- 1Laboratoire des Systèmes Perceptifs (LSP), CNRS, Ecole Normale Supérieure, Université Paris Sciences & Lettres, Paris, France
- 2Institut de Systématique, Évolution, Biodiversité (ISYEB), Muséum National d’Histoire Naturelle, CNRS, Sorbonne Université, Ecole Pratique des Hautes Etudes, Université des Antilles, Paris, France
The concept of soundscape was originally coined to study the relationship between humans and their sonic environment. Since then, several definitions of soundscapes have been proposed based on musical, acoustical and ecological perspectives. However, the causal mechanisms that underlie soundscapes have often been overlooked. As a consequence, the term “soundscape” is frequently used in an ambiguous way, alternatively pointing to objective realities or subjective percepts. Through an interdisciplinary review, we identified the main biotic and abiotic factors that condition non-anthropogenic terrestrial soundscapes. A source-filter approach was used to describe sound sources, sound propagation phenomena and receiver’s characteristics. Interdisciplinary information was cross-referenced in order to define relationships between factors, sound sources and filters. Those relationships and the associated references were organized into a functional block diagram. This representation was used to question the different uses and meanings of the soundscape concept found in the literature. Three separate categories were then suggested: distal soundscape, proximal soundscape and perceptual soundscape. Finally, practical examples of these different categories were described, in relation to the diagram. This new systemic approach to soundscapes should help ecoacousticians, bioacousticians, psychoacousticians and environmental managers to better understand soundscapes and protect natural areas in a more significant way.
Introduction
The concept of soundscape, which has been widely used in different scientific contexts during the last decades (Kang and Aletta, 2018), was originally introduced in by Southworth (1969) who was studying the perception of urban acoustic environment. Southworth first defined the soundscape as “the quality and type of sounds and their arrangements in space and time.” Schafer later popularized the term (Schafer, 1977). Through the study of the history of human soundscapes, Schafer exposed the rising emergence of noise pollution as a potential threat to human health and culture. Although Schafer did not have any scientific evidence at the time, he feared that the growth of what he called “low-fidelity soundscapes” at the expense of “high-fidelity soundscapes” would alter man’s relationship with nature and decrease his concern for ecosystem well-being. Later, with the emergence of soundscape ecology (e.g., Pijanowski et al., 2011) and more recently ecoacoustics (e.g., Sueur and Farina, 2015), the concept of soundscape evolved to designate an acoustic space that could be studied within the frame of ecology. Today, many studies show that the concerns of Schafer were justified and that soundscapes play a significant role in our understanding of natural environments, as well as our own well-being (Ratcliffe, 2021).
However, the definition of soundscape still appears as vague and ambiguous. Pijanowski et al. (2011) defined the soundscape as “the collection of biological, geophysical and anthropogenic sounds that emanate from a landscape and which vary over space and time reflecting important ecosystem processes and human activities.” Although this definition appears to be consensual and shared, at least in 2011, by Pijanowksy’s co-authors, the soundscape concept is actually associated with a wide variety of objects. As Farina and Pieretti (2012) noted, “The landscape can be defined in several ways according to the epistemological basis adopted and the discipline.”
In his seminal book Soundscape Ecology (2014), Farina proposed several definitions of the soundscape, two of them being: “an acoustical composition that results from the voluntary or involuntary overlap of different sounds of physical or biological origin” and “the acoustic context produced and, in turn, perceived in different ways by both animals and humans.” The first definition is parsimonious with that of Pijanowski, but the second explicitly relates the soundscape to perception of the acoustic environment. Farina will later make the distinction between the vibroscape, which represents all the vibrations present in an area, and the soundscape, that he finally defined as “the part of the vibroscape perceived as sound by an organism” (Farina et al., 2021). However, contemporary literature relates the vibroscape to substrate-borne sounds only (Šturm et al., 2022). Still, Farina’s last definition of soundscape suggests that animals play an active role in building the soundscape as an intellectual construct, which matches the definition from the International Standardisation Organisation (ISO, 2014) where the soundscape results from the listener’s understanding of an acoustic environment. Moreover, incorporating auditory perception aspects into the soundscape puts an emphasis on cognitive constraints that were not included in Pijanowski’s definition.
Barchiesi et al. (2015) presented the soundscape as an equivalent of the “acoustic scene”, designating the sound produced by the environment. In the context of acoustic scene classification, the acoustic scene pulls away from Pijanowski’s holistic soundscape and rather describes the sounds that arrive to an observer. Celis-Murillo et al. (2009) went further and suggested that a recording contained all the information that was embedded in a soundscape, and that a 360° display was a faithful replication of this soundscape. The idea that the soundscape and its recording are one and the same is common in soundscape composition. According to Westerkamp (2002), a soundscape may be understood as the result of the juxtaposition of environmental sound recordings that provide an “artistic, sonic transmission of meanings about place, time, environment and listening perception.” In soundscape composition, the ecological origin of sounds that is emphasized in Pijanowski’s definition has been replaced by the “meaning” that people attribute to the sounds. In Payne et al. (2009), merged both objective and subjective aspects of soundscapes into their own definition: “Soundscapes are the totality of all sounds within a location with an emphasis in the relationship between individual’s or society’s perception of, understanding of and interaction with the sonic environment.” However, this definition does not state whether the object of study is a physical (thus, external) phenomenon, or a perceptual (thus, internal) understanding of complex acoustic assemblages.
As Farina et al. rightfully said, “ecoacoustic research to date has focused predominantly on the development of tools for environmental monitoring, rather than theoretical and conceptual development and explication” (Farina et al., 2021). Some clarification could be obtained by identifying the causes and effects behind the soundscape concept. The description of the ecological factors that influence the production of sound sources and alter their acoustic qualities should help to better understand the dynamic relationship between sound sources, sound propagation, and sound perception. The causes and effects could be organized according to the principles of information theory, in which communication is the result of a source that generates a signal which passes through a transmission channel and conveys information to the receiver (Shannon and Weaver, 1949; see Reza, 1994). This approach allowed speech production and later animal communication research to tackle animal vocal communication through the source-filter theory. The source-filter theory decomposes vocal sound production into a larynx (the source) and a supralaryngeal vocal tract (the filter) (Lindblom et al., 2010; Taylor et al., 2016). Following these principles and taking a bioacoustic perspective, animal vocal production can be considered as a source signal that is filtered a first time by the acoustic particularities of the environment, and a second time by the auditory system of the receiver. In ecoacoustics, biophony and geophony may be considered as a collection of sound sources, and sound propagation and auditory perception as two different kinds of acoustic filters operating one after the other.
Here we aim at clarifying the terrestrial soundscape concept by listing and drawing the interactions between the causes and effects that explain non-anthropogenic soundscapes so that original ecological interactions, without human pressures, can be underlined. Soundscapes, as ecological phenomenons, were born and structured in non-anthropogenic environments. Although it is appropriate to say that anthropophony today represents a prevalent part of soundscapes around the world, including natural protected areas (Barber et al., 2011; Buxton et al., 2017), anthropophony is not indispensable to the clarification of the soundscape concept. Consequently, a source-filter approach was used, combined with an interdisciplinary review, in order to describe cause and effect relationships regarding biophony and geophony only. A systematic functional block diagram was then built in order to clarify factors, sources and filters. This description helped to unravel the soundscape conundrum. The resulting causal cartography offered a tool to deliberate on which meaningful concepts were hidden behind the soundscape polysemy. All of this led to the definition of three distinct soundscape categories: the distal soundscape, the proximal soundscape and the perceptual soundscape.
Methods
Studying Terrestrial Non-anthropogenic Acoustic Environments
Only non-anthropogenic terrestrial environments were considered in this study. Often referred to as “natural” or “pristine,” these environments do not contain any trace of human activity, that is any trace of anthropogenic sounds, also called “anthropophony.” It is acknowledged that this selection is a double simplification. However, terrestrial and marine soundscapes have been studied independently since the origin of soundscape ecology and ecoacoustics because air and water have different acoustic properties. In addition, excluding the anthropophony opens the possibility to focus on primary ecological processes that have occurred before the development of modern industries and the consequent rise of anthropophony.
Source-Filter Approach and Categories
In a source-filter approach, source signals (input) go through a filtering process, giving rise to a final signal (output). The properties of sources and filters depend on external or environmental factors which can affect the output signal properties (Figure 1). Soundscape components were therefore classified into environmental factors, sound sources and acoustic filters. Environmental factors were themselves divided into five categories: temporal factors, spatial factors, abiotic factors, biotic factors and acoustic factors. Sound sources were the primary sonic objects before considering any environmental alteration. Biotic sound sources were grouped into biophony and abiotic sound sources were grouped into geophony. Acoustic filters were separated into sound propagation filters, which depend on environmental conditions, and receiver filters, which depend on the receiver’s characteristics (i.e., location, structure) and acoustic sensitivity (i.e., auditory capacities).
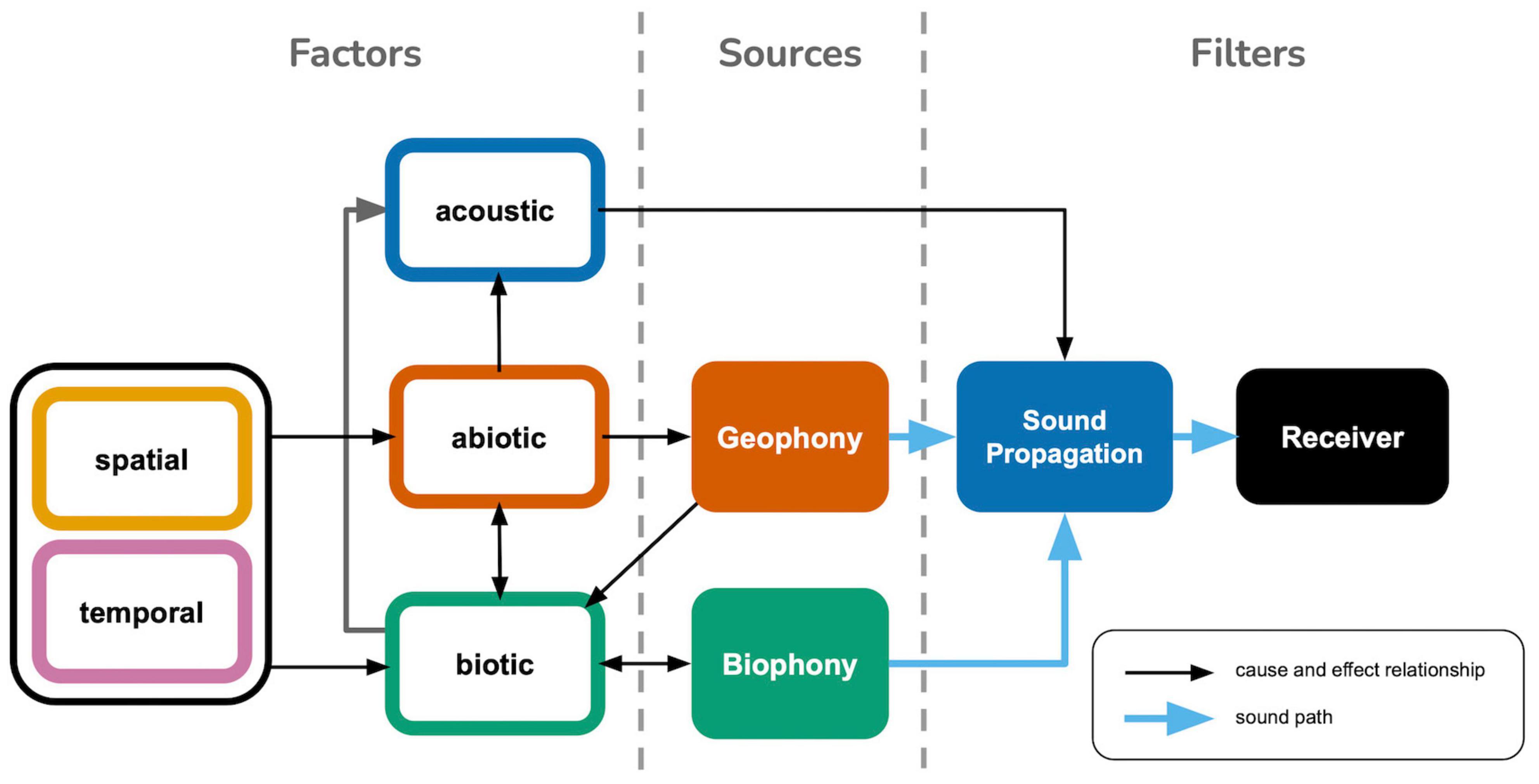
Figure 1. Simplified soundscape functional block diagram. Environmental factors, sound sources and acoustic filters are represented through a source-filter approach. The influence of geophony and biophony on biotic factors introduce feedback loops that partially explain the complexity of soundscape dynamics.
Interdisciplinary Literature Review
Environmental factors, sound sources and acoustic filters were listed and their relationships stated by conducting an interdisciplinary literature review on non-anthropogenic terrestrial soundscapes. The review covered animal behavior, animal physiology, community ecology, landscape ecology, meteorology, climatology, environmental acoustics, soundscape ecology, ecoacoustics and psychoacoustics. Because birds were overrepresented in papers dealing with biophony (Shannon et al., 2016), we cannot rule out a possible bias toward this taxonomic group when identifying the cause and effect mechanisms. Still, it is important to note that birds are, with insects, the main contributors to non-anthropogenic terrestrial soundscapes compared to amphibians and mammals (e.g., Phillips et al., 2018).
Semantics
As the literature review was interdisciplinary, several concepts were named differently according to the disciplines. Terms were therefore chosen by applying the following criteria in order of priority: (1) the term that was the least ambiguous, (2) the term that was the most shared by the scientific community, and (3) the term that would be the most understandable by the ecoacoustic community.
Functional Block Diagram
Functional block diagrams (FBD) are logic models that represent each object as a block, linked to one or more blocks by an arrow or different connectors (Papazoglou, 1998). FBDs, which are used in systems engineering, ecology modeling and risk management, help to visualize the relationships between objects, as well as specifying the nature of these relationships. Since each block represents a potential cause or effect, the construction of FBDs helps to consider any important causal links or objects in the literature. Here, each environmental factor, sound source and acoustic filter was represented as a block, which was connected to other blocks with directional arrows to symbolize cause and effect relationships. Using a color-blind safe color palette named “Okabe-Ito”, we colored the boxes according to their categories. The source-filter approach consisted in a linear approach that was translated into a linear diagram to be read from left to right.
Environmental Factors
Temporal Factors
Temporal factors take into account time changes at different scales. Animal and geophysical sounds produce acoustic variations at time scales ranging from milliseconds to minutes. Day hour has direct and indirect influences on animal behavior, known as diel activity (Balakrishnan, 2016; Phillips et al., 2018; Gil and Llusia, 2020). Lunar cycle is also known to regulate animal behavior, in particular for acoustic communication (Grant et al., 2013; York et al., 2014). Seasons through weather variations regulate yearly animal activity, known as phenology (Suthers et al., 2016; Phillips et al., 2018), and affect the composition of local species assemblages.
Spatial Factors
Spatial factors correspond to abiotic factors that are specifically linked to the geographical location of the soundscape, that is its geospatial coordinates. Topography and ground surface can impact sound propagation with obstacles and elevation inducing ground effects and sound scattering (cf. section “Acoustic Factors”).
Abiotic Factors
Climate regulates animal and vegetal biotic factors. Climate mostly depends on the studied area geographical location and the season.
Weather produces geophonic sound sources, impacts vegetation and alters animal behavior and distribution (Birch, 1957; Thuiller et al., 2008; Elkins, 2010). Rain also alters hydrologic landscape sounds such as rivers. Wind can produce a salient acoustic meteorological effect that generates amplitude fluctuations when it occurs in open areas such as meadows (cf. section “Acoustic Factors” and “Sound Propagation”).
Climate and weather, along with vegetation (cf. section “Biotic Factors”), influence the local microclimate, which can be described by the temperature, humidity and sun irradiance of a given area. Local microclimates influence animal behavior (Gil and Gahr, 2002), as well as acoustic meteorological effects such as atmospheric absorption (cf. section “Acoustic Factors”).
Biotic Factors
Biotic factors cover a large range of phenomena from physiological characteristics to ecological relationships. Here, we curated a list of biotic factors that have been frequently cited in the literature regarding biophony production and/or sound propagation. The first three factors (vegetation, acoustic community, and acoustic behavior) describe species and their intrinsic traits, whereas the last three factors (population density, territory distribution and trophic interactions) account for the complexity of intraspecific and interspecific dynamics.
Vegetation, either herbaceous or woody, affects the sound propagation. The thickness, geometry and porosity of plant components (stems, trunks, leaves) impact sound propagation through acoustic scattering and ground effects (cf. section “Acoustic Factors”). Vegetation is also a core determinant of the local microclimate, especially in closed habitats. The presence of vegetation near an open habitat can have an influence on wind currents and create specific sound speed profiles (Forrest, 1994).
In ecology, a community is an assemblage of species found in a given area and sharing the same resource. In soundscape ecology and ecoacoustics, an acoustic community is an assemblage of species sharing the same acoustic space (Gasc et al., 2015; Farina and James, 2016). Species assemblages vary geographically and can evolve through time depending on the season, environmental change and migration (Morin, 2009). Acoustic communities are the main elements of the biophony. Species assemblages are therefore crucial to obtain a good knowledge of local species dynamics.
Acoustic behavior is a behavior expressed by an individual emitting a sound. In terrestrial habitats, most animals produce intentional sounds for intraspecific communication. The information encoded in these signals includes courtship, territory defense, alarm, distress, kin contact, and parent-offspring interactions (Bradbury and Vehrencamp, 2011). Incidental sounds are mainly due to locomotion including walk and flight during, among others, habitat exploration, foraging, and prey escape. However, incidental sounds can appear as intentional and the line between the two can be blurry (Clark, 2016).
Population density is the number of acoustically active individuals in a given area and represents the abundance of sounds produced locally (Dawson and Efford, 2009; Thomas and Marques, 2012). On an ecological level, population density depends on population dynamics which are affected by trophic interactions and the species intrinsic rate of increase (Hanski and Gilpin, 1991).
Territory distribution is the position in space of any animal which can participate in biophony in a given area. Whereas the location of abiotic factors can be identified from topographical sources, the position of animals varies greatly due to individual movements in relation with the defense of their territory and with the exploration and exploitation of their home range (Birch, 1957). The position and trajectory of each biophonic animal is necessary to assess the spatial dynamics of the soundscape.
Trophic interactions are the core of inter-specific relationships in a given ecosystem. Trophic interactions are also influenced by abiotic factors (Rosenblatt and Schmitz, 2014). Fundamental trophic interactions such as prey-predator and plant-animal interactions considerably influence animal behavior and incidentally affect biotic sound sources through acoustic behavior (Siemers and Schaub, 2011; Medina and Francis, 2012).
Acoustic Factors
Ground effects describe the reflection of sound waves on the ground, which changes the distance that the sound wave can travel. This phenomenon produces destructive (attenuation) or constructive (amplification) interferences depending on the phasing of the resulting sound waves. Ground effects can therefore have a significant impact on sound propagation, especially at low frequencies (Embleton, 1996; Swearingen and White, 2007; Tarrero et al., 2008). The composition of the different layers determines the ground impedance which is responsible for the reflection. The magnitude of ground effects also depends on the sound source distance to the ground (Ellinger and Hödl, 2003).
Sound scattering occurs when sound wavelength is smaller than the dimension of surrounding objects such as tree trunks and foliage. Sound scattering consists of absorption, refraction and reverberation (reflection). Scattering impacts more high frequencies than low frequencies. Sound scattering depends on forest characteristics including tree density, foliage density, leaf shape, and rock configuration (Swearingen and White, 2007; Tarrero et al., 2008) and is more significant in closed habitats than in open habitats.
Meteorological effects regroups all abiotic and biotic phenomenons that impact sound propagation due to climate and weather. Humidity can facilitate atmospheric absorption. Ambient temperature which is linked to the canopy structure and solar irradiance changes sound speed (Swearingen and White, 2007). The combination of temperature fluctuations and wind currents can cause atmospheric turbulence that results in irregular amplitude fluctuations (cf. section “Sound Propagation”) (Embleton, 1996; Larom et al., 1997). Meteorological effects are more prominent in open habitats and for long distance communication.
Sound Sources
Geophony
Geophony is produced by abiotic sources (Figure 2). Here, we divide geophonic sounds into two main categories: weather sounds, like rain and wind, and hydrologic sounds, like waterfalls and rivers. Such sounds are dominated by relatively broadband and transient sounds (Lewicki, 2002; Theunissen and Elie, 2014). Other geophonic sound sources that have a low rate of occurrence and have been less studied, such as thunder, forest fire or seismic activity were not considered here. Still, it is important to state that such geophony can, during a certain timeframe, have a pervasive impact on soundscapes.
Weather sounds depend on meteorological variables, but also on biotic factors. The occurrence and power of weather sounds, like the force of the wind or the intensity of rain, are linked to climatic and meteorological factors, whereas the textural quality of weather sounds depends on the physical elements of the landscape with which weather phenomena interact. For example, the interaction between wind force (linked to abiotic factors) and tree foliage (linked to biotic factors) design wind sound. Similarly, leaf shape and soil texture change rain sound. During episodes of storms, high wind or heavy rain can generate a significant broadband noise that can alter animal behavior at various levels (Lengagne and Slater, 2002; Brumm, 2004; Tishechkin, 2013; Farji-Brener et al., 2018; Geipel et al., 2019).
Hydrologic sounds are produced by endemic moving bodies of water such as rivers or waterfalls. Unlike weather sounds, hydrologic landscape sounds are pervasive, although their presence and quality can depend on climatic and meteorological factors. A small stream can disappear during the dry season, whereas a river can become a prevalent sound source during a rainy day. The noise produced by rivers can have an impact on species territory distribution (Gomes et al., 2021).
Biophony
Biophony is produced by biotic sources, either intentionally or incidentally. Animals are the main sources of biophony. Each biophonic sound results from the species-specific behavior of an individual positioned in the landscape. Biophony encompasses a large variety of sounds that are themselves produced by a large variety of sound production systems (e.g., vocalization, stridulation, percussion): biophonic sounds range from periodic (as in the case of pure tones) to almost noisy sounds, may be stationary or fluctuating and range from narrowband to broadband sounds (Hauser, 1996; Tembrock, 1996; Bradbury and Vehrencamp, 2011).
Recent studies in sensory neuroscience that aimed to assess the acoustic statistics of natural scenes and isolated biological sounds suggest that most recorded animal vocalizations, that is to say animal sounds emanating from a vocal apparatus with vocal chords, are dominated by relatively slow amplitude modulations (below ∼10 Hz) with fine harmonic structure (Nelken et al., 1999; Lewicki, 2002; Theunissen and Elie, 2014). However, other frequent events such as stridulations have rather sudden onsets, often with fast fluctuations, and these studies still need to be extended to larger and more diverse sound databases. Biophony involves numerous types of biotic and abiotic factors, different modalities of these factors for each species, and complex internal dynamics such as prey-predator interactions with feedback loops (Figure 3).
Ambient Sounds
Ambient sounds, usually referred to as “background sounds,” “background noise,” “ambient noise” or “silence,” are the result of the combination of two types of sounds: external ambient sounds and internal ambient sounds. As their common appellation suggests, ambient sounds are often considered as background sounds, meaning that they are mostly understood as inherently undesirable sounds. Most of today’s terrestrial ecoacoustic literature intends to remove ambient sounds instead of studying them for their intrinsic qualities. But ambient sounds are not only a significant component of soundscapes, they also constitute the main, if not only, source of sound during periods of reduced biotic and abiotic activity such as nights or winters (e.g., Grinfeder et al., 2022).
External ambient sounds, also called “environmental noise,” consist in a mixture of biophonic and geophonic signals that are too attenuated and/or distorted to be separated and identified (Forrest, 1994). External ambient sounds are usually described as showing most energy below about 2 kHz, but it remains unknown to which extent biophony and geophony, respectively, influence the acoustic nature of external ambient sounds. However, one can make the assumption that biophonic and geophonic ambient sounds should occur on different parts of the amplitude spectrum, follow different periodicities, and overall possess distinguishable features.
Internal ambient sounds are sounds that are produced by the receiver’s body and can only be perceived by it. For animals, internal ambient sounds can have neural, vascular or pulmonary origins. For artificial recorders, internal ambient sounds are mechanical or electronic sounds that result from the recorder’s physical configuration and operation.
Acoustic Filters
Sound Propagation
During sound propagation, the acoustic characteristics of the environment filter the signal and produce attenuation and distortion (Figure 4). This filter can be characterized by a transfer function which captures the shape of a known signal after its transmission through the habitat. Signal attenuation and distortion may reduce the amount of information encoded or limit the transmission of the information over a specific distance, usually known as the active space.
Attenuation is the decrease of intensity of a sound traveling through a medium. Attenuation is mainly due to spreading loss and atmospheric absorption (cf. section “Acoustic Factors”). Sound attenuation is frequency dependent, with a greater effect on high frequencies (Wiley and Richards, 1978; Forrest, 1994). Moreover, ground effects can generate shadow zones that drastically attenuate sounds in areas that can be close to the source (Roberts et al., 1981).
Distortion mainly results from sound scattering and meteorological effects (cf. section “Acoustic Factors”). Time, amplitude and frequency alteration of sounds can occur such that temporal smearing or amplification can be observed after transmission. Temporal smearing, mainly due to reverberation, may mask high rate amplitude modulation. Irregular amplitude fluctuations, due to atmospheric turbulence, may mask low rate amplitude modulation (Richards and Wiley, 1980).
Receiver
A receiver is a system which operates a transduction of acoustic energy into mechanical or electrical energy. The receiver acts as a filter which can be defined with a transfer function. A receiver can be an animal, including a human, or a machine, in particular a microphone. The characteristics of the receiver include observational condition and transduction. Here, we consider the receiver as a passive observer of the soundscape. Consequently, the receiver does not retroactively act on factors, nor does it influence the sound sources.
Observation conditions consist of the position, orientation, structure (e.g., head, neck and torso for humans) and movement of the receiver’s body.
Transduction is constrained by the amplitude dynamic range, integration time and frequency response of the transducer. Each species and each individual may have specific transduction properties.
Building Operational Definitions
Operational definitions are warranted to specify the scientific value and usefulness of the soundscape concept. Such definitions should allow to formulate qualitative and quantitative hypotheses and predictions that could guide scientific investigations through experimental designs (Popper, 1959). As indicated below, we adopted a source-receiver approach and distinguished between different categories of soundscape events according to the configuration and nature of the potential receivers and their relationship to sound sources. Each category represents a different kind of possible semantic relationship to the soundscape and the three categories should be considered as complementary rather than contradictory (Figure 5).
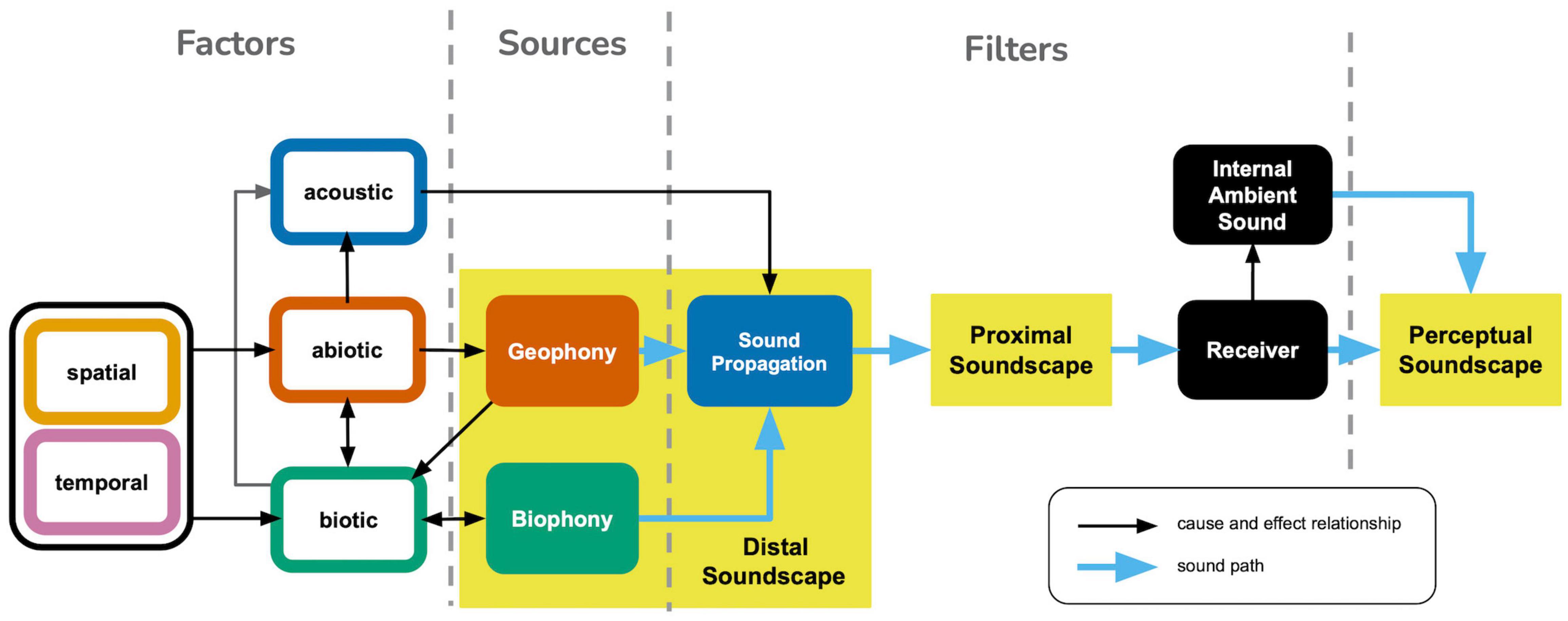
Figure 5. General soundscape functional block diagram. The distal soundscape is closer to environmental factors and their influence on sound production and propagation. The proximal soundscape is located just before the receiver and represents an ideal point of observation which includes external ambient sounds. The perceptual soundscape is the soundscape representation that the receiver progressively builds through its psychoacoustic apparatus. Compared to Figure 1, the “internal ambient sound” block was added in order to clarify the perceptual soundscape category.
Three Categories of Events
During the construction of the FBD, the question of the specific placement of “soundscape” arose several times. The soundscape was first placed after the receiver, implying that the soundscape was the result of the recording or perception of filtered sound signals. However, this choice seemed unsatisfying because of its inconsistency with other soundscape definitions such as Pijanoswky’s one. Three potential locations for the soundscape concept were identified on the diagram: (1) in the area of biophony, geophony and sound propagation blocks, (2) before the receiver block, and (3) after the receiver block. The first location corresponds to Pijanowski’s definition and consists of an “external” but purely theoretical event. The second location is similar to Barchiesi’s definition, consists of an “external” event that is not theoretical and represents the sonic information that is transformed by the environment but not yet recorded by a microphone or perceived by an observer. The third location corresponds to Farina’s definition where the soundscape is more subjective and consists of an “internal” event that can be attributed to a perceptual representation.
Farina et al. (2021) were the first to differentiate soundscape categories that they called “soundscape epithets.” The latent soundscape is “a portion of vibroscape that is not perceived by a particular individual as sound but that can be heard by others.” This concept (or any equivalent) does not seem to be included in ecoacoustic research yet. The sensed soundscape is “the portion of the acoustic information that a particular organism is sensitive to but are not necessarily integrated into a physiological or behavioral reaction.” Finally, the interpreted soundscape is “the subset of soundscape that makes a difference to the organisms.” This distinction between sensed and interpreted soundscape matches, to some extent, the hierarchy made by psychophysicists between low-level (i.e., sensory) and high-level (i.e., cognitive) processing stages. However, these soundscape epithets do not integrate the external-oriented uses of the soundscape concept. This led us to believe that there was a need to introduce a different operational categorization of the soundscape concept, aimed at discriminating the different usages present in the literature.
In the second half of the 20th century, psychologists and philosophers made the distinction between distal, proximal and perceptual events. A distal event is an event as it is produced at the source, far from the observer. A proximal event is an event as it arrives at the receiver and after it has been altered by its propagation from the distal location. Finally, a perceptual event is an event as it has been processed by the observer to link successive proximal events into a singular interpretation (Cooper, 1992). We followed this three-fold partition to divide the soundscape concept into three separate categories. This opened the possibility to draw a parallel between previous soundscape definitions and a new nomenclature of soundscape into distal soundscape, proximal soundscape and perceptual soundscape.
The Distal Soundscape
Landscape ecology, which can be considered as a parent of soundscape ecology, studies ecological invariant patterns of interest that emerge from a collection of singular events (McGarigal and Urban, 2001). Since a landscape event cannot be assimilated to a singular signal, it would be more accurate in this context to consider a soundscape event as a collection of sound signals in a prespecified area. This spatial and temporal distribution of sound signals is theoretical because no observer can receive at the same time the total acoustic information that occurs in a given area. It is the collection and identification of invariant spatial and temporal patterns, such as the bird dawn chorus, that gives external clues about the soundscape dynamics. Consequently, when we consider the soundscape as the acoustic equivalent of the landscape, we consider the distal soundscape.
This definition can still be seen as vague because the acoustic scale of the sound signals has not been defined. Should the sound of a worm moving in the soil be considered when studying the distal soundscape at the scale of vertebrates? Or more generally, what is the time period used to study the distal soundscape? The patterns that soundscape ecologists observe only occur at a specific time, frequency and amplitude range, which is often implied but rarely stated. These ranges define the acoustic scale of the distal soundscape and complete the spatial scale of the defined area. However, the acoustic scale is altered by acoustic factors which will alter the accessibility of sound information (cf. section “Sound Propagation”). In contrast with conventional distal events, the distal soundscape should therefore encompass sound propagation in order to correctly reflect its complexity. The distal soundscape is therefore defined as the spatial and temporal distribution of sounds in a prespecified area, in relation to sound propagation effects. When described, a distal soundscape should be associated with a specific time period and a specific acoustic range. This soundscape category can be represented by an acoustic cartography or a thorough description of the sound patterns that occur in a specific area.
The Proximal Soundscape
In visual psychophysics, the “ambient optic array” represents a visual point of observation (Gibson, 2014). Whereas the perception of the ambient optic array (the “visual scene”) should change from one observer to another, the ambient optic array remains consistent and represents all the potential information that can be retrieved by any observer at any point in time. Barchiesi et al. (2015) suggested that the acoustic scene could be thought of as an acoustic equivalent of the ambient optic array, but this would be a matter of interpretation. In order to clarify this, we suggest using the notion of proximal soundscape in this context. Where the distal soundscape requires the survey of all the potential effects of sound propagation that can occur on a given area, the proximal soundscape is the effective filtering of these sound signals at one point in space. Although there is only one distal soundscape for a given area, there is a multiplicity of proximal soundscapes occurring in the same area, corresponding to every potential receiver position. The proximal soundscape is therefore defined as the collection of propagated sound signals that occurs at a specific point in space. This soundscape category can be represented by an “ideal” recording with a limitless acoustic scale (cf. section “The Distal Soundscape”) and no internal ambient sound (cf. section “Ambient Sounds”).
The Perceptual Soundscape
A perceptual event consists of acquiring proximal events through time and/or space, and linking them into a dynamic “internal” representation. Consequently, a perceptual event is a subjective representation built by the observer, suited for a given task, in order to make sense of the acquired information. For any living observer, the analysis of a proximal soundscape involves multiple sensory and cognitive processes operating in a sequential and/or parallel fashion. These processes (or computations) take time. Some are automatic and fast, and others are more controlled and slow (see Neuhoff, 2004). Over the past century, research in auditory psychophysics, neuroscience and cognitive psychology has shown that the auditory processing of complex acoustic mixtures such as proximal soundscapes requires – among other things – the segregation of these scenes into “streams” or “auditory objects” on the basis of simultaneous and sequential grouping mechanisms (e.g., Bregman, 1990; Moore and Gockel, 2012; Młynarski and McDermott, 2019), and the computation of acoustic attributes such as pitch, loudness, timbre and dynamic patterns (e.g., Moore, 2012; Thoret et al., 2020). Auditory processing of proximal soundscapes also involves bottom-up attentional processes that enhance the sensory representation (the “salience”) of certain acoustic events (these events “pop out”; Kayser et al., 2005; Huang and Elhilali, 2017; Filipan et al., 2019) as well as memory and decision processes. Recent work in brain imaging (Irwin et al., 2011) reveals the existence of two distinct neural processing pathways recruited by soundscapes: (i) an auditory bottom-up analysis pathway (from the auditory periphery to the cortical centers) and (ii) an emotional processing pathway involving two central structures well known in emotional response. The observer eventually constructs a more elaborate “cognitive representation” that results from deeper (e.g., semantic) processing. This cognitive representation may finally be stored in episodic and semantic autobiographical memory (e.g., Tekcan et al., 2015).
Since there is a multiplicity of proximal soundscapes, there is an infinite number of perceptual soundscapes, depending on the receiver’s nature, observation conditions and processing stages. The perceptual soundscape is therefore defined as the individual subjective interpretation of a proximal soundscape. This soundscape category can be represented in many ways depending on the processing stage that is considered.
Applications and Implications
In this section, we propose applications of the distal, proximal and perceptual soundscape concepts. Each application refers to the global functional diagram (Figure 6).
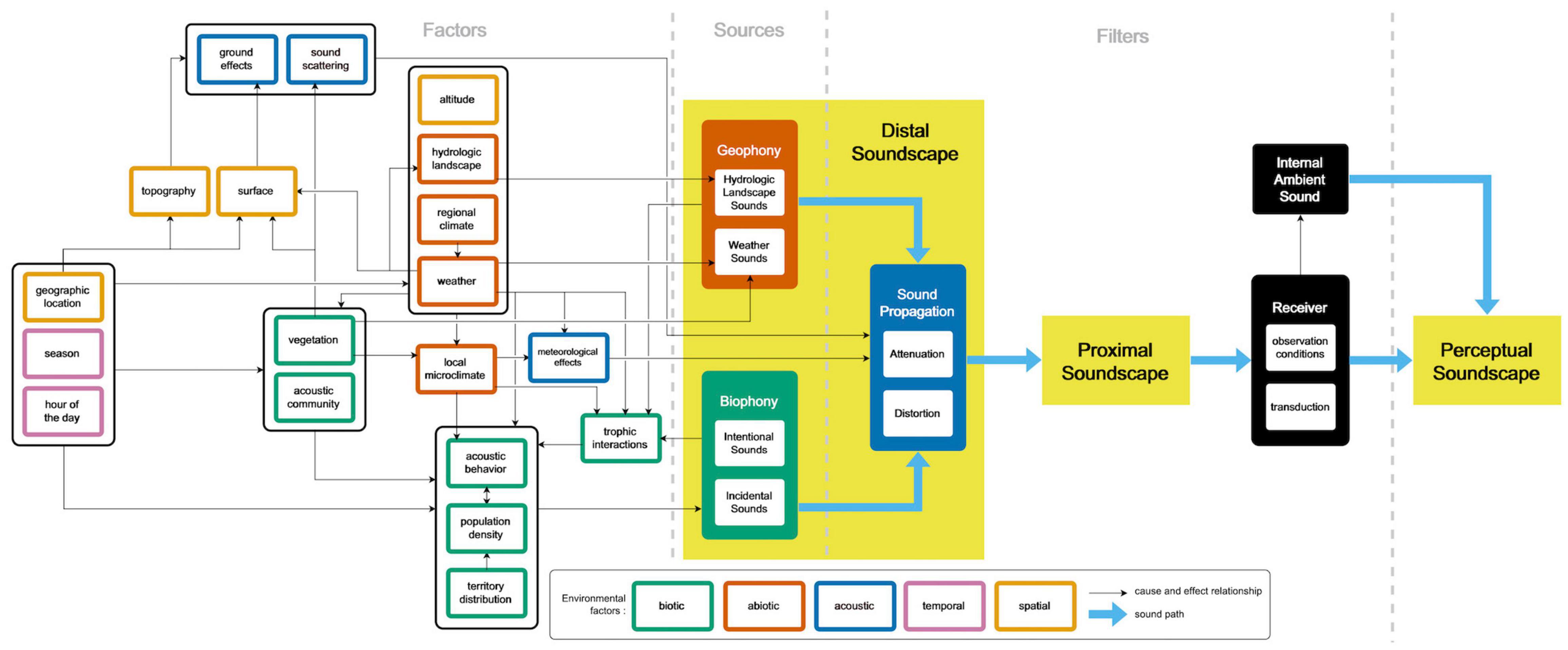
Figure 6. Detailed soundscape functional block diagram. The complex entanglement of the different types of environmental factors reveals the underlying complexity of soundscapes. This diagram can be used as a graphical display of current soundscape related knowledge, or as a tool to evaluate the potential impact of environmental change on non-anthropogenic terrestrial soundscapes.
Soundscape Recordings
Soundscape recordings are the fundamental material of soundscape ecology and ecoacoustics. Microphones receive a proximal soundscape that is then transformed by an analog to digital converter into a digital audio file. The digital signal is afterward converted into a given numerical representation thanks to specific mathematical operations. This can lead to different visual representations including waveforms, amplitude spectra, or spectrograms.
Due to the holistic dimension of distal soundscapes, it is not possible to consider a unique point of recording as an accurate reproduction of a distal soundscape. In other words, an infinity of soundscape recordings is theoretically required to properly assess a distal soundscape. Consequently, the use of the expression “soundscape recording” in this context can be inappropriate. However, it is reasonable to assume that a limited set of recordings provide a partial approximation of the distal soundscape and can be defined as a “distal soundscape recording.” Despite the fact that such apparatus could not encompass all the sounds that occur in a given area, the identification of sound patterns across recordings can give general but useful clues about spatio-temporal sound dynamics (e.g., Rodriguez et al., 2014).
For proximal soundscapes, soundscape recordings can be considered as an approximation of the absolute sonic object that proximal soundscapes represent. Since soundscape recordings are limited by their acoustic scale (cf. section “The Distal Soundscape”), it is important to note that soundscape recordings give an incomplete representation of the information available at a given point of observation. Soundscape recordings are often limited to the audible frequency range of humans, 20 Hz to 20 kHz, missing potentially important information in the infrasonic and ultrasonic domains.
With regard to perceptual soundscapes, soundscape recordings convey acoustic information that stimulates the sensory organ (e.g., the cochlea for humans) but only a fraction of it is taken into account by the sensory system of the observer, each species showing a unique “listening bandwidth” and spectro-temporal resolution. For those reasons, soundscape recordings should not be confused with perceptual soundscapes, even with “low-level” perceptual representations.
Consequently, any subsequent processing stage of the former soundscape recording, whether it is sonic or numerical, can also be seen as some form of soundscape recording or representation. This is why the use of “soundscape recording” in this context should be accompanied by the explicit soundscape category in question, that is “distal soundscape recording” or “proximal soundscape recording”. When referring to the subjective experience, the term “perceptual soundscape” should be used.
Distal Soundscapes and Temporal Patterns
As presented in Figure 5, the distal soundscape is the soundscape that is the least affected by filtering processes, which allows the study of sound sources and their relationship with environmental factors. Distal soundscape can therefore be useful to reveal the processes that drive the diel and seasonal patterns of biophony and geophony. If we take the example of a temperate cold forest, diel patterns can be predicted by assessing the relationship between biotic factors such as birds’ circadian rhythms and temporal factors such as moon and annual cycles. As shown in Grinfeder et al. (2022), yearly patterns can be predicted by combining biotic factors such as vegetation seasonal cycle and weather abiotic factors such as temperature, snowfall, rainfall and wind. In this study, the description of invariant features (i.e., the periodicity of a selection of acoustic sources) in a specific area (i.e., the same habitat) were used as evidence for the description of the forest’s distal soundscape dynamics.
Proximal Soundscapes and the Listening Experience
Whereas distal soundscapes are often used to describe sounds at an ecological scale, proximal soundscapes are usually considered as means to change the individual experience of the sonic environment. The work of Bernie Krause consists of recording natural non-anthropogenic environments in order to study their composition and reproduce the sonic, subjective experience of listening in non-anthropogenic areas through soundscape composition (Krause, 2015). Here, the task focuses not on studying the ecological dynamics of the soundscape but on using observation points as references to produce a work of art to share with an audience. The information that is retrieved from these references is the type of sound sources (geophony and biophony), as well as potential sound propagation effects if needed. Since soundscape composition aims at inspiring “environmental listening awareness” (Westerkamp, 2002), soundscape composers allow themselves to take creative liberties. However, linking sound sources and acoustic filters to the corresponding environmental factors could help building proximal soundscape “reconstructions”, which are consistent with validated ecological knowledge. The reconstruction of proximal soundscapes could then be improved, going beyond the raw superposition of bird song recordings (Gasc et al., 2015; Zhao et al., 2019; Morrison et al., 2021). Such a tool would provide a new kind of ecoacoustic ground-truth that may be useful to rigorously evaluate biodiversity indexes among others.
Perceptual Soundscapes
Tympanic ears appeared about 210–230 million years ago (Grothe and Pecka, 2014). It is thus reasonable to assume that through selective adaptation, the auditory system of many species has evolved to develop and optimize a capacity to analyze soundscapes, efficiently detect biological sound sources and discriminate levels of biodiversity in close environments (e.g., Webster et al., 2017). Indeed, soundscapes provide crucial information about potential resources, preys, predators, mates and habitat structure.
As discussed in section “The Perceptual Soundscape,” the existence of a multiplicity of perceptual processing stages within the observer’s auditory and cognitive system makes the conception of a singular comprehensive example of perceptual soundscape difficult. In addition, the understanding of perceptual soundscapes has more often been the source of speculations based on landscape ecology, such as the hypothesis of the “cognitive soundscape” (Farina, 2014; Barchiesi et al., 2015), rather than a source of objective data production. Cognitive psychology and neurosciences can be used to draw operational hypotheses aiming to assess basic aspects of soundscape perception and test the respective roles of low- and high-level auditory mechanisms (Theunissen and Elie, 2014). For instance, are we humans able to discriminate between soundscapes associated with distinct habitats - which represent a specific combination of environmental factors - or temporal factors such as seasons or moments of the day? The answer is probably “yes” but information about the capacities of human listeners is clearly lacking. Many other questions arise. Are biological sound sources processed differently from geophonic sound sources? To which extent are we able to distinguish levels of biodiversity with our ears? These questions among others pave the way for an entirely new research program in the cognitive sciences of audition.
Conclusion
Despite the ambiguity that the soundscape concept has been carrying since Schafer’s seminal work, there is an opportunity to distinguish three distinct but complementary categories. The distal soundscape is the spatial and temporal distribution of sounds in a prespecified area, in relation to sound propagation effects. The proximal soundscape is the collection of propagated sound signals that occurs at a specific position in space. The perceptual soundscape is the individual subjective interpretation of a proximal soundscape. By explicitly clarifying soundscape definitions, we hope to make soundscape ecology more operational. The soundscape, which is often summarized as a simple collection of individual sounds, underlies a complex association of sound sources and acoustic filters that are affected by an array of environmental factors. We hope that the graphical display of these relationships can help ecologists and environment managers to formulate relevant scientific hypotheses, anticipate the ecoacoustic impact of anthropogenic and non-anthropogenic environmental changes and guide conservation policies.
Data Availability Statement
The original contributions presented in the study are included in the article/supplementary material, further inquiries can be directed to the corresponding author.
Author Contributions
EG designed research, conducted the literature review, developed the conceptual framework, and the qualitative model. CL, SH, and JS contributed to the conceptual framework and the qualitative model. All authors wrote the manuscript.
Funding
This work was supported by ANR-20-CE28-0011 “Hearbiodiv”, by FrontCog grant ANR-17-EURE-001, and by the Parc Naturel Régional du Haut-Jura who received funding from the Région Bourgogne-Franche-Comté and the Région Auvergne-Rhône-Alpes.
Conflict of Interest
The authors declare that the research was conducted in the absence of any commercial or financial relationships that could be construed as a potential conflict of interest.
Publisher’s Note
All claims expressed in this article are solely those of the authors and do not necessarily represent those of their affiliated organizations, or those of the publisher, the editors and the reviewers. Any product that may be evaluated in this article, or claim that may be made by its manufacturer, is not guaranteed or endorsed by the publisher.
Acknowledgments
We are grateful to Adèle de Baudouin, Richard McWalter, and Léo Varnet for their valuable insight that led to significant improvement of the manuscript. We thank both referees for their valuable comments.
References
Balakrishnan, R. (2016). “Behavioral Ecology of Insect Acoustic Communication,” in Insect Hearing, eds G. Pollack, A. Mason, A. Popper, and R. Fay (New York, NY: Springer International Publishing), doi: 10.1007/978-3-319-28890-1_3
Barber, J. R., Burdett, C. L., Reed, S. E., Warner, K. A., Formichella, C., Crooks, K. R., et al. (2011). Anthropogenic noise exposure in protected natural areas: estimating the scale of ecological consequences. Lands. Ecol. 26, 1281–1295. doi: 10.1007/s10980-011-9646-7
Barchiesi, D., Giannoulis, D., Stowell, D., and Plumbley, M. D. (2015). Acoustic scene classification: classifying environments from the sounds they produce. IEEE Signal Proc. Mag. 32, 16–34. doi: 10.1109/MSP.2014.2326181
Birch, L. C. (1957). The role of weather in determining the distribution and abundance of animals. Cold Spring Harb. Symp. Quant. Biol. 22, 203–218. doi: 10.1101/SQB.1957.022.01.021
Bradbury, J. W., and Vehrencamp, S. L. (2011). Principles of Animal Communication. Sunderland, MA: Sinauer Associates.
Brumm, H. (2004). The impact of environmental noise on song amplitude in a territorial bird. J. Anim. Ecol. 73, 434–440. doi: 10.1111/j.0021-8790.2004.00814.x
Buxton, R. T., McKenna, M. F., Mennitt, D., Fristrup, K., Crooks, K., Angeloni, L., et al. (2017). Noise pollution is pervasive in US protected areas. Science 356, 531–533. doi: 10.1126/science.aah4783
Celis-Murillo, A., Deppe, J. L., and Allen, M. F. (2009). Using Soundscape Recordings to Estimate Bird Species Abundance, Richness, and Composition. J. Field Ornithol. 80, 64–78. doi: 10.1111/j.1557-9263.2009.00206.x
Clark, C. J. (2016). “Locomotion-Induced Sounds and Sonations: Mechanisms, Communication Function, and Relationship with Behavior,” in Vertebrate Sound Production and Acoustic Communication, eds R. A. Suthers, W. T. Fitch, R. R. Fay, and A. N. Popper (New York, NY: Springer International Publishing), 83–117. doi: 10.1007/978-3-319-27721-9_4
Cooper, R. (1992). Systems and Organizations: distal and Proximal Thinking. Syst. Pract. 5, 373–377. doi: 10.1007/BF01059829
Dawson, D. K., and Efford, M. G. (2009). Bird Population Density Estimated from Acoustic Signals. J. Appl. Ecol. 46, 1201–1209. doi: 10.1111/j.1365-2664.2009.01731.x
Ellinger, N., and Hödl, W. (2003). Habitat acoustics of a neotropical Lowland rainforest. Bioacoustics 13, 297–321. doi: 10.1080/09524622.2003.9753503
Embleton, T. F. (1996). Tutorial on sound propagation outdoors. J. Acoust. Soc. Am. 100, 31–48. doi: 10.1121/1.415879
Farina, A. (2014). “Soundscape and Landscape Ecology,” in Soundscape Ecology, ed. A. Farina (Dordrecht, NL: Springer Netherlands), 1–28. doi: 10.1007/978-94-007-7374-5_1
Farina, A., Eldridge, A., and Li, P. (2021). Ecoacoustics and multispecies semiosis: Naming, semantics, semiotic characteristics, and competencies. Biosemiotics 14, 141–165. doi: 10.1007/s12304-021-09402-6
Farina, A., and James, P. (2016). The acoustic communities: definition, description and ecological role. Biosystems 147, 11–20. doi: 10.1016/j.biosystems.2016.05.011
Farina, A., and Pieretti, N. (2012). The soundscape ecology: a new frontier of landscape research and its application to islands and coastal systems. J. Mar. Isl. Cult. 1, 21–26. doi: 10.1016/j.imic.2012.04.002
Farji-Brener, A. G., Dalton, M. C., Balza, U., Courtis, A., Lemus-Domínguez, I., Fernández-Hilario, R., et al. (2018). Working in the Rain? Why leaf-cutting ants stop foraging When It’s Raining. Insectes Soc. 65, 233–239. doi: 10.1007/s00040-018-0605-z
Filipan, K., De Coensel, B., Aumond, P., Can, A., Lavandier, C., and Botteldooren, D. (2019). Auditory sensory saliency as a better predictor of change than sound amplitude in pleasantness assessment of reproduced urban soundscapes. Build. Environ. 148, 730–741. doi: 10.1016/j.buildenv.2018.10.054
Forrest, T. G. (1994). From sender to receiver: propagation and environmental effects on acoustic signals. Am. Zool. 34, 644–654. doi: 10.1093/icb/34.6.644
Gasc, A., Pavoine, S., Lellouch, L., Grandcolas, P., and Sueur, J. (2015). Acoustic indices for biodiversity assessments: Analyses of bias based on simulated bird assemblages and recommendations for field surveys. Biol. Conserv. 191, 306–312. doi: 10.1016/j.biocon.2015.06.018
Geipel, I., Smeekes, M. J., Halfwerk, W., and Page, R. A. (2019). Noise as an informational cue for decision-making: the sound of rain delays bat emergence. J. Exp. Biol. 222:jeb192005. doi: 10.1242/jeb.192005
Gibson, J. J. (2014). The Ecological Approach to Visual Perception. Hove: Psychology Press, doi: 10.4324/9781315740218
Gil, D., and Gahr, M. (2002). The honesty of bird song: multiple constraints for multiple traits. Trends Ecol. Evol. 17, 133–141. doi: 10.1016/S0169-5347(02)02410-2
Gil, D., and Llusia, D. (2020). “The bird dawn chorus revisited,” in Coding strategies in Vertebrate Acoustic Communication, eds T. Aubin and N. Mathevon (New York, NY: Springer International Publishing), 45–90.
Gomes, D. G., Hesselberg, T., and Barber, J. R. (2021). Phantom river noise alters orb-weaving spider abundance, web size, and prey capture. Funct. Ecol. 35, 717–726. doi: 10.1111/1365-2435.13739
Grant, R., Halliday, T., and Chadwick, E. (2013). Amphibians’ response to the lunar synodic cycle—a review of current knowledge, recommendations, and implications for conservation. Behav. Ecol. 24, 53–62. doi: 10.1093/beheco/ars135
Grinfeder, E., Haupert, S., Ducrettet, M., Barlet, J., Reynet, M.-P., Sèbe, F., et al. (2022). Soundscape dynamics of a cold protected forest: dominance of aircraft noise. Lands. Ecol. 37, 567–582. doi: 10.1007/s10980-021-01360-1
Grothe, B., and Pecka, M. (2014). The natural history of sound localization in mammals–a story of neuronal inhibition. Front. Neural Circuits 8:116. doi: 10.3389/fncir.2014.00116
Hanski, I., and Gilpin, M. (1991). Metapopulation dynamics: brief history and conceptual domain. Biol. J. Linn. Soc. 42, 3–16. doi: 10.1111/j.1095-8312.1991.tb00548.x
Huang, N., and Elhilali, M. (2017). Auditory salience using natural soundscapes. J. Acoust. Soc. Am. 141, 2163–2176. doi: 10.1121/1.4979055
Irwin, A., Hall, D. A., Peters, A., and Plack, C. J. (2011). Listening to urban soundscapes: physiological validity of perceptual dimensions. Psychophysiology 48, 258–268. doi: 10.1111/j.1469-8986.2010.01051.x
ISO 12913-1 (2014). Acoustics — Soundscape — Part 1: Definition and conceptual framework. Geneva: International Organization for Standardization.
Kang, J., and Aletta, F. (2018). The impact and outreach of soundscape research. Environments 5:58. doi: 10.3390/environments5050058
Kayser, C., Petkov, C. I., Lippert, M., and Logothetis, N. K. (2005). Mechanisms for allocating auditory attention: an auditory saliency map. Curr. Biol. 15, 1943–1947. doi: 10.1016/j.cub.2005.09.040
Larom, D., Garstang, M., Payne, K., Raspet, R., and Lindeque, M. (1997). The influence of surface atmospheric conditions on the range and area reached by animal vocalizations. J. Exp. Biol. 200, 421–431. doi: 10.1242/jeb.200.3.421
Lengagne, T., and Slater, P. J. (2002). The effects of rain on acoustic communication: tawny owls have good reason for calling less in wet weather. Proc. Biol. Sci. 269, 2121–2125. doi: 10.1098/rspb.2002.2115
Lewicki, M. (2002). Efficient coding of natural sounds. Nat. Neurosci. 5, 356–363. doi: 10.1038/nn831
Lindblom, B., Sundberg, J., Branderud, P., Djamshidpey, H., and Granqvist, S. (2010). “The Gunnar Fant legacy in the study of vocal acoustics,” in Procedings of the 10ème Congrès Français d’Acoustique, Lyon.
McGarigal, K., and Urban, D. (2001). Introduction to Landscape Ecology. Durham: Landscape Ecology course notes, Duke University.
Medina, I., and Francis, C. D. (2012). Environmental variability and acoustic signals: a multi-level approach in songbirds. Biol. Lett. 8, 928–931. doi: 10.1098/rsbl.2012.0522
Młynarski, W., and McDermott, J. H. (2019). Ecological origins of perceptual grouping principles in the auditory system. Proc. Natl. Acad. Sci. U.S.A. 116, 25355–25364. doi: 10.1073/pnas.1903887116
Moore, B. C., and Gockel, H. E. (2012). Properties of auditory stream formation. Philos. Trans. R. Soc. Lond. B Biol. Sci. 367, 919–931. doi: 10.1098/rstb.2011.0355
Morrison, C. A., Auniòð, A., Benkõ, Z., Brotons, L., Chodkiewicz, T., Chylarecki, P., et al. (2021). Bird population declines and species turnover are changing the acoustic properties of spring soundscapes. Nat. Commun. 12:6217. doi: 10.1038/s41467-021-26488-1
Nelken, I., Rotman, Y., and Yosef, O. B. (1999). Responses of auditory-cortex neurons to structural features of natural sounds. Nature 397, 154–157. doi: 10.1038/16456
Papazoglou, I. A. (1998). Functional block diagrams and automated construction of event trees. Reliab. Eng. Syst. Saf. 61, 185–214. doi: 10.1016/S0951-8320(98)00011-8
Payne, S. R., Davies, W. J., and Adams, M. D. (2009). Research into the Practical and Policy Applications of Soundscape Concepts and Techniques in Urban Areas. London: HMSO.
Phillips, Y. F., Towsey, M., and Roe, P. (2018). Revealing the ecological content of long-duration audio-recordings of the environment through clustering and visualisation. PLoS One 13:e0193345. doi: 10.1371/journal.pone.0193345
Pijanowski, B. C., Farina, A., Gage, S. H., Dumyahn, S. L., and Krause, B. L. (2011). What is soundscape ecology? An introduction and overview of an emerging new science. Lands. Ecol. 26, 1213–1232. doi: 10.1007/s10980-011-9600-8
Ratcliffe, E. (2021). Sound and soundscape in restorative natural environments: a narrative literature review. Front. Psychol. 12:570563. doi: 10.3389/fpsyg.2021.570563
Reza, F. M. (1994). An Introduction to Information Theory. North Chelmsford, MA: Courier Corporation.
Richards, D. G., and Wiley, R. H. (1980). Reverberations and amplitude fluctuations in the propagation of sound in a forest: implications for animal communication. Am. Nat. 115, 381–399. doi: 10.1086/283568
Roberts, J., Hunter, M. L., and Kacelnik, A. (1981). The ground effect and acoustic communication. Anim. Behav. 29, 633–634. doi: 10.1016/S0003-3472(81)80132-7
Rodriguez, A., Gasc, A., Pavoine, S., Grandcolas, P., Gaucher, P., and Sueur, J. (2014). Temporal and spatial variability of animal sound within a neotropical forest. Ecol. Inform. 21, 133–143. doi: 10.1016/j.ecoinf.2013.12.006
Rosenblatt, A. E., and Schmitz, O. J. (2014). Interactive effects of multiple climate change variables on trophic interactions: a meta-analysis. Clim. Chang. Responses 1:8. doi: 10.1186/s40665-014-0008-y
Schafer, R. M. (1977). Our Sonic Environment and the Tuning of the World: The Soundscape. Rochester, VT: Destiny Books.
Shannon, C. E., and Weaver, W. (1949). The Mathematical Theory of Communication. Champaign, IL: Illinois University Press.
Shannon, G., McKenna, M. F., Angeloni, L. M., Crooks, K. R., Fristrup, K. M., Brown, E., et al. (2016). A synthesis of two decades of research documenting the effects of noise on wildlife. Biol. Rev. 91, 982–1005. doi: 10.1111/brv.12207
Siemers, B. M., and Schaub, A. (2011). Hunting at the highway: traffic noise reduces foraging efficiency in acoustic predators. Proc. Biol. Sci. 278, 1646–1652. doi: 10.1098/rspb.2010.2262
Southworth, M. (1969). The sonic environment of cities. Environ. Behav. 1, 49–70. doi: 10.1177/001391656900100104
Šturm, R., López Díez, J. J., Polajnar, J., Sueur, J., and Virant-Doberlet, M. (2022). Is it time for ecotremology? Front. Ecol. Evol. 152:828503. doi: 10.3389/fevo.2022.828503
Sueur, J., and Farina, A. (2015). Ecoacoustics: the ecological investigation and interpretation of environmental sound. Biosemiotics 8, 493–502. doi: 10.1007/s12304-015-9248-x
Suthers, R. A., Fitch, W. T., Fay, R. R., and Popper, A. N. (2016). Vertebrate Sound Production and Acoustic Communication. New York, NY: Springer International Publishing.
Swearingen, M. E., and White, M. J. (2007). Influence of scattering, atmospheric refraction, and ground effect on sound propagation through a pine forest. J. Acoust. Soc. Am. 122, 113–119. doi: 10.1121/1.2735108
Tarrero, A. I., Martín, M. A., González, J., Machimbarrena, M., and Jacobsen, F. (2008). Sound propagation in forests: a comparison of experimental results and values predicted by the Nord 2000 model. Appl. Acoust. 69, 662–671. doi: 10.1016/j.apacoust.2007.01.007
Taylor, A. M., Charlton, B. D., and Reby, D. (2016). “Vocal production by terrestrial mammals: Source, filter, and function,” in Vertebrate Sound Production and Acoustic Communication, eds R. A. Suthers, W. T. Fitch, R. R. Fay, and A. N. Popper (New York, NY: Springer International Publishing), 229–259. doi: 10.1007/978-3-319-27721-9_8
Tekcan, A. Ý, Yılmaz, E., Kaya Kızılöz, B., Karadöller, D. Z., Mutafoðlu, M., and Aktan Erciyes, A. (2015). Retrieval and phenomenology of autobiographical memories in blind individuals. Memory 23, 329–339. doi: 10.1080/09658211.2014.886702
Tembrock, G. (1996). Akustische Kommunikation bei Saeugetieren. Darmstadt, DE: Wissenschaftliche Buchgesellschaft.
Theunissen, F. E., and Elie, J. E. (2014). Neural processing of natural sounds. Nat. Rev. Neurosci. 15, 355–366. doi: 10.1038/nrn3731
Thomas, L., and Marques, T. A. (2012). Passive acoustic monitoring for estimating animal density. Acoust. Today 8, 35–44. doi: 10.1121/1.4753915
Thoret, E., Varnet, L., Boubenec, Y., Férriere, R., Le Tourneau, F. M., Krause, B., et al. (2020). Characterizing amplitude and frequency modulation cues in natural soundscapes: a pilot study on four habitats of a biosphere reserve. J. Acoust. Soc. Am. 147, 3260–3274. doi: 10.1121/10.0001174
Thuiller, W., Albert, C., Araujo, M. B., Berry, P. M., Cabeza, M., Guisan, A., et al. (2008). Predicting global change impacts on plant species’ distributions: future challenges. Perspect. Plant. Ecol. Evol. Syst. 9, 137–152. doi: 10.1016/j.ppees.2007.09.004
Tishechkin, D. Y. (2013). Vibrational background noise in herbaceous plants and its impact on acoustic communication of small Auchenorrhyncha and Psyllinea (Homoptera). Entomol. Rev. 93, 548–558. doi: 10.1134/S0013873813050035
Webster, P. J., Skipper-Kallal, L. M., Frum, C. A., Still, H. N., Ward, B. D., and Lewis, J. W. (2017). Divergent human cortical regions for processing distinct acoustic-semantic categories of natural sounds: animal action sounds vs. vocalizations. Front. Neurosci. 10:579. doi: 10.3389/fnins.2016.00579
Westerkamp, H. (2002). Linking soundscape composition and acoustic ecology. Organ. Sound 7, 51–56. doi: 10.1017/S1355771802001085
Wiley, R., and Richards, D. G. (1978). Physical constraints on acoustic communication in the atmosphere: implications for the evolution of animal vocalizations. Behav. Ecol. Sociobiol. 3, 69–94. doi: 10.1007/BF00300047
York, J. E., Young, A. J., and Radford, A. N. (2014). Singing in the moonlight: dawn song performance of a diurnal bird varies with lunar phase. Biol. Lett. 10:20130970. doi: 10.1098/rsbl.2013.0970
Zhao, Z., Xu, Z. Y., Bellisario, K., Zeng, R. W., Li, N., Zhou, W. Y., et al. (2019). How well do acoustic indices measure biodiversity? Computational experiments to determine effect of sound unit shape, vocalization intensity, and frequency of vocalization occurrence on performance of acoustic indices. Ecol. Ind. 107:105588. doi: 10.1016/j.ecolind.2019.105588
Keywords: soundscape, environmental factors, sound sources, sound propagation, distal soundscape, proximal soundscape, perceptual soundscape
Citation: Grinfeder E, Lorenzi C, Haupert S and Sueur J (2022) What Do We Mean by “Soundscape”? A Functional Description. Front. Ecol. Evol. 10:894232. doi: 10.3389/fevo.2022.894232
Received: 11 March 2022; Accepted: 24 May 2022;
Published: 15 June 2022.
Edited by:
Almo Farina, University of Urbino Carlo Bo, ItalyReviewed by:
Jenni Stanley, University of Waikato, New ZealandAlice Eldridge, University of Sussex, United Kingdom
Copyright © 2022 Grinfeder, Lorenzi, Haupert and Sueur. This is an open-access article distributed under the terms of the Creative Commons Attribution License (CC BY). The use, distribution or reproduction in other forums is permitted, provided the original author(s) and the copyright owner(s) are credited and that the original publication in this journal is cited, in accordance with accepted academic practice. No use, distribution or reproduction is permitted which does not comply with these terms.
*Correspondence: Elie Grinfeder, elie.grinfeder@gmail.com