- 1IGEPP, INRAE, Institut Agro, Univ Rennes, Le Rheu, France
- 2IGEPP, INRAE, Institut Agro, Univ Rennes, Rennes, France
Adoption of practices that reduce the risk of pest outbreaks is one of the pillars of agroecology and is largely based on biological control. Multiple infield and landscape parameters affect biocontrol, but the effects of conservation soil management on biological control have been poorly investigated over crop season. By comparing winter wheat fields within the same landscape but with different soil management, the direct and indirect effects of soil management (conservation and conventional systems) on natural enemies’ communities and their biological control on aphids was studied from the tillering stage to the harvest. In addition to aphid infestation, two families of the main natural enemies’ guilds were monitored, as well as their associated services: aphid parasitoid, a specialist and flying natural enemy, with parasitism service, and carabid beetles, a generalist and ground-dwelling predator, with aphidophagy service. Soil conservation system hosted more abundant and diverse carabid beetles’ assemblages, and received higher aphidophagy service in June than conventional system. However, neither parasitoid abundance, nor parasitism rates, were affected by soil management. Aphid infestation and its associated damage did not depend on soil management either. Our results suggest that ground-dwelling natural enemies are more impacted by soil management than foliage-dwelling natural enemies, which is partly reflected in aphid biocontrol. In agricultural systems with reduced soil perturbation, direct mortality on ground-dwelling communities due to tillage may be lower than in a conventional system, but habitat heterogeneity is also greater, increasing the number of ecological niches for natural enemies. Both factors are supposed to favor an early presence of natural enemies and a tendency toward a precocious aphidophagy service is indeed observed in conservation system.
Introduction
Faced with many global challenges, including increasing agricultural yields, food security, environmental protection and climate change mitigation, the European Union is aiming to promote practices combining viable agricultural production, sustainable management and territorial development with improved economic competitiveness (Kertész and Madarász, 2014). Biological control is one of the reliable levers of integrated pest management, significantly reducing yield loss caused by agricultural pests (Losey and Vaughan, 2006; Ali et al., 2018). However, by threatening biodiversity, including natural enemies’ communities, agricultural intensification jeopardizes the biocontrol service they provide (Geiger et al., 2010; Winqvist et al., 2012; Carbonne et al., 2022). By promoting agricultural practices which allow subsidiary resources being available for natural enemies and improving the number of biological interactions, biological control of pests could be favored, leading to a reduction in the use of pesticides.
Several natural enemies, with specialist or generalist diet, may reduce pest abundances with complementary effects (Snyder and Ives, 2003; Thies et al., 2011; Dainese et al., 2017). Biocontrol complementarity is based on facilitation between natural enemies, but also niche partitioning, resulting from attacking different life stages of the same pest or asynchronous complementarity, by exploiting the prey at different times of the season (Snyder, 2019). However, complementarity effects of natural enemies on pests are not systematic (Letourneau et al., 2009) and the complexity of trophic networks and the risk of antagonistic interactions, such as predation, increase with natural enemy diversity and could deter biological control (Schmidt et al., 2007; Martin et al., 2013).
Aphids are a major pest of cereal fields causing yield loss through sap-sucking but also through virus transmission (Ali et al., 2018; Nancarrow et al., 2021). Aphids and their natural enemies are diverse and well-studied, and their interaction-complex has become a model-system for trophic interactions and population dynamics studies. The effects of complementarity and interactions between aphid natural enemies on aphid biological control have also been the subject of numerous studies. Indeed, facilitation between coccinellids and carabids occurs when the foraging behavior of coccinellids causes aphids to fall off plants making it easier for carabids to capture preys (Losey and Denno, 1998). Likewise, niche partitioning occurs between coccinellids and aphid parasitoids that attack aphid populations on different parts of their host plant (Straub and Snyder, 2008). However, predation events also happen between several natural enemies of aphids. For instance, Staudacher et al. (2018) showed that spiders are an important part of the diet of carabids, reflecting intraguild predation. Parasitoid DNA was also detected in the gut content of both coccinellids and carabids (Traugott et al., 2012; Ortiz-Martínez et al., 2020).
According to Gonzalez-Sanchez et al. (2015), conservation agriculture is a sustainable agriculture production system “whose farming and soil management techniques protect the soil from erosion and degradation, improve its quality and biodiversity, and contribute to the preservation of the natural resources, water and air, while optimizing yields.” Conservation agriculture differs from conventional agriculture in three main ways: minimal soil perturbation (no- or reduced tillage), permanent soil cover and high diversity of cropping system with crop rotation (Bash et al., 2011; Gonzalez-Sanchez et al., 2015). Conservation systems stand out for their habitat heterogeneity as well, they provide more ecological niches and alternative preys to natural enemies’ communities (Finke and Denno, 2002; Langellotto and Denno, 2004), indirectly favoring their presence and diversity and consequently the biocontrol services they provide (Dainese et al., 2019). Positive relationship between soil management reduction, diversity and abundance of natural enemy communities and biocontrol services has already been proven for weeds or some ground dwelling pests like slugs (Honek et al., 2003; Menalled et al., 2007; Bohan et al., 2011; Scaccini et al., 2020) but still has to be demonstrated on insect pests, especially aphids (but see Tamburini et al., 2016).
Damage from aphid transmission of the Yellow Dwarf Virus have also been reported to be lower on barley cultivated with crop residues and no-tillage than on barley cultivated in a conventional system. Jordan and Hutcheon (2002) suggest that this is due to (1) the inability of aphids to recognize barley plants in presence of residues and (2) a more abundant and diverse presence of natural enemies in conservation system. Indeed, direct perturbation due to tillage is reduced in conservation systems and, as a result, direct mortality of underground and ground-dwelling communities, including predators like carabids, spiders or rove beetles, is also reduced (Thorbek and Bilde, 2004; Saska et al., 2008; Soane et al., 2012).
By comparing pairs of winter wheat fields that differ in soil management but are surrounded by the same landscape structure, we aimed to assess the effects of soil management on natural enemies’ communities, their biocontrol services and pest populations throughout the growing season. For the first time, both direct and indirect effects of soil management were studied diachronically, considering temporal variations in natural enemy communities, their biocontrol services and aphid infestation. From wheat tillering to harvest season, two communities of natural enemies, carabid beetles and aphid parasitoids, were monitored to illustrate the impact of soil management on both ground-dwelling and foliage-dwelling natural enemies. Carabids are ground-dwelling predators that can overwinter in fields and are supposed to be sensitive to soil management (Soane et al., 2012). Both overwintering and circulating communities of carabids were monitored to disentangle the direct and indirect effects of conservation soil management. The level of aphid infestation, the composition and abundance of natural enemies’ communities and their biocontrol services were assessed. Finally, damage were estimated using the Rautapää’s index (Rautapää, 1966) through the cumulative abundance of cereal aphids over time.
We hypothesized that fields cultivated under conservation soil management (1) host more abundant and diverse communities of natural enemies, especially carabid beetles, than conventional fields, and (2) benefit consequently from enhanced biological control services, especially aphidophagy, and reduced aphid infestation. At last, (3) carabid beetles’ communities are expected to be more precocious in conservation fields than in conventional ones, due to overwintering carabid populations not affected by tillage, which should result in earlier predation on sentinel prey.
Materials and Methods
Study Site
The study site was located in the western periphery of Rennes, Brittany, Western France. This site is a 50,000-ha farmland area that exhibits a “bocage” structure, with a dense hedgerow network, and is dominated by a polyculture-livestock system. Five locations were chosen in the study site, in which two paired fields in conservation and conventional soil management were selected (Figure 1). The distance between two locations was at least 1.9 km and at most 21.3 km. Locations were not supposed to be spatially auto-correlated, as previous studies in the same region have shown spatial independence (Puech et al., 2015; Djoudi et al., 2018). Conventional fields corresponded to crops where the soil management consisted in more than 25 cm depth tillage and inversion of the soil and conservation fields corresponded to crops where the soil management consisted in tillage of less than 10 cm depth, and non-inversed soil. Paired fields were less than 100 m apart and therefore assumed to share the same landscape environment. Reduced soil management was applied for at least 6 years in conservation fields except for one (Biodiversystem: 1 year). Fields surface varied from 0.25 ha to 2 ha. All fields were cultivated with winter wheat (Triticum aestivum), and previously cultivated with maize.
Arthropod Sampling
Aphids and Parasitoids Sampling
Aphid density was assessed at 6 times in each field from the start of the rise of aphid population in April to grain maturation stage in July 2019 (April 5th, April 18th, May 9th, May 28th, June 13th, July 12th). In the center of each field, seven spots were randomly selected, a total of 100 wheat tillers were sampled in 10 m transect and the sum of apterous aphid individuals was carefully collected alive by hand using small brushes. Individuals were identified to species level using taxonomic keys (Blackman and Eastop, 2000), counted and stored in laboratory under controlled conditions (20 ± 2°C, 60 ± 10% RH and LD 16:8 h photoperiod) in ventilated petri dishes up to 4 weeks or to the mummification of the parasitized ones. In the case of mummified aphids, they were isolated in individual 1.5 mL microcentrifuge tubes until the emergence of adult parasitoids, which were identified to species level (Hullé et al., 2020).
Carabid Sampling
Two carabid communities were studied: the circulating carabid community and the emerging carabid community. The circulating community is composed of carabids that are present in the field but that have not necessarily emerged in the field, and the emerging community is composed solely of carabids that have emerged in the field. Circulating carabid individuals were sampled for 1 week each month, from the start of carabid emergence, in March, to grain maturation, in July 2019 (March 21st, April 4th, May 9th, June 13th, July 11th), using pitfall traps. Those individuals compose the so-called circulating carabid community. Pitfall traps were filled to the third with a brine (100 g of salt for 1 L of water) mixed with 3–4 drops of soap (TEEPOL). Emerging carabid individuals were collected using emergence tents (60 cm2) in parallel of circulating communities. In each tent, two traps were set up: one pitfall trap at ground level to capture ground-dwelling invertebrates and another one placed at the top of the tent (50 cm high) to capture flying invertebrates. Those individuals compose the so-called emerging carabid community. In each field, four sampling spots, 10 m apart from each other, consisting of one emergence tent and two pitfall traps (10 m apart from each other), were set up at least 25 m away from the field border, to avoid edge effect. See the SM1 for more details. Traps were active for 7 days and, trapped carabid beetles were counted and identified at lowest taxonomical level using taxonomical keys (Roger et al., 2013).
Service and Damage Assessment
Aphid Damage
Damage was estimated using the Rautapää’s index (Rautapää, 1966) through the cumulative abundance of cereal aphids over time, all species combined.
Parasitism Service
Parasitism potential was measured by the ratio of parasitized aphids among all the aphids that were collected during wheat threshing and kept in isolation in the laboratory.
Aphidophagy Service
Predation potential on aphids was assessed using sentinel preys, once a month, from March to July 2019. Eight 2-weeks old broad bean (Vicia faba) plants infested with 10 apterous pea aphids (Acyrthosiphon pisum) were exposed in each field for 3 days. After 3 days, aphids remaining on the sentinel plants were counted and predation rate assessed. The aphids exposed in the fields were in their fourth nymphal stage to avoid reproduction and a skewed predation rate during their period in the field.
Statistical Analysis
All statistical analyses were carried out with the software R (R Core Team, 2019), version 3.6.1.
Impact of Soil Management on the Dynamics of Arthropod Communities
The sum of larvae and adult carabids were considered for abundance data, but only adults were considered for biodiversity index due to inability to identify larval state. Effects of soil management and the sampling session on the abundance of all arthropod communities of interest were assessed using generalized linear models fitted with the “nb.glmer()” function from “lme4” package (Bates et al., 2019), considering a negative binomial distribution and a log link function. Field factor, nested in location factor, was included as a random factor. If models did not converge, we followed the recommendations of the package’s authors (Bates et al., 2019), i.e., 3 corrections were applied one after another until models converged: first, scaling variables, second, increasing models’ iterations number, last changing optimization function from “nlminbwrap” to “bobyga.” Detection of overdispersion was tested using Pearson residual χ2 statistic. Significance of the explanatory variables was checked using the “Anova()” function from “car” package (Fox et al., 2019). Pairwise comparisons between levels of each factor were carried out using “emmeans()” and “pairs()” functions from “emmeans” package (Lenth et al., 2020).
Sampling completeness was estimated by calculating Chao1 index with the “specpool()” function from “vegan” package (Oksanen et al., 2019). The effect of soil management and of sampling session on Species richness and Shannon index of carabid communities was assessed using a generalized linear model fitted with the “glmer()” function from “lme4” package, considering a Poisson distribution and a log link function for Species richness, and with the “lmer()” function and a Gaussian distribution for Shannon index (Bates et al., 2019). Field factor, nested in Location factor, was also included as a random factor. Model convergence, overdispersion checking, significance of variable and pairwise comparisons were treated in the same way as for abundance models.
Permutational Multivariate Analysis of Variance (PERMANOVA) applied on Cao distance matrix was used to test the significance of differences in carabid assemblages between emerging communities captured in pitfall traps and circulating communities, between conservation and conventional systems and between sampling sessions, using the “adonis2()” function from “vegan” package (Oksanen et al., 2019). In a second time, PERMANOVA was applied separately on Bray-Curtis distance matrices of circulating and emerging communities to test the significance of differences in carabid assemblages between conservation and conventional systems and between sampling sessions, using the same function. Association between farming practices, or sampling sessions, and species patterns was tested with a multi-level pattern analysis, using the “multipatt()” function from “indicspecies” package (De Cáceres et al., 2010).
Due to the low number of species observed, differences in species richness, Shannon values and assemblage composition were not considered for parasitoids and aphids.
Impact of Soil Management on the Dynamics of Ecosystem Services and Damage
The effect of soil management and sampling session on aphid predation and parasitism rates were assessed using a generalized linear model fitted with the “glmer()” function from “lme4” package (Bates et al., 2019), considering a binomial distribution and a logit link function. The effect of soil management and sampling session on damage (cumulated number of aphids) were assessed using a generalized linear model fitted with the “nb.glmer()” function from “lme4” package (Bates et al., 2019), considering a negative binomial distribution and a log link function. Field factor, nested in Location factor, was also included as a random factor. Model convergence, overdispersion checking, significance of variable and pairwise comparisons were treated in the same way as for abundance model.
Results
Variations in Arthropod Communities of Agricultural Interest Over Time and According to Soil Management
Carabid Communities
A total of 6,334 carabid individuals belonging to 71 species were captured in pitfall traps and emergence tents. Observed specific richness of circulating and emerging carabid communities were 66% and 67% of Chao1 estimated richness respectfully (SM2). Considering the highly J-shaped structure of carabid communities in agroecosystems, those rates suggest good sampling completeness, so statistical analysis are presented on observed specific richness.
Differences Between Assemblages of Pitfall-Trapped Emerging and Circulating Carabids
The two carabid communities were trapped with different sampling methods, so the observed differences might be due to differences in carabids assemblages or differences in trapping efficiency and should be interpreted with caution. Permutation test showed that carabid assemblages significantly differed between emerging and circulating communities (R2 = 0.077, P = 0.001), but also between types of soil management (R2 = 0.007, P = 0.003). The difference in their interaction was also significant (emerging versus circulating, R2 = 0.005, P = 0.007), which is hardly attributable to the trapping method alone. Sampling session, and its interaction with soil management, had a significant influence on carabid assemblages (R2 = 0.126, P = 0.001, respectively, R2 = 0.017, P = 0.001). Some carabid species were significantly associated with specific soil management and nature of the community (Figure 2): Ophonus subquadratus was significantly associated with emerging communities in conservation fields (P = 0.035). Poecilus cupreus was significantly associated with circulating communities regardless of soil management (P = 0.005). Finally, Anchomenus dorsalis, Amara sp and Harpalus affinis were significantly associated with circulating communities regardless of soil management as well as with the emerging communities in conservation fields (respectively, P = 0.005, P = 0.005 and P = 0.045).
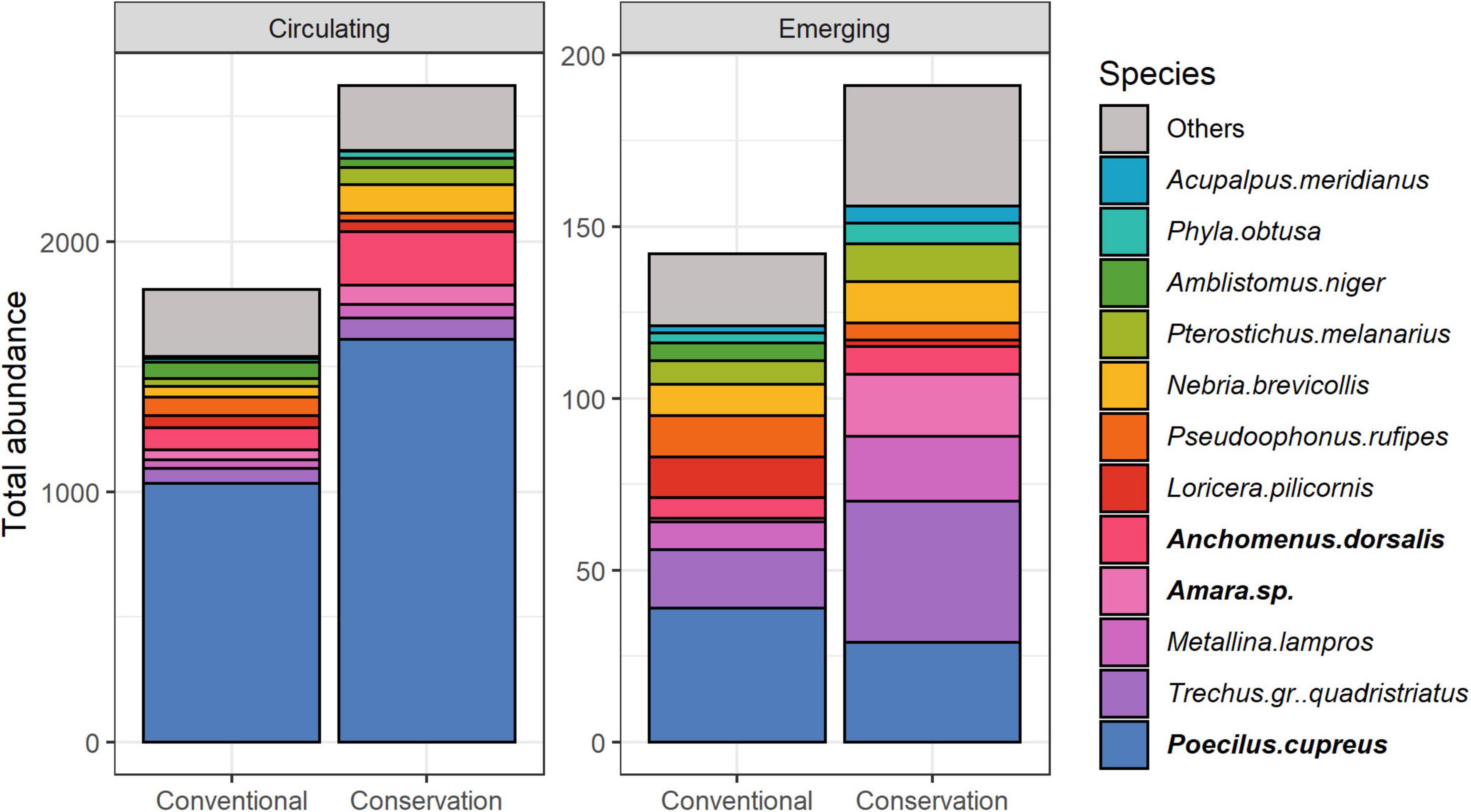
Figure 2. Variations of cumulative abundance of carabid species representing at least 75% of the total abundance depending on soil management and type of community. Indicator species are bolded. Take note that the scales are different between circulating and emerging carabid communities.
Circulating Carabid Communities
A total of 4,855 carabid individuals belonging to 61 species were captured in pitfall traps. Soil management, sampling session and their interaction all had a significant effect on the number of captured carabids per trap. Only soil management and sampling session had a significant effect on species richness per trap, and only sampling session and its interaction with soil management had a significant effect on Shannon index (Table 1 and Figure 3).
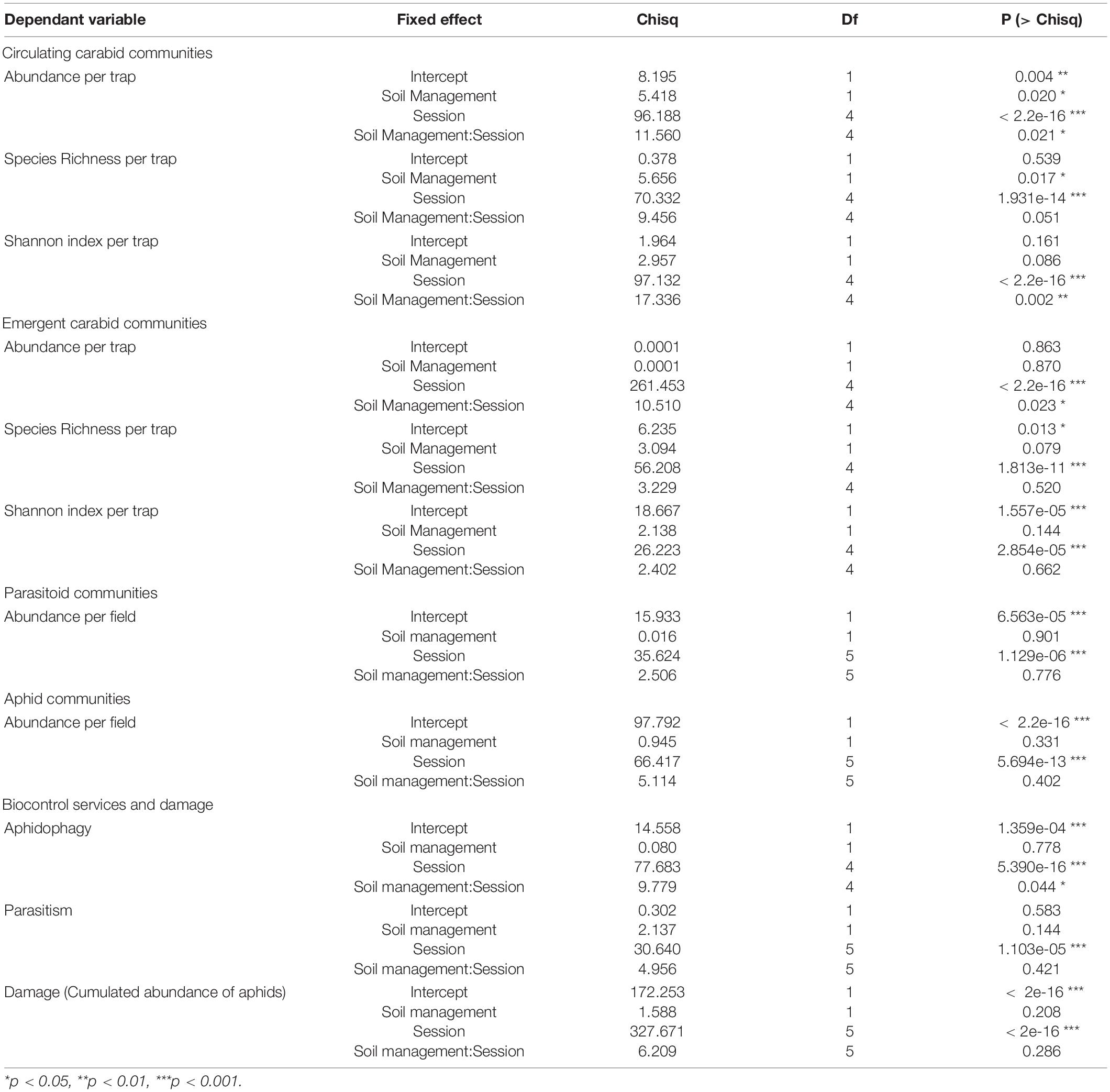
Table 1. Deviance table for the effect of soil management, sampling session and their interaction on arthropod communities, their ecosystem services and damage.
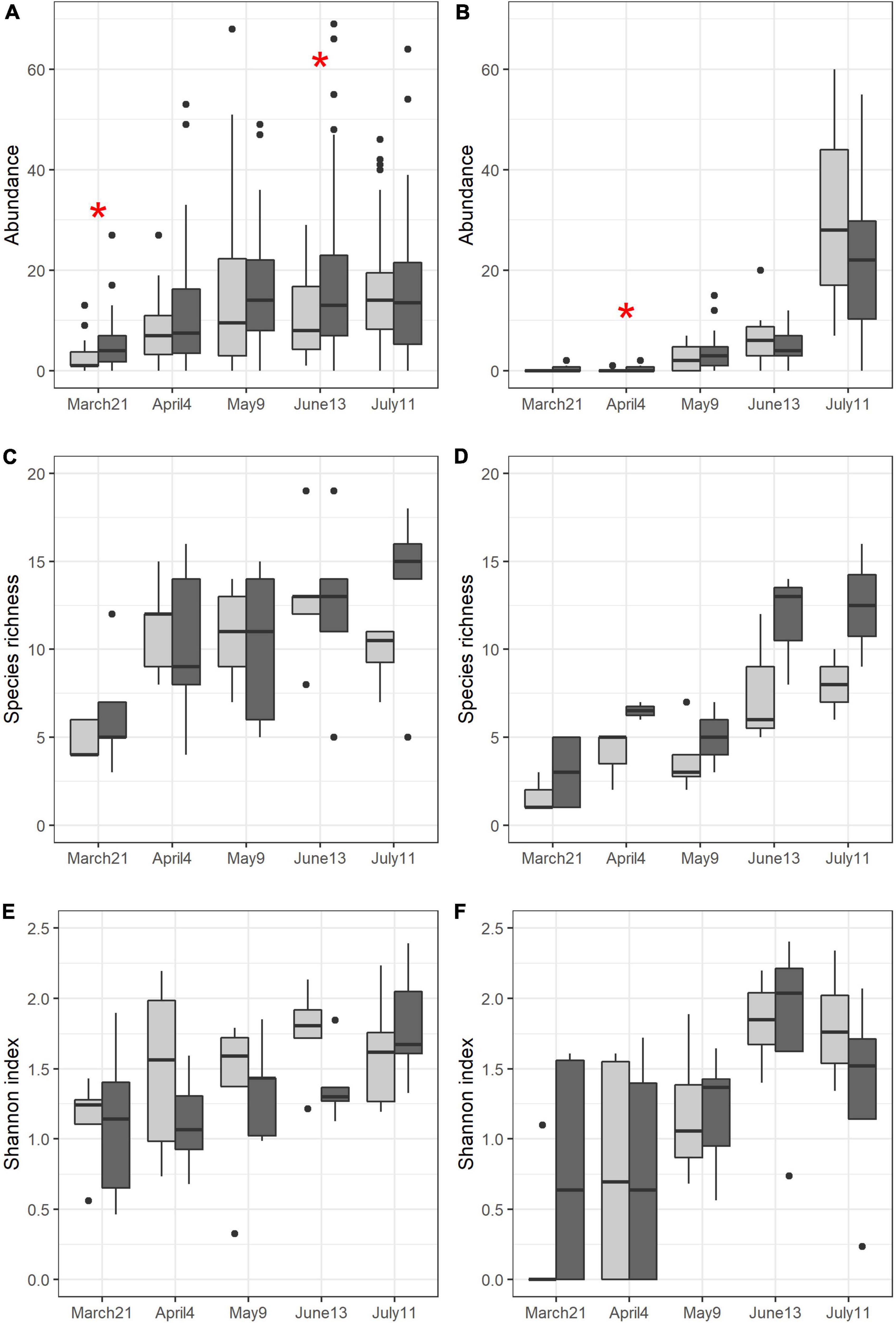
Figure 3. Variations of Abundance per trap (A,B), species richness per field (C,D) and Shannon index per field (E,F) for circulating (A,C,E) and emerging (B,D,F) carabid communities over time and according to soil management (light gray: conventional, dark gray: conservation). Red asterisk reflects significant difference between soil managements at a specific sampling session. To improve the readability of the figure, 2 outliers were ignored in panel (A) (1 in May in conservation and 1 in June in conservation), 1 outlier was ignored in panel (B) (in July in conservation) and 1 outlier was ignored in panel (C) (in July in conventional).
Overall, carabid abundance was greater in fields under conservation soil management than in fields under conventional soil management (P = 0.004). Differences were significant on March 21st (P = 0.020) and on June 13th (P = 0.030). Carabid abundance also increased over time (P < 2.2e-16) and significant differences were observed during Spring (March 21st < April 4th < May 9th/June 13th/July 11th, P < 0.0001 for all comparisons with March 21st and P = 0.012/0.006/0.001). Species richness was greater in fields under conservation soil management than in fields under conventional soil management (P = 0.017) and also increased from Spring to Summer (March 21st < April 4th < June 13th, July 11th, respectively, P < 0.0001, P = 0.0008, P = 0.0002). Finally, Shannon index also increased significantly over time from Spring to Summer (March 21st < April 4th < June 13th, July 11th, respectively, P = 0.0001 for all comparisons with March 21st, P = 0.0001, P < 0.0001).
Permutation test showed that carabid assemblages significantly differed between soil management (R2 = 0.006, P = 0.011), sampling session (R2 = 0.133, P = 0.001) and their interaction (R2 = 0.017, P = 0.009). Two species were significantly associated with each type of soil management: Nebria brevicollis (P = 0.005) and Notiophilus biguttatus (P = 0.025) for conservation soil management, and Demetrias atricapillus (P = 0.005) and Drypta dentata (P = 0.025) for conventional soil management (Figure 4). Differences between fields in conservation and conventional soil managements were significant on June 13th only (R2 = 0.030, P = 0.029), but no species was specifically associated to a type of soil management. On May 9th and July 11th, differences in community composition between soil management are close to significance (P = 0.072 and P = 0.06) with D. atricapillus significantly associated with conventional soil management on May 9th (P = 0.005), and N. brevicollis with conservation soil management both on May 9th and July 11th (P = 0.005 and P = 0.02). Several carabid species were also significantly associated with a sampling session (SM3).
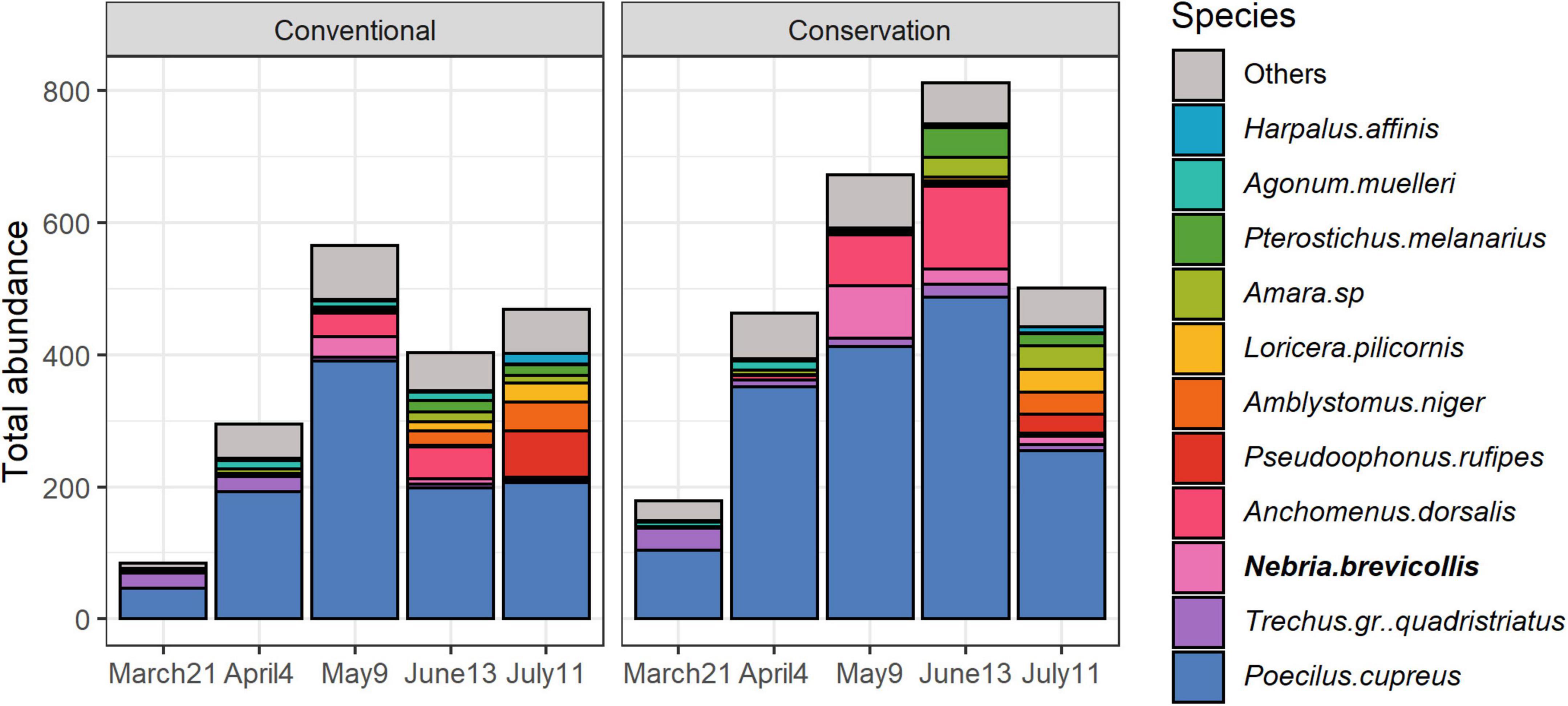
Figure 4. Variations over time and soil management of cumulative abundance of carabid species in the circulating community representing at least 80% of the total abundance. Indicator species are bolded.
Emerging Carabid Communities
A total of 1,479 carabid individuals belonging to 43 species were captured in emergence tents. Sampling session and its interaction with soil management had significant effects on the number of carabids captured per tent, but only sampling session significantly influenced species richness per tent and Shannon index (Table 1 and Figure 2).
Emerging carabid abundance significantly increased over time (April 4th < May 9th < June 13th < July 11th, respectively, P < 0.0001, P = 0.0005, P < 0.0001). In Spring (March 21st, April 4th, May 9th), the number of captured emerging carabids was greater in fields under conservation soil management than in fields under conventional soil management (significant difference on April 4th only, P = 0.044). In Summer (June 13th, July 11th) the trend was reversed. Species richness and Shannon index increased over time with significant differences between early Spring and Summer (Species richness: March 21st < May 9th < June 13th, July 11th, respectively, P = 0.002, P = 0.0001 and P < 0.0001; Shannon index: March 21st, April 4th, May 9th < June 13th, July 11th, respectively, March 21st: P < 0.0001 and P < 0.0001, April 4th: P = 0.001 and P = 0.002, May 9th: P = 0.014 and P = 0.031).
Permutation test showed that emerging carabid assemblages significantly changed depending on soil management (R2 = 0.020, P = 0.005) and sampling session (R2 = 0.107, P = 0.001) and but not with their interaction (Figure 5). One species was associated with conservation soil management: Amara sp. (P = 0.040). When analyses were repeated for each sampling session individually, the difference between fields in conservation and conventional soil managements was significant only on May 9th (R2 = 0.069, P = 0.041), and this month, Metallina lampros was associated with conservation soil management (P = 0.035). Some carabid species were also significantly associated to a sampling session (SM4).
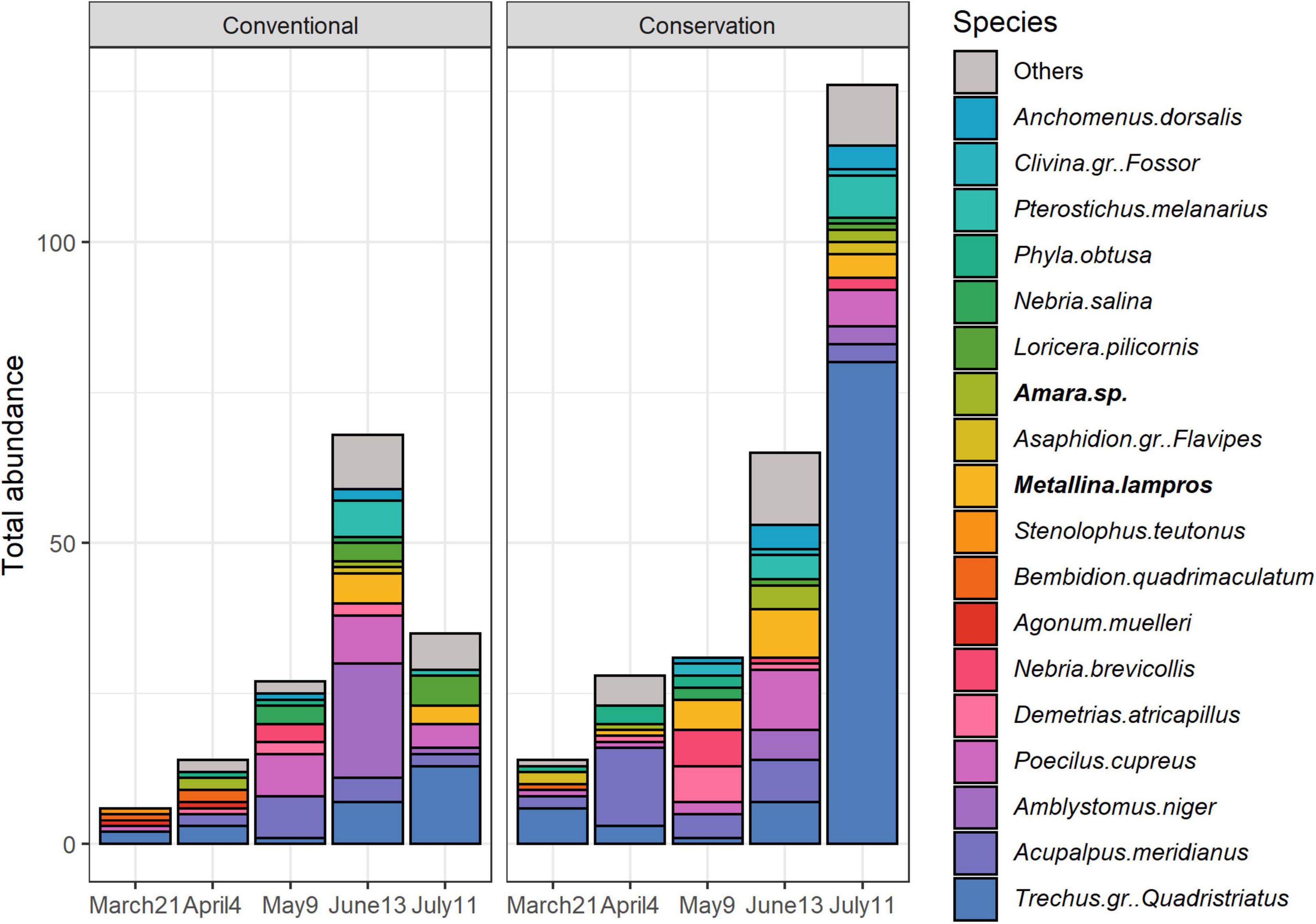
Figure 5. Variations over time and soil management of cumulative abundance of carabid species in the emerging community representing at least 80% of the total abundance. Indicator species are bolded.
Parasitoid Communities
A total of 403 parasitoids emerged from collected aphids. Sampling session had a significant effect on parasitoid abundance, but not soil management (Table 1 and Figure 6A). During the first three sampling sessions (April 5th, April 18th, May 9th), 6 species of parasitoids were identified (Figure 6B). Parasitoids were significantly more abundant at peak infestation (June 13th) compared to previous sampling sessions regardless of the type of soil management (P < 0.001 for all comparisons with previous sessions). Regardless of the sampling session, no difference between soil managements was significant.
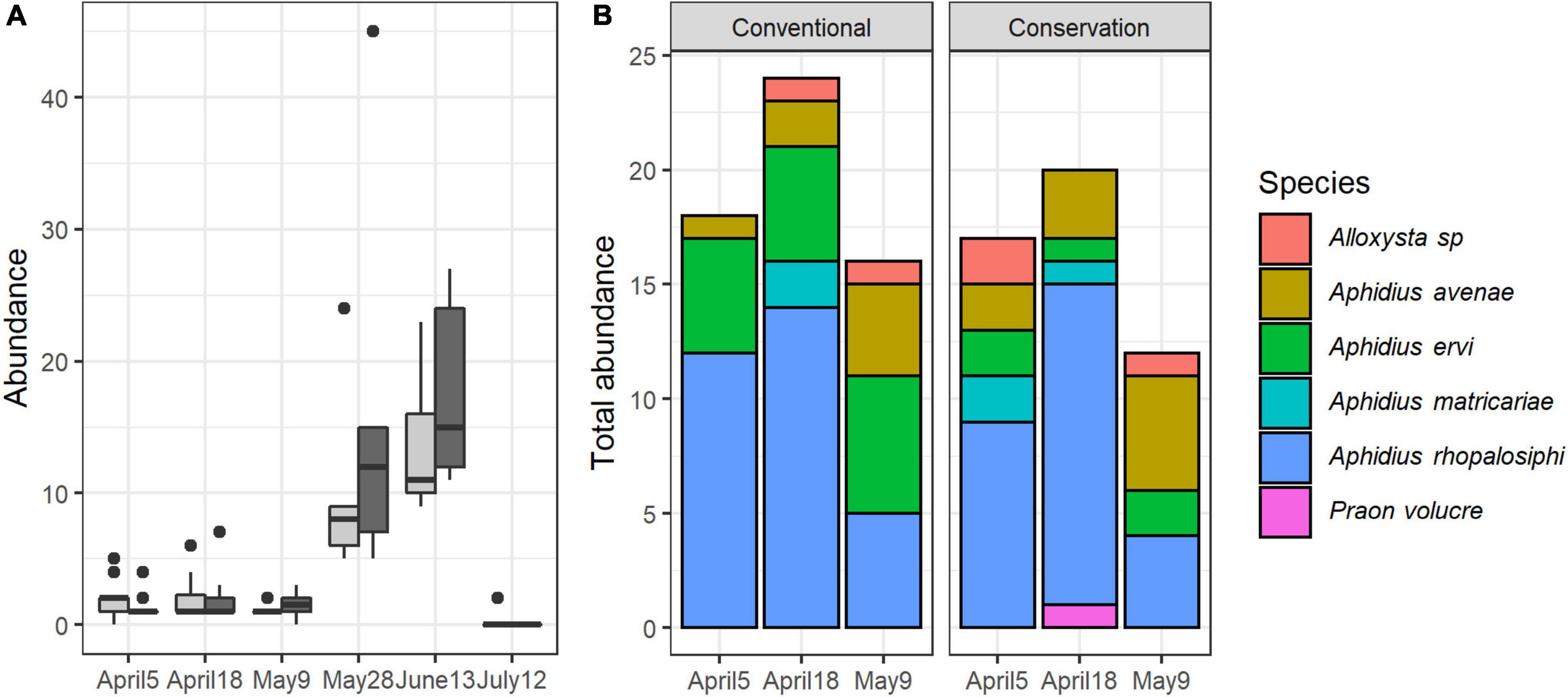
Figure 6. Variations of parasitoid abundance per field over time and according to soil management (A) and of the cumulative abundance of parasitoid species for the first three sampling sessions (B).
Aphid Communities
A total of 1,147 aphids were collected, comprising 610 Sitobion avenae, 464 Metopolophium dirhodum and 73 Rhopalosiphum padi (Figure 7). The colony structure of aphid populations resulted in a strong aggregation of data. Hence, despite the use of binomial negative distribution a significant remaining overdispersion (P = 0.003) after model fitting was observed. Therefore, the estimated probabilities should be interpreted with caution.
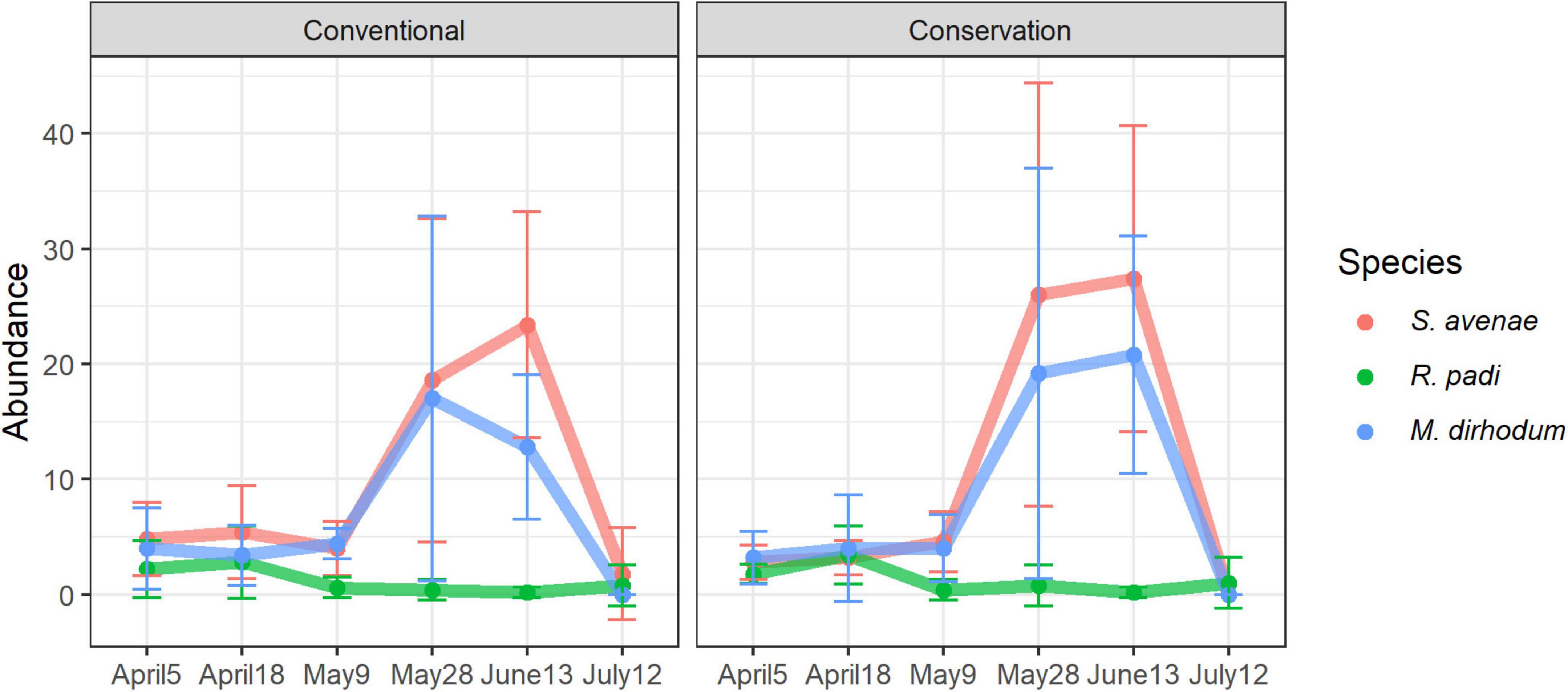
Figure 7. Temporal variations of the abundance per field of the three detected cereal aphids depending on soil management.
Only sampling session had a significant effect on aphid abundance (Table 1). Aphid abundance peaked on May 28th and June 13th and was significantly higher at these times than during all previous and subsequent sampling sessions (P < 0.001 for all comparisons with previous and subsequent Sessions).
Variations in Aphid Biological Control and Damages Over Time and According to Soil Management
Sampling session and its interaction with soil management had significant effects on aphidophagy, but only sampling session had a significant effect on parasitism service (Table 1). Aphidophagy significantly increased over time (March 21st, April 4th < May 9th, June 13th < July 11th, P < 0.0001 for all comparisons, Figure 8A), and on June 13th, aphidophagy service was greater in fields under conservation soil management than in fields under conventional soil management (P = 0.018). Reversely, parasitism service decreased over time (Figure 8B) with significant differences between April 5th and May 28th (P = 0.006), and between May 9th and June 13th (P = 0.0001).
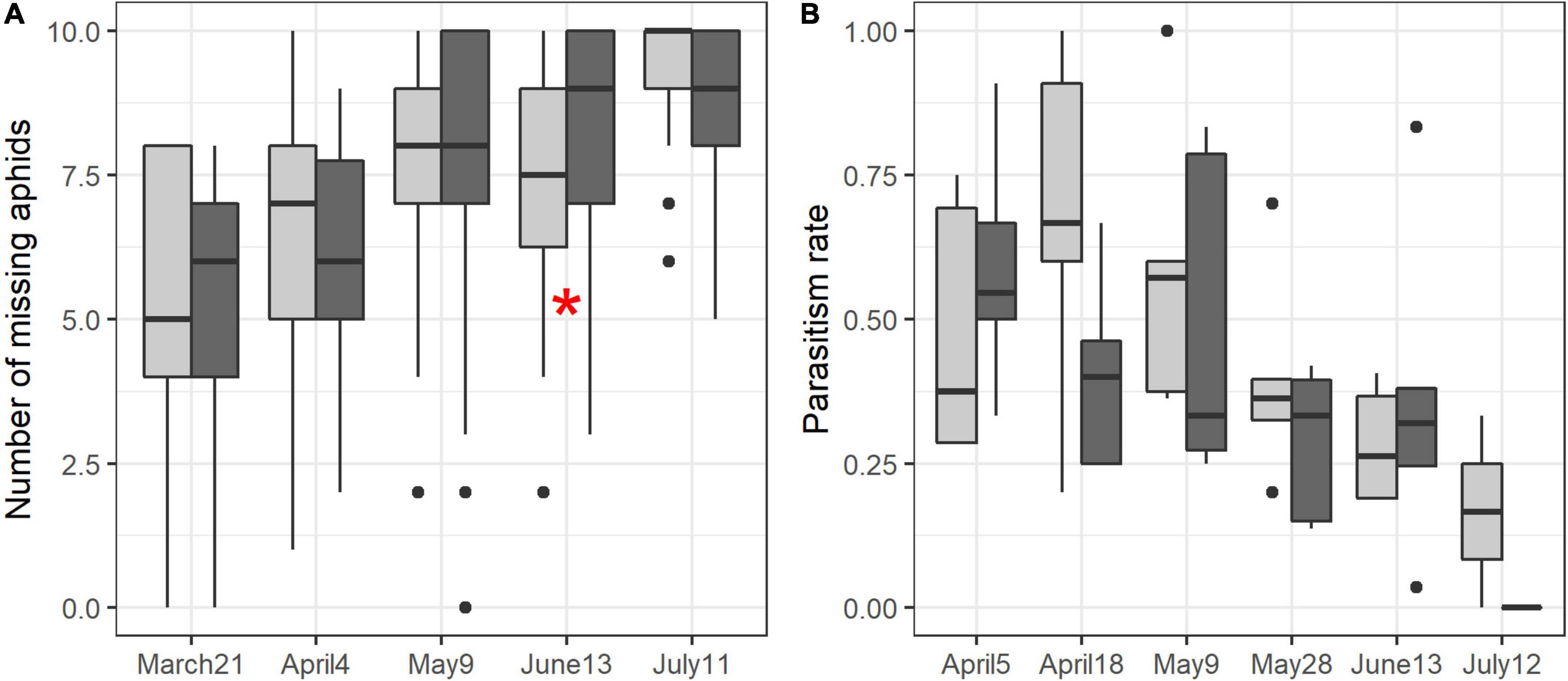
Figure 8. Variations of aphidophagy service (A) and parasitism service (B) over time and according to soil management (light gray: conventional, dark gray: conservation). Red asterisk reflects significant difference between soil managements at a specific sampling session.
Only sampling session had a significant effect on aphid damage (Table 1). Damage caused by aphids tended to be lower in fields under conservation soil management in Spring than in conventional fields but the trend was reversed from the end of May onward, however, the differences between soil management were not significant (SM5).
Discussion
In the present study, the influence of soil management practices on natural enemy communities was assessed. Simultaneously, the ecosystem service of biological control of aphids and their population dynamics were evaluated. In general, compared to conventional soil management, conservation soil practices had a positive effect on the abundance and diversity of carabids (ground dwelling natural enemies). Conversely, parasitoids (aerial natural enemies) were not influenced by soil management practices. Some variations over time in carabid community’s structure were observed and the effect on natural enemy communities was partially reflected in biocontrol services with higher aphidophagy services at peak aphid infestation. No differences were observed for parasitism rates and aphid abundances remained similar between the two types of soil management.
Influence of Soil Management on Natural Enemy Abundance and Diversity
Soil management is an important factor in determining soil biotope properties and its biocoenosis (Kladivko, 2001). Ground dwelling arthropods, such as carabids, are especially sensitive to soil management. In accordance, conservation fields with reduced perturbations harbored more diverse and abundant carabid communities than conventional fields in this study. Regarding diversity indices similar findings have been consistently observed in previous studies (Baguette and Hance, 1997; Hatten et al., 2007; Menalled et al., 2007; Shearin et al., 2007) but abundance responses to conservation soil management varied. Most previous studies also showed a positive impact of conservation practices with higher abundances of carabids in conservation systems than in conventional systems (Kromp, 1999; Holland and Reynolds, 2003; Kosewska et al., 2014). However, in some cases higher abundances were found in conventional systems (Baguette and Hance, 1997; Hatten et al., 2007; Menalled et al., 2007), but this was usually due to a single dominant species that was either indifferent to disturbance or resilient to it thanks to good colonization capabilities (Baguette and Hance, 1997; Hatten et al., 2007). Allema et al. (2019) also hypothesized that in disturbed open habitat, such as conventional fields, carabids exhibit a more intense foraging activity than in complex habitat, resulting in increased trapping efficacy rather than increased abundance.
In general, conservation soil management is expected to have direct beneficial impacts on communities that overwinter in fields (Soane et al., 2012). Direct mechanical disturbances, such as deep tillage applied in conventional fields, result in high mortality of field-overwintering carabid individuals. In fact, emerging communities of carabids have been recorded to be more abundant in conservation fields or in less disturbed habitats than in conventional fields (Holland and Reynolds, 2003; Thorbek and Bilde, 2004; Mesmin et al., 2020). Similarly, the number of individuals that emerged in conservation fields in our study was 1.3 more abundant than in conventional fields (SM6). Carabid species overwintering as larvae are suspected to be more sensitive to tillage than overwintering adults (Purvis and Fadl, 1996; Mesmin et al., 2020) with the exception of those that overwinter below plough depth (Barney and Pass, 1986). In our case, almost all emerged carabid species overwintered as adults, but Trechus gr. quadristriatus, the most abundant species in the emerging community overwintered as both larvae and adults and was 2.45 more abundant in conservation fields than in conventional ones (SM6), which may indeed suggest a greater sensitivity of the larval stage to tillage. Emergence dynamics also depended on soil management: emerging communities were more abundant in conservation fields than in conventional ones in Spring (see Figure 3), but the trend was reversed in Summer. This temporal change in carabids response to tillage can be explained by two non-exclusive hypotheses: first, the soil is more loosened in conventional fields than in conservation ones due to tillage which facilitates carabid burial and Summer-emerging species overwinter below plough depth escaping direct mortality. For instance, Pseudoophonus rufipes, that starts emerging and being active in July in our study, have previously been found buried at 45 cm and below (Briggs, 1965). Second, carabid emergence in conservation fields is earlier because of changes in soil properties. Indeed, heat diffuses faster in conservation soils than in conventional soils (Potter et al., 1985), leading to faster development of carabid eggs and larvae (Tenailleau et al., 2011) which could explain earlier emergence.
One of the main benefits of conventional deep tillage is weed suppression, which leads to habitat homogenization, in contrast to the more weed-colonized conservation fields (which was actually observed in our fields pairs), and to indirect negative impacts on natural enemies. MacArthur (1972) hypothesized that the number of available ecological niches increased with habitat complexity, which is expected to have a positive effect on the co-existence of species (Tews et al., 2004; Stein et al., 2014). A high habitat heterogeneity supports a wide range of alternative preys (Langellotto and Denno, 2004; Finke and Snyder, 2008) and brings stability in natural enemies food webs (Staudacher et al., 2018) and biocontrol services (Langellotto and Denno, 2004; Birkhofer et al., 2008; Finke and Snyder, 2008) by lessening interspecific competition (Finke and Denno, 2002; Staudacher et al., 2018). In consequences, high habitat heterogeneity is expected to be beneficial for generalist predators like carabid beetles, by providing a wider range of prey and refuge, which is in accordance with the higher abundance and species richness observed in circulating communities of conservation systems in our study. Parasitoids are also expected to benefit from habitat heterogeneity. Conservation fields by providing a greater abundance and diversity of weeds (Soane et al., 2012) might provide more nutritional resources, overwintering sites and alternative preys (Rusch et al., 2010; Araj et al., 2011). The number of parasitoids was slightly higher in conservation fields than in conventional ones at peak aphid infestation, in late May and early June in our study. On the contrary, more parasitoids were present at the beginning of the season in conventional fields than in conservation ones, possibly due to a bottom-up control of parasitoid populations by aphid resources since aphids were more abundant in April in those fields. In any case, no significant differences in parasitoid abundance were found according to the type of soil management, suggesting that if an effect exists, it is of low magnitude.
Influence of Soil Management on Biocontrol Services and Aphid Infestation
Aphid predation by carabid beetles increased over time and was similar between soil managements, except in June when this service was greater in conservation fields than in conventional fields. The mechanisms governing temporal variations in aphids place in the carabid diet have not been studied but they have been for seed predation. Given the generalist diet of carabid beetles, the same mechanisms could be at play for aphid predation. According to Saska et al. (2008), temporal variations in seed predation result from three mechanisms. First, there is a turnover in carabid communities; even if carabids are generalist predators, there is a wide gradient of diet among species and a different food demand is expected depending on community composition. Second, food demand is expected to change with phenological stage, even within the same species. Third, changes in environment temperature lead to changes in activity and metabolic rates of carabids and in consequences, in predation rates. Aphidophagy service was linked to carabid abundance in a previous study that used aphids as sentinel prey (Boetzl et al., 2020) and, regardless of soil management we can assume that carabid community turnover, changes in phenological stage and in environment temperature, all contributed to changes in aphidophagy rates, with an increase over time in our study. In June, aphid predation rate was higher in conservation fields than in conventional ones while no significant difference in carabid abundance was observed at this time. However, in June, a significant difference in carabid community composition was noted between fields managed with conservation practices and fields managed with conventional practices, suggesting that species composition rather than total abundance determines aphid predation rate.
In our study, parasitism rates did not differ according to soil management. Contrarily, Tamburini et al. (2016) found higher parasitism rates in fields under conservation soil management than in fields under conventional management. However, in this study, parasitism rates also depended on the proportion of semi-natural habitat in the landscape, but only for conventional fields, suggesting that habitat heterogeneity within the field could compensate for poor landscape quality. Brittany’s agricultural landscape exhibits a “bocage” structure with semi-natural field-borders that may provide refuge and resources to arthropod communities. This type of structure could compensate for the poor habitat heterogeneity in conventional fields and mitigate the positive response of parasitoids to conservation practices. Parasitism rates decreased over time in our study. Until May, aphid infestation remained low resulting in a shortage of resources for the already present parasitoids and parasitism rates reaching over 50% (see Figure 8). At peak aphid infestation, parasitism rates decreased, probably due to a rapid increase in resources, and varied between 25% and 35% regardless of soil management, an order of magnitude similar to a previous study performed in Brittany (Derocles et al., 2014). Although differences were not significant, peak parasitism rate in conservation fields was earlier than in conventional fields, which makes us wonder about the potential benefits of conservation practices on early service of biocontrol.
Aphidophagy and parasitism have reversed temporal dynamics suggesting a temporal complementarity of carabid and parasitoid communities in the provision of aphid control service. An effective complementarity between flying and ground dwelling guilds of natural enemies had already been observed through lower aphid infestation when both guilds were present (Schmidt et al., 2004; Tamburini et al., 2016), despite the risk of negative interactions between natural enemies, such as consumption of mummified aphids by predators (Traugott et al., 2012; Ortiz-Martínez et al., 2020). These opposed dynamics between natural enemies might be due to the removal by predators of aphid resources which the parasitoids can no longer access. Similarly to the delay between complex and simple landscape context, conservation soil management, by favoring a precocious presence of predators compared to conventional soil management, might explain the delay in parasitism response in conventional system (Ortiz-Martínez and Lavandero, 2018). Regardless of the interaction between natural enemies, aphid population dynamics or their damage do not change with soil management. Indeed, despite higher carabid abundances and aphidophagy, especially in June, conservation fields displayed similar aphid infestation dynamics as conventional fields.
Services were measured with various methods, inducing some biases: parasitism rate was assessed directly on local aphid populations, while aphidophagy was measured using introduced sentinel prey, pea aphids. As a result, the measures of aphid control by parasitoids and by predators are not strictly identical, making it difficult to compare the two. Furthermore, sentinel prey have been criticized for their immobility and suspected of not truly reflecting natural trophic interactions (Birkhofer et al., 2017) but they have been shown to be an efficient proxy for assessing predation in an ecosystem (Boetzl et al., 2020) and immobility bias was removed in our study by using live aphids as sentinel prey.
Natural Enemy Assemblage and Implications for Biocontrol Services
Parasitoid community did not seem to be impacted by soil management: both assemblages were composed of Aphidiinae parasitoids. In contrast to parasitoid assemblages and in accordance with previous studies, soil management influenced carabid assemblages (Baguette and Hance, 1997; Holland and Reynolds, 2003; Thorbek and Bilde, 2004; Kosewska et al., 2014). One species was dominant in circulating communities regardless of soil management (Poecilus cupreus), suggesting that this species mainly overwinters outside the fields and recolonizes them afterward. Some others were specific to the circulating communities and to the emerging community in conservation soil management (Anchomenus dorsalis, Amara sp, Harpalus affinis), suggesting that these species overwinter both inside and outside the fields and are directly negatively impacted by autumn tillage. Similar results have already been observed for Amara and Harpalus genera (Tonhasca, 1993; Baguette and Hance, 1997; Thorbek and Bilde, 2004; Pretorius et al., 2018) and for A. dorsalis (Thorbek and Bilde, 2004 but Baguette and Hance, 1997) and corroborate the existence of direct mortality caused by tillage. It is worth noting that those three taxa were especially active in June, when aphidophagy was significantly higher in the conservation fields than in the conventional ones, and are recognized biocontrol agents. Indeed, A. dorsalis is a well-known aphid consumer (Chiverton, 1987, p. 0; Sunderland and Vickerman, 1980; Staudacher et al., 2018) and could explain the difference in aphidophagy between systems. Amara sp and H. affinis have also been observed consuming aphids but at lower predation rates (Sunderland and Vickerman, 1980; Staudacher et al., 2018). However, Amara and Harpalus genera are known for their predation service on weed seeds (Honek et al., 2007; Saska et al., 2008), highlighting that other services not assessed in our study could be affected by conservation practices.
When considering only circulating carabid communities, some species were also characteristic of the soil management mode. Demetrias atricapillus and Drypta dentata were more abundant in conventional fields, while Nebria brevicollis and Notiophilus biguttatus were more abundant in conservation fields. Spring breeders that overwinter as adults, such as D. atricapillus and D. dentata, should be resistant to soil disturbance. In contrast, species that breed in autumn and overwinter in their larval form are more sensitive to perturbation (Purvis et al., 2001; Ribera et al., 2001 but Kosewska et al., 2014), which is consistent with the observed higher abundance of N. brevicollis and N. biguttatus in conservation fields as they breed in both Autumn and Spring. In addition, the ability to fly of D. atricapillus and D. dentata, which had functional wings, gives them good dispersal capabilities, which is advantageous in conventional fields to escape disturbance and recolonize (Ribera et al., 2001). D. atricapillus has been shown to be a good aphid-consumer (Sunderland and Vickerman, 1980) but the diet of N. brevicollis includes a wide variety of pests in addition to aphids: lepidopterans, crane flies or even slugs (Sunderland and Vickerman, 1980; Seric Jelaska et al., 2014; Reich et al., 2020), confirming the presence of multiservice-providing species in conservation fields.
Conclusion
This study assessed the impact of conservation soil management on some selected natural enemy characteristics of a single pest and their corresponding complementary services. Our results contributed to a better understanding of the sensitivity to soil management of ground-dwelling natural enemies like carabids and suggested a correlation between both abundance and assemblage of carabids and aphid predation. In contrast, foliage-dwelling natural enemies like parasitoid wasps had a moderate response to soil management, and so had their parasitism service. Despite an overall positive effect of conservation soil management compared to conventional soil management, aphid infestation remained similar between farming systems, suggesting soil management is not the only factor affecting aphid biological control.
Considering that one of the natural enemy communities was composed of generalist predators, variations of this community due to conservation soil management could also have an impact on the biocontrol of other pests. Other ecosystem services could be impacted altogether by conservation practices, such as decomposition of organic matter or pollination, and a multiservice approach would allow for the assessment of synergies and trade-offs between services.
Data Availability Statement
The raw data supporting the conclusions of this article will be made available by the authors, without undue reservation.
Author Contributions
EC and MP conceptualized and designed the study. ASMP, EC, and SO-M acquired fieldwork data. ASMP analyzed the data and wrote the first version of the manuscript. All authors contributed to the manuscript.
Funding
This study was funded by ANR NGB (ANR-17-CE32-0011), “Next Generation Biomonitoring of change in ecosystem structure and function.” SO-M would like to thank the CONICYT Becas Chile Postdoctoral Grant N° 74190108.
Conflict of Interest
The authors declare that the research was conducted in the absence of any commercial or financial relationships that could be construed as a potential conflict of interest.
Publisher’s Note
All claims expressed in this article are solely those of the authors and do not necessarily represent those of their affiliated organizations, or those of the publisher, the editors and the reviewers. Any product that may be evaluated in this article, or claim that may be made by its manufacturer, is not guaranteed or endorsed by the publisher.
Acknowledgments
We thank the farmers who allowed us to work in their fields. We also thank Yann Laurent and all the internees for their contribution in fieldwork and Franck Duval for his help in carabid identification.
Supplementary Material
The Supplementary Material for this article can be found online at: https://www.frontiersin.org/articles/10.3389/fevo.2022.893787/full#supplementary-material
References
Ali, A., Desneux, N., Lu, Y., and Wu, K. (2018). Key aphid natural enemies showing positive effects on wheat yield through biocontrol services in northern China. Agric. Ecosyst. Environ. 266, 1–9. doi: 10.1016/j.agee.2018.07.012
Allema, B., Hemerik, L., Rossing, W. A. H., Groot, J. C. J., van Lenteren, J. C., and van der Werf, W. (2019). Dispersal of a carabid beetle in farmland is driven by habitat-specific motility and preference at habitat interfaces. Entomol. Exp. Appl. 167, 741–754. doi: 10.1111/eea.12804
Araj, S.-E., Wratten, S., Lister, A., Buckley, H., and Ghabeish, I. (2011). Searching behavior of an aphid parasitoid and its hyperparasitoid with and without floral nectar. Biol. Control 57, 79–84. doi: 10.1016/j.biocontrol.2010.11.015
Baguette, M., and Hance, T. (1997). Carabid beetles and agricultural practices: influence of soil ploughing. Biol. Agric. Hortic. 15, 185–190. doi: 10.1080/01448765.1997.9755193
Barney, R. J., and Pass, B. C. (1986). Ground Beetle (Coleoptera: Carabidae) Populations in Kentucky Alfalfa and Influence of Tillage. J. Econ. Entomol. 79, 511–517. doi: 10.1093/jee/79.2.511
Bash, G., Kassam, A., Gonzalez-Sanchez, E., and Streit, B. (2011). Making Sustainable Agriculture Real in CAP 2020 - The Role of Conservation Agriculture. Évora: universidade de évora.
Bates, D., Maechler, M., Bolker, B., Walker, S., Christensen, R. H. B., Singmann, H., et al. (2019). lme4: Linear Mixed-Effects Models using “Eigen” and S4. Madison, WI: R Project.
Birkhofer, K., Bylund, H., Dalin, P., Ferlian, O., Gagic, V., Hambäck, P. A., et al. (2017). Methods to identify the prey of invertebrate predators in terrestrial field studies. Ecol. Evol. 7, 1942–1953. doi: 10.1002/ece3.2791
Birkhofer, K., Gavish-Regev, E., Endlweber, K., Lubin, Y. D., Berg, K., von Wise, D. H., et al. (2008). Cursorial spiders retard initial aphid population growth at low densities in winter wheat. Bull. Entomol. Res. 98, 249–255. doi: 10.1017/S0007485308006019
Blackman, R. L., and Eastop, V. F. (2000). Aphids on the World’s Crops: An Identification and Information Guide. New York, NY: Wiley.
Boetzl, F. A., Konle, A., and Krauss, J. (2020). Aphid cards – Useful model for assessing predation rates or bias prone nonsense? J. Appl. Entomol. 144, 74–80. doi: 10.1111/jen.12692
Bohan, D. A., Boursault, A., Brooks, D. R., and Petit, S. (2011). National-scale regulation of the weed seedbank by carabid predators: carabid seed predation. J. Appl. Ecol. 48, 888–898. doi: 10.1111/j.1365-2664.2011.02008.x
Briggs, J. B. (1965). Biology of some ground beetles (Col., Carabidae) injurious to strawberries. Bull. Entomol. Res. 56, 79–93. doi: 10.1017/S0007485300057060
Carbonne, B., Bohan, D. A., Foffová, H., Daouti, E., Frei, B., Neidel, V., et al. (2022). Direct and indirect effects of landscape and field management intensity on carabids through trophic resources and weeds. J. Appl. Ecol. 59, 176–187. doi: 10.1111/1365-2664.14043
Chiverton, P. A. (1987). Predation of Rhopalosiphum padi (Homoptera: Aphididae) by polyphagous predatory arthropods during the aphids’ pre-peak period in spring barley. Ann. Appl. Biol. 111, 257–269. doi: 10.1111/j.1744-7348.1987.tb01452.x
Dainese, M., Martin, E. A., Aizen, M. A., Albrecht, M., Bartomeus, I., Bommarco, R., et al. (2019). A global synthesis reveals biodiversity-mediated benefits for crop production. Sci. Adv. 5:eaax0121. doi: 10.1126/sciadv.aax0121
Dainese, M., Schneider, G., Krauss, J., and Steffan-Dewenter, I. (2017). Complementarity among natural enemies enhances pest suppression. Sci. Rep. 7:8172. doi: 10.1038/s41598-017-08316-z
De Cáceres, M., Legendre, P., and Moretti, M. (2010). Improving indicator species analysis by combining groups of sites. Oikos 119, 1674–1684. doi: 10.1111/j.1600-0706.2010.18334.x
Derocles, S. A. P., Le Ralec, A., Besson, M. M., Maret, M., Walton, A., Evans, D. M., et al. (2014). Molecular analysis reveals high compartmentalization in aphid–primary parasitoid networks and low parasitoid sharing between crop and noncrop habitats. Mol. Ecol. 23, 3900–3911. doi: 10.1111/mec.12701
Djoudi, E. A., Marie, A., Mangenot, A., Puech, C., Aviron, S., Plantegenest, M., et al. (2018). Farming system and landscape characteristics differentially affect two dominant taxa of predatory arthropods. Agric. Ecosyst. Environ. 259, 98–110. doi: 10.1016/j.agee.2018.02.031
Finke, D. L., and Denno, R. F. (2002). Intraguild predation diminished in complex-structured vegetation: implications for prey suppression. Ecology 83, 643–652. doi: 10.1007/s00442-006-0443-y
Finke, D. L., and Snyder, W. E. (2008). Niche Partitioning Increases Resource Exploitation by Diverse Communities. Science 321, 1488–1490. doi: 10.1126/science.1160854
Fox, J., Weisberg, S., Price, B., Adler, D., Bates, D., Baud-Bovy, G., et al. (2019). car: Companion to Applied Regression. Madison, WI: R Project.
Geiger, F., Bengtsson, J., Berendse, F., Weisser, W. W., Emmerson, M., Morales, M. B., et al. (2010). Persistent negative effects of pesticides on biodiversity and biological control potential on European farmland. Basic Appl. Ecol. 11, 97–105. doi: 10.1016/j.baae.2009.12.001
Gonzalez-Sanchez, E., Veroz-Gonzalez, O., Blanco-Roldan, G. L., Marquez-Garcia, F., and Carbonell, R. (2015). A renewed view of conservation agriculture and its evolution over the last decade in Spain. Soil Tillage Res. 146, 204–212. doi: 10.1016/j.still.2014.10.016
Hatten, T. D., Bosque-Pérez, N. A., Labonte, J. R., Guy, S. O., and Eigenbrode, S. D. (2007). Effects of Tillage on the Activity Density and Biological Diversity of Carabid Beetles in Spring and Winter Crops. Environ. Entomol. 36, 356–368. doi: 10.1093/ee/36.2.356
Holland, J. M., and Reynolds, C. J. M. (2003). The impact of soil cultivation on arthropod (Coleoptera and Araneae) emergence on arable land. Pedobiologia 47, 181–191. doi: 10.1078/0031-4056-00181
Honek, A., Martinkova, Z., and Jarosik, V. (2003). Ground beetles (Carabidae) as seed predators. Eur. J. Entomol. 100, 531–544. doi: 10.14411/eje.2003.081
Honek, A., Martinkova, Z., Saska, P., and Pekar, S. (2007). Size and taxonomic constraints determine the seed preferences of Carabidae (Coleoptera). Basic Appl. Ecol. 8, 343–353. doi: 10.1016/j.baae.2006.07.002
Hullé, M., Chaubet, B., Turpeau, E., and Simon, J.-C. (2020). Encyclop’Aphid: a website on aphids and their natural enemies. Entomol. Gen. 40, 97–101. doi: 10.1127/entomologia/2019/0867
Jordan, V. W. L., and Hutcheon, J. A. (2002). “Influence of Cultivation Practices on Arable Crop Diseases,” in Soil Tillage in Agroecosystems, ed. A. E. Titi (Boca Raton, FL: CRC Press), 187–205.
Kertész, Á, and Madarász, B. (2014). Conservation Agriculture in Europe. Int. Soil Water Conserv. Res 2, 91–96. doi: 10.1016/S2095-6339(15)30016-2
Kladivko, E. (2001). Tillage systems and soil ecology. Soil Tillage Res. 61, 61–76. doi: 10.1016/S0167-1987(01)00179-9
Kosewska, A., Skalski, T., and Nietupski, M. (2014). Effect of conventional and non-inversion tillage systems on the abundance and some life history traits of carabid beetles (Coleoptera: Carabidae) in winter triticale fields. Eur. J. Entomol. 111, 669–676. doi: 10.14411/eje.2014.078
Kromp, B. (1999). Carabid beetles in sustainable agriculture: a review on pest control efficacy, cultivation impacts and enhancement. Agric. Ecosyst. Environ. 74, 187–228. doi: 10.1016/S0167-8809(99)00037-7
Langellotto, G. A., and Denno, R. F. (2004). Responses of invertebrate natural enemies to complex-structured habitats: a meta-analytical synthesis. Oecologia 139, 1–10. doi: 10.1007/s00442-004-1497-3
Lenth, R. V., Buerkner, P., Herve, M., Love, J., Riebl, H., and Singmann, H. (2020). emmeans: Estimated Marginal Means, aka Least-Squares Means. Madison, WI: R Project.
Letourneau, D., Jedlicka, J., Bothwell, S., and Moreno, C. (2009). Effects of Natural Enemy Biodiversity on the Suppression of Arthropod Herbivores in Terrestrial Ecosystems. Annu. Rev. Ecol. Syst. 40, 573–592. doi: 10.1146/annurev.ecolsys.110308.120320
Losey, J. E., and Denno, R. F. (1998). Positive Predator–Predator Interactions: Enhanced Predation Rates and Synergistic Suppression of Aphid Populations. Ecology 79, 2143–2152.
Losey, J. E., and Vaughan, M. (2006). The Economic Value of Ecological Services Provided by Insects. BioScience 56, 311–323.
MacArthur, R. H. (1972). Geographical ecology: patterns in the distribution of species. Princeton, NJ: Princeton University Press.
Martin, E. A., Reineking, B., Seo, B., and Steffan-Dewenter, I. (2013). Natural enemy interactions constrain pest control in complex agricultural landscapes. Proc. Natl. Acad. Sci. U.S.A. 110, 5534–5539. doi: 10.1073/pnas.1215725110
Menalled, F. D., Smith, R. G., Dauer, J. T., and Fox, T. B. (2007). Impact of agricultural management on carabid communities and weed seed predation. Agric. Ecosyst. Environ. 118, 49–54. doi: 10.1016/j.agee.2006.04.011
Mesmin, X., Cortesero, A.-M., Daniel, L., Plantegenest, M., Faloya, V., and Le Ralec, A. (2020). Influence of soil tillage on natural regulation of the cabbage root fly Delia radicum in brassicaceous crops. Agric. Ecosyst. Environ. 293:106834. doi: 10.1016/j.agee.2020.106834
Nancarrow, N., Aftab, M., Hollaway, G., Rodoni, B., and Trębicki, P. (2021). Yield Losses Caused by Barley Yellow Dwarf Virus-PAV Infection in Wheat and Barley: A Three-Year Field Study in South-Eastern Australia. Microorganisms 9, 645. doi: 10.3390/microorganisms9030645
Oksanen, J., Blanchet, F. G., Friendly, M., Kindt, R., Legendre, P., McGlinn, D., et al. (2019). vegan: Community Ecology Package. Available online at: https://CRAN.R-project.org/package=vegan (accessed Jan 21, 2021).
Ortiz-Martínez, S., Staudacher, K., Baumgartner, V., Traugott, M., and Lavandero, B. (2020). Intraguild predation is independent of landscape context and does not affect the temporal dynamics of aphids in cereal fields. J. Pest. Sci. 93, 235–249. doi: 10.1007/s10340-019-01142-4
Ortiz-Martínez, S. A., and Lavandero, B. (2018). The effect of landscape context on the biological control of Sitobion avenae: temporal partitioning response of natural enemy guilds. J. Pest. Sci. 91, 41–53. doi: 10.1007/s10340-017-0855-y
Potter, K. N., Cruse, R. M., and Horton, R. (1985). Tillage Effects on Soil Thermal Properties. Soil Sci. Soc. Am. J. 49, 968–973. doi: 10.2136/sssaj1985.03615995004900040035x
Pretorius, R. J., Hein, G. L., Blankenship, E. E., Purrington, F. F., Wilson, R. G., and Bradshaw, J. D. (2018). Comparing the Effects of Two Tillage Operations on Beneficial Epigeal Arthropod Communities and Their Associated Ecosystem Services in Sugar Beets. J. Econ. Entomol. 111, 2617–2631. doi: 10.1093/jee/toy285
Puech, C., Poggi, S., Baudry, J., and Aviron, S. (2015). Do farming practices affect natural enemies at the landscape scale? Landsc. Ecol. 30, 125–140. doi: 10.1007/s10980-014-0103-2
Purvis, G., and Fadl, A. (1996). Emergence of Carabidae (Coleoptera) from pupation: a technique for studying the “productivity” of carabid habitats. Ann. Zool. Fenn. 33, 215–223.
Purvis, G., Fadl, A., and Bolger, T. (2001). A multivariate analysis of cropping effects on Irish ground beetle assemblages (Coleoptera: Carabidae) in mixed arable and grass farmland. Ann. Appl. Biol. 139, 351–360. doi: 10.1111/j.1744-7348.2001.tb00149.x
R Core Team. (2019). R: A Language and Environment for Statistical Computing. Vienna: R Foundation for Statistical Computing.
Rautapää, J. (1966). The effect of the english grain aphid Macrosiphum avenae (F.) (Hom. Aphididae) on the yield and quality of wheat. Ann. Agric. Fenn. Ser. Anim. Nocentia Hels. 5, 334–341.
Reich, I., Jessie, C., Ahn, S.-J., Choi, M.-Y., Williams, C., Gormally, M., et al. (2020). Assessment of the Biological Control Potential of Common Carabid Beetle Species for Autumn- and Winter-Active Pests (Gastropoda, Lepidoptera, Diptera: Tipulidae) in Annual Ryegrass in Western Oregon. Insects 11:722. doi: 10.3390/insects11110722
Ribera, I., Dolédec, S., Downie, I. S., and Foster, G. N. (2001). Effect of Land Disturbance and Stress on Species Traits of Ground Beetle Assemblages. Ecology 82, 1112–1129.
Roger, J.-L., Jambon, O., and Bouger, G. (2013). Clé de détermination des carabides. Canada: Nord Ouest de la France.
Rusch, A., Valantin-Morison, M., Sarthou, J.-P., and Roger-Estrade, J. (2010). “Chapter six - Biological Control of Insect Pests in Agroecosystems: Effects of Crop Management, Farming Systems, and Seminatural Habitats at the Landscape Scale: A Review,” in Advances in Agronomy, ed. D. L. Sparks (Cambridge, MA: Academic Press), 219–259. doi: 10.1016/B978-0-12-385040-9.00006-2
Saska, P., Werf, W., van der Vries, E., and de Westerman, P. R. (2008). Spatial and temporal patterns of carabid activity-density in cereals do not explain levels of predation on weed seeds. Bull. Entomol. Res. 98, 169–181. doi: 10.1017/S0007485307005512
Scaccini, D., Panini, M., Chiesa, O., Nicoli Aldini, R., Tabaglio, V., and Mazzoni, E. (2020). Slug monitoring and impacts on the ground beetle community in the frame of sustainable pest control in conventional and conservation agroecosystems. Insects 11:380. doi: 10.3390/insects11060380
Schmidt, M. H., Thewes, U., Thies, C., and Tscharntke, T. (2004). Aphid suppression by natural enemies in mulched cereals. Entomol. Exp. Appl. 113, 87–93. doi: 10.1111/j.0013-8703.2004.00205.x
Schmidt, N. P., O’Neal, M. E., and Singer, J. W. (2007). Alfalfa living mulch advances biological control of soybean aphid. Environ. Entomol. 36, 416–424. doi: 10.1603/0046-225x(2007)36[416:almabc]2.0.co;2
Seric Jelaska, L., Franjevic, D., Jelaska, S. D., and Symondson, W. O. C. (2014). Prey detection in carabid beetles (Coleoptera: Carabidae) in woodland ecosystems by PCR analysis of gut contents. Eur. J. Entomol. 111, 631–638. doi: 10.14411/eje.2014.079
Shearin, A. F., Reberg-Horton, S. C., and Gallandt, E. R. (2007). Direct Effects of Tillage on the Activity Density of Ground Beetle (Coleoptera: Carabidae) Weed Seed Predators. Environ. Entomol. 36, 1140–1146. doi: 10.1603/0046-225x(2007)36[1140:deotot]2.0.co;2
Snyder, W. E. (2019). Give predators a complement: Conserving natural enemy biodiversity to improve biocontrol. Biol. Control 135, 73–82.
Snyder, W. E., and Ives, A. R. (2003). Interactions Between Specialist and Generalist Natural Enemies: Parasitoids, Predators, and Pea Aphid Biocontrol. Ecology 84, 91–107.
Soane, B. D., Ball, B. C., Arvidsson, J., Basch, G., Moreno, F., and Roger-Estrade, J. (2012). No-till in northern, western and south-western Europe: A review of problems and opportunities for crop production and the environment. Soil Tillage Res. 118, 66–87. doi: 10.1016/j.still.2011.10.015
Staudacher, K., Rubbmark, O. R., Birkhofer, K., Malsher, G., Sint, D., Jonsson, M., et al. (2018). Habitat heterogeneity induces rapid changes in the feeding behaviour of generalist arthropod predators. Funct. Ecol. 32, 809–819.
Stein, A., Gerstner, K., and Kreft, H. (2014). Environmental heterogeneity as a universal driver of species richness across taxa, biomes and spatial scales. Ecol. Lett. 17, 866–880. doi: 10.1111/ele.12277
Straub, C. S., and Snyder, W. E. (2008). Increasing enemy biodiversity strengthens herbivore suppression on two plant species. Ecology 89, 1605–1615. doi: 10.1890/07-0657.1
Sunderland, K. D., and Vickerman, G. P. (1980). Aphid Feeding by Some Polyphagous Predators in Relation to Aphid Density in Cereal Fields. J. Appl. Ecol. 17, 389–396. doi: 10.2307/2402334
Tamburini, G., De Simone, S., Sigura, M., Boscutti, F., and Marini, L. (2016). Conservation tillage mitigates the negative effect of landscape simplification on biological control. J. Appl. Ecol. 53, 233–241. doi: 10.1111/1365-2664.12544
Tenailleau, M., Dor, C., and Maillet-Mezeray, J. (2011). Synthèse bibliographique sur l’écologie des Carabidae 73. Seoul: Etude House.
Tews, J., Brose, U., Grimm, V., Tielbörger, K., Wichmann, M. C., Schwager, M., et al. (2004). Animal species diversity driven by habitat heterogeneity/diversity: the importance of keystone structures. J. Biogeogr. 31, 79–92. doi: 10.1046/j.0305-0270.2003.00994.x
Thies, C., Haenke, S., Scherber, C., Bengtsson, J., Bommarco, R., Clement, L. W., et al. (2011). The relationship between agricultural intensification and biological control: experimental tests across Europe. Ecol. Appl. 21, 2187–2196. doi: 10.1890/10-0929.1
Thorbek, P., and Bilde, T. (2004). Reduced numbers of generalist arthropod predators after crop management. J. Appl. Ecol. 41, 526–538. doi: 10.1111/j.0021-8901.2004.00913.x
Tonhasca, A. (1993). Carabid beetle assemblage under diversified agroecosystems. Entomol. Exp. Appl. 68, 279–285. doi: 10.1111/j.1570-7458.1993.tb01714.x
Traugott, M., Bell, J. R., Raso, L., Sint, D., and Symondson, W. O. C. (2012). Generalist predators disrupt parasitoid aphid control by direct and coincidental intraguild predation. Bull. Entomol. Res. 102, 239–247. doi: 10.1017/S0007485311000551
Keywords: conservation agriculture, biological control, aphid, sentinel prey, parasitism, aphidophagy
Citation: Sacco–Martret de Préville A, Ortiz-Martinez S, Plantegenest M and Canard E (2022) Effect of Conservation Agriculture on Aphid Biocontrol by Generalist (Carabid Beetle) and Specialist (Parasitoids Wasp) Natural Enemy Communities in Winter Wheat. Front. Ecol. Evol. 10:893787. doi: 10.3389/fevo.2022.893787
Received: 10 March 2022; Accepted: 20 June 2022;
Published: 18 July 2022.
Edited by:
James Moran, Atlantic Technological University, IrelandReviewed by:
Gabor Pozsgai, University of the Azores, PortugalAbid Ali, University of Agriculture, Faisalabad, Pakistan
Copyright © 2022 Sacco–Martret de Préville, Ortiz-Martinez, Plantegenest and Canard. This is an open-access article distributed under the terms of the Creative Commons Attribution License (CC BY). The use, distribution or reproduction in other forums is permitted, provided the original author(s) and the copyright owner(s) are credited and that the original publication in this journal is cited, in accordance with accepted academic practice. No use, distribution or reproduction is permitted which does not comply with these terms.
*Correspondence: Elsa Canard, ZWxzYS5jYW5hcmRAaW5yYWUuZnI=
†These authors share last authorship