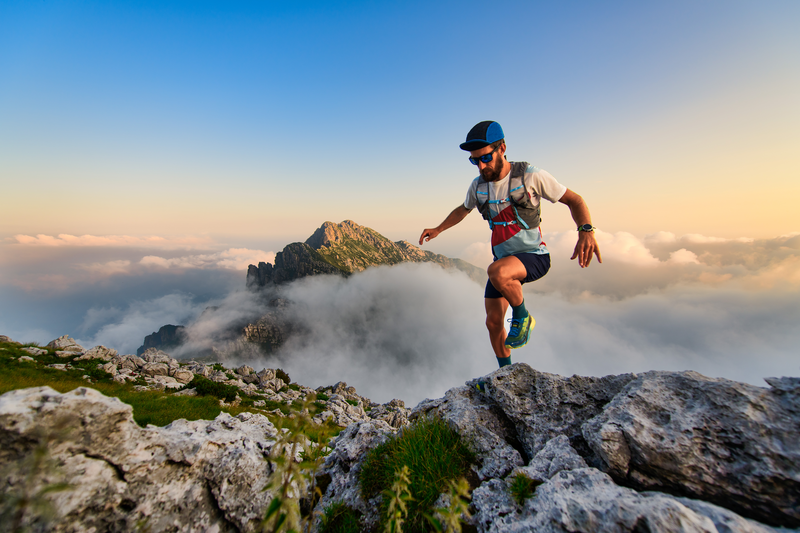
95% of researchers rate our articles as excellent or good
Learn more about the work of our research integrity team to safeguard the quality of each article we publish.
Find out more
REVIEW article
Front. Ecol. Evol. , 13 May 2022
Sec. Behavioral and Evolutionary Ecology
Volume 10 - 2022 | https://doi.org/10.3389/fevo.2022.879031
This article is part of the Research Topic Recent Advances in the Ecology and Evolution of the Bathyergidae View all 15 articles
Transmission of parasites between hosts is facilitated by close contact of hosts. Consequently, parasites have been proposed as an important constraint to the evolution of sociality accounting for its rarity. Despite the presumed costs associated with parasitism, the majority of species of African mole-rats (Family: Bathyergidae) are social. In fact, only the extremes of sociality (i.e., solitary and singular breeding) are represented in this subterranean rodent family. But how did bathyergids overcome the costs of parasitism? Parasite burden is a function of the exposure and susceptibility of a host to parasites. In this review I explore how living in sealed burrow systems and the group defenses that can be employed by closely related group members can effectively reduce the exposure and susceptibility of social bathyergids to parasites. Evidence suggests that this can be achieved largely by investment in relatively cheap and flexible behavioral rather than physiological defense mechanisms. This also shifts the selection pressure for parasites on successful transmission between group members rather than transmission between groups. In turn, this constrains the evolution of virulence and favors socially transmitted parasites (e.g., mites and lice) further reducing the costs of parasitism for social Bathyergidae. I conclude by highlighting directions for future research to evaluate the mechanisms proposed and to consider parasites as facilitators of social evolution not only in this rodent family but also other singular breeders.
Parasites (i.e., macroparasites such as ticks, fleas, lice, mites and helminths and microparasites such as viruses, bacteria and fungi) are important agents of selection. This is because they make up more than 50% of living organisms and by definition, they cause harm to their hosts (Poulin, 2007). This generates strong selective pressures which are considered one of the major evolutionary constraints that have made the evolution of sociality rare across the animal kingdom (Alexander, 1974). This is because the close proximity of individuals in a social group is assumed to facilitate the transmission of parasites between group members (Anderson and May, 1982; Rifkin et al., 2012; Patterson and Ruckstuhl, 2013).
Despite such potential evolutionary constraints, sociality has evolved repeatedly among an enigmatic group of subterranean rodents, African mole-rats of the family Bathyergidae (Faulkes et al., 1997). In fact, bathyergids exhibit a strict dichotomy between solitary species (genus Heliophobius, Georychus, and Bathyergus) and those exhibiting cooperative breeding (genus Heterocephalus, Fukomys, and Cryptomys) with a high reproductive skew (i.e., singular breeding) where usually only a single female and a small number of males per group breed (Faulkes and Bennett, 2021; Figure 1). With the exception of the genus Heterocephalus social genera are also more speciose than solitary ones (Faulkes and Bennett, 2021; Figure 1). Although based on phylogenetic analyses sociality may be the ancestral state for bathyergids (Faulkes and Bennett, 2021), this is not sufficient to account for the prevalence of this extreme form of sociality in this rodent family that has only been reported for about 3% of all bird and mammal species (Lukas and Clutton-Brock, 2012a). Similarly, only about 5% of mammals exhibit monogamy but this mating system is prevalent among social mole-rats and some of their close relatives although the degree of genetic monogamy differs between species and localities (Lukas and Clutton-Brock, 2012a,2013; Faulkes and Bennett, 2021). Monogamy cannot only limit the exposure to parasites, but has also been identified as a precursor for the evolution of cooperative breeding in singular breeding societies (Cremer et al., 2007; Lukas and Clutton-Brock, 2012b). For Bathyergidae ecological constraints to dispersal and the unpredictability of rainfall as well as encounters with food sources in habitats with high variance in rainfall have traditionally been proposed to be the driving force behind the evolution of sociality (Jarvis et al., 1994; Faulkes et al., 1997; Faulkes and Bennett, 2021). Large environmental variability, particularly with regards to rainfall has generally been proposed to be a key predictor of the evolution of cooperatively breeding bird and mammal species (Jetz and Rubenstein, 2011; Lukas and Clutton-Brock, 2017; Firman et al., 2020). However, this hypothesis has been criticized as singular breeding species also occur in relatively begin habitats (Shen et al., 2017). It would also not account for the occurrence of several cooperatively breeding bathyergid species in more mesic habitats while plural breeding or loosely social species are entirely absent from the Bathyergidae (Bennett and Faulkes, 2000; Šumbera et al., 2012; Patzenhauerová et al., 2013). While no other animal taxon exhibits this dichotomy of social systems, singular breeding is commonly found in social insects. Parasites have been implicated as important driver of the evolution of sociality in eusocial insects (Biedermann and Rohlfs, 2017; Cremer et al., 2018). To date, the possible role of parasites for other singular breeding species has not been investigated. In the remainder of this article, I will explore the role that parasites may have played in the evolution of sociality and singular breeding of bathyergids.
Figure 1. Simplified phylogenetic tree for the family Bathyergidae indicating their main genera based on mitochondrial and nuclear genes. Numbers in brackets indicate the numbers of species for the genus, the average and maximum group size reported for various species in the genus. © Images Marietjie Froneman.
Infection with parasites is a function of the exposure and susceptibility of individuals to parasites (Poulin, 2007). From a parasite’s perspective this requires a two-step process: encountering a host and invading it (Schmid-Hempel, 2021). Exploiting the subterranean niche may have aided in the evolution of sociality by reducing the exposure to parasites. It has been noted repeatedly that subterranean rodents have a significantly impoverished macroparasite species richness compared to similar sized terrestrial species while microparasite infection has rarely been studied (Scharff et al., 1996; Bartel and Gardner, 2000; Rossin and Malizia, 2002; Hubálek et al., 2005; Rossin et al., 2010; Viljoen et al., 2011b; Lutermann and Bennett, 2012; Cutrera et al., 2014; Lutermann et al., 2015, 2019; Archer et al., 2017; Fagir et al., 2021). However, since the majority of the other subterranean rodent families comprises mostly of solitary species and none are singular breeders (Jarvis and Bennett, 1993), a reduction of parasite exposure in the subterranean niche alone cannot account for the prevalence of singular breeding species among Bathyergidae. Regardless, bathyergids differ from most other subterranean families by living in sealed burrow systems, rarely venturing above ground and only some of the solitary species include above ground vegetation in their diet (Stein, 2000). At the same time, the buffered environmental conditions in the burrows of bathyergids with stable, warm temperatures and high humidity (Buffenstein, 2000; Šumbera, 2019) also provide ideal microhabitats for parasites such as fungi and arthropods that experience favorable conditions year-round (Marshall, 1981). Nevertheless, the subterranean niche may have been an ideal starting point for social evolution in bathyergids due to the constraints it puts on contact rates between hosts.
In a recent paper Schmid-Hempel (2021) proposed that from a parasite’s perspective the opportunity for transmission is the crucial difference between infecting solitary compared to a social host. This applies regardless of the vast range of group size and social organization in many social species. Several meta-analyses have concluded that group size per se affects parasite transmission or disease risk (Côté and Poulin, 1995; Rifkin et al., 2012; Patterson and Ruckstuhl, 2013). However, using group size as a proxy for sociality is a convenient but oversimplified approach that entirely ignores the many facets of group living. Instead Schmid-Hempel (2021) posits to use four key elements of sociality that affect what he calls “generalized transmission distances”: the temporal, spatial, genetic and ecological proximity of hosts that incorporate many dimensions of social organization. Group living may be temporary, e.g., for breeding purposes or perennial. In the case of bathyergids species, rainfall is a key determinant of group stability for social species but also affects breeding opportunities for both solitary and social species and foraging activity which may determine the encounter probability with parasites (Figure 2A; Spinks et al., 1999, 2000; Young et al., 2010; Torrents-Ticó et al., 2018). Transmission can occur within as well as between groups representing different spatial scales (Figure 2A). The patterns of genetic relatedness can vary widely between host species but relatedness is particularly high in cooperative breeders where breeding is limited to a small number of individuals and most group members are offspring of the breeders (i.e., singular breeders) as is the case in all social mole-rats (Bishop et al., 2004; Burland et al., 2004; Patzenhauerová et al., 2013; Ingram et al., 2015). If transmission of a parasite is determined by the genetic make-up of the host, closely related hosts in close spatial proximity for extended periods of time, such as in social Bathyergidae, could greatly facilitate the spread of a parasite (Schmid-Hempel, 2021). Lastly, their social organization and cooperation allows social species to extend their ecological niche (Schmid-Hempel, 2021). Consequently, in social bathyergids the exploitation of arid habitats with highly dispersed food sources is thought to be enabled by group-living (Bennett and Faulkes, 2000; Faulkes and Bennett, 2021). However, it also extends to the structure and dimensions of their burrow systems with larger systems potentially exposing individuals to more parasites.
Figure 2. Compositive distance space [i.e., generalized transmission distance (GTD) derived from spatiotemporal, genetic and ecological distance between hosts, a theoretical measure of transmission distance] and its predicted effects on parasite exposure and selection pressures on parasites and bathyergid hosts. This measure incorporates characteristics of the social organization and group size of a particular species. (A) GTD is unimodal for solitary hosts (black, dashed line) but bimodal for social hosts (yellow line) due to short GTDs between group members (within-group) but long GTDs between groups. (B) Living in a subterranean environment excludes mobile parasites and reduces exposure to environmentally-transmitted or vector-borne parasites (e.g., ticks, helminths). For parasites (open arrows) this reduces their degree of co-infection with variable host investment (solid arrows) in physiological defenses (i.e., resistance vs. tolerance). (C) In a subterranean environment GTDs for within and between-group transmission vary with rainfall and soil properties but are generally larger for the latter compared to terrestrial species. These conditions favor directly transmitted parasites (e.g., mites), but pose constraints on their host range and virulence with uncertain consequences for host investment in physiological defenses. (D) Behavioral responses of hosts (i.e., organizational immunity) can further generate differential patterns of GTDs depending on their presence (orange line) or absence (gray, dotted line). The former causes further parasite aggregation (curve on the bottom right) and reductions in population size. For parasites this can result in reductions in genome size and associated function as well as further decreases of virulence and potentially evolution of symbiotic relationships with unknown consequences for physiological defenses employed by hosts.
Incorporating these four elements into a “generalized transmission distance” suggests fundamental difference in the transmission dynamics between solitary and social hosts that parasites face (Schmid-Hempel, 2021; Figure 2A). As illustrated in Figure 2A parasites experience a unimodal, intermediate transmission distance across a host population when parasitizing solitary hosts (Schmid-Hempel, 2021). In contrast, distances within groups are short, and this is where most transmission occurs (Figure 2A). Conversely, between group transmissions distances are far and consequently transmission frequencies are low resulting in a bimodal distribution of transmission distances (Figure 2A). These differences lead to fundamentally different selection pressures in the host-parasite systems of solitary and social hosts. For subterranean species, the transmission distances between groups are further exaggerated due to the dispersal constraints posed by the challenges of below-ground dispersal (Figure 2C). The antagonistic relationship between parasites and their hosts suggests that the fitness implications of these differences in transmission distances and how these may have shaped the evolution of sociality in bathyergids should be considered separately (Schmid-Hempel, 2021). Accordingly, I will address these separately for hosts and parasites in the following.
Parasite infection depends on the exposure (i.e., encounter) as well as susceptibility (i.e., invasion and proliferation) of an individual. The highly skewed distribution of parasites (also called overdispersed) across host populations suggests that these two parameters can differ widely among host individuals (Poulin, 2007).
Hosts generally employ three strategies to reduce either exposure or susceptibility to parasites, i.e., by avoiding encounters with parasites, suppressing parasite proliferation and/or minimizing parasite damage (Råberg et al., 2009; Medzhitov et al., 2012; Hart and Hart, 2018). While parasite avoidance is largely limited to behavioral modifications, preventing parasite proliferation and reducing damage also requires physiological responses that are more costly (Viney et al., 2005; Boots et al., 2009; Medzhitov et al., 2012).
The most efficient and at the same time probably least costly anti-parasite strategy an individual can employ is the avoidance of parasites by actively reducing encounter probabilities (Hart and Hart, 2018). This includes the avoidance of areas with high exposure but also of infected conspecifics including when choosing mates (Hart and Hart, 2018). However, subterranean species may be limited in the choice of habitats they explore or in their mate choice due to the costs of digging (Vleck, 1979; Šumbera, 2019). This could have been one factor contributing to the differences in microhabitat choice between solitary and social bathyergids with the former often using more easily workable soil types (Romanach, 2005; Lövy et al., 2012). In addition, evidence suggests that solitary species more frequently venture above ground for breeding dispersal which may also permit more flexibility in mate choice (Stein, 2000). While in social Bathyergidae individuals may act aggressively toward unfamiliar conspecifics, active discrimination based on infection status has rarely been tested (Lutermann et al., 2022). Within a burrow system individuals can reduce their exposure to any parasites present by using several sleeping chambers and/or the use of distinct chambers for various purposes (Roper et al., 2002). The most obvious may be a toilet chamber that will limit the deposit of urine and feces, that could be the source of directly transmitted helminths or pathogens, spatially. Using these chambers only for brief periods would furthermore reduce the parasite exposure. Similarly, the use of food chambers reduces the chances of consuming contaminated food. Such a dedicated chambers are already apparent in the burrow systems of solitary bathyergids (Thomas et al., 2009, 2012; Šumbera et al., 2012).
Grooming is known to be an effective means of removing ectoparasites (Mooring et al., 2004; Hart and Hart, 2018). In addition, the incorporation of plant materials containing volatiles inhibiting the growth and survival of ectoparasites and bacteria in nesting material can reduce exposure (Hemmes et al., 2002). However, it is not known in how far this is employed by bathyergids and possible medicinal properties of the of the plants they use have rarely been explored.
Once parasite infection has occurred a host can employ several mechanisms to suppress its proliferation. Self-medication in the form of the consumption of plant material or substances with medicinal properties can be an effective behavioral strategy to limit the amplification of parasites once an infection has occurred (Hart and Hart, 2018). This anti-parasite behavior can be observed in a wide range of animal species (Neco et al., 2019). It is unknown whether bathyergids also practice this behavior. However, the physical constraints of the subterranean environment make it unlikely that they are selectively seeking such plants. Nevertheless, the “geophyte farming” described for some Bathyergidae may include bulbs and tubers with medicinal properties that are kept for such a purpose (Jarvis and Sale, 1971; Lovegrove and Knight-Eloff, 1988; Jarvis et al., 1998). In addition, the inclusion of bulbs and tubers with highly toxic secondary plant component reported for several bathyergids (Bennett and Faulkes, 2000) may also assist in suppressing the proliferation of pathogens. These plants often also have a high fiber content and require the aid of symbiotic microorganisms in the cecum to break down the cellulose (Buffenstein, 2000). It has been reported that such microorganisms can also help their host to combat parasites in some species (Kreisinger et al., 2015; Peachey et al., 2017; Leung et al., 2018). It remains to be seen whether they play a similar role in bathyergids although this has been suggested for at least one species (Debebe et al., 2017; Braude et al., 2021). Both, direct competition for resources with invading parasites but also the production of chemicals in response to infection have been reported (Kreisinger et al., 2015; Leung et al., 2018). In the case of mole-rats such toxins may act directly in the host’s body or could be taken up through the coprophagy practiced by these animals. Currently, however, no empirical data is available for bathyergids to support this hypothesis.
In adaption to the high temperatures and humidity in the sealed burrow systems mole-rats have lowered metabolic rates and less dense pelage to prevent the build-up of heat and facilitate heat dissipation (Bennett and Faulkes, 2000; Buffenstein, 2000; Šumbera, 2019). In addition, they may temporarily become hypothermic in response to hypoxia although this response appears to be restricted to social bathyergids (Cheng et al., 2021). Lowered body temperatures could also slow down the proliferation of some pathogens and parasites. If mole-rats would actively downregulate their body temperatures in response to infection, similar to the response to hypoxic conditions, they could thus contain infection. Although it is unclear whether body temperature was downregulated actively, resting metabolic rate of Natal mole-rats (Cryptomys hottentotus hottentotus) infested with cestodes (Raillientina sp.) was reduced providing indirect support for such a mechanism. However, this could only be sustained at a low to medium cestode abundance while it was similar to that of uninfested animals in those carrying large burdens of cestodes. Furthermore, mole-rats are known to regulate the temperature in their intestines to provide ideal conditions for their symbionts suggesting that they are able to make selective adjustments in body temperature (Buffenstein, 2000).
A suite of behavioral (i.e., sickness behaviors) and physiological changes in the host in response to microparasite infections form part of the acute phase response that can be observed across a wide range of taxa (Hart, 1988; Adelman and Martin, 2009). Sickness behaviors include reductions in overall activity, food intake, and libido but also increases in body temperature causing a fever are part of this phenomenon. It allows the host to conserve energy that can be channeled into immune responses but also deprives the parasite of essential nutrients for growth and replication (Adelman and Martin, 2009). Fever and sickness behaviors have also been recorded for several mole-rat species (Urison et al., 1993; Viljoen et al., 2011a; Lutermann et al., 2012) and likely play a role in combating infections with microparasites in bathyergids as well.
The immune system of mammals is complex and comprised of two arms, innate and adaptive immunity, and is probably the most important line of defense against parasites once transmission has occurred (Adelman, 2010). Innate immune responses are considered relatively cheap and broadly target foreign molecules. Conversely, adaptive immune responses are antigen-specific and induced by exposure to foreign antigens (Lochmiller and Deerenberg, 2000; Klasing, 2004). Triggering this arm of the immune system is energetically costly and requires trade-offs with other demands (e.g., growth, reproduction) an individual might experience and hence, affect individual fitness (Lochmiller and Deerenberg, 2000; Lee, 2006; Martin et al., 2008; Martin, 2009). In concert with the genetic make-up of an animal as well as co-infections with other parasites the costs of immune responses are likely to be the reason why immune responses vary considerably within and between individuals (Viney et al., 2005; Altizer et al., 2006; Råberg et al., 2009; Bordes et al., 2012). However, this investment can pay off when it confers immunity to re-infection with the same parasite species. In mammals it may furthermore be transmitted to offspring via the placenta or via antibodies in the milk produced by mothers (Boulinier and Staszewski, 2008; Roth et al., 2018). Apart from extensive studies in naked mole-rats (Heterocephalus glaber) the immune function of bathyergids has received limited attention (Lutermann and Bennett, 2008; Lutermann et al., 2012; Lin and Buffenstein, 2021). Innate immune responses of H. glaber to parasites are potentially modified as they lack natural killer cells. At the same time, their macrophages exhibit a higher production of pro-inflammatory cytokines and they possess a type of neutrophils that can produce several antimicrobials at large quantities properties that are not known from other rodents (Lin and Buffenstein, 2021). It is unknown whether similar modification are also present in other bathyergid species but the composition of blood cells in highveld mole-rats (Cryptomys hottentotus pretoriae) was modified by infection with parasites (Lutermann et al., 2012).
The costs associated with resistance as well as the possible tissue damage involved (i.e., immunopathologies) may be the reason why some individuals or species may opt for tolerance rather than resistance (Viney et al., 2005; Råberg et al., 2009; Schulenburg et al., 2009; Best et al., 2012; Medzhitov et al., 2012). Although well-established in plant hosts, this strategy has only recently garnered attention in animals and studies of tolerance in animals are limited (Baucom and de Roode, 2011; Medzhitov et al., 2012; Budischak and Cressler, 2018). The host can attempt to minimize the costs of tissue damage from either the parasite or the immune system using a variety of mechanisms (Medzhitov et al., 2012). These include cellular stress responses triggered by stress-response systems dedicated to particular stressors such as high levels of reactive oxygen species (ROS) or hypoxia (Medzhitov et al., 2012). ROS have been studied in all three social genera, most extensively in H. glaber, but not in relation to parasites (Mendonça et al., 2020; Braude et al., 2021; Buffenstein et al., 2022; Jacobs et al., 2021a,b). Like other subterranean rodents Bathyergidae are adapted to the hypoxic conditions in their burrow systems and this also includes range of physiological adaptations to reduce oxidative stress (Schülke et al., 2012; Ivy et al., 2020; Logan et al., 2020). Adaptations to the subterranean niche may hence, have equipped bathyergids with a physiological “tool kit” that could also prove useful in parasite tolerance and may make them particularly tolerant to parasite infections. Tolerance to infection with helminths has been shown in C. h. pretoriae where males chronically infested with Mathevotaenia sp. show neither reductions in body mass nor changes in androgen levels (Lutermann et al., 2012, 2022). However, simulation of a secondary bacterial infection resulted in significant reductions of testosterone levels for helminth-infested but not healthy males suggesting lower tolerance to invasion of a second parasite (Lutermann et al., 2012).
Hosts are unlikely to pursue only a single strategy but employ a mixture of all of these, potentially in a parasite-specific manner (Best et al., 2008; Boots et al., 2009; Råberg et al., 2009) as all are clearly beneficial. The use of the various strategies may also change temporally depending on other demands (e.g., reproduction) and resource availability (Altizer et al., 2006; Budischak and Cressler, 2018).
Cremer and colleagues suggested that singular breeding social insects (i.e., eusocial Hymenoptera and termites) achieve parasite protection at a group-level through the sum of defenses employed by group members that affect the exposure and susceptibility of social hosts to parasites and called this “social immunity” (Cremer et al., 2007, 2018). Similar to individual host defenses, social immunity can be distinguished into avoidance, resistance and tolerance. In addition to a behavioral and physiological component it has an organizational components that is unique to social organisms (Cremer et al., 2018). While aspects of social immunity have been the focus of many eusocial insects (Cremer et al., 2007, 2018; Stroeymeyt et al., 2014; Schmid-Hempel, 2021), its applicability to social vertebrate species has not been explored (Van Meyel et al., 2018).
Behavioral strategies play an important role in parasite avoidance at the colony level. Exploiting soil types that pose greater constraints to underground dispersal may already act as an effective defense against between-group parasite transmission (Figure 2B). In addition, Bathyergidae are known to be xenophobic (Riain et al., 1997; Spinks et al., 1998; Clarke and Faulkes, 1999; Ganem and Bennett, 2004; Bappert et al., 2012). Although this could be partially attributable to the risk of reproductive competition in social species, an alternative, and not mutually exclusive, function may be to reduce the risk of parasite transmission by intruding conspecifics (Freeland, 1976, 1979). This hypothesis has not yet been addressed for Bathyergidae, but does not appear to always apply (Lutermann et al., 2022).
In addition to self-grooming, allo-grooming, the grooming of conspecifics, can further reduce the risk of parasite transmission. Since the rate of allo-grooming should increase with group size this could be an effective way to reduce the risk of parasitism in social species including social bathyergids. It could account for the negative correlation between ectoparasite burdens and colony size found in several social bathyergids (Viljoen et al., 2011b; Lutermann et al., 2013; Archer et al., 2016). In eusocial insects allo-grooming also significantly affected the outcome of interspecific competition between co-infecting parasites (Milutinović et al., 2020). The possible role of allo-grooming for parasite control has not been explored in Bathyergidae.
While avoidance, resistance and tolerance are clearly beneficial to both solitary and social host individuals, social species may have more resources to invest (Ezenwa et al., 2016). Resource availability can constrain a host’s ability to use either resistance or tolerance as a strategy to combat parasite infection (Budischak and Cressler, 2018). However, if increased access to resources is one of the benefits of group-living, social species should have additional means to invest into parasite defenses (Ezenwa et al., 2016). For example, in Natal mole-rats energy stores in the form of fat increased with colony size (Lutermann et al., 2013). This is likely to translate into more energy being available for parasite defenses and although this has not been explicitly tested for any bathyergid, the negative correlations between colony size and parasite burden reported for several Cryptomys species suggests that this might be the case (Viljoen et al., 2011b; Lutermann et al., 2013). The additional resources available can either be used for stronger immune responses but can also increase the tolerance of social hosts to parasite infection as shown in other singular breeding mammals (Almberg et al., 2015).
Exposure to infected conspecifics can result in the activation of the immune system of uninfected individuals in anticipation of an infection (Kavaliers and Colwell, 1992, 1994). Hence, encounters with infected intruders as well as colony mates could prime individuals in social Bathyergidae and in turn lower their susceptibility to parasites. Furthermore, coprophagy between individuals or from adult offspring to newly recruited offspring may allow for the transmission of both immune-stimulating substances as well as a diverse symbiont community that could provide additional parasite defenses (Ezenwa et al., 2016) similar to what has been reported for some social insects (Leclaire et al., 2014; Powell et al., 2014; Cremer et al., 2018). These hypotheses have not been addressed for bathyergids but deserve attention in the future.
Parasite transmission is often linked to contact rates between individuals (May and Anderson, 1978; Altizer et al., 2003). This is illustrated by several network analyses showing that microbial fauna reflects contact networks of inter-individual contacts (Drewe, 2010; Blyton et al., 2014; VanderWaal et al., 2014). In addition, this may be facilitated by the close relatedness in singular breeding species (Cremer et al., 2007, 2018; Schmid-Hempel, 2021). First proposed for social insects, the organizational immunity hypothesis posits that division of labor as well as behavioral flexibility that modulates contact rates between group members in response to infection should constrain parasite spread within a colony (Naug and Camazine, 2002; Cremer et al., 2007; Stroeymeyt et al., 2014).
The temporal or spatial separation of group members can reduce parasite transmission by reducing contacts (i.e., exposure), both direct or indirect, between group members (Evans et al., 2020; Lucatelli et al., 2021). The use of different sections of the burrow system at different times could be a means of temporal separation. A number of studies has addressed activity patterns in Bathyergidae although mostly focusing on activity rhythms when in isolation (Oosthuizen and Bennett, 2022). Nevertheless, in laboratory conditions activity patterns differed between individuals for several social bathyergids (Oosthuizen et al., 2003; Hart et al., 2004; De Vries et al., 2008; Schielke et al., 2012; van Jaarsveld et al., 2019). Similarly, they differed between group members in the field (Šklíba et al., 2012, 2014, 2016; Šumbera et al., 2012; Lövy et al., 2013; Francioli et al., 2020; Finn et al., 2022). Several of the latter studies also observed distinct differences in activity patterns between breeding and non-breeding colony members with the former exhibiting less activity which could lead to differential exposure and susceptibility to parasites for colony members.
The greater complexity of burrow systems of social compared to solitary bathyergids is probably not simply a results of greater foraging efficiency or a larger number of foragers (Lovegrove, 1988; Spinks et al., 1999; Le Comber et al., 2002; Le Comber, 2006; Sichilima et al., 2008; Šumbera, 2019). Burrows containing several nesting and/or toileting areas allow colony members to rest or defecate separately, both in time and space, and this can impede within-group transmission via reduced exposure. In fact, several studies have reported the differential use of burrow systems by colony members (Lacey and Sherman, 1991; Šumbera et al., 2012; Lövy et al., 2013; Šklíba et al., 2016; Francioli et al., 2020) which is likely to affect parasite exposure. At the same time, singular breeding and high relatedness among colony members in social Bathyergidae also means that losing non-breeders due to parasite infection is less costly while these individuals still accrue inclusive fitness benefits (Cremer et al., 2018; Schmid-Hempel, 2021). To date, the removal of infected group members, either of their own volition or forcibly by other group members, as observed in social insects, has not been reported for bathyergids.
In addition to the reproductive division of labor, the division of labor of other tasks and/or specialization on particular tasks observed in eusocial insects can reduce the spread of parasites among colony members, particularly if those tasks are carried out in different parts of the nest or burrow (Cremer et al., 2007, 2018; Stroeymeyt et al., 2014). In more flexible eusocial insect species, usually those with smaller group sizes, task specialization changes with time and environmental conditions and may vary based on age, sex or infection status of the individual concerned (Tofts and Franks, 1992; Cremer et al., 2007, 2018; Stroeymeyt et al., 2014). Although task specialization has been suggested for naked mole-rats and several Fukomys species in early studies (Jarvis, 1981; Bennett and Jarvis, 1988; Lovegrove, 1988; Gaylard et al., 1998; Wallace and Bennett, 1998) this was not confirmed in later ones (Lacey and Sherman, 1991; Thorley et al., 2018; Siegmann et al., 2021). However, several studies have reported that an individual’s age, sex and/or breeding status correlates with its contributions to cooperative tasks in these bathyergid species (Thorley et al., 2018; Zöttl et al., 2018; Siegmann et al., 2021). No such division of labor could be identified in Cryptomys species, but few studies have addressed this in this genus (Bennett, 1989; Moolman et al., 1998). In eusocial insects age-related cooperative behavior also determines exposure to parasites (Stroeymeyt et al., 2014; Cremer et al., 2018). However, additional division of labor may also be constraint by group size and more likely in species achieving larger group sized (e.g., naked or Damaraland mole-rats). In accordance with this hypothesis, age-related cooperative behavior has also been identified in eusocial bathyergids but links to parasite transmission have not been explored. Similarly, age effects on parasite burden have not explicitly been addressed although effects of breeding status in some species may be an indication of this as breeders are presumably the oldest individuals in a group.
The task-specific division of labor can also affect the microbiome of individuals, either because they differ in their exposure to microorganisms or because exchange of microorganisms occurs predominately between individuals carrying out the same task (Münger et al., 2018; Sinotte et al., 2020). In eusocial insects these differences are assumed to also increase the efficiency with which certain tasks are carried out and increase the extraction of nutrient that also fuel immune responses (Iorizzo et al., 2020; Sclocco and Teseo, 2020). Although the number of studies investigating the effects of the division of labor on the microbiome remains limited, the available information suggests distinct microbiomes for breeders and non-breeders as well as different worker castes in eusocial insects (Sclocco and Teseo, 2020; Sinotte et al., 2020). These differences can also be linked to differences in metabolomics including more stimulated immune systems in foraging workers (Quque et al., 2021). Currently, no information is available in how far this may also apply to social Bathyergidae.
The above information suggests that social bathyergids have various additional avenues of parasite defense, many of which do not rely on costly mechanism. Behavioral mechanisms also allow for a great deal of flexibility and may only be employed after a parasite infection has occurred, further reducing their costs.
The fitness of parasites can be partitioned into two components the success within and that between hosts (Schmid-Hempel, 2021). The former requires the successful invasion and multiplication, growth or acquisition of resources to eventually produce transmission stages or offspring. Conversely, the latter is determined by the successful transmission of this parasite propagules to new hosts. In the following I will consider the two fitness determining processes separately.
There are three host traits that determine the within host-success of a parasite: the immune response of the host, host predictability and the presence of other parasites or symbionts that may compete for host resources (Schmid-Hempel, 2021).
As outlined above, hosts can employ one of two immune strategies in response to an infection; resistance or tolerance (Råberg et al., 2009; Baucom and de Roode, 2011; Medzhitov et al., 2012). While the former can effectively reduce parasite survival and proliferation the latter does not. At the same time, host tolerance extends the period of propagule production and hence, is likely beneficial for the success of parasites (Budischak and Cressler, 2018).
Overall, host individuals in bathyergid groups may differ in their suitability as hosts for parasites invading a social group. Indeed, asymmetric distribution of parasites between breeders and non-breeders or between the sexes has been reported for some parasite species of social bathyergids (Ross-Gillespie et al., 2007; Viljoen et al., 2011a; Archer et al., 2016, 2017; Fagir et al., 2021). However, this was not the case for other social Bathyergidae (Fagir et al., 2021). Similarly, such differences may be age-based (Silk and Fefferman, 2021).
Social rank can affect immune responses as shown in recent meta-analyses (Habig and Archie, 2015; Habig et al., 2018). Although patterns varied widely among the studies included, there was a general trend for dominant individuals to carry higher parasite burdens. The authors proposed that this was linked to the greater investments by dominants to achieve and maintain their status (Habig and Archie, 2015; Habig et al., 2018). However, this pattern was not apparent in cooperatively breeding species. For singular breeders such as social bathyergids the kin structure of the group would likely preclude such competition for dominance that is usually linked to reproductive activity. While studies exploring this relationship are limited for bathyergids, Natal mole-rats breeders exhibited stronger fever responses to a simulated infection and had significantly larger spleens, an organ important for the storage and circulation of immune cells (Lutermann and Bennett, 2008; Viljoen et al., 2011a). This may be due to energy savings of breeders that partake to a lesser extend in energetically costly cooperative tasks.
Hosts with larger body sizes, in better condition, that are more abundant and/or long-lived are a more predictable resource from a parasite perspective and this benefits its persistence and proliferation (Combes, 2001). With few exceptions (i.e., Giant mole-rats, Fukomys mechowi), social bathyergids are smaller in body size than solitary species however, they are more abundant due to living in groups (Bennett and Faulkes, 2000). At the same time, individuals are likely to be in better body condition in social species and fat stores increased with group size for Natal mole-rats (Lutermann et al., 2013). While longevity does not increase with sociality per se, it does in singular breeding species where it is mostly observed for reproductive individuals (Lucas and Keller, 2020; Downing et al., 2021; Kennedy et al., 2021; Korb and Heinze, 2021; Vágási et al., 2021). While there is currently no information on longevity for the genus Cryptomys, members of the other two social genera (i.e., Heterocephalus and Fukomys) are well-known for their long life-spans, particularly in breeders (Dammann and Burda, 2006; Dammann et al., 2011; Schmidt et al., 2013; Lewis and Buffenstein, 2016). This suggests that social Bathyergidae may be particularly predictable and localized hosts from a parasite’s perspective.
Infections with a single parasite are the exception rather than the rule in nature (Behnke et al., 2001; Cox, 2001), but is suggested to be more common for social species (Altizer et al., 2003; Schmid-Hempel, 2021). Since parasites rely entirely on their hosts for resources this can cause competition between co-infecting parasites for limiting host resources (Pedersen and Fenton, 2007). Such competition can reduce an individual parasite’s success but can also facilitate it (Knowles et al., 2013; Pedersen and Antonovics, 2013; Fagir et al., 2015; Hoffmann et al., 2016; Mabbott, 2018). In addition to this direct interaction between co-infecting parasite species, they can also interact indirectly via the host’s immune system and infection with one parasite may enhance or reduce the successful invasion and/or proliferation of another parasite (Pedersen and Fenton, 2007). Furthermore, the dependence on beneficial microorganisms bathyergids require to break down their unpalatable herbivorous diet (Bennett and Faulkes, 2000; Buffenstein, 2000) makes competition for resources between parasites and endosymbionts similarly likely.
Parasites are required to complete three steps to achieve between-host fitness gains starting with leaving the current host (Schmid-Hempel, 2021). While this step likely does not differ fundamentally between solitary and social hosts, the subsequent transmission steps (i.e., encountering and infecting) to a new hosts are characterized by substantial differences in general transmission distance (Schmid-Hempel, 2021; Figure 2).
By definition host-parasite relationships are antagonistic with each side attempting to increase their fitness at the expense of the other (Poulin, 2007). In the resulting evolutionary arms race parasites depend entirely on their hosts for their survival while this does not apply in a similar fashion to hosts (Dawkins and Krebs, 1979). Furthermore, the number of parasites is generally larger and their generation time usually shorter than that of their hosts suggesting that selection will mostly act on parameters of the host-parasite relationship that provide the greatest fitness returns for parasites (Poulin, 2007; Schmid-Hempel, 2021). In the case of social bathyergids this would be the within-group transmission which is likely to occur more frequently than that between colonies (Archer et al., 2016; Figure 2C). Consequently, selection of defense mechanisms reducing within-colony transmission should be under strong selection pressure in social bathyergids (Hawley et al., 2021; Schmid-Hempel, 2021). At the same time, parasites of social bathyergids should experience stronger selection pressures than their hosts, mainly on mechanisms aiming to increase the transmission rate for parasites. In the following I will address the resulting selection scenarios for hosts and parasites separately.
As behavioral measures can substantially affect parasite transmission and are highly flexible at comparatively low costs, selection pressures from parasites should act strongly on behavior (Ezenwa et al., 2016; Hawley et al., 2021). The presumed higher transmission in larger groups selects for smaller group sizes (Altizer et al., 2003; Rifkin et al., 2012; Patterson and Ruckstuhl, 2013). Under such circumstances selection can instead favor preferential social interactions with certain individuals that can ultimately lead to modularity to reduces parasites transmission (Freeland, 1976, 1979; Griffin and Nunn, 2012; Nunn et al., 2015). However, evidence for this is weak possibly due to the opposing selection pressures associated with the benefits of group-living including lower predation pressure, increased foraging efficiency, transfer of protective microbes and information that may counter pressures on reductions of group size (Ezenwa et al., 2016; Townsend et al., 2020; Hawley et al., 2021). In social bathyergids the ecological constraints posed by the subterranean niche already generate between-group modularity (Figure 2C). In addition to the benefits of improved foraging efficiency in groups, ecological and behavioral factors have likely played an important role in bathyergids in tipping the balance in favor of group-living (Faulkes and Bennett, 2021). Ecological constraints to dispersal likely have also relaxed selection pressures on avoiding encounters with parasites through infected intruders. This could also account for the lack of avoidance of odors from infected males observed in highveld mole-rats and the readiness of females of several bathyergid species to engage in copulations with unfamiliar males (Lutermann et al., 2022).
Modularity can occur between as well as within groups (e.g., organizational immunity) and the selection pressure exerted by parasites is likely to contribute to modularity at a colony level (Figure 2D). The predominance of within-group transmission in social bathyergids suggests that selection for organizational immunity, including the reproductive division of labor, should be a major evolutionary trajectory in this family. Avoidance and hygienic behaviors can be observed across the full spectrum of sociality (Meunier, 2015; Van Meyel et al., 2018). This often leads to asymmetries in inter-individual contact rates which can differ widely across a range of social systems from solitary to eusocial (Sah et al., 2018). Such modularity between less connected sub-groups is common among social species and increases with group size across species (Griffin and Nunn, 2012; Sah et al., 2018; Evans et al., 2021). At the same time, parasite-mediated selection should favor greater investment in hygienic behaviors including allo-grooming, burrow hygiene or the removal/isolation of infected group members, but reduce the incidence of social behaviors that can increase parasite transmission such as agonistic behaviors (Hawley et al., 2021). This is more likely to evolve in kin groups where inclusive fitness benefits reduce competition for resources. However, the evolution of full organizational immunity, including the reproductive division of labor, is likely constrained in species with multiple breeders due to the lack of inclusive fitness benefits as a result of lower relatedness. In contrast, in closely related hosts, parasites can favor the evolution of singular breeding and organizational immunity as has been proposed for eusocial insects (Naug and Camazine, 2002; Biedermann and Rohlfs, 2017; Cremer et al., 2018). Similarly, parasites could have generated an important selection pressure for the monogamous ancestors of social mole-rats to invest into organizational immunity in response to parasitism due to the high dispersal barriers for offspring (Figure 2D). Group sizes differ substantially between social bathyergids and social insects with the latter having group sizes that may exceed those of mole-rats by three orders of magnitude. This is relevant as implementing and maintaining organizational immunity may be constrained by group size. However, it has recently been shown for singular breeding ambrosia beetles (Xyleborinus saxesenii) living in small groups, that offspring delay breeding dispersal in the presence of microparasite infection (Nuotclà et al., 2019). The additional investment in hygienic behaviors by philopatric offspring significantly reduced the detrimental effects of parasite infection and hence, lowered the costs of sociality. If similar effects can be accrued by non-breeding group members in social bathyergids this could also account for the philopatry in bathyergid species where dispersal constraints are relaxed (e.g., F. mechowii or F. anselli). At the same time, such benefits would be an additional incentive for offspring in species with high dispersal constraints (e.g., H. glaber) to forgo investment into reproduction and hence, parasites may also have contributed to the evolution of singular breeding and reproductive suppression in Bathyergidae. The division of labor for other tasks has been questioned for social bathyergids (Lacey and Sherman, 1991; Thorley et al., 2018; Siegmann et al., 2021). Recent mathematical models suggest significant fitness benefits for such division of labor (Udiani and Fefferman, 2020). However, this only applied in the presence of parasites when age-based division of labor reaped more benefits than fixed task specialization. Consequently, some forms of organizational immunity may be more flexible in bathyergids.
While both resistance and tolerance are strategies available to solitary as well as social species and although parasite burden is frequently assumed to be larger in social species (Altizer et al., 2003; Rifkin et al., 2012; Patterson and Ruckstuhl, 2013), from the arguments outlined above it is not clear that social species should exhibit a greater investment in immune defenses than solitary species (Schmid-Hempel, 2021 and references therein, Figure 2). Comparative studies in social bees and cooperatively breeding birds suggest a greater investment in immune defenses in the latter (Stow et al., 2007; Spottiswoode, 2008). However, such increased investment in immunity is energetically costly and should more likely be favored in singular breeding species as breeders can benefit from the contributions of related non-breeders (e.g., greater access to food, less investment in foraging or parental care) that in turn increase their inclusive fitness in this way. Conversely, tolerance may carry fitness costs to the host as it does not affect the parasite burden (Medzhitov et al., 2012; Budischak and Cressler, 2018). Once again, the benefits of group-living in the form of greater access to resources can, however, alleviate these costs as has been shown for other singular breeding species (Almberg et al., 2015). Similarly, theoretical work suggests that high burdens of parasites with low virulence should select for hosts to employ tolerance rather than resistance as a defense strategy (Bonds et al., 2005). Thus, organizational immunity can provide effective protection against parasites in singular breeders while additional investment in immune response may not be required or even be lower in singular breeders (Figure 2).
Due to the reproductive division of labor the effective population size of singular breeding species is smaller than in communally breeding or solitary species and this can cause a loss of genetic diversity (Hoban et al., 2020). For social insects this results in reduced negative or purifying selection across the genome (Imrit et al., 2020). Similarly, there is evidence for a link between effective population size and selection of genes involved in parasite recognition and immune activation in Bathyergidae. Kundu and Faulkes (2004) report evidence for purifying selection of exon 3 of the MHC II BLA-DQα1 gene, coding for the transmembrane protein not directly involved with parasite recognition, from solitary (Heliophobius argenteocinereus) to social bathyergids (Cryptomys hottentotus hottentotus) that was less pronounced in the two species with the largest reproductive skew (F. damarensis and H. glaber) and the smallest effective population sizes. At the same time, evidence for positive selection on exon 2, coding for the antigen recognition site, was stronger in C. h. hottentus compared to the solitary as well as the other social species (F. damarensis and H. glaber; Kundu and Faulkes, 2004). Furthermore, high mortalities observed in laboratory colonies of H. glaber as a result of artificial viral infections suggest that immune responses may be weaker in the species with the lowest effective population size (Ross-Gillespie et al., 2007; Artwohl et al., 2009). Conversely, investment in immune priming via social cues from infested conspecifics should be strongly selected for in social bathyergids to reduce within-group transmission (Schmid-Hempel, 2021).
These examples illustrate that despite the costs of parasitism, selection against group-living should be reduced for singular breeding Bathyergidae. At the same time, selection on behaviors reducing parasite transmission, particularly within colonies such as organizational immunity, should be stronger than selection on physiological immunity, particularly if they provide protection from a range of parasite taxa (Hawley et al., 2021). The kin structure of social bathyergids is a key element affecting the inclusive fitness benefits of such anti-parasite strategies and similar advantageous effects cannot be expected for plural breeders.
Social behavior of hosts can have profound effects on the population structure and evolution of virulence of parasites (i.e., parasite-induced harm to the host) (Ezenwa et al., 2016; Hawley et al., 2021). The genetic diversity and effective population size of a parasite increases in gregarious species with large groups that exhibit only weak modularity (Figure 2D). Such species also tend to sustain a larger diversity of parasite species (Côté and Poulin, 1995; Rifkin et al., 2012; Patterson and Ruckstuhl, 2013). However, in species with high modularity, such as in social bathyergids, parasite transmission is greatly impeded and consequently the genetic population structure of parasites should be much more distinct and the effective population size smaller (Hawley et al., 2021; Figure 2D). In host species that exhibit organizational immunity these effects are further exacerbated as it constraints parasite transmission within groups (Cremer et al., 2018). This should also affect patterns of parasite aggregation (Figure 2D). Parasite populations are often characterized by a skewed distribution, also called overdispersion, with a small number of host individuals sustaining the majority of parasites (Woolhouse et al., 1997; Wilson et al., 2002). While such skews may be absent in host populations with frequent contact or close proximity between host individuals, it should be pronounced in hosts where inter-individual contact rates are low (Hawley et al., 2021). These effects are illustrated for bathyergid parasites by observations that group membership is a good predictor of parasite infection (Viljoen et al., 2011a; Lutermann et al., 2013; Archer et al., 2016). Evidence for similar effects at a colony level due to organizational immunity are less clear, but differences in parasite burden between breeders and non-breeders have been reported for some social bathyergids (Viljoen et al., 2011b; Lutermann et al., 2013; Archer et al., 2016). Overdispersion of parasites among hosts results in the aggregation of parasites on certain host individuals that in turn leads to increased competition between these parasites for host resources and greater variance in reproductive success of competing parasite individuals (Poulin, 2007; Hawley et al., 2021).
These effects of host behavior on parasite population structure and aggregation also affect selection on parasite virulence (Hawley et al., 2021; Schmid-Hempel, 2021). Reductions in host connectivity or increased modularity reduce transmission rates, conditions also favored by low between-group contacts and organizational immunity, select for greater parasite virulence (Ebert, 1998; Figures 2C,D). However, in host populations with distinct modularity high virulence effectively results in a depletion of hosts. This would negatively affect parasite fitness as observed in two incidences of viral infections of naked mole-rats in captivity (Ross-Gillespie et al., 2007; Artwohl et al., 2009). These fitness implications would exert selection pressures for the evolution of low virulence on parasites (Hawley et al., 2021), a scenario also applicable to social bathyergids. The fragmentation of parasite populations due to organizational immunity could furthermore lead to reductions in parasite genome size and possibly loss of functional abilities (Figure 2D) as observed in lineages that become commensals in eusocial insects (Leggett et al., 2013; Conlon et al., 2021). In highly modular social hosts dispersal constraints would also result in closely related parasite individuals exploiting hosts. Due to the inherent inclusive fitness benefits of such a scenario selection should favor decreased transmission rates and lowered virulence to reduce kin competition among such parasites (Hawley et al., 2021 and references therein). Thus, high modularity generated by low between-group contact rates (Figure 2C) as well as behavioral barriers to within-group transmission (Figure 2D) should lower the optima for both transmission and virulence for parasites exploiting hosts such as social Bathyergidae. At the same time, this can be expected to result in lower co-infection rates, further relaxing selection for virulence in co-infecting parasites (Hawley et al., 2021). In addition, the long life expectancy observed in social bathyergids and the related increased opportunities for transmission should lower selection pressures on parasite virulence (Bonds et al., 2005).
If social interactions of hosts are biased in favor of kin, parasites transmitted between such hosts exploit hosts with a similar genetic make-up. In singular breeding species with large group sizes (e.g., naked mole-rats) this does not only resemble the conditions experienced during the serial passage of parasites through the same host (Ebert, 1998), but it is also similar to those encountered by parasites in monocultures which facilitate transmission between hosts (Baer and Schmid-Hempel, 1999; Altermatt and Ebert, 2008; Ekroth et al., 2019). Ultimately, the high degree of relatedness between hosts, as observed within groups of social bathyergids, can favor the evolution of host specialization (Figure 2C) as successful transmission and proliferation in alternative hosts is no longer required (Kassen, 2002; Bono et al., 2017). In its extreme form such specialization can not only increase parasite fitness but can be a route for the evolution of benign symbionts (Hughes et al., 2008; Biedermann and Rohlfs, 2017; Figure 2D). Similarly, host predictability should facilitate host specialization in order to increase parasite fitness (Combes, 2001). This possibility has received limited attention in the literature to date (Hawley et al., 2021).
Selection pressures experienced by parasites will also differ with the immune strategy employed by the host; since host resistance reduces parasite fitness this strategy should result in selection for increased virulence in parasites. Empirical evidence of the benefits of sociality in the form of greater access to resources suggest that social species do not necessarily exhibit more investment in immunity thus relaxing selection for virulence in parasites (Almberg et al., 2015; Ezenwa and Snider, 2016). Both strategies might be equally employed by host individuals regardless of their social system and from an evolutionary perspective the mean and variance across a host population rather than individual differences are relevant for selection (Schmid-Hempel, 2021).
Based on the arguments laid out above, parasites of social mole-rats should experience population bottlenecks that reduce their species and genetic diversity while selecting for reductions in virulence, but possibly high transmission and host specialization (Figure 2). In fact, for bathyergids there is some evidence for such evolutionary forces having acted on mesostigmatid mites of the genus Androlaelaps. Although they are not necessarily restricted to social bathyergids, the majority of the species parasitizing Bathyergidae have not been reported for any other host family (Lutermann et al., 2019). Overall, it appears that selection pressures exerted by behavioral strategies employed by social bathyergids should be stronger than those posed by immune strategies as the latter are less likely to differ from those encountered in solitary hosts or those with multiple breeders.
The forces of selection acting on both hosts and parasites are also dependent on the mode of transmission, mobility and the type of life-cycle of a parasite with the former likely evolving in response to host defenses that also affect parasite virulence (Poulin, 2007; Antonovics et al., 2017; Hawley et al., 2021; Schmid-Hempel, 2021). The mode of transmission (i.e., method used by parasite) can be either vertical or horizontal. Horizontal transmission can further be distinguished into direct transmission via physical contact, airborne (i.e., micrcoparasites), or indirect, i.e., environmentally (e.g., contaminated food, soil or water) or vector-borne (Antonovics et al., 2017). For many parasites more than one mode of transmission may be used and the relative importance of each mode in a particular host-parasite system will be important for their evolution (Antonovics et al., 2017). Generally, parasites with a vertical mode of transmission decrease in virulence because only surviving offspring that reproduces can further transmit the parasite while those with horizontal mode of transmission increase in virulence (Ebert, 2013; Antonovics et al., 2017). However, the kin structure of social bathyergids makes distinctions between these two routes challenging. On the one hand, although vertical transmission is easily possible, the high reproductive skew in mole-rat societies means that the majority of parent-offspring transmissions will not be successful for parasites with obligate vertical transmission as these offspring will never breed and are thus unable to transmit the parasite to their progeny. At the same time, within a colony, transmission will mostly happen between colony members due to their close physical and genetic proximity (see details above). Although this is technically horizontal transmission, the distinction from vertical transmission is largely formal. In contrast, between-colony transmission is clearly horizontal but occurs much less frequently. Due, to low between-group contact rates, lack of a clear distinction between vertical and horizontal mode and the limited number of group members in the case of social bathyergid hosts, the evolution of virulence of parasites using a horizontal mode should be constrained for parasites (Hawley et al., 2021). This would be further exacerbated by organizational immunity that leads to modularity and hence, further constraints to transmission within bathyergid groups. Similarly, the monogamous mating strategy of social Bathyergidae is an effective measure against directly transmitted parasites during sexual contacts (Antonovics et al., 2017).
While horizontally transmitted parasites requiring direct contact between hosts, particularly virulent ones, should constrain the evolution of sociality the dilution effects provided by group members favor selection of sociality in the presence of mobile parasites that may also act as vectors for microparasites (Hart and Hart, 2018; Hawley et al., 2021). However, the subterranean environment effectively eliminates vector-borne microparasites relying on mobile vectors (e.g., mosquitoes) for bathyergids (Figure 2B). Consequently, selection on defenses against these types of parasites should be low in social bathyergids. While no flying vectors are known for Bathyergidae, a number of relatively mobile vectors such as fleas have been reported for several bathyergids (Lutermann et al., 2019; Fagir et al., 2021). Fleas can either be directly transmitted between hosts or via shared space use such as nests (Krasnov, 2008). Intriguingly, roughly half the flea species observed for bathyergids to date are host generalists and vectors of zoonotic pathogens while the other half appear to be host specialists that only exploit social bathyergids (Table 1). If opportunities for between-group transmission are limited, even for relatively mobile parasites, such specialization should be favored by selection. This should also remove opportunities for such vectors to contract microparasites. Thus, it may not be surprising that screenings for Bartonella spp., that are most likely vectored by fleas, have been negative for social common and Damaraland mole-rats but not solitary B. suillus (van Sandwyk, 2007). However, solitary G. capensis were also negative for these microparasites. Only two tick species, less mobile vectors with environmental transmission, have been reported for solitary Bathyergidae and both species appear to be host generalists parasitizing a range of small mammals (Horak et al., 2018; Lutermann et al., 2019; Table 2). Microparasites are the least studied parasites for Bathyergidae and natural infections have only been assessed for one fungal (Emmonsia parva) and two bacterial taxa (Bacillus cereus and Mycoplasma spp.). Of these Mycoplasma spp. could be vector-borne while the other two are environmentally transmitted via contamination of the soil (Hubálek et al., 2005; Retief et al., 2017, 2021). All of these microparasites appear to be host generalists. However, while prevalences were generally greater for social compared to one solitary hosts, this did not apply to B. suillus which had prevalences exceeding those of social species (C. h. hottentotus, F. damarensis) for both bacteria.
Table 1. Overview of insect parasite species, their mode of transmission and host range reported for the family Bathyergidae to date.
Table 2. Overview of acari parasite species, their mode of transmission and host range reported for the family Bathyergidae to date.
The most speciose ectoparasite taxon infecting Bathyergidae is represented by mites with at least 18 species (Table 2). For trombiculid mites (i.e., chiggers, genera: Austracarus, Euschöngastia, Gahrliepia, and Schoutedenichia) only the first instars are generalist parasites while all other stages are soil dwelling and consequently transmission is environmental (Shatrov and Kudryashova, 2006). Thus, it is not surprising that these parasites have been reported from a range of social bathyergids but only one solitary species as the larger burrow system of the former may increase their exposure to these mites. The remaining 14 mite species reported for bathyergids rely predominately on direct transmission and are often the most prevalent and abundant parasites reported for a host species (Viljoen et al., 2011b; Archer et al., 2014; Lutermann et al., 2015, 2019; Fagir et al., 2021). Only three of these species are known host generalists (Androlaelaps marshalli, Laelaps liberiensis and Orntihonyssus bacoti) while the remaining 11 species appear to be host specialists for Bathyergidae either at the family, genus or species level (Table 2; Lutermann et al., 2019). This suggests a potentially close co-evolutionary history between these mites and bathyergids that should help to shed light on the selection pressures experienced by both actors. Blood-sucking lice (Anoplura) are the most sedentary ectoparasite taxon of bathyergids and require direct contact between hosts for transmission (Kim, 2006). This is also why most louse species have a narrow host range, often using a single host species, which also appears to apply to the two species identified in Bathyergidae with one potentially specific to the host genus (Eulinognathus hilli) and another to the species (E. lawrensis) (Zumpt, 1966; Lutermann et al., 2019; Table 1). Thus, the patterns of host specialization across the ectoparasite communities described for bathyergids to date appears to support the hypothesis for a link between parasite transmission mode, host social behavior and parasite specialization. As laid out above this specialization should also include lowered virulence in these parasites and consequently, stronger selection pressures on behavioral rather than physiological immunity for social bathyergids.
The picture arising from the helminth community described for Bathyergidae is somewhat less clear. This is partially due to the lower taxonomic resolution with most parasites only being identified to genus level (Lutermann et al., 2019; Table 3). However, cestodes have complex life cycles that require an intermediate host (often an arthropod) in their life cycle (Georgiev et al., 2006). Hence, they need to adapt to both an invertebrate and vertebrate which makes host specialization much less likely as host encounters tend to depend mostly on stochastic processes (Antonovics et al., 2017). This could not only account for the limited number of cestode species observed in Bathyergidae to date, but would also make the evolution of specific behavioral or physiological responses less likely (Antonovics et al., 2017). At the same time, basic hygienic behaviors (e.g., grooming to reduce intermediate hosts such as mites or fleas) and organizational immunity in the form of dedicated toileting areas can be effective means to reduce the transmission of cestode propagules. Nematodes have a more diverse range of transmission modes including direct, environmental or transmission via an intermediate host, but this is often not well established for a species (Anderson, 2000). However, many of the genera observed to infect Bathyergidae are host generalists with a wide geographic distribution (Anderson, 2000; Table 3). Possible exceptions are four nematode species (Ortleppsrongylus bathyergid, Mammalakis macrospiculum, M. zambiensis, and Paralibyostrongylus bathyergid) that have to date only been found in bathyergids with three of them retrieved from B. suillus (Lutermann et al., 2019; Table 3). Helminths are famous for their ability to manipulate the immune responses of their hosts and may strongly interact with symbiotic microorganism (Maizels et al., 2004). Hence, they often do not trigger strong immune responses from their hosts, at the same time, they require host survival for successful proliferation. Consequently, they may favor tolerance responses in hosts.
Table 3. Overview of helminth parasite species, their mode of transmission or life-cycle and host range reported for the family Bathyergidae to date.
Rather than an individual parasites species the composition of parasite communities exploiting a host and the virulence of common parasites will determine selection on particular social behaviors as well as the extent to which such behaviors reduces the associated fitness costs (Hawley et al., 2021). This in turn will shape the evolutionary trajectories for the parasites involved. The majority of macroparasites exploiting Bathyergidae seem to fall into one of two broad categories: directly transmitted host specialists (at species, genus or family level) and indirectly (environmentally or via intermediate hosts) transmitted host generalists with the former occurring at the highest prevalences (Viljoen et al., 2011b; Lutermann et al., 2013, 2019; Archer et al., 2014, 2017; Fagir et al., 2021). Consequently, selection on the bathyergid host should be strongest on behavioral (e.g., hygienic behaviors, organizational immunity) instead of physiological control strategies while for those parasites selection will act to favor benign parasites with direct transmission.
Exploiting the subterranean niche has posed a number of challenges for Bathyergidae such as hypoxia, hypercapnia and foraging and dispersal constraints. However, the latter constraints likely have also limited the exposure and transmission to parasites which in turn should have made major contributions to the evolutionary steps of members of this family toward sociality and singular breeding. Living in sealed burrow system has reduced the exposure of bathyergids to mobile, environmentally and vector-transmitted parasites (Figure 2B). The resulting reductions in the prevalence, abundance, co-infection rate and parasite species diversity and hence, costs of parasitism should also have lowered the threshold for group-living. At the same time, the constant physical conditions and low generalized transmission distance within groups should favor directly transmitted arthropod parasites of bathyergids (Figure 2B). While social behaviors such as allo-grooming are effective in controlling such parasites the finite host size should induce evolution toward host specialization and lowered virulence in these parasites (Figure 2C). This can be facilitated further by organizational immunity including the reproductive division of labor observed in all social Bathyergidae (Figure 2D). This scenario also suggests that selection on behavioral defense strategies has been much stronger for Bathyergidae than that experienced on physiological defenses. Hence, venturing below ground and exploring soils that are difficult to work and regions of unpredictable rainfall have likely contributed to the evolution of extreme reproductive skew observed in naked and Damaraland mole-rats (Fukomys damarensis) that is unique among vertebrates with a social structure that bears resemblance to what can be found in eusocial insects. This is also illustrated by the stark contrast of social systems in bats that are not only extremely mobile and can occur in very large groups, but also harbor one of the most diverse assemblages of parasites among vertebrates (Luis et al., 2015; Han et al., 2016; Webber and Willis, 2016). However, although there might be some kin structure in bats, they do not exhibit singular breeding.
There are a number of hypotheses that require testing and research directions that can help to consolidate or revise the framework laid out here that I will outline below. Firstly, I anticipate that across the range of bathyergid species overall parasite burden (e.g., prevalence, abundance and/or diversity) should decrease from solitary to social species with increasing reproductive skew (i.e., increasing group size), but also with progressively more challenging soil properties and less predictable rainfall patterns. At a species level, similar patterns should be apparent between different geographic localities experiencing different climatic and soil conditions that affect between-colony contact rates. More specifically, the community composition of parasites can be expected to shift from more to less mobile parasites and reductions in vector-borne as well as environmentally-submitted parasites with increasing dispersal constraints (e.g., soil hardness, rainfall patterns) for the host. Specifically, low prevalences, abundance and species diversity of mobile and vector-borne parasites can be expected for social hosts in more arid localities or during periods of low rainfall and larger group sizes. At the same time, directly transmitted parasites such as relatively benign parasites, e.g. mites, could increase in species diversity. This is due to the genetic bottlenecks, they would experience but possibly also increases in prevalence and abundance due to hosts employing tolerance rather than resistance as defense strategy in larger groups.
Conversely, evidence for elements of organizational immunity should increase with increasing dispersal constraints and increasing group sizes both within and between bathyergid species. This is already evident in the degree of physiological suppression of reproduction of non-breeders (Faulkes and Bennett, 2021), but other aspects of organizational immunity need to be explored as well including but not limited to spatial and temporal segregation between colony members. The subterranean environment exploited by Bathyergidae hampers to some extend studies of behavioral mechanisms although technology can provide some remedy (Šklíba et al., 2016; Finn et al., 2022). Conversely, and despite their limitations, laboratory settings can allow for detailed behavioral observations. Such comparisons should be conducted at three levels: between bathyergid species that differ in group sizes (see Figure 1), across a range of colony sizes for each social species, as well as individual colonies that grow in size as they mature. At each of these levels the risk of social transmission and hence, the need for organizational immunity will change with group size and dispersal constraints. Importantly, a lack of evidence for organizational immunity beyond the reproductive division of labor does not inevitably disprove the role of parasites or the significance of organizational immunity as the presence of parasites may be required to induce such flexible behavioral responses (Nuotclà et al., 2019). Laboratory settings allow for experimental manipulations of parasite burdens and will thus be a good test of this hypothesis. Since the effectiveness of behavioral responses also depends on the mode of transmission such manipulations should be conducted with different parasite taxa.
Behavioral patterns such as contact rates can also be measured indirectly. Individually marked parasites can be used to track their transmission between group members and shed light on contact rates (Zohdy et al., 2012). The larger effective population size and shorter generation time of parasites also allow insights into host movements based on the genetic population structure of their parasites as well as co-speciation patterns between hosts and parasites (Hugot, 2006; Nieberding and Olivieri, 2007). Hence, studies of the population structure but also genome size of bathyergid parasites can provide insights into the interaction between these hosts and their parasites. Given their species diversity mites may prove particularly useful in this regard and mite species exploiting a large number of bathyergid species, such as Androlaelaps scapularis and Androlaelaps capensis, would allow for direct comparisons of population structure across a number of bathyergid species.
Virulence of parasite species can be tested by artificial infestation of individuals. For many of the bathyergid parasites this will, however, firstly require a better understanding of their life cycles. Nevertheless, infections with directly transmitted parasites (e.g., mites) should already be feasible and not only allow to assess their level of virulence but also the physiological defenses of their hosts. This can be carried out in isolated individuals and those in groups to evaluate the contributions of individual and social factors. By varying the resource availability (e.g., access to food) during such experimental manipulations one will furthermore be able to determine the role of resources and contributions of immunity vs. tolerance to host responses (Budischak and Cressler, 2018). Using different numbers or species of parasites (i.e., co-infection) can furthermore shed light on both the competitive ability of particular parasites and the resulting costs for hosts.
The assessments of the microbiome should be extended to a range of bathyergid species. Importantly, rather than simply providing inventories of the species composition they should be linked to group and individual characteristics that constitute once again a reflection of between and within-group contacts and possibly organizational immunity. Their potential role in mediating resistance or tolerance benefits can be evaluated by linking them to (natural or experimental) infection patterns. Lastly, the use of theoretical models using the biological parameters provided by such studies can allow the testing of assumptions and further specification of conditions that facilitated social evolution in bathyergids, similar to studies conducted for social insects (e.g., Udiani and Fefferman, 2020).
The scenario presented here is based on incomplete information as exhaustive and long-term parasite assessments (including those for microparasites) are lacking for many bathyergid species, most notably naked mole-rats. Also, more extensive behavioral observations are necessary and immunity and tolerance needs to be assessed in a greater range of species. Nevertheless, the scenario presented integrates knowledge on host and parasite biology to shed light on the relationships between bathyergids and their parasites and is the first to explore the role of the latter for social evolution in Bathyergidae. This illustrates how subterranean living and social structure can reduce the exposure to parasites and thus, substantially lower the costs associated with living in groups while the benefits of sociality such as better resource acquisition are apparent. In turn, bathyergid parasites have likely experienced substantial constraints to the evolution of virulence as well as experienced genetic bottlenecks that made them more benign. This will help to further shed light on social evolution in this unique family but also the role of social behaviors for parasite evolution.
The author confirms being the sole contributor of this work and has approved it for publication.
The author declares that the research was conducted in the absence of any commercial or financial relationships that could be construed as a potential conflict of interest.
All claims expressed in this article are solely those of the authors and do not necessarily represent those of their affiliated organizations, or those of the publisher, the editors and the reviewers. Any product that may be evaluated in this article, or claim that may be made by its manufacturer, is not guaranteed or endorsed by the publisher.
I thank Stan Braude, Sabine Begall, Susanne Holtze, and Nigel Bennett for the invitation to contribute to this Research Topic. I am indebted to Marietjie Froneman for permission to use her images and her invaluable help with Figure 1.
Adelman, J. S. (2010). “Immune systems and sickness behavior,” in Encyclopedia of Animal Behavior, eds M. D. Breed and J. Moore (Amsterdam: Elsevier), 133–137. doi: 10.1016/b978-0-08-045337-8.00263-1
Adelman, J. S., and Martin, L. B. (2009). Vertebrate sickness behaviors: adaptive and integrated neuroendocrine immune responses. Integr. Comp. Biol. 49, 202–214. doi: 10.1093/icb/icp028
Almberg, E. S., Cross, P. C., Dobson, A. P., Smith, D. W., Metz, M. C., Stahler, D. R., et al. (2015). Social living mitigates the costs of a chronic illness in a cooperative carnivore. Ecol. Lett. 18, 660–667. doi: 10.1111/ele.12444
Altermatt, F., and Ebert, D. (2008). Genetic diversity of Daphnia magna populations enhances resistance to parasites. Ecol. Lett. 11, 918–928. doi: 10.1111/j.1461-0248.2008.01203.x
Altizer, S., Dobson, A., Hosseini, P., Hudson, P., Pascual, M., and Rohani, P. (2006). Seasonality and the dynamics of infectious diseases. Ecol. Lett. 9, 467–484. doi: 10.1111/j.1461-0248.2005.00879.x
Altizer, S., Nunn, C. L., Thrall, P. H., Gittleman, J. L., Antonovics, J., Cunningham, A. A., et al. (2003). Social organization and parasite risk in mammals: integrating theory and empirical studies. Annu. Rev. Ecol. Evol. Syst. 34, 517–547. doi: 10.1146/annurev.ecolsys.34.030102.151725
Anderson, R. C. (2000). Nematode Parasites of Vertebrates: Their Development and Transmission, 2nd Edn. New York, NY: CABI Publishing.
Anderson, R. M., and May, R. M. (1982). Coevolution of hosts and parasites. Parasitology 85, 411–426. doi: 10.1017/s0031182000055360
Antonovics, J., Wilson, A. J., Forbes, M. R., Hauffe, H. C., Kallio, E. R., Leggett, H. C., et al. (2017). The evolution of transmission mode. Philos. Trans. R. Soc. B 372:20160083. doi: 10.1098/rstb.2016.0083
Archer, E. K., Bennett, N. C., Faulkes, C. G., and Lutermann, H. (2016). Digging for answers: contributions of frequency- and density-dependent mechanisms on ectoparasite burden in a social host. Oecologia 180, 429–438. doi: 10.1007/s00442-015-3494-0
Archer, E. K., Bennett, N. C., Junker, K., Faulkes, C. G., and Lutermann, H. (2017). The distribution of gastrointestinal parasites in two populations of common mole-rats (Cryptomys hottentotus hottentotus). J. Parasitol. 103, 786–790. doi: 10.1186/s12917-017-1175-4
Archer, E. K., Bennett, N. C., Ueckermann, E. A., and Lutermann, H. (2014). Ectoparasite burdens of the common mole-rat (Cryptomys hottentotus hottentotus) from the Cape Provinces of South Africa. J. Parasitol. 100, 79–84. doi: 10.1645/13-270.1
Artwohl, J., Ball-Kell, S., Valyi-Nagy, T., Wilson, S. P., Lu, Y., and Park, T. J. (2009). Extreme susceptibility of African naked mole rats (Heterocephalus glaber) to experimental infection with herpes simplex virus type 1. Comp. Med. 59, 83–90.
Baer, B., and Schmid-Hempel, P. (1999). Experimental variation in polyandry affects parasite loads and fitness in a bumble-bee. Nature 397, 151–154.
Bappert, M., Burda, H., and Begall, S. (2012). To mate or not to mate? Mate preference and fidelity in monogamous Ansell’s mole-rats, Fukomys anselli, Bathyergidae. Folia Zool. 61, 71–83. doi: 10.25225/fozo.v61.i1.a11.2012
Bartel, M. H., and Gardner, S. L. (2000). Arthropod and helminth parasites from the plains pocket gopher, Geomys bursarius bursarius from the hosts’ northern boundary range in Minnesota. J. Parasitol. 86, 153–156. doi: 10.1645/0022-33952000086
Baucom, R. S., and de Roode, J. C. (2011). Ecological immunology and tolerance in plants and animals. Funct. Ecol. 25, 18–28. doi: 10.1111/j.1365-2435.2010.01742.x
Behnke, J. M., Bajer, A., Sinski, E., and Wakelin, D. (2001). Interactions involving intestinal nematodes of rodents: experimental and field studies. Parasitology 122(Suppl.), S39–S49. doi: 10.1017/S0031182000016796
Bennett, N. C. (1989). The social structure and reproductive biology of the common mole-rat, Cryptomys h. hottentotus and remarks on the trends in reproduction and sociality in the family Bathyergidae. J. Zool. 219, 45–59. doi: 10.1111/j.1469-7998.1989.tb02564.x
Bennett, N. C., and Faulkes, C. G. (2000). African Mole-Rats: Ecology and Eusociality. Cambridge: Cambridge University Press.
Bennett, N. C., and Jarvis, J. U. M. (1988). The social structure and reproductive biology of colonies of the mole-rat Cryptomys damarensis. J. Mammal. 69, 293–302. doi: 10.2307/1381379
Best, A., Long, G., White, A., and Boots, M. (2012). The implications of immunopathology for parasite evolution. Proc. R. Soc. B 279, 3234–3240. doi: 10.1098/rspb.2012.0647
Best, A., White, A., and Boots, M. (2008). Maintenance of host variation in tolerance to pathogens and parasites. Proc. Natl. Acad. Sci. U.S.A. 105, 20786–20791. doi: 10.1073/pnas.0809558105
Biedermann, P. H., and Rohlfs, M. (2017). Evolutionary feedbacks between insect sociality and microbial management. Curr. Opin. Insect Sci. 22, 92–100. doi: 10.1016/j.cois.2017.06.003
Bishop, J. M., Jarvis, J. U. M., Spinks, A. C., Bennett, N. C., and O’Ryan, C. (2004). Molecular insight into patterns of colony composition and paternity in the common mole-rat Cryptomys hottentotus hottentotus. Mol. Ecol. 13, 1217–1229. doi: 10.1111/j.1365-294X.2004.02131.x
Blyton, M. D. J., Banks, S. C., Peakall, R., Lindenmayer, D. B., and Gordon, D. M. (2014). Not all types of host contacts are equal when it comes to E. coli transmission. Ecol. Lett. 17, 970–978. doi: 10.1111/ele.12300
Bonds, M. H., Keenan, D. C., Leidner, A. J., and Rohani, P. (2005). Higher disease prevalence can induce greater sociality: a game theoretic coevolutionary model. Evolution 59, 1859–1866. doi: 10.1554/05-028.1
Bono, L. M., Smith, L. B., Pfennig, D. W., and Burch, C. L. (2017). The emergence of performance trade-offs during local adaptation: insights from experimental evolution. Mol. Ecol. 26, 1720–1733. doi: 10.1111/mec.13979
Boots, M., Best, A., Miller, M. R., and White, A. (2009). The role of ecological feedbacks in the evolution of host defence: what does theory tell us? Philos. Trans. R. Soc. London B 364, 27–36. doi: 10.1098/rstb.2008.0160
Bordes, F., Ponlet, N., de Bellocq, J. G., Ribas, A., Krasnov, B. R., and Morand, S. (2012). Is there sex-biased resistance and tolerance in Mediterranean wood mouse (Apodemus sylvaticus) populations facing multiple helminth infections? Oecologia 170, 123–135. doi: 10.1007/s00442-012-2300-5
Boulinier, T., and Staszewski, V. (2008). Maternal transfer of antibodies: raising immuno-ecology issues. Trends Ecol. Evol. 23, 282–288. doi: 10.1016/j.tree.2007.12.006
Braude, S., Holtze, S., Begall, S., Brenmoehl, J., Burda, H., Dammann, P., et al. (2021). Surprisingly long survival of premature conclusions about naked mole-rat biology. Biol. Rev. 96, 376–393. doi: 10.1111/brv.12660
Budischak, S. A., and Cressler, C. E. (2018). Fueling defense: effects of resources on the ecology and evolution of tolerance to parasite infection. Front. Immunol. 9:2453. doi: 10.3389/fimmu.2018.02453
Buffenstein, R. (2000). “Ecophysiological responses of subterranean rodents to underground habitats,” in Life Underground: The Biology of Subterranean Rodents, eds E. A. Lacey, J. L. Patton, and G. N. Cameron (Chigago, IL: University of Chicago Press), 62–110. doi: 10.1152/ajpheart.00831.2013
Buffenstein, R., Amoroso, V., Andziak, B., Avdieiev, S., Azpurua, J., Barker, A. J., et al. (2022). The naked truth: a comprehensive clarification and classification of current ‘myths’ in naked mole-rat biology. Biol. Rev. 97. doi: 10.1111/brv.12791
Burland, T. M., Bennett, N. C., Jarvis, J. U. M., and Faulkes, C. G. (2004). Colony structure and parentage in wild colonies of co-operatively breeding Damaraland mole-rats suggest incest avoidance alone may not maintain reproductive skew. Mol. Ecol. 13, 2371–2379. doi: 10.1111/j.1365-294X.2004.02233.x
Cheng, H., Sebaa, R., Malholtra, N., Lacoste, B., El Hankouri, Z., Kirby, A., et al. (2021). Naked mole-rat brown fat thermogenesis is diminished during hypoxia through a rapid decrease in UCP1. Nat. Commun. 12:6801. doi: 10.1038/s41467-021-27170-2
Clarke, F. M., and Faulkes, C. G. (1999). Kin discrimination and female mate choice in the naked mole-rat Heterocephalus glaber. Proc. R. Soc. B 266, 1995–2002. doi: 10.1098/rspb.1999.0877
Combes, C. (2001). in Parasitism: The Ecology and Evolution of Intimate Interactions, ed. J. N. Thompson (Chicago, IL: University of Chicago Press).
Conlon, B. H., Gostinèar, C., Fricke, J., Kreuzenbeck, N. B., Daniel, J. M., Schlosser, M. S. L., et al. (2021). Genome reduction and relaxed selection is associated with the transition to symbiosis in the basidiomycete genus Podaxis. iScience 24:102680. doi: 10.1016/j.isci.2021.102680
Côté, I. M., and Poulin, R. (1995). Parasitism and group size in a social animals: a meta-analysis. Behav. Ecol. 6, 159–165. doi: 10.1093/beheco/6.2.159
Cox, F. E. (2001). Concomitant infections, parasites and immune responses. Parasitology 122, S23–S38. doi: 10.1017/S003118200001698X
Cremer, S., Armitage, S. A. O., and Schmid-Hempel, P. (2007). Social immunity. Curr. Biol. 17, R693–R702. doi: 10.1016/j.cub.2007.06.008
Cremer, S., Pull, C. D., and Fürst, M. A. (2018). Social immunity: emergence and evolution of colony-level disease protection. Annu. Rev. Entomol. 63, 105–123. doi: 10.1146/annurev-ento-020117-043110
Cutrera, A. P., Zenuto, R. R., and Lacey, E. A. (2014). Interpopulation differences in parasite load and variable selective pressures on MHC genes in Ctenomys talarum. J. Mammal. 95, 679–695. doi: 10.1644/13-MAMM-A-120
Dammann, P., and Burda, H. (2006). Sexual activity and reproduction delay ageing in a mammal. Curr. Biol. 16, R117–R118. doi: 10.1016/j.cub.2006.02.012
Dammann, P., Šumbera, R., Massmann, C., Scherag, A., and Burda, H. (2011). Extended longevity of reproductives appears to be common in Fukomys mole-rats (Rodentia, Bathyergidae). PLoS One 6:e18757. doi: 10.1371/journal.pone.0018757
Dawkins, R., and Krebs, J. R. (1979). Arms races between and within species. Proc. R. Soc. B 205, 489–511. doi: 10.1098/rspb.1979.0081
De Vries, J. L., Oosthuizen, M. K., Sichilima, A. M., and Bennett, N. C. (2008). Circadian rhythms of locomotor activity in Ansell’s mole-rat: are mole-rat’s clocks ticking? J. Zool. 276, 343–349. doi: 10.1111/j.1469-7998.2008.00496.x
Debebe, T., Biagi, E., Soverini, M., Holtze, S., Hildebrandt, T. B., Birkemeyer, C., et al. (2017). Unraveling the gut microbiome of the long-lived naked mole-rat. Sci. Rep. 7:9590. doi: 10.1038/s41598-017-10287-0
Downing, P. A., Griffin, A. S., and Cornwallis, C. K. (2021). Hard-working helpers contribute to long breeder lifespans in cooperative birds. Philos. Trans. R. Soc. B 376:20190742. doi: 10.1098/rstb.2019.0742
Drewe, J. A. (2010). Who infects whom? Social networks and tuberculosis transmission in wild meerkats. Proc. R. Soc. B 277, 633–642. doi: 10.1098/rspb.2009.1775
Ebert, D. (1998). Experimental evolution of parasites. Science 282, 1432–1436. doi: 10.1126/science.282.5393.1432
Ebert, D. (2013). The epidemiology and evolution of symbionts with mixed-mode transmission. Annu. Rev. Ecol. Evol. Syst. 44, 623–643. doi: 10.1146/annurev-ecolsys-032513-100555
Ekroth, A. K. E., Rafaluk-Mohr, C., and King, K. C. (2019). Host genetic diversity limits parasite success beyond agricultural systems: a meta-analysis. Proc. R. Soc. B 286:20191811. doi: 10.1098/rspb.2019.1811
Evans, J. C., Hodgson, D. J., Boogert, N. J., and Silk, M. J. (2021). Group size and modularity interact to shape the spread of infection and 1 information through animal societies. Behav. Ecol. Sociobiol. 75:163. doi: 10.1007/s00265-021-03102-4
Evans, J. C., Silk, M. J., Boogert, N. J., and Hodgson, D. J. (2020). Infected or informed? Social structure and the simultaneous transmission of information and infectious disease. Oikos 129, 1271–1288. doi: 10.1111/oik.07148
Ezenwa, V. O., and Snider, M. H. (2016). Reciprocal relationships between behaviour and parasites suggest that negative feedback may drive flexibility in male reproductive behaviour. Proc. R. Soc. B 283:20160423. doi: 10.1098/rspb.2016.0423
Ezenwa, V. O., Ghai, R. R., McKay, A. F., and Williams, A. E. (2016). Group living and pathogen infection revisited. Curr. Opin. Behav. Sci. 12, 66–72. doi: 10.1016/j.cobeha.2016.09.006
Fagir, D. M., Bennett, N. C., Ueckermann, E. A., Howard, A., and Hart, D. W. (2021). Ectoparasitic community of the Mahali mole-rat, Cryptomys hottentotus mahali: potential host for vectors of medical importance in South Africa. Parasites Vectors 14:24. doi: 10.1186/s13071-020-04537-w
Fagir, D. M., Horak, I. G., Ueckermann, E. A., Bennett, N. C., and Lutermann, H. (2015). Ectoparasite diversity in the eastern rock sengis (Elephantulus myurus): the effect of seasonality and host sex. Afr. Zool. 50, 109–117. doi: 10.1080/15627020.2015.1021173
Faulkes, C. G., and Bennett, N. C. (2021). “Social evolution in African mole-rats - a comparative overview,” in The Extraordinary Biology of the Naked Mole-Rat, eds R. Buffenstein, T. J. Park, and M. M. Holmes (Switzerland: Springer Nature), 157–195. doi: 10.1007/978-3-030-65943-1_1
Faulkes, C. G., Bennett, N. C., Bruford, M. W., O’Brien, H. P., Aguilar, G. H., and Jarvis, J. U. M. (1997). Ecological constraints drive social evolution in the African mole-rats. Proc. R. Soc. London B 264, 1619–1627. doi: 10.1098/rspb.1997.0226
Finn, K. T., Janse van Vuuren, A. K., Hart, D. W., Suess, T., Zottl, M., and Bennett, N. C. (2022). Seasonal changes in locomotor activity patterns of wild social Natal mole-rats (Cryptomys hottentotus natalensis). Front. Ecol. Evol. 10:819393. doi: 10.3389/fevo.2022.819393
Firman, R. C., Rubenstein, D. R., Moran, J. M., Rowe, K. C., and Buzatto, B. A. (2020). Extreme and variable climatic conditions drive the evolution of sociality in Australian rodents. Curr. Biol. 30, 691–697.e3. doi: 10.1016/j.cub.2019.12.012
Francioli, Y., Thorley, J., Finn, K., Clutton-Brock, T., and Zöttl, M. (2020). Breeders are less active foragers than non-breeders in wild Damaraland mole-rats: reduced foraging in mole-rat breeders. Biol. Lett. 16:20200475. doi: 10.1098/rsbl.2020.0475rsbl20200475
Freeland, W. J. (1976). Pathogens and the evolution of primate sociality. Biotropica 8, 12–24. doi: 10.2307/2387816
Freeland, W. J. (1979). Primate social groups as biological islands. Ecology 60, 719–728. doi: 10.2307/1936609
Ganem, G., and Bennett, N. C. (2004). Tolerance to unfamiliar conspecifics varies with social organization in female African mole-rats. Physiol. Behav. 82, 555–562. doi: 10.1016/j.physbeh.2004.05.002
Gaylard, A., Harrison, Y., and Bennett, N. C. (1998). Temporal changes in the social structure of a captive colony of the Damaraland mole-rat, Cryptomys damarensis: the relationship of sex and age to dominance and burrow-maintenance activity. J. Zool. 244, 313–321. doi: 10.1017/S095283699800301X
Georgiev, B. B., Bray, R. A., and Littlewood, D. T. J. (2006). “Cestodes of small mammals: taxonomy and life cycles,” in Micromammals and Macroparasites, from Evolutionary Ecology to Management, eds S. Morand, B. R. Krasnov, and R. Poulin (Tokyo: Springer Verlag), 29–62. doi: 10.1007/978-4-431-36025-4_3
Griffin, R. H., and Nunn, C. L. (2012). Community structure and the spread of infectious disease in primate social networks. Evol. Ecol. 26, 779–800. doi: 10.1007/s10682-011-9526-2
Habig, B., and Archie, E. A. (2015). Social status, immune response and parasitism in males: a meta-analysis. Philos. Trans. R. Soc. B 370:20140109. doi: 10.1098/rstb.2014.0109
Habig, B., Doellman, M. M., Woods, K., Olansen, J., and Archie, E. A. (2018). Social status and parasitism in male and female vertebrates: a meta- analysis. Sci. Rep. 8:3629. doi: 10.1038/s41598-018-21994-7
Han, B. A., Kramer, A. M., and Drake, J. M. (2016). Global patterns of zoonotic disease in mammals. Trends Parasitol. 32, 565–577. doi: 10.1016/j.pt.2016.04.007
Hart, B. L. (1988). Biological basis of the behavior of sick animals. Neurosci. Biobehav. Rev. 12, 123–137. doi: 10.1016/s0149-7634(88)80004-6
Hart, B. L., and Hart, L. A. (2018). How mammals stay healthy in nature: the evolution of behaviours to avoid parasites and pathogens. Philos. Trans. R. Soc. B 373:20170205. doi: 10.1098/rstb.2017.0205
Hart, L., Bennett, N. C., Malpaux, B., Chimimba, C. T., and Oosthuizen, M. K. (2004). The chronobiology of the Natal mole-rat, Cryptomys hottentotus natalensis. Physiol. Behav. 82, 563–569. doi: 10.1016/j.physbeh.2004.05.008
Hawley, D. M., Gibson, A. K., Townsend, A. K., Craft, M. E., and Stephenson, J. F. (2021). Bidirectional interactions between host social behaviour and parasites arise through ecological and evolutionary processes. Parasitology 148, 274–288. doi: 10.1017/S0031182020002048
Hemmes, R. B., Alvarado, A., and Hart, B. L. (2002). Use of California bay foliage by wood rats for possible fumigation of nest-borne ectoparasites. Behav. Ecol. 13, 381–385. doi: 10.1093/beheco/13.3.381
Hoban, S., Bruford, M., D’Urban Jackson, J., Lopes-Fernandes, M., Heuertz, M., Hohenlohe, P. A., et al. (2020). Genetic diversity targets and indicators in the CBD post-2020 Global Biodiversity Framework must be improved. Biol. Conserv. 248:108654. doi: 10.1016/j.biocon.2020.108654
Hoffmann, S., Horak, I. G., Bennett, N. C., and Lutermann, H. (2016). Evidence for interspecific interactions in the ectoparasite infracommunity of a wild mammal. Parasit. Vectors 9:58. doi: 10.1186/s13071-016-1342-7
Horak, I. G., Heyne, H., Williams, R., Gallivan, G. J., Spickett, A. M., Bezuidenhout, J. M., et al. (2018). The Ixodid Ticks (Acari: Ixodidae) of Southern Africa. Cham: Springer Nature.
Hubálek, Z., Burda, H., Scharff, A., Heth, G., Nevo, E., Šumbera, R., et al. (2005). Emmonsiosis of subterranean rodents (Bathyergidae, Spalacidae) in Africa and Israel. Med. Mycol. 43, 691–697. doi: 10.1080/13693780500179553
Hughes, D. P., Pierce, N. E., and Boomsma, J. J. (2008). Social insect symbionts: evolution in homeostatic fortresses. Trends Ecol. Evol. 23, 672–677. doi: 10.1016/j.tree.2008.07.011
Hugot, J.-P. (2006). “Coevolution of macroparasites and their small mammalian hosts: cophylogeny and coadaptation,” in Micromammals and Macroparasites - From Evolutionary Ecology to Management, eds S. Morand, B. R. Krasnov, and R. Poulin (Tokyo: Springer Verlag), 257–276. doi: 10.1007/978-4-431-36025-4_14
Imrit, M. A., Dogantzis, K. A., Harpur, B. A., and Zayed, A. (2020). Eusociality influences the strength of negative selection on insect genomes: negative selection in social genomes. Proc. R. Soc. B Biol. Sci. 287:20201512. doi: 10.1098/rspb.2020.1512rspb20201512
Ingram, C. M., Troendle, N. J., Gill, C. A., Braude, S., and Honeycutt, R. L. (2015). Challenging the inbreeding hypothesis in a eusocial mammal: population genetics of the naked mole-rat, Heterocephalus glaber. Mol. Ecol. 24, 4848–4865. doi: 10.1111/mec.13358
Iorizzo, M., Pannella, G., Lombardi, S. J., Ganassi, S., Testa, B., Succi, M., et al. (2020). Inter-and intra-species diversity of lactic acid bacteria in Apis mellifera ligustica colonies. Microorganisms 8:1578. doi: 10.3390/microorganisms8101578
Ivy, C. M., Sprenger, R. J., Bennett, N. C., van Jaarsveld, B., Hart, D. W., Kirby, A. M., et al. (2020). The hypoxia tolerance of eight related African mole-rat species rivals that of naked mole-rats, despite divergent ventilatory and metabolic strategies in severe hypoxia. Acta Physiol. 228:e13436. doi: 10.1111/apha.13436
Jacobs, P. J., Hart, D. W., and Bennett, N. C. (2021a). Plasma oxidative stress in reproduction of two eusocial African mole-rat species, the naked mole-rat and the Damaraland mole-rat. Front. Zool. 18:45. doi: 10.1186/s12983-021-00430-z
Jacobs, P. J., Hart, D. W., Suess, T., Van Vuuren, A. K. J., and Bennett, N. C. (2021b). The cost of reproduction in a cooperatively breeding mammal: consequences of seasonal variation in rainfall, reproduction, and reproductive suppression. Front. Physiol. 12:780490. doi: 10.3389/fphys.2021.780490
Jarvis, J. U. M. (1981). Eusociality in a mammal: cooperative breeding in naked mole-rat colonies. Science 212, 571–573. doi: 10.1126/science.7209555
Jarvis, J. U. M., and Bennett, N. C. (1993). Eusociality has evolved independently in two genera of bathyergid mole-rats - but occurs in no other subterranean mammal. Behav. Ecol. Sociobiol. 33, 253–260. doi: 10.1007/bf02027122
Jarvis, J. U. M., and Sale, J. B. (1971). Burrowing and burrow paterns of East African mole-rats Tachyoryctes, Heliophobius and Heterocephalus. J. Zool. London 163, 451–479. doi: 10.1111/j.1469-7998.1971.tb04544.x
Jarvis, J. U. M., Bennett, N. C., and Spinks, A. C. (1998). Food availability and foraging by wild colonies of Damaraland mole-rats (Cryptomys damarensis): implications for sociality. Oecologia 113, 290–298. doi: 10.1007/s004420050380
Jarvis, J. U. M., O’Riain, M. J., Bennett, N. C., and Sherman, P. W. (1994). Mammalian eusociality: a family affair. Trends Ecol. Evol. 9, 47–51. doi: 10.1016/0169-5347(94)90267-4
Jetz, W., and Rubenstein, D. R. (2011). Environmental uncertainty and the global biogeography of cooperative breeding in birds. Curr. Biol. 21, 72–78. doi: 10.1016/j.cub.2010.11.075
Junker, K., Lutermann, H., and Mutafchiev, Y. (2017). A new ascaridid nematode, Mammalakis zambiensis n. sp. (Heterakoidea: Kiwinematidae), from the mole rat Fukomys anselli (Burda, Zima, Scharff, Macholán & Kawalika) (Rodentia: Bathyergidae) in Zambia. Syst. Parasitol. 94, 557–566. doi: 10.1007/s11230-017-9721-9
Kassen, R. (2002). The experimental evolution of specialists, generalists, and the maintenance of diversity. J. Evol. Biol. 15, 173–190. doi: 10.1046/j.1420-9101.2002.00377.x
Kavaliers, M., and Colwell, D. D. (1992). Exposure to the scent of male mice infected with the protozoan parasite, Eimeria vermiformis, induces opioid-and nonopioid-mediated analgesia in female mice. Physiol. Behav. 52, 373–377. doi: 10.1016/0031-9384(92)90286-b
Kavaliers, M., and Colwell, D. D. (1994). Parasite infection attenuates nonopioid mediated predator-induced analgesia in mice. Physiol. Behav. 55, 505–510. doi: 10.1016/0031-9384(94)90108-2
Kennedy, A., Herman, J., and Rueppell, O. (2021). Reproductive activation in honeybee (Apis mellifera) workers protects against abiotic and biotic stress. Philos. Trans. R. Soc. B 376:20190737. doi: 10.1098/rstb.2019.0737
Kim, K. C. (2006). “Blood-sucking lice (Anoplura) of small mammals: True parasites,” in Micromammals and Macroparasites - From Evolutionary Ecology to Management, eds S. Morand, B. R. Krasnov, and R. Poulin (Tokyo: Springer Verlag), 141–160. doi: 10.1007/978-4-431-36025-4_9
Knowles, S. C. L., Fenton, A., Petchey, O. L., Jones, T. R., Barber, R., Amy, B., et al. (2013). Stability of within-host-parasite communities in a wild mammal system. Proc. R. Soc. B 280:20130598. doi: 10.1098/rspb.2013.0598
Korb, J., and Heinze, J. (2021). Ageing and sociality: why, when and how does sociality change ageing patterns? Philos. Trans. R. Soc. B Biol. Sci. 376:20190727. doi: 10.1098/rstb.2019.0727
Krasnov, B. R. (2008). Functional and Evolutionary Ecology of Fleas. Cambridge: Cambridge University Press.
Kreisinger, J., Bastien, G., Hauffe, H. C., Marchesi, J., and Perkins, S. E. (2015). Interactions between multiple helminths and the gut microbiota in wild rodents. Philos. Trans. R. Soc. B Biol. Sci. 370:20140295. doi: 10.1098/rstb.2014.0295
Kundu, S., and Faulkes, C. G. (2004). Patterns of MHC selection in African mole-rats, family Bathyergidae: the effects of sociality and habitat. Proc. R. Soc. B 271, 273–278. doi: 10.1098/rspb.2003.2584
Lacey, E. A., and Sherman, P. W. (1991). “Social organization of naked mole-rat colonies: evidence for divisions of labor,” in The Biology of the Naked Mole-Rat, eds P. W. Sherman, J. U. M. Jarvis, and R. D. Alexander (Princeton, NJ: Princeton Universtiy Press), 275–336. doi: 10.1515/9781400887132-013
Le Comber, S. C. (2006). Burrow fractal dimension and foraging success in subterranean rodents: a simulation. Behav. Ecol. 17, 188–195. doi: 10.1093/beheco/arj011
Le Comber, S. C., Spinks, A. C., Bennett, N. C., Jarvis, J. U. M., and Faulkes, C. G. (2002). Fractal dimension of African mole-rat burrows. Can. J. Zool. 441, 436–441. doi: 10.1139/Z02-026
Leclaire, S., Nielsen, J. F., and Drea, C. M. (2014). Bacterial communities in meerkat anal scent secretions vary with host sex, age, and group membership. Behav. Ecol. 25, 996–1004. doi: 10.1093/beheco/aru074
Ledger, J. A. (1980). The Arthropod Parasites of Vertebrates in Africa South of the Sahara. Volume IV. Phthiraptera (Insecta). Johannesburg: South African Institute for Medical Research.
Lee, K. A. (2006). Linking immune defenses and life history at the levels of the individual and the species. Integr. Comp. Biol. 46, 1000–1015. doi: 10.1093/icb/icl049
Leggett, H. C., Buckling, A., Long, G. H., and Boots, M. (2013). Generalism and the evolution of parasite virulence. Trends Ecol. Evol. 28, 592–596. doi: 10.1016/j.tree.2013.07.002
Leung, J. M., Graham, A. L., and Knowles, S. C. L. (2018). Parasite-microbiota interactions with the vertebrate gut: synthesis through an ecological lens. Front. Microbiol. 9:843. doi: 10.3389/fmicb.2018.00843
Lewis, K. N., and Buffenstein, R. (2016). “The naked mole-rat: a resilieint rodent model of aging, longevity and healthspan,” in Handbook of the Biology of Aging, eds M. R. Kaeberlein and G. M. Martin (Amsterdam: Academic Press), 179–204. doi: 10.1016/B978-0-12-411596-5.00006-X
Lin, T., and Buffenstein, R. (2021). “The unusual immune system of the naked mole-rat,” in The Extraordinary Biology of the Naked Mole-Rat, eds R. Buffenstein, T. J. Park, and M. M. Holmes (Switzerland: Springer Nature), 315–327. doi: 10.1007/978-3-030-65943-1_12
Lochmiller, R. L., and Deerenberg, C. (2000). Trade-offs in evolutionary immunology: just what is the cost of immunity? Oikos 88, 87–98. doi: 10.1034/j.1600-0706.2000.880110.x
Logan, S. M., Szereszewski, K. E., Bennett, N. C., Hart, D. W., van Jaarsveld, B., Pamenter, M. E., et al. (2020). The brains of six African mole-rat species show divergent responses to hypoxia. J. Exp. Biol. 223:jeb215905. doi: 10.1242/jeb.215905
Lovegrove, B. G. (1988). Colony size and structure, activity patterns and foraging behaviour of a colony of the social mole-rat Cryptomys damarensis (Bathyergidae). J. Zool. 216, 391–402. doi: 10.1111/j.1469-7998.1988.tb02437.x
Lovegrove, B. G., and Knight-Eloff, A. (1988). Soil and burrow temperatures, and the resource characteristics of the social mole-rat Cryptomys damarensis (Bathyergidae) in the Kalahari desert. J. Zool. 216, 403–416. doi: 10.1111/j.1469-7998.1988.tb02438.x
Lövy, M., Šklíba, J., and Šumbera, R. (2013). Spatial and temporal activity patterns of the free-living giant mole-rat (Fukomys mechowii), the largest social bathyergid. PLoS One 8:e55357. doi: 10.1371/journal.pone.0055357
Lövy, M., Šklíba, J., Burda, H., Chitaukali, W. N., and Šumbera, R. (2012). Ecological characteristics in habitats of two African mole-rat species with different social systems in an area of sympatry?: implications for the mole-rat social evolution. J. Zool. 286, 145–153. doi: 10.1111/j.1469-7998.2011.00860.x
Lucas, E. R., and Keller, L. (2020). The co-evolution of longevity and social life. Funct. Ecol. 34, 76–87. doi: 10.1111/1365-2435.13445
Lucatelli, J., Mariano-Neto, E., and Japyassú, H. F. (2021). Social interaction, and not group size, predicts parasite burden in mammals. Evol. Ecol. 35, 115–130. doi: 10.1007/s10682-020-10086-6
Luis, A. D., O’Shea, T. J., Hayman, D. T. S., Wood, J. L. N., Cunningham, A. A., Gilbert, A. T., et al. (2015). Network analysis of host-virus communities in bats and rodents reveals determinants of cross-species transmission. Ecol. Lett. 18, 1153–1162. doi: 10.1111/ele.12491
Lukas, D., and Clutton-Brock, T. (2012a). Cooperative breeding and monogamy in mammalian societies. Proc. R. Soc. B 279, 2151–2156. doi: 10.1098/rspb.2011.2468
Lukas, D., and Clutton-Brock, T. (2012b). Life histories and the evolution of cooperative breeding in mammals. Proc. R. Soc. B 279, 4065–4070. doi: 10.1098/rspb.2012.1433
Lukas, D., and Clutton-Brock, T. (2017). Climate and the distribution of cooperative breeding in mammals. R. Soc. Open Sci. 4:160897. doi: 10.1098/rsos.160897
Lukas, D., and Clutton-Brock, T. H. (2013). The evolution of social monogamy in mammals. Science 341, 526–530. doi: 10.1126/science.1238677
Lutermann, H., and Bennett, N. C. (2008). Strong immune function: a benefit promoting the evolution of sociality? J. Zool. 275, 26–32. doi: 10.1111/j.1469-7998.2007.00403.x
Lutermann, H., and Bennett, N. C. (2012). Determinants of helminth infection in a subterranean rodent, the Cape dune mole-rat (Bathyergus suillus). J. Parasitol. 98, 686–689. doi: 10.1645/GE-3024.1
Lutermann, H., Archer, E. K., Ueckermann, E. A., Junker, K., and Bennett, N. C. (2019). Surveys and literature review of parasites among African mole-rats: proposing hypotheses for the roles of geography, ecology, and host phylogenetic relatedness in parasite sharing. J. Parasitol. 106, 38–45. doi: 10.1645/GE-3119.1
Lutermann, H., Bennett, N. C., Speakman, J. R., and Scantlebury, M. (2013). Energetic benefits of sociality offset the costs of parasitism in a cooperative mammal. PLoS One 8:e57969. doi: 10.1371/journal.pone.0057969
Lutermann, H., Bodenstein, C., and Bennett, N. C. (2012). Natural parasite infection affects the tolerance but not the response to a simulated secondary parasite infection. PLoS One 7:e52077. doi: 10.1371/journal.pone.0052077
Lutermann, H., Butler, K. B., and Bennett, N. C. (2022). Parasite-mediated mate preferences in a cooperatively breeding rodent. Front. Ecol. Evol. 10:838076. doi: 10.3389/fevo.2022.838076
Lutermann, H., Carpenter-Kling, T., Ueckermann, E. A., Gutjahr, G. H., and Bennett, N. C. (2015). Ectoparasite burdens of the Damaraland mole-rat (Fukomys damarensis) from Southern Africa. J. Parasitol. 101, 666–671. doi: 10.1645/15-775
Lutermann, H., Haukisalmi, V., and Junker, K. (2018). First report of gastrointestinal parasites from Ansell’s mole-rat (Fukomys anselli) in Zambia. J. Parasitol. 104, 566–570. doi: 10.1645/17-123
Mabbott, N. A. (2018). The influence of parasite infections on host immunity to co-infection with other pathogens. Front. Immunol. 9:2579. doi: 10.3389/fimmu.2018.02579
Maizels, R. M., Balic, A., Gomez-Escobar, N., Nair, M., Taylor, M. D., and Allen, J. E. (2004). Helminth parasites – masters of regulation. Immunol. Rev. 201, 89–116. doi: 10.1111/j.0105-2896.2004.00191.x
Martin, L. B. (2009). Stress and immunity in wild vertebrates: timing is everything. Gen. Comp. Endocrinol. 163, 70–76. doi: 10.1016/j.ygcen.2009.03.008
Martin, L. B., Weil, Z. M., and Nelson, R. J. (2008). Seasonal changes in vertebrate immune activity: mediation by physiological trade-offs. Philos. Trans. R. Soc. London 363, 321–339. doi: 10.1098/rstb.2007.2142
May, R. M., and Anderson, R. M. (1978). Regulation and stability of host-parasite population interactions: II. destabilizing processes. J. Anim. Ecol. 47, 249–267. doi: 10.2307/3934
Medzhitov, R., Schneider, D. S., and Soares, M. P. (2012). Disease tolerance as a defense strategy. Science 335, 936–941. doi: 10.1126/science.1214935
Mendonça, R., Vullioud, P., Katlein, N., Vallat, A., Glauser, G., Bennett, N. C., et al. (2020). Oxidative costs of cooperation in cooperatively breeding Damaraland mole-rats. Proc. R. Soc. B Biol. Sci. 287:20201023. doi: 10.1098/rspb.2020.1023
Meunier, J. (2015). Social immunity and the evolution of group living in insects. Philos. Trans. R. Soc. B Biol. Sci. 370:20140102. doi: 10.1098/rstb.2014.0102
Milutinović, B., Stock, M., Grasse, A. V., Naderlinger, E., Hilbe, C., and Cremer, S. (2020). Social immunity modulates competition between coinfecting pathogens. Ecol. Lett. 23, 565–574. doi: 10.1111/ele.13458
Moolman, M., Bennett, N. C., and Schoeman, A. S. (1998). The social structure and dominance hierarchy of the highveld mole-rat Cryptomys hottentotus pretoriae (Rodentia: Bathyergidae). J. Zool. 246, 193–201. doi: 10.1111/j.1469-7998.1998.tb00148.x
Mooring, M. S., Blumstein, D. T., and Stoner, C. J. (2004). The evolution of parasite-defence grooming in ungulates. Biol. J. Linn. Soc. 81, 17–37. doi: 10.1111/j.1095-8312.2004.00273.x
Münger, E., Montiel-Castro, A. J., Langhans, W., and Pacheco-López, G. (2018). Reciprocal interactions between gut microbiota and host social behavior. Front. Integr. Neurosci. 12:21. doi: 10.3389/fnint.2018.00021
Naug, D., and Camazine, S. (2002). The role of colony organization on pathogen transmission in social insects. J. Theor. Biol. 215, 427–439. doi: 10.1006/jtbi.2001.2524
Neco, L. C., Abelson, E. S., Brown, A., Natterson-Horowitz, B., and Blumstein, D. T. (2019). The evolution of self-medication behaviour in mammals. Biol. J. Linn. Soc. 128, 373–378. doi: 10.1093/biolinnean/blz117
Nieberding, C. M., and Olivieri, I. (2007). Parasites: proxies for host genealogy and ecology? Trends Ecol. Evol. 22, 156–165. doi: 10.1016/j.tree.2006.11.012
Nunn, C. L., Jordan, F., McCabe, C. M., Verdolin, J. L., and Fewell, J. H. (2015). Infectious disease and group size: more than just a numbers game. Philos. Trans. R. Soc. B 370:20140111. doi: 10.1098/rstb.2014.0111
Nuotclà, J. A., Biedermann, P. H. W., and Taborsky, M. (2019). Pathogen defence is a potential driver of social evolution in ambrosia beetles. Proc. R. Soc. B 286:20192332. doi: 10.1098/rspb.2019.2332
Oosthuizen, M. K., Cooper, H. M., and Bennett, N. C. (2003). Circadian rhythms of locomotor activity in solitary and social species of African mole-rats (Family: Bathyergidae). J. Biol. Rhythms 18, 481–490. doi: 10.1177/0748730403259109
Oosthuizen, M. K., and Bennett, N.C. (2022). Clocks ticking in the dark: A review of biological rhythms in subterranean African mole-rats. Front. Ecol. Evol. 10:878533. doi: 10.3389/fevo.2022.878533
Patterson, J. E. H., and Ruckstuhl, K. E. (2013). Parasite infection and host group size: a meta-analytical review. Parasitology 140, 803–813. doi: 10.1017/S0031182012002259
Patzenhauerová, H., Sklíba, J., Bryja, J., and Šumbera, R. (2013). Parentage analysis of Ansell’ s mole-rat family groups indicates a high reproductive skew despite relatively relaxed ecological constraints on dispersal. Mol. Ecol. 22, 4988–5000. doi: 10.1111/mec.12434
Peachey, L. E., Jenkins, T. P., and Cantacessi, C. (2017). This gut ain’t big enough for both of us. Or is it? Helminth-microbiota interactions in veterinary species. Trends Parasitol. 33, 619–633. doi: 10.1016/j.pt.2017.04.004
Pedersen, A. B., and Antonovics, J. (2013). Anthelmintic treatment alters the parasite community in a wild mouse host. Biol. Lett. 9:20130205. doi: 10.1098/rsbl.2013.0205
Pedersen, A. B., and Fenton, A. (2007). Emphasizing the ecology in parasite community ecology. Trends Ecol. Evol. 22, 133–139. doi: 10.1016/j.tree.2006.11.005
Powell, J. E., Martinson, V. G., Urban-Mead, K., and Moran, N. A. (2014). Routes of acquisition of the gut microbiota of the honey bee Apis mellifera. Appl. Environ. Microbiol. 80, 7378–7387. doi: 10.1128/AEM.01861-14
Quque, M., Villette, C., Criscuolo, F., Sueur, C., Bertile, F., and Heintz, D. (2021). Eusociality is linked to caste-specific differences in metabolism, immune system, and somatic maintenance-related processes in an ant species. Cell. Mol. Life Sci. 79:29. doi: 10.1007/s00018-021-04024-0
Råberg, L., Graham, A. L., and Read, A. F. (2009). Decomposing health: tolerance and resistance to parasites in animals. Philos. Trans. R. Soc. B Biol. Sci. 364, 37–49. doi: 10.1098/rstb.2008.0184
Retief, L., Bennett, N. C., and Bastos, A. D. S. (2021). Molecular detection and characterization of novel haemotropic Mycoplasma in free-living mole rats from South Africa. Infect. Genet. Evol. 89:104739. doi: 10.1016/j.meegid.2021.104739
Retief, L., Bennett, N. C., Jarvis, J. U. M., and Bastos, A. D. S. (2017). Subterranean mammals: reservoirs of infection or overlooked sentinels of anthropogenic environmental soiling? Ecohealth 14, 662–674. doi: 10.1007/s10393-017-1281-6
Riain, M. J. O., Jarvis, J. U. M., and Town, C. (1997). Colony member recognition and xenophobia in the naked mole-rat. Anim. Behav. 53, 487–498. doi: 10.1006/anbe.1996.0299
Rifkin, J. L., Nunn, C. L., and Garamszegi, L. Z. (2012). Do animals living in larger groups experience greater parasitism? A meta-analysis. Am. Nat. 180, 70–82. doi: 10.1086/666081
Romanach, S. S. (2005). Influences of sociality and habitat on African mole-rat burrowing patterns. Can. J. Zool. 83, 1051–1058. doi: 10.1139/z05-099
Roper, T. J., Jackson, T. P., Conradtà, L., and Bennett, N. C. (2002). Burrow use and the influence of ectoparasites in Brants’ whistling rat Parotomys brantsii. Ethology 564, 557–564. doi: 10.1046/j.1439-0310.2002.00794.x
Ross-Gillespie, A., O’Riain, M. J., and Keller, L. F. (2007). Viral epizootic reveals inbreeding depression in an habitually inbreeding mammal. Evolution 61, 2268–2273. doi: 10.1111/j.1558-5646.2007.00177.x
Rossin, A., and Malizia, A. (2002). Relationship between helminth parasites and demographic attributes of a population of the subterranean rodent Ctenomys talarum (Rodentia: Octodontidae). J. Parasitol. 88, 1268–1270. doi: 10.1645/0022-3395(2002)088[1268:RBHPAD]2.0.CO;2
Rossin, M. A., Malizia, A. I., Timi, J. T., and Poulin, R. (2010). Parasitism underground: determinants of helminth infections in two species of subterranean rodents (Octodontidae). Parasitology 137, 1569–1575. doi: 10.1017/S0031182010000351
Roth, O., Beemelmanns, A., Barribeau, S. M., and Sadd, B. M. (2018). Recent advances in vertebrate and invertebrate transgenerational immunity in the light of ecology and evolution. Heredity 121, 225–238. doi: 10.1038/s41437-018-0101-2
Sah, P., Mann, J., and Bansal, S. (2018). Disease implications of animal social network structure: a synthesis across social systems. J. Anim. Ecol. 87, 546–558. doi: 10.1111/1365-2656.12786
Scharff, A., Burda, H., Tenora, F., Kawalika, M., and Barus, V. (1997). Parasites in social subterranean Zambian mole-rats (Cryptomys ssp., Bathyergidae, Rodentia). J. Zool. 241, 571–577. doi: 10.1111/j.1469-7998.1997.tb04848.x
Scharff, A., Tenora, F., Kawalika, M., Barus, V., and Burda, H. (1996). Helminths from Zambian mole-rats (Cryptomys, Bathyergidae, Rodentia). Helminthologia 33, 105–110.
Schielke, C. K. M., Begall, S., and Burda, H. (2012). Reproductive state does not influence activity budgets of eusocial Ansell’s mole-rats, Fukomys anselli (Rodentia, Bathyergidae): a study of locomotor activity by means of RFID. Mamm. Biol. 77, 1–5. doi: 10.1016/j.mambio.2011.09.004
Schmid-Hempel, P. (2021). Sociality and parasite transmission. Behav. Ecol. Sociobiol. 75:156. doi: 10.1007/s00265-021-03092-3
Schmidt, C. M., Jarvis, J. U. M., and Bennett, N. C. (2013). The long-lived queen: reproduction and longevity in female eusocial Damaraland mole-rats. Afr. Zool. 48, 193–196. doi: 10.1080/15627020.2013.11407583
Schulenburg, H., Kurtz, J., Moret, Y., and Siva-Jothy, M. T. (2009). Introduction. Ecological immunology. Philos. Trans. R. Soc. London B 364, 3–14. doi: 10.1098/rstb.2008.0249
Schülke, S., Dreidax, D., Malik, A., Burmester, T., Nevo, E., Band, M., et al. (2012). Living with stress: Regulation of antioxidant defense genes in the subterranean, hypoxia-tolerant mole rat, Spalax. Gene 500, 199–206. doi: 10.1016/j.gene.2012.03.019
Sclocco, A., and Teseo, S. (2020). Microbial associates and social behavior in ants. Artif. Life Robot. 25, 552–560. doi: 10.1007/s10015-020-00645-z
Segerman, J. (1995). Siphonaptera of Southern Africa: A Handbook for the Identification of Fleas. Johannesburg: South African Institute for Medical Research.
Shatrov, A. B., and Kudryashova, N. I. (2006). “Taxonomy, life cycles and the origin of parasitism in trombiculid mites,” in Micromammals and Macroparasites - From Evolutionary Ecology to Management, eds S. Morand, B. R. Krasnov, and R. Poulin (Tokyo: Springer Verlag), 119–140. doi: 10.1007/978-4-431-36025-4_8
Shen, S., Emlen, S. T., Koenig, W. D., and Rubenstein, D. R. (2017). The ecology of cooperative breeding behavior. Ecol. Lett. 20, 708–720. doi: 10.1111/ele.12774
Sichilima, A. M., Bennett, N. C., Faulkes, C. G., and Le Comber, S. C. (2008). Evolution of African mole-rat sociality: burrow architecture, rainfall and foraging in colonies of the cooperatively breeding Fukomys mechowii. J. Zool. 275, 276–282. doi: 10.1111/j.1469-7998.2008.00439.x
Siegmann, S., Feitsch, R., Hart, D. W., Bennett, N. C., Penn, D. J., and Zöttl, M. (2021). Naked mole-rats (Heterocephalus glaber) do not specialise in cooperative tasks. Ethology 127, 850–864. doi: 10.1111/eth.13160
Silk, M. J., and Fefferman, N. H. (2021). The role of social structure and dynamics in the maintenance of endemic disease. Behav. Ecol. Sociobiol. 75:122. doi: 10.1007/s00265-021-03055-8
Sinotte, V. M., Renelies-Hamilton, J., Taylor, B. A., Ellegaard, K. M., Sapountzis, P., Vasseur-Cognet, M., et al. (2020). Synergies between division of labor and gut microbiomes of social insects. Front. Ecol. Evol. 7:503. doi: 10.3389/fevo.2019.00503
Šklíba, J., Lövy, M., Burda, H., and Šumbera, R. (2016). Variability of space-use patterns in a free living eusocial rodent, Ansell’s mole-rat indicates age-based rather than caste polyethism. Sci. Rep. 6:37497. doi: 10.1038/srep37497
Šklíba, J., Lövy, M., Hrouzkova, E., Kott, O., Okrouhlik, J., and Šumbera, R. (2014). Social and environmental influences on daily activity pattern in free-living subterranean rodents: the case of a eusocial bathyergid. J. Biol. Rhythms 29, 203–214. doi: 10.1177/0748730414526358
Šklíba, J., Mazoch, V., Patzenhauerová, H., Hrouzková, E., Lövy, M., Kott, O., et al. (2012). A maze-lover’s dream: burrow architecture, natural history and habitat characteristics of Ansell’s mole-rat (Fukomys anselli). Mamm. Biol. 77, 420–427. doi: 10.1016/j.mambio.2012.06.004
Spinks, A. C., Branch, T. A., Croeser, S., Bennett, N. C., and Jarvis, J. U. M. (1999). Foraging in wild and captive colonies of the common mole-rat Cryptomys hottentotus hottentotus (Rodentia: Bathyergidae). J. Zool. 249, 143–152. doi: 10.1111/j.1469-7998.1999.tb00752.x
Spinks, A. C., Jarvis, J. U. M., and Bennett, N. C. (2000). Comparative patterns of philopatry and dispersal in two common mole-rat populations: implications for the evolution of mole-rat sociality. J. Anim. Ecol. 69, 224–234. doi: 10.1046/j.1365-2656.2000.00388.x/full
Spinks, A. C., O’Riain, M. J., and Polakow, D. A. (1998). Intercolonial encounters and xenophobia in the common mole rat, Cryptomys hottentotus hottentotus (Bathyergidae): the effects of aridity, sex, and reproductive status. Behav. Ecol. 9, 354–359. doi: 10.1093/beheco/9.4.354
Spottiswoode, C. N. (2008). Cooperative breeding and immunity: a comparative study of PHA response in African birds. Behav. Ecol. Sociobiol. 62, 963–974. doi: 10.1007/s00265-007-0521-0
Stein, B. R. (2000). “Morphology of subterranean rodents,” in Life Underground: The Biology of Subterranean Rodents, eds E. A. Lacey, J. A. Patton, and G. N. Cameron (Chigago, IL: The University of Chicago Press), 19–61.
Stow, A., Briscoe, D., Gillings, M., Holley, M., Smith, S., Leys, R., et al. (2007). Antimicrobial defences increase with sociality in bees. Biol. Lett. 3, 422–424. doi: 10.1098/rsbl.2007.0178
Stroeymeyt, N., Casillas-Pérez, B., and Cremer, S. (2014). Organisational immunity in social insects. Curr. Opin. Insect Sci. 5, 1–15. doi: 10.1016/j.cois.2014.09.001
Šumbera, R. (2019). Thermal biology of a strictly subterranean mammalian family, the African mole-rats (Bathyergidae, Rodentia) - a review. J. Therm. Biol. 79, 166–189. doi: 10.1016/j.jtherbio.2018.11.003
Šumbera, R., Mazoch, V., Patzenhauerová, H., Lövy, M., Šklíba, J., Bryja, J., et al. (2012). Burrow architecture, family composition and habitat characteristics of the largest social African mole-rat: the giant mole-rat constructs really giant burrow systems. Acta Theriol. 57, 121–130. doi: 10.1007/s13364-011-0059-4
Tenora, F., Barus, V., Prokes, M., Šumbera, R., and Koubková, B. (2003). Helminths parasitizing the silvery mole-rat, Heliophobius argenteocinereus (Rodentia: Bathyergidae) from Malawi. Helminthologia 40, 153–160.
Thomas, H. G., Bateman, P. W., Le Comber, S. C., Bennett, N. C., Elwood, R. W., and Scantlebury, M. (2009). Burrow architecture and digging activity in the Cape dune mole rat. J. Zool. 279, 277–284. doi: 10.1111/j.1469-7998.2009.00616.x
Thomas, H. G., Bateman, P. W., Scantlebury, M., and Bennett, N. C. (2012). Season but not sex influences burrow length and complexity in the non-sexually dimorphic solitary Cape mole-rat (Rodentia: Bathyergidae). J. Zool. 288, 214–221. doi: 10.1111/j.1469-7998.2012.00944.x
Thorley, J., Mendonça, R., Vullioud, P., Torrents-Ticó, M., Zöttl, M., Gaynor, D., et al. (2018). No task specialization among helpers in Damaraland mole-rats. Anim. Behav. 143, 9–24. doi: 10.1016/j.anbehav.2018.07.004
Till, W. (1963). Ethiopian mites of the genus Androlaelaps Berlese s. lat. (Acari: Mesostigmata). Bull. Br. Mus. Nat. Hist. 10, 1–104. doi: 10.5962/bhl.part.20524
Tofts, C., and Franks, N. R. (1992). Doing the right thing: ants, honeybees and naked mole-rats. Trends Ecol. Evol. 7, 346–349. doi: 10.1016/0169-5347(92)90128-X
Torrents-Ticó, M., Bennett, N. C., Jarvis, J. U. M., and Zöttl, M. (2018). Sex differences in timing and context of dispersal in Damaraland mole-rats (Fukomys damarensis). J. Zool. 306, 252–257. doi: 10.1111/jzo.12602
Townsend, A. K., Hawley, D. M., Stephenson, J. F., and Williams, K. E. G. (2020). Emerging infectious disease and the challenges of social distancing in human and non-human animals. Proc. R. Soc. B 287:20201039. doi: 10.1098/rspb.2020.1039
Udiani, O., and Fefferman, N. H. (2020). How disease constrains the evolution of social systems: social evolution under infection risk. Proc. R. Soc. B Biol. Sci. 287:20201284. doi: 10.1098/rspb.2020.1284
Urison, N. T., Goelst, K., and Buffenstein, R. (1993). A positive fever response by a poikilothermic mammal, the naked mole rat (Heterocephalus glaber). J. Therm. Biol. 18, 245–249. doi: 10.1016/0306-4565(93)90009-I
Vágási, C. I., Vincze, O., Lemaître, J.-F., Pap, P. L., Ronget, V., and Gaillard, J.-M. (2021). Is degree of sociality associated with reproductive senescence? A comparative analysis across birds and mammals. Philos. Trans. R. Soc. B 376:20190744. doi: 10.1098/rstb.2019.0744
van Jaarsveld, B., Bennett, N. C., Hart, D. W., and Oosthuizen, M. K. (2019). Locomotor activity and body temperature rhythms in the Mahali mole-rat (C. h. mahali): the effect of light and ambient temperature variations. J. Therm. Biol. 79, 24–32. doi: 10.1016/j.jtherbio.2018.11.013
Van Meyel, S., Körner, M., and Meunier, J. (2018). Social immunity: why we should study its nature, evolution and functions across all social systems. Curr. Opin. Insect Sci. 28, 1–7. doi: 10.1016/j.cois.2018.03.004
van Sandwyk, J. H. D. T. (2007). Identification and Classification of Endogenous Bacteria within Mole-Rats of the Family Bathyergidae. PhD Thesis. Pretoria: University of Pretoria.
VanderWaal, K. L., Atwill, E. R., Isbell, L. A., and McCowan, B. (2014). Linking social and pathogen transmission networks using microbial genetics in giraffe (Giraffa camelopardalis). J. Anim. Ecol. 83, 406–414. doi: 10.1111/1365-2656.12137
Viljoen, H., Bennett, N. C., and Lutermann, H. (2011a). Life-history traits, but not season, affect the febrile response to a lipopolysaccharide challenge in highveld mole-rats. J. Zool. 285, 222–229. doi: 10.1111/j.1469-7998.2011.00833.x
Viljoen, H., Bennett, N. C., Ueckermann, E. A., and Lutermann, H. (2011b). The role of host traits, season and group size on parasite burdens in a cooperative mammal. PLoS One 6:e27003. doi: 10.1371/journal.pone.0027003
Viney, M. E., Riley, E. M., and Buchanan, K. L. (2005). Optimal immune responses: Immunocompetence revisited. Trends Ecol. Evol. 20, 665–669. doi: 10.1016/j.tree.2005.10.003
Vleck, D. (1979). The energy cost of burrowing by the pocket gopher Thomomys bottae. Physiol. Biol. 52, 391–396.
Wallace, E. D., and Bennett, N. C. (1998). The colony structure and social organization of the giant Zambian mole-rat, Cryptomys mechowi. J. Zool. 244, 51–61. doi: 10.1111/j.1469-7998.1998.tb00006.x
Webber, Q., and Willis, C. (2016). “Sociality, parasites, pathogens in bats,” in Sociality in Bats, ed. J. Ortega (Switzerland: Springer Nature), 105–139. doi: 10.1007/978-3-319-38953-0
Wilson, K., Bjørnstad, O. N., Dobson, A. P., Merler, S., Poglayen, G., Randolph, S. E., et al. (2002). “Heterogeneities in macroparasite infections: patterns and processes,” in The Ecology of Wildlife Diseases, eds P. J. Hudson, A. Rizzoli, B. T. Grenfell, H. Heesterbeek, and A. P. Dobson (New York, NY: Oxford University Press), 6–44.
Woolhouse, E. M., Dye, C., Etard, J.-F., Smith, T., Charlwood, J. D., Garnett, G. P., et al. (1997). Heterogeneities in the transmission of infectious agents: Implications for the design of control programs. Proc. Natl. Acad. Sci. U.S.A. 94, 338–342. doi: 10.1073/pnas.94.1.338
Young, A. J., Oosthuizen, M. K., Lutermann, H., and Bennett, N. C. (2010). Physiological suppression eases in Damaraland mole-rat societies when ecological constraints on dispersal are relaxed. Horm. Behav. 57, 177–183. doi: 10.1016/j.yhbeh.2009.10.011
Zohdy, S., Kemp, A. D., Durden, L. A., Wright, P. C., and Jernvall, J. (2012). Mapping the social network: tracking lice in a wild primate (Microcebus rufus) population to infer social contacts and vector potential. BMC Ecol. 12:4. doi: 10.1186/1472-6785-12-4
Zöttl, M., Vullioud, P., Goddard, K., Torrents-Ticó, M., Gaynor, D., Bennett, N. C., et al. (2018). Allo-parental care in Damaraland mole-rats is female biased and age dependent, though independent of testosterone levels. Physiol. Behav. 193, 149–153. doi: 10.1016/j.physbeh.2018.03.021
Zumpt, F. (1961). The Arthropod Parasites of Vertebrates in Africa South of the Sahara. Vol. I (Chelicerata). Johannesburg: South African Institute of Medical Research.
Keywords: Bathyergidae, sociality, mode of transmission, generalized transmission distance, organizational immunity, social immunity, evolution
Citation: Lutermann H (2022) Socializing in an Infectious World: The Role of Parasites in Social Evolution of a Unique Rodent Family. Front. Ecol. Evol. 10:879031. doi: 10.3389/fevo.2022.879031
Received: 18 February 2022; Accepted: 05 April 2022;
Published: 13 May 2022.
Edited by:
Stan Braude, Washington University in St. Louis, United StatesReviewed by:
Marcela Lareschi, CEPAVE - Centro de Estudios Parasitológicos y de Vectores, ArgentinaCopyright © 2022 Lutermann. This is an open-access article distributed under the terms of the Creative Commons Attribution License (CC BY). The use, distribution or reproduction in other forums is permitted, provided the original author(s) and the copyright owner(s) are credited and that the original publication in this journal is cited, in accordance with accepted academic practice. No use, distribution or reproduction is permitted which does not comply with these terms.
*Correspondence: Heike Lutermann, aGx1dGVybWFubkB6b29sb2d5LnVwLmFjLnph
Disclaimer: All claims expressed in this article are solely those of the authors and do not necessarily represent those of their affiliated organizations, or those of the publisher, the editors and the reviewers. Any product that may be evaluated in this article or claim that may be made by its manufacturer is not guaranteed or endorsed by the publisher.
Research integrity at Frontiers
Learn more about the work of our research integrity team to safeguard the quality of each article we publish.