- 1Departamento de Biología y Geología, Física y Química Inorgánica, Universidad Rey Juan Carlos, Madrid, Spain
- 2Departamento de Biología, Área de Botánica, Universidad Autónoma de Madrid, Madrid, Spain
- 3Centro de Investigación en Biodiversidad y Cambio Global, Universidad Autónoma de Madrid, Madrid, Spain
- 4Oikobit LLC, Albuquerque, NM, United States
- 5Ecology and Evolutionary Biology, University of Michigan, Ann Arbor, MI, United States
A vast literature indicates that environment plays a paramount role in determining floristic composition in tropical forests. However, it remains unclear which are the most important environmental factors and their relative effect across different spatial scales, plant life forms or forest types. This study reviews the state of knowledge on the effect of soil and climate on floristic composition in tropical forests. From 137 publications, we collated information regarding: (1) spatial scale, continent, country, life form, and forest type; (2) proportion of variance in floristic composition explained by soil and climatic variables and how it varies across spatial scales; and (3) which soil and climate variables had a significant relationship on community composition for each life form and forest type. Most studies were conducted at landscape spatial scales (67%) and mainly in South America (74%), particularly in Brazil (40%). Studies majorly focused on trees (82%) and on lowland evergreen tropical forests (74%). Both soil and climate variables explained in average the same amount (14% each) of the variation observed in plant species composition, although soils appear to exert a stronger influence at smaller spatial scales while climate effect increases toward larger ones. Temperature, precipitation, seasonality, soil moisture, soil texture, aluminum, and base cations—calcium and magnesium–and their related variables (e.g., cation exchange capacity, or base saturation) were frequently reported as important variables in structuring plant communities. Yet there was variability when comparing different life forms or forest types, which renders clues about certain ecological peculiarities. We recommend the use of standardized protocols for collecting environmental and floristic information in as much as possible, and to fill knowledge gaps in certain geographic regions. These actions will be especially beneficial to share uniform data between researchers, conduct analysis at large spatial scales and get a better understanding of the link between soils and climate gradients and plant strategies, which is key to propose better conservation policies under the light of global change.
Introduction
Which factors determine organisms distribution and abundance patterns remains a paramount question in ecology (Sutherland et al., 2013). In this sense, two main groups of environmental variables have been of utmost interest: climate and soil-related factors. Identifying the relationships between floristic composition and these factors is a fundamental step to further comprehend the underlying mechanisms (Whittaker, 1960; Chave, 2008)—such as environmental filtering (Cadotte and Tucker, 2017)—but can also provide insights for ecosystems management and conservation (Libalah et al., 2020). Consequently, ecologists have largely investigated the effect of such factors over an array of biomes, among which tropical forests have attracted a special interest. Tropical forests are not only among the hottest terrestrial biodiversity hotspots (Myers et al., 2000; Slik et al., 2015) but also offer a vast variety of climatic and edaphic conditions (Townsend et al., 2008; Rahbek et al., 2019).
The role of climate in plant species distribution has been widely reported from earlier biogeographers (Humboldt and Bonpland, 1805) to contemporary ecologists (Willig et al., 2003; Morueta-Holme and Svenning, 2018), and thus climate has been acknowledged as a strong predictor of large-scale patterns of plant community composition across biomes (Hawkins et al., 2003; Woodward et al., 2004). Although more stable in terms of precipitation and temperature than other biomes, most tropical forests experience from mild to marked seasonal variation in rainfall (Golicher et al., 2008; Pennington et al., 2009) and stark temperature differences exist in tropical montane forests along elevation (Malhi et al., 2010). Those facts have been acknowledged to influence the distribution of species in tropical communities (Ter Steege et al., 2006; Engelbrecht et al., 2007; Esquivel-Muelbert et al., 2017).
The role of soil variation in determining floristic composition has been also broadly recognized, particularly at intermediate and landscape scales (Phillips et al., 2003a; Tuomisto et al., 2003b; Guevara Andino et al., 2021). In the hitherto most exhaustive review of the effects of soil factors on floristic composition in tropical forests, Sollins (1998) indicated that the most important edaphic variables that affect evergreen lowlands forests were, in a decreasing order of importance: phosphorus availability, aluminum content, moisture (in terms of drainage and water-holding capacity) and availability of base-metal cations (potassium, calcium, and magnesium). Recent studies, however, provide only partial support to these results, with differences arising mostly from variation in the soil variables measured, sampling methods, analytical approaches and spatial scales considered, making generalizations difficult to establish (Chave, 2008; Baraloto et al., 2013; Garzon-Lopez et al., 2014).
The main goal of this study is to examine whether a systematic literature review shows that variations in climate or soil account for variation in floristic composition at scales ranging from landscape to global. The specific goals were to: (1) describe the state of knowledge (by spatial scale, continent, country, life form, and forest type) concerning studies conducted in tropical forests that investigate the effects of environmental determinism in plant community composition; (2) ascertain the relative contribution of soil and climate drivers in determining floristic composition in terms of explained variance and how it varies across spatial scales; and (3) identify which soil and climate factors show a more significant influence with floristic composition for different life forms and forest types. Through this approach, we aim to shed light from scientific evidence compiled in the, to our knowledge, largest literature review to date to understand which soil and climate drivers influence floristic composition in tropical forests, identify potential particularities for different life forms or forest types and across spatial scales, and reveal gaps in knowledge.
Materials and Methods
Literature Search and Inclusion Criteria
We conducted a systematic search in the Web of Science and Scopus to identify publications that: (i) contained the strings “tropical forest” and “floristic composition” and [“environmental variables” or “climate” or “soil”] in their titles, abstracts and/or main text; and (ii) were published in English, Portuguese, or Spanish. We considered a climatic definition of the tropics (Feeley and Stroud, 2018).
We excluded from the analyses (i) works that focused exclusively on paleontology, remote sensing, agronomy, silviculture, restoration, etc.; (ii) studies on non-forest vegetation types (e.g., paramo, puna, or pampa); (iii) considering response variables other than species composition (e.g., species richness or diversity); and (iii) descriptive articles not assessing statistically the effect of soil or climatic variables on floristic composition. The literature browsing was finished in June 2020.
Data Retrieved
For the selected publications, we extracted data from 37 categorical, discrete, and/or continuous variables regarding: (i) the spatial scale of the study: landscape (<100 km), regional (100–1,000 km), continental (>1,000 km but one continent), and global (more than one continent); (ii) the continent and country investigated; (iii) as no studies consider the whole flora, the life form(s) studied: trees, shrubs, palms, ferns, lianas, and/or herbs; (iv) the forest type in relation to elevation (lowland or montane) and in relation to rainfall seasonality (evergreen or seasonal); (v) the sample vegetation grain size; (vi) the minimum individual size [such as diameter at breast height (DBH)]; (vii) the soil depth sampled; (viii) the percentage of variance explained by environmental variables, including soil variables, climatic variables, distance or space, and unexplained variation; and (ix) the specific soil variables [pH, phosphorus (P), potassium (K), calcium (Ca), magnesium (Mg), sodium (Na), iron (Fe), manganese (Mg), zinc (Zn), copper (Cu), aluminum (Al), silicon (Si), titanium (Ti), boron (B), nitrogen (N), carbon (C), carbon nitrogen ratio (C/N), Cation Exchange Capacity (CEC), electrical conductivity, exchangeable cations, base saturation, organic matter, moisture and texture], and/or climatic variables (precipitation, temperature, and seasonality) analyzed and whether they had (1) or not (0) a statistically significant effect on floristic composition.
Data Analysis
To describe the state of knowledge concerning studies investigating the effects of environmental determinism in plant communities (goal 1), we explored the distribution of studies by spatial scale, continent, country, life form, and forest type. To assess the relative contribution of climate and soil in plant species composition (goal 2), we estimated the frequency distribution of the explained variation in floristic composition with respect to soil and climatic variables, and how it changed across spatial scales. Finally, to identify key environmental variables driving floristic composition (goal 3), we calculated the percentage of studies where each soil and climate variable had a significant relationship on floristic composition for each life form and forest type. Publications encompassing several countries or considering multiple life forms, forest types or spatial scales, were accounted independently when possible, so one single study may eventually imply more than one record for data analyses purposes.
Results
Literature Search
We found 829 articles matching the search strings, of which only 124 met all the inclusion criteria. We incorporated 13 additional studies from our daily-working database not including some or any search strings (such as floristic composition) but falling into the scope of this work. Overall, we considered 137 publications (Supplementary Table 1). Yet a variable number of records were considered for the different analyses, as some of the publications reported results simultaneously at several spatial scales [e.g., landscape and regional (Normand et al., 2006)], and on different life forms [e.g., trees, shrubs, and lianas (López-Martínez et al., 2013)] or forest types [e.g., lowland and montane (Garibaldi et al., 2014)]. Additionally, for the analyses related to goals 2 and 3 there was no information available in all the publications, so the total number of records analyzed was lower than 137. Thus, hereinafter we will refer to publications (n = 137) or records (n ≠ 137) depending on the scope of the analysis. We indicate the sample size when presenting each of the specific results.
Most studies explored only soil effects on floristic composition (55%), followed by those exploring both soil and climate (31%), and those considering only climate (14%). A majority were in English (91%), with Portuguese (7%), and Spanish (2%) accounting for a minority.
Studies by Spatial Scales, Continents and Countries, Forest Types, and Life Forms
More than half of the publications (67%) reported results at landscape scale, whereas 32% had a regional extent and 12% covered a continental scope (Figure 1A and Supplementary Table 1). Only a minority (<1%) encompassed global scales.
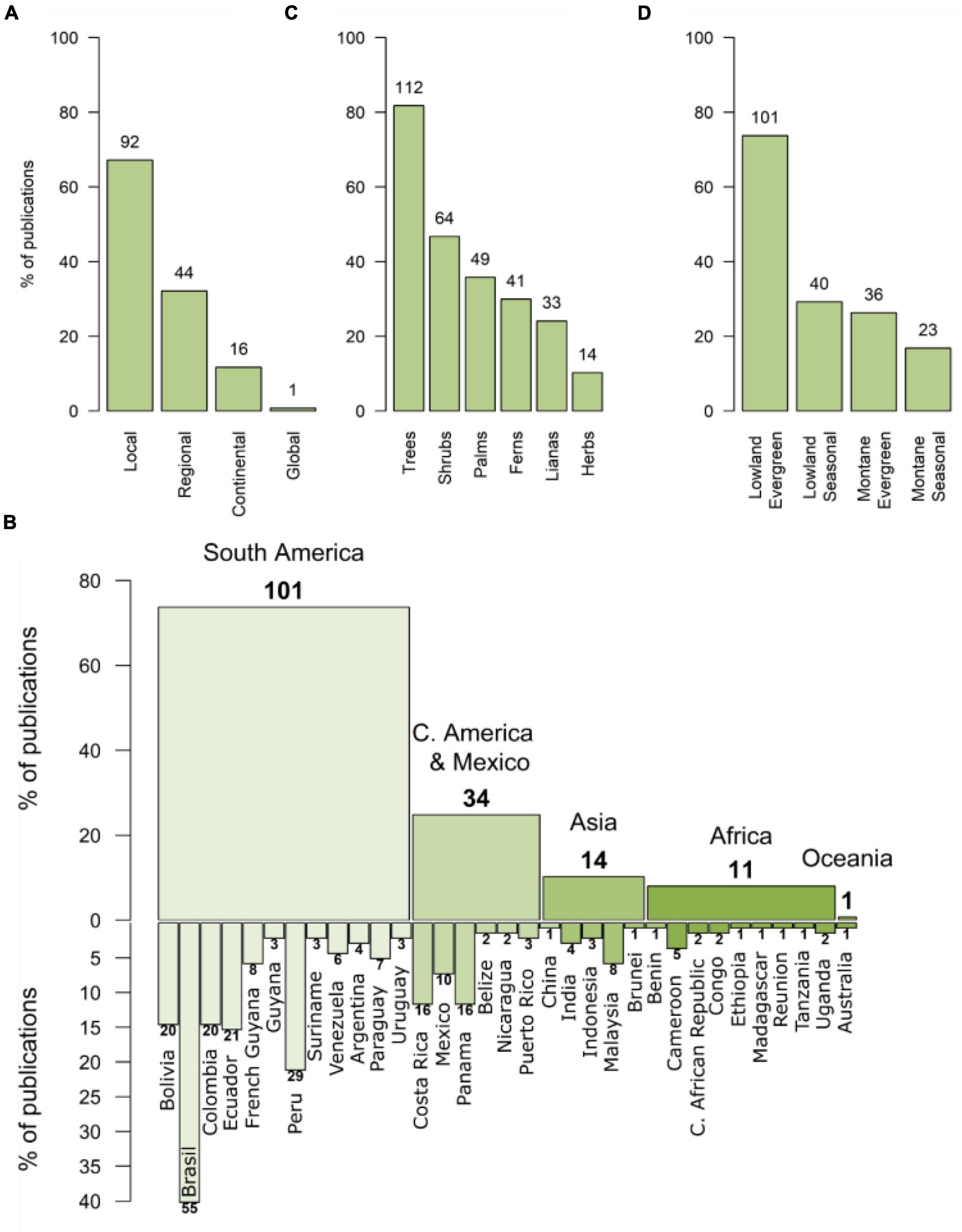
Figure 1. Distribution of 137 publications investigating the importance of soils and climate as drivers of floristic composition in tropical forests assorted by (A) spatial scale, (B) geographic region –continents and countries–, (C) life form, and (D) forest type. Numbers above the bars represent the number of records for each case. In (B) Guatemala, Honduras, El Salvador, and all Caribbean-island countries excepting Puerto Rico accounted for one record each but are not represented due to space limitation.
Regarding the geographical scope of the studies (Figure 1B and Supplementary Table 1), a large amount reported results from research conducted in South America (74%), with Brazil leading the ranking (40%), followed by Peru (21%) and Ecuador, Colombia, and Bolivia (15% each). Central America and Mexico were investigated in 24% of the studies, with Panama and Costa Rica concentrating the largest amount (12% each), followed by Mexico (7%). Only 10% of the publications contained results from Asia. These were spread over five countries, with Malaysia leading the ranking (6%), followed by India (3%). Publications for Africa were the scarcest, accounting only for 8%, and distributed over eight countries, being Cameroon the most representative (4%). Finally, just one publication (<1%) was conducted in Oceania.
Most publications reported results on the effects of environmental determinism on trees (82%) and shrubs (47%), followed by palms (36%), ferns (30%), lianas (24%), and lastly herbs (10%; Figure 1C and Supplementary Table 1). Concerning forest types, most publications, ca. two thirds (68.5%) were focused on evergreen forests, whereas seasonal forests accounted for near a third (31.5%; Figure 1D and Supplementary Table 1). A similar proportion exists between lowland and montane forests (70.5 and 29.5%, respectively).
There was a high heterogeneity among publications in terms of the soil and environmental variables considered, collection methods, inventory sizes, minimum plant size, laboratory protocols, and data analyses techniques (Supplementary Table 1). Plot area ranged from 0.0001 ha in studies focusing on herbs to 7,850 ha in studies considering trees, with plots of 0.25 (14%), 1 (11%), 0.01 (7%), 0.04, and 0.1 ha (ca. 6% each) being the most common. Minimum DBH ranged from 1 to 30 cm, being 10 (30%) and 5 cm (27%) the most common. Soil sampling depths ranged from 5 cm to 120 cm, depths of 20 (28%) and 10 cm (26%) being the most common. There was a plethora of approaches to analyze soil moisture, from measuring soil gravimetric water storage or qualitatively defining soil drainage levels to estimate moisture by considering distance to floodplains or the elevation relative to nearby streams.
Variance Explained by Soil and Climatic Drivers and Its Variation Across Spatial Scales
Almost a third of the publications (41/137; Supplementary Table 1) included analyses based on variance partitioning methods—for example, PCA, CCA, or RDA (Borcard et al., 1992; Legendre and Legendre, 2012)—to assess the effect of different groups of environmental variables in species composition. Albeit there was a high variability regarding the amount of variation explained by soils (from 1 to 58%) and climate (from 0.2 to 82%), both on average explained the same amount (14% each; Figure 2A). The amount of variance explained by soils and climate combined ranged from 0.4 to 21% (mean 9%). The amount of variance explained by distance and/or space ranged from 0 to 66% (mean 12%), whereas the amount of unexplained variance varied from 12 to 94% (mean 66%).
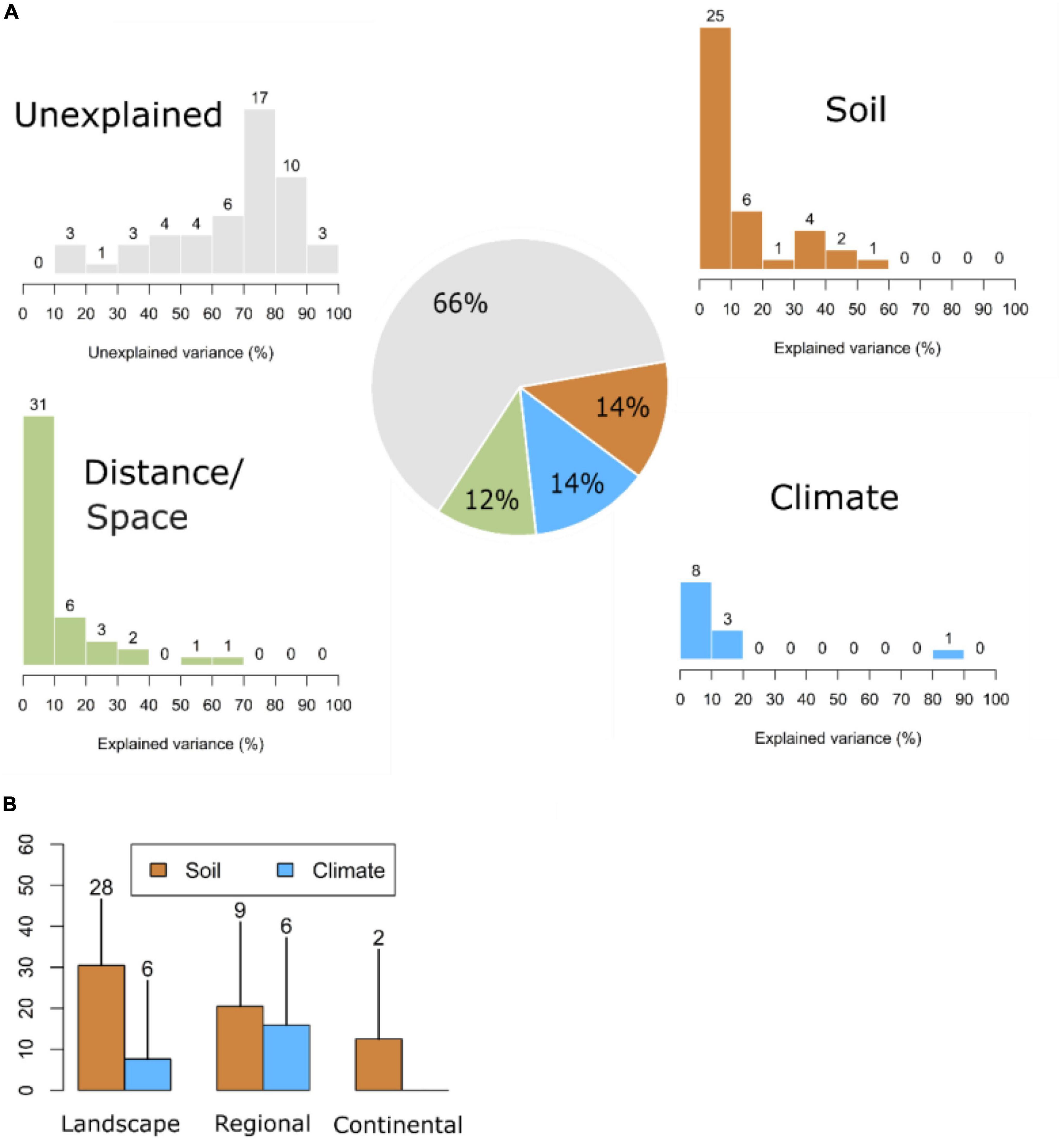
Figure 2. Percentage of variation (A) explained by soils, climate, distance/space, and unexplained and (B) by soil and climate across spatial scales in 41 publications investigating their relative importance as drivers of floristic composition in tropical forests using variance partitioning methods. Numbers above the bars represent the number of records (sample size) for each case and in the pie chart the average percentage of variation. Landscape scale (<100 km), regional scale (100–1,000 km), and continental scale (>1,000 km but one continent). Neither studies at global scales for any of the variables nor at continental scales for climate variables were found.
In relation to spatial scale (Figure 2B), our results showed that the explanatory power of soil variables decreased as the spatial extent of the study increased. Conversely, climate variables explained a larger variation of floristic composition as the spatial extent increased.
Effect of Soil and Climate Variables on Community Composition for Different Life Forms and Forest Types
Soil variables probed to drive floristic composition in most studies (statistically significant relationship in 96% of the records, n = 135; Supplementary Table 1). Among all, moisture (85%, n = 41), texture (66%, n = 95), Al (62%, n = 60), Ca (59%, n = 90), organic matter (58%, n = 53), C/N (58%, n = 12), and Mg (56%, n = 89) emerged as major drivers of floristic composition in tropical forests, regardless of the life form or forest type considered. Climate variables also emerged as important correlates of floristic composition (statistically significant relationship in 85% of the records, n = 65; Supplementary Table 1): temperature (75%, n = 44), precipitation (71%, n = 62), and seasonality (69%, n = 46).
For trees, soil moisture (87%), temperature (80%), precipitation and seasonality (78% each) were the most important variables driving floristic composition (Figure 3A and Supplementary Table 1). Other important variables were soil texture (66%), Al (63%), organic matter (61%), base saturation (57%), and CEC (56%). For shrubs, four variables were particularly relevant: soil moisture (81%), precipitation (80%), temperature and seasonality (75% each). Al (70%), soil texture (64%), Fe (62%), base saturation (57%), and pH (54%) also played an important role. For palms, the most important variables were soil moisture (94%), temperature (83%), precipitation and C/N (75% each), seasonality and Al (73% each), texture (72%), base saturation (70%), and organic matter (62%). Ferns were mainly associated to C/N (100%), soil moisture (90%), exchangeable cations (85%), soil texture (78%), precipitation (75%), temperature and organic matter (73% each), Mg (68%), and Al (67%). For lianas the main predictor was base saturation (100%), followed by soil moisture (83%), temperature (80%), organic matter (78%), Fe (75%), pH (74%), soil texture (71%), and seasonality. Herbs responded mainly to N and precipitation (100%), soil moisture (80%), Al and exchangeable cations (75% each) and P, Ca, Na, and temperature (67% each).
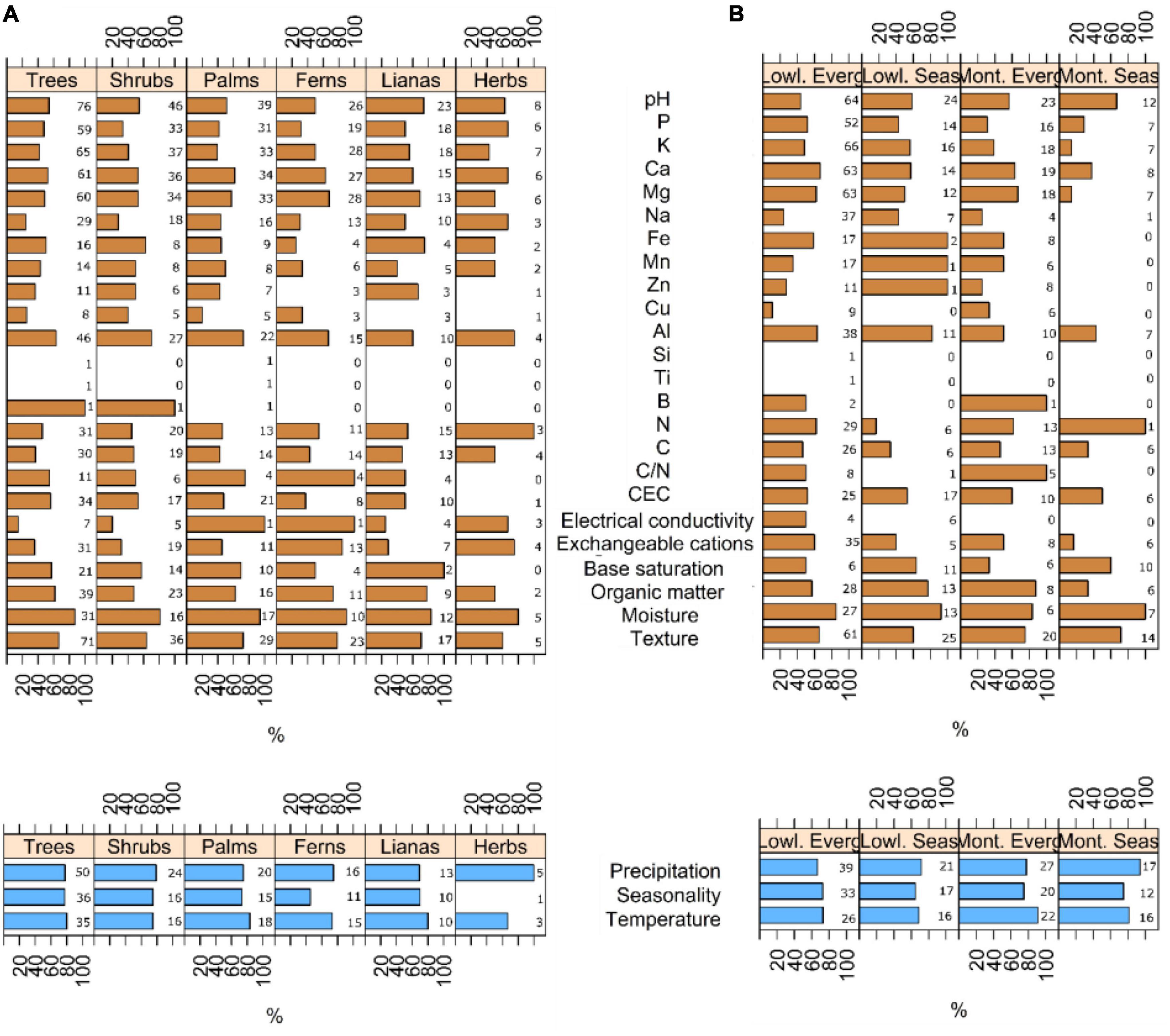
Figure 3. Percentage of publications in which soil variables (brown bars) and climate variables (blue bars) had effects on floristic composition in tropical forests attending to different (A) life forms and (B) forest types. Numbers rear to the bars represent the number of records (sample size) for each case.
Across forest types, soil moisture was the only soil variable consistently ranking among the three most important factors (Figure 3B and Supplementary Table 1). Soil moisture ranked higher in seasonal [both in lowlands (92%) and montane (100%)] than in evergreen forests [both in lowlands (85%) and montane (83%)]. Similarly, precipitation showed a larger effect on seasonal (71% in lowlands and 94% in montane) than in evergreen forests (67% in lowlands and 78% in montane). Temperature was more relevant in montane [both in evergreen (91%) and seasonal (81%)] than in lowland forests [both in evergreen (73%) and seasonal (69%)]. In the case of lowland evergreen forests, other important variables were seasonality (73%), Ca (67%), and texture (65%), whereas Al (82%), organic matter (77%), seasonality (65%), and base saturation (64%) showed a larger effect in lowland seasonal forests (Figure 3). For montane evergreen forests, other relevant predictors were C/N (100%), organic matter (87%), seasonality (75%), and moisture (67%). For montane seasonal forests, seasonality (75%), soil texture (71%), pH (67%), and base saturation (60%).
Discussion
Knowledge Availability by Spatial Scales, Continents and Countries, Life Forms, and Forest Types
Most publications investigated floristic composition at small spatial scales (landscape) and the number of studies decreased toward larger ones (Figure 1A). While studies at regional and especially landscape scales rely upon primary data, studies at continental and global scales typically use data acquired by different groups, which use different protocols in floristics (e.g., plot size, or minimum DBH) and soil characterization (e.g., soil depth or moisture, and/or nutrients quantification methods; see Supplementary Table 1). Despite this potentially leads to certain noise, these studies currently are the only alternative to conduct studies at continental and global scales. Given this need for collaborative science to gain insights at these scales, the more standardized the sampling protocols are, the better for the sake of data integration from different sources (Baker et al., 2021).
The Neotropics is the most intensely studied biogeographical region (Figure 1B). This is due, on one hand, to the creation of research centers (e.g., the Organization for Tropical Studies, the Smithsonian Tropical Research Institute or the Instituto Nacional de Pesquisas da Amazônia) and field stations (Whitesell et al., 2002), which have increased scientific research in countries as Costa Rica, Panama, and Brazil (Bawa et al., 2004) while others (e.g., Nicaragua or Honduras) remain understudied (Stocks et al., 2008). This problem is emphasized upon consideration of countries’ area, as few of the countries with larger number of studies are relatively small (such as Costa Rica)—and vice versa—which lead to certain regions being relatively understudied (such as the center of the Amazon basin). Besides, albeit many studies use external funds from temperate countries/institutions and those projects could be conducted anywhere in the tropics, there are practical limitations in some countries, such as weak systems of protected areas, tedious bureaucracy, or lack of political stability. All these factors hinder the capacity to conduct research even if funding is not a constraint (Watkins and Donnelly, 2005; Dangles et al., 2016). Meanwhile, on the other hand, certain tropical areas in Asia and Africa are poorly studied compared to the American countries (Clark, 1985), as most Asian an African countries lack a science-oriented political agenda in comparison to, for example, Brazil (Cayuela et al., 2018). By committing more resources to science, countries can skew their capacity toward increasing biodiversity research (Holmgren and Schnitzer, 2004; Malhado et al., 2014). At this point, it is illustrative how countries similar in size and incomes reveal contrasting number of studies: Malaysia (5) and Thailand (0; Abd Manaf et al., 2019). More resources are particularly needed to increase representativeness of remote, under sampled and even unsampled regions, –e.g., the central Congo Basin and New Guinea– (ForestPlots.net, 2021).
Trees are the dominant life form in forest ecosystems, and thus most research on forest environmental determinism focuses on trees (Figure 1C and Supplementary Table 1). Trees, however, neither are representative of the whole plant community nor always respond to ecological drivers in the same way as other life forms (Ruokolainen et al., 2007; Pasion et al., 2018). Hence, the consideration of life forms other than trees for which investigations are scarce (e.g., lianas and herbs) might render additional clues about the role of certain environmental variables in driving floristic community composition (see section “Particular Effects of Soil and Climate Across Different Life Forms and Forest Types”).
Most papers in this review investigate lowland forests (Figure 1D and Supplementary Table 1), which might be related to the fact that they cover a larger proportion in the tropics than montane forests (Bruijnzeel et al., 2011) and to the difficulty of working in montane slopes (Bubb et al., 2004). Concerning evergreen versus seasonal forests, the latter are understudied, not only because they occupy a smaller geographical extent, but also for their overexploited situation, consequence of agriculture and livestock farming (Sánchez-Azofeifa et al., 2005; DRYFLOR et al., 2016).
Soil and Climate Effects on Floristic Composition and Variation Across Spatial Scales
Our results indicated that, on average, 28% of the variance in floristic composition was explained by environmental variables (Figure 2A and Supplementary Table 1). This result is very similar (26%) to the meta-analysis conducted by Soininen (2014), which included organisms other than plants such as microorganisms, invertebrates and vertebrates. It also resembles the results found for plants in Baldeck et al. (2016; 24%), Neves et al. (2015; 21%), or Arellano et al. (2016b; 20%). We found, however, wide ranges in the variation explained by soil (from 1 to 58%) or climatic variables (from 0.2 to 82%). This variability in results from different studies reflects true ecological differences between the studied systems, but also spurious methodological differences associated with the great heterogeneity in the methods used (e.g., spatial scale and sample grain size considered, sampling, and analysis techniques, etc.; Phillips et al., 2003b; Magnusson et al., 2005; Arellano et al., 2016a). Without methodological standardization –which in practice is hardly achievable (see section “Conclusions and Future Directions”)– it is not possible to disentangle both.
Soil variables have been related to floristic composition in a large number of studies (e.g., Phillips et al., 2003a; Costa et al., 2005; Bohlman et al., 2008). We found soils correlated with plant composition to some degree in 96% of the records, explaining on average 14% of variation in floristic composition. On the other hand, climatic variables explained another 14% of the variation in plant assemblages, detecting positive correlations with floristic composition in 85% of the records. This influence of climate in tropical floristic composition was already described by some seminal papers and largely supported afterward (Gentry, 1988; Chust et al., 2006; Saiter et al., 2016; Borges-Prata et al., 2018).
Considering the spatial scale of the studies shed clues on the relative influence of soil versus climate variables on plant communities. Our results showed that soil variables and floristic composition were highly correlated at small spatial scales, particularly at landscape ones, while climate variables become more important as spatial scale increases (Figure 2B and Supplementary Table 1). Those findings are well in agreement with other studies (Palmer, 2007; Siefert et al., 2012) since climate conditions at small spatial scales are relatively homogeneous, whereas soil drivers at this scale can be highly heterogeneous within just few kilometers (Lechowicz and Bell, 1991; Bell et al., 1993; Ryel et al., 1996). For instance, soil moisture varies a lot depending on the microtopography of the terrain–even within a region of uniform precipitation– (Valencia et al., 2004; Ledo et al., 2013), and soil nutrients and texture can also depend on landscape topography (Porder et al., 2005; Weintraub et al., 2015) and rock outcrops (Isichei et al., 1990). Unfortunately, the number of studies analyzing variation partitioning in floristic composition at large spatial scales are scarce or inexistent due to the difficulty of obtaining the required data (see section “Knowledge by Spatial Scales, Continents, Countries, Life Forms, and Forest Types”). This limitation hampers our capacity to assess the dominant effect of different environmental drivers across the whole spectra of spatial scales.
Particular Effects of Soil and Climate Across Different Life Forms and Forest Types
Regarding the relative effect of soil variables in floristic composition, our results identified moisture (85% of the studies), texture (66%), organic matter (62%), Al (62%), Ca (59%), C/N (58%), and Mg (56%) as the most important drivers of floristic composition considering all life forms and forest types. This is well in agreement with the results reported by Sollins (1998), who found that for lowland evergreen tropical forests the most relevant factors were P, Al, moisture, availability of base cations (K, Ca, and Mg), micronutrients (Zn), and N.
Soil moisture has been broadly reported as one of the key edaphic variables driving floristic composition and plant distribution in the tropics since it is a proxy for plant water availability (Comita and Engelbrecht, 2009). Correspondingly, it systematically appeared among the top three most important soil variables across all life forms and forest types (Figure 3 and Supplementary Table 1). The same occurs with base cations (Ca, Mg, and K), which are crucial nutrients for plants and hence key for soil fertility (Marschnell, 2012). Across life forms, either single cations, their sum or other soil parameters that informs about their availability for plants [e.g., CEC or base saturation (Mikkelsen, 2011)] probed to play a crucial role in determining floristic composition (Tuomisto et al., 2003a; Guèze et al., 2013; Zuquim et al., 2019). Organic matter, by supplying nutrients and providing CEC through its decomposition (Ross, 1993), is also a relevant driver of floristic composition in tropical forests (e.g., White and Hood, 2004; Slik et al., 2009; Khairil et al., 2014).
Nitrogen is of essential importance for plants and, in many ecosystems, its limitation leads to problems in plant growth (Jarvis and Linder, 2000; Finzi et al., 2006). However, Sollins (1998) pointed out N as the less influential of the soil factors driving floristic composition in tropical forests. In the same way, our results (Figure 3 and Supplementary Table 1) revealed that N is of utmost relevance only for herbs (and C/N also for ferns and in montane evergreen forests). Such results could be explained by the fact that tropical species are more adaptable than temperate ones to use whatever source of N available in the soil, thus being this element of less significance than others (Houlton et al., 2007).
In the case of phosphorus, while Sollins (1998) found that it was the most critical soil variable, our review has shown it to be of relatively limited relevance (46% on average, up to a 52% in lowland evergreen forests and only ranking among the top six important soil variables for herbs; Figure 3A and Supplementary Table 1), and several authors neither identified this element as key for community composition in Neotropical forests (Johnston, 1992; Tuomisto et al., 2003a; Macía et al., 2007; Higgins et al., 2011). In fact, the role of P is one of the most controversial topics in tropical soil ecology (Vitousek, 1984; Turner et al., 2018). For instance, different soil parent materials, weathering intensities and topographies can cause differences in P content across landscapes (Walker and Syers, 1976; Mage and Porder, 2013). However, P depletion is thought to be unlikely in areas of moderate uplift, such us in Central America or Southeast Asia, due to the advection of P through the soil into the rooting zone (Porder et al., 2007), and the Amazon basin and the rear oriental Andean slopes might not be as impoverished in P as previously though considering that wind-carried African dust containing P—or other elements, such as Ca (Pett-Ridge et al., 2009)—fertilize these areas (Yu et al., 2015). Furthermore, the availability of P to plants is particularly challenging to measure given the great range of its organic and inorganic chemical forms occurring in soil and the variety of plant mechanisms acquiring them (Turner, 2008; Condit et al., 2013).
Another important element for plants is aluminum, which is toxic given its deleterious effects for plant growth and function (Brunner and Sperisen, 2013). Indeed, Al ranked among the top three soil variables for all life forms except for ferns and lianas (Figure 3A and Supplementary Table 1). Tropical ferns have been demonstrated to accumulate Al, what could explain their role as pioneer species in high Al content soils (Schmitt et al., 2017). Likewise, certain angiosperms families (such as Rubiaceae) have also been proved to accumulate Al (Jansen et al., 2002). Those facts could help to explain why lowlands forests’ composition is more influenced by Al content than montane one (Figure 3B; Trujillo et al., 2019).
Soil texture is another influential variable, as not only determines water availability for plants, but also other variables linked with plant growth such as CEC (Dodd and Lauenroth, 1997), root respiration (Walters et al., 1992), and microbial activity (Chau et al., 2011). Accordingly, soil texture was among the top five soil variables for all life forms except for herbs but even for this life form it had effect in 60% of the records (Figure 3A and Supplementary Table 1).
If focusing on forest types, results for soil variables (Figure 3B and Supplementary Table 1) were similar to those found for life forms (Figure 3A and Supplementary Table 1) being moisture, Al, texture and cations —and cations related variables– among the top relevant variables. The most interesting difference among forest types arises when focusing on pH. pH deeply affects soil chemical and physical properties ultimately affecting plants root system and nutrient uptake (Kidd and Proctor, 2001), and seem to have a larger influence on floristic composition in seasonal than in evergreen forests. This fact constitutes an interesting observation that requires further research, especially considering the soil acidification of tropical ecosystems (Lu et al., 2014).
Concerning the relative importance of climate variables in floristic composition (Figure 3B and Supplementary Table 1), our results show that temperature, precipitation, and seasonality play roles of utmost relevance in tropical forests. Those variables exert an influence on 75, 71, and 70% of the studies, respectively, independently of life forms or forest types considered.
In the case of precipitation, it showed a larger effect on seasonal (83% in average) than in evergreen forests (73%). Precipitation results resemble those of soil moisture, which similarly exerted a larger effect on seasonal (96% in average) than in evergreen forests (84%). Tropical seasonal forests, also known as dry forests, are featured by the existence of a season where rainfall is very scarce or even inexistent. Therefore, it seems logical that precipitation determines more than any other factor the floristic composition of such systems, since during the dry season water availability becomes a limiting factor (Murphy and Lugo, 1986; Pennington et al., 2009). In the case of herbs, precipitation seemed to play a role of utmost importance, being influential in 100% of the records. Although those values—far from the percentages for the rest of life forms—could be biased given the small number of records investigating this variable for herbs (n = 5), a great reliance of herbs on precipitation should not be unexpected given that their root system is more limited compared with other life forms and obtains water mainly from the upper soil, with no access to deep layers were water is stored during larger periods (Fan et al., 2017). In the case of temperature, it plays a larger effect on montane (86% in average) than in lowland forests (71%), a non-surprising fact given that temperature experiences large shifts along altitudinal gradients, filtering species ability to thrive on mountain slopes (Körner, 2007).
Conclusion and Future Directions
Our results highlight the major influence of soil and climate on floristic composition, with some particularities across spatial scales and for certain life forms and forest types. Nevertheless, results show a great variability given the great heterogeneity of variables investigated, and data collection and analyses methods used across studies. In fact, the heterogeneity in methods causes some limitations, especially when large scale studies using primary data from different sources are to be conducted or results from different studies are to be compared. In this sense, although many of these pitfalls could be overcome by using standardized field an laboratory protocols –such as the RAINFOR initiative proposal for 1-ha plots (Phillips et al., 2018), a modified Gentry’s system for 0.1-ha plots (Arellano et al., 2016a), or the soil protocols from the Embrapa (Teixeira et al., 2017)– this is far easier to be said than to be actually done. Each research’s particular ecological questions addressed, or budget limitations ultimately condition the sampling methods chosen. In any case, detailed information about the methods used should always be included in the publications, which is not always the case.
Finally, understanding soil and climate drivers of floristic composition is fundamental to support conservation policies aiming to protect species and their ecosystem services. In this sense, developing more accurate soil and climate maps will not only help us to envision toward which floristic composition patterns could global change reshape tropical forests (Colwell et al., 2008; Feeley et al., 2020), but also to map how environmental gradients cause variations in plant functional strategies and forest functioning across space (Asner et al., 2017) and time (NGEE-Tropics Project, 2022).
Data Availability Statement
The original contributions presented in the study are included in the article/Supplementary Material; further inquiries can be directed to the corresponding author.
Author Contributions
MM, GA, and LC conceived the study. GC and GB-d-D conducted the literature research and analyzed research items retrieved. GB-d-D and LC analyzed the results. GB-d-D wrote the manuscript with the substantial help of MM, GA, and LC. All authors contributed to the article and approved the submitted version.
Funding
GB-d-D was funded through a Postdoc grant by the Education and Research Department of Madrid Autonomous Region Government (REMEDINAL TE; S2018/EMT-4338). GC was funded through a Ph.D. abroad grant provided by the Brazilian Ministry of Science, (MCTIC) through the National Council of Scientific and Technological Development (CNPq). This study was supported through three grants from the Spanish Ministry of Economy (MINECO; CGL2013-45634-P, CGL2016-75414-P, and PID2019-105064GB-I00).
Conflict of Interest
GA is employed by Oikobit LLC.
The remaining authors declare that the research was conducted in the absence of any commercial or financial relationships that could be construed as a potential conflict of interest.
Publisher’s Note
All claims expressed in this article are solely those of the authors and do not necessarily represent those of their affiliated organizations, or those of the publisher, the editors and the reviewers. Any product that may be evaluated in this article, or claim that may be made by its manufacturer, is not guaranteed or endorsed by the publisher.
Acknowledgments
We are thankful to Marcos Méndez and the three reviewers for their commentaries.
Supplementary Material
The Supplementary Material for this article can be found online at: https://www.frontiersin.org/articles/10.3389/fevo.2022.866905/full#supplementary-material
References
Abd Manaf, Z., Mohd Zain, M. F., Cheng, W.-W., Huggett, S., Rohatgi, S., Reggio, D., et al. (2019). Malaysia: Knowledge Destination for Research Excellence. Amsterdam: Elsevier.
Arellano, G., Tello, J. S., Jørgensen, P. M., Fuentes, A. F., Loza, M. I., Torrez, V., et al. (2016b). Disentangling environmental and spatial processes of community assembly in tropical forests from local to regional scales. Oikos 125, 326–335. doi: 10.1111/oik.02426
Arellano, G., Cala, V., Fuentes, A., Cayola, L., Jorgensen, P. M., and Macía, M. J. (2016a). A standard protocol for woody plant inventories and soil characterisation using temporary 0.1-Ha plots in tropical forests. J. Trop. For. Sci. 28, 508–516.
Asner, G. P., Martin, R. E., Knapp, D. E., Tupayachi, R., Anderson, C. B., Sinca, F., et al. (2017). Airborne laser-guided imaging spectroscopy to map forest trait diversity and guide conservation. Science 355, 385–389. doi: 10.1126/science.aaj1987
Baker, T. R., Vicuña Miñano, E., Banda-R, K., del Castillo Torres, D., Farfan-Rios, W., Lawson, I. T., et al. (2021). From plots to policy: how to ensure long-term forest plot data supports environmental management in intact tropical forest landscapes. Plants People Planet 3, 229–237. doi: 10.1002/PPP3.10154
Baldeck, C. A., Tupayachi, R., Sinca, F., Jaramillo, N., and Asner, G. P. (2016). Environmental drivers of tree community turnover in western Amazonian forests. Ecography (Cop.) 39, 1089–1099. doi: 10.1111/ecog.01575
Baraloto, C., Molto, Q., Rabaud, S., Hérault, B., Valencia, R., Blanc, L., et al. (2013). Rapid simultaneous estimation of aboveground biomass and tree diversity across neotropical forests: a comparison of field inventory methods. Biotropica 45, 288–298. doi: 10.1111/btp.12006
Bawa, S. K., Kress, W. J., Nadkarni, M. N., and Lele, S. (2004). Beyond Paradise – meeting the challenges in tropical biology in the 21st Century. Biotropica 36, 437–446. doi: 10.1007/978-3-319-67208-3_5
Bell, G., Lechowicz, M. J., Appenzeller, A., Chandler, M., DeBlois, E., Jackson, L., et al. (1993). The spatial structure of the physical environment. Oecologia 96, 114–121. doi: 10.1007/BF00318038
Bohlman, A. S., Laurance, F. W., Laurance, G. N. S., Ascimento, E. M. H., Fearnside, M. P., and Andrade, A. (2008). Importance of soils, topography and geographic distance in structuring central Amazonian tree communities. J. Veg. Sci. 19, 863–874. doi: 10.3170/2008-8-18463
Borcard, D., Legendre, P., and Drapeau, P. (1992). Partialling out the spatial component of ecological variation. Ecology 73, 1045–1055. doi: 10.2307/1940179
Borges-Prata, E. M., Teixeira, A., de, P., Joly, C. A., and Assis, M. A. (2018). The role of climate on floristic composition in a latitudinal gradient in the Brazilian atlantic forest. Plant Ecol. Evol. 151, 303–313. doi: 10.5091/plecevo.2018.1407
Bruijnzeel, L. A., Scatena, F. N., and Hamilton, L. S. (2011). Tropical Montane Cloud Forest. Cambridge: Cambridge University Press.
Brunner, I., and Sperisen, C. (2013). Aluminum exclusion and aluminum tolerance in woody plants. Front. Plant Sci. 4:172. doi: 10.3389/fpls.2013.00172
Bubb, P., May, I., Miles, L., and Sayer, J. (2004). Cloud Forest Agenda. Cambridge: United Nations Environment Programme- World Conservation Monitoring Centre.
Cadotte, M. W., and Tucker, C. M. (2017). Should environmental filtering be abandoned? Trends Ecol. Evol. 32, 429–437. doi: 10.1016/j.tree.2017.03.004
Cayuela, L., Granzow-de la Cerda, Í., and Méndez, M. (2018). The state of European research in tropical biology. Biotropica 50, 202–207. doi: 10.1111/btp.12514
Chau, J. F., Bagtzoglou, A. C., and Willig, M. R. (2011). The effect of soil texture on richness and diversity of bacterial communities. Environ. Forensics 12, 333–341. doi: 10.1080/15275922.2011.622348
Chave, J. (2008). “Spatial variation in tree species composition across tropical forests: pattern and process,” in Tropical Forests Community Ecology, eds W. Carson and S. A. Schnitzer (Oxford: Wiley-Blackwell), 11–30.
Chust, G., Chave, J., Condit, R., Aguilar, S., Lao, S., and Pérez, R. (2006). Determinants and spatial modeling of tree β−diversity in a tropical forest landscape in Panama. J. Veg. Sci. 17, 83–92. doi: 10.1111/j.1654-1103.2006.tb02426.x
Clark, D. B. (1985). Ecological field studies in the tropics: geographical origin of reports. Bull. Ecol. Soc. Am. 66, 6–9.
Colwell, R. K., Brehm, G., Cardelús, C. L., Gilman, A. C., and Longino, J. T. (2008). Global warming, elevational range shifts, and lowland biotic attrition in the wet tropics. Science 322, 258–261. doi: 10.1126/science.1162547
Comita, L. S., and Engelbrecht, B. M. J. (2009). Seasonal and spatial variation in water availability drive habitat associations in a tropical forest. Ecology 90, 2755–2765. doi: 10.1890/08-1482.1
Condit, R., Engelbrecht, B. M. J., Pino, D., Pérez, R., and Turnera, B. L. (2013). Species distributions in response to individual soil nutrients and seasonal drought across a community of tropical trees. Proc. Natl. Acad. Sci. U.S.A. 110, 5064–5068. doi: 10.1073/pnas.1218042110
Costa, F. R. C., Magnusson, W. E., and Luizao, R. C. (2005). Mesoscale distribution patterns of Amazonian understorey herbs in relation to topography, soil and watersheds. J. Ecol. 93, 863–878. doi: 10.1111/j.1365-2745.2005.01020.x
Dangles, O., Loirat, J., Freour, C., Serre, S., Vacher, J., and Le Roux, X. (2016). Research on biodiversity and climate change at a Distance: collaboration networks between Europe and Latin America and the Caribbean. PLoS One 11:e0157441. doi: 10.1371/journal.pone.0157441
Dodd, M. B., and Lauenroth, W. K. (1997). The influence of soil texture on the soil water dynamics and vegetation structure of a shortgrass steppe ecosystem. Plant Ecol. 133, 13–28. doi: 10.1023/A:1009759421640
DRYFLOR, Banda-R, K., Delgado-Salinas, A., Dexter, K. G., Linares-Palomino, R., Oliveira-Filho, A., et al. (2016). Plant diversity patterns in neotropical dry forests and their conservation implications. Science 353, 1383–1387. doi: 10.1126/science.aaf5080
Engelbrecht, B. M. J., Comita, L. S., Condit, R., Kursar, T. A., Tyree, M. T., Turner, B. L., et al. (2007). Drought sensitivity shapes species distribution patterns in tropical forests. Nature 447, 80–82. doi: 10.1038/nature05747
Esquivel-Muelbert, A., Baker, T. R., Dexter, K. G., Lewis, S. L., ter Steege, H., Lopez-Gonzalez, G., et al. (2017). Seasonal drought limits tree species across the Neotropics. Ecography (Cop.) 40, 618–629. doi: 10.1111/ecog.01904
Fan, Y., Miguez-Macho, G., Jobbágy, E. G., Jackson, R. B., and Otero-Casal, C. (2017). Hydrologic regulation of plant rooting depth. Proc. Natl. Acad. Sci. U.S.A. 114, 10572–10577. doi: 10.1073/pnas.1712381114
Feeley, K. J., and Stroud, J. T. (2018). Where on Earth are the “tropics”? Front. Biogeogr. 10:e38649. doi: 10.21425/f5fbg38649
Feeley, K., Martinez-Villa, J., Perez, T., Silva Duque, A., Triviño Gonzalez, D., and Duque, A. (2020). The thermal tolerances, distributions, and performances of tropical montane tree species. Front. For. Glob. Chang. 3:25. doi: 10.3389/ffgc.2020.00025
Finzi, A. C., Moore, D. J. P., DeLucia, E. H., Lichter, J., Hofmockel, K. S., Jackson, R. B., et al. (2006). Progressive nitrogen limitation of ecosystem processes under elevated CO2 in a warm-temperate forest. Ecology 87, 15–25. doi: 10.1890/04-1748
ForestPlots.net (2021). Taking the pulse of Earth‘s tropical forests using networks of highly distributed plots. Biol. Conserv. 260, 108849. doi: 10.1016/j.biocon.2020.108849
Garibaldi, C., Nieto-Ariza, B., Macía, M. J., and Cayuela, L. (2014). Soil and geographic distance as determinants of floristic composition in the Azuero Peninsula (Panama). Biotropica 46, 687–695. doi: 10.1111/btp.12174
Garzon-Lopez, C. X., Jansen, P. A., Bohlman, S. A., Ordoñez, A., and Olff, H. (2014). Effects of sampling scale on patterns of habitat association in tropical trees. J. Veg. Sci. 25, 349–362. doi: 10.1111/jvs.12090
Gentry, A. H. (1988). Changes in plant community diversity and floristic composition on environmental and geographical gradients. Ann. Missouri Bot. Gard. 75, 1–34. doi: 10.2307/2399464
Golicher, D. J., Cayuela, L., Alkemade, J. R. M., González-Espinosa, M., and Ramírez-Marcial, N. (2008). Applying climatically associated species pools to the modelling of compositional change in tropical montane forests. Glob. Ecol. Biogeogr. 17, 262–273. doi: 10.1111/j.1466-8238.2007.00362.x
Guevara Andino, J. E., Pitman, N. C. A., ter Steege, H., Peralvo, M., Cerón, C., and Fine, P. V. A. (2021). The contribution of environmental and dispersal filters on phylogenetic and taxonomic beta diversity patterns in Amazonian tree communities. Oecologia 196, 1119–1137. doi: 10.1007/s00442-021-04981-0
Guèze, M., Paneque-Gálvez, J., Luz, A. C., Pino, J., Orta-Martínez, M., Reyes-García, V., et al. (2013). Determinants of tree species turnover in a southern Amazonian rain forest. J. Veg. Sci. 24, 284–295. doi: 10.1111/j.1654-1103.2012.01461.x
Hawkins, B., Field, R., Cornell, H., Currie, D., Guégan, J., Kaufman, D., et al. (2003). Energy, water, and broad-scale geographic patterns of species richness. Ecology 84, 3105–3117. doi: 10.1890/03-8006
Higgins, M. A., Ruokolainen, K., Tuomisto, H., Llerena, N., Cardenas, G., Phillips, O. L., et al. (2011). Geological control of floristic composition in Amazonian forests. J. Biogeogr. 38, 2136–2149. doi: 10.1111/j.1365-2699.2011.02585.x
Holmgren, M., and Schnitzer, S. A. (2004). Science on the rise in developing countries. PLoS Biol. 2:e1. doi: 10.1371/journal.pbio.0020001
Houlton, B. Z., Sigman, D. M., Schuur, E. A. G., and Hedin, L. O. (2007). A climate-driven switch in plant nitrogen acquisition within tropical forest communities. Proc. Natl. Acad. Sci. U.S.A. 104, 8902–8906. doi: 10.1073/pnas.0609935104
Humboldt, A. V., and Bonpland, A. (1805). Essai sur la Geographie des Plantes. Paris: Chez Levrault Schoell.
Isichei, A. O., Morton, A. J., and Ekeleme, F. (1990). Mineral nutrient flow from an inselberg in south-western Nigeria. J. Trop. Ecol. 6, 479–492. doi: 10.1017/s0266467400004892
Jansen, S., Broadley, M. R., Robbrecht, E., and Smets, E. (2002). Aluminum hyperaccumulation in Angiosperms: a review of its phylogenetic significance. Bot. Rev. 68, 235–269. doi: 10.1663/0006-8101(2002)068[0235:ahiaar]2.0.co;2
Jarvis, P., and Linder, S. (2000). Constraints to growth of boreal forests. Nature 405, 904–905. doi: 10.1038/35016154
Johnston, M. H. (1992). Soil vegetation relationships in Tabunoco forest reserve Puerto Rico. J. Trop. Ecol. 8, 253–263. doi: 10.3389/ffgc.2021.676691
Khairil, M., Wan Juliana, W. A., and Nizam, M. S. (2014). Edaphic influences on tree species composition and community structure in a tropical watershed forest in peninsular Malaysia. J. Trop. For. Sci. 26, 284–294.
Kidd, P. S., and Proctor, J. (2001). Why plants grow poorly on very acid soils: are ecologists missing the obvious? J. Exp. Bot. 52, 791–799. doi: 10.1093/jexbot/52.357.791
Körner, C. (2007). The use of ‘altitude’ in ecological research. Trends Ecol. Evol. 22, 569–574. doi: 10.1016/j.tree.2007.09.006
Lechowicz, M. J., and Bell, G. (1991). The ecology and genetics of fitness in forest plants. II. Microspatial heterogeneity of the edaphic environment. J. Ecol. 79, 687–696. doi: 10.2307/2260661
Ledo, A., Burslem, D. F. R. P., Condés, S., and Montes, F. (2013). Micro-scale habitat associations of woody plants in a neotropical cloud forest. J. Veg. Sci. 24, 1086–1097. doi: 10.1111/jvs.12023
Libalah, M. B., Droissart, V., Sonké, B., Barbier, N., Dauby, G., Fortunel, C., et al. (2020). Additive influences of soil and climate gradients drive tree community composition of Central African rainforests. J. Veg. Sci. 31, 1154–1167. doi: 10.1111/jvs.12918
López-Martínez, J. O., Sanaphre-Villanueva, L., Dupuy, J. M., Hernández-Stefanoni, J. L., Meave, J. A., and Gallardo-Cruz, J. A. (2013). β-Diversity of functional groups of woody plants in a tropical dry forest in Yucatan. PLoS One 8:e73660. doi: 10.1371/journal.pone.0073660
Lu, X., Mao, Q., Gilliam, F. S., Luo, Y., and Mo, J. (2014). Nitrogen deposition contributes to soil acidification in tropical ecosystems. Glob. Chang. Biol. 20, 3790–3801. doi: 10.1111/gcb.12665
Macía, M. J., Ruokolainen, K., Tuomisto, H., Quisbert, J., and Cala, V. (2007). Congruence between floristic patterns of trees and lianas in a southwest Amazonian rain forest. Ecography (Cop.) 30, 561–577. doi: 10.1111/j.2007.0906-7590.05124.x
Mage, S. M., and Porder, S. (2013). Parent material and topography determine soil phosphorus status in the Luquillo Mountains of Puerto Rico. Ecosystems 16, 284–294. doi: 10.1007/s10021-012-9612-5
Magnusson, W. E., Lima, A. P., Luizão, R., Costa, F. R., Volkmer de Castilho, C., and Kinupp, V. (2005). RAPELD: a modification of the Gentry method for biodiversity surveys in long-term ecological research sites. Biota Neotrop. 5, 19–24. doi: 10.1590/s1676-06032005000300002
Malhado, A. C. M., de Azevedo, R. S. D., Todd, P. A., Santos, A. M. C., Fabré, N. N., Batista, V. S., et al. (2014). Geographic and temporal trends in Amazonian knowledge production. Biotropica 46, 6–13. doi: 10.1111/btp.12079
Malhi, Y., Silman, M., Salinas, N., Bush, M., Meir, P., and Saatchi, S. (2010). Introduction: elevation gradients in the tropics: laboratories for ecosystem ecology and global change research. Glob. Chang. Biol. 16, 3171–3175. doi: 10.1111/j.1365-2486.2010.02323.x
Morueta-Holme, N., and Svenning, J.-C. (2018). Geography of plants in the new world: Humboldt’s relevance in the age of big data. Ann. Missouri Bot. Gard. 103, 315–329. doi: 10.3417/2018110
Murphy, P. G., and Lugo, A. E. (1986). Ecology of tropical dry forest. Annu. Rev. Ecol. Syst. 17, 67–88. doi: 10.1146/annurev.es.17.110186.000435
Myers, N., Mittermeier, R. A., Mittermeier, C. G., Fonseca, G. A. B., and Kent, J. (2000). Biodiversity hotspots for conservation priorities. Nature 403, 853–858. doi: 10.1038/35002501
Neves, D. M., Dexter, K. G., Pennington, R. T., Bueno, M. L., and Oliveira Filho, A. T. (2015). Environmental and historical controls of floristic composition across the South American Dry Diagonal. J. Biogeogr. 42, 1566–1576. doi: 10.1111/jbi.12529
NGEE-Tropics Project (2022). NGEE-Tropics Next Generation Ecosystem Experiments. Available online at: https://ngee-tropics.lbl.gov/ (accessed February 16, 2022).
Normand, S., Vormisto, J., Svenning, J. C., Grández, C., and Balslev, H. (2006). Geographical and environmental controls of palm beta diversity in paleo-riverine terrace forests in Amazonian Peru. Plant Ecol. 186, 161–176. doi: 10.1007/s11258-006-9120-9
Palmer, M. (2007). “Species-area curves and geometry of nature,” in Scaling Biodiversity, eds M. Storch, P. A. Marquet, and J. Brown (Cambridge: Cambridge University Press), 15–31. doi: 10.1111/j.1461-0248.2010.01449.x
Pasion, B. O., Roeder, M., Liu, J., Yasuda, M., Corlett, R. T., Slik, J. W. F., et al. (2018). Trees represent community composition of other plant life-forms, but not their diversity, abundance or responses to fragmentation. Sci. Rep. 8:11374. doi: 10.1038/s41598-018-29635-9
Pennington, R. T., Lavin, M., and Oliveira-Filho, A. (2009). Woody plant diversity, evolution, and ecology in the tropics: perspectives from seasonally dry tropical forests. Annu. Rev. Ecol. Evol. Syst. 40, 437–457. doi: 10.1146/annurev.ecolsys.110308.120327
Pett-Ridge, J. C., Derry, L. A., and Kurtz, A. C. (2009). Sr isotopes as a tracer of weathering processes and dust inputs in a tropical granitoid watershed, Luquillo Mountains, Puerto Rico. Geochim. Cosmochim. Acta 73, 25–43. doi: 10.1016/j.gca.2008.09.032
Phillips, O. L., Baker, T. R., Feldpausch, T. R., and Brienen, R. (2018). RAINFOR: Field manual for Plot Establishment and Remeasurement. Available online at: http://rainfor.org/upload/ManualsEnglish/RAINFOR_field_manual_version_2016.pdf (accessed April 2, 2022).
Phillips, O. L., Núñez Vargas, P., Monteagudo, A. L., Cruz, A. P., Zans, M. E. C., Sánchez, W. G., et al. (2003a). Habitat association among Amazonian tree species: a landscape-scale approach. J. Ecol. 91, 757–775. doi: 10.1046/j.1365-2745.2003.00815.x
Phillips, O. L., Vásquez Martínez, R., Núñez Vargas, P., Lorenzo Monteagudo, A., Chuspe Zans, M. E., Galiano Sánchez, W., et al. (2003b). Efficient plot-based floristic assessment of tropical forests. J. Trop. Ecol. 19, 629–645. doi: 10.1017/S0266467403006035
Porder, S., Asner, G. P., and Vitousek, P. M. (2005). Ground-based and remotely sensed nutrient availability across a tropical landscape. Proc. Natl. Acad. Sci. U.S.A. 102, 10909–10912. doi: 10.1073/pnas.0504929102
Porder, S., Vitousek, P. M., Chadwick, O. A., Chamberlain, C. P., and Hilley, G. E. (2007). Uplift, erosion, and phosphorus limitation in terrestrial ecosystems. Ecosystems 10, 158–170. doi: 10.1007/s10021-006-9011-x
Rahbek, C., Borregaard, M. K., Colwell, R. K., Dalsgaard, B., Holt, B. G., Morueta-Holme, N., et al. (2019). Humboldt’s enigma: what causes global patterns of mountain biodiversity? Science 365, 1108–1113. doi: 10.1126/science.aax0149
Ross, S. M. (1993). Organic matter in tropical soils: current conditions, concerns and prospects for conservation. Prog. Phys. Geogr. 17, 265–305. doi: 10.1177/030913339301700301
Ruokolainen, K., Tuomisto, H., Macía, M. J., Higgins, M. A., and Yli-Halla, M. (2007). Are floristic and edaphic patterns in Amazonian rain forests congruent for trees, pteridophytes and Melastomataceae? J. Trop. Ecol. 23, 13–25. doi: 10.1017/S0266467406003889
Ryel, R., Caldwell, M., and Manwaring, J. (1996). Temporal dynamics of soil spatial heterogeneity in sagebrush-wheatgrass steppe during a growing season. Plant Soil 184, 299–309. doi: 10.1007/bf00010459
Saiter, F. Z., Eisenlohr, P. V., Barbosa, M. R. V., Thomas, W. W., and Oliveira-Filho, A. T. (2016). From evergreen to deciduous tropical forests: how energy–water balance, temperature, and space influence the tree species composition in a high diversity region. Plant Ecol. Divers. 9, 45–54. doi: 10.1080/17550874.2015.1075623
Sánchez-Azofeifa, G. A., Quesada, M., Rodríguez, J. P., Nassar, J. M., Stoner, K. E., Castillo, A., et al. (2005). Research priorities for neotropical dry forests. Biotropica 37, 477–485. doi: 10.1111/j.1744-7429.2005.00066.x
Schmitt, M., Mehltreter, K., Sundue, M., Testo, W., Watanabe, T., and Jansen, S. (2017). The evolution of aluminum accumulation in ferns and lycophytes. Am. J. Bot. 104, 573–583. doi: 10.3732/ajb.1600381
Siefert, A., Ravenscroft, C., Althoff, D., Alvarez-Yépiz, J. C., Carter, B. E., Glennon, K. L., et al. (2012). Scale dependence of vegetation-environment relationships: a meta-analysis of multivariate data. J. Veg. Sci. 23, 942–951. doi: 10.1111/j.1654-1103.2012.01401.x
Slik, J. F., Arroyo-Rodríguez, V., Aiba, S.-I., Alvarez-Loayza, P., Alves, L. F., Ashton, P., et al. (2015). An estimate of the number of tropical tree species. Proc. Natl. Acad. Sci. U.S.A. 112, E4628–E4629. doi: 10.1073/pnas.1512611112
Slik, J. W. F., Raes, N., Aiba, S. I., Brearley, F. Q., Cannon, C. H., Meijaard, E., et al. (2009). Environmental correlates for tropical tree diversity and distribution patterns in Borneo. Divers. Distrib. 15, 523–532. doi: 10.1111/j.1472-4642.2009.00557.x
Soininen, J. (2014). A quantitative analysis of species sorting across organisms and ecosystems. Ecology 95, 3284–3292. doi: 10.1371/journal.pone.0062394
Sollins, P. (1998). Factors influencing species composition in tropical lowland rain forest: does soil matter? Ecology 79, 23–30. doi: 10.1890/0012-96581998079[0023:FISCIT]2.0.CO;2
Stocks, G., Seales, L., Paniagua, F., Maehr, E., and Bruna, E. M. (2008). The geographical and institutional distribution of ecological research in the tropics. Biotropica 40, 397–404. doi: 10.1111/j.1744-7429.2007.00393.x
Sutherland, W. J., Freckleton, R. P., Godfray, H. C. J., Beissinger, S. R., Benton, T., Cameron, D. D., et al. (2013). Identification of 100 fundamental ecological questions. J. Ecol. 101, 58–67. doi: 10.1111/1365-2745.12025
Teixeira, P., Donagemma, G., Fontana, A., and Teixeira, W. (2017). Manual de Métodos de Análise de Solo. Brasilia: Embrapa.
Ter Steege, H., Pitman, N. C. A., Phillips, O. L., Chave, J., Sabatier, D., Duque, A., et al. (2006). Continental-scale patterns of canopy tree composition and function across Amazonia. Nature 443, 444–447. doi: 10.1038/nature05134
Townsend, A. R., Asner, G. P., and Cleveland, C. C. (2008). The biogeochemical heterogeneity of tropical forests. Trends Ecol. Evol. 23, 424–431. doi: 10.1016/j.tree.2008.04.009
Trujillo, L. N., Granzow-de la Cerda, Í, Pardo, I., Macía, M. J., Cala, V., and Arellano, G. (2019). Niche-based processes outperform neutral processes when predicting distance decay in co-dominance along the Amazon – Andes rainforest gradient. J. Veg. Sci. 30, 644–653. doi: 10.1111/jvs.12761
Tuomisto, H., Ruokolainen, K., and Yli-Halla, M. (2003b). Dispersal, environment, and floristic variation of Western Amazonian forests. Science 299, 241–244. doi: 10.1126/science.1078037
Tuomisto, H., Poulsen, A. D., Ruokolainen, K., Moran, R. C., Quintana, C., Celi, J., et al. (2003a). Linking floristic patterns with soil heterogeneity and satellite imagery in Ecuadorian Amazonia. Ecol. Appl. 13, 352–371. doi: 10.1890/1051-0761(2003)013[0352:lfpwsh]2.0.co;2
Turner, B. L. (2008). Resource partitioning for soil phosphorus: a hypothesis. J. Ecol. 96, 698–702. doi: 10.1111/J.1365-2745.2008.01384.X
Turner, B. L., Brenes-Arguedas, T., and Condit, R. (2018). Pervasive phosphorus limitation of tree species but not communities in tropical forests. Nature 555, 367–370. doi: 10.1038/nature25789
Valencia, R., Foster, R. B., Villa, G., Condit, R., Svenning, J. C., Hernández, C., et al. (2004). Tree species distributions and local habitat variation in the Amazon: large forest plot in eastern Ecuador. J. Ecol. 92, 214–229. doi: 10.1111/j.0022-0477.2004.00876.x
Vitousek, P. M. (1984). Litterfall, nutrient cycling, and nutrient limitation in tropical Forests. Ecology 65, 285–298. doi: 10.2307/1939481
Walker, T. W., and Syers, J. K. (1976). The fate of phosphorus during pedogenesis. Geoderma 15, 1–19. doi: 10.1016/0016-7061(76)90066-5
Walters, D., Aulakh, M., and Doran, J. (1992). Effects of soil aeration, legume residue, and soil texture on transformations of macro- and micro nutrients in soils. Soil Sci. 153, 100–107. doi: 10.1097/00010694-199202000-00003
Watkins, G., and Donnelly, M. (2005). Biodiversity research in the Neotropics: from conflict to collaboration. Proc. Acad. Nat. Sci. Phila. 154, 127–136. doi: 10.1635/0097-3157(2004)154[0127:britnf]2.0.co;2
Weintraub, S. R., Taylor, P. G., Porder, S., Cleveland, C. C., Asner, G. P., and Townsend, A. R. (2015). Topographic controls on soil nitrogen availability in a lowland tropical forest. Ecology 96, 1561–1574. doi: 10.1890/14-0834.1
White, D. A., and Hood, C. S. (2004). Vegetation patterns and environmental gradients in tropical dry forests of the northern Yucatan Peninsula. J. Veg. Sci. 15, 151–160. doi: 10.1111/j.1654-1103.2004.tb02250.x
Whitesell, S., Lilieholm, R., and Sharik, T. (2002). A global survey of tropical biological field stations. Bioscience 52, 55–64. doi: 10.1002/rra.3476
Whittaker, R. H. (1960). Vegetation of the Siskiyou Mountains, Oregon and California. Ecol. Monogr. 30, 279–338. doi: 10.2307/1943563
Willig, M. R., Kaufman, D. M., and Stevens, R. D. (2003). Latitudinal gradients of biodiversity: pattern, process, scale, and synthesis. Annu. Rev. Ecol. Evol. Syst. 34, 273–309. doi: 10.1146/annurev.ecolsys.34.012103.144032
Woodward, F. I., Lomas, M. R., and Kelly, C. K. (2004). Global climate and the distribution of plant biomes. Philos. Trans. R. Soc. B Biol. Sci. 359, 1465–1476. doi: 10.1098/rstb.2004.1525
Yu, H., Chin, M., Yuan, T., Bian, H., Remer, L. A., Prospero, J. M., et al. (2015). The fertilizing role of African dust in the Amazon rainforest: a first multiyear assessment based on data from Cloud-Aerosol Lidar and Infrared Pathfinder Satellite Observations. Geophys. Res. Lett. 42, 1984–1991. doi: 10.1002/2015GL063040
Keywords: life forms, forest types, spatial scales, soil moisture, phosphorus, aluminum, temperature, precipitation
Citation: Bañares-de-Dios G, Macía MJ, de Carvalho GM, Arellano G and Cayuela L (2022) Soil and Climate Drive Floristic Composition in Tropical Forests: A Literature Review. Front. Ecol. Evol. 10:866905. doi: 10.3389/fevo.2022.866905
Received: 31 January 2022; Accepted: 30 March 2022;
Published: 27 April 2022.
Edited by:
Daniel de Paiva Silva, Goiano Federal Institute (IFGOIANO), BrazilReviewed by:
Maxime Réjou-Méchain, UMR 5120 Botanique et Modélisation de l’Architecture des Plantes et des Végétations (AMAP), FranceJoseph Yavitt, Cornell University, United States
Marcelo Leandro Bueno, Universidade Estadual de Mato Grosso do Sul, Brazil
Copyright © 2022 Bañares-de-Dios, Macía, de Carvalho, Arellano and Cayuela. This is an open-access article distributed under the terms of the Creative Commons Attribution License (CC BY). The use, distribution or reproduction in other forums is permitted, provided the original author(s) and the copyright owner(s) are credited and that the original publication in this journal is cited, in accordance with accepted academic practice. No use, distribution or reproduction is permitted which does not comply with these terms.
*Correspondence: Guillermo Bañares-de-Dios, Z2JhbmFyZXNkZEBnbWFpbC5jb20=