- MesoAmerican Research Center, Institute of Social, Behavioral and Economic Research, University of California, Santa Barbara, Santa Barbara, CA, United States
Human expansion into and occupation of the New World coincided with the great transition from the Pleistocene to the Holocene epoch, yet questions remain about how we detect human presence in the paleoecological record. In the Maya area of southern Mesoamerica, archeological evidence of the human imprint is largely invisible until ∼4,000 years ago. How do environmental changes after that time correspond and relate to human impacts? Are the archeological signatures of initial settlements in the Early Preclassic detected? Later, by ∼2,000 years ago when the Maya had fully settled the landscape, how does the evidence of forest compositional changes relate to human intervention? This paper evaluates published paleoecological data in light of the rise of the Maya civilization and reflects on interpretations of how swidden agriculture and the milpa cycle impacted the environment. Evaluating the contrast between the long archeological sequence of successful Maya development and paleoecological interpretations of destructive human-induced environmental impacts requires a concordance among pollen data, archeological evidence, ethnohistoric observations, ethnological studies of traditional Maya land use, and the historical ecology of the Maya forest today.
Introduction
Human groups entered the Americas at the threshold of the Holocene and quickly spread from the Arctic Circle to Tierre del Fuego, with pioneering populations adapting not only to new landscapes but also to the changing climatic regime after the Ice Age. The southern lowlands of Mesoamerica, home to the tropical Maya forest today, was cool and arid 10,000 years ago, presenting a temperate, dry climate to early hunter-gatherer groups. Within 2000 years, during the period known as the Holocene Thermal Maximum, the lowlands transformed to the warm and wet tropical forest contemporary visitors might recognize. Mobile populations were already manipulating the landscape, as evidenced by Archaic-period plant domestication. Within this predictably warm and wet climate regime, humans expanded their involvement with Mesoamerican vegetation, using multiple parts of many types of plants (Fedick and Santiago, 2022). As mobile horticulturalists, they likely employed open gaps in the forest for food production and used other natural resources to their advantage. This set the stage for Mesoamerican agricultural settlements that followed the worldwide climatic changes after 4,000 years ago. The rise of Maya civilization was a consequence of these later developments, which increased the imprint of people on the Maya forest landscape. Herein lies the source of debate over the relationship the Maya had with their forest environment.
Ancient Maya settlement expansion after 4,000 BP certainly impacted the landscape, but questions about the nature and scale of these impacts remain. Despite arguments that the Maya Lowlands were abandoned after the Classic period (c. 1,000 CE), ethnohistoric accounts show otherwise (Jones, 1998). In mining the ethnohistorical sources, Jones shows that there were long standing tensions and movements among resident populations in the greater Petén in preconquest times. Cortés (1971 [1525]), for example, used maps and resided in homes during his traverse across the “forested terrain” of the Central Lowlands in 1525 CE; he never mentioned issues finding housing or food for his retinue, which included 3,000 Mexica.
Current research on Mayan languages links the occupants of the region today to Landa’s observations (Landa, 1990) and from there to ancient Maya hieroglyphic texts (Macri and Ford, 1997; Houston et al., 2000). This continuity in language suggests continuity in land-use strategies (Terán and Rasmussen, 1995) and the botanical knowledge documented in ethnohistoric accounts (Roys, 1931; Steggerda, 1943). Maya knowledge of forest plants is well recognized, and recent research has shown that nearly 500 plants of the area are used in food preparations (Fedick, 2020). Moreover, most plants of the Maya forest, as registered by economic botanists (Campbell et al., 2006; Ross, 2011; see also Roys, 1931), are of significant economic use and value, and the dominant plants of the forest are noteworthy for the continuing role they play in the world economy (Schwartz, 1990; Mathews, 2019; Machuca et al., 2020). The economic significance inherent in these plants, and the Traditional Ecological Knowledge (TEK) that perpetuates their many and varied uses, indicates the Maya forest is a legacy of ancient Maya land-use and management practices (see Wilken, 1971, 1987; cf. Foster et al., 2003; Oswald et al., 2020).
This paper reflects on published paleoecological data and its generalized interpretation in the wider literature on the ancient Maya. The review is cast in light of the traditional Maya milpa-forest garden cycle as described at the time of conquest, identified in ethnohistories, and detailed in ethnologies that began in the 20th century. To accomplish this goal, I first provide an overview of contemporary Maya land-use systems, emphasizing the integral nature of the milpa cycle with respect to forest regeneration. This provides a framework for evaluating the chronology of Maya civilization and the paleoecological data. Through the lens of milpa forest garden practices, I address implications for pollen, rainfall, management of water and erosion, and the importance of maintaining soil fertility (Handelsman, 2021).
The importance of traditional Maya land-use systems is considered before discussion of the archeological record. The archeological chronology of the Maya highlights the successful developments that supported growing populations and expanding cities. The growing archeological data on plant materials demonstrate that natural resources in the ancient Maya forest are comparable to those used by the Maya today (Thompson et al., 2015; Morell-Hart et al., Forthcoming). The agricultural land-use system practiced by traditional small-holder Maya can be identified in ancient settlement patterns and in descriptions of Postclassic encounters with Spanish invaders (Ford et al., 2021).
Finally, I evaluate the paleoecological data on pollen, precipitation, and droughts. This critical examination considers the disparity between generalized paleoecological interpretations and specific data in the context of the milpa cycle, expectations of the interplay among people, land-use practices, plants and habitat distribution, and scales of precipitation. The combined paleoecological evidence provides a baseline for interpreting environmental interactions that must square with the archeological chronology. Taking these components together, I consider the co-creative processes that emerged with the interactions of cultural provisioning —the biological capital of the Maya forest— that could have derived from forest gardens.
Traditional agroecology of the Maya forest
Geography
Appreciation of the Maya area begins with an understanding of the landscape (West, 1964; White and Hood, 2004). The geological foundation of the Maya forest is a karst limestone platform that influences the spatial distribution of all resources. Local variations in limestone are expressed in drainage features and seasonal water distribution (Beach et al., 2009). The porous limestone absorbs rain, and local precipitation averages vary from 500 mm in the dry northwest Yucatan Peninsula to 4,000 mm in the far south. In the central area, where the majestic ancient cities of Tikal and El Pilar stand (Figure 1), rainfall ranges from 1,500 to 2,000 mm a year (West, 1964; White and Hood, 2004), with rainwater seeping down to form subterranean flows beneath the bedrock that determine access to surface water on the surface (Scarborough, 1993; Ford, 1996; Lucero, 2003; Ferrand et al., 2012; Šprajc et al., 2021). Water drains from the rocky hills, ridges, and escarpments to collect in scattered depressions, with an estimated 40% of the region comprising wetlands (Fedick and Ford, 1990; Schwartz, 1990; Dunning et al., 2002, 2020; Ford and Nigh, 2015). These variable environments provide the vital resources that influence the locations of ancient and modern settlements in the Maya lowlands (Beach, 1998; Dunning et al., 1998, 2009, 2012; Beach et al., 2002, 2006, 2018b; Ferrand et al., 2012; Liendo et al., 2014). Land cover over the limestone base depends on local climate, rainfall, and slope conditions, with upland forests replete with magnificent towering trees that thrive in the fertile shallow soils and are cultivated for a range of economic uses (Reina, 1984).
The milpa cycle
Understanding the traditional milpa system lies at the crux of debates about past and present Maya agriculture. Few Maya scholars recognize the effective role of fire in management (Hernández Xolocotzi et al., 1995; Eastmond and Faust, 2006; Nigh, 2008; Nigh and Diemont, 2013; see also Peters, 2000; Anderson, 2005; Abrams and Nowacki, 2020; Jurskis et al., 2020) or comprehend the systems’ integral role in shaping the landscape (see Conklin, 1957; Dove, 1983, 1993; Van Vliet et al., 2013), even as they negatively cast the milpa cycle as extensive “slash-and-burn” agriculture. The milpa system is based on a hand cultivated poly-cropping scheme that intimately manages microhabitats for water, drainage, erosion, and soil fertility while selecting and directing perennial succession processes (Chazdon, 2014; Ford and Nigh, 2015; see Robinson and McKillop, 2013; Lentz et al., 2021). Archeologists assumed that this system failed to produce sufficient food for growing populations in the ancient past and the intensification of this subsistence mode necessarily led to deforestation. The agroecological evidence for the Maya milpa-forest garden system tells an alternative story (Schwartz and Márquez, 2015).
Environmental and agroecological scholars point to the value of traditional agricultural practices and their relationships to the landscape (Barrera Vásquez et al., 1977; Gómez-Pompa and Kaus, 1990, 1999; Gómez-Pompa and Del Amo, 1994; Atran, 2003; Altieri and Toledo, 2011). These studies show how the Maya milpa system, with cyclic and strategic dry-season burns every ∼20 years (Hernández Xolocotzi et al., 1995; Faust, 1998, 2001; Terán et al., 1998; Ford and Nigh, 2014), is adaptive in the tropical forest ecosystem by reducing fuel loads and regenerating forests (see Altieri, 2002). Intensification is based on scheduled, skilled, and knowledgeable investments of manual labor with tools appropriate to the woodland landscape, not the plow and draft animals employed by European farmers.
In the context of Maya geography, contemporary traditional farming practices are distinct from so-called conventional agricultural practices (Sumberg and Giller, 2022). Modern conventional farming, developed with Western goals of uniformity to maximize profits, is based on monocropping arable land and cleared cattle pasture, with high external inputs to maintain uniform yields that rely on technology and labor efficiencies (Bray, 1994; Gold, 1999; Scarborough, 2003; Smith, 2005; Montgomery, 2007). Traditional farming developed to minimize risk on the basis of local human-environment relations, balancing family needs with local labor, skill, and scheduling (Dumond, 1961; Boserup, 1965; Reina, 1967; Netting, 1977, 1993; Stone and Downum, 1999; Schreinemachers and Berger, 2011).
Agroecologists, economic botanists, and evolutionary psychologists who work with traditional Maya agriculturalists and the plants of the Maya forest identify the milpa subsistence system as highly integrated with the environment (Nations and Nigh, 1980; Gliessman, 1982, 1992, 1998; Gómez-Pompa and Bainbridge, 1993; Terán and Rasmussen, 1994; Quintana-Ascencio et al., 1996; Atran, 1999, 2000; De Clerck and Negreros-Castillo, 2000; Anderson, 2003; Gómez-Pompa et al., 2003; Levy Tacher and Rivera, 2005; Campbell et al., 2006; Nigh, 2006, 2008; Campbell, 2007; Corzo Márquez and Schwartz, 2008; Ford, 2008; Ross, 2011; among many others). The milpa-forest garden cycle today has continuity with the past. Records from the conquest and colonial times show practices comparable with the present (Terán and Rasmussen, 1995; Graham, 2006; Altieri et al., 2012; Ford and Clarke, 2019; Sánchez-Suárez et al., 2021). This system, developed from ancient adaptations and historical practices (Roys, 1952; Farriss, 1984; Terán and Rasmussen, 1995; Alexander, 2006; Zetina Gutiérrez, 2007; Zetina Gutiérrez and Faust, 2011; Rushton et al., 2020; Slotten et al., 2020), presents an effective subsistence adaptation for ancient Maya land use.
The landscape created by the milpa cycle embraces infield home gardens and diverse, accessible outfields interspersed among secondary growth and mature, closed canopy forests. The field-to-forest cycle, described for the ethnohistoric and contemporary Maya (Roys, 1931; Villa Rojas, 1945; Hernández Xolocotzi et al., 1995; Zetina Gutiérrez, 2007; Cook, 2016; Evans et al., 2021; Ford et al., 2021), creates this patchwork and demonstrates how the mosaic landscape provides resources to fulfill daily requisites of food, condiments, fiber, oils, fuel, gum, furnishings, supplies, medicine, toys, construction materials for buildings, household utensils for cooking, spinning, baskets, and habitat for animals; in short, all the everyday household necessities (Fedick, 1996). If these resource strategies can be projected back in time (Morell-Hart et al., Forthcoming), the entire landscape, with soil characteristics, geological assets, and animal habitats, was part of environmental interactions undertaken to meet common human needs.
The human environmental imprint relates to permanent residential units within an asynchronous forest-to-field mosaic. This mosaic would hinge on complex infield home gardens covering less than one-half hectare (Netting, 1974; Fedick, 1992; Fisher, 2014), with approximately 70% of the infields in shaded orchards and 30% in sunny fields (Ford and Nigh, 2015). The legacy of this system is evident in the widely acknowledged biodiverse Maya forest today (Primack et al., 1998; Mittermeier et al., 2000; TNC, 2021). Use of the open field, like a forest gap (Hartshorn, 1989), initiates the cycle of regeneration from which the perennial forest products are nurtured, selected, planted, and grown (Ford, 2008; Lentz et al., 2014, 2018). Outfields are interspersed within the regenerating succession and mature forests, ensuring sufficient variety in land cover across the landscape, providing habitat for animals, construction materials for housing, as well as fruits and other useful perennial resources from the dominant plants of the Maya forest (Table 1).
Rather than creating a patchwork of shifting slash and burn maize fields and abandoned plots left to “fallow,” the diverse land use strategies of the contemporary Maya produce a varied horizontal and vertical vegetation landscape (Ford, 2020). The horizontal landscape mosaic includes fields, successional perennials, and mature canopy, while a variety of vertical growth habits exist in home gardens and in milpa fields. The inclusion of perennial seedlings and resprouts in the milpa hastens the regeneration of the plot when it transitions toward forest. Selection for utility as well as diversity keeps the milpa cycle viable in the long-term. The sun loving field crops give way to first low and then high shady forest over time. This land-use system disperses annuals and perennials, aiding in water conservation, building soil organics, and inhibiting erosion. Agricultural extension officers in Belize and Guatemala observe that erosion is regularly witnessed in plowed fields and rarely noted in milpa fields (Tzul, 2018, personal communication).
Vanguard trees and shrubs gain ascendency after 4 years of sharing the milpa field with forbs1 and grasses. It is the natural regeneration cycle, not neglect, that encourages the prevailing annuals to give way to perennials. Far from uncontrolled, and certainly not fallow (defined as an unseeded plowed field), this succession-building phase requires constant attention to direct secondary growth and provide opportunities to improve livelihoods with useful plants and animals (Emery and Thornton, 2008, 2012; Bongers, 2017; Levis et al., 2017). The succession provides a complex space, with sun-seeking annuals retreating around the emerging shade trees; it is a stepping stone in the forest transition where the farmer can influence land cover to meet social and economic needs (Guariguata, 2017). The value of this second growth is only now being realized (Chazdon, 2014).
It is critical to appreciate that all the necessities of ancient Maya life derived from their tropical landscape. Understanding the flexibility of the milpa-forest garden cycle and its importance to living in the forest is fundamental. Agricultural practices developed over millennia, working with natural processes to meet immediate food needs with annual crops and selecting significant perennials for long term requirements (Palerm, 1967, 1976; Wilken, 1971, 1987; Nations and Nigh, 1980; Gómez-Pompa, 1987; Hernández Xolocotzi et al., 1995; Terán and Rasmussen, 1995; Gliessman, 2001; Schwartz and Márquez, 2015; cf. Conklin, 1957). The Maya milpa cycle is an intensive system based on skill and scheduling that maintains biodiversity, lowers temperature, conserves water, checks erosion, and builds soil fertility.
Precipitation
Water, a critical resource for plants and animals, in the Maya forest is distributed unevenly over the year (e.g., Kramer and Hackman, 2021). There are three distinct seasons that impact land use recognized by local farmers: the cool wet, beginning in November-December, called Yaax Pak’al or first planting; the dry season, beginning around March, known as Yaax K’in or first sun; and the warm wet period, beginning in May–June, called Noh Pak’al or principal planting (Bricker, 2017). It is important to understand that this annual precipitation scheme is related to distant influences from the north, south, and east. The Atlantic brings the cool wet period that coincides with winter in North America and is characterized by weather systems known as nortes. The initiation of the warm wet period is dependent on the northward move of the Intertropical Convergence Zone (ITCZ) and the intensity and frequency of the rains depend on the eastern development of storms and hurricanes. In between these distinct wet periods is the dry season that varies in length according to the persistence of nortes and hurricanes.
Deluges as well as droughts, from the farmer’s point of view, are not measured annually. In fact, annual scales miss key information and interactions that impact farmers and farming (Tuxill, 2004; Kramer and Hackman, 2021). While relevant at the regional scale, coarse-grained temporal and spatial precipitation data of annual and even seasonal generalities fail to characterize patterns critical to the Maya farmer (Kramer and Hackman, 2021). Farmers in the Central Lowlands expect the annual cycle to give two maize yields; the largest and most reliable from the May–June planting with its September–October ripening depending on the maize race chosen (Reina, 1984; Tuxill et al., 2010). As insurance, there can be a dry season planting opportunity, depending on observations of precipitation (Reina, 1967). Outfield plots are strategically placed to take advantage of variability in drainage and aspect. The remarkable repertoire of edible plants number nearly 500 (Fedick, 2020), and many of these edibles are drought tolerant, thriving with intermittent or scarce rain fall (Fedick and Santiago, 2022), including specific maize races (Tuxill et al., 2010; Fenzi et al., 2017).
Of course, with rainfall agriculture (Tuxill, 2004; Whitmore and Turner, 2005), rain is required, but too much rain is just as menacing as too little (Lundell, 1978). Bad years are not measured by annual rainfall peaks or troughs, but rather on the timing related to harvest. High rainfall at a time when maize is maturing is devastating yet anticipated from tropical storms and hurricanes (Kramer and Hackman, 2021). Critical to successful crop yields are: (1) the timing of dry periods, when fields are burned; (2) sufficient rain for crops to mature; but (3) not too much rain to flood fields and damage crops. The reality on the ground is that the timing of the rain is much more important than overall rainfall (Tuxill, 2004). Low annual rainfall delivered to meet crop needs is good, while high annual rainfall delivered at the wrong time will destroy crops and lay waste the harvest. Precipitation cannot be measured at a coarse scale.
Land erosion
The nature of the milpa cycle provides for diverse land cover types at any given time. Open fields are rarely larger than a hectare (Schwartz and Márquez, 2015; Kramer and Hackman, 2021) and are interspersed within the many stages of forest regeneration. This variety enriches soil fertility with organic matter and inhibits erosion. Traditional milpa farming by small holders works to minimize risk over the long term, and field systems are monitored closely, designed to conserve water and check soil loss, factors that farmers everywhere are well attuned to (Handelsman, 2021).
For the Maya case, there is controversy over the proxies of instability and erosion that are generally predicated on a view of land cover that pits maize fields against forest. Lands that are found around settlements at centers, like Tikal (Carr and Hazard, 1961), are managed without landesque investment. Managing soil on slopes is important. The use of terraces and drainages are examples where marginal lands are brought into the agricultural regime, yet these systems are rare. Indeed, water travels too fast over steep slopes and still water in basins need drainage for crops. It is obvious that, in local situations, there may examples of erosion (Dunning and Beach, 2000; Carozza et al., 2007; Wahl et al., 2007; Beach et al., 2008, 2018a,b, 2019). These specific pulses identified at base of slopes, wetlands, as well as the occasional uplands, need to be carefully assessed in terms of regional implications. Specifics where erosion is identified need to be carefully assessed in terms of regional implications and alternative explanations.
While situations may vary, we have some idea of worldwide erosion rates for conventional vs. traditional systems (Hooke, 2000; Montgomery, 2007). Rates for preindustrial farming are very low, and rarely result in serious erosion. Montgomery (2007), the gold standard for evaluating erosion, indicates conventional agriculture is much more prone to impact erosion rates than traditional systems. This concords with field observations (Figure 2).
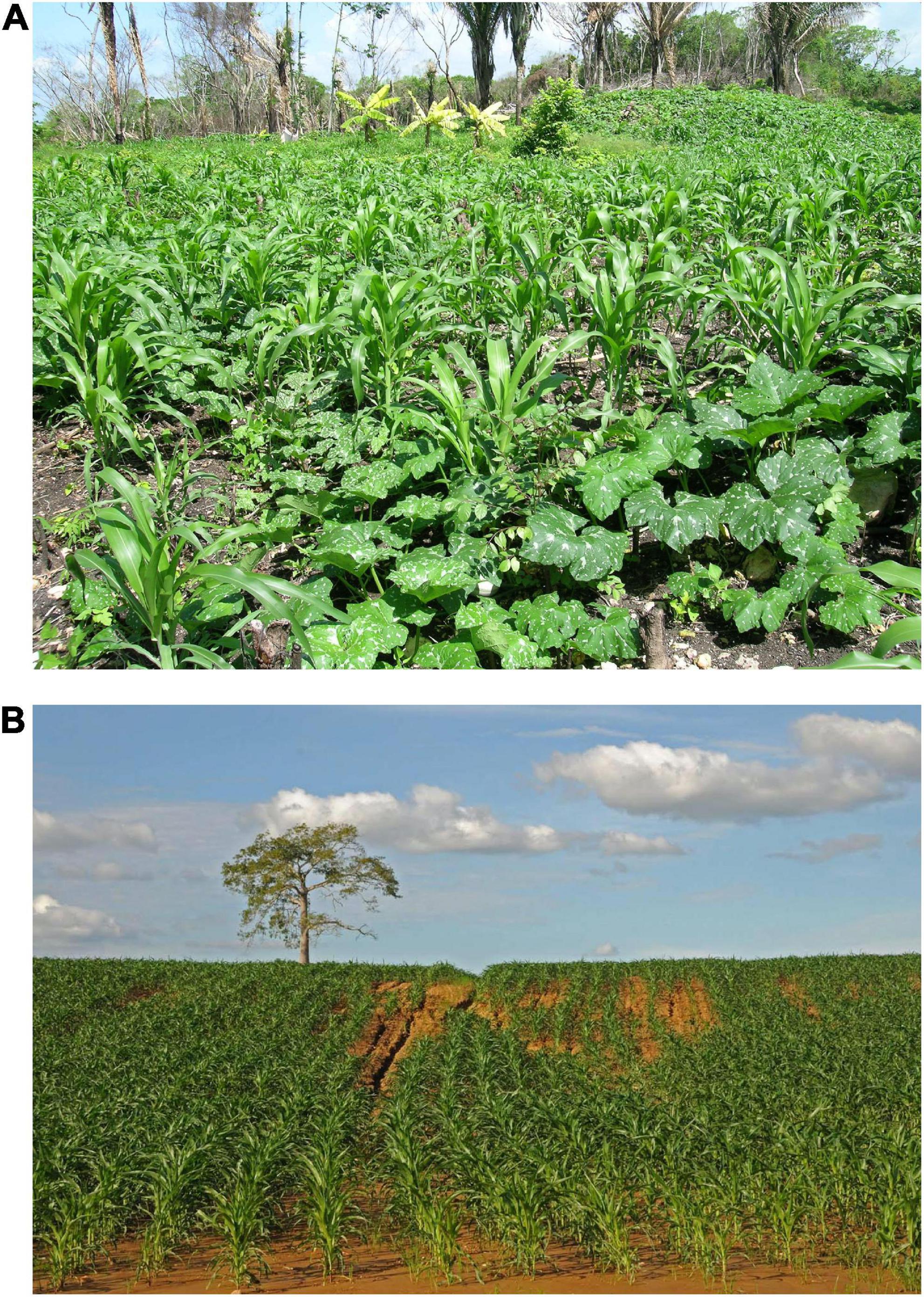
Figure 2. Comparison of fields (A) complex milpa field with little erosion, (B) plowed maize field with significant erosion. Credit ISBER/MesoAmerican Research Center, UCSB.
Overview
Maya farmers, like subsistence farmers throughout the world, maintain diverse home gardens (Caballero, 1992; Campbell, 2007) along with several outfield plots, which leverage different resources and incorporate well-drained and poorly drained lands to hedge against uncertainties of weather and crop yields (Hernández Xolocotzi et al., 1995). The home infield is the base operation; it is the core that is coordinated with multiple outfields. The land use cycles of open field crops, secondary shrub and tree cover, and mature canopy forest gardens are long-term, enhanced resource-regeneration systems that run for a period of about 20 years (Ford and Nigh, 2015). With variability in rainfall and the seasonal cycle of precipitation, farmers today, and certainly in the past, favor established cropping in home infield plots for their most intensive labor investments, while dispersed outfield areas mitigate production challenges that vary with precipitation levels. Areas of emerging and mature perennials provide specific animal habitats and resources, from homes for bees to fruits and construction materials. This system builds food sovereignty for the farmer and security for the family, supporting the redistribution of resources that are required by the local community.
This infield-outfield pattern of land use (Netting, 1989) contributes to a complex, asynchronous landscape mosaic that shifts from forest to field and back again with each family, generation, century, and millennial cycle (Table 2). By working within the natural regenerative system, swidden in general, and the Maya agricultural cycle in particular, is integral to forest maintenance (see Conklin, 1954). As recognized by palynologists, the open and succession phases of the cycle favor wind pollination produced by abundant sun-loving annuals and perennials, which are well represented by forbs in the pollen rain (Islebe et al., 2018; Vela-Pelaez et al., 2018). Investments in long-term perennials of the mature phases of the cycle are evident in the archeobotanical data (Trabanino-Garcia, 2014; Morell-Hart et al., Forthcoming) and are supported by animal pollination, a process identifiable in specific local captures of pollen rain (Wahl et al., 2007; Dunning and Beach, 2010; Bhattacharya et al., 2011; Dunning et al., 2012; Islebe et al., 2015). Built on a basic 20-year field-to-forest system, the Maya milpa-forest garden cycle intervenes in the natural succession process (Chazdon, 2014) to direct the production of the landscape to meet human needs. In forestry terms, the opening for crops is equivalent to the forest gap; this artificially constructed gap provides diverse fields filled with forbs and grasses in a manner similar to natural gaps (Hartshorn, 1989; Fredericksen and Pariona, 2002). Today the evidence of past land use is the feral forest itself, reflecting the legacy of a highly domesticated landscape (Ross, 2011; Thompson et al., 2015; Ford, 2020; Armstrong et al., 2021; cf. Michon et al., 2007; Iriarte et al., 2020).
The Maya chronology: Long, steady, successful cultural development
Occupation of the Maya lowlands of Mesoamerica began with the expansion of mobile foraging societies into the Americas more than 15,000 years ago (Steele et al., 1998; Goebel et al., 2003; Prufer et al., 2021). The archeological evidence for the early Holocene is growing (Neff et al., 2006; Rosenswig, 2006a,b), and data demonstrate that the Maya area was occupied early on (Kennett et al., 2002, 2020, 2022; Lohse, 2010; Lohse et al., 2006), as were most areas in the Americas (Steele et al., 1998; Voorhies, 2004; Kelly and Thomas, 2013). Early populations in the Maya Lowlands inhabited an arid and cool landscape (Steele et al., 1998; Leyden, 2002; Burroughs, 2005; Piperno, 2006, 2011; see also Caffrey et al., 2011) founded on the karstic limestone platform that forms the greater Petén of Guatemala, Belize, and the Yucatan Peninsula of Mexico. While the climate was different from the present, the geography these early inhabitants confronted was essentially the undulating ridges and hills interspersed by flatlands of today.
The early millennia of the Holocene witnessed significant changes in climate, and 8,000 years ago the region transformed to a warm and wet environment with tropical characteristics familiar today: broadleaf forested uplands, seasonally and permanently inundated wetlands, and water bodies represented by lakes in the south and cenotes in the north (Leyden et al., 1993; Leyden, 2002; Carillo-Bastos et al., 2012; see also Haug et al., 2001; Peterson et al., 2001; Hillesheim et al., 2005; Hodell et al., 2008). The region was—and remains—subject to periodic disturbance by hurricanes and volcanic eruptions, and the climate is structured by annual cycles recognized by contemporary local farmers.
As a biodiversity hotspot today (Mittermeier et al., 2000), it is worthwhile to note that past human impacts on the Maya forest likely reduced overall diversity, and one can even imagine anthropogenic extinctions (Braje and Erlandson, 2013; Abrams and Nowacki, 2020; Jurskis et al., 2020). Botanists studying the Maya forest show that it has a lower alpha diversity – the overall diversity of plant life – compared with the Amazon (Campbell et al., 2006). The signature of intensive management at dense ancient Maya settlement areas is evident today in the composition of perennial forest plants, where tall trees and understory shrubs are economically useful (Campbell et al., 2006; Ross, 2011). Interestingly, these zones of dense settlement show significant homogeneity, indicated by high beta diversity, a measure of similarity (Campbell et al., 2006), which contrasts with the low beta diversity of the Amazon. Outfields located in the well-drained and slow-drained areas show similar impact and homogeneity when compared with the Amazon, but lowland and wetland beta diversity is lower than in high-density settlement areas. Maya resource management documented in TEK strategies appears to have influenced the forest and is reflected in this high beta diversity, with human selection revealed in the economic utility of the dominant plants of the Maya forest today. This suggests that the long-term impact of Maya management may have simplified the enduring forest composition.
Time among the Maya
8,000–4,000 BP
Archeological data from the Archaic period is scant but growing (Awe et al., 2021; Prufer et al., 2021; Rosenswig, 2021; Valdez et al., 2021; Wrobel et al., 2021), yet evidence of plant domestication shows inhabitants of the Maya Lowlands were already manipulating flora. Maize, beans, squash, and chile supplemented hunting and gathering (McClung de Tapia, 1992; Betz, 1997; Piperno and Pearsall, 1998; Smith, 1998; Clark and Cheetham, 2002; Blake, 2015; Cagnato, 2021). Little is known of the probably sparse Archaic settlements or seasonal patterns (Voorhies, 1998; Lohse et al., 2006; Stemp and Harrison-Buck, 2019), and archeological remains are largely represented by stone tools (MacNeish, 1982).
4,000–3,000 BP
Major changes are recognized throughout Mesoamerica (Pohl et al., 1996; Vanderwarker, 2006), marked by the widespread emergence of permanent settlements. By 3,000 BP, settlements dominated Mesoamerica (Blake et al., 1992; Clark and Cheetham, 2002), including the Maya area (Puleston and Puleston, 1972; Rice, 1976; Ford, 1986; Fedick, 1989; also Neff et al., 2006). This marks the beginning of the processes that led to the proliferation of permanent structures, the use of pottery, and ultimately Maya civilization (Rosenswig, 2021; Stemp et al., 2021).
3,000–2000 BP
All major upland ridges in the Maya area contain evidence of settlements (Fedick and Ford, 1990). These communities formed the bases for Preclassic Maya cities, such as Nakbe and Mirador (Forsyth, 1989; Pohl et al., 1996; Pope et al., 2001; Clark and Cheetham, 2002; Hansen et al., 2002), and later Tikal and El Pilar (Coe, 1965; Haviland, 1969; Puleston, 1973; Fedick, 1989; Wernecke, 1994, 2005; Ford, 2004; Ford and Horn, 2017; Horn, 2020), as well as others (e.g., Holmul, Cahal Pech, Cuello). Small at the outset, many early centers later became major players in local and regional administrative hierarchies.
2,000–1,400 BP
Classic Maya civilization is marked by the growth of settlements in the Late Preclassic and Early Classic, characterized by increasing social complexity and the emergence of culturally distinctive features, such as the famous Maya hieroglyphs. Settlements expanded in all well-drained areas, revealing a concentration of occupation in the prime agricultural areas that compose about 25–49% of the region (Bullard, 1960; Fedick and Ford, 1990) and evidence of subsistence intensification (Johnston, 2003; Ford and Nigh, 2009; Robin, 2012). Settlements began encroaching on less preferred zones, such as transitional wetland areas, but these are characterized by lower population densities (Ford, 1986; Ford et al., 2009).
1,400–1,100 BP
The Late Classic was the apex of Maya civilization, with major centers focused on the Central Lowlands (Webster, 2002) and an increase of residential settlements (Culbert and Rice, 1990). Civic centers originally built in Preclassic times reached their most extensive size, as exemplified by the enormity of Tikal, which comprised more than 150 hectares of monumental architecture (half the area of all the medieval City of London). Large and dense settlements occupied the well-drained ridges first settled during the Preclassic (Ford, 1986; Robin, 2012; Ford and Nigh, 2015; Canuto and Auld-Thomas, 2021). This was also a time of diversification in ceramics, with the appearance of volcanic ash-tempered pottery prominent among fine wares, and volcanic ashfall is also suggested in reservoirs (Simmons and Brem, 1979; Jones, 1986; Ford and Glicken, 1987; Ford and Rose, 1995; Sunahara, 2003; Ford and Spera, 2007; Tankersley et al., 2011, 2016; Coffey et al., 2014).
While structure counts are straightforward and well-reported (Haviland, 1972; Puleston, 1973; Ford, 1986, 1990, 1991; Chase and Chase, 1987; Rice and Rice, 1990; Healy et al., 2007; Robin, 2012), and are a logical proxy for population (Turner, 1990), population density estimates for the Late Classic are the subject of major debate. High population estimates provide the foundation for interpretations of overpopulation and deforestation. For example, Chase et al. (2011) estimates the population density of regional Caracol at 1,000 persons/sq. km, while Ford and Clarke (2019) suggest regional El Pilar had 140 persons/sq. km. As defined by economic geographers (Boserup, 1981), “dense” populations range from 64 persons/sq. km. for the Ming Dynasty of China in 1500 CE to the “very dense” 128 persons/sq. km recorded in Edo Japan in 1750 CE. Populations only reached 256–512 persons/sq. km in Asia in 1975. In the Maya context, reasoned estimates of 100–200 persons/sq. km (Turner, 1990) are viable and conform to preliminary results for the 1,300 sq. km greater El Pilar area (Ford et al., 2011). Recent visual assessments of Lidar coverage present a more moderated view of settlement (Chase et al., 2011; Canuto et al., 2018) and surveys on the ground corroborate lower settlement densities and greater diversity (Reese-Taylor et al., 2016; Ford and Horn, 2018; Horn et al., 2019; Hutson et al., 2021). Indeed, population estimates are at the center of the debates on land use and environmental impacts.
1,100–1,000 BP
The end of Classic Maya civilization is dated by the last Maya stela erected in the core area of the Maya Lowlands (Sharer and Traxler, 2006). Known as the Terminal Classic, and lasting c. 100–300 years, this period is marked by increasing neglect of monumental infrastructure and has been associated with what has been called a prolonged drought (Hodell et al., 2001; Haug et al., 2003; Peterson and Haug, 2005; Kennett et al., 2012, 2013; Douglas et al., 2015, 2016; Hoggarth et al., 2015; Evans et al., 2018; Lucero and Larmon, 2018; Roman et al., 2018). The current leading candidate provoking this change is overpopulation, resulting in deforestation and soil degradation (summary given by Turner and Sabloff, 2012). The Classic Maya “collapse” has been recognized more recently as an environmental transformation with economic and political disruptions (Demarest, 2004; Lucero et al., 2015; Yaeger, 2020) and a concomitant redistribution of farming populations (Ford and Nigh, 2015).
The main evidence cited in support of environmental collapse hypotheses are generalized interpretations of paleoecological data based on the Petén lake sediment cores (Deevey et al., 1979; Vaughan et al., 1985; Binford et al., 1987). These generalizations are reproduced and emphasized repeatedly to the present (see Rice, 1996; Webster, 2002; Brenner, 2022). These repetitions have propagated and entrenched the position that the Maya overpopulated the region such that they negatively impacted the environment (see Diamond, 2005). Interpretation of these data, however, is ambiguous (Ford and Nigh, 2009; see also Gunn et al., 1995; Fedick, 2010; McNeil et al., 2010; McNeil, 2012). Mounting evidence from macrobotanical remains in archeological contexts demonstrates that the ancient forest landscape was comparable to that found today (Thompson et al., 2015; Dussol et al., 2017; Machuca et al., 2020; Morell-Hart et al., Forthcoming), highlighting the diversity that is latent in the pollen from lake cores.
1,000–500 BP
The Postclassic is a time of political transformation and reorganization following the upheavals that produced dilapidated monumental architecture in city centers across the Central Lowlands. Centers in the old core area gradually fell into disuse as counterparts in the north expanded. During this period, farming populations, unconstrained by taxation and corvee labor, continued living in the tropical woodlands (Fisher, 2020). Suggested as a diaspora (Lucero et al., 2015), there is no reason to suppose farmers left this area with its well-known natural resources. The populace endured social upheavals and changes until faced with the brutal Spanish conquest (Alexander, 2006), which culminated in the ultimate collapse of Maya social organization under the weight of the oppressive colonial regime.
The paleoenvironmental chronology: Pollen, sediments, and precipitation
The Maya paleoenvironmental record derives from several lines of evidence, most prominently analyses of lake core sediments and more recently from studies of speleothems (Deevey et al., 1979; Binford et al., 1987; Jacob, 1995; Webster et al., 2007; Hodell et al., 2012; Douglas et al., 2016; Medina-Elizalde et al., 2016; Akers et al., 2019; Castro-López et al., 2021; Brenner, 2022; James et al., 2022). Vegetation changes across the Holocene (Figure 3) are recorded in the pollen register from these lake cores (Vaughan et al., 1985; Leyden, 2002), which are dominated by wind-borne pollen (Kellman and Tackaberry, 1997; Bradley, 1999). Major and trace elements (e.g., Ti, Fe) are used as a proxy of variations in precipitation, with new data from speleothems adding another source of rainfall data. Together, these data sources provide insight into continuity and change in the Maya forest and must be brought into concordance with ancient Maya chronology and land use.
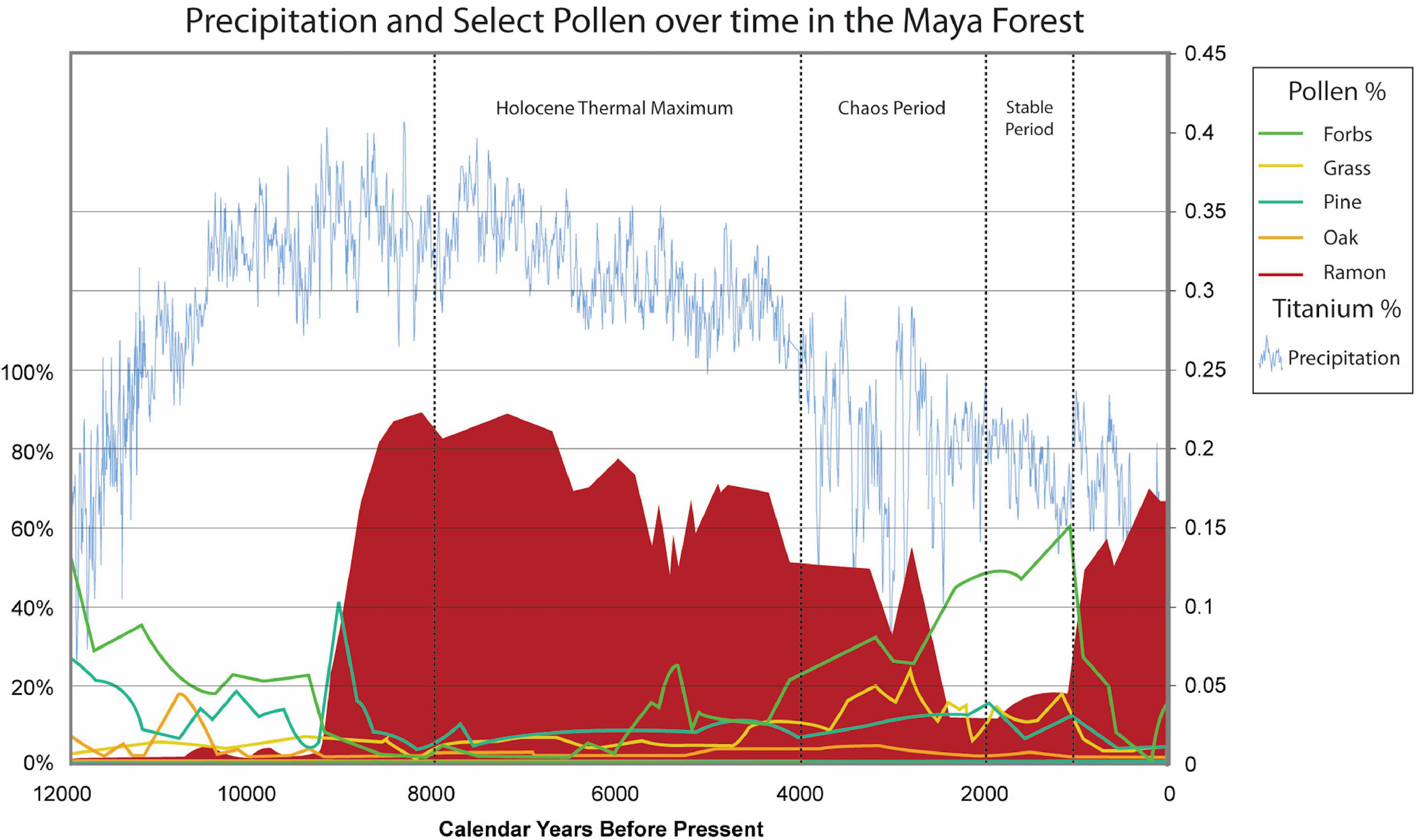
Figure 3. Precipitation proxy and key pollen over 12,000 years in the Maya forest. Pollen data from Curtis et al. (1998), Brenner et al. (2002), and Leyden (2002) with Moracea-type, Brosimum alicastrum/Ramon as the indicator of forest, and the Cariaco Ti precipitation proxy data from Haug et al. (2003). Compiled by ISBER/MesoAmerican Research Center, UCSB.
Lake core sediments from the central Petén reveal differences in the influx of materials called the “Maya clay” (Curtis et al., 1998; Anselmetti et al., 2007; Mueller et al., 2009, 2010; Battistel et al., 2018; Brenner, 2022), which researchers believe correspond to a widespread erosion event. These features may be interpreted in different ways. It is assumed to be allochthonous (Brenner, 2022), yet it could of turbidite origin (Mueller et al., 2010), accounted for by below water movement of materials. The evidence against deforestation is bolstered with the zooarchaeological record (Emery and Thornton, 2008, 2012) and with the archeobotanical record (Thompson et al., 2015; Dussol et al., 2017; Machuca et al., 2020; Morell-Hart et al., Forthcoming) that document the presences of forest habitats throughout the Maya area. It is noteworthy that “Maya clay” is not recognized just to the north in the Yucatan (Islebe et al., 2018).
A good record of past precipitation here is based on a proxy of the concentration and ratios of the minor element titanium (Ti) as recorded in the Cariaco ocean basin north of Venezuela. Other metals in lake sediments (Fe, Al) are used as a proxy indicator of rainfall (Haug et al., 2001; Mueller et al., 2009; Medina-Elizalde et al., 2016; Vela-Pelaez et al., 2018). These proxies are thought to be tracking the summer monsoonal rain associated with the movement of the ITCZ and hurricanes reflected in detailed sediment cores with Ti from the Cariaco Basin (Haug et al., 2001; Battistel et al., 2018; Supplementary Data) and corroborated with Fe of the homogenized data of the Petén Lakes (Hodell et al., 2008; Mueller et al., 2009; Metcalfe et al., 2010).
Another important component of soil is silica, and a known source of silica in the soils of the region—particularly clays—is volcanic ash, the interpretation of which also contributes to paleoenvironmental reconstructions (Harris, 1982). Volcanoes, located on the Mexican Volcanic Belt and the Central American Axis (Smithsonian Institution, 2022), are a preeminent feature of the greater Mesoamerican landscape, with a demonstrable impact on humans as both resource and threat (Sheets, 1981, 2001; Harris, 1982; Ford and Glicken, 1987; Ford and Rose, 1995; Siebe, 2000; Ford and Spera, 2007, 2016; Tankersley et al., 2011, 2016). The major eruption of Ilopango, sometime in the middle of the first millennium of our current era, is known to have had regional and hemispheric impact while the recorded historic eruptions of Santa Maria in 1902 and El Chichon in 1982 spread ash over much of the Maya area. Records of volcanic eruptions for the Maya area are presented as part of the environmental context of the region.
Time and paleoecology
8,000–4,000 BP
The Holocene Thermal Maximum, a period of four millennia noted worldwide (Burroughs, 2005; Rosen, 2007; Renssen et al., 2009; see also Burn and Mayle, 2008), is characterized by high precipitation in the Maya area (Haug et al., 2001; Mueller et al., 2009), set in the context of an overall drying trend (Wahl et al., 2014). Floristic evidence from lake core sediments demonstrates a major change to tropical megathermal plants (Deevey et al., 1979; Morley, 2000; Leyden, 2002; see also Kellman and Tackaberry, 1997; Morley, 2000) and features a rapid increase and ultimate dominance of Moraceae-type pollen (Figure 3), interpreted as representing mature forest cover (Brenner et al., 2002; Hillesheim et al., 2005). It is curious to note the perturbations in the forest signature around 5,000BP that coincides with a rise in forbs yet no change in grasses and continuity in precipitation (Figure 3). During this long period only seven major volcanic eruptions, from the highlands of Mexico and Central America (Smithsonian Institution, 2022), could have influenced the Maya area through inputs of tephra to the lowlands. One eruption is attributed to the western volcano, El Chichón (Espíndola et al., 2000).
4,000–3,000 BP
The end of the Holocene Thermal Maximum is marked by drastic precipitation fluctuations noted in the Cariaco as well as the Petén data (Haug et al., 2001; Mueller et al., 2009; see Ford and Nigh, 2009, 2015), with extremes of high and low rainfall set in an overall trend toward drier conditions that persist to the present (Haug et al., 2001; Mueller et al., 2006; Wahl et al., 2014). Amid this highly variable rainfall and continued drying trend (Wahl et al., 2014), floristic diversity increased, with greater abundances of forbs relative to trees and grasses remaining minimal throughout (Vaughan et al., 1985; Mueller et al., 2009; Figures 3, 4). This period also saw the initial influx of “Maya clay” in the Petén (Anselmetti et al., 2007; Mueller et al., 2010; Brenner, 2022), interpreted as the result of local soil erosion (Beach, 1998; Beach et al., 2002, 2006; Brenner et al., 2003; Mueller et al., 2006) but there are other possibilities that need to be explored as it not identified in the north (Torrescano and Islebe, 2015; Islebe et al., 2018). Volcanism increased, with seven major eruptions, including El Chichón.
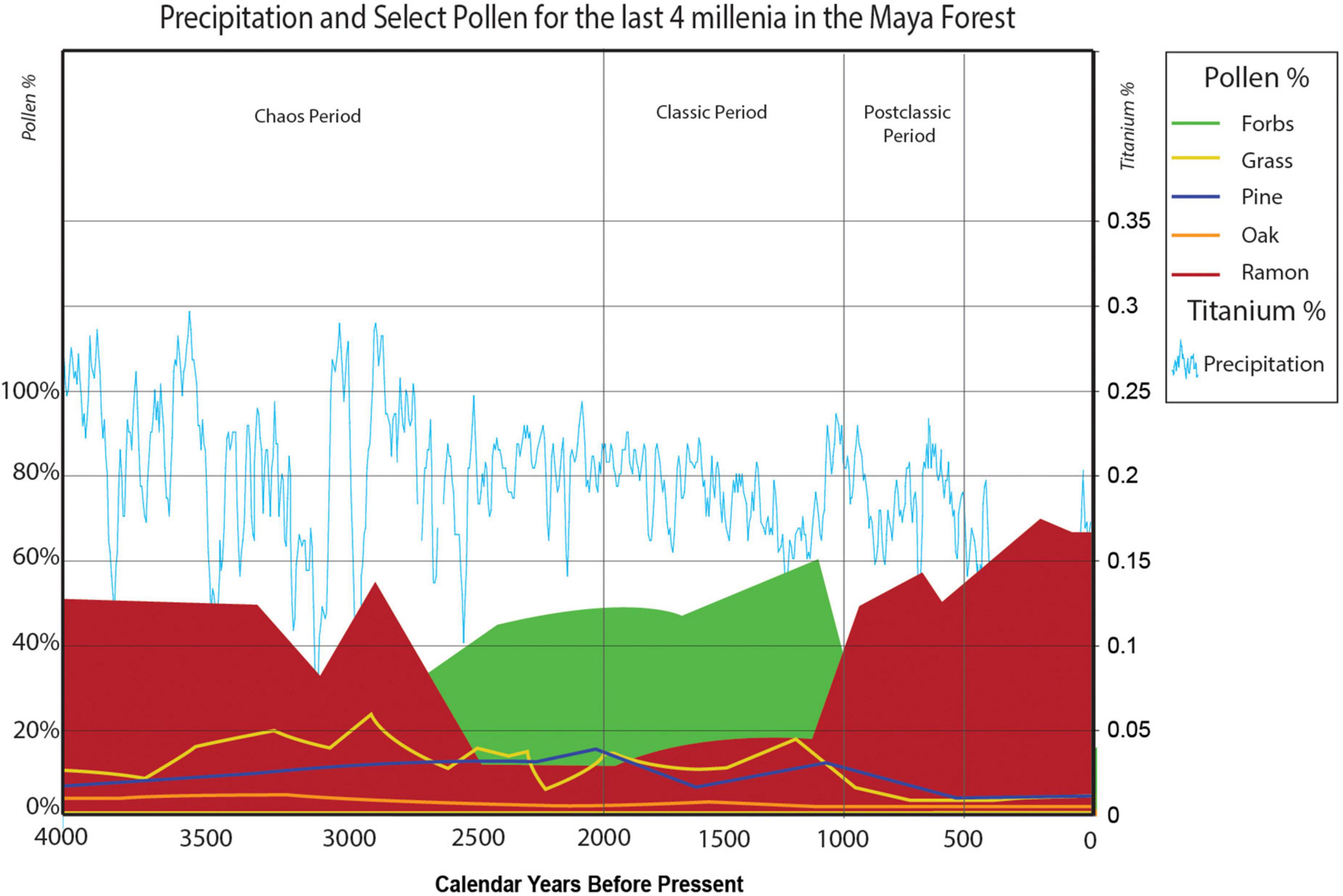
Figure 4. Precipitation proxy and key pollen for the last four millennia in the Maya forest. Scaled pollen data from Curtis et al. (1998), Brenner et al. (2002), and Leyden (2002) with Moracea-type, Brosimum alicastrum/Ramon as the indicator of forest, and the Cariaco Ti precipitation proxy data from Haug et al. (2003). Compiled by ISBER/MesoAmerican Research Center, UCSB.
3,000–2,000 BP
The era of chaotic precipitation was attenuating over the course of this period, and by 2,000 BP it stabilized under drier conditions than had existed during the Thermal Maximum, as inferred by a drop in Ti concentrations (Figure 3) from the Cariaco core. The composition and relative abundances of pollen taxa represented in lake core sediments continued to suggest a shift to higher species diversity, with a dominance of forbs and minimal grasses.
Simultaneous with the indicators of change in precipitation and flora, the amount of “Maya clay” rose to its highest levels (Anselmetti et al., 2007; Mueller et al., 2010). Although thought to be the result of erosion caused by agricultural deforestation, this is based on the wind pollen proxies. Curiously, this is a time period when population levels were low based on settlement chronologies and such radical soil movement would not be expected under traditional agricultural practices (Hooke, 2000; Montgomery, 2007). Further, there is no indication of animal habitat changes across the same area (Emery and Thornton, 2008) that concords with the archeobotanical data (Morell-Hart et al., Forthcoming). An alternative explanation could be the drier conditions or internal movement of lake sediments known as turbidites (Mueller et al., 2009). Volcanism decreased to just three major Mesoamerican eruptions recorded over this 1,000-year interval, including two from the nearest volcano, El Chichón (Espíndola et al., 2000; see also Battistel et al., 2018).
2,000–1,400 BP
Precipitation stabilized at a lower level than during the Holocene Thermal Maximum (Figure 3, see discussion Ford and Nigh, 2015). The pollen record comprises stable vegetation assemblages characterized by the continued dominance of forbs, with only 10% identified as forest taxa (Leyden, 1984, 1987). In addition, the influx of “Maya clay” stabilized at moderate levels (Brenner et al., 2002; Mueller et al., 2010), even as settlement, and presumably population, densities increase suggesting that land use is not related to the sediment feature. Interestingly, a significant rise in volcanism was evident, with 17 major eruptions, including three from El Chichón and one from the southern volcano Ilopango (Espíndola et al., 2000; Mehringer et al., 2005; Nooren et al., 2017).
1,400–1,100 BP
During this period there is continuity in the environmental indicators, including precipitation, pollen, and sedimentary inputs into the lakes. These data have been interpreted as representing continued deforestation (Rosenmeier et al., 2002; Wahl et al., 2006; Turner and Sabloff, 2012), the first evidence for which was initiated at least two millennia earlier based on the pollen proxy for forest, generalized as Brosimum alicastrum or ramon. Interestingly, significant volcanism is recorded with eight major eruptions, including El Chichón (Espíndola et al., 2000; Nooren et al., 2009; see also Battistel et al., 2018).
1,100–1,000 BP
While a single century may present an unrealistic time period in terms of the precision of paleoenvironmental records, this is a critical episode in Maya development that has generated speculations in terms of environmental interpretations. It is noteworthy that no truly dramatic climactic changes appear in the lake cores (Medina-Elizalde and Rohling, 2012; James et al., 2020, 2022). This is the case, despite the search for evidence of drought and other environmental impacts (Haug et al., 2003; Aimers and Hodell, 2011; Iannone et al., 2014). Based on the Cariaco core, precipitation was essentially stable, with some rise in levels of Ti, indicating increased rainfall (Haug et al., 2001), although research on speleothems has contributed to interpretations of drought (Webster et al., 2007; Douglas et al., 2015; Hoggarth et al., 2015; Ebert et al., 2017; James et al., 2022). These data, too, suffer from issues of precision.
“Maya clay” is reduced, and the vegetation remains diverse and dominated by forbs, which have been interpreted, using pollen proxy, as representing a backdrop of deforestation (Brenner, 1994, 2022; Rosenmeier et al., 2002; Douglas et al., 2015, 2016). Only two major volcanic eruptions are recorded for this short period (Smithsonian Institution, 2022).
1,000–500 BP
Precipitation in this period fluctuated and was unpredictable. Major pollen changes are registered in a significant rise in Moracea-type, or ramon, pollen, a decrease but lasting presence of forbs, and minimal grasses. The increase of the Moracea-type pollen in this period is comparable to the increase at the onset of the Holocene Thermal Maximum (Figure 3), where these perennials are prominent. The neglect of maintenance of ancient monuments provided new niches for pioneering and wind pollinated plants, and the Moracea-type pollen, associated with the tree Brosimum alicastrum or ramon, is well documented as a dominant plant on ancient Maya temples (Puleston, 1968; Lambert and Arnason, 1982; Schulze and Whitacre, 1999). No major volcanic eruptions are recorded from this period.
Concordance of Maya development with paleoenvironmental indicators
Deevey (1969) initiated his pioneering interdisciplinary work on human–environment interactions in the Maya forest of Petén, Guatemala, and the paleoecological database has grown and changed from these origins (Brenner et al., 2002). Increasing archeological evidence confirms the development of human presence over the past millennia, yet distinguishing climate change from human impact is nuanced and subtle (cf. Robinson et al., 2018). We know that humans inhabiting Mesoamerica were manipulating the landscape and involved with plants from the outset, yet early on, their presence was light, and environmental changes impacted human occupation more than humans influenced their surroundings. This is the case until there were permanent settlements across the landscape (see Mottl et al., 2021). The significant paleoenvironmental perturbations began a chaotic period c. 4,000 years ago (Figure 3) clearly constitutie a climate signature noted worldwide. In the Maya area this change is associated with the dramatic precipitation extremes identified in the Cariaco core and is reflected in the pollen data of the Maya area; there is a drop in the forest proxy and rise in forbs (Figure 4). More substantial presence of humans as indicated by the increase in archeological sites appear around this time, suggesting the emergence of agricultural settlements across Mesoamerica and the Maya area in the Early Preclassic was likely a response to the intense precipitation and natural resource uncertainties (Table 3).
The leading interpretations that the ancient Maya advanced unsustainable land use practices (summarized in Diamond, 2005) posit overpopulation and deforestation as destructive forces across the Maya lowlands (Figure 5; redrawn based on Binford et al., 1987; validated and reproduced many times: see Rice, 1996; Webster, 2002). This position is founded on the misunderstanding of swidden, derogatorily called shifting or slash-and-burn agriculture, that is contrasted with the high value placed on the achievements of the Maya civilization (Ford et al., 2021; see also Dove, 1983; Russell, 1988; Altieri, 2004; Mt. Pleasant, 2011, 2015). Recasting the nature of the Maya interactions with the forest to coincide with the historic and ethnographic land-use systems brings into focus alternative possibilities and opens the floor to different explanations.
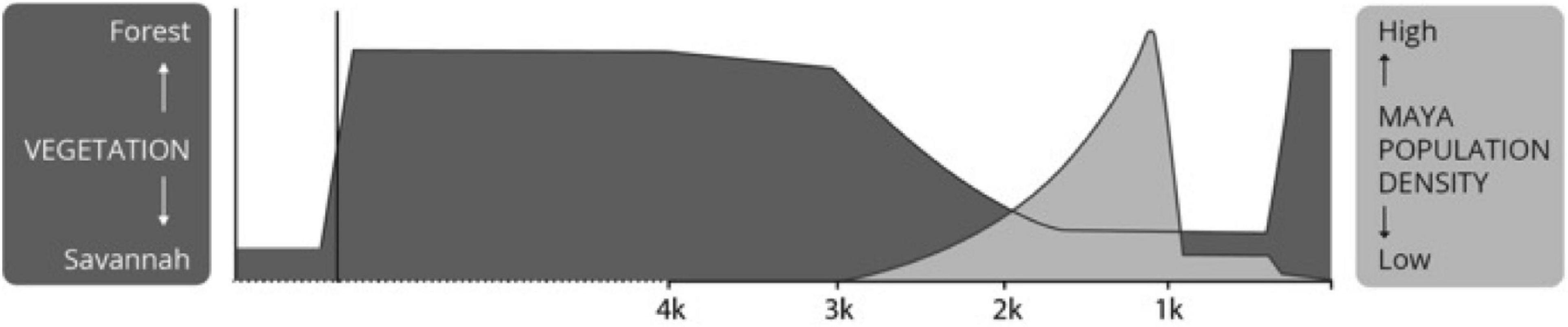
Figure 5. Interpretation of pollen in the Maya forest redrawn based on original presentations of Binford et al. (1987), Rice (1996), and Webster (2002) showing the impression of dramatic deforestation. Credit ISBER/MesoAmerican Research Center, UCSB.
As the Maya settled on the landscape, they increasingly shaped the forest through management in the context of the natural environment (Toledo et al., 2003; see also Iriarte et al., 2020; Bush et al., 2021), as revealed in the paleoethnobotanical evidence of diverse land-use and subsistence strategies employed by Maya ancestors (Morell Hart et al., in press). An assessment drawn from contemporary Maya agroforestry practices suggests adaptations based on the cyclic infield and outfield strategies previously discussed. Matching the archeological chronology with the paleoecological record through the lens of the forest garden milpa cycle can help to resolve the divergence between the successful growth of the Maya and the grim interpretations of paleoecological data.
It is reasonable to assume that, during the development of Maya civilization, changes on the landscape were due to human activity. Yet to turn a blind eye to traditional Maya agriculture and summarily cast these strategies and practices aside as inappropriate for the civilizational process and too primitive to account for the complexity of the Maya is short-sighted (see Terán and Rasmussen, 1995). As the contemporary Mayan languages are linked to the Classic Maya hieroglyphs (Macri and Ford, 1997; Houston et al., 2000), we can reasonably posit that Indigenous Maya knowledge – the local terminology of the forest and landscape—preserves traditional practices embedded in the language.
Indigenous skills and knowledge of the Maya forest are necessary for living in the Maya forest and are intertwined with the daily household economy (Sánchez-Suárez et al., 2021). Some Mayan expressions provide examples of this: Kanan K’aax is translated as “well cared for” forest, but also means to learn from and appreciate; K’axil kab refers to a forest with beehives; Ka’kab K’aax means a forest with good soil qualities; and Otoch K’aax literally means the forest is home, which can be interpreted as one feeling at home in the forest and that the forest provides the home. The milpa cycle has its particular terminology: short-term annuals (∼4 years) are found in the Kol, fast-growing perennials (∼8 years) are recognized as Pak Che’ Kol, and areas with mature canopy trees (∼8 years) can be called Känan K’aax. These Indigenous Mayan expressions are a key to the language of the Maya forest, relied upon by Master Forest Gardeners in their daily and seasonal field work (Campbell, 2007; Ellis and Ford, 2020; Ford et al., 2021). Evidence for diverse land-use and subsistence strategies in the past (Morell-Hart et al., Forthcoming) shows that the archeobotanical data conform with TEK evidence from contemporary Maya agricultural practices (Terán and Rasmussen, 1995; Anderson, 2003; Topsey et al., 2020).
Interpretations of the paleoecological data seem not to have considered the households of farmers and their critical needs beyond food. These views appear to imagine that one must necessarily reduce forest cover to increase food production. Major emphasis is put on landscape modifications, such as terraces (Healy et al., 1983; Dunning and Beach, 1994; Fedick, 1994; Chase and Chase, 2016) yet little thought given in labor investments and scheduling in food production (Ford and Nigh, 2015) nor to all the other necessities of life. Human interventions do change the forest, but that does not imply the removal of tree cover or the destruction of habitats (Chazdon, 2014; see also Roberts et al., 2021). Human-induced change has been envisioned as binary, where any disturbance to the forest is seen as damaging to the environment (Figure 5). Alternative views have largely been left unexpressed, unexplored, and untested for the Maya case (see Fedick, 2010, 2020; Ford and Nigh, 2015; Fisher, 2020).
Despite years of environmental and archeological research and data that increasingly reveal the sophistication of traditional Maya land use, the original idea that Maya deforestation and drought led to the collapse persists (Webster, 2002; Dunning et al., 2012; Turner and Sabloff, 2012; Kennett and Beach, 2013; Douglas et al., 2015, 2016; Marx et al., 2017; Evans et al., 2018; Lentz et al., 2018; Roman et al., 2018; James et al., 2020; among others). The relationships between paleoenvironmental reconstructions and ancient Maya prehistory have only recently been subjected to a long overdue review (Roberts, 2020; Roberts et al., 2021; see also Ford and Nigh, 2009, 2015; Fedick, 2010; McNeil et al., 2010; McNeil, 2020).
It is critical to appreciate that all necessities of ancient Maya life were derived from their tropical landscape. Daily, monthly, annually, generationally, adaptations were fundamental to everyday life. Understanding the flexibility of the milpa-forest garden cycle and its importance to living in the forest is essential. Agricultural practices developed over millennia advanced by managing the natural processes. The cycle had to meet the immediate food needs with annual crops while providing significant perennials for long term requirements. The Maya milpa cycle is essentially intensive system based on skills that monitor and address biodiversity, temperature, water, erosion, and soil fertility (Palerm, 1967, 1976; Wilken, 1971, 1987; Nations and Nigh, 1980; Gómez-Pompa, 1987; Hernández Xolocotzi et al., 1995; Terán and Rasmussen, 1995; Gliessman, 2001; Schwartz and Márquez, 2015; cf. Conklin, 1957).
Pollen signatures and the ascent of the milpa-forest garden cycle
The Maya forest is recognized today as anthropogenic in origin (Gómez-Pompa and Kaus, 1992, 1999; Gómez-Pompa et al., 2003; Gómez-Pompa, 2004; Barrera-Bassols and Toledo, 2005; among others), an obvious consequence of ancient human interactions with the environment (Campbell et al., 2006; Ford, 2008; Ross, 2011; see Covich, 1978). In the Archaic period, archeologists have identified domesticated crops such as chile, maize, squash, and beans, which made up the harvest of mobile horticulturalists, as well as the presence of palm fruits and avocado native to the lowlands (McClung de Tapia, 1992; Pope et al., 2001; Piperno and Stothert, 2003; Smalley and Blake, 2003; Neff et al., 2006; Casas et al., 2007; Pohl et al., 2007). This early experimentation represented the incipient agroecology from which the milpa cycle arose.
Tracking vegetation changes through time in the Maya forest area is challenging and the pollen record overrepresents wind pollinated plants. Woody plant species are overwhelmingly pollinated by animals, with only about 2% relying on wind to spread their pollen over the landscape (Kellman and Tackaberry, 1997; Bush and Rivera, 1998; Bradley, 1999; Ollerton et al., 2011). While some animal pollinated trees are represented in the local pollen rain of the Maya area, they are restricted to plants with open flower structures (Bush and Rivera, 2001), making it difficult to infer the presence and abundance of mature canopy forest species (Bush, 1995; Bhattacharya et al., 2011). Given these conditions, the pollen data can be quite revealing, and an understanding of potential land use strategies embedded in the milpa cycle can help to provide alternative explanations for discussion.
Of the dominant trees of the Maya forest (Table 1), only Brosimum alicastrum, commonly known as ramon, is wind pollinated. Ramon does not stand alone. It may indeed be a good proxy for the entire forest, but it would represent, at minimum, the presence of the dominant plants of the Maya forest. This is corroborated with the growing archeobotanical data that demonstrate the use of the dominant trees in prehistory (examples include Turner and Miksicek, 1984; Trabanino, 2012; Thompson, 2013; Trabanino-Garcia, 2014; Morehart and Morell-Hart, 2015; Thompson et al., 2015; Dussol, 2017; Dussol et al., 2017, Dussol et al., 2021; Varela Scherrer and Liendo Stuardo, 2021). The standard paleoecological interpretation has taken ramon as the indicator of the presence of forest and its absence an indication of deforestation (Figure 5). Clearly, the focus on pollen to understand the vegetation landscape is problematic and must be considered carefully (Islebe et al., 2015; Torrescano and Islebe, 2015; Torrescano et al., 2019).
The pollen signatures reveal, at first, worldwide climate changes, and later the complex interactions as the Maya adapted to their environment. The rise in temperature and precipitation attending the Holocene Thermal Maximum is mirrored well with the steep rise of the megathermal Moraceae and Brosimum-type pollen. The precipitation regime from 8,000 to 4,000 BP is consistent and relatively stable in the context of an overall drying trend noted for the region (Haug et al., 2001; Wahl et al., 2014; see Ford and Nigh, 2015). This setting of predictability and continuity is an important factor with respect to human adaptation. There is variation in the pollen signals, particularly in the relationship between forest and forbs. Forests dominate, yet forbs fluctuate from well below 5% to more than 20%, at the same time grasses hover around 5% (Figure 3). From the standpoint of the low-density, mobile horticulturalists of the Archaic, this was a time when natural resources were relatively predictable, and their impact on the forest was negligible. This relationship would later change with a shift in climate that brought radical highs and lows in precipitation creating instability.
Major climatic extremes that impacted the Maya region beginning in the Early Preclassic, and continuing from 4,000 and 2,000 years ago, provided an environmental impetus for adaptations that is detected archeologically in terms of permanent residential structures and incipient civic architecture. Maya farmers had to develop flexible strategies to cope with the radical shifts and unpredictability that characterized this chaotic period (Figure 3), and the successful adaptations developed at this difficult time must have laid the foundations for the later developments of the Maya civilization.
Originally interpreted simply as a shift from forest to savannah some 4,000 years ago (Binford et al., 1987; Rice, 1996; Webster, 2002, see Figure 5), a detailed examination of the pollen taxa reveals a much more diverse picture, where savannah grasslands play no role at all (compare Figure 3 with 5). The interpretation of savanna is driven by the classifications of “disturbance” and masks the existing variability evident in the pollen records (Brenner et al., 1990, 2002; Curtis et al., 1998; Leyden, 2002). Unpacking the generic disturbance taxa into forbs and grasses shows clearly that herbaceous forbs dominate grasses (see Figure 3 drawn from data presented in Brenner et al., 1990, 2002; Curtis et al., 1998; Leyden, 2002), exactly what one would expect from the implementation of milpa cycle agriculture (Slotten et al., 2020; Table 2). The growth of settled populations, and their expansion across the forested region around 3,000 years ago, corresponds to this “disturbance” land-cover signature, marking the early development of complex Maya communities in Preclassic times (Table 3).
Proportions of forest proxies to forbs appear reciprocal. The increase in the contribution of forbs, reflecting gaps in the forest (compare Table 3 and Figure 3), corresponds to changes in the forest recorded by the drop in the Moraceae and Brosimum-type pollen. While the interpretations have become more nuanced, the general perception that deforestation persists based on original presentations (Binford et al., 1987; see Figure 5), and persists among Maya scholars, despite the noteworthy evidence that grasses maintain a relatively low level throughout the sequence. The overall plant diversity increased with the proliferation of annual and perennial forbs representing c. 50% of identifiable taxa (Curtis et al., 1998; Brenner et al., 2002; Leyden, 2002). The drop in Moraceae/ramon more likely reflects the tree diversification preferences of the Maya, favoring trees useful for their fruit, construction materials, and other productive uses (see Cook, 2016; Ford, 2020), which are largely invisible because they are pollinated by birds, bees, and bats. Their pollen would therefore be less likely to settle into lakes and be retrievable by coring operations.
The climatic challenges the Maya faced during the Preclassic were enormous enough to produce the creative cultural adjustments that later underwrote their civilization. The uncertainties would have provided new opportunities in interactions with the landscape including the use of fire (Schüpbach et al., 2015; Anderson and Wahl, 2016; see also Abrams and Nowacki, 2020). Deluges followed by droughts in short sequence expedited the development of the milpa-forest garden system that endures today. One can only speculate about the triumphs and defeats this process of trial and error produced over the erratic centuries of this climate chaos period. There is no doubt, however, about the ultimate achievement: Maya settlements steadily grew and civic centers continued to be founded. These settlements flourished and ensured the prosperity of the Classic Period (Table 3), which influenced the composition of the Maya forest into the present day.
On the threshold of the Classic Period, some 2,000 years ago, irregularities in precipitation decreased, and there was more climatic stability. Classic Period paleoenvironmental indicators are largely constant and predictable in terms of precipitation and pollen (Figure 4). Precipitation ranges are comparable to those of the Holocene Thermal Maximum, but the overall climate was dryer. The uncertainties of the preceding centuries left their mark, and the land use strategies began during that period of climate chaos formed a solid base for growth and development. The cultural elaborations characteristic of Maya civilization—monumental temples, hieroglyphic texts, astronomical observations, and growing and diversifying settlements—were a consequence of continuity. With a more predictable environmental setting and less uncertainty, investments could be expanded in the civic realms.
Just as the human imprint of the Classic Period Maya is reflected in the paleoecological record, so is the evidence of the “collapse.” The reciprocal relationship of the forest proxies, Moraceae and Brosimum-type pollen (ramon) to forb pollen reverses without delay (cf Figure 4): as one rises the other drops, and the pollen record soon indicates the unrestrained expansion of pioneering ramon, known today to be competitive in the well-drained uplands (Schulze and Whitacre, 1999). As with the rise of ramon 8,000 years ago as it expanded into open niches during the warm and wet Holocene Thermal Maximum, evidence after 1,000 BP suggests expansion of ramon into a new, but different, niche (Figure 3). This new niche of open gaps was likely the neglected monumental architecture, left unmaintained to deteriorate, which the pioneering and limestone-loving ramon quickly exploited after being dispersed by animals, such as bats (Medellin and Gaona, 1999; Valdés Bérriz, 2015). The increase of ramon at Maya sites recognized today (Puleston, 1968, 1983; Lambert and Arnason, 1982; see also Burn and Mayle, 2008) may be best explained by the absence of monument management and ramon’s pioneering adaptation to flourish in rocky soils (Reina, 1984), just the habitat presented by derelict temples and palaces. Forbs stay between 20 and 40% until European contact and drop drastically after 500 BP. The presence of forbs is an indicator of the persistent practice of the milpa cycle by populations that continued to farm in the woodlands, like the contemporary Lacandon, without the overburden of political elites.
Precipitation scales
A preoccupation with drought has plagued the story of Maya civilization. Rainfall agriculture does need rain, but good farmers have developed practices and strategies based on keen observation to work with natural processes (Wilken, 1987; see also Dumond, 1961; Reina, 1967; Terán and Rasmussen, 1994; Hernández Xolocotzi et al., 1995; Tuxill, 2004; Schwartz and Márquez, 2015). Management efforts at the microhabitat level and observations that couple experience, skill, and knowledge form the basis for flexible adjustments to climate conditions. The intimate, local scale of small holder operations minimize risk over the long term and allow planning across time with expectations for good and bad harvests (Kramer and Hackman, 2021).
Maya farmers attune land use to the need for water conservation and favor specific trees in milpa fields for their economic values as well as their water conservation attributes. They make every effort to stagger field locations so that there is always some vegetation cover to conserve water with shade from trees, shrubs, and forbs (Ford et al., 2021). Skilled forest gardeners avoid broad clearings that expose land to excessive evapotranspiration and promote natural shade cover, even in fields. Home orchards, managed succession, and mature trees are of further importance to this cycle while providing necessary natural resources for food, construction, and medicine among other necessities (Ford, 2020).
Given the three recognized seasons, farmers identify their crop vulnerability to rainfall variation during the August dry period, known as the canicula, and the late growing season when plants reach maturity. Annual precipitation rates impact harvests less than a prolonged canicula or heavy rain when crops are ready to harvest. These are particular moments in the farming cycles that can arise in August or along the harvesting continuum from October to April.
While data on changing annual and decadal rainfall provide essential information on general environmental conditions within which the ancient Maya were living, they do not provide the appropriate scale for understanding farming strategies. These are coordinated at the local level, and even at the scale of individual plots, where understanding drainage qualities, provision of shade, and land cover to manage the water cycle and conserve moisture, as well as improve soil fertility and check erosion, are necessary to ensure the harvest.
Drought and deluge are factors that will menace the farmer’s harvest. Too little rain at the onset of planting is just as bad as too much rain at the climax of the crop. Climate modeling today shows that, in the Maya area, temperatures are increasing and precipitation is decreasing, but such trends do not reveal the timing of the precipitation. Farmers, at the local level, hedge their bets by dispersing their fields in wet and dry areas to leverage the uncertainties of seasonal weather dynamics (Morell-Hart et al., Forthcoming). Plots that cycle into the perennial phases break up the landscape between fields and forests, which assists water management and erosion by providing varied land cover.
Erosion
Much has been made of erosion as a consequence of forest clearing. The timing of the so-called erosion feature, “Maya clay,” is recognized in the Petén Lakes but not in other areas of the Maya lowlands. The relationship between deforestation and erosion as established through pollen reconstructions is ambiguous. The archeobotanical data, which demonstrate that a wide variety of woods were available to the Maya, need to be included in paleoenvironmental reconstructions (Sheets et al., 2012; Dunning et al., 2018; Morell-Hart et al., Forthcoming). Moreover, the timing of the proposed erosion events comes early in the development of Maya society, when population levels and density were very low. Rice and Rice (1990) estimate population at c. 43 persons per sq km at this time, which is about 20% of the estimates for the height of the Classic Period. Yet the interpreted erosion inexplicably diminishes with the growth and expansion of Maya settlements.
Montgomery (2007) provides a basis for evaluating erosion and a revealing review of global data on agriculture and erosion rates, which indicates that conventional agriculture — based on the Western model of arable monoculture — induces average soil movements between 1.5 and 3.94 mm per year. Contrast this with conservation agriculture, reflecting traditional systems of labor-based polyculture, which produce average movements of 0.08–0.124 mm per year. Conventional systems range from 19 to 32 times greater than the rates of the traditional systems today. The ancient Maya certainly impacted their local landscapes (e.g., Beach et al., 2006, 2008, 2018a; Dunning et al., 2012, Dunning et al., 2019; Wahl et al., 2019), but generalizing from the small populations of the Preclassic (Culbert and Rice, 1990), which relied entirely on stone tool technologies (Denevan, 1992) when facing the vigorous growth of tropical vegetation, it is difficult to accept “Maya clay” as major erosion feature. “Maya clay” in most prominent in Salpeten range from 6 to 7 m, requiring 1,700 years to accumulate based on erosional averages at the highest conventional agricultural rate of 3.94 mm/year. The highest traditional agriculture rates of 0.124 mm/year for the same length of time would accumulate only 0.21 m. The presumption that ancient Maya land use strategies from 3,000 years ago would have such an impact on the landscape needs to be directly tested.
In sum, viewing paleoecological data through the lens of traditional land use strategies can bring concordance to what appear as disparate lines of data. Pollen and precipitation data together provide the context for interpreting the successful Maya occupation of their forested landscape. In light of the new perspectives presented here, new challenges to accepted interpretations are clear. What data will indicate the transition from mobile horticulturalists to settled agriculturalists? How did the forest garden management system transform social hierarchies and fuel the development of Maya civilization? And, curiously, what were the economic, political, and social upheavals that caused the famous “collapse?” Maya farmers, who understood the art of not being governed, were there at the time of the conquest, so population abandonment provides no adequate explanation.
Discussion
There are compelling reasons to examine past societies to gain an understanding of interactions between humans and their environment, particularly in the tropics (Roberts, 2020). Archeological data span centuries and provide evidence for development, continuity, and change, yet, for the Maya case, major assumptions have been made in terms of land use impacts on soil, settlement impacts on the forest, and population impacts on the broader landscape (Webster, 2002, 2014; Turner and Sabloff, 2012). Reconsidering the record of human-environment interactions in the Maya area allows us to examine assumptions and explore alternative scenarios that may better encompass all the data at hand. Ancient Maya civilization grew and prospered for many centuries, and the roots of its success can be understood in terms of the traditional milpa-forest garden cycle.
Interpretations of lake-core pollen (Brenner et al., 2002; Leyden, 2002; Wahl et al., 2014) provide a broad picture of climate trends during the Holocene. As we look more deeply into the interactions that shaped the Holocene landscape, however, we must bring into focus the scale of human-environment dynamics in the Maya forest. The existing pollen record for the last 12,000 years provides one line of evidence to understand how the Maya forest developed. To appropriately interpret the pollen record, we need a clear appreciation of the archeological data and solid grasp of tropical forest agroecology.
Critically, regional pollen analyses have not taken into account the complexity of the landscape, comprising the diverse mosaic of well-drained uplands and humid lowlands, which ultimately contribute to interpretations of past land use and management. Nor do these analyses consider the cyclic nature and scale of land use characteristic of milpa cultivation, successional reforestation, and forest garden practices. The milpa-forest garden cycle (Table 2) can readily account for the distribution of wind-pollinated taxa identified in the fossil pollen record, particularly in the opportunities for the expansion of forbs as a signature of the fields and succession components. Consequently, the inference of widespread deforestation, beginning in the Preclassic and subsequent “forest recovery” after the so-called collapse are suspect.
The Maya forest today is a legacy of Maya landscape management strategies that must have included a complex settlement hierarchy, concentrated infield home gardens, and a wide array of intensive outfield and forest management systems across the region. Human impacts on the landscape are registered in the archeology and paleoecology of the Maya forest; the forest, however, with its diverse species has persisted and is comparable to what was in the past. There is little doubt that productivity of Maya land use strategies financed the development of the Maya civilization and its transformations. The ancestral Maya responded to uncertainties with the ongoing development of resource-management practices that interacted with and adapted to tropical forest ecological systems. This had to be a flexible system, amenable to varied circumstances, to support as complex a society as the Maya developed (cf. Scott, 2009). The milpa-forest garden cycle is a resource-management system that could have dynamically shaped the Maya forest and provided the basis to sustain early settlements and ultimately fueled the growth and development of the Classic period.
Conclusion and future directions
Mounting evidence on the diversity of contemporary Maya agriculture and the wealth of plant foods the Maya use today speaks to the resilience of the varied practices developed to mitigate the vagaries of deluge and drought over generations, centuries, and millennia. Bridging the convergent data on the ancient Maya and their descendent communities with paleoecological information casts doubt on the projection of human-induced environmental disaster.
The Maya forest management regime, as seen through contemporary agroforestry research and the dominant plants that constitute today’s forest, emphasizes beneficial plants that are economically valuable to contemporary Maya as well as the global world economy. These plant species confirm that the Maya had, and continue to have, a creative impact on forest composition, which stretches back to the very beginning of farming settlements in the region. By listening to traditional Maya farmers and learning from the body of TEK they draw on to sustain their household needs, archeologists and paleoecologists can formulate alternative hypotheses to explore the nature of ancient Maya human–environment interactions and impacts.
Recent studies document the wealth of plant foods available to the contemporary Maya and the resistance of many of these plants to varying levels of precipitation and drought. Archeobotanical research at ancient Maya sites, long neglected due to preservation issues posed by the tropical forest environment, is now expanding the catalog of identifiable floral remains used by urban and rural Maya alike. The identification of multiple tree, forb, grasses, and root-crop species in the archeological record that concord with those grown in contemporary Maya home gardens and milpa outfields indicates a level of continuity between past and present agricultural practices.
Prevailing environmental collapse models have three primary foundations: (1) interpretations of population estimates based on settlement pattern data, (2) inferences of climate change, erosion rates, and the composition of vegetation communities in the Maya forest area based on paleoenvironmental data, and (3) a conception of Maya agricultural strategies based on extensive slash-and-burn systems that expanded fields at the expense of the forest. Proxies recovered from paleoenvironmental investigations are interpreted in terms of these human activities. If ancient Maya land-management practices more closely resembled those of traditional Maya farmers today, however, extensive deforestation for milpa production—presumed as a driver of erosion rates and decline of forest-species—does not adequately describe human-landscape relationships. The linkage between recent archeobotanical data and studies centering on Maya TEK and land-management practices casts doubt on models of extensive forest clearance while providing new directions for testable hypotheses on ancient Maya human-environment interactions. Maya scholars, both archeologists and paleoenvironmental specialists alike, must be willing to engage with this new information, even if it means the revision of models that have dominated our thinking for decades.
Data availability statement
The original contributions presented in this study are included in the article/Supplementary material, further inquiries can be directed to the corresponding author.
Author contributions
The author confirms being the sole contributor of this work and has approved it for publication.
Acknowledgments
Reflection on Maya settlement and environment have been a focus of my career. Components of the research have been discussed and published over the past decades with a number of scholars who have been influential in developing ideas: Keith Clarke, SF, and Ronald Nigh. Sherman Horn was especially valuable in clarifying the complex themes that converge when comparing divergent data sets. Discussions and debates with Maya forest gardeners and work with these heroes has helped me to understand the milpa system: Narciso Torres, Alfonso Tzul, and Zacarias Quixchan.
Conflict of interest
The author declares that the research was conducted in the absence of any commercial or financial relationships that could be construed as a potential conflict of interest.
Publisher’s note
All claims expressed in this article are solely those of the authors and do not necessarily represent those of their affiliated organizations, or those of the publisher, the editors and the reviewers. Any product that may be evaluated in this article, or claim that may be made by its manufacturer, is not guaranteed or endorsed by the publisher.
Supplementary material
The Supplementary Material for this article can be found online at: https://www.frontiersin.org/articles/10.3389/fevo.2022.868660/full#supplementary-material
Footnotes
- ^ Forbs are herbaceous flowering plants separate from grass. Herbs include grasses.
References
Abrams, M. D., and Nowacki, G. J. (2020). Native american imprint in palaeoecology. Nat. Sustain. 3, 241–246. doi: 10.1038/s41893-020-0578-6
Aimers, J., and Hodell, D. (2011). Societal collapse: Drought and the maya. Nature 479, 44–45. doi: 10.1038/479044a
Akers, P. D., Brook, G. A., and Railsback, L. B. (2019). Integrating U-Th, 14C, and 210Pb Methods to Produce a Chronologically Reliable Isotope Record For the Belize River Valley Maya from a Low-Uranium Stalagmite. Holocene 29, 1234–1248. doi: 10.1177/0959683619838047
Alexander, R. T. (2006). Maya settlement shifts and agrarian ecology in yucatán. J. Anthropol. Res. 62:23. doi: 10.3998/jar.0521004.0062.401
Altieri, M. A. (2002). Agroecology: The science of natural resource management for poor farmers in marginal environments. Agric. Ecosyst. Environ. 93, 1–24. doi: 10.1016/S0167-8809(02)00085-3
Altieri, M. A. (2004). Linking ecologists and traditional farmers in the search for sustainable agriculture. Front. Ecol. Environ. 2, 35–42. doi: 10.1890/1540-92952004002[0035:LEATFI]2.0.CO;2
Altieri, M. A., and Toledo, V. M. (2011). The agroecological revolution in latin america: Rescuing nature, ensuring food sovereignty and empowering peasants. J. Peasant Stud. 38, 587–612. doi: 10.1080/03066150.2011.582947
Altieri, M. A., Funes-Monzote, F. R., and Petersen, P. (2012). Agroecologically efficient agricultural systems for smallholder farmers: Contributions to food sovereignty. Agron. Sustain. Dev 32, 1–13. doi: 10.1007/s13593-13011-10065-13596
Anderson, E. N. (2003). “Traditional Knowledge of Plant Resources,” in The Lowland Maya Area: Three Millennia at the Human-Wildland Interface, eds A. G. ómez-Pompa, M. F. Allen, S. L. Fedick, and J. J. Jiménez-Osornio (New York, NY: Food Products Press).
Anderson, L., and Wahl, D. (2016). Two Holocene paleofire records from Peten. Guatemala: Implications for natural fire regime and prehispanic maya land use. Glob. Planetary Change 138, 82–92. doi: 10.1016/j.gloplacha.2015.09.012
Anderson, M. K. (2005). Tending the Wild: Native American Knowledge and the Management of California’s Natural Resources. Berkeley, CA: University of California Press.
Anselmetti, F. S., Hodell, D. A., Ariztegui, D., Brenner, M., and Rosenmeier, M. F. (2007). Quantification of soil erosion rates related to ancient maya deforestation. Geology 35, 915–918. doi: 10.1130/G23834A.1
Armstrong, C. G., Miller, J. E. D., McAlvay, A. C., Ritchie, P. M., and Lepofsky, D. (2021). Historical indigenous land-use explains plant functional trait diversity. Ecol. Soc. 26:6. doi: 10.5751/ES-12322-260206
Atran, S. (1999). “Managing the Maya Commons: The Value of Local Knowledge,” in Ethnoecology: Situated Knowledge/Located Lives, ed. V. D. Nazarea (Tucson, AZ: University of Arizona Press), ages190–214. doi: 10.2307/j.ctv1gwqrkg.15
Atran, S. (2000). “The Vanishing Landscape of the Petén Maya Lowlands: People, Plants, Animals, Places, Words and Spirits,” in On Biocultural Diversity: Linking Language, Knowledge and the Environment, ed. L. Maffi (Washington, DC: Smithsonian Institution Press).
Atran, S. (2003). “Anthropogenic Vegetation: A Garden Experiment in the Maya Lowlands,” in The Lowland Maya Area: Three Millennia at the Human-Wildland Interface, eds A. G. ómez-Pompa, M. F. Allen, S. L. Fedick, and J. J. Jimenez-Osornio (New York, NY: Food Products Press), ages517–532.
Awe, J., Ebert, C., Stemp, W., Brown, M., Sullivan, L., and Garber, J. (2021). Lowland maya genesis: The late archaic to late early formative transition in the upper belize river valley. Anc. Mesoam. 32, 519–544. doi: 10.1017/S0956536121000420
Barrera Vásquez, A., Gómez-Pompa, A., and Vázquez-Yanes, C. (1977). El Manejo de las Selvas por los Mayas: Sus Implicaciones Silvícolas y Agrícolas. Biotica 2, 47–60.
Barrera-Bassols, N., and Toledo, V. M. (2005). Ethnoecology of the yucatec maya: Symbolism, knowledge and management of natural resources. J. Latin Am. Geogr. 4, 9–41. doi: 10.1353/lag.2005.0021
Battistel, D., Roman, M., Marchetti, A., Kehrwald, N. M., Radaelli, M., Balliana, E., et al. (2018). Anthropogenic impact in the maya lowlands of peten, guatemala, during the last 5500 years. J. Quat. Sci. 33, 166–176. doi: 10.1002/jqs.3013
Beach, T. (1998). Soil constraints on northwest yucatán, mexico: Pedoarchaeology and maya subsistence at chunchucmil. Geoarchaeology 13, 759–791. doi: 10.1002/(SICI)1520-6548(199812)13:8<759::AID-GEA1>3.0.CO;2-B
Beach, T., Dunning, N., Luzzadder-Beach, S., Cook, D. E., and Lohse, J. (2006). Impacts of the ancient maya on soils and soil erosion in the central maya lowlands. Catena 65, 166–178. doi: 10.1016/j.catena.2005.11.007
Beach, T., Luzzadder-Beach, S., Cook, D., Krause, S. M., Doyle, C., and Eshleman, S. (2018a). Stability and instability on Maya Lowlands tropical hillslope soils. Geomorphology 305, 185–208. doi: 10.1016/j.geomorph.2017.07.027
Beach, T., Luzzadder-Beach, S., Dunning, N., and Cook, D. (2008). Human and natural impacts on fluvial and karst depressions of the maya lowlands. Geomorphology 101, 308–331. doi: 10.1016/j.geomorph.2008.05.019
Beach, T., Ulmer, A., Cook, D., Brennan, M., Luzzadder-Beach, S., Doyle, C., et al. (2018b). Geoarchaeology and tropical forest soil catenas of northwestern Belize. Quaternary International 463, 198–217. doi: 10.1016/j.quaint.2017.02.031
Beach, T., Luzzadder-Beach, S., Dunning, N., Hageman, J., and Lohse, J. (2002). Upland agriculture in the maya lowlands: Ancient maya soil conservation in northwestern Belize. Geogr. Rev. 92, 372–397. doi: 10.2307/4140916
Beach, T., Luzzadder-Beach, S., Dunning, N., Jones, J., Lohse, J., Guderjan, T., et al. (2009). A review of human and natural changes in maya lowland wetlands over the holocene. Quat. Sci. Rev. 28, 1710–1724. doi: 10.1016/j.quascirev.2009.02.004
Beach, T., Luzzadder-Beach, S., Krause, S., Guderjan, T., Valdez, F Jr, and Fernandez-Diaz, J. C. (2019). Ancient Maya wetland fields revealed under tropical forest canopy from laser scanning and multiproxy evidence. PNAS 116, 21469–21477. doi: 10.1073/pnas.1910553116
Bhattacharya, T., Beach, T., and Wahl, D. (2011). An analysis of modern pollen rain from the Maya lowlands of northern Belize. Rev. Palaeobot. Palynol. 164, 109–120. doi: 10.1016/j.revpalbo.2010.11.010
Binford, M. W., Brenner, M., Whitmore, T. J., Higuera-Gundy, A., Deevey, E. S., and Leyden, B. (1987). Ecosystems, paleoecology and human disturbance in subtropical and tropical america. Quat. Sci. Rev. 6, 115–128. doi: 10.1016/0277-3791(87)90029-1
Blake, M. (2015). Maize for the Gods. Oakland, CA: University of California Press. doi: 10.1525/9780520961692
Blake, M., Chisholm, B. S., Clark, J. E., Voorhies, B., and Love, M. W. (1992). Prehistoric subsistence in the soconusco region. Curr. Anthropol. 33, 83–94. doi: 10.1086/204038
Bongers, F. (2017). Why secondary forests? Why now?. The Role of Tropical Secondary Forests in Conservation and Restoration. A joint webinar sponsored by the Society for Ecological Restoration and the Association for Tropical Biology and Conservation. Available online at: https://tropicalbiology.org/career-development/webinars/role-secondary-forests/ (accessed October, 2022).
Boserup, E. (1965). The Conditions of Agricultural Growth: The Economics of Agrarian Change Under Population Pressure. New York, NY: Aldine Pub. Co.
Boserup, E. (1981). Population and Technological Change: A Study of Long-Term Trends. Chicago, IL: University of Chicago Press.
Bradley, R. S. (1999). Paleoclimatology: Reconstructing Climates of the Quaternary, 2nd Edn. San Diego, CA: Academic Press.
Braje, T. J., and Erlandson, J. M. (2013). Human acceleration of animal and plant extinctions: A late pleistocene. holocene, and anthropocene continuum. Anthropocene 4, 14–23. doi: 10.1016/j.ancene.2013.08.003
Bray, F. (1994). Agriculture for developing nations. Sci. Am. 271, 30–37. doi: 10.1038/scientificamerican0794-30
Brenner, M. (1994). “Lakes Salpeten and Quexil, Petén, Guatemala, Central America,” in Global Geological Record of Lake Basins, eds E. Gierlowski-Kordesch and K. R. Kelts (Cambridge: Cambridge University Press).
Brenner, M. (2022). Classics revisited/from the archive mayan urbanism: Impact on a tropical karst environment. Prog. Physical Geogr. doi: 10.1177/03091333221112360
Brenner, M., Hodell, D. A., Curtis, J. H., Rosenmeier, M. F., Anselmetti, F. S., and Ariztegui, D. (2003). “Paleolimnological Approaches for Inferring Past Climate Change in the Maya Region: Recent Advances and Methodological Limitations,” in The Lowland Maya Area: Three Millennia at the Human-Wildland Interface, eds A. G. ómez-Pompa, M. F. Allen, S. L. Fedick, and J. J. Jimenez-Osornio (New York, NY: Haworth Press), ages45–75.
Brenner, M., Leyden, B., and Binford, M. W. (1990). Recent sedimentary histories of shallow lakes in guatemalan savannas. J. Paleolimnol. 4, 239–252. doi: 10.1007/BF00239697
Brenner, M., Rosenmeier, M. F., Hodell, D. A., and Curtis, J. H. (2002). Paleolimnology of the maya lowlands: Long-term perspectives on interactions among climate, environment, and humans. Ancient Mesoam. 13, 141–157. doi: 10.1017/S0956536102131063
Bullard, W. R. Jr. (1960). Maya settlement pattern in northeastern petén. Guatemala Am. Antiq. 25, 355–372. doi: 10.2307/277519
Burn, M. J., and Mayle, F. E. (2008). Palynological differentiation between genera of the moraceae family and implications for amazonian palaeoecology. Rev. Palaeobot. Palynol. 149:15. doi: 10.1016/j.revpalbo.2007.12.003
Burroughs, W. J. (2005). Climate Change in Prehistory: The End of the Reign of Chaos. New York, NY: Cambridge University Press. doi: 10.1017/CBO9780511535826
Bush, M. B. (1995). Neotropical plant reproductive strategies and fossil pollen representation. Am. Nat. 145, 594–609. doi: 10.1086/285757
Bush, M. B., and Rivera, R. (2001). Reproductive ecology and pollen representation among neotropical trees. Glob. Ecol. Biogeogr. 10, 359–367. doi: 10.1046/j.1466-822X.2001.00247.x
Bush, M. B., Nascimento, M. N., Åkesson, C. M., Maezumi, S. Y., Behling, H., Correa-Metrio, A., et al. (2021). Widespread reforestation before European influence on Amazonia. Science 372, 484–487. doi: 10.1126/science.abf3870
Bush, M., and Rivera, R. (1998). Pollen dispersal and representation in a neotropical rain forest. Glob. Ecol. Biogeogr. 7:14. doi: 10.2307/2997685
Caballero, J. (1992). The maya homegardens of the yucatan peninsula: Past. Present Fut. Etnoecol. 1, 35–54.
Caffrey, M. A., Taylor, M. J., and Sullivan, D. G. (2011). A 12,000-year record of vegetation and climate change from the sierra de los cuchumatanes, guatemala. J. Latin Am. Geogr. 10, 129–151. doi: 10.1353/lag.2011.0041
Cagnato, C. (2021). Gathering and sowing across the central maya lowlands: A review of plant use by preceramic peoples and the early to middle preclassic maya. Ancient Mesoam. 32, 486–501. doi: 10.1017/S0956536121000225
Campbell, D. G. (2007). Don Berto’s Garden: Language, Biodiversity, and a Story of Salvation. Great Barrington, MA: Orion Magazine.
Campbell, D. G., Ford, A., Lowell, K., Walker, J., Lake, J. K., Ocampo-Raeder, C., et al. (2006). “The Feral Forests of the Eastern Petén,” in Time and Complexity in the Neotropical Lowlands, eds C. Erickson and W. Baleé (New York, NY: Columbia University Press), ages21–55. doi: 10.7312/bale13562-004
Canuto, M. A., and Auld-Thomas, L. (2021). Taking the high ground: A model for lowland Maya settlement patterns. J. Anthropol. Archaeol. 64:101349. doi: 10.1016/j.jaa.2021.101349
Canuto, M. A., Estrada-Belli, F., Garrison, T. G., Houston, S. D., Acuña, M. J., Kováč, M., et al. (2018). Ancient lowland maya complexity as revealed by airborne laser scanning of northern guatemala. Science 361:eaau0137. doi: 10.1126/science.aau0137
Carillo-Bastos, A., Islebe, G. A., and Nuria, T.-V. (2012). Geospatial Analysis of Pollen Records from the Yucatan Peninsula. Mexico. Veget. Hist. Archaeobot. 21, 429–437. doi: 10.1007/s00334-012-0355-1
Carozza, J.-M., Galop, D., Metaile, J.-P., Vanniere, B., Bossuet, M., and Monna, F. (2007). Landuse and soil degradation in the Southern Maya Lowlands, from pre-classic to post-classic times: The case of La Joyanca (Petén, Guatemala). Geodinamica Acta 20, 195–207. doi: 10.3166/ga.20.195-207
Carr, R. F., and Hazard, J. E. (1961). Tikal Report 11: Maps of the Ruins of Tikal, El Peten, Guatemala. Philadelphia, AR: University Museum University of Pennsylvania.
Casas, A., Otero-Arnaiz, A., Perez-Negron, E., and Valiente-Banuet, A. (2007). In situ management and domestication of plants in mesoamerica. Ann. Bot. 100, 1101–1115. doi: 10.1093/aob/mcm126
Castro-López, V., Domínguez-Vázquez, G., Islebe, G. A., Priego-Santander, ÁG., and Velázquez, A. (2021). Modern pollen-vegetation relationships across a landscape mosaic in central México. Rev. Palaeobot. Palynol. 289:104362. doi: 10.1016/j.revpalbo.2020.104362
Chase, A. F., and Chase, D. Z. (1987). Investigations at the Classic Maya City of Caracol, Belize: 1985-1987. San Francisc, CA: Pre-Columbian Art Research Institute.
Chase, A. F., and Chase, D. Z. (2016). The ancient Maya city: Anthropogenic landscapes, settlement archaeology, and Caracol. Belize. Res. Rep. Belizean Archaeol. 13, 3–14.
Chase, A. F., Chase, D. Z., Weishampe, J. F., Drake, J. B., Shrestha, R. L., Slatton, K. C., et al. (2011). Airborne lidar, archaeology, and the ancient maya landscape at caracol, belize. J. Archaeol. Sci. 38, 387–398. doi: 10.1016/j.jas.2010.09.018
Chazdon, R. L. (2014). Second Growth: The Promise of Tropical Forest Regeneration in an Age of Deforestation. Chicago, IL: University of Chicago Press. doi: 10.7208/chicago/9780226118109.001.0001
Clark, J. E., and Cheetham, D. (2002). “Mesoamerica’s Tribal Foundations,” in The Archaeology of Tribal Societies, ed. W. A. Parkinson (Ann Arbor, CA: International Monographs in Prehistory), ages278–339. doi: 10.2307/j.ctv8bt29z.18
Coe, W. R. (1965). Tikal, guatemala and emergent maya civilization - excavations reveal evidence of early complex-living at a prime maya indian site. Science 147, 1401–1419. doi: 10.1126/science.147.3664.1401
Coffey, K. T., Schmitt, A. K., Ford, A., Spera, F. J., Christensen, C., and Garrison, J. (2014). Volcanic ash provenance from zircon dust with an application to maya pottery. Geology 42, 595–598. doi: 10.1130/G35376.1
Conklin, H. (1957). Hanunóo Agriculture: A Report on an Integral System of Shifting Cultivation in the Philippines. Rome: FAO Food and Agriculture Organization of the United Nations.
Conklin, H. C. (1954). “An Ethnoecological Approach to Shifting Agriculture,” in Transactions of the New York Academy of Sciences, ed. R. W. Miner (New York, NY: The New York Academy of Sciences), ages133–142. doi: 10.1111/j.2164-0947.1954.tb00402.x
Cook, S. (2016). The Forest of the Lacandon Maya- An Ethnobotaical Guide. New York, NY: Springer Science+Business Media. doi: 10.1007/978-1-4614-9111-8
Corzo Márquez, A. R., and Schwartz, N. B. (2008). Traditional home gardens of petén, guatemala: Resource management, food security, and conservation. J. Ethnobiol. 28, 305–317. doi: 10.2993/0278-0771-28.2.305
Covich, A. P. (1978). “A Reassessment of Ecological Stability in the Maya Area: Evidence from Lake Studies of Early Agricultural Impacts on Biotic Communities,” in Prehispanic Maya Agriculture, eds P. Harrison and B. L. Turner (Albuquerque, NM: University of New Mexico Press), ages145–155.
Culbert, T. P., and Rice, D. S. (1990). Precolumbian Population History in the Maya Lowlands. Albuquerque, NM: University of New Mexico Press.
Curtis, J. H., Brenner, M., Hodell, D. A., Balser, R. A., Islebe, G. A., and Hooghiemstra, H. (1998). A multi-proxy study of holocene environmental change in the maya lowlands of petén. Guatemala J. Paleolimnol. 19, 139–159. doi: 10.1023/A:1007968508262
De Clerck, F. A. J., and Negreros-Castillo, P. (2000). Plant species of traditional mayan homegardens of mexico as analogs for multistrata agroforests. Agrofor. Syst. 48, 303–317. doi: 10.1023/A:1006322612362
Deevey, E. S. (1969). Coaxing history to conduct experiments. BioScience 19, 40–43. doi: 10.2307/1294629
Deevey, E. S., Rice, D. S., Rice, P. M., Vaughan, H. H., Brenner, M., and Flannery, M. S. (1979). Mayan urbanism: Impact on a tropical karst environment. Science 206, 298–306. doi: 10.1126/science.206.4416.298
Demarest, A. A. (2004). Ancient Maya: The Rise and Fall of a Rainforest Civilization. Cambridge: Cambridge University Press.
Denevan, W. M. (1992). Stone vs metal axes: The ambiguity of shifting cultivation in prehistoric Amazonia. J. Steward Anthropol. Soc. 20, 153–165.
Diamond, J. (2005). Collapse: How Societies Choose to Fail or Succeed. New York, NY: The Penguin Group.
Douglas, P. M. J., Demares, A. A., Brenner, M., and Canuto, M. A. (2016). Impacts of climate change on the collapse of lowland maya civilization. Annu. Rev. Earth Planetary Sci. 44, 613–645 doi: 10.1146/annurev-earth-060115-012512
Douglas, P. M., Pagani, M., Canuto, M. A., Brenner, M., Hodell, D. A., Eglinton, T. I., et al. (2015). Drought, agricultural adaptation, and sociopolitical collapse in the Maya Lowlands. Proc. Natl. Acad. Sci. U.S.A. 112, 5607–5612. doi: 10.1073/pnas.1419133112
Dove, M. (1983). Theories of swidden agriculture and the political economy of ignorance. Agrofor. Syst. 1, 85–99. doi: 10.1007/BF00596351
Dove, M. R. (1993). A Revisionist View of Tropical Deforestation and Development. Evnviron. Conserv. 20:56. doi: 10.1017/S0376892900037188
Dumond, D. E. (1961). Swidden Agriculture and the Rise of Maya Civilization. Southwest. J. Anthropol. 17, 301–314. doi: 10.1086/soutjanth.17.4.3628942
Dunning, N. P., and Beach, T. (1994). Soil erosion, slope management, and ancient terracing in the Maya lowlands. Latin Am. Antiq. 5, 51–69. doi: 10.2307/971902
Dunning, N. P., and Beach, T. (2000). “Stability and Instability in Prehispanic Maya Landscapes,” in An Imperfect Balance: Landscape Transformations in the Precolumbian America, ed. D. L. Lentz (New York, NY: Columbia University Press), ages179–202. doi: 10.7312/lent11156-010
Dunning, N. P., and Beach, T. (2010). “Farms and Forests: Spatial and Temporal Perspectives on Ancient Maya Landscapes,” in Landscapes and Societies: Selected Cases, eds P. Martini and W. Chesworth (New York, NY: Springer Science+Business Media), ages381–382. doi: 10.1007/978-90-481-9413-1_23
Dunning, N. P., Beach, T. P., and Luzzadder-Beach, S. (2012). Kax and Kol: Collapse and resilience in lowland maya civilization. Proc. Natl. Acad. Sci. U.S.A. 109, 3652–3657. doi: 10.1073/pnas.1114838109
Dunning, N. P., Beach, T., and Luzzadder-Beach, S. (2020). “Ancient maya agriculture,” in The maya world, eds S. Hutson and T. Arden (New York, NY: Routledge), 501–518.
Dunning, N. P., Luzzadder-Beach, S., Beach, T., Jones, J. G., Scarborough, V., and Culbert, T. P. (2002). Arising from the bajos: The evolution of a neotropical landscape and the rise of maya civilization. Ann. Assoc. Am. Geogr. 92, 267–283. doi: 10.1111/1467-8306.00290
Dunning, N., Beach, T., Farrell, P., and Luzzadder-Beach, S. (1998). Prehispanic agrosystems: Adaptive regions in the maya lowlands. Cult. Agric. 20, 87–101. doi: 10.1525/cag.1998.20.2-3.87
Dunning, N., Beach, T., Graham, E., Lentz, D., and Luzzadder-Beach, S. (2018). “Maize, Manioc, Mamay, and More: Precolumbian Lowland Maya Agriculture,” in The Archaeology of Caribbean and Circum-Caribbean Farmers, ed. B. Reid (New York, NY: Routledge), ages329–352. doi: 10.4324/9781351169202-15
Dunning, N., Beach, T., Luzzadder-Beach, S., and Jones, J. G. (2009). “Creating Stable Landscape: Soil Conservation and Adaptation Among the Maya,” in The Archaeology of Environmental Change, eds C. T. Fisher, J. B. Hill, and G. M. Feinman (Tucson: University of Arizona Press), ages85–105. doi: 10.2307/j.ctv2k88sk5.7
Dunning, N. P., Hernández, A. A., Beach, T., Carr, C., Griffin, R., Jones, J., et al. (2019). Margin for error: Anthropogenic geomorphology of bajo edges in the maya lowlands. Geomorphology 331, 127–145. doi: 10.1016/j.geomorph.2018.09.002
Dussol, L. (2017). Feux et forêts mayas. Usages et gestion des combustibles ligneux dans les Basses Terres centrales mayas à la période Classique : le cas du site de Naachtun, Petén, Guatemala, approche anthracologique. Paris, FR: Université de Paris 1 Panthéon-Sorbonne.
Dussol, L., Elliott, M., and Théry-Parisot, I. (2017). Experimental anthracology: Evaluating the role of combustion processes in the representivity of archaeological charcoal records in tropical forests, a case study from the Maya Lowlands. J. Archaeol. Sci. 12, 480–490. doi: 10.1016/j.jasrep.2017.02.020
Dussol, L., Elliott, M., Michelet, D., and Nondédéo, P. (2021). Fuel economy, woodland management and adaptation strategies in a classic maya city: Applying anthracology to urban settings in high biodiversity tropical forests. Veg. His. Archaeobot. 30, 175–192. doi: 10.1007/s00334-020-00776-0
Eastmond, A., and Faust, B. (2006). Farmers, fires, and forests: A green alternative to shifting cultivation for conservation of the maya forest? Landscape Urban Planning 74, 267–284. doi: 10.1016/j.landurbplan.2004.09.007
Ebert, C. E., May, N. P., Culleton, B. J., Awe, J. J., and Kennett, D. J. (2017). Regional response to drought during the formation and decline of preclassic maya societies. Quat. Sci. Rev. 173, 211–235. doi: 10.1016/j.quascirev.2017.08.020
Emery, K. F., and Thornton, E. K. (2008). Zooarchaeological habitat analysis of ancient maya landscape changes. J. Ethnobiol. 28, 154–178. doi: 10.2993/0278-0771-28.2.154
Emery, K. F., and Thornton, E. K. (2012). “Using Animal Remains to Reconstruct Ancient Landscapes and Climate in the Central and Southern Maya Lowlands,” in Proceedings of the General Session of the 11th International Council for Archaeozoology Conference (Paris, 23-28 August 2010), ed. C. Lefèvre (Oxford: Archaeopress), ages203–225.
Espíndola, J. M., Macías, J. L., Tilling, R. I., and Sheridan, M. F. (2000). Volcanic History of El Chichón Volcano (Chiapas, Mexico) during the Holocene, and its impact on human activity. Bull. Volcanol. 62, 90–104. doi: 10.1007/s004459900064
Evans, D. L., Vis, B. N., Dunning, N. P., Graham, E., and Isendahl, C. (2021). Buried solutions: How maya urban life substantiates soil connectivity. Geoderma 377:114925. doi: 10.1016/j.geoderma.2020.114925
Evans, N. P., Bauska, T. K., ázquez-Sánchez, F. G., Brenner, M., Curtis, J. H., and Hodell, D. A. (2018). Quantification of drought during the collapse of the classic Maya civilization. Science 361, 498–501. doi: 10.1126/science.aas9871
Farriss, N. M. (1984). Maya Society Under Colonial Rule: The Collective Enterprise of Survival. Princeton, NJ: Princeton University Press. doi: 10.1515/9780691235400
Faust, B. B. (1998). Mexican Rural Development and the Plumed Serpent: Technology and Maya Cosmology in the Tropical Forest of Campeche, México. Westport, CT: Bergin & Garvey.
Faust, B. B. (2001). Maya environmental successes and failures in the yucatán peninsula. Environ. Sci. Policy 4, 153–169. doi: 10.1016/S1462-9011(01)00026-0
Fedick, S. L. (1989). “The Economics of Agricultural Land Use and Settlement in the Upper Belize River Valley,” in Prehistoric Maya Economies of Belize, eds P. A. McAnany and B. L. Isaac (Greenwich: JAI Press), ages215–254.
Fedick, S. L. (1992). “An agricultural perspective on prehistoric maya household location and settlement density,” in Proceedings of the memorias del primer congreso internacional de mayistas; Mesas redondas, arquelogía, epigrafía (Coyoacán: Universidad Nacional Autónoma de México), 87–108.
Fedick, S. L. (1994). Ancient maya agricultural terracing in the upper Belize river area: Computer-aided modeling and the results of initial field investigations. Ancient Mesoam. 5, 107–127. doi: 10.1017/S0956536100001073
Fedick, S. L. (1996). “Landscape Approaches to the Study of Ancient Maya Agriculture and Resource Use,” in The Managed Mosaic: Ancient Maya Agriculture and Resource Use, ed. S. L. Fedick (Salt Lake City, UT: University of Utah Press), ages335–348.
Fedick, S. L. (2010). The Maya forest: Destroyed or cultivated by the ancient maya. Proc. Natl. Acad. Sci. U.S.A. 107, 953–954. doi: 10.1073/pnas.0913578107
Fedick, S. L. (2020). “Maya Cornucopia Indigenous Food Plants of the Maya Lowlands,” in The Real Business of Ancient Maya Economies, eds D. A. Freidel, M. A. Masson, and A. A. Demarest (Gainesville, FL: University Press of Florida). doi: 10.5744/florida/9780813066295.003.0013
Fedick, S. L., and Ford, A. (1990). The prehistoric agricultural landscape of the central Maya Lowlands: An examination of local variability in a regional context. World Archaeol. 22, 18–33. doi: 10.1080/00438243.1990.9980126
Fedick, S. L., and Santiago, L. S. (2022). Large variation in availability of Maya food plant sources during ancient droughts. PNAS 119:e2115657118. doi: 10.1073/pnas.2115657118
Fenzi, M., Jarvis, D. I., Arias Reyes, L. M., Moreno, L. L., and Tuxills, J. (2017). Longitudinal analysis of maize diversity in Yucatan, Mexico: Influence of agro-ecological factors on landraces conservation and modern variety introduction. Plant Genet. Resour. 15, 51–63. doi: 10.1017/S1479262115000374
Ferrand, E. A., Dunning, N. P., Lentz, D. L., and Jones, J. G. (2012). Use of aguadas as water management sources in two southern Maya lowland sites. Ancient Mesoam. 23, 85–101. doi: 10.1017/S0956536112000065
Fisher, C. (2014). The role of infield agriculture in maya cities. J. Anthropol. Archaeol. 36, 196–210. doi: 10.1016/j.jaa.2014.10.001
Fisher, C. (2020). Archaeology for sustainable agriculture. J Archaeol Res. 28, 393–441. doi: 10.1007/s10814-019-09138-5
Ford, A. (1986). Population Growth and Social Complexity: An Examination of Settlement and Environment in the Central Maya Lowlands. Tempe, AZ: Arizona State University.
Ford, A. (1990). “Maya Settlement in the Belize River Area: Variations in Residence Patterns of the Central Maya Lowlands,” in Prehistoric Population History in the Maya Lowlands, eds T. P. Culbert and D. S. Rice (Albuquerque, NM: University of New Mexico Press), ages167–181.
Ford, A. (1991). Problems with the evaluation of population from settlement data: Examination of ancient maya residential patterns in the tikal-yaxhá intersite area. Estudios de Cultura Maya. 18, 157–186. doi: 10.19130/iifl.ecm.1991.18.175
Ford, A. (1996). “Critical Resource Control and the Rise of the Classic Period Maya,” in The Managed Mosaic: Ancient Maya Agriculture and Resource Use, ed. S. L. Fedick (Salt Lake City, UT: University of Utah Press), ages297–303.
Ford, A. (2004). “Integration Among Communities, Centers, and Regions: The Case from El Pilar,” in The Ancient Maya of the Belize Valley: Half a Century of Archaeological Research, eds J. Garber and D. Chase (Gainesville, FL: University Press of Florida), ages238–256.
Ford, A. (2008). Dominant plants of the Maya Forest and gardens of el pilar: Implications for paleoenvironmental reconstructions. J. Ethnobiol. 28, 179–199. doi: 10.2993/0278-0771-28.2.179
Ford, A. (2020). “The Maya Forest: A Domesticated Landscape,” in The Maya World, eds S. R. Hutson and T. Arden (London: Routledge). doi: 10.4324/9781351029582-33
Ford, A., and Clarke, K. C. (2019). “Linking the Past and Present of the Ancient Maya: Lowland Land Use, Populatioin Distribution, ad Density in the Late Classic Period,” in The Oxford Handbook of Histoical Ecology and Applied Archaeology, eds C. Isendahl and D. Stump (Oxford: Oxford University Press), ages156–183.
Ford, A., and Glicken, H. (1987). “The Significance of Volcanic Ash Tempering in the Ceramics of the Central Maya Lowlands,” in 1985 Maya Ceramics Conference, eds P. M. Rice and R. J. Sharer (Oxford: British Archaeo logical Reports), ages479–502.
Ford, A., and Horn, I. S. (2017). El Pilar monuments retrospective & prospective: Re-discovering el pilar. Res. Rep. Belizean Archaeol. 14, 87–95. doi: 10.1007/978-3-319-51726-1_3195-1
Ford, A., and Horn, S. (2018). Above and below the Maya forest: Advanced remote sensing technology raises questions about settlement and land use. Science 316, 1313–1314. doi: 10.1126/science.aav0887
Ford, A., and Nigh, R. (2009). Origins of the Maya forest garden: A resource management system. J. Ethnobiol. 29, 213–236. doi: 10.2993/0278-0771-29.2.213
Ford, A., and Nigh, R. (2014). “Climate Change in the Ancient Maya Forest: Resilience and Adaptive Management Across Millennia,” in The Great Maya Droughts In Cultural Context: Case Studies in Resilience and Vulnerability, ed. G. Iannone (Boulder,CO: University Press of Colorado), ages87–106.
Ford, A., and Nigh, R. (2015). The Maya Forest Garden: Eight Millennia of Sustainable Cultivation in the Tropical Woodlands. Santa Rosa, CA: Left Coast Press.
Ford, A., and Rose, W. I. (1995). Volcanic ash in ancient maya ceramics of the limestone lowlands: Implications for prehistoric volcanic activity in the guatemala highlands. J. Volcanol. Geothermal Res. 66, 149–162. doi: 10.1016/0377-0273(94)00068-R
Ford, A., and Spera, F. (2007). Fresh volcanic glass shards in the pottery shards of the maya lowlands. Res. Rep. Belizean Archaeol. 4, 111–118.
Ford, A., and Spera, F. (2016). “Petrography First: Issues of Identification and Sourcing Volcanic Ash Temper in Maya Pottery,” in Integrative Approaches in Ceramic Petrography, eds M. Ownby, I. Druc, and M. Masucci (Salt Lake City, UT: University of Utah Press), ages8–23.
Ford, A., Clarke, K. C., and Morlet, S. (2011). “Calculating Late Classic Lowland Maya Population for the Upper Belize River Area,” in Archaeological Investigations in the Eastern Maya Lowlands: Papers of the 2010 Belize Archaeology Symposium, eds J. Morris, J. Awe, G. Thompson, and M. Badillo (Belmopan,BZ: Institute of Archaeology), ages75–87.
Ford, A., Clarke, K. C., and Raines, G. (2009). Modeling settlement patterns of the late classic maya with bayesian methods and GIS. Ann. Assoc. Am. Geogr. 99, 496–520. doi: 10.1080/00045600902931785
Ford, A., Horn, S., Crimmel, T., and Tran, J. (2021). Conserving the American tropics: Exploring the cropscape of the ancient maya. Technol. Stories 9. Available online at: https://www.technologystories.org/conserving-the-american-tropics-exploring-the-cropscape-of-the-ancient-maya/
Forsyth, D. W. (1989). “La Cerámica Arqueológica de Nakbe y El Mirador, Peten,” in III Simposio de Arqueología Guatemalteca, eds P. J. P. Laporte, H. Escobedo, and Y. S. Villagrán (Guatemala City: Asociacion Tikal).
Foster, D., Swanson, F., Aber, J., Burke, I., Brokaw, N., Tilman, D., et al. (2003). The Importance of Land-Use legacies to ecology and conservation. BioScience 53, 77–88. doi: 10.1641/0006-3568(2003)053[0077:TIOLUL]2.0.CO;2
Fredericksen, T. S., and Pariona, W. (2002). Effect of Skidder Disturbance on Commercial Tree Regeneration in Logging Gaps in A Bolivian Tropical Forest. For. Ecol. Manag. 171, 223–230. doi: 10.1016/S0378-1127(01)00767-8
Gliessman, S. R. (1982). Nitrogen Distribution in Several Traditional Agro-ecosystems in the Humid Tropical Lowlands of South-eastern México. Plant Soil 67, 105–117. doi: 10.1007/BF02182759
Gliessman, S. R. (1992). Agroecology in the tropics: Achieving a balance between land use and preservation. Environ. Manag. 16, 681–689. doi: 10.1007/BF02645658
Gliessman, S. R. (1998). Agroecology: Ecological Processes in Sustainable Agriculture. Ann Arbor, MI: Ann Arbor Press.
Gliessman, S. R. (2001). Agroecosystem Sustainability: Developing Practical Strategies. Boco Raton, FL: CRC Press. doi: 10.1201/9781420041514
Goebel, T., Waters, M. R., and Dikova, M. (2003). The archaeology of Ushki Lake, Kamchatka, and the Pleistocene peopling of the Americas. Science 301, 501–505. doi: 10.1126/science.1086555
Gold, M. V. (1999). Sustainable Agriculture: Definitions and Terms. Special Reference Briefs Series 2011. Available online at: http://www.nal.usda.gov/afsic/pubs/terms/srb9902.shtml#toc9901 (accessed October, 2022).
Gómez-Pompa, A. (2004). The role of biodiversity scientists in a troubled world. BioScience 54:217. doi: 10.1641/0006-3568(2004)054[0217:TROBSI]2.0.CO;2
Gómez-Pompa, A., Allen, M. F., Fedick, S. L., and Jimenez-Osornio, J. J. (eds) (2003). The Lowland Maya Area: Three Millennia at the Human-Wildland Interface. New York, NY: Food Products Press.
Gómez-Pompa, A., and Bainbridge, D. (1993). México: In Sustainable Agriculture and the Environment in the Human Tropics. Washington, D.C: National Academy Press.
Gómez-Pompa, A., and Del Amo, R. (1994). “Sostenibilidad y Participación Campesina: Un Mecanismo para Establecer un Programa,” in Agroforestería en Desarrollo: Educación, Investigación y Extensión, eds L. Krishnamurthy and J. A. Leos-Rodriguez (Texcoco: Universidad Autónoma Chapingo), ages211–227.
Gómez-Pompa, A., and Kaus, A. (1990). “Traditional Management of Tropical Forests in México,” in Alternatives to Deforestation: Steps Toward Sustainable Use of the Amazon Rain Forest, ed. A. B. Anderson (New York, NY: Columbia University Press), ages45–64.
Gómez-Pompa, A., and Kaus, A. (1992). Taming the wilderness myth: Environmental policy and education are currently based on western beliefs about nature rather than on reality. BioScience 42, 271–279. doi: 10.2307/1311675
Gómez-Pompa, A., and Kaus, A. (1999). From pre-Hispanic to future conservation alternatives: Lessons from Mexico. Proc. Natl. Acad. Sci. U.S.A. 96, 5982–5986. doi: 10.1073/pnas.96.11.5982
Graham, E. (2006). “Due South: Learning from the Tropical Experience,” in Reconstructing the Past: Studies in Mesoamerican and Central American Prehistory, (eds) D. M. Pendergast and A. P. Andrews (England: Hadrian Books), ages151–158.
Guariguata, M. R. (2017). What Are the Knowledge Gaps for Better Understanding of Forest Regrowth in Tropical Human Modified Landscapes? Webinar sponsored by the Society for Ecological Restoration and the Association for Tropical Biology and Conservation. Available online at: https://tropicalbiology.org/webinars/role-secondary-forests/ (accessed October, 2022).
Gunn, J., Folan, W. J., and Robichaux, H. R. (1995). A landscape analysis of the candelaria watershed in méxico: Insights into paleoclimate affecting upland horticulture in the southern yucatán peninsula semi-karst. Geoarchaeology 10, 3–42. doi: 10.1002/gea.3340100103
Handelsman, J. (2021). A World Without Soil: The Past, Present, and Precarious Future of the Earth Beneath Our Feet. New Haven, CT: Yale Univerisyt Press. doi: 10.12987/9780300263107
Hansen, R. D., Bozarth, S., Jacob, J., Wahl, D., and Schreiner, T. (2002). Climatic and environmental variability in the rise of maya civilization: A preliminary perspective from northern petén. Anc. Mesoam. 13, 273–295. doi: 10.1017/S0956536102132093
Harris, S. (1982). “Archaeology and Volcanism,” in Encyclopedia of Volcanoes, ed. H. Sigurdsson (San Diego, CA: Academic Press San Diego), ages1301–1314.
Hartshorn, G. (1989). Application of gap theory to tropical forest management: Natural regeneration on strip clear-cuts in the peruvian Amazon. Ecol. Soc. Am. 70, 567–569. doi: 10.2307/1940208
Haug, G. H., Gunther, D., Peterson, L. C., Sigman, D. M., Hughen, K. A., and Aeschlimann, B. (2003). Climate and the Collapse of Maya Civilization. Science 299, 1731–1735. doi: 10.1126/science.1080444
Haug, G. H., Hughen, K. A., Sigman, D. M., Peterson, L. C., and Rohl, U. (2001). Southward Migration of the Intertropical Convergence Zone through the Holocene. Science 293, 1304–1308. doi: 10.1126/science.1059725
Haviland, W. A. (1969). Tikal, Guatemala and Mesoamerican Urbanism. World Archaeol. 2, 186–196. doi: 10.1080/00438243.1970.9979473
Haviland, W. A. (1972). Family Size, Prehistoric Population Estimates, and the Ancient Maya. Am. Antiq. 37, 135–139. doi: 10.2307/278895
Healy, P. F., Helmke, C. G. B., Awe, J. J., and Sunahara, K. S. (2007). Survey, Settlement, and Population History at the Ancient Maya Site of Pacbitun, Belize. J. Field Archaeol. 32, 17–39. doi: 10.1179/009346907791071737
Healy, P. F., Lambert, J. D., Arnason, J. T., and Hebda, R. J. (1983). Caracol, Belize: Evidence of ancient Maya agricultural terraces. J. Field Archaeol. 10, 397–410. doi: 10.1179/009346983791504200
Hernández Xolocotzi, E., Bello Baltazar, E., and Levy Tacher, S. I. (1995). La Milpa en Yucatán: Un Sistema de Producción Agrícola Tradicional, Vol. 1 y 2. México: Colegio de Postgraduados.
Hillesheim, M. B., Hodell, D. A., Leyden, B. W., Brenner, M., Curtis, J. H., Anselmetti, F. S., et al. (2005). Climate Change in Lowland Central America During the Late Deglacial and Early Holocene. J. Quater. Sci. 20, 363–376. doi: 10.1002/jqs.924
Hodell, D. A., Anselmetti, F. S., Ariztegui, D., Brenner, M., Curtis, J. H., Gilli, A., et al. (2008). An 85-ka Record of Climate Change in Lowland Central America. Quater. Sci. Rev. 27, 1152–1165. doi: 10.1016/j.quascirev.2008.02.008
Hodell, D. A., Brenner, M., Curtis, J. H., and Guilderson, T. (2001). Solar Forcing of Drought Frequency in the Maya Lowlands. Science 292, 1367–1370. doi: 10.1126/science.1057759
Hodell, D. A., Turchyn, A. V., Wiseman, C. J., Escobar, J., Curtis, J. H., Brenner, M., et al. (2012). Late Glacial Temperature and Precipitation Changes in the Lowland Neotropics by Tandem Measurement of d18O in Biogenic Carbonate and Gypsum Hydration Water. Geochimica Cosmochimica Acta 77, 352–368. doi: 10.1016/j.gca.2011.11.026
Hoggarth, J. A., Restall, M., Wood, J. W., and Kennett, D. J. (2015). Drought and Its Demographic Effects in the Maya Lowlands. Curr. Anthropol. 58, 82–113. doi: 10.1086/690046
Hooke, R. L. (2000). On the History of Humans as Geomorphic Agents. Geology 28, 843–846. doi: 10.1130/0091-7613(2000)28<843:OTHOHA>2.0.CO;2
Horn, I. I. I. S. W. (2020). Socioeconomic Networks and the Rise of Maya Civilization: The Web of complexity at Middle Preclassic Cahal Pech, Belize. BAR International Series 3009. Oxford: British Archaeological Reports. doi: 10.30861/9781407357546
Horn, S., Ford, A., and Morales, P. (2019). Lasers, lasers, everywhere -and all the trees did shrink: Reliable methods and results from lidar guided survey at el pilar. Res. Rep. Belizean Archaeol. 16, 143–155.
Houston, S., Robertson, J., and Stuart, D. (2000). The Language of Classic Maya Inscriptions. Curr. Anthropol. 41, 321–356. doi: 10.1086/300142
Hutson, S., Dunning, N. P., Cook, B., Ruhl, T., and Barth, N. (2021). Rural settlement patterns, household cooperation, and regional subsistence interdependency in the Rio Bec area, Mexico: Contributions from G-LiHT. J. Anthropol. Res. 77, 550–579. doi: 10.1086/716750
Iannone, G., Yaeger, J., and Hodell, D. (2014). “Assessing the Great Maya Droughts: Some Critical Issues,” in The Great Maya Droughts in Cultural Context: Case Studies in Resilience and Vulnerability, ed. G. Iannone (Boulder: University Press of Colorado), ages51–70.
Iriarte, J., Elliott, S., Maezumi, S. Y., Alves, D., Gonda, R., Robinson, M., et al. (2020). The origins of Amazonian landscapes: Plant cultivation, domestication and the spread of food production in tropical South America. Quater. Sci. Rev. 248:106582. doi: 10.1016/j.quascirev.2020.106582
Islebe, G. A., Sanchez, O. S., Valdez, M., and Weissenberger, H. (2015). “Distribution of vegetation types,” in Biodiversity and Conservation of the Yucatan Peninsula, eds G. A. Islebe, S. Calmé, J. L. León-Cortés, and B. Schmook (Berlin: Springer International Publishing), age401. doi: 10.1007/978-3-319-06529-8
Islebe, G. A., Torrescano-Valle, N., Aragón-Moreno, A. A., Vela-Peláez, A. A., and Valdez-Hernández, M. (2018). The Paleoanthropocene of the Yucatán Peninsula: Palynological evidence of environmental change. Boletín la Sociedad Geológica Mexicana 70, 49–60. doi: 10.18268/BSGM2018v70n1a3
Jacob, J. S. (1995). “Archaeological Pedology in the Maya Lowlands,” in Pedological Perspectives in Archeological Research, ed. M. Collins (Madison, WI: Soil Science Society of America), ages51–82. doi: 10.2136/sssaspecpub44.c4
James, D., Breitenbach, S., Cheng, H., Hartland, A., Orland, I., Brenner, M., et al. (2020). The maya terminal classic drought replicated in two stalagmites from columnas cave, nw yucatán. Available online at: https://www.sciencegate.app/document/10.5194/egusphere-egu2020-18326
James, D., Carolin, S., Breitenbach, S., Endsley, E., Gallup, C., Brenner, M., et al. (2022). “An ultra-high-resolution seasonal-scale stalagmite palaeoclimate record from the Yucatán peninsula, spanning the Maya Terminal Classic period,” in EGU General Assembly, EGU22–EGU7862. Available online at: https://meetingorganizer.copernicus.org/EGU22/EGU22-7862.html
Johnston, K. J. (2003). The Intensification of Pre-Industrial Cereal Agriculture in the Tropics: Boserup, cultivation lengthening, and the Classic Maya. J. Anthropol. Archaeol. 22, 126–161. doi: 10.1016/S0278-4165(03)00013-8
Jones, G. D. (1998). The Conquest of the Last Maya Kingdom. Redwood City, CA: University of Stanford Press. doi: 10.1515/9780804779326
Jones, L. D. (1986). Lowland Maya Pottery: The Place of Petrological Analysis. Oxford: British Archaeological Reports. doi: 10.30861/9780860543718
Jurskis, V., Underwood, R., and Burrows, N. (2020). How australian aborigines shaped and maintained fire regimes and the biota. Ecol. Evol. Biol. 5, 164–172. doi: 10.11648/j.eeb.20200504.17
Kellman, M., and Tackaberry, R. (1997). Tropical Environments: The Functioning and Management of Tropical Ecosystems. New York, NY: Routledge.
Kelly, R. L., and Thomas, D. H. (2013). Archaeology, 6th Edn. New York, NY: Wadsworth Cengage Learning.
Kennett, D. J., and Beach, T. P. (2013). Archeological and Environmental Lessons for the Anthropocene from the Classic Maya Collapse. Anthropocene 4, 88–100. doi: 10.1016/j.ancene.2013.12.002
Kennett, D. J., Breitenbach, S. F. M., Aquino, V. V., Asmerom, Y., Awe, J., Baldini, J. U. L., et al. (2012). Development and Disintegration of Maya Political Systems in Response to Climate Change. Science 338, 788–791. doi: 10.1126/science.1226299
Kennett, D. J., Hajdas, I., Culleton, B. J., Belmecheri, S., Martin, S., Neff, H., et al. (2013). Correlating the ancient maya and modern european calendars with high-precision AMS 14C dating. Sci Rep. 3:1597. doi: 10.1038/srep01597
Kennett, D. J., Lipson, M., Prufer, K. M., Mora-Marín, D., George, R. J., Rohland, N., et al. (2022). South-to-north migration preceded the advent of intensive farming in the Maya region. Nat. Commun. 13:1530. doi: 10.1038/s41467-022-29158-y
Kennett, D. J., Prufer, K. M., Culleton, B. J., George, R. J., Robinson, M., Trask, W. R., et al. (2020). Early isotopic evidence for maize as a staple grain in the Americas. Sci. Adv. 6:eaba3245. doi: 10.1126/sciadv.aba3245
Kennett, D. J., Voorhies, B., and McClure, S. B. (2002). Los Cerritos: An early fishing-farming community on the pacific coast of México. Antiquity 76, 631–632. doi: 10.1017/S0003598X00090992
Kramer, K. L., and Hackman, J. (2021). Scaling climate change to human behavior predicting good and bad years for Maya farmers. Am. J. Hum. Biol. 33:e23524. doi: 10.1002/ajhb.23524
Lambert, J. D. H., and Arnason, J. T. (1982). Ramon and Maya Ruins: An ecological not an economic relation. Science 216, 298–299. doi: 10.1126/science.216.4543.298
Lentz, D. L., Dunning, N. P., Scarborough, V. L., and Grazioso, L. (2018). Imperial resource management at the ancient Maya city of Tikal: A resilience model of sustainability and collapse. J. Anthropol. Archaeol. 52, 113–122. doi: 10.1016/j.jaa.2018.08.005
Lentz, D. L., Dunning, N., Scarborough, V. L., Magee, K., Thompson, K. M., Weaver, E., et al. (2014). Forests, fields, and the edge of sustainability at the ancient Maya City of Tikal. Proc. Natl. Acad. Sci.U.S.A. 111, 18513–18518. doi: 10.1073/pnas.1408631111
Lentz, D. L., Hamilton, T., Dunning, N. P., Tepe, E., Scarborough, V., Meyers, S., et al. (2021). Environmental DNA reveals arboreal cityscapes at the Ancient Maya Center of Tikal. Sci. Rep. 11:12725. doi: 10.1038/s41598-021-91620-6:12725
Levis, C., Costa, F. R. C., Bongers, F., Peña-Claros, M., Clement, C. R., Junqueira, A. B., et al. (2017). Persistent effects of pre-Columbian plant domestication on Amazonian forest composition. Science 355, 925–931. doi: 10.1126/science.aal0157
Levy Tacher, S. I., and Rivera, J. R. A. (2005). Successional Pathways Derived from Different Vegetation Use Patterns by Lacandon Maya. J. Sustain. Agricult. 26, 49–82. doi: 10.1300/J064v26n01_06
Leyden, B. W. (1984). Guatemalan Forest Synthesis After Pleistocene Aridity. Proc. Natl. Acad. Sci. U.S.A. 81, 4856–4859. doi: 10.1073/pnas.81.15.4856
Leyden, B. W. (1987). Man and Climate in the Maya Lowlands. Quater. Res. 28, 407–417. doi: 10.1016/0033-5894(87)90007-X
Leyden, B. W. (2002). Pollen Evidence for Climatic Variability and Cultural Disturbance in the Maya Lowlands. Ancient Mesoam. 13, 85–101. doi: 10.1017/S0956536102131099
Leyden, B. W., Brenner, M., Hodell, D. A., and Curtis, J. H. (1993). “Late Pleistocene Climate in the Central American Lowlands,” in Climate in Continental Isotopic Records, ed. P. K. Swart (Washington, D.C: American Geophysical Union), agesxiii,374.
Liendo, R., Solleiro-Rebolledo, E., Solis-Castillo, B., Sedov, S., and Ortiz-Perez, A. (2014). “Population Dynamics and Its Relation to Ancient Landscapes in the Northwestern Maya Lowlands: Evaluating Resilience and Vulnerability,” in The Resilience and Vulnerability of Ancient Landscapes: Transforming Maya Archaeology through IHOPE, eds A. F. Chase and V. L. Scarborough (Hoboken, NJ: Wiley), ages84–100. doi: 10.1111/apaa.12031
Lohse, J. C. (2010). Archaic origins of the lowland maya. Latin Am. Antiq. 21, 312–352. doi: 10.7183/1045-6635.21.3.312
Lohse, J. C., Awe, J., Griffith, C., Rosenswig, R. M., and Valdez, F. (2006). Preceramic occupations in Belize: Updating the paleoindian and archaic record. Latin Am. Antiq. 17, 209–226. doi: 10.2307/25063047
Lucero, L. J. (2003). The politics of ritual: The emergence of classic maya rulers. Curr. Anthropol. 44, 523–558. doi: 10.1086/375870
Lucero, L. J., and Larmon, J. (2018). “Climate Change, Mesoamerica, and the Classic Maya Collapse,” in Climate Changes in the Holocene: Impacts and Human Adaptation, ed E. Chiotis (London: CRC Press), ages165–181. doi: 10.1201/9781351260244-8
Lucero, L. J., Fletcher, R., and Coningham, R. (2015). From ‘collapse’ to urban diaspora: The transformation of low-density, dispersed agrarian urbanism. Antiquity 89, 1139–1154. doi: 10.15184/aqy.2015.51
Lundell, C. L. (1978). Some observations from fifty years of exploration of the yucatán Peninsula. Wrightia 6, 1–3.
Machuca, P., Pulido-Salas, M. T., and Trabanino, F. (2020). Past and present of allspice (Pimenta dioica) in Mexico and Guatemala: From traditional management to current large-scale markets. Rev. D’ethnoécol. 18. doi: 10.4000/ethnoecologie.6261
MacNeish, R. S. (1982). Third Annual Report of the Belize Archaic Archaeological Reconnaissance. Robert S. Andover, MA: Peabody Foundation for Archaeology.
Macri, M. J., and Ford, A. (eds) (1997). The Language of Maya Hieroglyphs. San Francisco: Pre-Columbian Art Research Institute.
Marx, W., Haunschild, R., and Bornmann, L. (2017). The role of climate in the collapse of the maya civilization: A bibliometric analysis of the scientific discourse. Climate 5:88. doi: 10.3390/cli5040088
Mathews, J. P. (2019). Chicle: The Chewing Gum of The Americas, From the Ancient Maya to William Wrigley. Tucson, AZ: University of Arizona Press.
McClung de Tapia, E. (1992). “The Origins of Agriculture in Mesoamerica and Central America,” in The Origins of Agriculture: An International Perspective, eds C. W. Cowan and P. J. Watson (Washington, D.C: Smithsonian Institution Press), ages143–171.
McNeil, C. L. (2012). Deforestation, Agroforestry, and Sustainable Land Management Pracitices among the Classic Period Maya. Quater. Int. 249, 19–30. doi: 10.1016/j.quaint.2011.06.055
McNeil, C. L. (2020). “Favored Plants of the Maya,” in The Maya World, eds S. R. Hutson and T. Arden (London: Routledge), ages183–202. doi: 10.4324/9781351029582-13
McNeil, C. L., Burney, D. A., and Burney, L. P. (2010). Evidence disputing deforestation as the cause for the collapse of the ancient maya polity of copan, Honduras. Proc. Natl. Acad. Sci. U.S.A. 107, 1017–1022. doi: 10.1073/pnas.0904760107
Medina-Elizalde, M., and Rohling, E. J. (2012). Collapse of classic maya civilization related to modest reduction in precipitation. Science 335, 956–959. 9 doi: 10.1126/science.1216629
Medina-Elizalde, M., Burns, S. J., Polanco-Martínez, J. M., Beach, T., Lases-Hernández, F., Shen, C.-C., et al. (2016). High-resolution speleothem record of precipitation from the Yucatan Peninsula spanning the Maya Preclassic Period. Glob. Planet. Change 138, 93–102. doi: 10.1016/j.gloplacha.2015.10.003
Medellin, R. A., and Gaona, O. (1999). Seed dispersal by bats and birds in forest and disturbed habitats of Chiapas, Mexico. Biotropica 31, 478–485. doi: 10.1111/j.1744-7429.1999.tb00390.x
Mehringer, J. P. J., Sama-Wojcicki, A. M., Wollwage, L. K., and Sheets, P. (2005). Age and Extent of the Ilopango TBJ Tephra Inferred from a Holocene Chronostratigraphic Reference Section. Lago De Yojoa, Honduras. Quarter. Res. 63, 199–205. doi: 10.1016/j.yqres.2004.09.011
Metcalfe, S. E., Jones, M. D., Davies, S. J., Noren, A., and MacKenzie, A. (2010). Climate Variability over the Last Two Millennia in the North American Monsoon Region, Recorded in Laminated Lake Sediments from Laguna de Juanacatlán, Mexico. Holocene 20, 1195–1206. doi: 10.1177/0959683610371994
Michon, G., De. Foresta, H., Levang, P., and Verdeaux, F. (2007). Domestic Forests: A new paradigm for integrating local communities’ forestry into tropical forest science. Ecol. Soc. 12:1. doi: 10.5751/ES-02058-120201
Mittermeier, R. A., Myers, N., and Mittermeier, C. G. (2000). Hotspots: Earth’s Biologically Richest and Most Endangered Terrestrial Ecoregions. San Pedro Garza García, MX: CEMEX.
Montgomery, D. R. (2007). Soil erosion and agricultural sustainability. Proc. Natl. Acad. Sci.U.S.A. 104, 13268–13272. doi: 10.1073/pnas.0611508104
Morehart, C. T., and Morell-Hart, S. (2015). Beyond the ecofact: Toward a social paleoethnobotany in Mesoamerica. J. Archaeol. Method Theory 22, 483–511. doi: 10.1007/s10816-013-9183-6
Morell-Hart, S., Dussol, L., and Fedick, S. L. (Forthcoming). Lowland Maya Agriculture: Paleoethnobotanical Residues and New Perspectives on Plant Management Journal of Archaeological Research.
Morley, R. J. (2000). Origin and Evolution of Tropical Rain Forests. New York, NY: John Wiley & Sons.
Mottl, O., Flantua, S. G. A., Bhatta, K. P., Felde, V. A., Giesecke, T., Goring, S., et al. (2021). Global acceleration in rates of vegetation change over the past 18,000 years. Science 372, 860–864. doi: 10.1126/science.abg1685
Mt Pleasant, J. (2011). The Paradox of Plows and Productivity: An agronomic comparison of cereal grain production under iroquois hoe culture and european plow culture in the seventeenth and eighteenth centuries. Agricult. Hist. 85, 460–492. doi: 10.3098/ah.2011.85.4.460
Mt Pleasant, J. (2015). A new paradigm for pre-columbian agriculture in North America. Early Am. Stud. 13, 374–412. doi: 10.1353/eam.2015.0016
Mueller, A. D., Anselmetti, F. S., Ariztegui, D., Benner, M., Hillersheim, M. B., Hodell, D. A., et al. (2006). Climate Drying and Forest Decline (~4.0-3.0 calkyrBP) Preceding Sedentary Maya Occupation in the Lowlands of the Peten, Guatemala. Geophys. Res. Abstracts 8:1784.
Mueller, A. D., Anselmetti, F. S., Ariztegui, D., Brenner, M., Hodell, D. A., Curtis, J. H., et al. (2010). Late quaternary palaeoenvironment of northern guatemala: Evidence from deep drill cores and seismic stratigraphy of Lake Petén Itza. Sedimentology 57, 1220–1245. doi: 10.1111/j.1365-3091.2009.01144.x
Mueller, A. D., Islebe, G. A., Hillesheim, M. B., Grzesik, D. A., Anselmetti, F. S., Ariztegui, D., et al. (2009). Climate drying and associated forest decline in the lowlands of northern guatemala during the late Holocene. Quater. Res. 71, 133–141. doi: 10.1016/j.yqres.2008.10.002
Nations, J. D., and Nigh, R. (1980). The evolutionary potential of lacandon maya sustained-yield tropical forest agriculture. J. Anthropol. Res. 36, 1–30. doi: 10.1086/jar.36.1.3629550
Neff, H., Pearsall, D. M., Jones, J. G., Arroyo, B., Collins, S. K., and Freidel, D. E. (2006). Early maya adaptive patterns: Mid-late holocene paleoenvironmental evidence from pacific guatemala. Latin Am. Antiq. 17, 287–315. doi: 10.2307/25063054
Netting, R. M. (1974). Agrarian Ecology. Annu. Rev. Anthropol. 3, 21–56. doi: 10.1146/annurev.an.03.100174.000321
Netting, R. M. (1977). “Maya Subsistence: Mythologies, Analogies, Possibilities,” in The Origins of Maya Civilization, ed. R. E. W. Adams (Albuquerque, NM: University of New Mexico Press), ages299–333.
Netting, R. M. (1989). “Smallholders, Householders, Freeholders: Why the Family Farm Works Well Worldwide,” in Household Economy: Reconsidering the Domestic Mode of Production, ed. R. R. Wilk (Boulder, CO: Westview Press), ages221–244.
Netting, R. M. (1993). Smallholders, Householders: Farm Families and the Ecology of Intensive, Sustainable Agriculture. Redwood City, CA: Stanford University Press. doi: 10.1515/9781503622067
Nigh, R. (2006). How the Maya Built their Landscapes: Agriculture, Successional Management and the Maya Forest in Long-Term Perspective. London: International Congress of Americanists.
Nigh, R. (2008). Trees, fire and farmers: Making woods and soil in the Maya Forest. J. Ethnobiol. 28, 231–243. doi: 10.2993/0278-0771-28.2.231
Nigh, R., and Diemont, S. (2013). The maya milpa: Fire and the legacy of living soil. Front. Ecol. Environ. 11, 45–54. doi: 10.1890/120344
Nooren, C. A. M., Hoek, W. Z., Tebbens, L. A., and Martin Del Pozzo, A. L. (2009). Tephrochronological Evidence for the Late Holocene Eruption History of El Chichón Volcano, México. Geofísica Int. 48, 97–112. doi: 10.22201/igeof.00167169p.2009.48.1.102
Nooren, K., Middelkoop, H., Nienhuis, J., and Hoek, W.Z. (2017). Holocene evolution of the Tabasco Delta-Mexico: Impact of Climate, Volcanism, and Humans. Utrecht: Ultrecht University.
Ollerton, J., Winfree, R., and Tarrant, S. (2011). How Many Flowering Plants are Pollinated by Animals? Oikos 120, 321–326. doi: 10.1111/j.1600-0706.2010.18644.x
Oswald, W. W., Foster, D. R., Shuman, B. N., Chilton, E. S., Doucette, D. L., and Duranleau, D. L. (2020). Conservation implications of limited Native American impacts in pre-contact New England. Nat. Sustain. 3, 241–246. doi: 10.1038/s41893-019-0466-0
Palerm, A. (1967). “Agricultural Systems and Food Patterns,” in Handbook of Middle American Indians, eds R. Wauchope and M. Nash (Austin, TX: University of Texas Press), ages26–52. doi: 10.7560/736665-004
Palerm, A. (1976). “Agriculture and Food Patterns,” in Handbook of Middle American Indians, eds R. Wauchope and N. Manning (Austin, TX: University of Texas Press), ages26–52.
Peters, C. M. (2000). “Precolumbian Silviculture and Indigenous Management of Neotropical Forests,” in Imperfect Balance: Landscape Transformations in the Precolumbian Americas, ed. D. L. Lentz (New York, NY: Columbia University Press), ages203–223. doi: 10.7312/lent11156-011
Peterson, J. B., Neves, E. G., and Heckenberger, M. J. (2001). “Gift from the Past: Terra Preta and Prehistoric Amerindian Occupation in Amazonia,” in Unknown Amazon: Culture in Nature in Ancient Brazil, ed. C. McEwan, C. Barreto, and EG. Neves (London: British Museum), ages86–105.
Peterson, L. C., and Haug, G. H. (2005). Climate and the collapse of maya civilization: A series of multi-year droughts helped to doom an ancient culture. Am. Sci. 93, 322–329. doi: 10.1511/2005.54.968
Piperno, D. (2011). “Prehistoric Human Occupation and Impacts on Neotropical Forest Landscapes During the Late Pleistocene and Early/Middle Holocene,” in Tropical Rainforest Response to Climatic Change, eds M. B. Bush, J. R. Flenley, and W. D. Gosling (Cambridge: Cambridge University Press). doi: 10.1007/978-3-642-05383-2_6
Piperno, D. R. (2006). Quaternary Environmental History and Agricultural Impact on Vegetation in Central America. Ann. Mo. Bot. Gard. 93, 274–296. doi: 10.3417/0026-6493(2006)93[274:QEHAAI]2.0.CO;2
Piperno, D. R., and Pearsall, D. M. (1998). The Origins of Agriculture in the Lowland Neotropics. San Diego, CA: Academic Press.
Piperno, D. R., and Stothert, K. E. (2003). Phytolith Evidence for Early Holocene Cucurbita Domestication in Southwest Ecuador. Science 299, 1054–1057. doi: 10.1126/science.1080365
Pohl, M. D., Pope, K. O., Jones, J. G., Jacob, J. S., Piperno, D. R., deFrance, S. D., et al. (1996). Early agriculture in the Maya Lowlands. Latin Am. Antiq. 7, 355–372. doi: 10.2307/972264
Pohl, M. E. D., Piperno, D. R., Pope, K. O., and Jones, J. G. (2007). Microfossil Evidence for Pre-Columbian Maize Dispersals in the Neotropics from San Andrés, Tabasco, México. Proc. Natl. Acad. Sci. U.S.A. 104, 6870–6875. doi: 10.1073/pnas.0701425104
Pope, K. O., Pohl, M. E., Jones, J. G., Lentz, D. L., Nagy, C. V., Vega, F. J., et al. (2001). Origin and Environmental Setting of Ancient Agriculture in the Lowlands of Mesoamerica. Science 292, 1370–1373. doi: 10.1126/science.292.5520.1370
Primack, R., Bray, D., Galleti, H., and Ponciano, I. (1998). Timber, Tourists, and Temples: Conservation and Development in the Maya Forest of Belize, Guatemala, and México. Washington, D.C: Island Press.
Prufer, K., Robinson, M., and Kennett, D. (2021). Terminal pleistocene through middle holocene occupations in southeastern mesoamerica: Linking ecology and culture in the context of neotropical foragers and Early Farmers. Ancient Mesoam. 32, 439–460. doi: 10.1017/S0956536121000195
Puleston, D. E. (1968). Brosimum Alicastrum as a Subsistence Alternative for the Classic Maya of the Central Southern Lowlands. Ph.D thesis. Philadelphia, PA: University of Pennsylvania.
Puleston, D. E. (1973). Ancient Maya Settlement Patterns and Environment at Tikal, Guatemala: Implications for Subsistence Models. Ph.D thesis. Philadelphia, PA: University of Pennsylvania.
Puleston, D. E. (1983). Tikal Report No. 13: The Settlement Survey of Tikal. Philadelphia, PA: University of Pennsylvania. doi: 10.9783/9781934536353
Puleston, D. E., and Puleston, O. (1972). “A Processual Model for the Rise of Classic Maya Civilization in the Southern Lowlands,” in 40th International Congress of Americanists, (Genoa: Roma).
Quintana-Ascencio, P. F., Gonzales-Espinosa, M., Ramiraz-Marcial, N., Domínguez-Vázquez, G., and Martinez-Ico, M. (1996). Soil seed banks and regeneration of tropical rain forest from milpa fields at the selva lacandona. Chiapas, México. Biotropica 28, 192–209. doi: 10.2307/2389074
Reese-Taylor, K., Hernández, A. A., Esquivel, F. C. A. F., Monteleone, K., Uriarte, A., Carr, C., et al. (2016). Boots on the ground at yaxnohcah: Ground-truthing lidar in a complex tropical landscape. Adv. Archaeol. Pract. 4, 314–338. doi: 10.7183/2326-3768.4.3.314
Reina, R. E. (1967). Milpas and Milperos: Implications for prehistoric times. Am. Anthropol. 69, 1–20. doi: 10.1525/aa.1967.69.1.02a00020
Renssen, H., Seppä, H., Heiri, O., Roche, D. M., Goosse, H., and Fichefet, T. (2009). The spatial and temporal complexity of the Holocene thermal maximum. Nat. Geosci. 2, 411–414. doi: 10.1038/ngeo513
Rice, D. S. (1976). The Historical Ecology of Lakes Yaxhá and Sacnab, El Petén, Guatemala. Ph.D thesis. State College, PA: Pennsylvania State University.
Rice, D. S. (1996). “Paleolimnological Analysis in the Central Petén, Guatemala,” in The Managed Mosaic: Ancient Maya Agriculture and Resource Use, ed. S. L. Fedick (Salt Lake City, UT: University of Utah Press), ages193–206.
Rice, D. S., and Rice, P. M. (1990). “Population Size and Population Change in the Central Peten Lakes Region, Guatemala,” in Precolumbian Population History in the Maya Lowlands, eds T. P. Culbert and D. S. Rice (Albuquerque, NM: University of New Mexico Press), ages123–148.
Roberts, P. (2020). Tropical Forests in Prehistory, History, and Modernity. Oxford: Oxford University Press. doi: 10.1093/oso/9780198818496.003.0005
Roberts, P., Hamilton, R., and Piperno, D. R. (2021). Tropical forests as key sites of the “Anthropocene”: Past and present perspectives. Proc. Natl. Acad. Sci. U.S.A. 118:e2109243118. doi: 10.1073/pnas.2109243118
Robin, C. (ed.) (2012). Chan: An Ancient Maya Farming Community. Gainesville: University Press of Florida. doi: 10.5744/florida/9780813039831.001.0001
Robinson, M. E., and McKillop, H. I. (2013). Ancient Maya wood selection and forest exploitation: A view from the Paynes Creek salt works, Belize. J. Archaeol. Sci. 40, 3584–3595. doi: 10.1016/j.jas.2013.04.028
Robinson, M., De Souza, J. G., Maezumi, S. Y., Cárdenas, M., Pessenda, L., Prufer, K., et al. (2018). Uncoupling human and climate drivers of late Holocene vegetation change in southern Brazil. Sci. Rep. 8:7800. doi: 10.1038/s41598-018-24429-5
Roman, S., Palmerc, E., and Bredea, M. (2018). The Dynamics of Human–Environment Interactions in the Collapse of the Classic Maya. Ecol. Econ. 146, 312–324. doi: 10.1016/j.ecolecon.2017.11.007
Rosen, A. M. (2007). Civilizing Climate: Social Responses to Climate Change in the Ancient Near East. Lanham: AltaMira Press.
Rosenmeier, M. F., Hodell, D. A., Brenner, M., Curtis, J. H., Martin, J. B., Anselmetti, F. S., et al. (2002). Influence of Vegetation Change on Watershed Hydrology: Implications for Paleoclimatic Interpretation of Lacustrine 8180 Records. J. Paleolimnol. 27, 117–131. doi: 10.1023/A:1013535930777
Rosenswig, R. M. (2006a). Sedentism and Food Production in Early Complex Societies of Soconusco. Mexico. World Archaeol. 38, 330–355. doi: 10.1080/00438240600694115
Rosenswig, R. M. (2006b). Northern Belize and the Soconusco: A comparison of the late archaic to formative transition. Res. Rep. Belizean Archaeol. 3, 59–71.
Rosenswig, R. M. (2021). Opinions on the Lowland Maya Late Archaic Period with Some Evidence from Northern Belize. Anc. Mesoam. 32, 461–474. doi: 10.1017/S0956536121000018
Ross, N. J. (2011). Modern Tree Species Composition Reflects Ancient Maya “Forest Gardens” in Northwest Belize. Ecol. Appl. 21, 75–84. doi: 10.1890/09-0662.1
Roys, R. L. (1952). Conquest Sites and Subsequent Destruction of Maya Architecture in the Interior of Northern Yucatán. Contr. Am. Anthropol. Hist. 11, 129–182.
Rushton, E. A. C., Whitney, B. S., and Metcalfe, S. E. (2020). A tale of maize, palm, and pine: Changing socio-ecological interactions from pre-classic maya to the present day in Belize. Quaternary 3:30. doi: 10.3390/quat3040030
Russell, W. M. S. (1988). Population, swidden farming and the tropical environment. Popul. Environ. 10, 77–94. doi: 10.1007/BF01359134
Sánchez-Suárez, A., Cervera-Montejano, M. D., Victoria-Ojeda, J., and Castillo-Burguete, M. T. (2021). Amarrando los saberes. Mérida: Universidad Autónoma de Yucatán.
Scarborough, V. L. (1993). “Water Management in the Southern Maya Lowlands: An Accretive Model for the Engineered Landscape,” in Economic Aspects of Water Management in the Prehispanic New World Greenwich, eds V. L. Scarborough and B. L. Isaac (Greenwich: JAI Press), ages17–69.
Scarborough, V. L. (2003). How to Interpret an Ancient Landscape. Proc. Natl. Acad. Sci. U.S.A. 100, 4366–4368. doi: 10.1073/pnas.0831134100
Schreinemachers, P., and Berger, T. (2011). An Agent-based Simulation Model of Human Environment Interactions in Agricultural Systems. Environ. Model. Softw. 26, 845–859. doi: 10.1016/j.envsoft.2011.02.004
Schulze, M. D., and Whitacre, D. F. (1999). A Classification and Ordination of The Tree Community of Tikal National Park, Petén, Guatemala. Bull. Fla. Mus. Nat. Hist. 41, 169–297.
Schüpbach, S., Kirchgeorg, T., Colombaroli, D., Beffa, G., Radaelli, M., Kehrwald, N. M., et al. (2015). Combining charcoal sediment and molecular markers to infer a Holocene fire history in the Maya Lowlands of Petén, Guatemala. Quat. Sci. Rev. 115, 123–131. doi: 10.1016/j.quascirev.2015.03.004
Schwartz, N. B. (1990). Forest Society: A Social History of Petén, Guatemala. Philadelphia: University of Pennsylvania Press. doi: 10.9783/9781512806786
Schwartz, N. B., and Márquez, A. R. C. (2015). Swidden Counts: A petén, guatemala milpa system: Production, carrying capacity and sustainability in the southern Maya Lowlands. J. Anthropol. Res. 71, 69–93. doi: 10.3998/jar.0521004.0071.104
Scott, J. C. (2009). The Art of Not Being Governed: An Anarchist History of Upland Southeast Asia. New Haven: Yale Univeresity Press.
Sharer, R. J., and Traxler, L. P. (2006). The Ancient Maya, 6th Edn. Redwood City: Stanford University Press. doi: 10.1515/9781503624221
Sheets, P. (2001). “The Effects of Explosive Volcanism on Simple to Complex Societies in Ancient Middle America,” in Interhemispheric Climate Linkages, ed. V. Markgraf (San Diego: Academic Press), ages73–86. doi: 10.1016/B978-012472670-3/50008-2
Sheets, P., Lentz, D. L., Piperno, D., Jones, J. G., Dixon, C., Maloof, G., et al. (2012). Ancient manioc agriculture south of the Cerén Village, El Salvador. Lat. Am. Antiq. 23, 259–281. doi: 10.7183/1045-6635.23.3.259
Siebe, C. (2000). Age and Archaeological Implications of Xitle Volcano, Southwestern Basin of México-City. J. Volcanol. Geotherm. Res. 104, 45–64. doi: 10.1016/S0377-0273(00)00199-2
Simmons, M. P., and Brem, G. F. (1979). The Analysis and Distribution of Volcanic Ash-Tempered Pottery in the Lowland Maya Area. Am. Antiq. 44, 79–91. doi: 10.2307/279191
Slotten, V., Lentz, D., and Sheets, P. (2020). Landscape management and polyculture in the ancient gardens and fields at Joya de Cerén. El Salvador. J. Anthropol. Archaeol. 59:101191. doi: 10.1016/j.jaa.2020.101191
Smalley, J., and Blake, M. (2003). Sweet beginnings: Stalk sugar and the domestication of Maize. Curr. Anthropol. 44, 675–703. doi: 10.1086/377664
Smith, B. D. (1998). Origins of agriculture enhanced: Between foraging and farming. Science 279, 1651–1652. doi: 10.1126/science.279.5357.1651
Smith, D. A. (2005). Garden Game: Shifting cultivation. indigenous hunting and wildlife ecology in Western Panama. Hum. Ecol. 33, 505–537. doi: 10.1007/s10745-005-5157-Y
Smithsonian Institution (2022). Global volcanism holocene volcano list, department of mineral sciences, national museum of natural history. Available online at: https://volcano.si.edu/volcanolist_holocene.cfm (Accessed October 2022).
Šprajc, I., Dunning, N. P., Štajdohar, J., Gómez, Q. H., López, I. C., Marsetič, A., et al. (2021). Ancient Maya Water Management, Agriculture, and Society in the Area of Chactún, Campeche, Mexico. J. Anthropol. Archaeol. 61:101261. doi: 10.1016/j.jaa.2020.101261
Steele, J., Adams, J., and Sluckin, T. (1998). Modelling Paleoindian Dispersals. World Archaeol. 30, 285–305. doi: 10.1080/00438243.1998.9980411
Steggerda, M. (1943). Some Ethnological Data Concerning One Hundred Yucatan Plants. Washington, DC: Bureau of American Ethnology.
Stemp, W. J., and Harrison-Buck, E. (2019). Pre-Maya Lithic Technology in the Wetlands of Belize: The chipped stone from Crawford Bank. Lithic Technol. 44, 183–198. doi: 10.1080/01977261.2019.1629173
Stemp, W., Awe, J., Marcus, J., Helmke, C., and Sullivan, L. (2021). The preceramic and early ceramic periods in Belize and the central Maya Lowlands. Anc. Mesoam. 32, 416–438. doi: 10.1017/S0956536121000444
Stone, G. D., and Downum, C. E. (1999). Non-Boserupian ecology and agricultural risk: Ethnic politics and land control in the Arid Southwest. Am. Anthropol. 101, 113–128. doi: 10.1525/aa.1999.101.1.113
Sumberg, J., and Giller, K. E. (2022). What is ‘conventional’ agriculture? Glob. Food Sec. 32:100617. doi: 10.1016/j.gfs.2022.100617
Sunahara, K. S. (2003). Ancient Maya Ceramic Economy in the Belize River Valley Region: Petrographic Analyses, Ph.D thesis. Hamilton: McMaster University.
Tankersley, K. B., Dunning, N. P., Scarborough, V., Huff, W. D., Lentz, D. L., and Carr, C. (2016). Catastrophic volcanism and its implication for agriculture in the Maya Lowlands. J. Archaeol. Sci. 5, 465–470. doi: 10.1016/j.jasrep.2015.12.019
Tankersley, K. B., Scarborough, V. L., Dunning, N., Huff, W., Maynard, B., and Gerke, T. L. (2011). Evidence for Volcanic Ash Fall in the Maya Lowlands from a Reservoir at Tikal. Guatemala. J. Archaeol. Sci. 38, 2925–2938. doi: 10.1016/j.jas.2011.05.025
Terán, S., and Rasmussen, C. H. (1994). La Milpa de los Mayas: La Agricultura de los Mayas Prehispánicas y Actuales en el Noreste de Yucatán. Mèrida: Gobierno del Estado de Yucatàn.
Terán, S., and Rasmussen, C. H. (1995). Genetic diversity and agricultural strategy in 16th century and present-day yucatecan milpa agriculture. Biodivers. Conserv. 4, 363–381. doi: 10.1007/BF00058422
Terán, S., Rasmussen, C. H., and Cauich, O. M. (1998). Las Plantas de la Milpa Entre los Mayas: Etnobotánica de las Plantas Cultivadas por Campesinos Mayas en las Milpas del Noreste de Yucatán, México. Yucatán: Fundación Tun Ben Kin, A.C.
Thompson, K. (2013). Biodiversity in Forests of the Ancient Maya Lowlands and Genetic Variation in a Dominant Tree, Manilkara zapota (Sapotaceae): Ecological and Anthropogenic Implications. Ann Arbor: ProQuest Dissertations Publishing.
Thompson, K. P., Hood, A., Cavallaro, D., and Lentz, D. L. (2015). “Connecting Contemporary Ecology and Ethnobotany to Ancient Plant Use Practices of the Maya at Tikal,” in Tikal: Paleoecology of an Ancient Maya City, eds D. L. Lentz, N. P. Dunning, and V. L. Scarborough (New York, NY: Cambridge University Press), ages124–151. doi: 10.1017/CBO9781139227209.008
TNC (2021). Maya forest. Available online at: https://www.nature.org/en-us/about-us/where-we-work/latin-america/mexico/maya-forest/.
Toledo, V. M., Ortiz Espejel, B., Cortés, L., Moguel, P., and Ordoñez, M. D. J. (2003). The multiple use of tropical forests by indigenous peoples in México: A case of adaptive management. Conserv. Ecol. 7:9. doi: 10.5751/ES-00524-070309
Topsey, C. E., Ford, A., and Horn, S. (2020). Different ways of knowing and a different way of being: On a path to reawakening legacy of the Maya Forest. Heritage 3, 493–510. doi: 10.3390/heritage3020029
Torrescano, N., and Islebe, G. A. (2015). Holocene Paleoecology, Climate History and Human Influence in the Southwestern Yucatan Peninsula. Rev. Palaeobot. Palynol. 217, 1–8. doi: 10.1016/j.revpalbo.2015.03.003
Torrescano, N., Gerald, I., and Roy, P. (eds) (2019). The Holocene and Anthropocene Environmental History of Mexico. Berlin: Springer. doi: 10.1007/978-3-030-31719-5
Trabanino, F. (2012). “Sistema de manejo del bosque tropical en Chinikihá a través de la etnoecología y la paleoetnobotánica,” in XXV Simposio de Investigaciones Arqueológicas, eds B. Arroyo and H. E. Mejía (Madrid: Ministerio de Cultura y Deportes), ages798–804.
Trabanino-Garcia, F. (2014). El Uso de las Plantas y el Manejo de la Selva por los Antiguos Mayas de Chinikiha: Interacciones Sociedad y Medio Ambiente a Traves de la Paleoetnobotanica y de la Antracologia. Mexico City: Universidada Nacional Autonoma de Mexico.
Turner, B. L. (1990). “Population Reconstruction of the Central Maya Lowlands: 1000 B.C. to A.D. 1500,” in Precolumbian Population History in the Maya Lowlands, eds T. P. Culbert and D. S. Rice (Albuquerque: University of New Mexico Press).
Turner, B. L., and Miksicek, C. H. (1984). Economic plant species associated with prehistoric agriculture in the Maya Lowlands. Econ. Bot. 38, 179–193. doi: 10.1007/BF02858831
Turner, B. L., and Sabloff, J. A. (2012). Classic period collapse of the central Maya Lowlands: Insights about human–environment relationships for sustainability. Proc. Natl. Acad. Sci. U.S.A. 109, 13908–13914. doi: 10.1073/pnas.1210106109
Tuxill, J. (2004). “Effects of a Regional Drought on Local Management of Seed Stocks of Maize, Beans, and Squash in Central Yucatan State, Mexico: Preliminary Findings,” in Manejo de la Diversidad de los Cultivos en los Agroecosistemas Tradicionales, eds J. L. Ch⋅vez-Servia, J. Tuxill, and D. I. Jarvis (Cali Columbia: Instituto Internacional de Recursos Fitogenéticos).
Tuxill, J., Reyes, L. A., Moreno, L. L., Uicab, V. C., and Jarvis, D. I. (2010). “All Maize Is Not Equal: Maize Variety Choice sand Mayan Foodways in Rural Yucatan, Mexico,” in Pre-columbian Foodways: Interdiscipilanary Approaches to Food, Culture, and Markets in Ancient Mesoamerica, eds J. E. Staller and M. D. Carrasco (Berlin: Springer Science). doi: 10.1007/978-1-4419-0471-3_19
Tzul, A. (2018). Reflections of an agricultural extension officer in belize. Santa Barbara, CA: MesoAmerican Research Center.
Valdés Bérriz, M. (2015). Dispersal of brosimum alicastrum seeds by tent-roosting bats and its relation to germination and seedling survival in the lacandon forest, Chiapas. Rufford Foundation. Available online at: https://www.rufford.org/projects/maripaula-vald%C3%A9s-b%C3%A9rriz/dispersal-of-brosimum-alicastrum-seeds-by-tent-roosting-bats-and-its-relation-to-germination-and-seedling-survival-in-the-lacandon-forest-chiapas/
Valdez, F., Sullivan, L., Buttles, P., and Aebersold, L. (2021). The Origins and Identification of the Early Maya from Colha and Northern Belize. Anc. Mesoam. 32, 502–518. doi: 10.1017/S0956536121000468
Van Vliet, N., Mertz, O., Birch-Thomsen, T., and Schmook, B. (2013). Is There a Continuing Rationale for Swidden Cultivation in the 21st Century? Hum. Ecol. 41, 1–5. doi: 10.1007/s10745-013-9562-3
Vanderwarker, A. M. (2006). Farming, Hunting, and Fishing in the Olmec World. Austin: University of Texas Press. doi: 10.7560/709805
Varela Scherrer, C., and Liendo Stuardo, R. (2021). Aprovechamiento Del Paisaje Y Manejo De La Fauna En Palenque, Chiapas. Anc. Mesoam. 33, 294–308. doi: 10.1017/S095653612100002X
Vaughan, H. H., Deevey, E. S. Jr., and Garrett-Jones, S. E. (1985). “Pollen Stratigraphy of Two Cores from the Petén Lake District, With an Appendix on Two Deep-Water Cores,” in Prehistoric Lowland Maya Environment and Subsistence Economy, ed. M. Pohl (Cambridge: Harvard University Press), 73–89.
Vela-Pelaez, A. A., Torrescano-Valle, N., Islebe, G. A., Mas, J. F., and Weissenberger, H. (2018). Holocene precipitation changes in the Maya forest, Yucatán peninsula, Mexico. Palaeogeogr. Palaeoclimatol. Palaeoecol. 505, 42–52. doi: 10.1016/j.palaeo.2018.05.024
Villa Rojas, A. (1945). The Maya of East Central Quintana Roo. Washington, D.C: Carnegie Institution.
Voorhies, B. (1998). “The Transformation from Foraging to Farming in Lowland Mesoamerica,” in The Managed Mosaic: Ancient Maya Agriculture and Resource Use, ed. S. L. Fedick (Salt Lake City: University of Utah Press), 17–29.
Voorhies, B. (2004). Coastal Collectors in the Holocene: The Chantuto People of Southwest Mexico. Gainesville: University Press of Florida.
Wahl, D., Anderson, L., Estrada-Belli, F., and Tokovinine, A. (2019). Palaeoenvironmental, epigraphic and archaeological evidence of total warfare among the Classic Maya. Nat. Hum. Behav. 3, 1049–1054. doi: 10.1038/s41562-019-0671-x
Wahl, D., Byrne, R., and Anderson, L. (2014). An 8700 year paleoclimate reconstruction from the southern Maya lowlands. Quat. Sci. Rev. 103, 19–25. doi: 10.1016/j.quascirev.2014.08.004
Wahl, D., Byrne, R., Schreiner, T., and Hansen, R. (2006). Holocene Vegetation Change in the Northern Petén and its Implications for Maya Prehistory. Quat. Res. 65, 380–389. doi: 10.1016/j.yqres.2005.10.004
Wahl, D., Schreiner, T., Byrne, R., and Hansen, R. (2007). A Paleoecological Record from a Late Classic Maya Reservoir in the North Peten. Lat. Am. Antiq. 18, 212–222. doi: 10.2307/25063105
Webster, D. (2002). The Fall of the Ancient Maya: Solving the Mystery of the Maya Collapse. London: Thames & Hudson, Ltd.
Webster, D. (2014). “Drought, Collapse, and Niche Inheritance,” in The Great Maya Droughts in Cultural Context: Case Studies in Resilience and Vulnerability, ed. G. Iannone (Boulder: University Press of Colorado), 333–358.
Webster, J. W., Brook, G. A., Railsback, L. B., Cheng, H., Edwards, R. L., Alexander, C., et al. (2007). Stalagmite evidence from Belize indicating significant droughts at the time of Preclassic Abandonment, the Maya Hiatus, and the Classic Maya collapse. Palaeogeogr. Palaeoclimatol. Palaeoecol. 250, 1–17. doi: 10.1016/j.palaeo.2007.02.022
Wernecke, D. C. (1994). Aspects of Urban Design in an Ancient Maya Center: El Pilar, Belize. Degree in the Master of Arts. Boca Raton: Florida Atlantic University.
Wernecke, D. C. (2005). A stone canvas: Interpreting maya building materials and construction technology. Ph. D. dissertation. Austin, TX: University of Texas.
West, R. C. (1964). “Surface Configuration and Associated Geology of Middle America,” in R. C. West (ed.), Handbook of Middle American Indians, Vol. 1: Natural Environment and Early Cultures. (University of Texas Press: Austin). doi: 10.7560/732599-002
White, D. A., and Hood, C. S. (2004). Vegetation Patterns and Environmental Gradients in Tropical Dry Forests of the Northern Yucatán Peninsula. J. Veg. Sci. 15, 151–160. doi: 10.1111/j.1654-1103.2004.tb02250.x
Whitmore, T. M., and Turner, B. L.II (2005). Cultivated Landscapes of Middle America on the Eve of Conquest. Oxford: Oxford University Press.
Wilken, G. C. (1971). Food-Producing Systems Available to the Ancient Maya. Am. Antiq. 36, 432–448. doi: 10.2307/278462
Wilken, G. C. (1987). Good Farmers: Traditional Agricultural Resource Management in México and Central America. Berkeley: University of California Press.
Wrobel, G., Hoggarth, J., and Marshall, A. (2021). Before the Maya: A review of paleoindian and archaic human skeletons found in the Maya Region. Anc. Mesoam. 32, 475–485. doi: 10.1017/S0956536121000250
Yaeger, J. (2020). “Collapse, transformation, reorganization The Terminal Classic transition in the Maya world,” The Terminal Classic transition in the Maya world. 777-793 in S. R. Hutson and T. Ardren eds (Routledge: London). doi: 10.4324/9781351029582-47
Zetina Gutiérrez, M. D. G. (2007). Ecología Humana de las Rancherías de Pich, Campeche: Un Análisis Diacrónic. Ph.D thesis. Yuanlin City: Merida.
Keywords: Maya forest, ancient Maya, tropical land use, pollen, paleoecology
Citation: Ford A (2022) Scrutinizing the paleoecological record of the Maya forest. Front. Ecol. Evol. 10:868660. doi: 10.3389/fevo.2022.868660
Received: 03 February 2022; Accepted: 26 September 2022;
Published: 22 November 2022.
Edited by:
Gerald Alexander Islebe, The South Border College (ECOSUR), MexicoReviewed by:
Heather McKillop, Louisiana State University, United StatesNicholas Dunning, University of Cincinnati, United States
Scott Fedick, University of California, Riverside, CA, United States
Copyright © 2022 Ford. This is an open-access article distributed under the terms of the Creative Commons Attribution License (CC BY). The use, distribution or reproduction in other forums is permitted, provided the original author(s) and the copyright owner(s) are credited and that the original publication in this journal is cited, in accordance with accepted academic practice. No use, distribution or reproduction is permitted which does not comply with these terms.
*Correspondence: Anabel Ford, Zm9yZEB1Y3NiLmVkdQ==