- 1Department of Zoology and Fisheries, Faculty of Agrobiology, Food and Natural Resources, Czech University of Life Sciences, Prague, Czechia
- 2Faculty of Fisheries and Protection of Waters, South Bohemia Research Center of Aquaculture and Biodiversity of Hydrocenoses, Research Institute of Fish Culture and Hydrobiology, University of South Bohemia in České Budějovice, Vodňany, Czechia
- 3Department of Probability and Mathematical Statistics, Faculty of Mathematics and Physics, Charles University, Prague, Czechia
In laboratory experiments, variously colored strains of animals, including those with albino phenotypes, are commonly used. The melanocortin theory suggests, however, that coloration phenotypes alter animal physiology and behavior. Animals with the albino phenotype show photoreceptor degradation associated with lowered visual accuracy, escape reactions, etc., presumably accompanied by prevailing nocturnal activity and lowered aggressiveness. This assumption was tested in small groups of albino and pigmented European catfish, Silurus glanis, during the diel cycle. The frequency of agonistic interactions was observed during mutual contests for shelters, and subsequently, blood plasma, brain, gill, and liver samples were collected to evaluate stress parameters. In an experimental arena with shelters, the light/dark rhythmicity of locomotor activity and aggressiveness of the two phenotypes were comparable; the peak was observed at night, and a lower peak was observed at dawn. In an experimental stream without shelters, the peak of locomotor activity occurred at night for only the pigmented phenotype. In the evaluation of 4 antioxidants and 1 oxidative stress indicator, representing a total of 15 indices, albino fish showed significant rhythmicity for 8 indices, whereas pigmented catfish showed significant rhythmicity for 5 indices. The production of blood stress parameters with the peak during the day occurred only in albino fish. A complex model was fitted with the aim of evaluating the links between behavioral and biochemical indices. Time periodicity was modeled using a sine wave and confirmed parallel courses of agonistic interactions in the catfish groups; the peak at dawn was associated with a 4.08-fold (conf. int. 3.53–4.7) increase in such interactions. The changes in glucose and superoxide dismutase concentrations varied with phenotype, while the effects of cortisol, lactate and catalase did not. In summary, the rhythmicity of locomotor activity and changes in the aggressiveness of catfish were influenced by shelter availability, and the effect of light-induced stress was more apparent in albino fish than in pigmented conspecific fish. The results suggested that laboratory-raised animals with pigmentation patterns naturally occurring in the wild show more reasonable values during experiments than those with an albino phenotype.
Introduction
In laboratory experiments, variously colored strains of animals, including those with albino phenotypes, are commonly used. The melanocortin theory suggests, however, that coloration phenotypes alter animal physiology and behavior (Ducrest et al., 2008; Santostefano et al., 2019). Albino animals exhibit physiological limitations that separate their behavior during the diurnal cycle from that of pigmented conspecifics. Ocular anomalies related to retinal degradation are most frequently mentioned (Oetting, 2000; Prusky et al., 2002). A lack of melanin can lead to an underdeveloped central part of the retina adapted for high-acuity vision (fovea centralis) and a reduction in rod number (Jeffery, 1997). Physiological changes are followed by reduced visual acuity (Balkema and Dräger, 1991; Buhusi et al., 2005), impaired perception of movement (Hupfeld and Hoffmann, 2006) and higher sensitivity to light (van Abeelen and Kroes, 1967; Kehas et al., 2005). The eyes of albino rodents show reduced adaptation to light, often leading to photoreceptor degradation (Prusky et al., 2002; Refinetti, 2007; Marc et al., 2008), which in turn can cause loss of vision (Buhusi et al., 2005) and, eventually, photophobia and acrophobia (van Abeelen and Kroes, 1967; Owen et al., 1970). Albino animals are less active (Defries, 1969) with prevailing nocturnal activity (Stryjek et al., 2013), and deteriorated spatial orientation is probably the reason why, e.g., albino fish, show a reduced escape response (Ren et al., 2002).
Light is an important zeitgeber that controls diurnal rhythms through the melanocortin system (Sánchez-Vázquez et al., 1997; Oliveira et al., 2013). Light information passes through the retina to the pineal organ and initiates the secretion of melatonin hormone, which is released to blood in low concentrations during the day and high concentrations during the night, thereby controlling the daily patterns of behavior (López-Olmeda et al., 2009; Oliveira et al., 2009; Sánchez-Vázquez et al., 2019). Fish specifically respond to light alternation by showing diurnal, nocturnal or crepuscular daily patterns of behavior (Madrid et al., 2001). The locomotor activity associated with light oscillation may be altered, however, due to food intake (Sánchez-Vázquez et al., 1995, 1997), the number of individuals (Boujard, 1995; Vera et al., 2011) or social relationships (Alanärä et al., 2001; Brännäs, 2008), and individuals can switch their activity from diurnal to nocturnal and vice versa or show dual activity (Alanärä and Brännäs, 1997; Vera et al., 2009; Slavík and Horký, 2012).
Light conditions are associated with the level of aggressiveness (Britz and Pienaar, 1992; Almazán-Rueda et al., 2004). Increasing melatonin secretion during low light intensities attenuates the activity and aggressiveness of organisms (Almazán-Rueda et al., 2004; Carvalho et al., 2012). Biological interpretation of a decrease in aggressiveness during the dark phase suggests that, e.g., fish, do not see each other, as well as prey, and hence, stimuli to be aggressive are waned (Valdimarsson and Metcalfe, 2001; Lopes et al., 2018). Agonistic interactions are accompanied by stress that can be observed as changes in blood plasma parameters (Pottinger and Carrick, 2001; Sloman et al., 2001), e.g., cortisol hormone concentration (Pickering, 1992; Øverli et al., 2004) and brain antioxidant activity (Costantini et al., 2011; Chowdhury and Saikia, 2020). For example, a lower level of cortisol is reported in dominant and more aggressive fish than in subordinate and less aggressive fish (Koolhaas et al., 1999; Sloman et al., 2001; Øverli et al., 2004). Agonistic behavior is a metabolically demanding process (Haller, 1995; Copeland et al., 2011) requiring mobilization of glucose from storage glycogen, leading to its increase in the blood (Fernandez et al., 1994); hence, it is accompanied by hepatic glycogen reduction and an increase in the glucose level in the plasma (Haller, 1991; Neat et al., 1998) that is higher for subdominant individuals and losers (Elofsson et al., 2000; Copeland et al., 2011). The cortisol level also corresponds with the light/dark (L/D) cycle and feeding schedule, while the glucose level response is weaker (Polakof et al., 2007; López-Olmeda et al., 2009; Oliveira et al., 2013). The activity of antioxidants in fish brains is reported as an oxidative stress, which is caused by an imbalance between harmful free radicals and antioxidants neutralizing these radicals (Chowdhury and Saikia, 2020). Oxidative stress can occur after acute stress as a response to increased glucocorticoids, which may induce oxidative stress by increasing the metabolic rate and thus free radicals (Costantini et al., 2011). The rise in antioxidant activity is associated with changes in light (Sreejith et al., 2007; Kim et al., 2016) and the level of aggressiveness (Almeida et al., 2009). In summary, the L/D cycle influences fish behavior, which can be recorded as changes in locomotor activity and aggressiveness accompanied by changes in physiological parameters in blood plasma and antioxidant activity.
Animals, including fish, with a higher level of melanin are more aggressive and dominant (Horth, 2003; Kittilsen et al., 2009) and vice versa; i.e., less pigmented individuals are more sensitive to stress and are often subdominant (Höglund et al., 2000; Kittilsen et al., 2012). In fish, individuals with an albino phenotype show lower aggressiveness, often being ostracized by pigmented conspecifics (Slavík et al., 2015, 2016b), and display higher sensitivity to stress (Slavík et al., 2022) than those with the pigmented phenotype. Data on the behavioral response of albino animals to light alterations during the L/D cycle are rare, and specific information about albino fish is not available.
In our study, we observed the locomotor activity and aggressiveness of albino and pigmented European catfish, Silurus glanis (Linnaeus, 1758), during the L/D cycle. We assumed that the locomotor activity of the albino phenotype would occur predominantly during the dark phase of a day and the frequency of aggressiveness would be higher during the light phase, corresponding to increasing avoidance of light and shelter availability. Blood plasma, brain, gill, and liver samples were collected to evaluate stress parameters accompanying aggressive behavior. Our study aimed to compare the behaviors of albino and pigmented phenotypes of catfish in terms of expected differences in aggressiveness during the L/D cycle. We issue a word of caution regarding the use of experimental albino strains of animals for the simulation of behavioral responses of animals in the wild.
Materials and Methods
Ethics Statements
All the experimental laboratory procedures complied with valid legislative regulations (Law no. 246/1992, §19, art. 1, letter c), which was derived from the Directive 2010/63/EU; the permit (no. CZ02233) was awarded to O. Slavík, qualified according to Law no. 246/1992, §17, art. 1. All laboratory sampling, including passive integrated transponder (PIT) implantation, was carried out with relevant permission (63479/2016-MZE-17214). The maintenance staff were trained by law in animal care to maintain the high quality of the experiment. The number of experimental animals and all methods used complied with the reduction, replacement, and refinement of animal experimentation. The study did not involve endangered species.
Experimental Animals
The European catfish is a large predator currently widely distributed outside its native range. Albino individuals are observed in the wild, and juveniles are commonly used for laboratory experiments (Dingerkus et al., 1991; Lechner and Ladich, 2011). Two groups of 7-month-old juvenile catfish from the Znojmo hatchery, Czechia, were transported to the laboratory 1 month prior to the start of the experiments. Both groups, the group of pigmented individuals (160 inds) and the group of albino individuals (160 inds), were from different pigmented parents. In the laboratory, experimental animals were further divided into 8 groups (4 albino and 4 pigmented groups), with 40 individuals each, that were kept in separate tanks (380 L each) for 1 month. Fish were placed under general anesthesia (2-phenoxyethanol, water bath concentration 0.2 ml × l−1), tagged with PITs with a unique code and kept under standard conditions. Water was purified using biological filters with an integrated UV sterilizer (Pressure-Flo 5000, Rolf C. Hagen Inc.1). Water temperature was controlled automatically using external air conditioning and held at an average of 20°C. Light was controlled to a 12-h day/12-h night regime. Fish were fed ad libitum with BioMar pellets (BioMar Group, Denmark2) once per day. Once per day, the tank was manually cleaned of unconsumed feed and other solid residuals. Each individual was used only once during the experiment.
Experimental Design
Experiment I: Locomotor Activity in an Experimental Stream Without Shelters
A total of 36 albino (mean weight 41.4 g, range 23–30 g) and 36 pigmented (mean weight 42.8 g, range 28–93 g) randomly selected juvenile catfish were used in this experiment. No significant size differences between the pigmented and albino catfish (P > 0.4) were detected. Locomotor activity was measured in a straight artificial stream (260 cm long, 70 cm wide, and 40 cm deep) equipped with a PIT system according to previous measures of catfish activity (Slavík et al., 2016a). The stream was divided into five subunits by using four equidistantly distributed PIT antennae. The antennae (inner area 49 cm × 25 cm) were designed as frames capable of detecting an individual swimming through them. There was no space between the antenna and the wall of the stream as antennas were inserted in a specific partition with a gap corresponding to the size of the antenna in the middle. The antennae were connected to a recorder to store collected data (PIT tag code, date, time and antenna number) in the internal memory. A group of four same-colored fish from the holding tank were released at the same end of the artificial stream and left there to habituate for 24 h. Their locomotor activity, considered throughout the manuscript as a spontaneous movement in the safe environment (Réale et al., 2007; Conrad et al., 2011) was continuously observed for the next 24 h, resulting in nine separate trials for albino catfish and nine separate trials for pigmented catfish (albino and pigmented catfish trials were regularly rotated). The whole experimental stream was cleaned and rinsed thoroughly after each trial. Fish were kept under a photoperiod of 12 h of light/12 h of darkness during the experiment, allowing us to distinguish between the day and night.
Experiment II: Locomotor Activity, Aggressiveness, and Shelter Reassignment in the Experimental Arena With a Shelter
A total of 56 albino (mean weight 28.2 g, range 20–43 g) and 56 pigmented (mean weight 28.3 g, range 12–49 g) randomly selected juvenile catfish were used in this experiment. No significant size differences between the pigmented and albino catfish (P > 0.85) were detected. The behavioral features (locomotor activity, aggressiveness, and shelter reassignment) were observed in a square experimental arena (footprint 30 cm × 30 cm) with one tube shelter (5 cm × 15 cm) placed in the middle of the arena. Fish were exposed to the same shelters in holding tanks, and the provision of only one shelter was chosen to stimulate competition for limited resources. Catfish behavior was recorded with a Bosch Dinion IP Starlight automatic digital camera (Bosch Sicherheitssysteme, GmbH, Germany) that is designed to provide clear images at night or under low-light conditions. A group of four same-colored fish from the same holding tank were released into the experimental arena and left there to habituate for 24 h. Their behavioral features were recorded for the next 24 h, resulting in 14 separate trials for albino catfish and 14 separate trials for pigmented catfish (albino and pigmented catfish trials were regularly rotated). The whole experimental arena was cleaned and rinsed thoroughly after each trial. Fish were kept under a specific photoperiod using custom-made programmable lights allowing simulation of 11.5 h of daylight, followed by a 0.5 h dusk, 11.5 h of darkness, and a 0.5 h dawn. Dawn and dusk were simulated as a stepwise increase/decline in light intensity during six 5-min intervals. Fish behavior was automatically recorded during each of these sampling intervals. Another set of equidistantly distributed evenly long 5-min records were collected during the day as well as during darkness, covering the whole 24 h period. As a result, there was an equal number of sampling intervals during every light interval (i.e., day, dusk, darkness, and dawn) to assure better comparability of the light intervals in further analyses.
Experiment III: Physiological Patterns in the Experimental Arena With a Shelter
The experiment followed the same design and used the same conditions as Experiment II, with the aim of observing physiological parameters. During the experiment, we collected blood and tissue (brain, gill, and liver) samples from tested individuals. In total, 32 albino (mean weight 47.2 g, range 25–97 g) and 32 pigmented (mean weight 53.1 g, range 29–86 g) randomly selected juvenile catfish were used in this experiment. No significant size differences were detected between the pigmented and albino catfish (P > 0.15). A group of four same-colored fish from the same holding tank were released into the experimental arena and left there to habituate for 24 h. Blood and tissues were sampled during the following 24 h in eight equidistantly distributed sampling intervals (three during daytime, one at dusk, three during darkness, and one at dawn). All four individuals in a group were sampled at the same time within each trial and the trial was terminated after sampling, resulting in eight trials/intervals of four individuals for albino catfish and eight trials/intervals of four individuals for pigmented catfish (albino and pigmented catfish trials were regularly rotated). The whole experimental arena was cleaned and rinsed thoroughly after every trial. Catfish blood was sampled from the vena caudalis using a heparinized syringe (heparin inj., Léčiva, Czechia) according to the unified methods for the hematological examination of fish (Borges et al., 2007; Sudová et al., 2009). For biochemical plasma analysis, blood was separated via centrifugation at 12,000 × g for 10 min at 4°C, and plasma samples were stored at −80°C until analysis. Cortisol, alkaline phosphatase (ALP), alanine aminotransferase (ALT), aspartate aminotransferase (AST), calcium (Ca), phosphorus (P), glucose (GLU), magnesium (Mg), triglyceride (TRIG), creatine kinase (CK), lactate (LAC), amylase (AMYL), lipase (LIPA), albumin (ALB) and globulin (GLOB) concentrations were measured using a VETTEST 8008 analyzer (IDEXX Laboratories Inc., Westbrook, ME, United States; Gallardo et al., 2010; Li et al., 2011). After blood sampling, fish were euthanized by severing the spinal cord, and samples of the brain, gills and liver were taken for an evaluation of oxidative stress. Samples for evaluation of oxidative stress biomarkers and antioxidant enzymes were homogenized and prepared for analysis according to Stara et al. (2013). Thiobarbituric acid reactive substances (TBARSs) were estimated according to the method of Lushchak et al. (2005), while total superoxide dismutase (SOD) activity was estimated by the method of Marklund and Marklund (1974). Catalase (CAT) activity was assayed using the method of Beers and Sizer (1952). Reduced glutathione (GSH) levels were assayed using the methods of Sedlak and Lindsay (1968) with the modification reported by Ferrari et al. (2007). The total catalytic concentration of glutathione-S-transferase (GST) was determined according to Habig et al. (1974). Protein levels were estimated spectrophotometrically by the method of Bradford (1976), using bovine serum albumin as a standard.
Data Analyses
Data from all experiments (Figure 1) were analyzed using the light intervals approach, distinguishing for ecologically relevant light levels across 24 h (e.g., Slavík et al., 2007), to facilitate comparability and mutual interpretation of various datasets. The datasets generated for this study can be found in the Dryad repository (Valchářová et al., 2022).
Experiment I
Over 160,000 raw data points were obtained during the artificial stream experiment. The “locomotor activity” of each fish was calculated as the number of artificial stream units passed within the 1-min time grid according to the activity measures of juvenile chub (Horký et al., 2014; Hubená et al., 2020) or catfish (Slavík et al., 2016a). All the values were subsequently grouped into four equidistantly distributed sampling intervals (two during the day and two during night) with the mean individual locomotor activity values. One pigmented catfish trial was not included in the final dataset due to technical difficulties, resulting in a total of eight pigmented and nine albino catfish trials.
Experiment II
Aggressive interactions that were accounted for included biting, chasing and frontal and lateral displays as described for catfish by Slavík et al. (2016b). The sum of all aggressive interactions within each group of four individuals, referred to as “aggressiveness,” was used in further analyses. Altogether, 724 aggressive interactions were detected. Locomotor activity was defined as the total number of all movements of the four individuals in a group. Altogether, 7246 movements were detected. “Shelter reassignment” was defined as the total number of reassignments of shelters within a group. Altogether, 131 shelter reassignments were recorded. Diel behavioral patterns were analyzed across four light intervals (dawn, day, dusk, and night). One albino catfish trial was not included in the final dataset due to technical difficulties, resulting in a total of 14 pigmented and 13 albino catfish trials.
Experiment III
Each physiological sample was successfully analyzed, resulting in 64 data points in the final dataset used to analyze diel physiological patterns across four light intervals (dawn, day, dusk, and night). Data from Experiments II and III were consequently merged to evaluate the links between behavioral and biochemical indices. For the purpose of this complex analysis, the “aggressiveness” and “shelter reassignment” variables were summed to form a single variable, “agonistic interactions,” including the total number of all recorded agonistic interactions per minute. The mean values of biochemical indices obtained during Experiment III were assigned corresponding values of agonistic interactions from Experiment II.
Statistical Analysis
The statistical analyses were performed using SAS software (SAS Institute Inc., version 9.43). The data from Experiments I to III were analyzed using separate mixed models with appropriate distributions (continuous variables – normal distribution; count variables – Poisson distribution) and random factors. The data were transformed for normality (logarithmic or square-root transformation) prior to analyses when needed. The random factors were used to account for the repeated measures collected for the same experimental units throughout the experiment. The significance of the explanatory variables was assessed using F tests. The differences between classes were examined with t tests, and Tukey–Kramer adjustment was used for multiple comparisons. The degrees of freedom were calculated using the Kenward–Roger method (Kenward and Roger, 1997).
The links between behavioral and biochemical indices were modeled using R software (R Core Team, 2019). Several different modeling approaches were applied, and a sine profile was found to have the best fit for the overall diel rhythmicity of agonistic interactions. The generalized linear model (GLM) regression framework was then applied, and a model for Poisson data with a logarithmic link was employed. The random effects were included to properly account for the dependence within the experiments and the variability between them. The sine baseline profile was included in the model at first, and the other covariates were added in a forward stepwise manner.
Results
The diel rhythms of the behavioral and biochemical indices generally varied in pigmented and albino catfish (Tables 1, 2). The locomotor activity in the artificial stream without shelters showed no rhythmicity in albino catfish, while pigmented catfish showed a peak during the nocturnal phase of the day (Figure 2). When subjected to limited shelter availability in the experimental arena, pigmented and albino catfish showed similar diel rhythmicities in locomotor activity (Figures 3A,B), aggressiveness (Figures 3C,D), and shelter reassignment (Figures 3E,F). The peak of locomotor activity occurred at night. High-level locomotor activity was also maintained during dawn together with the peak of aggressiveness and shelter reassignment. A nocturnal peak in locomotor activity indicates natural activity patterns, while a dawn peak reflects competition for limited resources (shelter) with the aim of avoiding daylight conditions. The avoidance of daylight was more pronounced in albino catfish, as the presence of a shelter induced their switch to diel rhythmicity, and their stress from daylight was apparent from the peaks in blood cortisol (Figure 4A), glucose (Figure 4B), and lactate (Figure 4C) levels. In contrast, pigmented catfish showed no diel rhythmicity in blood cortisol, glucose, or lactate level. Analogously, significant diel rhythmicity was detected in 11 of the 15 measured blood biochemical indices in albino catfish, while pigmented catfish showed rhythmicity in none of them (Table 1). Among the 4 antioxidants and 1 oxidative stress indicator detected in 3 tissues (brain, gill, and liver), with 15 indices in all, albino catfish showed significant diel rhythmicity in 8 of them, whereas pigmented catfish showed rhythmicity in 5 of them (Table 2). Peaks of antioxidants in the brain (SOD – Figure 5A; CAT – Figure 5B) or liver (SOD, GST, and GSH) of albino catfish were detected during the day, suggesting their relation to daylight stress. On the other hand, antioxidants (GST and GSH) in the gills of albino catfish peaked during dawn, suggesting their relation to the increase of aggressiveness and shelter reassignment. The antioxidants in the liver (SOD) and brain (CAT – Figure 5C) of pigmented catfish peaked at night, suggesting their relation to increased activity, while the antioxidants in gills peaked during the day (SOD) or at dusk (CAT). The oxidative stress indicator TBARS peaked during dusk in both catfish groups; however, in albino catfish, a significant trend was found in the liver, while in pigmented catfish, a significant trend was found in the gills.
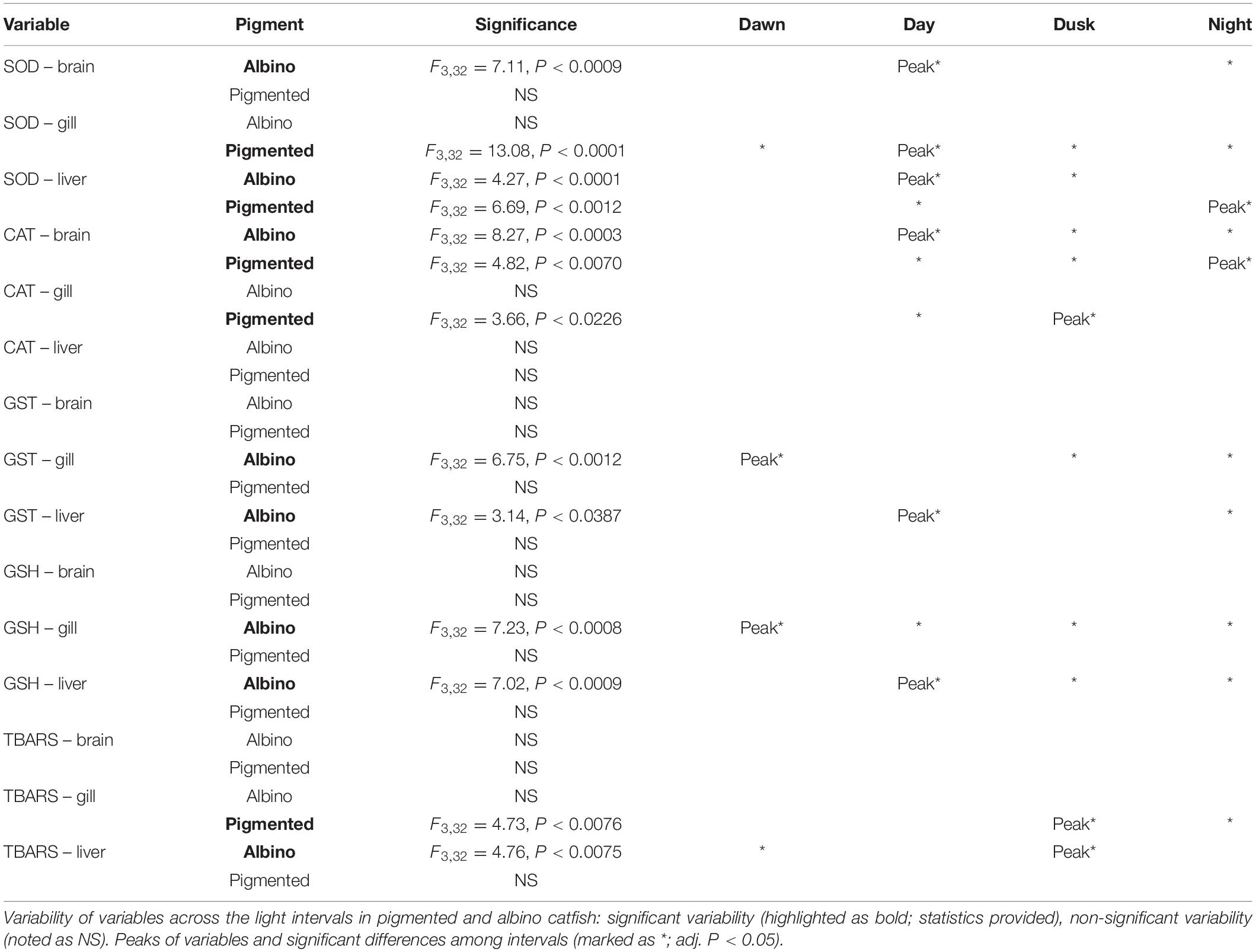
Table 2. Diel rhythms of antioxidants and oxidative stress indicators detected in fish tissues (brain, gill, and liver).
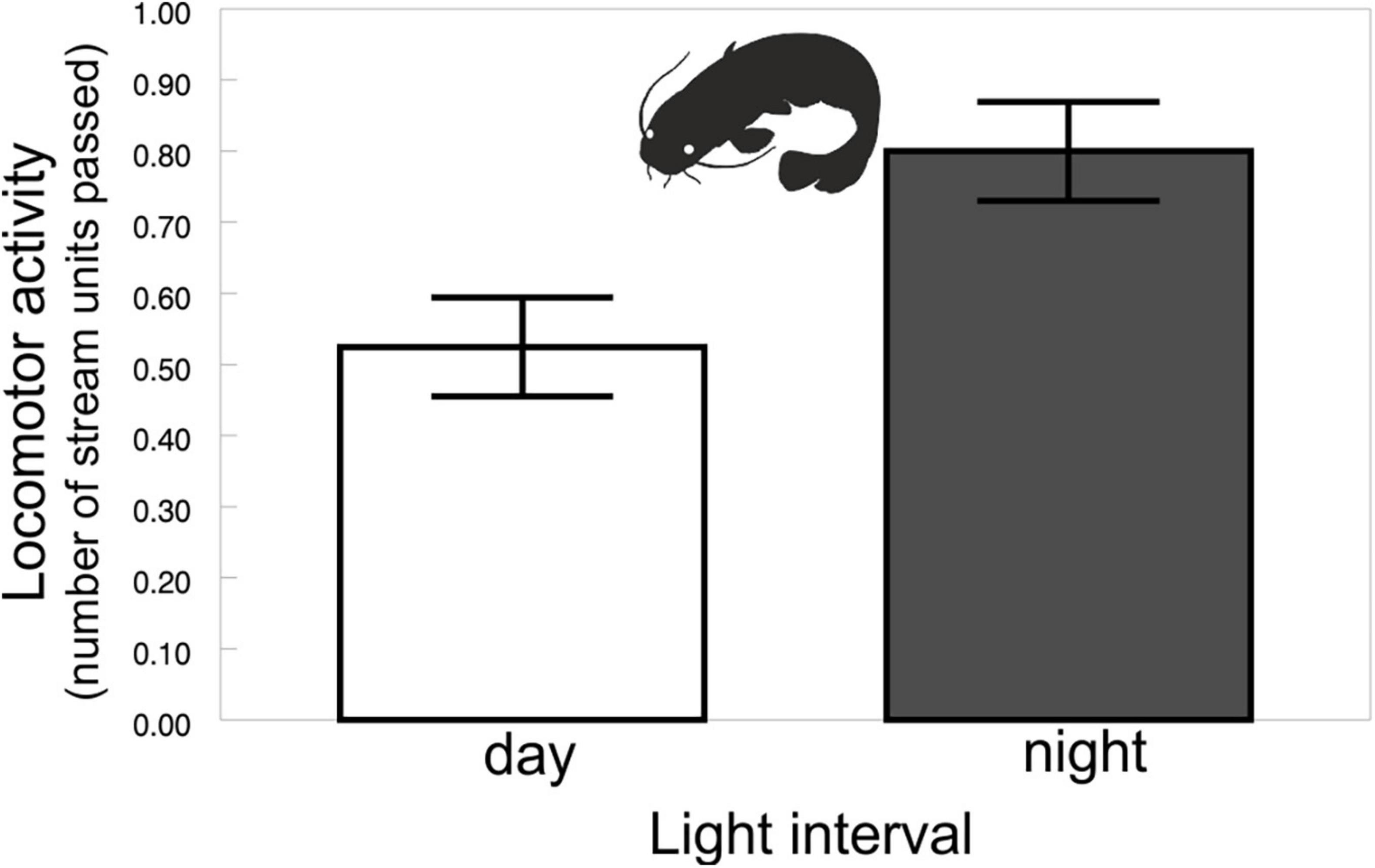
Figure 2. Pigmented catfish locomotor activity in the experimental stream without shelters across light intervals. Values (±SEs) are predicted from the mixed model (i.e., presented data are after the adjustment by the statistical model).
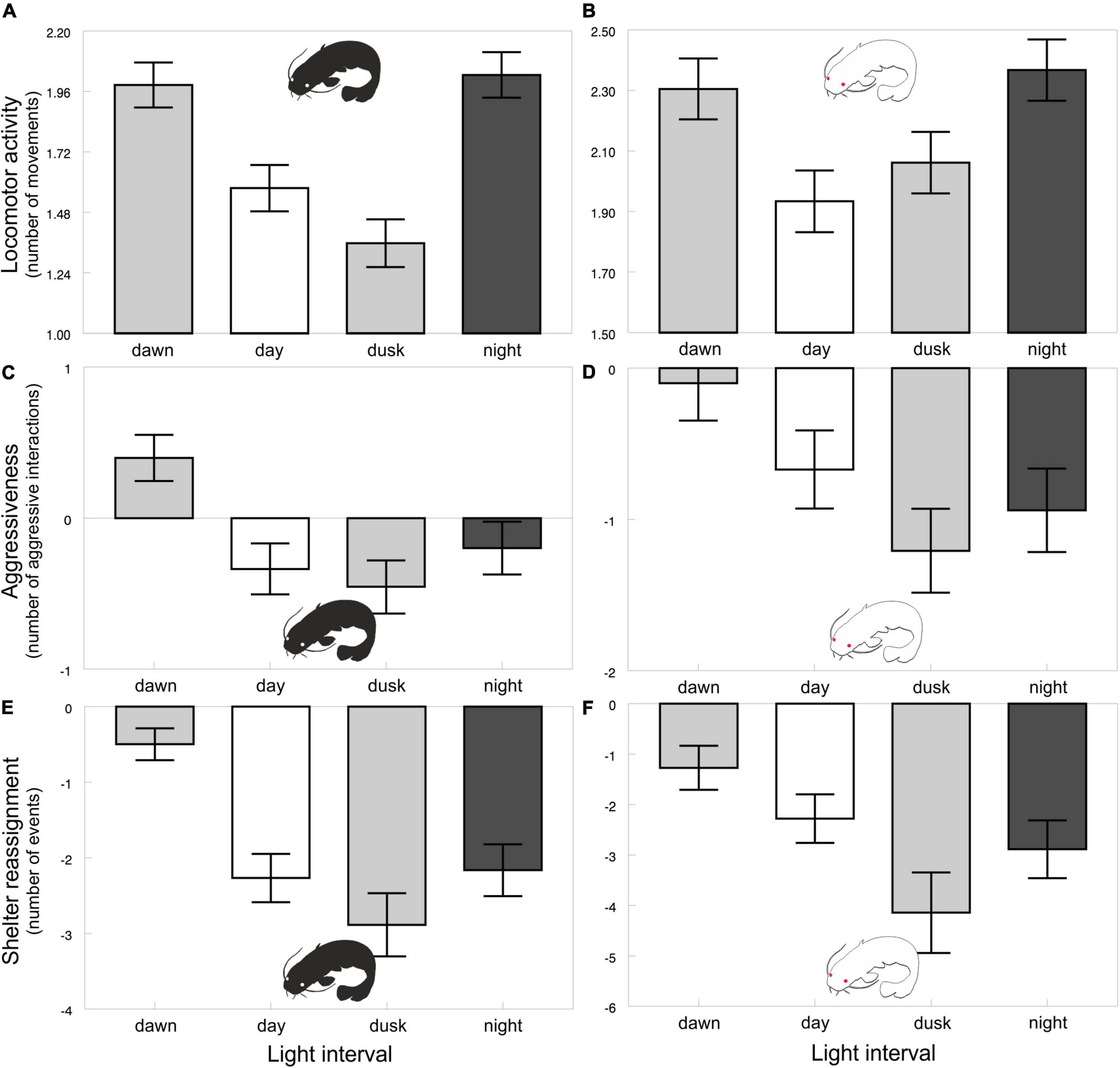
Figure 3. Locomotor activity of pigmented (A) and albino (B) catfish, aggressiveness of pigmented (C) and albino (D) catfish and shelter reassignment of pigmented (E) and albino (F) catfish in the arena with a shelter across light intervals. Values (±SEs) of behavioral indices are predicted from the mixed models (i.e., presented data are after the adjustment by the statistical model).
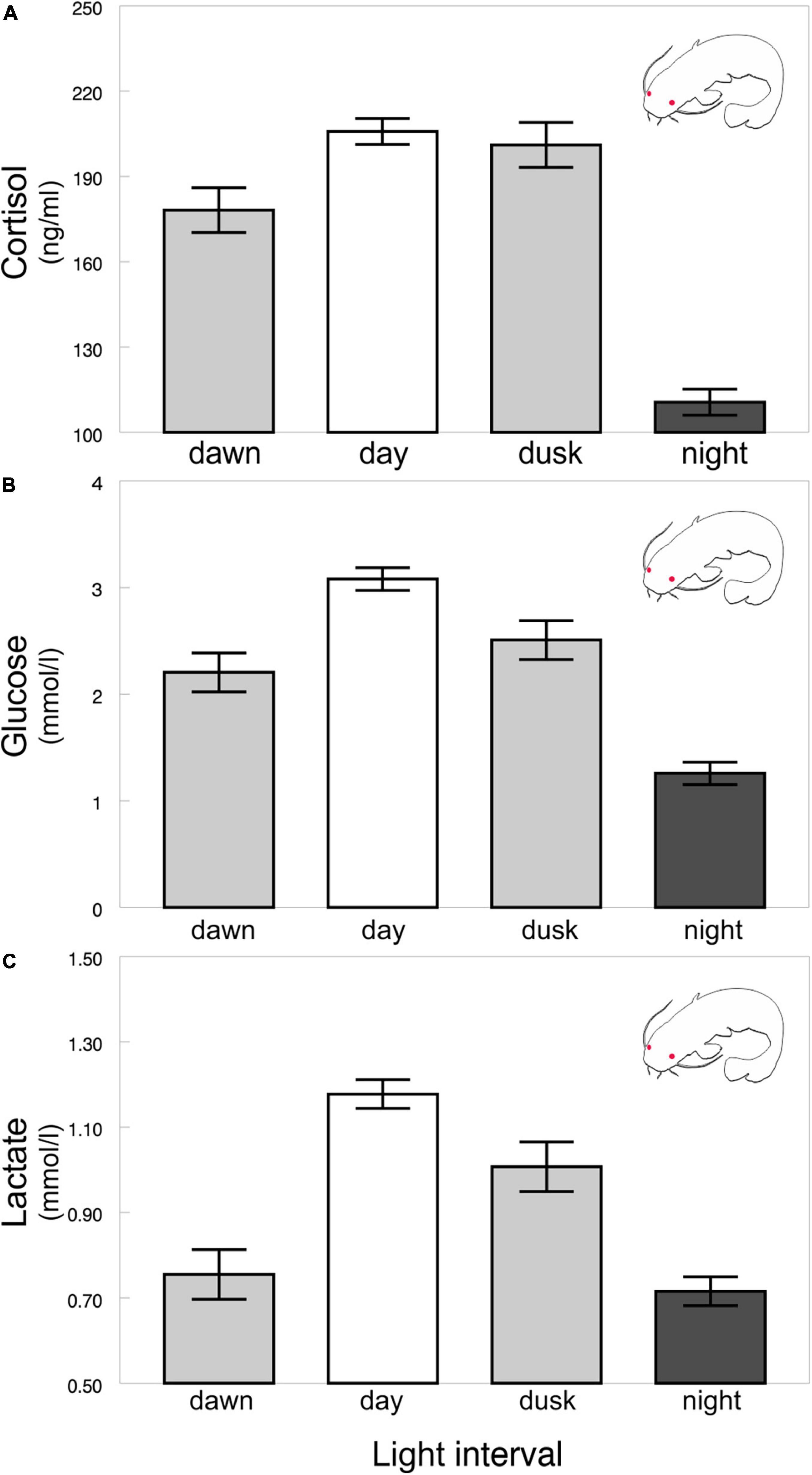
Figure 4. Albino catfish blood cortisol (A), glucose (B), and lactate (C) levels across light intervals. Values (±SEs) of biochemical indices are predicted from the mixed models (i.e., presented data are after the adjustment by the statistical model).
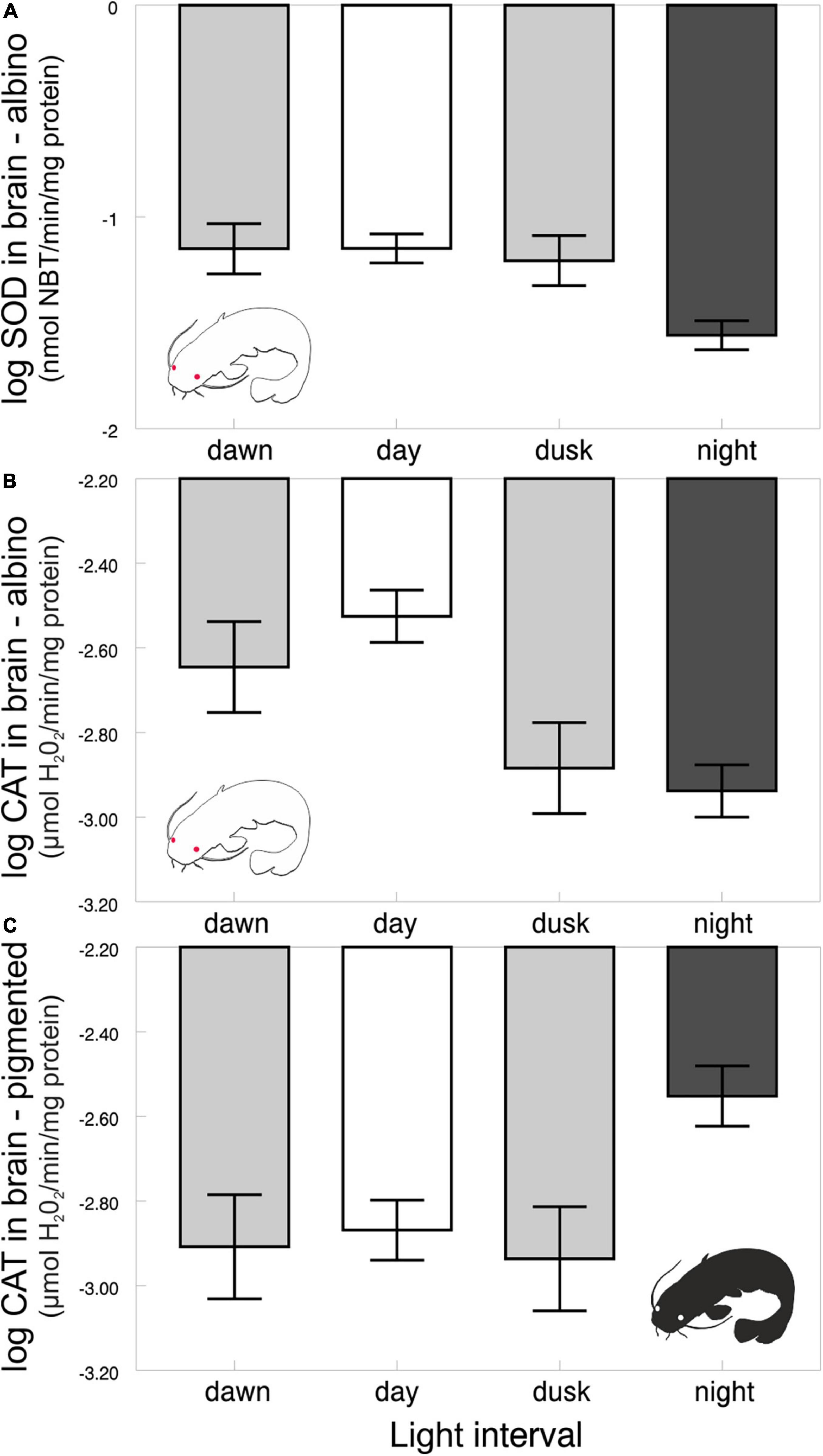
Figure 5. Levels of antioxidants in brain of albino (SOD – A; CAT – B) and pigmented (CAT – C) catfish across light intervals. Values (±SEs) of biochemical indices are predicted from the mixed models (i.e., presented data are after the adjustment by the statistical model).
A complex model was fitted with the aim of evaluating the links between behavioral and biochemical indices. Time rhythmicity was modeled using a sine wave and confirmed a parallel course of agonistic interactions in both catfish groups (P < 0.001; Figure 6), with the peak during dawn associated with a 4.08-fold (conf. int. 3.53–4.7) increase. The effect of SOD concentration in the brain was significant only in pigmented catfish (P < 0.01), in which a one unit increase in SOD (i.e., 10–2 nmol NBT/min/mg protein) was related to a 7% decrease in agonistic interactions. The effect of glucose was significant, but opposite effects were observed in pigmented (P < 0.001) and albino (P < 0.001) catfish. An increase in glucose by one unit (i.e., 10–1 mmol/L) was associated with a 14% decrease in agonistic interactions in albino catfish, while in pigmented catfish, agonistic interactions increased by 342%. The effects of blood cortisol, lactate, and catalase in the brain were significant (P < 0.001) but not influenced by catfish pigmentation. Thus, an increase in cortisol by one unit (i.e., 10–1 ng/ml) caused an increase in agonistic interactions of 177%, while an increase in lactate or catalase in the brain by one unit (i.e., 10–1 ng/ml; resp. 10–2 μmol H2O2/min/mg protein) was related to a 16% (LAC) or 39% (CAT) decrease in agonistic interactions in both catfish groups.
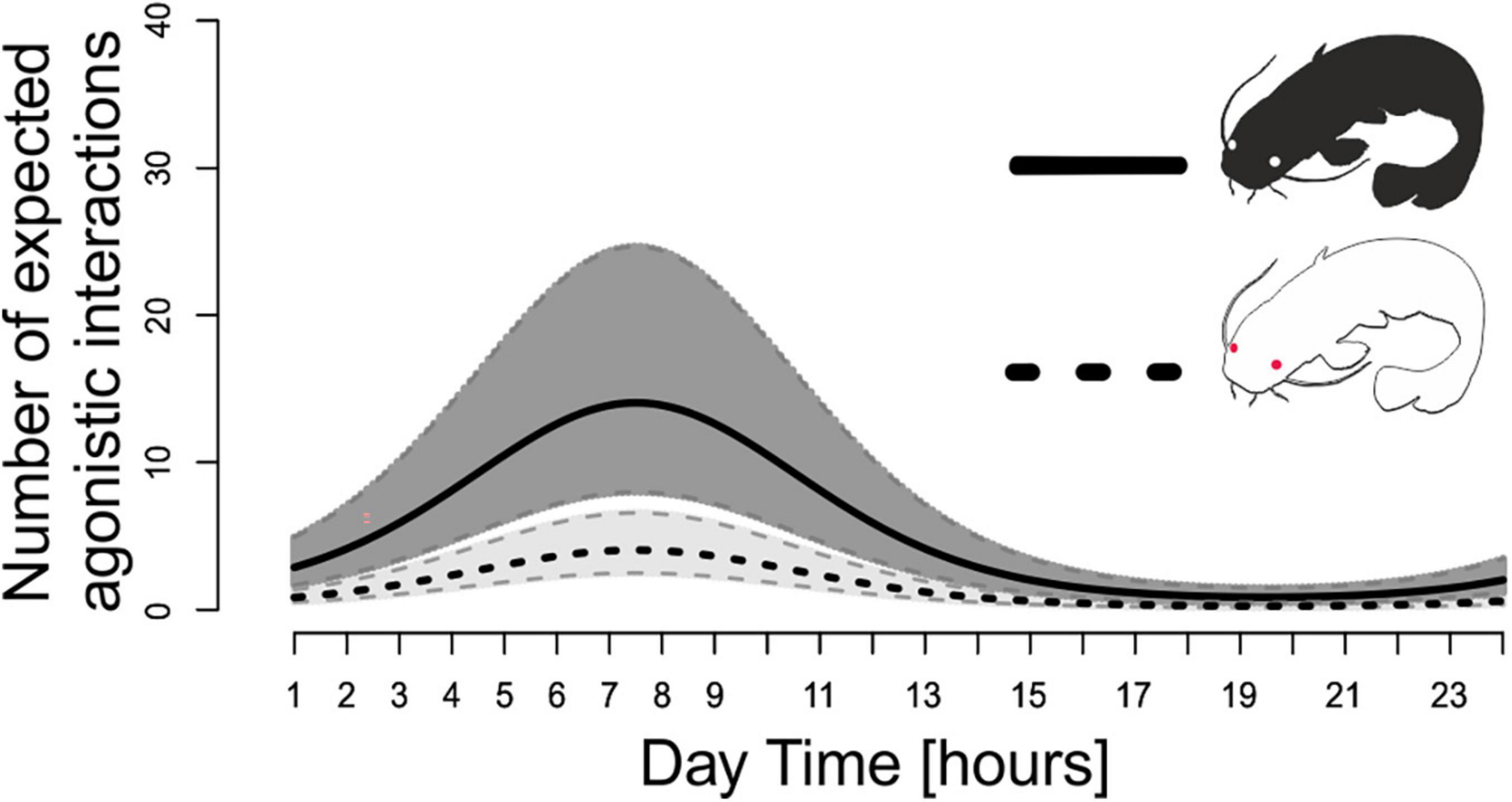
Figure 6. Number of expected agonistic interactions related to sampling interval of albino (dashed black line) and pigmented (solid black line) catfish throughout the diel cycle. Predicted lines are displayed with gray 95% pointwise confidence bands.
Discussion
Albinism is associated with higher light sensitivity and photoreceptor degradation (Prusky et al., 2002; Vihtelic et al., 2006) with behavioral repercussions (Ren et al., 2002). For example, increased sensitivity to sunlight was reported for albino Caribbean Sea urchins, Tripneustes ventricosus (Lamarck, 1816), which tend to cover themselves with plants more than pigmented individuals (Kehas et al., 2005). In albino rodents, avoidance of light and lower locomotor activity during daylight are generally known behavioral characteristics (Fuller, 1967; Defries, 1969; Stryjek et al., 2013), but information about the physiological processes underlying photophobia is rare, e.g., in the albino cave tetra Astyanax mexicanus (De Filippi, 1853), higher sensitivity to stress is associated with mutation of the oca2 gene and catecholamine synthesis (Kvetnansky et al., 2009; Bilandžija et al., 2013). In our study, we observed differences in behavior between albino and pigmented European catfish, S. glanis, during the L/D cycle influenced by the complexity of the environment. These differences were accompanied by changes in physiological parameters in blood plasma and the brain, gill, and liver. In nature, the locomotor activity of pigmented S. glanis follows the L/D cycle, being mostly nocturnal; however, it is highly variable across seasons (Slavík et al., 2007). Moreover, the activity of S. glanis is highly individualistic; some individuals show nocturnal activity, some show daylight activity, and some do not prefer any phase of the L/D cycle (Slavík and Horký, 2012), as commonly reported for other fish species (Madrid et al., 2001; Vera et al., 2009; Sánchez-Vázquez et al., 2019). Under experimental conditions, the locomotor activity and food intake of S. glanis during the L/D cycle are affected by the social environment; for example, individually bred fish are arrhythmic, while grouped fish are rhythmic (Boujard, 1995).
Rhythmicity of Locomotor Activity in the Experimental Stream Without Shelters
In our study, the locomotor activity of albino catfish in the environment without shelters was arrhythmic, while the activity of pigmented catfish was rhythmic. Animals use shelters to avoid risks from predators or adverse conditions (Álvarez and Nicieza, 2003; Weiss et al., 2008; Larranaga and Steingrímsson, 2015), and under laboratory conditions, S. glanis prefers an environment with shelters (Slavík et al., 2012). Behavioral rhythmicity during the diel cycle can be influenced by stressors (Sánchez-Vázquez et al., 2019), and the absence of shelters during our experiment represents such a stressor for the albino phenotype. For example, the lack of availability of shelters stressed the freshwater nocturnal predator burbot, Lota lota (Linnaeus, 1758), during daylight even when its predators were not present (Fischer, 2000). Hence, the explanation for why the pigmented phenotype displayed rhythmicity in locomotor activity while the albino phenotype did not is presumably related to their different sensitivities to stress induced by the lack of availability of shelters.
Rhythmicity of Locomotor Activity and Stress Parameters in the Experimental Arena With a Shelter
In the environment with a shelter, the locomotor activity of both the pigmented and albino phenotypes was rhythmic, with the peak during the dark, as observed for aggressiveness and shelter reassignment. Correspondingly, higher locomotor activity is associated with an increase in aggressiveness (Huntingford et al., 2012; Manuel et al., 2016), manifesting as an increasing frequency of changes in shelter use by S. glanis in our study. Analyses of biochemical indices from blood plasma, however, showed rhythmicity of stress parameters only in albino catfish. Circadian oscillation of cortisol levels in fish is species-specific and variable during the L/D cycle (Pickering and Pottinger, 1983; Lankford et al., 2003; López-Olmeda et al., 2009). Nocturnal species, e.g., the Senegalese sole Solea senegalensis (Kaup, 1858), show higher values at the end of the day (Oliveira et al., 2013). Conversely, a species with daylight activity, the green sturgeon Acipenser medirostris (Ayres, 1854), exposed to stressful conditions displayed an increased level of plasma cortisol with peak at night (Lankford et al., 2003). Stressed individuals of the sea bream Sparus aurata (Linnaeus, 1758) showed diurnal rhythmicity of cortisol with an increase at night, while unstressed conspecifics showed no rhythmicity (Vera et al., 2014), which corresponds with the differences between albino and pigmented S. glanis found in our study. We recorded rhythmicity, e.g., in cortisol, glucose and lactate, only in albino catfish with the peak at night and dawn. In fish, the diel rhythmicity of lactate is apparent (Lankford et al., 2003; Polakof et al., 2007), while that of glucose is not necessarily obvious (Lankford et al., 2003; López-Olmeda et al., 2009; Oliveira et al., 2013). Cortisol pronouncedly contributes to an increase in glucose via gluconeogenesis (Pankhurst, 2011), and anaerobic glycolysis during intense exercise leads to the increased production of lactate and its secretion into plasma (Begg and Pankhurst, 2004; Pankhurst, 2011). All three parameters have been used to determine stress (Øverli et al., 1999; Sloman et al., 2001; Begg and Pankhurst, 2004; Costas et al., 2011) in fish. Locomotor activity during the L/D cycle, production of hormones, e.g., cortisol, and expression of genes for biological rhythmicity are linked and can be assigned to fish personality, showing high rhythmicity in proactive individuals and no/low rhythmicity in reactive conspecifics (Tudorache et al., 2018). Albino individuals can be considered reactive, displaying lower aggressiveness (Slavík et al., 2016b), higher stress in response to variability in the physical and social environments (Slavík et al., 2022) and higher light-induced stress, including associated rhythmicity in stress parameters, as observed during this study. The higher stress induced by allocation and defense of resources, e.g., shelters, can be considered a key reason for the rarity of albino individuals in nature, also taking into account the previously considered significant influence of predation on their abundance (Ellegren et al., 1997), which does not seem to be as apparent (Stephenson et al., 2021) as previously expected.
Rhythmicity of Oxidative Stress in the Experimental Arena With a Shelter
Oxidative stress is associated with hormonal production during the stress response. Increased production of free radicals occurs with an increase in catecholamine (Smythies and Galzigna, 1998) and/or cortisol and glucose correspond to higher energy production (McIntosh et al., 1998; Liu and Mori, 1999). The variability in values of some antioxidants can be used as an indicator of oxidative stress. For example, Costantini et al. (2008) observed an increased antioxidant serum capacity in a group of mice characterized as reactive, showing lower levels of aggressiveness and shyness and higher sensitivity to stress, while in a group of proactive individuals usually displaying more aggression and a lower stress response, the antioxidative capacity was lower. The increased antioxidant capacity of reactive individuals can be considered a strategy for managing high levels of stress. For example, Almeida et al. (2009) reported higher CAT and SOD activities in dominant individuals of the Nile tilapia Oreochromis niloticus (Linnaeus, 1758) than in submissive conspecifics, indicating the influence of the social environment on the oxidative stress level. In our study, the evaluation of 4 antioxidants and 1 oxidative stress indicator in the tissues (brain, gill, and liver), representing a total of 15 indices, revealed significant rhythmicity for 8 indices in albino catfish, whereas pigmented catfish showed significant rhythmicity for only 5 indices. In albino catfish, the maximal level of antioxidants in the brain and liver was detected during daylight, while for gills, it was detected during dawn. This suggests that the light-induced stress from daylight was accompanied by an increase in fish aggressiveness during the effort of conspecifics to find shelters. In contrast, in the pigmented phenotype, the antioxidant levels in the brain and liver reached a maximum during dusk and at night, indicating their nocturnal activity. An increase in oxidative stress in fish with increasing light intensity (Giannetto et al., 2014; Kim et al., 2016) has been reported. For example, Sreejith et al. (2007) reported an increase in CAT and SOD activities in climbing perch, Anabas testudineus (Bloch, 1792), exposed to a continuous illumination photoperiod and a decrease in activity and glutathione content after melatonin treatment. These results suggested higher stress in albino individuals than in pigmented conspecifics during the light phase of the day.
Complex Model for Light/Dark Rhythmicity of Aggressiveness and Stress Parameters
Aggressiveness is influenced by L/D rhythmicity during a diel cycle (Hood and Amir, 2018). Hence, in our study, a complex statistical model was prepared to analyze aggressiveness, the biochemical indices from blood plasma, the level of oxidative stress and L/D rhythmicity. For the analyses of oxidative stress, brain samples with the closest relation to behavioral and stress patterns were used. For both phenotypes, the model displayed a sinusoid course with the peak of agonistic interactions during dawn. Both phenotypes showed predominantly nocturnal activity; hence, the importance of shelters increased during dawn. Sufficient shelter availability reduces overall aggressiveness (Höjesjö et al., 2004; Kobler et al., 2011), while a limited number of shelters encourages aggressive behavior (Mikheev et al., 2005). The effects of glucose and SOD varied with phenotype, while the effects of cortisol, lactate and catalase did not. An increase in SOD for only the pigmented phenotype manifested as a small decrease in aggressiveness, shows that oxidative stress accompanies the behavioral response of social interactions (Costantini et al., 2008; Almeida et al., 2009). Interestingly, a one-unit increase in glucose in the albino phenotype was associated with a 14% decrease in aggressiveness, while in the pigmented phenotype, a one-unit increase in glucose accompanied a 342% increase in aggressiveness. These apparent differences demonstrate physiological and behavioral variation between albino and pigmented phenotypes. The correlation between glucose level and aggressiveness (McIntosh et al., 1998; Liu and Mori, 1999) suggests that agonistic interactions are more exhausting for albino individuals than for pigmented conspecifics, indicating different behavioral strategies between the phenotypes characterized by an extreme increase in aggressiveness in the pigmented phenotype in response to glucose increase. This difference can be considered the reason why aggressive interactions are rarely observed in individuals with the albino phenotype (Slavík et al., 2016b). Hence, we can assume that light intensity was the key factor influencing the different levels of aggressiveness and stress indices between the two phenotypes.
Conclusion
Our results suggested that melanin absence is associated with the typical behavioral performance of the albino phenotype during the L/D cycle and showed more stress in the albino phenotype under light conditions than in pigmented conspecifics. Rare albino individuals observed in nature display different behavioral and physiological responses under experimental laboratory conditions compared to those of pigmented individuals, which show a lower stress response and behavioral patterns approaching those observed during ecological studies in natural conditions.
Data Availability Statement
The datasets generated for this study can be found in Dryad repository (Valchářová et al., 2022).
Ethics Statement
The animal study was reviewed and approved by the Ministry of Agriculture of the Czech Republic (63479/2016-MZE-17214).
Author Contributions
OS and PH conceptualized the research. PH, JV, MM, and TV managed the data curation. JV and AS conducted the biochemical analyses. PH, IH, MM, and MP conducted the statistical analyses. OS, JV, AS, PH, and TV conducted the investigation and sampling in the laboratory. OS, PH, JV, and AS developed the methodological approach. TV, OS, and PH wrote the manuscript with input from all authors.
Funding
The funding source of our study was European Regional Development Fund; submitted manuscript was supported within the frame of the comprehensive project named “Centre for the investigation of synthesis and transformation of nutritional substances in the food chain in inter-action with potentially harmful substances of anthropogenic origin: comprehensive assessment of soil contamination risks for the quality of agricultural products” (No. CZ.02.1.01/0.0/0.0/16_019/0000845).
Conflict of Interest
The authors declare that the research was conducted in the absence of any commercial or financial relationships that could be construed as a potential conflict of interest.
Publisher’s Note
All claims expressed in this article are solely those of the authors and do not necessarily represent those of their affiliated organizations, or those of the publisher, the editors and the reviewers. Any product that may be evaluated in this article, or claim that may be made by its manufacturer, is not guaranteed or endorsed by the publisher.
Acknowledgments
We thank two reviewers for their valuable comments and A. Slavikova for providing assistance with earlier versions of the manuscript.
Footnotes
References
Alanärä, A., and Brännäs, E. (1997). Diurnal and nocturnal feeding activity in Arctic char (Salvelinus alpinus) and rainbow trout (Oncorhynchus mykiss). Can. J. Fisher. Aquat. Sci. 54, 2894–2900. doi: 10.1139/f97-187
Alanärä, A., Burns, M. D., and Metcalfe, N. B. (2001). Intraspecific resource partitioning in brown trout: the temporal distribution of foraging is determined by social rank. J. Anim. Ecol. 70, 980–986. doi: 10.1046/j.0021-8790.2001.00550.x
Almazán-Rueda, P., Schrama, J. W., and Verreth, J. A. J. (2004). Behavioural responses under different feeding methods and light regimes of the African catfish (Clarias gariepinus) juveniles. Aquaculture 231, 347–359. doi: 10.1016/j.aquaculture.2003.11.016
Almeida, J. A., Barreto, R. E., Novelli, E. L. B., Castro, F. J., and Moron, S. E. (2009). Oxidative stress biomarkers and aggressive behavior in fish exposed to aquatic cadmium contamination. Neotropic. Ichthyol. 7, 103–108. doi: 10.1590/s1679-62252009000100013
Álvarez, D., and Nicieza, A. G. (2003). Predator avoidance behaviour in wild and hatchery-reared brown trout: the role of experience and domestication. J. Fish Biol. 63, 1565–1577. doi: 10.1046/j.1095-8649.2003.00267.x
Balkema, G. W., and Dräger, U. C. (1991). Impaired visual thresholds in hypopigmented animals. Visual Neurosci. 6, 577–585. doi: 10.1017/S095252380000256X
Beers, R. F. Jr., and Sizer, W. I (1952). A spectrophotometric method for measuring the breakdown of hydrogen peroxide by catalase. J. Biol. Chem. 195, 133–140.
Begg, K., and Pankhurst, N. W. (2004). Endocrine and metabolic responses to stress in a laboratory population of the tropical damselfish Acanthochromis polyacanthus. J. Fish Biol. 64, 133–145. doi: 10.1111/j.1095-8649.2004.00290.x
Bilandžija, H., Ma, L., Parkhurst, A., and Jeffery, W. R. (2013). A potential benefit of albinism in Astyanax cavefish: downregulation of the oca2 gene increases tyrosine and catecholamine levels as an alternative to melanin synthesis. PLoS One 8:1–14. doi: 10.1371/journal.pone.0080823
Borges, A., Scotti, L. v, Siqueira, D. R., Zanini, R., Amaral, F. d., Jurinitz, D. F., et al. (2007). Changes in hematological and serum biochemical values in jundiá Rhamdia quelen due to sub-lethal toxicity of cypermethrin. Chemosphere 69, 920–926. doi: 10.1016/j.chemosphere.2007.05.068
Boujard, T. (1995). Diel rhythms of feeding activity in the European catfish. Silurus glanis. Physiol. Behav. 58, 641–645. doi: 10.1016/0031-9384(95)00109-V
Bradford, M. M. (1976). Rapid and sensitive method for the quantitation of microgram quantities of protein utilising the principle of protein dye binding. Anal. Biochem. 72, 248–254.
Brännäs, E. (2008). Temporal resource partitioning varies with individual competitive ability: a test with Arctic charr Salvelinus alpinus visiting a feeding site from a refuge. J. Fish Biol. 73, 524–535. doi: 10.1111/j.1095-8649.2008.01941.x
Britz, P. J., and Pienaar, A. G. (1992). Laboratory experiments on the effect of light and cover on the behaviour and growth of African catfish, Clarias gariepinus (Pisces: Clariidae). J. Zool. 227, 43–62. doi: 10.1111/j.1469-7998.1992.tb04343.x
Buhusi, C. v, Perera, D., and Meck, W. H. (2005). Memory for Timing Visual and Auditory Signals in Albino and Pigmented Rats. J. Exp. Psychol. 31, 18-30. doi: 10.1037/0097-7403.31.1.18
Carvalho, T. B., Ha, J. C., and Gonçalves-de-Freitas, E. (2012). Light intensity can trigger different agonistic responses in juveniles of three cichlid species. Mar. Freshwater Behav. Physiol. 45, 91–100. doi: 10.1080/10236244.2012.690564
Chowdhury, S., and Saikia, S. K. (2020). Oxidative Stress in Fish: a Review. J. Sci. Res. 12, 145–160.
Conrad, J. L., Weinersmith, K. L., Brodin, T., Saltz, J. B., and Sih, A. (2011). Behavioural syndromes in fishes: a review with implications for ecology and fisheries management. J. Fish Biol. 78, 395–435. doi: 10.1111/j.1095-8649.2010.02874.x
Copeland, D. L., Levay, B., Sivaraman, B., Beebe-Fugloni, C., and Earley, R. L. (2011). Metabolic costs of fighting are driven by contest performance in male convict cichlid fish. Anim. Behav. 82, 271–280. doi: 10.1016/j.anbehav.2011.05.001
Costantini, D., Carere, C., Caramaschi, D., and Koolhaas, J. M. (2008). Aggressive and non-aggressive personalities differ in oxidative status in selected lines of mice (Mus musculus). Biol. Lett. 4, 119–122. doi: 10.1098/rsbl.2007.0513
Costantini, D., Marasco, V., and Møller, A. P. (2011). A meta-analysis of glucocorticoids as modulators of oxidative stress in vertebrates. J. Compar. Physiol. 181, 447–456. doi: 10.1007/s00360-011-0566-2
Costas, B., Conceição, L. E. C., Aragão, C., Martos, J. A., Ruiz-Jarabo, I., Mancera, J. M., et al. (2011). Physiological responses of Senegalese sole (Solea senegalensis Kaup, 1858) after stress challenge: effects on non-specific immune parameters, plasma free amino acids and energy metabolism. Aquaculture 316, 68–76. doi: 10.1016/j.aquaculture.2011.03.011
Defries, J. C. (1969). Pleiotropic Effects of Albinism on Open Field Behaviour in Mice. Nature 221, 65–66. doi: 10.1038/221065a0
Dingerkus, G., Séret, B., and Guilbert, E. (1991). The first albinos wels, Silurus glanis Linnaeus, 1758, from France, with a review of albinism in catfishes (Teleostei: siluriformes). Cybium 15, 185–188.
Ducrest, A., Keller, L., and Roulin, A. (2008). Pleiotropy in the melanocortin system, coloration and behavioural syndromes. Trends Ecol. Evol. 23, 502–510. doi: 10.1016/j.tree.2008.06.001
Ellegren, H., Lindgren, G., Primmer, C. R., and Møller, A. P. (1997). Fitness loss and germline mutations in barn swallows breeding in Chernobyl. Nature 389, 593–596. doi: 10.1038/39303
Elofsson, U. O. E., Mayer, I., Damsgård, B., and Winberg, S. (2000). Intermale competition in sexually mature Arctic charr: effects on brain monoamines, endocrine stress responses, sex hormone levels, and behavior. Gen. Compar. Endocrinol. 118, 450–460. doi: 10.1006/gcen.2000.7487
Fernandez, X., Meunier-Salaün, M. C., and Mormede, P. (1994). Agonistic behavior, plasma stress hormones, and metabolites in response to dyadic encounters in domestic pigs: interrelationships and effect of dominance status. Physiol. Behav. 56, 841–847. doi: 10.1016/0031-9384(94)90313-1
Ferrari, A., Venturino, A., Pechén and de D’Angelo, A. M. (2007). Effects of carbaryl and azinphos methyl on juvenile rainbow trout (Oncorhynchus mykiss) detoxifying enzymes. Pesticide Biochem. Physiol. 88, 134–142. doi: 10.1016/j.pestbp.2006.10.005
Fischer, P. (2000). An experimental test of metabolic and behavioural responses of benthic fish species to different types of substrate. Can. J. Fisher. Aquat. Sci. 57, 2336–2344. doi: 10.1139/f00-211
Fuller, J. L. (1967). Effects of the albino gene upon behaviour of mice. Anim. Behav. 15, 467–470. doi: 10.1016/0003-3472(67)90045-0
Gallardo, J. M., Alavi, S. M. H., Adámek, Z., and Drozd, B. (2010). External damage and changes in blood parameters in female tench, Tinca tinca (L.) retained in anglers’ keepnets. Rev. Fish Biol. Fisher. 20, 403–408. doi: 10.1007/s11160-009-9149-7
Giannetto, A., Fernandes, J. M. O., Nagasawa, K., Mauceri, A., Maisano, M., De Domenico, E., et al. (2014). Influence of continuous light treatment on expression of stress biomarkers in Atlantic cod. Dev. Compar. Immunol. 44, 30–34. doi: 10.1016/j.dci.2013.11.011
Habig, W. H., Pabst, M. J., and Jakoby, W. B. (1974). Glutathione S transferases. The first enzymatic step in mercapturic acid formation. J. Biol. Chem. 249, 7130–7139. doi: 10.1016/S0021-9258(19)42083-8
Haller, J. (1991). Biochemical cost of a fight in fed and fasted Betta splendens. Physiol. Behav. 49, 79–82. doi: 10.1016/0031-9384(91)90234-F
Haller, J. (1995). Biochemical background for an analysis of cost-benefit interrelations in aggression. Neurosci. Biobehav. Rev. 19, 599–604. doi: 10.1016/0149-7634(95)00053-4
Höglund, E., Balm, P. H. M., and Winberg, S. (2000). Skin darkening, a potential social signal in subordinate Arctic charr (Salvelinus alpinus): the regulatory role of brain monoamines and pro-opiomelanocortin-derived peptides. J. Exp. Biol. 203, 1711–1721. doi: 10.1242/jeb.203.11.1711
Höjesjö, J., Johnsson, J., and Bohlin, T. (2004). Habitat complexity reduces the growth of aggressive and dominant brown trout (Salmo trutta) relative to subordinates. Behav. Ecol. Sociobiol. 56, 286–289. doi: 10.1007/s00265-004-0784-7
Hood, S., and Amir, S. (2018). Biological clocks and rhythms of anger and aggression. Front. Behav. Neurosci. 12:1–12. doi: 10.3389/fnbeh.2018.00004
Horký, P., Douda, K., Maciak, M., Závorka, L., and Slavík, O. (2014). Parasite-induced alterations of host behaviour in a riverine fish: the effects of glochidia on host dispersal. Freshwater Biol. 59, 1452–1461. doi: 10.1111/fwb.12357
Horth, L. (2003). Melanic body colour and aggressive mating behaviour are correlated traits in male mosquitofish (Gambusia holbrooki). Proc. R. Soc. London. Ser. B: Biol. Sci. 270, 1033–1040. doi: 10.1098/rspb.2003.2348
Hubená, P., Horký, P., and Slavík, O. (2020). Test-dependent expression of behavioral syndromes: a study of aggressiveness, activity, and stress of chub. Aggressive Behav. 46, 412–424. doi: 10.1002/ab.21909
Huntingford, F. A., Kadri, S., and Jobling, M. (2012). Aquaculture and Behavior. Oxford, UK: Wiley-Blackwell.
Hupfeld, D., and Hoffmann, K. (2006). Motion perception in rats (Rattus norvegicus sp.): deficits in albino Wistar rats compared to pigmented Long-Evans rats. Behav. Brain Res. 170, 29–33. doi: 10.1016/j.bbr.2006.01.022
Jeffery, G. (1997). The albino retina: an abnormality that provides insight into normal retinal development. Trends Neurosci. 20, 165–169. doi: 10.1016/S0166-2236(96)10080-1
Kehas, A. J., Theoharides, K. A., and Gilbert, J. J. (2005). Effect of sunlight intensity and albinism on the covering response of the Caribbean sea urchin Tripneustes ventricosus. Mar. Biol. 146, 1111–1117. doi: 10.1007/s00227-004-1514-4
Kenward, M. G., and Roger, J. H. (1997). Small Sample Inference for Fixed Effects from Restricted Maximum Likelihood. Biometrics 53:983. doi: 10.2307/2533558
Kim, B., Jin, S., Jae, Y., Na, N., Young, C., and Kim, J. (2016). Effects of different light wavelengths from LEDs on oxidative stress and apoptosis in olive flounder (Paralichthys olivaceus) at high water temperatures. Fish Shellfish Immunol. 55, 460–468. doi: 10.1016/j.fsi.2016.06.021
Kittilsen, S., Johansen, I. B., Braastad, B. O., and Øverli, Ø (2012). Pigments, Parasites and Personalitiy: towards a Unifying Role for Steroid Hormones? PLoS One 7:e34281. doi: 10.1371/journal.pone.0034281
Kittilsen, S., Schjolden, J., Beitnes-Johansen, I., Shaw, J. C., Pottinger, T. G., Sørensen, C., et al. (2009). Melanin-based skin spots reflect stress responsiveness in salmonid fish. Horm. Behav. 56, 292–298. doi: 10.1016/j.yhbeh.2009.06.006
Kobler, A., Maes, G. E., Humblet, Y., Volckaert, F. A. M., Eens, M., Kobler, A., et al. (2011). Temperament traits and microhabitat use in bullhead, Cottus perifretum: fish associated with complex habitats are less aggressive. Behaviour 148, 603–625.
Koolhaas, J. M., Korte, S. M., De Boer, S. F., Van Der Vegt, B. J., Van Reenen, C. G., et al. (1999). Coping styles in animals: current status in behavior and stress-physiology. Neurosci. Biobehav. Rev. 23, 925–935. doi: 10.1016/s0149-7634(99)00026-3
Kvetnansky, R., Sabban, E. L., and Palkovits, M. (2009). Catecholaminergic systems in stress: structural and molecular genetic approaches. Physiol. Rev. 89, 535–606. doi: 10.1152/physrev.00042.2006
Lankford, S. E., Adams, T. E., and Cech, J. J. Jr. (2003). Time of day and water temperature modify the physiological stress response in green sturgeon, Acipenser medirostris. Compar. Biochem. Physiol. Part A: Mol. Integr. Physiol. 135, 291–302. doi: 10.1016/S1095-6433(03)00075-8
Lechner, W., and Ladich, F. (2011). How do albino fish hear? J. Zool. 283, 186–192. doi: 10.1111/j.1469-7998.2010.00762.x
Li, Z.-H., Velisek, J., Zlabek, V., Grabic, R., Machova, J., Kolarova, J., et al. (2011). Chronic toxicity of verapamil on juvenile rainbow trout (Oncorhynchus mykiss): effects on morphological indices, hematological parameters and antioxidant responses. J. Hazard. Mater. 185, 870–880. doi: 10.1016/j.jhazmat.2010.09.102
Liu, J., and Mori, A. (1999). Stress, aging, and brain oxidative damage. Neurochem. Res. 24, 1479–1497. doi: 10.1023/A:1022597010078
Lopes, A. C. C., Villacorta-Correa, M. A., and Carvalho, T. B. (2018). Lower light intensity reduces larval aggression in matrinxã, Brycon amazonicus. Behav. Proc. 151, 62–66. doi: 10.1016/j.beproc.2018.01.017
López-Olmeda, J. F., Montoya, A., Oliveira, C., and Sánchez-Vázquez, F. J. (2009). Synchronization to light and restricted-feeding schedules of behavioral and humoral daily rhythms in gilthead sea bream (Sparus aurata). Chronobiol. Int. 26, 1389–1408. doi: 10.3109/07420520903421922
Lushchak, V. I, Bagnyukova, T. v, Husak, V. v, Luzhna, L. I, Lushchak, O. v, and Storey, K. B. (2005). Hyperoxia results in transient oxidative stress and an adaptive response by antioxidant enzymes in goldfish tissues. Int. J. Biochem. Cell Biol. 37, 1670–1680. doi: 10.1016/j.biocel.2005.02.024
Madrid, J. A., Boujard, T., and Sánchez-Vázquez, F. J. (2001). “Feeding Rhythms,” in Food Intake in Fish, eds D. Houlihan, T. Boujard, and M. Jobling (Oxford: Blackwell Science, UK), 189–215.
Manuel, R., Boerrigter, J. G. J., Cloosterman, M., Gorissen, M., Flik, G., van den Bos, R., et al. (2016). Effects of acute stress on aggression and the cortisol response in the African sharptooth catfish Clarias gariepinus: differences between day and night. J. Fish Biol. 88, 2175–2187. doi: 10.1111/jfb.12989
Marc, R. E., Jones, B. W., Watt, C. B., Vazquez-Chona, F., Vaughan, D. K., and Organisciak, D. T. (2008). Extreme retinal remodeling triggered by light damage: implications for age related macular degeneration. Mol. Vis. 14, 782–806.
Marklund, S., and Marklund, G. (1974). Involvement of the Superoxide Anion Radical in the Autoxidation of Pyrogallol and a Convenient Assay for Superoxide Dismutase. Eur. J. Biochem. 47, 469–474. doi: 10.1111/j.1432-1033.1974.tb03714.x
McIntosh, L. J., Hong, K. E., and Sapolsky, R. M. (1998). Glucocorticoids may alter antioxidant enzyme capacity in the brain: baseline studies. Brain Res. 791, 209–214. doi: 10.1016/S0006-8993(98)00115-2
Mikheev, V. N., Pasternak, A. F., Tischler, G., and Wanzenböck, J. (2005). Contestable shelters provoke aggression among 0 + perch, Perca fluviatilis. Environ. Biol. Fishes 73, 227–231. doi: 10.1007/s10641-005-0558-8
Neat, F. C., Taylor, A. C., and Huntingford, F. A. (1998). Proximate costs of fighting in male cichlid fish: the role of injuries and energy metabolism. Anim. Behav. 55, 875–882. doi: 10.1006/anbe.1997.0668
Oetting, W. S. (2000). The Tyrosinase Gene and Oculocutaneous Albinism Type 1 (OCA1): a Model for Understanding the Molecular Biology of Melanin Formation. Pigment Cell Res. 13, 320–325. doi: 10.1034/j.1600-0749.2000.130503.x
Oliveira, C., Garcia, E. M., López-Olmeda, J. F., and Sánchez-Vázquez, F. J. (2009). Daily and circadian melatonin release in vitro by the pineal organ of two nocturnal teleost species: senegal sole (Solea senegalensis) and tench (Tinca tinca). Compar. Biochem. Physiol. Part A: Mol. Integr. Physiol. 153, 297–302. doi: 10.1016/j.cbpa.2009.03.001
Oliveira, C. C. V., Aparício, R., Blanco-Vives, B., Chereguini, O., Martín, I., and Javier Sánchez-Vazquez, F. (2013). Endocrine (plasma cortisol and glucose) and behavioral (locomotor and self-feeding activity) circadian rhythms in Senegalese sole (Solea senegalensis Kaup 1858) exposed to light/dark cycles or constant light. Fish Physiol. Biochem. 39, 479–487. doi: 10.1007/s10695-012-9713-2
Øverli, Ø, Korzan, W. J., Höglund, E., Winberg, S., Bollig, H., Watt, M., et al. (2004). Stress coping style predicts aggression and social dominance in rainbow trout. Horm. Behav. 45, 235–241. doi: 10.1016/j.yhbeh.2003.12.002
Øverli, Olsen, R. E., Løvik, F., and Ringø, E. (1999). Dominance hierarchies in Arctic charr, Salvelinus alpinus L.: differential cortisol profiles of dominant and subordinate individuals after handling stress. Aquacult. Res. 30, 259–264. doi: 10.1046/j.1365-2109.1999.00322.x
Owen, K., Thiessen, D. D., and Lindzey, G. (1970). Acrophobic and photophobic responses associated with the albino locus in mice. Behav. Genet. 1, 249–255. doi: 10.1007/BF01074656
Pankhurst, N. W. (2011). The endocrinology of stress in fish: an environmental perspective. Gen. Compar. Endocrinol. 170, 265–275. doi: 10.1016/j.ygcen.2010.07.017
Pickering, A. D. (1992). Rainbow trout husbandry: management of the stress response. Aquaculture 100, 125–139. doi: 10.1016/0044-8486(92)90354-N
Pickering, A. D., and Pottinger, T. G. (1983). Seasonal and diel changes in plasma cortisol levels of the brown trout, Salmo trutta L. Gen. Compar. Endocrinol. 49, 232–239. doi: 10.1016/0016-6480(83)90139-9
Polakof, S., Ceinos, R. M., Fernández-Durán, B., Míguez, J. M., and Soengas, J. L. (2007). Daily changes in parameters of energy metabolism in brain of rainbow trout: dependence on feeding. Compar. Biochem. Physiol. Part A: Mol. Integr. Physiol. 146, 265–273. doi: 10.1016/j.cbpa.2006.10.026
Pottinger, T. G., and Carrick, T. R. (2001). Stress responsiveness affects dominant-subordinate relationships in rainbow trout. Horm. Behav. 40, 419–427. doi: 10.1006/hbeh.2001.1707
Prusky, G. T., Harker, K. T., Douglas, R. M., and Whishaw, I. Q. (2002). Variation in visual acuity within pigmented, and between pigmented and albino rat strains. Behav. Brain Res. 136, 339–348. doi: 10.1016/S0166-4328(02)00126-2
R Core Team (2019). R: A Language and Environment for Statistical Computing. Vienna: R Foundation for Statistical Computing.
Réale, D., Reader, S. M., Sol, D., McDougall, P. T., and Dingemanse, N. J. (2007). Integrating animal temperament within ecology and evolution. Biol. Rev. 82, 291–318. doi: 10.1111/j.1469-185X.2007.00010.x
Refinetti, R. (2007). Enhanced circadian photoresponsiveness after prolonged dark adaptation in seven species of diurnal and nocturnal rodents. Physiol. Behav. 90, 431–437. doi: 10.1016/j.physbeh.2006.10.004
Ren, J. Q., McCarthy, W. R., Zhang, H., Adolph, A. R., and Li, L. (2002). Behavioral visual responses of wild-type and hypopigmented zebrafish. Vision Res. 42, 293–299. doi: 10.1016/S0042-6989(01)00284-X
Sánchez-Vázquez, F. J., López-Olmeda, J. F., Vera, L. M., Migaud, H., López-Patiño, M. A., and Míguez, J. M. (2019). Environmental cycles, melatonin, and circadian control of stress response in fish. Front. Endocrinol. 10:1–18. doi: 10.3389/fendo.2019.00279
Sánchez-Vázquez, F. J., Madrid, J. A., Zamora, S., and Tabata, M. (1997). Feeding entrainment of locomotor activity rhythms in the goldfish is mediated by a feeding-entrainable circadian oscillator. J. Compar. Physiol. - A Sensory, Neural, Behav. Physiol. 181, 121–132. doi: 10.1007/s003590050099
Sánchez-Vázquez, F. J., Zamora, S., and Madrid, J. A. (1995). Light-dark and food restriction cycles in sea bass: effect of conflicting zeitgebers on demand-feeding rhythms. Physiol. Behav. 58, 705–714. doi: 10.1016/0031-9384(95)00116-Z
Santostefano, F., Fanson, K. v, Endler, J. A., and Biro, P. A. (2019). Behavioral, energetic, and color trait integration in male guppies: testing the melanocortin hypothesis. Behav. Ecol. 30, 1539–1547. doi: 10.1093/beheco/arz109
Sedlak, J., and Lindsay, R. H. (1968). Estimation of total, protein-bound, and nonprotein sulfhydryl groups in tissue with Ellman’s reagent. Anal. Biochem. 25, 192–205. doi: 10.1016/0003-2697(68)90092-4
Slavík, O., and Horký, P. (2012). Diel dualism in the energy consumption of the European catfish Silurus glanis. J. Fish Biol. 81, 2223–2234. doi: 10.1111/j.1095-8649.2012.03436.x
Slavík, O., Horký, P., Bartoš, L., Koláøová, J., and Randák, T. (2007). Diurnal and seasonal behaviour of adult and juvenile European catfish as determined by radio-telemetry in the River Berounka. Czech Repub. J. Fish Biol. 71, 101–114. doi: 10.1111/j.1095-8649.2007.01471.x
Slavík, O., Horký, P., and Maciak, M. (2015). Ostracism of an Albino Individual by a Group of Pigmented Catfish. PLoS One 10:e0128279. doi: 10.1371/journal.pone.0128279
Slavík, O., Horký, P., and Wackermannová, M. (2016b). How does agonistic behaviour differ in albino and pigmented fish? PeerJ. 4, e1937. doi: 10.7717/peerj.1937
Slavík, O., Horký, P., Maciak, M., and Wackermannová, M. (2016a). Familiarity, prior residency, resource availability and body mass as predictors of the movement activity of the European catfish. J. Ethol. 34, 23–30. doi: 10.1007/s10164-015-0441-9
Slavík, O., Horký, P., Valcháøová, T., Pfauserová, N., and Velíšek, J. (2022). Comparative study of stress responses, laterality and familiarity recognition between albino and pigmented fish. Zoology 150, 125982. doi: 10.1016/j.zool.2021.125982
Slavík, O., Maciak, M., and Horký, P. (2012). Shelter use of familiar and unfamiliar groups of juvenile European catfish Silurus glanis. Appl. Anim. Behav. Sci. 142, 116–123. doi: 10.1016/j.applanim.2012.09.005
Sloman, K. A., Metcalfe, N. B., Taylor, A. C., and Gilmour, K. M. (2001). Plasma cortisol concentrations before and after social stress in rainbow trout and brown trout. Physiol. Biochem. Zool. 74, 383–389. doi: 10.1086/320426
Smythies, J., and Galzigna, L. (1998). The oxidative metabolism of catecholamines in the brain: a review. Biochim. et Biophys. Acta - General Subjects 1380, 159–162. doi: 10.1016/S0304-4165(97)00131-1
Sreejith, P., Beyo, R. S., Divya, L., Vijayasree, A. S., Manju, M., and Oommen, O. v (2007). Triiodothyronine and melatonin influence antioxidant defense mechanism in a teleost Anabas testudineus (Bloch): in vitro study. Ind. J. Biochem. Biophys. 44, 164–168.
Stara, A., Kristan, J., Zuskova, E., and Velisek, J. (2013). Effect of chronic exposure to prometryne on oxidative stress and antioxidant response in common carp (Cyprinus carpio L.). Pesticide Biochem. Physiol. 105, 18–23. doi: 10.1016/j.pestbp.2012.11.002
Stephenson, B. P., Velani, Z., and Ihász, N. (2021). The effect of albinism on avian predator attack rates in eastern garter snakes. Zoology 150:125987. doi: 10.1016/j.zool.2021.125987
Stryjek, R., Modlińska, K., Turlejski, K., and Pisula, W. (2013). Circadian Rhythm of Outside-Nest Activity in Wild (WWCPS), Albino and Pigmented Laboratory Rats. PLoS One 8:e66055. doi: 10.1371/journal.pone.0066055
Sudová, E., Piačková, V., Kroupová, H., Pijáček, M., and Svobodová, Z. (2009). The effect of praziquantel applied per os on selected haematological and biochemical indices in common carp (Cyprinus carpio L.). Fish Physiol. Biochem. 35, 599–605. doi: 10.1007/s10695-008-9269-3
Tudorache, C., Slabbekoorn, H., Robbers, Y., Hin, E., Meijer, J. H., Spaink, H. P., et al. (2018). Biological clock function is linked to proactive and reactive personality types. BMC Biol. 16:1–14. doi: 10.1186/s12915-018-0618-0
Valchářová, T., Slavík, O., Horký, P., Stará, A., Hrušková, I., Maciak, M., et al. (2022). Stressful Daylight: Differences in Diel Rhythmicity Between Albino and Pigmented Fish. Dryad Digital Repository. V1. doi: 10.5061/dryad.m0cfxpp5j
Valdimarsson, S. K., and Metcalfe, N. B. (2001). Is the level of aggression and dispersion in territorial fish dependent on light intensity? Anim. Behav. 61, 1143–1149. doi: 10.1006/anbe.2001.1710
van Abeelen, J. H. F., and Kroes, H. W. (1967). Albinism and mouse behaviour. Genetica 38, 419–429. doi: 10.1007/BF01507473
Vera, L. M., Al-Khamees, S., and Hervé, M. (2011). Stocking density affects circadian rhythms of locomotor activity in african catfish, Clarias gariepinus. Chronobiol. Int. 28, 751–757. doi: 10.3109/07420528.2011.606388
Vera, L. M., Cairns, L., Sanchez-Vazquez, F. J., and Migaud, H. (2009). Circadian rhythms of locomotor activity in the nile tilapia Oreochromis niloticus. Chronobiol. Int. 26, 666–681. doi: 10.1080/07420520902926017
Vera, L. M., Montoya, A., Pujante, I. M., Pérez-Sánchez, J., Calduch-Giner, J. A., Mancera, J. M., et al. (2014). Acute stress response in gilthead sea bream (Sparus aurata L.) is time-of-day dependent: physiological and oxidative stress indicators. Chronobiol. Int. 31, 1051–1061. doi: 10.3109/07420528.2014.945646
Keywords: albinism, melanocortin theory, locomotor activity, aggressiveness, oxidative stress
Citation: Valchářová T, Slavík O, Horký P, Stará A, Hrušková I, Maciak M, Pešta M and Velíšek J (2022) Stressful Daylight: Differences in Diel Rhythmicity Between Albino and Pigmented Fish. Front. Ecol. Evol. 10:890874. doi: 10.3389/fevo.2022.890874
Received: 06 March 2022; Accepted: 23 June 2022;
Published: 22 July 2022.
Edited by:
Rafał Stryjek, Institute of Psychology (PAN), PolandReviewed by:
Cristiano Bertolucci, University of Ferrara, ItalyDaniel Cerveny, Swedish University of Agricultural Sciences, Sweden
Copyright © 2022 Valchářová, Slavík, Horký, Stará, Hrušková, Maciak, Pešta and Velíšek. This is an open-access article distributed under the terms of the Creative Commons Attribution License (CC BY). The use, distribution or reproduction in other forums is permitted, provided the original author(s) and the copyright owner(s) are credited and that the original publication in this journal is cited, in accordance with accepted academic practice. No use, distribution or reproduction is permitted which does not comply with these terms.
*Correspondence: Ondřej Slavík, b3NsYXZpa0BhZi5jenUuY3o=