- 1UMR 5204 EDYTEM Environnement Dynamique et Territoire de la Montagne, CNRS/Université Savoie Mont Blanc, Le Bourget-du-Lac, France
- 2Paleobotalab, Le Bourget-du-Lac, France
- 3UMR 042 CARRTEL Centre Alpin de Recherche sur les Réseaux Trophiques et Ecosystèmes Limniques, Université de Savoie Mont Blanc/INRAE, Le Bourget-du-Lac, France
- 4UMR LECA Université Grenoble Alpes, CNRS, Université Savoie Mont Blanc, Grenoble, France
- 5UMR ISTerre Institut des Sciences de la Terre, Université Savoie Mont Blanc/Université Grenoble Alpes/CNRS/IRD/Université Gustave Eiffel, Chambéry, France
- 6UMR 6249 Laboratoire Chrono-Environnement, Université Franche Comté, Besançon, France
Over the last two millennia, European Alpine ecosystems have experienced major changes in response to the important, yet fluctuating, impact of human activities. This study aims to reconstruct the environmental history of the last 1800 years on the western edge of the Alps by analyzing sediments from Lake Aiguebelette, a large lake located in the perialpine area. We have combined analyses of pollen and other palynomorphs, such as coprophilous fungal spores, together with sedimentary DNA (from plants and mammals) in order to reconstruct both vegetation and land-use histories. A sedimentological and geochemical analysis was also conducted in order to gain an understanding of changes in erosion dynamics in response to landscape modifications that were influenced by climate and human activities. This work highlights alternating phases of anthropization and agricultural abandonment allowing forest recovery. While pollen reflects the major phases of regional deforestation and afforestation related to the dynamic of farming activities, plant DNA provides precise information on the plants cultivated in fields, orchards and vegetable gardens over the past centuries. The combination of mammal DNA and coprophilous fungal spores completes this work by documenting the history of pastoral practices.
Introduction
Current landscapes result from millennial-long evolutions of environments under the joint influence of human activities, climate changes, and ecosystem responses (Kaplan et al., 2009; Ellis et al., 2013; Mottl et al., 2021). In the European Alps (Mottl et al., 2021), this evolution of ecosystems during the Holocene has greatly expanded and accelerated in the last few millennia, as shown by numerous paleoecological and paleoenvironmental records (Tinner et al., 1996; Schmidt et al., 2002; Giguet-Covex et al., 2011, 2014; Doyen et al., 2013, 2016; Walsh et al., 2014; Bajard et al., 2016, 2017; Andrič et al., 2020; Dietre et al., 2020; etc.). These records highlight the growing impact of agricultural intensification both at low altitude (Doyen et al., 2013, 2016; Simonneau et al., 2013; Rapuc et al., 2021) and in Alpine pastures (Giguet-Covex et al., 2014; Rey et al., 2017) in a period when populations were growing and agricultural techniques were being improved. The last two millennia were also marked by major shifts in climatic conditions, such as the Medieval Climate Anomaly (MCA) and the Little Ice Age (LIA) (Büntgen et al., 2011; Le Roy et al., 2015). At the same time, important socio-economic/cultural shifts occurred, among which the fall of the Roman Empire, the agricultural and economic revolution of the Middle Ages (e.g., invention of the plow) or the current agricultural decline (Mazoyer and Roudart, 1997; Ferault and Le Chatelier, 2012) and introduction of “exotic plants” are likely to have a significant impact on landscape evolutions. How have human impacts and climatic variations modified and driven the trajectories of ecosystems over the last two millennia?
Although the identification of cultivated plants is a direct indicator of past agricultural strategies, the whole vegetation history can also be a reliable indicator of such strategies and associated agrarian practices (e.g., expansion of ruderal, nitrophilous plants, weeds, changes in forest composition due to clearance, etc.) (Behre, 1981; Brun, 2007). Moreover, analysis of non-pollen palynomorphs (spores of coprophilous fungi such as Sporormiella sp.) provides indirect and robust information about past pastoral pressure (Ejarque et al., 2011; Etienne et al., 2013; Doyen and Etienne, 2017). Therefore, palynological analyses are traditionally used as a fundamental tool to reconstruct agro-pastoral activities and paleoenvironmental dynamics. For the Alps, pollen analyses are commonly used to reconstruct the vegetation and land-use history (de Beaulieu et al., 1992; Tinner et al., 1996, 2003; Haas et al., 1998; Guiter et al., 2005; Finsinger and Tinner, 2007; David, 2010; Schwörer et al., 2015; Thöle et al., 2016; Pini et al., 2017).
In perialpine areas (the regions located immediately around the Alpine range), past vegetation cover and land-use have already been documented through pollen from lake sediments (Clerc, 1988; Lotter, 1999; Tinner et al., 1999; Doyen et al., 2013, 2016; Rey et al., 2017; Andrič et al., 2020). In these regions, farming activities are more developed and diversified than in the inner Alpine areas which are not always suitable for growing cultivated plants. Indeed, previous studies have described the development of mixed agro-pastoral activities comprised of cereal growing, fruit tree growing and grazing, that have shaped the regional vegetation and driven soil erosion dynamics (Jacob et al., 2009; Doyen et al., 2013, 2016; Simonneau et al., 2013). Nonetheless, pollen analysis presents some limitations in reconstructing plant cover, especially in foothill areas (Muller et al., 2006). Pollen assemblages deposited in lake sediment include pollen grains from both local and regional sources, a fact which limits the reconstruction of local vegetation and land-use. The low taxonomic resolution of most of the herbaceous families and the low dispersal capacity of insect-pollinated plants, represent important limitations to the precise identification of past plant communities and their intrinsic diversity. Spores of coprophilous fungi are robust proxies of past livestock farming but they cannot indicate the composition of past domestic herds. Consequently, the reconstructions of paleoecological trajectories and of the nature of past agro-pastoral activities are open to improvement.
In order to enhance our knowledge of past human-influenced environmental trajectories, it is possible to combine palynological data with plant and mammal DNA analyses. Lake sediment DNA (sedaDNA) analyses from plants was recently made possible thanks to the emergence of the DNA metabarcoding approach (Parducci et al., 2017), which takes advantage of the development of high-throughput sequencing techniques (Taberlet et al., 2012). This emerging indicator of past biodiversity has been successfully applied in various ecosystems ranging from tropical to boreal environments (Boessenkool et al., 2014; Epp et al., 2015; Alsos et al., 2016; Bremond et al., 2017; Sjögren et al., 2017; Ficetola et al., 2018; Tabares et al., 2020), including the Alps (Pansu et al., 2015; Bajard et al., 2017; Giguet-Covex et al., 2019). Lake sedaDNA analyses have also been successfully applied in different contexts to identify mammals (Giguet-Covex et al., 2014; Etienne et al., 2015; Graham et al., 2016; Pedersen et al., 2016; Sabatier et al., 2017; Ficetola et al., 2018; Brown et al., 2021). The combination of these micro-fossil and DNA-based approaches offers a new and more detailed image of past vegetation and of agricultural and pastoral activities (Giguet-Covex et al., 2014) at the local scale. Indeed, because plant DNA in lake sediments comes from the watershed (or parts of the watershed; Parducci et al., 2017; Sjögren et al., 2017; Alsos et al., 2018; Giguet-Covex et al., 2019), it potentially represents a key indicator allowing us to decipher the different pollen origins (regional/local) and to better reconstruct the spatial distribution and modification of vegetation at the catchment scale. Pollen and sedaDNA have already been used in combination in several studies (Parducci et al., 2005, 2013; Giguet-Covex, 2010; Jørgensen et al., 2012; Pedersen et al., 2013; Niemeyer et al., 2017; Sjögren et al., 2017). These studies have revealed the benefits of applying both methods since the results of DNA analysis provide invaluable information about past vegetation and complement the results of pollen analysis.
Therefore, in order to investigate the question of the influence of climate variations and/or human activities over the last two millennia in the perialpine area, we propose to study the Lake Aiguebelette sediment archive sequence, by combining different approaches in a multiproxy analysis: (i) sedimentological and geochemical analyses to trace the evolution of soil erosion and (ii) palynological (pollen grains and spores of Sporormiella sp.) and lake sedaDNA analyses targeting plants and mammals with a view to documenting past changes in both vegetation communities and agricultural/livestock farming activities/practices. Taken together, these proxies will provide a full picture of agricultural activities, their effects on the landscape (vegetation cover and soil erosion) and, ultimately, will give us a better understanding of the history of local livelihood strategies and land use over the past 1800 years, a period that witnessed deep social-economical mutations and significant climate changes.
Study Site
Physical Setting
Lake Aiguebelette (374 m a.s.l., 5.5 km2) and its watershed (58.9 km2) are located in Savoie (France), in the northern perialpine area. The lake is bordered by the southern fringe of the Jura mountain range. It is of glacial origin and was formed after the retreat of the glacier at the end of the Würm period (Coutterand, 2010). Its catchment area ranges in altitude from 374 to 1441 m a.s.l. The geological substratum is mainly composed of Jurassic to lower Cretaceous limestone and marls, Miocene marine sandstone and Quaternary deposits composed of moraine and limestone screes in the southern part of the lake and along the limestone cliff (Eastern part) (Figure 1). The lake, which is the 7th largest natural lake in France, is composed of three main basins, respectively 30, 46, and 71 m of water depth. The main tributaries are the Gua, the Leysse de Novalaise and the Tuilerie rivers. The outlet of the lake, the Tiers, flows into the Guiers River, which itself is a tributary of the Rhône.
The lake water hosts a rich sub-aquatic macroflora including taxa such as Nymphea alba, Nuphar lutea, Najas sp., Potamogeton sp., and Myriophyllum spicatum. The lake shores are occupied by riparian forests dominated by alder (Alnus glutinosa), swamps and reed beds. The eastern side of the catchment (the slopes of the Epine range) is covered by forests dominated by fir (Abies alba) and beech (Fagus sylvatica). A thin belt of white oak (Quercus pubescens) and box tree (Buxus sempervirens) can be found on the well-exposed outcrops of the limestone massif. On this side, just a few small patches of pasture occur at the present time. The western part is mainly occupied by grasslands, cereal fields and pastures. While the farming activities are not as intense as in past centuries, this region is still an agricultural region. Forests composed of oak, hornbeam (Carpinus betulus), maple (Acer spp.) and hazel (Corylus avellana), are also well represented in this part of the watershed (Figure 1). Chestnut trees (Castanea sativa) are widespread in the forests growing on the molasses. This region was formerly known for its fruit production and while orchards were numerous in the last centuries, only a few are still in existence today (Figure 1).
Materials and Methods
Coring
Several sediment cores were retrieved from the deepest part of the lake (71 m depth, 45,5506N; 5,80128E). In 2008, a 170-cm long sediment core (AIG08-04) was collected using an UWITEC gravity corer with hammer. The upper part of this core, dated with short-lived radionuclides was then correlated with new cores. In 2016, AIG16-08 (N° IGSN TOAE0000000168) and AIG16-06 (N° IGSN TOAE0000000166), measuring 256.5 and 125 cm respectively, were sampled using an UWITEC gravity corer with hammer.
Sedimentological and Geochemical Analysis
In the laboratory, the core was split, photographed, and logged in detail, and all physical sedimentary structures were noted as was the vertical succession of facies. From these two cores and the correlation of their sedimentary structures we were able to create a master core (AIG16-MC) measuring 261.6 cm in length (0–45.5 cm from AIG16-06, and 40.2–256.3 cm from AIG16-08). Following the standard procedure (Heiri et al., 2001), loss on ignition (LOI) analysis was performed on 179 samples taken along the AIG16-MC core in order to estimate organic matter (OM) and carbonate contents in the sediments. The non-carbonate ignition residue (NCIR) was obtained by subtracting the LOI at 550 and 950°C from the initial dry weight. X-ray fluorescence (XRF) analysis was performed on the surfaces of the split sediment cores at 1-mm intervals, using a non-destructive Avaatech core scanner (Richter et al., 2006). The relative geochemical compositions (intensities), expressed in counts per second, were obtained at various tube settings: 10 kV at 1.5 mA for Al, Si, P, S, K, Ca, Ti, Mn, and Fe; 30 kV at 1 mA for Cu, Ni, Zn, Br, Sr, Rb, Zr, and Pb with each run lasting 30 s. Principal component analysis (PCA) was performed on the geochemical results using R software (R Core Team, 2018) to identify principal sediment end-members, which were then used to better constrain each sedimentological facies and to select the best elements for tracing the erosion dynamic (e.g., Sabatier et al., 2010). Relative abundances of elements are expressed as centered log-ratio (CLR) to avoid dilution effects due to water (e.g., Weltje et al., 2015). The following elements (n = 15) were used to calculate the geometric mean: Si, P, S, K, Ca, Ti, Mn, Fe, Zn, Br, Rb, Sr, Zr, and Pb. For the same potential matrix effects, element ratios are expressed as logarithms (ln) of XRF count ratios that are linearly related to the log ratios of corresponding absolute concentrations (Weltje and Tjallingii, 2008).
Chronology
The core chronology is based on four AMS 14C dates, which were obtained on selected terrestrial plant macroremains (Table 1), and short-lived radionuclide measurements. Clam (Blaauw, 2010), written for the open-source statistical software ‘R’, was used to calibrate the 14C ages with the Intcal20 calibration curve (Reimer et al., 2020) in order to construct an age-depth model. For short-lived radionuclides, a continuous sampling step of 10 mm was applied over the first 22 cm of AIG08-04 to determine 210Pb, 226Ra, and 137Cs activities. The analysis was carried out on well-type, germanium detectors located at the Laboratoire Souterrain de Modane following the procedure described by Reyss et al. (1995). For each sample, the 210Pb excess activities were calculated by subtracting the 226Ra-supported activity from the total 210Pb activity. The age model was then computed using a Constant Flux Constant Sedimentation model on the R package serac (Bruel and Sabatier, 2020).
Pollen and Sporormiella sp. Spore Analysis
Forty-eight sediment subsamples of 1 cm3 were taken at a mean temporal interval of ca. 40 years for pollen and Non-Pollen Palynomorph (NPPs) analyses. As far as possible, event layers were avoided during the sampling in order to avoid the taphonomic problems caused by the different origins of such sediment deposits. Samples were prepared using the standard procedure described by Faegri and Iversen (1989). Lycopodium clavatum tablets were added to each subsample (Stockmaar, 1971) in order to calculate Sporormiella sp. (HdV-113) spore concentrations. Pollen grains were identified using atlases of European and Mediterranean pollen grains (Reille, 1992; Beug, 2004). The identification of Sporormiella sp. is based on several published photographs (van Geel and Aptroot, 2006; Cugny et al., 2010) and follows the nomenclature established by Miola (2012). Sporormiella spores were counted following the procedure described by Etienne and Jouffroy-Bapicot (2014) and expressed in accumulation rates (no.cm–2.yr–1). At least 500 pollen grains of terrestrial plants (TLP: Total Land Pollen) were identified. Pollen counts were expressed as percentages of TLP, excluding pteridophytes, aquatic plants and Cannabis/Humulus pollen-type from the total pollen sum. Pollen data have been divided into six Local Pollen Assemblage Zones (LPAZ) by constrained cluster analysis (CONISS) using Tilia software (Grimm, 1987). In order to identify the main changes in plant community composition according to the cultural periods, a non-metric multidimensional scaling (NMDS) was performed using the Bray-Curtis dissimilarity indices with the metaMDS function from the VEGAN package v2.5.6 in R (Kruskal, 1964; Legendre and Legendre, 2012; Oksanen et al., 2013). This analysis was performed including three dimensions and a color code was used to distinguish the main cultural periods.
DNA Analysis: From the Sampling to the Data Treatment
Twenty-four sediment slices, each 1 cm-thick, were taken with a temporal interval fluctuating between 15 and 120 years (mean 75 years). As in the case of pollen, event layers were avoided during sampling. The edges were removed to avoid contaminations and were used to determine the sediment water content. This measurement allowed the estimation of the dry sediment quantity used for the DNA extraction. This quantity varies between 1 and 4.4 g of dry sediments. The protocols applied for the sampling and the DNA extractions are described in detail on Protocols.io1. To summarize, we mixed the wet sediments with a phosphate buffer (for 1 g of dry sediment we added 2 ml of phosphate buffer) for 15 min in a falcon tube. After a first centrifugation, supernatant was transferred to Amicon ®ultra-15 10k centrifugal filters (Millipore) and centrifuged again to concentrate DNA and increase the detectability of DNA fragments of interest (Bremond et al., 2017; Capo et al., 2021). Between 500 and 700 μl of DNA extract were recovered and 400 μl was used as starting material for the DNA extraction, using a NucleoSpin ® Soil kit and starting at binding step (Macherey-Nagel, Düren, Germany). Then, all of the DNA extracts were quantified by using the Quantifluor ® ONE dsDNA system from Promega. Four extraction controls were performed.
The extracted DNA was then amplified using primers g and h for plants (Taberlet et al., 2007) and MamP007 (in combination with a human-specific blocking primer) for mammals (Giguet-Covex et al., 2014). For each sample and marker, nine PCR replicates were performed in order to improve the detection of rare taxa and the robustness of the data (Ficetola et al., 2015). Two negative PCR and two positive PCR controls were included. For the plants, the positive PCR controls contained 0.18 ng of DNA from a subtropical/tropical plant from the Melastomataceae family. For the mammals, the positive PCR controls contained 0.18 ng of DNA extracted from a marsupial (Didelphis marsupialis) that does not exist in Europe. The sequencing runs were performed by 2 × 125 bp pair-ends sequencing on an Illumina HiSeq 2500 platform. The sequences were filtered using the OBITOOLS software2 (Boyer et al., 2016) and following the procedure described in Giguet-Covex et al. (2019). Reference databases used for the assignations were built from the EMBL database with the ecoPCR program (gh database created from release r126, mamP007 database from release r133). For the plant DNA, only DNA sequences with a > 95% similarity with a sequence in the reference database were kept. For mammals, after having removed all sequences assigned to Hominidae, only DNA sequences with a > 97% similarity with a sequence in the reference database were kept. Additional filtering steps, relating to low or stochastic detections (minimum of five reads per PCR and in two sample replicates or one replicate but in two consecutive samples) and potential contaminations (based on the extraction and PCR controls and exotic assignations), were performed (Giguet-Covex et al., 2019). For the mammals, only one taxon was frequently detected in the negative controls (in 31 over 53) and excluded from the dataset. It is also assigned to an exotic taxon, the Meleagris gallopavo. Among the six remaining taxa, two were assigned to water birds (Fulica atra and Anas platyrhynchos), two to fish (Salvelinus sp. and Rutilus sp.), one to an exotic rodent (Thryonomys swinderianus) and one to a marine worm (Phascolosoma esculenta). These last two taxa are the most detected in the sediment record and because they were not frequently detected in the controls (in less than 5 over 53), we checked the suspicious assignments with Blast. The results proposed a 100% of identity with Sus scrofa for the rodent and with Bos taurus for the marine worm, which is highly plausible in our context. In this manuscript, we use only these mammals to document the past farming history. However, we note that the detection of taxa other than mammals with the primer pair MamP007 has already been demonstrated (Annelida, Lammers et al., 2018). Moreover, when we blast the primers, bony fish can also clearly be amplified. For the plants, no taxa were frequently detected in the controls (no more than four positive controls over 53). However, over the five sequences detected in four controls, two correspond also to exotic taxa (Cedrus sp. and Caricaceae) and were excluded from the dataset. The other excluded exotic taxa are Grubbia rosmarinifolia, Passiflora sp., Araceae, Millettieae, Musaceae, and Persicaria sp.
The results for each taxon are presented in numbers of positive PCR replicates, and for plants, we also integrated the sum of DNA reads. To highlight changes in groups of plants associated (or potentially associated) with human activities, we also calculated the proportion of DNA reads [log-transformed reads due to the exponential multiplication of the DNA sequences during the PCR; Log(N reads + 1)] represented by each of these groups, i.e., (1) trees and shrubs, (2) ruderal and nitrophilous plants related to pastoral activities and/or hay meadows (Urtica sp., Plantago sp., Rumex sp., Rhinanthus alectorolophus, Dactylis glomerata) and (3) cultivated taxa (fruit trees, cereals, hemp, hops, several garden plants, and associated weeds). Based on the sum of the DNA reads from the sample replicates, we determined the number of detected taxa. A taxon was considered in the calculation when the number of reads was higher than 45 (i.e., 5 reads × 9 replicates). As in the case of pollen data, a non-metric multidimensional scaling (NMDS) was performed using the Bray-Curtis dissimilarity indices with the metaMDS function from the VEGAN package v2.5.6 in R. For this analysis, we summed the number of reads of three PCR replicates to finally have three replicas per sample and so decrease the stochasticity of the PCR success. This analysis was performed including three dimensions and a color code was used to distinguish the main cultural periods.
Results and Interpretations
Sedimentary Units
The description of AIG16-MC led to the identification of different units characterized by thin laminae and interrupted by event deposits. The regular millimetric laminae are made up of an alternation of beige, grayish and green laminae. At certain depths, we observed homogenous olive-gray deposits topped by light gray mm-thick clay caps. The base of these deposits is sharp and presents high organic debris content but no visual grain size variation. Along the entire core, we observed 14 of these event deposits, with homogeneous facies ranging between 0.3 and 2.3 cm in thickness. These events are considered as instantaneous deposits (Sabatier et al., 2017) and were removed from the age-depth modeling. The origin of these deposits is currently being investigated (flood vs. earthquake induced deposit), but their nature (sudden erosive event) and composition mean that they fail to combine all data types for paleoecological studies. They were therefore not sampled and were omitted from the discussion regarding environmental variations.
The identification of each sedimentary unit (U1, U2, and U3) is based on sedimentary characteristics such as color, grain size and sediment structures with lamination frequency and LOI contents (Figure 2). Unit 1 (U1: 0 to 21 cm) is characterized by a silty to clayey sediment with regular alternation of millimetric laminae. Mean organic content (LOI550) and carbonate content (LOI950) are relatively high with 9.3 and 23.3%, respectively. Two dark laminae appear in this unit. Unit 2 (U2: 21 to 141.2 cm) is composed of brown silty-clay sediment and presents an irregular and low presence of rhythmic lamination. Mean organic (8.6%) and carbonate (16.3%) contents are relatively low. This unit comprises 9 event deposits. It evolves progressively downwards into a more laminated and darker sediment. Unit 3 (U3: 141.2–261.6 cm) is composed of an alternation of light olive gray millimetric to sub-millimetric silty/clayey laminae. Mean organic (10.4%) and carbonate contents (19%) are slightly higher than those of U2. Five instantaneous deposits were identified in U3.
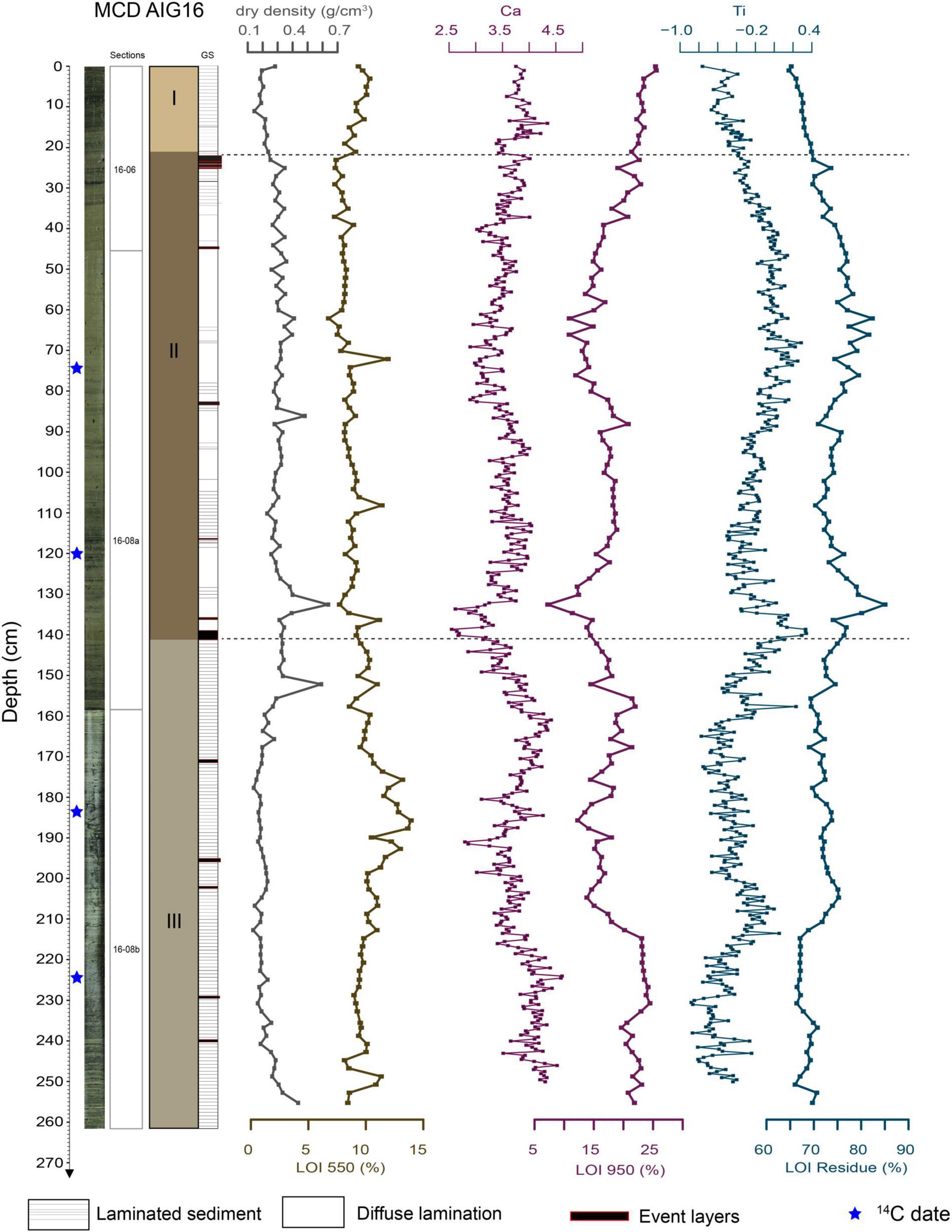
Figure 2. Sedimentological description of AIG16-MC including photography, lithological facies and units, dry density measurements, LOI analyses and selected XRF core scanner elements (centred-log ratios) reflecting the minerogenic fraction (Ti as proxy for the non-carbonated detrital compounds and Ca for the carbonated fraction).
Geochemistry
A PCA analysis was performed on the following raw elements, measured with the Avaatech XRF core scanner: P, K, Ti, S, Ca, Fe, Mn, Br, Zr, Rb, Zn, Sr, and Pb. The first axis explains 43.4% of the total variance and the second, 22.3% (Figure 3A). Five different end-members can be identified. With negative loading on the first PCA axis, Ca, S, and Br form an end-member (EM1) reflecting the autochtonous biogenic production made up of bio-precipitated calcite and lacustrine organic matter, as Br content is related to lake organic matter (Lefebvre et al., 2021). With positive loading on PC1, there are the carbonated and silicated coarse detrital components characterized by Sr and Zr, respectively (EM2). With positive loading on both the first and second axes, we find the siliciclastic fine detrital component made up of K and Ti (EM3). With negative loading of the first axis and positive of the second axis, the redox-dependent elements Fe and Mn are grouped together (EM4). Between this end-member and that of biogenic production, there is a last end-member (EM5) corresponding to elements typical of lead (Pb) and nutrient (P) pollution. The silicium (Si) has a positive loading on the second PCA axis. Its position between the detrital and biogenic components suggests a mixed origin, from both clastic inputs and diatoms. The first unit (U1) is mostly characterized by the pollution end-member, i.e., EM5. In the second unit (U2), the geochemical signature is dominated by the inputs of coarse carbonated and silicated detrital particles, but it also contains fine silicated particles, as indicated by the PCA analysis and the bi-plots (Figure 3B). In unit 3 (U3), some depths are characterized by very low detrital inputs and are dominated by autochtonous production (especially bio-induced calcite), while others are slightly enriched in fine and silicated detrital inputs and redox-dependent elements as indicated by the PCA analysis and the bi-plots (Figure 3). Based on the PCA results and in order to better qualify the erosion dynamic, we selected three ratios, (i) ln(Ti/Ca) reflecting the silicated relative to the carbonated detrital inputs, (ii) ln(Sr/Ca) reflecting the carbonated detrital inputs relative to the bio-precipitated calcite, and (iii) ln(Zr/Ti) reflecting the coarse relative to the fine silicated detrital inputs, and we compared them with each other (Figure 3B). The significant and high correlation (r2 = 0.81, p < 0.0001) between ln(Ti/Ca) and ln(Sr/Ca) suggests a common origin for the silicated and carbonated detrital materials. ln(Zr/Ti) and ln (Sr/Ca) (r2 = 0.81, p < 0.0001) are also correlated, but the lower correlation suggests the contribution of a distinct source of coarse silicated materials.
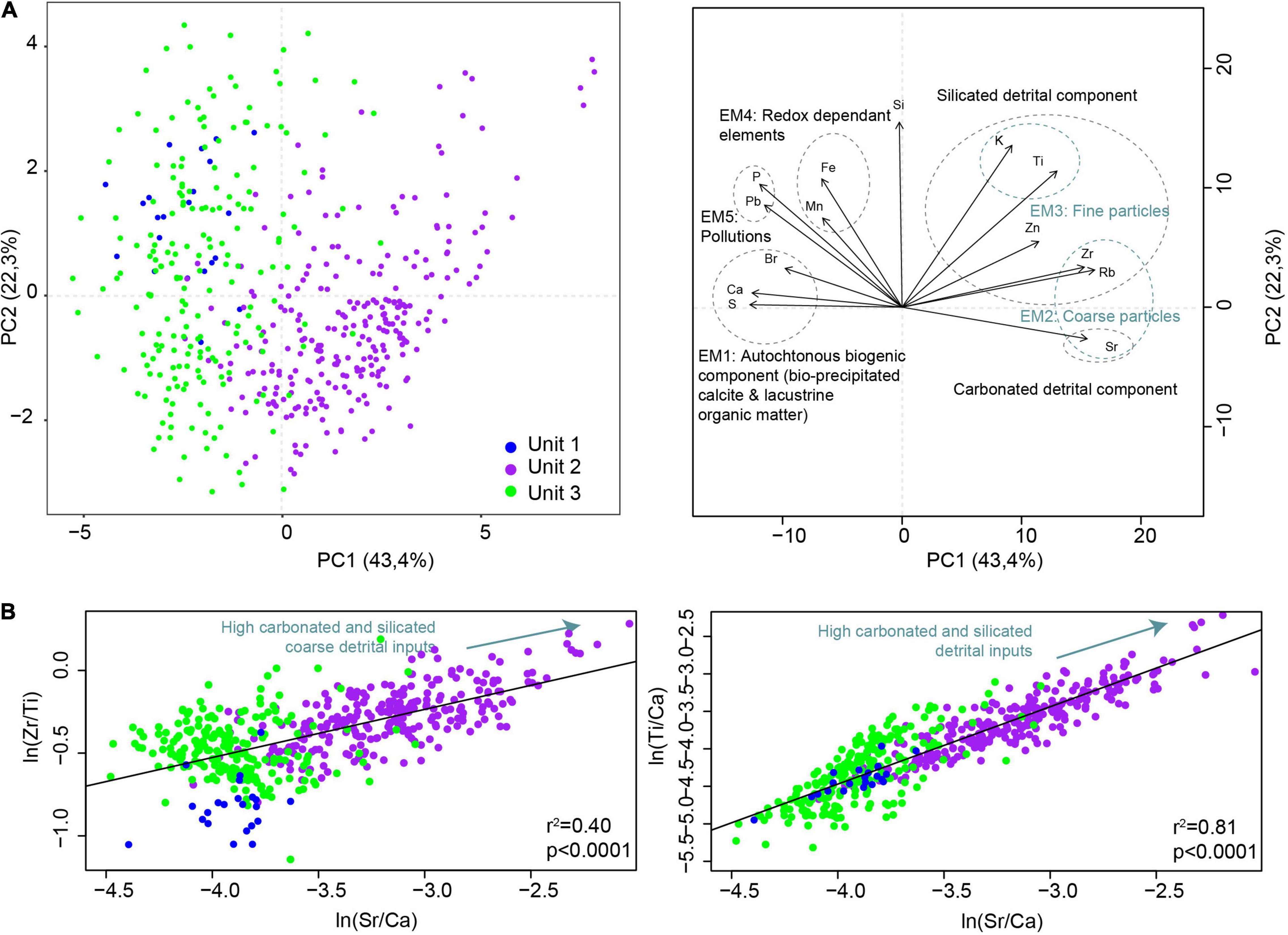
Figure 3. Geochemical patterns from AIG16-MC. (A) Results from the PCA. Five end-members corresponding to sediment fractions of different origins or related to different sedimentary processes are highlighted. (B) Biplots comparing Zr/Ti (proxy of coarse and silicated detrital inputs) with Sr/Ca (proxy of the carbonated detrital fraction) on the left side and Ti/Ca (proxy of silicated detrital inputs) with Sr/Ca (proxy of carbonated detrital inputs) on the right side.
Chronology
The excess 210Pb downcore profile plotted on a logarithmic scale revealed well constrained linear trends providing a mean sedimentation rate of 2.44 ± 0.1 mm.yr–1 for the upper 22 cm (Figure 4). The 137Cs profile presented two peaks, at 4.5 and 9.5 cm, which correspond respectively to the Chernobyl accident in 1986 AD and the maximum of Nuclear Weapon Tests (NWT) in the Northern Hemisphere in 1963 AD in this region (Sabatier et al., 2014). This interpretation is confirmed by the 241Am peak attributed to the NWT (Appleby, 1991; Figure 4). The first 137Cs fallout is observed at 13.5 cm and corresponds to 1955 AD (Robbins and Edgington, 1975). Ages were then calculated using the CFCS model thanks to serac R package (Bruel and Sabatier, 2020) to provide a continuous age-depth relationship (Figure 4).
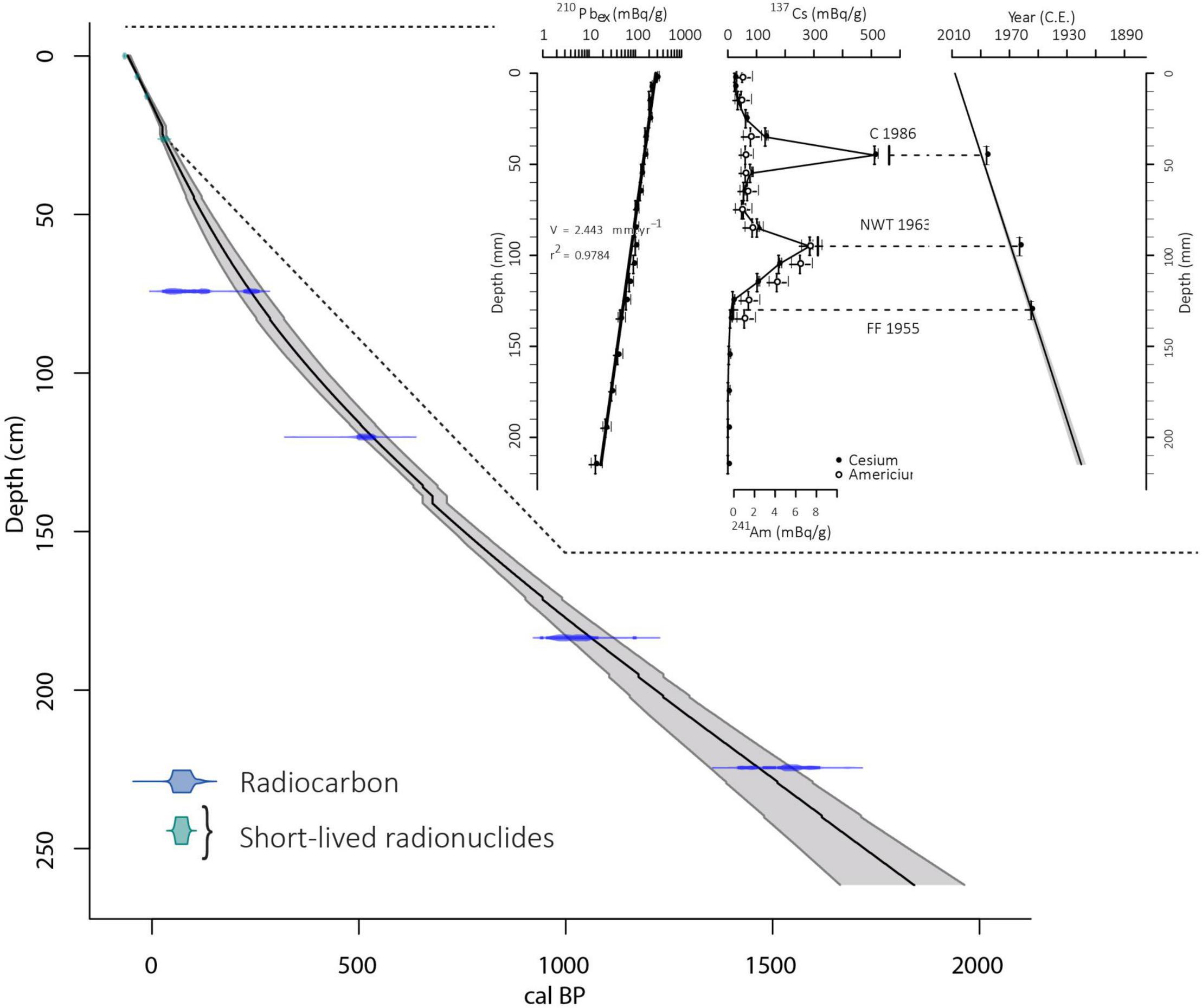
Figure 4. Age-depth model based on radiocarbon (blue) and short-lived radionuclides (green) for the entire AIG16-MC sediment sequence. The age-depth model based on radionuclides is derived from serac package with from left to right: 210Pbex, 137Cs and 241Am activities and CFCS age model.
A total of four terrestrial organic macroremains were dated by 14C dating (Table 1). The 14 deposits interpreted as event deposits (ED), representing a total of 9.9 cm, were removed. The remaining 251.7 cm were used to build an event-free sedimentary record (Goldberg, 1963; Wilhelm et al., 2012). We then calculated an age depth relationship by a smooth spline interpolation using the R code package “Clam” (Blaauw, 2010). The vertical bars represent the age of event deposits (Figure 4). This sediment sequence from Lake Aiguebelette covers the last 1816 ± 65 yr cal BP. The mean sedimentation rates estimated from the event-free sediment record vary between 3.2 and 0.9 mm.yr–1 with a higher sedimentation rate over the upper 60 cm (last 230 years).
Pollen and Sporormiella sp.
The NMDS analysis applied to pollen data (Figure 5A) highlights the difference (along the first axis) between samples corresponding to Roman and Early Middles Ages periods, and samples corresponding to the subsequent periods. In fact, samples dated to the Roman period and the Early Middle Ages are characterized by an abundance of trees, such as Fagus and Ulmus (Figure 5B), which drastically declined after 1050 cal CE (Figure 6). The samples dated to the High Middle Ages seem to represent a transition period (middle of the first Axis, Figure 5A), while samples dated to subsequent periods are marked by the amount of herbs (Figure 5C) and cultivated taxa they contain, apart that is from the very last samples (Contemporary), which are marked by the expansion of Populus, Fraxinus and the introduced taxon Ambrosia (Figure 5C).
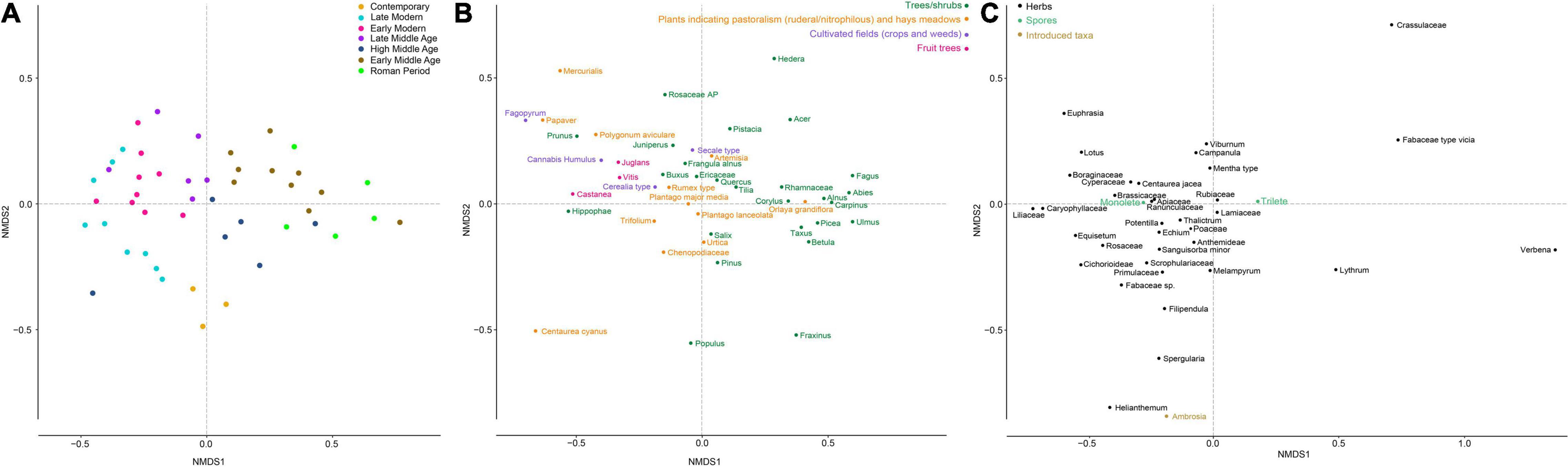
Figure 5. Temporal trajectory of plant composition as revealed by a non-metric multidimensional scaling (NMDS) analysis from pollen data (stress value = 0.14). (A) Sample distribution in the NMDS plot. The color code represents the different cultural periods. (B) Distribution of arboreal pollen taxa (AP) and taxa related (or potentially related to) human activities in the NMDS plot. (C) Distribution of all other taxa in the NMDS plot.
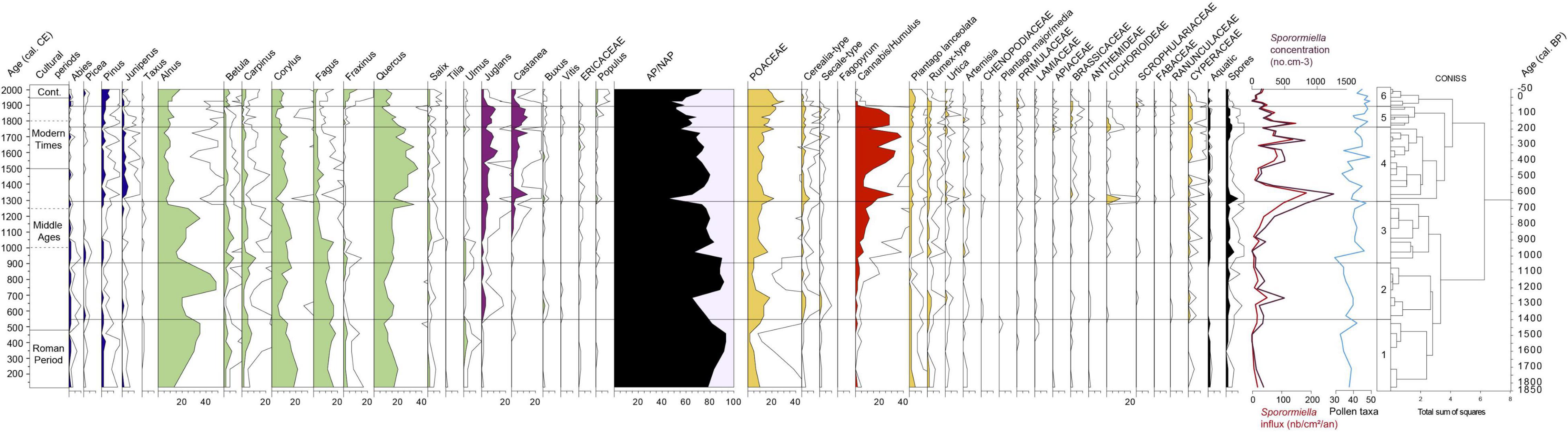
Figure 6. Pollen diagram from core AIG 16-MC presented as a percentage of the pollen sum. + Sporormiella sp. concentrations and accumulation rates. The exaggeration curves are x5.
Based on the pollen diagram (Figure 6), it is possible to describe 6 main palynozones, defined according to the CONISS analysis. As the age model is defined, all pollen data are presented relative to the chronology.
LPAZ 1 (120–550 cal CE)
The vegetation was dominated by arboreal pollen (Figure 6), AP/TLP percentages fluctuated between 80 and 95%. Four tree taxa recorded percentages above 10%: Alnus increased from 10 to 35%, Corylus and Quercus decreased from 20 to 10% and Fagus oscillated between 12 and 20%. Percentages of Betula, Pinus, Ulmus, Fraxinus, and Carpinus were all within a range of 1–10%. Other trees and shrubs remained below 1%.
Among herb taxa pollen, Poaceae dominated and decreased from 10% to 2% before slightly increasing to 10%. Several ruderal weeds were recorded (Plantago lanceolata, Rumex-type, Urticaceae, Artemisia, Chenopodiaceae, Plantago major/media) and all percentages decreased from 250 cal CE before finally increasing at the end of the zone. In each subsample, Cerealia-type and Secale-type were identified with percentages under 1%, while hemp pollen was recorded in some samples. The accumulation rate as well as the concentration of Sporormiella sp. stayed at low values (Figure 6).
LPAZ 2 (550–900 cal CE)
Alnus, the dominant taxon, decreased from 35 to 20% and reached its highest percentages of around 45% from 740 AD and then decreased once again from 800 AD (Figure 6). Percentages decreased and increased once again at the middle of the zone for Betula, Corylus, and Carpinus; and at the end of the zone for Ulmus and Tilia. Percentages of Quercus and Fagus remained at the same order of magnitude as in the previous zone. Juglans percentages exceeded 1% and rose to 4% around 650 cal CE. At the same period, peaks of Cerealia-type, Secale-type, Poaceae, ruderal plants (Rumex sp. and Urtica sp.) and Cannabis/Humulus were also recorded. The concentration and the accumulation rate of Sporormiella sp. also showed a small peak at 50 no.cm–2.yr–1 around 700 cal CE.
LPAZ 3 (900–1300 cal CE)
Alnus decreased to 14%, increased to around 25–35% and then decreased again to 9% at the end of the zone (Figure 6). Quercus remained stable at around 15%, except for a peak of 34% at 1270 cal CE. From 1050 cal CE, Fagus decreased from 16 to 7%, as did Ulmus and Tilia although to a lesser extent; at the same time, we observe an increase in Juglans and Castanea percentages.
In this zone, open areas were expanded (AP/TLP decreases from 90 to 75%), with an increase of Poaceae (to around 10%), ruderal plants and Cannabis/Humulus. A diversification of prairie and ruderal plants was also recorded. An important increase in Sporormiella sp. (accumulation rate and concentration) was recorded from 1200 cal CE (Figure 6).
LPAZ 4 (1300–1760 cal CE)
Quercus increased and became the dominant taxon with percentage values of around 20% and 35% (Figure 6). Juglans and Castanea percentages reached their highest values (13%). The percentages of Juniperus also increased. Most herb taxa remained at the same order of magnitude. Poaceae pollen grains were slightly more abundant than in the previous zone. It should be noted that at 1300 cal CE, a significant decrease in AP/TLP (to 45%) and peaks of Cerealia-type, Poaceae and Cichorioideae percentages probably correspond to an assemblage of spores and pollen grains brought into the lake by a flood. This sample is an outlier in the NMDS analysis due to the high values for Cichorioideae (Figure 5A). It will not be included in the environmental interpretation. Excluding this particular sample, in general Cerealia-type and Secale-type present values that are somewhat higher than those observed in LPAZ 3. Percentages of Cannabis/Humulus reached their highest values. Around 1350 cal CE, the accumulation rate and concentration of Sporormiella sp. reached its highest values (180 no.cm–2.yr–1) before decreasing to low values and then increasing again from 1550 cal CE (Figure 6).
LPAZ 5 (1760–1900 cal CE)
Quercus progressively decreased from 20 to 7% (Figure 6). The proportion of herbs increased (mean of AP/TLP decreased from 65 to 60%) mainly with the increase of Poaceae from 15% to 24%. The concentration and the accumulation rate of Sporormiella sp. remained relatively high (Figure 6).
LPAZ 6 (1900 cal CE to the Present Day)
Many tree taxa recorded an increase in their percentages, like Salix, Alnus (from 8 to 17%), Betula, Pinus, Fraxinus (from 1 to 12%), Fagus, Carpinus and Populus (Figure 6). On the other hand, Juglans and Castanea decreased from 10 to 1% and from 7 to 4% respectively. Among the herbs, Poaceae decreased from 30 to 15%. Cumulative percentages of ruderal plants decreased from 10 to 5% at the end of the zone, mainly due to decreases in Plantago lanceolata and Rumex-type. Cerealia-type and Secale-type decreased also, from 4 to 1%, while Cannabis/Humulus percentages decreased sharply. The accumulation rate of Sporormiella sp. decreased before increasing again in the recent period (Figure 6).
Plant DNA
The filtering procedure yielded 2,305,749 DNA reads and 235 unique sequences. We grouped all unique sequences with identical assignments and finally obtained 174 plant taxa including 23 aquatic taxa. Within the 151 remaining terrestrial taxa, five were assigned to moss or ferns, six to fruit trees and shrubs, 17 to cultivated fields (cultivated plants and weeds) and vegetable gardens, five to plants associated with pastoral activity and/or hay meadows, 93 to herbaceous plants and 25 to trees and shrubs.
In order to highlight the main changes in plant community composition, an NMDS analysis was performed, and samples were grouped by different cultural periods (Figure 7). The most important shift appeared along the first axis and separated the Roman period and the Early Middle Ages from the following periods (Figure 7). It should be noted however, that the end of the Roman period can be distinguished (Figure 7). Unlike the pollen data, the samples related to the High Middle Ages are clearly closer to those related to the following periods. Hence, we can summarize the NMDS analysis into two main phases. Before 1050 cal CE (Roman period and Early Middle Ages), the plant community composition was characterized by the presence of many trees, shrubs, (e.g., Pinus sp., Aquifoliaceae, Ulmaceae, Fagus sp., Picea sp., Alnus sp., Betulaceae, Hedera helix, and Tilia sp.) and a number of herbaceous taxa, some of which were growing in forest or forest edge environments (e.g., Veronica montana and Dipsacus sp.). From 1050 cal CE (High Middle Ages to nowadays), the plant community composition shifted to cultivated plants and plants associated with pastoral activities as well as different herbaceous taxa. This tipping point also corresponded to the period after which the proportion of tree and shrub DNA reads decreased (Figure 8). A new increase only occurred in the last 50 years. In contrast to the pollen data, the proportion of DNA reads was always dominated by herbaceous taxa. The number of terrestrial taxa also strongly increased since 1050 cal CE, from 43 taxa on average (min = 20; max = 60) to 78 (min = 60; max = 90).
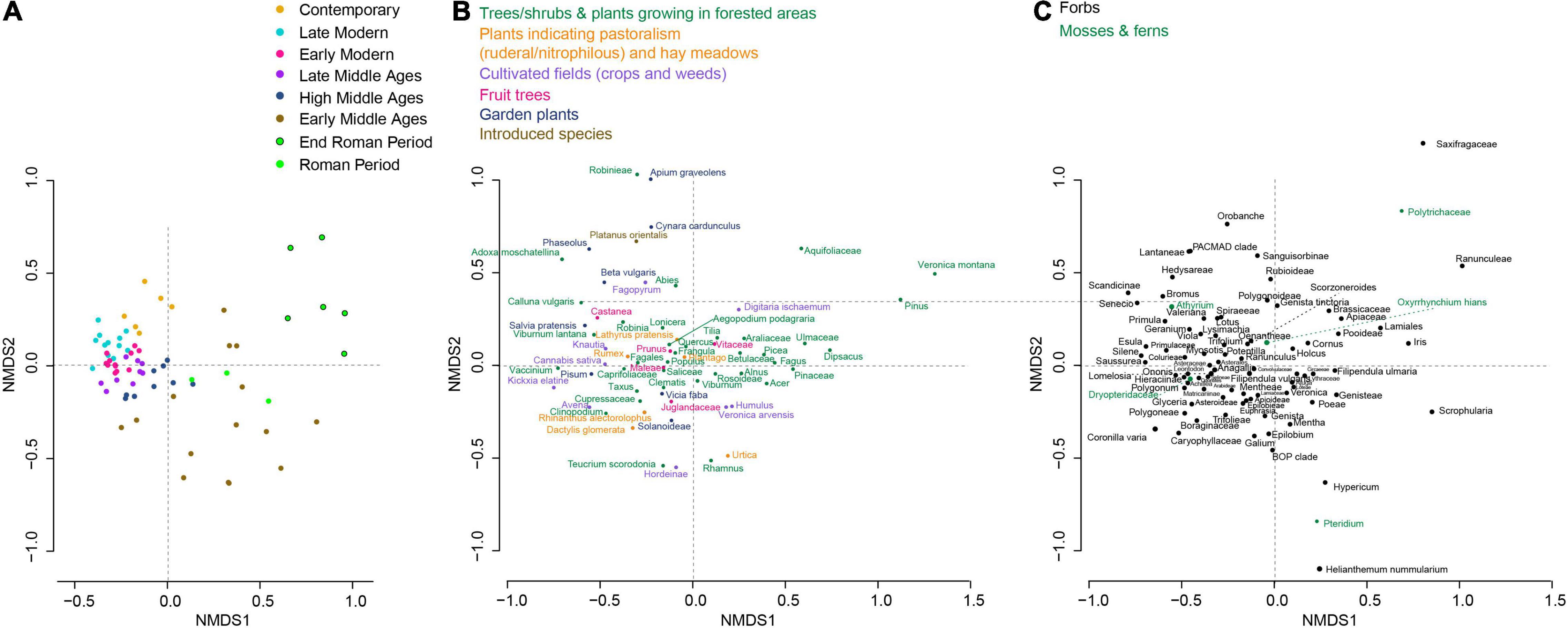
Figure 7. Temporal trajectory of plant composition as revealed by a non-metric multidimensional scaling (NMDS) analysis from lake sedaDNA data (stress value = 0.14). (A) Sample (each replicates) distribution in the NMDS plot. The color code represents the different cultural periods. (B) Distribution of taxa related to (or potentially related to) human activities in the NMDS plot. (C) Distribution of all other taxa in the NMDS plot.
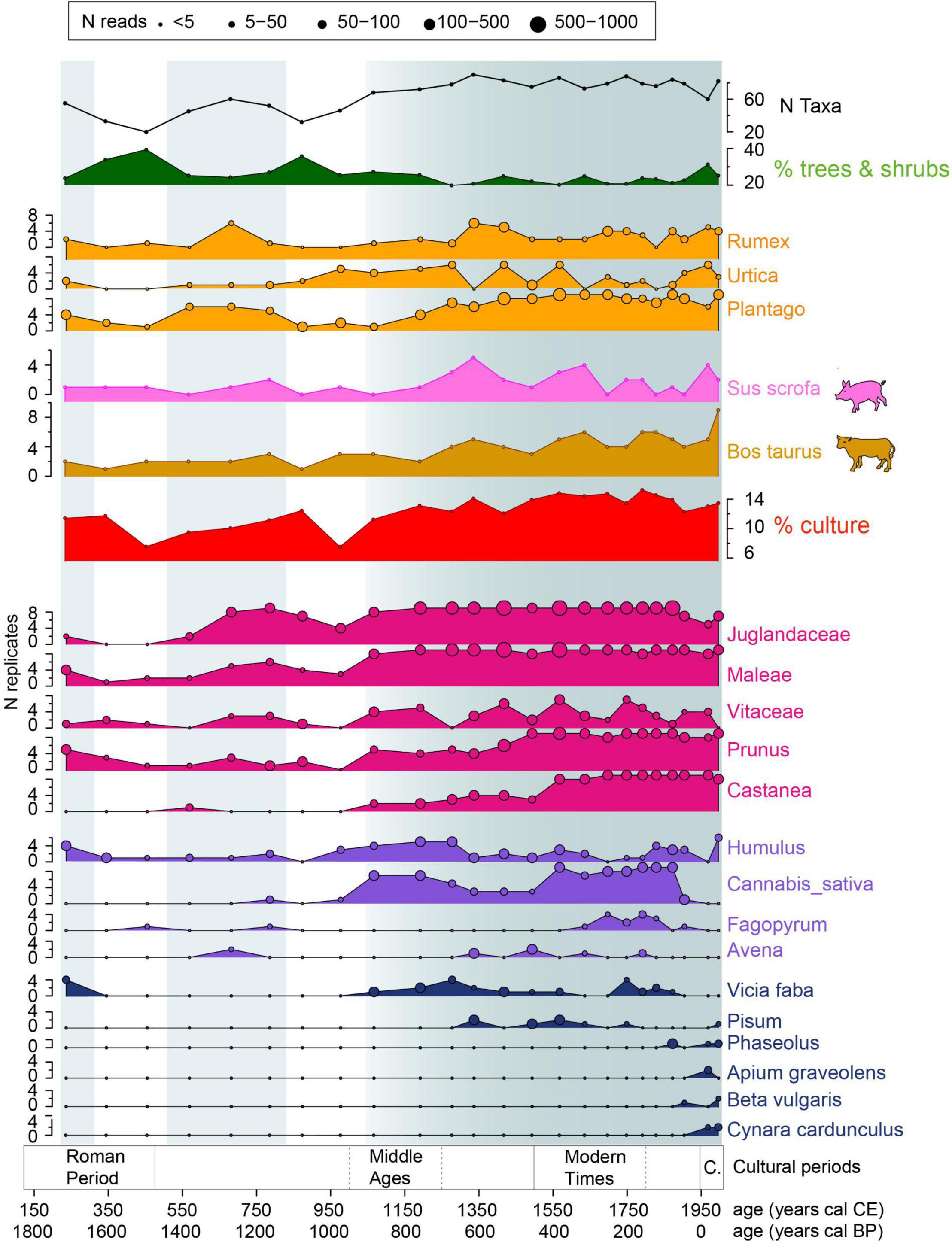
Figure 8. Main plant and mammal DNA results illustrating the evolution of forest cover (% of DNA reads from trees and shrubs), the number of taxa detected and the diverse farming activities (pastoralism, livestock farming, fruit tree cultivation, cultivated fields with cereals or other plants and garden plants). Blue areas highlight main phases of agricultural development.
Before 1050 cal CE, two phases characterized by a small increase in the number of taxa and a decrease in the proportion of DNA reads from trees and shrubs were recorded, around 250 cal CE and between 500 and 800 cal CE (Figure 8). During these phases of the Roman period and Early Middle Ages, we see the development of plants associated with pastoral activity (Plantago sp., Urtica sp. and a few Rumex sp.). Fruit trees (Vitaceae, Juglandaceae, and Maleae) were recorded (Figure 8). In the first phase, Vicia faba and Humulus sp. were also detected. However, this phase was only represented by one sample.
From 1050 cal CE, a general trend toward a progressive intensification and diversification of agropastoral activities can be observed. In detail, from 1050 cal CE, Castanea sp., Cannabis sativa and Vicia faba appeared, while Vitaceae, Prunus sp. and Humulus sp. increased considerably (high N replicates). Then at 1300 cal CE, Pisum sp. and Avena sp. were introduced. Moreover, some plants associated with pastoral activities (Lathyrus pratensis, Dactylis glomerata, Rhinanthus alectorolophus; Figure 7) appeared or began to be detected in large quantities (Rumex sp.). At that time, Cannabis sativa and Humulus sp. decreased. Cannabis sativa, Prunus sp. and Castanea sp. showed a new increase from 1500 to 1900 cal CE. During this period, Avena sp. and Pisum sp. decreased while Fagopyrum sp. started to be detected and Vicia faba appeared again. Finally, from 1850 cal CE, new taxa, i.e., Phaseolus, Beta vulgaris, Cynara cardunculus, and Apium graveolens, which we can see today in vegetable gardens were recorded. In this phase, most of the fruit tree taxa (Vitaceae, Juglandaceae, Prunus sp.) and Humulus sp. decreased and other crops (Fagopyrum sp., Cannabis sativa, and Vicia faba) disappeared.
Mammal DNA
Two taxa could be related to livestock farming activity, Bos taurus and Sus scrofa. They were detected almost throughout the record, but increased considerably from 1250 cal CE (Figure 8) as did the accumulation rate of Sporormiella sp. (Figure 6). These increases occurred when plants associated with pastoral activities (i.e., hay meadows or pastures; Dactylis glomerata, Rhinanthus alectorolophus) appeared or were detected in more PCR replicates (Rumex sp., Plantago sp.). However, the proportion of DNA from plants associated with pastoral activities remained relatively stable throughout the record. From this date, the DNA detection of Bos taurus presented an increasing trend until the present day, while Sus scrofa showed an important peak around 1350 cal CE and a long-lasting decrease between 1650 and 1950 cal CE.
Discussion
Interpretative Framework of DNA and Pollen Records: Taphonomic Benefits and Drawbacks of Each Method
Pollen and DNA analyses have been combined in numerous paleoecological studies and for a variety of biomes (e.g., boreal, arctic, alpine, tropical, semi-arid). Both convergent and divergent results have been recorded (e.g., Parducci et al., 2013, 2017; Sjögren et al., 2017; Clarke et al., 2018; Giguet-Covex et al., 2019, in revision; Liu et al., 2020; Tabares et al., 2020). For instance, in boreal and arctic environments, it has been shown that pollen and DNA records were more similar in lakes with large catchment areas compared to those with smaller catchments, as these catchments cover a more regional scale pollen source (Alsos et al., 2016; Clarke et al., 2018). In our context, plant DNA and pollen records provided very similar stories, but essentially for taxa associated with agricultural and pastoral activities, i.e., for taxa growing in open areas and where soils are worked, trampled and therefore are more exposed to erosion processes. In contrast, for trees and shrubs, i.e., in areas where erosion is more reduced, we observed almost no concordance (Supplementary Figure 4). It has been demonstrated that extracellular DNA (i.e., the DNA fraction targeted in the study) is quickly bound to particles such as clays, which protects the molecules from microbial degradation (Blum et al., 1997; Kanbar et al., 2020). This mechanism means that we can expect to find good DNA records, in terms of spatial representativeness, in erosive contexts and periods (Giguet-Covex et al., 2019). In contrast, in forested areas (and during forested periods) where erosion is more limited, a poor spatial representativeness of taxa is to be expected which explains the poor similarities between the DNA and pollen records for several forest taxa. Moreover, the proportion of forested lands may be underestimated by the proportion of DNA reads from trees and shrubs. This assumption is verified at least for the most recent sample (25% in the 1990s compared to the 55% shown by the Corine land cover data, see Figure 1), where pollen analysis revealed 70% arboreal pollen (a bit overestimated).
Given the taphonomic processes outlined above and because the landscape and erosion dynamic can change markedly, especially in man-made contexts, changes in tree/shrub taxa compositions must be interpreted with caution, while the evolution of anthropogenic taxa can be considered as robust. However, while it is difficult to use DNA as a proxy of tree cover, it can be very useful for confirming the presence of a specific tree/shrub taxon in a watershed. Indeed, taxa detected by DNA are necessarily present in the catchment area and probably close to the lake border and main tributaries (especially riparian species) (Sjögren et al., 2017; Alsos et al., 2018) and at the edges of open areas.
In contrast to sedaDNA data and as shown for the most recent sample, percentages of tree and shrub pollen grains are often overestimated relative to the real forest cover. This phenomenon is accentuated when the landscape becomes more open because of the increase in long-distance pollen inputs (Crump et al., 2019). Indeed, because most tree pollen grains are transported by winds, the eternal question is: is the pollen the echo of a regional vegetation pattern or of local changes? This obstacle can be overcome with different approaches. When they are preserved in lake sediment, the macrofossil remains, combined with pollen can help to disentangle local and regional vegetation. Another solution would be to use current modeling approaches to modulate the overrepresentation of tree pollen, based on pollen productivity estimates (Sugita, 2007a,b). The overestimation of the forest cover in pollen assemblages is accentuated by the poor representation (or the absence) of low pollen-producing entomophilous taxa such as forbes (Clarke et al., 2018). However, these entomophilous taxa are better represented in lake sedaDNA datasets (Parducci et al., 2017; Clarke et al., 2018, etc.). They are also often key ecological taxa or informative taxa relating to agricultural or pastoral activities and practices.
New Insights Into Paleoecological Interpretations and Human History Based on the Combination of Pollen and DNA Analyses
The different abilities of DNA, coprophilous fungal spores and pollen grains to mirror the development of trees, crops, vegetable cultivation and pastoral activities, makes their combination particularly effective for the reconstruction of past human activities and impacts.
The ability of DNA analysis to detect entomophilous taxa is a major bonus in paleoecological studies. It offers the opportunity to identify cultivated fruit trees, such as Prunus sp. (potentially plums and or cherries) and Maleae (potentially apple and/or pear). For instance, in the Aiguebelette record, the development of orchards is highlighted during medieval times and in the modern period (Figure 8). While Vitis was not identified through pollen analysis, numerous DNA sequences of Vitaceae are recorded for the Middle Ages (Figure 8).
DNA analysis also offers a new perspective for the identification of vegetable crops, such as beans. At Aiguebelette, this allows us to identify the local cultivation of Vicia faba from 1050 cal CE and Pisum sp. from 1350 cal CE (Figure 8). The present study brings to light this cultivation for the first time in a natural archive. It is worth noting that this interpretation of our dataset is consistent with documented medieval practices (Colardelle and Verdel, 1993; Ruas, 1997; Ferault and Le Chatelier, 2012). DNA approaches also allow the detection of new species, such as Apium graveolens, Beta vulgaris, Cynara cardunculus or Phaseolus sp, from 1900 cal CE in local cultivation.
In many pollen records from Western Europe, the Cannabis/Humulus pollen type is widely recorded from the last two millennia (Doyen, 2012). Most of the time, it is interpreted as the signal of hemp cultivation, but actually it is not easy to determine whether it reflects the growing of hemp and/or hops. This question can be resolved using DNA analysis because the technique allows us to distinguish between Humulus sp. and Cannabis sp. In the Aiguebelette sequence, the DNA analysis confirms that the high percentages of Cannabis/Humulus pollen type from 1050 cal CE reflect hemp cultivation (and certainly hemp retting in lake water). At the same time, the DNA analyses also demonstrate an important increase in Humulus (Figure 8), revealing that hops were grown too, probably for brewing. An identical decline in Cannabis/Humulus pollen percentages and Cannabis sp. DNA is concomitantly recorded from approximately 1850 cal CE and reflects the cessation of hemp cultivation in the watershed.
In paleoecological studies, cereal growing is usually recorded by two pollen types, namely “Cerealia-type” and “Secale-type.” Unfortunately, the plant DNA primers used for amplifications are not sufficiently resolutive for the Poaceae family (Yoccoz et al., 2012; Parducci et al., 2017), which means that the information provided by DNA about cereal cultivation is very limited. That represents a real weakness of sedaDNA analysis based on the gh primer, but this could be overcome by using a Poaceae-specific primer targeting the internal transcribed spacer region 1 (ITS1) of nuclear ribosomal DNA (Ait Baamrane et al., 2012; De Barba et al., 2014). Nevertheless, DNA approaches highlight the cultivation of more sporadically cultivated plants such as Avena sp. (oat) and Fagopyrum sp. (buckwheat), which display their highest values between 1650 and 1850 cal CE at Aiguebelette.
The evolution of pastoral pressure is generally described in pollen diagrams using the evolution of several herbaceous taxa, combining apophytes directly favored by pastoralism (Plantago lanceolata, Rumex acetosella, etc.) and other taxa which contribute to both pasture and hay meadows (Poaceae, Anthemideae, Cichorioideae, etc.). The analysis of strict coprophilous fungal spores (i.e., Sporormiella sp., Podospora sp.) archived in lacustrine sediments provides a more specific and very useful indicator of the abundance of herds at the watershed scale (Etienne et al., 2013). The DNA analysis is fully complementary as it provides information about the livestock composition (Giguet-Covex et al., 2014). In the Aiguebelette sequence, the pollen curves of Plantago and Rumex reveal that grazing occurred throughout the record but they do not allow us to identify specific periods of increasing pastoralism, except for the last century (Figure 6). However, the curve of Sporormiella concentrations and accumulation rates, and mammal DNA from cattle and pigs match well and highlight phases of intensified livestock farming which are discussed below (Figure 9).
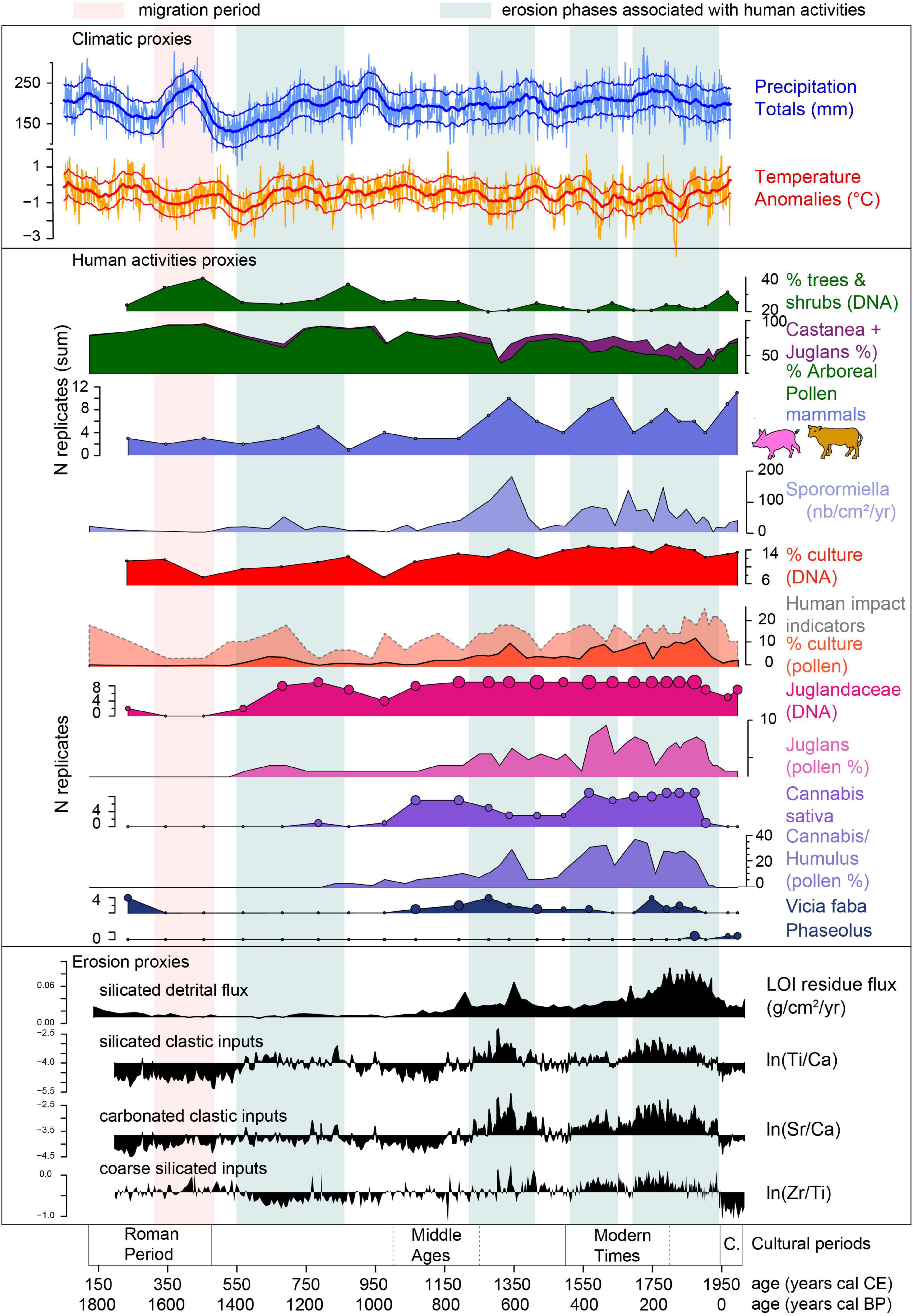
Figure 9. Synthesis of human and environmental histories recorded at Aiguebelette (this study) and regional climatic variations based on tree ring analysis (Büntgen et al., 2011).
Multi-Proxy and Multi-Record Approaches for a More Comprehensive History of Land-Use in Perialpine Areas
Pollen studies have been performed on two lake systems located only a few tens of kilometers away from Lake Aiguebelette within the same geographical and geomorphological context (Supplementary Figure 5): Lake Moras (43 km, Doyen et al., 2013) and Lake Paladru (20 km, Doyen et al., 2016). These three records (including Aiguebelette) display similar dynamics for the main tree taxa, as well as for the main agricultural and pastoral pollen indicators (Figure 10). These similarities can be explained by two main hypotheses: (i) the three lakes record the same regional pollen deposition due to overlapping RSAP (Relevant Source Area of Pollen) and their fairly large lake surface areas; (ii) the local environmental dynamics, agro-pastoral activities and socio-cultural histories were very similar within this small geographical region (i.e., they had similar trajectories).
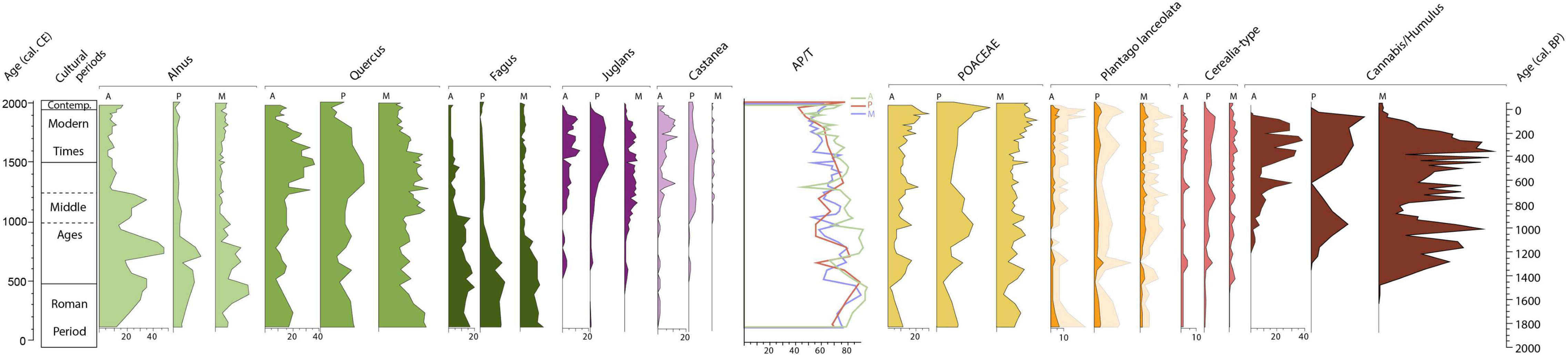
Figure 10. A compilation of key pollen taxa curves from 3 records: Aiguebelette (curve A), Paladru (curve P) and Moras (curve M). (Plantago lanceolata pollen curve is 4x exaggerated in light orange).
The aim of the following sections is to put into perspective the history of the vegetation and anthropogenic activities in this area based on the pollen records from lakes Paladru, Moras and Aiguebelette, and to potentially identify the local specificities of the Aiguebelette socio-ecosystem trajectory. In that context, analyzing DNA and coprophilous fungal spores, as well as sedimentary processes ongoing in the Aiguebelette watershed, is particularly relevant for deciphering the local history of the Aiguebelette basin. This combination of data will also help us to discuss the role of cultural and climatic forcing on the erosion dynamic in the watershed of Lake Aiguebelette.
The End of the Roman Period, Late Antiquity (150–500 cal CE)
The three pollen sequences record a decline in agriculture and an increase in tree cover (Figure 10). The DNA record from Aiguebelette reflects the same trend at the local scale and provides new data on what crops were declining in the area. Indeed, the DNA reveals the existence of orchards (apples or pears and plum or cherry trees) and the cultivation of broad beans (Vicia faba) and probably hops, during Antiquity, but all declined from the IInd century (Figure 9). Like the pollen records, the DNA data from Aiguebelette records a recovery of forests, probably due to a decrease in human impact. The rise in pioneer trees, such as Betula and Alnus, recorded by pollen, probably indicates the recolonization of abandoned pastures, attested to by the decline in Poaceae and taxa associated with pastures (Plantago, Rumex and Urtica in pollen and DNA records, Supplementary Figure 3). This phase of low human activity and forest recovery is accompanied by low detrital inputs as shown by low ln(Ti/Ca) and ln(Sr/Ca) and low silicated detrital flux (Figure 9). However, a small increase in ln(Zr/Ti), which represents a proxy of the silicated coarse detrital inputs, is recorded and may have been caused by the increase in precipitation recorded in Europe (Figure 9; Büntgen et al., 2011).
In the western Alps, the period of prosperity, characteristic of classical Antiquity (e.g., several sites have been identified to the south of Lake Aiguebelette, Nieloud-Muller, 2019), is followed by a phase of declining human impact and reforestation (Giguet-Covex et al., 2011, 2014; Doyen et al., 2013; Bajard et al., 2016). It is also well-recognized in other pollen records from the perialpine region (Clerc, 1988), as well as from other regions of the Alps. On the Swiss Plateau, pollen studies document a reduction in agricultural activities around the VIth century, accompanied by a short period of renaturation (Tinner et al., 1996, 2003). In Western Europe, this phase is a period of political turmoil, corresponding to the demise of the Roman Empire and the “Barbarian invasions,” also called the “Migration Period.” It is sometimes considered to be the greatest historical crisis to have occurred in central Europe. This socio-economic destabilization implied a reduced anthropogenic impact on the vegetation due to less farming activity and reduced tree harvesting (Rösch, 1992; McCormick, 2001). Additional hypotheses (other than socio-economic) have already been proposed to explain the decline in farming. Among these, the decrease in temperature and the rise of precipitation, from the mid-IIIrd century to the VIth century (Büntgen et al., 2011), could have significantly impacted agricultural production (Figure 10). An additional cause of the general degradation of production conditions might be the over-exploitation of farmland from the late Iron Age to the end of the Roman empire, which in turn would have generated the first major destabilization of the arable lands by erosion (Giguet-Covex et al., 2011, 2014; Doyen et al., 2013; Arnaud et al., 2016; Bajard et al., 2016).
Beginning of the Middle Ages (500–1000 cal CE)
In the three regional pollen records (Figure 10), the pollen values for grasses (and to a lesser extent for Plantago and Rumex) reflect an intensification of grazing. The local pastoral activity is confirmed at Aiguebelette by the DNA values of Plantago and Rumex, by the reappearance of coprophilous fungus spores (Sporormiella, Figure 9), and by a small increase in the detection of Bos taurus, all highlighting greater pastoral pressure in the watershed. This phase is also characterized by a similar decreasing pattern for Alnus pollen curves for the three regional records (a decrease from 500 to 650 cal CE, an increase from 650 to 800 cal CE and a main decrease from 800 to 950 cal CE) that could be interpreted as the result of human impact on wetlands and lake edges occupied by alder. It should be noted that the variations in Alnus are not easy to interpret because this tree grows in specific areas (swamps on waterlogged soils) directly connected to the lake. The very high quantity of Alnus DNA reads all along the sequence (higher values among all taxa, Supplementary Figure 2) reflects this specific situation. However, the DNA data records a slight decrease in Alnus reads from 800 to 1000 cal CE (Figure 8), probably confirming the decline of Alnus in the Aiguebelette watershed. In the pollen records, other trees such as Fagus and Quercus (Figure 6) are not affected by deforestation at this time, showing that it is possible that the humid lowlands previously occupied by Alnus may have been subjected to human pressure. The decline of swamps due to human impact has already been observed in the region (Clerc, 1988; Bernigaud, 2012). These areas could have been used for cultivation and pastures and probably to manage hay meadows, because of their waterlogged soils (Mazoyer and Roudart, 1997).
At the same time, the rise of both Cerealia and Secale reflects the development of cereal cultivation. A similar increase in cultivation has been observed in the Early Middle Ages on the Swiss Plateau (Tinner et al., 2003; Soepboer et al., 2010). The diversification of farming activities is attested by the DNA record which reveals the growing of fruit trees, such as apple and/or pear, plum and/or cherry and walnut trees, in the Aiguebelette area. Viticulture also developed during this phase. According to pollen records (Figure 6), it seems that walnut trees were cultivated in the region. The DNA data from Aiguebelette display significant values for Juglandaceae confirming local cultivation of walnut. The end of this phase (700–1000 cal CE) is characterized in all three pollen diagrams by an important re-extension of alder groves. This marked increase in Alnus could reflect the decline in human activities in the lowlands (Berger, 2010; Bernigaud, 2012), allowing the rise in Alnus.
Between 550 and 800 cal CE, i.e., during the maxima of landscape opening and farming activities, a first and low increase in erosion is evidenced principally by ln(Ti/Ca) (Figure 9). This phenomenon is also recorded at lakes Paladru (Simonneau et al., 2013; Doyen et al., 2016) and Annecy (Jones et al., 2013) and may have been slightly accentuated by a trend of increasing summer precipitation as reconstructed from tree rings in central Europe (see Figure 9, Büntgen et al., 2011).
From 1000 to 1350 cal CE, the End of the Middle Ages
From 1000 cal CE, the three regional pollen sequences show a diversification of farming practices, with the development of cereal and hemp growing (Figure 10). The DNA analysis at Aiguebelette also shows an important increase in Cannabis and Humulus, revealing that hemp was widely cultivated for textiles and was processed (retting) at Aiguebelette. DNA data show that hops were also abundant. The DNA from Humulus could come from natural hops growing in the surrounding wetlands, but its significant presence in the record could also reflect its cultivation for brewing. The production of beer during the Middle Age is already well known from historical archives (Poelmans and Swinnen, 2011).
The rise of Plantago, Rumex and Poaceae in pollen diagrams can be interpreted as the result of the expansion of pastoral activities. Both Plantago and Rumex, but mostly Urtica, are well-recorded by DNA analysis (Supplementary Figure 3). The Sporormiella accumulation rates (Figure 9) and the records of cow and pig DNA, which increase progressively up to a maximum value recorded at 1350 cal CE, confirm the importance of livestock farming activities at the end of this period in the Aiguebelette watershed.
The DNA record attests to the cultivation of various fruit trees, including walnuts, apples and/or pears, plums and/or cherries, grapes and chestnuts (Figure 8). Today grapes are not grown in the Aiguebelette area, but the historical existence of grapevines in Savoie is well-documented in written records relating to monastic and castle lands. These sources suggest an expansion of this activity before the XIIth century in some areas like the Combe de Savoie and the upper Arve Valley (Passy) (Soudan, 1978; Mouthon and Carrier, 2010). Grapevines were often planted alongside fruit trees, especially chestnuts, which acted as trellises or were planted with other trees to form hedges. Vitaceae DNA has also been detected in another nearby catchment, but at a higher altitude, between the XIth and XVIIth centuries (La Thuile in Bauges Massif; Bajard et al., 2017). The DNA record in Aiguebelette also shows the cultivation of fava beans (Figure 8). This result agrees with the medieval practice of legume growing (Colardelle and Verdel, 1993; Ruas, 1997; Ferault and Le Chatelier, 2012). The records for grape, hemp, cereals, hops, legumes and fruit trees reveal a transition toward a polyculture mode of farming in the Aiguebelette basin from the High Middle Ages onward.
At that time, all of these activities had an impact not only at the local scale (decrease in the DNA from trees and shrubs, Figure 9) but also affected regional forests. Indeed, the sum of tree pollen recorded in the three pollen sequences reflects an overall decrease in tree cover. At the regional scale, beech seems to have been considerably impacted by deforestation as early as 1050 cal CE (Figure 10). The abrupt decrease in the Alnus pollen curve, recorded between 1200 and 1300 cal CE, probably reflects other important forest clearances, but occurring in the lowlands and on the river banks.
From the Xth to the XIth century, the European population grew rapidly (Fossier, 1984), and this demographic explosion induced both an expansion and an intensification of farming activities (Mazoyer and Roudart, 1997; Ferault and Le Chatelier, 2012). The technical advances (plow, three-field rotation system, etc.) introduced to improve production (Leturcq, 2004; Ferault and Le Chatelier, 2012) caused growing disturbance to the environment (Rasmussen, 2005; Enters et al., 2008; Doyen et al., 2013, 2016). These farming activities had an impact on the forests around Aiguebelette, but the exploitation of trees for charcoal production (Crook et al., 2002; Py et al., 2012) and for the construction timber should also be considered. Another impact of the rising human activity is the increase in erosion, as evidenced first by the increase in silicated detrital flux (Figure 9), and then from 1200 cal CE, by the increase in fine silicated particles [ln(Ti/Ca)] as well as coarse particles [carbonated with ln(Sr/Ca) and sometimes silicate with ln(Zr/Ti)]. The contribution of coarse carbonated particles increases markedly for the first time in the record, showing new source(s) of eroded material and suggesting the exploitation of new areas (possibly on sandstone or molasses deposits Figure 1). The marked increase in erosion and the changes in erosion signatures occurred at a time when farming activity was highly developed, as shown by the mammal DNA and Sporormiella sp. records as well as the DNA and pollen indicators for cultivation. In the watershed of lakes Paladru and Annecy, new phases of soil erosion are also recorded (Jones et al., 2013; Simonneau et al., 2013; Doyen et al., 2016). Combined human activities (i.e., opening up of the landscape and agricultural developments) and climatic degradation, which included greater precipitation, undoubtedly contributed to this increase in erosion (Büntgen et al., 2011).
From 1350 to 1900 cal CE
In the regional pollen records, a short agro-pastoral decline occurs from the XIVth century (Figure 10). According to the pollen and DNA data, at Aiguebelette several cultivated plants (cereal, hemp, etc.) were less abundant from 1350 to 1500 cal CE. This period coincides with the recolonization of trees such as Corylus and Alnus and the main rising phase of oak, and also with a phase of decreased erosion as revealed by all geochemical indicators (Figure 10). The decrease in human impact at that time can be attributed to at least three main factors: (1) the effect of the second plague pandemic (Black Death); (2) the political instabilities affecting western Europe in the XIV-XVth centuries; (3) wetter and colder summers during the XIVth century, as attested by tree ring-based climate reconstructions for central Europe (Büntgen et al., 2011).
The next phase, spanning from 1500 to 1900 cal CE, is marked by renewed deforestation and a re-expansion of agro-pastoral activities in pollen diagrams (Figure 6), although it also corresponds to the Little Ice Age (Büntgen et al., 2011). The accumulation rates as well as the concentration of Sporormiella (Figure 9) indicate a constant high level of grazing throughout this period in the Aiguebelette watershed. As regards crop husbandry, cereals were still being grown in the watershed and Pollen and DNA attest to the introduction of buckwheat from around 1650 cal CE (Figures 6, 8). DNA results indicate an increase in fava bean and hemp growing. Both markers show that arboriculture reached its peak (Figures 8, 9). During the XIXth-XXth centuries, the Aiguebelette region was a region of apple production dedicated to fruit exportation (Dagenais, 1939). From 1500 cal CE, the arboreal pollen curves decrease continuously. The lowest values are recorded between the XVIIIth and XIXth centuries. Tree harvesting increased to meet the growing fuel needs of emerging industries.
In this phase, two periods of intense erosion are recorded by the geochemical tracers: from 1500 to 1650 cal CE and from 1700 to 1950 cal CE (Figure 9). These two phases may reflect a response to increased livestock farming, cultivation and summer precipitation (reconstructed from tree-rings in central Europe), especially between 1700 and 1800 cal CE (Büntgen et al., 2011).
From 1900 cal CE to the Present Day
From cal CE 1900 onward, all of the regional pollen records indicate a significant environmental change with an increase in total tree pollen, except for walnut which declines in regional pollen records (Figure 10). The proportion of tree taxa in the DNA records also increases (Figure 9). The number of positive DNA replicates of Juglans is also in decline (Figure 8), confirming the reduction of walnut cultivation in the Aiguebelette watershed. While nut production was progressively disappearing from the Aiguebelette watershed, DNA data show that orchards, composed of cherry or plum and apple trees, were still in activity at the beginning of the XXth century.
The drastic decline in Cannabis in both pollen and DNA records reflects the abandonment of hemp production in the area, a pattern that is also observed in the other pollen records (a bit earlier at Moras). The cultivation of cereals decreases slightly in the pollen records but to a lesser extent around Lake Aiguebelette than around Lake Paladru. Nowadays, the watershed of Aiguebelette is a rural terroir, still exploited, but cereal growing represents only a small portion of the agricultural activity (only 5.65% of the watershed area). The XXth century saw the appearance of new crops in the Aiguebelette area with DNA records revealing the development of vegetable gardens where Phaseolus, Cynara cardunculus, Apium graveolens, Pisum, and Beta vulgaris were grown.
The general decline in cultivation and reforestation observed at Aiguebelette are also recorded in many Alpine and perialpine lakes (Doyen et al., 2013, 2016; Bajard et al., 2016) through pollen and historical documents (Crook et al., 2002; Andrič et al., 2010). The decrease in erosion recorded at Aiguebelette (lowering of all erosion proxies from 1950, Figure 9) is a probable consequence of the lower human impact and of soil reforestation.
While cultivated plants seem to be less represented in regional pollen records, pastoralism indicators such as Rumex and Plantago are still well represented. At Aiguebelette, the mammal DNA (especially cattle) and Sporormiella spores demonstrate the continuation of grazing in the watershed after cal CE 1950. This persistence of grazing agrees with the high values in Poaceae recorded by pollen. This result probably illustrates the cattle rearing that is still a feature of the Aiguebelette area today, with most of the farms in the area being devoted to dairy farming for the production of milk for cheese.
Conclusion
This work highlights the excellent complementarity between the analysis of pollen grains, coprophilous fungal spores and environmental DNA and their value for reconstructing the history of vegetation and agropastoral practices. By combining these markers, it has been possible to reconstruct the pace, nature and sometimes the intensity of anthropization in the Lake Aiguebelette watershed over the last 1800 years.
The preliminary geochemical analysis carried out here to better understand the environmental impact of the agro-pastoral activities and climate reveals a pattern of erosion that fits very well with the dynamic of anthropization reconstructed using biotic proxies. A finer analysis of the potential sources of sediments (geochemical characterization of the watershed) would probably help to identify the different areas under human pressure throughout the record.
While the pollen seems to provide a vegetation history very similar to that recorded in two other regional lakes (Moras and Paladru), the results of the DNA analysis provide information on the agricultural activities carried out within the watershed. These results add greatly to our knowledge of the history of this terroir and encourage us to carry out the same type of analysis on the two other sites, to see how the exploitation of their terroirs differed (or not) from that of Aiguebelette.
Data Availability Statement
The datasets presented in this study can be found at: https://doi.org/10.6084/m9.figshare.19780297.v1. Any further queries should be directed to the corresponding author/s.
Author Contributions
ED, DE, and EM performed the spore and pollen analysis. CG-C and LG performed the DNA analysis. JD prepared the pollen samples. AJ managed the modern vegetation mapping. CG-C, A-LD, JP, PS, and FA performed the sedimentological and geochemical analysis. MB A-LD, CG-C, and PS managed the chronology. A-LD, CG-C, FA, and EM did the coring. All authors contributed to the article.
Conflict of Interest
The authors declare that the research was conducted in the absence of any commercial or financial relationships that could be construed as a potential conflict of interest.
Publisher’s Note
All claims expressed in this article are solely those of the authors and do not necessarily represent those of their affiliated organizations, or those of the publisher, the editors and the reviewers. Any product that may be evaluated in this article, or claim that may be made by its manufacturer, is not guaranteed or endorsed by the publisher.
Acknowledgments
We thank the CCLA (community of municipalities - Lake Aiguebelette) and the “Reserve Naturelle du Lac d’Aiguebelette” for coring authorizations and for their help. 14C dates were acquired with the help of Tomasz Goslar (Poznań Radiocarbon Laboratory). We express our gratitude to Rhoda Allanic for correcting the English text. We also thank Mathilde Fermond, a master’s degree student, for her help with DNA sampling and preparation.
Supplementary Material
The Supplementary Material for this article can be found online at: https://www.frontiersin.org/articles/10.3389/fevo.2022.866781/full#supplementary-material
Footnotes
- ^ dx.doi.org/10.17504/protocols.io.bdwsi7ee
- ^ https://git.metabarcoding.org/obitools/obitools/wiki/home
References
Ait Baamrane, M. A., Shehzad, W., Ouhammou, A., Abbad, A., Naimi, M., Coissac, E., et al. (2012). Assessment of the Food Habits of the Moroccan Dorcas Gazelle in M’Sabih Talaa, West Central Morocco, Using the trnL Approach. PLoS One 7:e35643. doi: 10.1371/journal.pone.0035643
Alsos, I. G., Lammers, Y., Yoccoz, N. G., Jørgensen, T., Sjögren, P., Gielly, L., et al. (2018). Plant DNA metabarcoding of lake sediments: how does it represent the contemporary vegetation. PLoS One 13:e0195403. doi: 10.1371/journal.pone.0195403
Alsos, I. G., Sjögren, P., Edwards, M. E., Landvik, J. Y., Gielly, L., Forwick, M., et al. (2016). Sedimentary ancient DNA from Lake Skartjørna, Svalbard: assessing the resilience of arctic flora to Holocene climate change. The Holocene 26, 627–642. doi: 10.1177/0959683615612563
Andrič, M., Martinčič, A., Štular, B., Petek, F., and Goslar, T. (2010). Land-use changes in the Alps (Slovenia) in the fifteenth, nineteenth and twentieth centuries AD: a comparative study of the pollen record and historical data. The Holocene 20, 1023–1037. doi: 10.1177/0959683610369506
Andrič, M., Sabatier, P., Rapuc, W., Ogrinc, N., Dolenec, M., Arnaud, F., et al. (2020). 6600 years of human and climate impacts on lake-catchment and vegetation in the Julian Alps (Lake Bohinj, Slovenia). Quatern. Sci. Rev. 227:106043. doi: 10.1016/j.quascirev.2019.106043
Appleby, P. G. (1991). 241Am dating of lake sediments. Hydrobiologia 214, 35–42. doi: 10.1007/978-94-011-3592-4_4
Arnaud, F., Poulenard, J. Giguet-Covex, C., Wilhelm, B. Révillon, S., Jenny, J. P., et al. (2016). Erosion under climate and human pressures: an alpine lake sediment perspective. Quat. Sci. Rev. 152, 1–18.
Bajard, M., Poulenard, J., Sabatier, P., Etienne, D., Ficetola, F., Chen, W., et al. (2017). Long-term changes in alpine pedogenetic processes: effect of millennial agro-pastoralism activities (French-Italian Alps). Geoderma 306, 217–236. doi: 10.1016/j.geoderma.2017.07.005
Bajard, M., Sabatier, P., David, F., Develle, A. L., Reyss, J. L., Fanget, B., et al. (2016). Erosion record in Lake La Thuile sediments (Prealps, France): evidence of montane landscape dynamics throughout the Holocene. The Holocene 26, 350–364.852. doi: 10.1177/0959683615609750
Behre, K. E. (1981). The interpretation of anthropogenic indicators in pollen diagrams. Poll. et Spor. 23, 225–245.
Berger, J.-F. (2010). Peuplement et Milieu en bas Dauphiné (Isle Crémieu) de l’apparition de l’agriculture à l’époque moderne. Rapport d’activité final de triennale du PCR « Peuplement et Milieu en bas-Dauphiné, Ile Crémieu, de l’apparition de l’agriculture à l’époque moderne »*. Lyon: SRA Rhône-Alpes.
Bernigaud, N. (2012). Les anthroposystèmes des marais de Bourgoin-la Verpillère (Isère) du Néolithique final à l’Antiquité tardive. Thèse, Université de Sophia Antipolis, Nice. The Holocene 23, 961–973.
Beug, H.-J. (2004). Leitfaden der Pollenbestimmung: Für Mitteleuropa und angrenzende Gebiete. München: Friedrich Pfeil.
Blaauw, M. (2010). Methods and code for “classical” age-modelling of radiocarbon sequences. Quater. Geochronol. 5, 512–518. doi: 10.1016/j.quageo.2010.01.002
Blum, S. A. E., Lorenz, M. G., and Wackernagel, W. (1997). Mechanism of Retarded DNA Degradation and Prokaryotic Origin of DNases in Nonsterile Soils. Syst. Appl. Microbiol. 20, 513–521. doi: 10.1016/S0723-2020(97)80021-5
Boessenkool, S., McGlynn, G., Epp, L. S., Taylor, D., Pimentel, M., Gizaw, A., et al. (2014). Use of Ancient Sedimentary DNA as a Novel Conservation Tool for High-Altitude Tropical Biodiversity: reconstructing Afro-Alpine Biodiversity. Conserv. Biol. 28, 446–455. doi: 10.1111/cobi.12195
Boyer, F., Mercier, C., Bonin, A., Le Bras, Y., Taberlet, P., and Coissac, E. (2016). OBITOOLS: aUNIX-inspired software package for DNAmetabarcoding. Mol. Ecol. Resour. 16, 176–182. doi: 10.1111/1755-0998.12428
Bremond, L., Favier, C., Ficetola, G. F., Tossou, M. G., Akouégninou, A., Gielly, L., et al. (2017). Five thousand years of tropical lake sediment DNA records from Benin. Quater. Sci. Rev. 170, 203–211. doi: 10.1016/j.quascirev.2017.06.025
Brown, A. G. Van Hardenbroek, M., Fonville, T., Davies, K., Mackay, H., Murray, E., et al. (2021). Ancient DNA, lipid biomarkers and palaeoecological evidence reveals construction and life on early medieval lake settlements. Sci. Rep. 11:11807.
Bruel, R., and Sabatier, P. (2020). Serac: aR package for ShortlivEd RAdionuclide Chronology of recent sediment cores. J. Environ. Radioact. 225:106449. doi: 10.1016/j.jenvrad.2020.106449
Brun, C. (2007). Archéophytes et Néophytes : pour Une Nouvelle Détermination Des Marqueurs Polliniques de l’anthropisation : le Cas Des Milieux cultivés et Rudéraux de Franche-Comté. Besançon: université de Franche-Comté. [PhD Thesis].
Büntgen, U., Tegel, W., Nicolussi, K., McCormick, M., Frank, D., Trouet, V., et al. (2011). 2500 years of European climate variability and human susceptibility. Science 331, 578–582. doi: 10.1126/science.1197175
Capo, E., Giguet-Covex, C., Rouillard, A., Nota, K., Heintzman, P. D., Vuillemin, A., et al. (2021). Lake Sedimentary DNA Research on Past Terrestrial and Aquatic Biodiversity: overview and Recommendations. Quaternary 4:6. doi: 10.3390/quat4010006
Clarke, C. L., Edwards, M. E., Brown, A. G., Gielly, L., Lammers, Y., Heintzman, P. D., et al. (2018). Holocene floristic diversity and richness in northeast Norway revealed by sedimentary ancient DNA (sed aDNA) and pollen. Boreas. Wiley 48, 299–316. doi: 10.1111/bor.12357
Clerc, J. (1988). Recherches pollenanalytiques sur la paléoécologie tardiglaciaire et holocène du Bas-Dauphiné. Marseille: Aix-Marseille University. [PhD Thesis].
Colardelle, M., and Verdel, E. (1993). Les Habitats du lac de Paladru (Isère) dans leur environnement : la formation d’un terroir au XIème siècle. Editions de la Maison des sciences et de l’homme, Paris.
Coutterand, S. (2010). Etude géomorphologique des flux glaciaires dans les Alpes nord-occidentales au Pléistocène récent : du maximum de la dernière glaciation aux premières étapes de la déglaciation. Chambéry: savoie University. [PhD Thesis].
Crook, D. S., Siddle, D. J., Dearing, J. A., and Thompson, R. (2002). Human Impact on the Environment in the Annecy Petit Lac Catchment, Haute-Savoie: a Documentary Approach. Environ. Hist. 10:284. doi: 10.3197/0967340041794312
Crump, S. E., Miller, G. H., Power, M., Sepúlveda, J., Dildar, N., Coghlan, M., et al. (2019). Arctic shrub colonization lagged peak postglacial warmth: molecular evidence in lake sediment from Arctic Canada. Glob. Chang. Biol. 25, 4244–4256. doi: 10.1111/gcb.14836
Cugny, C., Mazier, F., and Galop, D. (2010). Modern and fossil non-pollen palynomorphs from the Basque mountains (western Pyrenees, France): the use of coprophilous fungi to reconstruct pastoral activity. Veg. Hist. Archaeobot. 19, 391–408. doi: 10.1007/s00334-010-0242-6
Dagenais, P. (1939). Deuxième partie : l’homme et son śuvre. Revue de géographie alpine 27, 731–860.
David, F. (2010). An example of the consequences of human activities on the evolution of subalpine landscapes. Comptes. Rendus. Palevol. 9, 229–235. doi: 10.1016/j.crpv.2010.06.002
De Barba, M., Miquel, C., Boyer, F., Mercier, C., Rioux, D., Coissac, E., et al. (2014). DNA metabarcoding multiplexing and validation of data accuracy for diet assessment: application to omnivorous diet. Mol. Ecol. Res. 14, 306–323. doi: 10.1111/1755-0998.12188
de Beaulieu, J.-L., Kostenzer, J., and Reich, K. (1992). Dynamique forestière holocène dans la haute vallée de l’Arve (Haute-Savoie) et migrations de Abies et Picea dans les Alpes occidentales. Diss. Bot. 196, 387–398.
Dietre, B., Reitmaier, T., Walser, C., Warnk, T., Unkel, I., Hajdas, I., et al. (2020). Steady transformation of primeval forest into subalpine pasture during the Late Neolithic to Early Bronze Age (2300- 1700 BC) in the Silvretta Alps. Switzerland. The Holocene 30, 355–368. doi: 10.1177/0959683619887419
Doyen, E. (2012). 15 000 ans d’évolution des écosystèmes sous contrôle climatique et anthropique entre Jura et Bas Dauphiné : végétation, feu et érosion des sols. Besançon: université de Franche-Comté.
Doyen, E., Bégeot, C., Simonneau, A., Millet, L., Chapron, E., Arnaud, F., et al. (2016). Land use development and environmental responses since the Neolithic around Lake Paladru in the French Pre-alps. J. Archaeol. Sci.Rep. 7, 48–59. doi: 10.1016/j.jasrep.2016.03.040
Doyen, E., and Etienne, D. (2017). Ecological and human land-use indicator value of fungal spore morphotypes and assemblages. Veg. Hist. Archaeobot. 26, 357–367. doi: 10.1007/s00334-016-0599-2
Doyen, E., Vannière, B., Berger, J. F., Arnaud, F., Tachikawa, K., and Bard, E. (2013). Land-use changes and environmental dynamics in the upper Rhone valley since Neolithic times inferred from sediments in Lac Moras. The Holocene 23, 961–973. doi: 10.1177/0959683612475142
Ejarque, A., Miras, Y., and Riera, S. (2011). Pollen and non-pollen palynomorph indicators of vegetation and highland grazing activities obtained from modern surface and dung datasets in the eastern Pyrenees. Rev. Palaeobot. Palynol. 167, 123–139. doi: 10.1016/j.revpalbo.2011.08.001
Ellis, E. C., Fuller, D. Q., Kaplan, J. O., Lutters, W. G., and Blum, J. D. (2013). Dating the Anthropocene: towards an empirical global history of human transformation of the terrestrial biosphere. ElementaSci. Anthropoc. 1:000018.
Enters, D., Dörler, W., and Zolitschka, B. (2008). Historical soil erosion and land-use change during the last two millennia recorded in lake sediments of Frickenhauser See, northern Bavaria, central Germany. The Holocene 18, 243–254. doi: 10.1177/0959683607086762
Epp, L. S., Gussarova, G., Boessenkool, S., Olsen, J., Haile, J., Schrøder-Nielsen, A., et al. (2015). Lake sediment multi-taxon DNA from North Greenland records early post-glacial appearance of vascular plants and accurately tracks environmental changes. Quater. Sci. Rev. 117, 152–163. doi: 10.1016/j.quascirev.2015.03.027
Etienne, D., Destas, M., Lyautey, E., Marti, R., Ruffaldi, P., Georges-Leroy, M., et al. (2015). Two thousand–year reconstruction of livestock production intensity in France using sediment-archived fecal Bacteroidales and source-specific mitochondrial markers. The Holocene 25, 1384–1393. doi: 10.1177/0959683615585836
Etienne, D., and Jouffroy-Bapicot, I. (2014). Optimal counting limit for fungal spore abundance estimation using Sporormiella as a case study. Veg. Hist. Archaeobot. 23, 743–749. doi: 10.1007/s00334-014-0439-1
Etienne, D., Wilhelm, B., Sabatier, P., Reyss, J. L., and Arnaud, F. (2013). Influence of sample location and livestock numbers on Sporormiella concentrations and accumulation rates in surface sediments of Lake Allos. French Alps. J. Paleolimnol. 49, 117–127. doi: 10.1007/s10933-012-9646-x
Faegri, K., and Iversen, J. (1989). Textbook of pollen analysis, 4th edition. ed. Chichester: wiley & sons.
Ferault, C., and Le Chatelier, D. (2012). Une histoire des agricultures. France: campagne & compagnie.
Ficetola, G. F., Pansu, J., Bonin, A., Coissac, E., Giguet-Covex, C., De Barba, M., et al. (2015). Replication levels, false presences and the estimation of the presence/absence from eDNA metabarcoding data. Mol. Ecol. Resour. 15, 543–556. doi: 10.1111/1755-0998.12338
Ficetola, G. F., Poulenard, J., Sabatier, P., Messager, E., Gielly, L., Leloup, A., et al. (2018). DNA from lake sediments reveals long-term ecosystem changes after a biological invasion. Sci. Adv. 4:eaar4292. doi: 10.1126/sciadv.aar4292
Finsinger, W., and Tinner, W. (2007). Pollen and plant macrofossils at Lac de Fully (2135 m a.s.l.): holocene forest dynamics on a highland plateau in the Valais. Switzerland. The Holocene 17, 1119–1127. doi: 10.1177/0959683607082552
Giguet-Covex, C. (2010). Contribution des sédiments laminés lacustres à l’étude des changements Environnementaux Holocène Approche Couplée Sédimentologie/géochimie à Haute Résolution Application à Deux lacs Nord Alpins. Chambéry: université Savoie Mont Blanc.
Giguet-Covex, C., Arnaud, F., Poulenard, J., Disnar, J. R., Delhon, C., Francus, P., et al. (2011). Changes in erosion patterns during the Holocene in a currently treeless subalpine catchment inferred from lake sediment geochemistry (Lake Anterne, 2063 m a.s.l. NW French Alps): the role of climate and human activities. The Holocene 21, 651–665. doi: 10.1177/0959683610391320
Giguet-Covex, C., Ficetola, G. F., Walsh, K., Poulenard, J., Bajard, M., Fouinat, L., et al. (2019). New insights on lake sediment DNA from the catchment: importance of taphonomic and analytical issues on the record quality. Sci. Rep. 9:14676. doi: 10.1038/s41598-019-50339-1
Giguet-Covex, C., Pansu, J., Arnaud, F., Rey, P. J., Griggo, C., Gielly, L., et al. (2014). Long livestock farming history and human landscape shaping revealed by lake sediment DNA. Nat. Commun. 5:3211. doi: 10.1038/ncomms4211
Goldberg, E. D. (1963). “Geochronology with 210Pb,” in Proceedings of a Symposium of Radioactive Dating (Vienna: International Atomic Energy Agency), 121–130.
Graham, R. W., Belmecheri, S., Choy, K., Culleton, B. J., Davies, L. J., Froese, D., et al. (2016). Timing and causes of mid-Holocene mammoth extinction on St. Paul Island, Alaska. Proc. Nat. Acad. Sci. 113, 9310–9314. doi: 10.1073/pnas.1604903113
Grimm, E. C. (1987). CONISS: a FORTRAN 77 program for stratigraphically constrained cluster analysis by the method of incremental sum of squares. Comput. Geosci. 13, 13–35. doi: 10.1016/0098-3004(87)90022-7
Guiter, F., Andrieu-Ponel, V., de Beaulieu, J.-L., Ponel, P., Nicoud, G., and Blavoux, B. (2005). Impact anthropique sur la végétation de la région d’Évian depuis le Néolithique moyen : l’enregistrement pollinique de la Beunaz (971 m n.g.f. Haute-Savoie, France). Comptes Rendus Biol. 328, 661–673. doi: 10.1016/j.crvi.2005.03.002
Haas, J. N., Richoz, I., Tinner, W., and Wick, L. (1998). Synchronous Holocene climatic oscillations recorded on the Swiss Plateau and at timberline in the Alps. The Holocene 8, 301–309. doi: 10.1191/095968398675491173
Heiri, O., Lotter, A., and Lemcke, G. (2001). Loss on ignition as a method for estimating organic and carbonate content in sediments: reproducibility and comparability of results. J. Paleolimol. 25, 101–110.
Jacob, J., Disnar, J. R., Arnaud, F., Gauthier, E., Billaud, Y., Chapron, E., et al. (2009). Impacts of new agricultural practices on soil erosion during the Bronze Age in the French Prealps. The Holocene 19, 241–249. doi: 10.1177/0959683608100568
Jones, R. T., Reinhardt, L. J., Dearing, J. A., Crook, D., Chiverrell, R. C., Welsh, K. E., et al. (2013). Detecting climatic signals in an anthropogenically disturbed catchment: the late-Holocene record from the Petit Lac d’Annecy. French Alps. The Holocene 23, 1329–1339. doi: 10.1177/0959683613486940
Jørgensen, T., Haile, J., Möller, P. E. R., Andreev, A., Boessenkool, S., Rasmussen, M., et al. (2012). A comparative study of ancient sedimentary DNA, pollen and macrofossils from permafrost sediments of northern Siberia reveals long-term vegetational stability. Mol. Ecol. 21, 1989–2003. doi: 10.1111/j.1365-294X.2011.05287.x
Kanbar, H. J., Olajos, F., Englund, G., and Holmboe, M. (2020). Geochemical identification of potential DNA-hotspots and DNA-infrared fingerprints in lake sediments. Appl. Geochem. 11:104728. doi: 10.1016/j.apgeochem.2020.104728
Kaplan, J. O., Krumhardt, K. M., and Zimmermann, N. (2009). The prehistoric and preindustrial deforestation of Europe. Quater. Sci. Rev. 28, 3016–3034. doi: 10.1016/j.scitotenv.2006.08.013
Kruskal, J. B. (1964). Multidimensional scaling by optimizing goodness of fit to a nonmetric hypothesis. Psychometrika 29, 1–27. doi: 10.1007/BF02289565
Lammers, Y., Clarke, C. L., Erséus, C., Brown, A. G., Edwards, M. E., Gielly, L., et al. (2018). Clitellate worms (Annelida) in lateglacial and Holocene sedimentary DNA records from the Polar Urals and northern Norway. Boreas 48, 317–329. doi: 10.1111/bor.12363
Le Roy, M., Nicolussi, K., Deline, P., Astrade, L., Edouard, J. L., Miramont, C., et al. (2015). Calendar-dated glacier variations in the western European Alps during the Neoglacial: the Mer de Glace record. Mont Blanc massif. Quater. Sci. Rev. 108, 1–22. doi: 10.1016/j.quascirev.2014.10.033
Lefebvre, P., Sabatier, P., Mangeret, A., Gourgiotis, A., Le Pape, P., Develle, A. L., et al. (2021). Climate-driven uranium fluxes to lake sediments over the Holocene. Sci. Total Environ. 783:146878. doi: 10.1016/j.scitotenv.2021.146878
Leturcq, S. (2004). La vie rurale en France au Moyen Age - Xe- XVe siècle. Paris: editions Armand Colin.
Liu, S., Stoof-Leichsenring, K. R., Kruse, S., Pestryakova, L. A., and Herzschuh, U. (2020). Holocene Vegetation and Plant diversity changes in the North-Eastern Siberian treeline region from pollen and sedimentary ancient DNA. Front. Ecol. Evol. 8:560243. doi: 10.3389/fevo.2020.560243
Lotter, A. F. (1999). Late-glacial and Holocene vegetation history and dynamics as shown by pollen and plant macrofossil analyses in annually laminated sediments from Soppensee, central Switzerland. Veg. Hist. Archaeobot. 8, 165–184. doi: 10.1007/bf02342718
McCormick, M. (2001). Origins of the European Economy: communications and Commerce: a.D. Cambridge: cambridge Univ. Press, 300–900.
Miola, A. (2012). Tools for Non-Pollen Palynomorphs (NPPs) analysis: a list of Quaternary NPP types and reference literature in English language (1972–2011). Rev. Palaeobot. Palynol. 186, 142–161. doi: 10.1016/j.revpalbo.2012.06.010
Mottl, O., Flantua, S. G., Bhatta, K. P., Felde, V. A., Giesecke, T., Goring, S., et al. (2021). Global acceleration in rates of vegetation change over the past 18,000 years. Science 372, 860–864. doi: 10.1126/science.abg1685
Mouthon, F., and Carrier, N. (2010). Paysans des Alpes : les communautés montagnardes au Moyen Âge. France: pU Rennes.
Muller, S. D., Nakagawa, T., de Beaulieu, J. L., Court-Picon, M., Fauquette, S., and Genries, A. (2006). Paléostructures de végétation à la limite supérieure des forêts dans les Alpes françaises internes. Comptes Rendus Biol. 329, 502–511. doi: 10.1016/j.crvi.2006.05.002
Nieloud-Muller, S. (2019). Le lac d’Aiguebelette dans l’Antiquité. Les Dossiers du Musée Savoisien, Online journal. Available online at URL: https://patrimoines.savoie.fr/upload/docs/application/pdf/2020-05/rn_2019_lacaiguebelette_2020-05-18_18-21-59_837.pdf
Niemeyer, B., Epp, L. S. Stoof-Leichsenring, K. R., Pestryakova, L. A., and Herzschuh, U. (2017). A comparison of sedimentary DNA and pollen from lake sediments in recording vegetation composition at the Siberian treeline. Mol. Ecol. Res. 17, e46–e62.
Oksanen, J., Blanchet, F. G., Kindt, R., Legendre, P., Minchin, P. R., O’hara, R. B., et al. (2013). Package ‘vegan’. Commun. Ecol. Package Vers. 2, 1–295.
Pansu, J., Giguet-Covex, C., Ficetola, G. F., Gielly, L., Boyer, F., Zinger, L., et al. (2015). Reconstructing long-term human impacts on plant communities: an ecological approach based on lake sediment DNA. Mol. Ecol. 24, 1485–1498. doi: 10.1111/mec.13136
Parducci, L., Bennett, K. D., Ficetola, G. F., Alsos, I. G., Suyama, Y., Wood, J. R., et al. (2017). Ancient plant DNA in lake sediments. N. Phytol. 214, 924–942. doi: 10.1111/nph.14470
Parducci, L., Matetovici, I., Fontana, S. L., Bennett, K. D., Suyama, Y., Haile, J., et al. (2013). Molecular- and pollen-based vegetation analysis in lake sediments from central Scandinavia. Mol. Ecol. 22, 3511–3524. doi: 10.1111/mec.12298
Parducci, L., Suyama, Y., Lascoux, M., and Bennett, K. D. (2005). Ancient DNA from pollen: a genetic record of population history in Scots pine. Mol. Ecol. 14, 2873–2882.
Pedersen, M. W., Ginolhac, A., Orlando, L., Olsen, J., Andersen, K., Holm, J., et al. (2013). A comparative study of ancient environmental DNA to pollen and macrofossils from lake sediments reveals taxonomic overlap and additional plant taxa. Quat. Sci. Rev. 75, 161–168.
Pedersen, M. W., Ruter, A., Schweger, C., Friebe, H., Staff, R. A., Kjeldsen, K. K., et al. (2016). Postglacial viability and colonization in North America’s ice-free corridor. Nature 537, 45–49. doi: 10.1038/nature19085
Pini, R., Ravazzi, C., Raiteri, L., Guerreschi, A., Castellano, L., and Comolli, R. (2017). From pristine forests to high-altitude pastures: an ecological approach to prehistoric human impact on vegetation and landscapes in the western Italian Alps. J. Ecol. Gill J. 105, 1580–1597. doi: 10.1111/1365-2745.12767
Poelmans, E., and Swinnen, J. F. (2011). A brief economic history of beer. Oxford: oxford University Press.
Py, V., Ancel, B., and Durand, A. (2012). “La gestion de la forêt pour la mine et le charbonnage dans la Haute-Durance du Xe au XIIIe siècle,” in Colloque du Groupe d’Histoire des Forêts Françaises : « Forêt et montagne : évolution et aménagement », ed. A. Corvol (France: l’Harmattan), 53–75.
R Core Team (2018). R: A Language and Environment for Statistical Computing. Vienna: R Foundation for Statistical Computing.
Rapuc, W., Bouchez, J., Sabatier, P., Genuite, K., Poulenard, J., Gaillardet, J., et al. (2021). Quantitative evaluation of human and climate forcing on erosion in the alpine Critical Zone over the last 2000 years. Quatern. Sci. Rev. 268:107127. doi: 10.1016/j.quascirev.2021.107127
Rasmussen, P. (2005). Mid-to late-Holocene land-use change and lake development at Dallund S0, Denmark: vegetation and land-use history inferred from pollen data. The Holocene 15, 1116–1129. doi: 10.1191/0959683605hl884rp
Reille, M. (1992). Pollen et spores d’Europe et d’Afrique du Nord, Laboratoire de Botanique Historique et Palynologie U.R.A C.N.R.S 1152. France: marseille.
Reimer, P. J., Austin, W. E., Bard, E., Bayliss, A., Blackwell, P. G., Ramsey, C. B., et al. (2020). The IntCal20 northern hemisphere radiocarbon age calibration curve (0–55 cal kBP). Radiocarbon 62(4), 725–757.
Rey, F., Gobet, E., van Leeuwen, J. F., Gilli, A., van Raden, U. J., Hafner, A., et al. (2017). Vegetational and agricultural dynamics at Burgäschisee (Swiss Plateau) recorded for 18,700 years by multi-proxy evidence from partly varved sediments. Vegetat. Hist. Archaeobot. 26, 571–586. doi: 10.1007/s00334-017-0635-x
Reyss, J. L., Schmidt, S., Legeleux, F., and Bonté, P. (1995). Large, low background well-type detectors for measurements of environmental radioactivity. Nuclear Instruments Methods Phys. Res. Sec AacceleratorsSpectrom., Detect. a Assoc. Equipment 357, 391–397. doi: 10.1016/0168-9002(95)00021-6
Richter, T. O., Van der Gaast, S., Koster, B., Vaars, A., Gieles, R., de Stigter, H. C., et al. (2006). The Avaatech XRF Core Scanner: technical description and applications to NE Atlantic sediments. Geo. Soc. Lon. Special Publ. 267, 39–50. doi: 10.1144/gsl.sp.2006.267.01.03
Robbins, J. A., and Edgington, D. N. (1975). Determination of recent sedimentation rates in Lake Michigan using Pb-210 and Cs-137. Geochim. et Cosmochim. Acta 39, 285–304. doi: 10.1016/0016-7037(75)90198-2
Rösch, M. (1992). Human impact as registered in the pollen record: some results from the western Lake Constance region. South. Germ. Vegetat. Hist. Archaeobot. 1, 100–109.
Ruas, M. P. (1997). Les plantes consommées au Moyen Âge en France méridionale d’après les semences archéologiques. Archéologie du Midi médiéval 15, 179–204. doi: 10.3406/amime.1997.1323
Sabatier, P., Dezileau, L., Briqueu, L., Colin, C., and Siani, G. (2010). Clay minerals and geochemistry record from northwest Mediterranean coastal lagoon sequence: implications for paleostorm reconstruction. Sed. Geol. 228, 205–217.
Sabatier, P., Poulenard, J., Fanget, B., Reyss, J.-L., Develle, A.-L., Wilhelm, B., et al. (2014). Long-term relationships among pesticide applications, mobility, and soil erosion in a vineyard watershed. Proc. Nat. Acad. Sci. 111, 15647–15652. doi: 10.1073/pnas.1411512111
Sabatier, P., Wilhelm, B., Ficetola, G. F., Moiroux, F., Poulenard, J., Develle, A. L., et al. (2017). 6-kyr record of flood frequency and intensity in the western Mediterranean Alps – Interplay of solar and temperature forcing. Quatern. Sci. Rev. 170, 121–135. doi: 10.1016/j.quascirev.2017.06.019
Schmidt, R., Koinig, K. A., Thompson, R., and Kamenik, C. (2002). A multi proxy core study of the last 7000 years of climate and alpine land-use impacts on an Austrian mountain lake (Unterer Landschitzsee, Niedere Tauern). Palaeogeograph. Palaeoclimatol. Palaeoecol. 187, 101–120. doi: 10.1016/S0031-0182(02)00511-4
Schwörer, C., Colombaroli, D., Kaltenrieder, P., Rey, F., and Tinner, W. (2015). Early human impact (5000-3000 BC) affects mountain forest dynamics in the Alps. J. Ecol. Austin 103, 281–295. doi: 10.1111/1365-2745.12354
Simonneau, A., Doyen, E., Chapron, E., Millet, L., Vannière, B., Di Giovanni, C., et al. (2013). Holocene land-use evolution and associated soil erosion in the French Prealps inferred from Lake Paladru sediments and archaeological evidences. J. Archaeol. Sci. 40, 1636–1645. doi: 10.1016/j.jas.2012.12.002
Sjögren, P., Edwards, M. E., Gielly, L., Langdon, C. T., Croudace, I. W., Merkel, M. K. F., et al. (2017). Lake sedimentary DNA accurately records 20 th Century introductions of exotic conifers in Scotland. N. Phytol. 213, 929–941. doi: 10.1111/nph.14199
Soepboer, W., Sugita, S., and Lotter, A. F. (2010). Regional vegetation-cover changes on the Swiss Plateau during the past two millennia: a pollen-based reconstruction using the REVEALS model. Quatern. Sci. Rev. 29, 472–483. doi: 10.1016/j.quascirev.2009.09.027
Stockmaar, J. (1971). Tablets with Spores used in Absolute Pollen Analysis. Pollen et Spores 615–621.
Sugita, S. (2007a). Theory of quantitative reconstruction of vegetation I. Pollen from large lakes reveals regional vegetation. Holocene 17, 229–241.
Sugita, S. (2007b). Theory of quantitative reconstruction of vegetation II: all you need is LOVE. Holocene 17, 243—257.
Tabares, X., Zimmermann, H., Dietze, E., Ratzmann, G., Belz, L., Vieth-Hillebrand, A., et al. (2020). Vegetation state changes in the course of shrub encroachment in an African savanna since about 1850 CE and their potential drivers. Ecol. Evol. 10, 962–979. doi: 10.1002/ece3.5955
Taberlet, P., Coissac, E., Pompanon, F., Gielly, L., Miquel, C., Valentini, A., et al. (2007). Power and limitations of the chloroplast trnL (UAA) intron for plant DNA barcoding. Nucleic Acids Res. 35, e14–e14. doi: 10.1093/nar/gkl938
Taberlet, P., Prud’Homme, S. M., Campione, E., Roy, J., Miquel, C., Shehzad, W., et al. (2012). Soil sampling and isolation of extracellular DNA from large amount of starting material suitable for metabarcoding studies: extraction of extracellular DNA from Soil. Mol. Ecol. 21, 1816–1820. doi: 10.1111/j.1365-294X.2011.05317.x
Thöle, L., Schwörer, C., Colombaroli, D., Gobet, E., Kaltenrieder, P., van Leeuwen, J., et al. (2016). Reconstruction of Holocene vegetation dynamics at Lac de Bretaye, a high-mountain lake in the Swiss Alps. The Holocene 26, 380–396. doi: 10.1177/0959683615609746
Tinner, W., Ammann, B., and Germann, P. (1996). Treeline Fluctuations Recorder for 12,500 Years by Soil Profiles, Pollen, and Plant Macrofossils in the Central Swiss Alps. Arctic Alp. Res. 28, 131–147. doi: 10.2307/1551753
Tinner, W., Hubschmid, P., Wehrli, M., Ammann, B., and Conedera, M. (1999). Long-term forest fire ecology and dynamics in southern Switzerland. J. Ecol. 87, 273–289. doi: 10.1007/s10021-020-00587-4
Tinner, W., Lotter, A. F., Ammann, B., Conedera, M., Hubschmid, P., van Leeuwen, J. F. N., et al. (2003). Climatic change and contemporaneous land-use phases north and south of the Alps 2300 BC to 800 AD. Quater. Sci. Rev. 22, 1447–1460. doi: 10.1016/S0277-3791(03)00083-0
van Geel, B., and Aptroot, A. (2006). Fossil ascomycetes in Quaternary deposits. Nov. Hedwig. 82, 313–330. doi: 10.1127/0029-5035/2006/0082-0313
Walsh, K., Court-Picon, M., de Beaulieu, J. L., Guiter, F., Mocci, F., Richer, S., et al. (2014). A historical ecology of the Ecrins (Southern French Alps): archaeology and palaeoecology of the Mesolithic to the Medieval period. Quatern. Int. 353, 52–73. doi: 10.1016/j.quaint.2013.08.060
Weltje, G. J., Bloemsma, M. R., Tjallingii, R., Heslop, D., Röhl, U., and Croudace, I. W. (2015). “Prediction of geochemical composition from XRF core scanner data: a new multivariate approach including automatic selection of calibration samples and quantification of uncertainties,” in Micro-XRF studies of sediment cores, eds I. Croudace and R. Rothwell (Springer), 507–534. doi: 10.1007/978-94-017-9849-5_21
Weltje, G. J., and Tjallingii, R. (2008). Calibration of XRF core scanners for quantitative geochemical logging of sediment cores: theory and application. Ear. Planet. Sci. Lett. 274, 423–438. doi: 10.1016/j.epsl.2008.07.054
Wilhelm, B., Arnaud, F., Sabatier, P., Crouzet, C., Brisset, E., Chaumillon, E., et al. (2012). 1.4 kyrs of flash flood events in the Southern European Alps: implications for extreme precipitation patterns and forcing over the north western Mediterranean area. Quatern. Res. 78:1e12.
Keywords: land use history, vegetation history, Alps, last two millennia, pollen, erosion processes, sedaDNA
Citation: Messager E, Giguet-Covex C, Doyen E, Etienne D, Gielly L, Sabatier P, Banjan M, Develle A-L, Didier J, Poulenard J, Julien A and Arnaud F (2022) Two Millennia of Complexity and Variability in a Perialpine Socioecological System (Savoie, France): The Contribution of Palynology and sedaDNA Analysis. Front. Ecol. Evol. 10:866781. doi: 10.3389/fevo.2022.866781
Received: 31 January 2022; Accepted: 25 March 2022;
Published: 16 June 2022.
Edited by:
Triin Reitalu, Tallinn University of Technology, EstoniaReviewed by:
Christoph Schwörer, University of Bern, SwitzerlandKai Li, Zhejiang Normal University, China
Copyright © 2022 Messager, Giguet-Covex, Doyen, Etienne, Gielly, Sabatier, Banjan, Develle, Didier, Poulenard, Julien and Arnaud. This is an open-access article distributed under the terms of the Creative Commons Attribution License (CC BY). The use, distribution or reproduction in other forums is permitted, provided the original author(s) and the copyright owner(s) are credited and that the original publication in this journal is cited, in accordance with accepted academic practice. No use, distribution or reproduction is permitted which does not comply with these terms.
*Correspondence: Erwan Messager, ZXJ3YW4ubWVzc2FnZXJAdW5pdi1zbWIuZnI=