- Plant Conservation and Population Biology, Department of Biology, Katholieke Universiteit Leuven, Leuven, Belgium
While there is mounting evidence that ongoing changes in the climate system are shifting species ranges poleward and to higher altitudes, responses to climate change vary considerably between species. In general, it can be expected that species responses to climate change largely depend on how broad their ecological niches are, but evidence is still scant. In this study, we investigated the effects of predicted future climate change on the availability of suitable habitat for 14 Epipactis (Orchidaceae) species, and tested whether habitat specialists would experience greater changes in the extent of their habitats than habitat generalists. We used Maxent to model the ecological niche of each species in terms of climate, soil, elevation and land-use and projected it onto climate scenarios predicted for 2061–2080. To test the hypothesis that temperate terrestrial orchid species with small ranges or small niche breadths may be at greater risk under climate change than species with wide ranges or large niche breadths, we related niche breadth in both geographic and environmental space to changes in size and location of suitable habitat. The habitat distributions of half of the species shifted northwards in future projections. The area of suitable habitat increased for eight species but decreased for the remaining six species. If expansion at the leading edge of the distribution was not possible, the area of suitable habitat decreased for 12 species. Species with wide niche breadth in geographic space experienced greater northwards expansions and higher habitat suitability scores than species with small niche breadth. Niche breadth in environmental space was not significantly related to change in habitat distribution. Overall, these results indicate that terrestrial orchid species with a wide distribution will be more capable of shifting their distributions under climate change than species with a limited distribution, but only if they are fully able to expand into habitats at the leading edge of their distributions.
Introduction
Climate plays an important role in the distribution of plant and animal species and in light of the global climate crisis, the effects of changing climate on plant species distributions is a prominent topic in ecology (Chen et al., 2011; Tayleur et al., 2015; Lehikoinen and Virkkala, 2016). In order to survive climate change, species must either shift their range limits to environments that are able to support them or adapt to the new conditions in their current environments (Thuiller, 2007; Kelly and Goulden, 2008; Scheffers et al., 2016; Ash et al., 2017). Predicting how a species’ suitable habitat alters due to climate change is necessary when planning its long-term conservation, but can be difficult because of the wide variety of habitat needs and tolerances among species.
Species differ in their responses to climate change based on how broad their ecological niches are (Thuiller et al., 2005). Previous research has already shown that species within a genus can vary considerably in habitat preferences and distributions (Brown et al., 1996; Grossenbacher and Whittall, 2011; Anacker and Strauss, 2014; Duffy and Jacquemyn, 2019). Habitat generalists tend to have wider ranges of conditions where they can survive, grow and reproduce and are therefore assumed to be more adaptable to environmental change (Marvier et al., 2004; Thuiller et al., 2005). Specialist species, on the other hand, tend to have more specific environmental requirements and therefore can only occupy a narrow ecological niche. It is expected that species which have narrow temperature or precipitation tolerances are the most likely to be affected by climate change (Slatyer et al., 2013). However, empirical evidence is still limited (Shay et al., 2021) and for many species we do not know the factors that limit their distributions, whether leading edge expansions are sustainable, or how these species respond to climate change. Gaining a better understanding of the physical factors underlying the distribution of organisms is crucial to predict how species will respond to climate change (Hagsater et al., 1996; Tsiftsis et al., 2008).
Although orchids are generally considered rare and have small population sizes (Tremblay et al., 2005; Otero and Flanagan, 2006; Shefferson et al., 2020), there is often large variation in range size and environmental tolerance between species, both within and among orchid genera (McCormick and Jacquemyn, 2014; Evans and Jacquemyn, 2020). What drives variation in orchid species range size is not well known, but is likely a combination of factors including niche breadth, species age, niche availability and range position (Sheth et al., 2020). Previous research has shown that orchid species vary in their dependence on specific abiotic environmental conditions, with some species being limited primarily by temperature and precipitation (McCormick et al., 2009; Djordjević et al., 2016; Evans et al., 2020) and others being limited more by local growth conditions related to bedrock and soil (Bowles et al., 2005; Tsiftsis et al., 2008; Bunch et al., 2013). Consequently, specialist orchid species are often associated with the habitat types that arise from the specific combinations of these abiotic characteristics, from coastal dunes to temperate forests, and the spatial extent of these habitats therefore can limit the range of the species they support (McCormick and Jacquemyn, 2014; Djordjević and Tsiftsis, 2022). Species traits related to growth and reproduction in a habitat, such as root system and pollination, can affect spatial distribution. For example, wide spatial distributions of orchids in the Czech Republic were associated with a rhizomatous root system (Štípková et al., 2021), and the wide variety of pollinators utilised by the terrestrial orchid Epipactis helleborine is likely an important contributor to its large range and ability to colonise various habitats (Rewicz et al., 2017).
Recently, it has become clear that weather conditions can have a strong impact on orchid population dynamics, suggesting that changing climatic conditions have the potential to affect the geographic distribution of orchids. For example, climatic changes during the last three decades have been shown to have a positive effect on the survival of the terrestrial orchid Himantoglossum hircinum at the northern edge of its population in the United Kingdom (van der Meer et al., 2016) and warmer winter weather conditions have also been shown to be beneficial to German populations of this species (Pfeifer et al., 2006). Williams et al. (2015) demonstrated that the population dynamics, vital rates and reproduction of the lady orchid (Orchis purpurea) at the northern edge of its distribution were affected by seasonal temperature and precipitation and, specifically, that milder winters and wetter springs were beneficial for its population growth. These results suggest that a warmer climate will generally benefit orchids at the northern edges of their distributions. A recent modelling study has indeed shown that predicted changes in climatic conditions increased habitat suitability available to three Orchis species by 2050 at the northern edge of their distribution (Evans et al., 2020). However, given that these species showed very similar distribution areas and often co-occur, such a generalisation may not be appropriate and it remains unclear how differences in range size or environmental niche breadth predict vulnerability under global change (Shay et al., 2021).
In this study, we tested the hypothesis that orchid species with small ranges or small niche breadths may be at greater risk under climate change than species with wide ranges or large niche breadths. We used the orchid genus Epipactis as a study system. Epipactis is a widespread genus occurring throughout the European and Asian continents with 37 species according to the The Euro+Med Plantbase Project (2022) although the results of phylogenetic research in recent years has brought into question the status of many species (Sramkó et al., 2019; Bateman, 2020). Previous research has shown that among fourteen European Epipactis species, range size differed by more than three orders of magnitude between species with the smallest and largest ranges (Evans and Jacquemyn, 2020). The distribution of small-range species was strongly associated with local habitat conditions and landscape structure, while that of large-range species was more associated with climatic conditions (Evans and Jacquemyn, 2020). However, whether the habitat distributions of generalist species are more strongly affected by climate change than small-range, specialist species, is yet unknown. Specifically, we investigated how the habitat of the same fourteen Epipactis species would be affected by changes in temperature and precipitation in Europe predicted for 2061–2080, and assessed whether species with small ranges or narrow ecological niches would suffer greater changes in size and latitudinal position of habitat than species with large ranges.
Materials and Methods
Study Species and Occurrence Data
The genus Epipactis contains a large number of terrestrial orchids which vary greatly in distribution area and habitat type (Sramkó et al., 2019; Evans and Jacquemyn, 2020). Some species (e.g., E. dunensis and E. albensis) have very localised distributions and are restricted to particular habitats such as coastal dunes and beech forests, whereas others (e.g., E. helleborine and E. atrorubens) are widespread and can tolerate a relatively wide range of habitat conditions. There are several ecotypes of E. helleborine that can be found in specific habitats such as coastal dunes and forests (Jacquemyn et al., 2018). Species are autogamous, allogamous, or facultative allogamous (Claessens and Kleynen, 2011; Brys and Jacquemyn, 2016). The numerous seeds produced by Epipactis species are very small, dispersed by wind, and rely on the presence of mycorrhizal fungi in the soil to germinate and establish (Bidartondo and Read, 2008; Smith and Read, 2010; McCormick and Jacquemyn, 2014; Jacquemyn et al., 2018; Xing et al., 2020). Differences in mycorrhizal communities between localities may contribute to reproductive isolation and spatial distribution of Epipactis species and populations (Ogura-Tsujita and Yukawa, 2008; Jacquemyn et al., 2016, 2018; but see Těšitelová et al., 2012).
Records of each species’ occurrence from 2000 to 2020 on the continent of Europe were obtained from the online database GBIF1 (Supplementary Material). We discarded records with missing GIS coordinates, ambiguous species identification or with coordinates with a spatial resolution lower than 100 m. This resulted in between 31 (Epipactis lusitanica) and 45,354 (E. helleborine) occurrences per species. Records for each species were aggregated into 10 km2 grid cells to reduce the effects of spatial clustering resulting from sampling bias, by extracting the centre coordinates of each grid cell in which the species was recorded (Supplementary Table 1). Processing of occurrence data was performed in QGIS v3.4.9 (QGIS Development Team, 2019).
Ecogeographic Variables
Previous studies have shown that land cover, bedrock, precipitation, and temperature are important variables predicting the distributions of some Epipactis species (Tsiftsis et al., 2008; Djordjević et al., 2016; Evans and Jacquemyn, 2020). We therefore used nine raster-format predictor variables with <0.5 correlation with one other. Two of the 19 bioclimatic variables available at the WorldClim v2 online database (Fick and Hijmans, 20172) were used in our model, mean annual temperature and annual precipitation, projected for the near-present climate (1970–2000). These two variables were chosen because they are the most representative of the mean climate of an area, and are therefore appropriate for a continent-wide distribution study such as this. We also obtained the mean annual temperature and annual precipitation rasters predicted for the years 2061–2080 predicted by two Shared Socio-economic Pathways (SSPs), SSP 2-4.5 and SSP 5-8.5 from WorldClim.3 SSP 2-4.5 models the climate in a scenario where greenhouse gas emissions are at their highest (∼44 GT CO2) in 2040 and then decrease to 9.6 GT in 2100, while in SSP 5-8.5, emissions increase steeply until the year 2080 (∼130 GT) before starting to stabilise and decrease (Riahi et al., 2017). Maps of the distribution of temperature and precipitation values in Europe were created by calculating the mean temperature for each cell of a 50 km2 cell grid of Europe and summarising the values in QGIS.
The other seven variables used were the same as those used to model Epipactis species in Evans and Jacquemyn (2020). These include the first two components of two PCAs run on two topsoil datasets (physical and biochemical measures) acquired through the European Soil Data Centre (ESDAC) (Hiederer, 2013; Ballabio et al., 2019), dominant bedrock from the ESDAC database (Van Liedekerke et al., 2006), Corine Land Cover (CLC) from the Copernicus programme of the European Environmental Program (Heymann, 1994) and elevation (Amatulli et al., 2018). All raster processing was performed in RStudio v4.0.2 (R Core Team, 2021).
Ecological Niche Modelling
Defining and quantitatively comparing plant niches can be achieved using ecological niche models (ENMs). Ecological niche modelling has been applied successfully to numerous species to investigate ecological niches and to assess the impacts of climate change and land use on species ranges (Guisan and Thuiller, 2005). We used the programme Maxent v3.4.1. (Phillips et al., 2017) to model the effects of predicted climate change on species’ habitats. Maxent is a popular ENM tool that uses species occurrence data and environmental rasters to calculate a Gibbs value for each pixel of the study area, or the probability that the pixel has suitable habitat conditions for the species (Phillips, 2005) and performs well in comparison to other modelling methods (Elith et al., 2006; Phillips and Dudík, 2008; Valavi et al., 2021). Maxent creates habitat suitability maps over the study area from these data, as well as a table of the contribution of each predictor variable to the distribution of suitable habitat for each species. The choice of Maxent settings was informed by Barbet-Massin et al. (2012) and Merow et al. (2013). Each model was run using a random seed and 100 bootstrap replicates with 75% of the data used to train the model and 25% to test it. The rest of the settings were left as the default (convergence threshold of 0.00001, regularisation threshold of 1 and a maximum of 10,000 background points) and allowed for linear, quadratic, product and hinge features to be chosen automatically, producing a cloglog output. The models were run for the current climatic features and projected onto the SSP climate data to produce separate environmental niche map outputs for the current and future climate conditions.
Data Analysis
For each habitat suitability map, the mean Gibbs value with standard error was calculated for every latitudinal interval of 0.5 decimal degree of the study area (Europe) using the Zonal Statistics tool in QGIS. We ran a Wilcoxon signed rank test on these mean Gibbs values multiplied by their corresponding latitudes to test whether the suitable habitat of each species will shift in latitude in future climate scenarios. Before running the Wilcoxon tests, the set of Gibbs values for each climate scenario was centred by dividing by the mean to eliminate the influence of different mean Gibbs values between climate scenarios and test only for shifts in latitude.
The continuous probabilistic maps produced by Maxent were converted into binary presence maps using the Maximum Test Sensitivity plus Specificity (MTSS) value of each species as a threshold. The pixels with a Gibbs value of greater than the MTSS were extracted and plotted as a new map for each species, with each pixel representing the species being present at that location. The total numbers of pixels occupied by these habitat distribution maps were compared between current and future climate scenarios. The overlapping pixels between the current and future distributions (i.e., pixels for which occurrence equalled one for both maps) of each species were extracted and counted to provide a measurement of the area suitable for a species if it were unable to expand into any newly available areas created by future climate change.
Mean species occurrence per climate scenario and per species, for both the continuous Gibbs values and the number of pixels occupied of the binary maps, were compared between the climate scenarios using Kruskal–Wallis and Dunn tests with a Holm correction or ANOVA and Tukey tests if the data were normally distributed.
We calculated Levins’ B2 values of niche breadth in geographic (B2geo) and environmental space (B2env) for each species using the functions raster.breadth and env.breadth, respectively, in the ENMTools 1.0.5 R package (Warren et al., 2021). B2 ranges from 0 to 1, with values closer to 0 representing narrow (specialised) niche breadth and values closer to 1 representing wide (generalised) niche breadth. Finally, we investigated whether species niche breadth predicts changes in distribution in response to climate change by dividing the range change of a species from current to future scenarios, converting to the proportional change for each species, and comparing these values to each species’ B2 value using ordinary least-squares regression. Ordinary least-squares regression was also used to compare the Levins’ B2 values between geographic and environmental space. The difference in niche breadth between species with positive range changes and negative range changes in response to habitat change was investigated using Kruskal–Wallis tests. The effect of mating system on response to climate change was tested by comparing the mean changes in latitudinal habitat distribution and proportional range size between autogamous, allogamous and facultative autogamous species, using Kruskal–Wallis tests. All analyses were performed in RStudio v4.0.2 (R Core Team, 2021).
Results
The mean temperature in continental Europe will increase from a near-current mean of 9.21 ± 0.10°C (standard error) to 12.16 ± 0.09°C for SSP 2-4.5 and to 13.42 ± 0.09°C for SSP 5-8.5 (Figure 1) predicted for the years 2061–2080. The mean annual precipitation will increase slightly in the future projections, with a current mean of 742.74 ± 5.86–761.95 ± 5.86 mm3 for SSP 2-4.5 and 761.43 ± 5.93 mm3 for SSP 5-8.5.
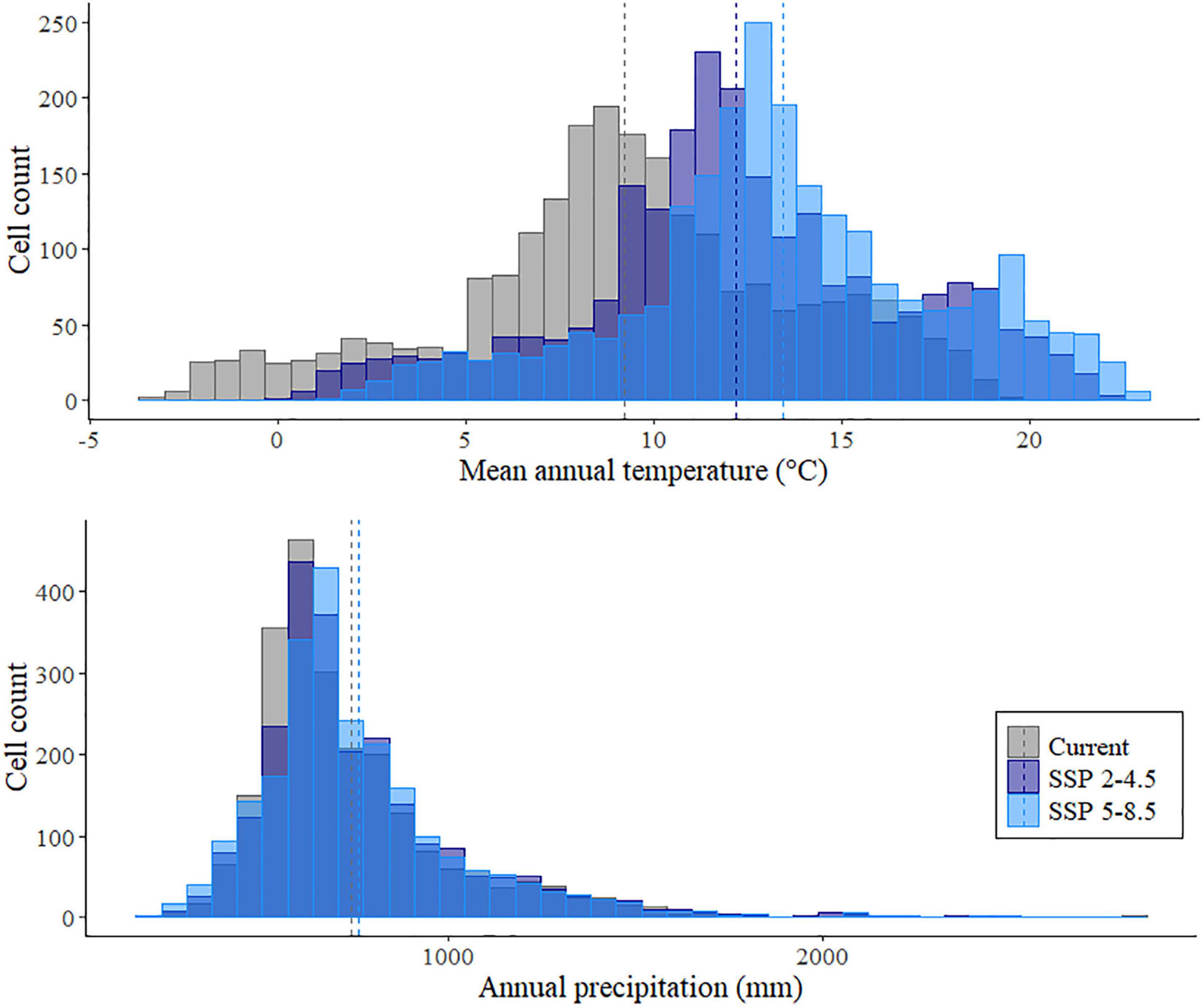
Figure 1. Distribution of mean annual temperature and annual precipitation in Europe predicted for the current climate, and projected to occur in the years 2061–2080 under two Shared Socio-economic Pathways (SSP2-4.5 and SSP5-8.5). Bars represent the number of 50 km grid squares of Europe with a corresponding mean temperature or precipitation value, and dotted lines represent the mean values.
The mean current habitat suitability or probability of occurrence (Gibbs p-value) predicted by the Maxent model ranged from 0.0026 ± 0.0006 for E. lusitanica to 0.29 ± 0.022 for E. helleborine. When the model was projected for the climate of 2061–2080, there was no significant difference in mean habitat suitability between the current climatic conditions and either of the two future climate scenarios (χ2 = 0.18, p-value = 0.91). Although the mean species’ habitat suitability did not change, when species were tested individually, the habitat suitability of E. helleborine, lusitanica, phyllanthes, and tremolsii significantly increased under both SSP scenarios, and E. albensis increased significantly for SSP 5-8.5 (Table 1; see Supplementary Table 2 for mean Gibbs values). Seven species (E. albensis, fageticola, kleinii, leptochila, microphylla, muelleri, and tremolsii) demonstrated significant northwards shifts in their habitat distributions in both future climate scenarios (Table 2 and Supplementary Material for illustration of individual range shifts).
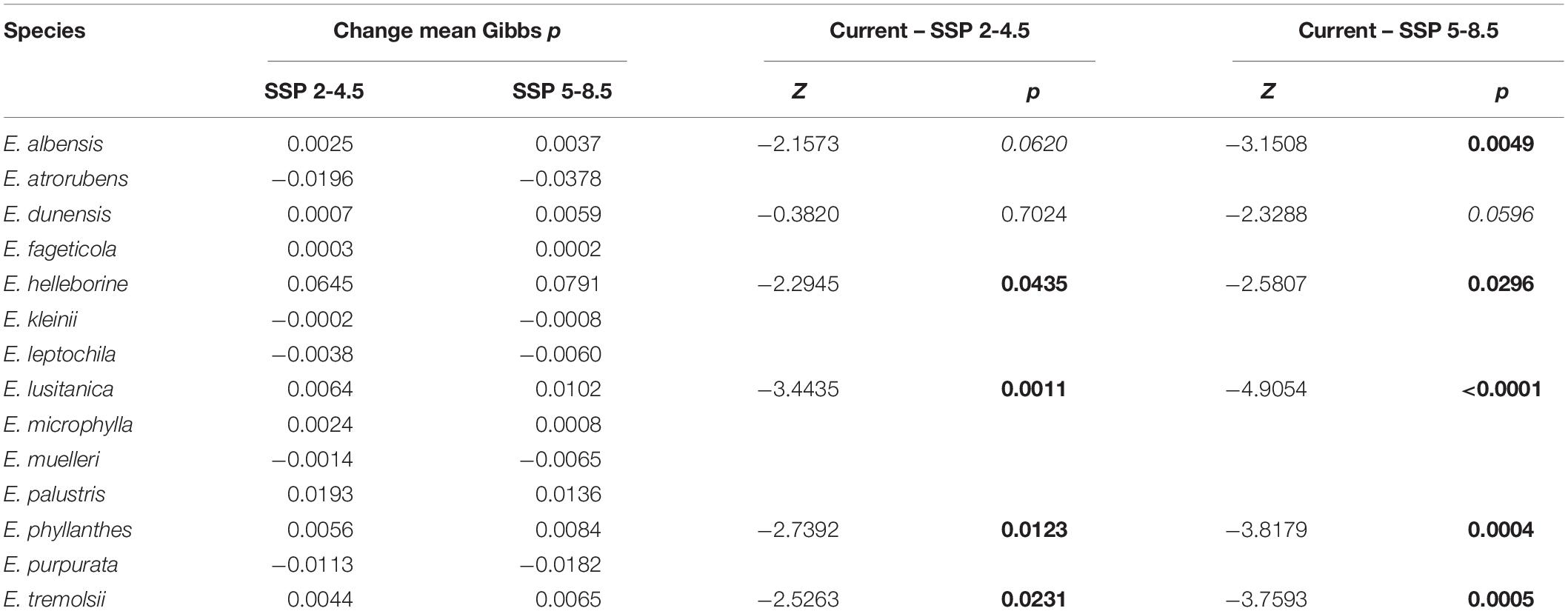
Table 1. Change in mean Gibbs values (habitat suitability) for Epipactis species from current to future (2061–2080) climate scenarios (SSP 2-4.5 and SSP 5-8.5) and results of Dunn tests comparing current and future mean Gibbs p-values (showing only results for significant differences, in bold, and marginally significant differences, in italics).
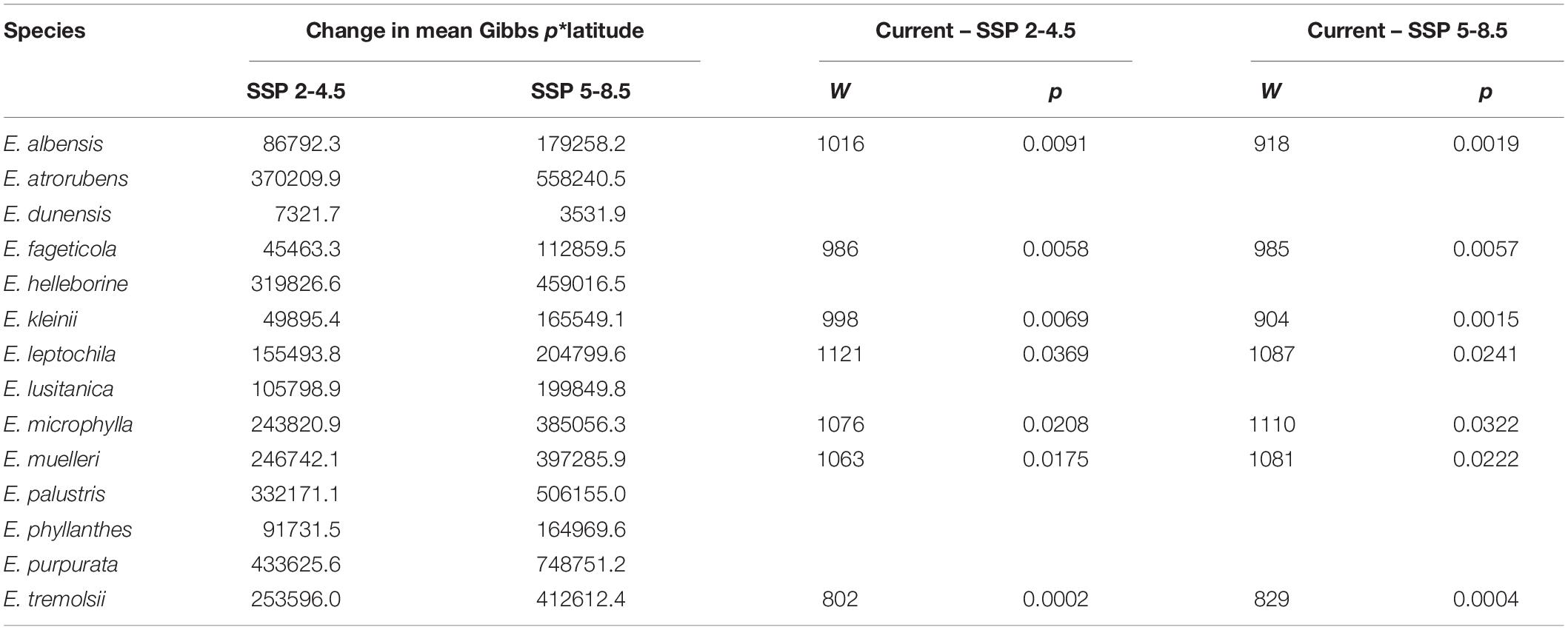
Table 2. Latitudinal shifts in habitat distribution from current to future climate scenarios and results of Wilcoxon tests of differences in habitat distributions (showing only results for significant differences).
The area of suitable habitat available (pixels where the Gibbs p-value was above the species’ MTSS threshold) increased for eight species (E. albensis, dunensis, fageticola helleborine, lusitanica, microphylla, phyllanthes, and tremolsii) in the future scenarios, but decreased for the remaining six species (E. atrorubens, kleinii, leptochila, muelleri, palustris, and purpurata; Table 3). For species that responded positively to the climatic changes, the increase in habitat ranged between 5 and 1000% (E. dunensis and E. lusitanica), while for those that responded negatively, decrease in habitat area ranged between 5% (E. kleinii, muelleri, and palustris) and 88% (E. purpurata).
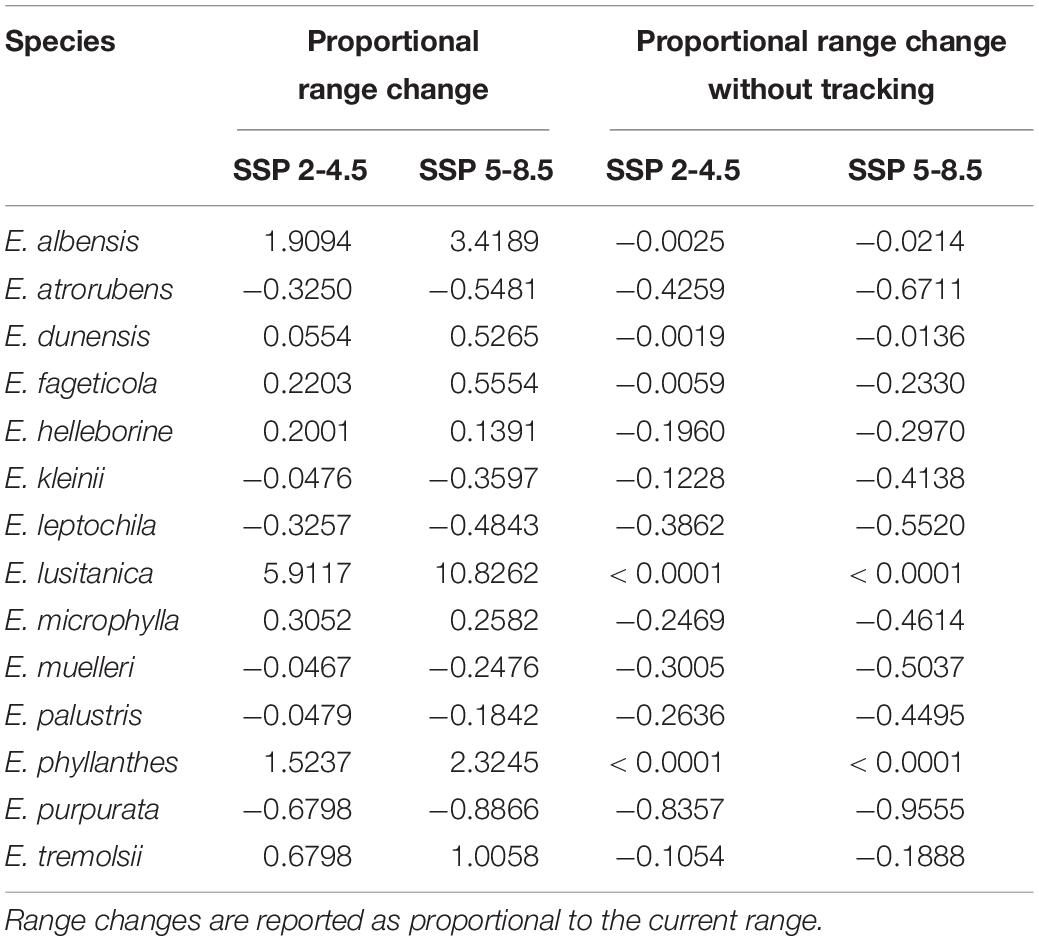
Table 3. Changes in area of suitable habitat above the Maximum Test Sensitivity and Specificity threshold of each species from the current climate conditions to future climate scenarios (SSP 2-4.5 and SSP 5-8.5), as well as changes if species are unable to expand their ranges into the climatic envelope of future scenarios (climate tracking).
Overlap in habitat distribution areas between current and future climate scenarios was fairly high, ranging from 57 to 100% for SSP 2-4.5 and 33 to 100% for SSP 5-8.5, except for E. purpurata which showed notably low overlap (16 and 4% for the two scenarios, respectively; see Supplementary Data for range values). The change in habitat area experienced by species if they would not be able to track the climate in the future decreased by up to 95% (E. purpurata) for all except two species, E. lusitanica and E. phyllanthes, which showed no decrease in distribution area (100% overlap, only expansion).
The niche breadth values of Levins’ B2geo (in geographic space) ranged from 0.39 for E. fageticola to 0.85 for E. palustris, while B2env ranged from 0.16 for E. dunensis to 0.90 for E. lusitanica (see Supplementary Material). B2 values in geographic and environmental spaces were not correlated with one another (p-value = 0.74). The means Gibbs value of habitat suitability was positively correlated with B2geo for both current (R2 = 0.33, F1,12 = 7.52, p-value = 0.012) and future (SSP 2-4.5: R2 = 0.50, F1,12 = 13.81, p-value = 0.0029; SSP 5-8.5: R2 = 0.53, F1,12 = 15.81, p-value = 0.0020) climate projections (Figure 2A). Similarly, B2geo had a positive relationship with range size for current (R2 = 0.32, F1,12 = 7.11, p-value = 0.021) and future (SSP 2-4.5: R2 = 0.53, F1,12 = 15.41, p-value = 0.0020; SSP 5-8.5: R2 = 0.52, F1,12 = 13.19, p-value = 0.0034) climate projections (Figure 2B). Species with higher B2geo values also experienced greater changes in Gibbs values between current and future climate scenarios (Figure 2C; SSP 2-4.5: R2 = 0.68, F1,12 = 17.93, p-value = 0.0039; SSP 5-8.5: R2 = 0.43, F1,12 = 7.09, p-value = 0.032). There was a positive relationship between B2geo and the change in mean Gibbs value between current climate and SSP 2-4.5 multiplied by latitude (Figure 2D; R2 = 0.24, F1,12 = 4.99, p-value = 0.045), indicating that species with higher B2geo values would experience a greater northwards shift in suitable habitat than those with low B2geo values, if they were able to track the suitable climate. This was also marginally significant for SSP 5-8.5 (R2 = 0.18, F1,12 = 3.77, p-value = 0.076). No comparisons involving B2env were significant at α = 0.05, but marginally significant positive relationships were detected between B2env and proportional range change (proportional to the species’ current range) from current to future climate scenarios (SSP 2-4.5: R2 = 0.31, F1,12 = 4.20, p-value = 0.086; SSP 5-8.5: R2 = 0.17, F1,12 = 3.64, p-value = 0.081). Species with higher B2env also showed some evidence for experiencing a greater northwards shift in suitable habitat from current to SSP 5-8.5 climate (R2 = 0.16, F1,12 = 3.77, p-value = 0.076). The mean proportional range change (with and without tracking) and change in latitudinal habitat distribution in response to climate change in either SSP scenario were not significantly different between mating systems (p-value > 0.05).
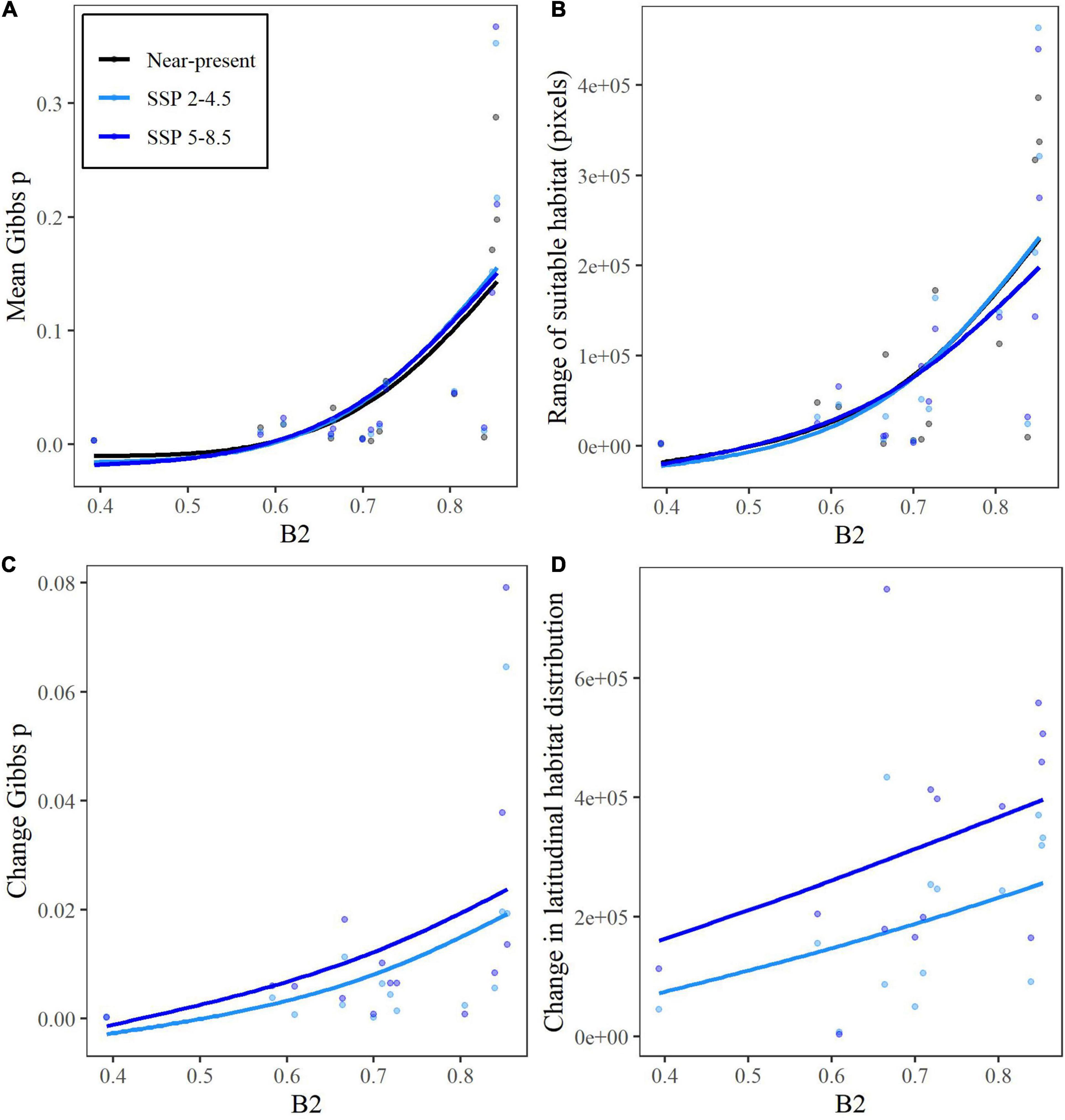
Figure 2. Relationships between Levins’ B2 measure for niche breadth in geographic space and (A) the mean Gibbs p-value (habitat suitability), (B) the spatial range of suitable habitat, (C) change in mean Gibbs p from current to future climate scenarios, and (D) change in latitudinal habitat distribution of 14 Epipactis species. Two climate scenarios were used, SSP 2-4.5 and SSP 5-8.5 to predict the climate for 2061–2080.
Discussion
In this study we investigated how the distribution of suitable habitat of Epipactis species would be affected by predicted climate change and whether species with small ranges or narrow niche breadths are at greater risk from climate change than species with wide ranges or large niche breadths. Our results showed that the habitat available increased on the leading (northern) edge of the distribution for half of the species but decreased for the remaining species, and decreased for all but two species if climate tracking was not possible. Levins’ B2 metric for niche breadth in geographic space was highly correlated with the spatial extent and mean Gibbs value (habitat suitability) of species habitat distributions and species with a higher B2 value were predicted to experience a greater northwards expansion in response to climate change. We did not detect significant effects of Levins’ B2 in environmental space, although there was marginally significant patterns similar to those of B2 in geographic space.
Impact of Climate Change on the Distribution of Epipactis in Europe
Although there was no change in the mean Gibbs value of the 14 species between current and future climate scenarios, the Gibbs values for the majority of the species individually was predicted to increase in 2061–2080. The area of suitable habitat increased into the north for some species and decreased in the south for most species in the future, resulting in a mean northern shift in habitat. When expansion into the north (climate tracking) was restricted, the area of habitat decreased by up to 95% for all except two small-range species.
Despite the expectation that species with narrow environmental tolerances are most threatened by climate change, in the case of Epipactis, the habitats of most of the small-range localised species that we investigated were predicted to increase with future climate change. Some northern hemisphere herbaceous species benefit from increased temperatures at the northern edge of their distribution through increased population growth, which in turn can lead to an increase in geographic range at this edge (Bremer and Jongejans, 2009). This includes orchids such as H. hircinum where climatic changes in the United Kingdom were shown to be partially responsible for the species’ expansion between 1991 and 2001, as well as for projected future scenarios (van der Meer et al., 2016). Similarly, Ongaro et al. (2018) predicted that the habitat range for nine orchid species will increase by 2070 on the island of Sardinia, although the probability of presence in the newly colonised habitats was not predicted to increase. However, Vogt-Schilb et al. (2015) found that the distributions of many orchids in Western Europe have declined in the last two decades due to land-use change, particularly in the northern parts of their distributions. If land-use continues to change in more northern latitudes, this could limit the areas into which Epipactis can move in response to climate change. The results of our study provide further support for the potential for orchid ranges to increase at their leading edges in response to climate change, but go further to demonstrate that this does not necessarily mean an increase in available habitat, particularly if they cannot move into the northern habitats in time.
Testing the Range Size Vulnerability Hypothesis
A widely supported paradigm is that the maximum range limits of a species coincide with its ecological niche limits and that, given the opportunity to disperse, range limits will shift to match the geographical extent of the niche under climate change (Reed et al., 2021; Shay et al., 2021). The pattern of species with wider niche breadths demonstrating greater latitudinal shifts in response to climate change has been documented in a number of terrestrial plant taxa (Thuiller et al., 2005; Alarcón and Cavieres, 2018). This was also demonstrated in orchids by Geppert et al. (2020) where the distributions of generalist orchid species and those inhabiting forests and semi-natural grasslands tended to be less affected than the more specialised and rare species in subalpine, natural grassland and wetland habitats, whose rear and leading edges shifted upward. This corresponds with our finding that Epipactis species with wider niche breadths (generalists) experience greater change in habitat area in response to changing climate than specialists. If we were to assign species to the groups of specialist and generalist based on current spatial ranges and values of B2 in geographic space, E. fageticola, albensis, kleinii, and lusitanica would be considered the most specialist (relative to the other species in this study), followed by E. tremolsii, dunensis, and leptochila as moderately specialist (Supplementary Table 2). E. muelleri, microphylla, and purpurata are moderately generalist, while E. helleborine, palustris, and atrorubens could be considered generalists. However, E. purpurata had a low B2 value but a fairly large spatial distribution and E. phyllanthes a high B2 value and small range, which is in contrast to this pattern. E. purpurata was predicted to experience a significant decrease in suitable habitat under climate change which may indicate that species with relatively large current ranges may still have fairly narrow niches which are nonetheless currently common in the environment, but are under threat from changing climate.
The distributions of all investigated species, even generalists, tended to lag behind climate warming, without being able to fully track the upward shift in suitable climate resulting in a range contraction, in both our study and Geppert et al. (2020). Plant species inhabiting forests may be somewhat buffered from the effects of climate warming (De Frenne et al., 2013; Zellweger et al., 2020) and those in grasslands tend to have high thermal ranges because of the lack of this buffering (Geppert et al., 2020). Similarly, Vogt-Schilb et al. (2015) found higher rates of disappearances in wetland orchid species in Western Europe than those in grassland, and more appearances in forest. There did not seem to be any clear pattern in response to climate change and habitat-use in our species (other than with niche breadth), with woodland species such as E. muelleri decreasing in suitable habitat and E. microphylla increasing. However, our study used a broad-scale specification of land cover, while more may be revealed at a finer resolution that captures microclimate gradients.
An important caveat to consider when carrying out niche breadth studies is that the metric used to describe niche breadth can greatly affect the results. Levin’s B2 is the reciprocal of Simpson’s diversity index (Levins, 1968) and has been a popular metric of niche breadth for more than 50 years. However, it has been noted that the traditional calculation of this metric is in geographic space (see Peterson and Soberón, 2012) for more on geographic and environmental space) and more accurately represents the “flatness” of the geographic distribution of suitable habitat (Warren et al., 2019), which may be a useful measure of spatial habitat-use but is not niche breadth in terms of specificity of resource-use. This is demonstrated clearly in our results, where B2 in geographic space was consistently correlated with measures of the size of the habitat distribution and the mean Gibbs value. B2 in environmental space as proposed by Warren et al. (2019) and developed in Warren et al. (2021) filters the geographic habitat suitability distribution through the set of environmental variables that was used to create the Maxent model, resulting in a B2 value that is closer to the concept of niche breadth as being the specificity in environmental conditions of a species’ habitat. It is important to note, however, that although closer to what we understand to be niche breadth, B2 in environmental space is still dependent on the availability of habitats in geographic space (Petraitis, 1979; Warren et al., 2019). Although the values of B2 in geographic and environmental space were not correlated, B2 in environmental space showed some evidence for having the same relationship with habitat changes as B2 in geographic space. This indicates that B2 in environmental space has the potential to be a useful representation of niche breadth for Epipactis in Europe, but further study is required to conclude this.
Other Factors Contributing to Range Shifts
Although the abiotic characteristics discussed here are important for predicting orchid ranges, biotic interactions and species-specific characteristics are also essential contributors to the realised niche, and including these interactions can improve the accuracy and performance of niche models (Flores-Tolentino et al., 2020; Phillips et al., 2020). Orchids rely on insect pollinators and mycorrhizal fungi to reproduce and germinate (Rasmussen, 2008; McCormick and Jacquemyn, 2014). As with other pollinator-reliant plants, allogamous Epipactis will only persist and track shifting climate if their pollinators are also able to disperse (Benning and Moeller, 2019; Shay et al., 2021), such as has been predicted for a Neotropical orchid bee which is predicted to persist and increase its habitat range under future climate change (Silva et al., 2015). Mating system was not significantly associated with changes in habitat distribution in response to climate change, indicating that in the specific case of these species, autogamous and allogamous species did differ in response to predicted climate change. This is not surprising, considering that mating system was not significantly associated with niche breadth or range size for Epipactis species in previous studies (Evans and Jacquemyn, 2020) and niche breadth in geographic space is directly linked to range size. The presence of soil microbes has also been linked to the ability of plants to expand into newly available habitats (David et al., 2019; Bueno de Mesquita et al., 2020; Benning and Moeller, 2021; Shay et al., 2021). The diversity of mycorrhizal fungi is linked to latitudinal gradients for some orchid species (Duffy et al., 2019), but it is unclear whether the northern shifts in orchid distributions will be supported by the lower diversity of fungi in more northern latitudes. Our understanding and predictions of orchid distribution changes in response to climate change would be greatly improved with the addition of pollinator and fungal symbiont distributional data.
Implications for Conservation
Studies that model the ecological niches of species are useful for conservation planning, particularly for identifying newly accessible areas available to plants (more so than predicting range contractions) and assessing the risk faced by populations as a consequence of their range size (Schwartz, 2012; Shay et al., 2021). This study provides a useful estimate of new areas into which Epipactis can expand, which in conjunction with more information on predicted land change in these areas, could be used in conservation schemes to allow the genus to flourish under climate change. However, it is important to assess the results in light of individual patterns in addition to drawing general conclusions. This is demonstrated in the contrast between mean change in Gibbs value (no change) and the change in Gibbs value for individual species, where a number of species were predicted to increase in the future and, the increase in habitat area for some species and the decrease for other. This disparity between general vs. individual species patterns has also been demonstrated in Geppert et al. (2020), who showed high interspecific variation among orchids grouped by habitat preference. We also show how some species with large areas of habitat such as E. purpurata should not be considered immune to the detrimental effects of future climate change as they may suffer considerable range reductions if they are not able to sufficiently disperse northwards.
Data Availability Statement
The original contributions presented in the study are included in the article/Supplementary Material, further inquiries can be directed to the corresponding author.
Author Contributions
AE and HJ: conceptualisation, methodology, writing, review and editing, and funding acquisition. AE: formal analysis, data curation, and visualisation. HJ: supervision. Both authors have read and agreed to the published version of the manuscript.
Funding
This work was supported by the Flemish Fund for Scientific Research (Grant: G093019N).
Conflict of Interest
The authors declare that the research was conducted in the absence of any commercial or financial relationships that could be construed as a potential conflict of interest.
Publisher’s Note
All claims expressed in this article are solely those of the authors and do not necessarily represent those of their affiliated organizations, or those of the publisher, the editors and the reviewers. Any product that may be evaluated in this article, or claim that may be made by its manufacturer, is not guaranteed or endorsed by the publisher.
Supplementary Material
The Supplementary Material for this article can be found online at: https://www.frontiersin.org/articles/10.3389/fevo.2022.894616/full#supplementary-material
Footnotes
- ^ www.GBIF.org
- ^ https://www.worldclim.org/data/worldclim21.html
- ^ https://www.worldclim.org/data/cmip6/cmip6climate.html
References
Alarcón, D., and Cavieres, L. A. (2018). Relationships between ecological niche and expected shifts in elevation and latitude due to climate change in South American temperate forest plants. J. Biogeography 45, 2272–2287. doi: 10.1111/jbi.13377
Amatulli, G., Domisch, S., Tuanmu, M.-N., Parmentier, B., Ranipeta, A., Malczyk, J., et al. (2018). A suite of global, cross-scale topographic variables for environmental and biodiversity modeling. Sci. Data 5:180040. doi: 10.1038/sdata.2018.40
Anacker, B. L., and Strauss, S. Y. (2014). The geography and ecology of plant speciation: range overlap and niche divergence in sister species. Proc. R. Soc. B: Biol. Sci. 281:20132980. doi: 10.1098/rspb.2013.2980
Ash, J. D., Givnish, T. J., and Waller, D. M. (2017). Tracking lags in historical plant species’ shifts in relation to regional climate change. Global Change Biol. 23, 1305–1315. doi: 10.1111/gcb.13429
Ballabio, C., Lugato, E., Fernández-Ugalde, O., Orgiazzi, A., Jones, A., Borrelli, P., et al. (2019). Mapping LUCAS topsoil chemical properties at European scale using Gaussian process regression. Geoderma 355:113912. doi: 10.1016/j.geoderma.2019.113912
Barbet-Massin, M., Jiguet, F., Albert, C. H., and Thuiller, W. (2012). Selecting pseudo-absences for species distribution models: how, where and how many? Methods Ecol. Evol. 3, 327–338. doi: 10.1111/j.2041-210x.2011.00172.x
Bateman, R. M. (2020). Implications of next-generation sequencing for the systematics and evolution of the terrestrial orchid genus Epipactis, with particular reference to the British Isles. Kew Bull. 75:4.
Benning, J. W., and Moeller, D. A. (2019). Maladaptation beyond a geographic range limit driven by antagonistic and mutualistic biotic interactions across an abiotic gradient. Evolution 73, 2044–2059. doi: 10.1111/evo.13836
Benning, J. W., and Moeller, D. A. (2021). Microbes, mutualism, and range margins: testing the fitness consequences of soil microbial communities across and beyond a native plant’s range. New Phytol. 229, 2886–2900. doi: 10.1111/nph.17102
Bidartondo, M. I., and Read, D. J. (2008). Fungal specificity bottlenecks during orchid germination and development. Mol. Ecol. 17, 3707–3716. doi: 10.1111/j.1365-294X.2008.03848.x
Bowles, M., Zettler, L., Bell, T., and Kelsey, P. (2005). Relationships between soil characteristics, distribution and restoration potential of the federal threatened eastern prairie fringed orchid, Platanthera leucophaea (Nutt.) Lindl. Am. Midland Natural. 154, 273–286. doi: 10.1674/0003-0031(2005)154[0273:rbscda]2.0.co;2
Bremer, P., and Jongejans, E. (2009). Frost and forest stand effects on the population dynamics of Asplenium scolopendrium. Popul Ecol. 52:211. doi: 10.1007/s10144-009-0143-7
Brown, J. H., Stevens, G. C., and Kaufman, D. M. (1996). The geographic range: size, shape, boundaries, and internal structure. Annu. Rev. Ecol. Syst. 27, 597–623. doi: 10.1146/annurev.ecolsys.27.1.597
Brys, R., and Jacquemyn, H. (2016). Severe outbreeding and inbreeding depression maintain mating system differentiation in Epipactis (Orchidaceae). J. Evol. Biol. 29, 352–359. doi: 10.1111/jeb.12787
Bueno de Mesquita, C. P., Sartwell, S. A., Schmidt, S. K., and Suding, K. N. (2020). Growing-season length and soil microbes influence the performance of a generalist bunchgrass beyond its current range. Ecology 101:e03095. doi: 10.1002/ecy.3095
Bunch, W. D., Cowden, C. C., Wurzburger, N., and Shefferson, R. P. (2013). Geography and soil chemistry drive the distribution of fungal associations in lady’s slipper orchid, Cypripedium acaule. Botany 91, 850–856. doi: 10.1139/cjb-2013-0079
Chen, I.-C., Hill, J. K., Ohlemuller, R., Roy, D. B., and Thomas, C. D. (2011). Rapid range shifts of species associated with high levels of climate warming. Science 333, 1024–1026. doi: 10.1126/science.1206432
Claessens, J., and Kleynen, J. (2011). The Flower of the European Orchid: form and Function. Voerendaal: Jean Claessens & Jacques Kleynen.
David, A. S., Quintana-Ascencio, P. F., Menges, E. S., Thapa-Magar, K. B., Afkhami, M. E., and Searcy, C. A. (2019). Soil microbiomes underlie population persistence of an endangered plant species. Am. Nat. 194, 488–494. doi: 10.1086/704684
De Frenne, P., Rodríguez-Sánchez, F., Coomes, D. A., Baeten, L., Verstraeten, G., Vellend, M., et al. (2013). Microclimate moderates plant responses to macroclimate warming. Proc. Natl. Acad. Sci. U S A. 110, 18561–18565. doi: 10.1073/pnas.1311190110
Djordjević, V., and Tsiftsis, S. (2022). “The role of ecological factors in distribution and abundance of terrestrial orchids,” in Orchids Phytochemistry, Biology and Horticulture Reference Series in Phytochemistry, eds J.-M. Mérillon and H. Kodja (Cham: Springer International Publishing), 3–72. doi: 10.1007/978-3-030-38392-3_4
Djordjević, V., Tsiftsis, S., Lakušić, D., Jovanović, S., and Stevanović, V. (2016). Factors affecting the distribution and abundance of orchids in grasslands and herbaceous wetlands. Systematics Biodiversity 14, 355–370. doi: 10.1080/14772000.2016.1151468
Duffy, K. J., and Jacquemyn, H. (2019). Climate change increases ecogeographical isolation between closely related plants. J. Ecol. 107, 167–177. doi: 10.1111/1365-2745.13032
Duffy, K. J., Waud, M., Schatz, B., Petanidou, T., and Jacquemyn, H. (2019). Latitudinal variation in mycorrhizal diversity associated with a European orchid. J. Biogeography 46, 968–980. doi: 10.1111/jbi.13548
Elith, J., Graham, C. H., Anderson, R. P., Dudík, M., Ferrier, S., Guisan, A., et al. (2006). Novel methods improve prediction of species’ distributions from occurrence data. Ecography 29, 129–151.
Evans, A., and Jacquemyn, H. (2020). Impact of mating system on range size and niche breadth in Epipactis (Orchidaceae). Ann. Bot. 126, 1203–1214. doi: 10.1093/aob/mcaa142
Evans, A., Janssens, S., and Jacquemyn, H. (2020). Impact of climate change on the distribution of four closely related orchis (Orchidaceae) species. Diversity 12:312. doi: 10.3390/d12080312
Fick, S. E., and Hijmans, R. J. (2017). WorldClim 2: new 1-km spatial resolution climate surfaces for global land areas. Int. J. Climatol. 37, 4302–4315. doi: 10.1002/joc.5086
Flores-Tolentino, M., García-Valdés, R., Saénz-Romero, C., Ávila-Díaz, I., Paz, H., and Lopez-Toledo, L. (2020). Distribution and conservation of species is misestimated if biotic interactions are ignored: the case of the orchid Laelia speciosa. Sci. Rep. 10:9542. doi: 10.1038/s41598-020-63638-9
Geppert, C., Perazza, G., Wilson, R. J., Bertolli, A., Prosser, F., Melchiori, G., et al. (2020). Consistent population declines but idiosyncratic range shifts in Alpine orchids under global change. Nat. Commun. 11:5835. doi: 10.1038/s41467-020-19680-2
Grossenbacher, D. L., and Whittall, J. B. (2011). Increased floral divergence in sympatric monkeyflowers. Evolution 65, 2712–2718. doi: 10.1111/j.1558-5646.2011.01306.x
Guisan, A., and Thuiller, W. (2005). Predicting species distribution: offering more than simple habitat models. Ecol. Lett. 8, 993–1009. doi: 10.1111/j.1461-0248.2005.00792.x
Hagsater, E., Dumont, V., and Pridgeon, A. M. (1996). Orchids: Status Survey and Conservation Action Plan. Gland: IUCN.
Heymann, Y. (1994). CORINE Land Cover: Technical Guide. Luxembourg.: Office for Official Publ. of the Europ. Communities.
Hiederer, R. (2013). Mapping Soil Properties for Europe—Spatial Representation of Soil Database Attributes. Luxembourg: Scientific and Technical Research series. Publications Office of the European Union, EUR26082EN.
Jacquemyn, H., Kort, H. D., Broeck, A. V., and Brys, R. (2018). Immigrant and extrinsic hybrid seed inviability contribute to reproductive isolation between forest and dune ecotypes of Epipactis helleborine (Orchidaceae). Oikos 127, 73–84. doi: 10.1111/oik.04329
Jacquemyn, H., Waud, M., Lievens, B., and Brys, R. (2016). Differences in mycorrhizal communities between Epipactis palustris, E. helleborine and its presumed sister species E. neerlandica. Annals of Botany 118, 105–114. doi: 10.1093/aob/mcw015
Kelly, A. E., and Goulden, M. L. (2008). Rapid shifts in plant distribution with recent climate change. Proc. Natl. Acad. Sci. U S A. 105, 11823–11826. doi: 10.1073/pnas.0802891105
Lehikoinen, A., and Virkkala, R. (2016). North by north-west: climate change and directions of density shifts in birds. Global Change Biol. 22, 1121–1129. doi: 10.1111/gcb.13150
Levins, R. (1968). Evolution in Changing Environments: Some Theoretical Explorations. Princeton, NJ: Princeton University Press.
Marvier, M., Kareiva, P., and Neubert, M. G. (2004). Habitat destruction, fragmentation, and disturbance promote invasion by habitat generalists in a multispecies metapopulation. Risk Anal. 24, 869–878. doi: 10.1111/j.0272-4332.2004.00485.x
McCormick, M. K., and Jacquemyn, H. (2014). What constrains the distribution of orchid populations? New Phytol. 202, 392–400. doi: 10.1111/nph.12639
McCormick, M. K., Whigham, D. F., O’Neill, J. P., Becker, J. J., Werner, S., Rasmussen, H. N., et al. (2009). Abundance and distribution of Corallorhiza odontorhiza reflect variations in climate and ectomycorrhizae. Ecol. Monographs 79, 619–635. doi: 10.1890/08-0729.1
Merow, C., Smith, M. J., and Silander, J. A. Jr. (2013). A practical guide to MaxEnt for modeling species’ distributions: what it does, and why inputs and settings matter. Ecography 36, 1058–1069. doi: 10.1111/j.1600-0587.2013.07872.x
Ogura-Tsujita, Y., and Yukawa, T. (2008). Epipactis helleborine shows strong mycorrhizal preference towards ectomycorrhizal fungi with contrasting geographic distributions in Japan. Mycorrhiza 18, 331–338. doi: 10.1007/s00572-008-0187-0
Ongaro, S., Martellos, S., Bacaro, G., Agostini, A. D., Cogoni, A., and Cortis, P. (2018). Distributional pattern of Sardinian orchids under a climate change scenario. Community Ecol. 19, 223–232. doi: 10.1556/168.2018.19.3.3
Otero, J. T., and Flanagan, N. S. (2006). Orchid diversity – beyond deception. Trends Ecol. Evol. 21, 64–65. doi: 10.1016/j.tree.2005.11.016
Peterson, A. T., and Soberón, J. (2012). Species distribution modeling and ecological niche modeling: getting the concepts right. NatCon 10, 102–107. doi: 10.2166/wst.2007.494
Petraitis, P. S. (1979). Likelihood measures of niche breadth and overlap. Ecology 60, 703–710. doi: 10.2307/1936607
Pfeifer, M., Wiegand, K., Heinrich, W., and Jetschke, G. (2006). Long-term demographic fluctuations in an orchid species driven by weather: implications for conservation planning. J. Appl. Ecol. 43, 313–324. doi: 10.1111/j.1365-2664.2006.01148.x
Phillips, R. D., Peakall, R., Niet, T., and van der Johnson, S. D. (2020). Niche perspectives on plant–pollinator interactions. Trends Plant Sci. 25, 779–793. doi: 10.1016/j.tplants.2020.03.009
Phillips, S. J., and Dudík, M. (2008). Modeling of species distributions with Maxent: new extensions and a comprehensive evaluation. Ecography 31, 161–175. doi: 10.1111/j.0906-7590.2008.5203.x
Phillips, S. J., Anderson, R. P., Dudík, M., Schapire, R. E., and Blair, M. E. (2017). Opening the black box: an open-source release of Maxent. Ecography 40, 887–893. doi: 10.1111/ecog.03049
Rasmussen, H. (2008). Terrestrial Orchids: from Seed to Mycotrophic Plant. Terrestrial Orchids: from Seed to Mycotrophic Plant. Cambridge: Cambridge University Press.
Reed, P. B., Peterson, M. L., Pfeifer-Meister, L. E., Morris, W. F., Doak, D. F., Roy, B. A., et al. (2021). Climate manipulations differentially affect plant population dynamics within versus beyond northern range limits. J. Ecol. 109, 664–675. doi: 10.1111/1365-2745.13494
Rewicz, A., Jaskuła, R., Rewicz, T., and Tończyk, G. (2017). Pollinator diversity and reproductive success of Epipactis helleborine (L.) Crantz (Orchidaceae) in anthropogenic and natural habitats. PeerJ 5:e3159. doi: 10.7717/peerj.3159
Riahi, K., van Vuuren, D. P., Kriegler, E., Edmonds, J., O’Neill, B. C., Fujimori, S., et al. (2017). The shared socioeconomic pathways and their energy, land use, and greenhouse gas emissions implications: an overview. Global Environ. Change 42, 153–168.
Scheffers, B. R., De Meester, L., Bridge, T. C., Hoffmann, A. A., Pandolfi, J. M., Corlett, R. T., et al. (2016). The broad footprint of climate change from genes to biomes to people. Science 354:aaf7671. doi: 10.1126/science.aaf7671
Schwartz, M. W. (2012). Using niche models with climate projections to inform conservation management decisions. Biol. Conserv. 155, 149–156. doi: 10.1016/j.biocon.2012.06.011
Shay, J. E., Pennington, L. K., Montiel-Molina, J. A. M., Toews, D., Hendrickson, B., and Sexton, J. (2021). Rules of plant species ranges: applications for conservation strategies. Front. Ecol. Evol. 9:700962. doi: 10.3389/fevo.2021.700962
Shefferson, R. P., Jacquemyn, H., Kull, T., and Hutchings, M. J. (2020). The demography of terrestrial orchids: life history, population dynamics and conservation. Botan. J. Linnean Soc. 192, 315–332. doi: 10.1093/botlinnean/boz084
Sheth, S. N., Morueta-Holme, N., and Angert, A. L. (2020). Determinants of geographic range size in plants. New Phytol. 226, 650–665. doi: 10.1111/nph.16406
Silva, D. P., Macêdo, A. C. B. A., Ascher, J. S., and De Marco, P. (2015). Range increase of a neotropical orchid bee under future scenarios of climate change. J. Insect. Conserv. 19, 901–910. doi: 10.1007/s10841-015-9807-0
Slatyer, R. A., Hirst, M., and Sexton, J. P. (2013). Niche breadth predicts geographical range size: a general ecological pattern. Ecol. Lett. 16, 1104–1114. doi: 10.1111/ele.12140
Sramkó, G., Paun, O., Brandrud, M. K., Laczkó, L., Molnár, A., and Bateman, R. M. (2019). Iterative allogamy–autogamy transitions drive actual and incipient speciation during the ongoing evolutionary radiation within the orchid genus Epipactis (Orchidaceae). Ann. Bot. 124, 481–497. doi: 10.1093/aob/mcz103
Štípková, Z., Tsiftsis, S., and Kindlmann, P. (2021). Distribution of orchids with different rooting systems in the czech republic. Plants 10:632. doi: 10.3390/plants10040632
Tayleur, C., Caplat, P., Massimino, D., Johnston, A., Jonzén, N., Smith, H. G., et al. (2015). Swedish birds are tracking temperature but not rainfall: evidence from a decade of abundance changes. Global Ecol. Biogeography 24, 859–872. doi: 10.1111/geb.12308
Těšitelová, T., Těšitel, J., Jersáková, J., Říhová, G., and Selosse, M. (2012). Symbiotic germination capability of four Epipactis species (Orchidaceae) is broader than expected from adult ecology. Am. J. Botany 99, 1020–1032. doi: 10.3732/ajb.1100503
The Euro+Med Plantbase Project (2022). The Euro+Med PlantBase - the Information Resource for Euro-Mediterranean Plant Diversity. Available online at: http://ww2.bgbm.org/EuroPlusMed/PTaxonDetail.asp?NameCache=Epipactis&PTRefFk=8000000 (accessed March 22, 2022).
Thuiller, W., Lavorel, S., and Araujo, M. B. (2005). Niche properties and geographical extent as predictors of species sensitivity to climate change. Global Ecol. Biogeography 14, 347–357. doi: 10.1111/j.1466-822x.2005.00162.x
Tremblay, R. L., Ackerman, J. D., Zimmerman, J. K., and Calvo, R. N. (2005). Variation in sexual reproduction in orchids and its evolutionary consequences: a spasmodic journey to diversification. Biol. J. Linnean Soc. 84, 1–54. doi: 10.1111/j.1095-8312.2004.00400.x
Tsiftsis, S., Tsiripidis, I., Karagiannakidou, V., and Alifragis, D. (2008). Niche analysis and conservation of the orchids of east Macedonia (NE Greece). Acta Oecol. 33, 27–35. doi: 10.1016/j.actao.2007.08.001
Valavi, R., Guillera-Arroita, G., Lahoz-Monfort, J. J., and Elith, J. (2021). Predictive performance of presence-only species distribution models: a benchmark study with reproducible code. Ecol. Monogr. 92:e01486.
van der Meer, S., Jacquemyn, H., Carey, P. D., and Jongejans, E. (2016). Recent range expansion of a terrestrial orchid corresponds with climate-driven variation in its population dynamics. Oecologia 181, 435–448. doi: 10.1007/s00442-016-3592-7
Van Liedekerke, M., Jones, A., and Panagos, P. (2006). ESDBv2 Raster Library—A Set of Rasters Derived from the European Soil Database Distribution v2.0. European Commission and the European Soil Bureau Network, CDROM, EUR 19945.
Vogt-Schilb, H., Munoz, F., Richard, F., and Schatz, B. (2015). Recent declines and range changes of orchids in Western Europe (France, Belgium and Luxembourg). Biol. Conservation 190, 133–141. doi: 10.1016/j.biocon.2015.05.002
Warren, D. L., Beaumont, L. J., Dinnage, R., and Baumgartner, J. B. (2019). New methods for measuring ENM breadth and overlap in environmental space. Ecography 42, 444–446. doi: 10.1111/ecog.03900
Warren, D. L., Matzke, N. J., Cardillo, M., Baumgartner, J. B., Beaumont, L. J., Turelli, M., et al. (2021). ENMTools 1.0: an R package for comparative ecological biogeography. Ecography 44, 504–511. doi: 10.1111/ecog.05485
Williams, J. L., Jacquemyn, H., Ochocki, B. M., Brys, R., and Miller, T. E. (2015). Life history evolution under climate change and its influence on the population dynamics of a long-lived plant. J. Ecol. 103, 798–808. doi: 10.1111/1365-2745.12369
Xing, X., Gao, Y., Zhao, Z., Waud, M., Duffy, K. J., Selosse, M., et al. (2020). Similarity in mycorrhizal communities associating with two widespread terrestrial orchids decays with distance. J. Biogeography 47, 421–433. doi: 10.1111/jbi.13728
Keywords: climate change, ecological niche, ENMTools, Epipactis, Maxent, range size
Citation: Evans A and Jacquemyn H (2022) Range Size and Niche Breadth as Predictors of Climate-Induced Habitat Change in Epipactis (Orchidaceae). Front. Ecol. Evol. 10:894616. doi: 10.3389/fevo.2022.894616
Received: 11 March 2022; Accepted: 25 March 2022;
Published: 15 April 2022.
Edited by:
Pavel Kindlmann, Charles University, CzechiaReviewed by:
Vladan Djordjević, University of Belgrade, SerbiaPrzemyslaw Baranow, University of Gdańsk, Poland
Copyright © 2022 Evans and Jacquemyn. This is an open-access article distributed under the terms of the Creative Commons Attribution License (CC BY). The use, distribution or reproduction in other forums is permitted, provided the original author(s) and the copyright owner(s) are credited and that the original publication in this journal is cited, in accordance with accepted academic practice. No use, distribution or reproduction is permitted which does not comply with these terms.
*Correspondence: Alexandra Evans, YWxleGFuZHJhLmV2YW5zQGt1bGV1dmVuLmJl