- 1Department of Migration, Max Planck Institute of Animal Behavior, Radolfzell, Germany
- 2Department of Biology, University of Konstanz, Konstanz, Germany
- 3Institute of Biological Problems of the North, Magadan, Russia
- 4Institute of Plant and Animal Ecology, Ural Branch of the Russian Academy of Sciences, Yekaterinburg, Russia
Migratory species have a limited time for habitat selection upon arrival at the breeding grounds. This is especially evident in arctic migrants, which are restricted by a narrow window of opportunity when environmental conditions are favorable for breeding. This general time constraint is amplified in rough-legged buzzards (Buteo lagopus) who, as many other arctic predators, rely on rodent (lemming) cycles during the breeding season, a 3–5 year period of waxing and waning local food abundance. It remains unclear how arctic predators, especially migrants, can find nesting areas where rodents are numerous when their selection time is so limited. We hypothesized that rough-legged buzzards select nesting areas during the previous breeding season. We tracked 43 rough-legged buzzards using GPS telemetry and assessed their movements post-breeding prospecting behavior to test our hypothesis. Here we show that rough-legged buzzards search for a nesting location during the previous breeding season in a post-breeding period. In the following year, individuals return to and attempt to breed in the area they inspected the year before. Rough-legged buzzards, regardless of breeding success, remained in the Arctic all breeding season until the end of September. Failed breeders prospected more than successful ones. At the same time, buzzards that bred in the rodent-free ecosystem prospected less and showed a high level of philopatry. Therefore, as rodent cycles have been predicted to collapse in the warming Arctic, we can expect arctic predators to change their movement patterns in the future with serious potential consequences for their conservation. We anticipate our study provides a step forward toward understanding movement and settlement decisions in animals experiencing high inter-annual environmental variation.
1. Introduction
The decision of animals to inhabit a specific area is of significant importance for their reproductive success and survival (Montgomery and Roloff, 2013). Particularly so in migratory species that travel large distances and thus have very little time after their arrival to the breeding grounds to choose an appropriate location for reproduction. This lack of time is especially evident in arctic migrants, which are particularly limited by a short breeding season (Bêty et al., 2004; Madsen et al., 2007; Lamarre et al., 2017). Another limitation for migratory arctic species is the timing of migration. Spring migration and the options for arrival to the breeding area are limited in time by the photoperiod (Pokrovsky et al., 2021) and extreme environmental conditions on the breeding grounds, such are low temperature, scarcity of food, and snow cover (Curk et al., 2020). Another key aspect to consider in the Arctic is the high inter-annual variability in environmental conditions (including food resources) during the reproductive season. A core component of life in the tundra habitat is the rodent cycle representing an abundant resource for numerous predators such as the stoat (Mustela erminea), arctic fox (Vulpes lagopus), long-tailed skua (Stercorarius longicaudus), snowy owl (Bubo scandiacus), and rough-legged buzzard (Buteo lagopus) every 3–5 years (Gilg et al., 2003, 2006; Sundell et al., 2004; Tast et al., 2010). These species have evolved to efficiently predict and track spatial variations in this variable resource (Sundell et al., 2004; Ims and Fuglei, 2005; Gilg et al., 2009; Schmidt et al., 2012).
The rodent cycle in the Arctic is defined by a period where lemming and vole abundance rises for a few years, reaches a peak and afterwards crashes (Oksanen and Oksanen, 1992; Angerbjörn et al., 2001). The rodent cycle, which appears as a pulsed resource, can be at the peak in one area while it might be at its lower point in another within the same season (Predavec et al., 2001; Krebs et al., 2002). While resident predator species staying year-round in the Arctic can track this pulsed and spatially heterogeneous resource (Tarroux et al., 2010; Therrien et al., 2014), it remains unclear how migratory species that spend only a limited time in the Arctic find the areas with rodents' peak during the concise settlement decision process. Moreover, due to climate warming, rodent cycles now appear to collapse and flatten in many Arctic regions (Kausrud et al., 2008; Gilg et al., 2009; Ims et al., 2011; Ehrich et al., 2020). Therefore, it raizes a question of how these changes in rodent cycles will affect habitat selection by Arctic predators.
To tackle these questions, we used a migratory arctic breeder, the rough-legged buzzard as a model species. Rough-legged buzzard specializes in small rodents during the breeding season. However, it can breed in areas with no rodents and shift to alternative prey (Ferguson-Lees and Christie, 2001; Bechard and Swem, 2002; Pokrovsky et al., 2014, 2015). Yet, small rodents are the preferred food source for buzzards and feeding on rodents (at its peak) during the breeding season results in higher breeding success of individuals (Sundell et al., 2004; Tast et al., 2010). Rough-legged buzzards in our study breed either in the areas with a cyclic density of rodents (Nenetsky, Vaigach, and Yamal) or in areas with no rodents (Kolguev Island), where a variety of geese species breed in large numbers annually (Mooij et al., 2011), providing a stable resource for the rough-legged buzzard (Pokrovsky et al., 2015). Thus, areas with rodent cycles are for the purpose of this study classified as “variable resource” areas and areas with no rodents as “stable resources”.
There are several hypotheses regarding the period in which rough-legged buzzards select breeding sites. One of them is that they do it during spring migration. While selecting nesting areas during spring migration is common to many species (Moore and Aborn, 2000; Newton, 2010), we believe it is unlikely for rough-legged buzzards. According to our data, the time between arrival in the Arctic and the start of incubation for rough-legged buzzards is 16 ± 4 (mean ± sd) days. At the same time, rough-legged buzzards build nests within 3–4 weeks between arrival and the start of egg-laying (Bechard et al., 2020). Thus, the nest-building behavior of rough-legged buzzards appears to be shorter than previously thought, and they start breeding immediately after migration and have no time for spring habitat selection. Snowy owls are known to search for a nesting site during their spring migration (Therrien et al., 2014; Brown et al., 2021), but unlike rough-legged buzzards, they are more adapted to Arctic conditions and can spend more time (up to 108 days) searching for suitable habitat. Therefore, here we focused on the investigation of the post-breeding prospecting movements.
Here, we hypothesize that rough-legged buzzards select nesting areas during the previous breeding season. The typical shape of the rodent cycle consists of 4 years with about 3 years of increasing rodent numbers (from low to medium to peak abundance) followed by a rapid crash thereafter (from the peak to low abundance) (Oksanen and Oksanen, 1992; Angerbjörn et al., 2001). Thus, if arctic predators were to predict rodent abundance for their next breeding season, they would win in three and lose in one out of four cases. Therefore, if a breeding area is in the “variable resource” region, buzzards after the nesting season would search for a suitable future nesting area for the following year. Following such a phase of prospecting movement, i.e., potential search for a nesting area, buzzards would migrate to the non-breeding area. Then, after return migration, buzzards would attempt to breed in a suitable nesting area that they had found in the previous summer. At the same time, if a breeding area is in the “stable resource” region (i.e., with no rodents), buzzards after breeding would have minimum or no prospecting movement. They would stay in their nesting area until the end of the breeding season, afterwards migrate South to overwinter, and return in spring to the same area for breeding. The hypothesis is illustrated on Figure 1.
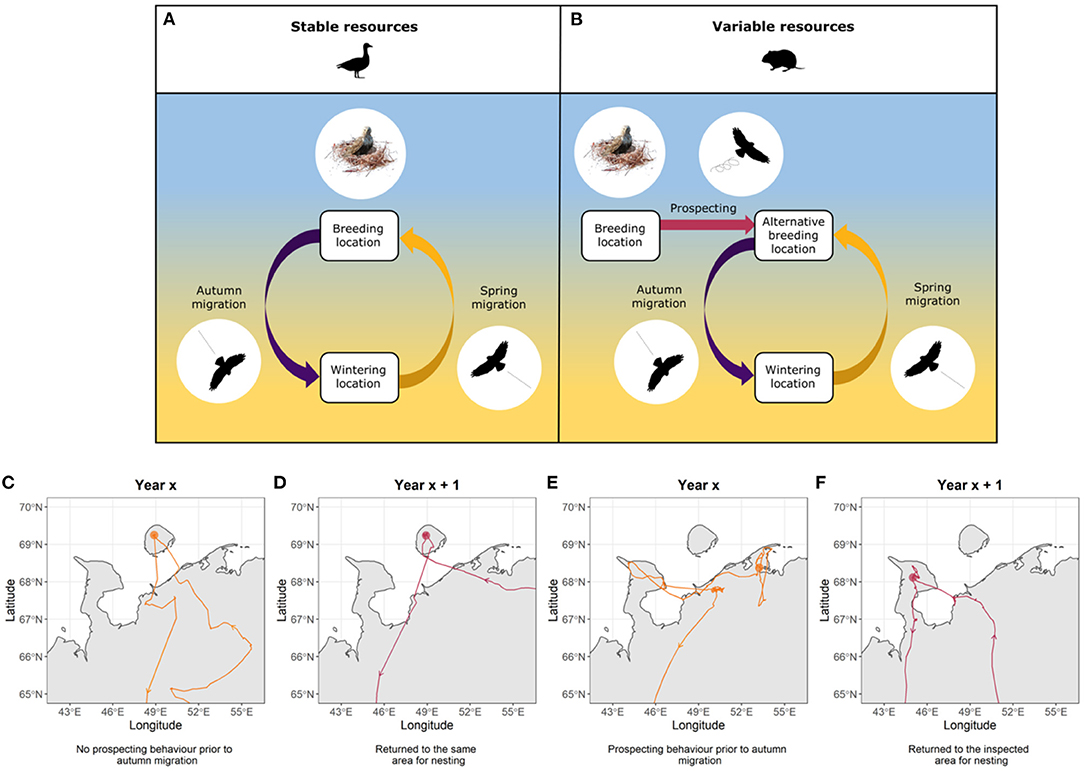
Figure 1. The overall scheme of the hypothesis. (A) If an individual breeds in areas with stable resources, it stays within the nesting area until the end of the breeding season, afterwards migrates to the south to overwinter and in spring returns to the same area for breeding. (B) If an individual breeds in areas with variable resources, it leaves the nesting area and searches for a suitable nesting area for the following year. After this phase of prospecting movement, it migrates to the wintering area and after spring migration, it attempts to breed in the suitable nesting area that it found in the previous summer. Exemplary trajectories for two consecutive years of (C,D) individual that breeds in areas with stable resources and (E,F) individual that breeds in areas with variable resources. Nest locations are marked with dots and arrows represent movement direction.
Prospecting movements have been linked to individual fitness and previous reproductive performance (Reed and Oring, 1992; Reed et al., 1999; Dittmann et al., 2005; Arlt and Pärt, 2008). Birds with bad breeding performance tend to change the nesting territory more often (Newton and Marquiss, 1982; Haas, 1998; Hoover, 2003), and therefore, they should prospect more than successful conspecifics. Thus, we could expect that rough-legged buzzards would stay in the Arctic during the whole breeding period regardless of their breeding success. At the same time, failed breeders will have extra time available during the breeding season to search for a suitable breeding area for the following year and, thus, will prospect more than successful breeders. Although the existing studies provide us with a good basis for assumptions of Arctic raptors post-breeding behavior, most of the studies on prospecting movements were on non-breeding birds (Reed and Oring, 1992; Dittmann et al., 2005) or breeding passerine birds (Hoover, 2003; Ward, 2005; Arlt and Pärt, 2008), which change their breeding site in a fairly limited space (5–10 sq km); and the main methods for these studies were ring recoveries and field observations. We ask whether this phenomenon can be relevant for species that change their breeding territory by hundreds or thousands of kilometers, such as rough-legged buzzards. And whether this phenomenon may thus explain the ability of arctic raptors to find regions with rodent peaks in the tundra. Modern tracking technologies allow us to monitor the prospecting behavior of the birds on a big scale with precise details and find out how raptors search for the rodent peaks and how they can adapt to the changes happening in the Arctic.
Our specific predictions are as follows: (i) buzzards, regardless of nesting success, will remain in the Arctic for the rest of the summer. Failed breeders will not migrate to the non-breeding grounds earlier. We expect no difference in departure times between successful, unsuccessful and non-breeding birds. (ii) Individuals who failed to breed will have extra time available during the breeding season to search for a suitable breeding area for the following year. Thus, failed breeders will prospect more (travel larger distances, cover larger areas, and move further away from the nest) than the successful breeders. (iii) In areas with stable resources, buzzards will prospect less than in the areas with variable resources since food resources are stable and individuals do not need to travel far to find alternative suitable nesting areas. (iv) Individuals will return to the area they explored during the previous breeding season. Regardless of areas with stable or variable resources, buzzards would return to the exploration area, i.e., the potential nesting area they selected during the previous post-breeding period.
2. Materials and Methods
2.1. Study Area
Fieldwork was conducted in June-August 2013-2019 in NW Russia on four study sites (Figure 2): Kolguev Island in the Barents Sea (hereafter Kolguev, 69°16′N, 48°87′E) in years 2013, 2015, 2017–2019; Nenetsky Nature Reserve in the Pechora river lowlands (hereafter Nenetsky, 68°20′N, 53°18′E) in 2014; Vaigach Island (hereafter Vaigach 69°43′N, 60°08′E) in 2015; and “Erkuta” tundra monitoring site in the southern part of Yamal peninsula (hereafter Yamal, 68°12′N, 68°59′E) in 2016. For the details on permits, see the Ethics Statement at the end of the article.
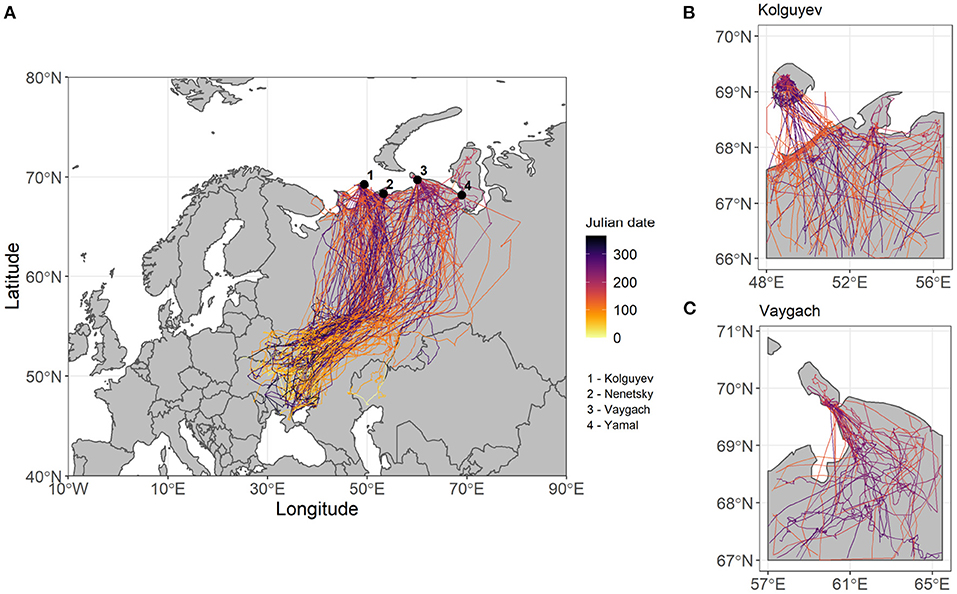
Figure 2. (A) GPS trajectories of 43 rough-legged buzzards (133 annual tracks). The color gradient represents Julian date. Locations of the study sites are marked with black dots. (B) Zoomed in plot of Kolguyev Island and (C) Vaigach Island.
2.2. Data Collection
Between 2013 and 2020, we tracked 43 adult rough-legged buzzards (35 females and 8 males). We caught birds with bow nets on the nests during the late incubation and nestling period and equipped them with 45g solar GSM-GPS-ACC loggers (e-obs GmbH) or 15g solar GSM-GPS loggers (UKn—University of Konstanz, Model “Lika”) using a Teflon harness. E-obs loggers were attached on 28 individuals, UKn loggers on 13 and two individuals were first equipped with UKn loggers that were later replaced with e-obs loggers. E-obs loggers recorded GPS locations and 3D body acceleration during 24 h/day. GPS positions were recorded every hour (full battery) and every 5 h (normal battery). Three-axial body acceleration was measured every 5 min for 3.8 s at 10.54 Hz (40 data points per axis and 120 data points per ACC burst). Data were stored and then downloaded via GSM mobile phone network using GPRS technology or via UHF radio link using handheld base stations. UKn loggers recorded GPS positions every hour (full battery) and every 12 h (normal battery) 24 h/day.
In addition to the GPS data, we also collected information on the nest locations of the breeding individuals and the nesting success for each year (n = 87). We estimated nest location and nesting success for 40 annual trajectories of individuals (9 for males and 31 for females) using direct field observations. For 47 annual trajectories (all of them females), we estimated the location of the nest and inferred nesting success from the bio-logging data (GPS and accelerometer) in the following way: if the bird stayed in one place (the difference between coordinates was <3 m) for more than 1 day (24 hourly positions), we considered it as the beginning of incubation and this position as the nest coordinates. We verified this assumption with information about the bird's body's position for birds for which we used loggers with accelerometers (28 bird-years). In all cases, the accelerometer showed that the birds at this time were in a horizontal position, which is possible if the bird is flying, or incubating a clutch or covering the nestlings. If the bird stayed on the nest for more than 50 days from the start of incubation, we considered it a successful nest. The threshold of 50 days was used because the incubation period in wild rough-legged buzzards is at least 31 days (Cramp and Simmons, 1980) and after hatching, a female stays at the nest more or less continuously until young are 17–22 days old (Bechard and Swem, 2002). If a bird stayed less than 50 days in a nest, we assumed that it failed to breed. We verified our distinction between the failed and successful breeders with the direct observations (n = 28), and in all cases, we correctly predicted nesting and breeding status. Therefore, for 87 annual trajectories, we estimated breeding attempts, and if a bird tried to breed, we estimated the nest coordinates, nesting success and nesting duration. For 70 annual trajectories, we estimated the nesting success (successful/failed) and nesting duration (number of days the bird was incubating and feeding nestlings).
2.3. Data Analyses
After removing outliers and duplicated timestamps, the data set comprised 43 individuals, 133 annual trajectories and 268977 positions (Figure 2).
First, we investigated the relationship between the departure day from the breeding grounds and nesting performance. For each individual trajectory (GPS locations of a bird during the specific year), we noted the date when it crossed the latitude of 64 degrees (the approximate southern border of the breeding area of the rough-legged buzzard in this region) during the autumn migration. If the difference in days between the first GPS position before and the first position after a bird crossed the 64 degrees latitude was less than 10 days, we used the mean value as a departure location and its corresponding date as departure day. If the difference was more than or equal to 10 days, we did not use the departure day for the analyses as the calculated mean location was not likely to represent the departure location and, thus, departure day reliably. In total, we had 71 departure days for 35 birds from 2013 to 2020. When individuals crossed a latitude of 64 degrees more than once, we recorded the first crossing as a departure/arrival location.
Second, we compared prospecting movement between the individuals that bred in an area with stable resources and those that bred in an area with variable resources (Figure 1). For variable resources, the number of tracked individuals was 23, annual trajectories 40 and positions 144087. For stable resources, the number of tracked individuals was 21, annual trajectories 45 and positions 110305. Furthermore, we compared prospecting movement after breeding attempt between the individuals who failed to breed and those who bred successfully. Prospecting movement was assessed for GPS locations between the nest location and the location corresponding to the date of 10 days before the departure location. The threshold of 10 days was selected based on a visual inspection of different thresholds (excluding 15, 10, and 5 days from the departure date). By removing 10 days before departure, we made sure to include only locations that are a part of prospecting movement and avoided including locations that were already part of autumn migration (for example, see Supplementary Figure 1). Prospecting movement was quantified by the cumulative distance (using “move” R package, Kranstauber et al., 2019), area covered using the 95% MCP—Minimum Convex Polygon estimator (“adehabitatHR” R package, Calenge, 2006), and the distance from the nest to each GPS location (using “raster” R package, Hijmans, 2020). The three parameters were calculated by including the first five data points (GPS locations) and every step adding an additional data point (e.g., 95% MCP was estimated for the first five locations, then again for six locations, for seven locations, etc. until all locations were included). In total, we calculated the three parameters for 14 individuals that bred in an area with stable resources (5006 data points) and 13 individuals that bred in an area with variable resources (4686 data points).
Third, we assessed whether individuals in the next breeding season returned to the territory they had inspected the year before. To test whether individuals inspected the area they returned to the following year to breed, we calculated the minimum distance between each GPS location of an individual's annual trajectory to the nest location of the following year (using “sp” R package, Pebesma and Bivand, 2005; Bivand et al., 2013). We calculated these trajectory-to-nest distances for individuals that failed to breed and those that bred successfully for a total of 35 events (18 individuals). To test if successful breeders return to the same area to breed and failed breeders to the different area, we calculated the distance between the nest locations of the current and the following year (37 events, 20 individuals) and compared them between the failed and successful breeders.
2.4. Statistical Analysis
To investigate whether buzzards' departure day from the breeding ground was related to breeding performance, we ran a linear mixed model (LMM) with departure day as a response variable, nesting success as a fixed effect and bird ID as a random effect, and a linear model (LM) with departure day as a response variable and nesting duration as a predictor. To account for pseudoreplication in the LM, we ran ten models each with 1 year per individual included (years per individual included in each model were randomly selected) and performed model averaging. We additionally checked whether the season (year) influences departure days. We used LMMs with departure days as a response variable, year as a fixed effect and bird ID as a random effect. We ran 10 LMs and performed model averaging as described above.
To compare prospecting behavior between individuals that bred successfully and those that failed to breed, we used LMMs with log cumulative distance, log MCP or log nest distance included as a response variable, an interaction between the Julian day and nesting success as a predictor and annual trajectory identity as a random effect. We used the interaction between Julian day and nesting success in the model because we wanted to test if cumulative distance, MCP and nest distance increase with time to a different extent for nesting success and nesting failure. We ran the three models separately for stable and variable resources and performed a model averaging of 10 models, so that in each model, only one bird ID per annual trajectory was included. The inclusion of annual trajectory in the models was randomly selected. To average the models, the sample sizes of data sets used for models had to be the same, thus we used an approximate minimum sample size of randomly selected rows per annual trajectory included (n = 1,800 for stable resources and n = 2,000 for variable resources).
To compare prospecting behavior between stable and variable resource sites, we used LMMs with log MCP or log trajectory-to-nest distance included as a response variable, prey variability as a predictor and bird ID as a random effect. In the case of log cumulative distance, the LMM did not converge so we ran ten models each with 1 year per individual included and performed model averaging as described above.
For all models, we first performed an overall test of full-null model comparison. We fitted the LMMs with a restricted maximum likelihood method using lmerTest (Kuznetsova et al., 2017). Model averaging was performed using the “MuMIn” R package (Barton, 2019). Assumptions of normally distributed and homogeneous residuals were fulfilled.
2.5. Terms
Note that throughout the text, we use the term “nesting area” for the area where rough-legged buzzards breed, “post-breeding period” for the period in the breeding season after a breeding attempt, “prospecting” for the potential search for a new nesting area during the post-breeding period, and “exploration area” for the area individuals prospected during the previous season and potentially return to in the following breeding season.
3. Results
3.1. Departure Days
Rough-legged buzzards stayed in the Arctic during the post-breeding period, and the timing of departure from the breeding grounds was similar for the individuals that bred successfully (mean ± SE: 276.4 ± 1.9 (hereafter day of the year), n = 29) and those that failed to breed (mean ± SE: 278.8 ± 1.6, n = 27). Five individuals that did not attempt to breed departed from the breeding grounds at the similar time than the other two groups (mean ± SE: 272.0 ± 4.3, n = 5). The model results confirmed that failed and successful breeders departed from the breeding grounds at the approximately same time (full-null model comparison: χ2 = 1.56, df = 1, P = 0.21; Supplementary Table 1 and Figure 3). Also, nesting duration of individuals that failed to breed did not influence the timing of departure (Supplementary Table 2 and Figure 3). Note that no full-null comparison is provided for this model since we performed model averaging and used only one annual trajectory per individual (Sum of squares = 3.65, df = 1, P = 0.814).
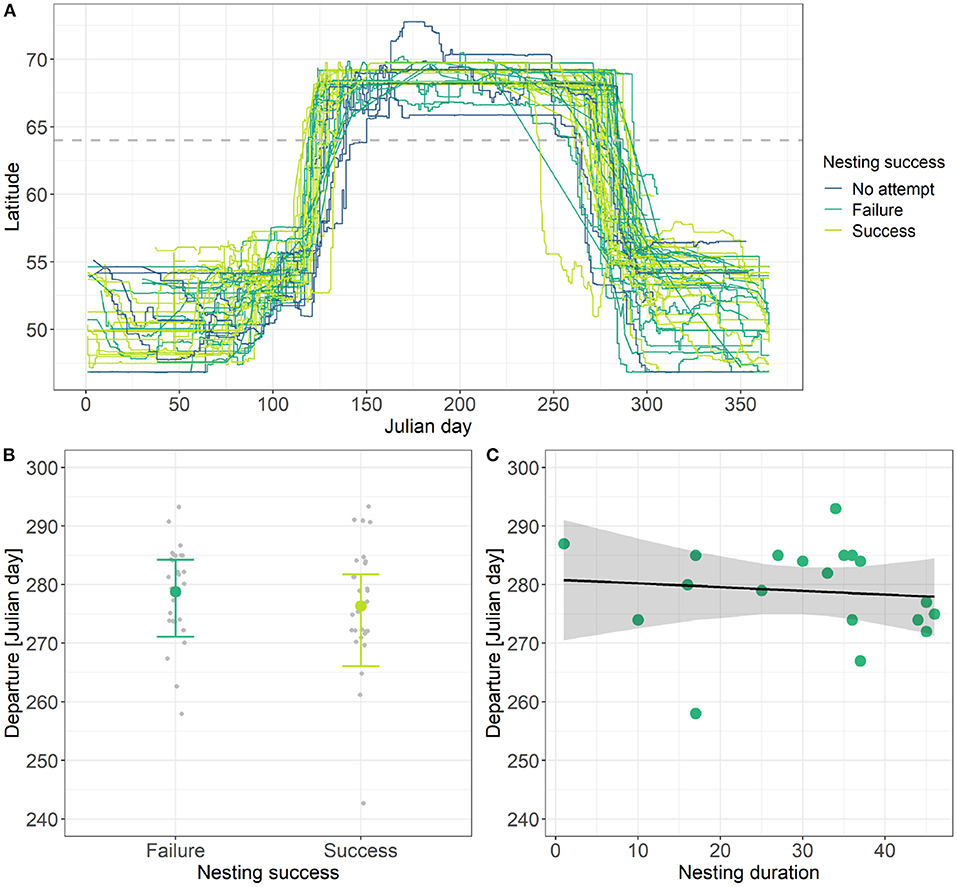
Figure 3. Relationship between the nesting success and departure days in rough-legged buzzards. (A) Latitude change during the annual life-cycle. (B) Departure day as a response of nesting success (predicted 95% CIs from LMM using the bootstrapping method and 500 simulations in “ciTools” R package (Haman and Avery, 2020) with lower limit representing minimum CI and upper limit representing maximum CI; gray dots represent raw data). (C) Departure day as a response of nesting duration of individuals that failed to breed (predicted CIs from LM with only 1 year of data per individual included; blue dots represent raw data).
Departure days significantly differed between the years (2013–2020) with the timing of departure becoming later every year (full-null model comparison: χ2 = 34.45, df = 7, P < 0.001; Supplementary Table 3 and Supplementary Figure 2). However, each year, we had a similar number of successful vs. failed breeders (mean ± SE; failed: 4.6 ± 1.2, successful: 4.8 ± 1.0), meaning that our results were not year-dependent.
3.2. Prospecting Behavior
All three parameters measuring prospecting behavior during the post-breeding period, cumulative distance, MCP and trajectory-to-nest distance, had higher values for failed than successful breeders.
Cumulative distance measured for birds breeding in stable resources increased with Julian day significantly more for individuals that failed to breed than for individuals that bred successfully (full-null model comparison: χ2 = 2287.8, df = 3, P < 0.001; Supplementary Table 4 and Figure 4A). For birds breeding in variable resources, the cumulative distance also increased with Julian day and was influenced by the nesting success (full-null model comparison: χ2 = 2772.6, df = 3, P < 0.001; Supplementary Table 5 and Figure 4B).
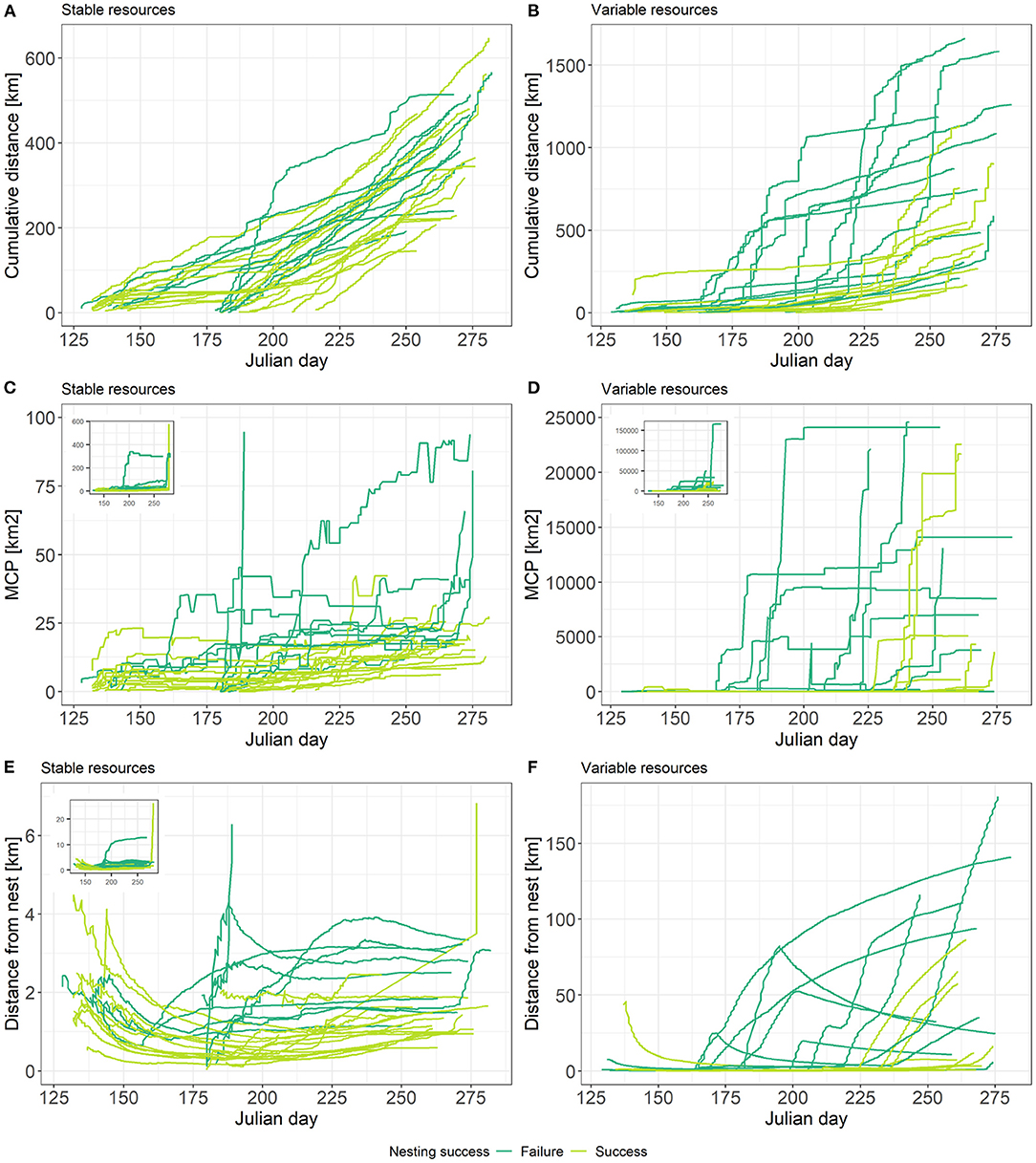
Figure 4. (A) Cumulative distance as a function of Julian date for stable resources. (B) Cumulative distance as a function of Julian day for variable resources. (C) MCP as a function of Julian day for stable resources. (D) MCP as a function of Julian day for variable resources. (E) Nest distance as a function of Julian day for stable resources. (F) Nest distance as a function of Julian day for variable resources.
MCP increased with the Julian date and was significantly larger for individuals that failed to breed than for individuals that bred successfully. This effect was seen in individuals that bred in stable resources (full-null model comparison: χ2 = 841.1, df = 3, P < 0.001; Supplementary Table 6 and Figure 4C) as also for individuals that bred in variable resources (full-null model comparison: χ2 = 1225.9, df = 3, P = 0.002; Supplementary Table 7 and Figure 4D).
Trajectory-to-nest distance increased with the Julian day and it was significantly larger for failed breeders than for successful breeders. This was the case for individuals breeding in stable resources (full-null model comparison: χ2 = 139.7, df = 3, P < 0.001; Supplementary Table 8 and Figure 4E) and also for individuals breeding in variable resources (full-null model comparison: χ2 = 1035.8, df = 3, P < 0.001; Supplementary Table 9 and Figure 4F).
Furthermore, individuals breeding in areas with variable resources explored larger areas and traveled more and further from the nest than those breeding in areas with stable resources (Supplementary Tables 10–12 and Figure 5). Full-null model comparison showed significant results for cumulative distance (Sum of Squares = 0.0, Res. df = 27, P < 0.001), MCP (χ2 = 5.8, df = 1, P = 0.016) and trajectory-to-nest distance (χ2 = 8.7, df = 1, P = 0.003).
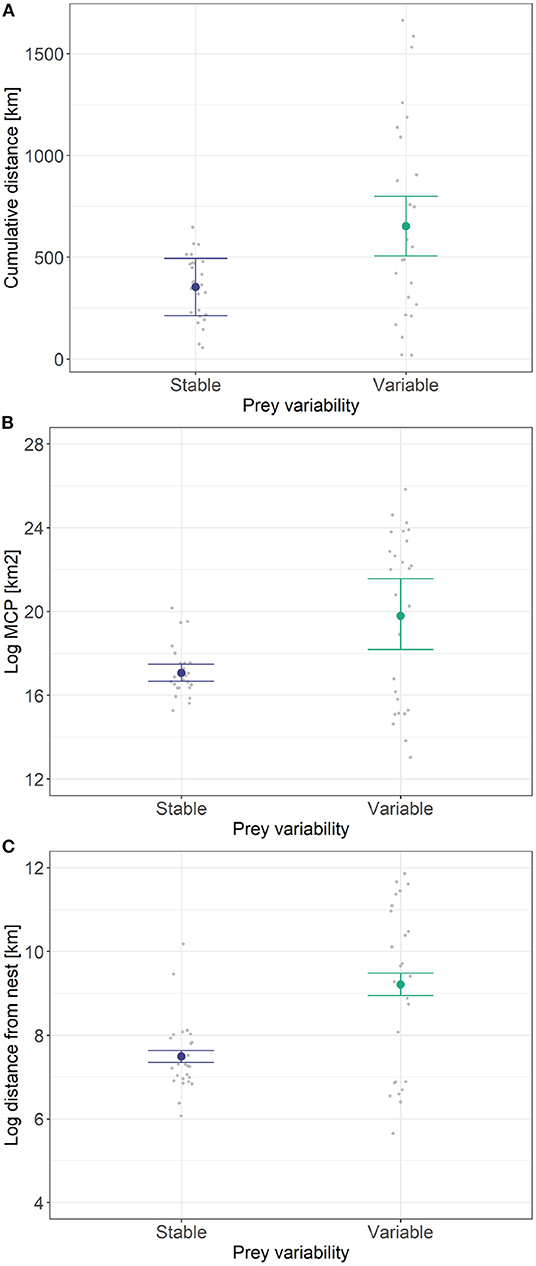
Figure 5. (A) Cumulative distance, (B) MCP, and (C) distance from nest as a function of prey variability (stable vs. variable).
3.3. Return to the Explored Area
Both successful and failed breeders returned to the area they explored during the previous breeding season. The minimum distance measured between an individual's annual trajectory and nest location of the following year was comparable between individuals in stable and those in areas with variable prey (Figure 6). The mean (± SE) distance for stable resources was 5.2 ± 4.8 km (range: 0.3 m–115.4 km, n = 24) and for variable prey was 8.7 ± 7.2 km (range: 1.5 m–80.5 km, n = 11). When removing outliers of 115.4 and 80.5 km, the mean ± SE is 0.4 ± 0.1 km (range: 0.3 m–2.2 km) for stable and 1.6 ± 0.8 km (range: 1.5 m–6.9 km) for variable prey. These are the two cases where individuals upon spring migration flew in the direction of the nest location of the previous year but decided to settle before reaching that location (Supplementary Figure 3).
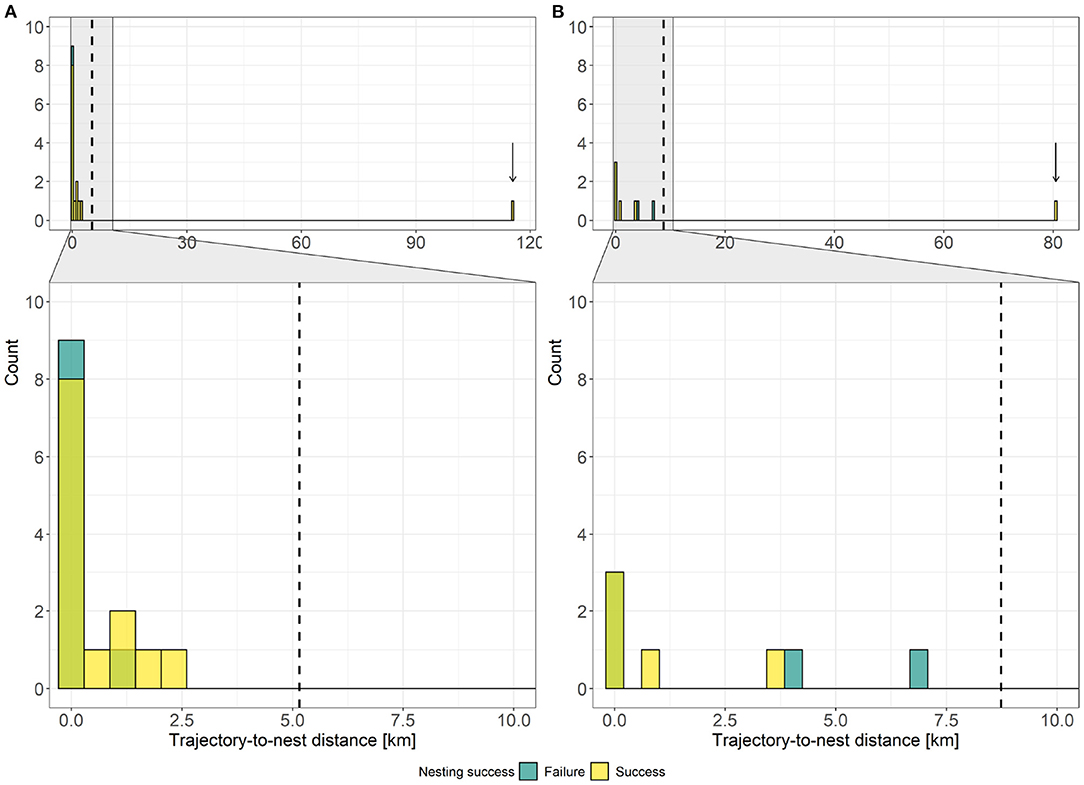
Figure 6. Histogram of the minimum distance between the trajectory of the current year and nest location of the following year (trajectory-to-nest distance) for (A) stable and (B) variable resources. Dashed line represents the mean value.
The distance between the nest locations of the current and the following year was smaller for stable then for variable prey (Figure 7). The mean (± SE) distance for stable prey was 2.2 ± 0.7 km (range: 2 m–17.2 km, n = 24) and for variable prey was 63.6 ± 27.9 km (range: 0.2–341.4 km, n = 13). When removing outlier of 341.4 km the mean ± SE is 40.5 ± 17.0 km (range: 0.2–139.0).
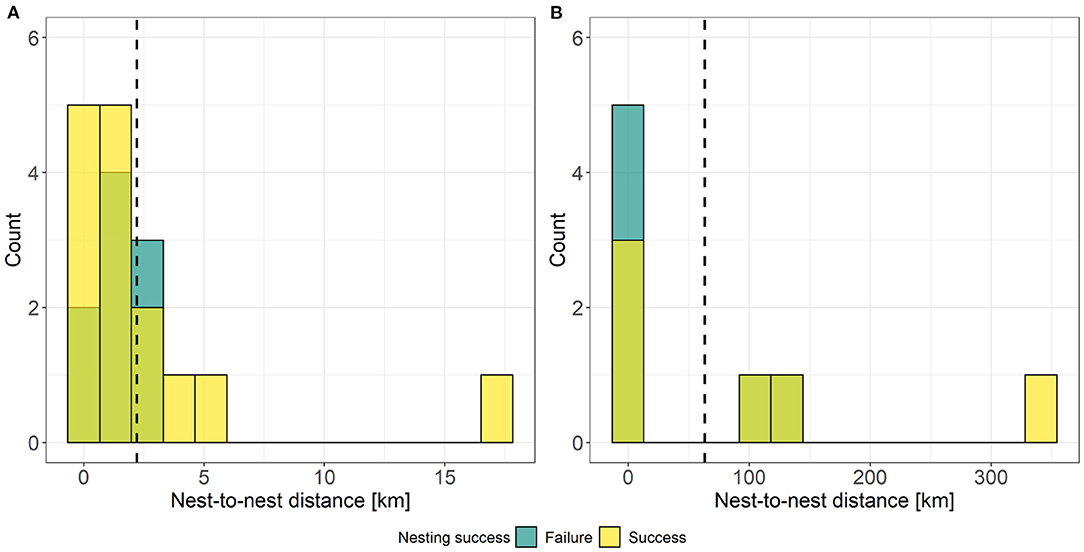
Figure 7. Histogram of the distance between the nests of the current and the following year (nest-to-nest distance) for (A) stable and (B) variable resources. Dashed line represents the mean value.
4. Discussion
We hypothesized that rough-legged buzzards select nesting areas during the previous breeding season. We found evidence for this behavior from our data, and our predictions came true.
First, we showed that the departure date from the breeding grounds did not differ between the individuals that failed to breed, bred successfully or those that did not attempt to breed. The average date at which all birds stayed in the arctic before departing is October 1. The reason for staying could be extra time available that they could use to search a nesting area for the following year. The alternative (but non-mutually exclusive) explanation is that rough-legged buzzards may remain in the Arctic to fatten up before starting their autumn migration. Pre-migratory fattening was shown for many species (Bibby and Green, 1981; Schaub and Jenni, 2000; Newton, 2010). However, raptors are known to feed regularly on migration and use soaring flight as energy sources for migration (Bildstein, 2006). At the same time, if the reason for post-breeding stay in the Arctic were only pre-migratory fattening, all birds would fatten up at different times, and the timing of their departure would differ accordingly. Therefore, we believe that the most likely explanation for a stay in the Arctic is searching for a nesting site for the following year.
Second, both failed and successful breeders prospected during the post-breeding period. Failed breeders prospected more than successful breeders, likely because they had more time to explore the area after the failed breeding attempt. However, this result could also suggest that failed breeders are more eager than successful breeders to find a suitable nesting area for the following year. The difference in prospecting between the failed and successful breeders was especially evident in areas with variable resources, while the difference in areas with stable resources was smaller. A likely explanation is that Kolguyev Island provides stable resource-rich habitat (Mooij et al., 2011), so individuals do not need to search for an area far away from their initial breeding site. During the entire study, not a single bird has left the island during the prospecting movement. This behavior indicates that the main reason for the prospecting period in the post-breeding period is the search for the territory with a high density of prey.
Third, rough-legged buzzards that failed to breed as well as those that bred successfully returned to the area they explored the year before. Breeding success did not determine if individuals will return to the same nesting area. In some cases, failed breeders could search for a nest location but still return to the same area to breed since they did not find a more suitable location. In contrast, successful breeders could find a more suitable nesting area during their prospecting movement and return to that area for breeding in the following year. However, regardless of the breeding success or how much they prospected, rough-legged buzzards bred in the area they had previously surveyed. We had only two exceptions to this rule.
Two individuals which bred successfully (both are island breeders), in the following year during the spring migration were moving toward their nest location of the previous year but stopped before the coast (Supplementary Figure 3 and Figure 6). The reason could be that they found a suitable nesting area on the way and decided to settle, or at the time of arrival to the coast, the wind conditions were not suitable for crossing, so they decided to settle on the mainland. Wind conditions are indeed an important factor when deciding to cross water bodies (Nourani et al., 2021). Yet, in the following years, individuals returned to the same area for breeding (Supplementary Figure 4). This behavior suggests that rough-legged buzzards could have mixed two-phase habitat selection. They select the future breeding territory during the post-breeding period and may refine their choice during the following year. In the second phase, they may either find a better territory en route to a previously selected area or decide not to breed if overall breeding conditions in the designated location have turned out to be poor.
Described two-step habitat selection could explain the asynchrony encountered in the density dynamic of rough-legged buzzards and their prey on the Nenetsky Ridge (Pokrovsky et al., 2014). While the density of rough-legged buzzards is usually highest in the years with a peak of rodents (Sundell et al., 2004; Tast et al., 2010), on the Nenetsky Ridge, it was highest in the year after the rodent peak (Pokrovsky et al., 2014). The latter type of predator-prey density dependence is well-known and explained by a series of time-delayed numerical responses. However, it is characteristic of sedentary resident specialist predators (Nielsen, 1999), and there was no clear explanation of this dynamic for migratory species. Rough-legged buzzards were assumed to find the territory with a high density of prey during spring migration. However, if habitat selection occurs a year before, as we showed in this study, rough-legged buzzard density should be highest in the year that follows the year with the peak rodent abundance. At the same time, predators who arrived on the territory with a low rodent density could decide not to nest in that season, especially if the number of alternative prey is low in that region. Thus, as a result, for rough-legged buzzards, we could meet both types of predator-prey density dynamic - either delayed or direct density dependency.
With the global change, rodent peaks are predicted to become less regular (Ims et al., 2011; Ehrich et al., 2020). In several areas across the Arctic, the typical shape of the cycle (several years of increasing rodent numbers and rapid crash) has recently become less frequent due to the increased incidence of spring warming (Kausrud et al., 2008). Disrupted rodent cycles can impact rough-legged buzzards' post-breeding prospecting behavior and modify the adaptive value of such movement strategy. That could explain why buzzards in an area with stable resources stay there rather than finding a place with rodent peak and hence, potentially higher fitness. Yet, in the future, we can expect that with less evident lemming cycles, rough-legged buzzards will likely switch to alternative prey and thus change their movement patterns. We speculate that prospecting movement during the breeding season in failed breeders would become less evident with shorter distances and smaller areas covered. This could potentially affect genetic diversity and cause populations to become more isolated, as was the case for peregrine falcons (Falco peregrinus) (Gu et al., 2021). However, further studies are needed to confirm this suggestion.
This study has two limitations that could be addressed in future research. First, we assumed that all the areas except Kolguev Island are the 'variable resource' areas. While the difference between rodent-free Kolguev Island with a high constant density of geese and the other sites is very pronounced, areas on the mainland also differ in rodent and alternative prey abundances (Ehrich et al., 2012; Pokrovsky et al., 2020). On some sites (e.g., Nenetsky Ridge), rough-legged buzzards could switch to alternative prey when the rodents are scarce (Pokrovsky et al., 2014). Thus, while rodent density in these regions will be cyclical, the overall resource availability for buzzards will be stable. This may explain considerable variation in prospecting values for the 'variable resource' group (Figure 5). Second, our data consists of 35 females and eight males. A skewed sex ratio could have potential consequences on the movement patterns observed in this study. Adult females could disperse further than males in several raptor species (Mearns and Newton, 1984; Serrano et al., 2001; Bildstein, 2006; Whitfield et al., 2009). Thus, such an overrepresentation of females in the study sample could bias observed prospecting behavior and overestimate movement parameters.
In summary, we showed that the prospecting movement during the post-breeding period plays an important role in finding a nesting area for the following year. Such a way of dealing with a lack of time and extreme arctic environment suggests that rough-legged buzzards have highly developed spatial memory due to memory-demanding ecological conditions, maybe more than it was previously thought (Pravosudov et al., 2006). We expect that this strategy is used by many migratory species, both non-breeders (Doligez et al., 2004; Dittmann et al., 2005) as well as breeders (Forstmeier, 2002; Ward, 2005; Arlt and Pärt, 2008) but especially Arctic birds or other animals that face limited time for breeding, fluctuating resources, and harsh environmental conditions. This study is a step forward in understanding movement and settlement decisions in animals experiencing changing environmental conditions and help us to predict future changes caused by climate warming in the Arctic.
Data Availability Statement
The datasets presented in this study can be found in online repositories. The names of the repository/repositories and accession number(s) can be found below: www.movebank.org, https://www.doi.org/10.5441/001/1.dg3sm625, https://www.movebank.org/cms/webapp?gwt_fragment=page=studies,path=study9493874.
Ethics Statement
Ethical review and approval was not required for the animal study because to carry out the work for this study, IP applied for and obtained permit No. 77-18/0854/4388 from The General Radio Frequency Centre, permit No. RU/2018/406 from Federal Service for Supervision of Communications, Information Technology and Mass Media (Roskomnadzor), and permit No. RU0000045099 from Federal Security Service. No specific permissions were required from Federal Service for Supervision of Natural Resources (Rosprirodnadzor) according to Section 44 and Section 6 of the Federal Law of the Russian Federation No. 52 from 24.04.1995 (last update 24.04.2020) On Wildlife, and from Federal Service for Technical and Export Control (FSTEC/FSTEK) according to Russian Federation government decree No. 633 from 29.08.2001 and Letter from FSTEK No. 240/33/1373 from 06.04.2015. There were no Special Protected Natural Territories in our study area, and our activities did not include withdrawal of investigated species from nature. All our protocols met the ABS/ASAB guidelines for the ethical treatment of animals. In Nenetsky, the work was carried out in agreement with the Nenetsky Nature Reserve in a buffer zone.
Author Contributions
IP developed the project idea. TC and IP analyzed the data and wrote the manuscript. IP, OK, and MW prepared the database. KS provided the valuable suggestions for data analyses. IP, OK, IF, MW, and TC conducted fieldwork. MW obtained the funding. All authors took part in the preparation of the manuscript including logical interpretation and presentation of the results. All authors approved the final version of the manuscript for publication.
Funding
This study was funded by the Max Planck Institute of Animal Behavior, and the German Aerospace Center (DLR).
Conflict of Interest
The authors declare that the research was conducted in the absence of any commercial or financial relationships that could be construed as a potential conflict of interest.
Publisher's Note
All claims expressed in this article are solely those of the authors and do not necessarily represent those of their affiliated organizations, or those of the publisher, the editors and the reviewers. Any product that may be evaluated in this article, or claim that may be made by its manufacturer, is not guaranteed or endorsed by the publisher.
Acknowledgments
We thank reviewers for valuable comments on the manuscript.
Supplementary Material
The Supplementary Material for this article can be found online at: https://www.frontiersin.org/articles/10.3389/fevo.2022.865482/full#supplementary-material
References
Angerbjörn, A., Tannerfeldt, M., and Lundberg, H. (2001). Geographical and temporal patterns of lemming population dynamics in fennoscandia. Ecography 24, 298–308. doi: 10.1034/j.1600-0587.2001.240307.x
Arlt, D., and Pärt, T. (2008). Post-breeding information gathering and breeding territory shifts in northern wheatears. J. Anim. Ecol. 77, 211–219. doi: 10.1111/j.1365-2656.2007.01329.x
Bechard, M. J., and Swem, T. R. (2002). Rough-Legged Hawk: Buteo Lagopus. American Ornithologists' Union. doi: 10.2173/tbna.641.p
Bechard, M. J., Swem, T. R., Orta, J., Boesman, P. F. D., and Marks, J. S. (2020). “Rough-legged hawk (Buteo lagopus), version 1.0,” in Birds of the World, ed S. M. Billerman (Ithaca, NY: Cornell Lab of Ornithology). doi: 10.2173/bow.rolhaw.01
Bêty, J., Giroux, J.-F., and Gauthier, G. (2004). Individual variation in timing of migration: causes and reproductive consequences in greater snow geese (Anser caerulescens atlanticus). Behav. Ecol. Sociobiol. 57, 1–8. doi: 10.1007/s00265-004-0840-3
Bibby, C., and Green, R. (1981). Autumn migration strategies of reed and sedge warblers. Ornis Scand. 12, 1–12. doi: 10.2307/3675898
Bildstein, K. L (2006). Migrating Raptors of the World: Their Ecology & Conservation. Ithaca, NY: Cornell University Press.
Bivand, R. S., Pebesma, E., and Gomez-Rubio, V. (2013). Applied Spatial Data Analysis with R, 2nd Edn. NY: Springer. doi: 10.1007/978-1-4614-7618-4
Brown, A., McCabe, R. A., Therrien, J.-F., Wiebe, K. L., Weidensaul, S., Brinker, D., et al. (2021). Nomadic breeders snowy owls (Bubo scandiacus) do not use stopovers to sample the summer environment. IBIS 163, 1271–1281. doi: 10.1111/ibi.12950
Calenge, C (2006). The package adehabitat for the r software: tool for the analysis of space and habitat use by animals. Ecol. Modell. 197, 1035. doi: 10.1016/j.ecolmodel.2006.03.017
Cramp, S., and Simmons, K. (1980). The Birds of the Western Palearctic. Vol. II. Hawks to Bustards. Oxford: Oxford University Press.
Curk, T., Pokrovsky, I., Lecomte, N., Aarvak, T., Brinker, D. F., Burnham, K., et al. (2020). Arctic avian predators synchronise their spring migration with the northern progression of snowmelt. Sci. Rep. 10, 1–11. doi: 10.1038/s41598-020-72948-x
Dittmann, T., Zinsmeister, D., and Becker, P. H. (2005). Dispersal decisions: common terns, sterna hirundo, choose between colonies during prospecting. Anim. Behav. 70, 13–20. doi: 10.1016/j.anbehav.2004.09.015
Doligez, B., Pärt, T., and Danchin, E. (2004). Prospecting in the collared flycatcher: gathering public information for future breeding habitat selection? Anim. Behav. 67, 457–466. doi: 10.1016/j.anbehav.2003.03.010
Ehrich, D., Henden, J.-A., Ims, R. A., Doronina, L. O., Killengren, S. T., Lecomte, N., et al. (2012). The importance of willow thickets for ptarmigan and hares in shrub tundra: the more the better? Oecologia 168, 141–151. doi: 10.1007/s00442-011-2059-0
Ehrich, D., Schmidt, N. M., Gauthier, G., Alisauskas, R., Angerbjörn, A., Clark, K., et al. (2020). Documenting lemming population change in the arctic: Can we detect trends? Ambio 49, 786–800. doi: 10.1007/s13280-019-01198-7
Forstmeier, W (2002). Benefits of early arrival at breeding grounds vary between males. J. Anim. Ecol. 71, 1–9. doi: 10.1046/j.0021-8790.2001.00569.x
Gilg, O., Hanski, I., and Sittler, B. (2003). Cyclic dynamics in a simple vertebrate predator-prey community. Science 302, 866–868. doi: 10.1126/science.1087509
Gilg, O., Sittler, B., and Hanski, I. (2009). Climate change and cyclic predator-prey population dynamics in the high arctic. Glob. Change Biol. 15, 2634–2652. doi: 10.1111/j.1365-2486.2009.01927.x
Gilg, O., Sittler, B., Sabard, B., Hurstel, A., Sané, R., Delattre, P., et al. (2006). Functional and numerical responses of four lemming predators in high arctic greenland. Oikos 113, 193–216. doi: 10.1111/j.2006.0030-1299.14125.x
Gu, Z., Pan, S., Lin, Z., Hu, L., Dai, X., Chang, J., et al. (2021). Climate-driven flyway changes and memory-based long-distance migration. Nature. 591, 259–264. doi: 10.1038/s41586-021-03265-0
Haas, C. A (1998). Effects of prior nesting success on site fidelity and breeding dispersal: an experimental approach. Auk 115, 929–936. doi: 10.2307/4089511
Haman, J., and Avery, M. (2020). ciTools: Confidence or Prediction Intervals, Quantiles, and Probabilities for Statistical Models. R package version 0.6.1.
Hoover, J. P (2003). Decision rules for site fidelity in a migratory bird, the prothonotary warbler. Ecology 84, 416–430. doi: 10.1890/0012-9658(2003)084(0416:DRFSFI)2.0.CO;2
Ims, R. A., and Fuglei, E. (2005). Trophic interaction cycles in tundra ecosystems and the impact of climate change. Bioscience 55, 311–322. doi: 10.1641/0006-3568(2005)055(0311:TICITE)2.0.CO;2
Ims, R. A., Yoccoz, N. G., and Killengreen, S. T. (2011). Determinants of lemming outbreaks. Proc. Natl. Acad. Sci. U.S.A. 108, 1970–1974. doi: 10.1073/pnas.1012714108
Kausrud, K. L., Mysterud, A., Steen, H., Vik, J. O., Østbye, E., Cazelles, B., et al. (2008). Linking climate change to lemming cycles. Nature 456, 93–97. doi: 10.1038/nature07442
Kranstauber, B., Smolla, M., and Scharf, A. K. (2019). move: Visualizing and Analyzing Animal Track Data. R package version 3.2.2.
Krebs, C. J., Kenney, A. J., Gilbert, S., Danell, K., Angerbjörn, A., Erlinge, S., et al. (2002). Synchrony in lemming and vole populations in the canadian arctic. Can. J. Zool. 80, 1323–1333. doi: 10.1139/z02-120
Kuznetsova, A., Brockhoff, P. B., and Christensen, R. H. B. (2017). lmerTest package: tests in linear mixed effects models. J. Stat. Softw 82, 1–26. doi: 10.18637/jss.v082.i13
Lamarre, V., Franke, A., Love, O. P., Legagneux, P., and Bêty, J. (2017). Linking pre-laying energy allocation and timing of breeding in a migratory arctic raptor. Oecologia 183, 653–666. doi: 10.1007/s00442-016-3797-9
Madsen, J., Tamstorf, M., Klaassen, M., Eide, N., Glahder, C., Rigét, F., et al. (2007). Effects of snow cover on the timing and success of reproduction in high-arctic pink-footed geese Anser brachyrhynchus. Polar Biol. 30, 1363–1372. doi: 10.1007/s00300-007-0296-9
Mearns, R., and Newton, I. (1984). Turnover and dispersal in a peregrine falco peregrinus population. IBIS 126, 347–355. doi: 10.1111/j.1474-919X.1984.tb00255.x
Montgomery, R. A., and Roloff, G. J. (2013). “Habitat selection,” in Encyclopedia of Biodiversity, 2nd Edn., ed S. A. Levin (Waltham, MA: Academic Press), 59–69. doi: 10.1016/B978-0-12-384719-5.00384-1
Mooij, J., Kruckenberg, H., Kondratyev, A., Zaynagutdinova, E., and Zöckler, C. (2011). Kolguev-island of geese. Goose Bull. 13, 24–33.
Moore, F. R., and Aborn, D. A. (2000). Mechanisms of en route habitat selection: How do migrants make habitat decisions during stopover? Stud. Avian Biol. 20, 34–42.
Newton, I., and Marquiss, M. (1982). Fidelity to breeding area and mate in sparrowhawks Accipiter nisus. J. Anim. Ecol. 51, 327–341. doi: 10.2307/4327
Nielsen, O. K (1999). Gyrfalcon predation on ptarmigan: numerical and functional responses. J. Anim. Ecol. 68, 1034–1050. doi: 10.1046/j.1365-2656.1999.00351.x
Nourani, E., Bohrer, G., Becciu, P., Bierregaard, R. O., Duriez, O., Figuerola, J., et al. (2021). The interplay of wind and uplift facilitates over-water flight in facultative soaring birds. Proc. R. Soc. B Biol. Sci. 288, 20211603. doi: 10.1098/rspb.2021.1603
Oksanen, L., and Oksanen, T. (1992). Long-term microtine dynamics in north Fennoscandian Tundra: the vole cycle and the lemming chaos. Ecography 15, 226–236. doi: 10.1111/j.1600-0587.1992.tb00029.x
Pebesma, E. J., and Bivand, R. S. (2005). Classes and methods for spatial data in R. R News 5, 9–13.
Pokrovsky, I., Ehrich, D., Fufachev, I., Ims, R. A., Kulikova, O., Sokolov, A., et al. (2020). Nest association between two predators as a behavioral response to the low density of rodents. Auk 137, ukz060. doi: 10.1093/auk/ukz060
Pokrovsky, I., Ehrich, D., Ims, R. A., Kondratyev, A. V., Kruckenberg, H., Kulikova, O., et al. (2015). Rough-legged buzzards, arctic foxes and red foxes in a tundra ecosystem without rodents. PLoS ONE 10, e0118740. doi: 10.1371/journal.pone.0118740
Pokrovsky, I., Ehrich, D., Ims, R. A., Kulikova, O., Lecomte, N., and Yoccoz, N. G. (2014). Diet, nesting density, and breeding success of rough-legged buzzards (Buteo lagopus) on the nenetsky ridge, arctic Russia. Polar Biol. 37, 447–457. doi: 10.1007/s00300-013-1441-2
Pokrovsky, I., Kolzsch, A., Sherub, S., Fiedler, W., Glazov, P., Kulikova, O., et al. (2021). Longer days enable higher diurnal activity for migratory birds. J. Anim. Ecol. 90, 2161–2171. doi: 10.1111/1365-2656.13484
Pravosudov, V. V., Kitaysky, A. S., and Omanska, A. (2006). The relationship between migratory behaviour, memory and the hippocampus: an intraspecific comparison. Proc. R. Soc. Biol. Sci. 273, 2641–2649. doi: 10.1098/rspb.2006.3624
Predavec, M., Krebs, C. J., Danell, K., and Hyndman, R. (2001). Cycles and synchrony in the collared lemming (Dicrostonyx groenlandicus) in arctic north america. Oecologia 126, 216–224. doi: 10.1007/s004420000516
Reed, J. M., Boulinier, T., Danchin, E., and Oring, L. W. (1999). “Informed dispersal,” in Current Ornithology, eds V. Nolan, E. D. Ketterson, and C. F. Thompson (New York, NY: Springer), 189–259. doi: 10.1007/978-1-4757-4901-4_5
Reed, J. M., and Oring, L. W. (1992). Reconnaissance for future breeding sites by spotted sandpipers. Behav. Ecol. 3, 310–317. doi: 10.1093/beheco/3.4.310
Schaub, M., and Jenni, L. (2000). Body mass of six long-distance migrant passerine species along the autumn migration route. J. Ornithol. 141, 441–460. doi: 10.1007/BF01651574
Schmidt, N. M., Ims, R. A., Høye, T. T., Gilg, O., Hansen, L. H., Hansen, J., et al. (2012). Response of an arctic predator guild to collapsing lemming cycles. Proc. R. Soc. B Biol. Sci. 279, 4417–4422. doi: 10.1098/rspb.2012.1490
Serrano, D., Tella, J. L., Forero, M. G., and Donázar, J. A. (2001). Factors affecting breeding dispersal in the facultatively colonial lesser kestrel: individual experience vs. conspecific cues. J. Anim. Ecol. 70, 568–578. doi: 10.1046/j.1365-2656.2001.00512.x
Sundell, J., Huitu, O., Henttonen, H., Kaikusalo, A., Korpimäki, E., Pietiäinen, H., et al. (2004). Large-scale spatial dynamics of vole populations in finland revealed by the breeding success of vole-eating avian predators. J. Anim. Ecol. 73, 167–178. doi: 10.1111/j.1365-2656.2004.00795.x
Tarroux, A., Berteaux, D., and Bêty, J. (2010). Northern nomads: ability for extensive movements in adult arctic foxes. Polar Biol. 33, 1021–1026. doi: 10.1007/s00300-010-0780-5
Tast, J., Kaikusalo, A., and Lagerström, M. (2010). Breeding biology of rough-legged buzzards Buteo lagopus at Kilpisjärvi, NW Finnish Lapland, in relation to rodent cycles. Kilpisjärvi Notes 22, 1–9.
Therrien, J.-F., Gauthier, G., Pinaud, D., and Bêty, J. (2014). Irruptive movements and breeding dispersal of snowy owls: a specialized predator exploiting a pulsed resource. J. Avian Biol. 45, 536–544. doi: 10.1111/jav.00426
Ward, M. P (2005). Habitat selection by dispersing yellow-headed blackbirds: evidence of prospecting and the use of public information. Oecologia 145, 650–657. doi: 10.1007/s00442-005-0179-0
Keywords: habitat selection, migration, arctic ecology, rodent cycles, rough-legged buzzard, movement ecology, prospecting movements
Citation: Curk T, Kulikova O, Fufachev I, Wikelski M, Safi K and Pokrovsky I (2022) Arctic Migratory Raptor Selects Nesting Area During the Previous Breeding Season. Front. Ecol. Evol. 10:865482. doi: 10.3389/fevo.2022.865482
Received: 29 January 2022; Accepted: 17 June 2022;
Published: 12 July 2022.
Edited by:
Guy Gilles Beauchamp, Concordia University, CanadaReviewed by:
Julien Terraube, University of the Sunshine Coast, AustraliaErica Nol, Trent University, Canada
Copyright © 2022 Curk, Kulikova, Fufachev, Wikelski, Safi and Pokrovsky. This is an open-access article distributed under the terms of the Creative Commons Attribution License (CC BY). The use, distribution or reproduction in other forums is permitted, provided the original author(s) and the copyright owner(s) are credited and that the original publication in this journal is cited, in accordance with accepted academic practice. No use, distribution or reproduction is permitted which does not comply with these terms.
*Correspondence: Teja Curk, curk@izw-berlin.de; Ivan Pokrovsky, ipokrovsky@ab.mpg.de