- 1Department of Zoology and Entomology, Mammal Research Institute, University of Pretoria, Pretoria, South Africa
- 2Department of Zoology, University of Cambridge, Cambridge, United Kingdom
- 3Department of Earth, Ocean and Ecological Sciences, University of Liverpool, Liverpool, United Kingdom
- 4Department of Biology and Environmental Science, Linnaeus University, Kalmar, Sweden
Dispersal from the natal site to breeding sites is a crucial phase in the life history of animals and can have profound effects on the reproductive ecology and the structure of animal societies. However, few studies have assessed dispersal dynamics in subterranean mammals and it is unknown whether dispersal distances are constrained by living underground. Here we show, in social, subterranean Damaraland mole-rats (Fukomys damarensis), that a subterranean lifestyle does not preclude long distance dispersal and that both sexes are capable of successfully dispersing long distances (>4 km). Body condition did not predict dispersal distance, but dispersers from larger groups traveled farther than individuals from smaller groups. Subsequently we show in a phylogenetically controlled comparative analysis of dispersal distances in subterranean and surface-dwelling rodents that living underground does not constrain dispersal distances and that dispersal capacity is mainly a consequence of body size in both lifestyles.
Introduction
The decision by individuals to disperse – leaving their natal territory in search of breeding opportunities (Greenwood, 1980) – and the distance that they travel, can be affected by inbreeding avoidance, by competition for mates, and by the distribution of resources in the environment (Gaines and McClenaghan, 1980; Bowler and Benton, 2009; Clutton-Brock, 2016). During dispersal, individuals must navigate through an unfamiliar environment, and for most terrestrial mammals this presents considerable mortality risks (Lambin et al., 2001; Maag et al., 2022). Such risks have been suggested to be particularly high in subterranean species whose morphological and ecological adaptations to underground living may make them ill-suited to dispersing long distances (Buffenstein, 2000; Stein, 2000). Because of their morphological adaptations and reliance on underground burrows, the natal dispersal distances of subterranean mammals have been suggested to be shorter than those of surface-dwelling species (Nevo, 1979).
Dispersal distances are known to vary widely within and between species, and the distribution of dispersal distances can provide information on a species’ ecology (Paradis et al., 2002; Nathan et al., 2012; Whitmee and Orme, 2012). For example, by comparing sex differences in dispersal across species it becomes apparent that the relative distance that males and females travel when dispersing is not necessarily directly associated with their overall tendency to disperse (Pope, 2000; Hanski and Selonen, 2009; Clutton-Brock, 2016). Despite such insights, collecting data on dispersal dynamics remains challenging. Following dispersing individuals is time and labor intensive and often requires tracking individuals beyond the bounds of a study area (Tomkiewicz et al., 2010; Maag et al., 2022), or relies on the deployment of biologgers which makes tracking possible without the need for direct resighting or recapture (Wilmers et al., 2015; Cozzi et al., 2020). These challenges have rarely been met for subterranean mammals and quantitative data on dispersal are relatively uncommon in these taxa (Busch et al., 2000). Recent molecular studies on subterranean rodents have shown that not all species have low vagility (Bray et al., 2012; Welborn and Light, 2014; Visser et al., 2018), but whether the dispersal distances of subterranean rodents are generally lower than those of surface-dwelling rodents remains unclear.
Damaraland mole-rats (Fukomys damarensis) are cooperatively breeding subterranean rodents (Family Bathyergidae) with high reproductive skew: a single reproductive pair monopolize reproduction, and their philopatric offspring delay dispersal until ecological constraints on burrowing (e.g., hard soils) are relaxed (Jarvis and Bennett, 1993; Bennett and Faulkes, 2000). Breeders can have long life spans, and non-breeders have a low chance of inheriting the burrow system (<1%; Schmidt et al., 2013; Torrents-Ticó et al., 2018; Thorley et al., 2021). Thus offspring of either sex must disperse from the natal group to acquire reproductive opportunities, even though strong ecological constraints make successful settlement rare. Indeed, very few individuals within a Damaraland mole-rat populations breed at some point in their lives (<8%; Jarvis and Bennett, 1993; Torrents-Ticó et al., 2018; Mynhardt et al., 2021; Thorley et al., 2021). Previous field studies of social mole-rats have shown that when individuals disperse, they frequently do so alone and rarely in coalitions (Jarvis and Bennett, 1993; Torrents-Ticó et al., 2018), and evidence suggests that dispersal occurs both above and below ground (Hazell et al., 2000; Bray et al., 2012; Patzenhauerová et al., 2013; Finn, 2021). By blocking themselves off into a section of the natal burrow, individuals can form a new territory via “territory budding” (Jarvis and Bennett, 1993; Lövy et al., 2013; Mynhardt et al., 2021). Genetic and re-trapping data suggest that new groups often form from unrelated pairs (Burland et al., 2002). Taken together, this demographic information identifies features that are likely to have been important selective forces in the formation of social groups in mole-rats. For example, the fact that males sometimes immigrate into established groups, whereas females rarely do (Patzenhauerová et al., 2013; Torrents-Ticó et al., 2018; Thorley et al., 2021), might explain the relatively large male-biased sexual size dimorphism in Fukomys mole-rats (Caspar et al., 2021). However, mole-rat populations are known to occupy habitats with varying climate and geology and the structure of groups often varies (Spinks et al., 2000; Finn et al., 2018). In this context, dispersal could play a crucial role in structuring population differences and it is important to understand sex-specific dispersal patterns across mole-rat populations.
In this study we investigate the dispersal distances of Damaraland mole-rats in the southern Kalahari Desert. Our study serves two aims. First, we use longitudinal data from a capture-mark-recapture study to test whether the distances traveled vary between the sexes or as a result of body condition or group size. Second, we conducted a phylogenetically controlled comparative analysis of published dispersal distances in rodents to test whether the below ground lifestyle constrains dispersal distances.
Methods
Study Site and Animal Capture Methods
We monitored 48 Damaraland mole-rat groups and 54 single individuals at the Kalahari Research Centre and an adjacent farm (26.98706°S, 21.81229°E; total area 1,850 ha) in the Northern Cape Province of South Africa between November 2013 and May 2020 (Supplementary Material). Groups are defined as two or more individuals which were recaptured repeatedly in the same location (Supplementary Material). Groups were recaptured at approximately 6-month intervals (mean ± SD = 7.5 ± 5.0 months, n = 403) and were recaptured 5.3 ± 3.5 (mean ± SD) times during the study period.
Animals were caught using Hickman traps baited with sweet potato (see Supplementary Material) for a total of 899 individual mole-rats (2,305 distinct animal captures across 403 group capture events). All individuals in a group were assumed to be captured when no activity was detected in the traps for 24 h, and these likely complete captures comprised 81.9% of capture events (n = 330; see Supplementary Material). Captured individuals were sexed, weighed to the nearest gram, and their total length was measured dorsally from nose to tip of tail with a ruler (±1 mm), and upper incisor width was measured with digital calipers (±0.01 mm). Animals were made individually identifiable by implanting them with a passive integrated transponder tag (Trovan Unique, DorsetID, Aalten, Netherlands). Thus, known and unknown individuals refer to animals with or without a transponder tag upon capture. Captured mole-rats were housed together with group members in artificial tunnel systems made from PVC pipes and provided with sweet potato ad libitum before they were released again in their burrow system (see Thorley et al., 2021).
The study site is divided into a core area where we focused most of our efforts and a western area where trapping was less intensive (Figure 1 and Supplementary Material). New groups were detected by the presence of mounds created during burrow construction while walking between groups when checking traps. To further detect new groups, the study area was systematically searched biannually by walking transects in areas where mole-rat mounds were absent. These searches maximized our likelihood of identifying dispersing individuals. Our study area was increased over time as a result of these searches (Figure 1).
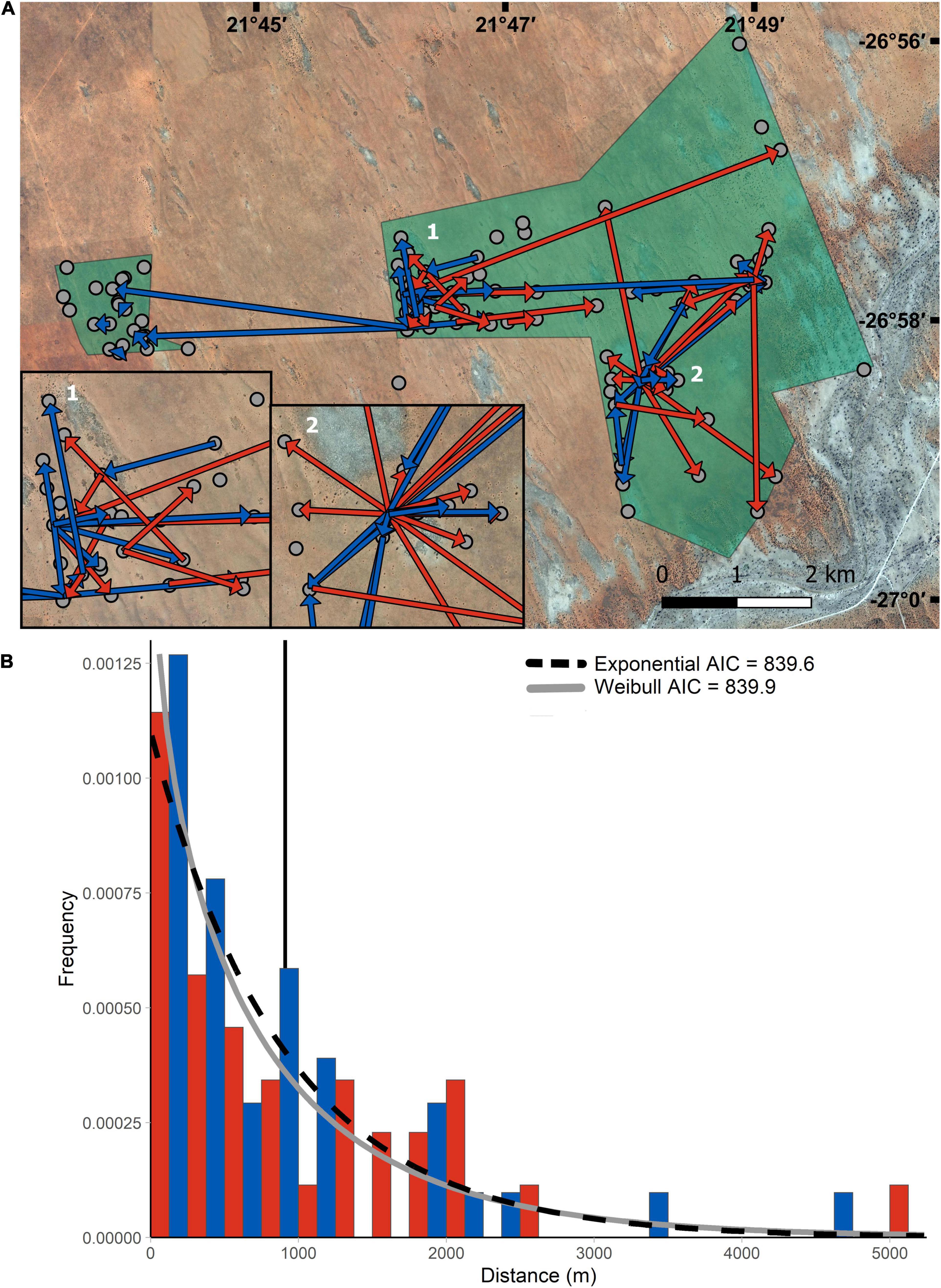
Figure 1. Dispersal distances in Damaraland mole-rats. The distance traveled was measured as the Euclidean distance between the natal group and new location. (A) Direction of travel in female (red), and male dispersers (blue) at the study site (green boxes). Group locations are indicated as gray circles. Inset 1 and 2 enlarge areas of multiple overlapping arrows. The areas we focused our search for dispersers is highlighted in green, with the core area being the larger area to the east. (B) Histogram of distances traveled during natal dispersal by Damaraland mole-rats (males in blue and females in red). The exponential (dashed) and Weibull (gray) density curves are overlaid on the graph. The solid vertical line indicates the mean distance traveled (910 ± 1,004 m). There was no significant difference in the mean distance traveled between the sexes (n = 76, p-value = 0.30) (doi:10.1093/jmammal/gyaa163).
When new locations of mounds were found, we captured the individuals present, and many dispersers were found in this way. Individuals with a transponder that were captured in a new location were considered as known dispersers. If adult individuals (>90 g) lacking a transponder were captured in a group within 6 months of a previous capture they were most likely unknown dispersers because wild juveniles will not reach 90 g in 6 months (Zöttl et al., 2016; Thorley et al., 2021). Due to the chance that some individuals may evade capture adult unknown individuals are therefore likely dispersers. When the capture interval was >6 months, new individuals >90 g may be the offspring of the dominants, and these individuals were therefore excluded from the analyses as we could not distinguish between in-group recruitment and out-of-group immigration. Our demographic data suggested that female dispersers frequently settle singly in a new location after dispersal, and rarely (n = 3) represent the last remaining individual in a previously established group (Thorley et al., 2021). Thus, we assume that unknown single individuals were also likely dispersers.
Dispersing mole-rats may block-off an unused section of their natal burrow, creating a new burrow adjacent to their natal burrow (Jarvis and Bennett, 1993; Lövy et al., 2013). We refer to these instances as “territory budding” (Supplementary Material). A radiofrequency identification (RFID) reader array was deployed in cases of suspected territory budding to ensure that the new group was physically separated from the natal group (Francioli et al., 2020). In short, this involved the placement of multiple RFID antennas above active foraging tunnels for 2–5 days. The antenna detects the transponder tag of individuals as they move through their burrow system. If individuals were living in physically separated burrow systems, then they would be detected on one antenna but not others with no overlap of individuals across antennas, thus implying the budding territory was distinct from the natal territory.
Dispersal Distances in Damaraland Mole-Rats
All statistical analyses were performed with R version 4.0.5 (R Core Team, 2021). Data are reported as mean ± 1 SD. A single GPS point was taken between trap sites at each group capture (Supplementary Material), and distances between capture locations of known dispersers were measured (±1 m) as an estimate of dispersal distance between locations with the Geosphere package (Hijmans et al., 2019). All unknown dispersers were removed from the distance analysis because they originated from unknown locations. We assigned each known disperser a body condition score which was computed as the residuals from a linear regression where body mass was the response and upper tooth width and body length were predictors. We analyzed the effects on dispersal distance in a linear mixed model with the natural log transformed dispersal distance as the response and sex, natal group size (number of individuals at last capture in their natal group), and the interaction between body condition and sex as predictors using nlme (Pinheiro et al., 2021). Group identity was added as a random effect to control for non-independence arising from dispersers that originated from the same group, and after fitting the model, we validated that model assumptions were met (Supplementary Material). Fisher’s exact tests were used to compare instances of territory budding to natal dispersal.
Dispersal Distances in Subterranean and Surface-Dwelling Rodents
To place the dispersal distance of Damaraland mole-rats into a broader context, we collated mean and maximum dispersal distances of rodents from the literature. We used the comparative analyses of Whitmee (2010) as the starting point for our literature search, and supplemented with further information, targeting subterranean and fossorial rodents in particular (Supplementary Table 4). We classed rodent species as fossorial if they spent nearly their entire lives underground and possess morphological adaptations for a burrowing lifestyle (Buffenstein, 2000; Stein, 2000; Gomes Rodrigues et al., 2016; Montoya-Sanhueza et al., 2019; see Supplementary Material). We further classified fossorial species into subterranean or not depending on whether they forage primarily below or above ground (Thorley, 2020). We added mean body mass of each species using the AnAge (de Magalhães and Costa, 2009) and Pantheria databases (Jones et al., 2009). In total, our data set included information from 57 studies, covering 53 species (Supplementary Table 4).
We fitted a series of Bayesian phylogenetic mixed models on our data set (PLMM) using the MCMCglmm package (Hadfield, 2010). PLMMs incorporate a phylogenetic tree as a random effect to control for the non-independence of comparative data due to the shared ancestry among species (Hadfield and Nakagawa, 2010). First, we explored the phylogenetic signal in both the mean and maximum dispersal distance by fitting a simple PLMM in which the natural log of the dispersal distance [ln(mean) or ln(maximum)] was modeled against the intercept -- an intercept-only model. The phylogenetic variance was fitted as a phylogenetic covariance matrix and included as a random effect, and was derived from a randomly chosen rodent phylogeny taken from Vertlife1. The phylogenetic signal was estimated as the phylogenetic heritability (h2phy) – the ratio of the phylogenetic variance to the total trait variance. Additional models then included predictors of the mean and maximum dispersal distance: ln(body mass), subterranean (categorical: yes or no), dispersal event sample size (categorical: very low, <10; low, 10–25; medium, 25–50; and >50, high). All models were fitted to a Gaussian error distribution and ran for 205,000 iterations, with a burn-in of 5000 and a thinning interval of 100; generating a posterior sample of 2,000 for all model estimates. A parameter expanded prior was specified for the phylogenetic covariance matrix (V = 1, nu = 1, alpha.mu = 0, and alpha.V = 1,000). Diagnostic checks indicated that the level of auto-correlation in chains was very low and that model residuals conformed to assumptions of normality and homogeneity of variance.
Results
Dispersal Distances of Damaraland Mole-Rats
A total of 76 known (35 females, 41 males) and 37 unknown (29 females, 8 males) dispersers were captured during the study, comprising 12.5% of the population and 4.9% of the total capture effort. The sex ratio of dispersers (males divided by total dispersers, 0.44) was the same as the mean population adult sex ratio (0.44 ± 0.23). We found four suspected dispersal coalitions (Supplementary Material). All known dispersers were never recaptured with members of their natal burrow after dispersal.
We found that the mean dispersal distance was not significantly different between the sexes (males = 834 ± 980 m; females = 998 ± 1,038 m; and LMM: Est. = –0.26, S.E. = 0.24, p-value = 0.30; Supplementary Table 2) and the majority of known dispersers traveled less than 1,000 m (68.8%; Figure 1B). However, two individuals traveled over 4.5 km during natal dispersal (female 4,597 m, male 5,232 m). We found that 57.1% of female dispersers (n = 20), and 48.8% of male dispersers (n = 20) moved >500 m, while 22.9% of females (n = 8) and 29.3% of males (n = 12) settled within 200 m of their natal group. Territory budding was rare and occurred less frequently than other forms of dispersal (5 instances; 11.6%; Fisher’s: df = 1, p < 0.001). When it occurred, individuals usually settled in parts of the natal territory and blocked of a subsection of the groups burrow (Supplementary Material).
We found that only natal group size (LMM: Est = 0.08, S.E. = 0.02, and p-value = 0.002) and not body condition (LMM: Est. = –0.01, S.E. = 0.01, and p-value = 0.45) were significant predictors of dispersal distance, where dispersal distance increased with group size for both sexes (Supplementary Figure 2 and Supplementary Table 2).
Dispersal Distances in Subterranean and Surface-Dwelling Rodents
The dispersal distances of subterranean rodents were similar to those of surface-dwelling rodents (Figure 2). When body mass was excluded, both the mean and maximum dispersal distance showed a strong phylogenetic signal (Table 1). After the strong positive association with body mass was included, the phylogenetic signal dropped substantially and was estimated with wide credible intervals. Sample size did not affect either the mean or maximum dispersal distance in rodents given the available data (Table 1).
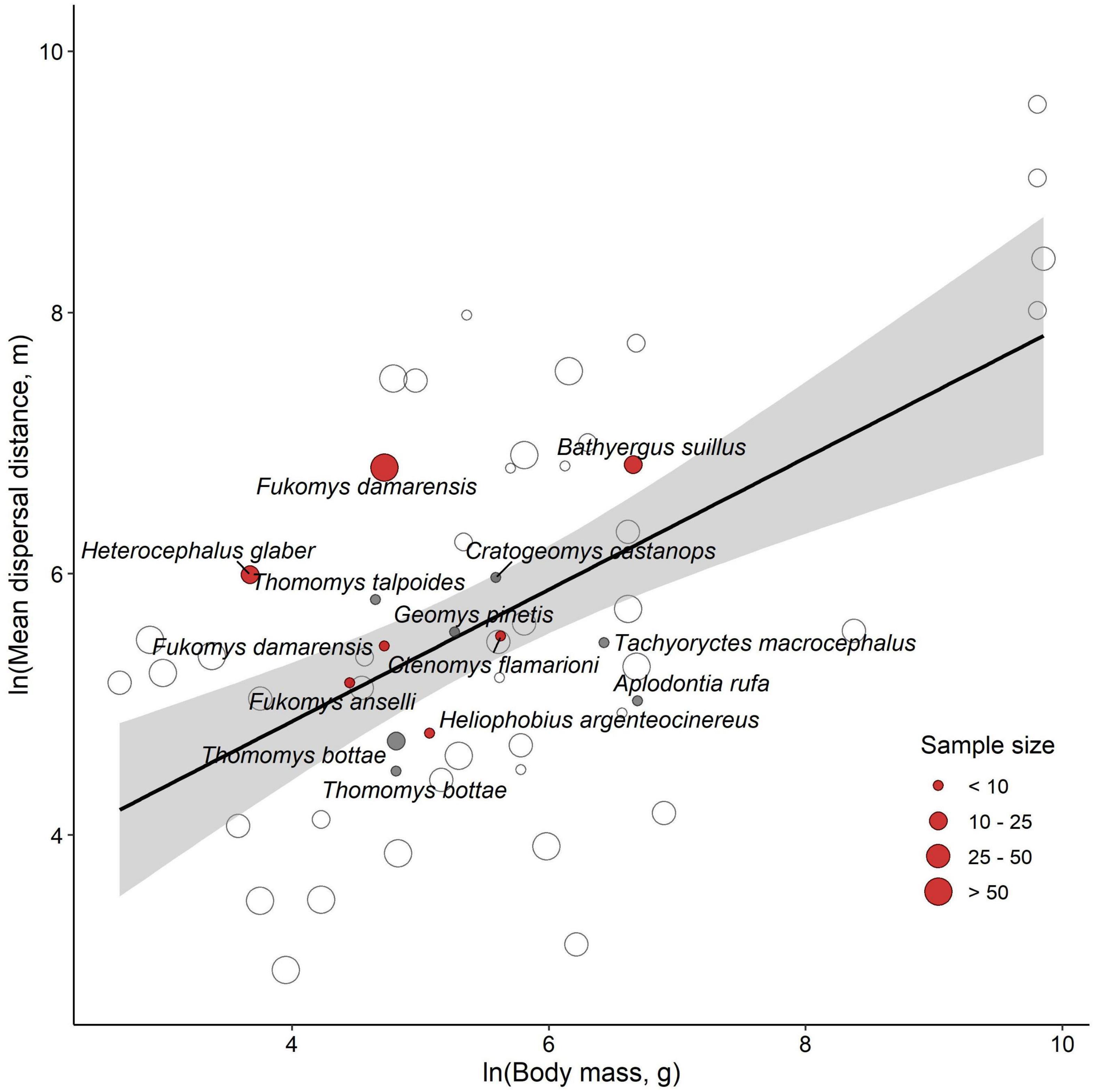
Figure 2. The relationship between the mean dispersal distance and body mass in rodents. Data points are separated according to whether the species is subterranean (red), fossorial (gray), or surface-dwelling (clear). Points are sized according to the number of observed dispersal records in the literature review as shown in the legend. The large Fukomys damarensis circle is from this study. The solid line indicates the line of best fit through the raw data for surface-dwelling rodents with shading denoting the 95% confidence intervals.
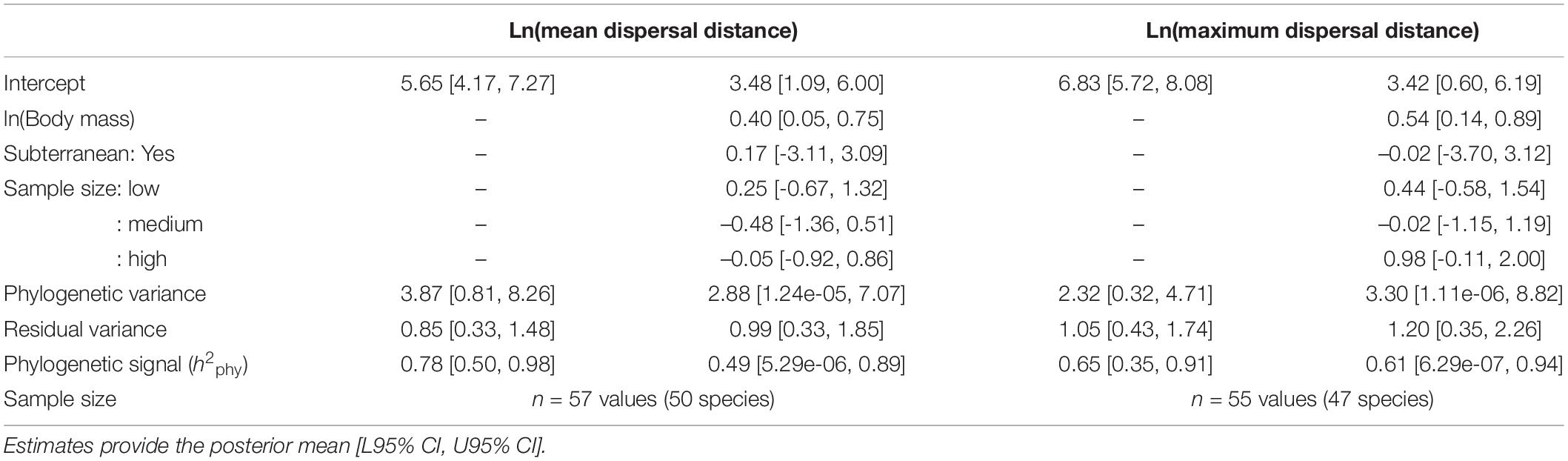
Table 1. Results of Bayesian phylogenetic mixed models fitted to comparative data on rodent dispersal.
Discussion
Our study investigated the dispersal dynamics of the Damaraland mole-rat. We did not find a significant difference in the dispersal distances of males and females. Both sexes were capable of traveling well over 1,000 m, and in two cases over 4,500 m. This similarity may be due to the social structure and mating system of social mole-rats, where mole-rat families are composed of an unrelated monogamous pair (Bennett and Faulkes, 2000). This structure forces both sexes to disperse in order to reproduce with unrelated individuals, rather than inheriting breeding positions in their natal burrow. Females can travel long distances searching for new territories with food resources (i.e., plant tubers), and males can travel long distances searching for mates.
In most monogamous and cooperatively breeding mammals, like the Damaraland mole-rat, females often settle in close proximity to their mother’s territory (Dobson, 2013). Therefore, we expected that females would also follow this pattern as observed in a related social mole-rat (Lövy et al., 2013). Whereas short dispersal distances do occur, we found that females mostly traveled more than 500 m from the natal burrow, and as shown previously, establish a new burrow system while waiting for dispersing males to join them (Thorley et al., 2021). The widely scattered food resources may force females to travel far to find suitable territory. Alternatively, females may disperse farther from the natal group to avoid competing with neighboring groups since over time burrow systems can become quite large. When the population density is low, the chance of an immigrant male locating a single female is low and therefore single females may have to wait several years before finding a mate (Thorley et al., 2021). The exceptional longevity of mole-rats for their size (Buffenstein et al., 2020; Thorley, 2020), combined with low predation rates once settled in a burrow, may allow females to endure these considerable periods without reproductive opportunities while waiting for male dispersers to find them.
Dispersal distance can be affected by body mass, territory size, availability of resources or vacant territories, and breeding opportunities (Bowman et al., 2002; Clobert et al., 2009; Whitmee and Orme, 2012). In our study, we found that animals from larger groups traveled further, and that natal group size had a stronger effect on dispersal distance than the body condition of dispersing individuals (Supplementary Material). The cause of this pattern is unclear, but one possibility is that group and burrow size may be influenced by the distribution of food resources in the landscape. In the Kalahari, the food resources for mole-rats exhibit a scattered but patchy distribution which may affect the dispersion and size of family groups in the landscape (Lovegrove, 1988). In particular, one could hypothesize that large groups occupy larger burrow systems and that this may force females to move further to find a vacant habitat with sufficient food resources. Thus, since natal group size and burrow size are closely related (Lovegrove, 1988; Thomas et al., 2016) the effect of group size may indicate that burrow size affects dispersal distances in mole-rats. Variation in group size might also reflect the localized abundance of plant tubers, and this in turn might affect the distances that individuals are required to move to find vacant habitat or receptive mates. Lastly, increases in group size may also lead to increased competition for food or space between individuals within a group (Cooney, 2002; Zöttl et al., 2016), which may cause heightened stress levels and trigger dispersal to avoid competition (Quirici et al., 2011). Heightened stress levels from increasing group size (and limited burrow capacity) may cause animals to disperse farther to escape a perceived stressor. Adult Damaraland mole-rats exhibit higher glucocorticoids than juveniles (Medger et al., 2018), and cortisol levels increase during the rainy season likely due to dispersal opportunities (Young et al., 2010), but the effects of group size on stress levels has not been investigated in mole-rats.
Despite the challenges of following dispersal in a subterranean rodent, the low rate of successful dispersal conforms to the general view that dispersal in mole-rats and other subterranean rodents carries a high mortality risk. Mole-rats are specialized diggers with low visual acuity (Kott et al., 2010; Gomes Rodrigues et al., 2016; Montoya-Sanhueza, 2020), and like other similarly adapted species, it has long been assumed that an underground lifestyle limits vagility (Nevo, 1979). Adaptations for subterranean life may make mole-rats particularly susceptible to predation while dispersing. Indeed, diet studies indicate that mole-rats may present a seasonally available prey item to jackals (Canis mesomelas) and serval (Leptailurus serval; Humphries et al., 2015; Ramesh and Downs, 2015). Despite the apparent constraints on dispersal, we present evidence showing that dispersal distances in subterranean rodents fall within the expected range of rodents based on their body size. Within the Family Bathyergidae previous studies have recorded a maximum dispersal distance of 2,149 m in Cape dune mole-rats (Bathyergus suillus; Bray et al., 2012), and 2,400 m in naked mole-rats (Heterocephalus glaber, Braude, 2000). Our study found that 5 dispersers, including individuals of both sexes, traveled over this distance, with the greatest distance traveled being 5,232 m from the natal burrow. Our study shows that the subterranean niche does not necessarily limit long distance dispersal, and some individuals can settle successfully far away from their natal territory. The long distances observed in this study suggest that traveling above ground may be the primary method of natal dispersal for Damaraland mole-rats and perhaps many other subterranean rodents, as previously suggested in geomyids (Welborn and Light, 2014).
Like in other field studies of natural populations the estimations of dispersal frequency and distance are likely to be conservative underestimates. The estimation of dispersal distances are based on the recapture of known dispersers and it is likely that the likelihood of finding these individuals decreases with increasing dispersal distance (Tomkiewicz et al., 2010; Maag et al., 2022). The number of unknown dispersers is also an approximation because we were unable to assess relatedness among individuals in each group. Some unknown dispersers found in established groups during the study may be recruits born to the reproductive pair which evaded capture and were not immigrants. However, our data suggests that these are rare occurrences because longitudinal resampling suggests that we rarely miss individuals repeatedly. Because of these reasons we believe that both of these sources of bias either lead to conservative underestimates or to very minor inaccuracies in frequencies of immigration.
By focusing on successfully established dispersers and the distances they moved, our study can only get us part way toward a complete understanding of sex-specific dispersal distances in Damaraland mole-rats. Dispersal is a three-stage process covering emigration, transience, and settlement, and different factors (e.g., mortality) may affect the sexes at each of these stages (Clobert et al., 2009; Maag et al., 2022). The examination of settlement patterns provides information on the probability that members of either sex acquire breeding opportunities, and the circumstances under which they do so, but additional sources of information must also be used to understand individual decisions during emigration and transience. As mole-rats cannot be observed continuously, emigration patterns have often been inferred from the duration of philopatry (Torrents-Ticó et al., 2018; Thorley et al., 2021), under the assumption that a sizeable proportion of disappearances are due to dispersal rather than in situ mortality. Such data suggests that in the social mole-rats, males emigrate from groups earlier than females (Torrents-Ticó et al., 2018). However, there is also no clear evidence of lifelong philopatry of non-breeding females (Torrents-Ticó et al., 2018; Thorley et al., 2021). While males may disperse earlier, the difference in the absolute number of male and female emigrants may be very small, at least in our population where the adult sex ratio is equal to that of dispersers (cf. Hazell et al., 2000). Direct information on the transience phase of dispersal in mole-rats is largely non-existent, save for one individually tracked animal (Lövy et al., 2013). It remains to be seen whether the two sexes face differing mortality risk during dispersal, such as from competitive aggression (Busch et al., 2000), or through increased search times (Šumbera et al., 2008). Molecular techniques have advanced our understanding of dispersal patterns in fossorial rodents by using microsatellite markers to determine the presence of sex biases in dispersal, gene flow between populations, and allow for an estimation of dispersal distances without the need for longitudinal sampling (Cutrera et al., 2005; Fernández-Stolz et al., 2007; Bray et al., 2012; Patzenhauerová et al., 2013; Welborn and Light, 2014; Visser et al., 2018; Mynhardt et al., 2021). Comparing variation in dispersal patterns within a single species across populations living in varying population densities, or environmental conditions (e.g., rainfall or soil type) would greatly assist in determining the extent to which demographic and ecological factors affect dispersal opportunities, frequency of intergroup movement, and gene flow in subterranean rodents.
Data Availability Statement
The datasets presented in this study can be found on the online repository FigShare: https://doi.org/10.6084/m9.figshare.19196732.v1.
Ethics Statement
The study was approved by the University of Pretoria Ethics Committee (EC032-13, EC006-15, EC050-16, and EC059-18) and subsequently approved by Northern Cape Nature Conservation.
Author Contributions
KF, JT, and MZ conceived and designed the study. KF, JT, HB, and MZ collected the data. KF and JT analyzed the data. KF wrote the first draft of the manuscript. All authors made significant contributions.
Funding
This research is part of a project that has received funding from the European Research Council (ERC) under the European Union’s Horizon 2020 research and innovation program (Grant agreement Nos. 294494 and 742808) and the Mammal Research Institute at the University of Pretoria, South Africa. MZ acknowledges funding from Vetenskapsrådet (2017–05296) and Crafoordska Stiftelsen (2018-2259 and 2020-0976).
Conflict of Interest
The authors declare that the research was conducted in the absence of any commercial or financial relationships that could be construed as a potential conflict of interest.
Publisher’s Note
All claims expressed in this article are solely those of the authors and do not necessarily represent those of their affiliated organizations, or those of the publisher, the editors and the reviewers. Any product that may be evaluated in this article, or claim that may be made by its manufacturer, is not guaranteed or endorsed by the publisher.
Acknowledgments
We thank Radim Šumbera for sharing dispersal distance data. We are grateful to Tim Clutton-Brock, Marta Manser, David Gaynor, the Kalahari Research Trust, and the Kalahari Research Centre (KRC) for access to facilities and for logistical support at the Kuruman River Reserve. We also thank Cobus Lampreigh for access to his farm. Thanks to Nigel Bennett for his continued support and helpful comments on earlier drafts of this manuscript. Thanks to our field assistants, other researchers, postdocs, managers, and staff at the KRC who assisted at various stages for this project. We would like to thank the Northern Cape Department of Environment and Nature Conservation and the University of Pretoria Ethics Committee for permission to conduct the research.
Supplementary Material
The Supplementary Material for this article can be found online at: https://www.frontiersin.org/articles/10.3389/fevo.2022.879014/full#supplementary-material
Footnotes
References
Bennett, N. C., and Faulkes, C. G. (2000). The African Mole-Rats: Ecology and Eusociality. Cambridge: Cambridge University Press.
Bowler, D. E., and Benton, T. G. (2009). Variation in dispersal morality and dispersal propensity among individuals: the effects of age, sex and resource availability. J. Anim. Ecol. 78, 1234–1241. doi: 10.1111/j.1365-2656.2009.01580.x
Bowman, J., Jaeger, J. A. G., and Fahrig, L. (2002). Dispersal distance of mammals is proportional to home range size. Ecology 83, 2049–2055.
Braude, S. H. (2000). Dispersal and new group formation in wild naked mole-rats: evidence against inbreeding as the system of mating. Behav. Ecol. 11, 7–12.
Bray, T. C., Bloomer, P., O’Riain, M. J., and Bennett, N. C. (2012). How attractive is the girl next door? An assessment of spatial mate acquisition and paternity in the solitary Cape dune mole-rat, Bathyergus suillus. PLoS One 7:e39866. doi: 10.1371/journal.pone.0039866
Buffenstein, R. (2000). “Ecophysiological responses of subterranean rodents to underground habits,” in Life Underground, eds E. A. Lacey, J. I. Patton, and G. N. Cameron (Chicago, IL: University of Chicago Press), 62–110.
Buffenstein, R., Lewis, K. N., Gibney, P. A., Narayan, V., Grimes, K. M., Smith, M., et al. (2020). Probing pedomorphy and prolonged lifespan in naked mole-rats and dwarf mice. Physiology 35, 96–111. doi: 10.1152/physiol.00032.2019
Burland, T. M., Bennett, N. C., Jarvis, J. U. M., and Faulkes, C. G. (2002). Eusociality in African mole-rats: new insights from patterns of genetic relatedness in the Damaraland mole-rat (Cryptomys damarensis). Proc. R. Soc. Lond. B 269, 1025–1030. doi: 10.1098/rspb.2002.1978
Busch, C., Antinuchi, C. D., de Valle, J. C., Kittlein, M. J., Malizia, A. I., Vassallo, A. I., et al. (2000). “Population ecology of subterranean rodents,” in Life Underground: The Biology of Subterranean Rodents, eds E. A. Lacey, J. L. Patton, and G. N. Cameron (Chicago, IL: University of Chicago Press), 183–226.
Caspar, K. R., Müller, J., and Begall, S. (2021). Effects of sex and breeding status on skull morphology in cooperatively breeding Ansell’s mole-rats and an appraisal of sexual dimorphism in the Bathyergidae. Front. Ecol. Evol. 9:638754. doi: 10.3389/fevo.2021.638754
Clobert, J., Le Galliard, J., Meylan, S., and Massot, M. (2009). Informed dispersal, heterogeneity in animal dispersal syndromes and the dynamics of spatially structured populations. Ecol. Lett. 12, 197–209.
Clutton-Brock, T. (ed.). (2016). “Male dispersal and its consequences,” in Mammal Societies, (Chichester: John Wiley & Sons, Ltd), 401–426.
Cooney, R. (2002). Colony defense in Damaraland mole-rats Cryptomys damarensis. Behav. Ecol. 13, 160–162.
Cozzi, G., Behr, D. M., Webster, H. S., Claase, M., Bryce, C. M., Modise, B., et al. (2020). African wild dog dispersal and implications for management. J. Wildl. Manag. 84, 614–621. doi: 10.1002/jwmg.21841
Cutrera, A. P., Lacey, E. A., and Busch, C. (2005). Genetic structure in a solitary rodent (Ctenomys talarum): implications for kinship and dispersal. Mol. Ecol. 14, 2511–2523. doi: 10.1111/j.1365-294X.2005.02551.x
de Magalhães, J. P., and Costa, J. (2009). A database of vertebrate longevity records and their relation to other life-history traits. J. Evol. Biol. 22, 1770–1774. doi: 10.1111/j.1420-9101.2009.01783.x
Dobson, F. S. (2013). The enduring question of sex-biased dispersal: Paul J. Greenwood’s (1980) seminal contribution. Anim. Behav. 85, 299–304. doi: 10.1016/j.anbehav.2012.11.014
Fernández-Stolz, G. P., Stolz, J. F. B., and de Freitas, T. R. O. (2007). Bottlenecks and dispersal in the tuco-tuco das dunas Ctenomys flamarioni (Rodentia: Ctenomyidae) in southern Brazil. J. Mammal. 88, 935–945.
Finn, K. T. (2021). Potential use of a magnetic compass during long-distance dispersal in a subterranean rodent. J. Mammal. 102, 205–257. doi: 10.1093/jmammal/gynaa163
Finn, K. T., Parker, D. M., Bennett, N. C., and Zöttl, M. (2018). Contrasts in body size and growth suggest that high population density results in a faster pace of life in Damaraland mole-rats (Fukomys damarensis). Can. J. Zool. 96, 920–927. doi: 10.1139/cjz-2017-0200
Francioli, Y., Thorley, J., Finn, K., Clutton-Brock, T., and Zöttl, M. (2020). Breeders are less active forager than non-breeders in wild Damaraland mole-rats. Biol. Lett. 16:20200475. doi: 10.1098/rsbl.2020.0475
Gaines, M. S., and McClenaghan, L. R. (1980). Dispersal in small mammals. Ann. Rev. Ecol. Syst. 11, 163–196. doi: 10.1371/journal.pone.0151500
Gomes Rodrigues, H., Šumbera, R., and Hautier, L. (2016). Life in burrows channelled the morphological evolution of the skull in rodents: the case of African mole-rats (Bathyergidae, Rodentia). J. Mamm. Evol. 23, 175–189. doi: 10.1007/s10914-015-9305-x
Greenwood, P. J. (1980). Mating systems, philopatry and dispersal in birds and mammals. Anim. Behav. 26, 645–652.
Hadfield, J. (2010). MCMC methods for multi-response generalized linear mixed models: the MCMCglmm R package. J. Stat. Softw. 33, 1–22.
Hadfield, J., and Nakagawa, S. (2010). General quantitative genetic methods for comparative biology: phylogenies, taxonomies and multi-trait models for continuous and categorical characters. J. Evol. Biol. 23, 494–508. doi: 10.1111/j.1420-9101.2009.01915.x
Hanski, I. K., and Selonen, V. (2009). Female-biased natal dispersal in the Siberian flying squirrel. Behav. Ecol. 20, 60–67. doi: 10.1093/beheco/arn115
Hazell, R. W. A., Bennett, N. C., Jarvis, J. U. M., and Griffin, M. (2000). Adult dispersal in the cooperatively breeding Damaraland mole-rat (Cryptomys damarensis): a case study from the Waterberg region of Namibia. J. Zool. 252, 19–25.
Hijmans, R. J., Williams, E., and Vennes, C. (2019). Geosphere: Spherical Trigonometry. R package version 1.5-10. Available Online at: https://CRAN.R-project.org/package=geosphere (accessed November 10, 2021).
Humphries, B. D., Ramesh, T., and Downs, C. T. (2015). Diet of black-backed jackals (Canis mesomelas) on farmlands in the KwaZulu-Natal Midlands. South Africa. Mammalia 80, 405–412. doi: 10.1515/mammalia-2014-0103
Jarvis, J. U. M., and Bennett, N. C. (1993). Eusociality has evolved independently in two genera of bathyergid mole-rats but occurs in no other subterranean mammal. Behav. Ecol. Sociobiol. 33, 253–260.
Jones, K. E., Bielby, J., Cardillo, M., Fritz, S. A., O’Dell, J., Orme, C. D. L., et al. (2009). PanTHERIA: a species-level database of life history, ecology, and geography of extant and recently extinct mammals. Ecology 90:2648.
Kott, O., Šumbera, R., and Nemec, P. (2010). Light perception in two strictly subterranean rodents: life in the dark or blue? PLoS One 5:e11810. doi: 10.1371/journal.pone.0011810
Lambin, X., Aars, J., and Piertney, S. B. (2001). “Dispersal, intraspecific competition, kin competition and kin facilitation: a review of the empirical evidence,” in Dispersal, eds J. Clobert, E. Danchin, A. A. Dhondt, and J. D. Nichols (Oxford: Oxford University Press), 110–122.
Lovegrove, B. G. (1988). Colony size and structure, activity patterns and foraging behaviour of a colony of the social mole-rat Cryptomys damarensis (Bathyergidae). J. Zool. 216, 391–402. doi: 10.1111/j.1469-7998.1988.tb02437.x
Lövy, M., Šklíba, J., and Šumbera, R. (2013). Spatial and temporal activity patterns in the free-living giant mole-rat (Fukomys mechowii), the largest social bathyergid. PLoS One 8:e55357. doi: 10.1371/journal.pone.0055357
Maag, N., Paniw, M., Cozzi, G., Manser, M., Clutton-Brock, T., and Ozgul, A. (2022). Dispersal decreases survival but increases reproductive opportunities for subordinates in a cooperative breeder. Am. Nat. 199, 679–690.
Medger, K., Bennett, N. C., Lutermann, H., and Ganswindt, A. (2018). Non-invasive assessment of glucocorticoid and androgen metabolite levels in cooperatively breeding Damaraland mole-rats (Fukomys damarensis). Gen. Comp. Endocrinol. 266, 202–210. doi: 10.1016/j.ygcen.2018.05.018
Montoya-Sanhueza, G. (2020). Functional Anatomy, Osteogenesis and Bone Microstructure of the Appendicular System of African Mole-Rats (Rodentia: Ctenohystrica: Bathyergidae). Ph.D. dissertation. Cape Town: University of Cape Town.
Montoya-Sanhueza, G., Wilson, L. A. B., and Chinsamy, A. (2019). Postnatal development of the largest subterranean mammal (Bathyergus suillus): morphology, osteogenesis, and modularity of the appendicular skeleton. Dev. Dyn. 248, 1101–1128. doi: 10.1002/dvdy.81
Mynhardt, S., Harris-Barnes, L., Bloomer, P., and Bennett, N. C. (2021). Spatial population genetic structure and colony dynamics in Damaraland mole-rats (Fukomys damarensis) from the southern Kalahari. BMC Ecol. 21:221. doi: 10.1186/s12862-021-01950-2
Nathan, R., Klein, R., Robledo-Arnuncio, J. J., and Revilla, E. (2012). “Dispersal kernels: review,” in Dispersal Ecology and Evolution, eds J. Clobert, M. Baguette, T. G. Benton, and J. M. Bullock (Oxford: Oxford University Press), 187–210.
Nevo, E. (1979). Adaptive convergence and divergence of subterranean mammals. Ann. Rev. Ecol. Syst. 10, 269–308.
Paradis, E., Baille, S. R., Sutherland, W. J., and Gregory, R. D. (2002). Patterns of natal and breeding dispersal in birds. J. Anim. Ecol. 67, 518–536. doi: 10.1046/j.1365-2656.1998.00215.x
Patzenhauerová, H., Šklíba, J., Bryja, J., and Šumbera, R. (2013). Parental analysis of Ansell’s mole-rat family groups indicates a high reproductive skew despite relatively relaxed ecological constraints on dispersal. Mol. Biol. 22, 4988–5000. doi: 10.1111/mec.12434
Pinheiro, J., Bates, D., DebRoy, S., and Sarkar, D. (2021). Linear and Nonlinear Mixed Effects Models. R package version 3.1-152. Available Online at: https://CRAN.R-project.org/package=nlme (accessed November 10, 2021).
Pope, T. R. (2000). “The evolution of male philopatry in neotropical monkeys,” in Primate Males: Causes and Consequences of Variation in Group Composition, ed. P. Kappeler (Cambridge: Cambridge University Press), 219–235.
Quirici, V., Faugeron, S., Hayes, L. D., and Ebensperger, L. A. (2011). The influence of group size on natal dispersal in the communally rearing and semifossorial rodent, Octodon degus. Behav. Ecol. Sociobiol. 65, 787–798. doi: 10.1007/s00265-010-1082-1
R Core Team (2021). R: A Language and Environment for Statistical Computing. Vienna: R Foundation for Statistical Computing.
Ramesh, T., and Downs, C. T. (2015). Diet of serval (Leptailurus serval) on farmlands in the Drakensberg Midlands, South Africa. Mammalia 79, 399–407. doi: 10.1515/mammalia-2014-0053
Schmidt, C. M., Jarvis, J. U. M., and Bennett, N. C. (2013). The long-lived queen: reproduction and longevity in female eusocial Damaraland mole-rats (Fukomys damarensis). Afr. Zool. 48, 193–196.
Spinks, A. C., Jarvis, J. U. M., and Bennett, N. C. (2000). Comparative patterns of philopatry and dispersal in two common mole-rat populations: implications or the evolution of mole-rat sociality. J. Anim. Ecol. 69, 224–234.
Stein, B. R. (2000). “Morphology of subterranean rodents,” in Life Underground, eds E. A. Lacey, J. I. Patton, and G. N. Cameron (Chicago, IL: University of Chicago Press), 19–61.
Šumbera, R., Šklíba, J., Elichová, M., Chitaukali, W. N., and Burda, H. (2008). Natural history and burrow system architecture of the silvery mole-rat from Brachystegia woodland. J. Zool. 274, 77–84. doi: 10.1111/j.1469-7998.2007.00359.x
Thomas, H. G., Swanepoel, D., and Bennett, N. C. (2016). Burrow architecture of the Damaraland mole-rat (Fukomys damarensis) from South Africa. Afr. Zool. 51, 29–36. doi: 10.1080/15627020.2015.1128355
Thorley, J. (2020). The case for extended lifespan in cooperatively breeding mammals: a re-appraisal. PeerJ 8:e9214. doi: 10.7717/peerj.9214
Thorley, J., Bensch, H., Finn, K., Clutton-Brock, T., and Zöttl, M. (2021). Fitness of breeders in social Damaraland mole-rats is independent of group size. bioRxiv [Preprint] doi: 10.1101/2021.12.08.471794
Tomkiewicz, S. M., Fuller, M. R., Kie, J. G., and Bates, K. K. (2010). Global positioning system and associated technologies in animal behaviour and ecological research. Philos. Trans. R. Soc. B Biol. Sci. 365, 2163–2176. doi: 10.1098/rstb.2010.0090
Torrents-Ticó, M., Bennett, N. C., Jarvis, J. U. M., and Zöttl, M. (2018). Sex differences in timing and context of dispersal in Damaraland mole-rats (Fukomys damarensis). J. Zool. 306, 252–257. doi: 10.1111/jzo.12602
Visser, J. H., Bennett, N. C., and Janse van Vuuren, B. (2018). Spatial genetic diversity in the Cape mole-rat, Georychus capensis: extreme isolation of populations in a subterranean environment. PLoS One 13:e0194165. doi: 10.1371/journal.pone.0194165
Welborn, S. R., and Light, J. E. (2014). Population genetic structure of the Baird’s pocket gopher, Geomys breviceps, in eastern Texas. West. N. Am. Nat. 74, 325–334. doi: 10.3398/064.074.0312
Whitmee, S. (2010). Dispersal and the Distributions of Mammals: Moving Towards Improved Predictions. Doctoral dissertation. London: Imperial College London.
Whitmee, S., and Orme, D. (2012). Predicting dispersal distance in mammals: a trait-based approach. J. Anim. Ecol. 82, 211–221. doi: 10.1111/j.1365-2656.2012.02030.x
Wilmers, C. C., Nickel, B., Bryce, C. M., Smith, J. A., Wheat, R. E., and Yovovich, V. (2015). Golden age of bio-logging: how animal-borne sensors are advancing the frontiers of ecology. Ecology 96, 1741–1753. doi: 10.1890/14-1401.1
Young, A. C., Oosthuizen, M. K., Lutermann, H., and Bennett, N. C. (2010). Physiological suppression eases in Damaraland mole-rat societies when ecological constraints on dispersal are relaxed. Horm. Behav. 57, 177–183. doi: 10.1016/j.yhbeh.2009.10.011
Keywords: natal dispersal, ecological constraints, subterranean, fossorial, rodents, Damaraland mole-rat, Bathyergidae, cooperative breeder
Citation: Finn KT, Thorley J, Bensch HM and Zöttl M (2022) Subterranean Life-Style Does Not Limit Long Distance Dispersal in African Mole-Rats. Front. Ecol. Evol. 10:879014. doi: 10.3389/fevo.2022.879014
Received: 18 February 2022; Accepted: 19 May 2022;
Published: 14 June 2022.
Edited by:
Sabine Begall, University of Duisburg-Essen, GermanyReviewed by:
Jan Šklíba, University of South Bohemia in České Budějovice, CzechiaEileen A. Lacey, University of California, Berkeley, United States
Copyright © 2022 Finn, Thorley, Bensch and Zöttl. This is an open-access article distributed under the terms of the Creative Commons Attribution License (CC BY). The use, distribution or reproduction in other forums is permitted, provided the original author(s) and the copyright owner(s) are credited and that the original publication in this journal is cited, in accordance with accepted academic practice. No use, distribution or reproduction is permitted which does not comply with these terms.
*Correspondence: Kyle T. Finn, a3lsZXRmaW5uQGdtYWlsLmNvbQ==; Markus Zöttl, bWFya3VzLnpvdHRsQGxudS5zZQ==
†ORCID: Kyle T. Finn, orcid.org/0000-0003-3119-9510; Jack Thorley, orcid.org/0000-0002-8426-610X; Hanna M. Bensch, orcid.org/0000-0002-8449-9843; Markus Zöttl, orcid.org/0000-0002-5582-2306