- Department of Biological Sciences, Auburn University, Auburn, AL, United States
Secondary sex characteristics, like beak color in some avian species, have indirect impacts on reproductive success, as they are considered to be honest indicators of condition, immunocompetence, and developmental history. However, little is known about the long-term effects of environmental perturbations on the production and maintenance of these secondary sex characteristics in avian species. In zebra finches (Taeniopygia guttata), redder beaks indicate increased carotenoid expression and implantation into beak tissue, and female zebra finches prefer males with pronounced bright red beaks as a mate. The present study examines the long-term effects of embryonic incubation temperature on the maturation of beak color in zebra finches. We also investigated the effects of embryonic incubation temperature on sensitivity to a handling and restraint stressor in adulthood. Specifically, the aims of this study were to examine: (1) whether suboptimal incubation temperatures affect the timing of beak color development and color characteristics before and after sexual maturity, (2) if repeated handling causes short-term changes in beak color and whether color changes are related to embryonic thermal environment, and (3) how thermal stress during incubation alters future responses to a repeated handling stressor. Zebra finch eggs were randomly assigned to one of three incubators: “Control,” “Low,” or “Periodic Cooling.” Beak color (hue, saturation, and value) was quantified before [45, 60, 75 days post-hatch (dph)] and after sexual maturity (95 dph), as well as after repeated handling stress later in adulthood (avg of 386 dph). We found that there were age- and sex- specific effects of incubation treatment on beak hue, where females from periodically cooled eggs had decreased hues (redder) in adulthood. Additionally, eggs laid later in a clutch had decreased beak saturation levels throughout life regardless of incubation environment. We found that females had lower beak hue and saturation following a capture and restraint stressor, while males showed increased beak saturation. Lastly, males subjected to the Low incubation treatment had relatively higher activity levels during restraint than those in the Control group. Overall, these findings suggest that fluctuating incubation temperatures combined with repeated, short-term stressors can have significant, sex-specific effects on sexual ornamentation and behavior.
Introduction
Anthropogenic disturbances to the environment such as climate change, habitat destruction, and introduction of environmental toxicants and pathogens pose novel threats to an organism’s health and can act as stressors [stimuli that have the potential to inflict damage at a molecular, cellular, organ, or organismal level (Wada, 2019)]. It is crucial to identify and understand the impacts of such stressors in order to relieve and conserve at-risk species (Carey, 2009). One major concern of biologists is climate change, as global annual temperature has increased, on average, at a rate of 0.08°C per decade since 1880 (NOAA, 2022). Furthermore, climate models predict that not only absolute temperature, but temperature variability will increase in the future (Bathiany et al., 2018), which may expose various species to temperature ranges that they have not previously encountered. Yet, many published laboratory studies that investigate the effects of temperature use absolute temperature changes (i.e., static increases/decreases in mean temperature), which may not give an accurate depiction of temperature fluctuations that occur in nature. One major developmental perturbation for avian species is temperature variation/fluctuations experienced during the incubation period. Parents of most avian species sit on their eggs and use their bodies to transfer heat to developing embryos (Bertin et al., 2018), ranging from temperatures of 36 to 40.5°C (Lundy, 1969; Conway, 2000), with 37–38°C being the optimal temperature range for normal development of most avian species (French, 2009). When parents leave the nest to forage, the egg is often susceptible to periodic cooling events until the parents return to continue incubating. Although it is difficult to mimic these fluctuations in the laboratory (Valenzuela et al., 2019), studies that account for fluctuating temperatures can shed light on the conditions that force parents to leave the nest more often, therefore resulting in high incubation temperature variability, which can influence development, survival, and reproductive abilities and attractiveness of the offspring.
In birds, secondary sex characteristics such as plumage, beak color, and song can strongly influence reproductive success. These ornamental traits can serve as signals to a potential mate to indicate overall quality of the contender and have also been shown to reflect an individual’s developmental history (Merrill et al., 2016). For instance, male zebra finches (Taeniopygia guttata) who received high quality food as young had larger cheek patches and were preferred by females than ones received a control diet (Naguib and Nemitz, 2007). Although secondary sex characteristics fully develop by sexual maturity in birds, some also change in relation to an individual’s condition (Rosenthal et al., 2012) and can indicate current condition of a mate. For example, unlike plumage, vascularized beaks are particularly susceptible to environmental perturbations over time (Schull et al., 2016). At a molecular level, beak color is derived from carotenoids (McGraw, 2006), which are lipid-soluble pigments synthesized by plants, algae, bacteria, and fungi. These carotenoid pigments that are solely obtained from diet, which in turn, circulate in the bloodstream and deposit into the highly vascularized beak tissue (Pérez-Rodríguez et al., 2010). Beak color in male zebra finches has been correlated with defense against parasites (Hamilton and Zuk, 1982; McGraw and Hill, 2000; Van Oort and Dawson, 2005) and resistance to oxidative damage (Pérez-Rodríguez et al., 2010). Beak color can change rapidly, as carotenoids are quickly deposited into the living tissue in the beak (Ardia et al., 2010; Rosenthal et al., 2012; Merrill et al., 2016), which makes beak color a well-suited indicator of an individual’s current condition to a potential mate. Additionally, individuals that maintain their beak color in the face of a stressor are deemed resistant to that specific stressor. This aforementioned stress resistance is defined as an organism’s ability to elicit anti-damage mechanisms (e.g., behavioral, physiological, or cellular responses to avoid persistent damage), so that they are more resilient to perturbations and overall performance is unaffected (Wada, 2019; Wada and Coutts, 2021). Therefore, if males can maintain a red and bright beak color despite facing a particular stressor, such as decreased food availability/quality, disturbances in environmental temperatures, and/or repeated handling or human interference, then they are considered resistant to that stressor, meaning better at regulating carotenoid distribution throughout the beak to attract a mate. Thus, male beak color can signal stress tolerance as well as developmental history, where a female can choose males with an attractive phenotype and/or genes to further pass on to offspring.
While stress resistance can be observed in beak color development and maintenance at the expense of attracting a suitable mate, stress tolerance can also be observed by the magnitude of a stress response an individual displays and its consequences. In response to a stressor, vertebrates elicit stress responses to escape or cope with that stressor, and these responses vary depending on the severity and persistence of the stressor in the environment. These include sympathetic nervous system and adrenocortical responses, prompting release of catecholamines (epinephrine and norepinephrine), and glucocorticoids (cortisol and corticosterone) into the bloodstream. Persistent physiological stress elicited during development have been shown to have long-lasting effects in avian species, including effects on body mass (Wada et al., 2015; Zito et al., 2017) and song learning (Lindström, 1999; Buchanan et al., 2003; Spencer et al., 2005; Wada and Coutts, 2021). In addition to the adrenocortical responses traditionally examined in relation to developmental stress, organisms display behavioral stress responses (e.g., anxiety-, fear-, and depression-related behaviors) due to variations in hormone signaling (Adkins-Regan, 2005; Myers et al., 2017). Although there are more studies that examined the effects of developmental nutritional stress and incubation temperature on corticosterone (CORT) levels, relatively little is known about how a developmental stressor affects behavioral stress responses later in life (Wada and Coutts, 2021) and how these short-term behavioral stress responses can impact secondary sex characteristics.
To address knowledge gaps related to stress cross-tolerance and effects of developmental conditions on secondary sex characteristic in adulthood when fitness-related traits like beak color would be most important, we explored the long-term consequences of fluctuating and low embryonic incubation temperatures on the maturation of beak color and sensitivity to a capture and restraint stressor in zebra finches. Specifically, we assessed three aims to elucidate the relationships between developmental stress, secondary sex characteristics, and the behavioral stress response: (1) the effects of suboptimal embryonic incubation temperatures on the development of beak hue, saturation, and value from juvenile stages to sexual maturity, (2) the short-term changes of beak coloration following a repeated capture and restraint (Cockrem et al., 2008; McGraw et al., 2011) associated with a behavioral response test [a “handling bag test” adapted from Martin and Réale (2008)] on beak color maintenance, and (3) the consequences of suboptimal embryonic incubation temperatures on responses to a repeated handling stressor. While the impacts of developmental stress may appear negative, there is increasing evidence for an adaptive role of low-level developmental stress in shaping animal phenotype throughout life (Crino and Breuner, 2015; Briga et al., 2017; Krause et al., 2017). Considering a fluctuating incubation temperature as a mild developmental stressor as opposed to constantly low incubation temperature, we hypothesized that fluctuating incubation temperatures promote cross-stress resistance (i.e., further resistant to future stressors) later in life when individuals are exposed to a capture and restraint stressor, as stress in early life has been shown to prepare an individual to future stressors in adulthood (Hoffman et al., 2018). Because developmental stress has been shown to have sex-specific effects on offspring phenotype in zebra finches where females are more negatively affected (Wada et al., 2008; Zito et al., 2017), we predicted that females incubated in the consistently low and periodic cooling incubation treatments would exhibit a more red beak color (increased hues/less attractive) across time, and short-term, repeated capture and restraint would cause a temporary decrease in female beak hue. Ultimately, the correlations between a developmental stressor and the impacts on an individual’s behavioral stress response are largely unknown aside from bird song (Buchanan et al., 2003; MacDougall-Shackleton and Spencer, 2012). Thus, the purpose of this study is to correlate a developmental stress, in the form of fluctuating embryonic incubation temperatures, experienced in early life to beak color maturation and behavioral stress response profiles of individuals later in life.
Materials and Methods
Animal Models and Husbandry
The animal husbandry and thermal manipulation protocols in this study have been extensively described in Rubin et al. (2021). Briefly, fertile eggs from 23 pairs (average of 3.7 eggs per nest) of zebra finches (Taeniopygia guttata) housed in at Auburn University, AL, United States were collected from April 2018 to December 2018. All breeding pairs had ad libitum access to seed [Kaytee Supreme (Finch) Chilton, WI, United States], water, grit, and cuttlefish bone pieces. Breeding pairs were given a tablespoon (9.50 ± 0.39 g) of egg food (boiled egg, bread, and cornmeal mixture) daily. Once a week, they were provided with spinach and a probiotic supplement (Bene-Bac, PetAg) mixed into egg food. Each breeding pair was provided an external nest box (19.5 cm × 14.5 cm × 14.5 cm) and nesting materials of shredded paper and irradiated hay (Rubin et al., 2021). To stimulate breeding, the birds were spritzed with water until egg laying began, as zebra finches use rainfall as a cue to initiate breeding (Zann et al., 1995). Each nest box was checked daily between 1,000 and 1,200 h for freshly laid eggs. Once collected, eggs were labeled with a non-toxic marker to reflect nest origin and laying order, and eggs were randomly assigned to one of three incubators (Brinsea Octagon EX incubators, Brinsea Products Inc., Titusville, FL, United States): (1) an incubator with a constant temperature of 37.4°C further referred to as “Control,” (2) an incubator that periodically cooled eggs by powering off five times a day for 30 min every 2 h further referred to as “Periodic,” and (3) an incubator with a constant low temperature of 36.4°C, which was the average temperature of the Periodic treatment, further referred to as “Low.” There was one incubator per experimental group. Each incubator was programmed to hold a constant humidity reading of 55%. Temperature readings on the incubators were confirmed using thermometers (Thermco, 25/45°C ACCI310) for the Low and Control incubators. The lowest average temperature that the Periodic incubator reached during periodic cooling events was 29°C. After hatch, hatchlings were marked with unique feather patterns and haphazardly placed in nests, as breeding pairs were in different stages of laying and incubation (Rubin et al., 2021). Parents were removed from the nests once the youngest offspring in a nest gained nutritional independence, which occurs at ∼45 dph. Additionally, birds were visually sexed and separated into isolation cages according to their sex between 55–60 dph, where they continued to receive ad libitum access to seed, water, grit, and cuttlefish bone pieces and weekly servings of spinach and egg food with probiotic mixture (Hoffman et al., 2018; Rubin et al., 2021). Each isolation cage held no more than three individuals. After individuals reached sexual maturity (∼95 dph), they were returned to communal flight cages. When individuals were re-captured in adulthood for this study, they were placed back into isolation cages (two individuals per cage, sex separated) for a 2-week acclimation period. Individuals were only disturbed for daily animal care, where they continued to receive ad libitum seed, acidified water, egg food mixture, and cuttlefish bone. This experiment utilizes the 22 individuals assigned to the Control incubator (12 female; 10 male), 22 assigned to the Low incubator (11 female; 11 male), and 28 individuals assigned to the Periodic incubator (13 female; 15 male; total of 72 individuals). All procedures listed above were completed at and approved by Auburn University, AL, United States under IACUC #2018-3274.
Beak Coloration Measurements
Beak photographs were taken using an iPhone 8 Plus camera set to standard settings (flash, HDR, Live Mode, Timers, and Filters disabled) within a Table–top photography studio with two light sources on either side of the photography arena. The light sources were marked using lab tape to ensure that light source positioning was consistent across all photos. The camera was secured on a tripod within the photography arena to maintain consistent distances between the bird and the iPhone. Red, blue, and green (RBG) coloration was quantified using ImageJ (Schneider et al., 2012). RGB values were then converted to hue, saturation, and value for each image using an online tool available from RapidTables.1 Beak color measures were cross-examined and confirmed with Adobe Photoshop CC using the Magic Wand, Histogram, and Color Picker tools with tolerance set to 60 for each photo. To explore the effects of suboptimal incubation temperatures on beak color maturation, measures for hue, saturation, and value were obtained for each bird at 45, 60, 75, and 95 dph (Figure 1). Beak coloration was again measured as adults (average age 385.82 ± 32.32 dph) prior to and after a “handling bag test” (described below) to test whether incubation temperature has a long-term effect on beak coloration, to examine a change in beak coloration due to repeated handling, and whether the degree of change is influenced by varying embryonic incubation temperatures. Photographs, ImageJ analyses, and Photoshop analyses were completed by the same individual blind to treatments.
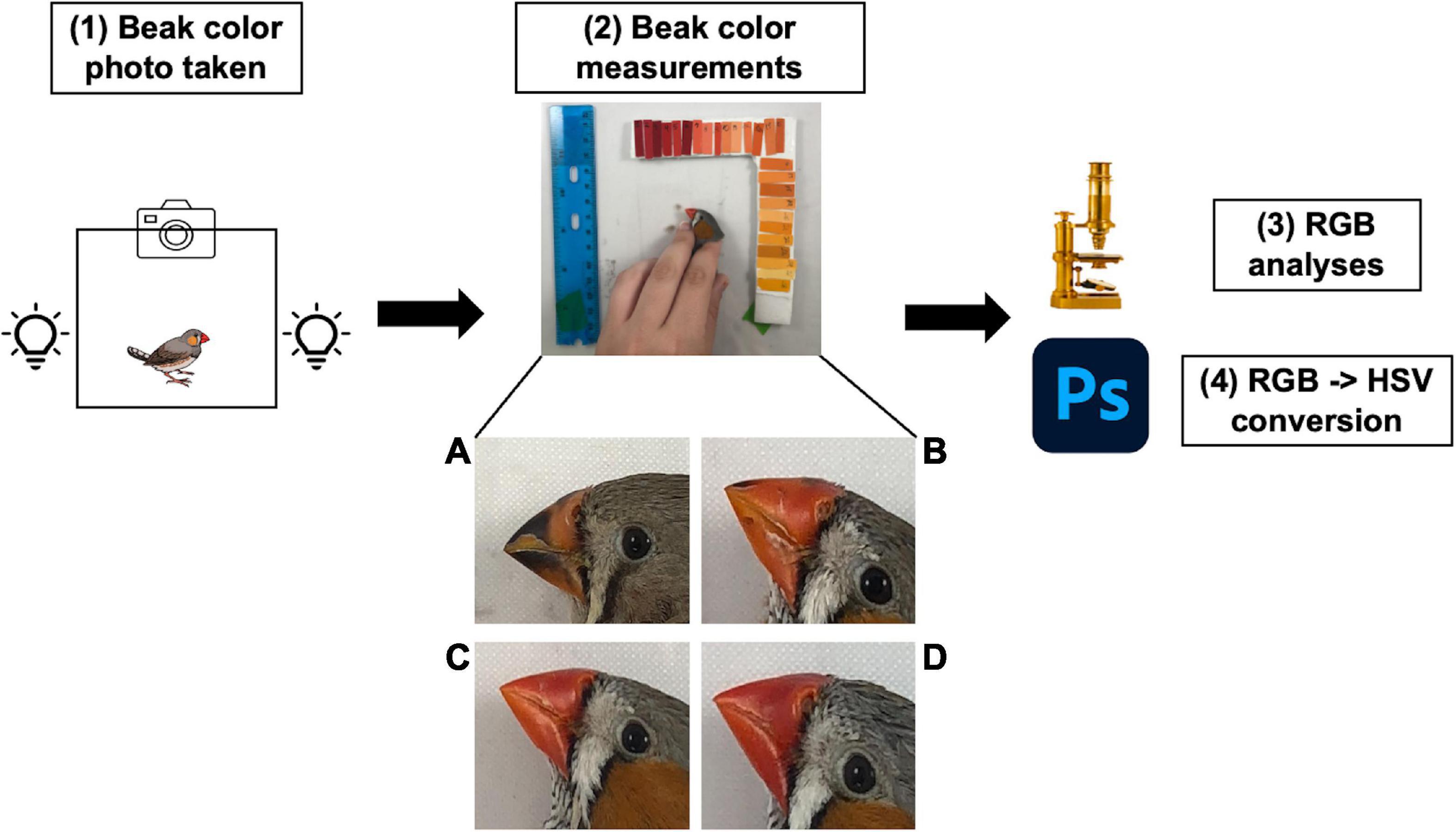
Figure 1. Beak color measurement and RGB/HSV conversion protocols. (1) Beak photos were taken using a standardized photography arena, with lights placed on either side of the arena and a tripod calibrated to a set distance to hold the iPhone. (2) The bird was laid flat onto the surface of the photography arena, ensuring visibility of the beak. Beak color/carotenoid accumulation is visualized in panels (A–D), which represents a male zebra finch at 45 (A), 60 (B), 75 (C), and 95 (D) dph. (3) Beak photos were then quantified for red, green, and blue (RGB) measurements using ImageJ, (4) those RGB values were converted into hue, saturation, and value (HSV) correlates and those HSV measurements were cross-examined using Adobe Photoshop.
To determine the effect of suboptimal incubation temperature on development and maintenance of beak coloration through an acute stressor, we examined three aspects of color: hue, saturation, and value (HSV). Hue refers to the actual color type, such as red, green, or blue and is measured in degrees on a color wheel, with 0° (and 360°) being red. Birds with lower hues have “redder” beaks, and birds with higher hues have lighter, more orange beaks. Saturation is the intensity (richness) of a color and is measured by a percent, with 0% meaning “no color” and 100% meaning “intense color.” Finally, value is the brightness of the color and is also measured by a percent, with 0% being black and 100% being white.
Handling Bag Test
To assess a behavioral stress response to a capture and restraint, we conducted a “handling bag test” adapted from Martin and Réale (2008), illustrated in Figure 2. To test the effect of incubation temperatures on behavioral stress responses and maintenance of beak coloration after repeated handling, 72 individuals (36 of each sex) exposed to the Control (n = 12 females; n = 10 males), Low (n = 11 females; n = 10 males), or Periodic (n = 13 females; n = 16 males) incubation regimes were used. Birds were placed in sex-specific cages with two birds per cage (temperature treatments randomized) for a period of 2 weeks, during this time they were not handled and only disturbed to perform daily care. Following this acclimation period, individuals were photographed to document a baseline beak coloration prior to the handling bag test and were then analyzed for their behavioral response to handling stress within 1 min of capture. Individual birds were caught and placed in an opaque brown paper bag suspended in the air with the bird inside for 60 s (Martin and Réale, 2008). During this time, each bird’s activity, described as any visible movement or rustling (visible shaking) of the bag during the measurement period, was recorded, and the proportion of time spent moving was calculated. The handling bag test occurred in a separate room from where conspecifics were housed to eliminate potential behavioral responses to chirps and song. An opaque brown paper bag was chosen instead of a mesh bag [as described in Martin and Réale (2008)] to (1) allow for disposal after each individual to prevent olfactory signals between individuals, (2) prevent visual cues while restrained in the opaque bag, and (3) the opaque bag is normally used in our field as a capture and restraint stress protocol. Bag tests were conducted in triplicate for each bird every other day over a period of 5 days, giving birds a day between replicates in which they were not handled and allowed to recover from the stress of capture and restraint. The order of birds that participated in these behavior trials was randomized daily. If two birds shared the same isolation cage, they were not tested in the same day to allow recovery of the individual that was not exposed to the handling bag test in that day. As behavioral responses can vary amongst each trial, three replicates across a period of 5 days were used to generate a “temperament” profile for each bird. In order to measure repeatability of these behavioral responses, we calculated an intra-class coefficient (ICC) to quantify the reliability of phenotypic variation as a fraction of variance among individuals and variance within individuals over time (Hayes and Jenkins, 1997; Bell et al., 2009; Nakagawa and Schielzeth, 2010; Wolak et al., 2012), listed in Table 3. The ICC values obtained in this study describe how similar the “time spent moving” replicates are to each other for each individual. Specifically, a value less than 0.5 indicates poor reliability, and a value greater than 0.9 indicates excellent reliability of the data. All handling bag tests, and beak measurements occurred between 08:00 and 09:30 a.m. to ensure that baseline measures were obtained prior to disturbance for daily care.
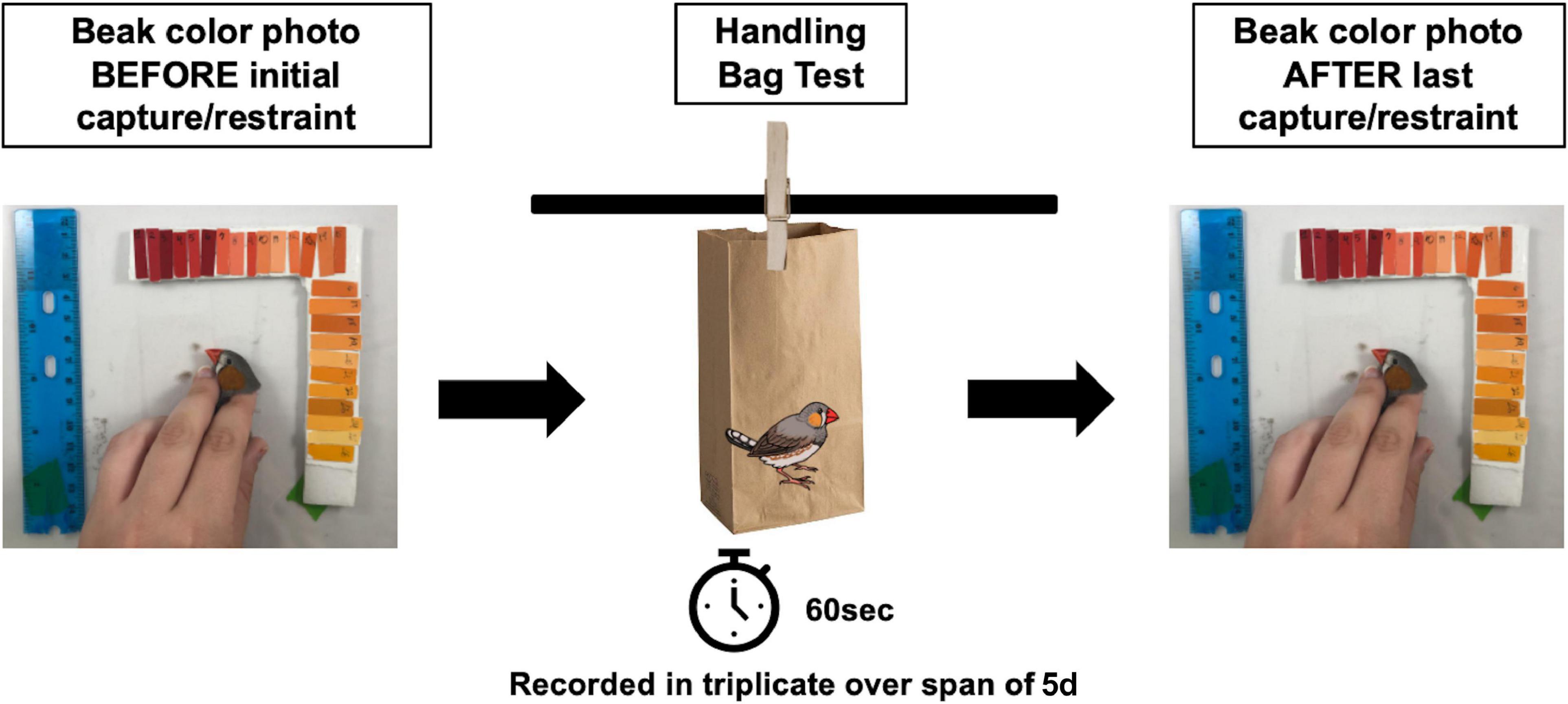
Figure 2. Handling bag test experimental design. Zebra finches at an average age of 386 dph participated in a handling bag test. Prior to the first handling bag test, beak color photos of each bird were taken. Then, each bird was placed in a brown, opaque paper bag and restrained for 1 min, and the time spent moving (“activity,”) was recorded. The handling bag test was conducted in triplicate every other day (over span of 5 days) for each bird to ensure for a recovery period. At the conclusion of the third handling bag test, another beak photo was taken.
Statistical Analyses
All statistical analyses were performed using RStudio version 4.0.5 “Ghost Orchid” (RStudio Team, 2021) using the lme4 (Bates et al., 2015), tidyverse (Wickham et al., 2019), and lmerTest (Kuznetsova et al., 2017) packages. All error metrics are reported as standard error unless otherwise noted. Aim 1 (beak color changes due to incubation temperature over time) was analyzed using linear mixed effects (LMER) models with a three-way interaction (along with corresponding two-way interactions) between age at measurement, incubation treatment, and sex, with numerical variables being scaled within the model. Additionally, all models for Aim 1 included an independent fixed effect of egg laying order and a random nested effect of nest of origin and individual to account for both genetic and individual differences. When interactions were significant in the global model (P < 0.05), those variables were analyzed separately. Hue, saturation, and value were used as measures of beak color. We used principal component analysis (PCA) to reduce collinearity between these variables. PCA showed that one component had an eigenvalue of 2.01, representing 67% of the variance in the data (Supplementary Figure 2 and Supplementary Table 5). When alternative models were performed, consistent results were obtained. Therefore, we are presenting hue, saturation, and value parameters separately.
Aim 2 (observing short-term changes in beak color from the handling bag test) was analyzed using LMER with a three-way interaction (along with corresponding two-way interactions) between sex, treatment, and timepoint (before/after the handling bag test), and a random effect of individual.
For Aim 3 (determining if thermal stress alters future responses to a stressor), we used a general linear model (lm) with fixed effects of treatment and sex and an interaction between the two variables. The independent variable in this case was the proportion of time spent moving while restrained in the bag. To verify the reliability of these repeated behavioral measures in Aim 3, we calculated an intra-class coefficient (ICC) using a generalized linear mixed effects model (binomial) with ID as a random effect using the rptR package (Stoffel et al., 2017). Statistical summaries are reported in Tables 1–4. A comprehensive table of estimates for each model are reported in Supplementary Tables 1–3.
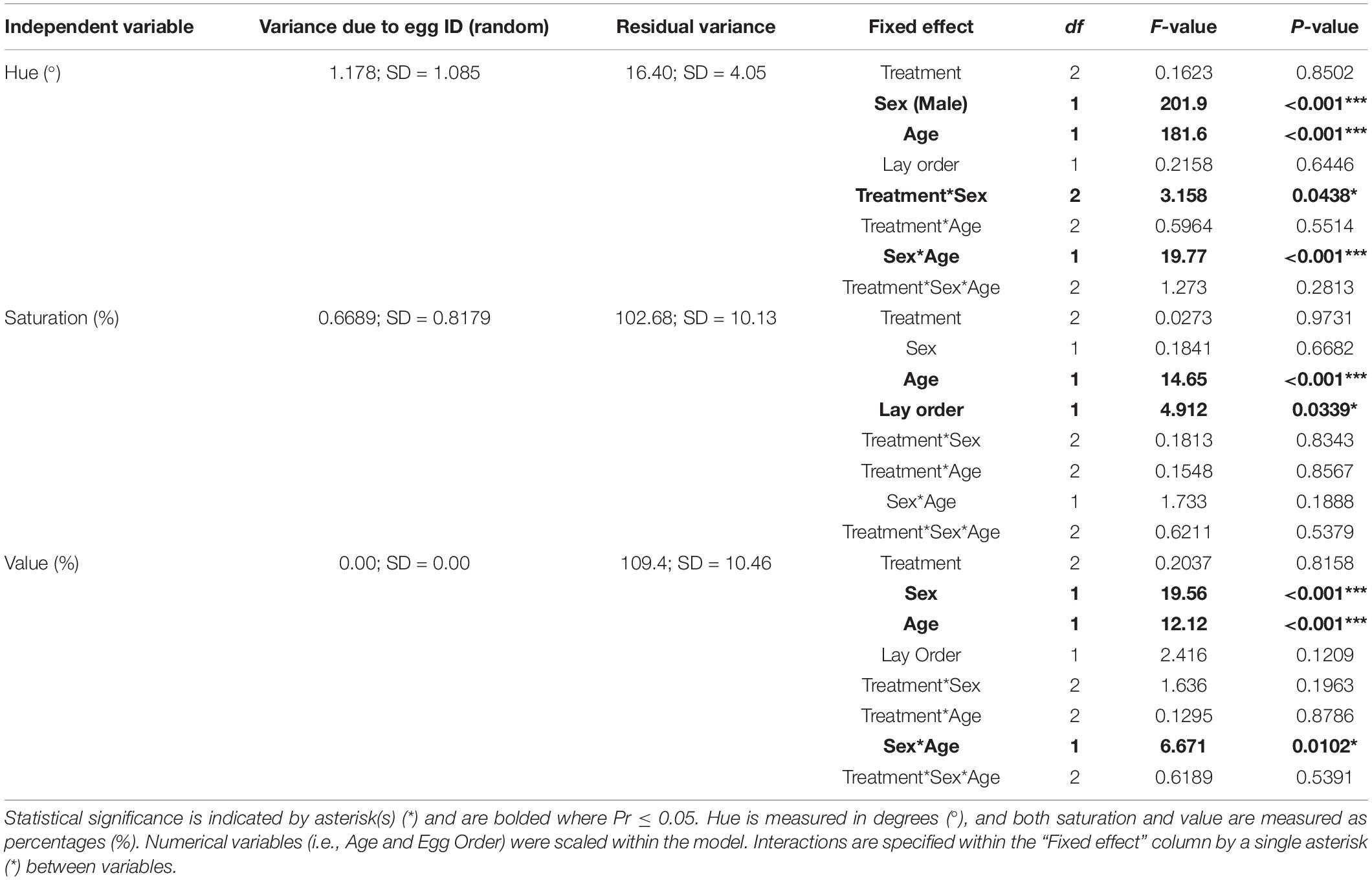
Table 1. Statistical summary of effects of incubation temperature on beak color before and after sexual maturity (Aim 1) using a global linear mixed effects model.
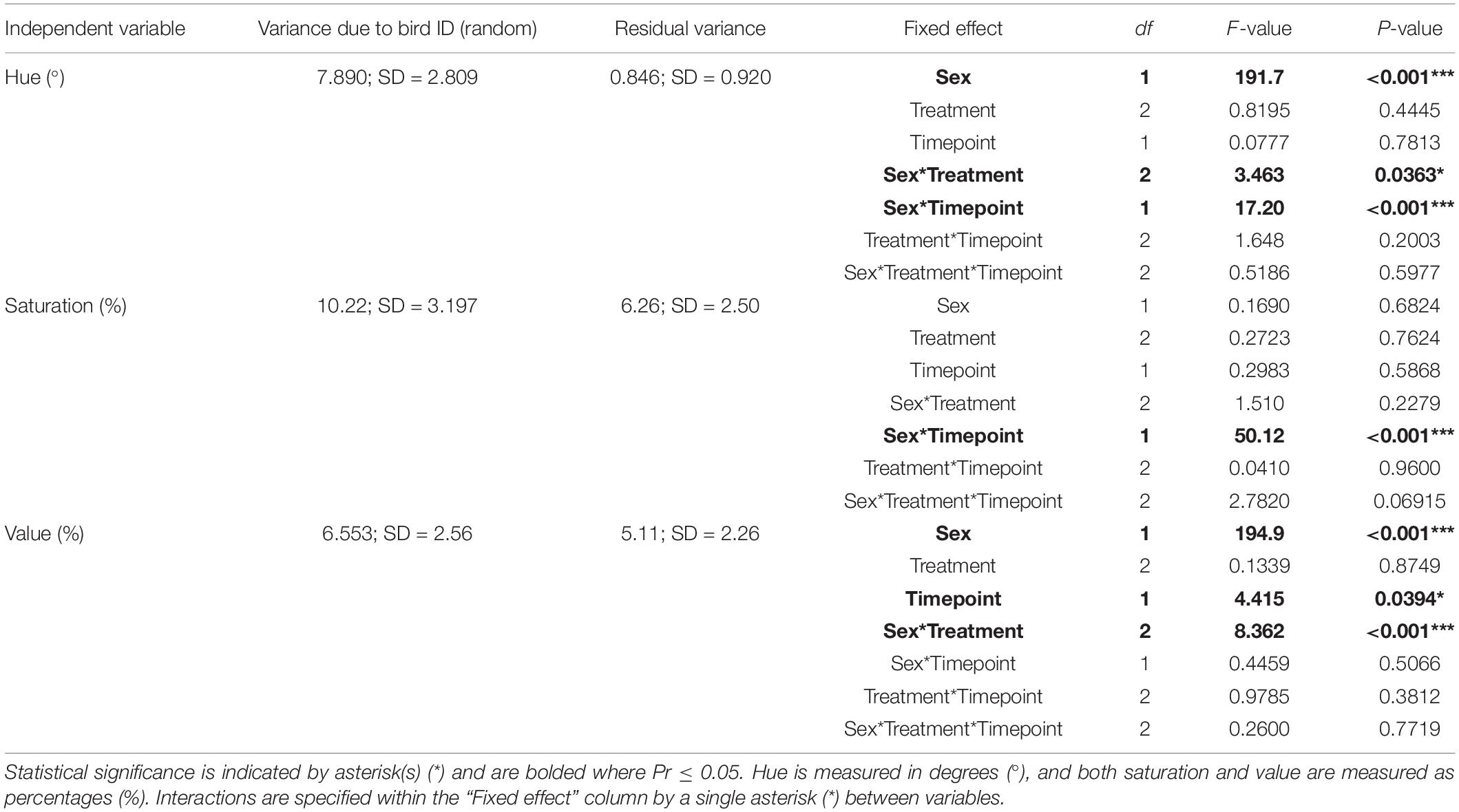
Table 2. Statistical summary of the short-term changes in beak color due to repeated handling and incubation temperature (Aim 2) using a global linear mixed effects model.
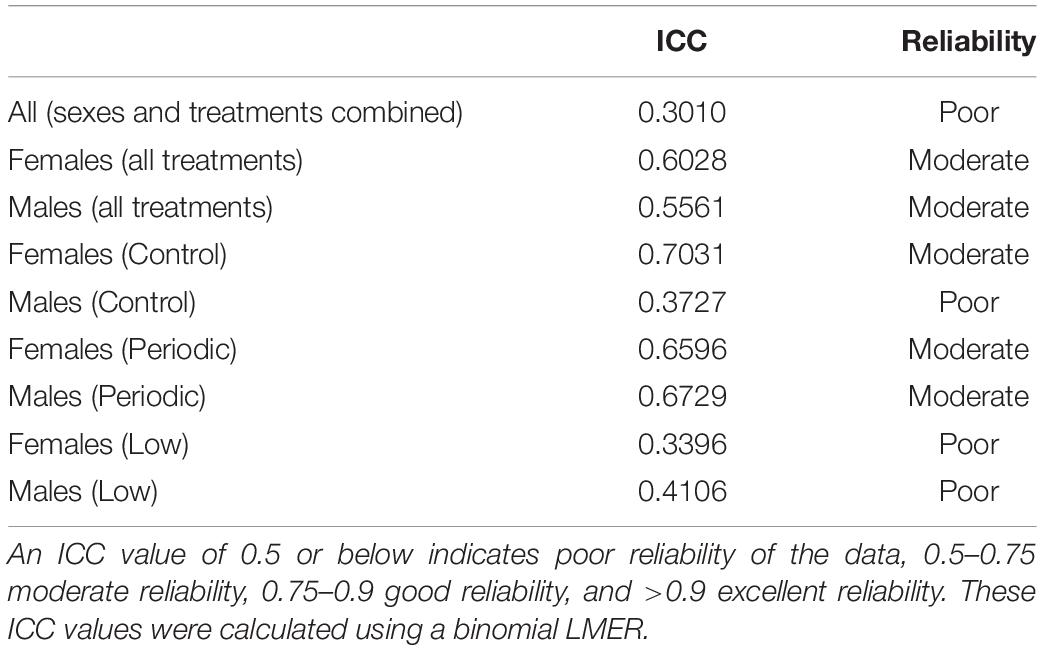
Table 3. Intra-class coefficient (ICC) calculations for repeated capture and restraint of zebra finches.
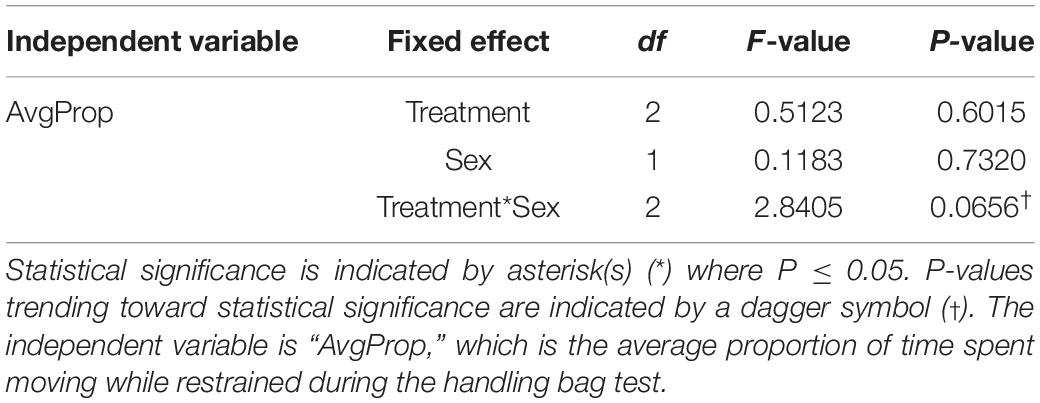
Table 4. Statistical summary elucidating the effects of suboptimal incubation temperature on activity levels (Aim 3) using a global linear model (LM).
Results
Aim 1: Effects of Suboptimal Incubation Temperature on Beak Coloration Throughout Development
All statistical measures for Aim 1 can be found in Table 1 and Supplementary Table 1. A graphical summary of the data can be found in Figure 3. We found that individuals in the Periodic group had significantly lower beak hues than those in the Control group [1.61° ± 0.734; t(371.00) = 2.19; P = 0.029]. Males also exhibited significantly lower beak hues than females [7.59° ± 0.825; t(329.14) = 9.20; P < 0.001]. We found a significant interaction between sex and age [−2.70 ± 0.753; F(1,348.65) = 19.77; P < 0.001]. When sexes were analyzed separately, we found that male hue decreased as they aged [3.84° ± 0.64; t(1932.00) = 6.00; P < 0.001, Figure 4]. In females, we found that female hue decreased with age [1.19° ± 0.379; t(145.15) = 3.14; P = 0.002, Figure 4].
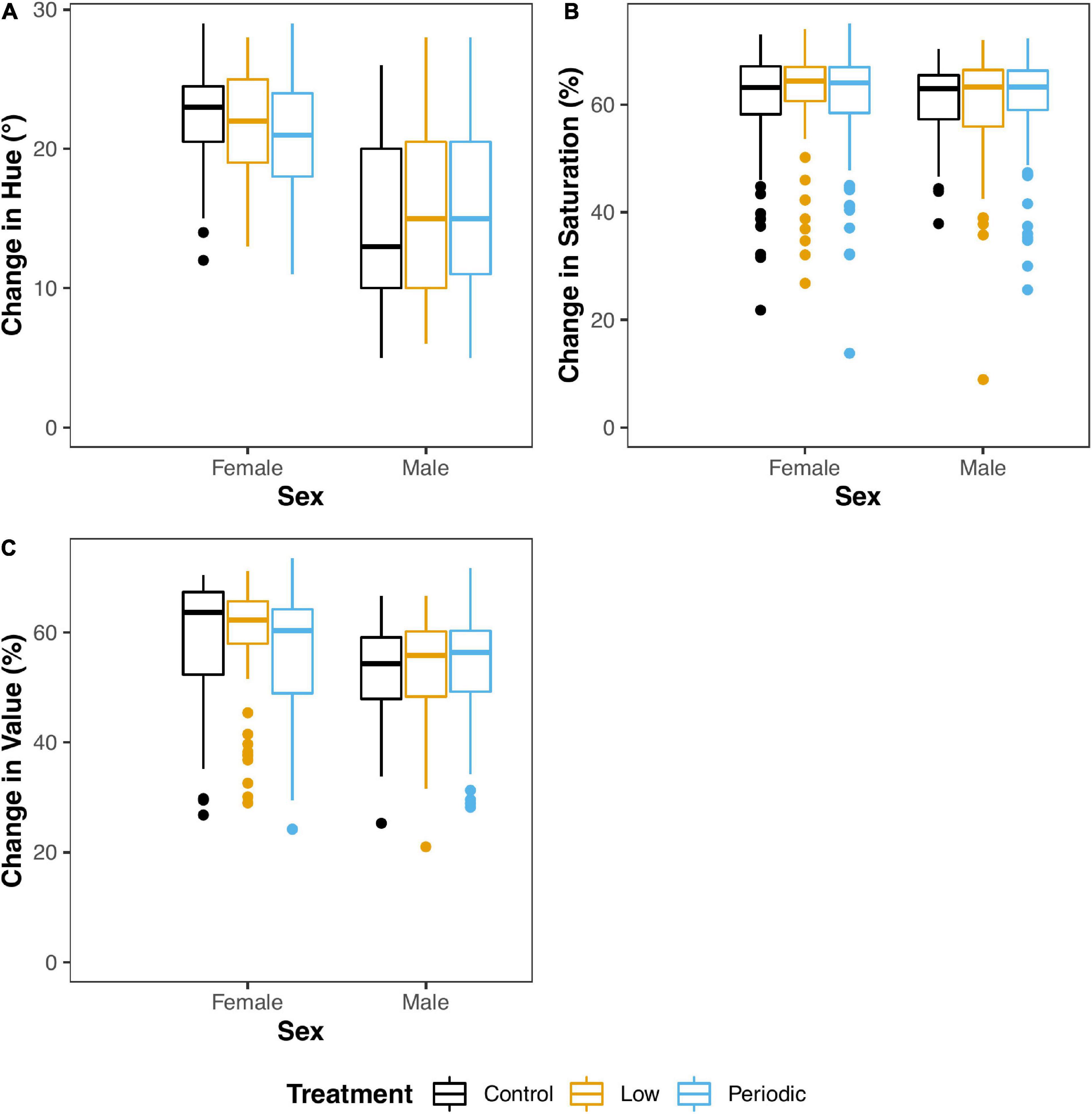
Figure 3. Suboptimal embryonic incubation temperatures do not impact beak color (hue, saturation, value) development. Control, Low, and Periodic treatments are shown in black, yellow, and blue, respectively. The changes in hue (A), saturation (B), and value (C) are measured on the y-axis, measured in degrees and percentage, respectively. Sex is indicated on the x-axis.
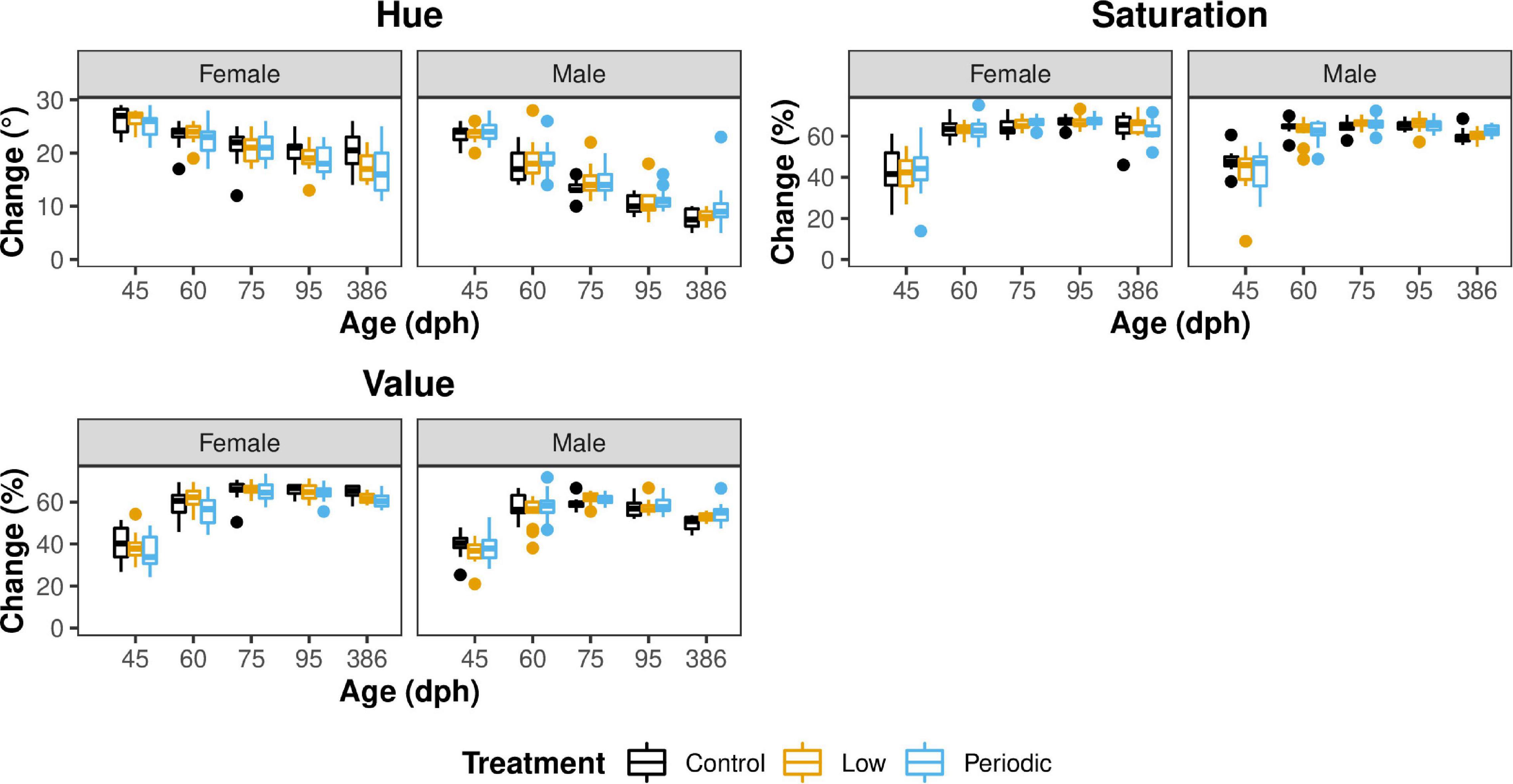
Figure 4. Male and female hue decreases over time, and saturation and value plateau on 60 and 75 dph, respectively. Females are shown in the left panel, and males are shown in the right panel. Control, Low, and Periodic treatments are shown in black, yellow, and blue, respectively. The changes in hue, saturation, and value are measured on the y-axis, measured in degrees and percentage, respectively. Age is indicated on the x-axis, including 45, 60, 75, 95, and 386 dph, respectively.
In contrast to hue, we found no significant interaction between sex and age on beak saturation. However, age significantly affected saturation, where beak saturation (intensity of color) increased by 2.77% ± 1.28 [t(355.43) = 2.17; P = 0.031] in both sexes (Figure 4) and plateaued around 75 dph. We did not find a significant effect of incubation treatment on neither male nor female beak saturation. Interestingly, we found that as egg laying order increased, saturation levels decreased [1.22% ± 0.552; t(31.97) = 2.22; P = 0.0339].
Males exhibited significantly lower beak values than females [5.92% ± 1.98; t(372.00) = 2.99; P = 0.003]. There was a significant interaction between sex and age on beak value [F(1,372) = 6.67; P = 0.010], so sexes were analyzed separately. There was no significance with regard to males. However, we found that female beak saturation increased [3.84% ± 1.43; t(179.00) = 2.69; P = 0.0078] with age.
Aim 2: Effects of Repeated Handling and Influence of Suboptimal Incubation Temperatures on Beak Coloration
Generally, we found that hues in all individuals were higher prior to the initial handling bag test compared to after the final bag test in the global model [1.0833° ± 0.3754; t(65.71) = 2.89; P = 0.0053], but this is dependent on sex [F(1,66) = 17.20; P < 0.001]. We also found a sex x treatment interaction [F(2,76) = 3.46; P = 0.036]. When sexes were analyzed separately, we found that males in the Periodic treatment had significantly higher hues [1.55° ± 0.77; t(43.52) = 2.02; P = 0.0496] than Control males. We found a significant interaction between sex x timepoint (P < 0.001). Females had significantly higher hues [1.08° ± 0.40; t(33.00) = 2.70; P = 0.011] before the handling bag test regardless of the incubation treatment, meaning that female beak color became redder after repeated handling. In contrast, male beak hue did not change with repeated handling [t(32.82) = 1.58; P = 0.124] (Figure 5A). We feel that it is important to mention that individuals in both the Periodic and Low treatments tended to have lower hues than Control birds [Periodic: t(73.32) = 1.91; P = 0.064; Low: t(73.32) = 1.67; P = 0.099, respectively].
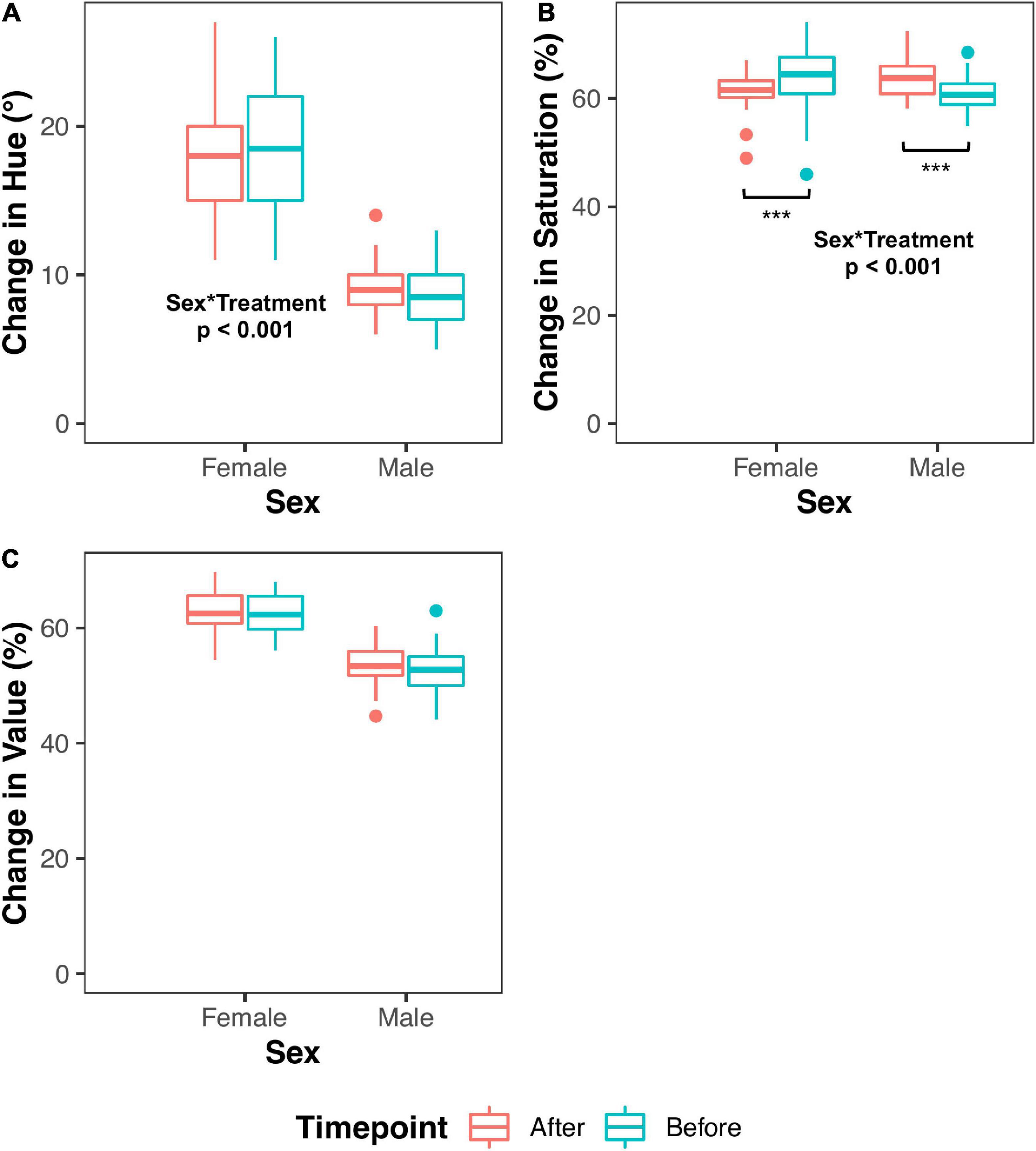
Figure 5. Beak hue and saturation are impacted by repeated capture and restraint. The change in the beak color parameter (hue, saturation, or value) is presented on the y-axis, and sex is shown on the x-axis. Data indicated in red boxplots are indicative of “Before” the handling bag test, whereas blue boxplots are indicative of “After” the handling bag test. Significant sex*timepoint interactions are mentioned where applicable. (A) Female beak hue significantly decreases following the initial handling bag test. (B) Both male and female beak saturation are inversely affected following the initial handling bag test. (C) There are no effects of the handling bag test on beak value. Three asterisks (***) indicate P ≤ 0.001.
We found a statistically significant sex x timepoint interaction [F(1,66) = 50.12; P < 0.001] when analyzing beak saturation. When sexes are analyzed separately, we found that males had significantly increased beak saturation following the last handling bag test [3.88% ± 1.19; t(32.95) = 3.26; P = 0.003; Figure 5B]. On the other hand, females had significantly decreased beak saturation following the last handling bag test [3.62% ± 0.95; t(33.00) = 3.80; P = 0.001; Figure 5B].
We found that males had significantly lower beak value (intensity) when compared to females in the global model [13.25% ± 1.46; t(100.40) = 9.06; P = 1.1 × 10–14; Figure 5C]. Additionally, a sex x treatment interaction was observed [F(2,69) = 8.36; P < 0.001], so sexes were analyzed separately. Compared to Control males, males in the Low group trended toward higher beak values [t(57.89) = 1.92; P = 0.060], and males in the Periodic group exhibited significantly higher beak values [3.54% ± 1.45; t(57.32) = 2.44; P = 0.018]. Females in the Periodic group exhibited significantly lower beak values [2.74% ± 1.30; t(42.77) = 2.10; P = 0.041] than females in the Control group. All statistical measures mentioned above can be found in Table 2 and Supplementary Table 2.
Aim 3: Fluctuating Incubation Temperature Alters Future Responses to a Handling Stressor
We estimated an ICC of 0.301 (95% CI: 0.080–0.432) for whether an animal moves during the one-minute stress test, indicating that the data is poorly reliable in quantifying an overall behavioral profile for each individual when both sexes and all treatments are considered. This ICC was calculated based on a binomial model, meaning that the data was coded by specifying whether the animal moved during the restraint period. ICCs were then calculated for each sex and treatment, which are listed in Table 3. Link- and original-scale approximation bootstrap repeatabilities for ID when calculating ICCs are listed in Supplementary Figure 1 and Supplementary Table 4. We found that males tended to elicit less activity (proportion of time spent moving) than females when restrained during the handling bag test [t(66.00) = 1.98; P = 0.051]. We did find a sex x treatment interaction trending toward statistical significance [F(2,66) = 2.84; P = 0.066], so we analyzed sexes separately due to biological significance. Males in the Low treatment group showed relatively higher activity levels than males in the Control group [0.26 ± 0.10; t(33.00) = 2.48; P = 0.018, Figure 6], but there was no effect of the Periodic group on activity levels in males [t(33.00) = 1.10; P = 0.28]. The incubation temperature treatment had no effect on activity levels in females. Statistical measures are summarized in Table 4 and Supplementary Table 3.
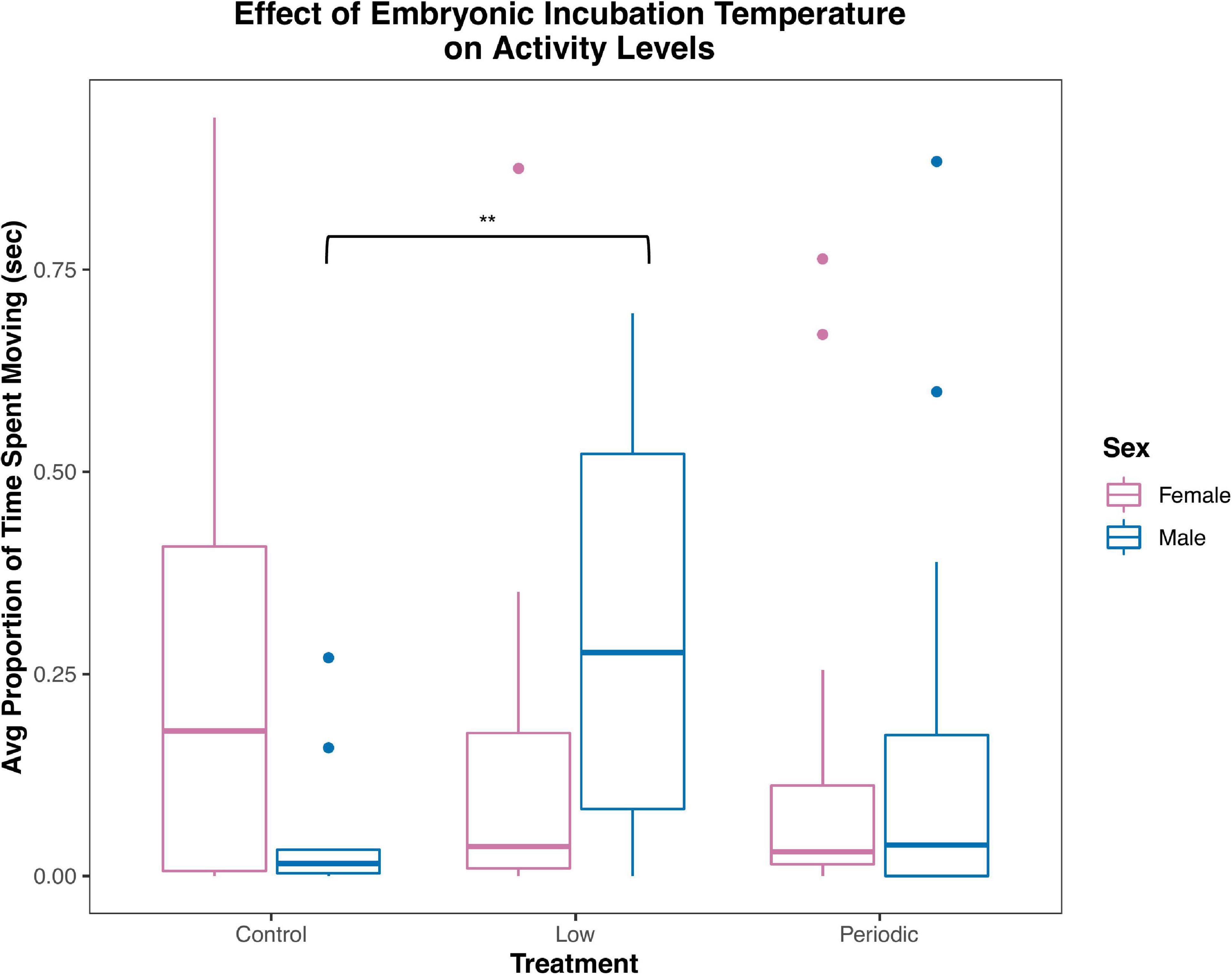
Figure 6. Males in the Low incubation treatment had higher activity levels than those in the Control treatment. The average proportion of time (seconds) spent moving during the handling bag test is recorded on the y-axis. The three incubation treatment groups are shown on the x-axis (Control, Low, and Periodic). Females are indicated by pink boxplots, and males are indicated by blue boxplots. Two asterisks (**) indicate P ≤ 0.05.
Discussion
Our study sheds light on the impacts of suboptimal embryonic incubation temperatures on beak coloration and the behavioral stress response in zebra finches throughout post-hatch development and into sexual maturity. Because fluctuating incubation temperatures are commonly experienced by avian eggs, in nature we sought to disentangle the effects of temperature variation from absolute temperature and to illuminate their downstream effects on beak color development using zebra finches as a model. We predicted that females in the Low and Periodic groups would decrease beak hue later in life, as females have been shown to be the most susceptible to environmental stressors. Because of this, we also predicted that females would exhibit higher activity levels during capture and restraint while birds from the Periodic group show improved stress tolerance due to frequent exposure to a mild stressor during development. In sum, we did not find an overall effect of incubation temperature on beak color development, but we found that periodically cooled females had decreased hues at 386 dph compared to Control females at that age. We also found that eggs laid later within a clutch had lower beak saturation than earlier laid eggs. When these individuals were subjected to repeated capture and restraint, females had lower beak hue and saturation following the final handling bag test. Conversely, we found that males had increased beak saturation following the final handling bag test. Lastly, we found that males within the Low incubation treatment had relatively higher activity levels than Control males when restrained.
Embryonic Incubation Temperature Had a Stronger Effect on Female Beak Color Maturation Than in Males
Many studies have explored the environmental, anthropogenic, and pathogenic effects of beak color in avian species. Beak color in goldfinches (Spinus tristis) is similarly regulated by carotenoid pigments and is impacted by repeated handling stress and immunostimulatory lipopolysaccharide (LPS) injection, as yellow saturation decreased within 6.5 h of repeated capture, and upon addition of LPS injection, beak hue and luminance drastically decreased (Rosenthal et al., 2012). Additionally, beak color in birds has been extensively experimentally modified via eliciting a stress response, modifying diet, or immune activity over a time period of weeks (Blount, 2003; Ardia et al., 2010; Rosenthal et al., 2012). Thus, it is apparent that maintaining beak color through the context of carotenoid distribution is a major tradeoff at the expense of reproduction (Bertrand et al., 2006). It is important to note that many studies that analyze beak color mainly use hue as a parameter, as hue is the main component of defining a particular color. However, data are lacking for other aspects of beak color such as saturation and value and so the consequences of changes in these traits on sexual ornamentation and reproduction is not well-understood.
First, we found that females at 386 dph had lower beak hues than Control females. This finding aligns with our prediction that females would be more affected than males, but the “attractiveness” of a redder beak in a female remains unclear without conducting mating trials. Specifically, females prefer males with the reddest, brightest beak color (Blount, 2003; Burley and Coopersmith, 2010; Merrill et al., 2016). Conversely, males prefer females whose beaks are not too red or too yellow but more orange with less intense coloration (Zann, 1996; McGraw, 2006). A previous study found that male and female zebra finches subjected to daily, 10-min handling treatments for 4 weeks displayed deeper orange/red beak coloration than control animals (McGraw et al., 2011). Although “attractiveness” of a redder beak on a female to a male beak is relatively unknown, a small set of evidence shows that males who experience no known stressor during development prefer females with orange beaks (higher hues) (Zann, 1996; McGraw, 2006). If a males’ preference is not influenced by a developmental stressor, high carotenoid allocation resulting in lower beak hue in a female may not be beneficial, as males may interpret this as a more “masculine” phenotype. Previous studies have shown that exogenous testosterone administration increases beak redness (lower hue) in female zebra finches (McGraw, 2006). Since testosterone increases redness in beaks but also suppresses immune functions, testosterone can mediate a trade-off between attractiveness and immune function. To fully understand these dynamics, future studies should evaluate how consistently low and fluctuating incubation temperature affects sex steroid levels, attractiveness via mate choice trails, and immunocompetence. It is possible that consistently low or periodic cooling events that occur during embryonic incubation of the parental generation may increase testosterone levels within the yolk of subsequent offspring (Gil et al., 2004). McGraw (2006) proposes that testosterone-induced immunosuppression in females with naturally high testosterone levels may divert carotenoids away from the beak and thus fading in beak color.
In contrast to females, we found that there was no significant effect of suboptimal embryonic incubation temperatures on male beak hue maturation. It is possible that males were able to maintain their beak hue despite embryonic perturbation as their post-hatch environment contained ad libitum access to food and nutrients. While there is strong evidence that an individual’s secondary sex characteristics can reflect developmental history (Merrill et al., 2016) especially in birdsong (Buchanan et al., 2003; Spencer et al., 2005), beak hue does not appear to be a strong indicator of developmental history with regard to male zebra finches. It has been shown that carotenoid-derived beak color in males is sex-steroid-dependent (e.g., androgens) (Nelson, 2005), where maternally-deposited androgens within the yolk increase offspring competitiveness and development (Schwabl, 1996). Female red-legged partridges (Alectoris rufa) have been found to mate with redder males and produce more eggs which could favor the survival of later hatchlings by increasing androgen allocation to eggs (Alonso-Alvarez et al., 2012). The next step would be to quantify circulating androgen levels to determine whether an ability to secrete comparable levels of androgen would explain how males from eggs incubated at Low or Periodic regime can maintain beak hue later in life.
We did not observe an effect of suboptimal incubation temperature on beak saturation in males nor females. However, we did confirm that beak saturation (color richness) increases with age in both sexes. Interestingly, we found that eggs laid later in a clutch had decreased beak saturation compared to earlier-laid eggs. It has previously been discovered that yolk testosterone levels increase as laying order increases in canaries and zebra finches (Schwabl, 1993), which could have implications of yolk androgen levels and its impacts on beak saturation later in life. This pattern can vary depending on other factors, including diet. When mothers were fed a low-quality diet, their yolk testosterone levels decreased with egg laying order (Sandell et al., 2007). Therefore, it is possible that the eggs utilized in this experiment that were laid later in the clutch had higher levels of testosterone by the mother, resulting in decreased beak saturation later in life. This is a loose speculation, as the parents of eggs from this experiment were not experimentally monitored nor exposed to any known stressors to cause variations in testosterone supplementation into the yolk.
Repeated Capture and Restraint Has Sex-Specific Effects on Beak Hue, Saturation, and Value
We found that females decreased beak hue and saturation while males increased beak saturation following the handling bag test. These findings are similar to a previous study where zebra finches of both sexes were subjected to daily handling stress over 4 weeks, and stressed males lost body mass and marginally decreased circulating carotenoid concentrations (McGraw et al., 2011). However, our results in females differed from the aforementioned study, where stressed females maintained their orange beak color. Both McGraw et al. (2011) and the current study show a sex-specific effect on handling stress on beak coloration in zebra finches, suggesting that males and females experience differential prioritization of beak color. It has been shown that carotenoids can be allocated to various parts of the body, such as within the integument, retina, and liver tissue (Rowe et al., 2012). Specifically, carotenoids can be stored within liver tissue until needed during periods of molt or migration (Negro et al., 2001). It is possible that, because of repeated capture and restraint, females mobilized carotenoid pigments, whereas males pulled carotenoid pigments from their beak tissue. The current study exposed finches to a handling stressor every other day for 5 days. Since this is much shorter and less frequent exposure than daily handling over 4 weeks used in McGraw et al. (2011), the data in the current study demonstrate that beak coloration can change with relatively mild handling stress. It is known that beak hue can change rapidly in response to a short-term stressor, as glucocorticoid-related stress impairs various condition-dependent traits (Buchanan, 2000). Generally, color saturation describes the vividness, richness, or intensity of a color. In this context, females had ‘less vivid’ beaks, whereas males had “more vivid” beaks because of the handling bag test. Males subjected to suboptimal incubation temperatures (both Low and Periodic groups) exhibited significantly higher beak values (brighter) compared to Control males following the capture and restraint protocol. It has been found that repeated handling significantly decreases body mass and depletes circulating carotenoid levels during the course of handling, but not long-term (McGraw et al., 2011). It is possible that short-term perturbation of carotenoids within beak tissue may make the beak itself less pigmented, thus affecting beak value, but our results show that individuals can potentially recover beak coloration by re-depositing carotenoid pigments within the beak. Unfortunately, we did not collect body mass/condition measures at or around the time of behavioral testing. However, our post-hoc testing of our previous study revealed that 10 dph individuals in the Periodic group were 14.2% lighter than individuals in the Low and Control groups (Rubin et al., 2021). Therefore, these results may be snapshots of the short-term changes in beak saturation because of repeated handling showing the movement of physiological and energetic resources away from secondary sex characteristics.
Embryonic Incubation Treatment Alters Future Responses to a Capture and Restraint Stressor
Overall, we found that males exhibited lower activity levels than females when restrained during the handling bag test. This is to be expected, as females generally elicit a more heightened stress response than males (Martins, 2004; Verhulst et al., 2006; Spencer et al., 2010; Marasco et al., 2012), which has been observed in rats in response to alcohol (Rivier, 1993), but females have been found to better cope with chronic stressors over time (Dalla et al., 2005). However, females do not always respond greater than males, as a previous study found that there is no sex differences in the CORT response to handling stress in 16 dph or as adults (Wada et al., 2008). However, we did find that males in the Low incubation group exhibited higher activity levels than males in the Control group. While the embryonic environment on the behavioral development of birds remains relatively unexplored, a recent study has shown that chronic exposure to suboptimal temperatures of 27.2°C for 1 h twice a day resulted in experimental chicks with elevated neophobic responses in novel food and novel environment tests, and these experimental chicks also showed higher corticotropin-releasing factor in the nuclei of the amygdala, which is involved in regulating fear-related behaviors (Bertin et al., 2018). Chronic exposure to low incubation temperatures has been shown to cause oxidative damage and changes in antioxidant pathways (Loyau et al., 2014; Bertin et al., 2018), which may have impacted the developmental programming of behavioral responses observed in males during the handling bag test. In fact, a study utilizing the same cohort of animals used in the present study found that individuals in the Periodic and Control groups were able to habituate, in terms of their adrenocortical response, to a repeated capture and restraint stress, whereas the individuals exposed to the Low incubation treatment were not (Rubin et al., 2021). This lack of the ability to habituate to a repeated stressor in the Low group viewed in conjunction with higher activity levels during restraint from the present study suggest a possible neuroendocrine link between heightened activity and elevated CORT levels. This inability to habituate to a subsequent stressor can lead to higher circulating CORT levels throughout life, which negatively impacts fitness-related traits, such as immunocompetence, growth, and survival (Sapolsky et al., 2000; Wada et al., 2015; Jimeno et al., 2018; Rubin et al., 2021). These hormonal responses are dependent on neuroendocrine pathways that are mostly established during early life. Lastly, there were no long-term effects of incubation temperature observed in females. Overall, these data suggest that incubation temperature and variability may contribute to the stress responses mounted later in life, which can have downstream effects on beak coloration and attractiveness.
Data Availability Statement
The raw data supporting the conclusions of this article will be made available by the authors, without undue reservation.
Ethics Statement
The animal study was reviewed and approved by Auburn University Institutional Animal Care and Use Committee.
Author Contributions
MC and AR carried out the experiment and collected the data. MC wrote the manuscript, analyzed the data, and generated graphical representations of the data. HW assisted with manuscript writing, proofreading, and statistical analyses. All authors designed the study, contributed to manuscript revision, and read and approved the submitted version.
Funding
This work was supported by the National Science Foundation grants (IOS-1553657 and IOS-2015802) and Alabama Agriculture Experiment Station awarded to HW.
Conflict of Interest
The authors declare that the research was conducted in the absence of any commercial or financial relationships that could be construed as a potential conflict of interest.
Publisher’s Note
All claims expressed in this article are solely those of the authors and do not necessarily represent those of their affiliated organizations, or those of the publisher, the editors and the reviewers. Any product that may be evaluated in this article, or claim that may be made by its manufacturer, is not guaranteed or endorsed by the publisher.
Acknowledgments
We would like to thank the 2018 undergraduate researchers of the Wada Lab for ensuring proper care for our zebra finches at our on-campus aviary. We would also like to thank the National Science Foundation (NSF) and Alabama Agriculture Experiment Station for funding this project. Additionally, we would like to thank Alexander Hoffman and Wonil Choi for their assistance in the rationale and statistical analyses for this study. We would also like to thank Matthew Wolak and Todd Steury for their help in the calculation and rationale of ICCs.
Supplementary Material
The Supplementary Material for this article can be found online at: https://www.frontiersin.org/articles/10.3389/fevo.2022.901303/full#supplementary-material
Footnotes
References
Adkins-Regan, E. (2005). Hormones and Animal Social Behavior. Princeton, NJ: Princeton University Press.
Alonso-Alvarez, C., Pérez-Rodríguez, L., Ferrero, M. E., de-Blas, E., Casas, F., and Mougeot, F. (2012). Adjustment of female reproductive investment according to male carotenoid-based ornamentation in a gallinaceous bird. Behav. Ecol. Sociobiol. 66, 731–742. doi: 10.1007/s00265-012-1321-8
Ardia, D. R., Broughton, D. R., and Gleicher, M. J. (2010). Short-term exposure to testosterone propionate leads to rapid bill color and dominance changes in zebra finches. Horm. Behav. 58, 526–532. doi: 10.1016/j.yhbeh.2010.04.004
Bates, D., Mächler, M., Bolker, B., and Walker, S. (2015). Fitting Linear Mixed-Effects Models Using lme4. J. Stat. Softw. 67, 1–48. doi: 10.18637/jss.v067.i01
Bathiany, S., Dakos, V., Scheffer, M., and Lenton, T. M. (2018). Climate models predict increasing temperature variability in poor countries. Sci. Adv. 4:eaar5809. doi: 10.1126/sciadv.aar5809
Bell, A. M., Hankison, S. J., and Laskowski, K. L. (2009). The repeatability of behaviour: a meta-analysis. Anim. Behav. 77, 771–783. doi: 10.1016/j.anbehav.2008.12.022
Bertin, A., Calandreau, L., Meurisse, M., Georgelin, M., Palme, R., Lumineau, S., et al. (2018). Incubation temperature affects the expression of young precocial birds’ fear-related behaviours and neuroendocrine correlates. Sci. Rep. 8, 1857. doi: 10.1038/s41598-018-20319-y
Bertrand, S., Alonso-Alvarez, C., Devevey, G., Faivre, B., Prost, J., and Sorci, G. (2006). Carotenoids modulate the trade-off between egg production and resistance to oxidative stress in zebra finches. Oecologia 147, 576–584. doi: 10.1007/s00442-005-0317-8
Blount, J. D. (2003). Carotenoid Modulation of Immune Function and Sexual Attractiveness in Zebra Finches. Science 300, 125–127. doi: 10.1126/science.1082142
Briga, M., Koetsier, E., Boonekamp, J. J., Jimeno, B., and Verhulst, S. (2017). Food availability affects adult survival trajectories depending on early developmental conditions. Proc. Biol. Sci. 284:20162287. doi: 10.1098/rspb.2016.2287
Buchanan, K. L. (2000). Stress and the evolution of condition-dependent signals. Trends Ecol. Evol. 15, 156–160. doi: 10.1016/S0169-5347(99)01812-1
Buchanan, K. L., Spencer, K. A., Goldsmith, A. R., and Catchpole, C. K. (2003). Song as an honest signal of past developmental stress in the European starling (Sturnus vulgaris). Proc. Biol. Sci. 270, 1149–1156. doi: 10.1098/rspb.2003.2330
Burley, N., and Coopersmith, C. B. (2010). Bill Color Preferences of Zebra Finches. Ethology 76, 133–151. doi: 10.1111/j.1439-0310.1987.tb00679.x
Carey, C. (2009). The impacts of climate change on the annual cycles of birds. Philos. Trans. R. Soc. B Biol. Sci. 364, 3321–3330. doi: 10.1098/rstb.2009.0182
Cockrem, J. F., Potter, M. A., Barrett, D. P., and Candy, E. J. (2008). Corticosterone Responses to Capture and Restraint in Emperor and Adelie Penguins in Antarctica. Zoolog. Sci. 25, 291–298. doi: 10.2108/zsj.25.291
Conway, C. J. (2000). Effects of ambient temperature on avian incubation behavior. Behav. Ecol. 11, 178–188. doi: 10.1093/beheco/11.2.178
Crino, O. L., and Breuner, C. W. (2015). Developmental stress: evidence for positive phenotypic and fitness effects in birds. J. Ornithol. 156, 389–398. doi: 10.1007/s10336-015-1236-z
Dalla, C., Antoniou, K., Drossopoulou, G., Xagoraris, M., Kokras, N., Sfikakis, A., et al. (2005). Chronic mild stress impact: Are females more vulnerable? Neuroscience 135, 703–714. doi: 10.1016/j.neuroscience.2005.06.068
French, N. A. (2009). The Critical Importance of Incubation Temperature. Avian Biol. Res. 2, 55–59. doi: 10.3184/175815509X431812
Gil, D., Heim, C., Bulmer, E., Rocha, M., Puerta, M., and Naguib, M. (2004). Negative effects of early developmental stress on yolk testosterone levels in a passerine bird. J. Exp. Biol. 207, 2215–2220. doi: 10.1242/jeb.01013
Hamilton, W., and Zuk, M. (1982). Heritable true fitness and bright birds: a role for parasites? Science 218, 384–387. doi: 10.1126/science.7123238
Hayes, J. P., and Jenkins, S. H. (1997). Individual Variation in Mammals. J. Mammal. 78, 274–293. doi: 10.2307/1382882
Hoffman, A. J., Finger, J. W., and Wada, H. (2018). Early stress priming and the effects on fitness-related traits following an adult stress exposure. J. Exp. Zool. Part Ecol. Integr. Physiol. 329, 323–330. doi: 10.1002/jez.2190
Jimeno, B., Hau, M., and Verhulst, S. (2018). Glucocorticoid-temperature association is shaped by foraging costs in individual zebra finches. J. Exp. Biol. 221:jeb187880. doi: 10.1242/jeb.187880
Krause, E. T., Krüger, O., and Schielzeth, H. (2017). Long-term effects of early nutrition and environmental matching on developmental and personality traits in zebra finches. Anim. Behav. 128, 103–115. doi: 10.1016/j.anbehav.2017.04.003
Kuznetsova, A., Brockhoff, P. B., and Christensen, R. H. B. (2017). lmerTest Package: tests in Linear Mixed Effects Models. J. Stat. Softw. 82, 1–26. doi: 10.18637/jss.v082.i13
Lindström, J. (1999). Early development and fitness in birds and mammals. Trends Ecol. Evol. 14, 343–348. doi: 10.1016/S0169-5347(99)01639-0
Loyau, T., Collin, A., Yenisey, Ç, Crochet, S., Siegel, P. B., Akşit, M., et al. (2014). Exposure of embryos to cyclically cold incubation temperatures durably affects energy metabolism and antioxidant pathways in broiler chickens. Poult. Sci. 93, 2078–2086. doi: 10.3382/ps.2014-03881
Lundy, H. (1969). “A review of the effects of temperature, humidity, turning and gaseous environment in the incubator on the hatchability of the hen’s egg,” in The Fertility and Hatchability of the Hen’s Egg. eds T. C. Carter and B. M. Freeman (Edinburgh, UK: Oliver and Boyd), 143–176.
MacDougall-Shackleton, S. A., and Spencer, K. A. (2012). Developmental stress and birdsong: current evidence and future directions. J. Ornithol. 153, 105–117. doi: 10.1007/s10336-011-0807-x
Marasco, V., Robinson, J., Herzyk, P., and Spencer, K. A. (2012). Pre- and post-natal stress in context: effects on the stress physiology in a precocial bird. J. Exp. Biol. 215, 3955–3964. doi: 10.1242/jeb.071423
Martin, J. G. A., and Réale, D. (2008). Temperament, risk assessment and habituation to novelty in eastern chipmunks. Tamias striatus. Anim. Behav. 75, 309–318. doi: 10.1016/j.anbehav.2007.05.026
Martins, T. L. F. (2004). Sex-specific growth rates in zebra finch nestlings: a possible mechanism for sex ratio adjustment. Behav. Ecol. 15, 174–180. doi: 10.1093/beheco/arg094
McGraw, K. (2006). Sex steroid dependence of carotenoid-based coloration in female zebra finches. Physiol. Behav. 88, 347–352. doi: 10.1016/j.physbeh.2006.04.003
McGraw, K. J., and Hill, G. E. (2000). Differential effects of endoparasitism on the expression of carotenoid- and melanin-based ornamental coloration. Proc. Biol. Sci. 267, 1525–1531. doi: 10.1098/rspb.2000.1174
McGraw, K. J., Lee, K., and Lewin, A. (2011). The effect of capture-and-handling stress on carotenoid-based beak coloration in zebra finches. J. Comp. Physiol. A 197, 683–691. doi: 10.1007/s00359-011-0631-z
Merrill, L., Naylor, M. F., and Grindstaff, J. L. (2016). Imperfect past and present progressive: beak color reflects early-life and adult exposure to antigen. Behav. Ecol. 27, 1320–1330. doi: 10.1093/beheco/arw029
Myers, B., Scheimann, J. R., Franco-Villanueva, A., and Herman, J. P. (2017). Ascending mechanisms of stress integration: implications for brainstem regulation of neuroendocrine and behavioral stress responses. Neurosci. Biobehav. Rev. 74, 366–375. doi: 10.1016/j.neubiorev.2016.05.011
Naguib, M., and Nemitz, A. (2007). Living with the Past: nutritional Stress in Juvenile Males Has Immediate Effects on their Plumage Ornaments and on Adult Attractiveness in Zebra Finches. PLoS One 2:e901. doi: 10.1371/journal.pone.0000901
Nakagawa, S., and Schielzeth, H. (2010). Repeatability for Gaussian and non-Gaussian data: a practical guide for biologists. Biol. Rev. 85, 935–956. doi: 10.1111/j.1469-185X.2010.00141.x
Negro, J. J., Figuerola, J., Garrido, J., and Green, A. J. (2001). Fat stores in birds: an overlooked sink for carotenoid pigments?: carotenoids in fat stores. Funct. Ecol. 15, 297–303. doi: 10.1046/j.1365-2435.2001.00526.x
Nelson, R. J. (2005). An introduction to behavioral endocrinology. 3rd ed. Sunderland, MA: Sinaeur Associates Inc.
NOAA (2022). NOAA National Centers for Environmental Information, State of the Climate: Global Climate Report for Annual 2021. Washington, DC: U.S. Department of Commerce.
Pérez-Rodríguez, L., Mougeot, F., and Alonso-Alvarez, C. (2010). Carotenoid-based coloration predicts resistance to oxidative damage during immune challenge. J. Exp. Biol. 213, 1685–1690. doi: 10.1242/jeb.039982
Rivier, C. (1993). Female Rats Release More Corticosterone Than Males in Response to Alcohol: influence of Circulating Sex Steroids and Possible Consequences for Blood Alcohol Levels. Alcohol. Clin. Exp. Res. 17, 854–859. doi: 10.1111/j.1530-0277.1993.tb00853.x
Rosenthal, M. F., Murphy, T. G., Darling, N., and Tarvin, K. A. (2012). Ornamental bill color rapidly signals changing condition. J. Avian Biol. 43, 553–564. doi: 10.1111/j.1600-048X.2012.05774.x
Rowe, M., Tourville, E. A., and McGraw, K. J. (2012). Carotenoids in bird testes: links to body carotenoid supplies, plumage coloration, body mass and testes mass in house finches (Carpodacus mexicanus). Comp. Biochem. Physiol. B Biochem. Mol. Biol. 163, 285–291. doi: 10.1016/j.cbpb.2012.06.005
RStudio Team (2021). RStudio: Integrated Development Environment for R. Boston, MA: Integrated Development Environment
Rubin, A. M., Choi, M. P., Hoffman, A. J., Beyl, H. E., Mendonça, M. T., and Wada, H. (2021). Periodic Cooling during Incubation Alters the Adrenocortical Response and Posthatch Growth in Zebra Finches. Physiol. Biochem. Zool. 94, 110–123. doi: 10.1086/713023
Sandell, M. I., Adkins-Regan, E., and Ketterson, E. D. (2007). Pre-breeding diet affects the allocation of yolk hormones in zebra finches Taeniopygia guttata. J. Avian Biol. 38, 284–290. doi: 10.1111/j.2007.0908-8857.03640.x
Sapolsky, R. M., Romero, L. M., and Munck, A. U. (2000). How Do Glucocorticoids Influence Stress Responses? Integrating Permissive, Suppressive, Stimulatory, and Preparative Actions. Endocr. Rev. 21, 55–89. doi: 10.1210/edrv.21.1.0389
Schneider, C. A., Rasband, W. S., and Eliceiri, K. W. (2012). NIH Image to ImageJ: 25 years of image analysis. Nat. Methods 9, 671–675. doi: 10.1038/nmeth.2089
Schull, Q., Dobson, F. S., Stier, A., Robin, J.-P., Bize, P., and Viblanc, V. A. (2016). Beak color dynamically signals changes in fasting status and parasite loads in king penguins. Behav. Ecol. 27, 1684–1693. doi: 10.1093/beheco/arw091
Schwabl, H. (1993). Yolk is a source of maternal testosterone for developing birds. Proc. Natl. Acad. Sci. 90, 11446–11450. doi: 10.1073/pnas.90.24.11446
Schwabl, H. (1996). Maternal testosterone in the avian egg enhances postnatal growth. Comp. Biochem. Physiol. A Physiol. 114, 271–276. doi: 10.1016/0300-9629(96)00009-6
Spencer, K. A., Heidinger, B. J., D’Alba, L. B., Evans, N. P., and Monaghan, P. (2010). Then versus now: effect of developmental and current environmental conditions on incubation effort in birds. Behav. Ecol. 21, 999–1004. doi: 10.1093/beheco/arq090
Spencer, K. A., Wimpenny, J. H., Buchanan, K. L., Lovell, P. G., Goldsmith, A. R., and Catchpole, C. K. (2005). Developmental stress affects the attractiveness of male song and female choice in the zebra finch (Taeniopygia guttata). Behav. Ecol. Sociobiol. 58, 423–428. doi: 10.1007/s00265-005-0927-5
Stoffel, M. A., Nakagawa, S., and Schielzeth, H. (2017). rptR: repeatability estimation and variance decomposition by generalized linear mixed-effects models. Methods Ecol. Evol. 8, 1639–1644. doi: 10.1111/2041-210X.12797
Valenzuela, N., Literman, R., Neuwald, J. L., Mizoguchi, B., Iverson, J. B., Riley, J. L., et al. (2019). Extreme thermal fluctuations from climate change unexpectedly accelerate demographic collapse of vertebrates with temperature-dependent sex determination. Sci. Rep. 9:4254. doi: 10.1038/s41598-019-40597-4
Van Oort, H., and Dawson, R. D. (2005). Carotenoid ornamentation of adult male Common Redpolls predicts probability of dying in a salmonellosis outbreak. Funct. Ecol. 19, 822–827. doi: 10.1111/j.1365-2435.2005.01035.x
Verhulst, S., Holveck, M.-J., and Riebel, K. (2006). Long-term effects of manipulated natal brood size on metabolic rate in zebra finches. Biol. Lett. 2, 478–480. doi: 10.1098/rsbl.2006.0496
Wada, H. (2019). Damage-Fitness Model: the missing piece in integrative stress models. Stress 22, 548–562. doi: 10.1080/10253890.2019.1614556
Wada, H., and Coutts, V. (2021). Detrimental or beneficial? Untangling the literature on developmental stress studies in birds. J. Exp. Biol. 224:jeb227363. doi: 10.1242/jeb.227363
Wada, H., Kriengwatana, B., Allen, N., Schmidt, K. L., Soma, K. K., and MacDougall-Shackleton, S. A. (2015). Transient and permanent effects of suboptimal incubation temperatures on growth, metabolic rate, immune function and adrenocortical responses in zebra finches. J. Exp. Biol. 218, 2847–2855. doi: 10.1242/jeb.114108
Wada, H., Salvante, K. G., Stables, C., Wagner, E., Williams, T. D., and Breuner, C. W. (2008). Adrenocortical responses in zebra finches (Taeniopygia guttata): individual variation, repeatability, and relationship to phenotypic quality. Horm. Behav. 53, 472–480. doi: 10.1016/j.yhbeh.2007.11.018
Wickham, H., Averick, M., Bryan, J., Chang, W., McGowan, L., François, R., et al. (2019). Welcome to the Tidyverse. J. Open Source Softw. 4:1686. doi: 10.21105/joss.01686
Wolak, M. E., Fairbairn, D. J., and Paulsen, Y. R. (2012). Guidelines for estimating repeatability: guidelines for estimating repeatability. Methods Ecol. Evol. 3, 129–137. doi: 10.1111/j.2041-210X.2011.00125.x
Zann, R. (1996). The Zebra Finch: A Synthesis of Field and Laboratory Studies. Oxford: Oxford University Press.
Zann, R. A., Morton, S. R., Jones, K. R., and Burley, N. T. (1995). The Timing of Breeding by Zebra Finches in Relation to Rainfall in Central Australia. Emu Austral. Ornithol. 95, 208–222. doi: 10.1071/MU9950208
Keywords: ornamentation, incubation temperature, beak coloration, zebra finch (Taeniopygia guttata), fluctuating temperature, behavioral stress response
Citation: Choi MP, Rubin AM and Wada H (2022) Suboptimal Embryonic Incubation Temperature Has Long-Term, Sex-Specific Consequences on Beak Coloration and the Behavioral Stress Response in Zebra Finches. Front. Ecol. Evol. 10:901303. doi: 10.3389/fevo.2022.901303
Received: 21 March 2022; Accepted: 08 June 2022;
Published: 24 June 2022.
Edited by:
John Cockrem, Massey University, New ZealandReviewed by:
Blanca Jimeno, University of Castilla La Mancha, SpainE. Tobias Krause, Friedrich-Loeffler-Institute, Germany
Copyright © 2022 Choi, Rubin and Wada. This is an open-access article distributed under the terms of the Creative Commons Attribution License (CC BY). The use, distribution or reproduction in other forums is permitted, provided the original author(s) and the copyright owner(s) are credited and that the original publication in this journal is cited, in accordance with accepted academic practice. No use, distribution or reproduction is permitted which does not comply with these terms.
*Correspondence: Madeline P. Choi, bXBsMDAxMUBhdWJ1cm4uZWR1
†Present address: Alexander M. Rubin, The University of Sydney, Sydney, NSW, Australia