- 1Laboratory of Zoology, Research Institute for Biosciences, University of Mons, Mons, Belgium
- 2International Center for Agricultural Research in the Dry Areas, Rabat, Morocco
- 3The Steinhardt Museum of Natural History, Tel Aviv University, Tel Aviv, Israel
- 4Operational Directorate Taxonomy and Phylogeny, Royal Belgian Institute of Natural Sciences, Brussels, Belgium
Agroecosystems are often impoverished ecosystems, but they can host diverse communities of insects which provide ecosystem services. Specifically, crops may benefit from insect pollinators that increase their quantity and quality of yields. Basic knowledge is still needed regarding the identity, diversity, abundance, and ecology of insect pollinators in many parts of the world, especially in low and middle-income countries. In this study we investigate the potential of agroecosystems and crops in Morocco to host a high diversity of insect pollinators. We sampled insects in four eco-climatic regions encompassing a total of 22 crops for 2 years (2018–2019). After describing the general pattern of diversity and abundance of insect pollinators, we focused our comparative analyses on bees as they are known to be the most efficient and abundant group of insect pollinators. We recorded a total of 53,361 insect pollinators in all agroecosystems among which 37,091 were visiting crop flowers. Bees were by far the most abundant group visiting crops. Honeybees represented 49% of crop visitors followed by wild bees representing 33% of relative abundance. Three genera (Lasioglossum, Andrena, and Xylocopa) represented 53% of the total abundance of wild bees visiting crops. We identified a total of 213 species visiting crops (22% of national wild bee species richness). A comparison of the abundance, species richness, and community composition of wild bees visiting the same crops showed significant inter-regional differences for zucchini, faba bean, and eggplant. This study highlights the high diversity of pollinators in Moroccan agroecosystems and represents an important step toward exploring the Moroccan pollinator fauna. It provides basic information for future studies on pollinator conservation and pollination services.
Introduction
For centuries, farmers have worked to find ways to increase their yields and improve harvest quality. Several controlled factors affect crop production, such as fertilizer use (Yousaf et al., 2017), irrigation (Temesgen et al., 2018; Tura and Tolossa, 2020), and pesticide use (Zhang et al., 2015). In addition to these agricultural inputs, crops benefit from different ecosystem services that are provided “free of charge,” and which have complementary or synergistic effects on crop yield such as nutrient cycling, pest regulation, and pollination (Losey and Vaughan, 2006; Garibaldi et al., 2018). A recent study comparing the effect of reduction of fertilizer input, irrigation, and pollination, found that a reduction of insect pollination has a stronger effect on crop yield than other agricultural inputs (Fijen et al., 2020). Furthermore, insect pollination enhances the yield and the quality of many crops at both the local and global scale, such as for faba bean (Aouar-sadli et al., 2008; Cunningham and Le Feuvre, 2013), strawberries (Abrol et al., 2019; MacInnis and Forrest, 2019), apple (Garratt et al., 2014a; Hünicken et al., 2021), eggplant (Jayasinghe et al., 2017), cucumber (Christmann et al., 2017, 2022), and tomato (Bashir et al., 2018; Toni et al., 2020). Moreover, many countries are becoming increasingly dependent on pollinators because production has shifted to more pollinator-dependent crops (Aizen et al., 2008; Potts et al., 2016). Pollination is therefore a key ecosystem service to conserve for crop production in and of itself, and also for agricultural development. On the other hand, many pollinator groups are experiencing widespread population declines (Powney et al., 2019; Zattara and Aizen, 2021) in different parts of the world. Dicks et al. (2021) demonstrated that land cover and land management are the major drivers of pollinator declines in most Western countries, while in Africa, pesticides, in addition to land cover was an important factor. Therefore, there is both a simultaneous increase in the demand for pollinators in agricultural regions, whilst many pollinators are concurrently declining in these same areas. The combination of these two phenomena could have a strong effect on crop production and food security (Reilly et al., 2020).
Despite the importance of pollinators, farmers often show little interest in wild pollinators and have a poor understanding about the ecosystem service they provide, especially in developing countries (Ali et al., 2020; Tarakini et al., 2020; Christmann et al., 2021). This suggests an urgent need to study pollinators and their contribution to crop production in these countries to identify measures to protect and enhance pollinator habitats. Therefore, the first step forward is to acquire basic knowledge on pollinator diversity in agricultural systems and crop-pollinator relationships (Garratt et al., 2014b).
Located in the extreme northwest of Africa, Morocco is characterized by a high diversity of bioclimatic regions. The northern regions are influenced by Mediterranean and Atlantic climates (humid, sub-humid, and semi-arid) and the southern regions have an arid to desertic climate (Mokhtari et al., 2013). Morocco constitutes an important hotspot of biodiversity and shows a high rate of endemism when compared to Mediterranean countries and in North Africa (Rankou et al., 2013, 2015). In particular, Lhomme et al. (2020) recorded a total of 961 wild bee species which ranks Morocco as the fifth most species rich country for bee richness in the Mediterranean Basin, confirming previous estimates that Morocco comprises a hotspot of wild bee diversity and pollinators in general (Patiny and Michez, 2007; Patiny et al., 2009). However, few publications exist that focus on insect pollinators and their importance in Moroccan agroecosystems (Christmann et al., 2017, 2021; Sabbahi, 2021; Sentil et al., 2021).
This study aims to test these previous assumptions with quantitative evidence based on extensive pollinator surveys, which comprised four regions in Morocco, 22 local entomophilous crops, and during two consecutive years. Our specific aims were: (1) to document the baseline abundance and diversity of the main insect pollinator groups in agroecosystems, specifically those of wild bees visiting crops in Morocco; (2) to characterize the bee assemblages visiting each crop; and (3) to compare the inter-regional variation in bee abundance, species richness and community composition associated with different crops.
Materials and Methods
Geographical Framework, Sites, and Crop Selection
Insect surveys were conducted in four regions in Morocco as follows (Figure 1 and Supplementary Table 1). (1) The Casablanca-Settat (CS) region in the north-center of Morocco, a semi-arid region dominated by cereal and legume crops. (2) The Rabat-Sale-Kenitra region (RSK) near Maamora forest (25 km from Kenitra city), characterized by sub-humid climate and small farms producing vegetables and avocado. (3) The Fes-Meknes region (FM), a mountainous zone with semi-arid climate located at the foot of the Middle Atlas in Sefrou province. This latter area is unique in Morocco for having Rosaceae orchards such as apple, plum, and cherry in addition to other crops like vegetables and cereals. (4) The Draa-Tafilalet (DT) arid region, including sites located between 4 and 20 km from Errich city and 8 and 20 km from Errfoud. Thus, sites were located between mountainous and oasis areas and characterized by apple orchard, alfalfa, some vegetables, and date palm (Ministry of Agriculture, Fisheries, Rural Development, Water, and Forests, 2020).
We considered nine main crops: faba bean, pumpkin, zucchini, melon, apple, cherry, tomato, eggplant, and okra (Supplementary Table 1). For each main crop in each region, we selected eight fields of 300 m2 (30 m × 10 m) (apple and cherry orchards excepted, see below). Five of the eight fields were under the Farming with Alternative Pollinators management approach (FAP) (Christmann and Aw-Hassan, 2012; Christmann et al., 2017). Under the FAP management, the main crop was planted in the central 75% of the field area and other crops (Marketable Habitat Enhancement Plants, MHEP) were planted at the edge of the fields. The three remaining fields were monoculture of the main crop. For apple and cherry, four orchards per crop (i.e., two regular orchards and two orchards with MHEP) were selected. For each orchard, two rows with seven trees were marked. All crops were planted (or orchards were surveyed) in 2018 and replicated in 2019, except pumpkin and faba bean in some regions (Supplementary Table 1). Crop planting date followed local farmer’s schedule in each region. Most crops were planted in different regions at the same time except for zucchini. Following the farmers’ choice, this was planted in spring in DT and FM regions and in summer in RSK and CS regions.
Overall, we completed 37 trials with 22 crops (including main crops and MHEP, see Supplementary Table 1), in a total of 263 fields.
Surveys of Pollinator Communities
For each trial, three or four insect sampling sessions were conducted depending on the flowering period of the main crop. Two types of sampling methods were used in each session: (1). Transect walks, including observations, and sweep net (about 30–35 cm in diameter) and vacuum collection on flowers (Popic et al., 2013), and (2). Trapping with colored pan traps (white, blue, and yellow) (Westphal et al., 2008).
Four transects were completed in each sampling session as follows: two inside the main crop for 10 min (5 min each), a third in the outer 25% of the field (i.e., in main crop or MHEP, for 5 min in 2018 and 10 min in 2019) and a fourth in the flowering plants (wildflowers and other crops) in the field margin outside the crop (for 5 min). The insect survey in orchards (apple and cherry) consisted of walking alongside trees of the two plots during 10 min, and visits in MHEP were assessed within a 10 min transect. All insects visiting the crops’ flowers were collected or noted if they could not be collected. Pan trapping was done by using two sets of plastic bowls with three colors (blue, white, and yellow). Pan traps were filled with water and a drop of liquid soap. The three sets of pan traps were put in each field in each sampling event for 2 days (one set inside the field and the other outside the field). Floral visitors were identified visually to species level for the bee species Apis mellifera, Bombus terrestris, and Xylocopa pubescens, all which can be unambiguously determined in the field.
Specimens collected from transects and pan traps were prepared and labeled for the following insect groups: Hymenoptera, Aculeata (i.e., bees and wasps), Lepidoptera (i.e., butterflies), and Diptera, Syrphidae (i.e., hoverflies) (Ollerton et al., 2011). Individuals were identified in the laboratory to genus level for bees (Michez et al., 2019) then sent to expert taxonomists for identification to species level (see “Acknowledgments” section). The remaining groups were identified to the lowest taxonomic level possible in the laboratory (Borror and White, 1991).
Data Analysis
We explored the records from both FAP and control fields. We assume that MHEP did not affect the crop pollinator community in FAP fields based on previous research in the same sites. This study has demonstrated using visitation data and pollen load analysis that MHEPs affected neither the crop pollinator’s abundance and richness nor the crop pollinator’s pollen diet (Sentil et al., 2021, 2022).
Initially, we classified insects recorded in this study into five insect groups: wild bees, honeybees, non-bee Hymenoptera, Syrphidae, and butterflies. The mean and the standard error of the relative abundance of each insect group was calculated using (1) the data of all insects recorded in agroecosystems and (2) the data of insects recorded visiting studied crops (22 different crops). We used the relative abundance for each insect group because the sampling effort was not standardized between the different agroecosystems and crops.
For the following analyses, we focused on wild bees. Data from all fields, all sampling dates and both years were pooled to generate a species accumulation curve using the “vegan” package in R. A heatmap was constructed using the “pheatmap” package to visualize interaction between bee genera and crops using the relative abundance of each genus visiting each crop.
To compare regions, we performed the analysis for each of the main crops which were studied in more than one region (zucchini, pumpkin, faba bean, and eggplant). We used data from the two transects sampled inside the main crop and only three sampling events for each crop in each year to remain with coherent sampling effort across all crops and regions. Differences in bee species richness and abundance were analyzed using a generalized linear mixed model “glmer.nb” in the “lme4” R package (Bates et al., 2015). The data distribution used was a negative binomial and we fitted a random intercept model. We used “region” as fixed factor, “year” as crossed random factor and “sampling” as nested random factor within “field.” Then we performed a pairwise comparison between crops and regions using the “glht” function in the “multcomp” R package (Hothorn et al., 2008).
Bee community composition across different regions was analyzed by Non-metric Multidimensional Scaling (NMDS) employing the function “metaMDS” in the R package “vegan.” NMDS was followed by statistical analyses: PERMANOVA (Permutational Multivariate Analysis of variance) and SIMPER (Similarity Percentage Analysis) to identify those species that contributed the most to the observed differences between pollinator communities. Indicator species were also identified using Indicator Value with the “multipatt” function in the package “indicspecies” in R.
Results
Insect Pollinators in Moroccan Agroecosystems
A total of 53,361 insects were collected or observed in all agroecosystems surveyed in 2018 and 2019. We recorded a total of 37,317 individuals within crop fields by direct observation and hand netting, of them 3,831 individuals vising wildflowers and additional 12,213 by using pan traps (Supplementary Figure 1). Honeybees and wild bees were the most abundant groups with 37 ± 2% and 45 ± 2% (mean ± SE; N = 263 fields), respectively. Among the other groups, non-bee Hymenoptera represented 12 ± 1%, Syrphidae with 4 ± 0.5% and Lepidoptera with 2 ± 0.5% (Supplementary Table 2). Among the insects visiting target crops, we counted a total of 24,553 honeybees which represented a mean of 49 ± 2%, and 8,400 wild bees that consisted of 33 ± 2% of the mean bee abundance per field (Supplementary Table 2).
Species accumulation curve showed that the number of bee species visiting crops did not reach an asymptote. Basing on the extrapolated values, we sampled as many as 88% of the expected wild bee species richness (Figure 2A and Supplementary Table 3).
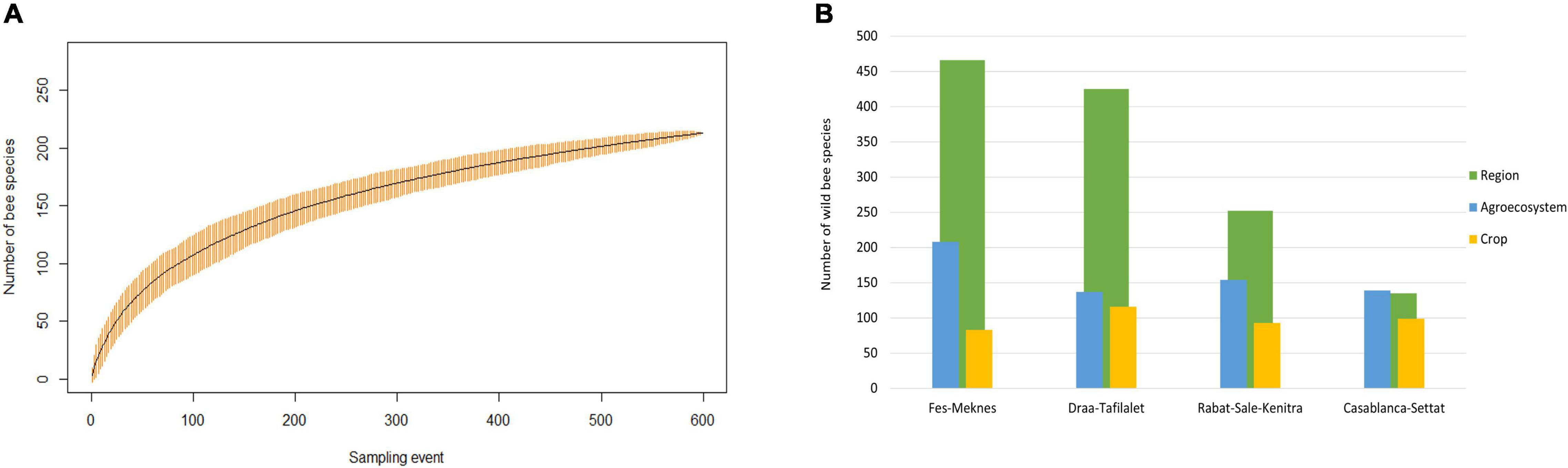
Figure 2. (A) Accumulated number of bee species visiting crops in Morocco. Curve was plotted based on data grouped across all sites and all sampling events. (B) Number of bee species known in each of the four studied region (Lhomme et al., 2020, green bars) compared to the number of bee species collected by different methods in all agroecosystems in each regions during 2018 and 2019 (blue bars) and the number of bee species visiting crops (22 crops; yellow bars).
A total of 213 species from 39 genera were identified as crop visitors (22% of the national richness) (Figure 2). The most abundant wild bee family visiting crops was the Halictidae (n = 3,940; 47% of the wild bees) followed by Apidae (n = 1,567; 19%), Andrenidae (n = 1,530; 18%), Colletidae (n = 432; 5%), and Megachilidae (n = 368; 4%). The most abundant genus was Lasioglossum (n = 2,046; 29%), followed by Andrena (n = 1,302; 19%), Xylocopa (n = 528; 8%), Amegilla (n = 450; 6%), Nomioides (n = 436; 6%), and Hylaeus (n = 391; 6%). Sixteen genera had an abundance less than 10 individuals and represented less than 1% of the total number of bee genera visiting crops. The most species-rich genus visiting crops was Andrena (53 species), followed by Lasioglossum (35 species), Anthophora (17 species), Hylaeus (16 species), and Eucera (12 species) (Supplementary Table 4).
Wild Bees Visiting Each Crop in Morocco
The heatmap revealed that most of the 22 crops were visited by dominant bee genera like Lasioglossum, Andrena, Xylocopa, and Amegilla, while some rare genera like Pseudapis, Lithurgus, and Melecta were visiting only one crop (Figure 3).
The family Fabaceae represented by faba bean, alfalfa, sweat pea, green bean, and grass pea, was visited mostly by the bee genera Anthophora, Amegilla, Eucera, Megachile, and Xylocopa, but also by some species of Andrena (such as A. abjecta, A. flavipes, and A. fulvicornis) and Lasioglossum. The Cucurbitaceae (zucchini, pumpkin, melon, armenian cucumber, and cucumber) was mostly visited by Lasioglossum, Ceratina, and X. pubescens. Crops of the family Solanaceae, including tomato and eggplant, had X. pubescens as the main visitor. However, we also collected bees from other genera visiting these crops like Amegilla, Halictus, and Lasioglossum but with a lower abundance. The Apiaceae (coriander and anise) and Brassicaceae (canola and arugula) attracted a high number of bees, these crops were visited mainly by Andrena, Hylaeus, and Lasioglossum. Okra (Malvaceae) was visited by a high abundance of Amegilla, Ceylalictus, Lasioglossum, and X. pubescens. In sunflower fields, the only crop which represented the Asteraceae family, we found that the most abundant species was X. pubescens, followed by the family Halictidae (genera Halictus, Lasioglossum, and Nomioides), but also with some bees of the genera Amegilla and Bombus. The family Lamiaceae represented by chia was frequented mostly by Ceratina cucurbitina, and two species of Osmia: O. caerulescens and O. tricornis. Finally, the wild bee species visiting apple flowers included two species of Andrena (A. flavipes, and A. propinqua), three species of Lasioglossum (L. lucidulum, L. malachurum, L. algericolellum), B. terrestris, Eucera nigrilabris, and Anthophora fulvitarsis, while cherry was mostly visited by Lasioglossum (L. algericolellum) and B. terrestris (Figure 3 and Supplementary Table 6).
Variation in Abundance, Species Richness and Community Composition of Wild Bees Visiting Crops Present in Different Regions
Accounting to how the abundance and species richness of wild bees visiting each crop differed between regions, the GLMM analysis revealed significant differences for zucchini, faba bean, and eggplant (all p < 0.05, Supplementary Table 7). Zucchini and faba bean in Draa-Tafilalet hosted the highest mean bee abundance (13.05 ± 3.2 individuals for zucchini and 10.08 ± 1.92 individuals for faba bean) and mean bee species richness (3.25 ± 0.54 for zucchini and 5.17 ± 0.65 for faba bean) followed by Fes-Meknes for zucchini (5.36 ± 1.03 individuals; 2.22 ± 0.31 species), and Casablanca-Settat for faba bean (4.49 ± 0.69 individuals, 2.20 ± 0.26 species).
For eggplant, the mean bee abundance and species richness were found to be higher in Rabat-Sale-Kenitra (1.23 ± 0.27 individuals: 0.65 ± 0.12 species) than in Casablanca-Settat region (0.36 ± 0.11 individuals, 0.31 ± 0.09 species) (Figure 4). However, no statistical differences were found between regions in the abundance and species richness of wild bees visiting pumpkin and apple fields (Supplementary Table 7).
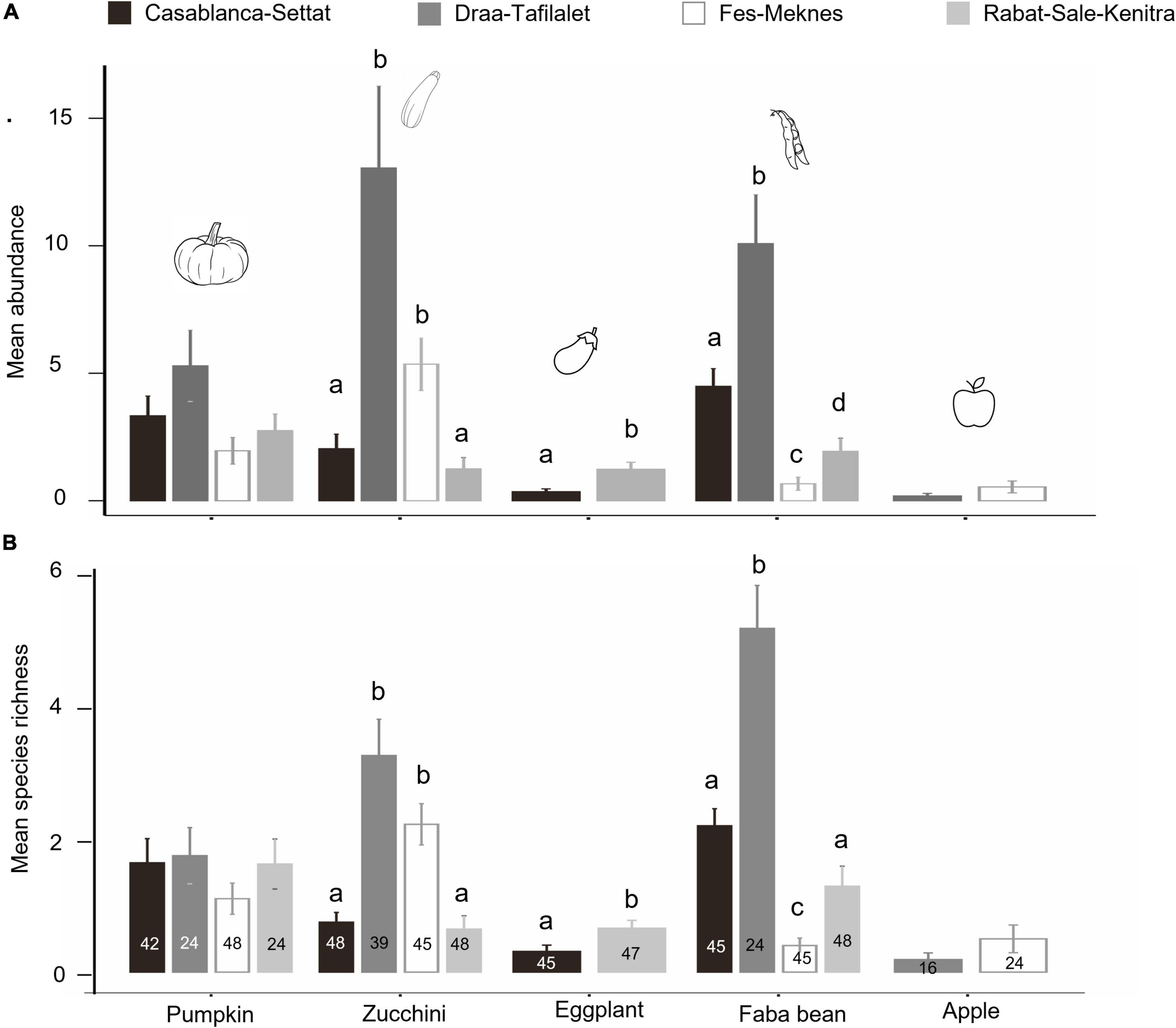
Figure 4. (A) Mean number of bee individuals visiting each crop in the areas where the crop was surveyed. (B) Mean number of bee species visiting each crop in the areas where the crop was surveyed. Means ± SE are shown. Small letters indicate significant differences between regions for each crop present in more than one region. Numbers indicate the number of observations (combination of year/field/sampling).
Wild bee composition differed between regions for all studied crops (PERMANOVA test: all p < 0.005). Based on pairwise analyses, we found differences in bee composition between all pairs of regions for each crop except between the regions Rabat-sale-Kenitra and Casablanca-Settat for wild bees visiting zucchini (p = 0.15), and between Rabat-Sale-Kenitra and Fes-Meknes for zucchini and pumpkin (p = 0.08 and p = 1, respectively) (Figure 5).
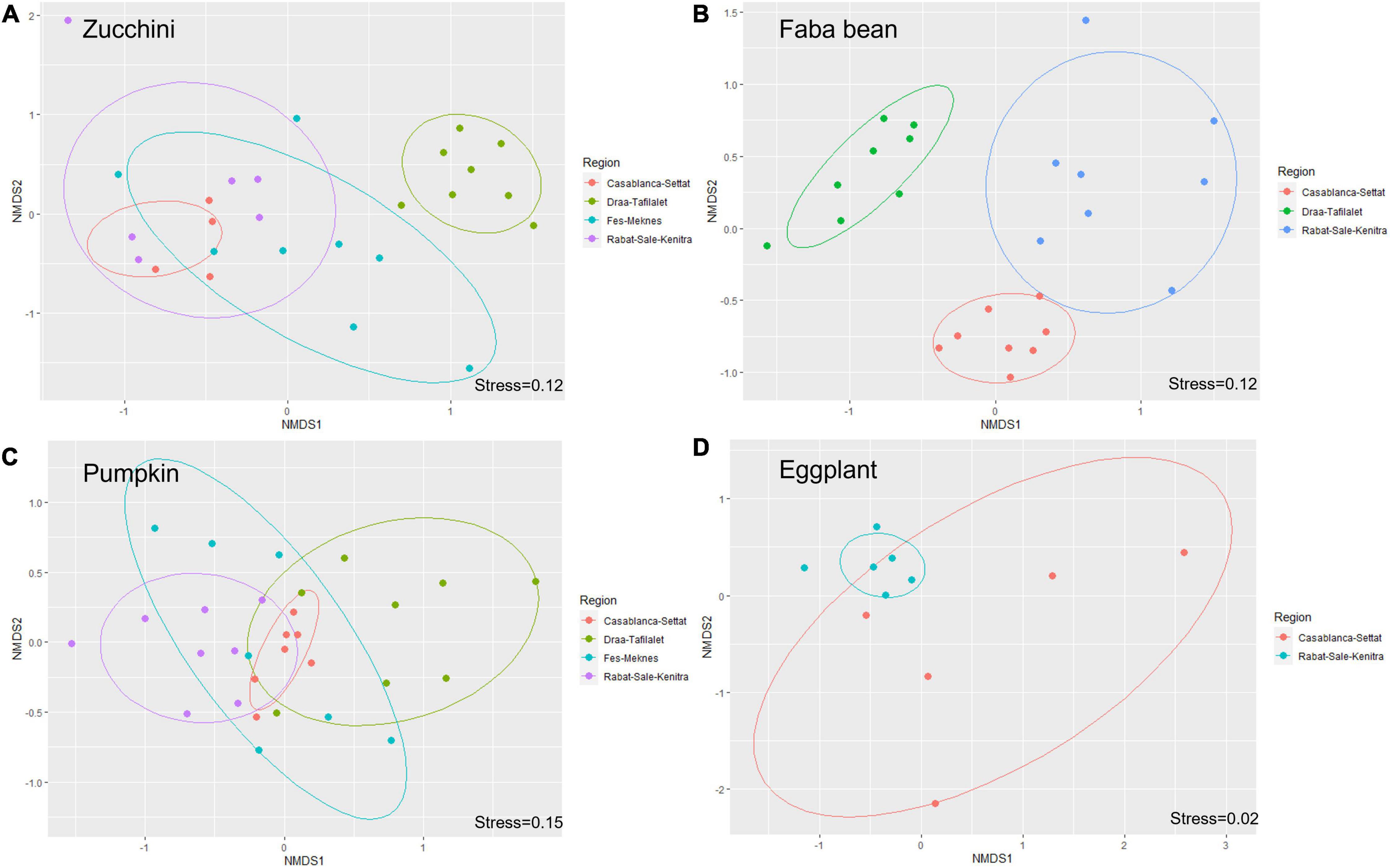
Figure 5. Non-metric multidimensional scaling (NMDS) ordination showing differences in bee community composition among regions for zucchini (A), faba bean (B), pumpkin (C), and eggplant (D).
SIMPER analysis showed that X. pubescens and halictid bees, especially Lasioglossum species, were the major contributors to the dissimilarity between regions for zucchini and pumpkin, whereas species of Eucera, Anthophora, Amegilla, and Xylocopa contributed to the compositional differences between regions for faba bean. Comparison between the two regions where eggplant was sampled revealed that only four species had a cumulative contribution of more than 70% to the dissimilarity index (X. pubescens with 53%, Anthophora sp. with 8%, Amegilla quadrifasciata with 7% and Halictus fulvipes with 5%) (Supplementary Table 8).
Based on the indicator species analysis, 14 bee species visiting zucchini were strongly associated with one or several regions. Seven species were associated with Draa-Tafilalet, two with Fes-Meknes and only one species was associated with Rabat-Sale-Kenitra. Four other species were found in more than one region: Lasioglossum villosulum, Lasioglossum leucozonium, and Lasioglossum interruptum in two regions and Lasioglossum malachurum in three regions (Tables 1, 2). Among the bee species visiting pumpkin, only four species were significantly associated with one region (Table 1) whereas Lasioglossum villosulum and Xylocopa pubescens were common and widespread species across regions (Table 2). For faba bean, six species were associated with Draa-Tafilaltet, two species were found to be indicators of the regions Rabat-Sale-Kenitra and Casablanca-Settat (Table 1) and only one species was associated with both Casablanca-Settat and Rabat-Sale-Kenitra (Table 2).
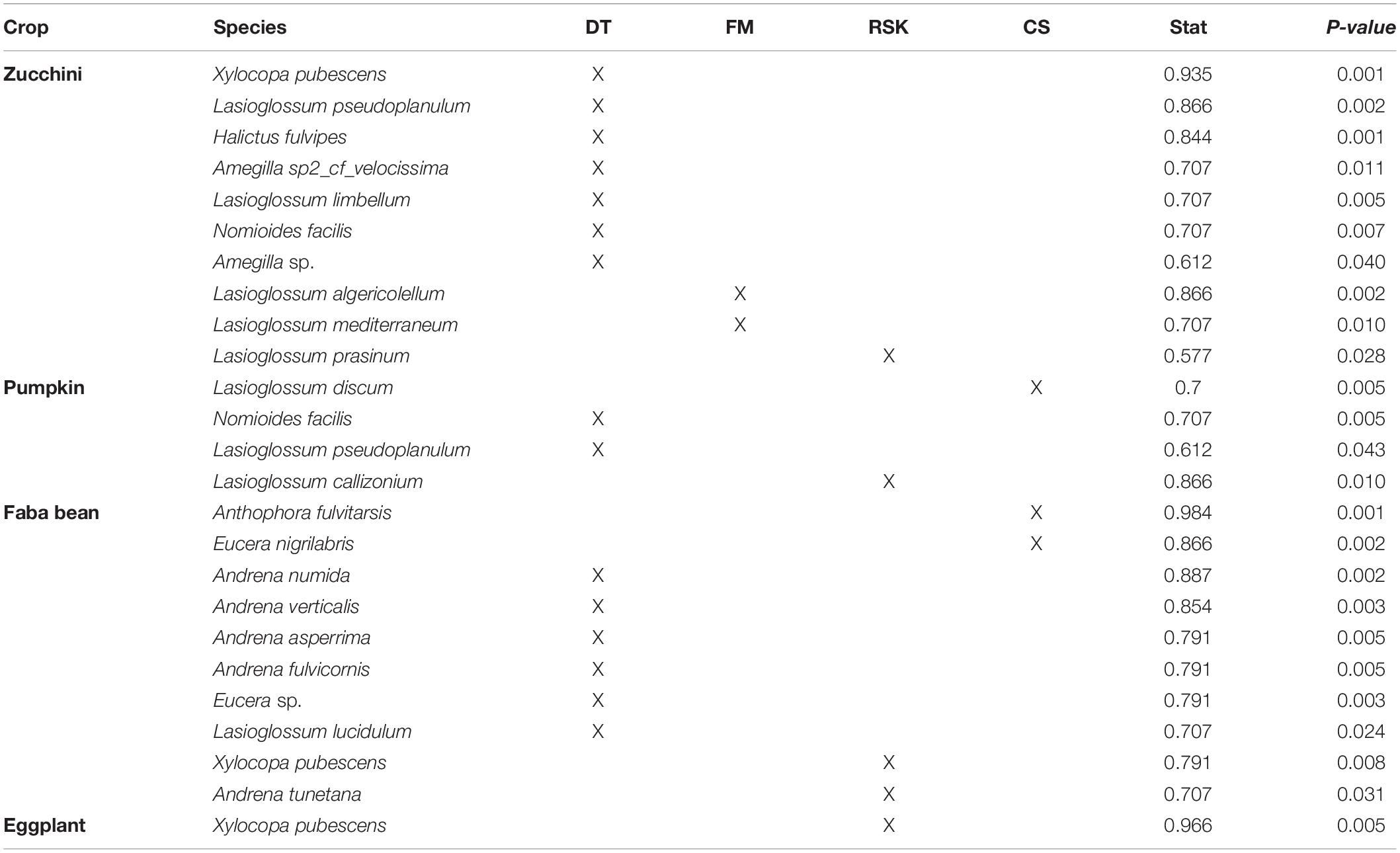
Table 1. Indicator bee species visiting crops significantly associated with only one region (DT, Draa-Tafilalet; FM, Fes-Mekenes; CS, Casablanca-Settat; RSK, Rabat-Sale-Kenitra).
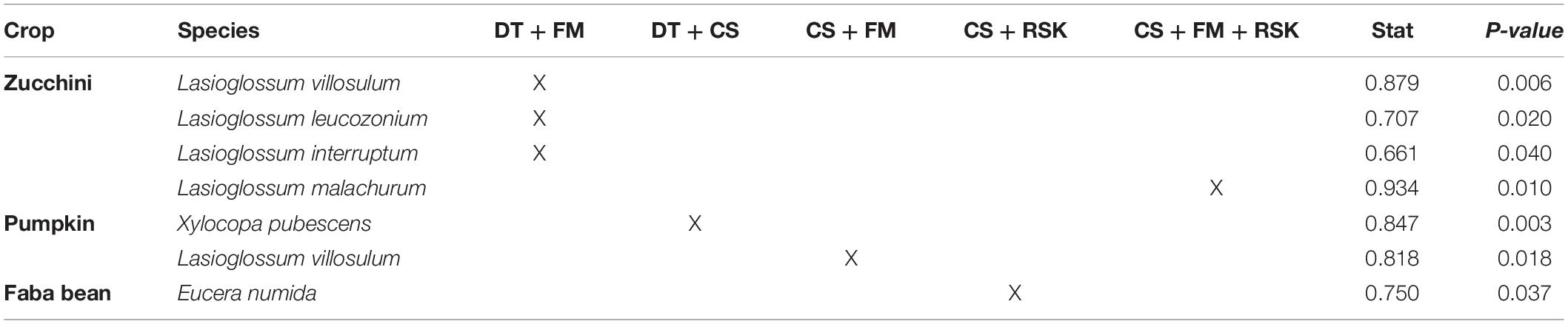
Table 2. Indicator bee species visiting crops significantly associated with more than one region (DT, Draa-Tafilalet; FM, Fes-Mekenes; CS, Casablanca-Settat; RSK, Rabat-Sale-Kenitra).
Discussion
Our study provides a first comprehensive and accurate estimation of the relative abundance and species richness of pollinators in agroecosystems in Morocco. It describes the relationship between wild bee species and crops from different plant families and across four ecoclimatic regions.
Wild Bees in Moroccan Agroecosystems
This study shows that agroecosystem in Morocco and particularly crop plants host high relative abundance of wild bee species, i.e., 45% in all agroecosystems, and 33% of all crop visitors, compared to that found in other countries. For example, in a study across five continents, wild bees accounted for 23% of crop visitors (Rader et al., 2016) and in the United States they accounted for 26% of the visitors (Reilly et al., 2020).
Furthermore, we recorded 213 wild bee species as crop visitors, i.e., 22% of national species richness. This number is high compared to the estimated global species richness of bees currently known to be crop visitors, 785 bee species, i.e., 4% of global species richness (Kleijn et al., 2015). The high species richness of wild bees found on crops further supports Morocco’s status as a hotspot of bee diversity (Michener, 1979; Lhomme et al., 2020).
Wild Bees Visiting Crops in Morocco
Our results are in accordance with many studies linking bee species and crops. We found that the most commonly represented genera in our study sites, like Lasioglossum, Andrena, and Xylocopa, were visiting multiple crops in agreement with the findings of Hutchinson et al. (2021).
Our results are also in line with studies showing a link between the floral traits of crops and their bee visitors (Garibaldi et al., 2015). We found that long tongued bees like Eucera, Anthophora, and Amegilla were more abundant at Fabaceae flowers that are characterized by flowers with a deep corolla tube (Aouar-sadli et al., 2008; Shebl and Farag, 2015; Marzinzig et al., 2018). Likewise, Solanaceae crops that typically require buzz pollination for fertilization were found to be visited mostly by X. pubescens and A. quadrifaciata, the two species which represent groups of efficient buzz pollinators (Gemmill-herren and Ochieng, 2008; Jayasinghe et al., 2017; Toni et al., 2020; Udayakumar et al., 2021).
Unlike other crops, we found a relatively small number of species and individuals visiting apple and cherry orchards. This could be explained by our sampling of these crops during cold and rainy weather conditions, when only a few species that are more tolerant to suboptimal conditions were active (including the genera Anthophora, Bombus, Eucera, and Lasioglossum). A similar pattern of floral visitation was found along a climatic gradient from Spain to the Netherlands with a growing dominance of the genera Bombus and Lasioglossum (Weekers et al., 2022). The higher abundance of Anthophora and Eucera in our study sites is typical to countries with a warmer Mediterranean climate, such as Morocco and Spain (Patiny and Michez, 2007; Weekers et al., 2022). Particularly in cherry orchards, we recorded mostly bees of the genus Lasioglossum while Andrena were less frequent, contrary to other studies in Germany and Belgium where these bees were the most abundant (Holzschuh et al., 2012; Eeraerts et al., 2020). Additionally, as woody Rosaceae are not a common element of the native Moroccan flora, the Moroccan Andrena community lacks many of the Eurasian species typically associated with these flowering plants, potentially explaining their low abundance in our surveys.
Regional Variability of Bee Abundance, Species Richness and Community Composition
We found that bee abundance, species richness and bee community composition were significantly different between regions for several of the studied crops.
There are several possible factors that can account for this variability between regions. Firstly, the studied regions are different in terms of the landscape composition and crop diversity that surround the surveyed fields. Studies have repeatedly shown that closer proximity of crop fields to semi-natural areas has a positive impact on wild bee diversity via augmentation of nesting resources in depauperate agricultural landscape (Ricketts et al., 2008; Kennedy et al., 2013; Chatterjee et al., 2020; Geeraert et al., 2020). In addition, higher crop diversity in agroecosystems may increase bee density (Raderschall et al., 2021). Secondly, the variability of bee diversity and community composition might be related to the climatic variability among regions in our study (sub humid, semi-arid, and arid regions). Bee species richness was found to be higher in arid and warm regions compared to wet regions (Michener, 2007; Patiny et al., 2009). This finding is in line with our result in Draa-Tafilalet (an arid region) where bee abundance and species richness in faba bean and zucchini fields were higher compared to other regions. Finally, seasonal differences throughout the year (Osorio-canadas et al., 2016). Previous studies have shown differences in bee abundance and species richness between dry and rainy seasons (Oertli et al., 2005; Samnegård et al., 2015; Balfour et al., 2018). These results may explain the differences that we found for zucchini in Draa-Tafilalet and Fes-Meknes where the crop was surveyed in early summer and was associated with a higher number of bee species as compared to Casablanca-Settat and Rabat-Sale-Kenitra, the two sites which were sampled in autumn.
Overall, 26 species were identified as indicator species of the regional crop-visiting bee community. The identified indicator species are among the most abundant species in agroecosystems (Supplementary Table 5) and are widespread in Morocco (Lhomme et al., 2020). Identifying indicator species by region and by crop could form the basis for selecting conservation priority species (Bladt et al., 2008). We found for example that only Lasioglossum species (three species) are indicators in Rabat-Sale-Kenitra and Fes-Meknes for zucchini compared to Draa-Tafilalet, in which seven species from six genera were found: Lasioglossum, Xylocopa, Amegilla, Halictus, and Nomioides. This suggests that different conservation strategies may be required between regions given the identified differences in the agriculturally relevant bee faunas.
Conservation Implications and Future Recommendations
This study provides basic information about wild bees in Moroccan agroecosystems and shows the importance of these habitats in hosting a high diversity of wild bees. Identifying the bee species associated with each crop and their abundance in different regions could help to identify conservation strategies for wild bees in Moroccan agroecosystems. For example, Lasioglossum and Andrena were the most abundant and species rich bee genera recorded in this study. These ground nesting bees could be at risk from intensive agriculture because of deep tillage (Ullmann et al., 2016; Christmann, 2022), soil compaction by heavy machinery, accumulation of pesticides as well as the combined effects of these factors. Reducing machinery or adopting no tillage in farms could conserve ground nesting bees (Shuler et al., 2005). Assessment of pesticide impact and risk to solitary ground-nesting bees as demonstrated by Christmann (2022), may be particularly important for the protection of pollinators in developing countries with high pesticide use (Dicks et al., 2021). For faba bean, cherry and apple that bloom in early season, seeding adequate complementary flowering crops (Christmann and Aw-Hassan, 2012; Sentil et al., 2021), wildflowers strips (Garibaldi et al., 2011; Sutter et al., 2017; Muñoz et al., 2021) or hedgerows (Morandin and Kremen, 2013) may help to attract early flying bees like the genera Eucera, Anthophora, and Bombus and help to conserve these bees in the agricultural landscape.
This first comprehensive study on the bee fauna associated with crops in Morocco highlights the relatively few knowledge available and the urgent need for future research in this country including: (a) research on the value of pollination services (Blaauw and Isaacs, 2014; Christmann et al., 2017, 2021, 2022; Anougmar, 2021; Sabbahi, 2021), (b) applied research to enhance pollinator diversity, and (c) assessment of different global change drivers (i.e., climate change) and their influence on pollinator distribution and diversity (Scheper et al., 2013).
Data Availability Statement
The raw data supporting the conclusions of this article will be made available by the authors, without undue reservation.
Author Contributions
IE and DM developed the specific research questions of this article. IE, PL, AS, LH, OI, and YB collected field data. AD, AP, SP, TW, DM, and PR identified bee species. IE analyzed data with the help of SR. SC developed FAP, designed, and coordinated the entire project. IE wrote the manuscript. All authors contributed to revisions.
Funding
This work was part of an ICARDA project funded by the German Federal Ministry for the Environment, Nature Protection and Nuclear Safety (BMU) within the International Climate Initiative (IKI). It was also partly supported by the “Research Foundation of Flanders (FWO)” and the “Fonds de la Recherche Scientifique (FNRS) under EOS Project CLIPS (no. 3094785)”. The publication was supported by the Institute for bioscience, Belgium.
Conflict of Interest
The authors declare that the research was conducted in the absence of any commercial or financial relationships that could be construed as a potential conflict of interest.
Publisher’s Note
All claims expressed in this article are solely those of the authors and do not necessarily represent those of their affiliated organizations, or those of the publisher, the editors and the reviewers. Any product that may be evaluated in this article, or claim that may be made by its manufacturer, is not guaranteed or endorsed by the publisher.
Acknowledgments
We would like to thank Holger H. Dathe, Michael Terzo, Andreas Müller, and Michael Kuhlmann for bee identification, and Axel Ssymank for Syrphid identification. We also received much support from Moulay Chrif Smaili who contributed to farmers selection and worked on pest and natural enemies. We would also like to thank Dimitri Evrard for his support in data management and digitization.
Supplementary Material
The Supplementary Material for this article can be found online at: https://www.frontiersin.org/articles/10.3389/fevo.2022.866581/full#supplementary-material
References
Abrol, D. P., Gorka, A. K., Ansari, M. J., Al-Ghamdi, A., and Al-Kahtani, S. (2019). Impact of insect pollinators on yield and fruit quality of strawberry. Saudi J. Biol. Sci. 26, 524–530. doi: 10.1016/j.sjbs.2017.08.003
Aizen, M. A., Garibaldi, L. A., Cunningham, S. A., and Klein, A. M. (2008). Long-Term Global Trends in Crop Yield and Production Reveal No Current Pollination Shortage but Increasing Pollinator Dependency. Curr. Biol. 18, 1572–1575. doi: 10.1016/j.cub.2008.08.066
Ali, M., Sajjad, A., Farooqi, M. A., Bashir, M. A., Aslam, M. N., Nafees, M., et al. (2020). Assessing indigenous and local knowledge of farmers about pollination services in cucurbit agro-ecosystem of Punjab. Pakistan Saudi J. Biol. Sci. 27, 189–194. doi: 10.1016/j.sjbs.2019.07.001
Anougmar, S. (2021). Economics Of Pollination In Drylands: Farmers’ And Consumers’ Perspectives In A Middle-Income Country.
Aouar-sadli, M., Louadi, K., and Doumandji, S. (2008). Pollination of the broad bean (Vicia faba L.var. major) (Fabaceae) by wild bees and honey bees (Hymenoptera: apoidea) and its impact on the seed production in the Tizi-Ouzou area (Algeria). Afr. J. Agric. Res. 3, 266–272.
Balfour, N. J., Ollerton, J., Castellanos, M. C., and Ratnieks, F. L. W. (2018). British phenological records indicate high diversity and extinction rates among late-summer-flying pollinators. Biol. Conserv. 222, 278–283. doi: 10.1016/j.biocon.2018.04.028
Bashir, M. A., Alvi, A. M., Khan, K. A., Rehmani, M. I. A., Ansari, M. J., Atta, S., et al. (2018). Role of pollination in yield and physicochemical properties of tomatoes (Lycopersicon esculentum). Saudi J. Biol. Sci. 25, 1291–1297. doi: 10.1016/j.sjbs.2017.10.006
Bates, D., Mächler, M., Bolker, B. M., and Walker, S. C. (2015). Fitting linear mixed-effects models using lme4. J. Stat. Softw. 67, 1–48
Blaauw, B. R., and Isaacs, R. (2014). Flower plantings increase wild bee abundance and the pollination services provided to a pollination-dependent crop. J. Appl. Ecol. 51, 890–898. doi: 10.1111/1365-2664.12257
Bladt, J., Larsen, F. W., and Rahbek, C. (2008). Does taxonomic diversity in indicator groups influence their effectiveness in identifying priority areas for species conservation? Anim. Conserv. 11, 546–554. doi: 10.1111/j.1469-1795.2008.00211.x
Borror, D. J., and White, R. E. (1991). Les Insectes De L’amérique Du Nord (Au Nord Du Mexique). Canada: Broquet Inc.
Chatterjee, A., Chatterjee, S., Smith, B., Cresswell, J. E., and Basu, P. (2020). Predicted thresholds for natural vegetation cover to safeguard pollinator services in agricultural landscapes. Agric. Ecosyst. Environ. 290:106785. doi: 10.1016/j.agee.2019.106785
Christmann, S. (2022). Regard and protect ground-nesting pollinators as part of soil biodiversity. Ecol. Appl. 32:e2564. doi: 10.1002/eap.2564
Christmann, S., Aw-Hassan, A., Güler, Y., Sarisu, H. C., Bernard, M., Smaili, M. C., et al. (2022). Two enabling factors for farmer-driven pollinator protection in low- and middle-income countries. Int. J. Agric. Sustain. 20, 54–67. doi: 10.1080/14735903.2021.1916254
Christmann, S., Aw-Hassan, A., Rajabov, T., Khamraev, A. S., and Tsivelikas, A. (2017). Farming with alternative pollinators increases yields and incomes of cucumber and sour cherry. Agron. Sustain. Dev. 37:24. doi: 10.1007/s13593-017-0433-y
Christmann, S., and Aw-Hassan, A. A. (2012). Farming with alternative pollinators (FAP)— An overlooked win-win-strategy for climate change adaptation. Agric. Ecosyst. Environ. 161, 161–164. doi: 10.1016/j.agee.2012.07.030
Christmann, S., Bencharki, Y., Anougmar, S., Rasmont, P., Smaili, M. C., Tsivelikas, A., et al. (2021). Farming with Alternative Pollinators benefits pollinators, natural enemies, and yields, and offers transformative change to agriculture. Sci. Rep. 11, 1–11. doi: 10.1038/s41598-021-97695-5
Cunningham, S. A., and Le Feuvre, D. (2013). Significant yield benefits from honeybee pollination of faba bean (Vicia faba) assessed at field scale. F Crop. Res. 149, 269–275. doi: 10.1016/j.fcr.2013.05.019
Dicks, L. V., Breeze, T. D., Ngo, H. T., Senapathi, D., An, J., Aizen, M. A., et al. (2021). A global-scale expert assessment of drivers and risks associated with pollinator decline. Nat. Ecol. Evol. 5, 1453–1461. doi: 10.1038/s41559-021-01534-9
Eeraerts, M., Vanderhaegen, R., Smagghe, G., and Meeus, I. (2020). Pollination efficiency and foraging behaviour of honey bees and non-Apis bees to sweet cherry. Agric. For. Entomol. 22, 75–82. doi: 10.1111/afe.12363
Fijen, T. P. M., Scheper, J. A., Vogel, C., Ruijven, J. V., and Kleijn, D. (2020). Insect pollination is the weakest link in the production of a hybrid seed crop. Agric. Ecosyst. Environ. 290:106743. doi: 10.1016/j.agee.2019.106743
Garibaldi, L. A., Aizen, M. A., Klein, A. M., Cunningham, S. A., and Harder, L. D. (2011). Global growth and stability of agricultural yield decrease with pollinator dependence. Proc. Natl. Acad. Sci. U. S. A. 108, 5909–5914. doi: 10.1073/pnas.1012431108
Garibaldi, L. A., Andersson, G. K. S., Requier, F., Fijen, T. P. M., Hipólito, J., Kleijn, D., et al. (2018). Complementarity and synergisms among ecosystem services supporting crop yield. Glob. Food Sec. 17, 38–47. doi: 10.1016/j.gfs.2018.03.006
Garibaldi, L. A., Bartomeus, I., Bommarco, R., Klein, A. M., Cunningham, S. A., Aizen, M. A., et al. (2015). Trait matching of flower visitors and crops predicts fruit set better than trait diversity. J. Appl. Ecol. 52, 1436–1444. doi: 10.1111/1365-2664.12530
Garratt, M. P. D., Breeze, T. D., Jenner, N., Polce, C., Biesmeijer, J. C., and Potts, S. G. (2014a). Avoiding a bad apple: insect pollination enhances fruit quality and economic value. Agric. Ecosyst. Environ. 184, 34–40. doi: 10.1016/j.agee.2013.10.032
Garratt, M. P. D., Coston, D. J., Truslove, C. L., Lappage, M. G., Polce, C., Dean, R., et al. (2014b). The identity of crop pollinators helps target conservation for improved ecosystem services. Biol. Conserv. 169, 128–135. doi: 10.1016/j.biocon.2013.11.001
Geeraert, L., Aerts, R., Berecha, G., Daba, G., De Fruyt, N., D’hollander, J., et al. (2020). Effects of landscape composition on bee communities and coffee pollination in Coffea arabica production forests in southwestern Ethiopia. Agric. Ecosyst. Environ. 288, 106706–106717. doi: 10.1016/j.agee.2019.106706
Gemmill-herren, B., and Ochieng, A. O. (2008). Role of native bees and natural habitats in eggplant (Solanum melongena) pollination in Kenya. Agric. Ecosyst. Environ. 127, 31–36. doi: 10.1016/j.agee.2008.02.002
Holzschuh, A., Dudenhöffer, J. H., and Tscharntke, T. (2012). Landscapes with wild bee habitats enhance pollination, fruit set and yield of sweet cherry. Biol. Conserv. 153, 101–107. doi: 10.1016/j.biocon.2012.04.032
Hothorn, T., Bretz, F., and Westfall, P. (2008). Simultaneous inference in general parametric models. Biom. J. 50, 346–363. doi: 10.1002/bimj.200810425
Hünicken, P. L., Morales, C. L., Aizen, M. A., Anderson, G. K. S., García, N., and Garibaldi, L. A. (2021). Insect pollination enhances yield stability in two pollinator-dependent crops. Agric. Ecosyst. Environ. 320:107573. doi: 10.1016/j.agee.2021.107573
Hutchinson, L. A., Oliver, T. H., Breeze, T. D., Bailes, E. J., Brünjes, L., Campbell, A. J., et al. (2021). Using ecological and field survey data to establish a national list of the wild bee pollinators of crops. Agric. Ecosyst. Environ. 315:107447. doi: 10.1016/j.agee.2021.107447
Jayasinghe, U. J. M. S. R., Silva, T. H. S. E., and Karunaratne, W. A. I. P. (2017). Buzzing Wild Bee Visits Enhance Seed Set in Eggplant, Solanum melongena. Hindawi 2017, 1–7. doi: 10.1155/2017/4624062
Kennedy, C. M., Lonsdorf, E., Neel, M. C., Williams, N. M., Ricketts, T. H., Winfree, R., et al. (2013). A global quantitative synthesis of local and landscape effects on wild bee pollinators in agroecosystems. Ecol. Lett. 16, 584–599. doi: 10.1111/ele.12082
Kleijn, D., Winfree, R., Bartomeus, I., Carvalheiro, L. G., Henry, M., Isaacs, R., et al. (2015). Delivery of crop pollination services is an insufficient argument for wild pollinator conservation. Nat. Commun. 6, 1–8. doi: 10.1038/ncomms8414
Lhomme, P., Michez, D., Christmann, S., Scheuchl, E., El Abdouni, I., Hamroud, L., et al. (2020). The wild bees (Hymenoptera: apoidea) of Morocco. Zootaxa 4892:zootaxa.4892.1.1. doi: 10.11646/zootaxa.4892.1.1
Losey, J. E., and Vaughan, M. (2006). The Economic Value of Ecological Services Provided by Insects. Bioscience 56:311.
MacInnis, G., and Forrest, J. R. K. (2019). Pollination by wild bees yields larger strawberries than pollination by honey bees. J. Appl. Ecol. 56, 824–832. doi: 10.1111/1365-2664.13344
Marzinzig, B., Brünjes, L., Biagioni, S., Behling, H., Link, W., and Westphal, C. (2018). Bee pollinators of faba bean (Vicia faba L.) differ in their foraging behaviour and pollination efficiency. Agric. Ecosyst. Environ. 264, 24–33. doi: 10.1016/j.agee.2018.05.003
Michener, C. D. (1979). Biogeography of bees. Ann. Missouri Bot. Gard. 66, 277–347. doi: 10.1016/j.ympev.2008.07.005
Michez, D., Rasmont, P., Terzo, M., and Vereecken, N. J. (2019). Bees Of Europe. France: NAP Editions.
Ministry of Agriculture, Fisheries, Rural Development, Water, and Forests (2020). Consolidation et archivage des statistiques produites par le ministère (superficie, Production, Effectifs d’élevage, …). Warsaw: Ministry of Agriculture, Fisheries, Rural Development, Water, and Forests.
Mokhtari, N., Mrabet, R., Lebailly, P., and Bock, L. (2013). Spatialisation des bioclimats, de l’aridité et des étages de végétation du Maroc. Rev. Marocaine Des Sci. Agron. Vét. 2, 50–66.
Morandin, L. A., and Kremen, C. (2013). Hedgerow restoration promotes pollinator populations and exports native bees to adjacent fields. Ecol. Appl. 23, 829–839. doi: 10.1890/12-1051.1
Muñoz, A. E., Plantegenest, M., Amouroux, P., and Zaviezo, T. (2021). Native flower strips increase visitation by non-bee insects to avocado flowers and promote yield. Basic Appl. Ecol. 56, 369–378. doi: 10.1016/j.baae.2021.08.015
Oertli, S., Müller, A., and Dorn, S. (2005). Ecological and seasonal patterns in the diversity of a species-rich bee assemblage (Hymenoptera: apoidea): apiformes. Eur. J. Entomol. 102, 53–63. doi: 10.14411/eje.2005.008
Ollerton, J., Winfree, R., and Tarrant, S. (2011). How many flowering plants are pollinated by animals? Oikos 120, 321–326. doi: 10.1111/j.1600-0706.2010.18644.x
Osorio-canadas, S., Arnan, X., Torné-Noguera, A., Molowny, R., Bosch, J., and Gómez, J. M. (2016). Body size phenology in a regional bee fauna : a temporal extension of Bergmann’s rule. Ecol. Lett. 19, 1395–1402. doi: 10.1111/ele.12687
Patiny, S., and Michez, D. (2007). Biogeography of bees (Hymenoptera, Apoidea) in Sahara and the Arabian deserts. Insect Syst. Evol. 38, 19–34. doi: 10.1163/187631207788784012
Patiny, S., Michez, D., Kuhlmann, M., Pauly, A., and Barbier, Y. (2009). Factors limiting the species richness of bees in Saharan Africa. Bull. Entomol. Res. 99, 337–346. doi: 10.1017/S0007485308006433
Popic, T. J., Davila, Y. C., and Wardle, G. M. (2013). Evaluation of Common Methods for Sampling Invertebrate Pollinator Assemblages: net Sampling Out-Perform Pan Traps. PLoS One 8:e66665. doi: 10.1371/journal.pone.0066665
Potts, S. G., Imperatriz-Fonseca, V., Ngo, H. T., Aizen, M. A., Biesmeijer, J. C., Breeze, T. D., et al. (2016). Safeguarding pollinators and their values to human well-being. Nature 540, 220–229. doi: 10.1038/nature20588
Powney, G. D., Carvell, C., Edwards, M., Morris, R. K. A., Roy, H. E., Woodcock, B. A., et al. (2019). Widespread losses of pollinating insects in Britain. Nat. Commun. 10:1018. doi: 10.1038/s41467-019-08974-9
Rader, R., Bartomeus, I., Garibaldi, L. A., Garratt, M. P. D., Howlett, B. G., Winfree, R., et al. (2016). Non-bee insects are important contributors to global crop pollination. Proc. Natl. Acad. Sci. U. S. A. 113, 146–151. doi: 10.1073/pnas.1517092112
Raderschall, C. A., Bommarco, R., Lindström, S. A. M., and Lundin, O. (2021). Landscape crop diversity and semi-natural habitat affect crop pollinators, pollination benefit and yield. Agric. Ecosyst. Environ. 306:107189. doi: 10.1016/j.agee.2020.107189
Rankou, H., Culham, A., Jury, S. L., and Christenhusz, M. J. M. (2013). The endemic flora of Morocco. Phytotaxa 78, 1–69. doi: 10.11646/phytotaxa.78.1.1
Rankou, H., Culham, A., Sghir Taleb, M., Ouhammou, A., Martin, G., and Jury, S. L. (2015). Conservation assessments and red listing of the endemic moroccan flora (monocotyledons). Bot. J. Linn. Soc. 177, 504–575. doi: 10.1111/boj.12258
Reilly, J. R., Artz, D. R., Biddinger, D., Bobiwash, K., Boyle, N. K., Brittain, C., et al. (2020). Crop production in the USA is frequently limited by a lack of pollinators: pollination limitation in US crops. Proc. R. Soc. B Biol. Sci. 287, 2–9. doi: 10.1098/rspb.2020.0922rspb20200922
Ricketts, T. H., Regetz, J., Steffan-Dewenter, I., Cunningham, S. A., Kremen, C., Bogdanski, A., et al. (2008). Landscape effects on crop pollination services: are there general patterns? Ecol. Lett. 11, 499–515. doi: 10.1111/j.1461-0248.2008.01157.x
Sabbahi, R. (2021). Economic value of insect pollination of major crops in Morocco. Int. J. Trop. Insect Sci. 42, 1275–1284.
Samnegård, U., Hambäck, P. A., Eardley, C., Nemomissa, S., and Hylander, K. (2015). Turnover in bee species composition and functional trait distributions between seasons in a tropical agricultural landscape. Agric. Ecosyst. Environ. 211, 185–194. doi: 10.1016/j.agee.2015.06.010
Scheper, J., Holzschuh, A., Kuussaari, M., Potts, S. G., Rundlof, M., Smith, H. G., et al. (2013). Environmental factors driving the effectiveness of European agri-environmental measures in mitigating pollinator loss – a. Ecol. Lett. 16, 912–920. doi: 10.1111/ele.12128
Sentil, A., Lhomme, P., Michez, D., Reverté, S., Rasmont, P., and Christmann, S. (2021). “Farming with Alternative Pollinators” approach increases pollinator abundance and diversity in faba bean fields. J. Insect Conserv. 26, 401–414
Sentil, A., Wood, T. J., Lhomme, P., Hamroud, L., El Abdouni, I., Ihsane, O., et al. (2022). Impact of the “Farming With Alternative Pollinators” Approach on Crop Pollinator Pollen Diet. Front. Ecol. Evol. 10:824474. doi: 10.3389/fevo.2022.824474
Shebl, M. A., and Farag, M. (2015). Bee diversity (Hymenoptera: apoidea) visiting Broad Bean (Vicia faba L.) flowers in Egypt. Zool. Middle East 61, 256–263. doi: 10.1080/09397140.2015.1069245
Shuler, R. E., Roulston, T. H., and Farris, G. E. (2005). Farming practices influence wild pollinator populations on squash and pumpkin. J. Econ. Entomol. 98, 790–795. doi: 10.1603/0022-0493-98.3.790
Sutter, L., Jeanneret, P., Bartual, A. M., Bocci, G., and Albrecht, M. (2017). Enhancing plant diversity in agricultural landscapes promotes both rare bees and dominant crop-pollinating bees through complementary increase in key floral resources. J. Appl. Ecol. 54, 1856–1864. doi: 10.1111/1365-2664.12907
Tarakini, G., Chemura, A., and Musundire, R. (2020). Farmers’ Knowledge and Attitudes Toward Pollination and Bees in a Maize-Producing Region of Zimbabwe: implications for Pollinator Conservation. Trop. Conserv. Sci. 13, 1–13. doi: 10.1177/1940082920918534
Temesgen, T., Ayana, M., and Bedadi, B. (2018). Evaluating the Effects of Deficit Irrigation on Yield and Water Productivity of Furrow Irrigated Onion (Allium cepa L.) in Ambo, Western Ethiopia. Irrig. Drain. Syst. Eng 07, 1–6. doi: 10.4172/2168-9768.1000203
Toni, H. C., Djossa, B. A., Ayenan, M. A. T., and Teka, O. (2020). Tomato (Solanum lycopersicum) pollinators and their effect on fruit set and quality. J. Hortic. Sci. Biotechnol. 96, 1–13. doi: 10.1080/14620316.2020.1773937
Tura, L. E., and Tolossa, T. T. (2020). Effect of Irrigation Water Quality and Deficit Irrigation on Crop Yield and Water Use efficiency. Turk. J. Agric. Food Sci. Technol. 8, 1201–1210.
Udayakumar, A., Chaubey, B. K., and Timalapur, M. (2021). Amegilla violacea (Lepeletier, 1841) (Anthophorini : apidae) – A native bee, an effective pollinator of eggplant (Solanum melongena). J. Apic. Res. 0, 1–7. doi: 10.1080/00218839.2020.1862393
Ullmann, K. S., Meisnera, M. H., and Williams, N. M. (2016). Impact of tillage on the crop pollinating, ground nesting bee, Peponapis pruinosa in California. Agr. Ecosyst. Environ. 232, 240–246.
Weekers, T., Marshall, L., Leclercq, N., Wood, T. J., Cejas, D., Drepper, B., et al. (2022). Dominance of honey bees is negatively associated with wild bee diversity in commercial apple orchards regardless of management practices. Agric. Ecosyst. Environ. 323:107697. doi: 10.1016/j.agee.2021.107697
Westphal, C., Bommarco, R., Carré, G., Lamborn, E., Morison, N., Petanidou, T., et al. (2008). Measuring bee diversity in different European habitats and biogeographical regions. Ecol. Monogr. 78, 653–671. doi: 10.1890/07-1292.1
Yousaf, M., Li, J., Lu, J., Ren, T., Cong, R., Fahad, S., et al. (2017). Effects of fertilization on crop production and nutrient-supplying capacity under rice-oilseed rape rotation system. Sci. Rep. 7, 1–9. doi: 10.1038/s41598-017-01412-0
Zattara, E. E., and Aizen, M. A. (2021). Worldwide Occurrence Records Reflect a Global Decline in Bee Species Richness. One Earth 4, 114–123. doi: 10.1101/869784
Keywords: honeybees, wild bees, Andrena, Lasioglossum, Xylocopa, pollination services
Citation: El Abdouni I, Lhomme P, Christmann S, Dorchin A, Sentil A, Pauly A, Hamroud L, Ihsane O, Reverté S, Patiny S, Wood TJ, Bencharki Y, Rasmont P and Michez D (2022) Diversity and Relative Abundance of Insect Pollinators in Moroccan Agroecosystems. Front. Ecol. Evol. 10:866581. doi: 10.3389/fevo.2022.866581
Received: 31 January 2022; Accepted: 26 May 2022;
Published: 11 July 2022.
Edited by:
Rory Mc Donnell, Oregon State University, United StatesReviewed by:
Alistair John Campbell, Embrapa Amazônia Oriental, BrazilGabor Pozsgai, University of the Azores, Portugal
Copyright © 2022 El Abdouni, Lhomme, Christmann, Dorchin, Sentil, Pauly, Hamroud, Ihsane, Reverté, Patiny, Wood, Bencharki, Rasmont and Michez. This is an open-access article distributed under the terms of the Creative Commons Attribution License (CC BY). The use, distribution or reproduction in other forums is permitted, provided the original author(s) and the copyright owner(s) are credited and that the original publication in this journal is cited, in accordance with accepted academic practice. No use, distribution or reproduction is permitted which does not comply with these terms.
*Correspondence: Insafe El Abdouni, aW5zYWZlLmVsYWJkb3VuaUBzdHVkZW50LnVtb25zLmFjLmJl