- 1Joint Nature Conservation Committee, Peterborough, United Kingdom
- 2Centre for Conservation and Restoration Science, Edinburgh Napier University, Edinburgh, United Kingdom
- 3Biological and Environmental Sciences and Engineering Division (BESE), King Abdullah University of Science and Technology (KAUST), Thuwal, Saudi Arabia
- 4Departamento de Genética Molecular y Microbiología, Pontificia Universidad Católica de Chile, Santiago, Chile
- 5Department of Biology, University of Padova, Padova, Italy
- 6Laboratorio de Ecofisiologia y Ecologia Evolutiva Marinas, Facultad de Ciencias del Mar y de Recursos Naturales, Universidad de Valparaiso, Valparaiso, Chile
Editorial on the Research Topic
Fluctuating Habitats: Ecological Relevance of Environmental Variability and Predictability on Species, Communities, and Ecosystems
The Importance of the Spatial and Temporal Variability in Ecology and Physiology
Temporal and spatial environmental variability are wide spread and life evolved adapting to constantly ever changing conditions (Desmond, 2021). In marine and freshwater productive ecosystems water temperature and light availability dictate diel change in oxygen, pH and CO2 by controlling the photosynthetic activity of the aquatic primary producers (Bitter et al., 2021a). Landward, intertidal environments are governed by the alternation of emerged and submerged periods that determine a temporal and spatial heterogeneity in salt stress and water availability to which species need to adapt. These diel changes are also observed in terrestrial ecosystems. For instance, in the desert, seasonal humidity and temperature fluctuation control the water availability create the conditions for life to thrive (Makhalanyane et al., 2015 and reference within). Although most of the models of species adaptation consider the biological response to one or few relevant environmental factors, such as temperature in climate change models, there are growing evidences that the cumulative effect of the variability of abiotic factors has to be considered. For instance, fluctuating conditions can mitigate the net impact of the corresponding stressor, increasing physiological tolerance and promoting adaptation to novel environmental conditions at all biological levels (Booth et al., 2021; Fusi et al., 2021). For microorganisms, in addition to spatial patterns, environmental fluctuations can determine localized spatial jackpot events that ensure persistence of strains across environmental dynamic conditions (Ciccarese et al., 2022). At the holobiont level, desert plants stabilize the associated bacterial community (e.g., stable composition) by modulating the release of exopolysaccharides therefore insulating the bacterial community around the rhizosphere, attaining the effect of daily and seasonal fluctuations. This process mediated by the plant holobiont ensures the persistence of important plant promoting bacteria that can support plant growth and homeostasis in such harsh environment (Marasco et al., 2022). In coastal areas, marine ectotherms experience such a high magnitude of environmental variability that significantly shapes their physiological response to climate change and stresses (Baldanzi et al., 2015; Giomi et al., 2019). Increasing number of studies highlight the importance of environmental fluctuations as main driver of all the eco-evolutionary processes of life on earth. However, when it comes to investigate the effect of the environmental change on physiological and ecological processes through both, laboratory and theoretical approaches, the “mean conditions paradigm” still prevail (Kroeker et al., 2020). This Research Topic brought together contributions that re-shape our understanding of how environmental heterogeneity and fluctuations drive population and community assembly across diverse habitats.
Considering the Temporal and Spatial Environmental Variability
Environmental fluctuations exert an impact on organism physiology which has been recognized either as a threat (e.g., marine heatwaves) or a benefit (Huey and Bennett, 1990; Bernhardt et al., 2020). Manríquez et al. studied the acclimatization to upwelling conditions (variable vs. stable) of the marine ectotherm Achanthopleura echinata of two Chilean populations and demonstrated that a fluctuating environment increases the thermal tolerance of A. echinata, however carrying a high energetic cost. Eaton et al. using thermal fluctuations to investigate the effect of heatwaves on pinfish, found that thermal stress induced metabolic plasticity as a response to thermal anomalies. As a results, the pinfish increased their resilience to heatwaves. Similarly, individual plastic response was investigated by El Moussaoui et al.. The authors find that American wisteria, a perennial climbing plant, responded to increasing stressing condition by modifying its morphology such that it could withstand aridity ad increasing temperatures. Likewise, a terrestrial orchid shows a degree of variability in growth, flowering and mortality over time as a respond to changing harvesting strategies and climate fluctuations occurring over the years (Timsina et al.).
Environmental variability results also in the variation of diel cycles along seasonal fluctuations. During summer the low pressure in the Indian ocean determined by the El Niño–Southern Oscillation reclaims water from the Red Sea basin and decreases its sea level and consequently its tidal range. Red Sea mangroves inhabiting landward zone experience almost 6 months of high salinity due to the absence of tide. The bacterial community of mangrove sediment in this area differs dramatically from area continuously inundated, significantly changing their ecosystem function (Thomson et al.). The importance of the variability of temperature fluctuation also has a significant impact on the algal biofilm formation. Cotiyane-Pondo and Bornman demonstrate that different localized fluctuations of nutrients, and temperature, at centimeter/millimeter scale, resulted in a differential colonization of diatoms, highlighting the importance of the local variability over large-scale patterns.
Spatial variability in environmental conditions can generate important consequences at species and community levels depending on the scale at which these variations is considered. Pérez-Matus et al. investigated the pattern of fish assemblages and their functional diversity across almost 150 degrees of longitude in eastern and western South Pacific Ocean. They found that, while at large scale, from west-to-east a decline in species diversity was evident, the patterns of variation in functional diversity depend on the environmental domains found at smaller scales. On a similar topic, Pattrick et al. investigated the effects of marine fronts on larval fish assemblages by jointly collecting environmental and biological data in the nearshore waters of the south coast of South Africa. They show that, the onshore retention of fish larvae is highly correlated with offshore larval mixing and the degree of environmental variability at local scales (current velocity and mixing).
Integrating Environmental Temporal and Spatial Variability in New Framework of Research
Temporal and spatial variability are central in many ecological processes driving the biological community assembly, from micro-scale with microorganisms (bacteria, archaea, fungi, viruses, and protists) to macro-scale at individual, community and ecosystem levels (Figure 1). Most importantly, the understanding of the predictability of the environmental variability plays a unique and fundamental role in shaping the phenotypic variation observed across natural populations and their organization (Bitter et al., 2021b). In-situ high frequency environmental logging, measured at ecological relevant spatial scale, can capture ecological patterns that models based on large spatial resolution often fail to detect (Lima and Wethey, 2012; Vargas et al., 2022). Environmental fluctuations have been proved to play a fundamental role in ecosystem resistance to climate change (Giomi et al., 2019). Overall, there is a general consensus that in future studies and models, multiple dimensions will need to be integrated to better describe the environmental complexity (Ciccarese and Johnson, 2019; Kroeker et al., 2020).
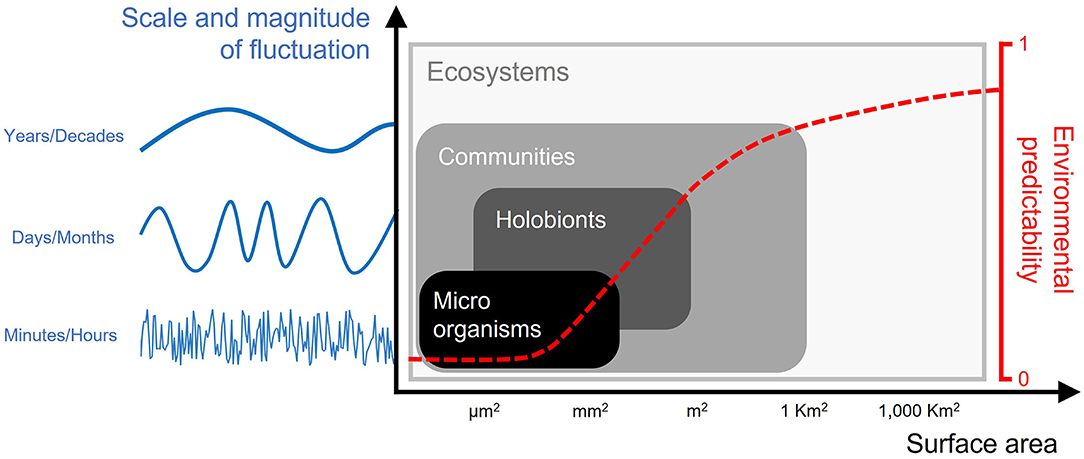
Figure 1. This figure aims to represent connections between the different scales of spatial and temporal variability (and predictability) that different levels of biological organization experience. Thus, showing the importance of integrating across different environmental scales that connect organisms/communities/ecosystems to climate and changes due to the anthropogenic impact. Spatial and temporal variability across different levels of biological organization vary in magnitude and scale. At micro/millimeters scales, environmental variables can display random variations across time (rather than a regular, e.g., cyclical, pattern) which make that variability difficult to predict. For example, cyanobacterial biofilms are characterized by a high variability of oxygen and pH fluctuations over time as a result of different micro-conditions (i.e., irradiance, photosynthetic performance). At larger scales, holobionts and communities experience more predictable patterns of variation. For example, hot desert organisms live in a predictable pattern of daily temperature variation; likewise, benthic communities in marine productive ecosystems experience regular daily fluctuations of dissolved oxygen driven by photosynthesis and community respiration. At the ecosystem level, the predictability of environmental variables further increases because of the seasonal pattern corresponding to the latitude where the ecosystem is located.
Measuring the environment as organisms experience it and respond to its variations within laboratory-based experiments will provide new insights to unveil the role of environmental variability in ecosystem structure and functioning (Tecon et al., 2019). This will help to provide a solid base to build more relevant models to predict the consequences of climate change on biodiversity and ecosystems across the planet and the services they provide.
Author Contributions
MF, SB, and RM led the article structure and the conceptual model. All authors contributed to the article and approved the submitted version.
Funding
SB acknowledges the funding from ANID-Fondecyt grant (n°11221161) and the University of Valparaiso through the grant INICI-UV (n°UVA20993). J-BR is supported by an ANID-Fondecyt grant (n°1210912).
Conflict of Interest
The authors declare that the research was conducted in the absence of any commercial or financial relationships that could be construed as a potential conflict of interest.
Publisher's Note
All claims expressed in this article are solely those of the authors and do not necessarily represent those of their affiliated organizations, or those of the publisher, the editors and the reviewers. Any product that may be evaluated in this article, or claim that may be made by its manufacturer, is not guaranteed or endorsed by the publisher.
Acknowledgments
We wish to thank all the contributing authors for their efforts to make this issue a success. We also wish to thank the Frontiers in Ecology & Evolution editorial and support teams for their patience and advice. We thank Dr. Davide Ciccarese for critical reviewing this editorial.
References
Baldanzi, S., Weidberg, N. F., Fusi, M., Cannicci, S., Mcquaid, C. D., and Porri, F. (2015). Contrasting environments shape thermal physiology across the spatial range of the sandhopper Talorchestia capensis. Oecologia 179, 1067–1078. doi: 10.1007/s00442-015-3404-5
Bernhardt, J. R., O'Connor, M. I., Sunday, J. M., and Gonzalez, A. (2020). Life in fluctuating environments. Philos. Trans. R. Soc. Lond. B. Biol. Sci. 375, 20190454. doi: 10.1098/rstb.2019.0454
Bitter, M. C., Kapsenberg, L., Silliman, K., Gattuso, J. P., and Pfister, C. A. (2021b). Magnitude and predictability of ph fluctuations shape plastic responses to ocean acidification. Am. Nat. 197, 486–501. doi: 10.1086/712930
Bitter, M. C., Wong, J. M., Dam, H. G., Donelan, S. C., Kenkel, C. D., Komoroske, L. M., et al. (2021a). Fluctuating selection and global change: A synthesis and review on disentangling the roles of climate amplitude, predictability and novelty. Proc. R. Soc. B Biol. Sci. 288, 20210727. doi: 10.1098/rspb.2021.0727
Booth, J., Fusi, M., Giomi, F., Chapman, E., Diele, K., and McQuaid, C. (2021). Diel oxygen fluctuation drives the thermal response and metabolic performance of coastal marine ectotherms. Proc. R. Soc. B Biol. Sci. 288, 20211141. doi: 10.1098/rspb.2021.1141
Ciccarese, D., and Johnson, D. (2019). “Functional microbial landscapes,” in Comprehensive Biotechnology, 3rd Edn, ed M. Moo-Young (Amsterdam: Elsevier), 42–51.
Ciccarese, D., Micali, G., Borer, B., Ruan, C., Or, D., and Johnson, D. R. (2022). Rare and localized events stabilize microbial community composition and patterns of spatial self-organization in a fluctuating environment. ISME J. 16, 1453–1463. doi: 10.1038/s41396-022-01189-9
Desmond, H. (2021). Adapting to environmental heterogeneity: selection and radiation. Biol. Theory 17, 80–93. doi: 10.1007/s13752-021-00373-y
Fusi, M., Daffonchio, D., Booth, J., and Giomi, F. (2021). Dissolved oxygen in heterogeneous environments dictates the metabolic rate and thermal sensitivity of a tropical aquatic crab. Front. Mar. Sci. 8, 767471. doi: 10.3389/fmars.2021.767471
Giomi, F., Barausse, A., Duarte, C. M., Booth, J., Agusti, S., Saderne, V., et al. (2019). Oxygen supersaturation protects coastal marine fauna from ocean warming. Sci. Adv. 5, 1–8. doi: 10.1126/sciadv.aax1814
Huey, R. B., and Bennett, A. F. (1990). “Physiological adjustments to fluctuating thermal environments : an ecological and evolutionary perspective,” in Stress Proteins in Biology and Medicine (Cold Spring Hahr Laboratory Press), 37–59.
Kroeker, K. J., Bell, L. E., Donham, E. M., Hoshijima, U., Lummis, S., Toy, J. A., et al. (2020). Ecological change in dynamic environments: accounting for temporal environmental variability in studies of ocean change biology. Glob. Chang. Biol. 26, 54–67. doi: 10.1111/gcb.14868
Lima, F. P., and Wethey, D. S. (2012). Three decades of high-resolution coastal sea surface temperatures reveal more than warming. Nat. Commun. 3, 704. doi: 10.1038/ncomms1713
Makhalanyane, T. P., Valverde, A., Gunnigle, E., Frossard, A., Ramond, J. B., and Cowan, D. A. (2015). Microbial ecology of hot desert edaphic systems. FEMS Microbiol. Rev. 39, 203–221. doi: 10.1093/femsre/fuu011
Marasco, R., Fusi, M., Mosqueira, M., Booth, J. M., Rossi, F., Cardinale, M., et al. (2022). Rhizosheath–root system changes exopolysaccharide content but stabilizes bacterial community across contrasting seasons in a desert environment. Environ. Microb. 17, 1–19. doi: 10.1186/s40793-022-00407-3
Tecon, R, Mitri, S., Ciccarese, D., Or, D., van der Meer, J. R., and Johnson, D. R. (2019). Bridging the holistic-reductionist divide in microbial ecology. mSystems. 4, e00265–e00218. doi: 10.1128/mSystems.00265-18
Keywords: environmental variability, environmental predictability, ecophysiology, climate change, biodiversity
Citation: Fusi M, Marasco R, Ramond J-B, Barausse A and Baldanzi S (2022) Editorial: Fluctuating Habitats: Ecological Relevance of Environmental Variability and Predictability on Species, Communities, and Ecosystems. Front. Ecol. Evol. 10:907622. doi: 10.3389/fevo.2022.907622
Received: 29 March 2022; Accepted: 15 April 2022;
Published: 03 May 2022.
Edited and reviewed by: Vera Maria Fonseca Almeida-Val, National Institute of Amazonian Research (INPA), Brazil
Copyright © 2022 Fusi, Marasco, Ramond, Barausse and Baldanzi. This is an open-access article distributed under the terms of the Creative Commons Attribution License (CC BY). The use, distribution or reproduction in other forums is permitted, provided the original author(s) and the copyright owner(s) are credited and that the original publication in this journal is cited, in accordance with accepted academic practice. No use, distribution or reproduction is permitted which does not comply with these terms.
*Correspondence: Marco Fusi, bWFyY28uZnVzaSYjeDAwMDQwO2thdXN0LmVkdS5zYQ==; Simone Baldanzi, c2ltb25lLmJhbGRhbnppJiN4MDAwNDA7dXYuY2w=