- Limnological Institute, University of Konstanz, Konstanz, Germany
Over the past decades, the zebra mussel (Dreissena polymorpha) and quagga mussel (D. rostriformis bugensis) invaded multiple freshwater systems and posed major threats to the overall ecosystem. In Lake Constance where zebra mussels invaded in the 1960s, the quagga mussel invasion progressed at a very high rate since 2016, providing an opportunity to study the ecological impact of both species at an early stage. We conducted a field experiment in the littoral region of the lake and monitored differences in macroinvertebrate community colonization. We used standardized stone substrates, which were blank, glued with empty shells of mussels, with living adult quagga mussels, and with living adult zebra mussels. Empty shells and the shells of both living adult quagga and zebra mussels created more colonization areas for newly settled macroinvertebrates. The abundance of newly settled quagga mussels was higher than zebra mussels, indicating the outcompeting behavior of quagga mussels. We used stable isotopes (δ13C and δ15N) of both dreissenids and their potential competitors, which include two snail species (New Zealand mud snail Potamopyrgus antipodarum and faucet snail Bithynia tentaculate) and additional invasive gammarid species (killer shrimp Dikerogammarus villosus), in order to investigate their feeding ecology and to evaluate their potential impacts on macroinvertebrate community. The δ13C and δ15N of neither the newly settled quagga mussels nor the well-established zebra mussels differed significantly among various treatments. Newly settled quagga mussels had higher δ13C values than newly settled zebra mussels and showed similar differences in all four stone setups. During the experimental period (with quagga and zebra mussels still coexisting in some regions), these two dreissenids exhibited clear dietary (isotopic) niche segregation. The rapid expansion of invasive quagga mussels coupled with the higher mortality rate of zebra mussels might have caused a dominance shift from zebra to quagga mussels. The study offers the first overview of the progressive invasion of quagga mussel and the reaction of zebra mussels and other newly settled macroinvertebrates, and compliments the hypothesis of facilitative associations between invasive dreissenids. Results provide an experimental benchmark by which future changes in trophic ecology and invasion dynamics can be measured across the ecosystem.
Introduction
The spread of invasive species in freshwater environments is observed as a global phenomenon (Lodge et al., 1998; Francis and Chadwick, 2012; Jones et al., 2021). While investigators have recorded multiple impacts of invasive species on native or established biological communities (Gherardi and Acquistapace, 2007; Stiers et al., 2011; Boltovskoy and Correa, 2015), the prediction of the impact of a newly established non-native species remains a challenge (Williamson, 1999; Roy et al., 2014). This is mainly due to the fact that impacts of a given species may vary over space and time, and might equally depend on the specificity of the invaded ecosystem (Strayer and Malcom, 2006; Karatayev et al., 2021). Moreover, the impact might be potentially measurable only after a considerable delay following invasion (Crooks, 2005; Ricciardi et al., 2013).
Ecologists have, therefore, exerted efforts to understand the potential impacts of invasive species on freshwater systems by utilizing different approaches that include documented knowledge of the literature (Copp et al., 2009; Roy et al., 2014) coupled with mathematical and statistical modeling (Ricciardi, 2003; Kulhanek et al., 2011). While these studies often depend on existing trends, it is more difficult to predict the impacts of the invasion when establishments occur in a novel location with no prior baseline data (Kulhanek et al., 2011). Moreover, some aquatic systems are invaded by more than one bio-physically similar or genetically related taxa, although the important species-specific traits seem to be underestimated (Ricciardi, 2003).
Two highly invasive taxa of particular concern in multiple freshwater lakes and rivers are dreissenids of the Ponto-Caspian origin (Son, 2007; Strayer et al., 2020): quagga mussel (Dreissena rostriformis bugensis) and zebra mussel (D. polymorpha) (Molloy et al., 2007; Evariste et al., 2018). The establishment of these two dreissenids has been extensively reported with synergistic and antagonistic shifts in the community structure of pre-existing freshwater ecosystems (Mörtl and Rothhaupt, 2003; Sousa et al., 2011). Specific changes following invasion include a measurable increase in benthic macroinvertebrate density (Ricciardi, 2003). As such, the physical structural complexity of the mussel bed provides a safe haven or fine-scale refugia against predators (González and Downing, 1999; Ward and Ricciardi, 2007), providing protection against unintended movement of waves that facilitate macroinvertebrates (Ricciardi et al., 1997; Stewart et al., 1998). Evidently, the biofouling of mussels has deleterious effects on certain macroinvertebrates (Sousa et al., 2011; Strayer and Malcom, 2018), and in many lakes, the native bivalves showed a large decrease in abundance after the invasion of dreissenids (Strayer and Malcom, 2007; Burlakova et al., 2014).
Most interestingly, detritivores and grazing herbivorous species may benefit from nutrient release following pseudo-feces excretion and biofilm deposition from dreissenids (Kobak et al., 2013). Following mussel invasion, remarkable increases in macroinvertebrate abundance and biomass of gammarids and snails have been documented (MacIsaac, 1996; Bially and MacIsaac, 2000). Finally, as posited by the invasional meltdown hypothesis, in multiple invaded ecosystems, the positive interactions among invaders initiate positive population-level feedback that intensifies influence and promotes further invasions (Simberloff and von Holle, 1999).
In the past decades, several invasive freshwater mussels have colonized most of the European perialpine lakes. In particular, the zebra and quagga mussels pose continuous challenges in Lake Constance. Adult quagga mussel was first reported in the lake in 2016 (Haltiner et al., 2022), while zebra mussel has colonized the lake since the late 1960s (Walz, 1973). In fact, the quagga mussel recently increased its range and density, and replaced the zebra mussel over most parts of the lake, only 2 years after the first record. These observations, combined with the existing increasing invasion rate and invasive species in Lake Constance, tentatively support the “invasional meltdown” model (Simberloff and von Holle, 1999). It could be argued, at least theoretically, that Lake Constance is experiencing an “easier” invasion as the cumulative number of introduced species increases and as facilitative interactions are expected to exacerbate the effect of these invaders. Indeed, the impacts of zebra mussels on the macroinvertebrate communities of Lake Constance have been well investigated (Mörtl and Rothhaupt, 2003; Gergs and Rothhaupt, 2008, 2015). However, the impacts of the recent invader quagga mussels remain unknown.
Therefore, the first objective of this study was to measure and compare the potential impacts of quagga and zebra mussels on macroinvertebrate communities in Lake Constance. Using an experimental approach, we aimed to observe macroinvertebrate colonization across four series of manipulated stone substrates treated with different specimens: blank stones, dead shell analogs of dreissenids, living adult quagga mussels, or living adult zebra mussels. By simulating these four potential substrate “scenarios” here, we aimed to improve our knowledge regarding these two highly concerning invasive species in the absence of robust baseline data (specifically for quagga mussels) for Lake Constance. Understanding food-web interaction using possible scenarios is critical for accurate prediction of future impacts, which would facilitate undertaking necessary prevention and management approaches (Byers et al., 2002). We expected that substrates with living mussel and shell treatments would cause higher macroinvertebrate abundance compared to the blank treatments.
Unraveling the mechanisms that allow multiple aquatic invasive species to coexist can provide insights into freshwater community ecology. However, dietary information on component species of these assemblages is often difficult to obtain. Therefore, our second objective was to identify potential food sources and estimate the relative trophic position of newly settled macroinvertebrate species (on the stone substrates). Therefore, we measured stable carbon and nitrogen isotopes (δ13C and δ15N) in soft tissue specimens obtained from five macroinvertebrate species (Gergs et al., 2011; Tran et al., 2015). Using the four manipulated substrate setups, we examined whether trophic niche differentiation and microhabitat segregation explain their co-existence in the lake. Both mussels are able to exploit phytoplankton or suspended detritus as significant energy sources. Yet, it is expected that the species might compete with each other in areas of sympatry. Therefore, we hypothesized that the presence of these mussels will influence not only the community composition but also the food resources of newly settled macroinvertebrates. Specifically, we measured and compared the isotopic niche size of each species and that of the whole community, and assessed isotopic niche overlap among the two invasive mussel species using the δ13C and δ15N values.
At present, the vast majority of dreissenids found in the littoral zone of Lake Constance are quagga mussels, while a short time ago, this area was dominated by zebra mussels. Species-specific population size may fluctuate because of the variation in the adult mortality rate, and the survival of adult mussels may be an important component of competitive displacement. Therefore, using this in situ setup, we opportunistically examined the survivorship of adult quagga and zebra mussels in the four treatments.
Materials and Methods
Study Area
The manipulated stone substrate experiment was conducted at the littoral area in Upper Lake Constance (N: 47°41′31.0″, E: 9°12′07.0″), one of the two major basins in Lake Constance, Germany. The littoral area has predominantly stony gravel and pebble substrate. It is surrounded by a semi-natural habitat, with scarce suburban housing and with hardly any extensive artificial land use (Gergs et al., 2011). The stone substrates were deployed at a depth of 0.80 m.
Field Experimental Design
The experimental design followed the former study by Mörtl and Rothhaupt (2003). The standardized stone substrates (commercially available concrete cobblestones) had an upper surface area of 121 cm2. The upper surface area of each stone substrate was manipulated to obtain four treatments with eight replicates each. The first control treatment (B treatment) consisted of blank and clean stones with no shells and no mussels on them. Treatment 2 (S treatment) consisted of stones with eviscerated shells of mussels that were glued together to mimic intact dead mussels (Botts et al., 1996). Shells were glued to the substrate at their posterior side and positioned in a similar direction, simulating field observations of adult dead mussels at the lakeshore. Treatment 3 (Q treatment) and treatment 4 (Z treatment) consisted of stones with upper surfaces covered by living adult quagga and zebra mussels, respectively. Living mussels of individual species were glued to the substrate at their posterior side. Care was taken to ensure that individual mussels could open and close their shells as in natural cases to ensure survival. The abundance of shells or living mussels on the upper surfaces of stones was equivalent to 3,719 ± 413 ind./m2. The substrate appearance resembled that of a natural mussel bed or druse, simulating field observations of living mussels. Caution was taken to ensure that glued individual mussels or shells were approximately equidistant.
To prepare the stone treatments, adult quagga and zebra mussels of ca. 20 mm shell length had been collected by hand at a littoral site of the lake where the two dreissenids coexisted by then (i.e., June 2018). Based on the morphological traits, mussels had been identified and separated as quagga or zebra mussels (Pathy and Mackie, 1993; Kerambrun et al., 2018). To prepare the shell treatments, shells of eviscerated mussels were washed and dried in the laboratory at room temperature for 72 h. Stones were deployed at the study site for the experiment between June and July 2018 (28 days). Elsewhere, artificial substrates have been reported to reach stabilized newly settled macroinvertebrate communities within 20–60 days (Roby et al., 1978; Wise and Molles, 1979). Therefore, through the experimental period of 28 days, we envisioned to capture the invasion processes and evaluate their effects on the density and taxonomic richness of newly settled macroinvertebrates within the first 4 weeks (short time duration).
On the last day of the experiment, all the stones were collected from the lake, thoroughly washed, and carefully inspected for attached (newly settled) macroinvertebrates. The newly settled macroinvertebrates were gently removed with a brush, washed, and collected from each stone after filtering through a 200 μm mesh. We took all the newly settled macroinvertebrates in the entire area of the respective stone into account. Afterward, living adult quagga and zebra mussels that had been glued on the stones were gently removed from their respective stones, and their survival was assessed by observing shell gape and soft tissue. If no soft tissue was present inside the shells, then mortality was recorded. One stone in the Z treatment was upside down at the end of the experiment, and this sample was omitted from further analysis.
Macroinvertebrate Counting
In the laboratory, all newly settled macroinvertebrate samples were sorted and counted under a dissecting microscope (× 10 magnification, Zeiss Stemi 2000-C). Identification was made to species or genus level, except for Diptera, which mainly included Chironomidae. Chironomidae were identified at the subfamily level due to morphological ambiguity at their smallest size ranges. The size of macroinvertebrates was measured using a microscope equipped with an ocular micrometer. Newly settled dreissenids larger than 1 mm in length were separated into quagga or zebra mussels based on morphological traits. We pooled the small dreissenids (≤ 1 mm in length) into one group.
Stable Isotope Analysis
The five common newly settled macroinvertebrate species recorded from the experiment, which included the two mussel species (quagga mussel D. rostriformis bugensis and zebra mussel D. polymorpha), two snail species (New Zealand mud snail Potamopyrgus antipodarum and faucet snail Bithynia tentaculata), and one gammarid species (killer shrimp Dikerogammarus villosus), were used for stable isotope measurement. The soft tissues of the two mussel species and two snail species were eviscerated for stable isotope analysis. Individuals of the gammarid species were used for stable isotope analysis.
Lipid content was removed from dried and pulverized samples (Post et al., 2007; Rothhaupt et al., 2014) and weighed (ca. 0.85 mg) in tin cups to the nearest 0.001 mg, using a micro-analytical balance. The 13C/12C or 15N/14N ratios of samples were determined using two instruments. Half of the samples were measured at the stable isotope lab of the University of Konstanz in Germany. Here, samples were combusted in a vario PYRO cube elemental analyzer (Elementar Analysensysteme, Germany) at 1,120°C. Resulting CO2 and N2 were separated by gas chromatography and passed into an IsoPrime isotope ratio mass spectrometer (IRMS; Isoprime Ltd., Manchester, United Kingdom). The rest of the samples were measured at the stable isotope laboratory of the University of Erlangen-Nürnberg, Germany. Here samples were combusted in a Costech Elemental Analyzer (ECS 4010; Costech International, Pioltello, Italy; now NC Technologies, Bussero, Italy). The resulting gases were separated and measured using Thermo Scientific Delta V plus IRMS (Thermo Fisher Scientific, Bremen, Germany). The repeatability and comparability of results obtained from the two laboratories were first ensured by measuring multiple macroinvertebrate samples (“dummy” samples) in both laboratories before proceeding with the samples used for this experiment.
All stable isotope values (δ13C or δ15N) were reported in the δ notation (per mill) where δ = [1,000 × (Rsample/Rstandard) –1] ‰, relative to the Pee Dee Belemnite (PDB) for carbon and atmospheric N2 for nitrogen in parts per thousand deviations (‰).
Data Analysis
Non-parametric Kruskal–Wallis test was used to analyze the differences in the abundances of newly settled macroinvertebrates among four different treatments, as the normality of the whole dataset was not reached (Shapiro–Wilk test). Dunn’s test was used for post hoc pairwise comparisons. Non-parametric Mann–Whitney Wilcoxon test was used to compare the abundances of quagga and zebra mussels in each treatment.
To identify differences in community composition across all treatments, we examined dissimilarities among macroinvertebrate assemblages by applying non-metric multidimensional scaling (NMDS) using Bray–Curtis dissimilarities. Mean abundance data (ind./m2 of newly settled macroinvertebrate per taxon) were used in the analysis, and data were standardized by the decostand function using the method “total.” We then assessed whether the composition of the macroinvertebrate community differed among treatments using analysis of similarity (ANOSIM). In order to reduce distortion of macroinvertebrate assemblage in the community, taxa accounting for less than 0.5% of the total mean abundance per treatment were excluded from the analysis.
Graphical summaries of the mean abundance of abundant newly settled macroinvertebrates (ind./m2 + SE) per treatment were made for taxa that can be identified up to the genus or species level, which included D. rostriformis bugensis, D. polymorpha, Dreissena (≤ 1 mm), P. antipodarum, B. tentaculata, D. villosus, Katamysis warpachowskyi, and Caenis (Figure 1). A summation of abundances for all the newly settled macroinvertebrates present in each sample is expressed as the total abundance.
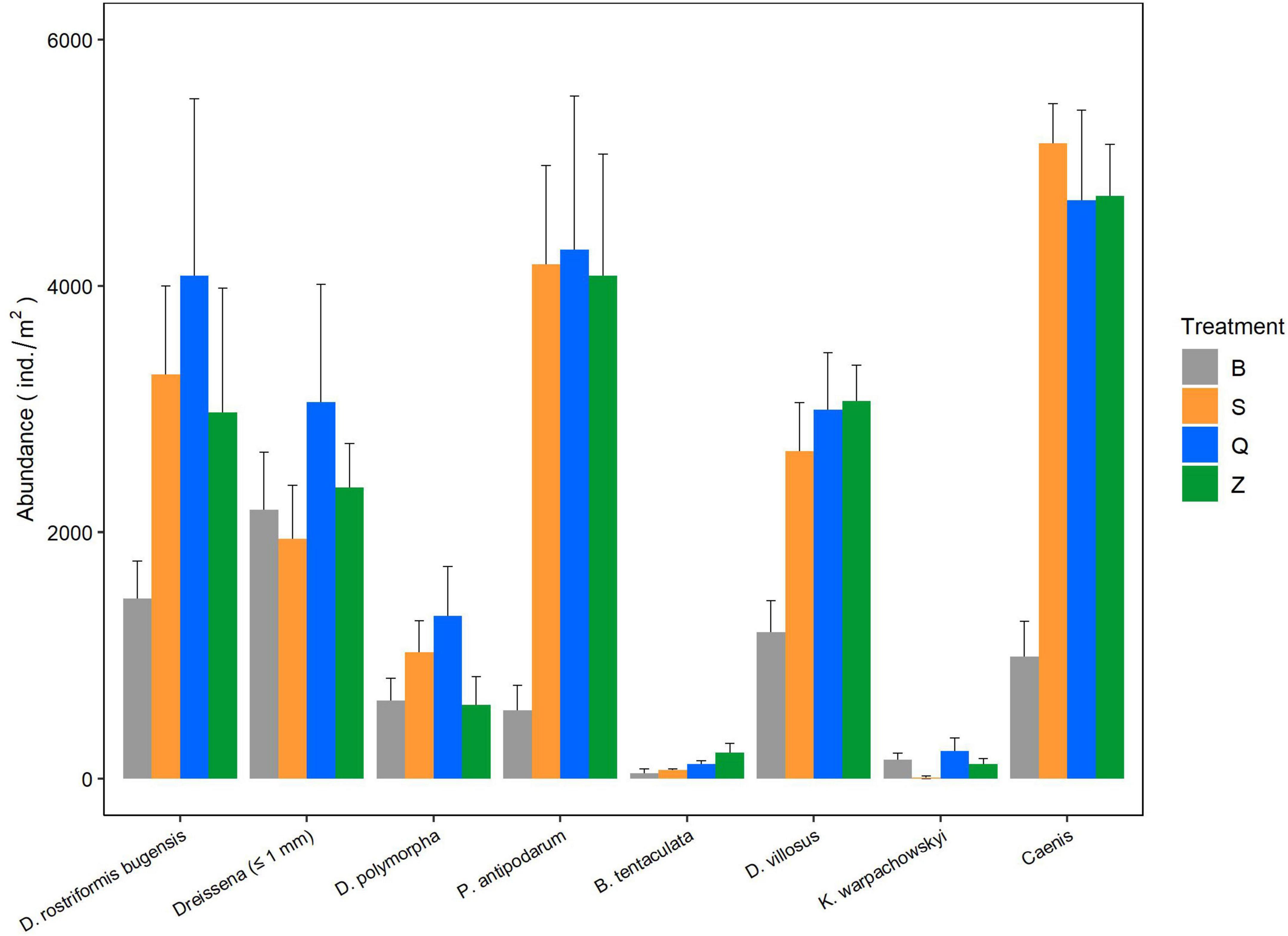
Figure 1. The abundance of abundant newly settled macroinvertebrates in four different treatments. Taxa that can be identified to species or genus level are presented. Error bars indicate standard errors.
The stable isotope values of newly settled macroinvertebrates were not normally distributed (Shapiro–Wilk test). Kruskal–Wallis test was used to analyze the differences in δ13C and δ15N values for the five main newly settled macroinvertebrate species among four treatments, and Dunn’s test was used for post hoc pairwise comparisons. Mann–Whitney Wilcoxon test was used to compare the δ13C and δ15N values of quagga and zebra mussels in each treatment. We used Bayesian standard ellipse area (SEA) and size-corrected standard ellipse area for small samples (SEAc) to describe species and community isotopic niche width of newly settled macroinvertebrates among different treatments. We also calculated and reported the 95% confidence intervals of SEA using 10,000 posterior Markov chain Monte Carlo draws (Jackson et al., 2011; Catry et al., 2016).
All calculations and figures were conducted using R (R Core Team, 2021) with the package readxl, tidyverse, DescTools, ggplot2, ggpubr, vegan, and SIBER, in order to import data, reshape data, conduct Dunn’s test, make figures, arranges figures, conduct NMDS analysis, and analyze Bayesian standard ellipse areas, respectively.
Results
The Abundance of Newly Settled Macroinvertebrates
The most abundant taxa in all the four treatments were mussels [D. rostriformis bugensis, D. polymorpha, and Dreissena spp. (≤ 1 mm)], snails (P. antipodarum and B. tentaculata), gammarids (D. villosus), mysids (K. warpachowskyi), mayfly nymphs (Caenis spp.), and chironomid larvae (Chironominae and Orthocladiinae) (Figures 1, 2). Other taxa, such as Trichoptera, that occur infrequently were used only for total abundance analysis.
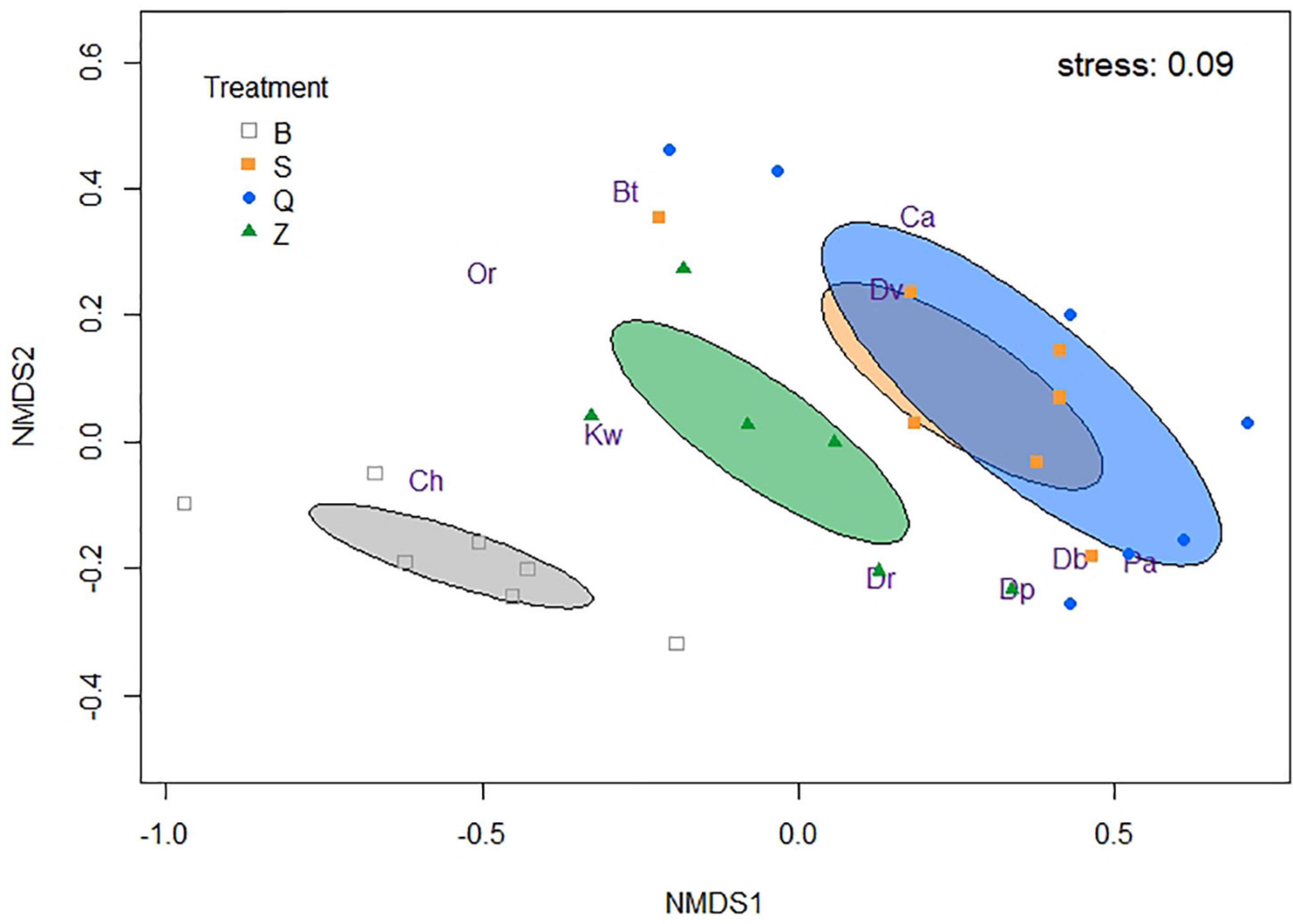
Figure 2. Non-metric multidimensional scaling (NMDS) ordination plot of newly settled macroinvertebrate abundances, representing the community structure in four different treatments. Ellipses draw standard errors of the treatments and show a 95% confidence interval. Db means D. rostriformis bugensis, Dp means D. polymorpha, Dr means Dreissena (≤ 1 mm), Pa means P. antipodarum, Bt means B. tentaculata, Dv means D. villosus, Kw means K. warpachowskyi, Ca means Caenis, Ch means Chironominae, and Or means Orthocladiinae.
Differences in the abundances of newly settled macroinvertebrates among the four treatments are presented in Table 1. The abundances of newly settled quagga mussels were not significantly different. The abundances of newly settled zebra mussels were also not significantly different. The abundances of newly settled snail P. antipodarum and mayfly nymphs Caenis were significantly different (p < 0.01), with the B treatment having the lowest numbers. The abundances of newly settled gammarid D. villosus were less in B treatments than in Q and Z treatments (p < 0.05). The abundances of newly settled chironomid larvae of subfamily Chironominae were higher in Z and B treatments than in S and Q treatments (p < 0.05). Total abundances of all newly settled macroinvertebrates were significantly higher in Z treatments than in B treatments (p = 0.002).
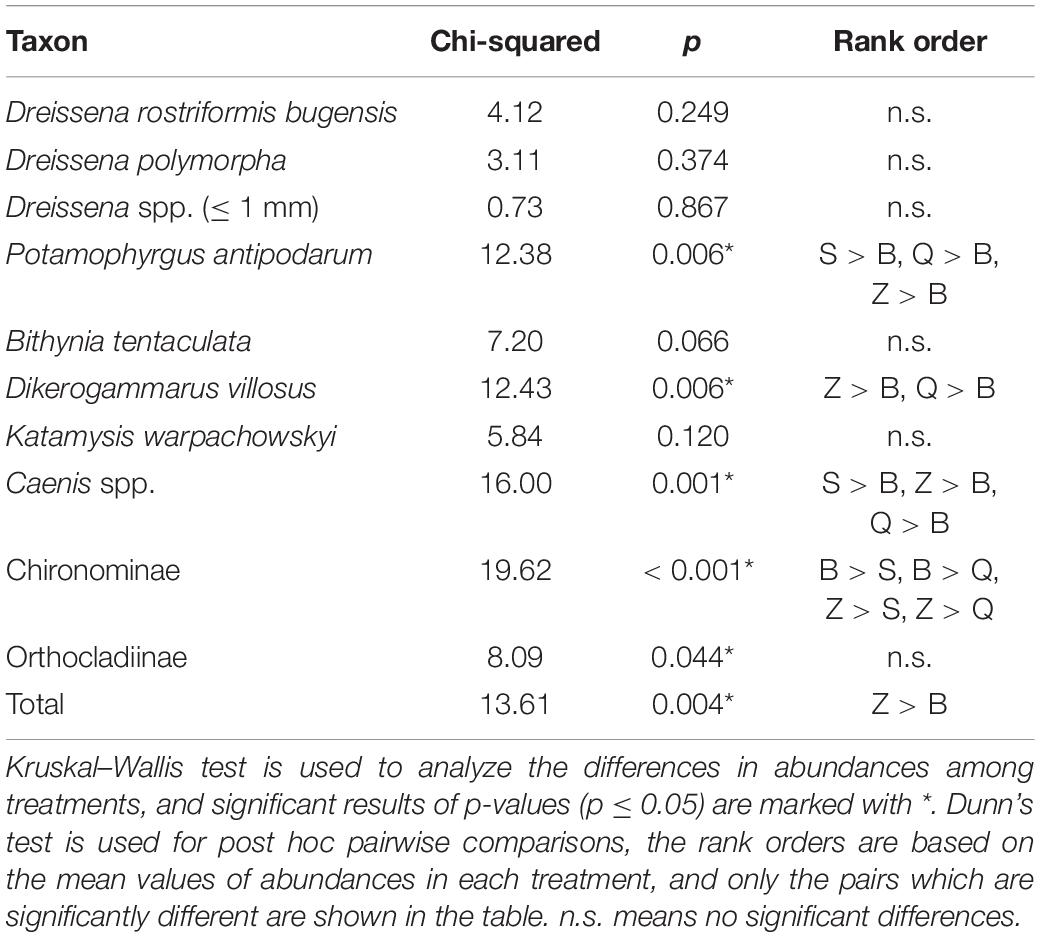
Table 1. The differences in abundances of newly settled macroinvertebrates among four different treatments.
The total abundance of quagga mussels in the entire experiment area was higher than the total abundance of zebra mussels. In Z treatments, the abundance of newly settled quagga mussels was significantly higher than that of zebra mussels (p = 0.021), and additional information is provided in Supplementary Table 1.
In the NMDS plot (stress 0.09), the average composition of colonizing macroinvertebrate community across treatments appeared to be most segregated by mussel treatments (ANOSIM global test R = 0.473, p = 0.001) (Figure 2). For this 28-day experiment, Q and S treatments were closely grouped and separated from the Z treatment, while the B treatment was more isolated on the plot.
The mortality rate of glued adult zebra mussels (24.00 ± 2.28%) was significantly higher than that of glued adult quagga mussels (7.33 ± 1.33%) (p < 0.01; Figure 3). The abundance of newly settled chironomid larvae (subfamily Chironominae) showed a positive correlation with the mortality rate of adult dreissenids (Figure 3).
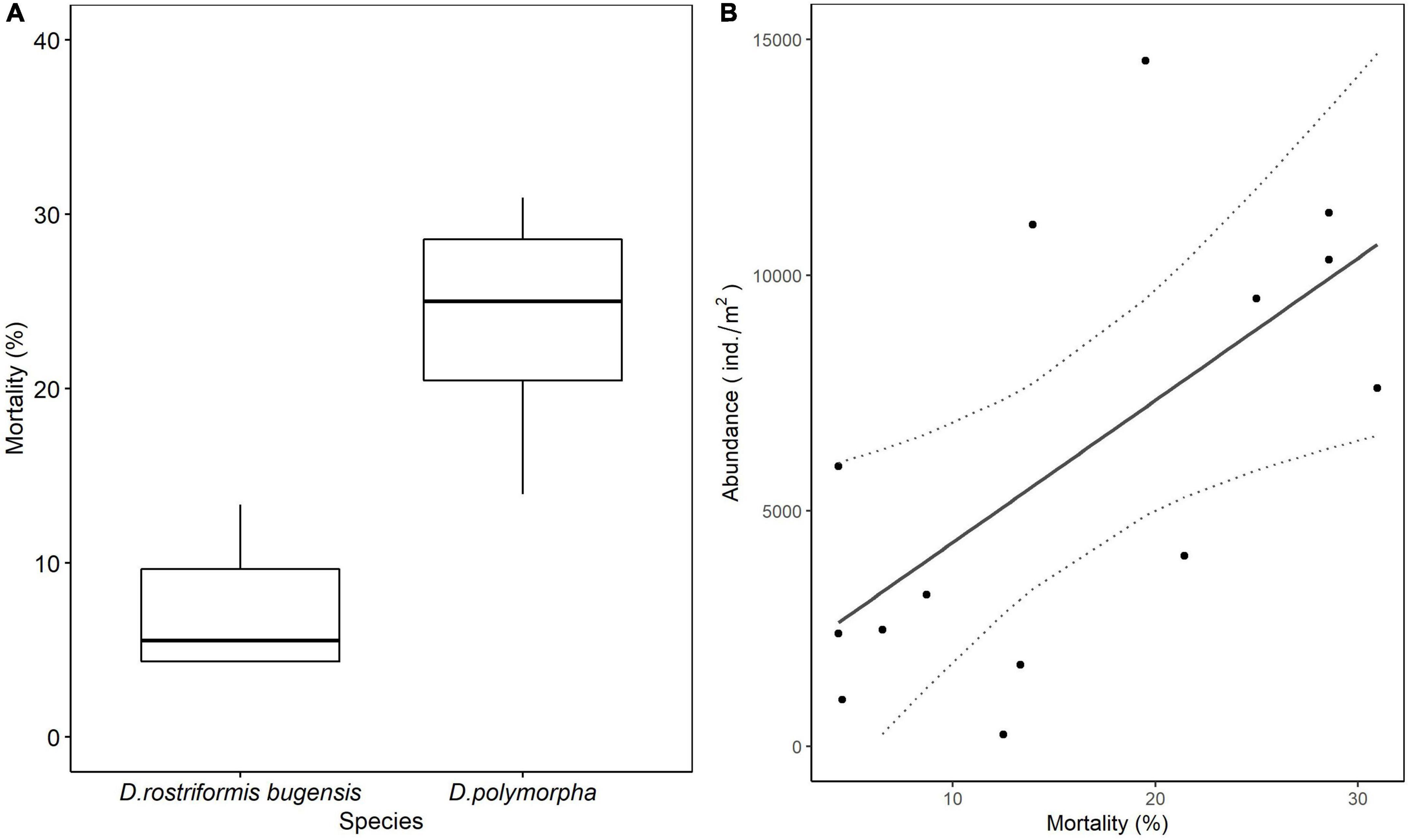
Figure 3. (A) Mortality rates of adult living quagga and zebra mussels glued on the stone substrates. D.rostriformis bugensis is the adult mussel used in Q treatments and D. ploymorpha is the adult mussel used in Z treatments. (B) Linear regression (solid line) and the 95% confidence interval of the regression (dotted line) for abundances of newly settled chironomid larvae (subfamily Chironominae) and mortality rates of adult dreissenids.
The Stable Isotope Values of Newly Settled Macroinvertebrates
The stable isotope values of five main macroinvertebrate species are shown in the stable isotope (δ13C and δ15N) bi-plots (Figure 4). Confidence intervals of the standard ellipse areas for different treatments and the whole experimental area are shown in the density plot (Figure 5 and Supplementary Figure 1).
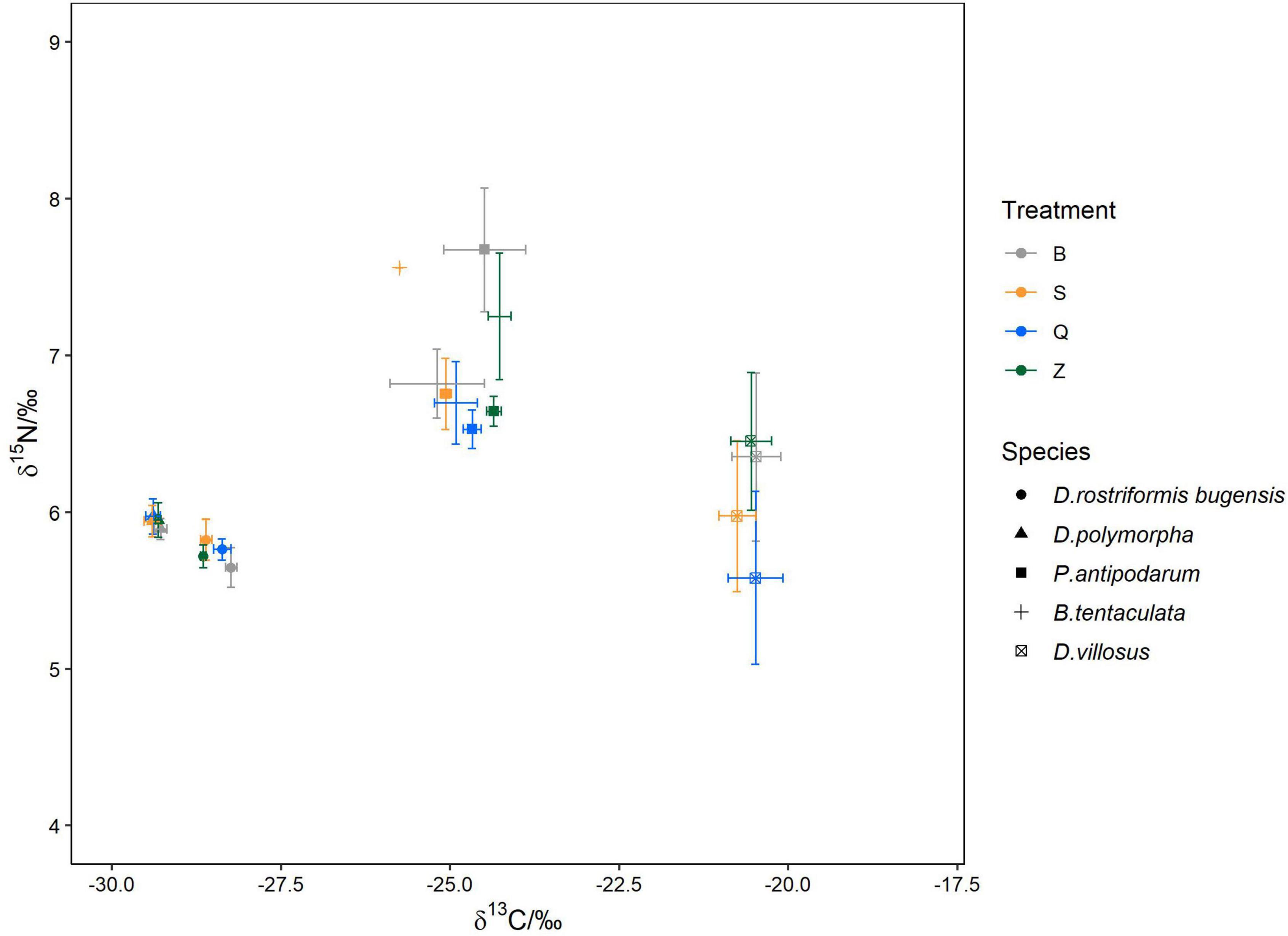
Figure 4. The stable isotope bi-plots (δ13C and δ15N) of five main newly settled macroinvertebrates in four different treatments. Error bars indicate standard errors.
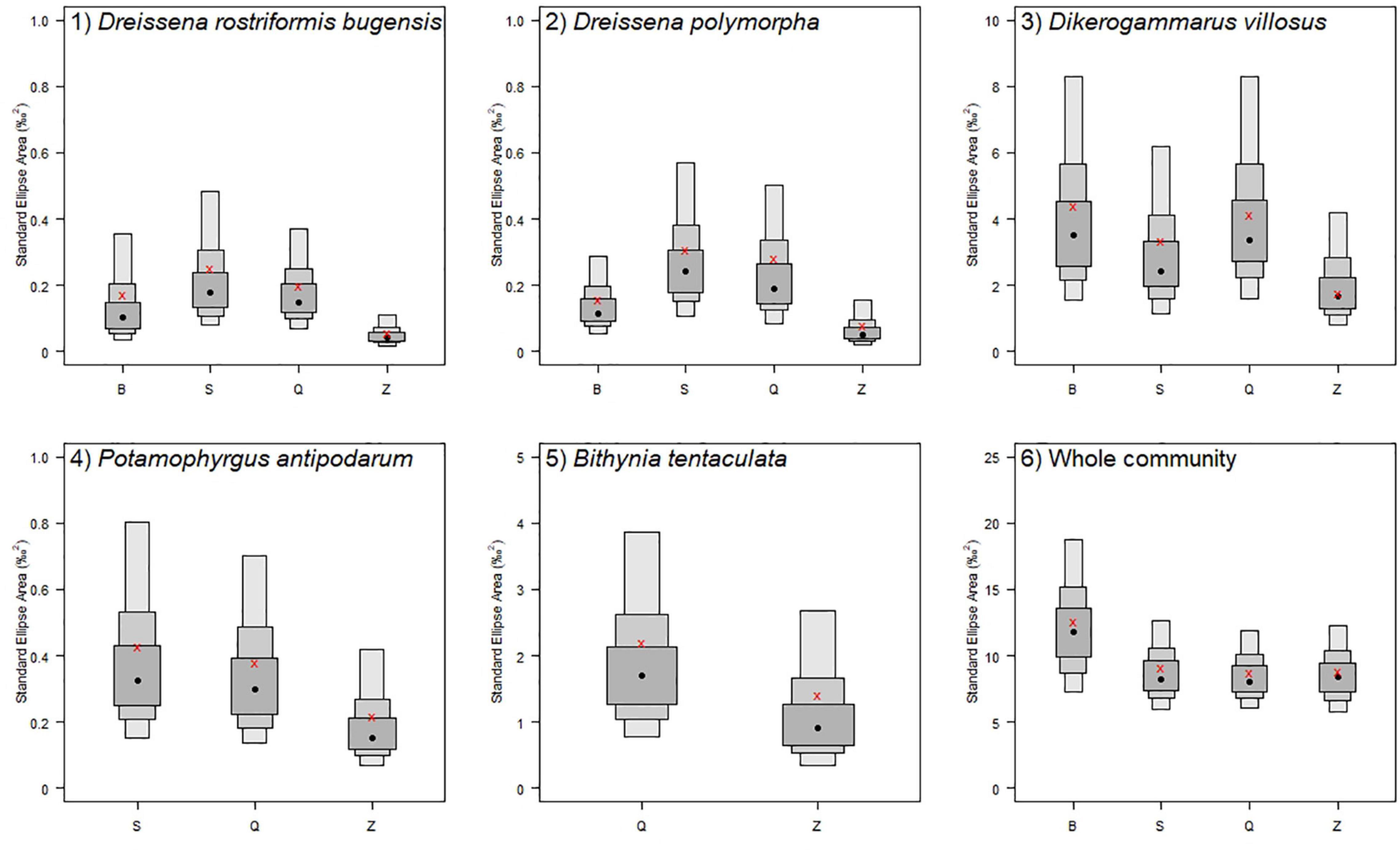
Figure 5. Density plot showing the confidence intervals of the standard ellipse area for five main newly settled macroinvertebrates and the whole community in four different treatments (B, S, Q, and Z). Red crosses correspond to the sample size-corrected standard ellipse areas (SEAc). Boxes shaded from dark to light gray represent the 50, 75, and 95% confidence intervals.
Among four treatments, the δ13C values of newly settled quagga mussels were significantly higher in the B treatment than in the Z treatment (p = 0.016; Table 2). The δ13C values of newly settled snail P. antipodarum were significantly higher in Z treatment than in S treatment (p = 0.014; Table 2). The other three species showed no significant differences in δ13C values among the four treatments. For all five main species, there were no significant differences in δ15N values among the four treatments.
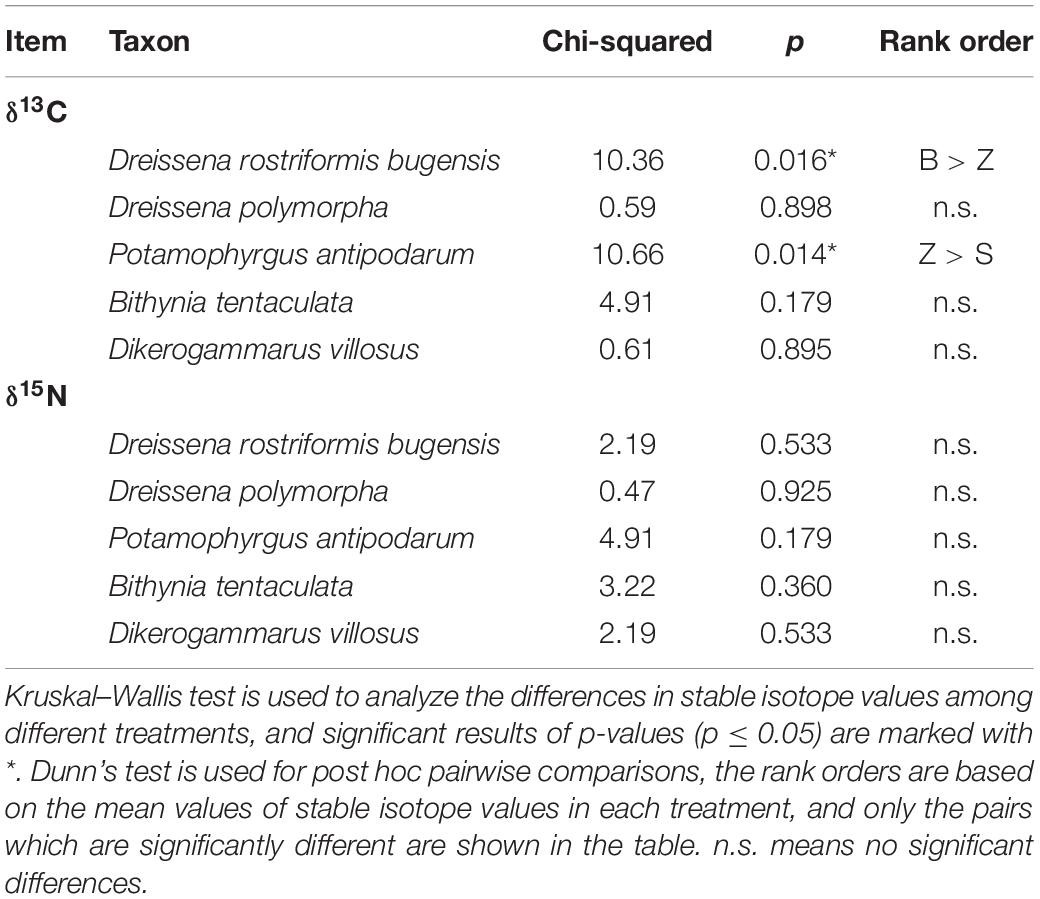
Table 2. The differences in stable isotope values of newly settled macroinvertebrates among four different treatments.
The SEAc results indicated that the newly settled quagga mussels showed a smaller isotopic niche in Z treatment than in B, S, and Q treatments (Figure 5 and Table 3). The newly settled zebra mussels also exhibited a smaller isotopic niche in Z treatment. The remaining three newly settled (frequent) macroinvertebrate species showed equivalent isotopic niches among the different treatments. Taken together, SEAc for the whole community was higher in B treatment.
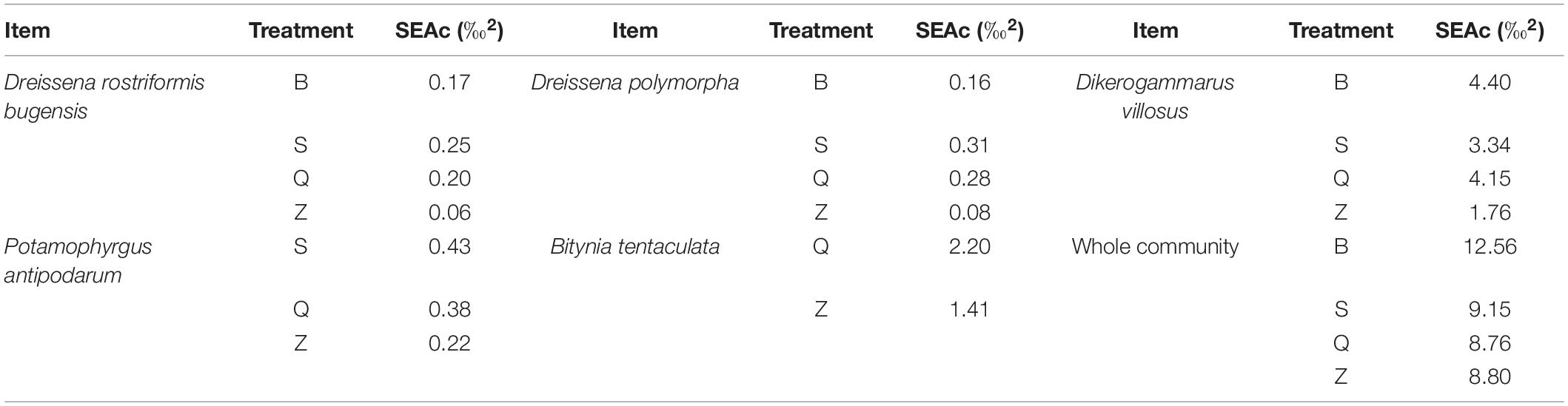
Table 3. Sample size-corrected standard ellipse areas (SEAc) of five newly settled macro-invertebrates and whole community from the four different treatments.
For the two dreissenids, the δ13C values of newly settled quagga mussels were higher than the δ13C values of newly settled zebra mussels. This pattern was evident in all four treatments (p ≤ 0.01; Supplementary Table 2). In each treatment, the δ15N values of all newly settled quagga mussels showed no significant differences from zebra mussels. Based on SEAc results, there were no overlaps in the isotopic niches between quagga and zebra mussels in each treatment.
Discussion
The Community Composition of Newly Settled Macroinvertebrates
The reaction of newly settled macroinvertebrates toward the stone substrate treatments complimented the hypothesis of facilitative associations between invasive dreissenids (Ward and Ricciardi, 2007; Ozersky et al., 2011), wherein one species has a positive effect on the establishment of another species. For example, the highest mean density of macroinvertebrates was consistently found in substrate treatments with shells or living mussels. By implication, the presence of mussels or shells was driving significant variation across experiments. Given the uniform in situ physicochemical conditions for stone substrate, this variation could be attributed to differences in the presence or absence of zebra and quagga mussels and their respective shells. Indeed, such effects were anticipated, since macroinvertebrates have shown a positive reaction to the physical structure of mussel shells (Ricciardi et al., 1997; Burlakova et al., 2012).
The most frequent newly settled macroinvertebrate taxa in our study, P. antipodarum, D. villosus, and Caenis spp., were mainly present at comparatively higher densities on stone substrate shells (S) and living mussel treatments (Q and Z). The rarity of settling macroinvertebrates on blank stone treatments (B) clearly indicates the feasible benefit of macroinvertebrates from the effects provided. In all three cases, each taxon could indeed benefit from the increased complexity of the substratum and surface area associated with natural dreissenid beds (Stewart et al., 1998; Ward and Ricciardi, 2007). Briefly, for P. antipodarum, shells may provide a bed substratum on which tubular refuges with protrusion could be constructed for grazing and scraping on detritus and sediments (Broekhuizen et al., 2001). In particular, the zebra mussel has shown mutualistic interactions with killer shrimp D. villosus and can benefit them in various ways. As shown during ex situ laboratory experiments on dreissenids, killer shrimp evidently utilizes mussel beds as protection from fish and allopatric predators more efficiently than other gammarids (Kobak et al., 2014). Similarly, juvenile zebra mussels can attach to the hard cover of the killer shrimp which could facilitate their dispersal (Yohannes et al., 2017), while the production of feces and pseudo-feces by zebra mussels may provide food (Klerks et al., 1996; Gergs and Rothhaupt, 2008). For Caenis, the taxon’s size might have allowed the use of the physical structure of dreissenids as nearby refuges through the exploitation of small interstices that are typical characteristics of dreissenid beds (Werner and Rothhaupt, 2007).
Like most of the three numerically dominant species, the abundances of newly settled chironomid larvae of subfamily Chironominae were higher in response to the presence of living zebra mussels (Z treatments). However, this species was one of the few species that showed higher density in the absence of mussels (B treatment). Almost all the newly settled chironomid larvae in B treatment were rather small with the average width of head measuring less than 180 μm. The observed pattern is presumably linked to the natural temporal or seasonal life history patterns (in growth, size, and abundance of the species), and perhaps this response was evident due to the 4 weeks of methodological effect. The overall pattern of chironomid larvae abundance and richness from our experimental study reinforces previous findings that zebra mussels facilitate the growth of benthic macroinvertebrate communities. This is in complement with previous studies that showed that the abundance of chironomid larvae increased after the colonization of zebra mussels (Botts et al., 1996; Gergs and Rothhaupt, 2008). Nevertheless, the abundance of chironomid larvae in Z treatments was higher than in Q treatments (Table 1). The high mortality rate of glued adult zebra mussels than quagga mussels might contribute to the differences, as the animal remains are part of the diet for chironomid larvae (Johnson, 1987).
The results of our analysis using analog shell and live quagga mussels should well be noted again, wherein no significant difference in the abundance of all taxa between S treatments and Q treatments was observed. This finding suggests that macroinvertebrates might primarily respond to the physical structure of quagga mussel shells, as discussed in previous investigations (Botts et al., 1996; Burlakova et al., 2012).
Although zebra mussels invaded the lake first, the rapid expansion in the range of the invasive quagga mussel in Lake Constance has caused a dominance shift from zebra to quagga mussels. However, specific effects of live mussels could offer additive benefits for multiple taxa, including newly settled conspecific zebra and quagga mussels. In fact, taking the whole experimental setup into consideration, the entire abundance of newly settled quagga mussels was higher than the entire abundance of newly settled zebra mussels. These data coupled with the current field observation in Lake Constance indicate that this and/or other similar facilitative invasions do cause major changes in biodiversity and ecosystem. The higher mortality rate of adult zebra mussels than adult quagga mussels may also contribute to the dominance shift. We also observed, based on a laboratory study (own unpublished data), that the quagga mussel has a faster growth rate than the zebra mussel.
Our experiment was conducted in 2018, only 2 years after the initial record of quagga mussels in the lake. Nevertheless, as shown by prior studies in other invaded systems, such initial reactions of macroinvertebrate communities toward dreissenids might reduce after several years (Strayer and Malcom, 2006; Karatayev et al., 2015). At the current particular population levels (5,708 ind./m2), we may conclude that quagga mussels would significantly impact the structure of the benthic community in this system. Indeed, this initial establishment of the species at this littoral site could be considered surprising; however, the highest densities of invasive populations have been recorded in higher depths of North American lakes, such as 342,000, 75,000, and 16,000 ind./m2 in Lakes Erie, Huron, and Michigan, respectively (Nalepa, 1995; Howell et al., 1996; Nalepa et al., 2009).
Food Resources of Newly Settled Macroinvertebrates
We were able to note significant differences in the food resources of newly settled macroinvertebrates using mainly δ13C values (Layman et al., 2012). Among the four treatments applied, the newly settled quagga mussels in B treatments showed higher δ13C values than in Z treatments, which was probably related to the smaller size of newly settled quagga mussels in B treatments (p < 0.001). The newly settled snail P. antipodarum is known as a grazer and feeds on periphyton (Larson and Ross Black, 2016), and as expected, showed higher δ13C values in Z treatments than in S treatments, thus the higher mortality of the adult zebra mussels in Z treatments may have generated a different nutrient resource. Comparing Q treatments and the other treatments, there were no significant differences in δ13C and δ15N values of newly settled macroinvertebrates. Previous studies by Gergs et al. (2011) found that the stable isotope values of gammarids were significantly and positively related to the biodeposition of zebra mussels. Here, the presence of the adult quagga mussels did not show a strong influence on the isotope values of the newly settled macroinvertebrates, which might be related to the smaller study area (experiment) and shorter study (invasion) period than the previous study.
Nonetheless, the newly settled quagga mussels had significantly higher δ13C values than the newly settled zebra mussels in each treatment. Quagga and zebra mussels attained from shallow depth of Lake Constance in 2018 also showed higher δ13C values of quagga mussels than that of zebra mussels (own unpublished data). The diet of dreissenids can be very flexible, and contains phytoplankton and suspended detritus (Garton et al., 2005). The benthic primary producers normally have a higher δ13C value compared to pelagic primary producers (more negative) (France, 1995; Mitchell et al., 1996). In our study period, the newly settled quagga mussels might consume a higher percentage of benthic food or a lower percentage of pelagic food, in comparison to newly settled zebra mussels. A similar result showed that after the invasion by smallmouth bass and rock bass, lake trout switched to consuming a lower percentage of littoral prey and had a lower δ13C value than the reference lakes (vander Zanden et al., 1999). One study found that the δ13C values of quagga mussels are more negative than those of zebra mussels (Verhofstad et al., 2013), and the explanation was that quagga mussels consumed more chemoautotrophs which have highly reduced δ13C values. The difference between our study and the study of Verhofstad et al. (2013) may be due to the differences in the substrates, depth ranges, or measurements. Some other studies did not find any difference in the stable isotope values between quagga and zebra mussels (Garton et al., 2005).
No overlaps in the isotopic niche of quagga and zebra mussels were observed in our field experiment. The lengths of newly settled quagga and zebra mussels in S, Q, and Z treatments did not show significant differences, thus the differences in stable isotope values of quagga and zebra mussels were not caused by the differences in the size of dreissenids. At our study site where quagga and zebra mussels coexisted, the dietary segregation or partition of colonized space may be the mechanism for the co-existence of quagga and zebra mussels (Diggins et al., 2004; Rothhaupt et al., 2014). Our experiment was conducted in 2018 in Lake Constance, only 2 years after the first record of quagga mussels. During the early colonization period, quagga mussels might occupy a different niche from zebra mussels to reduce resource competition with zebra mussels (Jackson and Britton, 2014).
Conclusion
In our study, the abundance of gastropods, gammarids, and some insect larvae increased with the presence of dreissenid shells in the littoral region. The macroinvertebrate communities might change after quagga mussels replace zebra mussels and occupy a high percentage in the total biomass of benthic macroinvertebrates, particularly in deep regions where adult quagga mussels can also live (Mills et al., 1993; Nalepa et al., 2020). After the invasion of dreissenids in the Great Lakes, the abundance of dressenids increased in the profundal region; however, the abundance of non-dreissenid profundal macroinvertebrates decreased, which is different from the changes in the littoral region (Burlakova et al., 2018). In our study, there was apparent niche partitioning between newly settled quagga and zebra mussels. The interesting patterns of dietary segregation between quagga and zebra mussels might be a common phenomenon in the early stage of quagga mussel invasions when the two dreissenids coexisted in lakes. These results provide a benchmark by which future changes in trophic ecology and invasion dynamics can be measured across the ecosystem. However, for future experiments, we recommend a longer stone substrate deployment duration encompassing standardized seasonal periods for individual species to achieve stabilized assemblages of wider coverage of macroinvertebrates. Yet, this study offers the first overview of the quagga mussel’s progressive invasion and the reaction of the zebra mussels and other newly settled macroinvertebrates, and contributes to the hypothesis of facilitative associations between invasive mussel species.
In summary, following the occurrence of quagga mussels in Lake Constance, significant changes in the benthic macroinvertebrate community are observed. Only about 5 years after the first detection of quagga mussel, it has become the most dominant macroinvertebrate in the littoral area of the lake. A recent study that analyzed the stomach content showed that benthic whitefish (Coregonus macrophthalmus), roach (Rutilus rutilus), and tench (Tinca tinca) showed high levels of consumption of quagga mussels (Baer et al., 2022). Although equivalent and empirical data on the actual impact of the invasive mussels on the accompanying macroinvertebrates are not available, these dietary shifts observed in multiple benthic dwelling fish species imply alternation in the dietary sources following quagga mussel colonization in Lake Constance.
Data Availability Statement
The raw data supporting the conclusions of this article will be made available by the authors, without undue reservation.
Author Contributions
K-OR, EY, and HZ designed the field experiment. K-OR secured the funding for the project. HZ conducted the field experiment, counted the macroinvertebrate samples, and prepared the samples for stable isotope analysis. HZ analyzed the data with guidance from EY and K-OR. HZ and EY wrote the draft. All authors revised the draft, read, and approved the submitted version.
Funding
This project was supported by funding from the Limnological Institute, University of Konstanz (AFF grant to K-OR). HZ received funding from the Chinese Scholarship Council (grant no. 201704910903) for a living stipend.
Conflict of Interest
The authors declare that the research was conducted in the absence of any commercial or financial relationships that could be construed as a potential conflict of interest.
Publisher’s Note
All claims expressed in this article are solely those of the authors and do not necessarily represent those of their affiliated organizations, or those of the publisher, the editors and the reviewers. Any product that may be evaluated in this article, or claim that may be made by its manufacturer, is not guaranteed or endorsed by the publisher.
Acknowledgments
We would like to thank Christian Fiek for his great help with conducting the experiment and counting macroinvertebrates. Ioanna Salvarina also provided guidance for counting macro-invertebrates. We would also like to thank Wolfgang Kornberger, Yao Zhiyijun, Christian Hanke, and Robert van Geldern for their assistance in the stable isotope analysis of the macroinvertebrates and the members of SeeWandel: Life in Lake Constance – the past, present and future for valuable discussions about the experiment. Martin Wolf also helped with the preparation of stone substrates for the experiment.
Supplementary Material
The Supplementary Material for this article can be found online at: https://www.frontiersin.org/articles/10.3389/fevo.2022.887191/full#supplementary-material
References
Baer, J., Spiessl, C., Auerswald, K., Geist, J., and Brinker, A. (2022). Signs of the times: isotopic signature changes in several fish species following invasion of Lake Constance by quagga mussels. J. Great Lakes Res. 48, 746–755. doi: 10.1016/j.jglr.2022.03.010
Bially, A., and MacIsaac, H. J. (2000). Fouling mussels (Dreissena spp.) colonize soft sediments in Lake Erie and facilitate benthic invertebrates. Freshwater Biol. 43, 85–97. doi: 10.1046/j.1365-2427.2000.00526.x
Boltovskoy, D., and Correa, N. (2015). Ecosystem impacts of the invasive bivalve Limnoperna fortunei (golden mussel) in South America. Hydrobiologia 746, 81–95. doi: 10.1007/s10750-014-1882-1889
Botts, P. S., Patterson, B. A., and Schloesser, D. W. (1996). Zebra mussel effects on benthic invertebrates: physical or biotic? J. North Am. Benthol. Soc. 15, 179–184. doi: 10.2307/1467947
Broekhuizen, N., Parkyn, S., and Miller, D. (2001). Fine sediment effects on feeding and growth in the invertebrate grazers Potamopyrgus antipodarum (Gastropoda, Hydrobiidae) and Deleatidium sp. (Ephemeroptera, Leptophlebiidae). Hydrobiologia 457, 125–132. doi: 10.1023/A:1012223332472
Burlakova, L. E., Barbiero, R. P., Karatayev, A. Y., Daniel, S. E., Hinchey, E. K., and Warren, G. J. (2018). The benthic community of the Laurentian Great Lakes: analysis of spatial gradients and temporal trends from 1998 to 2014. J. Great Lakes Res. 44, 600–617. doi: 10.1016/j.jglr.2018.04.008
Burlakova, L. E., Karatayev, A. Y., and Karatayev, V. A. (2012). Invasive mussels induce community changes by increasing habitat complexity. Hydrobiologia 685, 121–134. doi: 10.1007/s10750-011-0791-794
Burlakova, L. E., Tulumello, B. L., Karatayev, A. Y., Krebs, R. A., Schloesser, D. W., Paterson, W. L., et al. (2014). Competitive replacement of invasive congeners may relax impact on native species: interactions among zebra, quagga, and native unionid mussels. PLoS One 9:e114926. doi: 10.1371/journal.pone.0114926
Byers, J. E., Reichard, S., Randall, J. M., Parker, I. M., Smith, C. S., Lonsdale, W. M., et al. (2002). Directing research to reduce the impacts of nonindigenous species. Conservation Biol. 16, 630–640. doi: 10.1046/j.1523-1739.2002.01057.x
Catry, T., Lourenço, P. M., Lopes, R. J., Carneiro, C., Alves, J. A., Costa, J., et al. (2016). Structure and functioning of intertidal food webs along an avian flyway: a comparative approach using stable isotopes. Funct. Ecol. 30, 468–478. doi: 10.1111/1365-2435.12506
Copp, G. H., Vilizzi, L., Mumford, J., Fenwick, G. V., Godard, M. J., and Gozlan, R. E. (2009). Calibration of FISK, an invasiveness screening tool for nonnative freshwater fishes. Risk Anal. 29, 457–467. doi: 10.1111/j.1539-6924.2008.01159.x
Crooks, J. A. (2005). Lag times and exotic species: the ecology and management of biological invasions in slow-motion. Ecoscience 12, 316–329. doi: 10.2980/i1195-6860-12-3-316.1
Diggins, T. P., Weimer, M., Stewart, K. M., Baier, R. E., Meyer, A. E., Forsberg, R. F., et al. (2004). Epiphytic refugium: are two species of invading freshwater bivalves partitioning spatial resources? Biol. Invasions 6, 83–88.
Evariste, L., David, E., Cloutier, P. L., Brousseau, P., Auffret, M., Desrosiers, M., et al. (2018). Field biomonitoring using the zebra mussel Dreissena polymorpha and the quagga mussel Dreissena bugensis following immunotoxic reponses. is there a need to separate the two species? Environ. Pollut. 238, 706–716. doi: 10.1016/j.envpol.2018.03.098
France, R. L. (1995). Differentiation between littoral and pelagic food webs in lakes using stable carbon isotopes. Limnol. Oceanography 40, 1310–1313. doi: 10.4319/lo.1995.40.7.1310
Francis, R. A., and Chadwick, M. A. (2012). “Invasive alien species in freshwater ecosystems: a brief overview,” in A Handbook of Global Freshwater Invasive Species, ed. R. A. Francis (Abingdon: Earthscan), 3–21. doi: 10.1093/icb/icaa023
Garton, D. W., Payne, C. D., and Montoya, J. P. (2005). Flexible diet and trophic position of dreissenid mussels as inferred from stable isotopes of carbon and nitrogen. Canadian J. Fisheries Aquatic Sci. 62, 1119–1129. doi: 10.1139/f05-025
Gergs, R., and Rothhaupt, K. O. (2008). Effects of zebra mussels on a native amphipod and the invasive Dikerogammarus villosus: the influence of biodeposition and structural complexity. J. North Am. Benthol. Soc. 27, 541–548. doi: 10.1899/07-151.1
Gergs, R., and Rothhaupt, K. O. (2015). Invasive species as driving factors for the structure of benthic communities in Lake Constance, Germany. Hydrobiologia 746, 245–254. doi: 10.1007/s10750-014-1931-1934
Gergs, R., Grey, J., and Rothhaupt, K. O. (2011). Temporal variation in zebra mussel (Dreissena polymorpha) density structure the benthic food web and community composition on hard substrates in Lake Constance, Germany. Biol. Invasions 13, 2727–2738. doi: 10.1007/s10530-011-9943-9948
Gherardi, F., and Acquistapace, P. (2007). Invasive crayfish in Europe: the impact of Procambarus clarkii on the littoral community of a Mediterranean lake. Freshwater Biol. 52, 1249–1259. doi: 10.1111/j.1365-2427.2007.01760.x
González, M. J., and Downing, A. (1999). Mechanisms underlying amphipod responses to zebra mussel (Dreissena polymorpha) invasion and implications for fish-amphipod interactions. Canadian J. Fisheries Aquatic Sci. 56, 679–685. doi: 10.1139/f98-211
Haltiner, L., Zhang, H., Anneville, O., de Ventura, L., DeWeber, T. J., Hesselschwerdt, J., et al. (2022). The distribution and spread of quagga mussels in perialpine lakes north of the Alps. Aquatic Invasions 17, 153–173. doi: 10.3391/ai.2022.17.2.02
Howell, E. T., Marvin, C. H., Bilyea, R. W., Kauss, P. B., and Somers, K. (1996). Changes in environmental conditions during Dreissena colonization of a monitoring station in eastern Lake Erie. J. Great Lakes Res. 22, 744–756. doi: 10.1016/S0380-1330(96)70993-70990
Jackson, A. L., Inger, R., Parnell, A. C., and Bearhop, S. (2011). Comparing isotopic niche widths among and within communities: SIBER - Stable Isotope Bayesian Ellipses in R. J. Animal Ecol. 80, 595–602. doi: 10.1111/j.1365-2656.2011.01806.x
Jackson, M. C., and Britton, J. R. (2014). Divergence in the trophic niche of sympatric freshwater invaders. Biol. Invasions 16, 1095–1103. doi: 10.1007/s10530-013-0563-563
Johnson, R. K. (1987). Seasonal variation in diet of Chironomus plumosus (L.) and C.anthracinus Zett. (Diptera-Chironomidae) in mesotrophic Lake Erken. Freshwater Biol. 17, 525–532. doi: 10.1111/j.1365-2427.1987.tb01073.x
Jones, P. E., Tummers, J. S., Galib, S. M., Woodford, D. J., Hume, J. B., Silva, L. G. M., et al. (2021). The use of barriers to limit the spread of aquatic invasive animal species: a global review. Front. Ecol. Evol. 9:611631. doi: 10.3389/fevo.2021.611631
Karatayev, A. Y., Burlakova, L. E., and Padilla, D. K. (2015). Zebra versus quagga mussels: a review of their spread, population dynamics, and ecosystem impacts. Hydrobiologia 746, 97–112. doi: 10.1007/s10750-014-1901-x
Karatayev, A. Y., Karatayev, V. A., Burlakova, L. E., Mehler, K., Rowe, M. D., Elgin, A. K., et al. (2021). Lake morphometry determines Dreissena invasion dynamics. Biol. Invasions 23, 2489–2514. doi: 10.1007/s10530-021-02518-2513
Kerambrun, E., Delahaut, L., Geffard, A., and David, E. (2018). Differentiation of sympatric zebra and quagga mussels in ecotoxicological studies: a comparison of morphometric data, gene expression, and body metal concentrations. Ecotoxicol. Environ. Saf. 154, 321–328. doi: 10.1016/j.ecoenv.2018.02.051
Klerks, P., Fraleigh, P. C., and Lawniczak, E. (1996). Effects of zebra mussels (Dreissena polymorpha) on seston levels and sediment deposition in western Lake Erie. Canadian J. Fisheries Aquatic Sci. 53, 2284–2291. doi: 10.1139/f96-190
Kobak, J., Jermacz, Ł, and Płąchocki, D. (2014). Effectiveness of zebra mussels to act as shelters from fish predators differs between native and invasive amphipod prey. Aquatic Ecol. 48, 397–408. doi: 10.1007/s10452-014-9492-9491
Kobak, J., Kakareko, T., Jermacz, Ł, and Poznańska, M. (2013). The impact of zebra mussel (Dreissena polymorpha) periostracum and biofilm cues on habitat selection by a Ponto-Caspian amphipod Dikerogammarus haemobaphes. Hydrobiologia 702, 215–226. doi: 10.1007/s10750-012-1322-1327
Kulhanek, S. A., Leung, B., and Ricciardi, A. (2011). Using ecological niche models to predict the abundance and impact of invasive species: application to the common carp. Ecol. Appl. 21, 203–213. doi: 10.1890/09-1639.1
Larson, M. D., and Ross Black, A. (2016). Assessing interactions among native snails and the invasive New Zealand mud snail, Potamopyrgus antipodarum, using grazing experiments and stable isotope analysis. Hydrobiologia 763, 147–159. doi: 10.1007/s10750-015-2369-z
Layman, C. A., Araujo, M. S., Boucek, R., Hammerschlag-Peyer, C. M., Harrison, E., Jud, Z. R., et al. (2012). Applying stable isotopes to examine food-web structure: an overview of analytical tools. Biol. Rev. 87, 545–562. doi: 10.1111/j.1469-185X.2011.00208.x
Lodge, D. M., Stein, R. A., Brown, K. M., Covich, A. R., Christer, B., Garvey, J. E., et al. (1998). Predicting impact of freshwater exotic species on native biodiversity: challenges in spatial scaling. Australian J. Ecol. 23, 53–67. doi: 10.1111/j.1442-9993.1998.tb00705.x
MacIsaac, H. J. (1996). Potential abiotic and biotic impacts of zebra mussels on the inland waters of North America. Am. Zool. 36, 287–299. doi: 10.1093/icb/36.3.287
Mills, E. L., Dermott, R. M., Roseman, E. F., Dustin, D., Mellina, E., Conn, D. B., et al. (1993). Colonization, ecology, and population structure of the “quagga” mussel (Bivalvia: Dreissenidae) in the Lower Great Lakes. Canadian J. Fisheries Aquatic Sci. 50, 2305–2314. doi: 10.1139/f93-255
Mitchell, M. J., Mills, E. L., Idrisi, N., and Michener, R. (1996). Stable isotopes of nitrogen and carbon in an aquatic food web recently invaded by Dreissena polymorpha (Pallas). Canadian J. Fisheries Aquatic Sci. 53, 1445–1450. doi: 10.1139/f96-053
Molloy, D. P., Bij, De Vaate, A., Wilke, T., and Giamberini, L. (2007). Discovery of Dreissena rostriformis bugensis (Andrusov 1897) in Western Europe. Biol. Invasions 9, 871–874. doi: 10.1007/s10530-006-9078-9075
Mörtl, M., and Rothhaupt, K. O. (2003). Effects of adult Dreissena polymorpha on settling juveniles and associated macroinvertebrates. Int. Rev. Hydrobiol. 88, 561–569. doi: 10.1002/iroh.200310640
Nalepa, T. F. (1995). Initial colonization of the zebra mussel (Dreissena polymorpha) in Sagniaw Bay, Lake Huron: population recruitment, density, and size structure. J. Great Lakes Res. 21, 417–434.
Nalepa, T. F., Burlakova, L. E., Elgin, A. K., and Karatayev, A. Y. (2020). Abundance and biomass of benthic macroinvertebrates in Lake Michigan in 2015, with a summary of temporal trends. NOAA Techn. Memorandum GLERL 175, 1–41. doi: 10.25923/g0d3-3v41
Nalepa, T. F., Fanslow, D. L., and Lang, G. A. (2009). Transformation of the offshore benthic community in Lake Michigan: recent shift from the native amphipod Diporeia spp. to the invasive mussel Dreissena rostriformis bugensis. Freshwater Biol. 54, 466–479. doi: 10.1111/j.1365-2427.2008.02123.x
Ozersky, T., Barton, D. R., and Evans, D. O. (2011). Fourteen years of dreissenid presence in the rocky littoral zone of a large lake: effects on macroinvertebrate abundance and diversity. J. North Am. Benthol. Soc. 30, 913–922. doi: 10.1899/10-122.1
Pathy, D. A., and Mackie, G. L. (1993). Comparative shell morphology of Dreissena polymorpha, Mytilopsis leucophaeata, and the “quagga” mussel (Bivalvia: Dreissenidae) in North America. Canadian J. Zool. 71, 1012–1023. doi: 10.1139/z93-135
Post, D. M., Layman, C. A., Arrington, D. A., Takimoto, G., Quattrochi, J., and Montaña, C. G. (2007). Getting to the fat of the matter: models, methods and assumptions for dealing with lipids in stable isotope analyses. Oecologia 152, 179–189. doi: 10.1007/s00442-006-0630-x
R Core Team (2021). R: A Language and Environmental for Statistical Computing. Vienna: R Foundation for Statistical Computing.
Ricciardi, A. (2003). Predicting the impacts of an introduced species from its invasion history: an empirical approach applied to zebra mussel invasions. Freshwater Biol. 48, 972–981. doi: 10.1046/j.1365-2427.2003.01071.x
Ricciardi, A., Hoopes, M. F., Marchetti, M. P., and Lockwood, J. L. (2013). Progress toward understanding the ecological impacts of nonnative species. Ecol. Monographs 83, 263–282. doi: 10.1890/13-0183.1
Ricciardi, A., Whoriskey, F. G., and Rasmussen, J. B. (1997). The role of the zebra mussel (Dreissena polymorpha) in structuring macroinvertebrate communities on hard substrata. Canadian J. Fisheries Aquatic Sci. 54, 2596–2608. doi: 10.1139/f97-174
Roby, K. B., Newbold, J. D., and Erman, D. C. (1978). Effectiveness of an artificial substrate for sampling macroinvertebrates in small streams. Freshwater Biol. 8, 1–8. doi: 10.1111/j.1365-2427.1978.tb01420.x
Rothhaupt, K. O., Hanselmann, A. J., and Yohannes, E. (2014). Niche differentiation between sympatric alien aquatic crustaceans: an isotopic evidence. Basic Appl. Ecol. 15, 453–463. doi: 10.1016/j.baae.2014.07.002
Roy, H. E., Peyton, J., Aldridge, D. C., Bantock, T., Blackburn, T. M., Britton, R., et al. (2014). Horizon scanning for invasive alien species with the potential to threaten biodiversity in Great Britain. Global Change Biol. 20, 3859–3871. doi: 10.1111/gcb.12603
Simberloff, D., and von Holle, B. (1999). Positive interactions of nonindigenous species: invasional meltdown? Biol. Invasions 1, 21–32. doi: 10.1023/A:1010086329619
Son, M. O. (2007). Native range of the zebra mussel and quagga mussel and new data on their invasions within the Ponto-Caspian Region. Aquatic Invasions 2, 174–184. doi: 10.3391/ai.2007.2.3.4
Sousa, R., Pilotto, F., and Aldridge, D. C. (2011). Fouling of European freshwater bivalves (Unionidae) by the invasive zebra mussel (Dreissena polymorpha). Freshwater Biol. 56, 867–876. doi: 10.1111/j.1365-2427.2010.02532.x
Stewart, T. W., Miner, J. G., and Lowe, R. L. (1998). Quantifying mechanisms for zebra mussel effects on benthic macroinvertebrates: organic matter production and shell-generated habitat. J. North Am. Benthol Soc. 17, 81–94. doi: 10.2307/1468053
Stiers, I., Crohain, N., Josens, G., and Triest, L. (2011). Impact of three aquatic invasive species on native plants and macroinvertebrates in temperate ponds. Biol. Invasions 13, 2715–2726. doi: 10.1007/s10530-011-9942-9949
Strayer, D. L., and Malcom, H. M. (2006). Long-term demography of a zebra mussel (Dreissena polymorpha) population. Freshwater Biol. 51, 117–130. doi: 10.1111/j.1365-2427.2005.01482.x
Strayer, D. L., and Malcom, H. M. (2007). Effects of zebra mussels (Dreissena polymorpha) on native bivalves: the beginning of the end or the end of the beginning? J. North Am. Benthol Soc. 26, 111–122.
Strayer, D. L., and Malcom, H. M. (2018). Long-term responses of native bivalves (Unionidae and Sphaeriidae) to a Dreissena invasion. Freshwater Sci. 37, 697–711. doi: 10.1086/700571
Strayer, D. L., Fischer, D. T., Hamilton, S. H., Malcom, H. M., Pace, M. L., and Solomon, C. T. (2020). Long-term variability and density dependence in Hudson River Dreissena populations. Freshwater Biol. 65, 474–489. doi: 10.1111/fwb.13444
Tran, T. N. Q., Jackson, M. C., Sheath, D., Verreycken, H., and Britton, J. R. (2015). Patterns of trophic niche divergence between invasive and native fishes in wild communities are predictable from mesocosm studies. J. Animal Ecol. 84, 1071–1080. doi: 10.1111/1365-2656.12360
vander Zanden, M. J., Casselman, J. M., and Rasmussen, J. B. (1999). Stable isotope evidence for the food web consequences of species invasions in lakes. Nature 401, 464–467. doi: 10.1038/46762
Verhofstad, M. J., Grutters, B. M. C., van der Velde, G., and Leuven, R. S. E. W. (2013). Effects of water depth on survival, condition and stable isotope values of three invasive dreissenid species in a deep freshwater lake. Aquatic Invasions 8, 157–169. doi: 10.3391/ai.2013.8.2.04
Walz, N. (1973). Studies on the biology of Dreissena polymorpha in Lake Constance. Arch. Hydrobiol. Suppl. 42, 452–482.
Ward, J. M., and Ricciardi, A. (2007). Impacts of Dreissena invasions on benthic macroinvertebrate communities: a meta-analysis. Diversity Distrib. 13, 155–165. doi: 10.1111/j.1472-4642.2007.00336.x
Werner, S., and Rothhaupt, K.-O. (2007). Effects of the invasive bivalve Corbicula fluminea on settling juveniles and other benthic taxa. J. North Am. Benthol Soc. 26, 673–680. doi: 10.1899/07-017R.1
Wise, D. H., and Molles, M. C. (1979). Colonization of artificial substrates by stream insects: influence of substrate size and diversity. Hydrobiologia 65, 69–74. doi: 10.1007/BF00032721
Yohannes, E., Ragg, R. B., Armbruster, J. P., and Rothhaupt, K. O. (2017). Physical attachment of the invasive zebra mussel Dreissena polymorpha to the invasive gammarid Dikerogammarus villosus: supplementary path for invasion and expansion? Fundamental Appl. Limnol. 191, 79–85. doi: 10.1127/fal/2017/1063
Keywords: invasive species, macroinvertebrates, colonization, stable isotopes, Lake Constance
Citation: Zhang H, Yohannes E and Rothhaupt K-O (2022) The Potential Impacts of Invasive Quagga and Zebra Mussels on Macroinvertebrate Communities: An Artificial Stone Substrate Based Field Experiment Using Stable Isotopes. Front. Ecol. Evol. 10:887191. doi: 10.3389/fevo.2022.887191
Received: 01 March 2022; Accepted: 06 May 2022;
Published: 03 June 2022.
Edited by:
Gabriele Stowasser, British Antarctic Survey (BAS), United KingdomReviewed by:
Teresa Radziejewska, University of Szczecin, PolandJan Vanaverbeke, Royal Belgian Institute of Natural Sciences, Belgium
Copyright © 2022 Zhang, Yohannes and Rothhaupt. This is an open-access article distributed under the terms of the Creative Commons Attribution License (CC BY). The use, distribution or reproduction in other forums is permitted, provided the original author(s) and the copyright owner(s) are credited and that the original publication in this journal is cited, in accordance with accepted academic practice. No use, distribution or reproduction is permitted which does not comply with these terms.
*Correspondence: Karl-Otto Rothhaupt, karl.rothhaupt@uni-konstanz.de