- 1Laboratoire EDB Évolution and Diversité Biologique UMR 5174, Université Toulouse 3 Paul Sabatier, UPS, CNRS, IRD, Université de Toulouse, Toulouse, France
- 2Institut Méditerranéen de Biodiversité et d’Ecologie marine et continentale (IMBE), Univ Avignon, Aix Marseille Univ, CNRS, IRD, IUT site Agroparc, Avignon, France
- 3Centre d’Ecologie et des Sciences de la Conservation (CESCO), Muséum national d’Histoire naturelle, Centre National de la Recherche Scientifique, Sorbonne Université, UMR7204 Centre des Sciences de la Conservation (CESCO), Paris, Île-de-France, France
- 4Laboratoire Cogitamus, Paris, Languedoc-Roussillon, France
- 5Université de Montpellier, CNRS, EPHE, IRD, UMR5554 Institut des Sciences de l'Evolution de Montpellier (ISEM), Montpellier, France
- 6Copernicus Institute of Sustainable Development, Utrecht University, Utrecht, Netherlands
Grazing is recognized as a major process driving the composition of plant communities in grasslands, mostly due to the heterogeneous removal of plant species and soil compaction that results in a mosaic of small patches called micro-patterns. To date, no study has investigated the differences in composition and functioning among these micro-patterns in grasslands in relation to grazing and soil environmental variables at the micro-local scale. In this study, we ask (1) To what extent are micro-patterns different from each other in terms of species composition, species richness, vegetation volume, evenness, and functioning? and (2) based on multigroup structural equation modeling, are those differences directly or indirectly driven by grazing and soil characteristics? We focused on three micro-patterns of the Mediterranean dry grassland of the Crau area, a protected area traditionally grazed in the South-East of France. From 70 plant community relevés carried out in three micro-patterns located in four sites with different soil and grazing characteristics, we performed univariate, multivariate analyses and applied structural equation modeling for the first time to this type of data. Our results show evidence of clear differences among micro-pattern patches in terms of species composition, vegetation volume, species richness, evenness, and functioning at the micro-local scale. These differences are maintained not only by direct and indirect effects of grazing but also by several soil variables such as fine granulometry. Biological crusts appeared mostly driven by these soil variables, whereas reference and edge communities are mostly the result of different levels of grazing pressure revealing three distinct functioning specific to each micro-pattern, all of them coexisting at the micro-local scale in the studied Mediterranean dry grassland. This first overview of the multiple effects of grazing and soil characteristics on communities in micro-patterns is discussed within the scope of the conservation of dry grasslands plant diversity.
Introduction
Grasslands have been defined as ecosystems dominated by graminoids (i.e., grasses and grass-like plants) and forbs (Jacobs et al., 1999; Gibson, 2009). They constitute an important ecosystem type comprising about one-third of the Earth’s vegetative cover (Jacobs et al., 1999; Suttie et al., 2005) and covering over 900,000 km2 in Europe (Eurostat, 2017). Typical temperate grasslands are almost continuous ecosystems of grasses, sedges, and herbaceous dicotyledonous species in varying amounts, with scattered bushes and low tree cover (Jacobs et al., 1999). Their characteristics vary with local climate (i.e., water, wind, and light), soil, and topography (i.e., edaphic factors), biotic interactions but also natural and human disturbances such as fires and grazing (Burke et al., 1998; Jacobs et al., 1999; White et al., 2000; Gibson, 2009; Dantas et al., 2016).
Grazing is known to be a major driver of the composition of plant communities in grasslands, especially at the micro-local scale where grazing increases spatial heterogeneity and plant diversity relative to ungrazed habitat (McNaughton, 1983; Milchunas and Lauenroth, 1993; Adler et al., 2001; Ge and Liu, 2022). Grazing leads to the heterogeneous removal of plant species due to differences in plant palatabilities and animal selectivity (Bakker et al., 1983; Ge and Liu, 2022). These can yield a mosaic-like structure in vegetation, called micro-patterns (Bakker et al., 1983), where patches of tall vegetation coexist with shorter patches containing more palatable plants. This direct effect may be reinforced by indirect effects of grazers: for example, because grazers may forage more in patches with shorter plants, such patches can be denser, which further reduces plant development (Chenot et al., 2017). Both such direct and indirect effects combine with possible pre-existing spatial variations in soil characteristics to produce heterogeneity in vegetation composition, structure, and local functioning. Nevertheless, to our knowledge, no study has sought to disentangle so far the double effect of grazing and soil conditions on micro-pattern species composition and diversity (but see Bakker et al., 1983), especially to highlight the specific functioning at work due to grazing pressure and soil characteristics at a micro-local scale.
Yet, understanding these related effects on the species composition, abundance, and functioning of grassland communities remains crucial for the implementation of effective management strategies, restoration and conservation programs for species-rich grasslands. Indeed, some old-growth grasslands are composed of plant communities that require centuries or millennia to assemble (Henry et al., 2010; Poschlod and Baumann, 2010; Robin et al., 2018; Saatkamp et al., 2021) and exhibit an exceptional biodiversity both in terms of high plant species richness and endemism (Veldman et al., 2015). One example is the 26,000 km2 of dry grasslands of the Northwestern Mediterranean Basin (Buisson et al., 2020). These grasslands are typically resilient to endogenous disturbance regimes such as wildfires (Bond and Keeley, 2005; Vidaller et al., 2019) or grazing pressure variability (Saatkamp et al., 2017), but extremely vulnerable to human-caused exogenous perturbations, for example those arising from cultivation (Römermann et al., 2005; Coiffait-Gombault et al., 2012; Helm et al., 2019). They are also currently threatened by an increase in farmland abandonment leading to shrub encroachment, especially in the Mediterranean area where the decrease in livestock is considered a major cause of changes in landscapes (Buisson et al., 2020). Therefore, initiatives to conserve and restore old-growth Mediterranean grasslands are urgently needed based on our understanding of processes at work in maintaining their plant diversity and functions (Veldman et al., 2015).
To shed new light on the processes that maintain the heterogeneity and plant diversity in dry grasslands, we conducted our study at the micro-local scale, focusing on three micro-pattern plant communities of the Crau nature reserve, a protected Mediterranean dry grassland located in the South-East of France. This area is the only French Mediterranean pseudo-steppe, whose origin and sustainability arise from the combination action of climate, specific soil conditions, and the traditional practice of sheep grazing for several millennia (Buisson and Dutoit, 2006). At this micro-local scale, we addressed the following questions: (1) To what extent are the plant communities of the micro-patterns different from each other in terms of species composition, species richness, vegetation volume, evenness, and functioning? and (2) are those differences directly and/or indirectly driven by grazing and/or soil variables? In order to identify the processes maintaining heterogeneity in space, and potentially affecting the temporal dynamics of these micro-patterns, we performed univariate, multivariate analyses and applied structural equation modeling for the first time to this type of data to reveal direct and indirect effects of explanatory variables. We discussed our results within the scope of plant community ecology and applications to the biological conservation of species-rich Mediterranean dry grasslands.
Materials and methods
Study area and sites
Vegetation data were collected in the Crau nature reserve, hereafter called Crau area (Figure 1). This area is characterized by a Mediterranean climate (dry summers, mild winters, with rainfall peaking in autumn, some detailed temperature and precipitation data are available for the 1997–2006 period in Wolff et al., 2015), frequent strong northwesterly winds (Saatkamp et al., 2021) and is dominated by “pseudo-steppic” vegetation (Tatin et al., 2013). Dominant species composition is characteristic of Mediterranean Thero-Brachypodietea habitat “Pseudosteppe with grasses and annuals,” a high priority habitat for conservation (Corine Biotopes Code 34.512, Bissardon et al., 1997; Natura 2000 habitat type 6,220, San Miguel, 2008; EUNIS habitat E1.311 and E1.312, Louvel et al., 2013). This pseudo-steppic vegetation located in the fossil delta of the Durance river is characterized by a high stone cover (mainly pebbles) that limits the installation and the development of dense grasslands. Plant communities are highly diverse and dominated by low or very short herbaceous vegetation (49.4% of all plant species are annuals, Devaux et al., 1983; Helm et al., 2019; Pfeiffer et al., 2019), as a result of the combined effects of climate, soil, fire, and grazing. Grazing by itinerant sheep flocks has been reported since at least 2000 BP on the basis of Roman archeological remains (Badan et al., 1995; Henry et al., 2010; Robin et al., 2018; Saatkamp et al., 2021). This extensive grazing, occurring mostly from February to June, is heterogeneous throughout the steppe in the Crau area (Buisson and Dutoit, 2006; Saatkamp et al., 2017). These different parameters, Mediterranean climate, strong northwesterly winds, high stone cover, and two millennia of extensive grazing, all participate to shape this ecosystem.
We selected four study sites locally named in French “Coucou, Négreiron, Petit Carton, and Le Merle,” along a South-West/North-East gradient following the geomorphological-pedological-climatic gradient to cover all the environmental variability in the area (climate, topography, geology, pedology, and water accessibility) and in grazing pressures (Molinier and Tallon, 1950; Tatin et al., 2013; Table 1; Figure 1). An increase in fine elements (clay, silt) relative to decrease of coarse elements (sand, pebbles) has been shown to drive the presence of the biological crust micro-patterns in the south and their absence in the north of the plain (Rieux et al., 1977). Finally, the four sites are grazed by sheep kept in enclosures or led by a shepherd at an average grazing pressure of 400.8 ± 150 grazed day sheep/ha/year (see below, unpublished data from the Agricultural Chamber of the Bouches-du-Rhône department and the Le Merle Domain). Two out of the four sites had long-term, established ungrazed areas (exclosures): one of approximately 0.2 ha since 1982 at Le Merle and another of approximately 3 ha since 2001 at Négreiron (Bourrelly et al., 1983; Pilard and Brun, 2001).
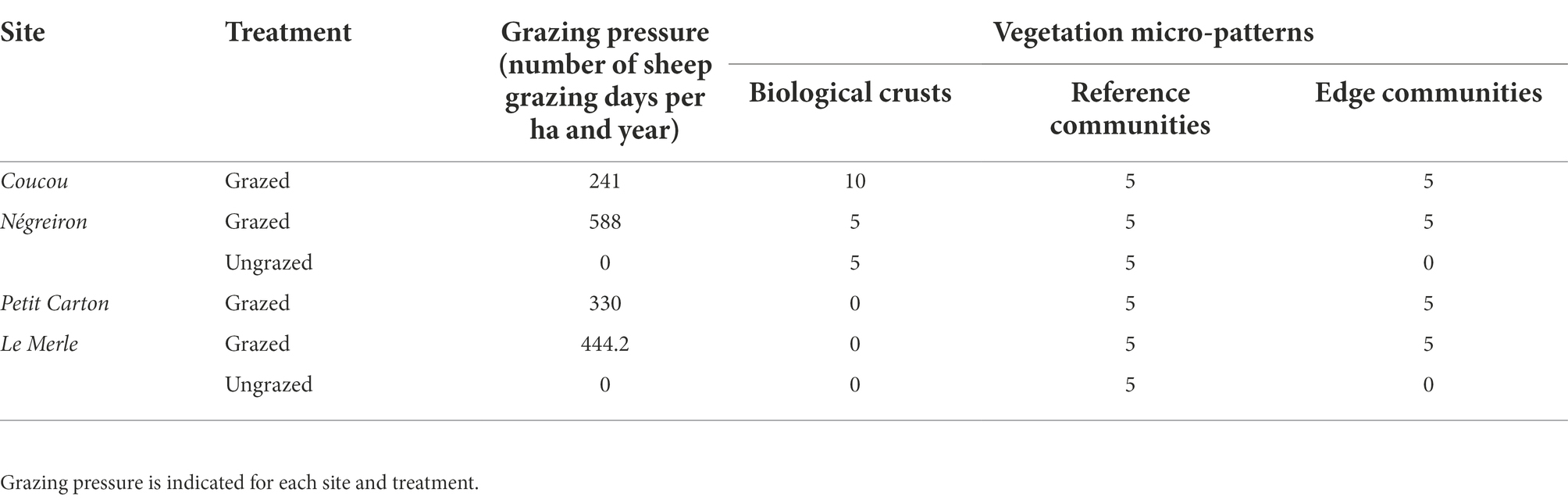
Table 1. Number of plant community relevés carried out for each micro-pattern and site of the Mediterranean dry grassland of the Crau area (South–East France), broken down by treatment (grazed vs. ungrazed in exclosures).
Sampling design and data collection
Within the four sites described above, three micro-patterns in plant communities have been primarily reported: (i) Mediterranean grasslands, locally named “coussoul” or Asphodeletum fistulosi (Thero-Brachypodietalia) following the phytosociological typology by Molinier and Tallon (1950), hereafter named as lightly grazed reference communities, (ii) biological crusts, areas dominated by lichens on shallower soils, locally named “tonsure” or Crassuletum tillaeae (Tuberarietalia guttati) following the phytosociological typology by Molinier and Tallon (1950), corresponding to present or historically high grazing pressure areas (Rieux et al., 1977), and (iii) edge communities dominated by the tussock perennial grass [Brachypodium retusum (Pers.) P.Beauv.] of this grassland, locally named “grossier” and associated to the absence of grazing or very low grazing pressure areas, often localized at the edge of grazing plots (Dureau and Bonnefon, 1998). A total of 70 relevés, i.e., a list of each vascular plant species in a community for a defined spatial area, were carried out in the three Mediterranean vegetation micro-patterns: 20 in biological crusts (5 in exclosures), 30 in reference communities (10 in exclosures) and 20 in edge communities (Table 1). This difference in the number of relevés was due to the absence of long-term exclosures at two sites (i.e., no exclosure in sites Coucou and Petit Carton) or the absence of biological crusts in the north of the plain (i.e., in sites Le Merle and Petit Carton). Each relevé delimitation was defined taking into account the floristic, structural, and ecological homogeneity necessary to set up a phytosociological survey and on previous phytosociological studies already realized in the Crau area (Molinier and Tallon, 1950; Rieux et al., 1977). A minimum distance of 10 m between relevés located in a same site was observed to avoid any influence of each type of plant community on one another, and relevés were done at least 10 m away from fences (Buisson and Dutoit, 2006; Saatkamp et al., 2017). The floristic data were gathered from April to May 2018, which is the period of the year during which most plants can be identified to the species level in South-East France. All vascular plants were recorded in each relevé, and abundance data were estimated for each species according to the six abundance classes defined by Braun-Blanquet (+, 1, 2, 3, 4, 5; Braun-Blanquet, 1932). The sampled areas of the 70 relevés ranged from 4 to 40 m2 (average area of grazed biological crusts 8.5 ± 7.9 m2 vs. ungrazed biological crusts 7.2 ± 2.2 m2; of grazed reference communities 14.4 ± 9.2 m2 vs. ungrazed reference communities 6.6 ± 2.8 m2; of edge communities 13.4 ± 7.8 m2, sampled area of each relevé are detailed in Supplementary Table A1). The variability of the sampled areas arose from differences in the area occupied by the three different micro-patterns and to their species richness, as we stopped samples when 95% of the species of the plant community was incorporated into the relevé, ratio also based on previous phytosociological surveys realized in the Crau area (Molinier and Tallon, 1950; Rieux et al., 1977). To avoid potential bias, the area was included in all subsequent analyses.
For each relevé, we defined five independent indices that captured the main local environmental parameters: (i) an index of vegetation volume, based on visual estimates of vegetation cover, bare ground, and maximum height of the herbaceous stratum; (ii) a soil fertility index, based on 12 parameters related to soil chemistry (cation exchange capacity, pH water, and pH KCI, available phosphorus, potassium oxide, magnesium oxide, calcium oxide, sodium oxide, total nitrogen, organic carbon, and carbon-to-nitrogen ratio); (iii) an amount of fine soil particles, measured as the amount of clay and fine silt; (iv) an amount of coarse soil particles, i.e., fine sand; and (v) an amount of pebbles (>5 cm), based on visual cover estimates. These indices capture known gradients at the scale of the Crau area (Details in Supplementary Material Appendix I; Supplementary Figure A1) that broadly influence vegetation characteristics. All visual estimations have been collected by the same observer to reduce subjective variability. Grazing pressure was retrieved from inquiries of pastoralists realized by the Agricultural Chamber of the Bouches-du-Rhône department and the Merle Domain for the four study sites concerning the 55 grazed relevés out of the 70 relevés (15 were located in exclosures) in 2018. We calculated the number of sheep grazing days per ha and per year as Ns * Nd/ha for the grazed season of the year 2018 (autumn 2017 and spring 2018, before the transhumance to the mountain pastures), where Ns the number of sheep in a herd, Nd the number of days of sheep presence on the grazed area, and ha the area of the site (Table 1). It is important to note that we used two grazing variables. We used first a quantitative variable, described above and named hereafter “grazing pressure.” This variable was mostly used in statistical analyses. Second, we compiled a qualitative variable, grazed vs. ungrazed in exclosures, hereafter named “treatment” and used in some graphical representations where appropriate.
Statistical analyses
We calculated the species richness and Pielou’s measure of species evenness, hereafter named evenness, which is weakly sensitive to rare taxa, not sensitive to median or dominant taxa, and independent of species richness for sites with more than 20 species (Beisel et al., 2003; Gosselin, 2006). Evenness was calculated as J = H′/ln(S) where H′ is the Shannon diversity index based on the abundance of species in a relevé and S is the total number of species in a relevé.
To explore the possible relationships between the three micro-patterns and the considered response variables, i.e., amount of clay and fine silt, amount of fine sand, soil fertility, pebble cover, vegetation volume, species richness, and evenness, we fitted linear mixed-effects models using the restricted maximum likelihood algorithm as recommended in case of unbalanced design associated to random effect (Pinheiro and Bates, 2000; Bolker et al., 2009). We included three fixed-effect predictors: micro-patterns (qualitative, three levels), grazing pressure (quantitative), and the interaction between both variables, and included the site identity as a random effect on the intercept. We included the logarithm of the sampled area as a control, fixed-effect variable for the models that included species richness, evenness, vegetation volume, and pebble cover as response variables. For the model with pebble cover as response, we did not include grazing pressure and the interaction between grazing pressure and micro-pattern as explanatory variable. Equations are detailed in Supplementary Material Appendix II. We adjusted the p-values obtained from the linear mixed-effects models (Kuznetsova et al., 2017) and from the ANOVA applying the Holm correction (Sinclair et al., 2013). We checked visually using quantile-quantile plots that the residuals were consistent with the assumption of a normal distribution. We calculated the pairwise comparison Wilcoxon tests between micro-pattern group levels according to each response variables with corrections for multiple testing (Benjamini and Hochberg, 1995; Benjamini and Yekutieli, 2001) and between grazed and exclosures micro-patterns.
Multivariate analyses were used to study the relationship between the species composition of plant communities, environmental variables, and grazing pressure. In order to analyze plant community data, Braun-Blanquet scores were transformed into percentage cover on the basis of the average percentage of cover of each class: + = 1%; 1 = 5.5%; 2 = 17.5%; 3 = 37.5%; 4 = 62.5%; 5 = 87.5%. We performed Adonis and pairwise Adonis post-hoc tests with Bonferroni adjustments to check whether the plant communities were significantly different between and within groups defined by the micro-pattern, the treatment (grazed vs. exclosure), and the interaction of both variables. Moreover, we implemented a redundancy analysis (RDA) to investigate the variability in plant community composition at the micro-local scale in the Crau area constrained by centered environmental variables and grazing pressure. Note that we considered site and area as conditional variables, to account for the spatial nesting of sampled areas in sites and for the variable area sampled per relevés, respectively. As recommended by Borcard et al. (2011), we Hellinger-transformed the abundance data to reduce the wide disparity in magnitude between species abundances prior to the analysis. We retained all environmental variables (i.e., amount of clay and fine silt, amount of fine sand, soil fertility, and pebble cover) and grazing pressure as explanatory variables according to the Variance Inflation Factor testing collinearity between explanatory variables (all VIF < 5; Borcard et al., 2011). We finally performed a global permutation test to assess the significance of the RDA and a test of the canonical axes significance.
Finally, in order to evaluate and compare direct and indirect effects of grazing versus soil variables on species richness, evenness, and vegetation volume of the three micro-patterns communities, we performed a single multigroup structural equation modeling (SEM), a probabilistic model including multiple predictors and response variables in a single causal network. It provides a means of representing complex hypotheses about causal networks, accounting for factors that are both causes and effects, taking into account interaction effects, and testing for model and data consistency (Grace, 2006; Lefcheck, 2019). We proposed an initial conceptual model containing six regression analyses based on an a priori knowledge about the relations among the variables that was both consistent with our data and which made biological sense (Supplementary Figure A2). We considered species richness, vegetation volume, and evenness as endogenous variables, i.e., responses variables, and amount of clay and fine silt, amount of fine sand, soil fertility, pebble cover, and grazing pressure as exogenous variables, i.e., predictors. Note that an endogenous variable can also predict other variables in the model and be characterized simultaneously as endogenous and exogenous variables. This is the case for vegetation volume, species richness, and amount of clay and fine silt which were both endogenous and exogenous variables. A logarithm term of the area was considered as an exogenous variable influencing species richness, vegetation volume, and evenness to account for the variable area sampled per relevé (Supplementary Figure A2). As expected, this term had a direct significant and positive effect on species richness of biological crusts (Standardized estimate = 0.72, value of p < 0.001), reference communities (Standardized estimate = 0.3, value of p < 0.001), and edge communities (Standardized estimate = 0.6, value of p < 0.001), i.e., the larger the sampled area, the higher the species richness. It has not been represented in figures as this variable is a sampling artifact. In each regression analyses of the multigroup SEM, we included an independent random effect to account for the spatial nesting of sampled areas in site. We performed a single multigroup SEM testing relationships between variables for the three micro-patterns of the Mediterranean dry grassland of the Crau area. This single multigroup SEM allows testing for significant differences in the relationships between variables across and between micro-patterns. According to the test of directed separation, no relation was missing in the conceptual model. To evaluate the model and the goodness-of-fit with the data, we used a Fisher’s C test (high p-values indicate a good fit, with values well above 0.05 considered acceptable since the null hypothesis here tested is that the data predicted by the model and the observed data are consistent; Lefcheck, 2019).
All analyses and figures were done using R version 3.2.4 (R Development Core Team, 2008), and the packages vegan v2.5.6 (Oksanen et al., 2019), nlme v3.1.142 (Pinheiro et al., 2017), piecewiseSEM v2.1.0 (Lefcheck, 2016), ggplot2 v3.3.1 (Wickham, 2009).
Results
Evidence of differences between the three micro-patterns of dry grasslands
We found differences between the three micro-patterns in terms of species composition. All groups constituted by grazed and ungrazed communities of the three micro-patterns of the Crau area were different from each other in terms of species composition (Pairwise Adonis test, adjusted value of p < 0.01; Supplementary Tables A2, A3) except grazed reference communities and grazed edge communities (Pairwise Adonis test, F value = 2.21, adjusted value of p = 0.41; Supplementary Tables A2, A3). We found a significant effect of the three micro-patterns (Adonis test, F value = 11.33, value of p < 0.001), of the treatment (Adonis test, F-value = 6.82, value of p < 0.001) and of the interaction of the both variables micro-patterns and the treatment (Adonis test, F-value = 0.38, value of p = 0.015) on the species composition of all groups.
We found differences between the three micro-patterns in terms of soil characteristics, vegetation volume, and species composition. Considering the RDA analysis, permutation tests supported the significance of the RDA (F-value = 4.03, value of p < 0.001; Supplementary Table A4): variance in plant community composition was explained to 20.55% by the explanatory variables and to 29.35% by the conditional variables (RDA adjusted R2 = 16.4; Supplementary Table A4). In addition, the first and second canonical axes were significant (Permutation test, RDA 1 F-value = 12.16, value of p < 0.001; RDA 2 F-value = 7, value of p <0.001; Supplementary Table A4) and accounted together for 79.17% of the total explained variance. The first RDA axis captured differences in composition of micro-patterns and vegetation volume: it contrasted biological crusts, which had higher level of fine sand and soil fertility, whereas reference communities and edge communities had higher levels of clay, fine silt, and vegetation volume (Figure 2). The second RDA axis separated grazed plant communities from ungrazed plant communities, i.e., exclosures.
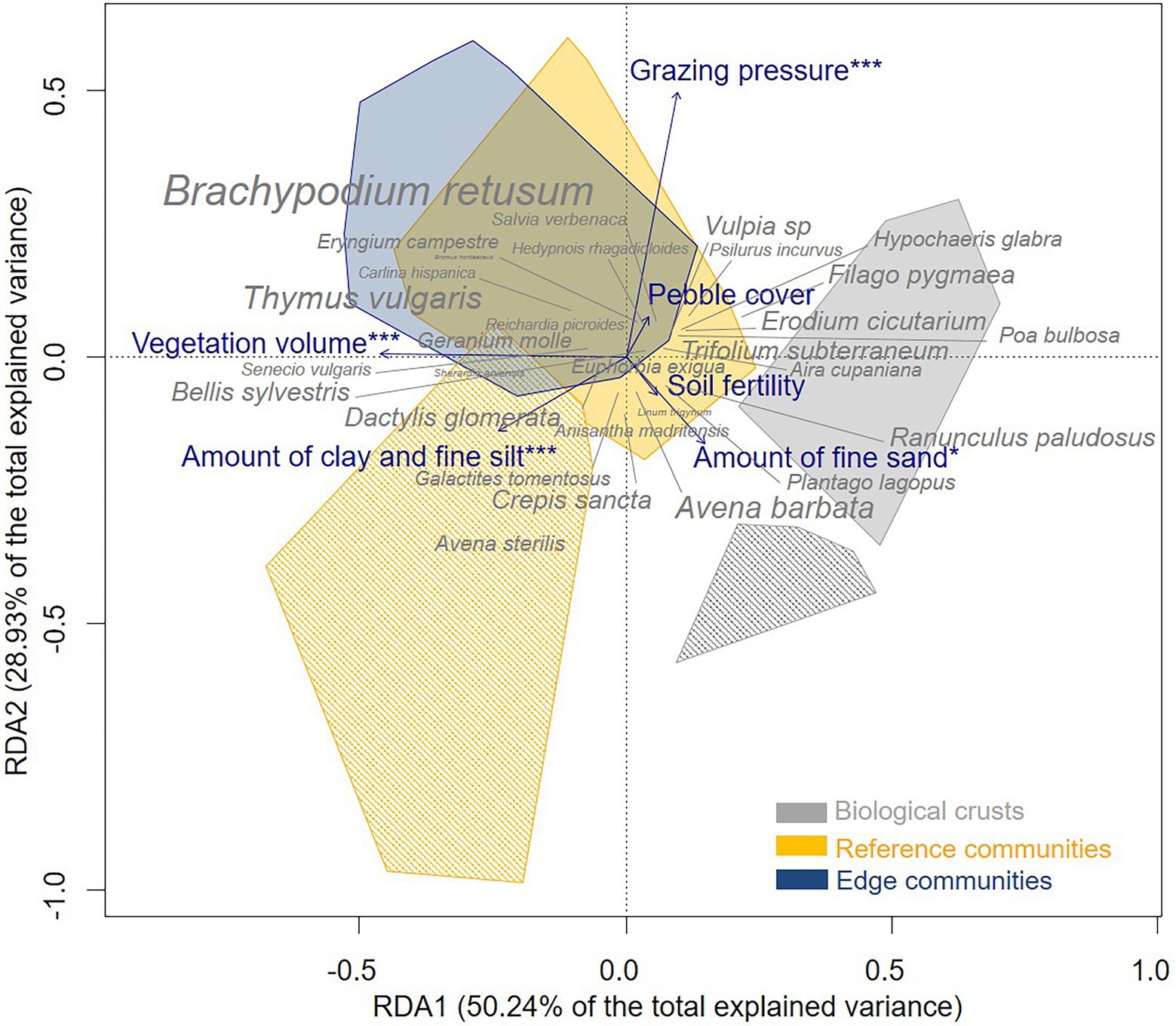
Figure 2. Ordination triplot of redundancy analysis (RDA) of the three micro-patterns of the Mediterranean dry grassland of the Crau area (full polygons for grazed relevés, hatched polygons for ungrazed relevés in exclosures) considering grazing and environmental variables (blue, stars precise significance) and two conditional variables (site and sampled area). Only the 30 most abundant species are represented and the size of species’ labels is proportional to their frequency.
We found evidence of differences between the three micro-patterns in terms of species richness, evenness, vegetation volume, and soil characteristics. Linear mixed models showed significantly higher levels of fine sand for biological crusts compared to the other micro-patterns, significantly higher levels of clay and fine silt, of vegetation volume for reference communities and edge communities compared to biological crusts (Table 2; Figure 3; Supplementary Tables A5, A6). Thus, biological crusts were characterized by coarse silt and fine sand, whereas reference communities and edge communities were characterized by a mixture of variables element’ size: clay and fine silt and coarse sand. Edge communities were characterized by the lower amount of fine sand in soil than for other micro-patterns (i.e., the higher amount of coarse sand (Figure 3). Finally, linear mixed models did not show significant differences among micro-patterns concerning soil fertility and pebble cover. We found significantly higher levels of clay and fine silt for ungrazed communities compared to grazed communities, higher levels of soil fertility for grazed biological crusts, lower levels of species richness, and evenness for ungrazed reference communities (Supplementary Figure A3).
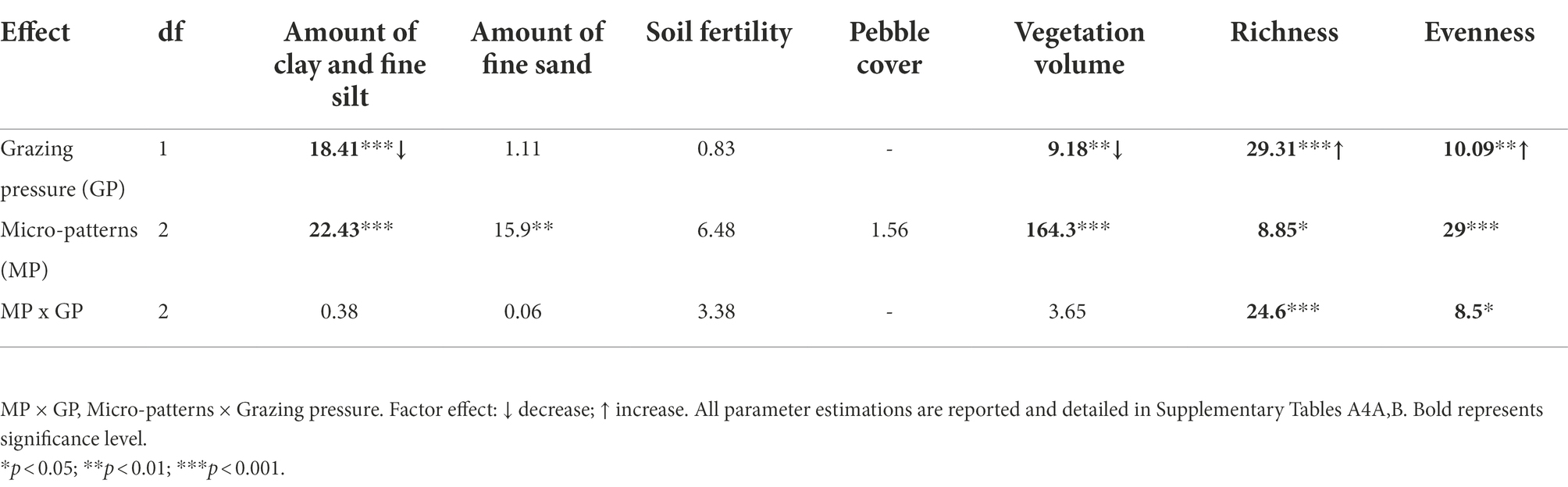
Table 2. ANOVA Type II (Chi-square tests) and significance level for effects of grazing pressure and micro-patterns against environmental variables, namely amount of clay and fine silt, amount of fine sand, soil fertility, pebble cover, and vegetation volume, species richness, and evenness.
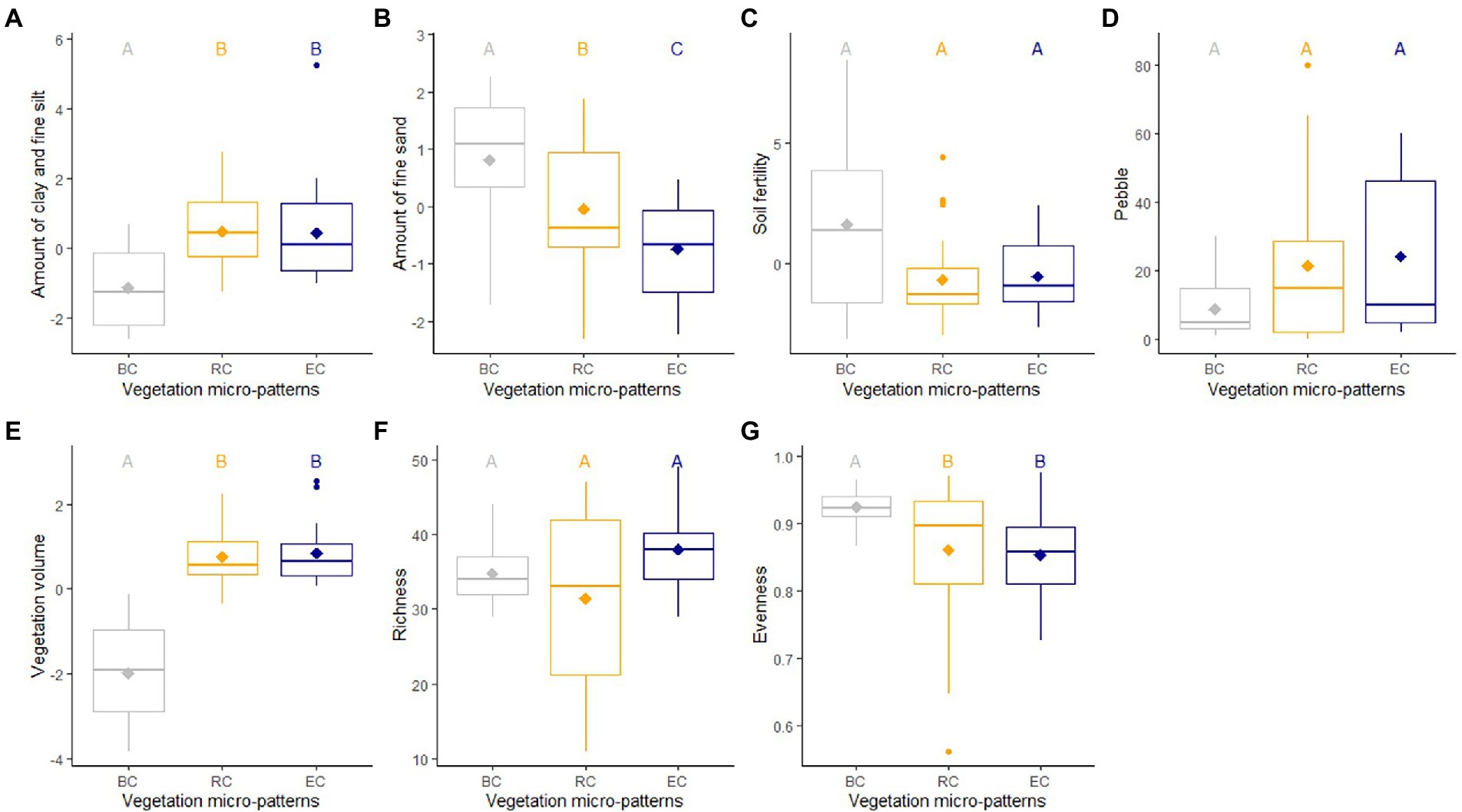
Figure 3. Comparison of levels of environmental variables, namely amount of clay and fine silt (A), amount of fine sand (B), soil fertility (C), pebble cover (D); vegetation volume (E), species richness (F), and evenness (G) according to the three micro-patterns (BC, biological crusts; RC, reference communities; EC, edge communities). Average (diamond), medians, quartiles, minimum and maximum are colored according to each micro-patterns. Significant differences between averages of each group are indicated with different letters, calculated with pairwise Wilcoxon tests.
Micro-patterns exhibit distinct functioning, all of them coexisting at the micro-local scale in dry grasslands
We investigated the direct and indirect effects of grazing pressure and soil variables on species richness, vegetation volume, and evenness of plant communities of the three micro-patterns and the fitting parameters of the adequate multigroup SEM were correct (Fisher’s C = 9.75, value of p = 0.78, ddl = 14, n = 70; Figure 4). This analysis showed that the direct effect of grazing varied across micro-patterns (significant interaction between grazing pressure and micro-patterns against species richness, value of p < 0.05), whereas effects of other predictors were similar across micro-patterns (value of p > 0.05; Figure 4). Effect sizes revealed three distinct functioning, each one specific of a micro-pattern. Soil variables and grazing had stronger direct and indirect effects on plant communities of biological crusts than on reference communities and edge communities. Direct effects of grazing on species richness differed among each micro-pattern, being negative in biological crusts and positive in reference and edge communities. Finally, soil fertility and pebble cover did not appear to influence plant communities of any micro-patterns according to the multigroup SEM.
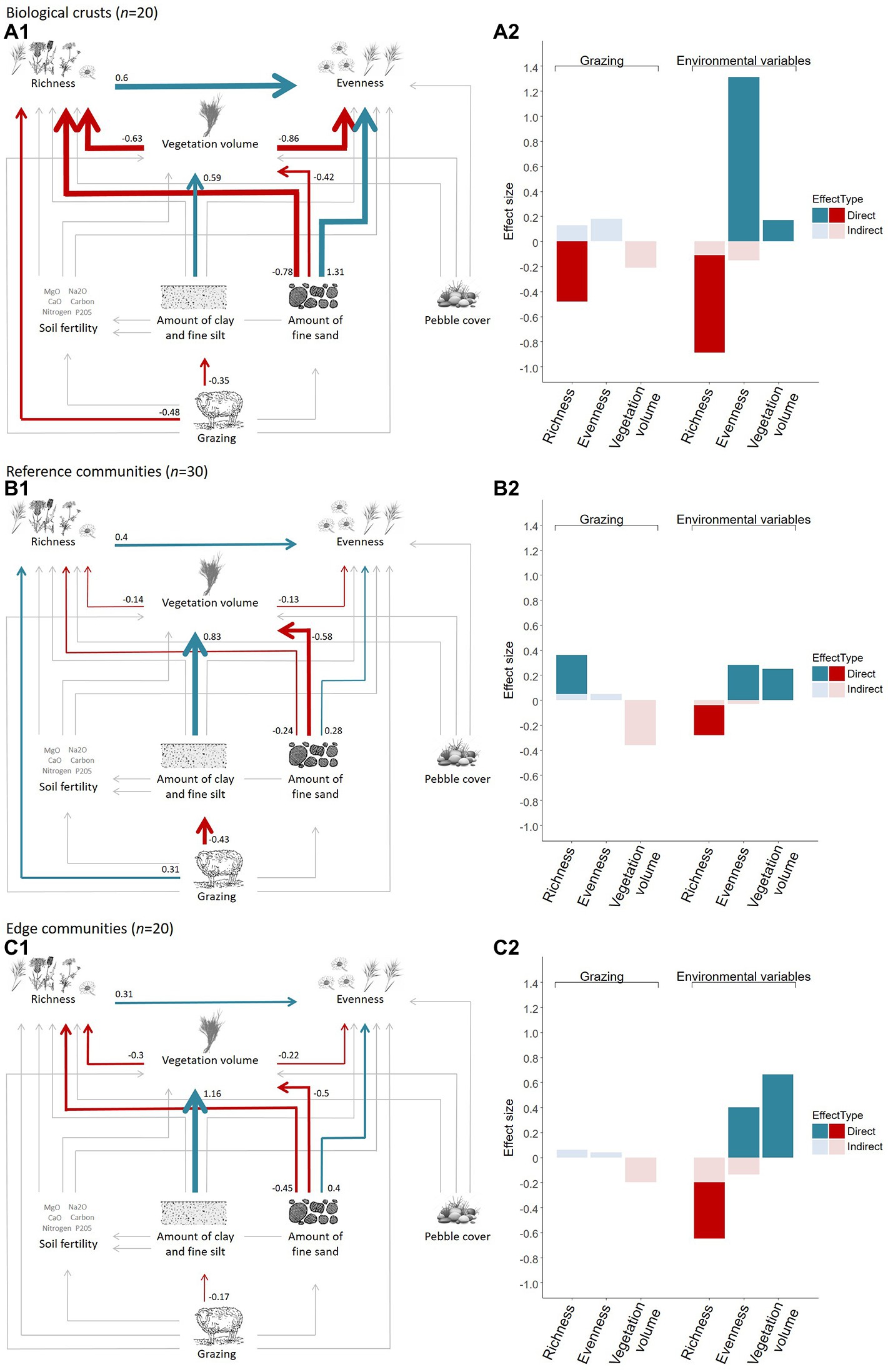
Figure 4. Direct and indirect effects of grazing and soil variables on vegetation richness, evenness and volume according to the three micro-patterns of the Mediterranean dry grassland of the Crau area (relations among variables on the left and total direct and indirect effect size on the right resulting from a piecewise multigroup structural equation model, coefficients correspond to standardized estimates). Red (negative effect) and blue (positive effect) arrow widths are proportional to the standardized estimate of each significant effect (numbers along arrows). Gray arrows are non-significant effects.
Discussion
For the first time, our work disentangles grazing and soil characteristics impacts on micro-pattern patches by showing differences in species composition, vegetation volume, species richness, evenness, and functioning at the micro-local scale among the micro-patterns of a Mediterranean grassland.
Origin and maintenance of micro-patterns
This work highlights the contrasted functioning across micro-patterns, as grazing had a variable impact on species richness and soil granulometry. Micro-patterns with higher vegetation cover and volume are those that tend to be less grazed (although this has not been quantified here)—biological crusts are thus likely to be where grazing is the highest, followed by the reference communities and edge communities. Grazing exhibited a negative effect on species richness in biological crusts versus a positive one on richness in reference communities and an indirect and smaller positive one on richness of edge communities. This is consistent with the theoretical impact of grazing on species richness—intense grazing decreases diversity (in biological crusts) but lower levels favor diversity, which is what we observe in other micro-patterns (Milchunas et al., 1988; Tatin et al., 2013).
Differences among micro-patterns were particularly stark between biological crusts and the two other micro-patterns, as the former showed very different soil granulometry and vegetation volume. Biological crusts appeared primarily driven by soil variables, and second by grazing. They were more homogeneous in terms of species composition, and had an upper soil containing more fine particles (fine sand, coarse silt) than the other two micro-patterns. Such differences in soil characteristics suggest that biological crust communities may have their origin in present or former grazing practices (Saatkamp et al., 2021). Indeed, soil characteristics are influenced by grazing, suggesting that trampling could be the main driver of local soil differences, which compound with already-existing larger-scale differences, such as the combined geomorphological-pedological-climatic gradient of the Crau area and the spatial heterogeneity of the vegetation (Molinier and Tallon, 1950; Adler et al., 2001; Tatin et al., 2013). Soil trampling and compaction by animals alter the soil structure by compressive deformation: particles are brought closer together by the applied charge, which reduces the total pore space and permanently expels air or water from the soil pores (Patto et al., 1978; Silva et al., 2003). These alterations of the upper part of the soil affect soil physical properties, density, water dynamics, mineral element cycle, and plant growth (Lowery and Schuler, 1991; Schlotzhauer and Price, 1999; De Neve and Hofman, 2000; Drewry et al., 2008). Biological crusts could correspond to past high-impact areas of grazing, which are known to have a long-lasting effect, and to finely textured soils, which are more compressible than others (Larson et al., 1980), especially under stronger grazing pressures. Indeed, the higher amount of fine sand in soil composition could promote biological crusts by compaction, which may impair the installation of the dominant and key species in the Crau area Brachypodium retusum. In contrast, the heterogeneity of soil particle sizes in reference and edge communities seems to promote Brachypodium retusum establishment, as observed in field. Also, the increased abundance of this perennial graminoid species has been related to lightly grazed edge communities (Porensky et al., 2017). The installation of this key species facilitated by soil grain size characteristics and fire resilience could then result in changes in the microenvironment by improving water infiltration, reducing radiation to the soil, and accumulating seeds, fine soil particles, and nutrients between plant roots (Aguiar and Sala, 1999; Vidaller et al., 2019; Davies et al., 2022). This could also reduce herbivory through a phenomenon of facilitation between species (survival of seedlings protected by the dominant species, Aguiar and Sala, 1999). Taken altogether, these processes could explain the persistence of such contrasted community types at a local scale (<10 m).
Reference and edge communities appeared much more similar to each other than to biological crusts, as they had similar species compositions and abundances, species richness, evenness, and vegetation volume, all consequences of variable levels of herbivory (Génin et al., 2021). However, they differ in terms of soil composition (amount of fine sand). Edge communities appeared directly driven by soil variables and in a minor way by grazing, thus contrasting with reference communities which were directly influenced by grazing. Edge communities are often associated with abiotic modifications such as water infiltration (Masson et al., 2015) and not only to low grazing pressure, which does not affect plant community composition unlike high grazing pressure. One notable implication in terms of conservation and restoration is that it is not enough to remove grazing to produce a transition from reference to edge community in the short term because changes must also occur in soil granulometry (i.e., high amount of coarse sands in the soils of edge community). In fact, only long-term grazing removal affects will change the species composition of reference communities into that of edge communities (Saatkamp et al., 2017). Despite being more similar, switching from one reference to edge community (or the other way around) may thus require more than short-term changes in grazing pressure.
No demonstrated effect of pebble cover and soil fertility on micro-patterns
No effect of pebble cover has been found, although it is widely thought to impact positively species richness, vegetation volume, and evenness, by constituting a root shelter allowing the installation of Brachypodium retusum (Bourrelly et al., 1983) and other species, improving microenvironmental conditions and reducing herbivory by physically protecting the species from sheep’s mouths (Dureau and Bonnefon, 1998; Aguiar and Sala, 1999). This is probably due to the differences in sampled area among micro-pattern (more pebbles in the less grazed areas are also the largest sampled areas), which is not independent from the variable size and density of pebble related to the gradient of the alluvial deposits of the Durance river (Devaux et al., 1983), the largest pebbles at the highest recovery being encountered in the north-west where we did not sample biological crusts (Le Merle site; Figure 1), while the fine elements in the superficial part of the soil are more present in the south of the Crau area (Coucou site; Figure 1). Likewise, no effect of soil fertility has been found and no difference among each micro-pattern even though the former over-grazed areas could be suspected to be characterized by accumulation of nutrients and animal waste, beneficial to plant growth, long-term fertility, and known to cause a shift in community diversity and composition (Matches, 1992; Liu et al., 2016). These persistent legacies of historical sheep concentration, as Roman ones, on soil properties have persistent effects on today’s vegetation in this area (Saatkamp et al., 2021).
Consequences for biological conservation and restoration of mediterranean dry grasslands
This work showed that ecosystem functioning varies over very small spatial scales: the three distinct communities at the micro-local scale are maintained by different direct and indirect effects of grazing and soil characteristics. Thus, there is no one-size-fits-all strategy to influencing the trajectory of the plant communities and one of the main levers of restoration strategies, changing grazing pressure, may have variable effects depending on the community type it is applied to. Grazing has a long-term residual effect on plant communities and soils, so it is not sufficient to remove it in the short term to restore a particular Mediterranean dry grassland community (Buisson et al., 2020). Indeed, grazing-induced changes in soil characteristics and fertility via long-term persistent eutrophication (soil phosphorus, calcium, and nitrogen) and trampling have effects on historical time-scales, as there is little opportunity to uncompact soil structure (Greenwood and McKenzie, 2001; Saatkamp et al., 2021). Grazing thus acts as a one-way lever: given the relative similarity between reference and edge community, it is likely that increased grazing pressure in edge communities would make them shift toward reference communities. However, the sole removal of grazing in reference communities is unlikely to drive them into edge-type communities on short time-scales, because of the need to reverse soil characteristics too. A similar and more pronounced effect is also likely in biological crusts: driving them toward other community types would require undoing the high soil compaction, which only occurs on very long time-scales (centuries) without drastic human intervention. Indeed, effect of past sheepfolds and intense grazing are still detectable on vegetation and soils, even after two millennia (Saatkamp et al., 2021).
In this situation, there is little opportunity to reverse such effects for restoration purposes. However, authors have already shown that a species of harvesting ant, Messor barbarus, could play an important role in the restoration of plant communities in the Crau area, by displacing a large quantity of plant seeds and changing physical and chemical soil characteristics toward that of the reference communities (Cramer et al., 2008; García-Ruiz and Lana-Renault, 2011; De Almeida et al., 2020). Typical dry grassland species are known to have low capacity to colonize new habitats or persist in the soil seed bank (Buisson et al., 2006; Metzner et al., 2017). Thus, increasing pebble cover and re-introducing Messor barbarus may provide an interesting and low-cost tool to reverse compaction and disperse seeds (Bulot et al., 2014; De Almeida et al., 2020).
In this work, we presented the first overview of the multiple effects of grazing and soil characteristics on plant communities of micro-patterns at the micro-local scale. Our results should enable conservation planners to use grazing and/or ecological engineer species as effective tools to influence plant community composition and soil structure. A more complete picture may emerge by measuring additional processes influencing the distribution of micro-patterns such as water infiltration capacity in case of compacted soil, wind-based redistribution of seeds, and fine soil elements that may accumulate in tall vegetation patches, and fire (Burke et al., 1998; Aguiar and Sala, 1999; Vidaller et al., 2019). Evaluating these additional effects would bring an understanding of the governing forces that shape community assembly in the context of Mediterranean dry grasslands conservation and restoration (Török et al., 2018).
Data availability statement
The original contributions presented in the study are included in the article/Supplementary material, further inquiries can be directed to the corresponding author. Full plant and soil data areavailable in the open repository Zenodo: https://doi.org/10.5281/zenodo.7222092.
Author contributions
AC, TD, and GM conceived the research idea. AC, HR, and TD collected data. GM performed statistical analyses. GM, with contributions from TD and AG wrote the manuscript. All authors contributed to the article and approved the submitted version.
Funding
This work was supported by grants from the French Ministry of Ecological and Solidarity Transition, i.e., Ministère de la Transition Écologique et Solidaire, within the framework of the national research project (CARHAB – project no. 2101536326, Approche multi-scalaire de l’organisation des parcours substeppiques des Thero-Brachypodietea dans le sud-est de la France: apport à la compréhension des liens entre géocurtaséries et impacts anthropiques actuels et/ou passés. Received by TD). AG has received funding from the European Union’s Horizon 2020 research and innovation programme under the Marie Sklodowska-Curie grant agreement no. 896159.
Acknowledgments
We thank Frédéric Bioret for his help initiating this project and Tania de Almeida, Elise Buisson, Julie Chenot, Aure Durbecq, Cannelle Moinardeau, and Daniel Pavon for their help with collecting floristic and soil data; Gaelle Besche and Fanny Sauguet for their help with obtaining grazing data; Christel Vidaller, Armin Bischoff, Olivier Blight, François Duchenne, and Mathieu Santonja for their constructive comments and advice during the data analyses; and the Federal State Nature Conservation Authority (CEN-PACA), the Crau nature reserve, and the Le Merle Domain and training center for shepherds for their permission to set up the experiments. We also thank two reviewers and one editor for their comments on a previous version of this manuscript.
Conflict of interest
The authors declare that the research was conducted in the absence of any commercial or financial relationships that could be construed as a potential conflict of interest.
Publisher’s note
All claims expressed in this article are solely those of the authors and do not necessarily represent those of their affiliated organizations, or those of the publisher, the editors and the reviewers. Any product that may be evaluated in this article, or claim that may be made by its manufacturer, is not guaranteed or endorsed by the publisher.
Supplementary material
The Supplementary material for this article can be found online at: https://www.frontiersin.org/articles/10.3389/fevo.2022.879060/full#supplementary-material
References
Adler, P., Raff, D., and Lauenroth, W. (2001). The effect of grazing on the spatial heterogeneity of vegetation. Oecologia 128, 465–479. doi: 10.1007/s004420100737
Aguiar, M. R., and Sala, O. E. (1999). Patch structure, dynamics and implications for the functioning of arid ecosystems. Trends Ecol. Evol. 14, 273–277. doi: 10.1016/S0169-5347(99)01612-2
Badan, O., Brun, J.-P., and Congès, G. (1995). Les bergeries romaines de La Crau d’Arles: Les origines de la transhumance en Provence. Gallia 52, 263–310. doi: 10.3406/galia.1995.3152
Bakker, J. P., de Leeuw, J., and van Wieren, S. E. (1983). Micro-patterns in grassland vegetation created and sustained by sheep-grazing. Vegetatio 55, 153–161. doi: 10.1007/BF00045017
Beisel, J.-N., Usseglio-Polatera, P., Bachmann, V., and Moreteau, J.-C. (2003). A comparative analysis of evenness index sensitivity. Internat. Rev. Hydrobiol. 88, 3–15. doi: 10.1002/iroh.200390004
Benjamini, Y., and Hochberg, Y. (1995). Controlling the false discovery rate: a practical and powerful approach to multiple testing. J. R. Stat. Soc Series B 57, 289–300. doi: 10.1111/j.2517-6161.1995.tb02031.x
Benjamini, Y., and Yekutieli, D. (2001). The control of the false discovery rate in multiple testing under dependency. Ann. Stat. 29, 1165–1188. doi: 10.1214/aos/1013699998
Bissardon, M., Guibal, L., and Rameau, J.-C. (1997). CORINE biotopes. Types d’habitats français Paris, France: ENGREF, MNHN.
Bolker, B. M., Brooks, M. E., Clark, C. J., Geange, S. W., Poulsen, J. R., Stevens, M. H. H., et al. (2009). Generalized linear mixed models: a practical guide for ecology and evolution. Trends Ecol. Evol. 24, 127–135. doi: 10.1016/j.tree.2008.10.008
Bond, W., and Keeley, J. (2005). Fire as a global ‘herbivore’: the ecology and evolution of flammable ecosystems. Trends Ecol. Evol. 20, 387–394. doi: 10.1016/j.tree.2005.04.025
Borcard, D., Gillet, F., and Legendre, P. (2011). Numerical ecology with R. New York Dordrecht London Heidelberg: Springer.
Bourrelly, M., Borel, L., Devaux, J., Louis-Palluel, J., and Archiloque, A. (1983). Dynamique annuelle et production primaire nette de l’écosystème steppique de Crau (Bouches du Rhône). Biologie et écologie méditerranéenne 10, 55–82.
Braun-Blanquet, J. (1932). Plant Sociology. The Study of Plant Communities. United States of America McGraw-Hill Book Co., Inc., New York and London, pp.xviii + 439.
Buisson, E., De Almeida, T., Durbecq, A., Arruda, A. J., Vidaller, C., Alignan, J., et al. (2020). Key issues in northwestern Mediterranean dry grassland restoration. Restor. Ecol. 29:rec.13258. doi: 10.1111/rec.13258
Buisson, E., and Dutoit, T. (2006). Creation of the natural reserve of La Crau: implications for the creation and management of protected areas. J. Environ. Manage. 80, 318–326. doi: 10.1016/j.jenvman.2005.09.013
Buisson, E., Dutoit, T., Torre, F., Römermann, C., and Poschlod, P. (2006). The implications of seed rain and seed bank patterns for plant succession at the edges of abandoned fields in Mediterranean landscapes. Agric. Ecosyst. Environ. 115, 6–14. doi: 10.1016/j.agee.2005.12.003
Bulot, A., Provost, E., and Dutoit, T. (2014). A comparison of different soil transfer strategies for restoring a Mediterranean steppe after a pipeline leak (La Crau plain, south-eastern France). Ecol. Eng. 71, 690–702. doi: 10.1016/j.ecoleng.2014.07.060
Burke, I. C., Lauenroth, W. K., Vinton, M. A., Hook, P. B., Kelly, R. H., Epstein, H. E., et al. (1998). “Plant-soil interactions in temperate grasslands,” in Plant-induced soil changes: Processes and feedbacks, ed. N. BreemenVan (Dordrecht: Springer Netherlands), 121–143.
Chenot, J., Gaget, E., Moinardeau, C., Jaunatre, R., Buisson, E., and Dutoit, T. (2017). Substrate composition and depth affect soil moisture behavior and plant-soil relationship on Mediterranean extensive green roofs. Water 9:817. doi: 10.3390/w9110817
Coiffait-Gombault, C., Buisson, E., and Dutoit, T. (2012). Are old Mediterranean grasslands resilient to human disturbances? Acta Oecol. 43, 86–94. doi: 10.1016/j.actao.2012.04.011
Cramer, V., Hobbs, R., and Standish, R. (2008). What’s new about old fields? Land abandonment and ecosystem assembly. Trends Ecol. Evol. 23, 104–112. doi: 10.1016/j.tree.2007.10.005
Dantas, V. L., Hirota, M., Oliveira, R. S., and Pausas, J. G. (2016). Disturbance maintains alternative biome states. Ecol. Lett. 19, 12–19. doi: 10.1111/ele.12537
Davies, K. W., Copeland, S. M., and Bates, J. D. (2022). Grazing effects on shrub-induced resource islands and herbaceous vegetation heterogeneity in sagebrush-steppe communities. Glob. Ecol. Conserv. 35:e02106. doi: 10.1016/j.gecco.2022.e02106
De Almeida, T., Blight, O., Mesléard, F., Bulot, A., Provost, E., and Dutoit, T. (2020). Harvester ants as ecological engineers for Mediterranean grassland restoration: impacts on soil and vegetation. Biol. Conserv. 245:108547. doi: 10.1016/j.biocon.2020.108547
De Neve, S., and Hofman, G. (2000). Influence of soil compaction on carbon and nitrogen mineralization of soil organic matter and crop residues. Biol. Fertil. Soils 30, 544–549. doi: 10.1007/s003740050034
Devaux, J., Archiloque, A., Borel, L., Bourrelly, M., and Louis-Alluel, J. (1983). Notice de la carte phytoécologique de la Crau (Bouches-du-Rhône). Biologie Ecologie Méditerranéenne, 10, 5–54.
Drewry, J. J., Cameron, K. C., and Buchan, G. D. (2008). Pasture yield and soil physical property responses to soil compaction from treading and grazing—a review. Soil Res. 46:237. doi: 10.1071/SR07125
Dureau, R., and Bonnefon, O. (1998). “Etude des pratiques de gestion pastorale des coussouls” in Patrimoine naturel et pratiques pastorales en Crau, CEN-PACA, CA 13, Saint-Martin de Crau, France. 61–89.
Eurostat (2017). Land cover and land use, landscape (LUCAS). Available at: http://ec.europa.eu/eurostat/web/lucas/data/database.
García-Ruiz, J. M., and Lana-Renault, N. (2011). Hydrological and erosive consequences of farmland abandonment in Europe, with special reference to the Mediterranean region – a review. Agric. Ecosyst. Environ. 140, 317–338. doi: 10.1016/j.agee.2011.01.003
Ge, Z., and Liu, Q. (2022). Foraging behaviours lead to spatiotemporal self-similar dynamics in grazing ecosystems. Ecol. Lett. 25, 378–390. doi: 10.1111/ele.13928
Génin, A., Dutoit, T., Danet, A., le Priol, A., and Kéfi, S. (2021). Grazing and the vanishing complexity of plant association networks in grasslands. Oikos 130, 541–552. doi: 10.1111/oik.07850
Gosselin, F. (2006). An assessment of the dependence of evenness indices on species richness. J. Theor. Biol. 242, 591–597. doi: 10.1016/j.jtbi.2006.04.017
Grace, J. B. (2006). Structural equation modeling and natural systems. Cambridge University Press. UK.
Greenwood, K. L., and McKenzie, B. M. (2001). Grazing effects on soil physical properties and the consequences for pastures: a review. Aust. J. Exp. Agric. 41, 1231–1250. doi: 10.1071/ea00102
Helm, J., Dutoit, T., Saatkamp, A., Bucher, S. F., Leiterer, M., and Römermann, C. (2019). Recovery of Mediterranean steppe vegetation after cultivation: legacy effects on plant composition, soil properties and functional traits. Appl. Veg. Sci. 22, 71–84. doi: 10.1111/avsc.12415
Henry, F., Talon, B., and Dutoit, T. (2010). The age and history of the French Mediterranean steppe revisited by soil wood charcoal analysis. Holocene 20, 25–34. doi: 10.1177/0959683609348841
Jacobs, B. F., Kingston, J. D., and Jacobs, L. L. (1999). The origin of grass-dominated ecosystems. Ann. Mo. Bot. Gard. 86:590. doi: 10.2307/2666186
Kuznetsova, A., Brockhoff, P. B., and Christensen, R. H. B. (2017). lmerTest package: tests in linear mixed effects models. J. Stat. Softw. 82, 1–26. doi: 10.18637/jss.v082.i13
Larson, W. E., Gupta, S. C., and Useche, R. A. (1980). Compression of agricultural soils from eight soil orders. Soil Sci. Soc. Am. J. 44, 450–457. doi: 10.2136/sssaj1980.03615995004400030002x
Lefcheck, J. (2016). Piecewise SEM: piecewise structural equation modelling in r for ecology, evolution, and systematics. Methods Ecol. Evol. 7, 573–579. doi: 10.1111/2041-210X.12512
Lefcheck, J. (2019). Structural equation modeling in R for ecology and evolution. Available at: https://jslefche.github.io/sem_book/index.html
Liu, C., Song, X., Wang, L., Wang, D., Zhou, X., Liu, J., et al. (2016). Effects of grazing on soil nitrogen spatial heterogeneity depend on herbivore assemblage and pre-grazing plant diversity. J. Appl. Ecol. 53, 242–250. doi: 10.1111/1365-2664.12537
Louvel, J., Gaudillat, V., and Poncet, L. (2013). EUNIS, European Nature Information System, Système d’information européen sur la nature. Classification des habitats. Traduction française. Habitats terrestres et d’eau douce Paris, France: MNHN-DIREV-SPN, MEDDE.
Lowery, B., and Schuler, R. T. (1991). Temporal effects of subsoil compaction on soil strength and plant growth. Soil Sci. Soc. Am. J. 55, 216–223. doi: 10.2136/sssaj1991.03615995005500010037x
Masson, S., Gauvain, M., Mesléard, F., and Dutoit, T. (2015). Impacts of water stress removal and disturbance regimes on Mediterranean dry grasslands diversity and succession. Plant Ecol. 216, 1351–1369. doi: 10.1007/s11258-015-0513-5
Matches, A. G. (1992). Plant response to grazing: a review. J. Prod. Agric. 5, 1–7. doi: 10.2134/jpa1992.0001
McNaughton, S. J. (1983). Serengeti grassland ecology: the role of composite environmental factors and contingency in community organization. Ecol. Monogr. 53, 291–320. doi: 10.2307/1942533
Metzner, K., Gachet, S., Rocarpin, P., and Saatkamp, A. (2017). Seed bank, seed size and dispersal in moisture gradients of temporary pools in southern France. Basic Appl. Ecol. 21, 13–22. doi: 10.1016/j.baae.2017.06.003
Milchunas, D. G., and Lauenroth, W. K. (1993). Quantitative effects of grazing on vegetation and soils over a global range of environments: ecological archives M063-001. Ecol. Monogr. 63, 327–366. doi: 10.2307/2937150
Milchunas, D. G., Sala, O. E., and Lauenroth, W. K. (1988). A generalized model of the effects of grazing by large herbivores on grassland community structure. Am. Nat. 132, 87–106. doi: 10.1086/284839
Molinier, R., and Tallon, G. (1950). La végétation de la Crau (Basse-Provence). Revue Générale de Botanique, 1, 1–111.
Oksanen, J., Blanchet, G., Friendly, M., Kindt, R., Legendre, P., Mcglinn, D., et al. (2019). Vegan: community ecology package. Available at: https://CRAN.R-project.org/package=vegan
Patto, P. M., Clement, C. R., and Forbes, T. J. (1978). Grassland Poaching in England and Wales. Grassland Research Institute. Maidenhead.
Pfeiffer, M., Langan, L., Linstädter, A., Martens, C., Gaillard, C., Ruppert, J. C., et al. (2019). Grazing and aridity reduce perennial grass abundance in semi-arid rangelands – insights from a trait-based dynamic vegetation model. Ecol. Model. 395, 11–22. doi: 10.1016/j.ecolmodel.2018.12.013
Pilard, P., and Brun, L. (2001). Guide de gestion des milieux en faveur du Faucon crécerellette de la plaine de Crau Saint-Martin de Crau, Unpublished report.
Pinheiro, J. C., Bates, D. M., Deb Roy, S., and Sarkar, D., R Development Core Team (2017). nlme: Linear and Nonlinear Mixed Effects Models. Available at: https://CRAN.R-project.org/package=nlme.
Porensky, L. M., Derner, J. D., Augustine, D. J., and Milchunas, D. G. (2017). Plant community composition after 75 Yr of sustained grazing intensity treatments in shortgrass steppe. Rangel. Ecol. Manage. 70, 456–464. doi: 10.1016/j.rama.2016.12.001
Poschlod, P., and Baumann, A. (2010). The historical dynamics of calcareous grasslands in the central and southern Franconian Jurassic mountains: a comparative pedoanthracological and pollen analytical study. Holocene 20, 13–23. doi: 10.1177/0959683609348843
R Development Core Team. (2008). R: A language and environment for statistical computing. Available at: http://www.R-project.org
Rieux, R., Ritschel, G., and Roux, C. (1977). Etude écologique et phytosociologique de Crassuletum tillaeae Molinier et Tallon 1949. Revue de biologie et d’écologie méditerranéenne 6, 117–143.
Robin, V., Nelle, O., Talon, B., Poschlod, P., Schwartz, D., Bal, M.-C., et al. (2018). A comparative review of soil charcoal data: spatiotemporal patterns of origin and long-term dynamics of Western European nutrient-poor grasslands. Holocene 28, 1313–1324. doi: 10.1177/0959683618771496
Römermann, C., Dutoit, T., Poschlod, P., and Buisson, E. (2005). Influence of former cultivation on the unique Mediterranean steppe of France and consequences for conservation management. Biol. Conserv. 121, 21–33. doi: 10.1016/j.biocon.2004.04.006
Saatkamp, A., Henry, F., and Dutoit, T. (2017). Vegetation and soil seed bank in a 23-year grazing exclusion chronosequence in a Mediterranean dry grassland. Plant Biosyst. 152, 1020–1030. doi: 10.1080/11263504.2017.1407375
Saatkamp, A., Henry, F., and Dutoit, T. (2021). Romans shape Today’s vegetation and soils: two millennia of land-use legacy dynamics in Mediterranean grasslands. Ecosystems 24, 1268–1280. doi: 10.1007/s10021-020-00581-w
San Miguel, A. (2008). Management of natura 2000 habitats. 6220* pseudo-steppe with grasses and annuals of the Thero-Brachypodietea ATECMA, Spain: European Commission.
Schlotzhauer, S. M., and Price, J. S. (1999). Soil water flow dynamics in a managed cutover peat field, Quebec: field and laboratory investigations. Water Resour. Res. 35, 3675–3683. doi: 10.1029/1999WR900126
Silva, A., Imhoff, S., and Corsi, M. (2003). Evaluation of soil compaction in an irrigated short-duration grazing system. Soil Tillage Res. 70, 83–90. doi: 10.1016/S0167-1987(02)00122-8
Sinclair, J. K., Taylor, P. J., and Hobbs, S. J. (2013). Alpha level adjustments for multiple dependent variable analyses and their applicability – a review. Int. J. Sports Sci. Eng. 7, 17–20.
Suttie, J. M., Reynolds, S. G., and Batello, C. (2005). Grasslands of the world, FAO, Rome, Italy. Food and Agriculture Org.
Tatin, L., Wolff, A., Boutin, J., Colliot, E., and Dutoit, T. (2013). Écologie et conservation d’une steppe méditerranéenne: La plaine de la Crau, Versailles, France: Quae éditions Edn.
Török, P., Helm, A., Kiehl, K., Buisson, E., and Valkó, O. (2018). Beyond the species pool: modification of species dispersal, establishment, and assembly by habitat restoration: restoration modify dispersal and establishment. Restor. Ecol. 26, S65–S72. doi: 10.1111/rec.12825
Veldman, J. W., Buisson, E., Durigan, G., Fernandes, G. W., Le Stradic, S., Mahy, G., et al. (2015). Toward an old-growth concept for grasslands, savannas, and woodlands. Front. Ecol. Environ. 13, 154–162. doi: 10.1890/140270
Vidaller, C., Dutoit, T., Ramone, H., and Bischoff, A. (2019). Fire increases the reproduction of the dominant grass Brachypodium retusum and Mediterranean steppe diversity in a combined burning and grazing experiment. Appl. Veg. Sci. 22, 127–137. doi: 10.1111/avsc.12418
White, R. P., Murray, S., and Rohweder, M. (2000). Pilot Analysis of Global Ecosystems: Grassland Ecosystems. Washington, DC: World Resources Institute.
Keywords: patchiness, soil nutrients and granulometry, grazing intensity, plant community composition, plant diversity, community functioning, micro-local scale, biological conservation
Citation: Martin G, Courtial A, Génin A, Ramone H and Dutoit T (2022) Why grazing and soil matter for dry grassland diversity: New insights from multigroup structural equation modeling of micro-patterns. Front. Ecol. Evol. 10:879060. doi: 10.3389/fevo.2022.879060
Edited by:
Orsolya Valkó, Hungarian Academy of Sciences, HungaryReviewed by:
Antonio Greco, University of Salento, ItalyGonzalo García-Baquero, University of the Basque Country, Spain
Copyright © 2022 Martin, Courtial, Génin, Ramone and Dutoit. This is an open-access article distributed under the terms of the Creative Commons Attribution License (CC BY). The use, distribution or reproduction in other forums is permitted, provided the original author(s) and the copyright owner(s) are credited and that the original publication in this journal is cited, in accordance with accepted academic practice. No use, distribution or reproduction is permitted which does not comply with these terms.
*Correspondence: Gabrielle Martin, Z2FicmllbGxlLm1hcnRpbkB1bml2LXRsc2UzLmZy