- 1Department of Geography and Spatial Information Techniques, Ningbo University, Ningbo, China
- 2State Key Laboratory of Freshwater Ecology and Biotechnology, Institute of Hydrobiology, Chinese Academy of Sciences, Wuhan, China
- 3Ningbo University Library (Information Center), Ningbo University, Ningbo, China
Being increasingly constructed worldwide, dams are a main driver of flow regime change and biodiversity decline. Although small run-of-river dams have exceeded the number of large dams, their impacts on taxonomic and functional β-diversity as well as community assembly process of aquatic organisms have been largely neglected. Ninety sites within twenty three small run-of-river dams in the Xiangxi River were selected, and the hydrological and physicochemical variables for each site were measured. We analyzed the traits and β-diversity of benthic diatoms, and explored the key driving mechanism of benthic diatom community assembly. Our results indicated that the construction of small run-of-river dams could affect the β-diversity of benthic diatoms and the mechanism of community assembly. Specifically, we found that small run-of-river dams could change the relative contribution of nestedness components to the trait-based β-diversity of benthic diatoms, but generally the taxonomy-based β-diversity was relatively higher than the trait-based β-diversity. Furthermore, the community assembly process of benthic diatoms was also affected. In areas affected directly by small run-of-river dams, dispersal assembly was the key mechanism for community assembly. Compared to unregulated habitats, the dispersal assembly process between the impacted and the unregulated habitats has been enhanced. We advocate that this study can be expanded to other organisms (such as macroinvertebrates, phytoplankton, fish) in future to fully understand impacts of small run-of-river dams on biodiversity from a multi-trophic level aspect. Based on our results, we suggest that maintaining genetic and ecological connectivity based on an effective impact assessment in dry seasons is a potential solution to mitigate the impacts of such dams, as key to adaptive management and sustainability.
Introduction
Due to its economic benefits, hydropower, as a clean and renewable energy source, often becomes the first choice in most countries (Huang and Yan, 2009). The hydropower plants can be divided into large hydropower plants (LHPs) and small hydropower plants (SHPs). The construction of LHPs brings huge social and ecological costs, such as negative impacts on water volume and quality, biodiversity, fisheries and other ecosystem services (Ansar et al., 2014; Kahn et al., 2014; Deemer et al., 2016). In contrast, SHP, as an important part of the future energy strategy, has become the consensus of all countries in the world due to its relatively small impact on the environment (Paish, 2002; Tang et al., 2012). Studies have shown that there are 82,891 SHPs in operation or under construction around the world. If all potential power generation capacity is to be developed, the number of SHPs will be twice as many as it is now (Couto and Olden, 2018). The construction of hydropower plants will affect the ecosystem in various ways, such as changing the continuity of rivers, changing water quality, increasing habitat fragmentation and degradation, and loss of biodiversity (Mallik and Richardson, 2009; Vaikasas, 2010). The research on the impact of LHPs on freshwater biological communities is relatively mature, but the research on the environmental impact of SHPs is still rare (Jia et al., 2009; Atilgan and Azapagic, 2016; de Faria et al., 2017; Aung et al., 2020). With the increasing number of SHPs, more and more scholars are aware of their impact on river ecosystems (Baskaya et al., 2011; Lange et al., 2018; Sarauskiene et al., 2021).
β-diversity not only includes the relationship between α-diversity and γ-diversity, but also can show the changes in species composition on a temporal and spatial scale. Recent advances in ecology believe that differences in species composition between communities originate from two different processes: species turnover and nestedness (Baselga, 2010). Among them, species turnover means species replacement between different communities, while a nestedness pattern presents if the communities with lower species richness is a subset of the communities with higher species richness (James et al., 2012; Staniczenko et al., 2013). β-diversity decomposition is to distinguish the effects of these two processes on total β-diversity, and to explore how these two processes together affect species distribution patterns in different temporal and spatial dimensions (Baselga, 2010; Podani and Schmera, 2011). Decomposition facilitates a deeper understanding of the distribution pattern of biodiversity and its maintenance mechanism (Baselga and Leprieur, 2015; Wu et al., 2022).
The formation of β-diversity is considered to be the result of combined effects of the two ecological processes of niche and dispersal (Soininen et al., 2007). The niche process believes that the range of the distribution area of a species is determined by the adaptation characteristics of the environment, while the dispersal process believes that the spreading ability of the species determines the distribution of the species (Green and Ostling, 2003). Probing the importance of niche and dispersal process in community assembly is conducive to strengthen the understanding of community assembly and protect biodiversity. Gaining more information on β-diversity allows for the correct identification of key mechanisms of community assembly for efficient selection of suitable protected areas (Sarkar, 2006). However, there are still controversies about how to apply appropriate statistical analysis methods to study β-diversity (Legendre et al., 2005; Tuomisto and Ruokolainen, 2006, 2008). The null model and variation partitioning methods are commonly used methods to quantify the process of community assembly, but they also have limitations. For example, the null model method relies on detailed data on community composition, environmental factors, and geographic location. Variation partitioning method cannot correctly represent the environmental and spatial components of community variation, making it difficult to explain the underlying process (Smith and Lundholm, 2010; Clappe et al., 2018). To overcome the data and methodological limitations of previous methods, a dispersal-niche continuum index (DNCI) has been proposed based on PER-SIMPER, which can effectively identify community assembly mechanisms (Vilmi et al., 2021). DNCI measures the relative strength of the community assembly process in a simple and easy way, which can be compared across data sets. Vilmi et al. (2021) developed DNCI based on stream diatoms, macroinvertebrates, fish, bacteria, and macroinvertebrates data and has been implemented in fleas and small mammals community assembly (Gibert et al., 2021). The construction of small run-of-river dams has caused the fragmentation of rivers and is likely to change the community construction mechanism. Currently, relevant research is still blank.
Benthic algae in rivers have the advantages of short life cycle, easy access, sensitive to physical and chemical changes in water bodies. As a consequence, they are increasingly used as indicators for water quality assessment (Lepisto et al., 2004; Wu et al., 2017). However, the current research on the impact of small run-of-river dam construction is mainly focusing on taxonomy-based α-diversity. For example, Wu et al. (2010) found that the construction of small run-of-river dams had affected the richness, diversity and traits of benthic diatoms. To our best knowledge, there are no studies on the impact of biological β-diversity yet.
In this study, we examined the potential impacts of small run-of-river dams from a Chinese mountain catchment (i.e., the Xiangxi River with 5 different habitat groups: H01–H05) on taxonomy- and trait-based β-diversity of benthic diatom communities and their components (i.e., turnover and nestedness) (Figure 1). We had three main research questions: (i) can small run-of-river dams change the relative contributions of turnover and nestedness components to total taxonomy- and trait-based β-diversity of benthic diatom communities? (ii) what is the key mechanism of community assembly (dispersal assembly, niche assembly or joint dispersal-niche assembly) in the area impacted by small run-of-river dams? (iii) do small run-of-river dams affect the community assembly process of benthic diatoms? Since species sharing similar niches are grouped into same traits, taxonomic species replacements do not always result in traits turnover. Furthermore, anthropogenic activities such as dam construction often cause segmentation and homogenization of stream habitats, and taxa in similar environments tend to share common traits (Goldenberg Vilar et al., 2014; Castro et al., 2018). Thus, we hypothesized that taxonomy-based β-diversity should be relatively higher, particularly a higher turnover, than trait-based β-diversity (HY1); the two facets of β-diversity at impacted habitats (e.g., H03 and H05) should be relatively lower than those at unregulated habitats (HY2). Since the main consequences from dam construction are losses of stream connectivity, we expected the dominant community assembly processes of both species and trait compositions will be affected. More specifically, the dispersal assembly process should be intensified between the impacted habitats (e.g., H03 and H05) and unregulated habitats (HY3).
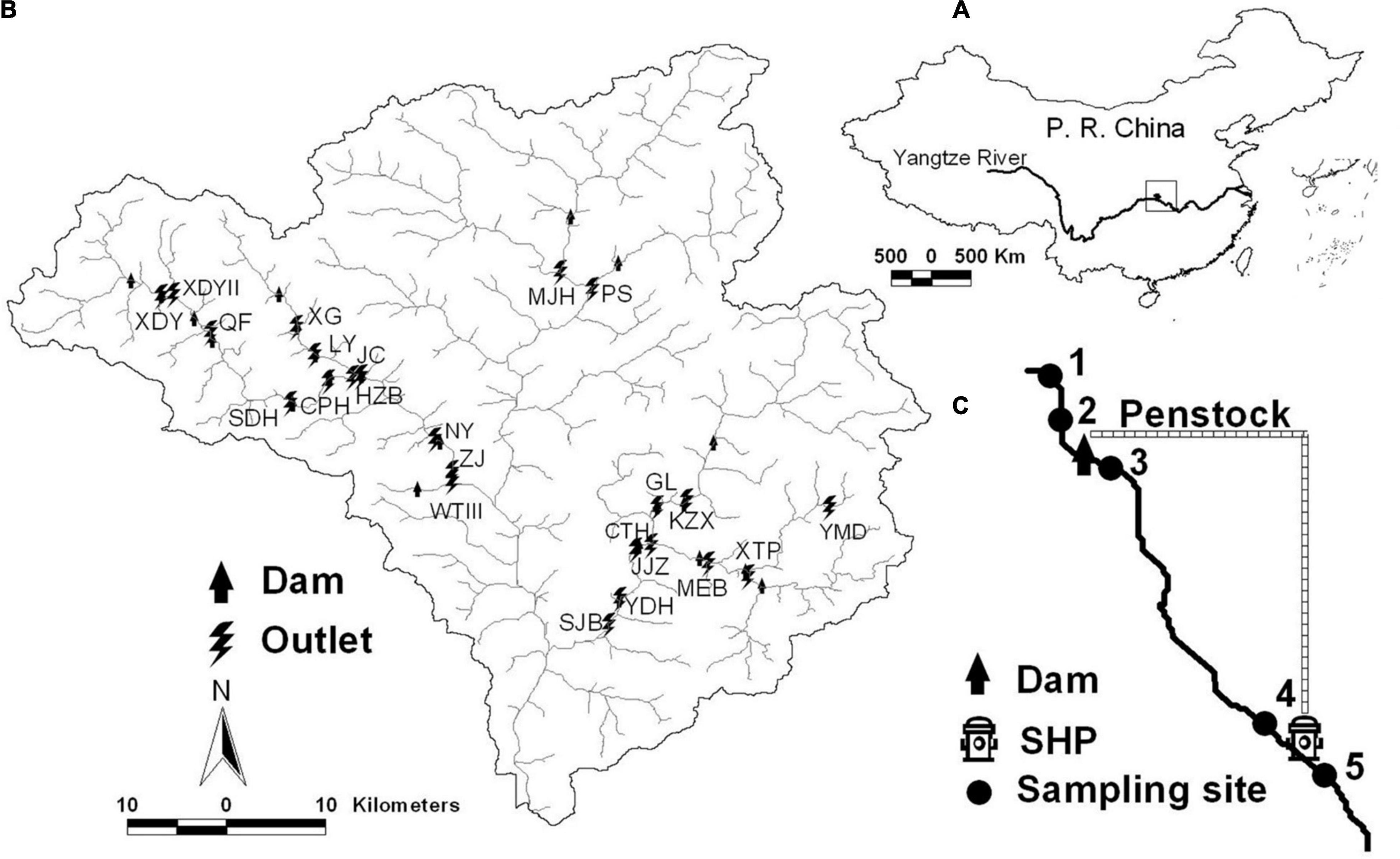
Figure 1. Small run-of-river dams within the Xiangxi River watershed (B) in the People’s Republic (P. R.) of China (A) and sketch map of sampling sites (C). SHP, small hydropower station; letters in (B) are names of SHPs. The figure was modified from Wang et al. (2022).
Materials and Methods
Study Area
Xiangxi River, originating from Shennongjia Mountain, lies in the west of Hubei Province. It is the largest river in Hubei Province and the closest tributary to the Three Gorges Dam. It has a total length of 469.7 km and an area of 3,099.4 km2 (Fu et al., 2006). The characteristic of the river course is typical, and the basin is very rich in precipitation and water resources. In order to make full use of hydropower resources, many small run-of-river dams have been built on both sides of the Xiangxi River. Most of the water in the river channel is introduced into special penstocks by using the elevation difference to generate electricity, and only a small part of the river water is left to maintain the normal function of the river channel. In the Xiangxi River Basin, 23 small run-of-river dams and 5 sites on each of the river sections for investigation were selected (S1–S5) (Figure 1). Since the small run-of-river dams constructed by cascades caused site overlap, we selected a total of 90 sites for sampling in October 2005. 5 sites were selected before and after the small dams. S1 and S2 were located upstream of the water intake. S1 selected a point where the river course was not affected by the dam, which was located about 50–100 m upstream of the dam. S2 was located at upstream of the dam and was less affected. S3 and S4 were located between the water intake and the water outlet. S3 was a pool formed by overflow and erosion in the rainy season under the water intake. S4 was located at upstream of the water outlet, and the water flow could only be restored when the downstream of S3 collects water. S5 was a deep pool formed downstream of the water outlet. We divided the 90 sites into 5 habitat groups (H01–H05) according to the impact degree of small run-of-river dams. H01–H05 correspond to S1–S5, respectively.
Field Sampling and Processing
The sampling process was divided into two parts: sampling and identification. Algae samples were collected at the 90 sites. At each sample point, three to five representative stones with a diameter of ∼25 cm were selected, and the algae in the sampling area with a diameter of 2.7 cm was brushed, and then rinsed with 350 ml distilled water. Algae samples were then preserved with 4% formalin for subsequent identification. The identification of benthic algae included two steps, the identification of non-diatoms and the preparation of permanent diatom slides. First, a 400× magnification microscope was used to identify non-diatoms in the 0.1 ml counting chamber. Second, permanent diatom slides were prepared with nitric acid and sulfuric acid. We counted at least 300 diatom valves using a 1,000× magnification microscope under oil immersion conditions. We used available information to determine algae as the lowest classification level from Anonymous (1992). Hu et al. (1980), Zhang and Huang (1991), and Zhu (2000). Ind/m2 represented the unit of density of benthic algae. As the most abundant group of benthic algae in the Xiangxi River was diatoms (about 80% of the total abundance) (Wu et al., 2010, 2012), we focused on diatoms in the following analyses.
Environmental Variables
Hydrological factors such as river width (Width), water depth (Depth), and velocity (Velocity) (using LJD-10 water current meter; Chongqing hydrological machines manufactory, Chongqing, China) were measured at each point. Meanwhiles, water temperature (WT), dissolved oxygen (DO), calcium concentration (Ca), pH, conductivity (COND), turbidity (TURB), total dissolved solids (TDS), salinity (Sal), Chloride (Cl), and oxidation reduction potential (orp) were measured in situ using a Horiba W-23XD (multiprobe sonde). ∼1 L of river water was collected in a pre-cleaned bottle to measure the two chemical variables of phosphate (PO4) and total phosphorus (TP) in the laboratory (Chinese Environmental Protection Bureau, 1989).
Diatom Traits
The benthic diatoms collected at 90 sampling points were classified by trait. The trait information of all observed species comes from the literature (Passy, 2007; Wagenhoff et al., 2013; Wu et al., 2017; Witteveen et al., 2020). We divided it into three categories: cell size, guild, and life form, which included 15 different traits: cell size (large, macro, meso, micro, or nano), guild (high profile, low profile, motile, or planktonic guild), and life form (filamentous, unicellular, or colonial life form; high, medium, or low attachment) (Table 1). We have chosen as the study area the reaches of the river where small run-of-river dams are located to ensure that all communities at least partially share the same regional species pool.
Data Analysis
R (version 4.0.2) (R Core Team, 2020) was used to perform all analyses. First, we decomposed β-diversity into two aspects: taxonomy- and trait-based β-diversity. Using the beta.pair function in the R package betapart, based on presence-absence data (using Sørensen distance), the total taxonomy-based β-diversity was divided into two parts: turnover and nestedness (Baselga and Orme, 2012). The trait-based β-diversity matrix included three components: total β-diversity, turnover, and nestedness, which were generated using the above 15 characteristics (using Sørensen distance). First, the gowdis function in the R package FD was used to calculate the distance between species based on trait data. Second, the Gower distance was used for principal coordinate analysis (PCoA), and the pco function in the R package labdsv was used to generate the trait vector. Third, the function functional.beta.pair in the R package betapart was used to generate three trait-based β-diversity matrices, using the first two PCoA vectors and species data. By decomposing β-diversity, we can know the relative contribution of turnover and nestedness components to the total taxonomy- and trait-based β-diversity, and compare the differences among the 5 different habitats (H01–H05) (question i). Furthermore, we ran Mantel test (using the function mantel in R package vegan) (Oksanen et al., 2019) to test the relationship between taxonomy- and trait-based β-diversity components to examine whether both facets of β-diversity provide complementarity of ecological information.
To explore what is the key mechanism of community assembly processes in the study area impacted by small run-of-river dams (question ii) and how do they affect community assembly processes of benthic diatoms (question iii), we calculated and compared the pairwise DNCI values (function DNCI_multigroup in R package DNCImper) (Gibert, 2021) for both species and trait composition of benthic diatoms among 5 different habitats. The DNCI can effectively estimate whether dispersal or niche processes dominate community assembly without environmental or spatial data, and it allows the comparisons of processes among different datasets (Vilmi et al., 2021). At the same time, the DNCI method relies on the distribution of taxa, which can better clarify the importance of the niche and dispersal process in the community assembly, and strengthen the understanding of the community assembly (Chase et al., 2011; Vilmi et al., 2021). In addition, the interpretation of DNCI values is relatively simple: if the DNCI is not significantly different from 0, we can assume that the dispersal and niche processes contribute equally to variations in community composition; if the DNCI is significantly lower than 0, dispersal processes are the primary determinants of community composition, whereas if the DNCI is significantly higher than 0, community composition is mainly driven by niche processes. In order to produce more robust results, we made pairs of groups even by random sampling of the largest groups (i.e., symmetrize = TRUE).
Results
Environmental Variables
Hydrological factors such as velocity, width and depth were obviously affected by the construction of small run-of-river dams (Supplementary Table 1), especially habitats such as H03, H05 that were disconnected due to the small dams. The rest 12 environmental variables (i.e., WT, DO, TP, PO4, pH, Sal, COND, orp, TURB, TDS, Cl, and Ca) had no significant differences among the 5 habitat groups (H01–H05). It could be seen that the construction of small run-of-river dams mainly affected hydrological factors, while no obvious impact was found on other physical and chemical variables.
Small Run-of-River Dams Affect Two Facets of β-Diversity and Its Components
The main patterns observed in the Xiangxi River Basin were turnover of taxonomy-based β-diversity and nestedness of trait-based β-diversity, and the contribution of turnover (0.489) was greater than nestedness (0.338) (Figure 2). As expected by HY1, taxonomy-based β-diversity (0.584) was relatively higher than trait-based β-diversity (0.243). The contribution of nestedness to trait-based β-diversity was significantly lower in impacted habitats (e.g., H03: 0.147, H05: 0.143) than unregulated habitats (e.g., H01: 0.214, H02: 0.253, H04: 0.232). From the perspective of environmental variables, the construction of small run-of-river dams had a great impact on the hydrological factors of H03 and H05 two types of habitats. The difference between habitats had a great influence on the trait of the benthic diatom community, and changed the relative contribution of nestedness components to the total trait-based β-diversity of the benthic diatom community, which answered our first question (question i). It could be seen from the Figure 2 that the total trait-based β-diversity of impacted habitats (e.g., H03: 0.192, H05: 0.168) were lower than the unregulated habitats (e.g., H01: 0.257, H02: 0.296, H04: 0.269), partially supporting our HY2.
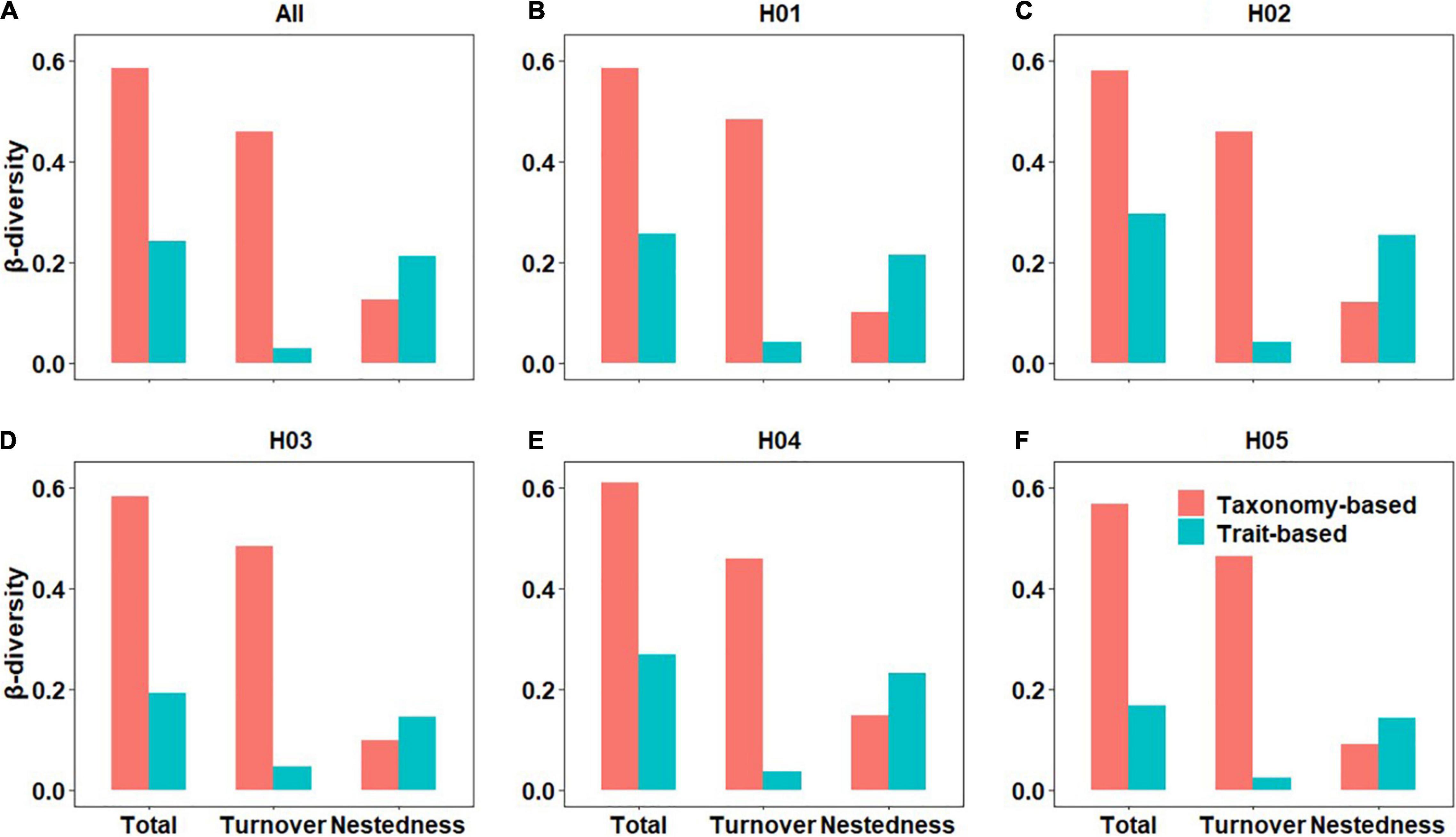
Figure 2. Statistical descriptions of two facets (taxonomy- and trait-based) of the β-diversity components (i.e., total, turnover, and nestedness) for benthic diatoms at all sites (A) and different habitat groups (B–F, H01–H05).
The Mantel test showed that the correlation between taxonomy- and trait-based β-diversity components were significant (p < 0.001), but correlation coefficient (i.e., Mantel r) was not high, indicating that the two provide different ecological information. Among them, the Mantel correlation coefficient between nestedness was the highest (r = 0.474), followed by total β-diversity (r = 0.188) and turnover (r = 0.149) (Supplementary Figure 1). In different habitat groups, the results of the Mantel test were different. Compared with other habitat groups, the Mantel test showed that the nestedness in the two impacted habitats H03 (r = 0.413) and H05 (r = 0.558) was higher, followed by turnover (H03: r = 0.302, H05: r = 0.364), the correlation was significant (p < 0.001) (Supplementary Figures 2–6). However, Mantel correlation coefficient between nestedness in H04 (r = 0.837) was the highest, which was significant (p < 0.001) (Supplementary Figure 5). There were also insignificant correlations in different habitat groups.
Small Run-of-River Dams Influence Community Assembly Process
The pairwise DNCI values of 5 different habitat groups of benthic diatom species and traits were all negative (Figure 3), demonstrating that dispersal was the main assembly process. This answered our second question (question ii), and we found that the key mechanism of small run-of-river dams affecting benthic diatom communities was dispersal assembly. Among the pairwise DNCI values based on species, the highest absolute value appeared in the comparison between H03 and H05 (DNCI = –11.01), indicating that the potential intensity of the dispersal-dominant assembly process between H03 and H05 was the largest, while the absolute value of DNCI between H02 and H04 was the lowest (DNCI = –6.27), indicating that the intensity of dispersal was the smallest (Supplementary Table 2). However, in the pairwise DNCI values based on trait, the absolute value of DNCI between H02 and H03 was the highest (DNCI = –16.90), followed by H03 and H05 (DNCI = –10.76), and the smallest absolute value between H01 and H04 (DNCI = –6.27) (Supplementary Table 2). The absolute value of the pairwise DNCI average value based on trait (DNCI = –8.74) was greater than the absolute value of the pairwise DNCI average value based on species (DNCI = –8.37), reflecting the greater intensity of the dominant process of dispersal of the trait (Supplementary Table 2). We found that whether it is based on species or trait, the absolute values of DNCI between other habitats and H03 and H05 are relatively large, which answered our third question (question iii). Small run-of-river dams affected the community assembly process of benthic diatoms. Due to the construction of small run-of-river dams, the dispersal assembly process between impacted habitats (e.g., H03 and H05) and unregulated habitats has been strengthened, confirming our third hypothesis (HY3).
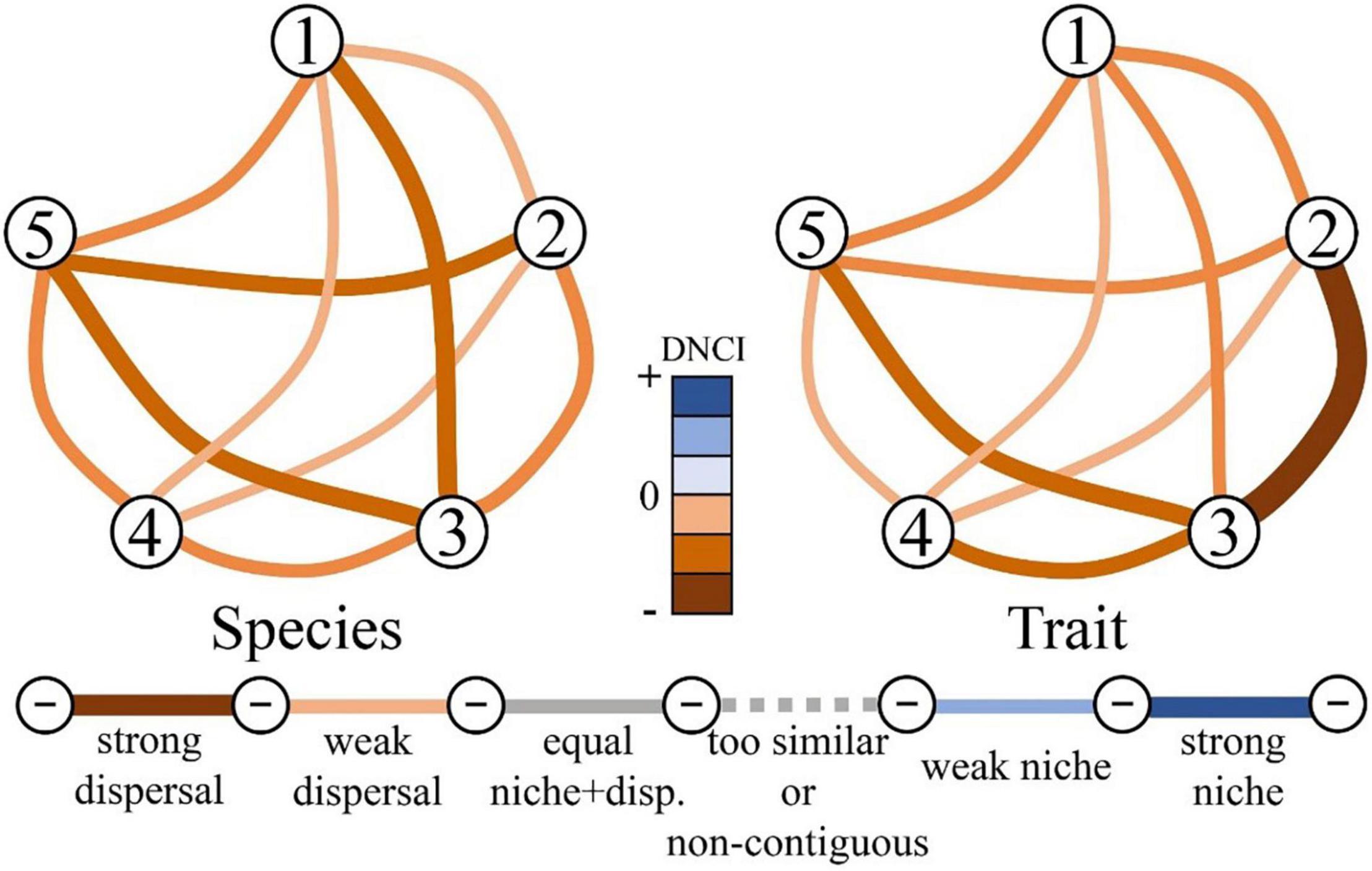
Figure 3. Dispersal-niche continuum index (DNCI) value calculated based on species and trait. The overall and pairwise analysis are pictured as a colored gradient bar and network plots, respectively. The width and color of the links between nodes correspond to assembly process intensity. The darker and wider the link, the stronger the assembly process. The figure on the left is based on species, whereas figure on the right is based on trait. ➀–➄ corresponds to H01–H05.
Discussion
Small Run-of-River Dams Changed the Contribution Rate of Turnover and Nestedness
As hypothesized by HY1, for the benthic diatom assemblages in the entire study area, taxonomy-based β-diversity was higher, particularly a higher turnover, than trait-based β-diversity. In the context of spatial and environmental gradients, factors such as competition, geographic barriers, and environmental filtering can all lead to species turnover (Angeler, 2013; Gutiérrez-Cánovas et al., 2013; Legendre, 2014). We speculated that the reason why the species turnover of the Xiangxi River Basin was much higher than that of nestedness may be as follows. First of all, the construction of small run-of-river dams led to the fragmentation of habitats, and there were obvious differences in hydrological variables in the watershed. Geographical isolation might limit the dispersal of benthic diatoms that were originally continuously dispersal, leading to the formation of allopatric speciation (Leprieur et al., 2011). Through species filtering on environmental gradients, different species appeared in their specific habitats suitable for survival, which led to rapid species turnover among communities. Second, huge human disturbances such as the construction of intensive small run-of-river dams have provided abundant hydropower resources, but they have caused huge changes to the river habitat and overall environmental conditions of the Xiangxi River Basin, and may also affect the current benthic diatom communities. Priority effect might also play a role here. In a specific habitat, species that colonize first would reject species that arrive later, resulting in a higher rate of species turnover between communities (Fukami, 2015). The main contribution of turnover to taxonomy-based total β-diversity was also found in previous studies (Rocha et al., 2019; Branco et al., 2020; Wu et al., 2021).
The trait-based β-diversity in the impacted habitats (such as H03, H05) was relatively lower than that of unregulated habitats, but the taxonomy-based β-diversity was not much different, which partially supported our HY2. The construction of small run-of-river dams changed the relative contribution of nestedness component to the trait-based β-diversity of benthic diatom communities, but had little effect on the taxonomy-based β-diversity. Due to the construction of small run-of-river dams, the two habitat groups H03 and H05 have been greatly affected. The hydrological factors of H03 and H05, such as velocity, width and depth, were very different from other habitat groups. Similarly, H05 was a deep pool formed by the outlet of a small run-of-river dams. Habitat homogeneity and dispersal limitations due to huge human disturbance might cause the traits of benthic diatoms to converge, resulting in lower trait-based β-diversity (Logez et al., 2010; Svenning et al., 2011). For example, benthic diatoms in high-velocity habitats mostly exhibit high attachment (Wang et al., 2022). In some highly heterogeneous habitats, benthic diatoms also showed the characteristics of high heterogeneity. Perhaps we could also use selective extinction to explain why nestedness had little effect on trait-based β-diversity in these two habitats. Because different benthic diatoms had different sensitivity to environmental changes, species with higher sensitivity were likely to disappear in habitats with higher environmental pressure due to selective extinction, but species with higher tolerance to environmental changes could survive in the habitat (Gutiérrez-Cánovas et al., 2013; Si et al., 2017). Nestedness was dominant in trait-based β-diversity patterns, which was in the line with previous studies (Rocha et al., 2019; Wu et al., 2021).
Mantel test showed that the correlation coefficient between taxonomy- and trait-based β-diversity components was not high, indicating that they provided different ecological information. We compared the contribution of turnover and nestedness to β-diversity, and found that the taxonomy-based total β-diversity was much higher than that of total trait-based β-diversity. The reason was that the turnover of trait-based β-diversity was lower. Due to the limited dispersal caused by the construction of small run-of-river dams, the functional convergence between benthic diatoms might lead to lower trait-based β-diversity (Logez et al., 2010; Svenning et al., 2011). In trait-based β-diversity, the contribution of nestedness was greater than the turnover. This indicated that the benthic diatom community in the Xiangxi River Basin has experienced a high level of trait convergence. For the nestedness contribution of trait-based β-diversity, impacted habitats (e.g., H03 and H05) were lower than unregulated habitats. This showed that the construction of small run-of-river dams had a great impact on the trait of the benthic diatom community, and reduced the relative contribution of nestedness components to its total β-diversity.
The large amount of overlap between benthic diatom assemblages in the functional space could explain the low level of functional conversion. Among the 5 habitat groups, the water flow of H04 can only be restored after H03 water is collected. Human disturbance led to high homogeneity of the habitat, and the nestedness of environmental conditions led to the high nestedness of trait (Rocha et al., 2019). H03 and H05 were also seriously affected by the construction of small run-of-river dams. Compared with turnover, nestedness also played a greater role in community composition.
Assembly Process
Community assembly is affected by both the dispersal process and the niche process, but the relative importance of the two processes differs depending on the scale, geographic area, and species group (Qian and Ricklefs, 2007; Buckley and Jetz, 2008). The dispersal process considers that the dispersal of a single species tends to be concentrated, and the ability of dispersal determines the distribution of species (Ney-Nifle and Mangel, 1999; Green and Ostling, 2003). The significantly negative DNCI result did not indicate that the niche-based process did not play a role in the community assembly process, but represented that the niche process was weak, and its role in species assembly was not as important as the dispersal-based process. They always appear in natural combinations (Araújo and Rozenfeld, 2014). By comparing multiple DNCI values, the differences in the assembly process between different groups can be more accurately identified. In our research, all 5 different habitats had the advantage of dispersal-based assembly process. The species and trait of benthic diatoms mainly depended on historical processes (dispersal). This answered our second question (question ii). In areas impacted by small run-of-river dams, dispersal assembly was a key mechanism for community assembly.
Studies have found that the structure of diatom communities can be explained by environmental and spatial variables in river, such as altitude, substrate type, and water quality effects (Schmera et al., 2018; Wang et al., 2020). Hydrological, physical and chemical factors might also affect the process of community assembly (Isabwe et al., 2018). Wu et al. (2021) found that spatial and local environmental factors exceeded the geographic climate gradient when constructing the β-diversity of benthic diatoms based on taxonomy and trait. The increase in flood residence time leads to changes in the chemical and physical properties of the water body, which affects community assembly and abundance (Corline et al., 2021). The construction of small run-of-river dams has led to changes in the hydrological and environmental factors between habitat groups, and also has a certain impact on hydrological connectivity. Studies have found that hydrological connectivity was a key factor affecting the assembly of phytoplankton communities (Lopez-Delgado et al., 2020; Meng et al., 2020). Hydrological connectivity affected the structure and assemblage of phytoplankton by affecting the spatial variability of CDOM, and might also interact with species dispersal functions, thereby affecting community assembly (Mayora et al., 2016). The penstock connecting H02 and H05 transports river water directly from H02 to H05, causing the connected environment to be cut off, but not completely. The different intensities of the dispersal capacity among these habitats might be caused by changes in some environmental factors between the habitat groups due to the construction of small run-of-river dams, such as velocity, width, and depth. The extremely small negative value of DNCI indicated severe habitat homogeneity, resulting in no significant difference in species richness and trait richness of benthic diatom communities. Whether based on species or trait, the absolute value of DNCI between other habitat groups and H03 and H05 was relatively large, this showed that especially in the habitat separated by the construction of small run-of-river dams, the signs of dispersion driving benthic diatom communities were particularly obvious. The construction of small run-of-river dams seriously affected the environmental factors between habitats, resulting in insufficient ecological differences between habitats. It answered our third question (question iii). Small run-of-river dams affected the community assembly process of benthic diatoms, especially the impacted habitats (e.g., H03 and H05). This also supported HY3 that the construction of small run-of-river dams have affected the community assembly process of benthic diatoms, and strengthened the dispersal assembly process between the impacted habitat and the unregulated habitat.
Management Implications
β-diversity decomposition is to explore how the two processes of species turnover and nestedness together affect species distribution patterns in different spatial and temporal dimensions, and helps to understand the distribution patterns and driving mechanisms of various biological groups (Baselga, 2010; Podani and Schmera, 2011). But at present, the research on the β-diversity of benthic diatoms is mostly based on taxonomy, whereas little attention is paid to the trait-based β-diversity (Rocha et al., 2019; Branco et al., 2020; Wu et al., 2021). In the spatial dimension, the species turnover and nestedness components of β-diversity can reflect different conservation strategies. Exploring how the two processes of species turnover and nestedness together affect species distribution patterns is particularly critical in the research of biogeography and conservation biology (Williams, 1996; Baselga and Leprieur, 2015; Socolar et al., 2016). In our research, the turnover process dominated the taxonomy-based total β-diversity, which means that all research sites contribute similarly to β-diversity, which can help decision makers decide on protected areas and conduct restoration progress assessments (Lopez-Delgado et al., 2020). Therefore, in river management, in addition to considering severely homogenized habitats, it should also include highly heterogeneous habitats without priority (Baselga, 2010; Gutiérrez-Cánovas et al., 2013).
In order to better protect biodiversity and maintain the healthy development of ecosystems, areas with high trait diversity should be protected first, and then the trait diversity of impacted habitats should be restored. Due to the differences in β-diversity among taxa, a conservation plan based on one taxon may not be able to protect the diversity of other taxa well. In the future, this study can be expanded to other organisms (such as macroinvertebrates, phytoplankton, fish) to study species distribution patterns and interactions, so as to fully understand the impact of small run-of-river dams on biodiversity from a multi-trophic level aspect, and provide suggestions on the planning and layout of the protected area.
From the research results, species with similar biological taxonomy would replace each other, and dispersal assembly was the key mechanism of community assembly in the study area. Changes in the composition of benthic diatom communities may be better explained by spatial distance and environmental variables. Due to the construction of small run-of-river dams, river connectivity has been interrupted, and there were large differences in hydrological variable between habitat environments. The distribution of species with strong dispersal ability may be more restricted by the niche process. The stronger the dispersal ability, the more likely it can occupy a suitable habitat. Ecological flow refers to the minimum flow required to maintain or restore the basic structure and function of the river, lake, and reservoir ecosystem in order to ensure the ecological service function of the river (Poff and Matthews, 2013). Our research results showed that the ecological flow should not only consider water quality and the structure and function of the river ecosystem, but also include the protection of biodiversity. We hereby suggest that maintaining genetic and ecological connectivity based on an effective impact assessment in dry seasons.
Conclusion
Our research proved that the construction of small run-of-river dams affected the β-diversity of benthic diatoms and the community assembly mechanism, thus verifying our hypothesis. We concluded that small run-of-river dams changed the relative contribution of nestedness components to the trait-based total β-diversity of benthic diatoms, and the taxonomy-based β-diversity was relatively higher than the trait-based β-diversity. Another important result was the discovery that small run-of-river dams affect the community assembly process of benthic diatoms. In areas impacted by small run-of-river dams, dispersal assembly was a key mechanism for community assembly. Compared to unregulated habitats, the dispersal assembly process between the impacted habitat and the unregulated habitats has been enhanced. Therefore, our research suggests that maintaining river connectivity and approving ecological flows, and maintaining genetic and ecological connectivity based on an effective impact assessment in dry seasons to reduce the impact of such dams, as key to adaptive management and sustainability.
Data Availability Statement
The raw data supporting the conclusions of this article will be made available by the authors, without undue reservation.
Author Contributions
NW and QC conceived the ideas. YW and NW performed the data analyses and led the writing. NW, TT, and SZ performed the sampling collection and processing. All authors contributed to the article and approved the submitted version.
Funding
This study was funded by the National Key R&D Program of China (No. 2017YFC0506406), the Strategic Priority Research Program of the Chinese Academy of Sciences (No. XDA23080101), and the National Natural Science Foundation of China (No. 30330140).
Conflict of Interest
The authors declare that the research was conducted in the absence of any commercial or financial relationships that could be construed as a potential conflict of interest.
Publisher’s Note
All claims expressed in this article are solely those of the authors and do not necessarily represent those of their affiliated organizations, or those of the publisher, the editors and the reviewers. Any product that may be evaluated in this article, or claim that may be made by its manufacturer, is not guaranteed or endorsed by the publisher.
Acknowledgments
We thank Xiaocheng Fu, Fengqing Li, and Wanxiang Jiang for their field supports. Jianjun Wang and Kun Guo helped with data analysis.
Supplementary Material
The Supplementary Material for this article can be found online at: https://www.frontiersin.org/articles/10.3389/fevo.2022.895328/full#supplementary-material
References
Angeler, D. G. (2013). Revealing a conservation challenge through partitioned long-term beta diversity: increasing turnover and decreasing nestedness of boreal lake metacommunities. Divers. Distrib. 19, 772–781. doi: 10.1111/ddi.12029
Anonymous, (1992). The Comprehensive Scientific Expedition to the Qinghai-Xizang Plateau, Academia Sinica. The Algae of the Xizang Plateau (in Chinese). Beijing: Science Press.
Ansar, A., Flyvbjerg, B., Budzier, A., and Lunn, D. (2014). Should we build more large dams? The actual costs of hydropower megaproject development. Energy Policy 69, 43–56. doi: 10.1016/j.enpol.2013.10.069
Araújo, M. B., and Rozenfeld, A. (2014). The geographic scaling of biotic interactions. Ecography 37, 406–415. doi: 10.1111/j.1600-0587.2013.00643.x
Atilgan, B., and Azapagic, A. (2016). Renewable electricity in Turkey: life cycle environmental impacts. Renew. Energy 89, 649–657. doi: 10.1016/j.renene.2015.11.082
Aung, T. S., Fischer, T. B., and Azmi, A. S. (2020). Are large-scale dams environmentally detrimental? Life-cycle environmental consequences of mega-hydropower plants in Myanmar. Int. J. Life Cycle Assess. 25, 1749–1766. doi: 10.1007/s11367-020-01795-9
Baselga, A. (2010). Partitioning the turnover and nestedness components of beta diversity. Glob. Ecol. Biogeogr. 19, 134–143. doi: 10.1111/j.1466-8238.2009.00490.x
Baselga, A., and Leprieur, F. (2015). Comparing methods to separate components of beta diversity. Methods Ecol. Evol. 6, 1069–1079. doi: 10.1111/2041-210x.12388
Baselga, A., and Orme, C. D. L. (2012). betapart: an R package for the study of beta diversity. Methods Ecol. Evol. 3, 808–812. doi: 10.1111/j.2041-210x.2012.00224.x
Baskaya, S., Baskaya, E., and Sari, A. (2011). The principal negative environmental impacts of small hydropower plants in Turkey. Afr. J Agric. Res. 6, 3284–3290.
Berthon, V., Bouchez, A., and Rimet, F. (2011). Using diatom life-forms and ecological guilds to assess organic pollution and trophic level in rivers: a case study of rivers in south-eastern France. Hydrobiologia 673, 259–271. doi: 10.1007/s10750-011-0786-1
Biggs, B. J. F., Stevenson, R. J., and Lowe, R. L. (1998). A habitat matrix conceptual model for stream periphyton. Arch. Fur. Hydrobiol. 143, 21–56. doi: 10.1127/archiv-hydrobiol/143/1998/21
Branco, C. C. Z., Bispo, P. C., Peres, C. K., Tonetto, A. F., Krupek, R. A., Barfield, M., et al. (2020). Partitioning multiple facets of beta diversity in a tropical stream macroalgal metacommunity. J. Biogeogr. 47, 1765–1780. doi: 10.1111/jbi.13879
Buckley, L. B., and Jetz, W. (2008). Linking global turnover of species and environments. Proc. Natl. Acad. Sci. U.S.A. 105, 17836–17841. doi: 10.1073/pnas.0803524105
Castro, D. M. P., Dolédec, S., and Callisto, M. (2018). Land cover disturbance homogenizes aquatic insect functional structure in neotropical savanna streams. Ecol. Indic. 84, 573–582. doi: 10.1016/j.ecolind.2017.09.030
Chase, J. M., Kraft, N. J. B., Smith, K. G., Vellend, M., and Inouye, B. D. (2011). Using null models to disentangle variation in community dissimilarity from variation in α−diversity. Ecosphere 2, 1–11.
Chinese Environmental Protection Bureau (1989). Monitoring and Analyzing Methods for Water and Sewage. Beijing: China Environment Science Press. (in Chinese).
Clappe, S., Dray, S., and Peres-Neto, P. R. (2018). Beyond neutrality: disentangling the effects of species sorting and spurious correlations in community analysis. Ecology 99, 1737–1747. doi: 10.1002/ecy.2376
Corline, N. J., Peek, R. A., Montgomery, J., Katz, J. V. E., and Jeffres, C. A. (2021). Understanding community assembly rules in managed floodplain food webs. Ecosphere 12:e03330.
Couto, T. B. A., and Olden, J. D. (2018). Global proliferation of small hydropower plants – science and policy. Front. Ecol. Environ. 16, 91–100. doi: 10.1002/fee.1746
de Faria, F. A. M., Davis, A., Severnini, E., and Jaramillo, P. (2017). The local socio-economic impacts of large hydropower plant development in a developing country. Energy Econ. 67, 533–544. doi: 10.1016/j.eneco.2017.08.025
Deemer, B. R., Harrison, J. A., Li, S., Beaulieu, J. J., Delsontro, T., Barros, N., et al. (2016). Greenhouse gas emissions from reservoir water surfaces: a new global synthesis. Bioscience 66, 949–964. doi: 10.1093/biosci/biw117
Fu, C. Y., Tao, F., and Deng, N. S. (2006). The research of phosphorus of Xiangxi River nearby the Three Gorges, China. Environ. Geol. 49, 923–928. doi: 10.1007/s00254-005-0124-x
Fukami, T. (2015). “Historical contingency in community assembly: integrating niches, species pools, and priority effects,” in Annual Review of Ecology, Evolution, and Systematics, Vol. 46, ed. D. J. Futuyma (Palo Alto, CA: Annual Reviews), 1–23. doi: 10.1146/annurev-ecolsys-110411-160340
Gibert, C. (2021). DNCImper: Assembly Process Identification based on SIMPER Analysis. R Package Version 0.0.1.0000.
Gibert, C., Shenbrot, G. I., Stanko, M., Khokhlova, I. S., and Krasnov, B. R. (2021). Dispersal-based versus niche-based processes as drivers of flea species composition on small mammalian hosts: inferences from species occurrences at large and small scales. Oecologia 197, 471–484. doi: 10.1007/s00442-021-05027-1
Goldenberg Vilar, A., van Dam, H., van Loon, E. E., Vonk, J. A., van Der Geest, H. G., and Admiraal, W. (2014). Eutrophication decreases distance decay of similarity in diatom communities. Freshw. Biol. 59, 1522–1531. doi: 10.1111/fwb.12363
Green, J. L., and Ostling, A. (2003). Endemics-area relationships: the influence of species dominance and spatial aggregation. Ecology 84, 3090–3097. doi: 10.1890/02-3096
Gutiérrez-Cánovas, C., Millán, A., Velasco, J., Vaughan, I. P., and Ormerod, S. J. (2013). Contrasting effects of natural and anthropogenic stressors on beta diversity in river organisms. Glob. Ecol. Biogeogr. 22, 796–805. doi: 10.1111/geb.12060
Hu, H.-J., Li, R., Wei, Y., Zhu, H., Chen, J., and Shi, Z. (1980). Freshwater Algae in China. Shanghai: Shanghai Science and Technology Press. (in Chinese).
Huang, H., and Yan, Z. (2009). Present situation and future prospect of hydropower in China. Renew. Sustain. Energy Rev. 13, 1652–1656. doi: 10.1016/j.rser.2008.08.013
Isabwe, A., Yang, J. R., Wang, Y., Liu, L., Chen, H., and Yang, J. (2018). Community assembly processes underlying phytoplankton and bacterioplankton across a hydrologic change in a human-impacted river. Sci. Total Environ. 630, 658–667. doi: 10.1016/j.scitotenv.2018.02.210
James, A., Pitchford, J. W., and Plank, M. J. (2012). Disentangling nestedness from models of ecological complexity. Nature 487, 227–230. doi: 10.1038/nature11214
Jia, X.-H., Jiang, W.-X., Li, F.-Q., Tang, T., Duan, S.-G., and Cai, Q.-H. (2009). Impacts of large hydropower station on benthic algal communities. J. Appl. Ecol. 20, 1731–1738.
Kahn, J. R., Freitas, C. E., and Petrere, M. (2014). False shades of green: the case of Brazilian Amazonian hydropower. Energies 7, 6063–6082. doi: 10.3390/en7096063
Lange, K., Meier, P., Trautwein, C., Schmid, M., Robinson, C. T., Weber, C., et al. (2018). Basin-scale effects of small hydropower on biodiversity dynamics. Front. Ecol. Environ. 16, 397–404. doi: 10.1002/fee.1823
Lange, K., Townsend, C. R., and Matthaei, C. D. (2016). A trait- based frame-work for stream algal communities. Ecol. Evol. 6, 23–36. doi: 10.1002/ece3.1822
Legendre, P. (2014). Interpreting the replacement and richness difference components of beta diversity. Glob. Ecol. Biogeogr. 23, 1324–1334.
Legendre, P., Borcard, D., and Peres-Neto, P. R. (2005). Analyzing beta diversity: partitioning the spatial variation of community composition data. Ecol. Monogr. 75, 435–450. doi: 10.1890/05-0549
Lepisto, L., Holopainen, L. L., and Vuoristo, H. (2004). Type-specific and indicator taxa of phytoplankton as a quality criterion for assessing the ecological status of Finnish boreal lakes. Limnologica 34, 236–248. doi: 10.1016/s0075-9511(04)80048-3
Leprieur, F., Tedesco, P. A., Hugueny, B., Beauchard, O., Durr, H. H., Brosse, S., et al. (2011). Partitioning global patterns of freshwater fish beta diversity reveals contrasting signatures of past climate changes. Ecol. Lett. 14, 325–334. doi: 10.1111/j.1461-0248.2011.01589.x
Logez, M., Pont, D., and Ferreira, M. T. (2010). Do Iberian and European fish faunas exhibit convergent functional structure along environmental gradients? J. North Am. Benthol. Soc. 29, 1310–1323. doi: 10.1899/09-125.1
Lopez-Delgado, E. O., Winemiller, K. O., and Villa-Navarro, F. A. (2020). Local environmental factors influence beta-diversity patterns of tropical fish assemblages more than spatial factors. Ecology 101:e02940. doi: 10.1002/ecy.2940
Mallik, A. U., and Richardson, J. S. (2009). Riparian vegetation change in upstream and downstream reaches of three temperate rivers dammed for hydroelectric generation in British Columbia, Canada. Ecol. Eng. 35, 810–819. doi: 10.1016/j.ecoleng.2008.12.005
Mayora, G., Devercelli, M., and Frau, D. (2016). Spatial variability of chromophoric dissolved organic matter in a large floodplain river: control factors and relations with phytoplankton during a low water period. Ecohydrology 9, 487–497. doi: 10.1002/eco.1651
Meng, F., Li, Z., Li, L., Lu, F., Liu, Y., Lu, X., et al. (2020). Phytoplankton alpha diversity indices response the trophic state variation in hydrologically connected aquatic habitats in the Harbin Section of the Songhua River. Sci. Rep. 10:21337. doi: 10.1038/s41598-020-78300-7
Ney-Nifle, M., and Mangel, M. (1999). Species-area curves based on geographic range and occupancy. J. Theor. Biol. 196, 327–342. doi: 10.1006/jtbi.1998.0844
Oksanen, J., Blanchet, F. G., Friendly, M., Kindt, R., Legendre, P., McGlinn, D., et al. (2019). vegan: Community Ecology Package. R Package Version 2.5-6.
Paish, O. (2002). Small hydro power: technology and current status. Renew. Sustain. Energy Rev0 6, 537–556. doi: 10.1016/s1364-0321(02)00006-0
Passy, S. I. (2007). Diatom ecological guilds display distinct and predictable behavior along nutrient and disturbance gradients in running waters. Aquat. Bot. 86, 171–178. doi: 10.1016/j.aquabot.2006.09.018
Podani, J., and Schmera, D. (2011). A new conceptual and methodological framework for exploring and explaining pattern in presence-absence data. Oikos 120, 1625–1638. doi: 10.1111/j.1600-0706.2011.19451.x
Poff, N. L., and Matthews, J. H. (2013). Environmental flows in the Anthropocence: past progress and future prospects. Curr. Opin. Environ. Sustain. 5, 667–675. doi: 10.1016/j.cosust.2013.11.006
Qian, H., and Ricklefs, R. E. (2007). A Latitudinal Gradient in Large-Scale Beta Diversity for Vascular Plants in North America, Vol. 10. John Wiley & Sons, Ltd, 737–744.
R Core Team (2020). R: A Language and Environment for Statistical Computing. Vienna: R Foundation for Statistical Computing.
Rimet, F., and Bouchez, A. (2012). Life-forms, cell-sizes and ecological guilds of diatoms in European rivers. Know. Manag. Aquat. Ecosyst. 406:01. doi: 10.1051/kmae/2012018
Rocha, M. P., Bini, L. M., Gronroos, M., Hjort, J., Lindholm, M., Karjalainen, S.-M., et al. (2019). Correlates of different facets and components of beta diversity in stream organisms. Oecologia 191, 919–929. doi: 10.1007/s00442-019-04535-5
Sarauskiene, D., Adzgauskas, G., Kriauciuniene, J., and Jakimavicius, D. (2021). Analysis of hydrologic regime changes caused by small hydropower plants in lowland rivers. Water 13:1961. doi: 10.3390/w13141961
Sarkar, S. (2006). Ecological diversity and biodiversity as concepts for conservation planning: Comments on Ricotta. Acta Biotheor. 54, 133–140. doi: 10.1007/s10441-006-8259-z
Schmera, D., Arva, D., Boda, P., Bodis, E., Bolgovics, A., Borics, G., et al. (2018). Does isolation influence the relative role of environmental and dispersal-related processes in stream networks? An empirical test of the network position hypothesis using multiple taxa. Freshw. Biol. 63, 74–85. doi: 10.1111/fwb.12973
Si, X., Zhao, Y., Chen, C., Ren, P., Zeng, D., Wu, L., et al. (2017) Beta-diversity partitioning: methods, applications and perspectives. Biodivers. Sci. 25, 464–480. doi: 10.17520/biods.2017024
Smith, T. W., and Lundholm, J. T. (2010). Variation partitioning as a tool to distinguish between niche and neutral processes. Ecography 33, 648–655. doi: 10.1111/j.1600-0587.2009.06105.x
Socolar, J. B., Gilroy, J. J., Kunin, W. E., and Edwards, D. P. (2016). How Should Beta-Diversity Inform Biodiversity Conservation? Trends Ecol. Evol. 31, 67–80. doi: 10.1016/j.tree.2015.11.005
Soininen, J., McDonald, R., and Hillebrand, H. (2007). The distance decay of similarity in ecological communities. Ecography 30, 3–12. doi: 10.1111/j.1461-0248.2008.01202.x
Staniczenko, P. P. A., Kopp, J. C., and Allesina, S. (2013). The ghost of nestedness in ecological networks. Nat. Commun. 4:1391. doi: 10.1038/ncomms2422
Svenning, J.-C., Flojgaard, C., and Baselga, A. (2011). Climate, history and neutrality as drivers of mammal beta diversity in Europe: insights from multiscale deconstruction. J. Anim. Ecol. 80, 393–402. doi: 10.1111/j.1365-2656.2010.01771.x
Tang, X., Li, Q., Wu, M., Tang, W., Jin, F., Haynes, J., et al. (2012). Ecological environment protection in Chinese rural hydropower development practices: a review. Water Air Soil Pollut. 223, 3033–3048. doi: 10.1007/s11270-012-1086-8
Tuomisto, H., and Ruokolainen, K. (2006). Analyzing or explaining beta diversity? understanding the targets of different methods of analysis. Ecology 87, 2697–2708. doi: 10.1890/0012-9658(2006)87[2697:aoebdu]2.0.co;2
Tuomisto, H., and Ruokolainen, K. (2008) Analyzing or explaining beta diversity? Reply Ecol. 89, 3244–3256. doi: 10.1890/08-1247.1
Vaikasas, S. (2010). Mathematical modelling of sediment dynamics and their deposition in lithuanian rivers and their deltas (case studies). J. Environ. Eng. Landsc. Manag. 18, 207–216. doi: 10.3846/jeelm.2010.24
Vilmi, A., Gibert, C., Escarguel, G., Happonen, K., Heino, J., Jamoneau, A., et al. (2021). Dispersal–niche continuum index: a new quantitative metric for assessing the relative importance of dispersal versus niche processes in community assembly. Ecography 44, 370–379. doi: 10.1111/ecog.05356
Wagenhoff, A., Lange, K., Townsend, C. R., and Matthaei, C. D. (2013). Patterns of benthic algae and cyanobacteria along twin-stressor gradients of nutrients and fine sediment: a stream mesocosm experiment. Freshw. Biol. 58, 1849–1863. doi: 10.1111/fwb.12174
Wang, J., Hu, J., Tang, T., Heino, J., Jiang, X., Li, Z., et al. (2020). Seasonal shifts in the assembly dynamics of benthic macroinvertebrate and diatom communities in a subtropical river. Ecol. Evol. 10, 692–704. doi: 10.1002/ece3.5904
Wang, Y., Wu, N., Tang, T., Wang, Y., and Cai, Q. (2022). Small run-of-river hydropower dams and associated water regulation filter benthic diatom traits and affect functional diversity. Sci. Total Environ. 813:152566. doi: 10.1016/j.scitotenv.2021.152566
Williams, P. H. (1996). Mapping variations in the strength and breadth of biogeographic transition zones using species turnover. Proc. R. Soc. Lond. B Biol. Sci. 263, 579–588. doi: 10.1098/rspb.1996.0087
Witteveen, N. H., Freixa, A., and Sabater, S. (2020). Local and regional environmental factors drive the spatial distribution of phototrophic biofilm assemblages in Mediterranean streams. Hydrobiologia 847, 2321–2336. doi: 10.1007/s10750-020-04258-2
Wu, N., Cai, Q., and Fohrer, N. (2012). Development and evaluation of a diatom-based index of biotic integrity (D-IBI) for rivers impacted by run-of-river dams. Ecol. Indic. 18, 108–117. doi: 10.1016/j.ecolind.2011.10.013
Wu, N., Dong, X., Liu, Y., Wang, C., Baattrup-Pedersen, A., and Riis, T. (2017). Using river microalgae as indicators for freshwater biomonitoring: review of published research and future directions. Ecol. Indic. 81, 124–131. doi: 10.1016/j.ecolind.2017.05.066
Wu, N., Tang, T., Fu, X., Jiang, W., Li, F., Zhou, S., et al. (2010). Impacts of cascade run-of-river dams on benthic diatoms in the Xiangxi River, China. Aquat. Sci. 72, 117–125. doi: 10.1007/s00027-009-0121-3
Wu, N., Wang, Y., Wang, Y., Sun, X., Faber, C., and Fohrer, N. (2022). Environment regimes play an important role in structuring trait- and taxonomy-based temporal beta diversity of riverine diatoms. J. Ecol.
Wu, N., Zhou, S., Zhang, M., Peng, W., Guo, K., Qu, X., et al. (2021). Spatial and local environmental factors outweigh geo-climatic gradients in structuring taxonomically and trait-based β-diversity of benthic algae. J. Biogeogr. 48, 1842–1857. doi: 10.1111/jbi.14108
Zhang, Z. S., and Huang, X. F. (1991). Research Methods for Freshwater Plankton. Beijing: Science Press. (in Chinese).
Keywords: β-diversity decomposition, DNCI, nestedness, small hydropower, turnover, Xiangxi River
Citation: Wang Y, Wu N, Tang T, Zhou S and Cai Q (2022) Small Run-of-River Dams Affect Taxonomic and Functional β-Diversity, Community Assembly Process of Benthic Diatoms. Front. Ecol. Evol. 10:895328. doi: 10.3389/fevo.2022.895328
Received: 13 March 2022; Accepted: 11 April 2022;
Published: 29 April 2022.
Edited by:
Tatenda Dalu, University of Mpumalanga, South AfricaReviewed by:
Weiju Zhu, Qiongtai Normal University, ChinaChipo Perseverance Mungenge, Rhodes University, South Africa
Copyright © 2022 Wang, Wu, Tang, Zhou and Cai. This is an open-access article distributed under the terms of the Creative Commons Attribution License (CC BY). The use, distribution or reproduction in other forums is permitted, provided the original author(s) and the copyright owner(s) are credited and that the original publication in this journal is cited, in accordance with accepted academic practice. No use, distribution or reproduction is permitted which does not comply with these terms.
*Correspondence: Qinghua Cai, qhcai@ihb.ac.cn
†These authors have contributed equally to this work and share first authorship