- 1Research School of Biology, Australian National University, Canberra, ACT, Australia
- 2Department of Biological Sciences, Colorado Mesa University, Grand Junction, CO, United States
- 3Department of Biology, University of Utah, Salt Lake City, UT, United States
- 4Hawkesbury Institute for the Environment, Western Sydney University, Penrith, NSW, Australia
- 5Centre for Tropical Environmental and Sustainability Studies, James Cook University, Cairns, QLD, Australia
Ambient temperature is an underappreciated determinant of foraging behaviour in wild endotherms, and the requirement to thermoregulate likely influences food intake through multiple interacting mechanisms. We investigated relationships between ambient temperature and hepatic detoxification capacity in two herbivorous marsupials, the common ringtail possum (Pseudocheirus peregrinus) and common brushtail possum (Trichosurus vulpecula) that regularly feed on diets rich in plant toxins. As an indicator of hepatic detoxification capacity, we determined the functional clearance rate of an anaesthetic agent, Alfaxalone, after possums were acclimated to 10°C [below the thermoneutral zone (TNZ)], 18°C [approximately lower critical temperature (LCT)], and 26°C [approximately upper critical temperature (UCT)] for either 7 days or less than 24 h. We then measured intake of foods with high or low plant secondary metabolite (PSM) concentrations under the same temperature regimes. After 7 days of acclimation, we found a positive correlation between the functional clearance rate of Alfaxalone and ambient temperature, and a negative relationship between ambient temperature and intake of foods with high or low PSM concentrations for both species. The effect of ambient temperature on intake of diets rich in PSMs was absent or reduced when possums were kept at temperatures for less than 24 h. Our results underscore the effects of ambient temperature in hepatic metabolism particularly with respect intake of diets containing PSMs. Given that the planet is warming, it is vital that effects of ambient temperature on metabolism, nutrition and foraging by mammalian herbivores is taken into account to predict range changes of species and their impact on ecosystems.
Introduction
Herbivory is the most common feeding strategy amongst mammalian species, and interactions between plants and herbivores are a fundamental component of ecosystem dynamics (Foley and Moore, 2005). It is well known that animals in production systems consume less food at higher ambient temperatures (Ta) (e.g., Bernabucci et al., 1999; Ominski et al., 2002; Renaudeau et al., 2002) due to lower energy demands and limits on the dissipation of heat from digestion and metabolism (Dale and Fuller, 1979; Renaudeau et al., 2012). However, there has been little recognition that the same processes could have significant implications for wild mammals in a warming climate (Youngentob et al., 2021), by reducing their intake of critical nutrients.
Ambient temperature also influences the toxicity of many xenobiotics, including plant secondary metabolites (PSMs), by affecting rates of hepatic detoxification (Keplinger et al., 1959; Kaplanski and Ben-Zvi, 1980; Gordon et al., 2014). This phenomenon, known as temperature-dependent toxicity (TDT; Dearing, 2013), makes many xenobiotics administered to laboratory rodents more toxic at warmer temperatures. Furthermore, the variation in food intake by herbivores eating diets containing PSMs at different Ta is attributed to TDT. For example, herbivorous rodents (genus Neotoma) have greater tolerance for PSMs at cooler than at warmer Ta (Kurnath et al., 2016). Understanding the extent of TDT in other species under natural circumstances is crucial for developing robust mechanistic niche models that can predict the likely future impacts of climate change on ecosystems (Kearney et al., 2012; Forbey et al., 2018).
Measuring rates of detoxification of PSMs in wild herbivores is challenging because the specific detoxification pathways of many PSMs are unknown, and can be complex (Marsh et al., 2005). Even when pathways have been characterised, it is often difficult to collect blood or urine samples repeatedly from wild animals in order to estimate clearance times of xenobiotics. Other traditional measures of toxicity, such as LD50, can be ethically and ecologically questionable when studying wildlife species. As an alternative, some studies have used anaesthetic agents as indicators to determine the functional clearance time of hepatically metabolised xenobiotics, since their effects are easily observed in hypnotic state assays (Dearing et al., 2006; Kurnath and Dearing, 2013). During such assays, the total time for which animals sleep reveals the functional clearance time of the drug. Hypnotic state assays have the benefit of being non-lethal, and allowing the comparison of multiple treatments within one individual.
To advance our understanding of the effects of ambient temperature on detoxification we interrogated whether Ta influences the rates of hepatic metabolism and feeding behaviour in two folivorous marsupial species, the common brushtail possum (Trichosurus vulpecula) and common ringtail possum (Pseudocheirus peregrinus). Common ringtail possums are small (700–900 g) folivores that predominantly eat tree leaves, including significant amounts from Eucalyptus species (Pahl, 1987a), which contain variable concentrations of several PSMs that influence feeding behaviour (Marsh et al., 2003, 2015). Common brushtail possums are larger (2–4 kg) generalist herbivores that consume Eucalyptus leaves and a range of leaves, flowers and fruits from other plant species (McDowell and McLeod, 2007). We measured the functional clearance time of a modern anaesthetic agent, Alfaxalone, when both species of possums were acclimated to three ambient temperatures (10, 18, and 26°C) and with short and long acclimation periods. Understanding whether animals respond differently depending on their length of exposure will help to elucidate whether temperature fluctuations over different timescales are likely to affect hepatic metabolism in different ways. We expected that the functional clearance rate of Alfaxalone in both possum species would increase with increasing Ta, consistent with observations of reduced hepatic metabolism at high Ta in some other herbivore species (Dearing et al., 2006; Kurnath and Dearing, 2013). We also tested whether Ta affected the voluntary food intake of brushtail and ringtail possums. We expected that changes in energy requirements and heat dissipation capacity would mean that possums would reduce their intake of low-PSM diets with increasing Ta. We also expected possums to reduce their intake of diets containing PSMs as Ta increased if Ta reduced the functional clearance of ingested toxins.
Materials and Methods
Husbandry of Animals
All animal work was approved by the Australian National University Animal Experimentation Ethics Committee (A2015/24) and conforms to the Australian Code of Practice for the Care and Use of Animals for Scientific Purposes.
Common Brushtail Possums
Twelve adult male common brushtail possums (mean body mass ± sd = 3.04 ± 0.38 kg) were caught on The Australian National University (ANU) campus, Australian Capital Territory (ACT) in March of 2015, using traps baited with apple and peanut butter. These possums were used for the week-long exposure functional clearance and feeding experiments, and the short exposure feeding experiment. Another 12 adult male brushtail possums were caught on the ANU campus in January 2016 for the short exposure functional clearance assay (mean body mass ± sd = 3.03 ± 0.25 kg). The health and age (by tooth wear; Winter, 1980; Pahl, 1987b) of all possums was checked following capture.
Brushtail possums were housed individually in aviaries (90 cm × 70 cm × 152 cm) inside constant temperature rooms (initially set to 18°C) on a 12:12 h light:dark cycle with a 30 min change in the intensity of light to simulate dusk and dawn, and a 15 W red light during the dark cycle. All possums were provided with wooden platforms with two sides (18 cm × 18 cm × 34 cm) to provide some shelter, but to prevent any temperature buffering. We measured the body mass of brushtail possums weekly, and changes in mass were calculated relative to the weight recorded the previous week.
Brushtail possums were initially provided with fresh fruit and mixed leaves of what species daily. Fresh water was always available. Over a period of 5 weeks, they were transitioned to a basal diet of fruit and cereals [42.5% pureed apple, 28% pureed banana, 10% pureed carrot, 6% ground rice hulls, 6% rolled oats, 4% ground lucerne chaff, 1.67% acid casein, 1.25% vegetable oil, 0.3% NaCl, 0.25% dicalcium phosphate, and 0.03% vitamin and mineral mix (Vitagran, Multicrop, VIC, Australia), all on a wet matter basis] that was prepared fresh daily. Food was offered at 1630 h (possums are nocturnal) and uneaten food was collected at 0900 h daily throughout all experiments. The dry matter content of the offered food was measured daily by drying a subsample (∼ 20 g) to constant mass at 60°C. Dry matter intake (DMI) was determined by drying all uneaten food, and subtracting this from the calculated amount of dry matter offered. Gross energy content of the basal diet approximates 13.4 kJ/g.
Common Ringtail Possums
Twelve common ringtail possums (five non-lactating females and seven males; mean body mass ± sd = 743 ± 24 g) were caught by hand or from nest boxes on Black Mountain, ACT in autumn and early winter 2015. Ringtail possums were weighed weekly, and at the start and finish of each respirometry experiment. Changes in mass were calculated relative to the weight recorded for the previous week. During respirometry, possums were housed individually in large 6 m × 3 m × 3 m (l × w × h) aviaries at ambient temperature (10–20°C) and light. For the later functional clearance rate and feeding experiments the animals were housed individually in smaller aviaries (66 cm × 65 cm × 92 cm) in the same temperature controlled rooms described for brushtail possums. Ringtail possums were provided with nest boxes (15 cm × 15 cm × 20 cm) with one side formed from wire mesh to minimise temperature buffering.
Ringtail possums were fed Eucalyptus foliage (primarily E. rossii) ad libitum daily at 1630 h, and food was removed at 0900 h the following morning. The stems were placed in containers of water to prevent drying. A control bunch of leaves was used to monitor changes in mass, but these changes were always small (average of 0.5 g out of 250 g total), so no correction was made during calculations of foliage intake. DMI was determined by drying a subsample of leaves at 50°C from the control bunch, and multiplying this by the mass of fresh leaves consumed. Water was available ad libitum.
Respirometry
To determine appropriate temperatures for TDT studies, we first collected data on the resting metabolism of ringtail possums between 4 and 35°C. We measured metabolic rate across a range of Ta for both brushtail and ringtail possums using respirometry, however, half of the brushtail possums suffered a stress induced colitis following their first day of measurements, and, despite attempts to acclimate them to the procedure, the colitis returned. Therefore, only data for ringtail possums is described and we relied on data from the literature for information on the thermal neutral zone and critical temperatures for the brushtail possums (Cooper et al., 2018).
Resting metabolism and body temperature in common ringtail possums were measured using positive pressure flow through respirometry as described by Krockenberger et al. (2012) with minor modifications. All measurements were taken within the daylight hours, between 0800 and 1730 h, which is the resting phase for this species. We did not fast ringtail possums prior to measurements of thermal tolerance, because they are caecotrophs, and are prone to developing gastrointestinal disturbances when stressed (Vogelnest and Woods, 2008). Even though feeding would have ceased at about 0400 h (P. Beale, personal observations), undoubtedly food would have been in the digestive system when the measurements began. Since possums do not go for long periods of time without eating in the wild, the results are relevant indicators of temperature responses in wild animals.
Measurements of gas exchange were taken at six different temperatures (three temperatures on each of two separate days in the chamber) for each ringtail possum. The temperatures were stratified so that possums experienced one low (4–15°C), one medium (15–25°C) and one high temperature (25–35°C) on each day in the chamber. In this way, the maximum number of temperatures within the 4–35°C range was covered, while allocation of temperatures to individuals within the categories was random. No animal experienced the same temperature twice, and at least two animals were exposed to every temperature. Only one individual was used each day and there was at least a week between the 2 days that each individual was in the metabolic chamber.
Resting metabolic rate (RMR) calculations were made using Warthog lab analyst software. Flow was taken as actual flow for each time-period and air was assumed to be dry. There were in total 75 data points (individual-temperature combinations) after faulty files and or experiments where equipment failure occurred were excluded. In R studio, the 1st, 2nd, 3rd, and 4th order polynomial regressions were calculated on RMR and body temperature data, with RMR or body temperature as the dependent variable and the temperature of the metabolic chamber as the independent variable. These were compared using AIC model selection criteria. Using the R package “segmented” the models were then assessed for potential breakpoints. This method tests for piecewise relationships in regression models using an iterative approach (Muggeo, 2003).
Functional Clearance Assays
Week-Long Temperature Exposure
Four possums of each species were randomly allocated to each of three ambient temperature treatments (10, 18, and 26°C). These temperatures were chosen from the respirometry data, indicating that 10°C is below the LCT, 18°C is close to the LCT and 26°C is likely to be just below the UCT of the TNZ of ringtail possums. Predictions for the TNZ of brushtail possums are similar (Porter and Kearney, 2009; Riek and Geiser, 2013).
Possums were maintained at the experimental temperatures for 1 week. After this, the clearance time assay was conducted over 2 days, between 0900 and 1200 h, to ensure that all individuals were tested during a similar period of the circadian cycle. On the seventh day, two possums of each species from each temperature were administered 5 mg.kg–1 of Alfaxalone (Jurox, Rutherford, NSW, Australia) intramuscularly. The chosen dose was at the lower end of the range recommended for fast-acting and short-term veterinary anaesthesia (Miller and Folwer, 2015). Alfaxalone undergoes hepatic phase 1 (Cytochrome P450 dependent) and phase 2 (glucuronide and sulphate conjugation dependent) metabolism in a variety of species (Warne et al., 2015), making it an ideal proxy compound for PSMs. We chose Alfaxalone because it is a neuroactive steroid used routinely in veterinary medicine as a safe and effective anaesthetic (Warne et al., 2015). Alfaxalone It is preferable to the drug that has been used more commonly in functional clearance assays, hexobarbital (e.g., Dearing et al., 2006; Kurnath and Dearing, 2013), because hexobarbital is a highly alkaline barbiturate drug (pH 11.5), and is thus an irritant when injected intraperitoneally, intramuscularly, or subcutaneously (Barron and Dundee, 1961). Animals were held in bags under observation until drowsy, and then removed and placed on their backs in an observation cage. The length of time that each possum stayed on its back until righting itself was used as the functional drug clearance time (Keplinger et al., 1959). On the eighth day, the procedure was repeated for the remaining possums from each temperature. The clearance time assays were repeated over three rounds in a randomised crossover design such that each possum was tested at all three experimental temperatures. The order of administration was randomised by temperature between rounds to account for any effects of the time of administration. A period of 7 days elapsed between rounds to allow hepatic enzymes to return to baseline levels (Pass et al., 2001).
Short Temperature Exposure
Twelve brushtail possums were held at 18°C for at least 1 week prior to the start of the experiment. Then, at 1600 h, the ambient temperature was reset to either 10°C (six possums) or 26°C (six possums). The temperature of both rooms stabilised within 1 h. The clearance time assay was conducted between 0800 and 1200 h the following morning, when possums had been exposed to the experimental temperatures for between 16 and 20 h. The procedure for the assay was otherwise identical to the week-long exposure assay. Possums were returned to 18°C for another week, and the clearance time assay was repeated at the other temperature.
Feeding Experiments
Diets
Brushtail possums were offered a formulated diet with or without the PSM, flavanone. Flavanone is a representative of the unsubstituted B-ring flavanone (UBF) class of PSMs present in the foliage of many eucalypt species (Saraf et al., 2017; Marsh et al., 2019), and is known to deter brushtail possums from feeding (Marsh et al., 2015). One week before feeding experiments to reduce any effects of novelty, brushtail possums were offered the basal diet containing 0.625% DM flavanone (Sigma-Aldrich, Sydney, NSW, Australia) on one night, and 1.25% DM flavanone the next night.
Ringtail possums are difficult to maintain on an equivalent formulated diet, so they were offered Eucalyptus melliodora leaves that naturally contained either a low (0.3 mg.g–1 DM) or high (2.2 mg.g–1 DM), concentration of another PSM, sideroxylonal, a formylated phloroglucinol compound (FPC; a combination of the three isomers sideroxylonal A, B, and C), that strongly deters them from feeding at high concentrations (Marsh et al., 2003; Wiggins et al., 2006; Jensen et al., 2014). Ringtail possums were offered leaves from the two experimental E. melliodora trees 1 week in advance of the trials to reduce any effects of novelty.
Week-Long Temperature Exposure
Possums were allocated to starting temperatures of either 10, 18, or 26°C (four possums per species per temperature) using a randomised crossover design. Animals were exposed to these temperatures for seven nights, followed by two experimental feeding nights. Ringtail possums were offered E. rossii, a staple food tree, during the first seven nights, followed by leaves from one of the two E. melliodora trees on night eight, with the leaves from the alternative tree being offered on the ninth night. In contrast, brushtail possums were offered the basal diet during the first seven nights, and were offered either the basal diet or the basal diet containing 1.25% DM flavanone on night eight. The alternative diet was offered on the ninth night. The experiment was repeated until the intake of both of the respective diets had been measured for every possum at all three temperatures that is, a total of 27 days of experimentation.
Short Temperature Exposure
Ringtail possums were held at 18°C for 1 week preceding the experiment and offered branches of E. rossii leaves as the basal diet for this time. At 1600 h on the eighth day, they were divided equally into high (26°C) and low (10°C) temperature treatment groups. At 1630 h, half of the ringtail possums in each temperature treatment were offered the E. melliodora leaves with a higher sideroxylonal concentration while the other half were offered the E. melliodora leaves with a low sideroxylonal concentration. Food was removed and food intake measured at 0900 h the following morning. That afternoon, at 1600 h, all possums were transferred back to 18°C and offered the alternative diet. The experiment was repeated over the next two nights so that each possum received both high and low sideroxyonal leaves at 18°C, and at one of the experimental temperatures.
Brushtail possums were held at 18°C for 1 week preceding the experiment and offered the basal artificial diet during this time. At 1600 h on the eighth day, they were divided equally into high (26°C) and low (10°C) temperature treatment groups. At 1630 h, half of the brushtail possums in each temperature treatment were offered the diet containing 1.25% DM flavanone, while the other half were offered the basal diet. Food was removed and food intake measured at 0900 h the following morning. That afternoon, at 1600 h, all possums were transferred back to 18°C and offered the alternative diet. The experiment was repeated over the next two nights so that each possum received both of the diets at 18°C, and at one of the experimental temperatures.
In other words, six possums of each species were exposed to the high temperature treatment, and six the low, with the order of treatments randomised for each possum in a balanced design.
Statistical Analysis
Functional Clearance Assays
Six possums were either mis-injected or were too aroused from handling prior to injection, and did not fall asleep. These data points (one ringtail possum, four brushtail possums from the week-long exposure experiment, and one from the short exposure experiment) were excluded from statistical analyses.
Data from the week-long temperature exposure experiments were analysed in R studio using a generalised linear mixed model (Packages lme4, lmeTest) to test the effect of temperature on functional clearance times. We made an initial model with temperature and possum species and an interaction between the two as fixed effects. The model also included three random effects; individual possum identity, day on which the anaesthetic was administered, and the order in which each possum was subjected to the temperature treatments. Using an ANOVA (type III) we determined that the interaction was not significant, so the same model without the interaction term was compared using an ANOVA with a null model containing only random effects.
Data from the short-term exposure experiment were also analysed using a generalised linear mixed model with temperature as the fixed effect, and possum identity, and the day of anaesthetic administration as random effects. The model with temperature as the fixed effect was compared to a null model with random variables using an ANOVA.
Feeding Experiments
Feeding experiments were analysed separately for each possum species and each length of exposure using generalised mixed models (packages; lme4, MuMIn, lmerTest, plyr) in R studio. We included temperature, diet, and a temperature x diet interaction as fixed effects, and animal identity and experimental period as the random effects, with DMI as the dependent variable. An ANOVA was used to determine whether the fixed effects and the interaction term between fixed effects had a significant effect on the model.
Results
Resting Metabolic Rate and Body Temperature in Ringtail Possums
A second order polynomial best explained the relationship between RMR and Ta (F2,72 = 76.91, P < 0.001, R2 = 0.68). A single breakpoint in the curve was estimated at 19.3 ± 1.4°C (Figure 1A). However, this breakpoint was not significant (P = 0.454), and when AIC model selection criteria were used to select the best model to fit the data, the original second order polynomial model with no breakpoint was selected.
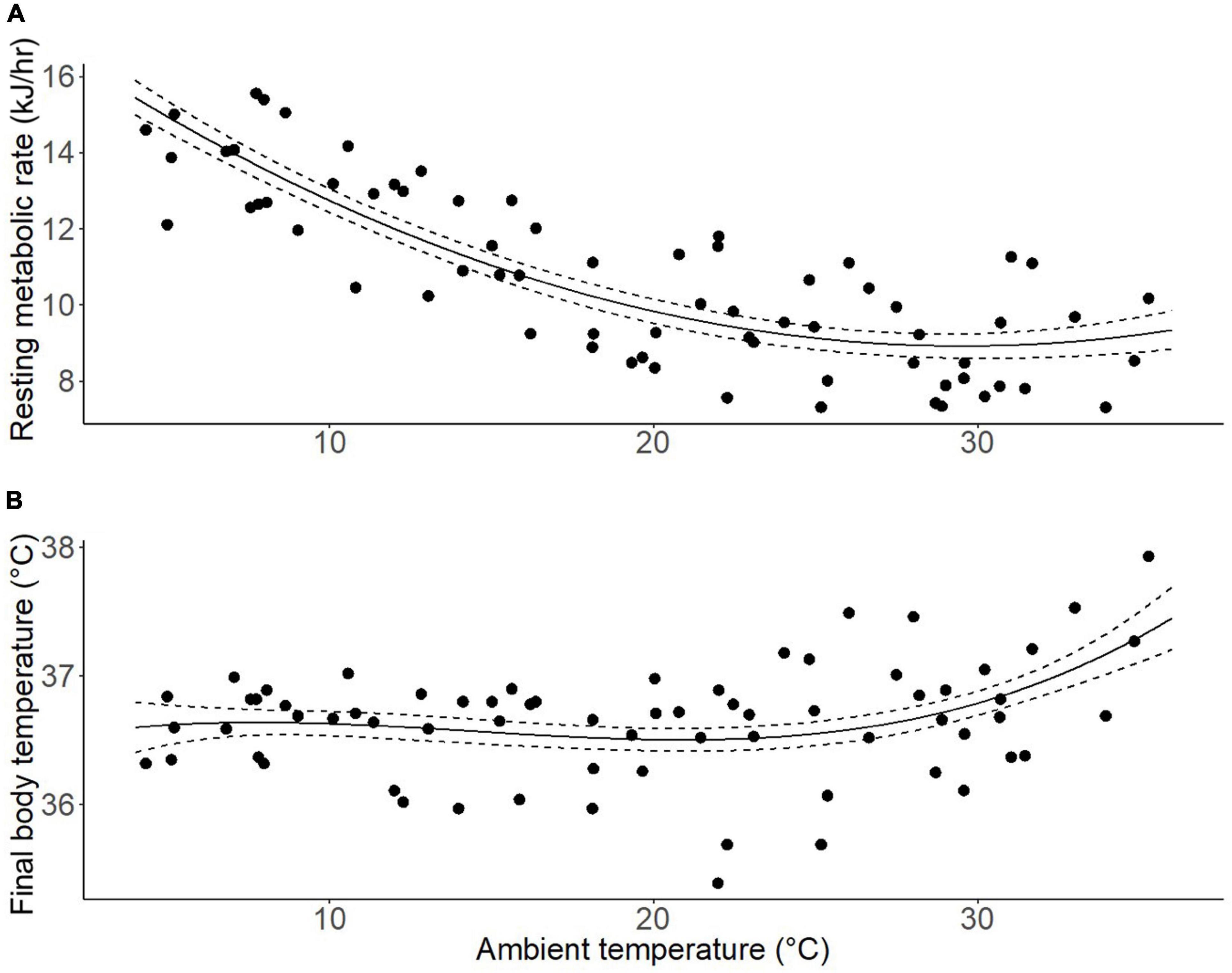
Figure 1. The relationship between ambient temperature and (A) resting metabolic rate (RMR), and (B) body temperature of ringtail possums (n = 12) at temperatures between 4 and 35°C. The solid lines are the best fit from the statistical model, with standard error (dashed lines); points are individual measurements.
The relationship between body temperature at the end of each respirometry measurement period and chamber temperature for that period was best explained by a third order polynomial (F3,65 = 4.63, P = 0.005, R2 = 0.18). A single breakpoint in the curve was estimated at 26.3 ± 0.9°C (Figure 1B). There was a trend for this breakpoint to be significant (P = 0.09), but when AIC model selection criteria was used to select the best model to fit the data, the original third order polynomial model with no breakpoint was selected.
Functional Clearance Assays
Week-Long Temperature Exposure
Both temperature (F2,35.15 = 24.70, P < 0.001) and possum species (F1,18.05 = 57.46, P < 0.001) significantly affected functional clearance times, and the model with temperature and possum species as fixed effects was significantly better than the null model (χ2 = 61.25, df = 3, P < 0.001). In both species, functional clearance times were 43% longer at 26°C compared to 10°C after possums were exposed for 7–8 days (Figure 2A). Ringtail possums always had a shorter functional clearance time than did brushtail possums (Figure 2A).
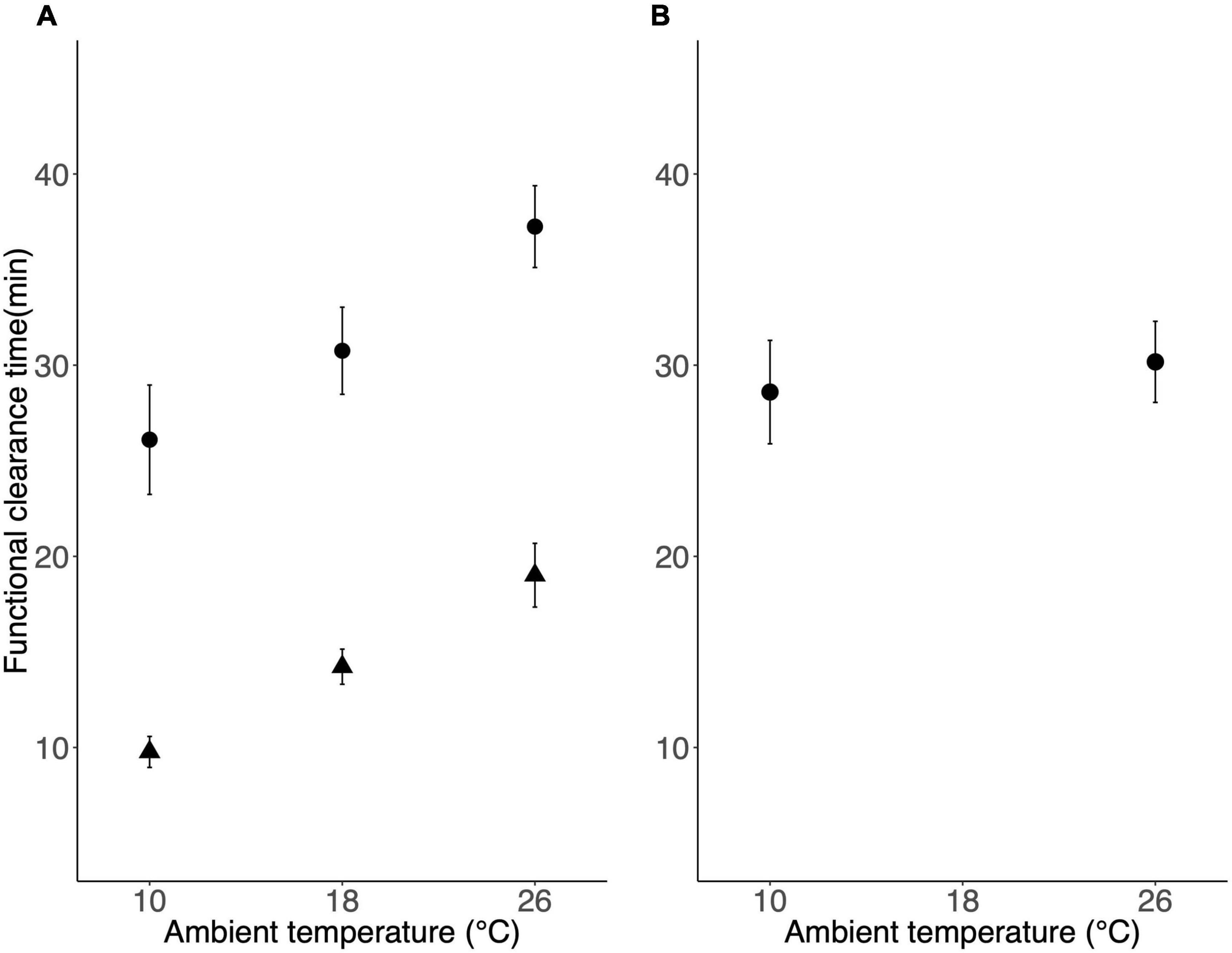
Figure 2. Functional clearance times (mean ± SE) of animals injected with 5 mg.kg–1 Alfaxalone for (A) ringtail possums (triangles, n = 11) and brushtail possums (circles, n = 9), following 7–8 days of exposure to different ambient temperatures and (B) for brushtail possums (n = 10) following short-term (<24 h) exposure to different ambient temperatures.
Short Temperature Exposure
There was no significant effect of ambient temperature on functional clearance times in common brushtail possums after a temperature exposure period of less than 24 h (χ2 = 1.26, df = 1, P = 0.26; Figure 2B). Mean functional clearance times differed by only 1.6 min between 10 and 26°C (Figure 2B), compared to the 10 min average difference after an exposure period of 1 week (Figure 2A).
Feeding Experiments
Week-Long Temperature Exposure
Brushtail possums ate less of the diet containing flavanone than of the basal diet following a week of exposure to each ambient temperature (F1,55 = 80.66, P < 0.001). There was also a negative relationship between food intake and temperature for brushtail possums (F2,55 = 25.29, P < 0.001). The interaction term was not significant, indicating that brushtail possums responded to Ta in a similar manner on both diets (Figure 3A).
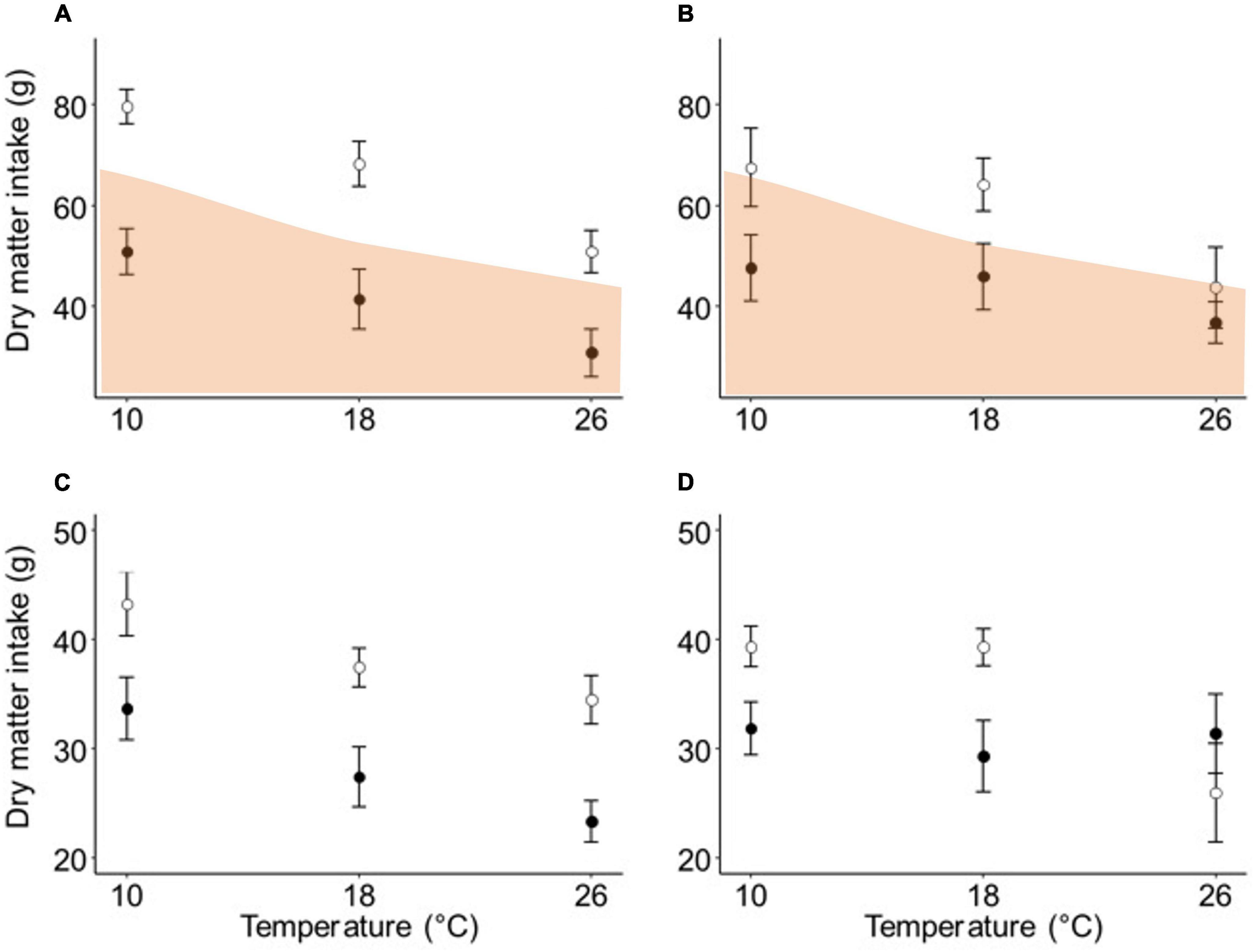
Figure 3. The effect of (A) week-long exposure, and (B) <24 h exposure to three ambient temperatures on the mean (± SE) food intake of brushtail possums (n = 12) offered a diet with (filled) or without (unfilled) flavanone. An estimate of minimum daily energy requirements (Cooper et al., 2018) is shown in orange. The effect of (C) week-long exposure, and (D) <24 h exposure to three ambient temperatures on the mean (± SE) food intake of ringtail possums (n = 12) offered Eucalyptus melliodora foliage with a high (filled) or low (unfilled) concentration of sideroxylonal.
After a week of exposure to each ambient temperature, ringtail possums ate less of the tree with the higher sideroxylonal concentration than the tree with the low concentration (F1,49.06 = 54.68, P < 0.001). There was a negative relationship between food intake and temperature (F2,55.78 = 14.59, P < 0.001), but the interaction between diet and temperature was not significant, showing that ringtail possums responded similarly to Ta for both trees (Figure 3C).
Short Temperature Exposure
In the short exposure experiment, brushtail possums ate less of the diet containing flavanone than of the basal diet (F1,28.36 = 16.83, P < 0.001), and there was a negative relationship between food intake and temperature (F2,31.59 = 6.08, P = 0.005). The interaction term was not significant, indicating that their response to Ta was similar for both diets (Figure 3B).
Ringtail possums again ate less of the tree with the higher sideroxylonal concentration relative to the tree with a low concentration (F1,26.64 = 3.33, P = 0.079), and, on average, there was a negative relationship between food intake and temperature (F2,32.14 = 3.48, P = 0.043). However, there was a significant interaction between temperature and diet (F2,27.87 = 5.21, P = 0.012), whereby ringtail possums maintained their intake of the tree with the higher sideroxylonal concentration across all ambient temperatures, but ate significantly less of the tree with a low sideroxylonal concentration at 26°C compared to 10 or 18°C (Figure 3D).
Changes in Body Mass
During the week-long acclimation feeding trials, relative to the mass the previous week, ringtail possums gained body mass after 1 week at 10°C, lost mass at 18°C, and lost mass at 26°C. Brushtail possums, gained body mass after 1 week at 10°C, gained at 18°C, and lost mass at 26°C (Table 1).
Discussion
The detoxification of PSMs presents a physiological challenge for mammalian herbivores, and our results demonstrate that this challenge is exacerbated at warmer ambient temperatures. We found a positive relationship between the functional clearance rate of the anaesthetic agent, Alfaxalone, and Ta when brushtail and ringtail possums were acclimated to ambient temperatures for 1 week. This change in detoxification rate was reflected in their feeding response to PSMs; possums ate less of PSM-rich diets after 1 week of exposure to higher ambient temperatures than they did at cooler temperatures. Our results support previous studies that have also documented temperature-mediated shifts in xenobiotic metabolism, PSM tolerance and PSM intake in herbivorous rodents (Dearing et al., 2008; Kurnath and Dearing, 2013; Kurnath et al., 2016; Windley and Shimada, 2020) and omnivorous birds (Chatelain et al., 2013).
Our results implicate depressed hepatic detoxification as the mechanism underpinning the reduced intake of foods containing sideroxylonal or flavanone. Although it would be ideal to demonstrate this directly, the specific detoxification pathways of sideroxylonal and flavanone have not been characterised in possums. Nevertheless, Alfaxalone is metabolised by hepatic pathways used by possums for other PSMs (i.e., oxidation by Cytochrome P450 enzymes and conjugation to glucuronic acid; McLean and Duncan, 2006; Warne et al., 2015). In further support, possums have previously been shown to regulate their intake of PSMs to remain below the limits of their detoxification capacity, and avoid toxicity (Marsh et al., 2005, 2006). Sideroxylonal and flavanone represent the two main classes of PSMs that strongly influence which eucalypts marsupial folivores choose to feed from Scrivener et al. (2004) and Marsh et al. (2014), and how much they eat from those trees (Jensen et al., 2014; Marsh et al., 2014, 2015). This means, if there was no effect of temperature on PSM tolerance, so long as PSMs had deterred feeding to below the level of food intake required to meet energy demands, intake of high PSM diets should be the same at different temperatures. Thus, the tolerance of herbivores to eucalypt PSMs (and any other plants containing PSMs that require hepatic metabolism) is reduced when possums are exposed to high ambient temperatures for prolonged periods. This could be considered a change in the effective toxicity of PSMs to herbivores. To further confirm this assertion a dose-response experiment with animals housed at different temperatures could be used.
It is notable that there was no difference in functional clearance rates of Alfaxalone between 10 and 26°C after short exposure of brushtail possums to these temperatures, and a reduced effect of short, relative to longer temperature exposure on the intake of PSM-rich diets in both possum species. This differs from the findings of Kurnath and Dearing (2013) who showed that there were changes in the functional clearance rate of hexobarbital in the desert woodrat (Neotoma lepida) after 3 h of exposure to different Ta. One reason for the different responses could be the large difference in body mass among these species. Common brushtail possums are much larger than woodrats (3 kg vs. 120 g). Body mass can increase thermal tolerance via heat storage such that small mammals are more metabolically responsive to shifts in ambient temperature compared to larger mammals (Riesenfeld, 1981; Porter and Kearney, 2009; Gardner et al., 2011). Ultimately, while mammals across a range of body sizes likely experience negative effects due to TDT, the physiological trade-off between thermoregulation and PSM detoxification could be more costly for smaller-bodied mammals.
The strong effect of Ta on feeding has been overlooked in mechanistic and habitat models that attempt to predict the responses of wild mammals to climate change. Instead, food intake is generally assumed to remain constant (e.g., Kearney et al., 2010). This oversight means that some species and populations may be at greater risk than is currently recognised. For example, Zhang et al. (2018) investigated whether areas considered to be suitable habitat for the giant panda (Ailuropoda melanoleuca) will be reduced in the future due to changing thermal environments, or due to loss of food from climate change, when, in fact, one may dramatically accelerate the other. If Ta, PSM tolerance, and feeding are integrated with nutrient availability, mechanistic models could be used more effectively to investigate how herbivore populations are likely to respond to future climate change (Youngentob et al., 2021).
Interestingly, higher temperatures alone limited nutrient intake by possums as effectively as high concentrations of PSMs. Possums offered foods with low PSM concentrations at 26°C ate little more than possums offered foods with high PSM concentrations at 10°C. Since we did not have respirometry data for brushtail possums we overlayed previous estimates from the literature of minimum daily energy requirements for this species on food intake in this experiment (Figure 3; Cooper et al., 2018). Brushtail possums would likely have met their minimum energy requirements when eating the basal diet (although our estimate of energy in the basal diet is total energy not digestible energy) but not when eating the flavanone diet in both experiments, excepting the 26°C treatment in the short exposure experiment. As a result, even possums maintained on low-PSM diets lost body mass at warmer Ta although some of this can be explained by changes in gut fill. The same phenomenon has been observed in domestic animals, with cattle (e.g., Bernabucci et al., 1999; Ominski et al., 2002), pigs (e.g., Close et al., 1971; Renaudeau et al., 2002), and poultry (e.g., Dale and Fuller, 1979; Baziz et al., 1996) all reducing food intake at high Ta to minimise metabolic heat production, despite increased metabolic rates. It is critical that researchers recognise that warmer average temperatures and more frequent heat wave events have the potential to substantially alter the nutritional landscape for wild mammalian herbivores, and to impede their nutrient intake.
While we can detect where an UCT and LCT of the classical “broken stick” model of thermoneutrality may be, our data suggest that physiological adjustments in response to Ta occur continuously. We agree with King (1964) that, while breakpoint regressions provide a useful way to statistically determine a traditional broken stick representation of thermoneutrality, in reality, this model is oversimplified and the transition zones are actually curvilinear. Furthermore, since most existing respirometry is done with fasted animals and over a short time, the effects on nutrient intake have been underappreciated. Thermoneutrality in free living animals might only be achievable by forgoing feeding. Despite this, estimating when temperatures are sufficiently high to necessitate heat reduction mechanisms is still useful. One approach could be to measure when the voluntary intake of food is no longer determined by requirements, but instead by limitations to heat dissipation, in the absence of PSMs. In this way, it would be possible to integrate how energy is being balanced, in terms of both food and heat, in a meaningful sense for future adaptation to changing temperatures. Animals eating less in the heat, must either do less, or must be more efficient in how they utilise what they eat.
Taken together, our results suggest that rising ambient temperatures have important implications for the ecology of mammalian herbivores. For example, possums may be forced to be more selective for individual trees with lower PSM concentrations (if present) within their environment, leading to a change in plant-herbivore interactions, which could have significant flow-on effects (Foley and Moore, 2005), and a need for conserved areas that encompass this variation in plant chemistry. Furthermore, relatively small changes in protein intake can lead to reduced reproductive success (White, 1983; DeGabriel et al., 2009), which could potentially result in large changes at the population level. These “amplifier” effects mean that even small changes in diet should be considered significant (White, 1983). For animals that obtain most of their water from food, reduced food intake could have additional critical impacts on their capacity to regulate body temperature at elevated Ta (Beale et al., 2018). It is imperative that future studies investigating the possible impacts of climate change on wild mammals consider that elevated ambient temperatures can moderate food intake and foraging behaviour, which is likely to impact populations and ecosystems.
Data Availability Statement
The raw data supporting the conclusions of this article will be made available by the authors, without undue reservation.
Ethics Statement
The animal study was reviewed and approved by the Australian National University Animal Experimentation Ethics Committee (A2015/24), and conforms to the Australian Code of Practice for the Care and Use of Animals for Scientific Purposes.
Author Contributions
MD, WF, BM, and KM conceived the ideas and designed methodology. PB, PC, and KM collected the data and led the writing of the manuscript. PB analysed the data with advice from PC and AK. All authors contributed critically to the drafts and gave final approval for publication.
Funding
This work was supported by grants from the Australian Research Council (DP140100228 to WF, MD, and BM, and DE120101263 to KM), and the National Science Foundation and the Australian Academy of Science (East Asia Pacific Summer Institute Fellowship to PC).
Conflict of Interest
The authors declare that the research was conducted in the absence of any commercial or financial relationships that could be construed as a potential conflict of interest.
Publisher’s Note
All claims expressed in this article are solely those of the authors and do not necessarily represent those of their affiliated organizations, or those of the publisher, the editors and the reviewers. Any product that may be evaluated in this article, or claim that may be made by its manufacturer, is not guaranteed or endorsed by the publisher.
Acknowledgments
We thank Johan Larson for advice and troubleshooting with the respirometry experiments, and Liam Bailey, Hwan-Jin Yoon and James Ruff for assistance with statistical analyses. Ivan Lawler and Ewen Lawler helped to catch ringtail possums, while Hannah Windley, Sarah Buchen and Sarah Webber assisted with the care of possums.
References
Barron, D. W., and Dundee, J. W. (1961). The recently introduced rapidly acting barbiturates; a review and critical appraisal in relation to thiopentone. Br. J. Anaesth. 33, 81–91. doi: 10.1093/bja/33.2.81
Baziz, H. A., Geraert, P. A., Padilha, J. C. F., and Guillaumin, S. (1996). Chronic heat exposure enhances fat deposition and modifies muscle and fat partition in broiler carcasses. Poultry Sci. 75, 505–513. doi: 10.3382/ps.0750505
Beale, P. K., Marsh, K. J., Foley, W. J., and Moore, B. D. (2018). A hot lunch for herbivores: Physiological effects of elevated temperatures on mammalian feeding ecology. Biol. Rev. Camb. Philos. Soc. 93, 674–692. doi: 10.1111/brv.12364
Bernabucci, U., Bani, P., Ronchi, B., Lacetera, N., and Nardone, A. (1999). Influence of short- and long-term exposure to a hot environment on rumen passage rate and diet digestibility by friesian heifers. J. Dairy Sci. 82, 967–973. doi: 10.3168/jds.S0022-0302(99)75316-6
Chatelain, M., Halpin, C. G., and Rowe, C. (2013). Ambient temperature influences birds’ decisions to eat toxic prey. Animal Behav. 86, 733–740. doi: 10.1016/j.anbehav.2013.07.007
Close, W. H., Mount, L. E., and Start, I. B. (1971). The influence of environmental temperature and plane of nutrition on heat losses from groups of growing pigs. Animal Product. 13, 285–294.
Cooper, C. E., Withers, P. C., Munns, S. L., Geiser, F., and Buttemer, W. A. (2018). Geographical variation in the standard physiology of brushtail possums (trichosurus): Implications for conservation translocations. Conserv. Physiol. 6:coy042 doi: 10.1093/conphys/coy042
Dale, N. M., and Fuller, H. L. (1979). Effect of low temperature, diet density, and pelleting on the preference of broilers for high fat rations. Poultry Sci. 58, 1337–1339.
Dearing, M. D. (2013). Temperature-dependent toxicity in mammals with implications for herbivores: A review. J. Compar. Physiol. B 183, 43–50. doi: 10.1007/s00360-012-0670-y
Dearing, M. D., Forbey, J. S., McLister, J. D., and Santos, L. (2008). Ambient temperature influences diet selection and physiology of an herbivorous mammal, neotoma albigula. Physiol. Biochem. Zool. 81, 891–897. doi: 10.1086/588490
Dearing, M. D., Skopec, M. M., and Bastiani, M. J. (2006). Detoxification rates of wild herbivorous woodrats (neotoma). Comp. Biochem. Physiol. A Mol. Integrat. Physiol. 145, 419–422. doi: 10.1016/j.cbpa.2006.07.016
DeGabriel, J. L., Moore, B. D., Foley, W. J., and Johnson, C. N. (2009). The effects of plant defensive chemistry on nutrient availability predict reproductive success in a mammal. Ecology 90, 711–719. doi: 10.1890/08-0940.1
Foley, W. J., and Moore, B. D. (2005). Plant secondary metabolites and vertebrate herbivores – from physiological regulation to ecosystem function. Curr. Opinion Plant Biol. 8, 430–435. doi: 10.1016/j.pbi.2005.05.009
Forbey, J. S., Liu, R., Caughlin, T. T., Matocq, M. D., Vucetich, J. A., Kohl, K. D., et al. (2018). Review: Using physiologically based models to predict population responses to phytochemicals by wild vertebrate herbivores. Animal 12:s383–s398. doi: 10.1017/S1751731118002264
Gardner, J. L., Peters, A., Kearney, M. R., Joseph, L., and Heinsohn, R. (2011). Declining body size: A third universal response to warming? Trends Ecol. Evol. 26, 285–291. doi: 10.1016/j.tree.2011.03.005
Gordon, C. J., Johnstone, A. F. M., and Aydin, C. (2014). Thermal stress and toxicity. Comp. Physiol. 4, 995–1016. doi: 10.1002/cphy.c130046
Jensen, L. M., Wallis, I. R., Marsh, K. J., Moore, B. D., Wiggins, N. L., and Foley, W. J. (2014). Four species of arboreal folivore show differential tolerance to a secondary metabolite. Oecologia 176, 251–258. doi: 10.1007/s00442-014-2997-4
Kaplanski, J., and Ben-Zvi, Z. (1980). Effect of chronic heat exposure on in-vitro drug metabolism in the rat. Life Sci. 26, 639–642.
Kearney, M. R., Simpson, S. J., Raubenheimer, D., and Kooijman, S. A. L. M. (2012). Balancing heat, water and nutrients under environmental change: A thermodynamic niche framework. Funct. Ecol. 27, 950–966.
Kearney, M. R., Wintle, B. A., and Porter, W. P. (2010). Correlative and mechanistic models of species distribution provide congruent forecasts under climate change. Conser. Lett. 3, 203–213. doi: 10.1371/journal.pone.0139194
Keplinger, M. L., Lanier, G. E., and Deichmann, W. B. (1959). Effects of environmental temperature on the acute toxicity of a number of compounds in rats. Toxicol. Appl. Pharmacol. 1, 156–161. doi: 10.1016/0041-008x(59)90136-x
King, J. R. (1964). Oxygen consumption and body temperature in relation to ambient temperature in the white-crowned sparrow. Compar. Biochem. Physiol. 12, 13–24. doi: 10.1016/0010-406x(64)90044-1
Krockenberger, A. K., Edwards, W., and Kanowski, J. (2012). The limit to the distribution of a rainforest marsupial folivore is consistent with the thermal intolerance hypothesis. Oecologia 168, 889–899. doi: 10.1007/s00442-011-2146-2
Kurnath, P., and Dearing, M. D. (2013). Warmer ambient temperatures depress liver function in a mammalian herbivore. Biol. Lett. 9:20130562. doi: 10.1098/rsbl.2013.0562
Kurnath, P., Merz, N. D., and Dearing, M. D. (2016). Ambient temperature influences tolerance to plant secondary compounds in a mammalian herbivore. Proc.R. Soc. B 283, 20152387. doi: 10.1098/rspb.2015.2387
Marsh, K. J., Foley, W. J., Cowling, A., and Wallis, I. R. (2003). Differential susceptibility to eucalyptus secondary compounds explains feeding by the common ringtail (pseudocheirus peregrinus) and common brushtail possum (trichosurus vulpecula). J. Comp. Physiol. B 173, 69–78. doi: 10.1007/s00360-002-0318-4
Marsh, K. J., Moore, B. D., Wallis, I. R., and Foley, W. J. (2014). Feeding rates of a mammalian browser confirm the predictions of a ‘foodscape’ model of its habitat. Oecologia 174, 873–882. doi: 10.1007/s00442-013-2808-3
Marsh, K. J., Saraf, I., Hocart, C. H., Youngentob, K., Singh, I.-P., and Foley, W. J. (2019). Occurrence and distribution of unsubstituted b-ring flavanones in eucalyptus foliage. Phytochemistry 160, 31–39.
Marsh, K. J., Wallis, I. R., and Foley, W. J. (2005). Detoxification rates constrain feeding in common brushtail possums (trichosurus vulpecula). Ecology 86, 2946–2954.
Marsh, K. J., Wallis, I. R., McLean, S., Sorensen, J. S., and Foley, W. J. (2006). Conflicting demands on detoxification pathways influence how common brushtail possums choose their diets. Ecology 87, 2103–2112. doi: 10.1890/0012-9658(2006)87[2103:cdodpi]2.0.co;2
Marsh, K. J., Yin, B., Singh, I. P., Saraf, I., Choudhary, A., Au, J., et al. (2015). From leaf metabolome to in vivo testing: Identifying antifeedant compounds for ecological studies of marsupial diets. J. Chem. Ecol. 41, 513–519. doi: 10.1007/s10886-015-0589-3
McDowell, A., and McLeod, B. J. (2007). Physiology and pharmacology of the brushtail possum gastrointestinal tract: Relationship to the human gastrointestinal tract. Adv. Drug Delivery Rev. 59, 1121–1132. doi: 10.1016/j.addr.2007.06.012
McLean, S., and Duncan, A. J. (2006). Pharmacological perspectives on the detoxification of plant secondary metabolites: Implications for ingestive behavior of herbivores. J. Chem. Ecol. 32, 1213–1228. doi: 10.1007/s10886-006-9081-4
Miller, E. R., and Folwer, M. E. (2015). “Fowler’s zoo and wild animal medicine,” in Fowler’s Zoo and Wild Animal Medicine, eds E. R. Miller and M. E. Folwer (St. Louis: Elsevier).
Muggeo, V. M. R. (2003). Estimating regression models with unknown break-points. Statist. Med. 22, 3055–3071. doi: 10.1002/sim.1545
Ominski, K. H., Kennedy, A. D., Wittenberg, K. M., and Nia, S. A. M. (2002). Physiological and production responses to feeding schedule in lactating dairy cows exposed to short-term, moderate heat stress. J. Dairy Sci. 85, 730–737. doi: 10.3168/jds.S0022-0302(02)74130-1
Pahl, L. (1987a). Feeding-behavior and diet of the common ringtail possum, pseudocheirus-peregrinus, in eucalyptus woodlands and leptospermum thickets in southern victoria. Aust. J. Zool. 35, 487–506.
Pahl, L. (1987b). Survival, age-determination and population age structure of the common ringtail possum, pseudocheirus peregrinus, in a eucalyptus woodland and a leptospermum thicket in southern victoria. Aust. J. Zool. 35, 625–639.
Pass, G. J., McLean, S., Stupans, I., and Davies, N. (2001). Microsomal metabolism of the terpene 1,8-cineole in the common brushtail possum (trichosurus vulpecula), koala (phascolarctos cinereus), rat and human. Xenobiotica 31, 205–221. doi: 10.1080/00498250110043535
Porter, W. P., and Kearney, M. (2009). Size, shape, and the thermal niche of endotherms. Proc.Natl. Acad. Sci. 106, 19666–19672. doi: 10.1073/pnas.0907321106
Renaudeau, D., Collin, A., Yahav, S., de Basilio, V., Gourdine, J. L., and Collier, R. J. (2012). Adaptation to hot climate and strategies to alleviate heat stress in livestock production. Animal 6, 707–728. doi: 10.1017/S1751731111002448
Renaudeau, D., Quiniou, N., and Dubois, S. (2002). Effects of high ambient temperature and dietary protein level on feeding behavior of multiparous lactating sows. Animal Res. 51, 227–243.
Riek, A., and Geiser, F. (2013). Allometry of thermal variables in mammals: Consequences of body size and phylogeny. Biol. Rev. 88, 564–572. doi: 10.1111/brv.12016
Riesenfeld, A. (1981). The role of body mass in thermoregulation. Am. J. Phys. Anthropol. 55, 95–99.
Saraf, I., Marsh, K. J., Vir, S., Foley, W. J., and Singh, I. P. (2017). Quantitative analysis of various b-ring unsubstituted and substituted flavonoids in ten australian species of eucalyptus. Nat. Product Commun. 12, 1695–1699.
Scrivener, N. J., Johnson, C. N., Wallis, I. R., Takasaki, M., Foley, W. J., and Krockenberger, A. K. (2004). Which trees do wild common brushtail possums (trichosurus vulpecula) prefer? Problems and solutions in scaling laboratory findings to diet selection in the field. Evol. Ecol. Res. 6, 77–87.
Vogelnest, L., and Woods, R. (2008). Medicine of australian mammals. Medicine of australian mammals.
Warne, L. N., Beths, T., Whittem, T., Carter, J. E., and Bauquier, S. H. (2015). A review of the pharmacology and clinical application of alfaxalone in cats. Vet. J. 203, 141–148. doi: 10.1016/j.tvjl.2014.12.011
White, R. G. (1983). Foraging patterns and their multiplier effects on productivity of northern ungulates. Oikos 40, 377–384.
Wiggins, N. L., Marsh, K. J., Wallis, I. R., Foley, W. J., and McArthur, C. (2006). Sideroxylonal in eucalyptus foliage influences foraging behaviour of an arboreal folivore. Oecologia 147, 272–279. doi: 10.1007/s00442-005-0268-0
Windley, H. R., and Shimada, T. (2020). Cold temperature improves tannin tolerance in a granivorous rodent. J. Anim. Ecol. 89, 471–481. doi: 10.1111/1365-2656.13119
Winter, J. (1980). Tooth wear as an age index in a population of the brush-tailed possum, trichosurus vulpecula (kerr). Wildlife Res. 7, 359–363.
Youngentob, K. N., Lindenmayer, D. B., Marsh, K. J., Krockenberger, A. K., and Foley, W. J. (2021). Food intake: An overlooked driver of climate change casualties? Trends Ecol. Evol. 36, 676–678. doi: 10.1016/j.tree.2021.04.003
Keywords: climate change, Eucalyptus, functional clearance rate, foraging, temperature-dependent toxicity, detoxification, marsupial, folivory
Citation: Beale PK, Connors PK, Dearing MD, Moore BD, Krockenberger AK, Foley WJ and Marsh KJ (2022) Warmer Ambient Temperatures Depress Detoxification and Food Intake by Marsupial Folivores. Front. Ecol. Evol. 10:888550. doi: 10.3389/fevo.2022.888550
Received: 03 March 2022; Accepted: 13 May 2022;
Published: 09 June 2022.
Edited by:
Jordi Figuerola, Doñana Biological Station (CSIC), SpainReviewed by:
Perry S. Barboza, Texas A&M University, United StatesZhu Wanlong, Yunnan Normal University, China
Copyright © 2022 Beale, Connors, Dearing, Moore, Krockenberger, Foley and Marsh. This is an open-access article distributed under the terms of the Creative Commons Attribution License (CC BY). The use, distribution or reproduction in other forums is permitted, provided the original author(s) and the copyright owner(s) are credited and that the original publication in this journal is cited, in accordance with accepted academic practice. No use, distribution or reproduction is permitted which does not comply with these terms.
*Correspondence: Phillipa K. Beale, cGhpbGxpcGEuYmVhbGVAZ21haWwuY29t