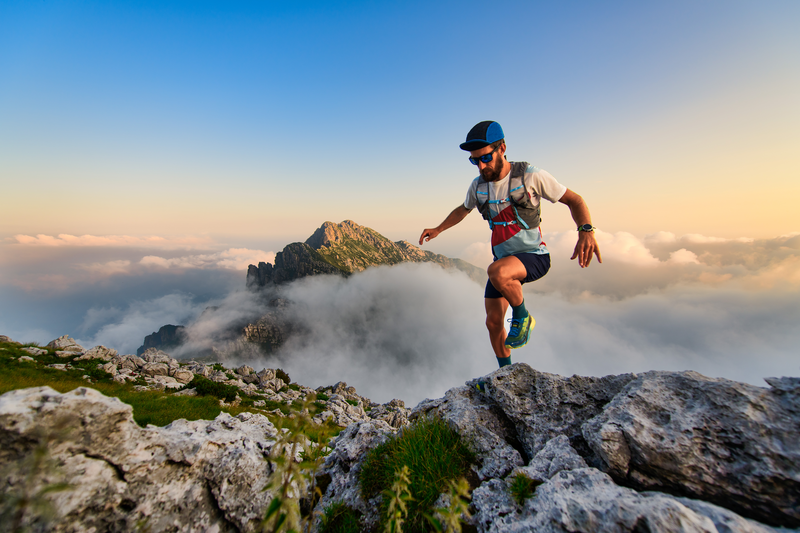
94% of researchers rate our articles as excellent or good
Learn more about the work of our research integrity team to safeguard the quality of each article we publish.
Find out more
ORIGINAL RESEARCH article
Front. Ecol. Evol. , 15 April 2022
Sec. Biogeography and Macroecology
Volume 10 - 2022 | https://doi.org/10.3389/fevo.2022.869258
The contributions and interaction of biotic and abiotic processes in community assembly are crucial for understanding the elevational patterns of biodiversity. The combined analyses of taxonomic, phylogenetic, and functional diversity are necessary to resolve this issue. By investigating vegetation in 24 transects sampled on Hongla Snow Mountain, in the central Hengduan Mountain Ranges in Southwest China, we delineated the elevational vegetation spectrum on the eastern and western slopes, analyzed the elevational variation in taxonomic, phylogenetic, and functional diversity of woody plant species, and compared the community structure of phylogeny and function in the low-elevational shrublands, mid-elevational forests, and alpine shrubs and meadows. The species richness, phylogenetic diversity, and functional diversity of woody plants showed nonstandard hump-shaped patterns with two peaks along the elevational gradient. The community structure of phylogeny and function (including tree height, leaf area, specific leaf area, leaf thickness, bark thickness, and wood density) clustered in the low-elevation shrub communities, being random and over-dispersed in mid-elevational forests. The phylogenic structure was over-dispersed in alpine communities, whereas the functional structure was clustered. Elevational patterns in taxonomic, phylogenetic, and functional diversity, together with the mean and variation in woody plant functional traits, suggested drought stress and freeze stress as environmental filters dominating the assembly of low and high elevation non-forest communities, and a conspicuous effect of biotic facilitation was also suggested for alpine habitats. By contrast, interspecific competition dominated the community assembly of forests at mid-elevations. The difference in biodiversity indices between the west and east slopes reflected the effects of the Indian Monsoon on the geomorphic patterns of ecosystem structure. These results increased our understanding of biodiversity patterns and underlying mechanisms in the Hengduan Mountains of Southwest China and highlighted the priorities for biodiversity conservation in this region.
Understanding the spatialvariation in species diversity and exploring the underlying mechanisms are core issues in community ecology and biogeography (Hubbell, 2001). The elevational variation in species diversity is one of the most classic and widely studied biodiversity patterns (Rahbek et al., 2019). Elevational gradients incorporate gradient effects of multiple environmental factors. Therefore, studying elevational patterns of species diversity is important for exploring species’ responses to environmental dynamics, understanding the processes of community assembly, for predicting future impacts of climate change on biodiversity (Corlett and Westcott, 2013; Sundqvist et al., 2013).
Mechanisms of species maintenance can be divided into deterministic processes based on niche theory (i.e., environmental filtering and interspecific competition) (Webb et al., 2002; Dante et al., 2013) and stochastic processes based on neutral theory (e.g., dispersal limitation) (Hubbell, 2001; Vellend, 2010), both of which jointly shape diversity patterns in variable formats (Adler et al., 2007; Wennekes et al., 2012). While environmental filtering has been found to be more important for community assembly in a stressful habitat, competitive exclusion is generally more crucial in ecologically healthy conditions (Coyle et al., 2014). By including information about community phylogeny and species functional traits, researchers can verify biodiversity pattern hypotheses from the perspective of evolutionary history and ecological adaptation, which is helpful to understand the imprints of long-term processes on community assembly and species coexistence (Swenson and Enquist, 2009; Purschke et al., 2013).
Plant functional traits are generally variable and indicate plant responses and adaptations to environmental changes. Maximum plant height (MH) represents the ability of a species to compete for light and is significantly limited by water availability (Moles et al., 2009). Leaf traits, such as size, thickness, and specific leaf area (SLA), can represent a strategic trade-off between carbon assimilation and water-use efficiency and indicate the capacity of a species for temperature regulation (Michaletz et al., 2016; Fauset et al., 2018). Bark thickness (BT) indicates the degree of stem protection and the capacity of the stem to store water (Rosell, 2016). Wood density (WD) is related to the growth rate (Falster, 2006; Preston et al., 2006). Therefore, multiple plant functional traits can be combined to reflect the intensity of different environmental stresses on plants along an elevational gradient and reveal the primary drivers of plant community assembly (Reich, 2014; Simova et al., 2017).
Many functional traits of species are phylogenetically conserved (Chazdon et al., 2003; Swenson et al., 2007). The more closely the species relate, the more similar their traits are likely to be, and the more consistent their adaptations to environmental filters are. Therefore, phylogenetic diversity (PD) provides more information about community assembly mechanisms than taxonomic diversity (Shi et al., 2021). In contrast, other functional traits, such as leaf morphological traits (SLA and leaf size), are more adaptive (Kraft and Ackerly, 2010) and thus, can provide direct indications of environmental pressures. The degree of similarity between phylogenetic relationships and functional traits depends on the intensity of the phylogenetic signals controlling those traits (Chalmandrier et al., 2016). Therefore, biodiversity analyses combining taxonomic, phylogenetic, and functional information are of practical importance to reveal the roles of the ecological and evolutionary process for species maintenance and have been developed as effective analytical protocols (Kraft et al., 2011; Cadotte et al., 2013; de Bello et al., 2017).
Studies have shown that community assembly along elevational gradients is nonrandom and that abiotic filtering dominates in areas with harsh environments (Siefert et al., 2013). Therefore, under the hypothesis that functional traits are phylogenetically conservative, plant phylogenetic or functional diversity (FD) will be lower than randomly expected in a harsh environment due to environmental filtering (Webb et al., 2002). However, environmental filtering effects on phylogeny and function are variable in communities among different environmental contexts. For example, at high elevations, where plant traits are generally clustered (i.e., similar among species) to adapt to low temperatures, the phylogenic structure of the community could also be clustering, because species with similar traits tend to be more closely related because of phylogenetic conservatism (Xu et al., 2017). In another case, the phylogenic structure can also be over-dispersed, when interspecific facilitation (e.g., nurse effects) helps tolerance to the harsh environment (Schoeb et al., 2012; Culmsee and Leuschner, 2013). At low elevations, the results are also differentiated. For example, Kluge and Kessler (2011) studied fern communities in the mountain forests of Costa Rica, and found that the structure of phylogeny and function both clustered in the low-elevational areas. In contrast, a study in the Sierra Nevada showed that the phylogenetic structure of plant communities was over-dispersed while the structure of function was clustered at low elevations (Molina-Venegas et al., 2016). Another possibility is that convergent evolution occurs due to environmental filtering, and species from different lineages adapt to the harsh environment with similar functional traits, leading to a scenario of high PD but low FD. Thus, patterns of community assembly along elevation gradients are complex and variable among regions in terms of the roles of biotic interactions, abiotic filtering, as well as historical processes of environmental changes and speciation.
The Hengduan Mountain Ranges region in Southwest China is one of 35 global biodiversity hotspots (Mittermeier et al., 2004). The collision of the Indian Plate northward to the Eurasian Plate results in the intensively folded geomorphology in this region (Zhu et al., 2004), with the uplift of the Tibetan Plateau intensifying the Southern Asian Monsoon and its influence on the climate in Southwest China (Li, 1999). The geomorphic framework of parallel mountains and valleys extending roughly in a latitudinal direction forms regional vegetation and environmental differentiation (Zhang et al., 1997; He et al., 2005). Significant and variable vertical climate gradients have enriched the habitat types, with prominent longitudinal connectivity and latitudinal isolation. This environmental context provides a unique combination of active speciation (i.e., “cradle”) and stable preservation (i.e., “museum”) mechanisms for the maintenance of biodiversity in this region (Thorne, 1999; Chen et al., 2018).
The vertical vegetation spectrum in the Hengduan Mountains is characterized by a significant continuous band of forests at mid-elevation, and non-forest vegetation in both alpine (shrubs and meadow) and dry-hot valley (i.e., savanna-like vegetation) environments (Liu et al., 2016). Recent studies have explored plant diversity patterns along elevational gradients (Yang et al., 2016; Luo et al., 2019), the unique environmental filtering effects, and biotic interactions on speciation processes in the extreme climate of the alpine “sky islands” (Chen et al., 2015; Niu et al., 2017). Meanwhile, studies have also estimated the effects of environmental isolation on the species turnover (Han et al., 2016) of shrub communities and the phylogeography of endemic plant species (Wang et al., 2020) in the dry valleys. In addition to evolutionary and modern ecological processes, geological and historical climatic processes are thought to jointly contribute to the existing spatial patterns of extraordinarily high biodiversity in this region (Rana et al., 2020; Wang et al., 2020). However, a general understanding of the elevational patterns of plant species diversity and the roles of underlying mechanisms remains lacking. In particular, phylogenetic and functional information has rarely been integrated with details of species composition. However, this is necessary to determine the crucial factors and processes driving plant community assembly and regulating the elevational patterns of species diversity in this region.
The present study systematically sampled the species compositions and plant traits along elevational gradients on the west and east slopes of Hongla Snow Mountain, at the center of the Hengduan Mountain Ranges. We integrated taxonomy, community phylogeny, and function to explore the determinants of woody plant diversity and investigated how these communities respond to different biotic or abiotic gradients along elevational gradients. Based on these patterns, we tested the following hypotheses about the underlying mechanisms involved: (1) the assembly processes in low and high elevation non-forest communities are subjected to strong environmental stress, which leads to structural clustering of phylogeny and function. In the habitats of mid-elevation, interspecific competition has a leading role in community assembly, so that the structure of phylogeny and function is dispersed; (2) plants in the dry valleys are primarily stressed by water deficiency, whereas those in alpine habitats are limited by energy. This difference and the plant responses should be reflected by community structure in phylogeny and function; and (3) differences in precipitation on the two aspects of the mountain, caused by topographical effects of the monsoon (Yang et al., 2016), should also affect their elevational patterns of plant PD and FD.
As one of 35 global biodiversity hotspots, the Hengduan Mountains have the most intact native ecosystems and complete elevational vegetation spectrum in China. The Ningjing-Yunling Mountain range is at its core, with two large rivers (Lancang and Jinsha) running in parallel on its west and east side. The Ningjing-Yunling Mountain Range has a typical elevational vegetation spectrum, characterized by shrubs in arid and warm low elevation valleys, mixed broad-leaved and coniferous forests in the mid-elevation, and evergreen shrubs and meadow above the alpine treeline (Yang et al., 2016). Such conspicuous variation in vegetation appearances indicates a strong environmental gradient and divergence in primary plant assembly processes. Our study site, the Hongla Snow Mountain (29°40′ 48′ N, 98°35′ 35′ E), is located in the northern Ningjing-Yunling Mountain range (Figure 1a). The summit of Hongla Snow Mountain is 5,036 m a.s.l., whereas the water surface elevation is at 2,620 m for the Lancang River and 2,495 m for the Jinsha River.
Figure 1. The geographical location of the study area and sampling sites. (a) Location of the study area; (b,c) the locations of the sampling sites on the west and east slopes of Hongla Snow Mountain; (d) the setting diagram of the transects, each transect of 0.1 hm2 is composed of ten 10 m × 10 m plots.
The climate of this region is characterized by marked synchronous seasonality in precipitation and temperature, mainly influenced by the Indian Ocean monsoon coming from the west in summer (Li et al., 2011), and a cold high pressure that forms over the Tibetan Plateau in winter. The interaction between the monsoon and the steep mountain range creates vertical bands of vegetation with significant differences. The valleys at the bottom of the mountain range have a dry-hot climate, resulting in a savanna-like vegetation type, dominated by shrubs and grass with scattered trees; the mid-elevations have a cool and humid climate, resulting in forests dominated by evergreen broad-leaved and coniferous trees; and the high elevations have a cold and humid climate, resulting in alpine vegetation dominated by dwarf evergreen scrubs and meadow.
In July–August 2020, we established 25 transects along the elevation gradient on the west and east slopes of Hongla Snow Mountain, including 12 on the west slope and 13 on the east slope (Figures 1b,c). Each transect area was 0.1 ha (10 m × 100 m), with the long side perpendicular to the slope. The elevations of the transects ranged from 2,700 to 4,900 m on the west slope, and from 2,500 m to 4,700 m on the east slope. The elevational difference between adjacent transects was 200 m. Each transect was divided into ten plots of 100 m2 (10 m × 10 m) (Figure 1d). We investigated the plants in the community at the plot scale. We recorded the species name, diameter at breast height (DBH), stem height, population abundance, and coverage for woody plants. The species name, clump stem, height, population abundance, and coverage for herbaceous plants. After the survey, 74 woody species were recorded, with 48 occurring on the western slope and 56 on the eastern slope, and 348 herbaceous species were recorded, with 197 occurring on the western slope and 261 on the eastern slope. In each transect, we collected four or more samples of leaves, bark, and wood for each woody species. All samples were collected from at least four healthy individuals with average DBH, growing under a normal light condition. Fully developed mature leaves were collected on the sunny surface of the outer canopy of each plant. The wood and bark of trees were cut together in a 10 cm × 5 cm area from the main trunk. A 10-cm section of the trunk was cut from each plant for shrubs. All samples were packed in self-sealing bags and taken to the laboratory for the following processing.
A total of 45 species of woody plants were collected on the west and east slopes, with 353 individual plants of 34 species on the west slope and 424 individuals of 38 species on the east. The total coverage of woody species in the plots on the east and west slopes were 95.28 and 92.93% on average (Supplementary Table 1), respectively. The fresh mass of leaf samples was measured using 1/1,000 analytical balance, and the leaf area was measured using the CanoScan LiDE 300 portable scanner. Three points were uniformly selected to measure the thickness along the direction of the central leaf vein using vernier calipers (0.01 mm resolution), avoiding the veins. The thickness of a leaf is the mean of three thickness measurements, and the mean leaf thickness of three measured individuals represents that of a species. The fresh weight of wood samples was weighed with bark stripped, and the volume was measured using the drainage method. In addition, bark thickness was measured with the method of leaf thickness measurement to avoid bark bumps. The leaf samples were oven-dried at 105°C for 20 min, then at 65°C for 72 h to a constant mass, and weighed for dry mass. The wood samples were first exposed to air for about 1 month, then dried at 65°C for 72 h to reach a constant mass and weighed for dry weight. The leaf, bark, and wood traits were calculated according to the following (Cornelissen et al., 2003):
MH = Maximum plant height of a species measured in all transects (m).
LA = Average leaf area of all measured woody individuals (mm2).
LT = Average leaf thickness of all measured woody individuals (mm).
BT = Average bark thickness of all measured woody individuals (mm).
SLA = Fresh leaf area (mm2)/Dry leaf mass (mg).
WD = Dry wood mass (g)/Wood volume (cm3).
Two chloroplast gene regions (rbcL and matK) and the internal transcribed spacer (ITS) of nuclear ribosomal DNA were downloaded from The National Center for Biotechnology Information (NCBI)1 using accession numbers for phylogenetic reassembly. For species with no sequences in NCBI, the sequences of their close relatives in the same genus were used as a proxy (Supplementary Table 2). The sequences were aligned in MAFFT version 7 and then concatenated in PhyloSuite v1.2.2. The best substitution model for each gene region was chosen by using jModelTest 2.1.7. Bayesian reconstructions were performed to estimate phylogenetic relationships and divergence times using BEAST v2.6.3. We performed the phylogeny reconstructions with an uncorrelated lognormal relaxed molecular clock model and a Yule speciation tree prior. Six-time calibration priors were set according to Zanne et al. (2014; Supplementary Table 3). A Markov chain Monte Carlo simulation for 100 million generations was set for the reconstructions. Tracer v1.7.1 was used to examine the log file and ensure that all effective sample size values were above 200. The output tree was annotated using TreeAnnotator v2.6.3, with 25% pre-burn-in (see Figure 2).
Figure 2. The phylogenetic tree of woody plant species was recorded in all plots on the west and east slopes of Hongla Snow Mountain, assembled from molecular sequences.
The plant species richness (SR) in each sample plot was selected to express the taxonomic α diversity, and this was counted on both the 100-m2 plot and the 1,000-m2 transect scales for all species and each growth form (trees, shrubs, and herbs). To compare the SR, PD and FD of the communities using consistent information, we specifically focused the indices on woody plant species. The minimum spanning tree distance (Faith, 1992) was calculated to obtain the original phylogenetic diversity (PD), and functional diversity (FD) was quantified by referring to the algorithm for PD (Petchey and Gaston, 2002; Liu et al., 2013). To eliminate the effect of SR on PD and FD, we use a simple linear model with the SR to predict the residuals of PD and FD (hereafter Corrected PD and FD) to represent their diversity. We also used a null model (independent swap) of the independent exchange algorithm (Gotelli, 2000) to standardize the mean pairwise distance (MPD) of the phylogeny using the formula in the “Phylocom” package in R (Webb et al., 2008):
We applied the formula to the standardized mean pairwise distance of function as calculated for MPD. We then repeated the calculation 999 times (Proches et al., 2006; Webb et al., 2008) and drew a line with zero values to represent the random state to estimate the divergence or clustering of phylogeny and function on the elevational gradient and to explore the corresponding ecological processes. Positive SES values indicated that the phylogeny (or function) had a tendency to diverge, and high quantiles (p > 0.95) indicate that the phylogeny (or function) was significantly divergent, or the distance of the phylogeny (or function) between coexisting species was larger than the randomly expected value. Negative SES values indicated that the phylogeny (or function) had a significant tendency to cluster, and low quantiles (p < 0.05) indicate that the phylogeny (or function) clustered significantly, or the distance of the phylogeny (or function) between coexisting species was smaller than the randomly expected value (Webb et al., 2008).
Based on the above methods, we first studied the elevational patterns in taxonomic diversity, PD, and FD on the west and east slopes at the transect scale. We then calculated the standardized average pairwise distance (SESMPD) of the phylogeny and function by comparing the data with the null models.
The community-weighted mean (CWM) values of six functional traits were calculated by relative coverage weighting and fitted using the locally weighted scatter plot smoothing method. The coefficient of variation (CV) was used to measure the differences in functional traits at the community level, and to infer the strategy of plant adaptation to the dominant environmental stress. The Kruskal–Wallis test was used to compare the values of taxonomic diversity, PD, and FD, as well as CWM and CV of the community structure of phylogeny and function at low, middle, and high elevations on the west and east slopes. All analyses were performed using R v4.1.0.
The elevational vegetation spectrums on the west and east slopes of Hongla Snow Mountain were similarly composed of a wide band of shrubs communities in the low-elevation dry valley, a forest band at mid-elevations, and an alpine band of shrubs and meadow (Figure 3A). On the west slope, shrub communities of variable densities extended from the river bank (2,650 m a.s.l.) upward to 3,300–3,500 m a.s.l., mainly dominated by deciduous species Sophora davidii, Indigofera calcicola, and Ceratostigma minus. The lower part of the forest zone comprised temperate forests ranging from 3,300 to 4,100 m a.s.l., jointly dominated by sclerophyllous evergreen Quercus aquifolioides and coniferous Pinus densata in a patchy pattern. The upper part of the forest zone started from ∼4,100 m, mostly dominated by Picea likiangensis var. balfouriana, and extended to the alpine treeline at ∼4,500 m a.s.l. Alpine dwarf shrubs and cushion vegetation patches extended up to 4,900 m a.s.l., with Sabina pingii var. wilsonii, several species of Rhododendron, and Arenaria densissima as the dominant species. On the east slope, the shrubs extending in the dry-hot valley at 2,500–3,100 m a.s.l. were dominated by Sageretia horrida and Bauhinia brachycarpa; The forest zone at 3,100–3,900 m a.s.l. comprised broad-leaved forest patches dominated by Q. aquifolioides, and the subalpine forests at 3,900–4,300 m a.s.l. were generally dominated by Abies georgei var. smithii. The alpine vegetation at 4,200-4,700 m is composed of patches of shrubs and meadow. The evergreen R. nivale and deciduous Potentilla fruticosa were dominant shrub species, whereas multiple species of Pedicularis, Saussurea, Primula, Poa, and Carex comprised the colorful alpine meadows.
Figure 3. The elevational patterns of vegetation types and taxonomic, phylogenetic, and functional diversity on two aspects of Hongla Snow Mountain. (A) Vegetation spectrum on both aspects. Red lines represent the boundaries between vegetation zones on the west slope, and blue lines represent those on the east slope. (B–D) Reflect the elevational patterns of SR, Corrected PD, and Corrected FD of woody species.
The mean plant species density (per 100 m2) showed two peaks at similar elevational patterns on the east and west slopes of Hongla Mountain (Supplementary Figure 1). The first peak appeared at 3,100–3,300 m a.s.l. and was mainly induced by a peak of SR in shrubs and herbaceous species, whereas a richness peak mainly caused the second peak only in herbaceous species. In contrast, tree SR, which was very low compared with that of shrubs and herbs, showed a standard hump-shaped pattern along the elevational gradient, peaking at 3,900–4,300 m a.s.l. in the mixed forests of deciduous and coniferous species.
For woody plants measured at the transect scale (1,000 m2), the elevational patterns of SR on the west and east slopes of the mountain both revealed a nonstandard hump-shaped pattern with two peaks. A primary peak similarly appeared at the boundary between the valley shrub zone and the forest zone (3,100 and 3,300 m on the east and west slopes, respectively), and a secondary peak just above the alpine treeline (∼4,300 m a.s.l.). SR of the east slope was higher than that of the west slope at the same elevation, except in the alpine vegetation above 4,300 m, where it was lower (Figure 3B). After controlling for the effect of SR, the pattern of Corrected PD showed an increasing trend along with elevation, with two peaks on the western slope and three peaks on the eastern slope. The trend of Corrected PD turned decreasing above 4300 m a.s.l. on both slopes (Figure 3C). The elevational patterns of Corrected FD increased upward from the dry valleys, peaked at around the alpine treeline, and then turned to decrease at higher elevations (Figure 3D).
Measured by the SESMPD, the community structure of phylogeny and function showed comparable elevational patterns on both aspects of the mountain (Figure 4). In general, the phylogeny and function of the shrub communities in the dry-hot valleys tended to be clustered (or converged) rather than random; at mid-elevations, the community structure of phylogeny and function changed gradually from clustered to over-dispersed. At elevations above the alpine treeline, the community phylogeny remained over-dispersed, whereas the function turned to be clustered again. These contrasting patterns between the phylogenetic and functional traits structure occurred on the west and east slopes.
Figure 4. The elevational patterns of community phylogenetic (red) and functional (blue) structure on the west (A) and east (B) slopes of the Hongla Snow Mountain. The asterisks indicate statistic significance (P < 0.05).
Concerning the six functional traits of woody plants, the CWM values of five traits showed unimodal elevational patterns on both the west and the east slopes; among which MH, LT, and BT had similar patterns, peaking in the subalpine conifer forests, whereas SLA showed an opposite pattern, with its minimum value occurring at the same elevation. In contrast, peak CWM values of LA occurred in the middle of the forest zone, corresponding to the temperate mixed forests of deciduous and coniferous species. The WD value decreased from low to high elevations (Figure 5). When the complete elevational gradient was classified into three sections (i.e., low-elevation shrublands, mid-elevation forests, and alpine shrubs and meadow), the (log-transformed) median value of the six functional traits were all significantly different (P < 0.05) among the sections, and the values of MH, LA, SLA, BT, and WD of non-forest communities were all higher in the dry valleys than in the alpine habitats, whereas LT was higher in the alpine than in the dry valley habitats (Figure 6).
Figure 5. The elevational patterns of abundance-weighted community mean values of six functional traits of woody plants on two slopes of Hongla Snow Mountain. Dots are plot values and lines represent theestimates of Lasso regression of transect value; Red indicates the west slope, blue indicates the east slope. (A) MH, maximum plant height; (B) LA, leaf area; (C) LT, leaf thickness; (D) SLA, specific leaf area; (E) BT, bark thickness; (F) WD, wood density. Vertical solid and dash lines represent lower and upper boundaries of the forest belt on thewest and east slopes.
Figure 6. Differences of the abundance-weighted community (log-transformed) mean value of functional traits in different vegetation types on two aspects of the mountain. Low, shrubs at low elevation; Mid, forests at middle elevations; High, alpine shrubs and meadow. (a–c) Indicate different levels among three sections with statistical significance. (A–F) Corresponds to the conditions of six plant functional traits.
The CVs of six functional traits from large to small were LA, MH, BT, LT, SLA, and WD. The CVs of alltrait values were larger at mid-elevations than in the dry-hot valleys and cold alpine habitats (Supplementary Figure 2). Compared with the mid-elevation forest communities, the CV values of six traits were not significantly different between the non-forest sections at lower and higher elevations (Supplementary Figure 3).
The elevational patterns of SR on both aspects of Hongla Snow Mountain differed from either the declining pattern typical of humid tropical mountains (Homeier et al., 2010; Clark et al., 2015; Worthy et al., 2019), or the hump-shaped unimodal pattern often observed in temperate regions (Rahbek, 1995; Shen et al., 2004). Instead, it showed a bimodal distribution (Figure 3B), which was similar to the results of the surrounding area (Wang et al., 2007; Wu et al., 2014; Yang et al., 2016). The primary peaks in SR on the east and west slopes occurred at 3,100 and 3,300 m, respectively, representing the ecotones between dry-hot valley shrubland and the mid-elevation forest belt. The difference between the two aspects of the mountain was that the lower part of the forest belt comprised mixed deciduous-coniferous forests and the sclerophyllous evergreen broadleaf Q. aquifolioides forest, respectively. The secondary peaks in SR occurred at the alpine treeline ecotone, the upper boundary of the forest belt. It is notable that, from the valley bottom upward, the elevations of vegetation belt boundaries on the west slope were all higher than those on the east slope, which appears to be determined by the elevation of the valley bottom, an indicator of the mass elevation effect on climate (Zhao et al., 2015). The trend of Corrected PD on the east and west slopes is not consistent (Figure 3C), and the absence of a peak in the low-elevation dry-hot valley on the west slope compared to the east slope may be related to the severe drought stress on the west slope. In tropical mountains areas, FD generally decreases with increasing elevation (Ding et al., 2019; Worthy et al., 2019). However, in the current study, the trend in Corrected FD showed non-standard hump-shaped curves with smaller values only in the lower-elevation section (Figure 3D). This may be related to the convergent evolution due to intense drought stress at low altitudes.
The SR values of shrubland in the valley on the east slope were higher than those on the west slope. Meanwhile, plant functional traits, including MH, LA, BT, and WD, also showed larger variability on the east slope than on the west slope. This indicated that environmental stress at low elevations on the west slope is more intensive, consistent with conditions on Baima Snow Mountain in the central section of the Ningjing-Yunling Mountain range (Yang et al., 2016). The SR, Corrected PD, and Corrected FD values of forest communities on the east slope were also higher than those on the west slope, which might be related to the higher precipitation in this section, especially in the upper part of the forest belt (Zhang, 1998), as also found by Yang et al. (2016). However, the SR, Corrected PD, and Corrected FD values of alpine vegetation on the west slope were higher than those on the east slope. In the field, the alpine landscape on the east slope was characterized by shrub patches scattered among homogeneous alpine meadows, whereas, on the west slope, the alpine landscape comprised amore heterogeneous stony scrubland with smaller meadow patches (Figure 3A). This difference in the heterogeneity of micro-topography could have a significant role in assembling different plant communities at different elevations.
Hongla Snow Mountain has an elevational vegetation spectrum typical of the Hengduan Mountains region, which comprises a mid-elevation forest belt with shrub communities at high and low elevations (Liu et al., 1985). In this study, the community structure of phylogeny and function tended to cluster on both the west and east slopes at low elevations (Figure 4), suggesting environmental filtering as a dominant driver of community assembly. This is generally linked to the typical hot and arid climate in the valleys, which forms a unique “valley savanna” type vegetation dominating arid valleys along the main rivers in the Hengduan Mountain region (Jin and Ou, 2000; Yang, 2007). The dry-hot valley environmental context results from interactions among historical, geomorphological, and anthropogenic drivers (Xu et al., 1985; Zhang et al., 1997; Shen et al., 2016), and has significant effects on the flora composition, phylogeography, and plant trait evolution (Liu et al., 2011). The community structure of phylogeny and function at mid-elevations went from random to over-dispersed. This is generally interpreted as enhanced interspecific competition having a dominant role in community assembly under suitable mid-elevational environmental conditions. Plants living above the alpine treeline face extreme freezing stress; thus, environmental filtering has a prominent role in community assembly, as supported by unique evidence of plant trait adaption, such as flower morphology evolution (Peng et al., 2012) and plant camouflage (Niu et al., 2017). However, unlike at low elevations, the over-dispersed phylogenetic structure in the alpine communities implies a distinct assembly process, which could be linked to facilitation among species (Callaway et al., 2002; Schoeb et al., 2012). For example, studies in the surrounding areas have also discovered interspecies facilitation that helps plants to adapt to the extreme alpine climate and maintain SR (Luo et al., 2016). Yang et al. (2010) found that associations between the cushion plant Arenaria polytrichoides (Caryophyllaceae) and other (less related) plant species indicated a positive effect of species coexistence in these communities.
The CWM and CV of the functional traits were higher in the mid-elevation forest zone than in the non-forest vegetation zones, and the community structure of phylogeny and function were random to over-dispersed, indicating that environmental stress was weak and that interspecific competition was dominant in community assembly (Kluge and Kessler, 2011; Montano-Centellas et al., 2020). In contrast, the community structure of function was clustered in the dry valley and alpine habitats, showing similar trends although resulting from different environmental filters. For example, low plant heights occurred in arid environments because evapotranspiration from a high canopy could not be supported by the water supply from the root system (Hartmann, 2011), whereas tree height was limited in the alpine environment by the temperature threshold that blocked the formation of the plant cell (Körner, 1998). Small leaf area was also common but induced by distinct mechanisms. To avoid excessive transpiration and water loss in arid environments, plants tended to form a small leaf area (Shields, 1950), whereas, in a low-temperature environment, such as alpine meadows, the growing season for leaves was short. Plants can maintain a stable photosynthetic carbon acquisition capacity by increasing the nitrogen content of leaves, whereas the cost of respiration and transpiration of leaflets is lower (Reich et al., 1992). The smaller mean BT is mainly related to stem size (Lawes et al., 2011; Rosell et al., 2017). Shrubs dominated in the dry valley and alpine habitats, whereas trees had a much larger DBH; thus, the CWM value of BT was generally much higher in forests. In contrast, woody density showed a clear decreasing trend with increasing elevation (Chave et al., 2006). The wood density of drought-tolerant species was higher (Niinemets, 2001; Poorter and Markesteijn, 2008), whereas drought-intolerant species tended to have the lower woody density to store water in the stem (Markesteijn and Poorter, 2009), which was related to increasing precipitation with elevation.
Ecosystems in dry-hot valleys and alpine regions are subjected to strong environmental stresses and, thus, are very sensitive to climate change and natural disasters (Yand et al., 2007; Kim and Donohue, 2013). Species living in such environments have evolved over millions of years to adapt to such unique and extreme conditions (Engler et al., 2011). Therefore, anthropogenic global warming at an unprecedented rate, accompanied by extreme climatic events, poses particularly significant threats to these ecosystems and the species living in them. Thus, the precise identification of such threats, and protection of these ecosystems using suitable and sustainable approaches should take priority in biodiversity conservation policies and action plans.
The contributions of multiple mechanisms to elevational patterns of biodiversity pose a lasting challenge despite decades of research efforts. Scale-dependent and regionalized processes add extra complexity to fully understanding such patterns. With regard to the typical elevational vegetation spectrum in the Hengduan Mountain region, our combined analyses of taxonomic, phylogenetic, and functional diversities revealed the zonal differentiation of dominant community assembly factors. Interspecific competition should have a leading role at mid-elevations with the appropriate environment. Whereas environmental filtering dominated community assembly at low and high elevations, the phylogenetic structure of alpine communities also implied an effect of biotic facilitation. The difference in biodiversity indices between the west and east slopes of the mountain also reflected the imprints of the Indian Monsoon on the geomorphological patterns of ecosystem structure. These results increased our understanding of biodiversity patterns and underlying mechanisms in the Hengduan Mountains of Southwest China and clarified the urgent need for biodiversity conservation in this region.
The original contributions presented in the study are included in the article/Supplementary Material, further inquiries can be directed to the corresponding author/s.
ZS and GL designed the study. FZ, TY, CL, and WR collected the data. FZ, TY and GL analyzed the data. GY formulated the phylogeny tree. FZ and ZS wrote the first draft of the manuscript. All authors reviewed the manuscript.
This study was sponsored by the Second Tibetan Plateau Scientific Expedition and Research Program (STEP) grant no. 2019QZKK0402 and Biodiversity Investigation and Assessment Project of the Ministry of Ecology and Environment (2019-Huanbaogongzuo-061-N-001-B-008).
The authors declare that the research was conducted in the absence of any commercial or financial relationships that could be construed as a potential conflict of interest.
All claims expressed in this article are solely those of the authors and do not necessarily represent those of their affiliated organizations, or those of the publisher, the editors and the reviewers. Any product that may be evaluated in this article, or claim that may be made by its manufacturer, is not guaranteed or endorsed by the publisher.
The Supplementary Material for this article can be found online at: https://www.frontiersin.org/articles/10.3389/fevo.2022.869258/full#supplementary-material
Adler, P. B., HilleRisLambers, J., and Levine, J. M. (2007). A niche for neutrality. Ecol. Lett. 10, 95–104. doi: 10.1111/j.1461-0248.2006.00996.x
Cadotte, M., Albert, C. H., and Walker, S. C. (2013). The ecology of differences: assessing community assembly with trait and evolutionary distances. Ecol. Lett. 16, 1234–1244. doi: 10.1111/ele.12161
Callaway, R. M., Brooker, R. W., Choler, P., Kikvidze, Z., Lortie, C. J., Michalet, R., et al. (2002). Positive interactions among alpine plants increase with stress. Nature 417, 844–848. doi: 10.1038/nature00812
Chalmandrier, L., Münkemüller, T., Lavergne, S., and Thuiller, W. (2016). Effects of species’ similarity and dominance on the functional and phylogenetic structure of a plant meta-community. Ecology 96, 143–153. doi: 10.1890/13-2153.1
Chave, J., Muller-Landau, H. C., Baker, T. R., Easdale, T. A., Easdale, T. A., ter Steege, H., et al. (2006). Regional and phylogenetic variation of wood density across 2456 neotropical tree species. Ecol. Appl. 16, 2356–2367. doi: 10.1890/1051-0761(2006)016[2356:RAPVOW]2.0.CO;2
Chazdon, R. L., Careaga, S., Webb, C., and Vargas, O. (2003). Community and phylogenetic structure of reproductive traits of woody species in wet tropical forests. Ecol. Monogr. 73, 331–348. doi: 10.1890/02-4037
Chen, J., Schöb, C., Zhou, Z., Gong, Q., Li, X., Yang, Y., et al. (2015). Cushion plants can have a positive effect on diversity at high elevations in the Himalayan Hengduan Mountains. J. Veg. Sci. 26, 768–777.
Chen, Y., Deng, T., Zhou, Z., and Sun, H. (2018). Is the East Asian flora ancient or not? Natl. Sci. Rev. 5, 920–932. doi: 10.1093/nsr/nwx156
Clark, D. B., Hurtado, J., and Saatchi, S. S. (2015). Tropical rain forest structure, tree growth and dynamics along a 2700-m elevational transect in Costa Rica. PLoS One 10:e0122905. doi: 10.1371/journal.pone.0122905
Corlett, R. T., and Westcott, D. A. (2013). Will plant movements keep up with climate change? Trends Ecol. Evol. 28, 482–488. doi: 10.1016/j.tree.2013.04.003
Cornelissen, J. H. C., Lavorel, S., Garnier, E., Diaz, S., Buchmann, N., Gurvich, D. E., et al. (2003). A handbook of protocols for standardised and easy measurement of plant functional traits worldwide. Aust. J. Bot. 51, 335–380. doi: 10.1071/Bt02124
Coyle, J. R., Halliday, F. W., Lopez, B. E., Palmquist, K. A., Wifahrt, P. A., and Hurlbert, A. H. (2014). Using trait and phylogenetic diversity to evaluate the generality of the stress-dominance hypothesis in eastern North American tree communities. Ecography 37, 814–826. doi: 10.1111/ecog.00473
Culmsee, H., and Leuschner, C. (2013). Consistent patterns of elevational change in tree taxonomic and phylogenetic diversity across Malesian Mountain forests. J. Biogeogr. 40, 1997–2010. doi: 10.1111/jbi.12138
Dante, S. K., Schamp, B. S., and Aarssen, L. W. (2013). Evidence of deterministic assembly according to flowering time in an old-field plant community. Funct. Ecol. 27, 555–564. doi: 10.1111/1365-2435.12061
de Bello, F., Smilauer, P., Diniz, J. A. F., Carmona, C. P., Lososová, Z., Herben, T., et al. (2017). Decoupling phylogenetic and functional diversity to reveal hidden signals in community assembly. Methods Ecol. Evol. 8, 1200–1211. doi: 10.1111/2041-210x.12735
Ding, Y., Zang, R., Lu, X., Huang, J., and Xu, Y. (2019). The effect of environmental filtering on variation in functional diversity along a tropical elevational gradient. J. Veg. Sci. 30, 973–983. doi: 10.1111/jvs.12786
Engler, R., Randin, C. F., Thuiller, W., Dullinger, S., Zimmermann, N. E., Araújo, M. B., et al. (2011). 21st century climate change threatens mountain flora unequally across Europe. Glob. Chang. Biol. 17, 2330–2341. doi: 10.1111/j.1365-2486.2010.02393.x
Falster, D. S. (2006). Sapling strength and safety: the importance of wood density in tropical forests. New Phytol. 171, 237–239. doi: 10.1111/j.1469-8137.2006.01809.x
Fauset, S., Freitas, H. C., Galbraith, D. R., Sullivan, M. J. P., Aidar, M. P. M., Joly, C. A., et al. (2018). Differences in leaf thermoregulation and water use strategies between three co-occurring Atlantic forest tree species. Plant Cell Environ. 41, 1618–1631. doi: 10.1111/pce.13208
Gotelli, N. J. (2000). Null model analysis of species co-occurrence patterns. Ecology 81, 2606–2621. doi: 10.2307/177478
Han, J., Shen, Z., Shi, S., and Peng, P. (2016). Comparison of plant species diversity and composition in the dry valleys of Yalong River and Dadu River: evaluating the effects of climate, topography and space. Biodivers. Sci. 24, 421–430. doi: 10.17520/biods.2015300
Hartmann, H. (2011). Will a 385 million year-struggle for light become a struggle for water and for carbon? – How trees may cope with more frequent climate change-type drought events. Glob. Chang. Biol. 17, 642–655. doi: 10.1111/j.1365-2486.2010.02248.x
He, D., Wu, S., Peng, H., Yang, Z., Ou, X., and Cui, B. (2005). A study of ecosystem changes in longitudinal range-gorge region and transboundary eco-security in southwest china. Adv. Earth Sci. 20, 338–344.
Homeier, J., Breckle, S.-W., Guenter, S., Rollenbeck, R. T., and Leuschner, C. (2010). Tree diversity, forest structure and productivity along altitudinal and topographical gradients in a species-rich Ecuadorian Montane rain forest. Biotropica 42, 140–148. doi: 10.1111/j.1744-7429.2009.00547.x
Hubbell, S. P. (2001). The Unified Neutral Theory of Biodiversity. Princeton: Princeton University Press. doi: 10.1515/9781400837526
Jin, Z., and Ou, X. (2000). The Vegetation of Dry Hot Valley of Yuanjiang River, Nujiang River, Jinsha River, Lancang River. Kunming: Yunnan Science and Technology Press.
Kim, E., and Donohue, K. (2013). Local adaptation and plasticity of Erysimum capitatum to altitude: its implications for responses to climate change. J. Ecol. 101, 796–805. doi: 10.1111/1365-2745.12077
Kluge, J., and Kessler, M. (2011). Phylogenetic diversity, trait diversity and niches: species assembly of ferns along a tropical elevational gradient. J. Biogeogr. 38, 394–405. doi: 10.1111/j.1365-2699.2010.02433.x
Körner, C. (1998). A re-assessment of high elevation treeline positions and their explanation. Oecologia 115, 445–459. doi: 10.1007/s004420050540
Kraft, N. J. B., and Ackerly, D. D. (2010). Functional trait and phylogenetic tests of community assembly across spatial scales in an Amazonian forest. Ecol. Monogr. 80, 401–422. doi: 10.1890/09-1672.1
Kraft, N. J. B., Comita, L. S., Chase, J. M., Sanders, N. J., Swenson, N. G., Crist, T. O., et al. (2011). Disentangling the drivers of beta diversity along latitudinal and elevational gradients. Science 333, 1755–1758. doi: 10.1126/science.1208584
Lawes, M. J., Adie, H., Russell-Smith, J., Murphy, B., and Midgley, J. J. (2011). How do small savanna trees avoid stem mortality by fire? The roles of stem diameter, height and bark thickness. Ecosphere 2, 1–13. doi: 10.1890/Es10-00204.1
Li, J. J. (1999). Studies on the geomorphological evolution of Qinghai-Xizang (Tibetan) Plateau and Asian monsoon. Mar. Geol. Quat. Geol. 19, 1–11.
Li, Z. X., He, Y. Q., Wang, C. F., Wang, X. F., Xin, H., Zhang, W., et al. (2011). Spatial and temporal trends of temperature and precipitation during 1960-2008 at the Hengduan Mountains, China. Quat. Int. 236, 127–142. doi: 10.1016/j.quaint.2010.05.017
Liu, L., Yu, Y., and Zhang, J. (1985). Discussion upon the regularities of vegetational distribution in the Hengduan Mountains. Acta Bot. Yunnanica 7, 323–335.
Liu, X., Swenson, N. G., Zhang, J., and Ma, K. (2013). The environment and space, not phylogeny, determine trait dispersion in a subtropical forest. Funct. Ecol. 27, 264–272. doi: 10.1111/1365-2435.12018
Liu, X., Zhou, G., Wang, X., Wang, H., and Gu, Z. (2011). Studies on drought stress of two kinds of Selaginella P. Beauv. plants. North Hort. 5, 102–105.
Liu, Y., Li, P., Xu, Y., Shi, S., Ying, L., Zhang, W., et al. (2016). Quantitative classification and ordination for plant communities in dry valleys of Southwest China. Biodivers. Sci. 24, 378–388. doi: 10.17520/biods.2015241
Luo, Y.-H., Cadotte, M. W., Burgess, K. S., Liu, J., Tan, S.-L., Xu, K., et al. (2019). Forest community assembly is driven by different strata dependent mechanisms along an elevational gradient. J. Biogeogr. 46, 2174–2187. doi: 10.1111/jbi.13669
Luo, Y.-H., Liu, J., Tan, S.-L., Cadotte, M. W., Wang, Y.-H., Xu, K., et al. (2016). Trait-based community assembly along an elevational gradient in subalpine forests: quantifying the roles of environmental factors in inter- and intraspecific variability. PLoS One 11:e0155749. doi: 10.1371/journal.pone.0155749
Markesteijn, L., and Poorter, L. (2009). Seedling root morphology and biomass allocation of 62 tropical tree species in relation to drought- and shade-tolerance. J. Ecol. 97, 311–325. doi: 10.1111/j.1365-2745.2008.01466.x
Michaletz, S. T., Weiser, M. D., McDowell, N. G., Zhou, J. Z., Kaspari, M., Helliker, B. R., et al. (2016). The energetic and carbon economic origins of leaf thermoregulation. Nat. Plants 2:16129. doi: 10.1038/Nplants.2016.147
Mittermeier, R. A., Robles, G. P., Hoffmann, M., Pilgrim, J., Brooks, T., Mittermeier, C. G., et al. (2004). Hotspots Revisited: Earth’s Biologically Richest and Most Endangered Ecoregions. Mexico City: CEMEX.
Moles, A. T., Warton, D. I., Warman, L., Swenson, N. G., Laffan, S. W., Zanne, A. E., et al. (2009). Global patterns in plant height. J. Ecol. 97, 923–932. doi: 10.1111/j.1365-2745.2009.01526.x
Molina-Venegas, R., Aparicio, A., Lavergne, S., and Arroyo, J. (2016). How soil and elevation shape local plant biodiversity in a Mediterranean hotspot. Biodivers. Conserv. 25, 1133–1149. doi: 10.1007/s10531-016-1113-y
Montano-Centellas, F. A., McCain, C., and Loiselle, B. A. (2020). Using functional and phylogenetic diversity to infer avian community assembly along elevational gradients. Glob. Ecol. Biogeogr. 29, 232–245. doi: 10.1111/geb.13021
Niinemets, U. (2001). Global-scale climatic controls of leaf dry mass per area, density, and thickness in trees and shrubs. Ecology 82, 453–469. doi: 10.2307/2679872
Niu, Y., Chen, Z., Stevens, M., and Sun, H. (2017). Divergence in cryptic leaf color provides local camouflage in an alpine plant. Proc. Biol. Sci. 284:20171654. doi: 10.1098/rspb.2017.1654
Peng, D. L., Zhang, Z. Q., Xu, B., Li, Z., and Sun, H. (2012). Patterns of flower morphology and sexual systems in the subnival belt of the Hengduan Mountains, SW China. Alpine Bot. 122, 65–73.
Petchey, O. L., and Gaston, K. J. (2002). Functional diversity (FD), species richness and community composition. Ecol. Lett. 5, 402–411. doi: 10.1046/j.1461-0248.2002.00339.x
Poorter, L., and Markesteijn, L. (2008). Seedling traits determine drought tolerance of tropical tree species. Biotropica 40, 321–331. doi: 10.1111/j.1744-7429.2007.00380.x
Preston, K. A., Cornwell, W. K., and DeNoyer, J. L. (2006). Wood density and vessel traits as distinct correlates of ecological strategy in 51 California coast range angiosperms. New Phytol. 170, 807–818. doi: 10.1111/j.1469-8137.2006.01712.x
Proches, S., Wilson, J. R. U., and Cowling, R. M. (2006). How much evolutionary history in a 10x10 m plot? Proc. R. Soc. B-Biol. Sci. 273, 1143–1148. doi: 10.1098/rspb.2005.3427
Purschke, O., Schmid, B. C., Sykes, M. T., Poschlod, P., Michalaski, S. G., Durka, W., et al. (2013). Contrasting changes in taxonomic, phylogenetic and functional diversity during a long-term succession: insights into assembly processes. J. Ecol. 101, 857–866. doi: 10.1111/1365-2745.12098
Rahbek, C. (1995). The elevational gradient of species richness: a uniform pattern? Ecography 18, 200–205.
Rahbek, C., Borregaard, M. K., Colwell, R. K., Dalsgaard, B., Holt, B. G., Morueta-Holme, N., et al. (2019). Humboldt’s enigma: what causes global patterns of mountain biodiversity? Science 365, 1108–1113. doi: 10.1126/science.aax0149
Rana, H. K., Luo, D., Rana, S. K., and Sun, H. (2020). Geological and climatic factors affect the population genetic connectivity in Mirabilis himalaica (Nyctaginaceae): insight from phylogeography and dispersal corridors in the Himalaya-Hengduan biodiversity hotspot. Front. Plant Sci. 10:1721. doi: 10.3389/fpls.2019.01721
Reich, P. B. (2014). The world-wide ‘fast-slow’ plant economics spectrum: a traits manifesto. J. Ecol. 102, 275–301. doi: 10.1111/1365-2745.12211
Reich, P. B., Walters, M. B., and Ellsworth, D. S. (1992). Leaf life-span in relation to leaf, plant, and stand characteristics among diverse ecosystems. Ecol. Monogr. 62, 365–392. doi: 10.2307/2937116
Rosell, J. A. (2016). Bark thickness across the angiosperms: more than just fire. New Phytol. 211, 90–102. doi: 10.1111/nph.13889
Rosell, J. A., Olson, M. E., Anfodillo, T., and Martinez-Mendez, N. (2017). Exploring the bark thickness-stem diameter relationship: clues from lianas, successive cambia, monocots and gymnosperms. New Phytol. 215, 569–581. doi: 10.1111/nph.14628
Schoeb, C., Butterfield, B. J., and Pugnaire, F. I. (2012). Foundation species influence trait-based community assembly. New Phytol. 196, 824–834. doi: 10.1111/j.1469-8137.2012.04306.x
Shen, Z., Hu, H., Zhou, Y., and Fang, J. (2004). Altitudinal patterns of plant species diversity on the southern slope of Mt. Shennongjia, Hubei, China. Biodivers. Sci. 12, 99–107.
Shen, Z., Zhang, Z., Hu, J., Han, J., Yang, J., and Ying, L. (2016). Protection and utilization of plant biodiversity resources in dry valleys of Southwest China. Biodivers. Sci. 24, 475–488. doi: 10.17520/biods.2016022
Shi, W., Wang, Y.-Q., Xiang, W.-S., Li, X.-K., and Cao, K. F. (2021). Environmental filtering and dispersal limitation jointly shaped the taxonomic and phylogenetic beta diversity of natural forests in southern China. Ecol. Evol. 11, 8783–8794. doi: 10.1002/ece3.7711
Shields, L. M. (1950). Leaf xeromorphy as related to physiological and structural influences. Bot. Rev. 16, 399–447. doi: 10.1007/bf02869988
Siefert, A., Ravenscroft, C., Weiser, M. D., and Swenson, N. G. (2013). Functional beta-diversity patterns reveal deterministic community assembly processes in eastern North American trees. Glob. Ecol. Biogeogr. 22, 682–691. doi: 10.1111/geb.12030
Simova, I., Rueda, M., and Hawkins, B. A. (2017). Stress from cold and drought as drivers of functional trait spectra in North American angiosperm tree assemblages. Ecol. Evol. 7, 7548–7559. doi: 10.1002/ece3.3297
Sundqvist, M. K., Sanders, N. J., and Wardle, D. A. (2013). Community and ecosystem responses to elevational gradients: processes, mechanisms, and insights for global change. Ann. Rev. Ecol. Evol. Syst. 44, 261–280. doi: 10.1146/annurev-ecolsys-110512-135750
Swenson, N. G., and Enquist, B. J. (2009). Opposing assembly mechanisms in a Neotropical dry forest: implications for phylogenetic and functional community ecology. Ecology 90, 2161–2170. doi: 10.1890/08-1025.1
Swenson, N. G., Enquist, B. J., Thompson, J., and Zimmerman, J. K. (2007). The influence of spatial and size scale on phylogenetic relatedness in tropical forest communities. Ecology 88, 1770–1780. doi: 10.1890/06-1499.1
Thorne, R. F. (1999). Eastern Asia as a living museum for archaic angiosperms and other seed plants. Taiwania 44, 413–422.
Vellend, M. (2010). Conceptual synthesis in community ecology. Q. Rev. Biol. 85, 183–206. doi: 10.1086/652373
Wang, Z., Tang, Z., and Fang, J. (2007). Altitudinal patterns of seed plant richness in the Gaoligong Mountains, south-east Tibet, China. Divers. Distrib. 13, 845–854. doi: 10.1111/j.1472-4642.2007.00335.x
Wang, Z.-W., Zhang, T.-C., Luo, D., Sun, W.-G., and Sun, H. (2020). Phylogeography of Excoecaria acerifolia (Euphorbiaceae) suggests combined effects of historical drainage reorganization events and climatic changes on riparian plants in the Sino–Himalayan region. Bot. J. Linnean Soc. 192, 350–368.
Webb, C. O., Ackerly, D. D., McPeek, M. A., and Donoghue, M. J. (2002). Phylogenies and community ecology. Ann. Rev. Ecol. Syst. 33, 475–505. doi: 10.3417/2009136
Webb, C. O., Ackerly, D. D., and Kembel, S. W. (2008). Phylocom: software for the analysis of phylogenetic community structure and trait evolution. Bioinformatics 24, 2098–2100. doi: 10.1093/bioinformatics/btn358
Wennekes, P. L., Rosindell, J., and Etienne, R. S. (2012). The neutral-niche debate: a philosophical perspective. Acta Biotheor. 60, 257–271. doi: 10.1007/s10441-012-9144-6
Worthy, S. J., Jimenez Paz, R. A., Perez, A. J., Reynolds, A., Cruse-Sanders, J., Valencia, R., et al. (2019). Distribution and community assembly of trees along an Andean elevational gradient. Plants (Basel) 8:326. doi: 10.3390/plants8090326
Wu, Y., Colwell, R. K., Han, N., Zhang, R., Wang, W., Quan, Q., et al. (2014). Understanding historical and current patterns of species richness of babblers along a 5000-m subtropical elevational gradient. Glob. Ecol. Biogeogr. 23, 1167–1176. doi: 10.1111/geb.12197
Xu, J. S., Chen, Y., Zhang, L., Chai, Y., Wang, M., Guo, Y., et al. (2017). Using phylogeny and functional traits for assessing community assembly along environmental gradients: a deterministic process driven by elevation. Ecol. Evol. 7, 5056–5069. doi: 10.1002/ece3.3068
Xu, Z., Tao, G., Yu, P., and Wang, Y. (1985). An approach to the vegetational changes from dry-hot valley of Yunan in the last 500 years. Acta Bot. Yunnanica 7, 403–411.
Yand, Z., Su, J., Luo, D., Li, Z., and Chen, X. (2007). Progress and perspectives on vegetation restoration in the dry-hot valley. For. Res. 20, 563–568.
Yang, Q. (2007). Study on the arid valley scrubs in the upper reaches of Minjiang River. J. Mountain Sci. 25, 1–32.
Yang, Y., Niu, Y., Cavieres, L. A., and Sun, H. (2010). Positive associations between the cushion plant Arenaria polytrichoides (Caryophyllaceae) and other alpine plant species increase with altitude in the Sino- Himalayas. J. Veg. Sci. 21, 1048–1057.
Yang, Y., Shen, Z. H., Han, J., and Ciren, Z. Y. (2016). Plant diversity along the eastern and western slopes of Baima Snow Mountain, China. Forests 7:89. doi: 10.3390/f7040089
Zanne, A. E., Tank, D. C., Cornwell, W. K., Eastman, J. M., Smith, S. A., FitzJohn, R. G., et al. (2014). Three keys to the radiation of angiosperms into freezing environments. Nature 506, 89–92. doi: 10.1038/nature12872
Zhang, R., Zheng, D., Yang, Q., and Liu, Y. (eds) (1997). Physical Geography of Hengduan Mountains. Beijing: Science Press.
Zhang, Y. G. (1998). Several issues concerning vertical climate of the Hengduan Mountains. Resour. Sci. 20, 12–19.
Zhao, F., Zhang, B., Zhang, S., Qi, W., He, W., Wang, J., et al. (2015). Contribution of mass elevation effect to the altitudinal distribution of global treelines. J. Mountain Sci. 12, 289–297. doi: 10.1007/s11629-014-3223-x
Keywords: community assembly, elevation, phylogeny, plant functional traits, species diversity
Citation: Zhao F, Yang T, Luo C, Rao W, Yang G, Li G and Shen Z (2022) Comparing Elevational Patterns of Taxonomic, Phylogenetic, and Functional Diversity of Woody Plants Reveal the Asymmetry of Community Assembly Mechanisms on a Mountain in the Hengduan Mountains Region. Front. Ecol. Evol. 10:869258. doi: 10.3389/fevo.2022.869258
Received: 04 February 2022; Accepted: 24 March 2022;
Published: 15 April 2022.
Edited by:
Manuel B. Morales, Autonomous University of Madrid, SpainReviewed by:
Gang Feng, Inner Mongolia University, ChinaCopyright © 2022 Zhao, Yang, Luo, Rao, Yang, Li and Shen. This is an open-access article distributed under the terms of the Creative Commons Attribution License (CC BY). The use, distribution or reproduction in other forums is permitted, provided the original author(s) and the copyright owner(s) are credited and that the original publication in this journal is cited, in accordance with accepted academic practice. No use, distribution or reproduction is permitted which does not comply with these terms.
*Correspondence: Guo Li, bGlndW9AY3JhZXMub3JnLmNu; Zehao Shen, c2h6aEB1cmJhbi5wa3UuZWR1LmNu
†These authors have contributed equally to this work
Disclaimer: All claims expressed in this article are solely those of the authors and do not necessarily represent those of their affiliated organizations, or those of the publisher, the editors and the reviewers. Any product that may be evaluated in this article or claim that may be made by its manufacturer is not guaranteed or endorsed by the publisher.
Research integrity at Frontiers
Learn more about the work of our research integrity team to safeguard the quality of each article we publish.