- 1CIBIO/InBio, Centro de Investigação em Biodiversidade e Recursos Genéticos, Laboratório Associado, Universidade do Porto, Vairão, Portugal
- 2CIBIO/InBio, Centro de Investigação em Biodiversidade e Recursos Genéticos, Laboratório Associado, Instituto Superior de Agronomia, Universidade de Lisboa, Lisbon, Portugal
- 3BIOPOLIS Program in Genomics, Biodiversity and Land Planning, CIBIO, Vairão, Portugal
- 4Centro de Estudos Florestais, Instituto Superior de Agronomia, Universidade de Lisboa, Lisbon, Portugal
- 5Laboratório Associado Terra, Portugal
- 6Centro de Estudos Geográficos, Instituto de Geografia e Ordenamento do Território, Universidade de Lisboa, Lisbon, Portugal
The number of alien plant species is growing steadily across all world regions. These numbers tend to be exceptionally high in riparian ecosystems, often with substantial negative consequences for native species communities and ecosystem services provision. Here, we map the richness of invasive alien plant species in riparian ecosystems of continental Portugal, assess the relative importance of human and natural factors in shaping the uncovered patterns, and predict richness values along watercourses and at the municipal level for the whole study area. We found a higher richness of invasive alien plants in low altitudes and in downstream areas where human concentration is high. As time progresses, ongoing and increasing levels of socio-economic activity and globalization of plant trade will conceivably lead to a higher number of alien species becoming established. National and sub-national measures aiming to prevent and manage biological invasions in riparian ecosystems require coordinated efforts involving both local entities and those with responsibilities in the management of upstream catchment areas. These efforts must also be targeted to achieve future biodiversity protection goals as part of the EU Biodiversity Strategy for 2030.
Introduction
Global trade and travel activities are leading to an increasing accumulation of alien species across regions of the world (CBD, 2014; Seebens et al., 2017; Beaury et al., 2021). The establishment and subsequent spread of these species in non-native regions have major negative impacts on economic activities and human wellbeing (IPBES, 2019; Diagne et al., 2021), and is among the major threats to biodiversity and species extinction worldwide (Clavero and Garcia-Berthou, 2005; Bellard et al., 2016). Recovering from ecosystem degradation has been one major motivation of global restoration effort and current policies (e.g., UN Decade on Ecosystem Restoration and EU Green Deal). However, managing and controlling invasive alien plant species (i.e., the subset of alien plant species that become established and cause negative impacts; hereafter “IAP”) can be expensive, laborious, and often technically difficult to achieve success (Marais et al., 2004; Haubrock et al., 2021). In fact, previous actions against IAP have not been sufficient to counteract their increasing globalization. To support these actions, substantial research has been done to understand the main dispersal pathways (Pyšek and Richardson, 2010), and spatial distribution patterns of IAP (Vilà et al., 2010). However, research on the distribution patterns of IAP or alien species in general has, thus far, focused mostly on large scales, like global or national studies (Chytrý et al., 2009; Seebens et al., 2017; Essl et al., 2019; Capinha et al., 2020), but detailed knowledge on the patterns and drivers of invasion at local scales—which are key for supporting management and restoration efforts—remains missing for many regions and taxonomic groups.
Here we use a comprehensive local scale data set to examine and describe the geography of invasions by alien plants in riparian ecosystems of continental Portugal. Continental Portugal harbors at least 3,314 vascular plant species (de Sequeira et al., 2012), of which at least 772 are alien (de Almeida, 2018) and 113 are listed as invasive species in the Portuguese law (Law No. 92/2019, 2019). Previous works have addressed plant invasions in Portuguese riverine systems (Bernez et al., 2006; Aguiar and Ferreira, 2013), however, how IAP are distributed in riparian ecosystems across the country remains largely unknown, making it difficult to identify areas under higher pressure from invasions and for which management or restoration efforts would be most needed. This occurs despite riparian ecosystems often being biodiversity hotspots (Duarte et al., 2004; Stella et al., 2013) providing a range of ecosystem functions and services related to water quality, microclimate, structural habitat, a bottom-up energy provider for the food web, and riverbank stability (Naiman et al., 2005), and being one of the most susceptible habitats to invasion (Richardson et al., 2007), often hosting a high diversity of IAP (Vilà et al., 2007; Chytrý et al., 2008).
In light of previous findings for other regions and taxa, a few hypotheses can be made about the drivers of invasion patterns in Portuguese riparian ecosystems. Propagule pressure resulting from proximity to urban areas and human activities may lead to the introduction of cosmopolitan, ornamental, nitrophilous, or cultivated species, originating hotspots of alien plant species (Hruska et al., 2008; Hulme, 2009; Pyšek et al., 2010, 2020). Land-use change is also an important driver of biodiversity change (Sala, 2000), disrupting ecological equilibrium and creating opportunities for alien species to establish (Richardson et al., 2007). Similarly, natural regular disturbances and the diversity of microhabitats, as well as movement of organisms, nutrients, and sediments create establishment opportunities and make riparian areas vulnerable to biological invasions (Pyšek and Prach, 1994; Catford et al., 2014). Seasonal patterns of summer droughts and winter floods, typical of Mediterranean rivers, lead to nutrient pulses and extreme natural disturbances, conditions that are also considered to favor invasions (Gasith and Resh, 1999; Davis et al., 2000; Stella et al., 2013). Finally, climatic variation across wide spatial extents is also a likely relevant factor in determining the distribution of alien plant species (Thuiller et al., 2008).
In this context, the goals of this study are to (i) map the richness of invasive alien plant species in riparian ecosystems of continental Portugal, (ii) assess the human and natural factors related to spatial variation in the values of this variable, and (iii) predict richness values along water courses and at the municipal level for the whole study area.
Materials and Methods
Study Area
Continental Portugal is in southwestern Europe, on the Iberian Peninsula. The climate is characterized by mild winters and dry summers and has a strong latitudinal and altitudinal gradient (Stella et al., 2013). Mean annual temperatures range between 7°C in the mountains and 18°C along the southern coastline. In the northwest region, mean annual precipitation is the highest (>3,000 mm/year), decreasing toward the south and amounting to around 500 mm/year (Miranda et al., 2002). Two bioclimatic regions are distinguished, the Temperate in the north-west and the Mediterranean in the remainder of the territory (Rodríguez-González et al., 2008; Rivas-Martínez et al., 2011). While coastal regions are densely populated and heavily affected by agriculture and industry, the southern and eastern regions are characterized by scattered settlements and extensive agricultural fields (Aguiar et al., 2009).
Data Collection
We used data on vegetation collected between 2003 and 2006 in 404 sites located in 29 river basins in continental Portugal (Instituto da Água, 2012) (Figure 1). This work was conducted during the pre-assessment surveys for the implementation of the Water Framework Directive (WFD) (WFD EU/2000/60; European Council, 2000). Field work was done according to the protocol established for the WFD implementation. In each site, all macrophyte species (excluding macroalgae) occurring in the water channel and banks along a 100 m length of the river were identified and their percentage cover was visually estimated (Aguiar et al., 2009). The distribution of sampling sites evenly covers all types of rivers in Portugal, providing a very broad coverage for statistical modeling. To ensure a representative analysis we only considered river basins with at least five samples available, which resulted in the use of 15 basins and 382 samples. For each site we measured the total number of IAP. Species were classified as invasive according to the latest listing of invasive species defined in the Portuguese Law (Law No. 92/2019, 2019) and specifically concerning continental Portugal - as IAP are also listed for the regions of Azores and Madeira. According to it, an invasive species is an alien species whose introduction into the wild or its spread within a given territory threatens or has an adverse impact on biological diversity, on ecosystem services associated with it, or has other adverse impacts. This list of invasive species includes mainly terrestrial species (Supplementary Table 1), therefore we refer to riparian habitats throughout our work.
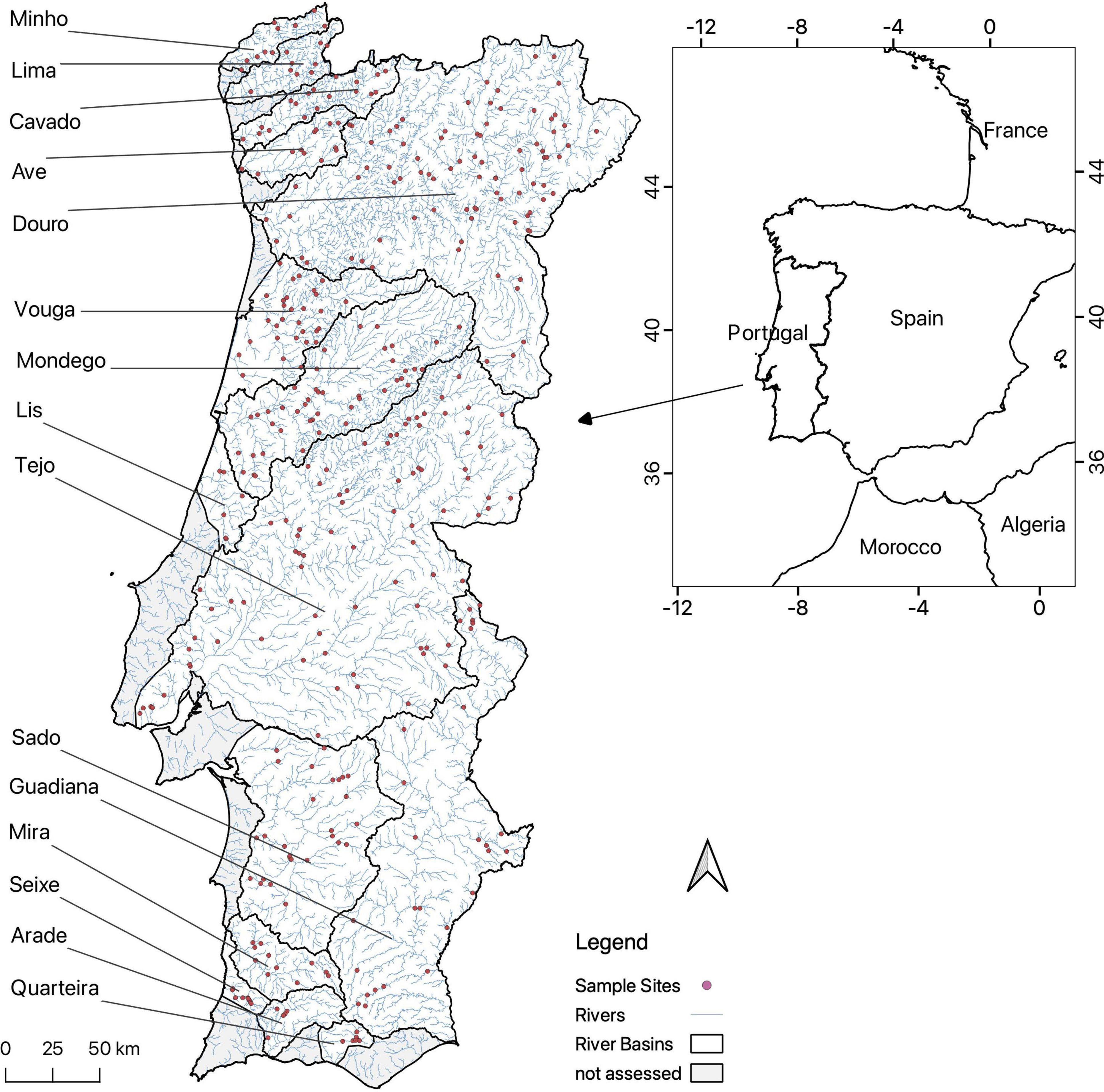
Figure 1. Map of continental Portugal with the location of the 382 analyzed vegetation plots (red dots) and the 15 river basins that were considered; the black lines delimit the river basins. This figure was created using QGIS Version 3.14 with the coordinate reference system ETRS 89/Portugal TM06 (QGIS Development Team, 2020).
Predictor Variables
Based on relevant literature (Richardson et al., 2007; Stella et al., 2013; Pyšek et al., 2020) and on our own expertise, we selected a set of variables that reflect expected relationships between natural and human factors and patterns of invasion by alien plants in riparian ecosystems. These variables reflect topographic and hydrological features as well as land use and socioeconomic settings. The identification, description, source, and spatial resolution of each variable are given in Table 1. To extract the value of each variable for each sampled site, we used a circular buffer with a 500 m radius within the centroid of the site. By extracting values within this radius of the sampled site, we aim to capture the effect of local conditions and of the surrounding landscape, which jointly shape the invasion patterns at local scales (Novák and Konvic̆ka, 2006). The only exception to this procedure was the variable slope, for which we used a 10 m radius, as we expect its effect to be mainly local, and primarily concerning water flow speed at the site. For climate variables, we used data from the 5 years prior to the recording period, as this period seems to have the strongest effect on the ecological conditions (Dias et al., 2015). The processing of all variables was performed in QGIS Version 3.14 (QGIS Development Team, 2020).
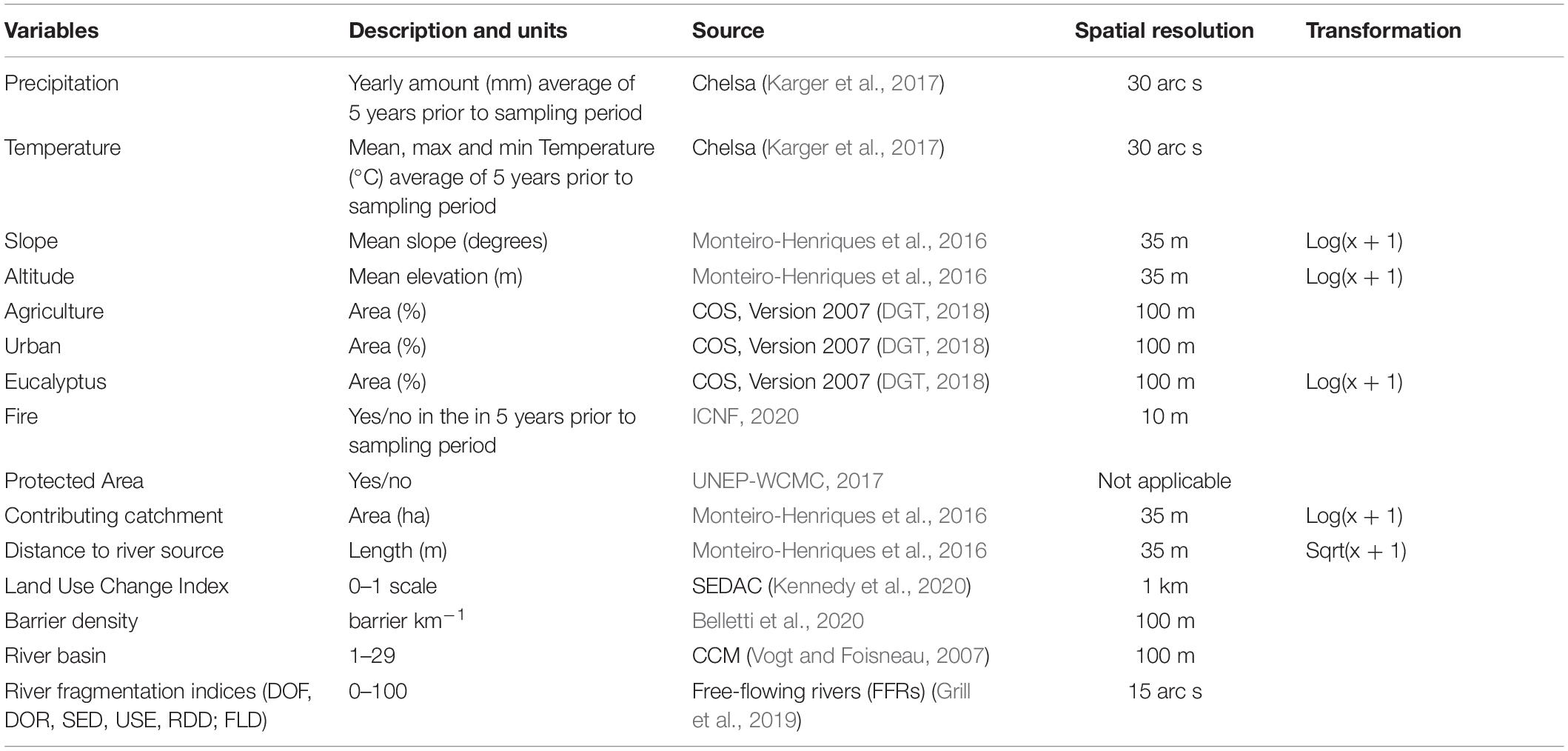
Table 1. Covariates used in the generalized additive model aiming to explain and predict spatial variation in values of richness of invasive alien plants in riparian ecosystems.
Statistical Analysis
To model the number of invasive alien plant species as a function of covariates, we used a generalized additive model (GAM), which accounts for linear and non-linear relationships between the response variable and the covariates. Since the response variable is discrete (i.e., a count), we considered three distributions, the Poisson distribution, the Tweedie distribution, and the negative binomial distribution. We fitted models with these three distributions and selected the one with the lowest Akaike information criterion (AIC) (Burnham et al., 2011). We used thin plate regression splines (Wood, 2003) as the basis for the model’s smooth terms. The model was initiated by considering that the fit is highly “wiggly”, so a “wiggliness” penalty is added during the model fitting process, determined by the data (Wood, 2017). To reduce redundancy among covariates we selected a set of those with pairwise Pearson correlation coefficients below |0.7| and variance inflation factor below 3 (Zuur et al., 2009). During model fitting, we selected covariates by adding an additional penalty that allowed each smooth term to be removed (Marra and Wood, 2011). We used a significance level (α) of 0.05. To account for spatial autocorrelation, we added a two-dimension smoothing function with the x and y coordinates of the centroid of sampled sites. River basins were included in the model as a random effect to account for basin-level dependencies among samples (Wood, 2013). We used the R package “mgcv” version 1.8-34 (Wood, 2011) for model fitting. To assess the robustness of the fitted model we analyzed deviance residuals and checked for normal distribution and constant variance using the gam.check function from the “mgcv” package. To assess the amount of deviance explained by the model we also obtained the adjusted-R2 (Wood, 2017).
We also evaluated the predictive accuracy of the model. For this purpose, we used two cross-validation approaches. First, we used a leave one out cross validation (LOOCV), which consists in removing one site at a time, fitting the model with the remaining sites, and then making a prediction for the site that was left out. We repeated this process for each of the 382 sites and recorded the error estimates. Second, we used a repeated k-fold cross validation that consisted in randomly choosing 80% of the survey sites as a training set, fitting the model with those data, and making predictions for the remaining 20%. This procedure was repeated 100 times to maximize the representativity of sampled conditions in both calibration and validation partitions. To measure the agreement of predictions and left out observations, we used the Mean Absolute Error (MAE) from the package “metrics” (Hamner and Frasco, 2018) and Relative Absolute Error (RAE) from the package “caret” (Kuhn, 2021). A valuable property of the latter metric is that it provides a threshold beyond which predictions are considered uninformative. This threshold corresponds to a value of 100, whereby evaluation results higher than this threshold have a predictive accuracy worse than the one obtained by simply using the average of richness values in the evaluation data (Witten et al., 2017; Capinha et al., 2018).
Finally, we used the final model to make predictions for unsampled sites. This model used three predictor variables (see below), so we calculated the values of each in the same way we did for model fitting, but for 1 km grid cells along the river network supplied by Vogt and Foisneau (2007). This grid cell resolution roughly matches the spatial area represented in the variables used for model calibration (i.e., a 500 m radius). We then applied the model to predict IAP richness in each cell. In addition, we also averaged the predicted values within each municipality, because this is an administrative division commonly considered in the development and implementation of environment-related initiatives, including biological invasion management and ecological restoration actions. All calculations were performed in R version 4.0.2 (R Core Team, 2021).
Results
A total of 960 plant species were found in riparian ecosystems of continental Portugal. In 382 sites 97 alien species were found and of these 34 are also considered as invasive plant species. The highest number of alien species found in a single plot was 15, and the highest number of invasive alien species was 10. Of the 382 sites, IAP were recorded in 297 sites (77.75%). The IAP species recorded more frequently were Bidens frondosa (207 sites), Conyza bonariensis (131 sites), Phytolacca americana (96 sites), and Arundo donax (83 sites) (Supplementary Table 1).
The model results suggest that richness of invasive plant species is negatively associated with altitude, and positively with the size of upstream catchment and percentage of urban area (Figure 2 and Table 2). The model explained a substantial amount of variability in richness values, as indicated by an adjusted-R2 of 0.63.
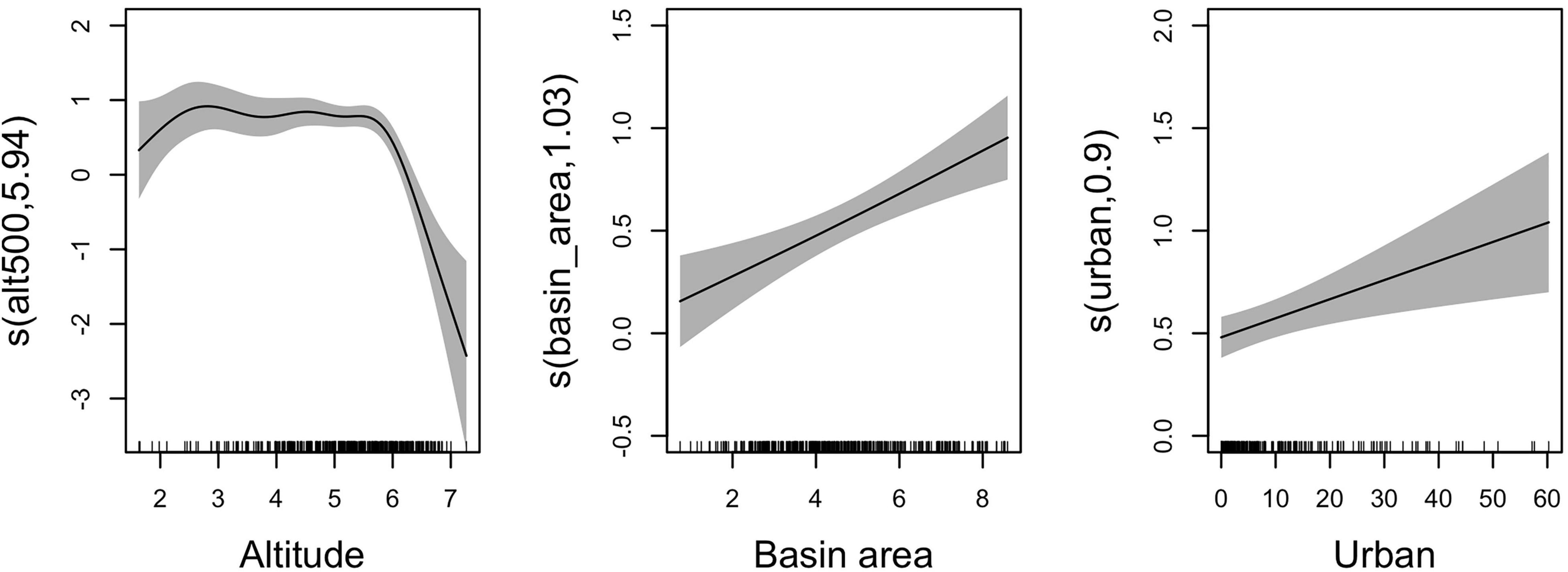
Figure 2. Smooth functions (black lines) for the predictor variables “altitude”, “basin area”, and “urban area”. The gray shading represents the 95% confidence intervals. The number in brackets on the vertical axis labels is the effective degrees of freedom of the smooth term (1 corresponds to a linear term).
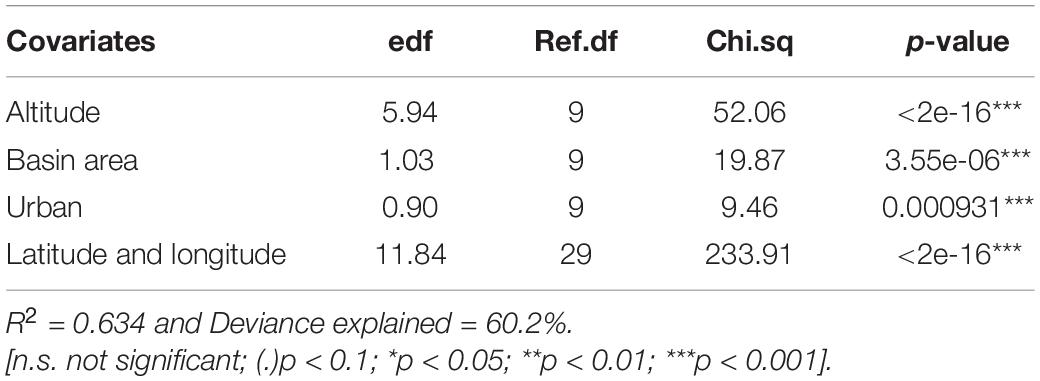
Table 2. Results of the generalized additive model explaining values of richness of invasive alien plants in riparian ecosystems.
The k-fold validation procedure recorded a mean absolute error (MAE) of 1.03 ± 0.1 species and relative absolute error (RAE) of 0.63 ± 0.06 (Table 3). The error values of the LOOCV show similar values (Table 3). The relatively small deviations between predicted and observed richness values support the robustness of the model and its use for predictive purposes.
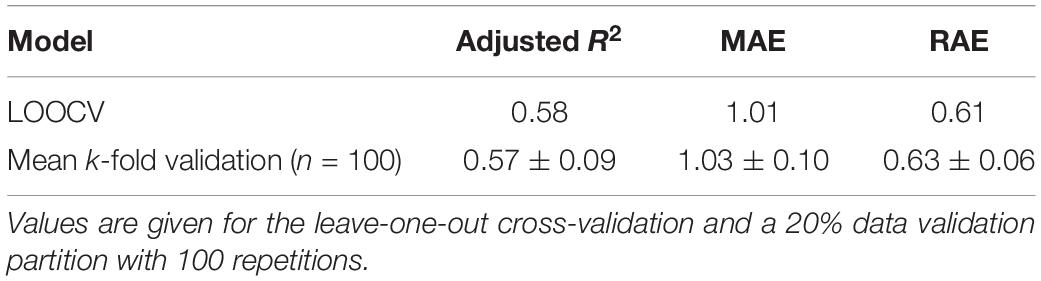
Table 3. Average and standard deviation of error measures of generalized additive model predicting values of richness of invasive alien plants in riparian ecosystems.
The prediction maps show that there is a higher diversity of IAP in the central and northwestern, lowland, regions of continental Portugal (Figures 3, 4). Low richness values were predicted for river sections in inland regions, regions south of Lisbon, and for mountainous areas. Our model predicts that for 43.1% of river sections of continental Portugal there are one to two invasive plant species, for 11.5% there are three to five, and for 2.2% there are five or more invasive plant species (Figure 3). At the municipal level, the highest average richness of invasive alien plants occurs around the country’s two main cities, Porto and Lisbon (Figure 4). High richness is generally found throughout the central to north-western continental Portugal. Although the highest numbers are concentrated around the larger cities, nearby areas belonging to the same river basins are also considerably affected. River basins with the highest average IAP richness are those of the rivers Lis (3.42 ± 0.97), Vouga (3.13 ± 0.97), Ave (2.88 ± 1.40), and Mondego (2.72 ± 1.64). The basins that harbor more IAP overall are Douro (20), Mondego (20), and Vouga (18) (Supplementary Table 1).
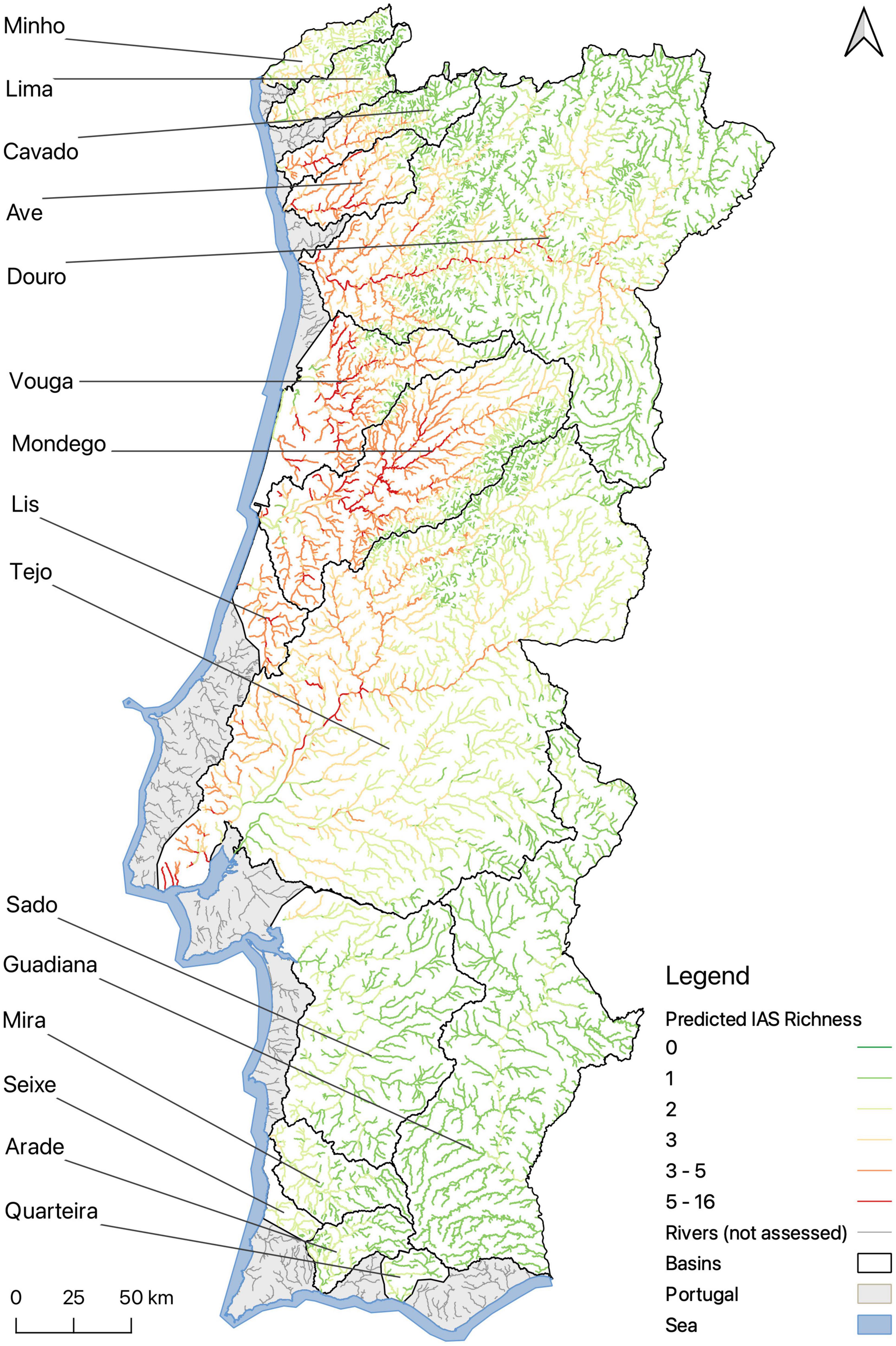
Figure 3. Predictions of invasive alien plant species richness for the Portuguese river network. Richness values are calculated for each 1 km grid cell corresponding to a river section. Gray areas correspond to river basins that did not have sufficient data to be included in the analysis. This figure was created with QGIS Version 3.14 (QGIS Development Team, 2020).
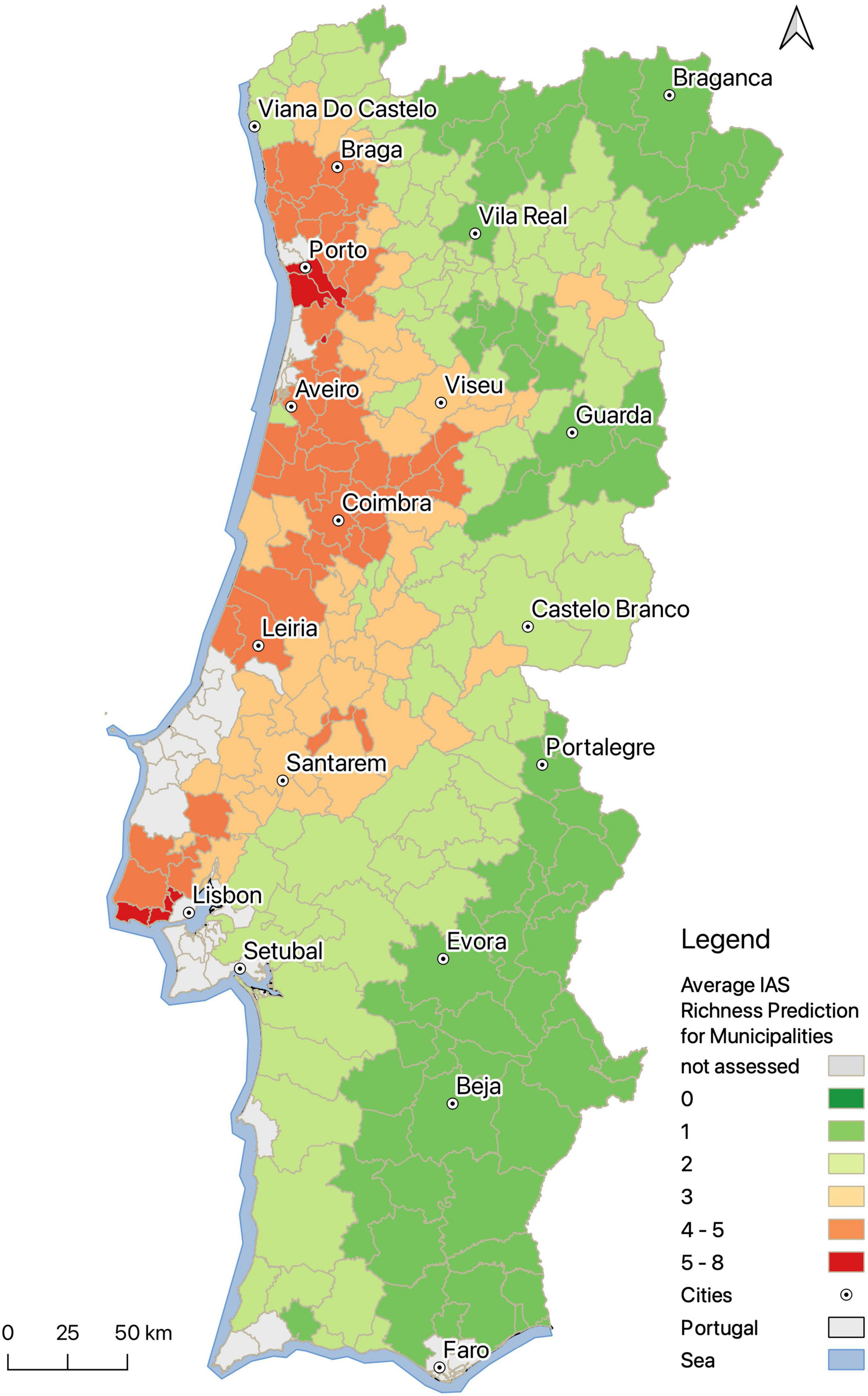
Figure 4. Predictions of invasive alien plant species richness for municipalities in Portugal. Predictions correspond to mean values of richness obtained for the 1 km grid cells representing river sections located within the municipality. Gray areas correspond to river basins that were not analyzed due to insufficient data. This figure was created with QGIS Version 3.14 (QGIS Development Team, 2020).
Discussion
Our results identified a marked distribution pattern in the richness of invasive alien species in riparian environments of continental Portugal. This pattern could be explained, to a large extent, by a few variables, namely altitude, size of the catchment area and the proportion of urban area in a buffer of 500 m around the target site. In addition, a model using these variables was able to predict the distribution of richness values in unsampled sites with good accuracy and at a high spatial resolution, enabling us to identify the river sections most strongly affected by invasions and for which management or restoration actions are more pressing.
Model results show that the richness of invasive alien plants was higher at sites up to 300 meters above sea level, and far from river sources. At higher altitudes, richness values of invasive alien plants decrease quickly. The relationship between increasing altitude and decreasing IAP richness has been often observed (Pyšek et al., 2002; Chytrý et al., 2005, 2009; Anđelković et al., 2022), and has been attributed to the generally milder climatic conditions found in low-altitude areas (Chytrý et al., 2005), benefiting IAP that mostly originate from warm areas (Pyšek et al., 2003). On the other hand, the IAP found at higher altitudes are mostly generalists with broad climate tolerances and able to thrive across a wide range of altitudes (Alexander et al., 2011; Essl et al., 2019). In our data set, 8 species were found at elevations above 500 m. The two most common were Bidens frondosa and Phytolacca americana; both native to North America and invasive in many temperate regions of the world (Huang and Ding, 2015; Ronzhina, 2017).
The positive relationship found between the size of the contributing catchment area and IAP richness likely reflects a scaling effect played by the dendritic structure of riparian networks, suggesting a key role of hydrochory in the accumulation of IAP (Rodríguez-González et al., 2019). A larger catchment area may result in a higher number of IAP simply because more species accumulate in downstream areas. Additionally, it draws attention to the interconnection of river segments along the same river basin, which therefore should not be considered or treated separately (Fausch et al., 2002; Rodríguez-González et al., 2019). In our study area, the higher Strahler stream segments of the larger river basins Tejo, Mondego, and Douro hold higher IAP numbers, and across all river basins, an increase toward the coast can be observed (Figure 3).
Socioeconomic influences are important drivers of invasive species accumulation, often more important than the physical environment (Dawson et al., 2017; Essl et al., 2019). Here, we also identified a significant positive relationship between richness of invasive alien plant species and the percentage of urban area surrounding each sampled site. Urban areas can favor invasions in multiple ways, very often through high colonization and propagule pressures that result from ornamental horticultural activities promoting the plantation of alien species in urban parks and gardens (van Kleunen et al., 2018), from accidental introductions resulting from trading or tourism activities (Hulme, 2009), and in general due to global plant trade (Beaury et al., 2021). At the same time, intense human activity also provides conditions that can favor the establishment of alien species, including disturbances or the creation of novel habitats that provide niche opportunities by reducing natural enemies, such as native plant competitors (McKinney, 2006). Although it is not possible to distinguish the relative contribution of these and other urban-related mechanisms in shaping the observed patterns of IAP richness, it seems likely that the number of IAP in the vicinity of these areas will continue to grow driven by high levels of socio-economic activity.
Previous research has found important limitations in the capacity of statistical models to predict regional-scale richness of alien species (Capinha et al., 2018). We found a good accuracy in predicting IAP richness, with error levels substantially below the threshold indicating uninformative predictions. The reasons for the high accuracy achieved here could be related to the lower diversity of human and natural conditions represented in our model compared to those tested in Capinha et al. (2018), and which comprised mostly continental to global extents. Furthermore, our data sample most of continental Portugal, ensuring that predictions are within the range of conditions used for model calibration, thus improving their reliability (Capinha et al., 2018). This provides good support for the use of our predictions to support decision-making in ongoing and future efforts to reduce IAP pressures on riparian ecosystems. In this regard, it is worth mentioning that the model is based on richness data collected between 2003 and 2006—the most recent available—so although the spatial patterns of variation are unlikely to have changed significantly, the current figures of IAP richness may be even slightly higher than predicted, reflecting the continued accumulation of alien plants worldwide as time progresses (Seebens et al., 2017, 2021).
According to the OECD (2019), in order to reach the goal of the EU Biodiversity Strategy for 2030 to decrease the number of Red List species they threaten by 50% (European Commission, 2020), invasive species must be controlled or eradicated by 2030 from 80% of the most important areas of plant diversity in Europe. Future work could thus aim at crossing the richness patterns we uncovered with observed or potential distribution maps of endangered species listed in the recently published Red List of Vascular Plants of Mainland Portugal (Carapeto et al., 2020) to help pinpoint locations where threatened riparian plant species could be under greater pressure from IAP. Similarly, our estimates could also be combined with estimates of richness of native plant species to map the areas where the proportion of alien invasive species on overall species diversity is higher (Catford et al., 2012). Results from these analyses could then be used to inform national governmental entities in the definition of priority areas for conservation or restoration efforts and by local entities, such as municipalities or environmental NGOs in directing future efforts of eradication or introduction prevention of riparian invasive species. Future work could also consider differing invasion pressures caused by individual species. For example, some areas may still be strongly affected by single invasive species like Acacia dealbata, which might propagate very fast and cover wide areas (Lorenzo et al., 2010; Souza-Alonso et al., 2017). Our estimates of species richness weighted by the magnitude of species-level impacts could provide a deeper understanding of the impact of invasion pressures on riparian ecosystems.
We provided a basis for understanding the distribution patterns of invasive alien plant species in riparian areas of continental Portugal. We found a consistent geographical clustering of highly invaded areas in central and northern coastal areas, which appears to be driven, to a large extent, by the joint effect of a high human population concentration, low altitude values, and the convergence of species spread in downstream areas. Our results also showed many contiguous municipalities sharing similar levels of invasion, thus recommending cross-municipal cooperation and coordination when addressing the prevention and management of invasive plants species. Given also the role identified for species spread through the hydrographic network, these efforts would also benefit from the involvement of entities with responsibilities over upstream river stretches (Fausch et al., 2002; Rodríguez-González et al., 2019).
Data Availability Statement
Publicly available datasets were analyzed in this study. These data can be found here: https://www.apambiente.pt/dqa/index.html.
Author Contributions
CC, FSD, LB-de-Á, PMR-G, and RP designed the original research. RP did the analytic work with contributions from FSD. All authors contributed to writing up the article.
Funding
RP, FSD, LB-de-Á, and PMR-G were financed by the FEDER Funds through the Operational Competitiveness Factors Program—COMPETE and the National Funds through Fundação para a Ciência e a Tecnologia (FCT). RP and FSD were supported through project PTDC/BIA-ECO/28729/2017—POCI-01-0145-FEDER-028729 (RIVERSCALE project), LB-de-Á under the Norma Transitória—L57/2016/CP1440/CT0022, and CC and PMR-G through FCT-CEEC Individual Programme CEECIND/02037/2017 and 2020/03356/CEECIND, respectively. Forest Research Centre was a research unit funded by FCT (UIDB/00239/2020).
Conflict of Interest
The authors declare that the research was conducted in the absence of any commercial or financial relationships that could be construed as a potential conflict of interest.
Publisher’s Note
All claims expressed in this article are solely those of the authors and do not necessarily represent those of their affiliated organizations, or those of the publisher, the editors and the reviewers. Any product that may be evaluated in this article, or claim that may be made by its manufacturer, is not guaranteed or endorsed by the publisher.
Acknowledgments
We thank contributions from three reviewers who helped improve the final version of the work.
Supplementary Material
The Supplementary Material for this article can be found online at: https://www.frontiersin.org/articles/10.3389/fevo.2022.875578/full#supplementary-material
References
Aguiar, F. C., Ferreira, M. T., Albuquerque, A., Rodríguez-González, P. M., and Segurado, P. (2009). Structural and functional responses of riparian vegetation to human disturbance: performance and spatial scale-dependence. Fundam. Appl. Limnol. 175, 249–267. doi: 10.1127/1863-9135/2009/0175-0249
Aguiar, F., and Ferreira, M. (2013). Plant invasions in the rivers of the Iberian Peninsula, south-western Europe: a review. Plant Biosyst. 147, 1107–1119. doi: 10.1080/11263504.2013.861539
Alexander, J. M., Kueffer, C., Daehler, C. C., Edwards, P. J., Pauchard, A., Seipel, T., et al. (2011). Assembly of nonnative floras along elevational gradients explained by directional ecological filtering. Proc. Natl. Acad. Sci. U.S.A 108, 656–661. doi: 10.1073/pnas.1013136108
Anđelković, A. A., Pavlović, D. M., Marisavljević, D. P., Živković, M. M., Novković, M. Z., Popović, S. S., et al. (2022). Plant invasions in riparian areas of the middle danube basin in Serbia. NeoBiota 71, 23–48.
Beaury, E. M., Patrick, M., and Bradley, B. A. (2021). Invaders for sale: the ongoing spread of invasive species by the plant trade industry. Front. Ecol. Environ. 19:550–556. doi: 10.1002/fee.2392
Bellard, C., Cassey, P., and Blackburn, T. M. (2016). Alien species as a driver of recent extinctions. Biol. Lett. 12:20150623. doi: 10.1098/rsbl.2015.0623
Belletti, B., de Leaniz, C., Jones, J., Bizzi, S., Börger, L., Segura, G., et al. (2020). More than one million barriers fragment Europe’s rivers. Nature 588, 436–441. doi: 10.1038/s41586-020-3005-2
Bernez, I., Aguiar, F., Violle, C., and Ferreira, T. (2006). Invasive river plants from Portuguese floodplains: what can species attributes tell us? Hydrobiologia 560, 3–9. doi: 10.1007/s10750-006-0155-7
Burnham, K. P., Anderson, D. R., and Huyvaert, K. P. (2011). AIC model selection and multimodel inference in behavioral ecology: some background, observations, and comparisons. Behav. Ecol. Sociobiol. 65, 23–35. doi: 10.1007/s00265-010-1029-6
Capinha, C., Essl, F., Seebens, H., Pereira, H. M., and Kühn, I. (2018). Models of alien species richness show moderate predictive accuracy and poor transferability. NeoBiota 38, 77–96. doi: 10.3897/neobiota.38.23518
Capinha, C., Marcolin, F., and Reino, L. (2020). Human-induced globalization of insular herpetofaunas. Glob. Ecol. Biogeogr. 29, 1328–1349. doi: 10.1111/geb.13109
Carapeto, A., Francisco, A., Pereira, P., and Porto, M. (2020). Lista Vermelha Da Flora Vascular De Portugal Continental. Lisboa: Imprensa Nacional.
Catford, J. A., Morris, W. K., Vesk, P. A., Gippel, C. J., and Downes, B. J. (2014). Species and environmental characteristics point to flow regulation and drought as drivers of riparian plant invasion. Divers. Distrib. 20, 1084–1096. doi: 10.1111/ddi.12225
Catford, J. A., Vesk, P. A., Richardson, D. M., and Pyšek, P. (2012). Quantifying levels of biological invasion: towards the objective classification of invaded and invasible ecosystems. Glob. Change Biol. 18, 44–62. doi: 10.1111/j.1365-2486.2011.02549.x
CBD. (2014). Global biodiversity Outlook 4. Secretariat of the Convention on Biological Diversity. Montréal: CBD, 155.
Chytrý, M., Maskell, L. C., Pino, J., Pyšek, P., Vilà, M., Font, X., et al. (2008). Habitat invasions by alien plants: a quantitative comparison among Mediterranean, subcontinental and oceanic regions of Europe. J. Appl. Ecol. 45, 448–458. doi: 10.1111/j.1365-2664.2007.01398.x
Chytrý, M., Pyšek, P., Tichý, L., Knollová, I., and Danihelka, J. (2005). Invasions by alien plants in the Czech Republic: a quantitative assessment across habitats. Preslia 77, 339–354.
Chytrý, M., Pyšek, P., Wild, J., Pino, J., Maskell, L. C., and Vilà, M. (2009). European map of alien plant invasions based on the quantitative assessment across habitats. Divers. Distrib. 15, 98–107. doi: 10.1111/j.1472-4642.2008.00515.x
Clavero, M., and Garcia-Berthou, E. (2005). Invasive species are a leading cause of animal extinctions. Trends Ecol. Evol. 20:110. doi: 10.1016/j.tree.2005.01.003
Davis, M. A., Grime, J. P., and Thompson, K. (2000). Fluctuating resources in plant communities: a general theory of invasibility. J. Ecol. 88, 528–534. doi: 10.1046/j.1365-2745.2000.00473.x
Dawson, W., Moser, D., Van Kleunen, M., Kreft, H., Pergl, J., Pyšek, P., et al. (2017). Global hotspots and correlates of alien species richness across taxonomic groups. Nat. Ecol. Evol. 1, 1–7. doi: 10.1038/s41559-017-0186
de Almeida, J. (2018). New additions to the exotic vascular flora of continental Portugal. Flora Mediterr. 28, 259–278. doi: 10.7320/FlMedit28.259
de Sequeira, M., Espírito-Santo, D., Aguiar, C. C. J., and Honrado, J. (2012). Checklist da Flora de Portugal (Continental, Açores e Madeira). Lisboa: Associação Lusitana de Fitossociologia. Available online at: http://ipt.gbif.pt/ipt/resource.do?r=alfa_checklist_florapt#anchor-citation [Accessed February 1, 2022]
DGT (2018). Especificações técnicas da Carta de Uso e Ocupação do Solo (COS) de Portugal Continental para 1995, 2007, 2010 e 2015. Relatório Técnico. Lisbon: Direção-Geral Territ, 103–103.
Diagne, C., Leroy, B., Vaissière, A.-C., Gozlan, R. E., Roiz, D., Jarić, I., et al. (2021). High and rising economic costs of biological invasions worldwide. Nature 592, 571–576. doi: 10.1038/s41586-021-03405-6
Dias, F. S., Bugalho, M. N., Rodríguez-González, P. M., Albuquerque, A., and Cerdeira, J. O. (2015). Effects of forest certification on the ecological condition of Mediterranean streams. J. Appl. Ecol. 52, 190–198. doi: 10.1111/1365-2664.12358
Duarte, M., Moreira, I., and Ferreira, M. (2004). Flora de ecossistemas aquáticos e ribeirinhos portugueses: delimitação taxonómica, tipológica e espacial. Recursos Hídricos 25, 67–94.
Essl, F., Dawson, W., Kreft, H., Pergl, J., Pyšek, P., Van Kleunen, M., et al. (2019). Drivers of the relative richness of naturalized and invasive plant species on Earth. AoBP 11, 1–13. doi: 10.1093/aobpla/plz051
European Commission. (2020). EU biodiversity strategy for 2030. Off. J. Eur. Communities 53, 1689–1699.
Fausch, K. D., Torgersen, C. E., Baxter, C. V., and Li, H. W. (2002). Landscapes to riverscapes: bridging the gap between research and conservation of stream fishes: a continuous view of the river is needed to understand how processes interacting among scales set the context for stream fishes and their habitat. BioScience 52, 483–498. doi: 10.1641/0006-3568(2002)052[0483:ltrbtg]2.0.co;2
Gasith, A., and Resh, V. H. (1999). Streams in mediterranean climate regions: abiotic influences and biotic responses to predictable seasonal events. Annu. Rev. Ecol. Syst. 30, 51–81. doi: 10.1146/annurev.ecolsys.30.1.51
Grill, G., Lehner, B., Thieme, M., Geenen, B., Tickner, D., Antonelli, F., et al. (2019). Mapping the world’s free-flowing rivers. Nature 569, 215–221. doi: 10.1038/s41586-019-1111-9
Hamner, B., and Frasco, M. (2018). Metrics: Evaluation Metrics for Machine Learning. R Package Version 014.
Haubrock, P. J., Turbelin, A. J., Cuthbert, R. N., Novoa, A., Taylor, N. G., Angulo, E., et al. (2021). Economic costs of invasive alien species across Europe. NeoBiota 67, 153–190. doi: 10.3897/neobiota.67.58196
Hruska, K., Dell’uomo, A., Staffolani, L., and Torrisi, M. (2008). Influence of urbanization on riparian and algal species composition in two rivers of Central Italy. Écoscience 15, 121–128. doi: 10.2980/1195-6860(2008)15[121:iouora]2.0.co;2
Huang, W., and Ding, J. (2015). Effects of generalist herbivory on resistance and resource allocation by the invasive plant, Phytolacca americana. Insect Sci. 23, 191–199. doi: 10.1111/1744-7917.12244
Hulme, P. E. (2009). Trade, transport and trouble: managing invasive species pathways in an era of globalization. J. Appl. Ecol. 46, 10–18. doi: 10.1111/j.1365-2664.2008.01600.x
Instituto da Água (2012). Nota Explicativa Dos Dados Recolhidos No Âmbito Dos Trabalhos De Implementação Da Directiva Quadro Da Água. Available online at: https://www.apambiente.pt/dqa/assets/nota-explicativa-dos-dados-recolhidos.pdf [Accessed November 02, 2021]
IPBES (2019). “Key messages - B. Direct and indirect drivers of change,” in Summary for Policymakers of the Global Assessment Report on Biodiversity and Ecosystem Services of the Intergovernmental Science-Policy Platform on Biodiversity and Ecosystem Services, eds J. S. S. Díaz, E. S. Brondízio, et al. (Bonn: IPBES Secretariat), 56.
Karger, D. N., Conrad, O., Böhner, J., Kawohl, T., Kreft, H., Soria-Auza, R. W., et al. (2017). Climatologies at high resolution for the earth’s land surface areas. Sci. Data 4:170122.
Kennedy, C., Oakleaf, J. R., Theobald, D. M., Baruch-Mordo, S., and Kiesecker, J. (2020). Global Human Modification of Terrestrial Systems. Palisades, NY: NASA Socioeconomic Data and Applications Center (SEDAC). Accessed 29042020. doi: 10.7927/edbc-3z60
Law No. 92/2019 (2019). Assembleia da república, 10 de julho de 2019. Diário Repúb. 130, 5688–5724. (in Portuguese),
Lorenzo, P., González, L., and Reigosa, M. J. (2010). The genus acacia as invader: the characteristic case of acacia dealbata link in Europe. Ann. For. Sci. 67, 101–101. doi: 10.1051/forest/2009082
Marais, C., Van Wilgen, B. W., and Stevens, D. (2004). The clearing of invasive alien plants in South Africa: a preliminary assessment of costs and progress. South Afr. J. Sci. 100, 97–103.
Marra, G., and Wood, S. N. (2011). Practical variable selection for generalized additive models. Comput. Stat. Data Anal. 55, 2372–2387. doi: 10.1016/j.csda.2011.02.004
McKinney, M. L. (2006). Urbanization as a major cause of biotic homogenization. Biol. Conserv. 127, 247–260. doi: 10.1016/j.biocon.2005.09.005
Miranda, P., Coelho, F., Tomé, A., and Valente, M. (2002). Climate Change in Portugal. Scenarios, Impacts and Adaptation Measures. SIAM Proj. Gradiva. Available online at: https://www.academia.edu/13392269/20th_Century_Portuguese_Climate_and_Climate_Scenarios_in_Climate_Change_in_Portugal_Scenarios_Impacts_and_Adaptation [Accessed February 3, 2022]
Monteiro-Henriques, T., Martins, M. J., Cerdeira, J. O., Silva, P., Arsénio, P., Silva, Á, et al. (2016). Bioclimatological mapping tackling uncertainty propagation: application to mainland Portugal. Int. J. Climatol. 36, 400–411. doi: 10.1002/joc.4357
Naiman, R. J., Décamps, H., McClain, M. E., and Likens, G. E. (2005). Riparia: Ecology, Conservation And Management Of Streamside Communities. New York, NY: Academic Press. doi: 10.1016/B978-0-12-663315-3.X5000-X
Novák, J., and Konvic̆ka, M. (2006). Proximity of valuable habitats affects succession patterns in abandoned quarries. Ecol. Eng. 26, 113–122. doi: 10.1016/j.ecoleng.2005.06.008
OECD (2019). The Post-2020 Biodiversity Framework: Targets, Indicators And Measurability Implications At Global And National Level. Novemb. Version. Paris: OCED.
Pyšek, P., and Prach, K. (1994). “How important are rivers for supporting plant invasions?,” in Ecology and management of invasive riverside plants, eds L. C. Waal, L. E. Child, P. M. Wade, and J. H. Brock Wiley (Chichester: University of Chichester), 19–26.
Pyšek, P., and Richardson, D. M. (2010). Invasive species, environmental change and management, and health. Annu. Rev. Environ. Resour. 35, 25–55. doi: 10.1146/annurev-environ-033009-095548
Pyšek, P., Hulme, P. E., Simberloff, D., Bacher, S., Blackburn, T. M., Carlton, J. T., et al. (2020). Scientists’ warning on invasive alien species. Biol. Rev. 95, 1511–1534. doi: 10.1111/brv.12627
Pyšek, P., Jarošík, V., and Kuc̆era, T. (2002). Patterns of invasion in temperate nature reserves. Biol. Conserv. 104, 13–24. doi: 10.1016/S0006-3207(01)00150-1
Pyšek, P., Jarošík, V., Hulme, P. E., Kühn, I., Wild, J., Arianoutsou, M., et al. (2010). Disentangling the role of environmental and human pressures on biological invasions across Europe. Proc. Natl. Acad. Sci. U.S.A 107, 12157–12162. doi: 10.1073/pnas.1002314107
Pyšek, P., Sádlo, J., Mandák, B., and Jarosík, V. (2003). Czech alien flora and the historical pattern of its formation: what came first to Central Europe? Oecologia 135, 122–130. doi: 10.1007/s00442-002-1170-7
R Core Team (2021). R: A Language and Environment for Statistical Computing. R Foundation for Statistical Computing. Available online at: https://www.r-project.org/ (accessed January, 2021).
Richardson, D. M., Holmes, P. M., Esler, K. J., Galatowitsch, S. M., Stromberg, J. C., Kirkman, S. P., et al. (2007). Riparian vegetation: degradation, alien plant invasions, and restoration prospects. Divers. Distrib. 13, 126–139. doi: 10.1111/j.1366-9516.2006.00314.x
Rivas-Martínez, S., Rivas-Sáenz, S., and Penas, Á (2011). “Worldwide bioclimatic classification system,” in Global Gebotany, 1st Edn, 1–638.
Rodríguez-González, P. M., Ferreira, M. T., Albuquerque, A., Santo, D. E., and Rego, P. R. (2008). Spatial variation of wetland woods in the latitudinal transition to arid regions: a multiscale approach. J. Biogeogr. 35, 1498–1511. doi: 10.1111/j.1365-2699.2008.01900.x
Rodríguez-González, P. M., García, C., Albuquerque, A., Monteiro-Henriques, T., Faria, C., Guimarães, J. B., et al. (2019). A spatial stream-network approach assists in managing the remnant genetic diversity of riparian forests. Sci. Rep. 9:6741. doi: 10.1038/s41598-019-43132-7
Ronzhina, D. (2017). Distribution, competitive ability, and seed production of Bidens frondosa L. in the Middle Urals. Russ. J. Biol. Invasions 8, 351–359. doi: 10.1134/S2075111717040099
Sala, O. E. (2000). Global biodiversity scenarios for the year 2100. Science 287, 1770–1774. doi: 10.1126/science.287.5459.1770
Seebens, H., Bacher, S., Blackburn, T. M., Capinha, C., Dawson, W., Dullinger, S., et al. (2021). Projecting the continental accumulation of alien species through to 2050. Glob. Change Biol. 27, 970–982. doi: 10.1111/gcb.15333
Seebens, H., Blackburn, T. M., Dyer, E. E., Genovesi, P., Hulme, P. E., Jeschke, J. M., et al. (2017). No saturation in the accumulation of alien species worldwide. Nat. Commun. 8:14435. doi: 10.1038/ncomms14435
Souza-Alonso, P., Rodríguez, J., González, L., and Lorenzo, P. (2017). Here to stay. recent advances and perspectives about acacia invasion in Mediterranean areas. Ann. For. Sci. 74:55. doi: 10.1007/s13595-017-0651-0
Stella, J. C., Rodríguez-González, P. M., Dufour, S., and Bendix, J. (2013). Riparian vegetation research in Mediterranean-climate regions: common patterns, ecological processes, and considerations for management. Hydrobiologia 719, 291–315. doi: 10.1007/s10750-012-1304-9
Thuiller, W., Albert, C., Araújo, M. B., Berry, P. M., Cabeza, M., Guisan, A., et al. (2008). Predicting global change impacts on plant species’ distributions: future challenges. Perspect. Plant Ecol. Evol. Syst. 9, 137–152. doi: 10.1016/j.ppees.2007.09.004
UNEP-WCMC. (2017). World Database on Protected Areas User Manual 1.5. UNEP-WCMC Camb. UK. Available online at: http://wcmc.io/WDPA_Manual (accessed October 28, 2020).
van Kleunen, M., Essl, F., Pergl, J., Brundu, G., Carboni, M., Dullinger, S., et al. (2018). The changing role of ornamental horticulture in alien plant invasions. Biol. Rev. 93, 1421–1437. doi: 10.1111/brv.12402
Vilà, M., Basnou, C., Pyšek, P., Josefsson, M., Genovesi, P., Gollasch, S., et al. (2010). How well do we understand the impacts of alien species on ecosystem services? A pan-European, cross-taxa assessment. Front. Ecol. Environ. 8:135–144. doi: 10.1890/080083
Vilà, M., Pino, J., and Font, X. (2007). Regional assessment of plant invasions across different habitat types. J. Veg. Sci. 18, 35–42. doi: 10.1111/j.1654-1103.2007.tb02513.x
Vogt, J., and Foisneau, S. (2007). CCM River and Catchment Database - Version 2.0 Analysis Tools. JRC36122.
WFD EU/2000/60; European Council. (2000). Directive 2000/60/EC of the european parliament and of the council of 23 October 2000 establishing a framework for community action in the field of water policy. Off. J. Eur. Commun. 327, 1–73. doi: 10.1016/j.bios.2004.07.033
Witten, I. H., Frank, E., Hall, M. A., and Pal, C. J. (2017). Data Mining: Practical Machine Learning Tools and Techniques, 4th Edn. Amsterdam: Elsevier. 1–621.
Wood, S. N. (2003). Thin plate regression splines. J. R. Stat. Soc. Ser. B Stat. Methodol. 65, 95–114. doi: 10.1111/1467-9868.00374
Wood, S. N. (2011). Fast stable restricted maximum likelihood and marginal likelihood estimation of semiparametric generalized linear models. J. R. Stat. Soc. B 73, 3–36. doi: 10.1111/j.1467-9868.2010.00749.x
Wood, S. N. (2013). A simple test for random effects in regression models. Biometrika 100, 1005–1010. doi: 10.1093/biomet/ast038
Wood, S. N. (2017). Generalized Additive Modles: An Introduction with R, 2nd Edn. Boca Raton, FL: Chapman and Hall/CRC. 1–496.
Keywords: alien invasive plants, biological invasions, restoration, riparian ecosystems, distribution modeling, invasion hotspots
Citation: Pabst R, Dias FS, Borda-de-Água L, Rodríguez-González PM and Capinha C (2022) Assessing and Predicting the Distribution of Riparian Invasive Plants in Continental Portugal. Front. Ecol. Evol. 10:875578. doi: 10.3389/fevo.2022.875578
Received: 14 February 2022; Accepted: 30 March 2022;
Published: 06 May 2022.
Edited by:
Jorge Capelo, University of Porto, PortugalReviewed by:
Hélia Marchante, Instituto Politécnico de Coimbra, PortugalAlbano Augusto Figueiredo, University of Coimbra, Portugal
Sílvia Ribeiro, Universidade de Évora, Portugal
Copyright © 2022 Pabst, Dias, Borda-de-Água, Rodríguez-González and Capinha. This is an open-access article distributed under the terms of the Creative Commons Attribution License (CC BY). The use, distribution or reproduction in other forums is permitted, provided the original author(s) and the copyright owner(s) are credited and that the original publication in this journal is cited, in accordance with accepted academic practice. No use, distribution or reproduction is permitted which does not comply with these terms.
*Correspondence: Rebecca Pabst, cGFic3QucmViZWNjYUBnbWFpbC5jb20=; César Capinha, Y2VzYXJjYXBpbmhhQGNhbXB1cy51bC5wdA==