- 1CNRS, UMR Ecologie de Forêts de Guyane (AgroParisTech, CIRAD, INRAE, Université de Guyane, Université des Antilles), Campus Agronomique, Kourou, France
- 2CEFE, Université Montpellier, CNRS, EPHE, IRD, Montpellier, France
- 3University of Grenoble-Alpes, Laboratoire Interdisciplinaire de Physique (LIPhy), Saint-Martin-d’Hères, France
- 4Institute of Organismic and Molecular Evolution, Johannes Gutenberg University, Mainz, Germany
Competition for limited resources can yield two contrasting outcomes in community structure, namely, either (i) dominance of most competitive species (with functional convergence of the traits conferring this ability), or (ii) niche partitioning of species using distinct resources. In addition, varying resource availability in different environmental contexts is expected to yield varying community dynamics and composition between the contexts (habitat filtering). We addressed resource-based ant community structure in a tropical ecosystem. We expected ant species to display varying trophic preferences and foraging behaviors, allowing habitat selection and niche differentiation in ant assemblages. Furthermore, we expected habitat filtering to occur between open and forested areas in the landscape mosaic, and competition to further influence local species co-occurrence. We assessed resource use in nine ant assemblages distributed in two habitats (i.e., forests and croplands), devising two separate experiments using bait-traps to characterize ant species’ trophic preference (e.g., eating prey, seeds, sugars) and their ability to obtain a same resource in heterogeneous forms (e.g., on vegetation, litter, with variable amounts…). The majority of baits offered were rapidly exploited in the two habitats suggesting important resource limitations. Forest and cropland ant communities differed, however, in the proportions of resources exploited, suggesting different competitive pressures toward specific resources between habitats. Within each habitat, ants preferentially exploited the same resources, suggesting habitat filtering, but locally, interspecific resource partitioning resulted in a reduction of resource overlap compared to habitat scale. Our study provides evidence of the effects of habitat filtering and competition for resource in tropical ant community structure. Our findings also suggest that niche filtering and niche partitioning are co-variant forces determining the identity of the species present in local assemblages.
Introduction
A major goal of community ecology has been to explain the occurrence patterns of species within a region as a consequence of assembly rules (Keddy, 1992; Weiher et al., 2011; Götzenberger et al., 2012; Hille Ris Lambers et al., 2012). The expectation is that interspecific niche differences determine the suitability of species to different habitat patches as well as their interactions in local communities. Niche filtering is explained by common adaptations of species sharing a habitat, relevant for their survival and competitive performance. Particularly, species foraging effort is expected to primarily target resources and strategies providing maximal reward in a given environments (Pyke et al., 1977). Consequently, within a same habitat, species are in average more likely foraging for the same abundant/high quality resources, resulting in interspecific resource overlap (Fox and Vasseur, 2008). In absence of mechanisms regulating species’ populations (e.g., natural enemies), resources may become limiting (Wiens, 1977; Schoener, 1983). In that case, dominant species (i.e., competitively superior phenotypes) may displace other species from local assemblages acting as a biological filter. The most frequently invoked mechanism preventing competitive exclusion is interspecific niche partitioning as explained by the limiting similarity theory (MacArthur and Levins, 1967; Abrams, 1983). Indeed, species differing in their resource requirements, or in their performance to exploit different resources, are more likely to co-occur, because competition intensity is greater between ecologically redundant species than between complementary ones. Another major concern is how assembly rules operate at different organizational levels within a region. Habitat filters may favor niche overlap among species sharing a particular habitat, but similarity may be limited within assemblages as a consequence of competitive interactions (Figure 1).
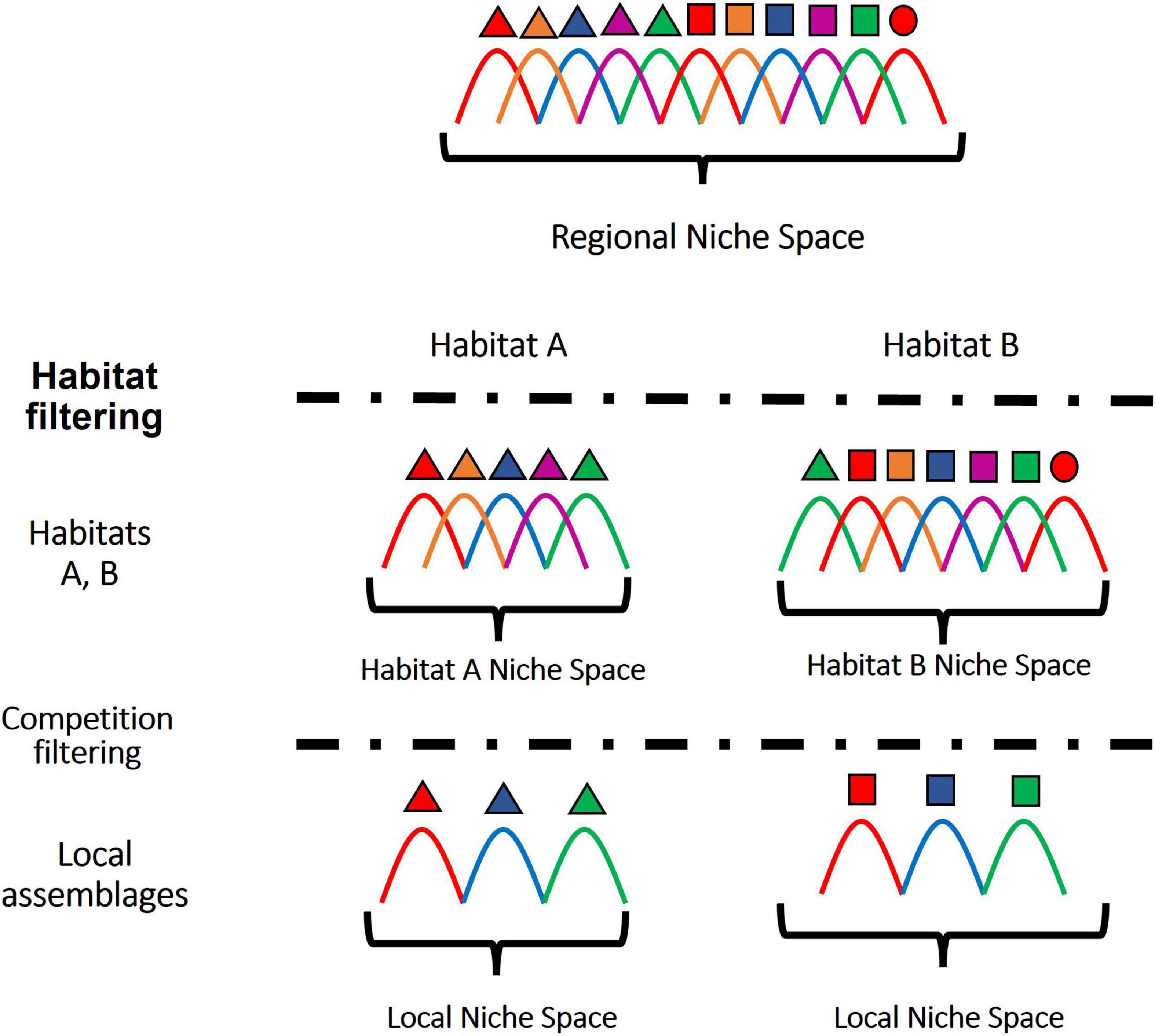
Figure 1. Habitat and competition filters simultaneously shape the niche space of local assemblages. Species are represented with different shapes and colors. Each species niche width and abundance is represented with a distribution curve positioned along an ecological gradient that represents the regional niche space. Within each habitat, only a narrower set of species is able to survive in relation to their traits. In local assemblages, competition may result in the local extinction of similar competitors, due to the limiting similarity principle, selecting for an overdispersed niche space compared to the potential niche range within that habitat.
In ants, competition has been considered the key-stone of ant assembly rules (Andersen, 1992; Davidson, 1998; Parr and Gibb, 2010). Ant abundance is huge, particularly in the tropics, where ants represent an important fraction of animal biomass (Davidson and Patrell-kim, 1996). Consequently, the foraging activity of ants for food resources is very intense, as demonstrated by many studies using baits to attract ant species (Parr and Gibb, 2010). Indeed, food baits are frequently exploited in a few hours or even minutes, and aggressive behaviors between species over food resources are frequently observed (Savolainen and Vepsäläinen, 1988; Hölldobler and Wilson, 1990; Andersen, 1992). Besides, due to the sessile nature of ant nests, the majority of ant species have a limited territory to forage for their food resources (Andersen, 1991). The intensity different resources are exploited is frequently considered as a consequence of environmental limitation in nutrient availability (Kaspari and Yanoviak, 2001; Hahn and Wheeler, 2002; Bihn et al., 2008; Peters et al., 2014). This is basically deduced from the stoichiometric ecology framework, where species require a balanced set of nutrients, and compete for the most limiting. Moreover, in addition to specific nutritional requirements, the access to nutrients is affected by how these become available in natura. For instance, the same nutrients are accessible to different species depending on whether they are contained on dead organic matter, prey, plants, or depending on microhabitat variations (Cerdá et al., 1998; McGlynn and Kirksey, 2000; Agarwal and Rastogi, 2009). In particular, the abundance of a thick litter layer or the vegetation cover, are important factors filtering different ant species (Gibb and Parr, 2013; Gibb et al., 2015; Nooten et al., 2019). Also, some ants forage on vegetation while many others search resources within the litter or foraging on the ground (Sarty et al., 2006; Brandão et al., 2012). Finally, some species are fierce defenders of food resources, while others survive by finding and exploiting faster such resources (Pearce-Duvet et al., 2011; Cerdá et al., 2013). Therefore, interspecific niche differences in nutritional requirements or in the ability to exploit different resources may be essential to explain species co-occurrence within assemblages (Luque and Reyes López, 2007; Lanan, 2014; Houadria et al., 2015). In this matter, a special place in ant community assembly has been attributed to dominant species. Indeed, some ant species excel at exploiting very important amounts of resources and aggressively displace competitors affecting the structure of assemblages (Andersen, 1992; Holway, 1999; Dejean et al., 2007; Parr, 2008). This may lead to intraspecific resource use variations depending on the outcomes of competition (Savolainen and Vepsäläinen, 1988; Sanders and Gordon, 2003; Blüthgen and Fiedler, 2004). Such competitive outputs may be influenced by the abundance of different microhabitats and resources affecting the foraging conditions and available resources (Fowler et al., 2014; Ipser and Gardner, 2020).
In this study we investigated interspecific niche partitioning in ant assemblages in relation to habitat condition and hypothesized that local assembly results from niche processes related to the use of food resources. We assessed ant occurrence patterns at food baits in nine ant assemblages in two habitat types within the same region. In two separate experiments, we devised two major dimensions of niche partitioning, namely, trophic differences and resource acquisition strategies. The first can be affected by the nutritional requirements of different species as well as ant ability to handle different food types. Resource acquisition strategies refer to the ability of ant species to exploit a same type of resource in different displays representing the heterogeneous access to food resources in the environment. First, we assessed and compared the exploitation intensity of different food resources within assemblages. The objective here was to confirm that resource limitations exist, as a preliminary condition to hypothesize that competition may be important; and to provide evidence of resource limitation differences between the two habitats to support habitat filtering. Second, we investigated intraspecific niche differences among assemblages, and interspecific niche differences within them. We examined general ant assemblages and particularly dominant species (i.e., those exploiting the largest fraction of resources). The aim was again two-fold, to confirm that intraspecific resource use is not contingent to local conditions, and that niche differences at intraspecific level are lower than between species, as expected from the limiting similarity theory. Finally, we tested whether resource-based filtering existed at the habitat level, and at the assemblage level. We expected a significant niche overlap among species sharing a habitat, but this can be reversed at the assemblage level due to the effects of competition, in which case, the strength of the filtering should be lower than at the habitat level.
Materials and Methods
Study Site and Sampling Scheme
The study area is located in the coastal part of French Guiana at La Montagne des Singes (5°04’19”N; 52°41’42”W). In total, nine plots were sampled in two habitat types: five forests and four slash-and-burn croplands. Forests presented mixed vegetation representative of rainforests of the facies Fabaceae, Chrysobalanaceae, Lecythidaceae of the coastal part of French Guiana. Croplands consisted of traditional young mixed crops (3–5 years) of different plant species, mainly manioc, pineapple, sugar cane, pepper, and fruit trees. The two habitats were selected to represent contrasting environmental conditions regarding habitat complexity, litter density, shade, and disturbance regularity, resulting in an almost full turnover of ant community composition across habitats (personal observation). Still, assemblages within the same habitat type shared an important fraction of species. Plots consisted of 20 sampling locations separated by 10 m were set up on a rectangular grid (1200 m2). This sample size was chosen to represent local assemblages, and the number of points based on a recommended minimum for characterizing ant communities (Agosti and Alonso, 2000). A similar display in previous experiments dealing with trophic differentiation of ants showed, that this number of samples adequately captured the largest fraction of ants foraging for food resources on the ground (Houadria et al., 2015; Salas-López et al., 2017). A distance of at least 250 m was left between the plots, and all of them were situated within a radius of 3 km and relatively interspersed [described in Salas-López et al. (2017, 2018)]. The distance between plots is enough to limit autocorrelation, and the plots are still not too far away to prevent large-scale environmental variation, and we could consider a single, consistent pool of species. Sampling was carried out between March and October 2013, alternating croplands and forests in dates, always in the morning (8:00–11:00 a.m.) and only in dry conditions (i.e., in the absence of rain and/or flooding) to avoid, as much as possible, sampling biases linked to soil conditions and thermal stress.
Ant Communities and Foraging Strategies
The sampling scheme enabled to obtain at the same time data about community composition, and interspecific niche differences.
First, we devised a food type experiment (hereafter referred as trophic) to investigate ant foraging behavior depending on the nature of the resource. Seven different food items representing some of the most frequently available sources of essential nutritional requirements for ants, including carbohydrates, proteins or lipids [see Houadria et al. (2015)]. Sucrose and melezitose constitute the main sugar sources for ants obtained from plant nectar and sap-sucking insects, respectively (3 ml 25% w/w dropped onto paper toweling). Insects constitute the main source of proteins and lipids for most ant species; however, insects can be differently accessible depending on their size or whether they are dead or alive. We offered dead insects (3 g of dead, crushed Tenebrio molitor mealworms), small living prey (at least 20 Anoplotermes sp. termites) and large living prey (two, living 1.5 and 3 cm-long mealworms). Seeds were offered as a mixture of peanuts and rice to represent a highly lipidic and a mainly starch composed seed. Finally, excrements are exploited by some species, and therefore we used bird droppings (3 g of chicken excrement).
Second, we devised a resource heterogeneity experiment (hereafter referred as acquisition strategies) to investigate ant foraging behavior depending on the location of the resource. We offered dead insects (i.e., the most attractive resource considering the number of ant genera attracted and of recruited individuals in the first experiment) in different bait displays (Figure 2). These baits were presented as a standard resource on the leaf litter (3 g of dead Tenebrio molitor as in the previous experiment), as interstitial litter resource [a plastic container where an open Eppendorf containing the resource was placed inside containing with six 0.5 cm diameter holes providing access to the ants; e.g., Sarty et al. (2006)], as a resource on vegetation (presented in an open Eppendorf which was tacked to the trunks of trees at a height of 1.3 m; Kaspari and Yanoviak, 2001), as small particles (close to 0.1 g of dried mealworms ground into powder; McGlynn and Kirksey, 2000), and rapid discovery (3 g of DT were placed in Petri dishes, surveyed every 5 min, and then closed as soon as any ant activity was observed; Pearce-Duvet et al., 2011).
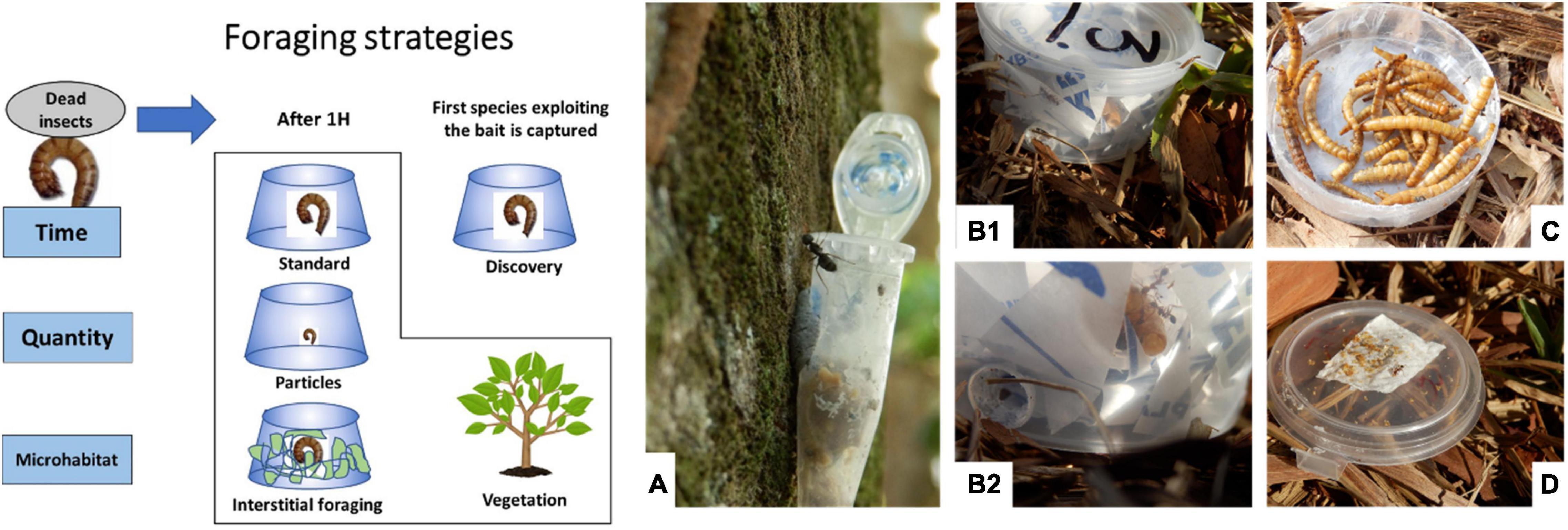
Figure 2. Foraging strategies sampling protocol. The left side of the figure illustrates which environmental factors and niche partitioning dimensions were aimed. At the right, the sampling devices used to emulate different resource acquisition strategies. (A) Vegetation foraging. (B1,B2) Interstitial foraging. (C) The standard bait display also used for the trophic experiment, and applied for both discovery and dominance, and (D) the small particles.
With the exception of the rapid discovery bait, the other bait traps were surveyed every 5–10 min to monitor ant activity (i.e., the species consuming the items were noted), and collected after 1 h. After each sampling session, the bait traps were closed to capture the ants. The traps were then taken to the laboratory, and the specimens were killed by freezing at −20°C. The ant specimens were then counted and sorted to genera using the identification key by Bolton (2003) and then to morphospecies based on morphological differences. A reference collection was built and can be found at the UMR Ecofog, Kourou, France.
Statistical Tests
We used incidence data for analyses (e.g., the number of times each of the species was found at a given bait type for an observation scale considered). Within each assemblage, a species can be present as much as 20 times for a given resource (i.e., the number of sampling locations). The trophic experiment enabled the capture of a total of 81 species in the forest habitat and 26 in the cropland habitat. In the acquisition strategies experiment, 66 species were captured in the forest habitat and 15 in the cropland habitat. Species captured in five or more occasions were retained for analyses. This was the minimal information to discriminate differences in the resource use patterns between two species according to the Chi-square (χ2) test (McCrum-Gardner, 2008). All the tests were performed separately for the two data sets (trophic experiment and resource acquisition). The statistical tests used consist on standard procedures suitable to investigate niche patterns on incidence data in discrete resource categories (Gotelli and Graves, 1996).
Resource Limitations
We first assessed the intensity of exploitation of different food types (using the trophic experiment data) as the percentage of baits exploited and the average number of species exploiting baits in the lapse of an hour. To test whether resource limitations differed between habitats and among assemblages, the incidence data of all species was summed up to account for exploitation intensity of the different resources. Then a contingency test based on the Chi2 distribution was conducted (here after proportionality test). For each habitat type, the algorithm generated random incidence data by keeping constant the sums in the rows (assemblages/habitat) and columns (resource types). A significant result in the test indicates that resources were consumed in different proportions between the elements compared.
Species Level Resource Use Differences
We characterized ant niches based on their incidence on different resource categories. Then intraspecific and interspecific resource use variations were assessed using the proportionality test. Intraspecific resource use variations were examined on species sufficiently represented at different assemblages (i.e., 10 or more incidences were found for at least three plots). Based on these conditions, a total of 24 tests were performed, where rows were assemblages, and resource categories were columns. Then, interspecific niche differences were investigated within assemblages. Only species with an incidence of five or more were retained for analyses. Rows were species and columns resources. In keeping with the limiting similarity principle, we expected interspecific differences within assemblages but not intraspecific resource use variations. Moreover, the finding of intraspecific level variations within a same habitat would compromise the interpretation of species suitability to different environments as a consequence of their resource use, as well as its competitive effect on other species.
Niche Overlap at the Habitat and the Assemblage Levels
We assessed the extent of habitat filtering among ant species within communities and habitats, based on the null expectation that species can use random proportions of resources (Gotelli and Graves, 1996). We used Ecosim routines of niche overlap implemented in R, at the habitat level first, and then on the different assemblages. The options RA3 algorithm and the Pianka overlap index were chosen as the most frequent procedure for resource overlap hypothesis (Gotelli and Entsminger, 2001). Observed niche overlap in different levels of organization was compared to the null distributions using a two-tailed statistical test. Any deviation from the null models indicated an influence of niche variation among species. Values lower than the 2.5% quantile indicated that species differ more in their resource use than expected by chance. Values over the 97.5% indicated more similarity than expected by chance. In addition, we calculated the standard effect size (SES) of observed overlap values. The absolute values of SES represent the standardized deviation from the null distribution. SES was particularly useful to compare resource use overlap patterns at the habitat and the assemblage levels.
Multiple Comparison Correction
To deal with the problem of the multiple comparisons, we used the method of false discovery rates (FDR), and the algorithms described in Pike (2011). This post-hoc method is based on the evaluation of the distribution of P-values to evaluate their signification, instead of a normative reduction of the confidence interval in more standard methods (e.g., Bonferroni). The key benefit of these methods is that they are much more powerful than Bonferroni-type comparisons to determine which results are true positives. In addition, they are also capable of detecting false negatives. In the presentation of our data, we maintain the P-values provided by the original statistical tests, and only report FDR results when discordance was found.
Ordinations
To identify the major components of species niche differences within habitats, we performed Principal Component Analyses using the software Past 3.0 (Hammer et al., 2001). Species of both habitats were ordinated together in a resource space, where the components were the different resources projected. Only species presenting an incidence of five or more at the habitat level were retained for the ordination. Accordingly, in the trophic experiment, 47 and 15 species were retained for forests and croplands, respectively and 40 and 12 in the resource acquisition experiment. Dominant species were considered apart. We chose those exploiting together the 75% or more of resources at the habitat level, which distinguished 7 species in the forest habitat and 3 species in the cropland habitat. These species are expected to reflect the habitat effect more clearly in favoring specific ecological strategies but also competition effects on ecological similarity.
Species and principal components were normalized prior to ordination, to avoid inertia biases related to differences in the exploitation intensity of different resources or related to species abundances. The niche space occupied by the ants in each habitat, and by dominant ants, was delimited with convex hulls. These join the peripheral points of the ecological volume of a specified group, and are frequently related to ecological filtering (Cornwell et al., 2006).
Results
Resource Limitations
The percentage of baits exploited was very high for most resources. Particularly in just 1 h, the great majority of baits containing dead insects, sugars, seeds, and termites were exploited, frequently, by several species simultaneously (Table 1). Despite the lower species diversity in croplands at assemblage and habitat level, the average number of species per bait was similar than in forests.
We found no differences in the exploitation intensity of different food resources among assemblages of the same habitat type, neither for the trophic experiment nor foraging strategies according to the proportionality test. Significant differences were found, however, between forests and croplands (χ2 = 85.23, P < 0.001) supporting the idea that the ants in each habitat differed in their resource requirements. Additionally, the Principal Component Analysis (Figure 3) illustrated that the dominant species of each habitat type consumed relatively different resources. Species in croplands, occupied a more central place, and consumed insects in several forms, while forest species presented a greater tendency to consume sugars and seeds. In contrast, no differences were found in the intensity of resource acquisition strategies by dominant species between habitats despite the important difference in the niche space delimited by the convex hulls in these two habitats when considering the whole pool of species. The absence of differences can be explained, however, because the reduced acquisition strategies niche space observed in croplands constitute a subassembly of those observed in forests by both dominants and subordinate ants.
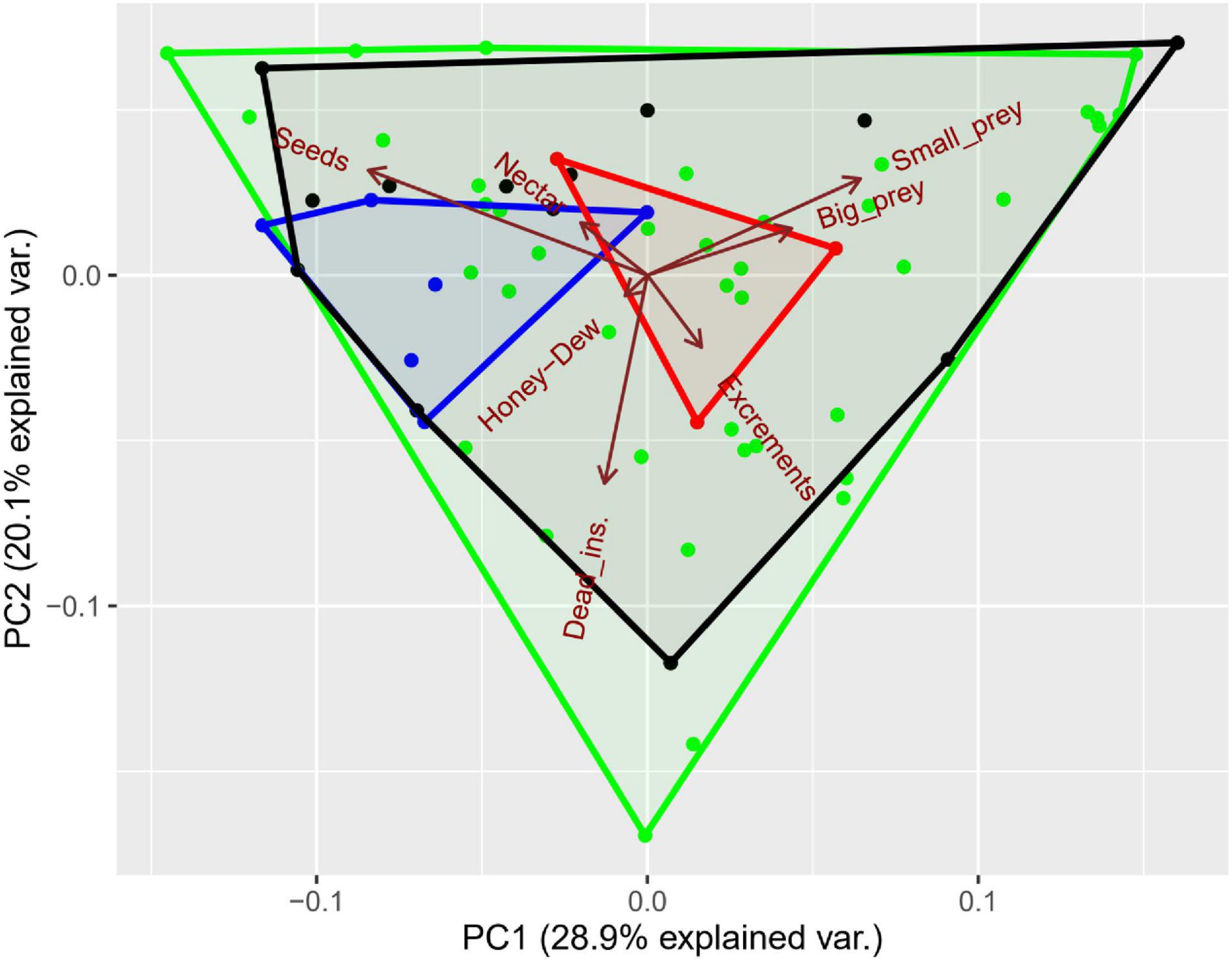
Figure 3. Principal Component Analysis of the trophic niche space. The first and second axes represent the 28.9% and 20% of the variance, respectively. Dots and crosses correspond to forest and cropland species, respectively, distributed along resource axes. Convex hulls encompass four groups of species: in red and blue are dominant species in croplands and forests, respectively. In black and green are the remaining species for croplands and forests. The food types are indicated as follows: termites (Small), mealworms (Large), excrements (Excrements), dead mealworms (Dead), melezitose (Honey-dew), sugar (Nectar), and Seeds.
Species Level Resource Use Differences
Variations in resource use patterns at the intraspecific level were extremely rare for the two experiments performed. Out of a total of 24 proportionality tests (Table 2), significant differences were only found in the means of the resource acquisition of Nylanderia sp.1. This result disappeared after applying a correction for multiple comparisons (FDR adjusted-P = 0.16). The absence of intraspecific resource use variations provides robust evidence of the constancy of the species’ response to resource limitations and effect of other species.
Interspecific trophic partitioning was found in all the assemblages after applying multiple comparison corrections (Table 3). These patterns were also suggested by the wide trophic space delimited with the convex hulls in the Principal Component Analysis (PCA) in both habitats (Figure 3). Trophic partitioning was also important among dominant species in all the cropland assemblages, but only in a 40% of the cases in forest assemblages (Table 3). These patterns are also reflected by the PCA, which reveals that the three species dominant in croplands were distantly positioned from one another (Figure 3). Conversely, forest dominant species were very closely positioned in the trophic space, and only one of them appears as relatively different to the others exploiting insects with greater frequency (Figure 3).
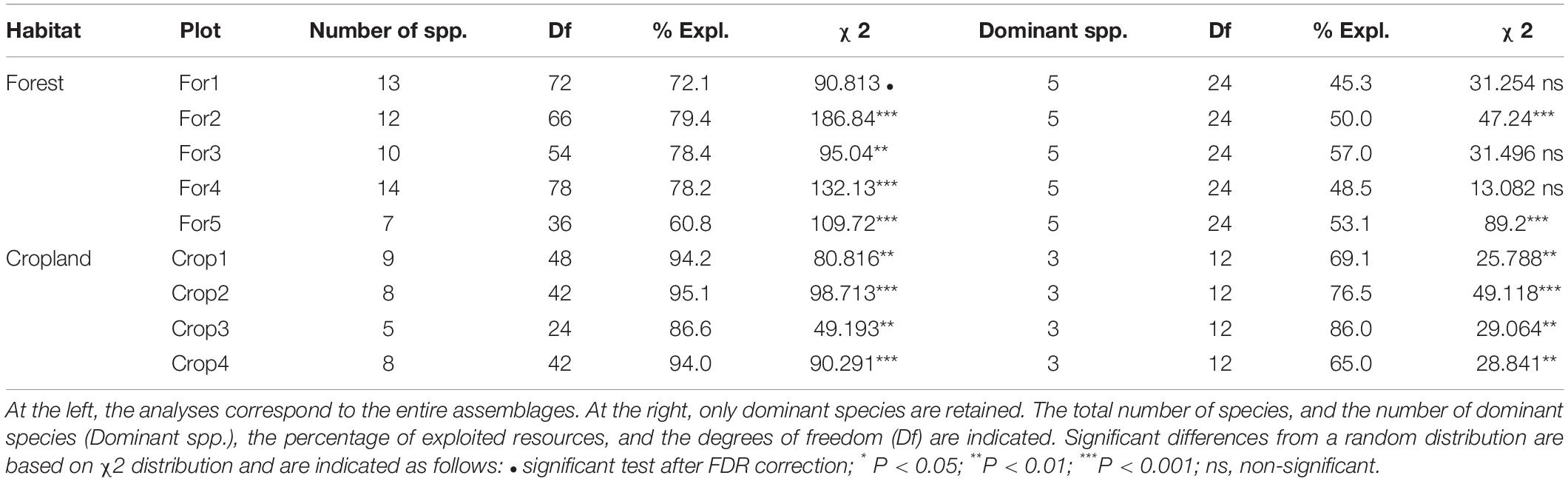
Table 3. Contingency tests calculated on trophic partitioning matrices on five forest and four cropland assemblages.
Partitioning in resource acquisition strategies was detected in all the forest assemblages after applying multiple comparisons correction, but in only one of the cropland assemblages (Table 4). The number dropped to four (80%) in forest assemblages when only the dominant species were considered and remained equal (25%) for croplands. Indeed, it can be observed (Figure 4) that species in forest habitat present a greater diversity of foraging preferences in relation to resource heterogeneity, and particularly dominant species which were quite evenly distributed covering an important fraction of this volume.
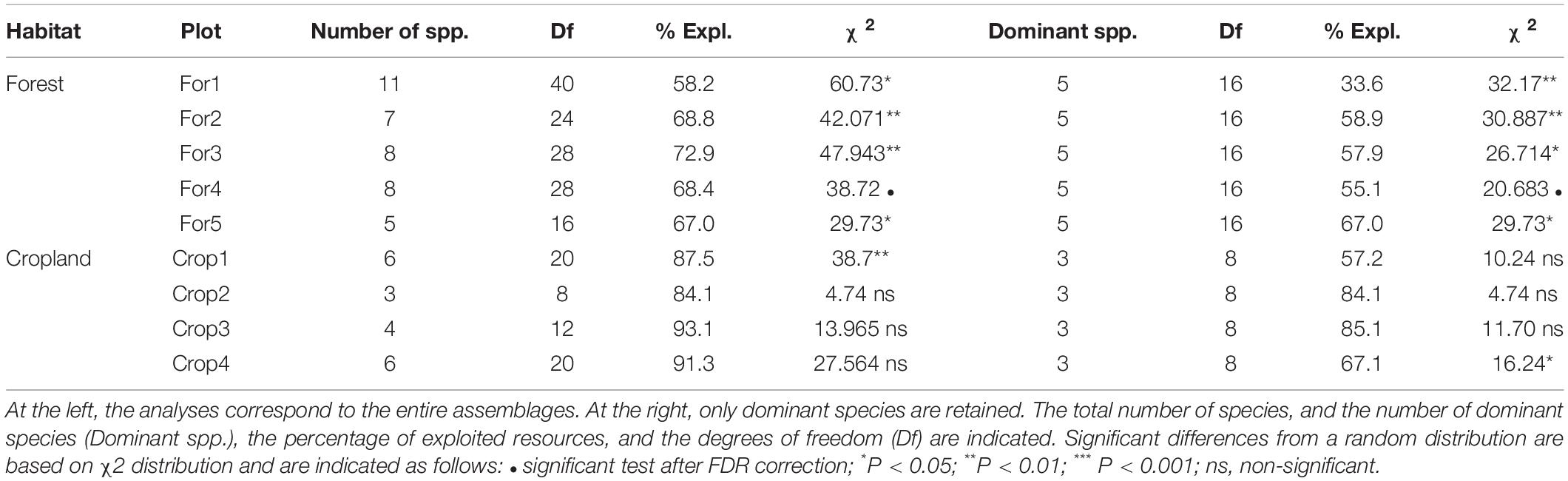
Table 4. Contingency tests calculated on acquisition strategy partitioning matrices on five forest and four cropland assemblages.
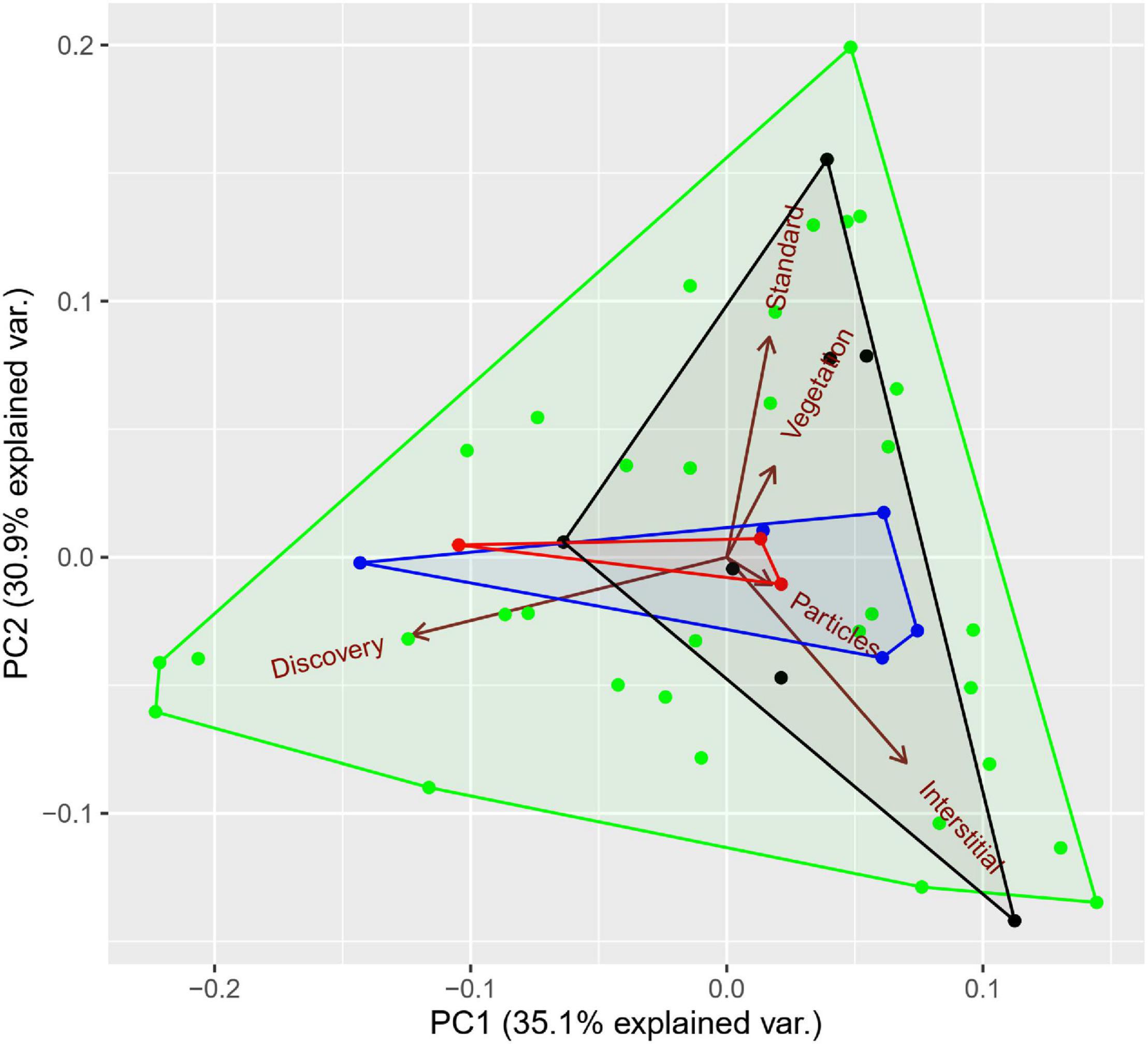
Figure 4. Principal Component Analysis of the acquisition strategies niche space. The first and second axes represent the 35 and 31% of the variance, respectively. Dots and crosses correspond to forest and cropland species respectively distributed along resource axes. Convex hulls encompass four groups of species: in red and blue are dominant species in croplands and forests, respectively. In black and green are the remaining species for croplands and forests. The abbreviations correspond to acquisition strategies as follows: Standard, standard reference bait; Interstitial, litter-surrounded bait; Vegetation, baits placed on vegetation; Particles, small amounts; Discovery, rapid exploitation.
High Niche Overlap at the Habitat Level, Decreases in Local Assemblages
At the habitat scale (pooled assemblages), the observed trophic overlap was greater than expected by chance for forests (Piankaobs = 0.54 vs. Piankanull = 0.50; P < 0.001) and croplands (Piankaobs = 0.65 vs. Piankanull = 0.57; P < 0.001) (Table 5). This indicates that some types of resources were more frequently exploited than others by most of the species. At the assemblage level, trophic overlap was also larger than expected by chance in four of the five forest assemblages (Table 5), but only in two of the cropland assemblages. Indeed, in croplands, the degree of deviation from the null expectation was considerably larger at the habitat scale than for the average assemblage (SESHabitat = 5.53 vs. a local mean of 1.39). The same result was observed in forest habitat compared to local assemblages (SESHabitat = 6.83 vs. a local mean of 2.73).
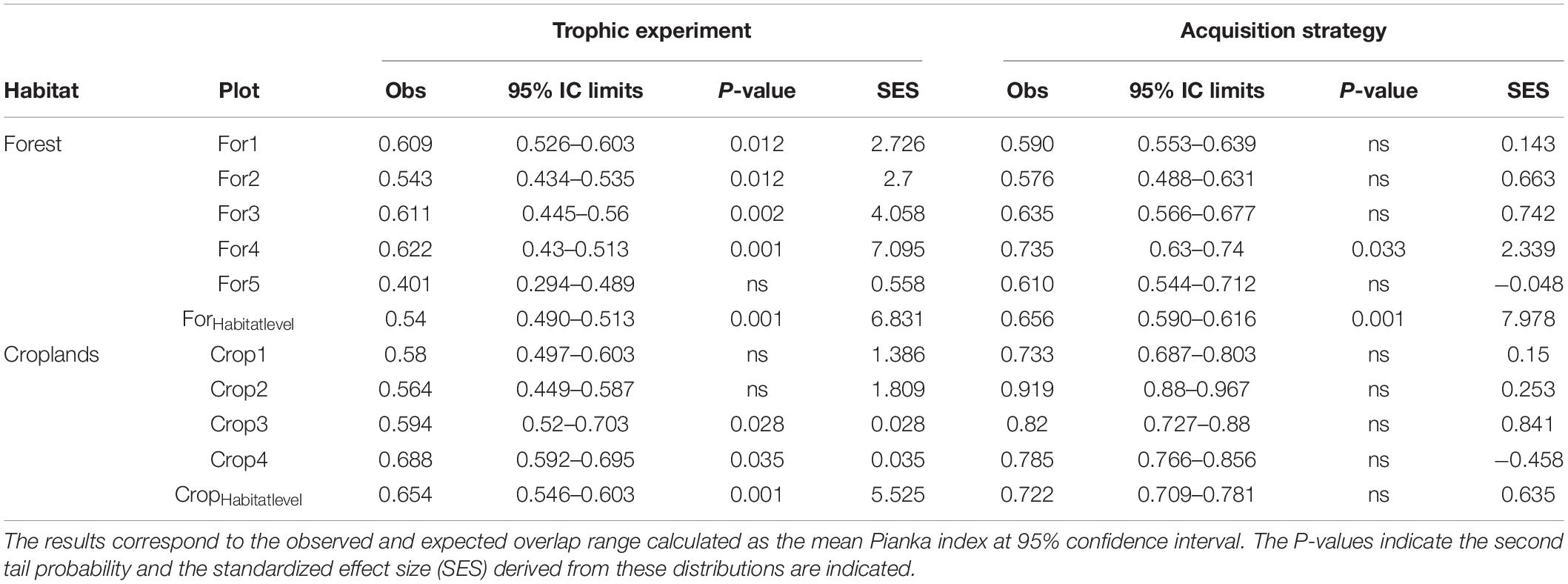
Table 5. Observed and expected range of trophic niche overlap measured at assemblage and habitat level.
In the acquisition strategies experiment, forest species overlapped more than expected by chance in the displays used at the habitat scale (Piankaobs = 0.66 vs. Piankanull = 0.60, P < 0.001), but this pattern was only maintained in one of the local assemblages (Table 5). Accordingly, the standardized effect size at the habitat scale was more than three times larger than in any of the local assemblages. In the croplands, the species presented a random pattern of resource use at the habitat and assemblage scales (Table 5).
Discussion
In this work we investigated the patterns of resource use in nine ant assemblages in two contrasted habitats. We used two different experiments representing important dimensions of niche partitioning, namely food type and resource acquisition strategies. In all the assemblages studied we found intense ant activity at food baits, which suggested resource limitations. Also, interspecific niche differences were found in all the habitats studied. Finally, species associated to forests and to croplands used more frequently the same resources and/or resource displays at the habitat level as well as at the assemblage level, suggesting niche filtering for each of these habitats. While we found filtering and partitioning in forests and croplands, they affected different ecological dimensions. We try to explain the observed patterns as a consequence of habitat filters and competitive interactions.
Prior to interpretations of assembly patterns, we investigated whether resource use intensity was a consistent property of individual species and entire assemblages. This was confirmed by each of the species examined displaying similar resource exploitation patterns in the different assemblages where they were present. Also, the characteristic assemblages of ants associated to forests and croplands respectively, presented specific patterns of resource exploitation that were consistent between assemblages of the same habitat. Because we are using resource use patterns to characterize the niche of species, as well as an indicator of resource limitations between assemblages, it seems necessary to confirm that resource use proportions are constant for a given species within assemblages of the same type. For example, different resource limitations may alter species competitive outputs (Kaspari et al., 2012; Correa and Winemiller, 2014; Nooten et al., 2019). And also, intraspecific resource use variations have been reported in response to environmental and competition effects (Savolainen and Vepsäläinen, 1988; Pfeiffer et al., 2014).
In our study, the intensity food resources were exploited differed between forests and croplands. An important trophic overlap was found in both habitats, although for different resources. Particularly, dominant species in forests consumed more sugars and seeds, while the dominant species in croplands consumed relatively more insects and appeared as more generalized in their trophic preferences. Such differences may be explained by environmental differences in resource availability between the two habitat types. For instance, a greater use intensity of proteinaceous resources in cropland vs. forests was found in previous studies (Bihn et al., 2008; Peters et al., 2014). Moreover, different resource limitations between environments may filter different species in relation to their ability to use the available forms of resources (McKane et al., 2002; Kaspari et al., 2012; Fowler et al., 2014). In addition, species in forest assemblages presented an important overlap in their acquisition strategies at the habitat level, but also occupied a large niche space indicating an important diversity of strategies in this habitat. This contrasted with cropland ants, for which niche overlap was not found and the niche space occupied was quite small. These findings may be explained by the denser litter and plant diversity found in forest. Microhabitat heterogeneity provides a wide range of spatio-temporal foraging possibilities not present in croplands, enabling niche partitioning and selection of different foraging strategies by forest ants.
Ants in the studied assemblages were likely structured, at least in part, by competition. This claim is frequently controverted, but we report four findings that together suggest an important effect of biotic filters in ant community structure. In first place, the majority of baits were rapidly exploited, often, by several species, supporting resource limitations, which is the essential driver of competition. Secondly, we confirmed that intraspecific level variations of resource use in different assemblages were unimportant in comparison to interspecific variations within assemblages. This is the most basic assumption of the limiting similarity prediction: species are more limited by conspecifics than by other species (Abrams, 1983). In third place, niche partitioning was the rule in the studied assemblages, in at least one of the two ecological dimensions considered (trophic partitioning and foraging strategies). Finally, the finding of interspecific niche differences is no proof of competition, so it must be evidenced that partitioning indeed relates to community structure (Connell, 1980; Cadotte and Tucker, 2017). Congruently, we found that when significant niche overlap was found at the habitat level, the average values of niche overlap were lower at the assemblage level. This finding suggest that local assemblages more likely contained subsets of species foraging for different resources, among the set of possible species from the habitat pool suitable for that habitat type (Levine and Hille Ris Lambers, 2009; Kraft and Ackerly, 2010). Likewise, resource dominance in the different local assemblages was shared by species belonging to a diversity of genera. This is important, because phylogenetic niche conservatism is an important factor explaining habitat filtering and niche partitioning (Andersen, 1995; Losos, 2008; Salas-López, 2017). In forests, every ant assemblage contained Ochetomyrmex neopolitus, Wasmannia auropunctata, and a Solenopsis species, as well as one or two dominant Pheidole species and a dominant Crematogaster species. Likewise, three ant species dominated croplands: Solenopsis saevissima, Pheidole (cf. fallax), and a Crematogaster species. Other traits such as the number of workers and soldiers captured were recorded but will be studied elsewhere in addition to trophic and morphological traits.
Another striking finding was that habitat filtering and niche partitioning were more intense for the same combination of habitat and ecological dimension. For example, the greatest overlap was found in acquisition strategies of forest ants at the habitat level, suggesting an important competition for some strategies. This finding contrasted however, with the absence of overlap in the majority of forest assemblages, together with the finding of niche partitioning in acquisition strategies in all of these assemblages. Additionally, by partitioning on acquisition strategies, forest dominant ants may be less constrained by their trophic similarity than cropland ants, as was also reported in a previous study (Jacquemin et al., 2012). Furthermore, neither niche overlap nor partitioning was found in acquisition strategies in croplands, suggesting that in general, species were unspecialized to use a particular resource or another. These findings suggest that interspecific niche partitioning was more important when important fractions of resources were shared among species. Our explanation is that, if adaptation to a specific set of conditions is important for the survival of species within a specific habitat, it is likely that related functional traits, will be selected, promoting species filtering and, therefore, niche overlap. Yet, competition may select for the maximal differences within the range of conditions and/or resources available in that habitat. This is supported by the central place that dominant species held in the PCAs compared to the overall niche space, and yet their important interspecific differences. Another related finding was that, despite important differences in species richness between habitats, the average number of species at baits was similar. We believe that for a given area, even if the number of species is greater in forest habitats, the competitive pressure for limiting resources can only allow a certain number of ant competitors to stand the presence of other species at a given bait. We have no quantitative evidence to confirm this, but we suggest that future studies reporting the number of ant species discovering a bait in the lapse of an hour would greatly help us to understand this pattern.
In combination, the presented results provide a strong support of the relevance of habitat filtering and competition in structuring ant assemblages. Resource availabilities in different habitats may have resulted in the filtering of species with similar ecological traits, but at the assemblage level, limiting similarity may result in non-overlapping or, at least, less overlapping assemblages (Ackerly and Cornwell, 2007; Algar et al., 2011). Finally, we could observe, a functional homogenization in agricultural-forest gradients as recurrent in many studies concerning ants and other organisms (Bihn et al., 2010; Clavel et al., 2011; Peters et al., 2014).
Conclusion
We provide evidence of the relevance of habitat and niche differentiation in resource use patterns of species and their interplay in community structure. Our study joins more and more studies providing similar evidence (Ackerly and Cornwell, 2007; Gilbert et al., 2008; Kraft and Ackerly, 2010; Algar et al., 2011). Niche overlap and partitioning are simultaneous covariant forces of community structure, since species must compete to exploit the highest quality resources within their habitats while preventing the depletion of identical sources. For a given habitat, niche overlap is expected in all its extension, but the limits of such overlap depend on the competitive exclusion of redundant competitors in local assemblages. We thus recommend the separation of overlap and partitioning hypotheses in different ecological dimensions while trying to understand assembly processes, as well as in considering the resource limitations in different environments (Fox and Vasseur, 2008; Correa and Winemiller, 2014).
Data Availability Statement
The original contributions presented in this study are included in the article/supplementary material, further inquiries can be directed to the corresponding author.
Author Contributions
AS-L, JO, and FMe presented the idea. AS-L carried out the experiment, identified the biological material, and wrote the main version of the manuscript. AS-L, CV, and FMu developed the theory and performed the statistical analyses. All authors contributed to the final version of the manuscript.
Funding
Financial support for this study was provided by a Ph.D. fellowship from the LabEx CEBA (Centre d’Etude de la Biodiversité Amazonienne) and the Fond Social Européen (FSE) to AS-L, and by an “Investissement d’Avenir” grant managed by the Agence Nationale de la Recherche (CEBA, ref. ANR-10- LABX-25- 01).
Conflict of Interest
The authors declare that the research was conducted in the absence of any commercial or financial relationships that could be construed as a potential conflict of interest.
Publisher’s Note
All claims expressed in this article are solely those of the authors and do not necessarily represent those of their affiliated organizations, or those of the publisher, the editors and the reviewers. Any product that may be evaluated in this article, or claim that may be made by its manufacturer, is not guaranteed or endorsed by the publisher.
Acknowledgments
We are most grateful to the landowners who generously allowed us to carry out the experiments on their properties, to Julian Frederick, Pierre Freyre, Isabelle Kozon, and Jean-Romain Roussel for field assistance and to Andrea Dejean for proofreading this manuscript.
References
Abrams, P. (1983). The theory of limiting similarity. Annu. Rev. Ecol. Syst. 14, 359–376. doi: 10.1146/annurev.es.14.110183.002043
Ackerly, D. D., and Cornwell, W. K. (2007). A trait-based approach to community assembly: partitioning of species trait values into within- and among-community components. Ecol. Lett. 10, 135–145. doi: 10.1111/j.1461-0248.2006.01006.x
Agarwal, V. M., and Rastogi, N. (2009). Food resource and temporal partitioning amongst a guild of predatory agroecosystem - inhabiting ant species. Curr. Zool. 55, 366–375.
Agosti, D., and Alonso, L. E. (2000). “The ALL protocol: a standard protocol for the collection of ground-dwelling ants,” in Ants: Standard Methods for Measuring and Monitoring Biodiversity, eds T. R. S. D. Agosti and J. D. Majer (Washington DC: Smithsonian Institution Press), 204–206.
Algar, A. C., Kerr, J. T., and Currie, D. J. (2011). Quantifying the importance of regional and local filters for community trait structure in tropical and temperate zones. Ecology 92, 903–914. doi: 10.1890/10-0606.1
Andersen, A. N. (1991). “Parallels between ants and plants: implications for community ecology,” in Ant-plant interactions, eds C. R. Huxley and D. F. Cutler (Oxford: Oxford University Press), 539–553.
Andersen, A. N. (1992). Regulation of “momentary” diversity by dominant species in exceptionally rich ant communities of the Australian seasonal tropics. Am. Nat. 140, 401–420. doi: 10.1086/285419
Andersen, A. N. (1995). A classification of australian ant communities, based on functional groups which parallel plant life-forms in relation to stress and disturbance. J. Biogeogr. 22, 15–29. doi: 10.2307/2846070
Bihn, J. H., Gebauer, G., and Brandl, R. (2010). Loss of functional diversity of ant assemblages in secondary tropical forests. Ecology 91, 782–792. doi: 10.1890/08-1276.1
Bihn, J. H., Verhaagh, M., and Brandl, R. (2008). Ecological stoichiometry along a gradient of forest succession: bait preferences of litter ants. Biotropica 40, 597–599. doi: 10.1111/j.1744-7429.2008.00423.x
Blüthgen, N., and Fiedler, K. (2004). Preferences for sugars and amino acids and their conditionality in a diverse nectar-feeding ant community. J. Anim. Ecol. 73, 155–166. doi: 10.1111/j.1365-2656.2004.00789.x
Bolton, B. (2003). Synopsis and classification of formicidae. Memoirs Am. Entomol. Institute 71, 1–370.
Brandão, C., Silva, R., and Delabie, J. (2012). “Neotropical ants (Hymenoptera) functional groups: nutritional and applied implications,” in Insect Bioecology and Nutrition for Integrated Pest Management, eds A. R. Panizzi and J. R. P. Parra (Boca Raton, FL: CRC Press, Taylor & Francis group), 213–236. doi: 10.1201/b11713-13
Cadotte, M. W., and Tucker, C. M. (2017). Should environmental filtering be abandoned? Trends Ecol. Evol. 32, 429–437. doi: 10.1016/J.TREE.2017.03.004
Cerdá, X., Arnan, X., and Retana, J. (2013). Is competition a significant hallmark of ant (Hymenoptera: Formicidae) ecology? Myrmecol. News 18, 131–147.
Cerdá, X., Retana, J., and Cros, S. (1998). Prey size reverses the outcome of interference interactions of scavenger ants. Oikos 82, 99–110. doi: 10.2307/3546920
Clavel, J., Julliard, R., and Devictor, V. (2011). Worldwide decline of specialist species: toward a global functional homogenization? Front. Ecol. Environ. 9:80216. doi: 10.1890/080216
Connell, J. H. (1980). Diversity and the coevolution of competitors, or the ghost of competition past. Oikos 35, 131–138. doi: 10.2307/3544421
Cornwell, W. K., Schwilk, D. W., and Ackerly, D. D. (2006). A trait-based test for habitat filtering: convex hull volume. Ecology 87, 1465–1471. doi: 10.1890/0012-9658(2006)87[1465:attfhf]2.0.co;2
Correa, S. B., and Winemiller, K. O. (2014). Niche partitioning among frugivorous fishes in response to fluctuating resources in the Amazonian floodplain forest. Ecology 95, 210–224. doi: 10.1890/13-0393.1
Davidson, D. W. (1998). Resource discovery versus resource domination in ants: a functional mechanism for breaking the trade-off. Ecol. Entomol. 23, 484–490. doi: 10.1046/j.1365-2311.1998.00145.x
Davidson, D. W., and Patrell-kim, L. (1996). “Tropical arboreal ants: why so abundant?,” in Neotropical Biodiversity and Conservation, ed. A. C. Gibson (Los Angeles, CA: Mildred E. Mathias Botanical Garden, University of California press), 127–140.
Dejean, A., Corbara, B., Orivel, J., and Leponce, M. (2007). Rainforest canopy ants: the implications of territoriality and predatory behavior. Funct. Ecosyst. Commun. 1, 105–120.
Fowler, D., Lessard, J. P., and Sanders, N. J. (2014). Niche filtering rather than partitioning shapes the structure of temperate forest ant communities. J. Anim. Ecol. 83, 943–952. doi: 10.1111/1365-2656.12188
Fox, J. W., and Vasseur, D. A. (2008). Character convergence under competition for nutritionally essential resources. Am. Nat. 172, 667–680. doi: 10.1086/591689
Gibb, H., and Parr, C. L. (2013). Does structural complexity determine the morphology of assemblages? an experimental test on three continents. PLoS One 8:e64005. doi: 10.1371/journal.pone.0064005
Gibb, H., Stoklosa, J., Warton, D. I., Brown, A. M., Andrew, N. R., and Cunningham, S. A. (2015). Does morphology predict trophic position and habitat use of ant species and assemblages? Oecologia 177, 519–531. doi: 10.1007/s00442-014-3101-3109
Gilbert, B., Srivastava, D. S., and Kirby, K. R. (2008). Niche partitioning at multiple scales facilitates coexistence among mosquito larvae. Oikos 117, 944–950. doi: 10.1111/j.0030-1299.2008.16300.x
Gotelli, N. J., and Entsminger, G. L. (2001). EcoSim: Null Models Software for Ecology. Version 7.0.
Gotelli, N. J., and Graves, G. R. (1996). Null Models in Ecology. Washington D.C: Smithsonian Institution Press, doi: 10.1007/BF01199989
Götzenberger, L., de Bello, F., Bråthen, K. A., Davison, J., Dubuis, A., Guisan, A., et al. (2012). Ecological assembly rules in plant communities-approaches, patterns and prospects. Biol. Rev. 87, 111–127. doi: 10.1111/j.1469-185X.2011.00187.x
Hahn, D. A., and Wheeler, D. E. (2002). Seasonal foraging activity and bait preferences of ants on Barro Colorado island, Panama. Biotropica 34, 348–356. doi: 10.1111/j.1744-7429.2002.tb00548.x
Hammer, Ø, Harper, D. A. T., and Ryan, P. D. (2001). Paleontological statistics software package for education and data analysis. Palaeontol. Electron. 4, 9–18.
Hille Ris, Lambers, J., Adler, P. B., Harpole, W. S., Levine, J. M., and Mayfield, M. M. (2012). Rethinking community assembly through the lens of coexistence theory. Annu. Rev. Ecol. Evol. Syst. 43, 227–248. doi: 10.1146/annurev-ecolsys-110411-160411
Holway, D. A. (1999). Competitive mechanisms underlying the displacement of native ants by the invasive argentine ant. Ecology 80, 238–251.
Houadria, M., Salas-López, A., Bluthgen, N., Orivel, J., and Menzel, F. (2015). Dietary and temporal niche differentiation in in tropical ants - can they explain local tropical ant coexistence? Biotropica 47, 208–217.
Ipser, R. M., and Gardner, W. A. (2020). Abundance, activity, diversity, and species interactions of ground-dwelling ants (Hymenoptera: Formicidae) in open versus canopied habitats in Central Georgia (USA). J. Entomol. Sci. 55, 469–486. doi: 10.18474/0749-8004-55.4.469
Jacquemin, J., Maraun, M., Roisin, Y., and Leponce, M. (2012). Differential response of ants to nutrient addition in a tropical Brown Food Web. Soil Biol. Biochem. 46, 10–17. doi: 10.1016/j.soilbio.2011.11.007
Kaspari, M., and Yanoviak, S. P. (2001). Bait use in tropical litter and canopy ants - evidence of differences in nutrient limitation. Biotropica 33, 207–211.
Kaspari, M., Donoso, D., Lucas, J. A., Zumbusch, T., and Kay, A. D. (2012). Using nutritional ecology to predict community structure: a field test in neotropical ants. Ecosphere 3, 1–15. doi: 10.1890/ES12-00136.1
Keddy, P. A. (1992). Assembly and response rules: two goals for predictive community ecology. J. Veg. Sci. 3, 157–164. doi: 10.2307/3235676
Kraft, N. J. B., and Ackerly, D. D. (2010). Functional trait and phylogenetic tests of community assembly across spatial scales in an Amazonian forest. Ecol. Monogr. 80, 401–422. doi: 10.1890/09-1672.1
Lanan, M. (2014). Spatiotemporal resource distribution and foraging strategies of ants (Hymenoptera: Formicidae). Myrmecol. News 20, 53–70.
Levine, J. M., and Hille Ris, Lambers, J. (2009). The importance of niches for the maintenance of species diversity. Nature 461, 254–257. doi: 10.1038/nature08251
Losos, J. B. (2008). Phylogenetic niche conservatism, phylogenetic signal and the relationship between phylogenetic relatedness and ecological similarity among species. Ecol. Lett. 11, 995–1003. doi: 10.1111/j.1461-0248.2008.01229.x
Luque, G. M., and Reyes López, J. (2007). Effect of experimental small-scale spatial heterogeneity on resource use of a Mediterranean ground-ant community. Acta Oecol. 32, 42–49. doi: 10.1016/j.actao.2007.03.003
MacArthur, R., and Levins, R. (1967). The limiting similarity, convergence, and divergence of coexisting species. Am. Nat. 101, 377–385. doi: 10.1086/282505
McCrum-Gardner, E. (2008). Which is the correct statistical test to use? Br. J. Oral Maxillofac. Surg. 46, 38–41. doi: 10.1016/J.BJOMS.2007.09.002
McGlynn, T. P., and Kirksey, S. E. (2000). The effects of food presentation and microhabitat upon resource monopoly in a ground-foraging ant (Hymenoptera: Formicidae) community. Rev. Biol. Trop. 48, 629–642.
McKane, R. B., Johnson, L. C., Shaver, G. R., Nadelhoffer, K. J., Rastetter, E. B., Fry, B., et al. (2002). Resource-based niches provide a basis for plant species diversity and dominance in arctic tundra. Nature 415, 68–71. doi: 10.1038/415068a
Nooten, S. S., Schultheiss, P., Rowe, R. C., Facey, S. L., and Cook, J. M. (2019). Habitat complexity affects functional traits and diversity of ant assemblages in urban green spaces (Hymenoptera: Formicidae). Myrmecol. News 29, 67–77. doi: 10.25849/myrmecol.news_029067
Parr, C. L. (2008). Dominant ants can control assemblage species richness in a South African savanna. J. Anim. Ecol. 77, 1191–1198. doi: 10.1111/j.1365-2656.2008.01450.x
Parr, C. L., and Gibb, H. (2010). “Competion and the role of dominant ants,” in Ant Ecology, eds L. Lach, C. Parr, and K. L. Abbott (New York, NY: Oxford University Pess), 77–96. doi: 10.1093/acprof:oso/9780199544639.003.0005
Pearce-Duvet, J. M. C., Elemans, C. P. H., and Feener, D. H. (2011). Walking the line: search behavior and foraging success in ant species. Behav. Ecol. 22, 501–509. doi: 10.1093/beheco/arr001
Peters, M. K., Mayr, A., Röder, J., Sanders, N. J., and Steffan-Dewenter, I. (2014). Variation in nutrient use in ant assemblages along an extensive elevational gradient on Mt Kilimanjaro. J. Biogeogr. 41, 2245–2255. doi: 10.1111/jbi.12384
Pfeiffer, M., Mezger, D., and Dyckmans, J. (2014). Trophic ecology of tropical leaf litter ants (Hymenoptera: Formicidae) - a stable isotope study in four types of Bornean rain forest. Myrmecol. News 19, 31–41.
Pike, N. (2011). Using false discovery rates for multiple comparisons in ecology and evolution. Methods Ecol. Evol. 2, 278–282. doi: 10.1111/j.2041-210X.2010.00061.x
Pyke, G. H., Pulliam, H. R., and Charnov, E. L. (1977). Optimal foraging: a selective review of theory and tests. Q. Rev. Biol. 52, 137–154.
Salas-López, A. (2017). Predicting resource use in ant species and entire communities by studying their morphological traits: Influence of habitat and subfamily. Ecol. Indic. 78, 183–191. doi: 10.1016/j.ecolind.2017.02.034
Salas-López, A., Mickal, H., Menzel, F., and Orivel, J. (2017). Ant-mediated ecosystem processes are driven by trophic community structure but mainly by the environment. Oecologia 183, 249–261. doi: 10.1007/s00442-016-3741-z
Salas-López, A., Violle, C., Mallia, L., and Orivel, J. (2018). Land-use change effects on the taxonomic and morphological trait composition of ant communities in French Guiana. Insect Conserv. Divers. 11, 162–173. doi: 10.1111/icad.12248
Sanders, N. J., and Gordon, D. M. (2003). Resource-dependent interactions and the organization of desert ant communities. Ecology 84, 1024–1031.
Sarty, M., Abbott, K. L., and Lester, P. J. (2006). Habitat complexity facilitates coexistence in a tropical ant community. Oecologia 149, 465–473. doi: 10.1007/s00442-006-0453-459
Savolainen, R., and Vepsäläinen, K. (1988). A competition hierarchy among boreal ants: impact on resource partitioning and community structure. Oikos 51, 135–155. doi: 10.2307/3565636
Weiher, E., Freund, D., Bunton, T., Stefanski, A., Lee, T., and Bentivenga, S. (2011). Advances, challenges and a developing synthesis of ecological community assembly theory. Philos. Trans. R. Soc. Lond. B. Biol. Sci. 366, 2403–2413.
Keywords: assembly rules, niche partitioning, habitat filtering, Formicidae, resource limitation, community ecology, foraging ecology
Citation: Salas-López A, Violle C, Munoz F, Menzel F and Orivel J (2022) Effects of Habitat and Competition on Niche Partitioning and Community Structure in Neotropical Ants. Front. Ecol. Evol. 10:863080. doi: 10.3389/fevo.2022.863080
Received: 26 January 2022; Accepted: 16 May 2022;
Published: 17 June 2022.
Edited by:
Gengping Zhu, Tianjin Normal University, ChinaReviewed by:
Flavia Maria Darcie Marquitti, State University of Campinas, BrazilHenintsoa Onivola Minoarivelo, University of Cape Town, South Africa
Copyright © 2022 Salas-López, Violle, Munoz, Menzel and Orivel. This is an open-access article distributed under the terms of the Creative Commons Attribution License (CC BY). The use, distribution or reproduction in other forums is permitted, provided the original author(s) and the copyright owner(s) are credited and that the original publication in this journal is cited, in accordance with accepted academic practice. No use, distribution or reproduction is permitted which does not comply with these terms.
*Correspondence: Alex Salas-López, YWxleC5zYWxhc2xvcGV6QGdtYWlsLmNvbQ==
†Present address: Alex Salas-López, Ávila, Spain