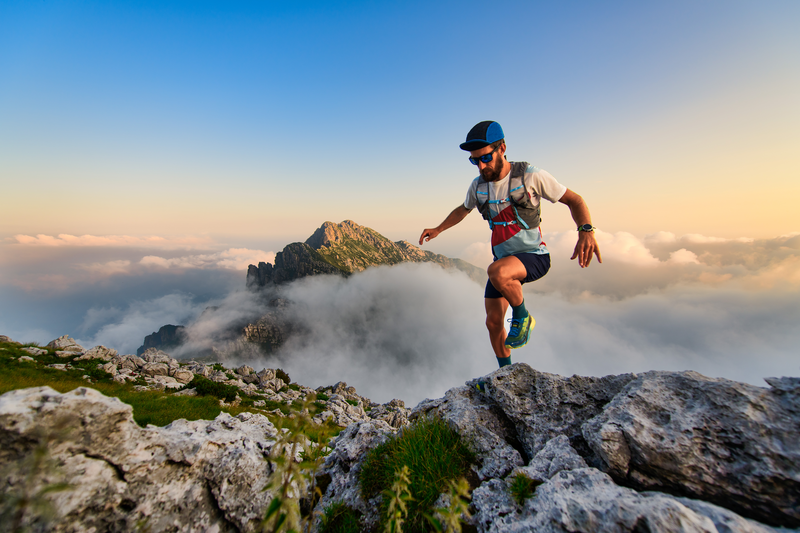
95% of researchers rate our articles as excellent or good
Learn more about the work of our research integrity team to safeguard the quality of each article we publish.
Find out more
ORIGINAL RESEARCH article
Front. Ecol. Evol. , 30 June 2022
Sec. Behavioral and Evolutionary Ecology
Volume 10 - 2022 | https://doi.org/10.3389/fevo.2022.894344
This article is part of the Research Topic The Evolutionary Roots of Reproductive Ageing and Reproductive Health Across the Tree of Life View all 17 articles
The relationship between age and reproductive performance is highly variable across species. Humans and some cetaceans exhibit an extreme form of reproductive senescence in that female reproduction ceases years or even decades before average life expectancy is reached. However, neither the existence of reproductive senescence in some taxa nor its absence in others is fully understood. Comparative data from other long-lived mammals may contribute to a more comprehensive understanding of the evolution of menopause, but data from wild primates, in particular, are scarce. We therefore investigated age-related female reproductive performance in two wild sympatric populations of Malagasy primates: Verreaux’s sifakas (Propithecus verreauxi) and redfronted lemurs (Eulemur rufifrons), which have a maximal longevity of more than 20 years. Based on 25 years of long-term demographic data, we extracted information on reproductive output of 38 female Verreaux’s sifakas and 42 female redfronted lemurs. We modeled variation in female reproductive performance and interbirth intervals as a function of age, the number of adult females within a group to account for female competition, and rainfall as a proxy for annual variation in food availability. We also compared our results for these two species with data on captive populations of the same two genera that are buffered from fluctuations in environmental variables. Our analyses disclosed statistical evidence for reproductive senescence in three out of four populations (captive Coquerel’s sifakas, wild redfronted lemurs, and captive red lemurs) but not for wild Verreaux’s sifakas. Compared to wild populations, reproductive senescence was therefore not less pronounced in captive animals, even though the latter are buffered from environmental adversities. In wild redfronted lemurs, mothers were more likely to give birth in years with more rainfall, but neither the number of co-resident females, nor annual rainfall did predict variation in the probability of giving birth in wild Verreaux’s sifakas. Thus, our study contributes valuable comparative information on reproductive senescence in a basal group of primates, and offers insights into the modulating effects of environmental, social and phylogenetic factors on patterns and dynamics of age-specific female reproduction.
The optimal allocation of reproduction and fertility across the lifespan constitutes a key life-history problem (Stearns, 1976; Roff, 1992; Healy et al., 2019). Theoretical frameworks that predict a trade-off between life-history traits therefore include reproductive senescence, i.e., a decline in reproductive performance, with age (Lemaître et al., 2015, Lemaître and Gaillard, 2017). While some authors have distinguished between aging (the process) and senescence (the outcome), we follow others (e.g., Monaghan et al., 2008; Lemaître et al., 2015) in not making this distinction and using only senescence below. In humans and a few other mammalian species, reproductive senescence terminates in menopause, i.e., the complete cessation of fertility before the average lifespan is reached (Walker and Herndon, 2008; Johnstone and Cant, 2010, 2019; Lieberman et al., 2021). Despite long-held skepticism (Medawar, 1952), there is now compelling evidence for the widespread existence of senescence in a variety of animal species (Monaghan et al., 2008; Nussey et al., 2013). Comparative studies based on long-term data from various wild vertebrate populations have confirmed the presence of early late life trade-offs, suggesting that resource limitation leads individuals to trade somatic maintenance later in life for high allocation to reproduction early in life (Lemaître et al., 2015), and have begun to identify environmental factors that modulate their timing and intensity (Gaillard and Lemaître, 2020). Studying intra- and interspecific variation in longevity and fertility, their complex relationship, as well as the factors modulating them, is therefore crucial for understanding variation in fitness, but our understanding of these processes remains limited, partly because data from very long-lived taxa remain scarce (Gaillard and Lemaître, 2020). Here, we present results of a comparative study exploring these patterns and sources of age-specific female reproduction in relatively long-lived basal primates to contribute to a redressal of this data gap.
As in the vast majority of mammalian species with relatively long lifespan (Lemaître et al., 2020), such as African elephants (Hayward et al., 2014), reproductive rates of female primates are expected to decline with age. Gestation and lactation are energetically demanding, and older female mammals should balance the successful rearing of current offspring against the probability to survive and to produce future offspring (Lemaître and Gaillard, 2017). Indeed, in wild, and thus unprovisioned, chimpanzees and yellow baboons, an age-related decline in female reproductive output was found, but only at relatively old maternal ages and at the same rate as the probability to survive declined (Thompson et al., 2007; Altmann et al., 2010). In some baboon populations, female reproductive activity even ceased before individuals reached their average lifespan (Packer et al., 1998). Further comparisons among six anthropoid (baboons, blue monkeys, capuchins, chimpanzees, gorillas, muriquis) and one lemur species (Verreaux’s sifakas) also suggested that reproductive senescence was present – at least in baboons and sifakas statistically significant effects could be demonstrated – but most individuals died before fertility cessation (Alberts et al., 2013). Another comparative study of 13 primate species showed that age-specific fertility declined with increasing age, also in captivity (Caro et al., 1995). Furthermore, in wild but provisioned rhesus macaques, rates of reproductive senescence were disproportionately higher than those of somatic decline (Lee et al., 2021). Finally, female muriquis, blue monkeys, baboons, gorillas and chimpanzees showed longer interbirth intervals (IBIs) in the oldest or youngest age classes, or both, and the oldest females also showed relatively fewer completed IBIs (Campos et al., 2022). Thus, under various conditions of food availability, reproductive senescence has been reported for various primate species. However, the majority of field studies used estimated or statistically modeled age classes and could not draw on known-aged individuals; one of the practical challenges of long-term field studies (Kappeler et al., 2012, 2017).
Whenever longitudinal data on reproductive rates are not available, cross-sectional data on IBI may provide relevant information on reproductive senescence because the probability of prolonged IBIs is predicted to increase with age. The current evidence for this effect is mixed, however. In Barbary macaques, for example, younger mothers wean their offspring indeed significantly earlier than older ones (Paul et al., 1993), indicating that the successful rearing of offspring is age-related, at least in some species (Atsalis and Margulis, 2008). In particular, IBIs were the longest for very young and very old females in this population (Paul et al., 1993). In chimpanzees, IBIs also increased significantly with maternal age, but the effect size was rather small and this effect was probably more due to individual maternal health, as IBI length in this species is closely related to somatic senescence (Thompson et al., 2007). In the comparative study of 13 captive primate species, the majority of correlations between IBIs and female age were negative, but only significant in humans, orangutans and chimpanzees (Caro et al., 1995). Female baboons seemed to experience shorter IBIs with advancing age in this study, but again this correlation was not significant. In wild capuchin, howler and spider monkeys, IBIs were not affected by age, but also not by parity, rank or body weight (Fedigan and Rose, 1995). Among several populations of captive common marmosets, Smucny et al. (2004) found a significant non-linear correlation between maternal age and IBI length. Thus, female primates appear to account for the increasing costs of reproduction with increasing age by adjusting IBIs in some cases.
If the detrimental age-effects cannot be fully compensated by reduced reproductive rates, infant survival may also be compromised. Even if females continue to reproduce at regular intervals throughout their lifetime, advanced maternal age may result in increased rates of miscarriages or negatively affect offspring survival because of reduced birth weights, milk production or other complications (Atsalis and Margulis, 2008; Lemaître and Gaillard, 2017; Comizzoli and Ottinger, 2021). In fact, infant survival in female olive baboons rapidly declined with age, and mothers beyond 21 years of age were more likely to experience a miscarriage (Packer et al., 1998). Yet, although the maternal age effect on offspring survival in semi-free ranging Barbary macaques was statistically not significant, infants of older mothers were more likely to survive than those of younger females (Paul et al., 1993). Thus, the efforts by senescence mothers to reproduce successfully may also be compromised by reduced infant survival.
The social system of a species may also have evolutionary consequences for age-dependent female reproductive strategies. Humans and beluga whales, killer whales, narwhales and short-finned pilot whales have evolved an extreme form of reproductive senescence (i.e., menopause), where the post-reproductive phase can last at least as long as the reproductive phase (Ward et al., 2009; Ellis et al., 2018; Johnstone and Cant, 2019). In these species, menopause appears to be the outcome of kin selection in social systems characterized by appreciable or effective female dispersal by reducing the costs of intergenerational reproductive conflict (Johnstone and Cant, 2019). Reproductive effort can also be compromised by competition with other females independent of age, however (Beehner and Lu, 2013). For example, in Hanuman langurs conception probability was lower and IBIs were longer in groups containing more females (Sommer and Rajpurohit, 1989; Sommer et al., 1992). Thus, the evolution of key life-history traits appears to be also shaped by features of the social system, including patterns of female kinship, mate quality and reproductive competition.
Other factors can modulate reproductive performance on more immediate levels and time scales. In the wild, animals are exposed to natural fluctuations in food availability, rainfall, temperature and parasites, all of which can have instantaneous consequences for the current or next reproductive cycle, and thus, for reproductive rates. These relationships can be investigated by either cross-sectional comparisons across multiple populations or groups, longitudinal contrasts across multiple seasons or years for the same individuals, or by comparing wild and captive populations of the same species or genus. In the present study, we will adopt the latter two approaches, focusing on two lemur genera in the wild and in captivity.
Long-term studies of wild populations are often compromised by practical challenges related to the longevity of the study subjects, which may exceed the academic lifespans of the researchers, and other practical difficulties (see above). In contrast, unmanaged captive populations represent a convenient opportunity to investigate the dynamics of maximal female reproductive rates under conditions of food abundance, veterinary care and reduced parasite exposure. Such effects might be far more significant and easier to detect in species with short lifespans and high reproductive rates (Tidière et al., 2016). Nonetheless, compared to their wild relatives, semi-captive ring-tailed lemurs did not seem to experience reproductive senescence (Lemaître and Gaillard, 2017). However, in two species of captive ruffed lemurs, litter size as well as infant survival follow an inverted U-shaped pattern, with a decreasing point after mothers reached the age of 9 and 11.5 years, respectively (Tidière et al., 2018). In Hanuman langur females, members of free-ranging populations exhibited a later onset of about 10 months of first conception than they do in captivity (Sommer et al., 1992). Similarly, the timing of first reproduction in wild female common marmosets was delayed by several months compared to their captive counterparts (Tardif et al., 2008). Comparisons of reproductive effort between wild and captive populations can therefore provide information about the magnitude of phenotypic plasticity in these traits, offering a benchmark for maximal reproductive effort to which data from populations with various environmental constraints can be compared.
Non-human primates share fundamental features of their life histories with humans (van Schaik and Isler, 2012), so that studying a basal group of primates can contribute valuable comparative information on the evolution of reproductive senescence in general and (human) menopause in particular. Despite their relatively long lifespans, slow development and reproductive rates, long-term field studies of primates have generated valuable demographic data for comparative studies of fundamental questions in life-history evolution (Bronikowski et al., 2011; Morris et al., 2011; Colchero et al., 2016, 2021; Campos et al., 2017, 2022). Lemurs, the endemic primates of Madagascar, have been underrepresented in these comparative studies because of the limited number of long-term study sites (Jolly, 2012; Kappeler and Fichtel, 2012a; Sussman et al., 2012; Wright et al., 2012; Kappeler et al., 2017). They are nonetheless interesting for studies of reproductive senescence because they tend to live longer than most anthropoid primates of similar body mass, with a remarkable maximum longevity of over 30 years for mammals of < 10 kg, at least in captivity (Zehr et al., 2014). Lemurs also exhibit impressive interspecific variation in age at first reproduction, litter size and reproductive rates (Kappeler, 1995, 1996; Ross, 1998; Tecot et al., 2013), but patterns and drivers of intraspecific variation have not yet been studied in great detail.
Representatives of two lemur families (Indriidae and Lemuridae) that evolved diurnal activity, group living and single births independently (Kappeler, 1998; Kappeler and Pozzi, 2019), thus resembling anthropoid primates in key life-history features, have been subjected to long-term field studies. The available published information about lemur reproductive senescence can be summarized as follows (for wild mouse lemurs see Rina Evasoa et al., 2018; for captive lemurs: Zehr et al., 2014). First, one study of Milne Edward’s sifakas (Propithecus edwardsi) revealed that, although no overall effect of age on fertility could be found, offspring survival was significantly decreased in years with below-average rainfall during the lactation period, but the age classification in this study was based on estimates of tooth wear rather than known ages (Wright et al., 2008). Second, in wild Verreaux’s sifakas (P. verreauxi) at Beza Mahafaly, which were also assigned to one of 5 age classes based on tooth wear analyses, females began reproducing surviving infants with 5 years of age, followed by a sharp increase in reproductive rates over subsequent years and a steady phase of about a decade before a decline in average fertility set in around the age of 18 (Richard et al., 2002). Nonetheless, many females in their early 20 s continued to reproduce regularly, and the oldest mother was estimated to be 28 years old. Controlling for body mass, these sifakas had a greater age at last reproduction than 14 other primate species for which these data were available at the time. Female reproductive performance in this species was also related to unpredictable climate because a severe drought decreased birth rates by almost 20%, and only few offspring born that year survived (Richard et al., 1991, 2000). At Kirindy Forest, the proportion of female Verreaux’s sifakas reproducing varied widely across years, and part of this variation was explained by variation in the number of co-resident females (Kappeler and Fichtel, 2012a). Finally, wild female ring-tailed lemurs, which were also grouped into age-classes, reproduced irrespective of age, but birth rates declined in groups with larger numbers of females and in years with a drought (Gould et al., 2003). In another population, old female ring-tailed lemurs reproduced at slightly, but not significantly, reduced rates, presumably because most of them had already died or disappeared before a clear pattern could have been detected (Ichino et al., 2015).
Additional data from long-term studies of age-specific fertility in the wild are obviously needed to further investigate reproductive senescence and its underlying mechanisms in lemurs. Here, we present such data on sympatric wild Verreaux’s sifakas and redfronted lemurs (Eulemur rufifrons), offering an opportunity for contrasting the life histories of representatives of independently evolved lineages to identical (fluctuations in) environmental conditions. Both species live in small multi-male, multi-female groups and feed on a broad range of fruits and leaves (Koch et al., 2017). Verreaux’s sifakas are slightly larger than redfronted lemurs (2.9 vs. 2.2 kg), their groups are of similar size (6.1 vs. 5.4 individuals), female reproduction begins later (5 vs. 3 years), but both species typically produce singletons and can live well over 20 years (Richard et al., 2000, 2002; Kappeler and Fichtel, 2012a,2016; Zehr et al., 2014). Despite female philopatry in both species, female Verreaux’s sifakas occasionally emigrate into other groups (Kappeler and Fichtel, 2012a), and female redfronted lemurs very rarely succeed in immigrating into other groups after being evicted from their natal one (Kappeler and Fichtel, 2012b). In order to assess the effects of ecological challenges on female reproduction, we compare our results with data from closely related captive Propithecus and Eulemur species, for which the Duke Lemur Center (DLC) (Durham, NC, United States) has accumulated an impressive data set under uniform captive conditions (Zehr et al., 2014).
The main aims of our study are therefore to evaluate female reproductive output and IBIs in two sympatric wild lemur populations as a function of age, the number of adult females (to account for female competition) as well as rainfall as a proxy for variation in food availability. To further investigate the relationship between ecology and life-history dynamics, we compare data on wild and captive populations of the same two genera to account for annual environmental variation and its consequences for reproductive senescence in the unpredictable environment of Madagascar. In line with the relevant evolutionary theory, we expected reproductive senescence (i) to occur in these relatively long-lived small mammals, and (ii) to be less pronounced in captive animals because they are buffered from natural hazards.
The data presented in this study come from two sources. First, we collected demographic and life-history data from sympatric populations of Verreaux’s sifakas and redfronted lemurs at Kirindy Forest, western Madagascar, a dry deciduous forest exposed to pronounced climatic seasonality (Kappeler and Fichtel, 2012a). Beginning in 1995 (sifakas) and 1996 (redfronted lemurs), members of several adjacent groups of both species, including immigrants and newborns, have been regularly captured, measured and individually marked with Passive Integrated Transponder (PIT) tags and unique nylon collars. One individual per group has been equipped with a radio collar to facilitate multiple censuses per week during which each group’s composition, including the number of co-resident adult females, has been recorded. Birth dates of newborn infants are therefore known with a precision of a few days; in most cases we know the exact birthday. Ages of individuals at the beginning of the study could be determined with an accuracy of a year because both species are strict annual breeders and juveniles of different ages clearly differ in body mass from each other; ages of adults during first captures were estimated based on patterns of tooth wear. We therefore created two data sets for the analyses of age-dependent reproductive rates of these two species: dataset A contains only individuals with exactly known ages; dataset B also includes females that were adults at the beginning of the study and whose ages have been estimated. Finally, because climatic data were not collected regularly during the early years of the study, we used published rainfall data from the CHIRPS (n.d.) data base to assess interannual variation on precipitation.
Second, in February 2022 we extracted life-history data from the published records of the Duke Lemur Center (DLC; Zehr et al., 2014), which houses captive colonies of sister species of the two species studied at Kirindy. Because P. verreauxi and E. rufifrons are not kept at the DLC, we extracted reproductive data (i.e., age at first reproduction, IBIs, age at all subsequent births) for Coquerel’s sifaka (P. coquereli) and red lemur (E. rufus) females of known ages. Both species are similar to P. verreauxi and E. rufifrons in body size, social system and also inhabit the dry deciduous forests of western Madagascar in the wild (Mayor et al., 2004; Razafindramanana et al., 2020).
We compared the age at first reproduction of females with known age between the captive and wild populations using a Mann–Whitney U-test. Age at last reproduction was compared between captive and wild lemurs with known and estimated age using a Kruskal–Wallis test. Since age was estimated for only three captive red lemur females, we did not include them in this comparison.
To estimate the effect of age on the likelihood of giving birth to an offspring, we fitted Generalized Linear Mixed Models (GLMM; Baayen, 2008) with binomial error structure and logit link function, with giving birth as the binary response (“yes” or “no”). For wild lemurs, we included as fixed effects, female’s age, the number of adult females present in the month of birth, and annual cumulative rainfall as control factors. Since reproductive senescence is a within-individual process, we separated within-individual aging patterns from between-individual heterogeneity, i.e., selective appearance or disappearance of individuals, by including longevity or age at first or last reproduction as additional control factors (van de Pol and Verhulst, 2006; Nussey et al., 2008). We fitted this model on each of the two data sets (A and B) for wild lemurs, one on all females including those for which the exact age was not known and the one including only females with known age. Longevity was included in the model including all females, whereas in the subset of females with known age, we included age at first and last birth to account for both, selective appearance and disappearance (van de Pol and Verhulst, 2006; Nussey et al., 2008). As random effects, we included female and group identity. For wild lemurs we included the random slopes of female’s age, the number of adult females and rainfall within mothers and groups. Originally, we also included correlations between random slopes and intercepts, but as the models did not converge, we had to exclude them again. As information on group composition as well as group identity was not available for data on captive lemurs, we included as fixed effects only female’s age and longevity because birth rates might have been influenced by husbandry decisions. Female’s age within the random slope of female’s identity was included as random factor. In Coquerel’s sifakas, we had to remove the correlation between random slopes and intercepts, as the models did not converge.
For each data set, we fitted three models, one including only “age,” one including “age and quadratic age,” and a threshold model (see Supplementary Material for a detailed description of the threshold model). Most, except three models, including age and quadratic age did not reveal a negative estimate for quadratic age, thus, not matching the expected inverted U-shape. Therefore, we report these models only in the Supplementary Material (Supplementary Tables 1–4). For models where the estimate for quadratic age was negative, we compared AIC values between the model including only age and age as well as quadratic age, and present the models with a delta AIC < 2 in the main text. Since the results of all threshold models, except one, revealed negative estimates for both, the lower threshold age as well as the upper threshold age, not matching the roughly expected inverted U-shape, we do not report model outputs. Finally, we explored whether females experienced reproductive completion. Females, that lived longer than the mean IBI + 2*SD after having given birth to the last live infant were considered as having completed reproduction (Alberts et al., 2013).
We compared all models to a null model, comprising all random and fixed effects except for age, with a likelihood ratio test (Schielzeth and Forstmeier, 2009). This full-null model serves to avoid “cryptic multiple testing” (Forstmeier and Schielzeth, 2011). Sample sizes for the reproductive output models can be found in Tables 1, 4.
Table 1. Overview of life-history milestones and sample sizes of wild Verreaux’s and captive Coquerel’s sifakas.
To determine whether female age has an effect on IBIs, we fitted Generalized Linear Mixed Models with a Gamma-distribution and a log link. For the two wild populations, we used IBIs as the response and female’s age, number of adult females present at birth, annual cumulative rainfall and longevity or age at first and last birth as fixed effects, as well as individual and group identity as random effects. For Verreaux’s sifakas we included the random slopes of age, the number of adult females and rainfall within mothers as well as age, longevity and rainfall within groups without correlations between random slopes and intercepts. For wild redfronted lemurs, we included the random slopes of age, the number of adult females and rainfall within mothers as well as age, the number of adult females, longevity and rainfall within groups. As in the reproductive output models, we estimated a model including only “age” and one including “age” and “quadratic age,” respectively. Similar to the reproductive output models, we compared AIC values of models including age or age and quadratic age, when the estimate of quadratic age was positive. In addition, all full models were compared to a null model, comprising all random and fixed effects except for age, and we checked for overdispersion, which revealed that all models were highly underdispersed. Therefore, we did not fit additional threshold models. Sample sizes for IBI models can be found in Table 1. For the two captive populations, we did not estimate whether IBIs were influenced by mothers age because some females were put on contraception due to husbandry decisions.
Analyses were conducted using R (version 4.1.0; R Core Team, 2020), applying the function glmer and lmer from the package “lme4” (version 1.1-21; Bates et al., 2015). We centered all quantitative predictors to a mean of zero and a standard deviation of one before including them into the models, to facilitate model convergence. All theoretically identifiable random slopes were included to avoid Type I errors (Barr et al., 2013). Confidence intervals were obtained for all models by means of parametric bootstraps using the function “bootMer” of the package “lme4,” applying 1000 parametric bootstraps. We checked for collinearity by determining Variance Inflation Factors (VIF; Dobson and Barnett, 2018) for a standard linear model without random effects using the package “car” (version 3.0.11; Field, 2005). VIF values were smaller than 1.9 in all cases. We estimated model stability by dropping levels of the random effect one at a time from the data set and compared the obtained estimates to the estimates obtained for the full data set. This revealed that all models exhibited good stability.
In total, 38 wild sifaka females gave birth to 225 singleton infants across 25 years. All females, except three, gave birth to more than one infant during our study. The exact age was known for 13 females, born 1995 or later, who had an age at first reproduction of 5.56 ± 1.06 (mean ± SD; Table 1) years. Of these 13 females, seven had already died at the time of this study. These females had an age at last reproduction of 11.65 ± 4.39 years. Of the other females (N = 25), for which we estimated age, one was still alive, and the remaining 24 females had an age at last reproduction of 11.97 ± 4.88 years. Only two of these females lived longer than the mean IBI + 2*SD after having given birth to the last live infant (Figure 2A). They were 11.98 and 21.08 years old, respectively. Two females that were still alive also experienced a long IBI, but they were rather young, about 8 years each, indicating that these long IBIs might have been due to other reasons than reproductive completion.
Overall, Verreaux’s sifaka females gave birth to 5.92 ± 3.83 infants during their entire lifespan, with a range of 1 and 16 infants. Focusing on females of known reproductive lifespan only (N = 7), we found that they produced on average 5.86 ± 3.49 infants. The average IBI for all females was 1.25 ± 0.44 years (Table 1). Following 74% of births, females gave birth again after about 1 year later, in 22% of cases they gave birth again about 2 years later, and only in two cases (2%) females gave birth again after 3 years (Figure 1C). The latter two cases occurred toward the end of the reproductive lifespan (i.e., at 9 and 11 years, respectively).
Figure 1. Probability of giving birth as a function of mother’s age for (A) wild Verreaux’s sifakas and (B) captive Coquerel’s sifakas. (C) Frequency distribution of interbirth intervals of wild Verreaux’s sifakas (light gray; N = 193) and captive Coquerel’s sifakas (dark gray; N = 161). Dashed lines represent the regression lines, gray polygons represent the 95% confidence intervals, and dots represent the number of observations with the area of dots being proportionate to the number of observations per binned age (Verreaux’s sifakas: range 2–33, Coquerel’s sifakas: 2–26). Note that the dots in panels (A,B) are not to the same scale.
Figure 2. Number of (A) wild Verreaux’s sifaka and (B) captive Coquerel’s sifaka females that gave birth (orange) or not (yellow) in their last year of life or observation (age in years).
In the model including all females, the probability of giving birth was independent of female’s age, the number of co-resident females, annual rainfall and longevity (Figure 1A and Table 2A). Including quadratic age in the model did not result in a better fit (AIC: age = 312, age and quadratic age = 318). In the subset of females with known age, the probability of giving birth was also independent of female’s age, the number of co-resident females, annual rainfall, and age at first or last birth (Table 2B). Adding quadratic age did not improve the model fit (AIC: age = 127, age and quadratic age = 133).
Table 2. Results of the models in wild Verreaux’s sifakas on (A) the probability of giving birth for all females, (B) for female’s with known age, and variation in interbirth intervals (C) for all females and (D) for females with known age.
The models on variation in IBIs including age revealed a better fit than those including age and quadratic age (AIC: all females: age = 155, age and quadratic age = 159; females with known age: age = 61, age and quadratic age = 67). Variation in IBIs was neither predicted by mother’s age, nor number of adult females in a group, rainfall or longevity (Table 2C). Similarly, in the subset of females with known age, variation in IBIs was neither predicted by mother’s age, number of adult females in a group, rainfall nor age at first or last birth (Table 2D). Both models where highly under-dispersed (all females: dispersions parameter = 0.074, p = 1, females with known age: dispersions parameter = 0.078, p = 1), so that results should be treated cautiously. Hence, our analyses revealed no evidence for wild Verreaux’s sifakas to experience reproductive senescence, neither in the probability of giving birth, nor in terms of extending their IBIs.
In total, 35 captive sifaka females gave birth to 196 singleton infants across 41 years. The exact age was known for all females. Age at first reproduction was on average 5.85 ± 1.96 years, which did not differ between captive and wild sifakas (Mann–Whitney U-test: z = 0.034 P = 0.972; Table 1). Four females gave birth only once, three of them were alive at the time of the census, and one had already died. Thirty-one females gave birth to more than one infant. Of those, 15 females were still alive at the time of the census and 16 had already died. The latter subsample had an age at last birth of 13.21 ± 4.20. Age at last reproduction did not differ among captive and wild sifakas with known or estimated age (Kruskal–Wallis: χ2 = 0.317, P = 0.853). After having given birth to the last live infant, six females lived longer than the mean IBI + 2*SD (Figure 2B). One of them died at an age of 11.91 years and gave birth to the last live infant 4.99 years before. The other five females were still alive, two were 5 and 7 years old, and three between 9 to 13 years, suggesting that the latter three ones might have already experienced reproductive completion. However, since we do not know whether some females failed to give birth because of management decisions, these IBIs should be treated with caution. Therefore, we did not estimate whether variation in IBIs covaried with mother’s age.
Overall, Coquerel’s sifaka females gave birth to an average of 5.6 ± 3.88 infants, with a range between 1 and 13 infants (Table 1). Mothers of known reproductive lifespan (N = 16) produced on average 6.77 ± 3.83 infants. The average IBI was 1.21 ± 0.7 years and they did not exhibit such a clear annual pattern as wild sifakas (Table 1 and Figure 1C). The model fitting the probability of giving birth in captive sifakas revealed a better fit when was age included in comparison to age and quadratic age (AIC: age = 270, age and quadratic age = 273). Older females were less likely to give birth, and birth rates were influenced by longevity only by trend (Figure 1B and Table 3). Hence, captive Coquerel’s sifakas appear to have experienced reproductive senescence.
Table 4. Overview of life-history milestones and sample sizes of wild redfronted lemurs and captive red lemurs.
In total, 42 redfronted lemur females gave birth to 201 singleton infants, and one female gave birth to twins across 25 years. Eight females gave birth to only one infant during our study period. The exact age was known for 26 females, who had an age at first reproduction of 3.59 ± 0.82 years (Table 4). Of these 26 females born in 1996 or later, 24 had already died by the time of this study. They had an age at last reproduction of 8.22 ± 4.22 years. Of the other females (N = 16), for which we estimated their age, one was still alive, and the remaining 15 females had an age at last reproduction of 8.24 ± 4.67 years. After having given birth to the last live infant, seven females lived longer than the mean IBI + 2*SD (Figure 3A). Their age ranged between 5.75 and 20.54 years, with only two females being older than the mean age of last reproduction. They were 18.50 and 20.54 years old at the time of death and gave birth to their last infant at an age of 16.07 and 18.04 years, respectively. Hence, these two mothers may have completed reproduction but were reproductively active until a very old age.
Figure 3. Number of (A) wild redfronted and (B) captive red lemur females that gave birth (orange) or not (yellow) in their last year of life or observation (age in years).
Overall, redfronted lemur females gave birth to 4.83 ± 3.52 infants, with a range between 1 and 17 infants. Females of known reproductive lifespan (N = 24), produced on average 4.75 ± 3.19 infants. The average IBI was 1.20 ± 0.50 years (Figure 4C and Table 4). Following 83% of births, females gave birth again after about 1 year later, in 14% of cases they gave birth again about 2 years later, and only in five cases (3%) females gave birth again after 3 years or more. One case occurred already at age of 3.99 years, whereas the other four cases occurred toward the end of the reproductive lifespan (i.e., at 8, 11, 18 and 15 years, respectively). Hence, wild redfronted lemurs might have experienced an effect of reproductive senescence, but average IBIs did not change with age (Figure 4C).
Figure 4. Probability of giving birth as a function of mother’s age for (A) wild redfronted lemur and (B) captive red lemur. (C) Frequency distribution of interbirth intervals of wild redfronted lemurs (light gray; N = 132) and captive red lemurs (dark gray; N = 103). Dashed lines represent the regression lines, gray polygons represent the 95% confidence intervals, and dots represent the number of observations with the area of dots being proportionate to the number of observations per binned age (redfronted lemurs: range 2–23, red lemurs: 2–26). Note that the dots in panels (A,B) are not to the same scale.
The model including age provided a better fit than the model including age and quadratic age (AIC: all females: age = 259, age and quadratic age = 263). The probability of giving birth was influenced by female’s age and rainfall (Figure 4A and Table 5A). Older females were less likely to give birth and females were more likely to give birth in years with more rainfall. Longevity did not influence the probability of giving birth. In the subset of females with known age, the probability of giving birth was also better predicted by the model including age than by the one including age and quadratic age (AIC: all females: age = 189, age and quadratic age = 194). Older females were less likely to give birth, and females were more likely to give birth when more adult females were in the group as well as in years with more rainfall (Table 5B). Age at first and last infant did not co-vary with the probability of giving birth. In both data sets (all females, females with known age), variation in IBIs was better explained by the model including age and quadratic age than only age (AIC: all females: age = 158, age and quadratic age = 129, females with known age: age = 125, age and quadratic age = 121). In the model including all females, interbirth length was not influenced by female’s age, number of adult females, longevity but by rainfall. IBIs were longer in years with less rainfall (Table 5C). In the data set including only females with known age, interbirth length was influenced by none of the factors, and rainfall influenced the length of IBIs only by trend (Table 5D). Since both models were highly under-dispersed (all females: dispersions parameter = 0.09, p = 1, females with known age: dispersions parameter = 0.11, p = 1) and only by trend significantly different from the null model (Tables 5C,D), results should be treated with caution.
Table 5. Results of the models in wild redfronted lemurs on (A) the probability of giving birth for all females, (B) for female’s with known age, and variation in interbirth intervals (C) for all females and (D) for females with known age.
In total, 35 captive red lemur females gave birth to 130 singleton infants and five mothers gave birth to 11 twins across 56 years. Six females gave birth to only one infant. The exact age was known for all females, which had an age at first reproduction of 3.64 ± 3.493 years. In comparison to wild redfronted lemurs, captive red lemurs were on average slightly older at first reproduction (Mann–Whitney U-test: z = 2.456, p = 0.014). Of these 35 females, 17 are known to have already died at the time of this study. These females had an age at last reproduction of 6.91 ± 5.81 years. Age at last reproduction did not differ between captive and wild redfronted lemurs with known or estimated age (Kruskal–Wallis: χ2 = 2.037, P = 0.361). After having given birth to the last live infant, 18 females lived longer than the mean IBI + 2*SD (Figure 3B). They were between 6.79 and 32.67 years old (mean = 20.02, SD = 7.66), with almost all females being older than the age of last reproduction of females with known reproductive lifespan, i.e., 6.91 ± 5.81 years. Since we do not know whether some females failed to give birth because of management decisions, these IBIs should be treated with caution. Therefore, we did not estimate whether variation in IBIs covaried with mother’s age.
Overall, red lemur females gave birth to 4.34 ± 4.65 infants, with a range between 1 and 19 infants. Females of known reproductive lifespan (N = 17) produced on average 3.71 ± 4.01 infants. The average IBI was 1.39 ± 1.11 years (Figure 4C and Table 4). The model on the probability of giving birth in red lemurs including age revealed a better fit than the one including age and quadratic age (AIC: age = 330. age and quadratic age = 354). We found an effect of female’s age but not of longevity on the probability of giving birth, with older females being less likely to give birth (Figure 4B and Table 6). Hence, captive red lemurs might also have experienced an effect of reproductive senescence.
Previous research indicated that lemurs do not experience any form of reproductive cessation and thus, are principally capable of reproducing until death (Wright et al., 2008), whereas other studies revealed reproductive senescence late in life (Alberts et al., 2013). The present analyses revealed strong evidence that females in three out of four populations experience reproductive senescence, thereby offering a basis for an exploration of the sources and dynamics of age-specific female reproduction in independently evolved lineages under different environmental conditions. In line with our first prediction, we did find evidence for reproductive senescence in three populations of these relatively long-lived small mammals. In wild redfronted lemurs, captive red lemurs and captive Coquerel’s sifakas, the probability of giving birth decreased with mother’s age, but it was unaffected by maternal age in wild Verreaux’s sifakas. While the pattern observed in the first three of our study populations corresponds to the pattern also observed in about 70% of mammalian species to date (Lemaître et al., 2020), the absence of evidence for reproductive senescence in Verreaux’s sifaka is intriguing, also because it only partly corroborates results of previous studies with data from another population of this species, which reported reproductive senescence (Alberts et al., 2013) but no change in IBIs with age (Campos et al., 2022). Thus, populations may vary in patterns of reproductive senescence and/or different components of reproductive senescence may vary independently.
This outcome across populations rejects our second prediction, stating that reproductive senescence might be less pronounced in captive animals because they are buffered from external adversities. With respect to the relative importance of age in modulating reproductive rates, we found that neither the number of co-resident females, nor annual rainfall did predict variation in the probability of giving birth in wild Verreaux’s sifakas either. In wild redfronted lemurs, in contrast, mothers were more likely to give birth in years with more rainfall. Finally, birth rates did not co-vary with any proxy indicating potential selective appearance or disappearance in the population. i.e., longevity or age at first or last reproduction (van de Pol and Verhulst, 2006; Nussey et al., 2008). Only in captive Coquerel’s sifakas longevity co-varied by trend with birth rates, suggesting that longer-lived females might have been more efficient at giving birth throughout their life.
Precise age estimates of relatively long-lived mammals like these lemurs require year- or even decade-long investment into long-term field studies. Because such studies are rare or challenging to sustain long enough (Kappeler et al., 2012, 2017), age has also been estimated from tooth wear or other morphological traits, or individuals have been grouped into coarse age classes. While estimates are clearly a less preferred, albeit often unavoidable option, the magnitude of the discrepancies between the two approaches remain poorly known. Our study offered a rare opportunity to compare classes of females of the same populations with either precisely known or estimated ages. In both Verreaux’s sifakas and redfronted lemurs, age at first or last reproduction as well as total number of infants did not differ widely between these groups of females, suggesting that their ages can be reliably estimated and that the age effects found in studies that relied on age estimates (see above) are probably robust.
In wild Verreaux’s sifakas, four females were still alive after having given birth for at least an IBI + 2*SD, which is suggestive of reproductive completion (Alberts et al., 2013), but two of them were only about 8 years old, indicating that these long IBIs might be due to other reasons, like poor health or undetected abortions. Only one very old captive Coquerel’s sifaka female did not reproduce, and her long IBI is also difficult to interpret because it might have been the result of husbandry decisions. In wild and captive Eulemur populations, we found differences in the number of females reaching various ages as well as in the proportion of them giving birth in their respective last year of life, with females in the wild reproducing almost every year until very old age. Also, after having given birth to the last live infant, only five wild redfronted lemur females lived beyond the mean IBI + 2*SD. In captive red lemur females, even 18 females lived longer than the mean IBI + 2*SD, but because of management interventions, these data have to be treated with great caution (see below). In any event, there is evidence to suggest that – as in many other mammals – there is little scope for these lemur females to exhibit grandmaternal care, but we do not know at present whether females live on average longer than males; a pattern found among the few species with grandparental care (Péron et al., 2019). The significant decline in reproductive rates in wild redfronted lemurs contrasts with previous reports for other members of both, the Lemuridae (Gould et al., 2003; Ichino et al., 2015) and Indridae (Richard et al., 2002; Wright et al., 2008), making it difficult at present to derive general conclusions about this aspect of life-history evolution in this basal group of primates.
In captivity, animals are buffered from fluctuations and limitations of food availability as well as from adverse environmental factors like droughts, extreme temperatures and parasitism. In addition, veterinary care contributes to improved health, so that overall, more energy should be available for growth and reproduction. As a result, captive females should be able to sustain higher reproductive rates, which should be reflected by shorter IBIs and attenuated or delayed reproductive senescence. Because the most common cause of environmentally driven mortality in the wild, i.e., predation, is essentially eliminated in captivity, females should on average also live longer than in the wild.
These predictions were only partially supported empirically in our study. In the Eulemur species, reproductive senescence occurred independent of the environmental conditions they found themselves in. However, although captive and wild sifakas did not differ in their maximal ages at reproduction or IBIs (Figure 2), reproductive senescence occurred only in captive, but not in the wild sifakas. The latter finding is in contrast to findings of reproductive senescence in another wild population of Verreaux’s sifakas in Beza Mahafaly (Richard et al., 2002; Alberts et al., 2013; Campos et al., 2022). At Beza Mahafaly, older females were less likely to give birth and they were less likely to close long IBIs by having another offspring; an aspect that we did not explicitly model in our approach. The differences between the two populations might be due to differences in sample size (Beza: 116 females; Kirindy: 38 females, 228 births), but a targeted comparison would be required to test this notion explicitly. These two populations differ, however, in the abundance of the fosa, which is absent at Beza Mahafaly, but common in Kirindy Forest (Kappeler and Fichtel, 2012b). Hence, at Kirindy, more older females, which are likely in a less good condition, might have fallen victim to predation, thereby obscuring potential effects of reproductive senescence. This notion is supported by a study of wild female Edward’s sifakas, where the fosa is also abundant, but where no indication of a decline in fertility in dentally senescent (aged > 18 years) females was found (Wright et al., 2008). Thus, variation in predation risk needs to be acknowledged in future demographic studies of wild primates.
Whereas more captive red lemurs lived indeed beyond 20 years than wild redfronted lemurs, their IBIs did not differ, and reproductive rates declined with age in both populations (Figures 4A,B). IBIs were longer in the captive population, which might be due to husbandry decisions. In the wild, however, IBIs did not co-vary with female’s age. Overall, the IBIs of wild and captive congers in our study nevertheless exhibited generally similar frequency distributions, with the vast majority of females giving birth every year. IBIs may exhibit such limited plasticity because of the photoperiodic control of lemur reproduction (Rasmussen, 1985; Heldstab et al., 2021), which limits ovulations to a narrow time window of 2–3 months, presumably as an adaptation to pronounced seasonality of most lemur natural habitats (Meyers and Wright, 1993).
Data on reproductive performance in captivity have to be treated with caution, however. Hormonal birth control and management decisions have presumably impacted the data on the onset of reproduction and individual fertility. Females that are capable of reproducing and housed with an adult male, but are not recommended to breed, receive contraception, but these decisions are re-evaluated every year. Thus, these data should therefore be treated and interpreted carefully and serve to illustrate the general point about shortcomings and limitations of life-history data from captive animals.
The present study revealed that neither the number of co-resident females nor annual rainfall predicted variation in the probability of giving birth for wild Verreaux’s sifakas. Reproductive rates of wild redfronted lemurs were higher in years with more rainfall, but unaffected by the number of co-resident females. There is evidence that lemur reproduction is challenged by resource availability (reviewed in Kappeler and Fichtel, 2015, Kappeler et al., 2022), so that more rain may improve food availability for reproducing females. The different responses by Verreaux’s sifakas and redfronted lemurs might be due to the fact that sifaka reproduction at Kirindy is embedded within an 8-month dry season (Kappeler and Fichtel, 2012b) with little phenological tree activity, whereas redfronted lemur lactation coincides with the onset of the production of flowers and young leaves by most trees, which may benefit from more rain. Similarly, birth rates of ring-tailed lemurs, Milne Edward’s sifakas and Verreaux’s sifakas at Beza Mahafaly also exhibited some reduction in response to low rainfall or droughts (Richard et al., 2000; Gould et al., 2003; Wright et al., 2008).
Competition by other females did not impact reproductive rates in Verreaux’s sifakas. However, in the redfronted lemur data set of females with known age, we found a positive effect on the probability of giving birth with an increase in the number of adult females, even though a previous analysis (Kappeler and Fichtel, 2012b) indicated an increase in the intensity of female competition with increasing numbers of resident females. Thus, more data from other species and study sites are required for a more robust assessment, but, overall, the effect of age on female reproduction appears to be more pervasive than those of ecological and social factors, for which this study revealed no consistent effects.
We investigated effects of age, female competition and variation in food availability on female reproductive performance and IBIs in two sympatric wild populations of true lemurs and sifaka and compared these data with captive populations of the same two genera to identify consequences for reproductive senescence. We found some negative effects of age on reproductive performance in three out of four populations. Wild and captive populations did not differ in these life-history traits in the predicted manner, but data from the captive populations were presumably compromised by husbandry decisions. In the wild, only some females live long beyond their last birth, offering no evidence for fertility completion in these basal primates, but reproductive senescence appears to set in shortly before the end of their natural lifespan. Our study contributes additional data on age-related life-history adaptations in monotocous primates and, hence, to a more comprehensive appraisal of reproductive senescence.
The datasets presented in this study can be found in online repositories. The names of the repository/repositories and accession number(s) can be found 10.6084/m9.figshare.20036558.
The animal study was reviewed and approved by the Caff/Core Ministry of the Environment, Madagascar.
PK and CF collected the data and conceived research. LPr and CF analyzed data. LPe and PK drafted the manuscript. All authors contributed to writing the final manuscript.
This research was grants by the Deutsche Forschungsgemeinschaft to PK and CF and institutional support by the Deutsches Primatenzentrum over the past 25 years ascertained our long-term data collection.
The authors declare that the research was conducted in the absence of any commercial or financial relationships that could be construed as a potential conflict of interest.
All claims expressed in this article are solely those of the authors and do not necessarily represent those of their affiliated organizations, or those of the publisher, the editors and the reviewers. Any product that may be evaluated in this article, or claim that may be made by its manufacturer, is not guaranteed or endorsed by the publisher.
We thank Michael Cant for very constructive comments and stimulating discussions, Roger Mundry for statistical advice, and the Kirindy field assistants for their hard work in the field. Comments by Jean-Francois Lemaître and the referees prompted important new analyses and improved the first version of this manuscript.
The Supplementary Material for this article can be found online at: https://www.frontiersin.org/articles/10.3389/fevo.2022.894344/full#supplementary-material
Alberts, S. C., Altmann, J., Brockman, D. K., Cords, M., Fedigan, L. M., Pusey, A., et al. (2013). Reproductive aging patterns in primates reveal that humans are distinct. PNAS 110, 13440–13445. doi: 10.1073/pnas.1311857110
Altmann, J., Gesquiere, L., Galbany, J., Onyango, P. O., and Alberts, S. C. (2010). Life history context of reproductive aging in a wild primate model. Ann. N.Y. Acad. Sci. 1204, 127–138. doi: 10.1111/j.1749-6632.2010.05531.x
Atsalis, S., and Margulis, S. (2008). Primate reproductive aging: from lemurs to humans. Interdiscip. Top. Gerontol. 36, 186–194. doi: 10.1159/000137710
Baayen, R. H. (2008). Analyzing Linguistic Data: A Practical Introduction to Statistics using R. Cambridge: Cambridge University Press, doi: 10.1017/CBO9780511801686
Barr, D. J., Levy, R., Scheepers, C., and Tily, H. J. (2013). Random effects structure for confirmatory hypothesis testing: keep it maximal. J. Mem. Lang. 68, 255–278. doi: 10.1016/j.jml.2012.11.001
Bates, D., Mächler, M., Bolker, B., and Walker, S. (2015). Fitting linear mixed-effects models using lme4. J. Stat. Softw. 67, 1–48. doi: 10.18637/jss.v067.i01
Beehner, J. C., and Lu, A. (2013). Reproductive suppression in female primates: a review. Evol. Anthropol. 22, 226–238. doi: 10.1002/evan.21369
Bronikowski, A. M., Altmann, J., Brockman, D. K., Cords, M., Fedigan, L. M., Pusey, A., et al. (2011). Aging in the natural world: comparative data reveal similar mortality patterns across primates. Science 331, 1325–1328. doi: 10.1126/science.1201571
Campos, F. A., Altmann, J., Cords, M., Fedigan, L. M., Lawler, R., Lonsdorf, E. V., et al. (2022). Female reproductive aging in seven primate species: patterns and consequences. Proc. Nat. Acad. Sci. U.S.A. 119:e2117669119. doi: 10.1073/pnas.2117669119
Campos, F. A., Morris, W. F., Alberts, S. C., Altmann, J., Brockman, D. K., Cords, M., et al. (2017). Does climate variability influence the demography of wild primates? Evidence from long-term life-history data in seven species. Glob. Change Biol. 23, 4907–4921. doi: 10.1111/gcb.13754
Caro, T. M., Sellen, D. W., Parish, A., Frank, R., Brown, D. M., Voland, E., et al. (1995). Termination of reproduction in nonhuman and human female primates. Int. J. Primatol. 16, 205–220. doi: 10.1007/bf02735478
CHIRPS (n.d.). CHIRPS: Climate Hazards Group InfraRed Precipitation With Station Data. Available online at: https://developers.google.com/earth-engine/datasets/catalog/UCSB-CHG_CHIRPS_DAILY?hl=en (accessed May 10, 2022).
Colchero, F., Aburto, J. M., Archie, E. A., Boesch, C., Breuer, T., Campos, F. A., et al. (2021). The long lives of primates and the ‘invariant rate of senescence’ hypothesis. Nat. Commun. 12:3666. doi: 10.1038/s41467-021-23894-3
Colchero, F., Rau, R., Jones, O. R., Barthold, J. A., Conde, D. A., Lenart, A., et al. (2016). The emergence of longevous populations. PNAS 113, E768–E7690. doi: 10.1073/pnas.1612191113
Comizzoli, P., and Ottinger, M. A. (2021). Understanding reproductive aging in wildlife to improve animal conservation and human reproductive health. Front. Cell Dev. Biol. 9:680471. doi: 10.3389/fcell.2021.680471
Dobson, A. J., and Barnett, A. G. (2018). An Introduction to Generalized Linear Models. Boca Raton, FL: CRC press, doi: 10.1201/9781315182780
Ellis, S., Franks, D. W., Nattrass, S., Cant, M. A., Bradley, D. L., Giles, D., et al. (2018). Postreproductive lifespans are rare in mammals. Ecol. Evol. 8, 2482–2494. doi: 10.1002/ece3.3856
Fedigan, L. M., and Rose, L. M. (1995). Interbirth interval variation in three sympatric species of neotropical monkey. Am. J. Primatol. 37, 9–24. doi: 10.1002/ajp.1350370103
Field, A. (2005). Discovering Statistics Using SPSS: Introducing Statistical Methods Series. Thousand Oaks, CA: Sage Publications.
Forstmeier, W., and Schielzeth, H. (2011). Cryptic multiple hypotheses testing in linear models: overestimated effect sizes and the winner’s curse. Behav. Ecol. Sociobiol. 65, 47–45. doi: 10.1007/s00265-010-1038-5
Gaillard, J.-M., and Lemaître, J.-F. (2020). An integrative view of senescence in nature. Funct. Ecol. 34, 4–16.
Gould, L., Sussman, R. W., and Sauther, M. L. (2003). Demographic and life-history patterns in a population of ring-tailed lemurs (Lemur catta) at Beza Mahafaly Reserve, Madagascar: a 15-year perspective. Am. J. Phys. Anthropol. 120, 182–194. doi: 10.1002/ajpa.10151
Hayward, A., Mar, K. U., Lahdenperä, M., and Lummaa, V. (2014). Early reproductive investment, senescence and lifetime reproductive success in female Asian elephants. J. Evol. Biol. 27, 772–783. doi: 10.1111/jeb.12350
Healy, K., Ezard, T. H. G., Jones, O. R., Salguero-Gómez, R., and Buckley, Y. M. (2019). Animal life history is shaped by the pace of life and the distribution of age-specific mortality and reproduction. Nat. Ecol. Evol. 3, 1217–1224. doi: 10.1038/s41559-019-0938-7
Heldstab, S. A., van Schaik, C. P., Müller, D. W. H., Rensch, E., Lackey, L. B., Zerbe, P., et al. (2021). Reproductive seasonality in primates: patterns, concepts and unsolved questions. Biol. Rev. 96, 66–88. doi: 10.1111/brv.12646
Ichino, S., Soma, T., Miyamoto, N., Chatani, K., Sato, H., Koyama, N., et al. (2015). Lifespan and reproductive senescence in a free-ranging ring-tailed lemur (Lemur catta) population at Berenty. Madagascar. Folia Primatol. 86, 134–139. doi: 10.1159/000368670
Johnstone, R. A., and Cant, M. A. (2010). The evolution of menopause in cetaceans and humans: the role of demography. Proc. Biol. Sci. 277, 3765–3771. doi: 10.1098/rspb.2010.0988
Johnstone, R. A., and Cant, M. A. (2019). Evolution of menopause. Curr. Biol. 29, R112–R115. doi: 10.1016/j.cub.2018.12.048
Jolly, A. (2012). “Berenty Reserve, Madagascar: a long time in a small space,” in Long-Term Field Studies of Primates, eds P. M. Kappeler and D. P. Watts (Berlin: Springer), doi: 10.1007/978-3-642-22514-7_2
Kappeler, P. M. (1995). “Life history variation among nocturnal prosimians,” in Creatures of the Dark: The Nocturnal Prosimians, eds L. Alterman, M. K. Izard, and G. A. Doyle (New York, NY: Plenum Press), 75–92. doi: 10.1007/978-1-4757-2405-9_6
Kappeler, P. M. (1996). Causes and consequences of life history variation among strepsirhine primates. Am. Nat. 148, 868–891. doi: 10.1086/285960
Kappeler, P. M. (1998). Nests, tree holes, and the evolution of primate life histories. Am. J. Primatol. 46, 7–33. doi: 10.1002/(SICI)1098-2345(1998)46:1<7::AID-AJP3>3.0.CO;2-#
Kappeler, P. M., Cuozzo, F. P., Fichtel, C., Ganzhorn, J. U., Gursky-Doyen, S., Irwin, M. T., et al. (2017). Long-term field studies of lemurs, lorises, and tarsiers. J. Mammal. 98, 661–669. doi: 10.1093/jmammal/gyx013
Kappeler, P. M., and Fichtel, C. (2012a). “A 15-year perspective on the social organization and life history of sifaka in Kirindy Forest,” in Long-Term Field Studies of Primates, eds P. M. Kappeler and D. P. Watts (Berlin: Springer), 101–121. doi: 10.1007/978-3-642-22514-7
Kappeler, P. M., and Fichtel, C. (2012b). Female reproductive competition in Eulemur rufifrons: eviction and reproductive restraint in a plurally breeding Malagasy primate. Mol. Ecol. 21, 685–698. doi: 10.1111/j.1365-294X.2011.05255.x
Kappeler, P. M., and Fichtel, C. (2015). Eco-evo-devo of the lemur syndrome: did adaptive behavioral plasticity get canalized in a large primate radiation? Front. Zool. 12:S15. doi: 10.1186/1742-9994-12-S1-S15
Kappeler, P. M., and Fichtel, C. (2016). The evolution of Eulemur social organization. Int. J. Primatol. 37, 10–28. doi: 10.1007/s10764-015-9873-x
Kappeler, P. M., and Pozzi, L. (2019). Evolutionary transitions toward pair living in nonhuman primates as stepping stones toward more complex societies. Sci. Adv. 5:eaay1276. doi: 10.1126/sciadv.aay1276
Kappeler, P. M., Fichtel, C., and Radespiel, U. (2022). The island of female power? Intersexual dominance relationships in the lemurs of Madagascar. Front. Ecol. Evol. 10:858859. doi: 10.3389/fevo.2022.858859
Kappeler, P. M., Schaik, C. P., and Watts, D. P. (2012). “The values and challenges of long-term field studies,” in Long-Term Field Studies of Primates, eds P. M. Kappeler and D. P. Watts (Berlin: Springer), 3–18. doi: 10.1007/978-3-642-22514-7_1
Koch, F., Ganzhorn, J. U., Rothmann, J. M., Chapman, C. A., and Fichtel, C. (2017). Sex and seasonal differences in diet and nutrient intake in Verreaux’s sifakas (Propithecus verreauxi). Am. J. Primatol. 79, 1–10. doi: 10.1002/ajp.22595
Lee, D. S., Kang, Y. H. R., Ruiz-Lambides, A. V., and Higham, J. P. (2021). The observed pattern and hidden process of female reproductive trajectories across the life span in a non-human primate. J. Anim. Ecol. 90, 2901–2914. doi: 10.1111/1365-2656.13590
Lemaître, J.-F., Berger, V., Bonenfant, C., Douhard, M., Gamelon, M., Plard, F., et al. (2015). Early-late life trade-offs and the evolution of ageing in the wild. Proc. Biol. Sci. 282:20150209. doi: 10.1098/rspb.2015.0209
Lemaître, J.-F., and Gaillard, J.-M. (2017). Reproductive senescence: new perspectives in the wild. Biol. Rev. 92, 2182–2199. doi: 10.1111/brv.12328
Lemaître, J.-F., Ronget, V., and Gaillard, J.-M. (2020). Female reproductive senescence across mammals: a high diversity of patterns modulated by life history and mating traits. Mech. Ageing Dev. 192:111377. doi: 10.1016/j.mad.2020.111377
Lieberman, D. E., Kistner, T. M., Richard, D., Lee, I.-M., and Baggish, A. L. (2021). The active grandparent hypothesis: physical activity and the evolution of extended human healthspans and lifespans. PNAS 118:e2107621118. doi: 10.1073/pnas.2107621118
Mayor, M. I., Sommer, J. A., Houck, M. L., Zaonarivelo, J. R., Wright, P. C., Ingram, C., et al. (2004). Specific status of Propithecus spp. Int. J. Primatol. 25, 875–900. doi: 10.1023/B:IJOP.0000029127.31190.e9
Meyers, D. M., and Wright, P. C. (1993). “Resource tracking: food availability and Propithecus seasonal reproduction,” in Lemur Social Systems and Their Ecological Basis, eds P. M. Kappeler and J. U. Ganzhorn (New York, NY: Plenum Press), 179–192.
Monaghan, P., Charmantier, A., Nussey, D. H., and Ricklefs, R. E. (2008). The evolutionary ecology of senescence. Funct. Ecol. 22, 371–378.
Morris, W. F., Altmann, J., Brockman, D. K., Cords, M., Fedigan, L. M., Pusey, A. E., et al. (2011). Low demographic variability in wild primate populations: fitness impacts of variation, covariation, and serial correlation in vital rates. Am. Nat. 177, E14–E28. doi: 10.1086/657443
Nussey, D. H., Froy, H., Lemaitre, J.-F., Gaillard, J.-M., and Austad, S. N. (2013). Senescence in natural populations of animals: widespread evidence and its implications for bio-gerontology. Ageing Res. Rev. 12, 214–225. doi: 10.1016/j.arr.2012.07.004
Nussey, D. H., Wilson, A. J., Morris, A., Pemberton, J., Clutton-Brock, T., and Kruuk, L. E. B. (2008). Testing for genetic trade-offs between early- and late-life reproduction in a wild red deer population. Proc. Royal Soc. Lon. B 275, 745–750. doi: 10.1098/rspb.2007.0986
Packer, C., Tatar, M., and Collins, A. (1998). Reproductive cessation in female mammals. Nature 392, 807–811. doi: 10.1038/33910
Paul, A., Kuester, J., and Podzuweit, D. (1993). Reproductive senescence and terminal investment in female barbary macaques (Macaca sylvanus) at Salem. Int. J. Primatol. 14, 105–124. doi: 10.1007/BF02196506
Péron, G., Bonenfant, C., Lemaitre, J.-F., Ronget, V., Tidiere, M., and Gaillard, J.-M. (2019). Does grandparental care select for a longer lifespan in non-human mammals? Biol. J. Linn. Soc. 128, 360–372.
R Core Team (2020). R: A Language and Environment for Statistical Computing. Vienna: R Foundation for Statistical Computing.
Rasmussen, D. T. (1985). A comparative study of breeding seasonality and litter size in eleven taxa of captive lemurs (Lemur and Varecia). Int. J. Primatol. 6, 501–517. doi: 10.1007/BF02735573
Razafindramanana, J., Eppley, T. M., Rakotondrabe, R., Rakotoarisoa, A. A., Ravaloharimanitra, M., and King, T. (2020). Eulemur rufus. IUCN Red List e.T8209A115562696. Gland: IUCN. doi: 10.2305/IUCN.UK.2020-2.RLTS.T8209A115562696.en
Richard, A. F., Dewar, R. E., Schwartz, M., and Ratsirarson, J. (2000). Mass change, environmental variability and female fertility in wild Propithecus verreauxi. J. Hum. Evol. 39, 381–391. doi: 10.1006/jhev.2000.0427
Richard, A. F., Dewar, R. E., Schwartz, M., and Ratsirarson, J. (2002). Life in the slow lane? Demography and life histories of male and female sifaka (Propithecus verreauxi verreauxi). J. Zool. 256, 421–436. doi: 10.1017/S0952836902000468
Richard, A. F., Rakotomanga, P., and Schwartz, M. (1991). Demography of Propithecus verreauxi at Beza Mahafaly, Madagascar: sex ratio, survival and fertility, 1984-1988. Am. J. Phys. Anthropol. 84, 307–322. doi: 10.1002/ajpa.1330840307
Rina Evasoa, M., Radespiel, U., Hasiniaina, A. F., Rasoloharijaona, S., Randrianambinina, B., Rakotondravony, R., et al. (2018). Variation in reproduction of the smallest-bodied primate radiation, the mouse lemurs (Microcebus spp.): A synopsis. Am. J. Primatol. 80:e22874. doi: 10.1002/ajp.22874
Roff, D. A. (1992). The Evolution of Life Histories: Theory and Analyses. New York, NY: Chapman & Hall.
Ross, C. (1998). Primate life histories. Evol. Anthropol. 6, 54–63. doi: 10.1002/(SICI)1520-650519986:2<54:AID-EVAN3>3.0.CO;2-W
Schielzeth, H., and Forstmeier, W. (2009). Conclusions beyond support: overconfident estimates in mixed models. Behav. Ecol. 20, 416–420. doi: 10.1093/beheco/arn145
Smucny, D. A., Abbott, D. H., Mansfield, K. G., Schultz-Darken, N. J., Yamamoto, M. E., Alencar, A. I., et al. (2004). Reproductive output, maternal age and survivorship in captive common marmoset females (Callithrix jacchus). Am. J. Primatol. 64, 107–121. doi: 10.1002/ajp.20065
Sommer, V., and Rajpurohit, L. S. (1989). Male reproductive success in harem troops of Hanuman langurs (Presbytis entellus). Int. J. Primatol. 10, 293–317. doi: 10.1007/BF02737419
Sommer, V., Srivastava, A., and Borries, C. (1992). Cycles, sexuality, and conception in free-ranging langurs (Presbytjs entellus). Am. J. Primatol. 28, 1–27. doi: 10.1002/ajp.1350280102
Stearns, S. C. (1976). Life-history tactics: a review of the ideas. Q. Rev. Biol. 51, 3–47. doi: 10.1086/409052
Sussman, R. W., Richard, A. F., Ratsiarson, J., Sauther, M. L., Brockman, D. K., Gould, L., et al. (2012). “Beza Mahafaly Special Reserve: Long-Term Research on Lemurs in Southwestern Madagascar,” in Long-term field studies of primates, eds P. M. Kappeler and D. P. Watts (Berlin: Springer), 45–66. doi: 10.1007/978-3-642-22514-7_3
Tardif, S. D., Araujo, A., Arruda, M. F., French, J. A., Sousa, M. B. C., and Yamamoto, M. E. (2008). Reproduction and aging in marmosets and tamarins. Interdiscip. Top. Gerontol. 36, 29–48. doi: 10.1159/000137678
Tecot, S., Baden, A. L., Romine, N., and Kamilar, J. M. (2013). “Reproductive strategies and infant care in the Malagasy primates,” in Building Babies: Primate Development in Proximate and Ultimate Perspectives, eds K. B. H. Clancy, K. Hinde, and J. N. Rutherford (Berlin: Springer) 37, 321–359. doi: 10.1007/978-1-4614-4060-4_15
Thompson, M. E., Jones, J. H., Pusey, A. E., Brewer-Marsden, S., Goodall, J., Marsden, D., et al. (2007). Aging and fertility patterns in wild chimpanzees provide insights into the evolution of menopause. Curr. Biol. 17, 2150–2156. doi: 10.1016/j.cub.2007.11.033
Tidière, M., Gaillard, J.-M., Berger, V., Müller, D. W. H., Bingaman Lackey, L., Gimenez, O., et al. (2016). Comparative analyses of longevity and senescence reveal variable survival benefits of living in zoos across mammals. Sci. Rep. 6:3636. doi: 10.1038/srep36361
Tidière, M., Thevenot, X., Deligiannopoulou, A., Douay, G., Whipple, M., Siberchicot, A., et al. (2018). Maternal reproductive senescence shapes the fitness consequences of the parental age difference in ruffed lemurs. Proc. Biol. Sci. 285:20181479. doi: 10.1098/rspb.2018.1479
van de Pol, M., and Verhulst, S. (2006). Age-dependent traits: a new statistical model to separate within-and between-individual effects. Am. Nat. 167, 766–773. doi: 10.1086/503331
van Schaik, C. P., and Isler, K. (2012). “Life-history evolution in primates,” in The Evolution of Primate Societies, eds J. C. Mitani, J. Call, P. M. Kappeler, R. Palombit, and J. B. Silk (Chicago, IL: Chicago University Press), 220–244. doi: 10.5167/uzh-71056
Walker, M. L., and Herndon, J. G. (2008). Menopause in nonhuman primates? Minireview 79, 398–406. doi: 10.1095/biolreprod.108.068536
Ward, E. J., Parsons, K., Holmes, E. E., Balcomb, K. C., and Ford, J. K. B. (2009). The role of menopause and reproductive senescence in a long-lived social mammal. Front. Zool. 6:4. doi: 10.1186/1742-9994-6-4
Wright, P., King, S. J., Baden, A., and Jernvall, J. (2008). Aging in wild female lemurs: sustained fertility with increased infant mortality. Interdiscip. Top. Gerontol. 36, 17–28. doi: 10.1159/000137677
Wright, P. C., Erhart, E. M., Tecot, S., Baden, A. L., Arrigo-Nelson, S. J., Herrera, J., et al. (2012). “Long-Term Lemur Research at Centre Valbio, Ranomafana National Park, Madagascar,” in Long-Term Field Studies of Primates, eds P. M. Kappeler and D. P. Watts (Berlin: Springer), 67–100. doi: 10.1007/978-3-642-22514-7_4
Keywords: reproduction, senescence, interbirth interval, captivity, menopause, lemurs
Citation: Kappeler PM, Pethig L, Prox L and Fichtel C (2022) Reproductive Senescence in Two Lemur Lineages. Front. Ecol. Evol. 10:894344. doi: 10.3389/fevo.2022.894344
Received: 11 March 2022; Accepted: 01 June 2022;
Published: 30 June 2022.
Edited by:
Jean-Francois Lemaitre, UMR 5558 Biométrie et Biologie Evolutive (LBBE), FranceReviewed by:
Morgane Tidière, University of Southern Denmark Odense, DenmarkCopyright © 2022 Kappeler, Pethig, Prox and Fichtel. This is an open-access article distributed under the terms of the Creative Commons Attribution License (CC BY). The use, distribution or reproduction in other forums is permitted, provided the original author(s) and the copyright owner(s) are credited and that the original publication in this journal is cited, in accordance with accepted academic practice. No use, distribution or reproduction is permitted which does not comply with these terms.
*Correspondence: Peter M. Kappeler, cGthcHBlbEBnd2RnLmRl
Disclaimer: All claims expressed in this article are solely those of the authors and do not necessarily represent those of their affiliated organizations, or those of the publisher, the editors and the reviewers. Any product that may be evaluated in this article or claim that may be made by its manufacturer is not guaranteed or endorsed by the publisher.
Research integrity at Frontiers
Learn more about the work of our research integrity team to safeguard the quality of each article we publish.