- 1Department of Paleobiology and Paleoecology, Institute of Geology of the Czech Academy of Sciences, Prague, Czechia
- 2Institute of Geology and Palaeontology, Charles University, Prague, Czechia
- 3Private Researcher, Prague, Czechia
- 4Palaeontological Department, Natural History Museum, National Museum, Prague, Czechia
- 5Department of Geological Sciences, Faculty of Sciences, Masaryk University, Brno, Czechia
- 6Private Researcher, Rosenfeld, Germany
The calcareous tubes inhabited by some polychaetes (some Serpulidae and the sabellid Glomerula) which are adapted to live sticking in soft ground, starting from the Permian, represent widespread but widely neglected and understudied substrates for domichnial bioerosion. Serpulids can be considered small macrofauna. However, due to the thinness of serpulid tubes, borings in them are sized in the order of 0.01–0.9 mm in diameter and thus rather considered micropaleontological objects. Extensive and methodologically broad search (vacuum castings studied at SEM; micro-computed tomography) for and study of borings in these specific substrates was performed on material from the Cenomanian of Le Mans area (France) and the Cenomanian and Turonian of the Bohemian Cretaceous Basin (Czechia). It shows that the bioerosive traces can be assigned to the existing ichnogenera Rogerella, Trypanites, Entobia, Maeandropolydora, and Iramena. Somewhat surprising is the frequency and disparity of dwelling borings. Several clues, especially in the more abundant ichnogenera Rogerella, Trypanites, and Entobia, support the hypothesis that the tracemakers of these borings adapted to the small size of their substrates by necessarily staying very small by themselves but nevertheless living to adulthood.
Introduction
One of the most problematic attributes of systematic ichnology, and thus ichnology in general, is the size of biogenic structures as ichnotaxobase and as a basis for further (e.g., paleobiological) interpretations (Bertling et al., 2006, 2022). For example, it has been tested that the diameter of a horizontal tunnel produces a continuous spectrum when measuring a large number of samples of different ages, from different environments and different substrates (e.g., Pemberton and Frey, 1982). It makes no sense to determine ichnotaxa by only one dimension, as they would be completely formal (Bertling et al., 2006). On the other hand, the size of a biogenic structure cannot be completely ignored, because extreme size differences point to, for example, different burrowing techniques or different ethologies (Bertling et al., 2022). Therefore, ichnotaxa that visibly violate the size rule as non-compliant ichnotaxobases are maintained in ichnological literature (e.g., Megaplanolites isp; Calvo et al., 1987).
A very specific example of ichnofossils of various sizes are borings, which fill virtually the entire space of particularly small grain (most often calcite or aragonite bioclast) and, as the small size of the grain fundamentally limits further boring enlargement, fall into the category of stenomorphic ichnofossils (Bromley, 1996). Recently, small boreholes have been found in the tubes of the serpulid genera Cementula Brünnich Nielsen, 1931, Placostegus Philippi, 1844 and Pyrgopolon de Montfort, 1808 from the Cenomanian and Turonian of the Czech Cretaceous Basin. In particular, however, the borings in the tubes of Pyrgopolon from the Cenomanian of the locality Le Mans ring road (France; Kočová Veselská et al., 2021; Figure 1) were evaluated in many ways. This work was based on the collections of Manfred Jäger from 2002. Tubes from these collections were selectively processed as vacuum epoxy castings and then studied by means of a scanning electron microscope; selected tubes were studied by computer tomography. The team of authors thoroughly studied hundreds of tubes of the genus Pyrgopolon and came to the following conclusions: (1) Borings found in the tubes show a relatively low diversity; yet several different, repetitive shapes were recognized. Borings of the ichnogenus Rogerella are among the best-preserved. (2) The tubes of Pyrgopolon represent the second known case of the interaction between boring barnacles (Rogerella tracemakers) and serpulids. (3) Short shafts perpendicular to the tube surfaces can be attributed to the Trypanites isp. (4) Irregularly meandering tunnels usually have more than one entrance opening, which indicates that they belong to the ichnogenus Maeandropolydora. (5) Another boring is an ovoid chamber with a relatively large mouth and several narrow threads, Entobia isp., caused by clionid borings. (6) Sclerozoans include encrusting oysters, cheilostome and cyclostome bryozoans, serpulid and sabellid worms, and foraminifera.
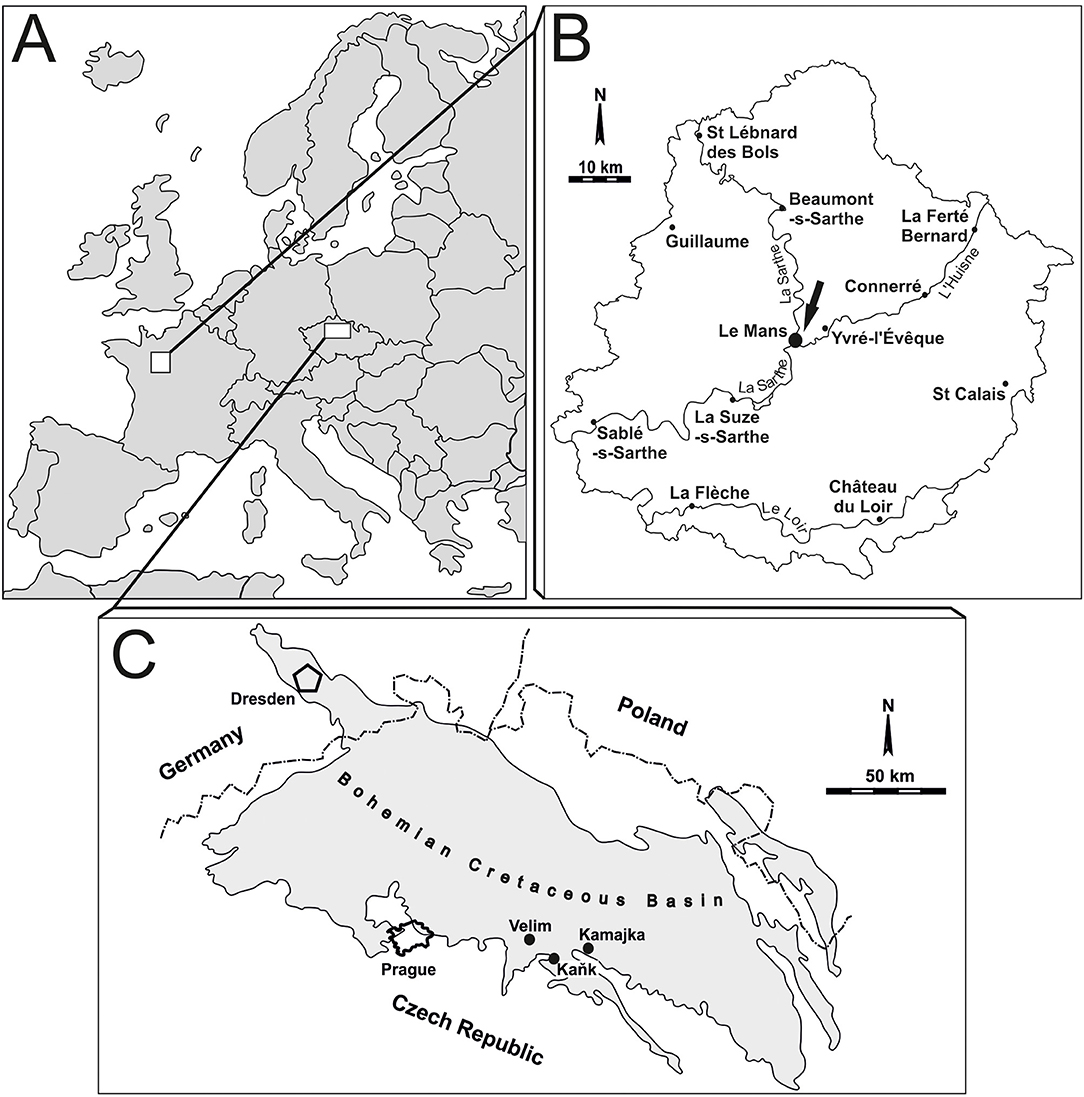
Figure 1. Location map. (A) Simplified map of Europe with the studied areas. (B) Le Mans area (France), the locality is marked by an arrow. (C) A sketch of the Bohemian Cretaceous Basin indicating the location of the studied sites.
The study of Kočová Veselská et al. (2021) acquaints the reader with the hitherto overlooked paleobiological and ichnological phenomenon. The study asked several questions, which only partially provide direct answers. The aim of our present follow-up study is to clarify the preliminary answers offered by the previous study and specially to add the knowledge gained from the material obtained by Tomáš Kočí in 2000–2020 from the so-called surf facies of the Bohemian Cretaceous Basin (BCB) in central Bohemia, Czech Republic, to Le Mans material. This material from the BCB, like the material from Le Mans (involved in Kočová Veselská et al., 2021), was studied by means of a scanning electron microscope and microcomputer tomography.
Geological Setting
A large amount of material representing 400 serpulid tubes has been found by Manfred Jäger in Le Mans area (France) in a temporary outcrop at a building site of Le Mans ring road (D 313) between Gazonfier and Yvré l'Évêque (Kočová Veselská et al., 2021). This outcrop represents the basal part of the Craie à Terebratella carantonensis and can be assigned to the uppermost Cenomanian Neocardioceras juddii Zone (Morel, 2015). The thickness of this formation varies between 1 and 3 m. It consists of a sandy and glauconitic chalk with abundant fragments of bivalves and planktonic foraminifera, which indicates pelagic carbonate sedimentation. The high homogeneity of environmental conditions during sedimentation indicates a wide-open sea (Juignet, 1974). The serpulids were collected by MJ in 2002; the bored specimens represent 58.75% (i.e., 235 out of the 400 collected serpulid tubes).
Additional material of 1,350 serpulid tubes comes from the late Cenomanian–early Turonian nearshore, shallow-water facies at Velim, Kamajka, and Kank “Na Vrších” in the Bohemian Cretaceous Basin (Czechia; Figure 1). All these deposits are situated along the southern and eastern margin of the Bohemian Cretaceous Basin and are interpreted to have been laid down under high-energy conditions, where nearshore sediments are exposed in depressions of metamorphic rocks (Žítt et al., 1997). Whereas strata containing bioeroded serpulids at Kank and Kamajka are exclusive of early Turonian age, those from Velim are late Cenomanian in age. The horizon yielding serpulid polychaetes is developed in characteristic facies, consisting of calcareous claystones at Kank “Na Vrších,” organodetritic clay limestones at Kamajka, and calcareous siltstones with abundant organodetritus at Velim and Kamajka. The studied serpulid tubes were collected by TK from 2010 to 2020; the bored specimens represent only 1.1% (i.e., 15 out of 1,350 gathered serpulids).
Methodology
Bioerosion in tubes of serpulid polychaetes from the late Cenomanian and early Turonian of the Bohemian Cretaceous Basin and the late Cenomanian of Le Mans region were studied by a combination of non-invasive and invasive methods: micro-CT and vacuum cast embedding (Struers CitoVac) (Charles University, Prague) technique producing polymer resin casts. Both micro-CT and epoxy vacuum embedding followed by SEM observations is widely regarded as the standard tools for reasonable 3D visualization and spatial distribution of inner structures inside various substrates (Tapanila, 2008; Knaust, 2012; Wisshak, 2012).
For visualizing bioerosion structures, X-ray micro-tomography SkyScan 1172 (Bruker) in the National Museum in Prague was chosen first for its non-destructive nature. To enhance better contrast between calcitic/aragonitic serpulid tubes from Le Mans and their infilling, Al (0.5 mm), Al + Cu, or Cu (1 mm) pre-filters were used. With a Cu filter, the X-ray microfocus tube operated at 100 μA and 100 kV. The rotation Step was 0.2°, 4 frames for one step, Random Movement was 10, and 180° rotation was used. For specimens NM O8727 and NM O8728 from the Bohemian Cretaceous Basin, an X-ray microfocus tube operated at 140 μA and 70 kV with an Al + Cu pre-filter. The rotation Step was 0.2°, with 2 frames for one step. Random Movement was 2, and 180° rotation was used. An average image pixel size was 2.84 μm for specimen NM O8727 and 2.44 μm for specimen NM O8728. Regarding specimen CZ2, the X-ray microfocus tube operated at 124 μA and 80 kV with an Al (0.5 mm) filter. The rotation Step was 0.2°, 2 frames for one step, Random Movement was 10, and 180° rotation was used. The average image pixel size was 1.96 μm. Software for reconstruction N-Recon (Bruker) Avizo 9.1 software (National Museum in Prague) was used, and photographs were created by Avizo 9.1 software. Revealed information was examined using the Volume Rendering and Ortho-slice mode of surface and/or sections. We also applied Isosurface visualizing and segmentation to effectively display tube-dwelling boring structures.
Specimens were then placed in a small vacuum chamber for cast embedding (Struers CitoVac) and infiltrated with a mixture of low-viscosity epoxy resin (EpoFix) and hardener (EpoFix) (property of the Charles University, Prague) in a ratio of 15/2 ml to make polymer resin casts. After curing, the samples were longitudinally cut in a half with a rock saw, decalcified by treatment with 10% HCl, rinsed in distilled water to prevent further etching and possible chemical precipitation, and dried. The polymer resin casts were then mounted on stubs before gold coating and SEM analysis (Hitachi S-3700 N SEM in the National Museum in Prague). The SEM images of the resulting casts have significantly better resolution than micro-CT to display and quantify details of borings. However, epoxy cast-embedding is unsuitable for poorly soluble substrates (Tapanila, 2008; Knaust, 2012; Wisshak, 2012) as shown in the silicified tube of specimen NM O8727 which could not be dissolved in either concentrated HCl or HF.
Material from Le Mans is kept in the collection of the Musée Vert, Muséum d'histoire naturelle du Mans, under inventory numbers MHNLM EMV 2016.3.1.−2016.3.88. Material from the Bohemian Cretaceous Basin is housed in the National Museum in Prague, under inventory numbers NM O8727, NM O8728, and CZ2.
Systematics
Trypanites Mägdefrau, 1932
Trypanites isp.
Figures 2A–F, 3B,C, 4A.
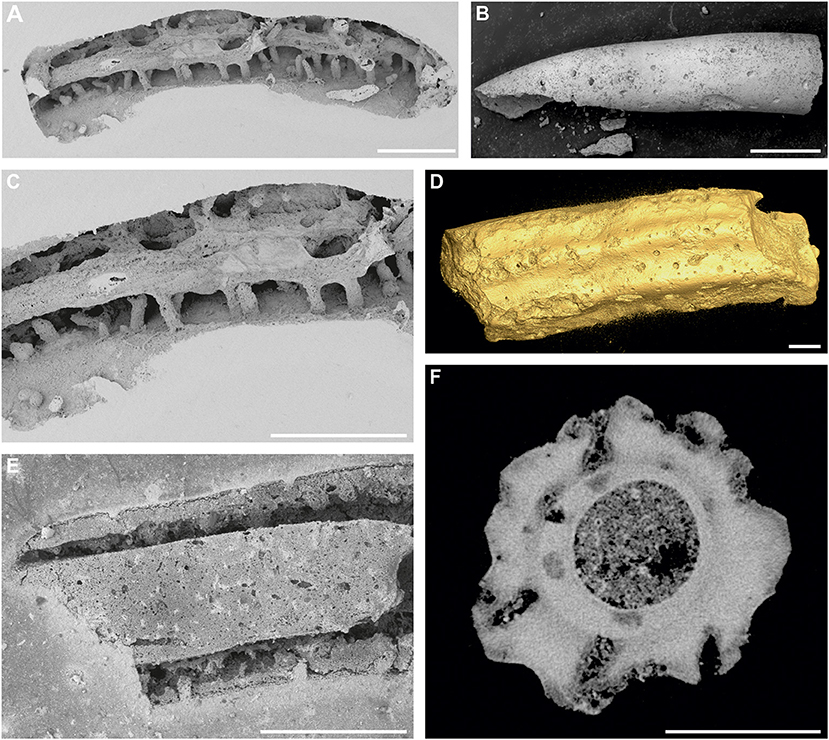
Figure 2. Trypanites isp. in various frequency and spatial distribution. (A,C) Pyrgopolon (Septenaria) cf. tricostata; numerous shafts of Trypanites isp. cut by the longitudinal boring Maeandropolydora isp.; SEM images of casted NM O8728; Kank “Na Vrších,” Bohemian Cretaceous Basin, Czechia. (B) Widely-spaced openings of Trypanites isp. on the outer surface of the inner tube of Pyrgopolon (Pyrgopolon) deforme; SEM image of MHNLM EMV 2016.3.88; Le Mans, France. (D) More densely crowded openings of Trypanites isp. on the outer tube of Pyrgopolon (Pyrgopolon) deforme; Micro-CT isosurface visualization of MHNLM EMV 2016.3.22; Le Mans, France. (E) SEM image of casted, partly dissolved silicified tube of Placostegus zbyslavus, with relatively frequent shallow shafts of Trypanites isp. NM O8727; Kamajka, Bohemian Cretaceous Basin, Czechia. (F) Numerous shafts of Trypanites isp. found in Pyrgopolon (Pyrgopolon) deforme on a computed tomography cross-section of the serpulid tube (note their preferred position on the longitudinal ribs) and smaller circular sections which probably represent the galleries and exploratory threads of the ichnogenus Entobia. MNHLM EMV 2016.3.44; Le Mans, France. Scale bars = 1 mm.
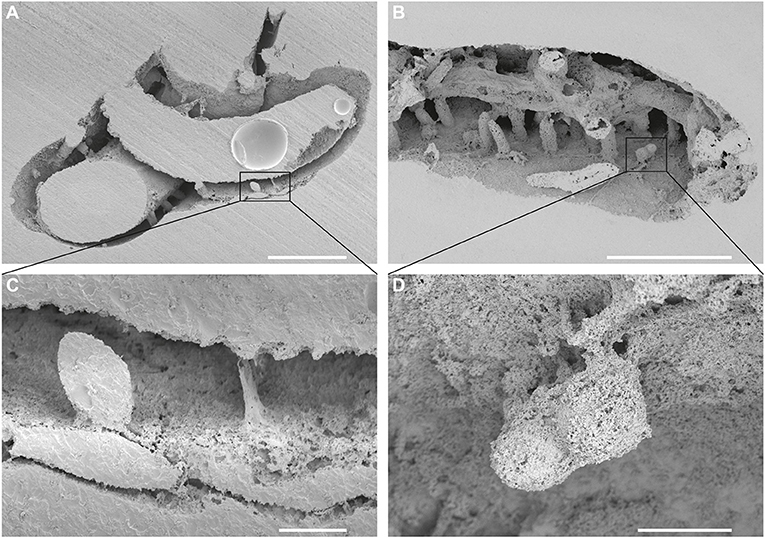
Figure 3. Entobia isp. (A,C) A single-chamber specimen of Entobia isp. (in a square), from which a thin fiber protrudes from the bottom right and a short shaft at the bottom in the middle. The substrate is Cementula sp.; SEM images of casted CZ2; Velim, Bohemian Cretaceous Basin, Czechia. (B,D) A serpulid tube Pyrgopolon (Septenaria) cf. tricostata bored by Trypanites, Maeandropolydora, and two bi-camerate specimens of Entobia isp. (in a square). Note the very short and thin threads only on the one side of the chambers; SEM images of casted NM O8728; Kank “Na Vrších,” Bohemian Cretaceous Basin, Czechia. Scale bars = 1 mm (A,B), 100 μm (C,D).
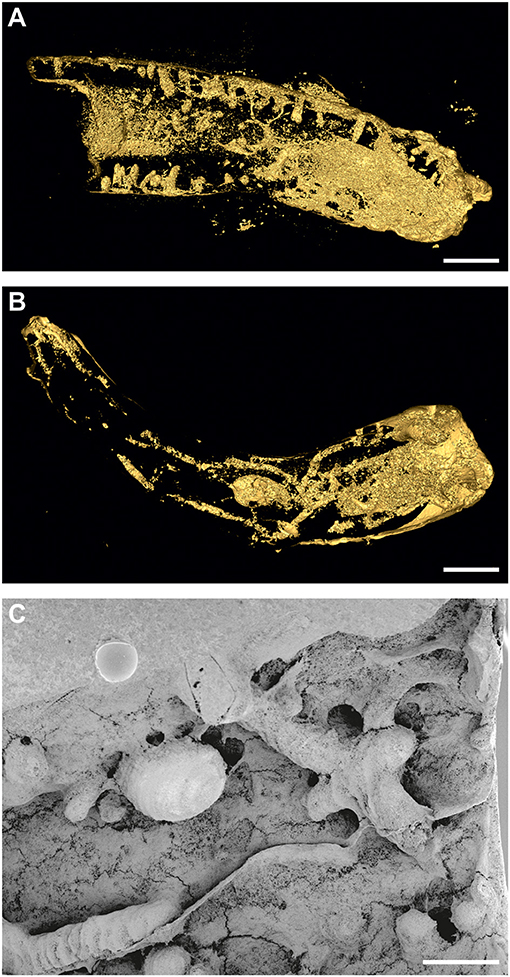
Figure 4. (A) Pyrgopolon (P.) deforme with frequent, equally wide, and deep shafts of Trypanites isp.; MHNLM EMV 2016.3.22; three-dimensional micro-CT reconstruction. Le Mans, France (cf. Kočová Veselská et al., 2021, Figure 6B). (B) single-chamber specimen of Entobia isp. with long branching galleries occupying the whole specimen of Pyrgopolon (P.) deforme, MHNLM EMV 2016.3.14, three-dimensional micro-CT reconstruction. Le Mans, France (cf. Kočová Veselská et al., 2021, Figure 7C). (C) Pyrgopolon (P.) deforme with network of Entobia exploratory tunnels and one larger, smooth chamber, SEM image of casted MHNLM EMV 2016.3.18, Le Mans, France (cf. Kočová Veselská et al., 2021, Figure 8A). Scale bars = 1 mm (A), 2 mm (B), and 400 μm (C).
Material
Le Mans: generally fewer than 20 borings per tube were ascertained in 15 serpulid tubes examined by micro-CT and vacuum epoxy method. Among them, the specimen MHNLM EMV 2016.3.22 (Pyrgopolon (P.) deforme) was studied in the most detail (Kočová Veselská et al., 2021). At least 31 boreholes are visible in the CT-shot on this individual (Figure 4A). Some other tubes studied with an SEM have up to 100 burrow openings; without the use of CT or epoxy casting, however, the mouth of Trypanites cannot be reliably distinguished from the entrance shafts of Entobia isp. From the Bohemian Cretaceous Basin (Czechia), the specimen O8728 displays a minimum of 30 borings on the epoxy cast, which includes about one-half of the original serpulid tube. Specimen O8727: Minimum 12 discernible borings on the epoxy cast done from an incompletely dissolved tube. Specimen CZ2: Minimum three incompletely preserved borings on the epoxy cast.
Description
Thin, short, smooth shafts usually pass the whole preserved wall of a serpulid tube. The diameter of individual shafts tends to be constant, but there are notable exceptions (Snapshot 23.tif the specimen upper right; diameter ranges from 0.035 to 0.05 mm). The Axis of the shaft is rectangular or steeply inclined toward the surface of the host tube. The usual length/depth of the tubes is 0.1 to 0.3 mm which corresponds to the thickness of the serpulid tube walls.
Remarks
Kočová Veselská et al. (2021) studied in detail sclerobionts and borings of the locality Le Mans. These authors stated that in 15 serpulid tubes examined by micro-CT and vacuum epoxy method, about 30 complete borings are oriented perpendicular to the substrate. Another 20 tunnels are inclined at angles of up to 45° to the vertical axis. The perpendicular borings are considered to belong to Trypanites, while the oblique tubes were preliminarily included in the ichnogenus Entobia. However, this attitude gives only approximate results in terms of the number of Trypanites and Entobia individuals. A specimen of Entobia usually has several openings connecting the domichnial chambers to the substrate surface; however, the number of these connections is very variable within the ichnogenus Entobia (e.g., Bromley and D'Alessandro, 1983); thereby, the number of oblique borings cannot be easily recalculated to the number of individuals.
For trypanites, Bromley (1972, pp. 95, 96, Figure G) reported diameter approx. 1 mm and length up to 4 cm. Taylor et al. (2013) stated the diameter of borings 0.35–0.4 mm in belemnites from the Early Cretaceous Speeton Clay Formation. The borings studied in our present publication are substantially smaller than those cited in Bromley's revised diagnosis of the ichnospecies, measuring only about 0.05 mm in diameter. Bertling et al. (2006) stated that the size itself is not a valid ichnotaxobase; thereby, the small size of the finds cannot be considered a clue to discard the above-described finds from Trypanites.
Following this practice, numerous authors (e.g., Nielsen et al., 2003) accommodated microscopic drillings into foraminifer shells to the ichnogenus Oichnus Bromley, 1981 which originally had been established for macroscopic drilling of naticid gastropods through shells of various mollusks (namely gastropods, bivalves, and scaphopods). The present article follows this practice and supports the validity of the approach. Also, other widespread borings than Oichnus may have their microscopic varieties.
Entobia Bronn, 1837
Entobia isp.
Material
On the surfaces of certain tubes from Le Mans more than 100 orifices are visible which could represent the mouths of Entobia isp. (Kočová Veselská et al., 2021). The CT or SEM studies of the epoxy vacuum cast showed that a significant portion of these openings belongs to Trypanites instead (see above) and that the individual of Entobia usually consists of one or two chambers and several (3–7) mouth tubes. Thereby, only a dozen specimens of Entobia isp. are well documented from Le Mans. From the BCB, a sole well casted unicamerate specimen has been found on the sample CZ2. A trinity of two-camerate specimens is present on O8728.
Description
Ovoid or bag-like chambers, 0.2 to 0.7 mm in diameter, connected to the surface by narrow tunnels, usually inclined (angle to the serpulid tube's longitudinal axis ~50°, rarely only 30°). As the chambers occur very close to the surface of the serpulid tubes, some connecting tunnels are very short (length ranges from 0.1 to 2 mm) and narrow (diameter varies from 0.01 to 0.4 mm).
Remarks
The boring found on CZ2, fortunately, preserved near the cut of the serpulid tube for casting, can be compared with several common ichnogenera: Gastrochaenolites Leymerie, 1842 is typical for rockgrounds but also firmgrounds from the Ordovician till the present; pholadid bivalves are its typical tracemakers, often preserved in situ (Mikuláš et al., 2006). However, these are geometrically exactly bored, due to the use of a mechanical rotational move of the shell toward the substrate. Another possibility, Rogerella isp., is excluded due to the relatively broad aperture in contrast with the slit-like or “comma-like” aperture of Rogerella. On the other hand, the bag-like shape is typical for certain camerate entobians (e.g., E. devonica); these do not show a geometrical exactness of the resultant chamber as it grows using a chemical dissolution of the surrounding substrate. The specimen from O8728 shows a couple of small (up to 100 μm) spherical bodies, partially penetrating each other. From the outside of the substrate, the spheres are smooth, resp. their surfaces correspond to the grain size of the mineralized filling (<10 μm). On the sediment-facing side, approximately six “stems,” shorter than 10 μm, protrude from the two spherical bodies, corresponding to exploratory threads of entobians (Bromley and D'Alessandro, 1984). Another two specimens of E. isp. on the sample O8728 show the comparative morphology and dimensions but are in the back of the epoxy cast and are not easily accessible for study.
Shortly after the year 2000, the vast majority of then known findings of the ichnogenus Entobia were relatively homogeneous in size, with the diameter of the chambers resp. galleries approximately a few millimeters, exceptionally over 1 cm: Entobia gigantea up to 50 mm, Entobia magna 20 mm, Entobia isp. B sensu Bromley and D'Alessandro, 1984 chamber size up to 15 mm. Wisshak (2008) summarized the findings of smaller entobians; he described the rich material from the Lower Pleistocene of the island of Rhodes and recent analogies from various aphotic, cold-water environments, mostly on the NE coast of the Atlantic. After studying a very large material, he described in detail two new ichnospecies of the ichnogenus Entobia, both of microscopic dimensions: E. mikra Wisshak, 2008 has the form of grape or lump clusters and reaches 60–330 μm; E. nana Wisshak, 2008 presents mostly solitary cavities/chambers with a size of 100–750 μm.
The holotypes of the two new entobians determined by Wisshak come from the early Pleistocene (Rhodes Formation), from the coral skeletons of the genus Lophelia. This genus with a finely acicular aragonite structure provides an ideal environment for the formation of idiomorphic (i.e., not taking into account the shape of the substrate) borings (Bromley, 2005). In addition to the ichnospecies E. mikra and E. nana, there are much larger residues of entobians in the bioclasts of the Rhodes Formation, identifiable only to the ichnogeneric level. Other ichnotaxa found include very abundant Saccomorpha clava Radtke, 1991, Orthogonum lineare Glaub, 1994 and Semidendrina pulchra Bromley et al., 2007. Rarely have been found Podichnus centrifugalis, Bromley et Surlyk, 1973, Caulostrepsis cretacea Voigt, 1971 Maeandropolydora isp., Palaeosabella isp., Scolecia serrata Radtke, 1991 and Oichnus simplex Bromley, 1981.
Within the ichnogenus Entobia, it is rather exceptional that direct fibers, known as exploratory threads (Bromley and D'Alessandro, 1984) do not protrude from the chambers in all or several prevailing directions. The behavior of entobians living in the tiny space inside the serpulid shells was apparently directed so that communication with the inside of the tube was not desirable because an exploratory thread would not have an opportunity to be useful in any way. There is no usable space for the boring organism inside the lumen of the tube because it is inhabited by the serpulid animal (except if the tube is already empty because either the serpulid animal has grown and moved to a more anterior position inside its tube, or the serpulid has already died and the lumen is empty.). Similar behavior is recorded by the ichnospecies E. solaris Mikuláš, 1992, from the Lower Cretaceous of the Outer Western Carpathians. Additionally, for these ichnospecies, the exploratory threads are limited to the surface of the substrate (Mikuláš, 1992).
The determination of this ichnofossil at the ichnospecific level is complicated. It is obvious that it is a camerate ichnospecies (typical examples are E. cretacea and E. ovula; Bromley and D'Alessandro, 1984). Non-camerate examples, i.e., cylindrical or conical ichnospecies would certainly create a gallery at first and not spherical hollows. It is also clear that the microscopic ichnospecies described by Wisshak (2008), i.e., E. mikra and E. nana, are morphologically different from E. isp. from both Le Mans and the BCB. Le Mans findings compared to the BCB collections may represent a single ichnospecies, but the comparative material is still relatively small. For the same reason, we also abandon the determination of new ichnospecies based on the material described herein.
From a couple of E. mikra and E. nana, none of them can be considered a typical product of chamber growth on exploratory threads. Wisshak (2008; p. 221, Figure 4) showed epoxy castings of E. mikra as grape-like forms with individual chambers measuring 3–6 μm in diameter. The chambers intersect the neighboring ones; therefore, they cannot be connected by galleries or threads. In the case of the specimen shown by Wisshak (2008, Figures 4A,B), 2–3 threads are clearly visible in an area limited by approximately 10 chambers. The specimen in Figures 4E,F resembles an elongated, partially branched grape composed of approximately one hundred balls. Tubular or fibrous forms are not recognizable here at all. Closer to the “classic” form of Entobia isp. with one or more chambers of “potato” shape and thin but distinct network of exploratory threads are Entobia nana Wisshak, 2008. A detailed numerical analysis of the ichnospecies E. mikra and E. nana was given by Wisshak in Tables 2 and 4. Interestingly, Wisshak (2008; p. 219) stated that the chambers of most entobians, if well preserved, have a cuspate microsculpture that may be lacking in gerontic individuals. In terms of these data, Entobia isp. studied herein, with a relatively smooth surface (the grain size of the surface is related to the grain size of the surrounding substrate) and the lack of exploratory threads represent an adult, if not a gerontic specimen. In addition, in the BCB findings, none of the studied substrates found “transient” forms between small entobians in serpulid shells and large, mostly as yet unstudied E. cf. cretacea from more massive substrates (mostly oysters; Žítt et al., 1997).
Maeandropolydora Voigt, 1965
Maeandropolydora isp.
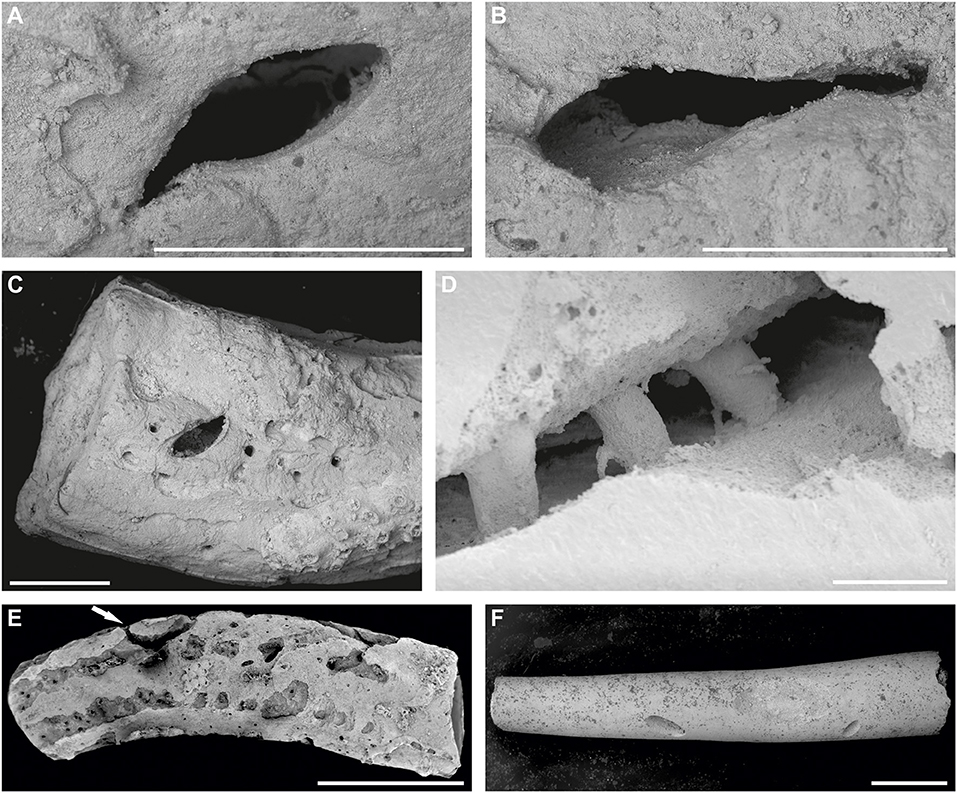
Figure 5. (A–C) SEM images of Pyrgopolon (P.) deforme bored by Rogerella isp. showing in detail the most frequent shapes of ventricular outlets: (A,B) slit-like; MHNLM EMV 2015.3.13. (C) Almond-like; MHNLM EMV 2015.3.57. Specimens from Le Mans, France (cf. Kočová Veselská et al., 2021, Figures 4A,B). (D) Detail of the casted Cementula sp. with three robust shafts, two of them are slightly conical, and the third is barrel-shaped, interpreted as Iramena isp. The shafts are also connected to the surface of the substrate by arcuate tunnels. Specimen CZ2; Velim, Bohemian Cretaceous Basin, Czechia. (E) SEM image of Pyrgopolon (P.) deforme with a variety of sclerobionts and borings; arrow points to Maeandropolydora isp.; MHNLM EMV 2016.3.9, Le Mans, France (cf. Kočová Veselská et al., 2021). (F) SEM image of the inner tube of Pyrgopolon (P.) deforme, showing bottoms of roughly U-shaped borings, probably Rogerella isp.; MHNLM EMV 2015.3.83, Le Mans, France (cf. Kočová Veselská et al., 2021). Scale bars = 1 mm (A–C,E,F), 200 μm (D).
Material
From Le Mans site, two tubes probably corresponding to Maeandropolydora were found in a CT projection of two specimens of Pyrgopolon (Kočová Veselská et al., 2021; Figures 7E,F). From the Bohemian Cretaceous Basin, a huge specimen is developed inside the O8728 sample.
Description
Specimens from Le Mans appear as rather short, winding cylindrical galleries, constant in diameter (0.2–0.3 mm), branched, and thereby have 2–4 openings. The galleries tend to follow the surface of tubes (Kočová Veselská et al., 2021). The specimen O8728 from the Kaňk locality is much larger. Long, cylindrical galleries have several apertures, running through the substrate in irregular, not too sharp turns. Some of the views of the epoxy cast show that the galleries are duplicated, partially touching each other; in the remaining part, they are somewhat less densely spaced but remain more or less parallel. The length of the individual boring is 5.8 mm, which corresponds to the overall length of the studied serpulid. The diameter of the galleries is somewhat variable but basically circular; it ranges from 0.3 to 0.5 mm. The surface of the galleries is smooth.
Remarks
Contrary to the diagnosis of Maeandropolydora proposed by Bromley and D'Alessandro (1983), no enlarged sections (i.e., vanes and pouches) were observed. The serpulid tube O8728 was apparently filled with calcium carbonate and was redeposited under a high physical energy environment of the rocky shore. Then this cylindrical object was available for drilling. The resulting configuration of borings is therefore quite different from the other studied specimens, which have the character of relatively thin-walled tubes.
Iramena Boekschoten, 1970
?Iramena isp.
Material
Three possible fragments of ?Iramena isp. in the serpulid specimen no. CZ2.
Description
Three short cylindrical or somewhat conical fillings of borings through the entire wall of the serpulid specimen CZ2. The epoxy casts show the stolons of the main borings, which are characterized by a significantly smaller, variable diameter compared to the main boring. The axes of the main bored cylinders/cones form an angle of 70–85° with the surfaces of the tubes, which is close to the values found for Trypanites. The axes of the stolons make an angle of 30–60° with the axis of the main borings.
Remarks
Ichnotaxonomic determination of the borings described above is problematic. However, the presence of tunnels of different diameters and their branching resembles the ichnogenus Iramena, Boekschoten (1970), which is typically diagnosed as “borings consisting of long tunnels in an irregular network, with a round to reniform apertures situated in alternating position laterally to and close by the tunnels” (Boekschoten, 1970). The stenomorphic form of Iramena in a thin tube could look just like an irregular letter “V.” However, it is necessary to make this determination with caution. In the specimen described, the ichnogenus Conchotrema Teichert, 1945 has a similar potential for making “V”-shaped borings (for details on Conchotrema see, e.g., Bromley and D'Alessandro, 1987).
Rogerella de Saint-Seine, 1951
Rogerella isp.
Material
From Le Mans, 209 Rogerella specimens were ascertained on 55 serpulid specimens selected for detailed statistics (Kočová Veselská et al., 2021); they occur in 68.75% of the non-moldic serpulid specimens (55 specimens out of 80).
Description
Elongated, almond-shaped, unornamented pits. Dimensions of borehole openings vary from 0.2 to 2.7 mm in length and 0.1–0.9 mm in width. The depth ranging from 0.4 to 0.6 mm was measured using micro-CT and polymer resin casts. For further details concerning morphology, taphonomy, and interactions, see Kočová Veselská et al. (2021).
The Tracemakers
The Tracemakers of Trypanites
Trypanites is morphologically the simplest and, in terms of the width of environments in which it occurs, a truly universal boring. Bromley (1992) stated that the proven tracemakers of Trypanites are Polychaeta, the crustacean genus Lithotrya, and a few specimens of Sipuncula. It is obvious that among the just mentioned tracemakers are those whose adults are too large to colonize the thin walls of serpulid tubes (e.g., Lithotrya), but also those whose body plan is downscalable in size by one to two orders of magnitude without becoming less functional.
The Tracemakers of Entobia
It is a fact that Porifera (especially Clioniadae) are the makers of Entobia (e.g., Bromley and D'Alessandro, 1984), the ichnogenus widespread in shallow-sea high-energy environments, occurring sporadically also in environments with a lower wave and current energies. Historically, ichnofossils of Entobia have very often been mistaken for Cliona which is the name of the body-fossil. Indeed, the difference in shape between the individual Cliona and corresponding Entobia is usually negligible; however, this does not authorize the use of the tracemaker's name for the domichnion.
Reproduction of Clionaid Sponges
Reproduction of clionaid sponges (i.e., the causative agents of most representatives of the ichnogenus Entobia). The Porifera group (sponges) belongs to the relatively diverse and biologically important, but still relatively little studied representatives of benthic fauna, especially (but not only) in shallow tropical and subtropical seas (Schönberg, 2021). A separate ecological group of boring sponges was soon recognized, initially often called “parasitic” due to their destructive activity on the shells of living bivalves of various genera (Nasonov, 1883), on coral skeletons, and also in the limestone pebbles of the Northumberland coast (Hancock, 1849). These sponges were classified by the mentioned authors into the genera Cliona Grant, 1826 and Vioam Nardo, 1839. Nasonov (1883) approached the question of the technique of boring clionaid sponges into limestone substrates through detailed observations in aquariums; his observations were specified and supplemented by Topsent (1900). Nasonov returned to the subject in 1924. The process of reproduction, colonization of the substrate, and its drilling is described in detail by the mentioned authors, while it is evident that within the family Clionaidae there is a certain diversity. For example, Nasonov (1883) observed that clionaid sponges in aquariums produced eggs, while Topsent (1900) observed larval hatching. The same authors described the process of nesting the larva into the substrate.
This process can be observed by placing thin slices of a prismatic layer of bivalve shells in an aquarium containing the larvae of the sponges. The larvae attach to the surface of these slices and the part of their body facing the substrate emits short, thin protoplasmic expansions, which sink into the substrate and, when viewed from above, the sponge has a rosette structural pattern at this stage. Through these expansions, the larva separates small particles that the larva draws into the body. The particles then pass through the soft tissue and then leave the body of the sponge. (This creates a large amount of micritic fraction, which can be transported and accumulated in other parts of the sedimentary basin; cf. Flügel, 2004). We can conclude that the work of piercing the chambers and galleries of the sponge into bivalve substrates, whose shell construction also contains substances other than CaCO3, is carried out first by a chemical process, i.e., by the excretion of acid and an enzyme that promotes the dissolution of conchiolin. In some sponges, the authors observed the ability to dissolve resistant substances, such as chitin. In the boring sponges, the mechanical process is manifested by the ejection of cut particles out of the body. According to Nasonov (1924) and other authors cited above, the minimum dimensions of the structure attributable to the ichnogenus Entobia are microscopic: young sponges begin drilling at a diameter of about 0.06 mm. The measured values of the diameter of the chambers of the entobians on the Pyrgopolon tubes slightly exceed 0.1 mm; within the above-mentioned morphological and physiological elasticity of this group, it is possible to imagine the formation of an egg in a chamber of this diameter, from which a larva equipped with a flagellum emerging very quickly.
Palaeobiological Positioning of Porifera Living in Small Bioclasts
Due to the need to study benthic communities based on mere pictorial documentation which becomes an often-pronounced requirement, Schönberg (2021) introduced a classification system of Porifera based on a strictly functional interpretation of traditional spongal morphologies. The goal is to deliver a standardized approach that can be optionally display-based and can be applied anywhere. A sophisticated system was introduced which works with 21 morphologies in its end categories; 4 morphologies are considered basic: 1—true crusts, endolithic bio-eroding, and creeping sponges, 2—simple massive, globular massive, composite massive, and tubular sponges, 3—bowls and barrels, 4—one-dimensional, two-dimensional and three-dimensionally erected forms; stalked sponges. From this point of view, based on a study of the extensive literature on recent sponges from various environments, our presented endolithic microforms represent a specialized sub-group within basic morphology no. 1. Their difference/specialization lies mainly in the following aspects: (1) Typical endolithic sponges usually inhabit the environment with the highest hydrodynamics; (2) endolithic sponges have to protect themselves from being buried by rapid sedimentation, and therefore erect forms are common in these environments. Both of these facts do not apply to sponge domichnia in serpulid tubes. The location of Le Mans ring road is characterized by a rather low moderate energy environment (Kočová Veselská et al., 2021) and the absolute predominance of small bioclasts.
The Tracemakers of Maeandropolydora
Maeandropolydora
Maeandropolydora is produced by polychaetes, mostly of the family Spionidae (Bromley and D'Alessandro, 1983, 1987; Farinati and Zavala, 2002) which ranges from intertidal to the depth of 50 m (Wisshak et al., 2005). Spionids are very small polychaete worms (lengths from a few millimeters up to 2–3cm) living in a wide variety of substrates and various habitats (e.g., Blake and Evans, 1973; Blake et al., 2019; Rouse et al., 2022).
Spionids may feed by gathering particles that deposit on the surface of the sea floor using a pair of long, grooved feeding palps with cilia that transport the particles along the palps to the mouth. Spionid tubeworms are selective deposit feeders (Fauchald, 1977) with two grooved palps for caption and prey. Palps may also be used to gather plankton and particles suspended in the water. Their glandular pouches produce mucus that cements sand grains and detritus for building their tubes. These worms are capable of chemical and mechanical boring into different calcareous substrates, including corals, mollusk shells, coralline algae, barnacles, and brachiopods (e.g., Abe et al., 2019; Blake et al., 2019; Abe and Sato-Okoshi, 2020; Malan et al., 2020; Demircan et al., 2021; Villas et al., 2021; Rouse et al., 2022). Shell boring ability is considered species-specific (Sato-Okoshi, 1999; Sato-Okoshi and Okoshi, 2000), albeit some exceptions are mentioned in Radashevsky and Pankova (2013). Some spionid worms may live freely. Spinoid traces described as Caulostrepsis Clarke, 1908 and Maeandropolydora Voigt, 1965 are known from the fossil record as well as from recent environments. The genus Caulostrepsis is characterized by U-shaped boring of various shapes, whereas the genus Maeandropolydora is characterized by more complex, multiple U-shaped, occasionally branching boring systems with often welldeveloped cylindrical galleries (Wisshak and Neumann, 2006). Both ichnogenera are found in many different calcareous and non-calcareous hard substrates. Their fossil record reaches back to the Palaeozoic (Bromley, 2004). The central portion of U-shaped boreholes is filled with detritus and particles of the dissolved substratum. The two openings communicate with the outside of the substratum, and the mud tube protrudes from each opening (Sato-Okoshi and Okoshi, 1997, 2000). Blake and Evans (1973) summarized all known records of polydorid borings in calcareous substrates, boring patterns, specificity of the attack, and larval development. These authors mentioned three main types of polydorid borings described in bivalve shells: surface fouling, U-shaped borings, and mud-blisters. The morphological variations of the traces depend on the type of substrate, abrasion, and density of occupation, and are determined by the changing trajectories of the boring tunnels and channels. Circular to elliptical boreholes in our material show maximum diameter varying between 0.3 and 0.5 mm and length up to 5.8 mm. Compared to dimensions of various Maeandropolydora in fossil and recent substrates (Zottoli and Carriker, 1974; Diez et al., 2011; Demircan, 2012), Maeandropolydora tunnels in our studied serpulids are very tiny; therefore, the tracemaker polydorid worms must have been juveniles or dwarfed adults (Kočová Veselská et al., 2021).
The Tracemakers of Iramena
Boring bryozoans are exclusively members of the class Gymnolaemata. This class encompasses two orders: Cheilostomatida and Ctenostomatida. The boring potential of Ctenostomatida is well known since the nineenth century (d'Orbigny, 1847; Ulrich, 1879; Seguenza, 1880). In contrast, Cheilostomes were regarded as borers in ichnological literature only recently (Taylor et al., 1999; Rosso, 2008).
The current taxonomic position of Ctenostomatida borings is quite confused and therefore problematic. The problem arose due to the mixing of zootaxonomy and ichnotaxonomy approaches and consequently remains a topic of long-lasting and ongoing debate (e.g., Rosso, 2008; Buatois et al., 2017; Wisshak et al., 2019). Ctenostome boring bryozoans are endolithic, living inside the borings in a calcareous substrate, only autozooids' lophophores are reaching out. This adaptation protects their soft, non-mineralized bodies against predation or mechanical disruption (Pohowsky, 1978). Ctenostome bryozoans were also reported to be able to bore into a wooden substrate as exemplified by brackish Bulbella abscondita (Boekschoten, 1966) or semi-boring freshwater form Potsiella erecta (Smith et al., 2003). The boring process begins soon after the larval settlement as the ancestrula is fully embedded with the substrate. The exact substrate selection process during larval settlement is unknown among the boring ctenostomates but presumably, the larvae can select the right type of substrate according to the chemistry of the substrate. This ability was described amid free-living cheilostome bryozoans Cupuladria and Discoporella which can differentiate calcareous from non-calcareous substrates during larval settlement (Driscoll et al., 1971). Astogeny continues with the production of one or more thin stolons (Pohowsky, 1978; Taylor, 2020). Boring by ctenostome bryozoans is presumably done by chemical etching but some degree of mechanical action of soft tissue may be involved as well (Rosso, 2008). The fossil record of ctenostomate borings extends from Ordovician to recent (Taylor, 2020).
Cheilostomatida borings are represented by the ichnogenus Finichnus (Taylor et al., 2013), originally described as Leptichnus (see Taylor et al., 1999, 2013), with three valid ichnospecies: F. peristroma (Taylor et al., 1999), F. dromeus (Taylor et al., 1999) and F. tortus (Rosso, 2008). Cheilostome borings are characterized by shallow pits etched in a hard calcareous substrate whereas the tracemaker is mostly living above the substrate. Boring by cheilostome bryozoans is presumably done by chemical etching (Taylor et al., 1999; Rosso, 2008). The purpose of boring behavior among the Cheilostomatida was interpreted as protection against dislodgement by bioerosion or abrasion (Taylor et al., 1999). Numerous not closely related families within Cheilostomatida are able to bore (e.g., Membraniporidae, Hippothoidae, or Romancheinidae), and therefore this ability is most likely a polyphyletic feature (Taylor et al., 1999; Rosso, 2008). The fossil record of cheilostome borings extends from the late Cretaceous (Maastrichtian) to recent (Taylor, 2020).
The Tracemaker of Rogerella
Although these boreholes produced by Acrothoracica (a group of cirriped barnacles) have been described in many different hard substrates from the Ordovician (Taylor and Wilson, 2003; Blissett and Hunter, 2005; Vogel and Brett, 2009), Middle Devonian, late Paleozoic, Jurassic (Breton et al., 2020) and Cretaceous (e.g., von Lukeneder, 1999; Breton, 2011; Donovan and Jagt, 2013; Donovan et al., 2015; Brezina et al., 2017), the tubes from the locality Le Mans represent the first known case of interaction between boring barnacles and serpulid worms (Kočí et al., 2017; Kočová Veselská et al., 2021). Seilacher (1969) noted that Acrothoracica shows a preference for skeletal carbonates, particularly epibenthonic sessile hosts, and that although differences in mineralogy and ecology of the substrate do not limit acrothoracican distribution, these cirripedes are more selective than boring sponges and annelids. Acrothoracicans thus infested Pyrgopolon tubes probably due to the absence of any other suitable thick-shelled substrate on the soft bottom. Seilacher (1969) also observed that acrothoracican borings are often clustered in certain areas, but boreholes in the examined belemnite rostra are randomly distributed without obvious grouping.
In the case of substrates as small as serpulid tubes, the question arises as to whether these were early ontogenetic phases of acrothoracicans or whether they were miniature but already adult individuals. In other words, we are looking for an ontogenetic series comparable to the growth stages of the ichnogenus Entobia (Bromley and D'Alessandro, 1983). Fortunately, there is a relatively sufficient supply of literature data to find the answer. Brezina et al. (2017) stated that Rogerella consists of a hole and a discernible slot. The slot, seen by the present authors, resembles the edge of the eye of birds or mammals. Brezina et al. (2017) stated that recent acrothoracicans penetrate the substrate during the larval stage by a combination of chemical dissolution and mechanic processes enabled by chitinous bristles. Lambers and Boekschoten (1986) wrote a pioneering work comparing recent drilling of common species of acrothoracicans with fossil borings. Their Figure 2 (page 260) shows that the length of the borehole (Rogerella isp.) is typically greater than the depth of the burrow. The figures also demonstrate that the length of the opening represents approximately 2/3 of the burrowing depth. Regarding the distribution of borings on the substrate surface, the above-mentioned authors stated that the perforation shows a preferred orientation when they appear in clusters; however, Rogerella occurs in the largest numbers on belemnite rostra are randomly distributed over the entire surface.
The oldest so far known (i.e., Ordovician) finds of Rogerella are also some of the smallest ones described so far: 0.2–0.4 mm long at the base, about 0.03–0.09 mm wide, and about 0.02–0.2 mm in depth (Vogel and Brett, 2009).
From the description of acrothoracican nesting and growth, Brezina et al. (2017) conclude that the boring process begins at the slot location; then it spreads, probably relatively quickly, into the main chamber. The growth of the acrothoracicans is apparently ontogenetically limited, as several authors describe and depict Rogerella fissures of the same size on large surfaces (typically belemnites, but virtually all other mollusk shells, and biodetritic limestones). Thereby, Rogerella described from Le Mans (Kočová Veselská et al., 2021) can be considered small but fully-grown and mature.
Discussion
Ordinary Residents or Doomed Pioneers?
Miniature domichnia in sub-macroscopic bioclasts is a seldom-studied group of ichnofossils; an exception is, among others, the work of Kočová Veselská et al. (2021), to which the present report is directly related. The work of Kočová Veselská et al. (2021) deals with serpulid communities and their boreholes (especially the boreholes of Acrothoracica, the ichnogenus Rogerella) from a single locality on Le Mans ring road. The present work, moreover, first adds information from three localities (Cenomanian to Turonian) of the Bohemian Cretaceous Basin; secondly, it pays equal attention to the ichnogenera Entobia, Trypanites, and Iramena. The questions to be asked are the same for all of these borings: Did the larvae of the makers of these borings suffer the fate of “doomed pioneers” (Föllmi and Grimm, 1990), or did the colonizers of the tiny substrates represent a prosperous, sophisticated population?
There are several clues that may suggest answers to these questions in specific cases. Most of them point to the answer that the boring community of serpulid tubes was a functioning population. In the case of small representatives of the ichnogenus Trypanites, such an indication is the uniformity of the substrate coverage by the openings of the borings (cf. Figures 2A–D); there are tubes typically densely covered with mouths (Figures 2A,D) and, conversely, weaker but also evenly colonized tubes (Figure 2B). The tracemaker precisely determined the depth of the shafts so that the inner surface of the inner tube of the serpulid was not perforated and there is no unwanted flow through the domestic space. The larvae settling in the future mouths of Trypanites appear to have communicated accurately with surrounding individuals, apparently chemically; otherwise, it would be difficult to achieve even coverage of the tube surface.
The morphology of the ichnogenus Entobia provides a stronger indication of beneficial functioning in the benthos association. Bromley and D'Alessandro (1983, 1987) provided a classical overview of the taxonomy of the ichnogenus Entobia. This taxonomy is extremely difficult mainly because most entobians (or their originators from the family Clionidae) go through a series of up to five growth stages. The criterion of aging is usually to increase the volume of the chambers at the expense of galleries and exploratory threads. While the very early stages have only embryo(s) of a chamber(s) and exploratory threads, the elderly specimens have fully developed chambers or, in the case of non-camerate entobians, long and wide galleries and a small volume of exploratory threads. Our findings, both from Le Mans (Kočová Veselská et al., 2021: Figure 8) and from the Bohemian Cretaceous Basin, have bag-like chambers, connected by thin exploratory threads only to the immediate vicinity of the mouth. The ichnospecies Entobia solaris Mikuláš, 1992 has similar relations to exploratory threads; in that case, the chamber has the shape of a hemisphere, so the mouth is very wide, and exploration takes place only on the surface of the rockground (Mikuláš, 1992, 2004). A calm environment of relatively deep-sea crevices or olistolith walls is assumed (Mikuláš, 2004).
In any case, the configuration of Entobia isp. indicates a recurring event after the larva has settled on the surface of the serpulid tube. Even in this case, the circumstances point to a specific, functioning component of the ecosystem, rather than the result of the “wandering” of random larvae of clionid sponges into an environment unfavorable to them.
The ichnogenus Iramena was found only in one serpulid specimen from the Bohemian Cretaceous Basin. However, it is well preserved, including sloping partitions with a minimum diameter of 0.03 mm. The group of conical cavities identified herein as ?Iramena isp. is not damaged by any subsequent drilling; it was therefore probably the last boring performed on the tube. In summary, regardless of the small size, the found individual has all the characteristics of an adult.
The tracemakers of the ichnogenus Rogerella in serpulid tubes were discussed in detail by Kočová Veselská et al. (2021). The obtained data indicate a shape agreement (relatively shallow slits maintaining the necessary distance from the inner surface of the tubes) with groups of Rogerella of usual (macroscopic) individuals. The findings do not cast doubt on the fact that cirripeds drilling into serpulid tubes in the studied areas reached adulthood; both the slit considered the work of larval stages, and the rest of the boring is clearly discernible.
How and why Are Le Mans Sites and the BCB Surf Facies Area Different?
The difference in the number of bored serpulids in Le Mans ring road and the Bohemian Cretaceous Basin is probably caused by the quantity of thick-shelled organisms at localities. The nearshore Czech late Cenomanian to early Turonian deposits yielded numerous bivalves, brachiopods, echinoids, and other shelly fauna (e.g., Žítt et al., 1997, 1998, 2006, 2015; Vodrážka et al., 2013; Kočová Veselská et al., 2015). These are more suitable for constructing domichnia than serpulids with tube walls ranging between 0.3 and 0.6 mm only. This may also explain the absence of Rogerella boreholes in serpulids at Czech localities where thicker-shelled invertebrates were more attractive to acrothoracicans than the relatively thin-shelled serpulids. In the area of Le Mans ring road, free-lying serpulid tubes acted as small solid benthic islands for colonization by invertebrate fauna due to the sparsity of suitable hard substrate on the soft bottom. Serpulid tubes were thus highly infested by various epi- and endobionts, including acrothoracicans, whose boreholes occurred on 35.25% of all Pyrgopolon specimens and simultaneously on 60% of 235 bored tubes, with a range of 0–11 Rogerella borings per tube (Kočová Veselská et al., 2021). It is obvious that tiny serpulid walls had to be very uncomfortable for acrothoracicans because they often reached the tube-wall bottoms (Figure 5F) in an effort to maximize the use of space for boreholes (Kočová Veselská et al., 2021). It should be noted that other late Cenomanian open sea localities of Le Mans region described by Breton et al. (2017) and Kočí et al. (2017) are very rich in thicker-shell marine fossils, especially mollusks, bryozoans, brachiopods, and echinoderms. Serpulids and sabellids found there are often not free-lying but attached to oyster valves, pectinid valves, ammonite shells, sabellid (Glomerula), and other serpulid tubes, bryozoans, and cobbles. Because there was a lot of more suitable hard substrate, similar to the Czech late Cenomanian to early Turonian nearshore deposits, thin serpulid, and sabellid tubes show no Rogerella boreholes and overall less domichnial borings than Pyrgopolon specimens from the ring road.
Conclusion
1. Small tubes of serpulids (length about 25–30 mm, width at tube near anterior end usually 2–4 mm) provided in the late Cretaceous (and probably until today) a specific habitat for specialized boring organisms. Predation on serpulids is common and may leave traces, e.g., Oichnus. However, also domichnia, borings created in such small tubes for living, are common. The basic ichnotaxa left behind by the miniature drillings are Trypanites, Rogerella, and Entobia, to a lesser extent Maeandropolydora and Iramena. In the case of Trypanites, Polychaeta and Sipuncula can be considered as the tracemakers. Crustacean Lithotrya cannot be taken into consideration; its relatives have never reached such a small size that they can act as borers with a diameter of <0.5 mm. Rogerella is undoubtedly formed by Acrothoracica (cirripedes). Construction details, such as the presence of a slut and a wellfunctioning equation determining the relationship between the length of the opening and the depth of the living chamber, can be considered the ichnological signature of the cirripedes.
2. Entobia isp. is found relatively abundantly in small serpulid tubes preserved in the fine sandy substrate (Le Mans ring road), but much rarer in mixed variable substrates of the surf facies of the BCB. In both cases, however, the morphology corresponds to the oldest developmental stages in terms of the growth phases as defined by Bromley and D'Alessandro (1983). A similar situation concerns the ichnogenus Rogerella, which is very abundant in serpulids from Le Mans ring road due to the fact that there was no more suitable hard substrate for boring organisms. On the contrary, Rogerella was not found in serpulids from the surf facies of the BCB with various offerings of hard substrates.
3. The clastic substrate with carbonate bioclasts ranging from tenths of a millimeter to a few millimeters represents a yet unrecognized environment for the existence of Porifera. To the 21 categories introduced by Schönberg (2021), we can supplement “isolated microscopic individuals of sponges in carbonate-clastic substrates.” Given the easy availability of these substrates for sampling, it would be desirable to focus on finding a recent analogy.
Data Availability Statement
The original contributions presented in the study are included in the article/supplementary material, further inquiries can be directed to the corresponding author.
Author Contributions
RM formulated the basic ideas of the study, addressed the author's team, wrote initial versions of ichnological parts and paragraphs dealing with sponges, gradually compiled, and critically revised the emerging text with the assistance of MK. Together with MK, he selected, prepared illustrations, and wrote the epoxy-casting methodology. MK and TK provided all the studied material and wrote initial forms of chapters on serpulids and worm tracemakers, they also made a significant contribution with their ichnological knowledge. During field studies, TK and MK obtained all the material described here from the Bohemian Cretaceous Basin. JŠ wrote draft paragraphs on bryozoans and read an early draft. MJ provided field data and material from Le Mans and critically read and supplemented the early draft of the article. TK determined serpulid material in this study. ZH processed the material from Le Mans area on micro-CT, wrote a micro-CT methodology, and critically read the whole manuscript. JB processed the material from the Bohemian Cretaceous Basin on micro-CT and contributed to the methodology. All authors contributed to the article and approved the submitted version.
Funding
RM, MK, ZH and JB were financially supported by the Czech Science Foundation (GACR 18-05935S). Additional support for RM and MK was obtained from the institutional project of the Czech Academy of Sciences, Institute of Geology (RVO 67985831). MK was supported by the Centre for Geosphere Dynamics (UNCE/SCI/006). TK, JB, and ZH obtained funding from the Ministry of Culture of the Czech Republic (DKRVO 2019–2023/ 2.I.d, National Museum, 00023272). JŠ acknowledges research project MUNI/A/1394/2021.
Conflict of Interest
The authors declare that the research was conducted in the absence of any commercial or financial relationships that could be construed as a potential conflict of interest.
Publisher's Note
All claims expressed in this article are solely those of the authors and do not necessarily represent those of their affiliated organizations, or those of the publisher, the editors and the reviewers. Any product that may be evaluated in this article, or claim that may be made by its manufacturer, is not guaranteed or endorsed by the publisher.
Acknowledgments
The authors thank Jan Sklenár and Lenka Váchová (NM Prague) for access to SEM facilities of the National Museum in Prague. Dr. Nicolas Morel (Musée Vert, Le Mans) is acknowledged for the extraordinary care of the serpulid collection from Le Mans. Max Wisshak (Wilhelmshaven) is thanked for making several epoxy casts of the studied material.
References
Abe, H., and Sato-Okoshi, W. (2020). Novel symbiotic relationship between a spionid polychaete and Lingula (Brachiopoda: Lingulata: Lingulidae), with description of Polydora lingulicola sp. nov. (Annelida: Spionidae). Zoosymposia 19, 103–120. doi: 10.11646/zoosymposia.19.1.13
Abe, H., Takeuchi, T., and Taru, M. (2019). Habitat availability determines distribution patterns of spionid polychaetes (Annelida: Spionidae) around Tokyo Bay. Mar. Biodivers. Rec. 12, 7, doi: 10.1186/s41200-019-0167-4
Bertling, M., Braddy, R., Bromley, R. G., Demathieu, G., Genise, J., Mikuláš, R., et al. (2006). Names for trace fossils: a uniform approach. Lethaia 39, 265–286. doi: 10.1080/00241160600787890
Bertling, M., Buatois, L. A., Knaust, D., Laing, B., Mángano, M. G., and Meyer, N. (2022). Names for trace fossils 2.0: theory and practice in ichnotaxonomy. Lethaia.
Blake, J. A., and Evans, J. W. (1973). Polydora and related genera as borers in mollusks shells and other calcareous substrates. Veliger 15, 235–250.
Blake, J. A., Maciolek, N. J., and Meissner, K. (2019). “Spionidae Grube, 1850,” in Annelida, Volume 2: Pleistoannelida, Sedentaria II, eds. G. Purschke, M. Böggemann, and W. Westheide (Berlin/Boston: Walter de Gruyter GmbH and Co. KG), p. 1–103.
Blissett, D. J., and Hunter, A. W. (2005). Review of “marine hard substrates: colonization and evolution,” a thematic session at the Geological Society of America Annual Meeting, Denver, USA, November 7–10, 2004. Ichnos 12, 301–302. doi: 10.1080/10420940500311095
Boekschoten, G. J. (1966). Shell borings of sessile epibiontic organisms as palaeoecological guides (with examples from the Dutch coast). Palaeogeogr. Palaeoecl. 2, 333–379. doi: 10.1016/0031-0182(66)90023-X
Boekschoten, G. J. (1970). “On bryozoan borings from the Danian at Fakse, Denmark,” in Trace fossils, eds. T.P. Crimes and J.C. Harper. Geol. J. (Spec. Issue) 3 43–48.
Breton, G. (2011). Le Gault du Perthois: terriers, phosphates et petites crottes. Bull. Ass. Géologique Auboise 31, 3–66.
Breton, G., Jäger, M., and Kočí, T. (2020). The sclerobionts of the Bajocian Oolithe ferrugineuse de Bayeux Formation from Calvados (Paris Basin, Normandy, France). Ann. Paléontol. 106, 102361. doi: 10.1016/j.annpal.2019.07.002
Breton, G., Wisshak, M., Néraudeau, D., and Morel, N. (2017). Parasitic gastropod bioerosion trace fossil on Cenomanian oysters from Le Mans, France and its ichnologic and taphonomic context. Acta Palaeontol. Pol. 62, 45–57. doi: 10.4202/app.00304.2016
Brezina, S. S., Romero, V., and Casadío, S. A. (2017). Encrusting and boring barnacles through the Cretaceous/Paleogene boundary in northern Patagonia (Argentina). Ameghiniana 54, 107–123. doi: 10.5710/AMGH.11.10.2016.2969
Bromley, R. G. (1972). On some ichnotaxa in hard substrates, with a redefinition of Trypanites Mägdefrau. Paläont. Z. 46, 93–98. doi: 10.1007/BF02989555
Bromley, R. G. (1981). Concepts in ichnotaxonomy illustrated by small round holes in shells. Acta Geol. Hisp. 16, 55–64.
Bromley, R. G. (1992). Bioerosion: eating rocks for fun and profit. Short Course Paleontol. 5, 121–129. doi: 10.1017/S2475263000002312
Bromley, R. G. (1996). Trace Fossils—Biology, Taphonomy and Applications. London: Chapman and Hall p. 347.
Bromley, R. G. (2004). “A stratigraphy of marine bioerosion,” in The Application of Ichnology to Palaeoenvironmental and Stratigraphic Analysis, ed. D. McIlroy (London: Special Publication) p. 455–479.
Bromley, R. G. (2005). “Preliminary study of bioerosion in the deep-water cora Lophelia, Pleistocene, Rhodes, Greece,” in Cold-Water Corals and Ecosystems, eds Freiwald, A., Roberts, J.M. (Berlin, Heidelberg: Springer), pp. 895–914.
Bromley, R. G., and D'Alessandro, A. (1983). Bioerosion in the Pleistocene of Southern Italy: Ichnogenera Caulostrepsis and Maeandropolydora. Riv. Ital. Paleontol. S. 89, 283–309.
Bromley, R. G., and D'Alessandro, A. (1984). The ichnogenus Entobia from the miocene, pliocene and pleistocene of Southern Italy. Riv. Ital. Paleontol. S. 90, 227–296.
Bromley, R. G., and D'Alessandro, A. (1987). Bioerosion of the Plio-Pleistocene transgression of Southern Italy. Riv. Ital. Paleontol. S. 93, 379–442.
Bromley, R. G., Wisshak, M., Glaub, I., and Botquelen, A. (2007). “Ichnotaxonomic review of dendriniform borings attributed to foraminiferans: Semidendrina igen. nov.” in Trace Fossils: Concepts, Problems, Prospects, ed. W. Miller, III(Amsterdam: Elsevier), p. 518–530.
Bronn, H. G. (1837). Lethaea Geognostica, Abbildungen und Beschreibungen der für die Gebirgs-Formationen bezeichnendsten Versteinerungen 1. Stuttgart: Schweizerbart.
Brünnich Nielsen, K. (1931). Serpulidae from the Senonian and Danian deposits of Denmark. Medd. Dansk geol. Foren. 8, 71–113.
Buatois, L. A., Wisshak, M., Wilson, M. A., and Mángano, M. G. (2017). Categories of architectural designs in trace fossils: a measure of ichnodisparity. Earth Sci. Rev. 164, 102–181. doi: 10.1016/j.earscirev.2016.08.009
Calvo, J. M., Gil, E., and Meléndez, G. (1987). Megaplanolites ibericus (ichnogen. et ichnosp. nov.), a new trace fossil from the Upper Jurassic (uppermost Oxfordian) of Bueña (Teruel Province, Iberian Chain, Spain). Palaeogeogr. Palaeoecl. 61, 199–204. doi: 10.1016/0031-0182(87)90049-6
de Montfort, D. (1808). Conchyliologie systématique et classification méthodique des coquilles, 1. Coquilles univalves, cloisonées. Paris: F. Schoell.
de Saint-Seine, R. (1951). Un Cirripède acrothoracique du Crétacé: Rogerella lecontrei n.g., n. sp. C. R. Acad. Sci. 233, 1051–1054.
Demircan, H. (2012). Determination of a late miocene rocky palaeoshore by bioerosion trace fossils from the Bozcaada Island, Çanakkale, Turkey. C. R. Palevol. 11, 331–344. doi: 10.1016/j.crpv.2011.11.002
Demircan, H., El Sorogy, A., and Alharbi, T. (2021). Bioerosional structures from the Late Pleistocene coral reef, Red Sea coast, northwest Saudi Arabia. Turk. J. Earth Sci. 30, 22–37. doi: 10.3906/yer-2005-7
Diez, M. E., Radashevsky, V. I., Orensanz, J. M., and Cremonte, F. (2011). Spionid polychaetes (Annelida: Spionidae) boring into shells of molluscs of commercial interest in northern Patagonia, Argentina. Ital. J. Zool. 78, 497–504. doi: 10.1080/11250003.2011.572565
Donovan, S. K., and Jagt, J. W. M. (2013). Rogerella isp. infesting the Pore Pairs of Hemipneustes striatoradiatus (Leske) (Echinoidea: Upper Cretaceous, Belgium). Ichnos 20, 153–156. doi: 10.1080/10420940.2013.845098
Donovan, S. K., Jagt, J. W. M., and Nieuwenhuis, E. (2015). Site selectivity of the boring Rogerella isp. infesting Cardiaster granulosus (Goldfuss) (Echinoidea) in the type Maastrichtian (Upper Cretaceous, Belgium). Geol. J. 51, 789–793. doi: 10.1002/gj.2692
d'Orbigny, A. D. (1847). “Zoophytes,” in Voyage dans l'Amerique méridionale, ed. P. Bertrand (Paris and Strasbourg), p. 7–28.
Driscoll, E. G., Gibson, J. W., and Mitchell, S. W. (1971). Larval selection of substate by the Bryozoa Discoporella and Cupuladria. Hydrobiologia 37, 347–359. doi: 10.1007/BF00015580
Farinati, E., and Zavala, C. (2002). Trace fossils on a shelly substrate. an example from the Miocene of Patagonia, Argentina. Acta Geol. Hisp. 37, 29–36. Available online at: https://raco.cat/index.php/ActaGeologica/article/view/75730
Fauchald, K. (1977). The Polychaete worms definitions and keys to the orders, families and genera. Nat. Hist. Mus. L. A. County, Sci. Ser. 28, 1–190.
Flügel, E. (2004). Microfacies of Carbonate Rocks. Analysis, Interpretation and Application. Berlin, Heidelberg, New York: Springer.
Föllmi, K. B., and Grimm, K. A. (1990). Doomed pioneers: gravity-flow deposition and bioturbation in marine oxygen-deficient environments. Geology 18, 1069–1072.
Glaub, I. (1994). Mikrobohrspuren in ausgewählten Ablagerungsräumen des europäischen Jura und der Unterkreide (Klassifikation und Palökologie). Cour. Forsch. Senck. 174, 1–324.
Grant, R. E. (1826). Notice of a New Zoophyte (Cliona celata Gr.) from the Firth of Forth. Edinburgh New Phil. J. 1, 78–81.
Hancock, A. (1849). On the excavating powers of certain sponges belonging to the genus Cliona; with descriptions of several new species, and an allied generic form. Annals Magazine Nat History Zool 2, 321–348. doi: 10.1080/03745485909494773
Juignet, P. (1974). La transgression crétacée sur la bordure orientale du Massif armoricain. Aptien, Albien, Cénomanien de Normandie et du Maine. Le stratotype du Cénomanien. [dissertation thesis]. [Caen (FR)]: Université de Caen.
Knaust, D. (2012). “Methodology and techniques,” in Trace fossils as indicators of sedimentary environments, eds Knaust, D., and Bromley, R.G., pp. 245–271.
Kočí, T., Jäger, M., and Morel, N. (2017). Sabellid and serpulid worm tubes (Polychaeta, Canalipalpata, Sabellida, Sabellidae, Serpulidae) from the Cenomanian (Late Cretaceous) stratotype (Le Mans area, Sarthe, Pays de la Loire, France). Ann. Paleontol. 103, 45–80. doi: 10.1016/j.annpal.2016.11.004
Kočová Veselská, M., Kočí, T., Collins, J. S. H., and Gale, A. S. (2015). A new species of scalpelliform cirripede (Crustacea, Cirripedia) from the Upper Cenomanian–Lower Turonian shallow-water facies at Velim (Bohemian Cretaceous Basin) and its palaeoecological implications. Neues Jahrb. Geol. P.-A. 278, 201–211. doi: 10.1127/njgpa/2015/0525
Kočová Veselská, M., Kočí, T., Jäger, M., Mikuláš, R., Hermanová, Z., Morel, N., et al. (2021). Sclerobionts on tubes of the serpulid Pyrgopolon (Pyrgopolon) deforme (Lamarck, 1818) from the upper Cenomanian of Le Mans region, France. Cretaceous Res. 125, 104873. doi: 10.1016/j.cretres.2021.104873
Lambers, P., and Boekschoten, G. J. (1986). On fossil and recent borings produced by acrothoracic cirripeds. Geol. Mijnbouw 65, 257–268.
Leymerie, A. (1842). Suite du mémoire sur le terrain crétacé du Département de l'Aube. Seconde partie. Mem. S. Geo. F. 5, 1–34.
Mägdefrau, K. (1932). Über einige Bohrgänge aus dem Unteren Muschelkalk von Jena. Palaont. Z. 14, 150–160. doi: 10.1007/BF03041628
Malan, A., Williams, J. D., Abe, H., Sato-Okoshi, W., Matthee, C. A., and Simon, C. A. (2020). Clarifying the cryptogenic species Polydora neocaeca Williams and Radashevsky, 1999 (Annelida: Spionidae): a shell-boring invasive pest of molluscs from locations worldwide. Mar. Biodivers. 50, 1–19. doi: 10.1007/s12526-020-01066-8
Mikuláš, R. (2004). Bioerosion in the geologic record of the Czech Republic. Field trip guide for the pre-conference excursion 4th International Bioerosion Workshop August 22–August 28, Czech Republic. Institute of Geology of the Czech Republic, Prague. ISSN: 80-9019404-9-9.
Mikuláš, R., Kadlecová, E., Fejfar, O., and Dvorák, Z. (2006). Three new ichnogenera of biting and gnawing traces on reptilian and mammalian bones: a case study from the Miocene of the Czech Republic. Ichnos 13, 113–127. doi: 10.1080/10420940600850729
Morel, N. (2015). Stratotype Cénomanien. Patrimoine géologique 6. Paris, Biotope, Mèze: Muséum national d'Histoire naturelle.
Nardo, D. G. (1839). Sopra un nuovo genere di spugne, le quali perforano le pietri ed i gusci marini. Letta al veneto Ateneo nel giorno 29 Aprile 1839, ed all' Assemblea de' Naturalisti tenutasi in Pisa il giorno 7 Ottobre dell' anno stesso. Ann. Sci. Regno Lombardo-Veneto 9, 221–226.
Nasonov, N. (1924). Sur l'éponge perforante Cliona stationis Nason. et le procédé du creusement des laeries dans les valves des huîtres. Dokl. Akad. Nauk SSSR—Comptes Rendus de l'Académie des Sciences de l'URSS, Ser. A 113–115.
Nielsen, K. S. S., Nielsen, J. K., and Bromley, R. G. (2003). Palaeoecological and ichnological significance of microborings in Quaternary foraminifera. Palaeontol. Electron. 6, 13. Available online at: http://palaeo-electronica.org/paleo/2003_1/ichno/issue1_03.htm
Pemberton, S. G., and Frey, R. W. (1982). Trace fossil nomenclature and the Planolites-Palaeophycus dilemma. J. Paleontol. 56, 843–881.
Philippi, A. (1844). Einige Bemerkungen über die Gattung Serpula, nebst Aufzählung der von mir im Mittelmeer mit dem thier beobachteten Arten. Arch. Naturgeschichte 10, 186–198. doi: 10.5962/bhl.part.29558
Pohowsky, R. A. (1978). The boring ctenostomate Bryozoa: taxonomy and paleobiology based on cavities in calcareous substrata. Bull. Am. Paleontol. 73, 1–192.
Radashevsky, V. I., and Pankova, V. V. (2013). Shell-boring versus tube-dwelling: is the mode of life fixed or flexible? two cases in spionid polychaetes (Annelida, Spionidae). Mar. Biol. 160, 1619–1624. doi: 10.1007/s00227-013-2214-8
Radtke, G. (1991). Die mikroendolithischen Spurenfossilien im Alt-Tertiär West-Europas und ihre palökologische Bedeutung. Cour. Forsch. Senck. 138, 1–150.
Rosso, A. (2008). Leptichnus tortus isp. nov., a new cheilostome etching and comments on other bryozoan-produced trace fossils. Studi Trent. Sci. Nat., Acta Geol. 83, 75–85.
Rouse, G. W., Pleijel, F., and Tilic, E. (2022). Annelida. London/New York :Oxford University Press.
Sato-Okoshi, W. (1999). Polydorid species (Polychaeta: Spionidae), Japan, with descriptions of morphology, ecology and burrow structure. 1. Boring species. J. Mar. Biol. Assoc. U. K. 79, 831–848. doi: 10.1017/S0025315498001003
Sato-Okoshi, W., and Okoshi, K. (1997). Survey of the genera Polydora, Boccardiella and Boccardia (Polychaeta, Spionidae) in Barkley Sound (Vancouver Island, Canada), with special reference to boring activity. B. Mar. Sci. 60, 482–493.
Sato-Okoshi, W., and Okoshi, K. (2000). Structural characteristics of self-excavated burrows by boring polydorid species (Polychaeta, Spionidae). B. Mar. Sci. 67, 235–248.
Schönberg, C. H. L. (2021). No taxonomy needed: sponge functional morphologies inform about environmental conditions. Ecol. Indic. 126, 107806. doi: 10.1016/j.ecolind.2021.107806
Seilacher, A. (1969). Paleoecology of boring barnacles. Am. Zool. 9, 705–719. doi: 10.1093/icb/9.3.705
Smith, D. G., Werle, S. F., and Klekowski, E. (2003). The anatomy and brooding biology of Pottsiella erecta (Potts, 1884) (Ectoprocta: Gymnolaemata: Ctenostomata), with an expanded diagnosis of the Pottsiellidae. Hydrobiologia 490, 135–145. doi: 10.1023/A:1023422814468
Tapanila, L. (2008). “The medium is the message: imaging a complex microboring (Pyrodendrina cupra igen. n., isp. n.) from the early Paleozoic of Anticosti Island, Canada,” in Current Developments in Bioerosion, eds M. Wisshak and L. Tapanila, L., (Erlangen Earth Conference Series, Berlin: Springer), p. 123–145.
Taylor, P. D., and Wilson, M. A. (2003). Palaeoecology and evolution of marine hard substrate communities. Earth-Sci. Revs. 62, 1–103. doi: 10.1016/S0012-8252(02)00131-9
Taylor, P. D., Wilson, M. A., and Bromley, R. G. (1999). A new ichnogenus for etchings made by cheilostome bryozoans into calcareous substrata. Palaeontology 42, 595–604. doi: 10.1111/1475-4983.00087
Taylor, P. D., Wilson, M. A., and Bromley, R. G. (2013). Finichnus, a new name for the ichnogenus Leptichnus Taylor, Wilson and Bromley, 1999, preoccupied by Leptichnus Simroth, 1896 (Mollusca, Gastropoda). Palaeontology 56, 456. doi: 10.1111/pala.12000
Teichert, C. (1945). Parasitic worms in Permian brachiopod and pelecypod shells in Western Australia. Am. J. Sci. 243, 197–209. doi: 10.2475/ajs.243.4.197
Topsent, E. (1900). Étude monographique des spongiaires de France. III. Monaxonida (Hadromerina). Arch. Zool. Exp. Gen. 3, 1–331.
Ulrich, E. O. (1879). Descriptions of new genera and species of fossils from the Lovuer Silurian about Cincinnati. J. Cincinnati Soc. Nat. Hist. 2, 8–30.
Villas, E., Mayoral, E., Santos, A., Colmenar, J., and Gutiérrez-Marco, J. C. (2021). Annelid borings on brachiopod shells from the upper ordovician of peru. a long-distance co-migration of biotic partners. Front. Ecol. Evol. 9, 766290. doi: 10.3389/fevo.2021.766290
Vodrážka, R., Bubík, M., Švábenická, L., and Žítt, J. (2013). late cretaceous (upper cenomanian–lower turonian) transgressive deposits near kutná hora and kolín (central bohemia, bohemian cretaceous basin). Prague: The Micropaleontological Society, Spring Meeting 2013, Excursion Guide, Faculty of Science, Charles University.
Vogel, K., and Brett, C. E. (2009). Record of microendoliths in different facies of the Upper Ordovician in the Cincinnati Arch region USA: the early history of light-related microendolithic zonation. Palaeogeogr. Palaeocl. 281, 1–24. doi: 10.1016/j.palaeo.2009.06.032
Voigt, E. (1965). Über parasitische Polychaeten in Kreide-Austern sowie einige andere in Muschelschalen bohrende Würmer. Paleont. Z. 39, 193–211. doi: 10.1007/BF02990164
von Lukeneder, A. (1999). Acrothoracica-Bohrspuren an einem Belemnitenrostrum (Unterkreide; Obervalangium; Oberösterreich). Ann. Naturhist. Mus. Wien 101 A, 137–143.
Wisshak, M. (2008). “Two new dwarf Entobia ichnospecies in a diverse aphotic ichnocoenosis (Pleistocene / Rhodes, Greece),” in Current Developments in Bioerosion. eds M.Wisshak, and L. Tapanila, (Berlin: Springer Erlangen Earth Conference Series), p. 213–233.
Wisshak, M. (2012). “Microbioerosion,” in Trace Fossils as Indicators of Sedimentary Environments, eds. D. Knaust, and R.G. Bromley (Amsterdam: Elsevier) p. 213–243.
Wisshak, M., Gektidis, M., Freiwald, A., and Lundälv, T. (2005). Bioerosion along a bathymetric gradient in a cold temperate setting (Kosterfjord, SW Sweden): an experimental study. Facies 51, 93–117. doi: 10.1007/s10347-005-0009-1
Wisshak, M., Knaust, D., and Bertling, M. (2019). Bioerosion ichnotaxa: review and annotated list. Facies 65, 24. doi: 10.1007/s10347-019-0561-8
Wisshak, M., and Neumann, C. (2006). A symbiotic association of a boring polychaete and an echinoid from the Late Cretaceous of Germany. Acta Palaeontol. Pol. 51, 589–597.
Žítt, J., Nekvasilová, O., Bosák, P., and Svobodová, M. (1997). Rocky coast facies of the Cenomanian-Turonian boundary interval at Velim (Bohemian Cretaceous Basin, Czech Republic). Bull. Czech Geol. Surv. First Part 72, 83–102; Second Part 72, 141–155.
Žítt, J., Nekvasilová, O., Hradecká, L., Svobodová, M., and Záruba, B. (1998). Rocky coast facies of the Unhošt-Tursko High (Late Cenomanian-Early Turonian, Bohemian Cretaceous Basin). Acta Mus. Nat. Pragae, Ser. B—Hist. Nat. 54 79–116.
Žítt, J., Vodrážka, R., Hradecká, L., Svobodová, M., Štastný, M., and Švábenická, L. (2015). Depositional and palaeoenvironmental variation of lower Turonian nearshore facies in the Bohemian Cretaceous Basin, Czech Republic. Cretaceous Res. 56, 293–315. doi: 10.1016/j.cretres.2015.05.007
Žítt, J., VodráŽka, R., Hradecká, L., Svobodová, M., and Zágoršek, K. (2006). Late Cretaceous environments and communities as recorded at Chrtníky (Bohemian Cretaceous Basin, Czech Republic). Bull. Geosci. 81, 43–79. doi: 10.3140/bull.geosci.2006.01.043
Keywords: bioerosion, Serpulidae, Cenomanian, Turonian, stenomorphism
Citation: Mikuláš R, Kočová Veselská M, Kočí T, Šamánek J, Jäger M, Heřmanová Z and Bruthansová J (2022) Domichnial Borings in Serpulid Tube Walls: Prosperous Benthic Assemblages in the Cretaceous of France and the Czech Republic. Front. Ecol. Evol. 10:882450. doi: 10.3389/fevo.2022.882450
Received: 23 February 2022; Accepted: 08 April 2022;
Published: 16 May 2022.
Edited by:
Barbara Calcinai, Polytechnic University of Marche, ItalyReviewed by:
Francisco J. Rodriguez-Tovar, University of Granada, SpainOlev Vinn, University of Tartu, Estonia
Copyright © 2022 Mikuláš, Kočová Veselská, Kočí, Šamánek, Jäger, Heřmanová and Bruthansová. This is an open-access article distributed under the terms of the Creative Commons Attribution License (CC BY). The use, distribution or reproduction in other forums is permitted, provided the original author(s) and the copyright owner(s) are credited and that the original publication in this journal is cited, in accordance with accepted academic practice. No use, distribution or reproduction is permitted which does not comply with these terms.
*Correspondence: Radek Mikuláš, bWlrdWxhc0BnbGkuY2FzLmN6