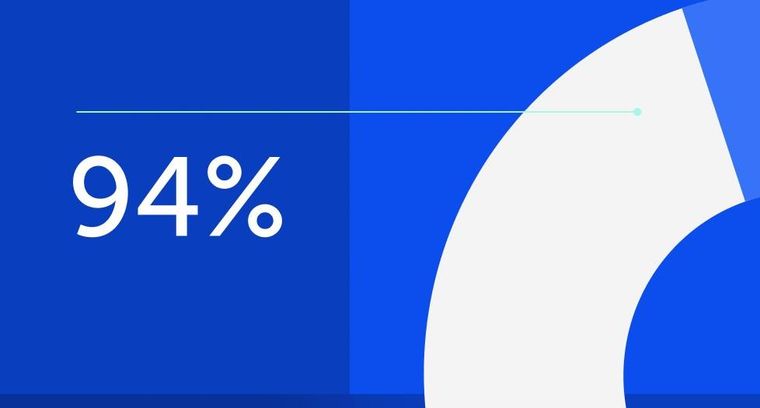
94% of researchers rate our articles as excellent or good
Learn more about the work of our research integrity team to safeguard the quality of each article we publish.
Find out more
ORIGINAL RESEARCH article
Front. Ecol. Evol., 20 June 2022
Sec. Behavioral and Evolutionary Ecology
Volume 10 - 2022 | https://doi.org/10.3389/fevo.2022.877221
This article is part of the Research TopicRecent Advances in the Ecology and Evolution of the BathyergidaeView all 15 articles
The social mole-rats of the family Bathyergidae show elaborate social organisation that may include division of labour between breeders and non-breeders as well as across non-breeders within their groups. However, comparative behavioural data across the taxa are rare and contrasts and similarities between species are poorly understood. Field studies of social bathyergids usually involve capturing all group members until the entire group is captured. Because each animal is only captured once and traps are typically placed in close proximity to active foraging areas, the order in which animals are captured provides an indication of the foraging activity of different individuals and of the spatial organisation of the group within the burrow system. Here, we compare the association of capture order with breeding status, sex, and body mass in four species and subspecies of social bathyergids, which vary in group size and represent all three social genera within the family Bathyergidae. We show that in naked and Damaraland mole-rats (Heterocephalus glaber and Fukomys damarensis), male and female breeders are captured later than non-breeders, whereas in two different subspecies of the genus Cryptomys only female breeders are captured later than non-breeders. The effect sizes vary largely and are 10 times larger in naked mole-rats as compared to Fukomys and 3–4 times larger than in Cryptomys. Among non-breeders, sex effects are notably absent in all species and body mass predicted capture order in both naked and Damaraland mole-rats. In naked mole-rats, larger non-breeders were captured earlier than smaller ones, whereas in Damaraland mole-rats intermediate-sized non-breeders were captured first. Our data suggest that there are similarities in behavioural structure and spatial organisation across all social bathyergid species, though the most pronounced differences within groups are found in naked mole-rats.
The African mole-rats (family Bathyergidae) currently consist of 30 species with varying degrees of sociality, from solitary living to cooperatively breeding groups (Jarvis and Bennett, 1993; Faulkes et al., 1997; Bennett and Faulkes, 2000; Visser et al., 2019; Faulkes and Bennett, 2021). Group living occurs in three genera (Heterocephalus, Fukomys, and Cryptomys) and has probably evolved twice independently, once in Heterocephalus and once in the ancestral state of the Fukomys/Cryptomys complex (Jarvis and Bennett, 1993; Faulkes et al., 1997; Faulkes and Bennett, 2016). Although there are large differences in the mean and the maximum group sizes across these three genera, there are also important similarities in the social organisation of mole-rats (Bennett and Faulkes, 2000). Mole-rat groups mostly consist of a monogamous pair and their offspring that delay dispersal and remain philopatric for extended periods, generating groups with high reproductive skew and high average relatedness between individuals (Burland et al., 2002; Bishop et al., 2004; Patzenhauerová et al., 2013). The main cooperative activity of all group members is to contribute to excavating a large underground burrow system that provides access to their main food source, which are plant tubers and roots (Brett, 1991; Bennett and Faulkes, 2000; Šumbera et al., 2012; Thomas et al., 2016). The extensive involvement of non-breeders in these activities and the possibility that, like in social insects, some individuals may specialise in certain activities have generated substantial research interest in division of labour in social mole-rat groups. However, data from wild populations are rare, and it remains unclear to what extent different species show similarities in their behavioural organisation (Jarvis, 1981; Bennett, 1990; Braude, 1991; Lacey and Sherman, 1991; Lacey and Sherman, 1997; Scantlebury et al., 2006; Zöttl et al., 2016a; Siegmann et al., 2021).
Studies of social mole-rats in captivity highlight that investment in cooperative burrowing often differs between breeders and non-breeders and across non-breeders of different sizes. In captive naked mole-rats (Heterocephalus glaber), both male and female breeders may be substantially less involved in burrowing than non-breeders (Jarvis, 1981; Jarvis et al., 1991). Some studies have also suggested that smaller non-breeding individuals spend more time excavating than larger non-breeders (Jarvis, 1981; Faulkes et al., 1991; Lacey and Sherman, 1991; Jacobs and Jarvis, 1996) though other studies identified large variation around these patterns and suggest that body size may not be a particularly good predictor of burrowing activity (Jarvis et al., 1991; Lacey and Sherman, 1991, 1997; Mooney et al., 2015; Gilbert et al., 2020; Siegmann et al., 2021). In the second social species that has been studied in detail in captivity, the Damaraland mole-rat (Fukomys damarensis), breeders show substantial contributions to burrowing behaviour, especially in relatively small groups, whereas in larger groups the relative time investment of breeders in burrowing behaviour is lower than that of non-breeders (Houslay et al., 2020). Some studies suggested that small non-breeding individuals invest more time in burrowing behaviour than larger non-breeders (Bennett, 1990; Jacobs et al., 1991; Gaylard et al., 1998) though later studies on a large number of known-age individuals showed that, as individuals grow, they increase their investment in burrowing behaviour until they reach the age of 1–1.5 years when rates of burrowing start to decline (Zöttl et al., 2016a; Thorley et al., 2018). This decline coincides with the age of dispersal in wild groups and produces a pattern where individuals of intermediate body sizes may be the individuals with the highest time investment in cooperative burrowing (Zöttl et al., 2016a; Thorley et al., 2018; Torrents-Ticó et al., 2018a,b). Substantially less research has focussed on the cooperative behaviour of other species in the genera Fukomys and Cryptomys; those studies that have done so find that while age effects on general activity are often present, there are rarely consistent differences related to breeder status or body mass (Bennett, 1989; Burda, 1989, 1990; Moolman et al., 1998; Schielke et al., 2012; Van Daele et al., 2019).
Behavioural studies of social mole-rats in the wild are rare, and it is possible that the effects of breeding status and body mass on cooperative behaviour may be more pronounced than in captivity. For example, in Damaraland mole-rats, the difference in foraging investment between breeders and non-breeders was larger in a wild population than in a captive population (Francioli et al., 2020; Houslay et al., 2020). Additionally, radio tracking of giant mole-rats and Ansell’s mole-rats (Fukomys mechowii and Fukomys anselli) suggested that space use and activity patterns differed between breeders and non-breeders and possibly also across non-breeders of different sizes (Lövy et al., 2013; Šklíba et al., 2014, 2016). By contrast, a recent study on Natal mole-rats (Cryptomys hottentotus natalensis) did not detect any differences in foraging activity of breeders and non-breeders, or across non-breeders of different sizes (Finn et al., 2022). However, it remains difficult to compare the results from these studies quantitatively because they employ different biologging methodologies–either radio-tracking or RFID-based monitoring systems (radio-frequency identification)–leading to different types of data and often largely contrasting sample sizes. Additionally, the species of different genera often differ in mean group size and the comparability of data across different species of mole-rats would be greatly improved if the data were collected with similar methods and normalised across group sizes to control for differences in mean group size between species.
One source of information on the behavioural organisation of social mole-rats in the wild is the order in which individuals are captured from groups (“capture order”; Jarvis, 1985; Bennett, 1989; Brett, 1991; Bennett and Faulkes, 2000). When capturing social mole-rats, Hickman traps baited with sweet potato are placed in underground tunnels close to active foraging areas (Hickman, 1979). This means that within groups, individuals that are more active in these foraging areas will have a higher probability of being captured early on, whereas individuals that visit these areas less often will likely be captured later. As a consequence, capture order can give an indication of division of labour and spatial organisation within groups of social mole-rats and has the advantage of using a standardised method across species. Previous field studies of naked and Damaraland mole-rats suggested that reproductive females are often captured among the last animals within groups (Brett, 1991; Jacobs et al., 1991). Across non-breeders, body mass may affect capture order in naked mole-rats, though the evidence is mixed with some studies finding a negative correlation between body mass and capture order in a majority of groups (5 out of 6 groups, Brett, 1991) and other studies only finding such a relationship in some of the groups (4 out of 12, Jarvis, 1985). Among Damaraland mole-rats and common mole-rats (Cryptomys hottentotus), such correlations have rarely been found (Bennett, 1989; Jacobs et al., 1991; Bennett et al., 1994; Voigt et al., 2019).
Here, we use capture order data from field studies of four species and subspecies of bathyergid mole-rats (naked mole-rats, Damaraland mole-rats, Natal mole-rats [Cryptomys hottentotus natalensis], and Mahali mole-rats [Cryptomys hottentotus mahali]), representing all three major social taxa Heterocephalus, Fukomys, and Cryptomys, as an index of variation of cooperative behaviour across these species. To ensure comparability between the species we use the same trapping techniques in all four species and use a z-transformation to control for differences in mean group size across the species (Cheadle et al., 2003; Curtis et al., 2016; Verhulst, 2020). This transformation produces a normalised capture index where capture rank is represented in standard deviations from the mean capture rank within each group ensuring that model estimates of effect sizes from species with different group and sample sizes are directly comparable. We first assess whether breeding males and breeding females are captured later than non-breeders and secondly analyse the association between capture order and body mass and sex in all four species. Finally, we compare the effect sizes of breeder status and body mass across all four species to assess their similarities in division of labour and spatial organisation.
We captured naked mole-rats (Heterocephalus glaber) in Meru National Park, Kenya within a 5 km radius of the Bawatherongi campsite (0.163389°, 38.212167°) between May 1 and August 20, 1989. Each group was trapped only once that year using a modification of the Hickman trap (Hickman, 1979). Active burrows were identified by the fresh volcano shaped pile of soil kicked up by the mole-rats. The tunnels beneath the volcanoes were excavated for as little as 10 cm and no more than 1 m. The traps were placed at opened tunnels, covered with soil, and shaded. Subsequently, we visually monitored the traps and removed the animals immediately upon capture. Trapping began at sunrise and ended at sunset due to the high density of lions within the park. Up to 6 traps were set in a cluster of volcanoes. Mole-rats were numbered with a permanent marker and kept in a plastic basin full of soil in the shade until the end of the trapping day. Sweet potato was placed in the tunnel openings overnight to prevent untrapped mole-rats from blocking the tunnels and to encourage them to continue visiting the location. All animals were housed in 1 × 0.5 m rectangular metal boxes in the laboratory until the entire group was captured. Complete capture was determined by the observation that no sweet potato was eaten overnight, no mole-rats were captured, and tunnels remained unblocked for 3 days. The animals were weighed, sexed, and their breeding status was determined by visual inspection (Braude et al., 2001). Breeding females were identified by their elongated bodies, perforate vagina, and prominent nipples. Breeding males were identified by their prominent genitalia and very thin bodies. Animals were then permanently marked by toe-clipping and were released back into the burrow from which they were captured.
In total, the dataset consisted of 11 groups, including 676 individuals (23 breeders and 653 non-breeders). Protocols were approved by and conducted under the auspices of the Research Division of the Office of the President of Kenya, Permit #15C/116.
We captured Damaraland mole-rats (Fukomys damarensis) in the area surrounding the Kuruman River Reserve (−26.978560°, 21.832459°) in the Kalahari Desert of South Africa between September 2013 and May 2020 (Finn et al., 2018; Thorley et al., 2021). The groups were trapped every 6–12-months using modified Hickman traps that were baited with sweet potato (Hickman, 1979). When foraging and expanding their tunnel systems, mole-rats create characteristic mounds of sand and we accessed their burrows by digging a trench between mounds and exposing the tunnel. Subsequently, we trapped all individuals, one by one, until each individual of the group was captured and all traps remained untouched for at least 24 h. During capturing, the traps were checked every 2–3 h throughout the day. After capture, the mole-rats were kept in a closed box and transported to the laboratory where they were sexed, weighed to the nearest gram and their breeding status was determined. For each individual we also recorded the capture time and derived a capture order as a rank-index where the first individual captured in a group received the index “1,” the second the number “2,” etc. Individuals that were captured at the same time, both received a tied rank index number (e.g., 7.5 if they were captured as 7th and 8th individual). The presence of ties may slightly reduce existing variance in capture ranks but is unlikely to bias patterns of capture order. While in the laboratory the captured animals were housed in custom built tunnel systems made out of PVC tubes with Perspex windows and provided with nesting material and sweet potato. Breeding females are commonly the largest females in their group and can be identified from their perforated vagina and prominent teats, whereas breeding males were identified using longitudinal capture records and body mass data. All captured individuals were marked with passive integrated transponder (PIT; Trovan Unique, DorsetID, Netherlands) tags on the first capture to facilitate individual identification throughout the study.
In total, the dataset consisted of 91 groups, 1,209 individuals, and 2,588 individual capture events (507 breeders and 2,081 non-breeders).
The fieldwork protocols were reviewed and approved by the Animal ethics committee of the University of Pretoria (EC032-13, EC006-15, EC050-16, EC059-18) and subsequently approved by Northern Cape Nature Conservation.
We captured Natal mole-rats (Cryptomys hottentotus natalensis) during field work at Glengarry Holiday Farm in KwaZulu-Natal, South Africa (−29.322530°, 29.712982°) from August 2019 to July 2021. The groups were captured with the same methods as described for Damaraland mole-rats, except that traps were checked from sunrise through to 10 p.m., and the door to the trap closed overnight during winter if the air temperature was near freezing. The groups were recaptured at 6-month intervals. Group membership was initially determined by capture location, a lack of aggression between individuals, and later confirmed by recapture of individuals in the same group during subsequent captures. For each individual we also recorded the capture time and derived a capture order as a rank-index where the first individual captured in a group received the index “1,” the second the number “2,” etc. Individuals that were captured at the same time, both received a tied rank index number (e.g., 7.5 if they were captured as 7th and 8th individual). The captured mole-rats were housed with family members at ambient temperature (15–25°C) in large boxes provided with wood shavings as bedding and fed sweet potato ad libitum. All animals were sexed, weighed to the nearest gram, and assigned a reproductive status at capture. Reproductive females were identified by a perforate vagina and prominent teats (Bennett and Faulkes, 2000). Male reproductive status was determined by body mass, presence of testes, and prolonged presence in the group. All individuals greater > 20 g were implanted with a subcutaneous PIT tag (Trovan Unique, DorsetID, Netherlands) to uniquely identify them. After 48 h without any signs of individuals present in the burrow system (i.e., no blocked tunnels, fresh mounds, triggered traps, or bait eaten), the group was assumed to be completely captured and the group was released back into their burrow.
In total, the dataset consisted of 47 groups, 385 individuals, and 577 individual capture events (125 breeders and 452 non-breeders). The field protocols were approved by the animal ethics committee of the University of Pretoria (EC001-19) and the Department of Forestry and Fisheries (DALRRD section 20 12/11/1/8, 1468). Permission to trap the mole-rats was granted by Ezemvelo Nature Conservation (Permit OP27-2020, OP1545-2021).
We captured Mahali mole-rat (Cryptomys hottentotus mahali) groups between October 2016 and September 2017 at Patryshoek, Pretoria (−25.663333°, 28.039583°), South Africa. The mole-rats were captured using Hickman traps, baited with a small piece of sweet potato (Hickman, 1979). The traps were placed at the entrance of excavated burrows, in close proximity to mole-rat mounds. We captured the entire group, and a group was considered completely captured when no trap activity (i.e., tunnel blocking or animal capture) was observed within five consecutive days. For a different study purpose, the animals were permanently removed and subsequently sacrificed (Hart et al., 2021). On capture, the body mass of each animal was recorded to the nearest 0.01 g. The breeding males were distinguishable from non-breeding males by their large descended inguinal testes and yellow staining around the mouth. Furthermore, the breeding males were usually, but not always, the largest male in each group (Bennett and Faulkes, 2000; Hart et al., 2021). The breeding females possessed prominent axillary teats and a perforated vagina, which was absent in the non-breeding females. During the dissection process, the breeding status of females was confirmed by the presence of foetuses or placental scars on the uterine horns of breeding females.
In total, the dataset consisted of 32 groups including 265 individuals (65 breeders and 200 non-breeder). The field protocols were approved by the Animal Ethics Committee of the University of Pretoria (No. EC044-16) and the Department of Forestry and Fisheries DALRRD section 20 12/11/1/8 (MG). Permission to trap the mole-rats was granted by the Gauteng Department of Nature Conservation (Permit No. CPF6-0127).
For all four species we removed groups where the group size was smaller than three individuals and groups where no reproductive females were captured. We also removed juvenile individuals that are known to contribute very little to cooperative behaviour in the groups (Lacey and Sherman, 1991; Zöttl et al., 2016a) with cut-off weights that correspond roughly to 20% of adult body mass (naked mole-rat 15 g, Damaraland mole-rat 50 g, Natal mole-rat, and Mahali mole-rat 40 g).
To facilitate direct comparability of the effect sizes and alleviate the differences arising from contrasts in mean group size across species, we z-transformed the capture index for each group capture event using the “scale” function in R (hereafter referred to as z-score). Transforming data to a z-score is achieved by first subtracting the mean capture index for a given group capture from each individual capture index. This results in negative values for early captured individuals and positive values for late captured individuals. Subsequently, we divided the mean centred data by the standard deviation of the capture index per group. The result of this transformation is that each individual’s z-score now represents the capture order expressed in standard deviations (SD) distance from the mean group size. This z-score is directly comparable between species that differ in group size and similar transformations are commonly used in meta-analyses or for comparing results that are biassed by analytical batch effects (Cheadle et al., 2003; Curtis et al., 2016; Verhulst, 2020).
To estimate the effects of breeding status and sex on capture order we fitted a linear mixed effect model with the capture order z-score as the response variable, and sex and breeding status as fixed factors, including the interaction between sex and breeding status. Because some individuals were captured repeatedly over the study population in Damaraland mole-rats and Natal mole-rats, we also included a random effect of animal identity for these species. In contrast, in naked mole-rats and in Mahali mole-rats no repeated captures of the same individuals were recorded, so we instead fitted the equivalent linear model (omitting the random effect).
To assess whether body mass among non-breeding individuals is associated with capture order, we excluded all breeders from the data set and carried out the z-transformations for capture order index and body mass. Because males and females in three of the four species show pronounced sexual dimorphism in body mass, we applied the same z-transformation for females and males within each species (Schielzeth, 2010). Subsequently, we fitted a linear mixed effect model with the capture order index (z-score) as the response variable, sex as fixed factor, body mass (z-score) and squared body mass (z-score, allowing non-linear relationships) as covariates. Again, in models for Damaraland mole-rats and Natal mole-rats we included the animal identity as a random effect, whereas for naked and Mahali mole-rats we fitted the equivalent linear model, omitting any random effects.
All analyses were carried out in R (R Core Team, 2019).
In both naked mole-rats and Damaraland mole-rats breeders were captured later than non-breeders. Though the results are qualitatively similar, the effect size of breeders being captured later was about 10 times higher in naked mole-rats than in Damaraland mole-rats (Tables 1A,B and Figure 1). It is important to note that because the response variable was z-transformed within each group, differences in group size cannot explain differences in effect size. Similarly, breeding females of Natal mole-rats and Mahali mole-rats were captured later than non-breeders (Tables 1C,D and Figure 1). However, in contrast to naked and Damaraland mole-rats, breeding males of these species clustered with non-breeders and were captured significantly earlier than breeding females (Tables 1C,D and Figure 1). In these two species, the effect sizes of the breeding females being captured later were about 3–4 times higher than in Damaraland mole-rats and about 3–4 times lower than in naked mole-rats (Figure 1).
Table 1. The effects of breeder status, sex, and body mass on capture order in four species of social bathyergid mole-rats.
Figure 1. Effect size estimates for breeder status and sex on capture order across naked mole-rats, Damaraland mole-rats, Natal mole-rats, and Mahali mole-rats. Error bars represent 95% confidence intervals. Photographs by Kyle T. Finn and Yannick Francioli.
Across non-breeders, in naked mole-rats we found that larger individuals were captured earlier than smaller group members and there was no indication of a convincing quadratic effect of body mass on capture order (Table 1A and Figure 2A). In contrast, in Damaraland mole-rats, body mass has a quadratic effect on capture order, indicating that intermediate sizes of non-breeders were captured first, and smaller and larger non-breeders both were captured later (Table 1B and Figure 2B). Also, with respect to this result, it is noteworthy that the effect size in naked mole-rats was larger than the body mass effect in Damaraland mole-rats. We did not find an effect of body mass on capture order in Natal and Mahali mole-rats. Because the sample sizes in these two species were substantially smaller than in Damaraland mole-rats, effects of similar size and uncertainty as in Damaraland mole-rats would not have been statistically significant in these species (Tables 1C,D and Figures 2C,D).
Figure 2. The predicted effects of body mass on capture order of male and female non-breeding group members in (A) Naked mole-rats, (B) Damaraland mole-rats, (C) Natal mole-rats, and (D) Mahali mole-rats. The shaded areas represent 95% confidence intervals and the test statistics can be found in Table 1. Note that the effects displayed in panels (C,D) do not represent statistical significant effects. Photographs by Kyle T. Finn and Yannick Francioli.
Because of the potential similarities to the social organisation of eusocial insects, the social organisation of the African mole-rats has been a widely and controversially debated topic over the last 40 years (Jarvis, 1981; Bennett, 1990; Bennett and Faulkes, 2000; Burda et al., 2000; Scantlebury et al., 2006; Faulkes and Bennett, 2016, 2021; Braude et al., 2021; Thorley et al., 2021; Buffenstein et al., 2022). Despite this interest, our understanding of the behavioural organisation of social mole-rats has been limited by the difficulty of collecting behavioural data from wild populations and by the lack of comparative data across different species of social mole-rats. We used capture order data from wild populations of four species of social bathyergid mole-rats to assess similarities in the behavioural and spatial organisation across the three social genera (Heterocephalus, Fukomys and Cryptomys). Our results suggest that across social mole-rats, breeding females are less active and less involved in cooperative burrowing and foraging. Although there are qualitative similarities in the social organisation of different mole-rat species, our results show that there are large quantitative differences between species. The differences between breeders and non-breeders in cooperative investment are much larger in naked mole-rats than in the Damaraland mole-rat, the Mahali mole-rat, and the Natal mole-rat. Additionally, only in naked mole-rats was capture order among non-breeders strongly associated with body mass, whereas such body mass effects were small in Damaraland mole-rats and possibly absent in both subspecies of Cryptomys hottentotus.
The results of our study align well with some previous studies of naked mole-rat behaviour and help to clarify some of the open questions about labour division in this species. In naked mole-rats, one of the most critical parts of cooperative burrowing is that individuals need to eject the surplus sand from their burrows through open mounds. This is energetically costly and potentially risky because of the exposure to predators (Jarvis, 1981; Braude, 1991; Brett, 1991) and previous studies suggested that large individuals may engage more in this activity than small individuals (Braude, 1991). Our data corroborate these suggestions by showing that in naked mole-rats, large non-breeders are captured earlier than smaller ones when the traps baited with sweet potato are placed in close proximity to active volcanoes. Because burrowing and ejecting sand from tunnels is not possible in captivity, studies in artificial tunnels systems have quantified this behaviour by recording how much substrate is moved by individuals and by the amount of time individuals spend gnawing on obstructions or the tunnel walls. These studies show that sometimes smaller individuals are more active (Jarvis, 1981; Jarvis et al., 1991; Jacobs and Jarvis, 1996) and that there is large behavioural variation that cannot be explained by differences in body mass (Lacey and Sherman, 1991, 1997; Gilbert et al., 2020; Siegmann et al., 2021). Our results are inconsistent with a clear negative relationship between cooperative burrowing and body mass and largely consistent with studies emphasising that there is substantial between-individual variation in burrowing activity that is unrelated to body mass. Additionally, it is important to appreciate that capture order in naked mole-rats is probably a better indicator of sand ejecting behaviour (i.e., volcanoeing) than of general activity or digging activity in more central areas of the burrow.
Across all social African mole-rats, we have the best understanding of the cooperative behaviour in Damaraland mole-rats and our comparative data yield insights into division of labour of other social mole-rat species by extension (Bennett, 1990; Gaylard et al., 1998; Scantlebury et al., 2006; Zöttl et al., 2016a; Thorley et al., 2018). In Damaraland mole-rats digging activity increases with body mass early in life before it starts to decline around the time of dispersal when individuals also reach their maximum body mass (Zöttl et al., 2016a,b; Thorley et al., 2018) and estimates of relative foraging activity in wild groups show that breeders exhibit a 50% reduction of foraging activity as compared to non-breeders (Francioli et al., 2020). These patterns are also reflected in our capture order data by showing that breeders of both sexes are captured later, and that intermediate sizes of non-breeders are captured earlier than smaller and larger individuals. If the effect size of capture order in Damaraland mole-rats reflects a 50% decrease in cooperative burrowing by breeders, this would suggest by extension that in naked mole-rats breeders may only show around 5% of the burrowing investment of non-breeders (10 fold difference in effect size) and that in the species of the genus Cryptomys breeding females may show intermediate levels of relative burrowing investment. In contrast to Damaraland mole-rats and Naked mole-rats, breeding males in Natal and Mahali mole-rats were not captured later than non-breeding males, which indicates that their levels of cooperative burrowing may be similar to those non-breeding group members. In line with other studies (Bennett, 1989; Moolman et al., 1998; Bennett and Faulkes, 2000), in both subspecies of Cryptomys we did not detect any significant body mass related patterns of capture order and it is likely that if such effects existed then they would be small, as in Damaraland mole-rats.
Previous studies in different mole-rat species suggested that although some species show pronounced size dimorphism, this rarely translates into behavioural differences between the sexes (Jarvis et al., 1991; Schielke et al., 2012; Zöttl et al., 2016a,b, 2018; Gilbert et al., 2020; Caspar et al., 2021; Siegmann et al., 2021). Also, in our study, behavioural sex differences are notably absent in all species of social mole-rats. This pattern is unusual across cooperative breeders where sex differences in helping behaviour are common and often linked to sex differences in philopatry and the relatedness (Clutton-Brock et al., 2002; Russell, 2004; Stiver et al., 2006; Downing et al., 2018) though may be expected for a breeding system as in social mole-rats where some of the key incentives for the evolution of sex differences of helping behaviours are missing. In social mole-rats mean relatedness of the sexes to the offspring is often similar because monogamous pairs are common (Burland et al., 2002; Patzenhauerová et al., 2013). Additionally, males and females only differ marginally in the duration of philopatry, disperse at similar rates and rarely inherit a breeding territory (Hazell et al., 2000; Torrents-Ticó et al., 2018a,b; Mynhardt et al., 2021; Thorley et al., 2021), which is expected to limit the opportunity for delayed direct fitness benefits to disproportionately favour one sex helping more than the other.
In conclusion, our study of capture rates across social mole-rats suggests that there are broad similarities in the division of labour across all three main social genera Heterocephalus, Fukomys, and Cryptomys. However, by controlling for differences in mean group size across species through normalising the capture order data we were able to show that the effect sizes of differences between breeders and non-breeders, and across non-breeders of different sizes were many-fold larger in naked mole-rats than in any other species included in this study. Our results suggest that while some behavioural differences in burrowing activity between breeders and non-breeders and across non-breeders occur in most social mole-rat species, the naked mole-rat may show substantially more pronounced behavioural contrasts than all other species.
All data and code is available in a public repository (https://figshare.com/articles/dataset/Data_and_R-Code_for_Capture_ order_across_social_bathyergids_indicate_similarities_in_behav ioural_and_spatial_organisation/19524937).
The animal study was reviewed and approved by Animal Ethics Committee of the University of Pretoria (EC032-13, EC006-15, EC050-16, EC059-18, EC044-16, and EC001-19).
MZ conceived to the idea and analysed the data together with HB. MZ wrote the manuscript with input from HB, KF, DH, JT, NB, and SB. All authors contributed to the data collection and approved the final submission.
MZ acknowledged funding from Vetenskapsrådet (2017-05296) and Crafoordska Stiftelsen (2018-2259 and 2020-0976). NB acknowledged funding from the Department of Science and Technology and National Research Foundation (GUN 64756).
The authors declare that the research was conducted in the absence of any commercial or financial relationships that could be construed as a potential conflict of interest.
All claims expressed in this article are solely those of the authors and do not necessarily represent those of their affiliated organizations, or those of the publisher, the editors and the reviewers. Any product that may be evaluated in this article, or claim that may be made by its manufacturer, is not guaranteed or endorsed by the publisher.
We thank Shay Rotics and Oscar Nordahl for helpful discussions and all co-workers and field assistants involved in the field studies. In particular, we want to thank Tim Clutton-Brock for his contribution to the data collection for Damaraland mole-rats and to Marta Manser for her contribution to managing the Kalahari River Reserve. We are grateful to the Mammal Research Institute at the University of Pretoria for their continuous support and the Northern Cape Department of Environment and Nature Conservation for permission to conduct research in the Northern Cape. We are grateful to the Gauteng Department of Nature Conservation, Ezemvelo National Parks for permits to trap Mahali and Natal mole-rats, respectively. Finally, we aslo thank DALRRD for the issuing of section 20 permits to undertake research in South Africa and the Office of the President of Kenya for permission to conduct research in Meru National Park.
Bennett, N. (1989). The social structure and reproductive biology of the common mole-rat, Cryptomys h. hottentotus and remarks on the trends in reproduction and sociality in the family Bathyergidae. J. Zool. 219, 45–59. doi: 10.1111/j.1469-7998.1989.tb02564.x
Bennett, N. (1990). Behaviour and social organization in a colony of the Damaraland mole-rat Cryptomys damarensis. J. Zool. 220, 225–247. doi: 10.1111/j.1469-7998.1990.tb04305.x
Bennett, N., Jarvis, J., and Cotterill, F. (1994). The colony structure and reproductive biology of the afrotropical Mashona mole-rat, Cryptomys darlingi. J. Zool. 234, 477–487. doi: 10.1111/j.1469-7998.1994.tb04861.x
Bennett, N. C., and Faulkes, C. G. (2000). African Mole-Rats: Ecology and Eusociality. Cambridge: Cambridge University Press.
Bishop, J., Jarvis, J., Spinks, A., Bennett, N., and O’ryan, C. (2004). Molecular insight into patterns of colony composition and paternity in the common mole-rat Cryptomys hottentotus hottentotus. Mol. Ecol. 13, 1217–1229. doi: 10.1111/j.1365-294X.2004.02131.x
Braude, S. (1991). “Which naked mole-rats volcano?,” in The Biology of the Naked Mole-Rat, eds P. W. Sherman, U. M. Jarvis, and R. D. Alexander (Princeton, NJ: Princeton University Press).
Braude, S., Ciszek, D., Berg, N. E., and Shefferly, N. (2001). The ontogeny and distribution of countershading in colonies of the naked mole-rat (Heterocephalus glaber). J. Zool. 253, 351–357. doi: 10.1017/S0952836901000322
Braude, S., Holtze, S., Begall, S., Brenmoehl, J., Burda, H., Dammann, P., et al. (2021). Surprisingly long survival of premature conclusions about naked mole-rat biology. Biol. Rev. 96, 376–393. doi: 10.1111/brv.12660
Brett, R. A. (1991). “The ecology of naked mole-rat colonies: burrowing, food, and limiting factors,” in The Biology of the Naked Mole-Rat, eds P. W. Sherman, J. U. M. Jarvis, and R. D. Alexander (Princeton, NJ: Princeton University Press), 137–184. doi: 10.1515/9781400887132-008
Buffenstein, R., Amoroso, V., Andziak, B., Avdieiev, S., Azpurua, J., Barker, A. J., et al. (2022). The naked truth: a comprehensive clarification and classification of current ‘myths’ in naked mole-rat biology. Biol. Rev. 97, 115–140. doi: 10.1111/brv.12791
Burda, H. (1989). Reproductive biology (behaviour, breeding, and postnatal development) in subterranean mole-rats, Cryptomys hottentotus (Bathyergidae). Z. Säugetierkd. 54, 360–376.
Burda, H. (1990). Constraints of pregnancy and evolution of sociality in mole-rats With special reference to reproductive and social patterns in Cryptomys hottentotus (Bathyergidae, Rodentia) 1. J. Zool. Syst. Evol. Res. 28, 26–39.
Burda, H., Honeycutt, R. L., Begall, S., Locker-Grütjen, O., and Scharff, A. (2000). Are naked and common mole-rats eusocial anf if so, why? Behav. Ecol. Sociobiol. 47, 293–303. doi: 10.1111/j.1439-0469.1990.tb00362.x
Burland, T. M., Bennett, N. C., Jarvis, J. U., and Faulkes, C. G. (2002). Eusociality in African mole-rats: new insights from patterns of genetic relatedness in the Damaraland mole-rat (Cryptomys damarensis). Proc. R. Soc. Lond. Ser. B Biol. Sci. 269, 1025–1030. doi: 10.1098/rspb.2002.1978
Caspar, K. R., Müller, J., and Begall, S. (2021). Effects of sex and breeding status on skull morphology in cooperatively breeding ansell’s mole-rats and an appraisal of sexual dimorphism in the bathyergidae. Front. Ecol. Evol. 9:638754. doi: 10.3389/fevo.2021.638754
Cheadle, C., Vawter, M. P., Freed, W. J., and Becker, K. G. (2003). Analysis of microarray data using Z score transformation. J. Mol. Diagn. 5, 73–81. doi: 10.1016/S1525-1578(10)60455-2
Clutton-Brock, T. H., Russell, A. F., Sharpe, L. L., Young, A. J., Balmforth, Z., and Mcilrath, G. M. (2002). Evolution and development of sex differences in cooperative behavior in meerkats. Science 297, 253–256. doi: 10.1126/science.1071412
Curtis, A. E., Smith, T. A., Ziganshin, B. A., and Elefteriades, J. A. (2016). The mystery of the Z-score. Aorta 4, 124–130. doi: 10.12945/j.aorta.2016.16.014
Downing, P. A., Griffin, A. S., and Cornwallis, C. K. (2018). Sex differences in helping effort reveal the effect of future reproduction on cooperative behaviour in birds. Proc. R. Soc. B 285:20181164. doi: 10.1098/rspb.2018.1164
Faulkes, C., Abbott, D., Liddell, C., George, L., and Jarvis, J. (1991). “Hormonal and behavioral aspects of reproductive suppression in female naked mole-rats,” in The Biology of the Naked Mole-Rat, eds P. W. Sherman, J. U. M. Jarvis, and R. D. Alexander (Princeton, NJ: Princeton University Press), 426–445. doi: 10.1515/9781400887132-017
Faulkes, C. G., and Bennett, N. C. (2016). Damaraland and Naked Mole-Rats: Convergence of Social Evolution. Cooperative Breeding in Vertebrates: Studies of Ecology, Evolution, and Behavior. Cambridge: Cambridge University Press, 338. doi: 10.1017/CBO9781107338357.020
Faulkes, C. G., and Bennett, N. C. (2021). “Social evolution in African mole-rats–a comparative overview,” in The Extraordinary Biology of the Naked Mole-Rat, eds R. B. Buffenstein, T. J. Park, and M. M. Holmes (Cham: Springer), 1–33. doi: 10.1007/978-3-030-65943-1_1
Faulkes, C. G., Bennett, N. C., Bruford, M. W., O’brien, H. P., Aguilar, G. H., and Jarvis, J. U. M. (1997). Ecological constraints drive social evolution in the African mole–rats. Proc. R. Soc. Lond. B Biol. Sci. 264, 1619–1627. doi: 10.1098/rspb.1997.0226
Finn, K., Parker, D. M., Bennett, N. C., and Zöttl, M. (2018). Contrasts in body size and growth suggest that high population density results in faster pace of life in Damaraland mole-rats (Fukomys damarensis). Can. J. Zool. 96, 920–927. doi: 10.1139/cjz-2017-0200
Finn, K. T., Janse Van Vuuren, A. K., Hart, D. W., Süess, T., Zöttl, M., and Bennett, N. C. (2022). Seasonal changes in locomotor activity patterns of wild social natal mole-rats (Cryptomys hottentotus natalensis). Front. Ecol. Evol. 10:819393. doi: 10.3389/fevo.2022.819393
Francioli, Y., Thorley, J., Finn, K., Clutton-Brock, T., and Zöttl, M. (2020). Breeders are less active foragers than non-breeders in wild Damaraland mole-rats. Biol. Lett. 16:20200475. doi: 10.1098/rsbl.2020.0475
Gaylard, A., Harrison, Y., and Bennett, N. C. (1998). Temporal changes in the social structure of a captive colony of the Damaraland mole-rat, Cryptomys damarensis: the relationship of sex and age to dominance and burrow-maintenance activity. J. Zool. 244, 313–321. doi: 10.1111/j.1469-7998.1998.tb00035.x
Gilbert, J. D., Rossiter, S. J., and Faulkes, C. G. (2020). The relationship between individual phenotype and the division of labour in naked mole-rats: it’s complicated. PeerJ 8:e9891. doi: 10.7717/peerj.9891
Hart, D. W., Medger, K., Van Jaarsveld, B., and Bennett, N. C. (2021). Filling in the holes: the reproductive biology of the understudied Mahali mole-rat (Cryptomys hottentotus mahali). Can. J. Zool. 99, 801–811.
Hazell, R., Bennett, N., Jarvis, J., and Griffin, M. (2000). Adult dispersal in the co-operatively breeding Damaraland mole-rat (Cryptomys damarensis): a case study from the Waterberg region of Namibia. J. Zool. 252, 19–25. doi: 10.1111/j.1469-7998.2000.tb00816.x
Hickman, G. (1979). A live-trap and trapping technique fort fossorial mammals. Afr. Zool. 14, 9–12. doi: 10.1080/02541858.1979.11447641
Houslay, T. M., Vullioud, P., Zöttl, M., and Clutton-Brock, T. H. (2020). Benefits of cooperation in captive Damaraland mole-rats. Behav. Ecol. 31, 711–718.
Jacobs, D., Bennett, N., Jarvis, J., and Crowe, T. (1991). The colony structure and dominance hierarchy of the Damaraland mole-rat, Cryptomys damarensis (Rodentia: Bathyergidae), from Namibia. J. Zool. 224, 553–576. doi: 10.1111/j.1469-7998.1991.tb03785.x
Jacobs, D. S., and Jarvis, J. U. M. (1996). No evidence for the work-conflict hypothesis in the eusocial naked mole-rat (Heterocephalus glaber). Behav. Ecol. Sociobiol. 39, 401–409. doi: 10.1007/s002650050307
Jarvis, J. (1985). Ecological studies on Heterocephalus glaber, the naked mole-rat. Kenya. Natl. Geogr. Soc. Res. Rep. 20, 429–437.
Jarvis, J., and Bennett, N. (1993). Eusociality has evolved independently in two genera of bathyergid mole-rats—but occurs in no other subterranean mammal. Behav. Ecol. Sociobiol. 33, 253–260. doi: 10.1007/BF02027122
Jarvis, J., O’riain, M., and Mcdaid, E. (1991). “Growth and factors affecting body size in naked mole-rats,” in The Biology of the Naked Mole-Rat, eds P. W. Sherman, J. U. M. Jarvis, and R. D. Alexander (Princeton, NJ: Princeton University Press), 358–383. doi: 10.1515/9781400887132-015
Jarvis, J. U. (1981). Eusociality in a mammal: cooperative breeding in naked mole-rat colonies. Science 212, 571–573. doi: 10.1126/science.7209555
Lacey, E. A., and Sherman, P. W. (1991). “Social organization of Naked Mole-Rat colonies: evidence for divisions of labor,” in The Biology of the Naked Mole-Rat, eds P. W. Sherman, J. U. M. Jarvis, and R. D. Alexander (Princeton NJ: Princeton University Press).
Lacey, E. A., and Sherman, P. W. (1997). “Cooperative breeding in naked mole-rats,” in Cooperative Breeding in Mammals, eds N. G. Solomon and J. A. French (Cambridge: Cambridge University Press).
Lövy, M., Šklíba, J., and Šumbera, R. (2013). Spatial and temporal activity patterns of the free-living giant mole-rat (Fukomys mechowii), the largest social Bathyergid. PLoS One 8:e55357. doi: 10.1371/journal.pone.0055357
Moolman, M., Bennett, N., and Schoeman, A. (1998). The social structure and dominance hierarchy of the highveld mole-rat Cryptomys hottentotus pretoriae (Rodentia: Bathyergidae). J. Zool. 246, 193–201. doi: 10.1111/j.1469-7998.1998.tb00148.x
Mooney, S. J., Filice, D. C., Douglas, N. R., and Holmes, M. M. (2015). Task specialization and task switching in eusocial mammals. Anim. Behav. 109, 227–233. doi: 10.1016/j.anbehav.2015.08.019
Mynhardt, S., Harris-Barnes, L., Bloomer, P., and Bennett, N. C. (2021). Spatial population genetic structure and colony dynamics in Damaraland mole-rats (Fukomys damarensis) from the southern Kalahari. BMC Ecol. Evol. 21:221. doi: 10.1186/s12862-021-01950-2
Patzenhauerová, H., Šklíba, J., Bryja, J., and Šumbera, R. (2013). Parentage analysis of A nsell’s mole-rat family groups indicates a high reproductive skew despite relatively relaxed ecological constraints on dispersal. Mol. Ecol. 22, 4988–5000. doi: 10.1111/mec.12434
R Core Team. (2019). R: A Language and Environment for Statistical Computing. Vienna: R Foundation for Statistical Computing. Available online at: https://www.R-project.org/
Russell, A. F. (2004). “Mammals: comparisons and contrasts,” in Ecology and Evolution of Cooperative Breeding in Birds, eds W. D. Koenig and J. L. Dickinson (Cambridge: Cambridge University Press). doi: 10.1017/CBO9780511606816.014
Scantlebury, M., Speakman, J., Oosthuizen, M., Roper, T., and Bennett, N. (2006). Energetics reveals physiologically distinct castes in a eusocial mammal. Nature 440, 795–797. doi: 10.1038/nature04578
Schielke, C. K., Begall, S., and Burda, H. (2012). Reproductive state does not influence activity budgets of eusocial Ansell’s mole-rats, Fukomys anselli (Rodentia, Bathyergidae): a study of locomotor activity by means of Rfid. Mammal. Biol. 77, 1–5. doi: 10.1016/j.mambio.2011.09.004
Schielzeth, H. (2010). Simple means to improve the interpretability of regression coefficients. Methods Ecol. Evol. 1, 103–113. doi: 10.1016/j.ejrad.2008.03.019
Siegmann, S., Feitsch, R., Hart, D. W., Bennett, N. C., Penn, D. J., and Zöttl, M. (2021). Naked mole-rats (Heterocephalus glaber) do not specialise in cooperative tasks. Ethology 127, 850–864.
Šklíba, J., Lövy, M., Burda, H., and Šumbera, R. (2016). Variability of space-use patterns in a free living eusocial rodent, Ansell’s mole-rat indicates age-based rather than caste polyethism. Sci. Rep. 6:37497. doi: 10.1038/srep37497
Šklíba, J., Lövy, M., Hrouzková, E., Kott, O., Okrouhlík, J., and Šumbera, R. (2014). Social and environmental influences on daily activity pattern in free-living subterranean rodents: the case of a eusocial bathyergid. J. Biol. Rhythms 29, 203–214. doi: 10.1177/0748730414526358
Stiver, K. A., Fitzpatrick, J., Desjardins, J. K., and Balshine, S. (2006). Sex differences in rates of territory joining and inheritance in a cooperatively breeding cichlid fish. Anim. Behav. 71, 449–456. doi: 10.1016/j.anbehav.2005.06.011
Šumbera, R., Mazoch, V., Patzenhauerová, H., Lövy, M., Šklíba, J., Bryja, J., et al. (2012). Burrow architecture, family composition and habitat characteristics of the largest social African mole-rat: the giant mole-rat constructs really giant burrow systems. Acta Theriol. 57, 121–130. doi: 10.1007/s13364-011-0059-4
Thomas, H. G., Swanepoel, D., and Bennett, N. C. (2016). Burrow architecture of the Damaraland mole-rat (Fukomys damarensis) from South Africa. Afr. Zool. 51, 29–36.
Thorley, J., Bensch, H., Finn, K., Clutton-Brock, T., and Zöttl, M. (2021). Fitness of breeders in social Damaraland mole-rats is independent of group size. bioRxiv[Preprint]. 2021.12.08.471794
Thorley, J., Mendonça, R., Vullioud, P., Torrents-Ticó, M., Zöttl, M., Gaynor, D., et al. (2018). No task specialization among helpers in Damaraland mole-rats. Anim. Behav. 143, 9–24. doi: 10.1016/j.anbehav.2018.07.004
Torrents-Ticó, M., Bennett, N. C., Jarvis, J. U., and Zöttl, M. (2018a). Growth affects dispersal success in social mole-rats, but not the duration of philopatry. Biol. Lett. 14:20180005. doi: 10.1098/rsbl.2018.0005
Torrents-Ticó, M., Bennett, N. C., Jarvis, J. U., and Zöttl, M. (2018b). Sex differences in timing and context of dispersal in Damaraland mole-rats (Fukomys damarensis). J. Zool. 306, 252–257. doi: 10.1111/jzo.12602
Van Daele, P. A., Desmet, N., Šumbera, R., and Adriaens, D. (2019). Work behaviour and biting performance in the cooperative breeding Micklem’s mole-rat Fukomys micklemi (Bathyergidae, Rodentia). Mammal. Biol. 95, 69–76.
Verhulst, S. (2020). Improving comparability between qPCR-based telomere studies. Mol. Ecol. Resour. 20, 11–13. doi: 10.1111/1755-0998.13114
Visser, J. H., Bennett, N. C., and Van Vuuren, B. J. (2019). Phylogeny and biogeography of the African Bathyergidae: a review of patterns and processes. PeerJ 7:e7730. doi: 10.7717/peerj.7730
Voigt, C., Ter Maat, A., and Bennett, N. C. (2019). No evidence for multimodal body mass distributions and body mass-related capture order in wild-caught Damaraland mole-rats. Mammal. Biol. 95, 123–126. doi: 10.1016/j.mambio.2018.09.012
Zöttl, M., Vullioud, P., Mendonça, R., Ticó, M. T., Gaynor, D., Mitchell, A., et al. (2016a). Differences in cooperative behavior among Damaraland mole rats are consequences of an age-related polyethism. Proc. Natl. Acad. Sci. U.S.A. 113, 10382–10387. doi: 10.1073/pnas.1607885113
Zöttl, M., Thorley, J., Gaynor, D., Bennett, N. C., and Clutton-Brock, T. (2016b). Variation in growth of Damaraland mole-rats is explained by competition rather than by functional specialization for different tasks. Biol. Lett. 12:20160820. doi: 10.1098/rsbl.2016.0820
Keywords: cooperative breeding, division of labour, helping behaviour, cooperation, Bathyergidae, social evolution, eusociality, cooperative behaviour
Citation: Zöttl M, Bensch HM, Finn KT, Hart DW, Thorley J, Bennett NC and Braude S (2022) Capture Order Across Social Bathyergids Indicates Similarities in Division of Labour and Spatial Organisation. Front. Ecol. Evol. 10:877221. doi: 10.3389/fevo.2022.877221
Received: 16 February 2022; Accepted: 20 May 2022;
Published: 20 June 2022.
Edited by:
Martin J. Whiting, Macquarie University, AustraliaReviewed by:
Hynek Burda, University of Duisburg-Essen, GermanyCopyright © 2022 Zöttl, Bensch, Finn, Hart, Thorley, Bennett and Braude. This is an open-access article distributed under the terms of the Creative Commons Attribution License (CC BY). The use, distribution or reproduction in other forums is permitted, provided the original author(s) and the copyright owner(s) are credited and that the original publication in this journal is cited, in accordance with accepted academic practice. No use, distribution or reproduction is permitted which does not comply with these terms.
*Correspondence: Markus Zöttl, bWFya3VzLnpvdHRsQGxudS5zZQ==
Disclaimer: All claims expressed in this article are solely those of the authors and do not necessarily represent those of their affiliated organizations, or those of the publisher, the editors and the reviewers. Any product that may be evaluated in this article or claim that may be made by its manufacturer is not guaranteed or endorsed by the publisher.
Research integrity at Frontiers
Learn more about the work of our research integrity team to safeguard the quality of each article we publish.