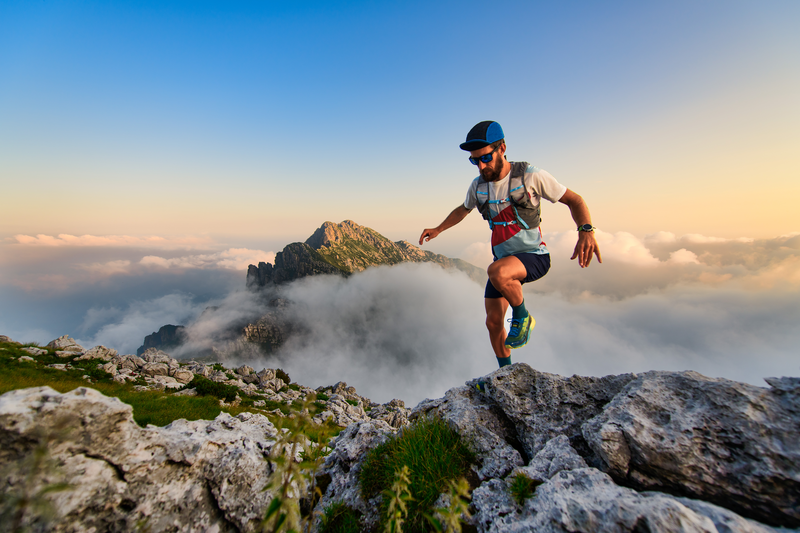
95% of researchers rate our articles as excellent or good
Learn more about the work of our research integrity team to safeguard the quality of each article we publish.
Find out more
ORIGINAL RESEARCH article
Front. Ecol. Evol. , 15 December 2022
Sec. Conservation and Restoration Ecology
Volume 10 - 2022 | https://doi.org/10.3389/fevo.2022.866947
This article is part of the Research Topic Conservation of Invertebrates in Agricultural Landscapes View all 13 articles
Agri-environment schemes (AES) are expected to counteract the negative impacts of intensive agriculture on biodiversity. These schemes were specifically designed to target farmland biodiversity and included, for instance, ecological focus areas (EFAs). In Switzerland, in order to qualify for direct payments, farmers must manage 7% or more of their land as biodiversity promotion areas (BPAs). BPAs encompass extensively managed and low intensity hay meadows, fallows (wildflower strips), traditional orchards with high-stem trees and hedgerows. Evaluation of AES delivery for biodiversity is of crucial importance but must be performed across several years and considering the various components of species diversity to avoid incomplete or wrong conclusions. From a complex study design comprising 478 fields in three regions and sampling over 7 years with four sampling times, spider assemblages of BPA habitats were compared to corresponding conventionally managed fields. A battery of investigations was performed including alpha- and beta-diversity analysis, multivariate dispersion, indicator species and species specificity to understand what BPAs deliver for spiders in the habitat scale and farming landscape. Results showed that alpha-diversity (average number of species) was usually higher in BPA habitats than in conventionally managed fields but the species composition (beta-diversity) had more power to perceive AES impact. Furthermore, the various environmental conditions of BPAs in the farming landscape led to highly diverse spider assemblages (multivariate dispersion) emphasizing that not only the agricultural management plays a role in determining species diversity but the environmental heterogeneity. Indicator (and rare) species were mostly found in woody BPAs (hedges and high-stem tree orchards) revealing the high importance of these BPA habitats for spider conservation. At regional scale, BPA hedges contributed most to the regional diversity of spiders in grassland and mixed regions while BPA meadows and wildflower strip BPAs were first delivering in the region of arable crops. Recommendations highlight the role of the woody habitats and of the environmental heterogeneity in the farming landscape as well as of regional planning to make AES effective.
Agriculture occupies about 34% of the total land area of the member countries of OECD and more than 50% for about half of the member countries, most of them European (OECD, 2008). These figures explain why Europe’s biodiversity is inextricably linked to agriculture and agricultural practices. Yet, agricultural intensification is among the main drivers of biodiversity loss in the last decades (Diaz et al., 2019). The main impacts resulting from modern agriculture are related to habitat loss and fragmentation as well as environmental degradation, which reduces the amount of habitat available to sustain wild species in farming landscapes (Dudley and Alexander, 2017). To counteract these negative impacts of agriculture, agri-environment schemes (AES) offered by the OECD member countries were designed to specifically target farmland biodiversity (OECD, 2011). AES are considered the most important policy instruments to protect biodiversity in agricultural landscapes and are associated with considerable spending (Herzog et al., 2005; Batáry et al., 2015). However, as they seem to have had inconsistent environmental success (Batáry et al., 2010; Mccracken et al., 2015; Biffi et al., 2021) there is a need for evaluation and improvement (Peer et al., 2019; Candel et al., 2021; Tyllianakis and Martin-Ortega, 2021).
In Switzerland, an AES scheme comprising biodiversity promotion areas (BPA; Supplementary Table 1) was introduced in year 1993 and perpetuated in a cross-compliance mechanism since 1999. In order to qualify for direct payments, farmers must manage 7% or more of their land as biodiversity promotion areas including extensively managed and low intensity hay meadows, traditional orchards with high-stem trees, fallows (sown with seed mixtures of 20 to 40 herbaceous plant species), and hedgerows. These are the most important and frequent BPAs implemented in Switzerland, and their management is strictly regulated (late cut of meadows, restrictions in fertilization, pesticide use, etc.) in order to achieve environmental goals.
The role of AES in preserving and promoting biodiversity has been subject to debate in Europe, since their success seems to be landscape and context-dependent (Kleijn et al., 2006; Batáry et al., 2010; Whittingham, 2011; Martínez-Núñez et al., 2020). In Switzerland, diverse evaluation projects have been conducted to assess the effectiveness of the BPA scheme for several taxa (vascular plants, bryophytes, birds, hares, pollinators, bugs, butterflies, carabid beetles, spiders and grasshoppers) and results have been published (Jeanneret et al., 2003a; Herzog et al., 2005; Knop et al., 2006; Birrer et al., 2007; Roth et al., 2008; Aviron et al., 2009; Albrecht et al., 2010; Riedel et al., 2019; Ravetto Enri et al., 2020; Bisang et al., 2021). Almost all studies showed that AES can be effective in protecting and promoting biodiversity. Still, the effect also depends on the group of organisms investigated and on the landscape context.
Most evaluations of the effectiveness of AES have traditionally consisted of assessing in situ biodiversity in fields under scheme compared to conventionally managed control fields. However, unproductive and less productive perennial woody elements such as orchards with high-stem trees and hedgerows have received little attention although they may play a substantial role in preserving biodiversity (Garratt et al., 2017). Also, most of our knowledge about the effectiveness of AES to date comes from single year studies, which does not consider temporal variation and the possible ecological time lag in community responses (time necessary for species to respond to conservation measures; Watts et al., 2020). Therefore, studies addressing the effectiveness of AES and the persistence of their benefits through years are key to improve our understanding on this matter. Finally, intensified agricultural landscapes can affect diversity at different levels beyond local species loss (i.e., decreased alpha diversity) such as, for instance, a reduced beta-diversity leading to biotic homogenization across sites (Gossner et al., 2016; Larsen et al., 2018). Although an increasing number of studies recently examined the effects of AES on complex components of diversity such as beta-diversity (Warzecha et al., 2021), functional diversity (Gallé et al., 2020; Feng et al., 2021; García-Navas et al., 2022) or interaction networks (Martínez-Núñez et al., 2019) they are still scarce and rarely use a multilevel approach. In this study we aim to fill some of these gaps of knowledge by following the conceptual framework developed by Legendre et al. (2005) and further discussed by authors (e.g., Legendre et al., 2008; Tuomisto and Ruokolainen, 2008) for analyzing data with respect to diversity components. In this regard, we recognized three levels of possible impact of BPAs implementation and management in the farmland landscape across 7 years. By deciding to manage a field as BPA instead of a production field at the same location, farmers will change the abiotic conditions of fields, thereby affecting the local communities in the farm. Consequently, the average number of species in BPAs and production fields (alpha-diversity) may differ (first level). Similarly, the species assemblages (beta-diversity) may vary between both types of habitats (second level). In addition, or in combination to the management effect, farmers will increase environmental heterogeneity by placing BPAs and production fields at various locations in the farmland landscape which may also influence the number of species and the species assemblages at the landscape level (Kovács-Hostyánszki et al., 2021). This is caused by the environmental control of the species distribution (sensu Whittaker, 1956) determining then the species assemblages in fields. Not only the difference in alpha- and beta-diversity between BPAs and production fields may be influenced by the environmental heterogeneity but also the variation in beta-diversity among groups of fields (so called “variation in variation in community composition data,” Legendre et al. (2008).
Beyond investigating diversity patterns of species assemblages, the identification of characteristic or indicator species is key to assist efficient conservation and management. This is particularly important due to the increasing focus on result-based agri-environmental payment frameworks (Chaplin et al., 2021). This approach arises as a more cost-effective alternative, but there is no consensus among farmers and it might be logistically difficult to implement (Zabel and Roe, 2009; Niskanen et al., 2021). The use of indicator species could solve some of these problems (Ruas et al., 2021), but few studies have tried to exploit this tool in the context of AES until now (but see Wittig et al., 2006; Martínez-Núñez et al., 2020).
Here, we use a unique and large data set collected in 478 fields over 7 years (four sampling times) in three regions of Switzerland, to study the effect of BPAs on spider assemblages. Focusing on spiders is particularly interesting, since they are a highly diverse and sensitive group that can play a key role in ecosystems as both predators and prey. Surprisingly, studies focusing on spider assemblages in the context of AES are underrepresented. Our approach aimed, first, at integrating traditional semi-natural habitats like orchards and hedges as part of the AES of the farming landscape in the evaluation, besides extensively managed fields in Switzerland. Second, we examined how AES performs over time in the mid-term with data collected bi-annually from 1997 to 2003. Third, we extended the usual analysis of alpha-diversity (i.e., the number of species) to further components of diversity (i.e., beta-diversity and the variability of alpha-diversity) because AES are expected to act in diverse ways on biodiversity. Fourth, we searched for indicator species that inform on the habitat conditions in BPA so that recommendations for the management can be derived. Fifth, we investigated the relative contribution of BPA to the diversity of spider assemblages at regional scale, so that recommendations for landscape planning can be made. Lastly, this study represents a good opportunity to set a baseline to compare the effect of future successive agricultural policy reforms, and to analyze long term trends of spider communities in multiple habitat types.
Based on ecological theory, we hypothesize that: i) alpha-diversity (species replacement) of spider assemblages will be higher in BPA than in production fields because these habitats provide more resources, more niches, and less perturbation, favoring the establishment and coexistence of more species (Benton et al., 2003; Chesson, 2000); ii) beta-diversity (community composition) and the variability of beta-diversity (multivariate dispersion) among spider assemblages of BPA and production fields will differ, because these different habitats will provide complementary niches for different species (Schoener, 1974; Chesson, 2000); and iii) we will be able to detect bioindicator species for each BPA and the contribution of each habitat to regional diversity: we expect BPA to contribute more specialized (or habitat-dependent) species because conventionally managed agricultural habitats will mainly support generalist frequent species (Robinson and Strauss, 2020).
We carried out investigations in three regions representative of the different farming types (arable, mixed arable–grassland, and grassland) in central Switzerland.
The study areas were each 8–10 km2. They were located in the Swiss lowlands, in (1) region one, mixed arable–grassland (7.2 km2, 6°49′ 30″ N/46°46′30″ E, 650 m above sea level [asl]; annual precipitation 900 mm; average annual temperature 8.4°C), (2) region two, grassland dominated (8.8 km2, 8°7′ N/47°6′ E, 750 m asl; annual precipitation 1,400 mm; average annual temperature 6.8°C), and (3) region three, arable land dominated (8.1 km2, 8°32′ N, 47°35 30″ E; 450 m asl; annual precipitation 900 mm; average annual temperature 8.5°C).The types of BPA and their share (percentage) of the farmland in the three regions were representative of the three larger biogeographic regions in which they were located.
The diversity of spiders was investigated bi-annually between 1997 and 2003 in both BPA and conventionally managed fields (total number of fields = 478). As not all BPA and fields were sampled every year and in every region due to study constrains, i.e., wildflower strips BPA were absent of regions 1 and 2 (Supplementary Methods), BPA habitats were first compared to a corresponding conventionally managed field category on a pairwise basis: BPA meadow versus conventional meadow (regions one, two and three, bi-annually from 1999 to 2003, n = 163 vs. 71), BPA orchard versus conventional meadow (region one and three, bi-annually from 1999 to 2003, n = 46 vs. 56), BPA hedge versus production field (encompasses conventional meadows and crops; region one and three, bi-annually from 1999 to 2003, n = 38 vs. 70), and wildflower strip BPA versus crop field (region three, bi-annually from 1997 to 2003, n = 40 vs. 82). Paired were defined on the basis of the production fields that would occur in case of conversion of the BPA, e.g., a crop field instead of a BPA hedge. The design of these paired comparisons is a factorial design, each of the habitat type being sampled in each of the regions and every sampling year (see Supplementary Table 2 for details about number of samples per habitat type, region and year). Second, the relative contribution of BPA habitats to the diversity of spider assemblages was investigated at regional scale by analyzing spider assemblages in BPA and production fields within regions.
In each field, spiders were collected using three pitfall traps located in the center of the field and spaced 3 m apart from one another during 5 weeks between May and July in two periods (3 and 2 weeks). In addition to pitfall trapping, sweep net method was used to collect spiders in the vegetation of meadows, BPA orchards, wildflower strips BPA and crop fields, and the beating method in BPA hedges and on trees of BPA orchards. Sweep netting and beating were applied five times from May to August and samples pooled per sampling year for analysis (see Supplementary Methods for details about trapping methods).
We defined alpha-diversity of a habitat type as the mean number of species in fields of this habitat type per region and year. This response variable was based on species density, the total number of species collected in three pitfall traps, in sweep net and in beating samples per field over a sampling year. Measures of species density reflect both the species richness of the community and the number of individuals collected (Gotelli and Colwell, 2001). Catches are affected by - as for example in our study - habitat structure, i.e., individuals more likely fall in pitfall traps placed in habitats with sparse vegetation (e.g., crops at early development stages) than in habitats with dense and diverse vegetation (e.g., meadows; Topping and Luff, 1995). Therefore, we used rarefaction to adjust for differing densities of individuals, i.e., we standardized fields to a common number of 100 individuals (Supplementary Methods) and estimated the species richness of fields using R (R Development Core Team, 2018).
We defined beta-diversity as the variation in species composition among fields per region and year. Differences in the species composition among the spider assemblages of fields were investigated and visualized using non-metric multi-dimensional scaling (MDS). Following Anderson et al. (2006) we used of the following dissimilarity measures that increasingly emphasize the relative abundance of the species: Jaccard dissimilarity index dJ (pure species composition = presence/absence list), the modified Gower’s dissimilarity measure (excluding joint absences) with data log10(x) + 1 transformed dMG10, and data log2(x) + 1 transformed dMG2 (Supplementary Methods, formula of dissimilarity measures). Non-metric MDS plots were constructed with dMG10 to allow for an intermediate emphasis of the relative abundance of the species. Polygons enclosing sites of habitat types per region and year were drawn on the plots by connecting the outermost sites. Together with the polygons, line segments linking each site to its centroid plotted per habitat type, region and year were drawn to visualize beta-diversity.
As a measure of the variation in beta-diversity among group of fields we used the multivariate homogeneity of group dispersions following concepts and methods devised by Anderson et al. (2006), and used recently in other studies (Martínez-Núñez et al., 2019). The multivariate dispersion is measured as the average distance (or dissimilarity) from an individual unit (a field) to the group centroid, using a dissimilarity measure. In our case, a group was composed of the sites of a habitat type in a region and a year. Analysis was again performed with dissimilarity matrices based on measures with increasing emphasis on the relative abundance of the species, i.e., dJ, dMG10 and dMG2 (Supplementary Methods). Multivariate dispersion was calculated per habitat type, region and year with R (R Development Core Team, 2018).
Characteristic spider species were identified for habitat types, sampling years and regions using the indicator value method (Dufrêne and Legendre, 1997). This method combines measures of specificity and fidelity and provides an indicator value (IndVal) for each species, as a percentage (Supplementary Methods). First, indicator species were searched for pairs of habitat types across regions for each year separately (two groups of sites, i.e., BPA habitats vs. conventionally managed fields, for instance BPA meadows vs. conventional meadows). Indicator fidelity was specified according to the number of sampling years for which the species has a significant indicator value, i.e., regular for 2 years significant, and very regular for 3 years significant. Then, indicator species were identified for the habitats within the three regions to examine whether indicator species for a particular habitat type were specific to a particular region, namely six groups of sites (BPA meadows vs. conventional meadows in the three regions), four groups of sites (BPA orchards vs. conventional meadows and BPA hedges vs. production fields in two regions) and two groups of sites (wildflower strip BPA vs. crop fields in region three). A rarity value for Switzerland was given for indicator species, from one (very common) to six (very rare; comm. Pers., Pozzi et al., 1998). The ecological requirements of species were derived from Maurer and Hänggi (1990).
As agri-environmental measures should be implemented according to region-specific goals, BPAs performance as conservation tool has to be assessed at a regional level. This can be approached by investigating the specificity of species for their habitat to derive the contribution of BPA fields to the regional diversity as shown by Wagner and Edwards (2001) with coarse types of land uses, plants and snails. We adapted this concept by summing the specificity of the species for the habitats, which is a component of the indicator value according to Dufrêne and Legendre (1997), in a score per habitat to compare the contribution of each habitat to the regional species richness (see Supplementary Methods for formula). This is a method commonly used to assess the uniqueness or contribution of different habitat types to the meta-community (e.g., García-Navas et al., 2022).
Multifactorial mixed-model ANOVAs with permutations (Anderson and Ter Braak, 2010) were performed to test differences between habitat types, regions and years, for alpha-diversity and multivariate dispersion (variation in beta-diversity) using the DISTLM procedure (Anderson, 2001, 2004). This procedure was originally introduced as a distance-based multivariate analysis for a linear model, but can be used for a single response variable (univariate case) by choosing the Euclidean distance to calculate the distance matrix between samples. The beta-diversity among habitat types, regions and years was analyzed with a distance-based multivariate ANOVA (Anderson, 2001; McArdle and Anderson, 2001), using again the DISTLM procedure. Within the analyses, the factors “habitat type” (two levels, BPA habitats versus conventionally managed fields, pooled and pairwise analyzed) and “region” (two or three levels depending on the pairs of habitat types compared, region one to three) were treated as fixed factors and “year” as a random factor (three or four levels depending on the pairs of habitat types compared, year one to three or one to four). Region was a fixed factor because regions were chosen regarding their main agricultural land use, i.e., arable, mixed arable–grassland, grassland. To test particular terms in the permutational analysis of variance, permutation strategies followed a design involving three crossed factors, two of them fixed and one random after Anderson and ter Braak (2010; Supplementary Methods). In case of significant interaction terms, the second order habitat type x region interaction was further considered by analyzing the habitat type effect per region separately, or in region three the habitat type effect in years. Permutational analysis of variance (univariate and multivariate) were performed with 4,999 permutations to calculate the significance of the pseudo-F statistic.
The significance of the indicator values (IndVal) of each species was tested with the random reallocation procedure of sites among site groups (habitat types, regions) according to Dufrêne and Legendre (1997). Each species has a percentage IndVal with an associated measure of significance, with high and significant percentages designating good indicator species. Because several tests of significance are performed simultaneously in this analysis, the Holm’s procedure to adjust probability values was performed as proposed by Legendre and Legendre (1998). Furthermore, after calculation of the mean indicator value per species over the sampling years, mean indicator values over all species (mean of the means) were compared among paired habitat types with the Wilcoxon rank sum test per year separately. Multiple comparisons of habitats in regions were done with the Tukey’s honest significant difference (Tukey’s hsd). Analyses were completed with R (R Development Core Team, 2018).
Canonical redundancy analysis (RDA) with principal coordinates of neighbor matrices (PCNM variables) as model for the spatial structure was used to check for independence of samples of pairs or fields compared within regions and years following the procedure of Borcard and Legendre (2002) and Borcard et al. (2004; Supplementary Methods). No significant autocorrelation has been detected in any of the pairs of habitats compared in any region and year at p = 0.05 level (RDA, 0.063 < p < 0.91).
To check for possible influence of sample size on the results, we looked at the linear correlation between dissimilarity matrices calculated with the Chao’s abundance-based Jaccard and its bias-corrected version (Chao et al., 2005) as proposed by Anderson et al. (2006). Chao’s abundance-based Jaccard and its bias-corrected version were calculated with EstimateS (Colwell and Elsensohn, 2014). The linear correlation between these two matrices ranged between 0.92 and 0.95 depending on the pair of habitats, suggesting that the sample size cannot be responsible for the observed pattern (Supplementary Methods).
In total, 180,987 individuals were collected and 284 species identified. Pooled BPA habitats (BPA meadows, BPA orchards, BPA hedges and wildflower strip BPAs, n = 276) showed a significantly higher alpha-diversity (rarefied to a common abundance level of 100 individuals per site) than pooled conventionally managed fields (crops and conventional meadows, n = 171) with, respectively, 17.1 ± 0.4 and 13.9 ± 0.3 species (mixed-model ANOVA with permutations, F = 55.9, df = 1, p < 0.05, see Supplementary Table 4 for the full ANOVA table). Alpha-diversity was significantly influenced by the region (R1 = 17.5 ± 0.5, R2 = 13.1 ± 0.3, R3 = 16.9 ± 0.4, F = 14.0, df = 2, p < 0.05) but not by the sampling year (F = 1.2, df = 2, p = 0.3). As the interaction habitat type x region was significant (p < 0.05), tests were performed per region separately. They revealed that habitat type was still significant with higher alpha-diversity values in BPA habitats than in conventionally managed fields in region one and three but not in region two (mixed-model ANOVA with permutations, region one, F = 25.5, df = 2, p < 0.05; region two, F = 15.4, df = 2, p = 0.06; region three, F = 64.2, df = 2, p < 0.05).
Furthermore, effects of habitat types, regions and years on alpha-diversity were tested in paired comparisons. Results showed that BPA habitats demonstrated a significantly higher alpha-diversity than conventionally managed fields except for BPA meadow vs. conventional meadow (habitat type effect, Table 1). The largest difference occurred between BPA hedges and production fields and was particularly remarkable in region one (Figure 1). Region showed significant effect excepted for BPA hedge vs. production field (Table 1). On average in meadow habitats, region three demonstrated the highest alpha-diversity (Figure 1, R3 = 17.8 ± 0.8, R1 = 14.4 ± 0.4, R2 = 11.4 ± 0.2) while in region one we found more species than in region two when comparing BPA orchards with conventional meadows (R1 = 17.4 ± 0.7, R2 = 11.9 ± 0.4). In addition, the sampling year had a significant effect in BPA orchard vs. conventional meadow comparison (Table 1), 1999 being on average the most species rich sampling year and 2003 the most species poor (1999 = 16.2 ± 0.8, 2001 = 14.8 ± 0.7, 2003 = 13.6 ± 0.8). Within this comparison, the habitat type, region and sampling year had a significant interaction effect (Table 1); region one showed a higher alpha-diversity than region two in both habitat types (Figure 1); the habitat type effect was stronger in region one (2001 and 2003 showed a significant difference between BPA orchard and conventional meadow, ANOVA with permutations, p < 0.005) than in region two (2001 only showed significant difference, p < 0.05) and alpha-diversity decreased along the sampling years excepted for BPA orchards in region one (Figure 1). Neither sampling year nor region had a significant effect on alpha-diversity in the BPA hedge vs. production field comparison. The three-way interaction was significant but the differences between BPA hedge and production field were significant each sampling year in region one (ANOVA with permutations, p < 0.001) and in region two (1999, p < 0.05, 2001, and 2003, p < 0.005). The highest alpha-diversity was recorded in BPA hedges in 2003 and in 2001 in region one and two, respectively (Figure 1). The habitat type and the sampling year effects were significant in the wildflower strip BPA vs. crop field comparison as well as the interaction between both (Table 1). Alpha-diversity strongly increased after the first year (1997) for which the difference between both habitat types was not significant (ANOVA with permutations, p > 0.05) and then slowly decreased from 1999 to 2003 in wildflower strips BPA while alpha-diversity remained remarkably stable in crops (Figure 1; 1999, 2001, and 2003, p < 0.001).
Table 1. Effects of habitat type (pairwise, BPA habitat types vs. conventionally managed fields), region and sampling year on alpha-diversity of spiders (rarefied at 100 individuals) in three regions of the Swiss plateau (mixed-model ANOVA with permutations).
Figure 1. Alpha-diversity (mean number of species +1SE) of spider species (rarefied at 100 individuals) in BPAs and conventionally managed fields. Habitat types are compared by pairs, i.e., (A) BPA vs. conventional meadow, (B) BPA orchard vs. conventional meadow, (C) BPA hedge vs. production field (encompasses crop fields and conventional meadows without pastures), (D) wildflower strip BPA vs. crop field. The comparison of wildflower strip BPA vs. crop field occurs in region three only. Each bar represents alpha-diversity per year, i.e., 1999, 2001, 2003 from the left to the right (1997 in addition for crop field vs. wild flower strip BPA). The scale among graphs is kept the same to allow vertical comparisons.
Assemblages were characterized by the dominance of a few species as it very often is in arthropod communities in agricultural landscapes. However, crop fields and conventional meadows were more strongly dominated than BPA habitats. The first five most dominant species encompassed 81% of the individuals in conventional meadows, 81% in crop fields, 73% in BPA meadows, 73% in BPA orchards, 44% in BPA hedges, and 63% in wildflower strips BPA (rank-abundance curves, Supplementary Figure 1).
All explanatory factors and interactions except the interaction habitat type x sampling year showed significant effects on the beta-diversity of pooled BPA habitats (BPA meadows, BPA orchards, BPA hedges, and wildflower strip BPAs, n = 276) versus pooled conventionally managed fields (crops and conventional meadows, n = 171) irrespective of the dissimilarity measure used, i.e., dJ, dMG10 and dMG2 (all p < 0.001, see Supplementary Table 5 for the full table of the distance-based multivariate ANOVA with permutations). As the interaction habitat type x region was significant (p < 0.05), tests were performed separately per region and revealed that beta-diversity was still significantly different according to the habitat type in the three regions and for the three dissimilarity measures involved (distance-based multivariate ANOVA with permutations, habitat type in all three regions: p < 0.005 for dJ, dMG10 and dMG2).
In paired comparisons, non-metric MDS plots indicated effect of region for meadow comparison but also revealed a set of very particular sites for spiders (Figure 2A). The effect of the habitat type was the most obvious by BPA hedge compared to production field (Figure 2C) while a regional effect was clear by BPA orchard compared to conventional meadow (difference not apparent in region two, Figure 2B), and a year effect by wildflower strip BPA compared to crop field (Figure 2D). BPA habitat types and conventionally managed fields revealed significantly different species composition (based on dMG10) for every pair of habitats compared (habitat type effect, Table 2). Regions and sampling years also showed significantly different species composition. As interactions were significant, we further investigated the habitat type effect in regions separately for BPA orchard vs. conventional meadow (sampling year is considered random factor), in regions and sampling years separately for BPA meadow vs. conventional meadow and BPA hedge vs. production field, and according to sampling years for wildflower strip BPA vs. crop field. In both regions 1 and 2, the species composition was still different among BPA orchards and conventional meadows (distance-based multivariate ANOVA with permutations, p < 0.001 and p < 0.005 in regions one and two, respectively). The difference between BPA and conventional meadow was not significant in any regions and sampling years (p > 0.05). The species composition of BPA hedges was significantly different from production fields in both regions during each sampling year (p < 0.001) except 1999 in region three (p = 0.08) with a larger dissimilarity in region one as shown on the non-metric MDS (Figure 2C). While the difference in species composition between wildflower strips and crop fields was lower in the first sampling year 1997 than during the subsequent years 1999, 2001, and 2003, as shown on the non-metric MDS (Figure 2D), the differences per year separately were still all significant (p < 0.005). Interestingly, among the main explanatory factors, the habitat type had the strongest effect for BPA hedge vs. production field comparison while the difference BPA vs. conventional meadows, and BPA orchards vs. conventional meadows was better explained by the region (Table 2, habitat type and region effects). This was confirmed by the non-metric MDS plots (Figure 2A) which showed a more apparent grouping of BPA meadows according to the region than to the habitat type. In particular, region two revealed similar species composition in BPA and conventional meadows. In contrast, the species composition of BPA hedges was very clearly separated from production fields for both regions. Apart from some differences in the significance level, increasing consideration of the species abundance by calculating the dissimilarity matrix with dMG2 and considering the presence/absence list with dJ did not change the results (see Supplementary Table 6, 7 for the full table of the distance-based multivariate ANOVA with permutations).
Figure 2. Non-metric MDS plots showing the pattern of dissimilarities and distance to centroid (using the Gower dissimilarity measure, excluding double zeros) with log10 + 1 transformed data among spider assemblages in BPA and conventionally managed fields. Habitat types are compared by pairs, i.e., (A) BPA vs. conventional meadow, (B) BPA orchard vs. conventional meadow, (C) BPA hedge vs. production field (encompasses crop fields and conventional meadows), (D) wildflower strip BPA vs. crop field. R1 = region one, R2 = region two, R3 = region three. Centroids, hull envelope and multivariate dispersion (distance between each site and the centroid to which it belongs) are shown for groups combining each habitat type per region. The comparison of wildflower strip BPA vs. crop field occurs in region three only for which centroids, hull envelope and multivariate dispersion combine habitat type and sampling year (1997, 1999, 2001, 2003).
Table 2. Effects of habitat type pairwise (BPA habitat types vs. conventionally managed fields), region and sampling year on the species composition (beta-diversity) of spiders in three regions of the Swiss plateau (distance-based multivariate ANOVA with permutations, mixed-model).
Pooled BPA habitats (BPA meadows, BPA orchards, BPA hedges and wildflower strip BPAs, n = 276) showed a significantly higher multivariate dispersion based on Jaccard’s dissimilarity index dJ and both Gower’s dissimilarity measures dMG10 and dMG2 than pooled conventionally managed fields (crops and conventional meadows, n = 171; p < 0.05, see Supplementary Table 8 for the full table of the multifactorial mixed-model ANOVA with permutations). By taking more account of relative abundance information, i.e., with dMG2, difference between regions was significant (p < 0.05), region having the highest multivariate dispersion followed by regions one and two (0.263 ± 0.004, 0.257 ± 0.004, 0.231 ± 0.004, respectively). In contrast, multivariate dispersion values were not significantly different among sampling years with any dissimilarity measure. Furthermore, multivariate dispersion based on dJ was significantly dependent on the interaction term region x sampling year (p < 0.05), the highest values being recorded in 2003 for region one (0.416 ± 0.008) and three (0.416 ± 0.009) but in 2001 for region two (0.409 ± 0.009). Multivariate dispersion based on dMG2 revealed a significant interaction term habitat type x region x sampling year (p < 0.05).
In paired comparisons, BPA habitat types demonstrated significantly higher multivariate dispersion based on dMG10 than conventionally managed fields for BPA meadow vs. conventional meadow (0.315 ± 0.004 vs. 0.272 ± 0.006) and BPA hedge vs. production field (0.339 ± 0.006 vs. 0.299 ± 0.005) but neither for BPA orchard vs. conventional meadow (0.321 ± 0.006 vs. 0.310 ± 0.008) nor for wildflower strip BPA vs. crop field (0.325 ± 0.005 vs. 0.300 ± 0.005; habitat type effect, Table 3). This was confirmed by the non-metric MDS plot (Figure 2). A larger multivariate dispersion of the BPA meadows occurred, irrespective of the region, than of the conventional meadows around their centroids. However, two conventional meadows in region three were more distant to their centroid than the average. For BPA meadows, the multivariate dispersion was larger in region one (0.316 ± 0.007) and three (0.343 ± 0.008) than in region two (0.297 ± 0.006). Compared to BPA hedges, production fields were remarkably grouped except two fields in region one (Figure 2C) and had consequently shorter distances to their centroids. As noticeable on the non-metric MDS plot (Figures 2B,C) multivariate dispersion among BPA orchards and conventional meadows, as well as among wildflower strips BPA and crop fields did not obviously differ (no significant habitat type effect, Table 3). In one case, namely BPA orchard vs. conventional meadow, the sampling year had a significant effect on multivariate dispersion, 2001 having the highest multivariate dispersion value and 1999 the lowest (1999: 0.296 ± 0.011, 2001: 0.330 ± 0.007, 2003: 0.313 ± 0.009). Adding abundance information to the pairwise comparisons by calculating the multivariate dispersion based on dMG2 did not qualitatively change the main results but some factors became significant (see Supplementary Table 9 for detailed results), i.e., the region in BPA meadow vs. conventional meadow, the interaction term habitat type x region x sampling year in BPA hedge vs. production field, and interestingly, the habitat type in wildflower strip BPA vs. crop field. In contrast, the habitat type became insignificant by the BPA hedge vs. production field comparison. Comparing multivariate dispersion based on the presence/absence list with dJ (eliminating the relative abundance effect) caused three additional factors to become significant, i.e., the interaction term region x sampling year in BPA meadow vs. conventional meadow, the sampling year in BPA hedge vs. production field, and the interaction term habitat type x sampling year in wildflower strip BPA vs. crop field (see Supplementary Table 10 for detailed results).
Table 3. Effects of habitat type pairwise (BPA habitat types vs. conventionally managed fields), region and sampling year on the multivariate dispersion of spiders in three regions of the Swiss plateau (mixed-model ANOVA with permutations).
From 284 species, preference for BPA habitats occurred by 41 species with a significant indicator value for BPA hedge, 22 for wildflower strip BPA and 11 for BPA orchard but less for BPA meadow (three species) while four and two species had significant values for crop fields and conventional meadow, respectively (list of species with indicator value IndVal in Supplementary Table 11).
Across regions and over the three sampling years, 22 of 231 species (about 10%, Figure 3) had a significant indicator value (p < 0.05, Holm-corrected) for conventional meadows while none had a significant indicator value for BPA meadows. In all other pairwise comparisons, the mean indicator value of species was significantly higher in BPA habitats than in non-BPA controls. For BPA meadow, though, the difference was relatively modest (Wilcoxon rank sum test, p < 0.05, over the years and every year; Figure 3). Some indicator species were also detected when considering different regions, years and sampling methods (Supplementary Results).
Figure 3. Percentage of significant indicator species per habitat type across regions and years (bars), and mean indicator value (+ 1SE) of all species (black dots). A: conventional meadow, B: BPA meadow, C: conventional meadow, D: BPA orchard, E: production field, F: BPA hedge, G: crop field, H: wildflower strip BPA.
Altogether, region one was the region with the highest number of species in this study, being slightly ahead of region three while region two had ¼ less species.
At the regional level, BPA hedges showed the highest numbers of characteristic species with significant indicator values in regions one and two(about 25 and 23% of the total regional number of species, respectively, Figure 4). Low numbers of indicator species were counted in crop field sites (4 and 5.5% in regions one and two, respectively). The conventional meadow was the poorest indicator species habitat over the regions one and two (1 and 0%, respectively) but not in region three where it was the richest (2.8%). However, in region three where no BPA hedge sites had been sampled, the number of species with significant indicator value was low, i.e., under 3%. Further interesting information was provided by the mean indicator value over all the species per habitat type. BPA hedge had the significantly highest mean indicator value in regions one and two (Tuckey’s hsd, p < 0.05, Figure 4). Although a similar number of species with significant indicator values were found in conventional meadows, crop fields and BPA orchards of region one, the mean indicator value over all species of the later habitat was significantly higher (Tuckey’s hsd, p < 0.05, Figure 4).
Figure 4. Percentage of significant indicator species per habitat type in three regions across years (bars), and mean indicator value of all species (black dots). A: conventional meadow, B: crop field, C: BPA meadow, D: BPA orchard, E: BPA hedge, F: wildflower strip BPA. Points with the same letter are not significantly different (Tukey’s hsd, p < 0.05).
The sum of the species specificity for habitats was the highest in region one, followed by regions three and two (Figure 5). BPA habitats had higher specificity values than production fields in the three regions although not always significant. BPA hedges in regions one and two (Figure 5E), and BPA meadows in region three showed the highest values (Figure 5C). In the three regions, conventional meadows exhibited the lowest specificity value.
Figure 5. Mean specificity score of habitats and standard error over three (four for region three) years in the three regions (comparative contribution to regional diversity). (A) conventional meadow, (B) crop field, (C) BPA meadow, (D) BPA orchard, (E) BPA hedge, (F) wildflower strip BPA. Specificity values with the same letter are not significantly different (Tukey’s hsd, p < 0.05).
Studies assessing the effectiveness of AES have traditionally concentrated on the number of species found in fields under the scheme compared to conventional production fields. However, the number of species (α-diversity) represents only a part of the diversity of a given species group. Indeed, as we show, agricultural intensification can have more pernicious and hidden effects on other diversity components such as beta-diversity, conducting to (meta-) community homogenization (also shown by Gabriel et al., 2006 and Tarifa et al., 2021). Indeed, alpha- and beta-diversity can be decoupled in their response to environmental perturbation (Smart et al., 2006). Simplification of species diversity to the number of species or to a composite diversity index leads to a substantial loss of information that may be crucial in examining the effect of environmental factors as shown in this study. This might be especially important in a context where the anthropic-driven simplification of ecosystems leads to biotic homogenization and the preponderance of generalist species (McKinney and Lockwood, 1999; Kehinde and Samways, 2014; García-Navas et al., 2022).
Comparison of diversity patterns of spider assemblages in BPA habitats and corresponding production fields showed that farmers act in different ways on diversity components by implementing BPA habitats. While alpha-diversity was on average higher in BPA hedges, BPA orchards and wildflower strip BPA compared to the corresponding production fields, alpha-diversity of BPA meadows did not significantly differ from conventional ones. Similar results were obtained by Knop et al. (2006) but not by Albrecht et al. (2010), who also compared the number of spider species between BPA meadows and conventional ones. Contrasting results likely reflect the longer survey period by Albrecht et al. (2010) including autumn assessment while both other studies were capturing spider during springtime and early summer. Vascular plants, bees and grasshoppers responded positively to the scheme in both investigations. Comparing several AES across Europe and their impact on biodiversity, Kleijn et al. (2006) found a low effectiveness of schemes on the species density of arthropods. A more recent study showed an overall positive effect of AES on multiple taxa (Boetzl et al., 2021). In a recent study, Gayer et al. (2021) showed that promoting diverse habitats such as flower strips, hedgerows and organic farms promotes the diversity of plants and arthropods in croplands. Our results show that conclusions may differ if, in addition to species richness, species composition is taken into account (see also Smart et al., 2006; Clough et al., 2007). Furthermore, our study revealed that traditional woody elements of the agricultural landscape, i.e., hedgerows and orchards with high-stem trees, differ most from production fields, with higher species richness and particular species composition. The value of these hedgerows and orchards in supporting arthropod diversity has been emphasized recently by other authors (Garratt et al., 2017; Kolb et al., 2020).
Analyzing the diversity components of spider assemblages in meadows in more detail allows deeper insights. The main effect (BPA vs. conventional) was observed on the species composition and this is probably due to a large number of particular BPA meadows which strongly differed from a group gathering BPA and conventional ones as shown on the MDS plot (Figure 2) and quantified by the multivariate dispersion (Table 3). This dispersion is very likely to be the consequence of the environmental heterogeneity across BPA meadows and not of the management intensity because all BPA meadows were managed similarly, according to the same – BPA – rules. Then, it can be concluded that extensively managed BPA meadows have the potential to support many different species and the specific assemblage found in this habitat will be more strongly determined by the environmental heterogeneity around them. Spiders may then profit from the choice of farmers, deciding to allocate BPA meadows in diverse environments related to low productivity and/or plots which are more difficult to manage (e.g., steep slope, forest shadow) as shown by Herzog et al. (2005). Nonetheless, benefits for spider communities have been reported after the implementation of uncut meadow strips AES management (Řezáč and Heneberg, 2018). No indicator species were found (according to IndVal) for BPA meadows while 10% of the species were indicators of conventional meadows. This is due, in one side, to particular species occurring in some BPA meadows but not in others (fidelity component of IndVal was low), and on the other side, to the particular species assemblages of conventional meadows in region three. In this region, one site was characterized by a concentration of rare species for Switzerland (rarity values from three to five according to Pozzi and Borcard, 2016). Again, this demonstrates the need to focus on multiple components on diversity beyond species richness. In this situation, farmers of region three should be encouraged to maintain the current management of the particular meadows to sustain species diversity at regional level.
On average more species were found in BPA orchards than in conventional meadows, and this had an effect on the species composition, which was also significantly different. In region one, however, beta-diversity of both habitat types was highly different in that region while less preeminent in region two, where assemblages of conventional meadows can be considered as a subset of the BPA orchards. The similar multivariate dispersion of both habitat types suggests that the environmental heterogeneity acted similarly on spider assemblages among fields in both habitat types and regions. Furthermore, BPA orchards are particularly interesting habitats for spiders because they combine both trees and a meadow. Although 1/3 of the 54 indicator species were characteristic species of tree canopy (caught by beating), a similar proportion of species were captured simultaneously in the tree canopy and in the vegetation (sweep net) or on the ground (pitfall trap) of BPA orchards. For the major part of these species which were not caught at all in conventional meadows, BPA orchards represent a unique habitat combination in the landscape that even BPA hedges do not provide. Published studies relating effect of orchards with high stem fruit trees on biodiversity have been traditionally underrepresented (e.g., but see Bailey et al., 2010; Samnegård et al., 2019; Martínez-Núñez et al., 2021), although the role of such agro-ecosystems in providing particular environmental conditions by combining trees with meadows or crops has for long been recognized in the agroforestry literature (Herzog, 1998; Jose, 2009).
BPA hedges had the most characteristic spider assemblages of BPA habitats compared to corresponding production fields in the agricultural landscape. This is reflected in the higher number of species, a highly distinctive assemblage of species and a high number of regular indicator species showing high specificity and fidelity, irrespective of the region and the sampling year (47% of species are indicators of BPA hedges compared to production fields). However, the species composition of BPA hedges in regions one and two largely differed. The multivariate dispersion was higher among BPA hedges than among production fields, reflecting both different environmental conditions of the BPA hedges and a possible difference of management within them. Investigations have emphasized the important role of hedgerows as conservation element for biodiversity in the agricultural landscape (e.g., Forman and Baudry, 1984; Rey et al., 2021) and also for biocontrol purposes (e.g., Marino and Landis, 1996; Kolb et al., 2020; Martínez-Núñez et al., 2021).
In our study, hedgerows were demonstrated to play a significant role for spider conservation by providing habitat for a number of species that did not occur neither in production fields nor in other BPA habitats (see below effect at region level). Further investigations would be necessary to know whether these species spillover to adjacent fields to their hunt territory, and then provide biocontrol ecosystem services (Tscharntke et al., 2016; Růžičková et al., 2020). A recent study has shown that spiders are more abundant and species-rich in set-aside fields, but their dispersal capacity is limited and their pest-control service might depend importantly on habitat proximity to the crop fields (Růžičková et al., 2020).
Wildflower strip BPA are inserted in landscapes dominated by crops in Switzerland, and are part of the crop rotation (may move every 6 years). This condition strongly influenced the spider assemblages occurring there because most of the species were typical crop species during the first 2 years after the strip has been sown. However, after 2 years in our study, spider assemblages of wildflower strip BPA were highly distinct from those of the crops with about 32% of significant indicator species. After the colonization period, species assemblages remained stable. This suggests, that the role played by the wildflower strip BPA as refuge in the landscape for typical crop species during unsuitable periods of cropping operations, and as reservoir for further re-colonization of the crop, which should deliver biological control effects. Such species are Diplostyla concolor (Wider, 1834) and Mangora acalypha (Walckenaer, 1802; see Supplement for the IndVal values of species). On the other side, wildflower strip BPA provide suitable habitat for particular species that can permanently benefit after rapid colonization (corroborated by Frank and Nentwig, 1995). Such species are Argiope bruennichi (Scopoli, 1772) and Aulonia albimana (Walckenaer, 1805). Similarly, fallow vineyards grown in steep slopes have also been identified as important habitats and reservoirs for rare species (Wersebeckmann et al., 2021).
Together with the comparative contribution of habitats to the regional diversity, results on alpha- and beta-diversity, and the multivariate dispersion suggest that AES were more effective in the region where the spider diversity was higher as a rule. This might be due to the fact that species-diverse regions have a higher potential to allocate species with contrasting habitat requirements in different BPAs while simplified regions probably host a pool of species that has already been strongly filtered, minimizing the potential impact of AES (Smart et al., 2006; Gámez-Virués et al., 2015). Indeed, observed regional differences in spider diversity can be explained by three factors: regional land use, management intensity and the farming history. Region two is characterized by a high production level of its dominating land use, namely grassland, with about 42% more hay produced on average by conventional meadows and about twice more livestock units per ha, compared to the mixed farming region one, and the crops’ region three (Table 4). This production level can only be reached by intensive management of the grasslands, also reflected by the number of cuts which averaged 4.6 in region two compared to 3.1 and 3.0 cuts per year in regions one and three, respectively. As a consequence, differences between BPA habitats and production fields, as well as habitat specificity by spider species were lower in region two. Land use history can be a main driver of current diversity levels of multiple taxa (Le Provost et al., 2020). Therefore, it is not surprising that it also plays an important role when it comes to BPA effectiveness. For instance, the high importance of BPA meadows for spiders in region three can be explained by land-use history during the last century. In this region, meadows were gradually replaced by more productive arable crops on the plateau, but some of them were kept and are still extensively managed on slopes around the plateau, some of which were now declared as BPA (on average 27.2 dt/ha hay yield). Apparently, spider assemblages in these meadows were quite characteristic and remarkably enriched the regional diversity.
Table 4. Major land use categories, agricultural characteristics of BPA, and production fields in the 3 regions.
Comparison of BPA habitats with production fields, together with land use and production data of the regions suggests that management intensity of the main land use and of particular BPA are the most important drivers for spider diversity at regional scale. A high proportion of BPA in the landscape does not ensure high species diversity depending on the BPA type and its management, as shown in region two which encompassed the higher proportion of BPA (16.2% against 8, 5 and 2.9% in regions one and three, respectively) but with a large area covered by BPA orchards (11.1%) where the undergrowth meadow is unfortunately very often intensively managed.
Ecological theory predicts that AES demonstrate higher effectiveness for biodiversity in simple landscape types compared to cleared and complex one’s when complexity is measured as the proportion of non-crop areas (Tscharntke et al., 2005). In our opinion, this is not true in all circumstances. Indeed, our data showed that the effectiveness of the scheme, i.e., the difference between BPA habitats and production fields in terms of diversity (alpha and beta) is the most effective for spiders in a landscape with primarily less intensively managed fields (that can also be grasslands) and a balanced proportion of crops, grasslands and BPA (i.e., region one). Furthermore, regions with high percentage of crops but with valuable BPA (e.g., wildflower strip BPA) as well as less intensively managed grasslands (region three in this study) may also demonstrate high diversity value. In our study, region two had the lowest proportion of crops but grasslands including meadows of BPA orchards were intensively managed preventing then a high spider diversity to occur even in BPA habitats. Investigations have shown that many factors may confound the effect of AES on biodiversity. One of them is the landscape configureuration and the proportion of non-crop habitats in the surrounding of AES fields versus conventionally managed fields (Clough et al., 2005; Schmidt et al., 2005, 2008; Gámez-Virués et al., 2015). However, effects were demonstrated for large gradients in these studies, i.e., from 0 to 80% of non-crop area. In our study, gradient was comparatively very low with values of 0 to 20% and consequently landscape effects were minor (Jeanneret et al., 2003b).
BPA habitats were characterized by significantly higher mean indicator values of all species calculated with IndVal (Dufrêne and Legendre, 1997) than production fields. The mean indicator value indicates how unique the habitat in the farmland landscape is for spiders. In paired comparison of BPA habitats vs. production fields, significant differences in favor of BPA habitats demonstrated their importance for a majority of species. The results of the regional analysis point into the same direction, BPA hedges showing in particular highly characteristic species assemblages which were not found in other habitat types. Remarkably, a large part of the species indicators for BPA hedges came from pitfall trapping. This indicates that not only species of shrubs and trees were characteristic but also soil dwelling species, due to particular micro-climatic conditions. Compared to conventional meadows, BPA orchards showed a high percentage of species preferably occurring there, but their value as particular habitat for spiders decreased in the regional analysis. This is due to the other habitat types, BPA meadows, BPA hedges and crop fields, which were part of the analysis, and where species of BPA orchards also occurred. However, detailed analysis of the species distribution revealed that the combination of a meadow with traditional high-stem trees favored two rare species of Switzerland known to live on the trunk of trees and which were exclusively caught in BPA orchards in the branches of the trees as well as in the low vegetation. Despite being isolated in a crop dominated landscape and regularly re-sown, wildflower strip BPA were rapidly colonized by species that were then recorded as indicator species because they were not occurring in the other habitats of region three. Crops, meadows and wildflower strip BPA are highly contrasted habitats resulting in a high specificity of spiders for them. Similarly, Rischen et al. (2021) reported positive effects of non-crop habitats in ground-dwelling beetle diversity, showing also that different habitat types contributed particular species assemblages to the landscape. These results might explain, at least partially, why schemes aimed at set aside areas (non-productive habitats) seem to be more effective (Ekroos et al., 2014; Batáry et al., 2015) and a mosaic of different habitat types in the landscape increase regional diversity of arthropod predators (Mader et al., 2017).
To investigate the effectiveness of agri-environmental measures on biodiversity, replicated spatial and temporal designs are necessary. Temporal and spatial variability in experimental designs may affect conclusion as results may depend on sampling years and regions. Indeed, in our study alpha-diversity of BPA orchards and conventional meadows were not significantly different for one and two sampling years in regions one and two, respectively. Based on those findings, it is difficult to draw conclusions about the success of the AES with respect to BPA orchards compared to conventional meadows for spiders. Analyzing beta-diversity in addition to alpha-diversity revealed significant differences in species composition among habitat types in both regions and across all years. This emphasizes the importance of investigating effects at several diversity levels and across several years. In 1997, the recently sown wild flower strip BPA harbored the same number of species on average and a similar species composition than the crop fields but were clearly more species-rich and with specific species composition in the following years. Those results suggest succession processes with a somewhat delayed response of spider assemblages, representing a clear case supporting the recovery debt hypothesis (Moreno-Mateos et al., 2017). If succession processes had not been considered in the evaluation design, wrong conclusions would have been drawn, i.e., no success in 1997. When time is replaced by space in snapshot studies, evaluation should take place once the effects can be expected to be established. This is actually very challenging. Indeed, even if targets of AES are precisely defined, e.g., the occurrence of a target species, goal achievement can only be recorded by monitoring data over time in both control and treatment fields following a before-after-control-impact design, as recommended by Kleijn et al. (2006). To our knowledge, our investigation is the first one to assess the effectiveness of an AES across a 7-years period of time, and covering all habitat types belonging to the scheme in a common analysis. In addition, data used in this study were collected ca. two decades ago, at the beginning of the implementation of these measures. This adds value to the work since it can be used as a baseline over which to compare more recently collected data.
This study highlights that, schemes aimed at promoting set-aside habitats (areas out of production) interspersed in farmland landscapes increase spider diversity at multiple levels. In addition, focusing only on α-diversity may bias conclusions about the benefits delivered by AES. To properly assess the efficacy of AES and derive useful recommendations, a comprehensive analysis of the species composition and the indicator species is necessary. Our findings on spider assemblages support that the conservation of semi-natural elements in the agricultural landscape such as hedgerows and traditional orchards with high-stem trees may be of crucial importance for biodiversity. The key role AES play in preserving species diversity in cultivated landscapes not only relies on harboring particular assemblages related to the habitat itself (e.g., hedgerows, wild flower strips) but also on increasing the range of environmental conditions in which these habitats usually occur (grasslands). The range of environmental conditions encountered even in regions of about 7 to 8 km2 can increase the regional diversity by acting on the β-diversity component and its variation. This has important implications because it is easier to maximize environmental heterogeneity by establishing less or non-productive habitats like BPA in the farmland landscape compared to changing local crop conditions or management. Restoring intensively managed agricultural fields for biodiversity conservation purposes proved to be difficult under certain circumstances and may even fail (grassland restoration). Therefore, maintaining and conserving present small fragments of semi-natural habitats in the agricultural landscape (“preserving what is still occurring”), and planting or sowing new elements should be the first priority of AES.
The original contributions presented in the study are included in the article/Supplementary materials, further inquiries can be directed to the corresponding author.
PJ participated to the elaboration of the study, performed the analysis, and wrote the manuscript. SP identified the spiders. CM reviewed the manuscript. All authors contributed to the article and approved the submitted version.
The authors thank the farmers for their cooperation, G. Blandenier, X. Heer, J. Steiger for the spider identification, D. Berner, F. Bosshart, S. Breitenmoser, S. Buholzer, J. Dudler, T. Gerdil, M. Hunziker, B. Leroy-Beaulieu, B. Peter, C. Rösli, S. Seidel, N. Suarez, M. Waldburger and K. Zobrist for field collection and sorting. We thank Felix Herzog for critical review of the manuscript and suggestions. This study comes from evaluation projects initiated by the Swiss Federal Government.
The authors declare that the research was conducted in the absence of any commercial or financial relationships that could be construed as a potential conflict of interest.
All claims expressed in this article are solely those of the authors and do not necessarily represent those of their affiliated organizations, or those of the publisher, the editors and the reviewers. Any product that may be evaluated in this article, or claim that may be made by its manufacturer, is not guaranteed or endorsed by the publisher.
The Supplementary material for this article can be found online at: https://www.frontiersin.org/articles/10.3389/fevo.2022.866947/full#supplementary-material
Albrecht, M., Schmid, B., Obrist, M. K., Schüpbach, B., Kleijn, D., and Duelli, P. (2010). Effects of ecological compensation meadows on arthropod diversity in adjacent intensively managed grassland. Biol. Conserv. 143, 642–649. doi: 10.1016/J.BIOCON.2009.11.029
Anderson, M. J. (2001). A new method for non-parametric multivariate analysis of variance. Austral. Ecol. 26, 32–46. doi: 10.1111/J.1442-9993.2001.01070.PP.X
Anderson, M. J. (2004). DISTLM v. 5: a FORTRAN computer program to calculate a distance-based multivariate analysis for a linear model. Department of Statistics, University of Auckland, New Zealand, 10:2016.
Anderson, M. J., Ellingsen, K. E., and McArdle, B. H. (2006). Multivariate dispersion as a measure of beta diversity. Ecol. Lett. 9, 683–693. doi: 10.1111/j.1461-0248.2006.00926.x
Anderson, M., and Ter Braak, C. (2010). Permutation tests for multi-factorial analysis of variance. J. Stat. Comput. Simul. 73, 85–113. doi: 10.1080/00949650215733
Aviron, S., Nitsch, H., Jeanneret, P., Buholzer, S., Luka, H., Pfiffner, L., et al. (2009). Ecological cross compliance promotes farmland biodiversity in Switzerland. Front. Ecol. Environ. 7, 247–252. doi: 10.1890/070197
Bailey, D., Schmidt-Entling, M. H., Eberhart, P., Herrmann, J. D., Hofer, G., Kormann, U., et al. (2010). Effects of habitat amount and isolation on biodiversity in fragmented traditional orchards. J. Appl. Ecol. 47, 1003–1013. doi: 10.1111/J.1365-2664.2010.01858.X
Batáry, P., Báldi, A., Sárospataki, M., Kohler, F., Verhulst, J., Knop, E., et al. (2010). Effect of conservation management on bees and insect-pollinated grassland plant communities in three European countries. Agric. Ecosyst. Environ. 136, 35–39. doi: 10.1016/J.AGEE.2009.11.004
Batáry, P., Dicks, L. V., Kleijn, D., and Sutherland, W. J. (2015). The role of agri-environment schemes in conservation and environmental management. Conserv. Biol. 29, 1006–1016. doi: 10.1111/cobi.12536
Benton, T. G., Vickery, J. A., and Wilson, J. D. (2003). Farmland biodiversity: is habitat heterogeneity the key? Trends Ecol. Evol. 18, 182–188. doi: 10.1016/S0169-5347(03)00011-9
Biffi, S., Traldi, R., Crezee, B., Beckmann, M., Egli, L., Epp Schmidt, D., et al. (2021). Aligning agri-environmental subsidies and environmental needs: a comparative analysis between the US and EU. Environ. Res. Lett. 16:054067. doi: 10.1088/1748-9326/ABFA4E
Birrer, S., Spiess, M., Herzog, F., Jenny, M., Kohli, L., and Lugrin, B. (2007). The Swiss Agri-environment scheme promotes farmland birds: but only moderately. J. Ornithol. 148, 295–303. doi: 10.1007/S10336-007-0237-Y/FIGURES/7
Bisang, I., Lienhard, L., and Bergamini, A. (2021). Three decades of field surveys reveal a decline of arable bryophytes in the Swiss lowlands despite agri-environment schemes. Agric. Ecosyst. Environ. 313:107325. doi: 10.1016/J.AGEE.2021.107325
Boetzl, F. A., Krauss, J., Heinze, J., Hoffmann, H., Juffa, J., König, S., et al. (2021). A multitaxa assessment of the effectiveness of Agri-environmental schemes for biodiversity management. Proc. Natl. Acad. Sci. U. S. A. 118:e2016038118. doi: 10.1073/pnas.2016038118
Borcard, D., and Legendre, P. (2002). All-scale spatial analysis of ecological data by means of principal coordinates of neighbour matrices. Ecol. Model. 153, 51–68. doi: 10.1016/S0304-3800(01)00501-4
Borcard, D., Legendre, P., Avois-Jacquet, C., and Tuomisto, H. (2004). Dissecting the spatial structure of ecological data at multiple scales. Ecology 85, 1826–1832. doi: 10.1890/03-3111
Candel, J. J. L., Lakner, S., and Peer, G. (2021). Europe’s reformed agricultural policy disappoints. Nature 595:650. doi: 10.1038/D41586-021-02047-Y
Chao, A., Chazdon, R. L., and Shen, T. J. (2005). A new statistical approach for assessing similarity of species composition with incidence and abundance data. Ecol. Lett. 8, 148–159. doi: 10.1111/J.1461-0248.2004.00707.X
Chaplin, S., Mills, J., and Chiswell, H. (2021). Developing payment-by-results approaches for agri-environment schemes: experience from an arable trial in England. Land Use Policy 109:83. doi: 10.1016/j.landusepol.2021.105698
Chesson, P. (2000). Mechanisms of maintenance of species diversity. Annu. Rev. Ecol. Evol. Syst. 31, 343–366.
Clough, Y., Holzschuh, A., Gabriel, D., Purtauf, T., Kleijn, D., Kruess, A., et al. (2007). Alpha and beta diversity of arthropods and plants in organically and conventionally managed wheat fields. J. Appl. Ecol. 44, 804–812. doi: 10.1111/J.1365-2664.2007.01294.X
Clough, Y., Kruess, A., Kleijn, D., and Tscharntke, T. (2005). Spider diversity in cereal fields: comparing factors at local, landscape and regional scales. J. Biogeogr. 32, 2007–2014. doi: 10.1111/J.1365-2699.2005.01367.X
Colwell, R. K., and Elsensohn, J. E. (2014). EstimateS turns 20: statistical estimation of species richness and shared species from samples, with non-parametric extrapolation. Ecography 37, 609–613. doi: 10.1111/ECOG.00814
Diaz, S., Settele, J., Brondízio, E., Ngo, H., Guèze, M., and Agard, J., … Butchart, S. (2019). Summary for policymakers of the global assessment report on biodiversity and ecosystem services of the intergovernmental science-policy platform on biodiversity and ecosystem services. Report, Paris, France. Available at: https://bit.ly/37HN35q (Accessed December 2, 2021).
Dudley, N., and Alexander, S. (2017). Agriculture and biodiversity: a review. Biodiversity 18, 45–49. doi: 10.1080/14888386.2017.1351892
Dufrêne, M., and Legendre, P. (1997). Species assemblages and indicator species: the need for a flexible asymmetrical approach. Ecol. Monogr. 67, 345–366.
Ekroos, J., Olsson, O., Rundlöf, M., Wätzold, F., and Smith, H. G. (2014). Optimizing agri-environment schemes for biodiversity, ecosystem services or both? Biol. Conserv. 172, 65–71. doi: 10.1016/J.BIOCON.2014.02.013
Feng, L., Arvidsson, F., Smith, H. G., and Birkhofer, K. (2021). Fallows and permanent grasslands conserve the species composition and functional diversity of carabid beetles and linyphiid spiders in agricultural landscapes. Insect Cons. Divers. 14, 825–836. doi: 10.1111/ICAD.12520
Forman, R. T. T., and Baudry, J. (1984). Hedgerows and hedgerow networks in landscape ecology. Environ. Manag. 8, 495–510. doi: 10.1007/BF01871575
Frank, T., and Nentwig, W. (1995). Groung dwelling spiders (Araneae) in sown weed strips and adjacent fields. Acta Oecol. 16, 179–193.
Gabriel, D., Roschewitz, I., Tscharntke, T., and Thies, C. (2006). Beta diversity at different spatial scales plant communities in organic and conventional agriculture. Ecol. Appl. 16, 2011–2021. doi: 10.1890/1051-0761(2006)016[2011:BDADSS]2.0.CO;2
Gallé, R., Geppert, C., Földesi, R., Tscharntke, T., and Batáry, P. (2020). Arthropod functional traits shaped by landscape-scale field size, local Agri-environment schemes and edge effects. Bas. Appl. Ecol. 48, 102–111. doi: 10.1016/J.BAAE.2020.09.006
Gámez-Virués, S., Perović, D. J., Gossner, M. M., Börschig, C., Blüthgen, N., De Jong, H., et al. (2015). Landscape simplification filters species traits and drives biotic homogenization. Nat. Commun. 6, 1–8. doi: 10.1038/ncomms9568
García-Navas, V., Martínez-Núñez, C., Tarifa, R., Molina-Pardo, J. L., Valera, F., Salido, T., et al. (2022). Partitioning beta diversity to untangle mechanisms underlying the assembly of bird communities in Mediterranean olive groves. Divers. Distrib. 28, 112–127. doi: 10.1111/DDI.13445
Garratt, M. P. D., Senapathi, D., Coston, D. J., Mortimer, S. R., and Potts, S. G. (2017). The benefits of hedgerows for pollinators and natural enemies depends on hedge quality and landscape context. Agric. Ecosyst. Environ. 247, 363–370. doi: 10.1016/j.agee.2017.06.048
Gayer, C., Berger, J., Dieterich, M., Gallé, R., Reidl, K., Witty, R., et al. (2021). Flowering fields, organic farming and edge habitats promote diversity of plants and arthropods on arable land. J. Appl. Ecol. 58, 1155–1166. doi: 10.1111/1365-2664.13851
Gossner, M. M., Lewinsohn, T. M., Kahl, T., Grassein, F., Boch, S., Prati, D., et al. (2016). Land-use intensification causes multitrophic homogenization of grassland communities. Nature 540, 266–269. doi: 10.1038/nature20575
Gotelli, N. J., and Colwell, R. K. (2001). Quantifying biodiversity: procedures and pitfalls in the measurement and comparison of species richness. Ecol. Lett. 4, 379–391. doi: 10.1046/J.1461-0248.2001.00230.X
Herzog, F. (1998). Streuobst: a traditional agroforestry system as a model for agroforestry development in temperate Europe. Agrofor. Syst. 42, 61–80. doi: 10.1023/A:1006152127824
Herzog, F., Dreier, S., Hofer, G., Marfurt, C., Schüpbach, B., Spiess, M., et al. (2005). Effect of ecological compensation areas on floristic and breeding bird diversity in Swiss agricultural landscapes. Agric. Ecosyst. Environ. 108, 189–204. doi: 10.1016/j.agee.2005.02.003
Jeanneret, P., Schüpbach, B., Pfiffner, L., Herzog, F., and Walter, T. (2003a). The Swiss agri-environmental programme and its effects on selected biodiversity indicators. J. Nat. Conserv. 11, 213–220. doi: 10.1078/1617-1381-00049
Jeanneret, P., Schüpbach, B., Pfiffner, L., and Walter, T. (2003b). Arthropod reaction to landscape and habitat features in agricultural landscapes. Landsc. Ecol. 18, 253–263. doi: 10.1023/A:1024496712579
Jose, S. (2009). Agroforestry for ecosystem services and environmental benefits: an overview. Agrofor. Syst. 76, 1–10. doi: 10.1007/S10457-009-9229-7/TABLES/2
Kehinde, T., and Samways, M. J. (2014). Effects of vineyard management on biotic homogenization of insect-flower interaction networks in the Cape Floristic Region biodiversity hotspot. J. Insect Conserv. 18, 469–477. doi: 10.1007/s10841-014-9659-z
Kleijn, D., Baquero, R. A., Clough, Y., Díaz, M., Esteban, J., Fernández, F., et al. (2006). Mixed biodiversity benefits of Agri-environment schemes in five European countries. Ecol. Lett. 9, 243–254. doi: 10.1111/j.1461-0248.2005.00869.x
Knop, E., Kleijn, D., Herzog, F., and Schmid, B. (2006). Effectiveness of the Swiss Agri-environment scheme in promoting biodiversity. J. Appl. Ecol. 43, 120–127. doi: 10.1111/J.1365-2664.2005.01113.X
Kolb, S., Uzman, D., Leyer, I., Reineke, A., and Entling, M. H. (2020). Differential effects of semi-natural habitats and organic management on spiders in viticultural landscapes. Agric. Ecosyst. Environ. 287:106695. doi: 10.1016/J.AGEE.2019.106695
Kovács-Hostyánszki, A., Soltész, Z., Szigeti, V., Somay, L., and Báldi, A. (2021). Non-rotational set-aside fields improve reproductive success of cavity-nesting bees and wasps at the landscape scale, but have no effect on other wild bees and hoverflies in mid-summer. Agric. Ecosyst. Environ. 308:107255. doi: 10.1016/J.AGEE.2020.107255
Larsen, S., Chase, J. M., Durance, I., and Ormerod, S. J. (2018). Lifting the veil: richness measurements fail to detect systematic biodiversity change over three decades. Ecology 99, 1316–1326. doi: 10.1002/ecy.2213
Le Provost, G., Badenhausser, I., Le Bagousse-Pinguet, Y., Clough, Y., Henckel, L., Violle, C., et al. (2020). Land-use history impacts functional diversity across multiple trophic groups. Proc. Natl. Acad. Sci. 117, 1573–1579. doi: 10.1073/pnas.1910023117
Legendre, P., Borcard, D., and Peres-Neto, P. R. (2005). Analyzing beta diversitu: partitioning the spatial variation of community composition data. Ecol. Monogr. 75, 435–450. doi: 10.1890/05-0549
Legendre, P., Borcard, D., and Peres-Neto, P. R. (2008). Analyzing or explaining beta diversity? Com. Ecol. 89, 3238–3244. doi: 10.1890/07-0272.1
Mader, V., Diehl, E., Fiedler, D., Thorn, S., Wolters, V., and Birkhofer, K. (2017). Trade-offs in arthropod conservation between productive and non-productive agri-environmental schemes along a landscape complexity gradient. Insect Cons. Divers. 10, 236–247. doi: 10.1111/ICAD.12220
Marino, P. C., and Landis, D. A. (1996). Effect of landscape structure on parasitoid diversity and parasitism in agroecosystems. Ecol. Appl. 6, 276–284. doi: 10.2307/2269571
Martínez-Núñez, C., Manzaneda, A. J., Isla, J., Tarifa, R., Calvo, G., Molina, J. L., et al. (2020). Low-intensity management benefits solitary bees in olive groves. J. Appl. Ecol. 57:13511. doi: 10.1111/1365-2664.13511
Martínez-Núñez, C., Manzaneda, A. J., Lendínez, S., Pérez, A. J., Ruiz-Valenzuela, L., and Rey, P. J. (2019). Interacting effects of landscape and management on plant–solitary bee networks in olive orchards. Funct. Ecol. 33:13465. doi: 10.1111/1365-2435.13465
Martínez-Núñez, C., Manzaneda, A. J., and Rey, P. J. (2020). Plant-solitary bee networks have stable cores but variable peripheries under differing agricultural management: bioindicator nodes unveiled. Ecol. Indic. 115:106422. doi: 10.1016/j.ecolind.2020.106422
Martínez-Núñez, C., Rey, P. J., Salido, T., Manzaneda, A. J., Camacho, F. M., and Isla, J. (2021). Ant community potential for pest control in olive groves: management and landscape effects. Agric. Ecosyst. Environ. 305:107185. doi: 10.1016/j.agee.2020.107185
Maurer, R., and Hänggi, A.. 1990. Katalog der schweizerischen Spinnen. 1. Auflage edition. Schweizerisches Zentrum für die kartographische Erfassung der Fauna (SZKF), Neuchâtel.
McArdle, B. H., and Anderson, M. J. (2001). Fitting multivariate models to community data: a comment on distance-based redundancy analysis. Ecology 82, 290–297. doi: 10.1890/0012-9658(2001)082[0290:FMMTCD]2.0.CO;2
Mccracken, M. E., Woodcock, B. A., Lobley, M., Pywell, R. F., Saratsi, E., Swetnam, R. D., et al. (2015). Social and ecological drivers of success in agri-environment schemes: the roles of farmers and environmental context. J. Appl. Ecol. 52, 696–705. doi: 10.1111/1365-2664.12412
McKinney, M. L., and Lockwood, J. L. (1999). Biotic homogenization: a few winners replacing many losers in the next mass extinction. Trends Ecol. Evol. 14, 450–453. doi: 10.1016/S0169-5347(99)01679-1
Moreno-Mateos, D., Barbier, E. B., Jones, P. C., Jones, H. P., Aronson, J., López-López, J. A., et al. (2017). Anthropogenic ecosystem disturbance and the recovery debt. Nat. Commun. 8:14163. doi: 10.1038/ncomms14163
Niskanen, O., Tienhaara, A., Haltia, E., and Pouta, E. (2021). Farmers’ heterogeneous preferences towards results-based environmental policies. Land Use Policy 102:105227. doi: 10.1016/J.LANDUSEPOL.2020.105227
OECD 2008. Environmental performance of agriculture in OECD countries since 1990. Paris, France: OECD.
Peer, G., Zinngrebe, Y., Moreira, F., Sirami, C., Schindler, S., Müller, R., et al. (2019). A greener path for the EU common agricultural policy. Science 365, 449–451. doi: 10.1126/SCIENCE.AAX3146/SUPPL_FILE/AAX3146_RAWDATA.XLSX
Pozzi, S., and Borcard, D. (2016). Effects of dry grassland management on spider (Arachnida: Araneae) communities on the Swiss occidental plateau 8, 32–44. doi: 10.1080/11956860.2001.11682628,
Pozzi, S., Gonseth, Y., and Hänggi, A. (1998). Evaluation de l'entretien des prairies sèches du plateau occidental suisse par le biais de leurs peuplements arachnologiques (Arachnida: Araneae). Rev. Suisse Zool. 105, 465–485.
R Development Core Team 2018. R: A language and environment for statistical computing. R Foundation for Statistical Computing, Vienna, Austria.
Ravetto Enri, S., Nucera, E., Lonati, M., Alberto, P. F., and Probo, M. (2020). The biodiversity promotion areas: effectiveness of agricultural direct payments on plant diversity conservation in the semi-natural grasslands of the southern Swiss Alps. Biodivers. Conserv. 29, 4155–4172. doi: 10.1007/s10531-020-02069-4
Rey, P. J., Camacho, F. M., Tarifa, R., Martínez-Núñez, C., Salido, T., Pérez, A. J., et al. (2021). Persistence of seed dispersal in agroecosystems: effects of landscape modification and intensive soil management practices in avian Frugivores, Frugivory and seed deposition in olive croplands. Front. Ecol. Evol. 0:871. doi: 10.3389/FEVO.2021.782462
Řezáč, M., and Heneberg, P. (2018). Effects of uncut hay meadow strips on spiders. Biologia 73, 43–51. doi: 10.2478/S11756-018-0015-8/TABLES/2
Riedel, S., Lüscher, G., Meier, E., Herzog, F., and Hofer, G. (2019). Qualité écologique des prairies qui bénéficient des contributions à la biodiversité. Rech. Agron. Suisse 10, 80–87.
Rischen, T., Frenzel, T., and Fischer, K. (2021). Biodiversity in agricultural landscapes: different non-crop habitats increase diversity of ground-dwelling beetles (Coleoptera) but support different communities. Biodivers. Conserv. 30, 3965–3981. doi: 10.1007/S10531-021-02284-7
Robinson, M. L., and Strauss, S. Y. (2020). Generalists are more specialized in low-resource habitats, increasing stability of ecological network structure. Proc. Natl. Acad. Sci. 117, 2043–2048. doi: 10.1073/pnas.1820143117
Roth, T., Amrhein, V., Peter, B., and Weber, D. (2008). A Swiss agri-environment scheme effectively enhances species richness for some taxa over time. Agric. Ecosyst. Environ. 125, 167–172. doi: 10.1016/J.AGEE.2007.12.012
Ruas, S., Rotchés-Ribalta, R., Uallacháin, D., Ahmed, K. D., Gormally, M., Stout, J. C., et al. (2021). Selecting appropriate plant indicator species for result-based Agri-environment payments schemes. Ecol. Indic. 126:107679. doi: 10.1016/J.ECOLIND.2021.107679
Růžičková, J., Kádár, F., Szalkovszki, O., Kovács-Hostyánszki, A., Báldi, A., and Elek, Z. (2020). Scale-dependent environmental filtering of ground-dwelling predators in winter wheat and adjacent set-aside areas in Hungary. J. Insect Conserv. 24, 751–763. doi: 10.1007/S10841-020-00249-9/FIGURES/5
Samnegård, U., Alins, G., Boreux, V., Bosch, J., García, D., Happe, A. K., et al. (2019). Management trade-offs on ecosystem services in apple orchards across Europe: direct and indirect effects of organic production. J. Appl. Ecol. 56, 802–811. doi: 10.1111/1365-2664.13292
Schmidt, M. H., Roschewitz, I., Thies, C., and Tscharntke, T. (2005). Differential effects of landscape and management on diversity and density of ground-dwelling farmland spiders. J. Appl. Ecol. 42, 281–287. doi: 10.1111/J.1365-2664.2005.01014.X
Schmidt, M. H., Thies, C., Nentwig, W., and Tscharntke, T. (2008). Contrasting responses of arable spiders to the landscape matrix at different spatial scales. J. Biogeogr. 35, 157–166. doi: 10.1111/J.1365-2699.2007.01774.X
Schoener, T. W. (1974). Resource partitioning in ecological communities. Science 185, 27–39. doi: 10.1126/science.185.4145.27
Smart, S. M., Thompson, K., Marrs, R. H., Le Duc, M. G., Maskell, L. C., and Firbank, L. G. (2006). Biotic homogenization and changes in species diversity across human-modified ecosystems. Proc. R. Soc. B Biol. Sci. 273, 2659–2665. doi: 10.1098/RSPB.2006.3630
Tarifa, R., Martínez-Núñez, C., Valera, F., González-Varo, J. P., Salido, T., and Rey, P. J. (2021). Agricultural intensification erodes taxonomic and functional diversity in Mediterranean olive groves by filtering out rare species. J. Appl. Ecol. 58, 2266–2276. doi: 10.1111/1365-2664.13970
Topping, C. J., and Luff, M. L. (1995). Three factors affecting the pitfall trap catch of linyphiids (Araneae: Linyphiidae). Bull. Br. Arachnol. Soc. 10, 33–36.
Tscharntke, T., Karp, D. S., Chaplin-Kramer, R., Batáry, P., DeClerck, F., Gratton, C., et al. (2016). When natural habitat fails to enhance biological pest control – five hypotheses. Biol. Conserv. 204, 449–458. doi: 10.1016/J.BIOCON.2016.10.001
Tscharntke, T., Klein, A. M., Kruess, A., Steffan-Dewenter, I., and Thies, C. (2005). Landscape perspectives on agricultural intensification and biodiversity – ecosystem service management. Ecol. Lett. 8, 857–874. doi: 10.1111/j.1461-0248.2005.00782.x
Tuomisto, H., and Ruokolainen, K. (2008). Analyzing or explaining beta diversity? Reply. Ecology 89, 3244–3256. doi: 10.1890/08-1247.1
Tyllianakis, E., and Martin-Ortega, J. (2021). Agri-environmental schemes for biodiversity and environmental protection: how we are not yet “hitting the right keys.”. Land Use Policy 109:105620. doi: 10.1016/J.LANDUSEPOL.2021.105620
Wagner, H. H., and Edwards, P. J. (2001). Quantifying habitat specificity to assess the contribution of a patch to species richness at a landscape scale. Landsc. Ecol. 16, 121–131. doi: 10.1023/A:1011118007670
Warzecha, D., Diekötter, T., Wolters, V., and Jauker, F. (2021). Spatial configureuration and landscape context of wildflower areas determine their benefits to pollinator α- and β-diversity. Bas. Appl. Ecol. 56, 335–344. doi: 10.1016/J.BAAE.2021.08.001
Watts, K., Whytock, R. C., Park, K. J., Fuentes-Montemayor, E., Macgregor, N. A., Duffield, S., et al. (2020). Ecological time lags and the journey towards conservation success. Nat. Ecol. Evol. 4, 304–311. doi: 10.1038/s41559-019-1087-8
Wersebeckmann, V., Kolb, S., Entling, M. H., and Leyer, I. (2021). Maintaining steep slope viticulture for spider diversity. Glob. Ecol. Cons. 29:e01727. doi: 10.1016/J.GECCO.2021.E01727
Whittaker, R. H. (1956). Vegetation of the Great Smoky Mountains. Ecol. Monogr. 26, 1–80. doi: 10.2307/1943577
Whittingham, M. J. (2011). The future of agri-environment schemes: biodiversity gains and ecosystem service delivery? J. Appl. Ecol. 48, 509–513. doi: 10.1111/j.1365-2664.2011.01987.x
Wittig, B., Kemmermann, A. R., gen,, and Zacharias, D. (2006). An indicator species approach for result-orientated subsidies of ecological services in grasslands – a study in northwestern Germany. Biol. Conserv. 133, 186–197. doi: 10.1016/J.BIOCON.2006.06.004
Keywords: agri-environment scheme, semi-natural habitats, Araneae, alpha diversity, beta diversity, multivariate dispersion, indicator species
Citation: Jeanneret P, Pozzi S and Martinez Nuñez C (2022) Spiders indicate delivery of an agri-environment scheme at multiple diversity levels. Front. Ecol. Evol. 10:866947. doi: 10.3389/fevo.2022.866947
Received: 31 January 2022; Accepted: 18 November 2022;
Published: 15 December 2022.
Edited by:
James Moran, Atlantic Technological University, IrelandReviewed by:
Paulo A. V. Borges, University of the Azores, PortugalCopyright © 2022 Jeanneret, Pozzi and Martinez Nuñez. This is an open-access article distributed under the terms of the Creative Commons Attribution License (CC BY). The use, distribution or reproduction in other forums is permitted, provided the original author(s) and the copyright owner(s) are credited and that the original publication in this journal is cited, in accordance with accepted academic practice. No use, distribution or reproduction is permitted which does not comply with these terms.
*Correspondence: Philippe Jeanneret, cGhpbGlwcGUuamVhbm5lcmV0QGFncm9zY29wZS5hZG1pbi5jaA==
Disclaimer: All claims expressed in this article are solely those of the authors and do not necessarily represent those of their affiliated organizations, or those of the publisher, the editors and the reviewers. Any product that may be evaluated in this article or claim that may be made by its manufacturer is not guaranteed or endorsed by the publisher.
Research integrity at Frontiers
Learn more about the work of our research integrity team to safeguard the quality of each article we publish.