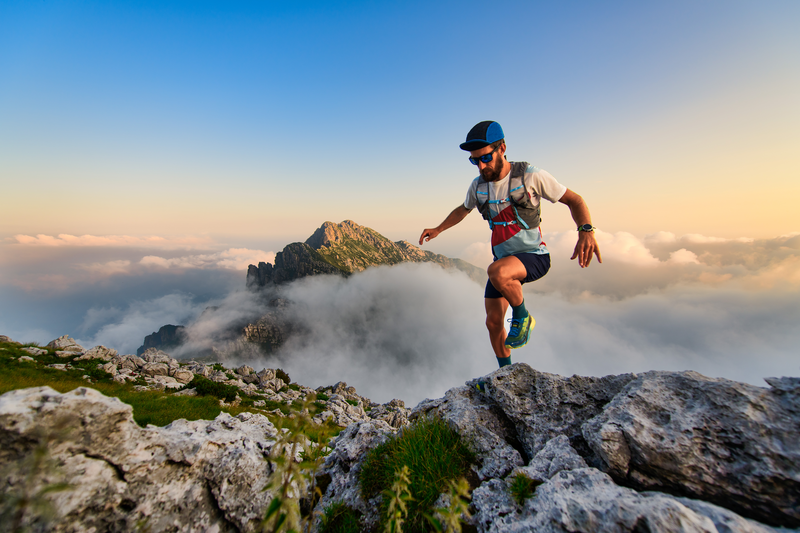
95% of researchers rate our articles as excellent or good
Learn more about the work of our research integrity team to safeguard the quality of each article we publish.
Find out more
ORIGINAL RESEARCH article
Front. Ecol. Evol. , 24 March 2022
Sec. Evolutionary Ecology of Social Behaviour
Volume 10 - 2022 | https://doi.org/10.3389/fevo.2022.860407
Explaining why animal groups vary in size is a fundamental problem in behavioral ecology. One hypothesis is that life-history differences among individuals lead to sorting of phenotypes into groups of different sizes where each individual does best. This hypothesis predicts that individuals should be relatively consistent in their use of particular group sizes across time. Little is known about whether animals’ choice of group size is repeatable across their lives, especially in long-lived species. We studied consistency in choice of breeding-colony size in colonially nesting cliff swallows (Petrochelidon pyrrhonota) in western Nebraska, United States, over a 32-year period, following 6,296 birds for at least four breeding seasons. Formal repeatability of size choice for the population was about 0.41. About 45% of individuals were relatively consistent in choice of colony size, while about 40% varied widely in the colony size they occupied. Birds using the smaller and larger colonies appeared more consistent in size use than birds occupying more intermediate sized colonies. Consistency in colony size was also influenced by whether a bird used the same physical colony site each year and whether the site had been fumigated to remove ectoparasites. The difference between the final and initial colony sizes for an individual, a measure of the net change in its colony size over its life, did not significantly depart from 0 for the dataset as a whole. However, different year-cohorts did show significant net change in colony size, both positive and negative, that may have reflected fluctuating selection on colony size among years based on climatic conditions. The results support phenotypic sorting as an explanation for group size variation, although cliff swallows also likely use past experience at a given site and the extent of ectoparasitism to select breeding colonies.
One of the more challenging problems in behavioral ecology is explaining why animal group sizes often vary by several orders of magnitude even within a single population (Jarman, 1974; Brown et al., 1990; Avilés, 1997; Jovani et al., 2016). A popular explanation is spatial variation in resource availability, with animals hypothesized to settle together in ways broadly proportional to local resource abundance or habitat quality (Lack, 1968; Fretwell and Lucas, 1970; Brown and Rannala, 1995; Danchin and Wagner, 1997; Safran et al., 2007; Spottiswoode, 2009). Most empirical work has focused on this general class of hypotheses (Rypstra, 1985; Gibbs et al., 1987; Danchin et al., 1998; Davis and Brown, 1999; Forbes et al., 2000; Ainley et al., 2003; Nuechterlein et al., 2003; Votier et al., 2007; Ventura et al., 2017), but the evidence remains mixed for most species that group size can be reliably predicted by spatial heterogeneity in resource distributions alone (Brown et al., 2002; Safran et al., 2007; Brown, 2016).
Another possibility is that the distribution of group sizes reflects variation in individuals’ life-history characteristics that confer preferential advantages in groups of different sizes (Brown, 1982; Brown et al., 1990; Höglund et al., 1993; Brown and Brown, 1996; Spottiswoode, 2007). For example, constellations of traits such as stress hormone profiles, brain size and resultant cognitive abilities, inherent aggressiveness, neophobia, and susceptibility to disease or parasites can differ systematically among individuals in large versus small groups (Bukacińska et al., 1993; Brown et al., 2005; Møller, 2010; Dardenne et al., 2013; Minias et al., 2020; Wagnon and Brown, 2020). This phenotypic specialization can maintain variation in group size as long as individual fitness remains on average equivalent among animals in the different social environments (Brown, 2016; Brown et al., 2016). If these suites of phenotypic life-history traits have any genetic basis, group-size choice can have a heritable component (Møller, 2002; Roche et al., 2011). Phenotypic environmental matching can lead to either genetic divergence (for example, between large- and small-group phenotypes) or selection on plasticity (Scheiner, 2016; Edelaar et al., 2017) to be able to choose the best social situation given the environmental conditions.
Most work on how phenotypic sorting of individuals causes variation in group size has been done with colonially nesting birds [reviewed in Brown (2016)]. Significant heritability in colony-size preference has been shown or strongly suggested in a few species (Brown and Brown, 2000; Møller, 2002; Brown et al., 2003; Serrano and Tella, 2007; Spottiswoode, 2009), while studies of non-colonial species have shown some genetic basis to other aspects of sociality (Bacon and Andersen-Harild, 1987; Magurran et al., 1995; Charmantier et al., 2007). Still, the extent to which individuals have consistent preferences or specializations for different colony sizes remains largely unstudied for most species. Selecting a colony size is likely a complex process that may also be influenced by prior experience or familiarity at a particular physical location (Hoogland et al., 2006; Brown et al., 2008), by annual variation in the numbers of local predators or parasites at a site (Brown and Brown, 1986; Danchin, 1992; Martínez-Abraín et al., 2003; Brown et al., 2017; Natusch et al., 2017), an individual’s own age (Coulson and White, 1956, 1958; Kharitonov and Siegel-Causey, 1988; Burger and Gochfeld, 1990; Brown et al., 2014), local resource availability or site quality as assessed by conspecifics (Danchin and Wagner, 1997; Danchin et al., 1998; Evans et al., 2016), and incomplete information on how many other animals are likely to settle or remain at a site (Matthiopoulos et al., 2005; Russell and Rosales, 2010; Schippers et al., 2011).
Our understanding of the importance of group size per se in dictating individual settlement decisions is compromised by having almost no information on the consistency of animals’ group-size choices over their lifetimes. Most work on colonial birds, for example, has consisted of short-term studies that have followed individuals over only 1 or 2 years of their lives (Brown and Brown, 2001; Brown, 2016). By monitoring the same animals over multiple years and knowing what colony sizes they choose each year, we can determine how their consistency in choice (if any) is influenced by past familiarity with a location or other factors thought to influence choice of a nesting colony.
In addition to its value in evaluating different hypotheses for the maintenance of colony-size variation, knowing individual consistency in selection of colony size across years allows statistical estimates of the repeatability of size choice. Repeatability, defined as the proportion of the observed variance in a trait attributable to differences among individuals (Wilson, 2018), formally captures the likelihood of individuals exhibiting the same trait at different times, and is increasingly being used in studies of behavior (Bell et al., 2009; Dochtermann and Royauté, 2019). Because heritability of behavioral traits is difficult to measure under field conditions in most cases, measures of repeatability can provide an upper estimate on heritability and thus the extent to which the trait may be subject to natural selection (Dohm, 2002; Bell et al., 2009; Wolak et al., 2012; Wilson, 2018).
The only study to date that has measured choice of colony size over individuals’ lifetimes was that of Roche et al. (2011) on colonially nesting cliff swallows (Petrochelidon pyrrhonota). Colony-size choices of birds in two cohorts were followed over an 11-year period from birth until none remained in the study area. These birds were all part of a cross-fostering experiment, in which portions of broods were moved between colonies of different sizes to estimate heritability of colony-size choice (Brown and Brown, 2000). The individuals that were re-caught were mostly found in only one or two seasons as breeders, and only about 70 birds were followed for 4 or more years of their lives (Roche et al., 2011). With half of these birds from an experimental treatment (transferred between colonies as young nestlings), the sample size was insufficient to examine the multiple factors potentially affecting colony-size choice over the birds’ lifetimes or to rigorously estimate repeatability of size choice (sensu Wolak et al., 2012).
In this study we used a 32-year mark-recapture dataset to investigate the colony-size choices made by individuals over multiple years. We restricted the analysis to 6,296 cliff swallows for which breeding-colony sizes were known in 4 or more years. Our general objectives were to use the individual histories to describe the extent of repeatability in individuals’ colony-size choice across years, and to determine whether this repeatability is consistent with earlier estimates of colony-size heritability in this species (Brown and Brown, 2000; Roche et al., 2011) and the sorting of individuals among colony sizes (Brown and Brown, 1996, 2003; Brown et al., 2005; Smith et al., 2005; Wagnon and Brown, 2020). The earlier work suggested moderate levels of heritability in colony-size choice in cliff swallows, perhaps driven by phenotypic differences in individual susceptibility to parasites, testis and brain size, and hormonal response to stress that varied among birds in different sized groups.
In this article, we provide repeatability estimates for colony-size choice in cliff swallows. In addition to formal estimates of repeatability as represented by the intraclass correlation coefficient (ICC), which is a population-level metric, we also examine repeatability by using each individual’s coefficient of variation (CV) in colony size, allowing us to investigate ecological correlates affecting colony-size choice at the individual level. These correlates include factors that potentially influence settlement, such as familiarity (experience) at a given colony site (Brown et al., 2008) and the number of ectoparasites in the nests at a site (often a legacy from past site use; Brown and Brown, 1986, 1996). We use these results to better understand the basis for colony-size variation in cliff swallows, what affects how consistent an individual’s choice of colony size may be across years, whether birds show net changes in the colony sizes used over their lifetimes, and how colony-size choice varies in different years. The extent to which resource availability (e.g., food) affects colony choice is not considered explicitly in this study, as earlier work has not shown strong relationships between local resources and colony size in cliff swallows (Brown, 1988; Brown and Brown, 1996; Brown et al., 2002).
The cliff swallow is a migratory, sparrow-sized passerine bird found commonly throughout the Great Plains and westward to the Pacific coast of North America (Brown et al., 2020). In its original habitat, the species built its gourd-shaped mud nests underneath horizontal overhangs on the sides of steep cliffs, but now many cliff swallows nest under the sides of bridges and buildings or inside concrete culverts underneath roads (Brown et al., 2013). The birds arrive in our study area beginning in late April, with most colony sites being occupied in May and early June, but colonies can begin as late as early July. Some colony sites are occupied synchronously by 75–100% of the eventual residents that arrive within periods as short as 4 days, while other sites (especially early-starting ones) gradually accumulate residents over a period of up to 2 weeks (Brown and Brown, 1996). Most colonies have completed nesting by late July. The species winters in southern South America, primarily Argentina (Brown et al., 2020).
We studied cliff swallows near the Cedar Point Biological Station (41.2097° N, 101.6480° W) in western Nebraska, United States, along the North and South Platte rivers. The study area includes portions of Keith, Garden, Deuel, Lincoln, and Morrill counties. Our work was done primarily at cliff swallow colonies on highway bridges and box-shaped culverts underneath roads or railroad tracks (Brown et al., 2013). Colonies were defined as birds from groups of nests that interacted at least occasionally in defense against predators or by sharing information on the whereabouts of food (Brown and Brown, 1996). Typically, all the nests on a given bridge or culvert constituted a colony. In rare cases, nests in different culverts that were as close as 0.1 km were considered separate colonies because adjacent residents did not interact, although most colonies were at least 0.5 km from the next nearest. Cliff swallows were well suited to a study of colony-size choice because in our study area colony size varied widely, ranging from 2 to 6000 nests (mean ± SE: 404 ± 13, n = 2318 colonies), with some birds also nesting solitarily. The birds had multiple colonies to choose from each year: over 220 colony sites were available within a 50 km × 150 km region (Brown et al., 2013). In parts of the study area, up 20 sites can be within 10 km of each other (Hannebaum et al., 2019a), which is within the distance that radio-tagged cliff swallows often moved early in the year while assessing sites (Brown and Brown, 1996). We use the term “colony” to refer to the birds occupying a structure in a given year, whereas “colony site” refers to the physical substrate.
We used mark-recapture data collected over a 32-year period, 1982–2013, in which we banded ∼229,000 cliff swallows with United States Geological Survey (USGS) bands and had ∼405,000 total bird captures in mist nets at up to 40 different colony sites each year (Brown et al., 2016). As swallows exited their nests, they were captured by putting nets across the entrance of highway culverts or along the sides of bridges that contained swallow colonies. Each bird received a unique USGS band and was sexed by the presence or absence of a cloacal protuberance (males) or a brood patch (females). In order to achieve roughly equal recapture probability across the study area, we shifted our recapture efforts among accessible colony sites, netting at each several times each season (Brown, 1998; Brown and Brown, 2004b; Roche et al., 2013). Over the summer, we typically captured 10–60% of the residents at a colony, as inferred from a colony’s capture total and the colony size. Most colonies netted were in a region of about 15 km × 15 km in Keith County (Hannebaum et al., 2019a), although the total study area over which netting occurred was about 20 km × 125 km, from near Paxton, Nebraska, to near Broadwater, Nebraska.
We used the pattern of recaptures to assign each individual as a resident breeder at a given colony site (Roche et al., 2011). Individuals caught at only a single colony in a given year were assumed to be residents of that site. For any bird caught at more than one colony site within a season, we categorized it as a resident at a given colony if it was caught at that colony site at least twice prior to 20 June. Cliff swallows caught at multiple colonies after 20 June were not assumed to be residents at any of those sites, due to widespread colony visitation by non-breeding and post-breeding birds later in the summer (Brown, 1998). Additional details and rationale for using the 20 June cut-off date are given in Roche et al. (2011).
Colony size in all cases refers to the maximum number of active nests at a site in a season, with an active nest defined as one in which one or more eggs were laid. Colony sizes were determined by direct counts of all active nests (from inspecting nest contents) or by estimation based both on nest counts of portions of a colony site and on the number of birds present at a site (Brown and Brown, 1996; Brown et al., 2013).
Some colony sites in the study area were fumigated each year to remove ectoparasitic swallow bugs (Hemiptera: Cimicidae: Cimex vicarius) as part of other research (Brown and Brown, 1986, 1996, 2004a). Swallow bugs are the major nest parasite of cliff swallows. At 7–10 day intervals throughout the breeding season, nests were lightly sprayed with a dilute solution of Dibrom, an organophosphate insecticide that is highly effective against swallow bugs (Brown and Brown, 2004a; Runjaic et al., 2017). Two colony sites were fumigated each year (beginning in 1984), while 12 additional ones were fumigated in subsets of 1–5 years. In analyses, sites were treated as either fumigated or non-fumigated in a given year depending on whether they were sprayed that year, and no lag effects of fumigation were considered. This was justified because only a single spraying at a colony site can effectively eliminate bugs there for the entire season (Runjaic et al., 2017), whereas bugs can rapidly re-colonize a site the year after fumigation (Brown, pers. obs.).
We used the mark-recapture database to identify all individuals that were caught at a designated breeding colony in at least 4 years. While 4 years was an arbitrary designation, it seemed appropriate in reflecting most of a cliff swallow’s lifetime. Given the variability in recapture probability for birds in the study area (Roche et al., 2013), many of the birds caught only two or three times may have been missed in other years, and thus we felt their observed colony-size histories may not have been representative of their lives. Of the 6,296 individuals across all years that met the 4-year criterion, 63.3% were caught in 4 years, 24% in 5 years, and the remaining 12.7% in 6–11 years. For analyses involving year, the first year a bird was caught at a breeding colony was its designated Year. Only birds through 2010 were included; this allowed the 2010 cohort to have had the opportunity to have been caught four times through 2013 (when the mark-recapture project ended). We noted whether birds in the dataset had originally been banded as nestlings (5.6%), fledged juveniles (12.2%), or adults (82.2%), and refer to this variable as Age. We did not have exact ages for the majority of birds (those banded as adults) and could not explore age effects beyond these crude categories.
The years in which we knew breeding-colony sizes for an individual were sometimes consecutive, but often gaps existed in between the years of capture. Because repeatability of behavioral traits can be affected by the duration over which the traits are measured (Bell et al., 2009), we designated a metric (Interval) to express the time period over which the colony sizes were known for each bird. A pair of consecutive years received a 1, whereas captures in 2 years separated by 1 missing year received a 2. These intervals were added across all captures for each individual. Thus, a bird caught in 4 consecutive years would have the minimum Interval of 3. The mean (±SE) Interval over all birds was 4.9 (±0.02, n = 6296), with 1473 (23.4%) having the minimum score of 3 and 11 birds with the maximum score of 11.
For each individual, we calculated the maximum proportion of times it used the same colony site (Samesite) across its capture years. Birds always changing sites got a 0.000, whereas those using the same site each year received a 1.000. If, for example, a bird caught five times used the same site in 3 years and another site in the other 2 years, it received a 0.600. Birds caught in 4 years that may have used two sites each in 2 years received a 0.500. For each bird, we also determined the proportion of years it occupied a fumigated colony site (Fumsite), its average colony size (Meansize), its difference in colony size between its first and last years (after minus before; Diffsize) as a measure of any long-term shift in colony size, and the coefficient of variation (CV) in its colony size across all years.
For population-level repeatability of colony size, we determined the intraclass correlation coefficient (ICC) by (1) performing a general linear model (one-way ANOVA) in SAS (SAS Institute, 2004), with individual as the independent predictor and colony size as the dependent variable and (2) using the SAS output to calculate the ICC that also accounted for different numbers of measurements (years with a colony size) for different individuals, as recommended by Lessells and Boag (1987). The standard error of the ICC was determined from the formulae in Zar (1999). Repeatability was calculated for subsets of our sample (males, females, Year), but we used the CV of colony size in most analyses. Comparisons of the CV and ICC are given in the section “Results.”
Statistical analyses were designed to identify factors potentially predicting the CV of colony size and the colony-size difference between a bird’s first and last capture years (Diffsize). Independent predictors included the first-year cohort a bird belonged to (Year), its proportion of sites used in 2 or more years (Samesite), its proportion of years occupying a fumigated site (Fumsite), its banding age (Age), the number of years for which its colony size was known (N), the time period over which its colony sizes were measured (Interval, see above), and its mean colony size (Meansize). In order to account for birds that might have preferred to settle at either the same site each year or to not move far from the first-year site (Brown et al., 2017; Hannebaum et al., 2019a), we used the first-year’s colony site (Yr1site) as a random effect to account for any sort of spatial dependence.
For each dependent variable, we constructed a global model containing all main effects and biologically plausible interactions (including non-linear ones) among the main effects. Interactions that proved to be non-significant were removed from the final model. Modeling was done with Proc Mixed in SAS that included the random effect of Yr1site. We did no formal model selection because we had a priori reason to examine each variable that was included. Predicted values of dependent variables were generated with Proc PLM in SAS from the final model.
For all years and sexes combined (n = 6296), repeatability as measured by the ICC (±SE) for colony-size choice was 0.416 (±0.0115); for males separately (n = 3689), 0.422 (±0.0149), and for females separately (n = 2607), 0.406 (±0.0179). When ICCs were calculated for each year cohort, 1983–2010 (excluding 1982 where n = 1 bird), there was no trend for the ICC to change over this period (Figure 1).
Figure 1. Repeatability of cliff swallow colony size for each year-cohort as measured by the intraclass correlation coefficient (ICC) (±SE) did not vary significantly across years (r = 0.07, P = 0.71, n = 28 years). Solid line indicates best-fit least-squares regression and dotted lines the 95% CI. Sample size for each year-cohort is given in Figure 9.
Figure 2. The mean (±SE) coefficient of variation (CV) in cliff swallow colony size for each year-cohort CV declined significantly with year (r = –0.51, P = 0.0055, n = 28 years). 1983 is not shown for reasons of scale (mean = 0.72, SE = 0.058, n = 5). Solid line indicates best-fit least-squares regression and dotted lines the 95% CI. Sample size for each year-cohort is given in Figure 9.
Figure 3. Frequency distribution of the individual coefficient of variation (CV) in colony size for 6,296 cliff swallows in western Nebraska.
Figure 4. Representative examples of individual cliff swallows’ choice of colony sizes across years for coefficient of variation (CV) values binned into groups of 0.10 (A–K). Each color or dot style per panel indicates a different individual.
Figure 5. The coefficient of variation (CV) in colony size for individual cliff swallows in different year-cohorts (Year) tended to be smaller for birds occupying relatively small and large mean colony sizes over their lifetimes (Meansize). Each dot is an individual bird although there is some overlap. Lines show predicted values (±95% CI) from the model in Table 1. All years (1983–2010) are shown in Supplementary Figure 1.
Table 1. Predictor variables (and interactions among predictors) of an individual’s coefficient of variation (CV) in colony size (a measure of colony-size consistency) for cliff swallows followed over at least four breeding seasons.
Figure 6. (A) Predicted values (±95% CI) of the coefficient of variation (CV) in colony size for individual cliff swallows declined with a bird’s mean colony size (Meansize) more for birds not using the same colony site each year than for birds always using the same site (Samesite). (B) Predicted values (±95% CI) of the coefficient of variation (CV) in colony size for individual cliff swallows showed different relationships with a bird’s mean colony size (Meansize) depending on whether the bird consistently used a fumigated site (Fumsite). Predicted values in both (A,B) come from the model in Table 1.
Figure 7. Predicted values (±95% CI) of the coefficient of variation (CV) in colony size of cliff swallows increased with a bird’s reuse of the same site (Samesite) for sites that were never fumigated but decreased with site reuse for sites that were fumigated (Fumsite). Predicted values come from the model in Table 1.
Figure 8. Frequency distribution of the net change between an individual’s final and initial colony sizes (Diffsize) for 6,296 cliff swallows in western Nebraska.
Figure 9. Net change (mean ± SE) between a cliff swallow’s final and initial colony sizes (Diffsize) were positive for some year-cohorts and negative for others, with asterisks denoting a mean that differed significantly from zero (one-sample t-test, P < 0.05). Numbers by dots indicate sample sizes (no. birds) in each year-cohort. 1983 is not shown for reasons of scale (mean = 1590, SE = 228.9, n = 5).
Averaged over all years and sexes combined (n = 6296), the mean (±SE) individual CV of colony size was 0.475 (±0.00367); for males separately (n = 3689), 0.486 (±0.00490), and for females separately (n = 2607), 0.459 (±0.00553). The mean CV for all individuals in each year-cohort showed a strong significant decline over 1983–2010 (Figure 2). Despite the difference in the yearly trends (Figures 1, 2), across the 28 year-cohorts there was a significant inverse correlation between the yearly ICC and the yearly mean CV (r = −0.675, P < 0.001, n = 28), showing as expected that higher within-population repeatability values were associated with lower mean CV values (less individual variation in colony size).
The distribution of individual CVs of colony size showed the most common category to be 0.200–0.299 (Figure 3), with 46.2% of all (n = 6296) below 0.400 and 42.2% ≥ 0.500. Representative individuals from different CV groups illustrate that cliff swallows of <0.300 showed relatively high consistency in colony-size choice regardless of colony size initially occupied (Figures 4A–C). Those with CVs of ≥0.700 showed considerable yearly variation (Figures 4H–K). Birds initially occupying the very largest colonies (>2000 nests) almost always chose smaller colonies in later years, sometimes with their later size choices relatively consistent (Figure 4).
An individual’s CV of colony size was significantly predicted by its mean colony size (Meansize), its year-cohort (Year), its proportion of years in which it occupied the same site (Samesite), and the proportion of years it occupied a fumigated site (Fumsite), but banding age (Age), sex, the number of years for which we knew its colony size (N), and the length of time over which its colony size was measured (Interval) were not significant predictors of the CV (Table 1). We detected interactions among the significant predictors (Table 1).
The effect of an individual’s average colony size (Meansize) on its CV was non-linear, with higher CVs for the intermediate means, but the strength of this effect varied across years (Table 1 and Figure 5). Birds in the smallest and largest colonies tended to have the lowest CVs (Figure 5). To some degree, this result is a statistical artifact of birds occupying colony sizes near either size extreme not having the option of moving to one side of the extreme, and this alone could result in a lower CV for them. The yearly distributions of CVs suggest that the most variable colony sizes tend to be among individuals with mean sizes of 500–1000 nests, but even for those, some individuals consistently chose intermediate sizes (i.e., had lower CVs, Figure 5). Results for all years are included in Supplementary Figure 1.
The CV was also predicted by an interaction between Meansize and both the proportion of years the same colony site was used (Samesite; Table 1 and Figure 6A) and the proportion of years a fumigated site was used (Fumsite; Table 1 and Figure 6B). Consistency in colony-size choice increased markedly with mean colony size when a different site was used each year, whereas consistency seemed less affected by mean colony size when the same site was always used (Figure 6A). Birds never using a fumigated site had highest colony-size consistency at the smallest and largest mean colony sizes, whereas birds using fumigated sites each year had lowest consistency in size-choice in the smallest mean colony sizes and highest at the largest mean colony sizes (Figure 6B).
The significant interaction between Samesite and Fumsite (Table 1) indicated that an individual’s CV declined as the proportion of times a bird used the same colony site increased when the site was regularly fumigated, but there was an opposite effect of using the same site on the CV at completely non-fumigated sites (Figure 7).
For all birds combined across all years, the net change in colony size between a cliff swallow’s final colony size and its initial colony size (Diffsize) averaged (±SE) +4.4 (±9.8) nests and did not differ significantly from zero (one-sample t-test, t = 0.45, P = 0.65). The distribution of size differences showed a roughly symmetrical distribution around zero (Figure 8). About 31% of birds showed a change of <250 nests between their final and initial colony sizes (Figure 8). However, when dividing the birds by year cohort, the change in colony size differed significantly from zero in most years, with birds exhibiting a net reduction in colony size on average in some years and a net increase in colony size in other years (Figure 9).
Diffsize was significantly predicted by the length of time over which a bird’s colony size was known (Interval), an individual’s first-year cohort (Year), and the proportion of years an individual used a fumigated site (Fumsite), but banding age (Age), sex, the number of years monitored (N), and the proportion of years it used the same site (Samesite) were not significant (Table 2). A longer interval led to a more positive Diffsize (β = 14.39, SE = 4.71; Table 2). The effect of Year was non-linear and interacted with Fumsite (Table 2 and Figure 10). Individual cliff swallows that used exclusively fumigated sites showed a net increase in colony size in all years, although this trend diminished slightly in the later years, while those never using fumigated sites showed a net reduction in colony size that was more apparent in the earlier years of the study (Figure 10).
Table 2. Predictor variables (and interactions among predictors) of an individual’s net change between its final and initial colony size (Diffsize) for cliff swallows followed over at least four breeding seasons.
Figure 10. Predicted values (±95% CI) of the net change between a cliff swallow’s final and initial colony sizes trended more toward zero over the years of the study regardless of how much a bird used a fumigated site (Fumsite), but birds always occupying fumigated sites consistently had more positive net size change than those never using a fumigated site. Predicted values come from the model in Table 2.
Our results indicate that choice of colony size in cliff swallows is significantly repeatable, with some birds consistently occupying colonies of similar sizes throughout their lives. Other birds, however, varied widely in the colony size they selected. The extent to which an individual was observed to use similar colony sizes over its life depended partly on its mean colony size, the extent of ectoparasitism at a site (i.e., whether the site had been fumigated), and whether it re-used the same colony site in more than 1 year, but we found no effect of sex, age, or over how much of its life it was monitored on its consistency in colony size. Comparison of the net change in an individual’s colony size over its life found no net deviation from zero for the population as a whole, although most year-cohorts showed either significant negative or positive change in colony size.
Because group size at a site can depend in part on whether other individuals join or leave a group (Sibly, 1983; Pulliam and Caraco, 1984), choosing a particular colony size could be subject to constraints on what others do. Cliff swallows “solve” this problem in part in at least two ways: they make collective decisions on site use by often arriving at a colony site together and apparently assessing it en masse (Brown and Brown, 1996), and by delaying egg-laying at a site until most of the daily size fluctuations have ceased, which gives an individual the opportunity to reject a colony if it increases or declines to an unacceptable degree, at least early in the season.
Our overall estimate of colony-size repeatability (using the ICC) for the population, 0.416, was relatively high and in line with repeatability estimates of other behavioral traits (Bell et al., 2009; Mitchell et al., 2020). Repeatability is often assumed to represent an upper limit on a trait’s heritability (Boake, 1989; Falconer and Mackay, 1996; Wilson, 2018), and our result is consistent with mid-parent heritability estimates of 0.326–0.377 from a cross-sectional dataset of >1000 birds (Brown and Brown, 2000) and 0.415–0.433 from a cohort of about 300 non-cross-fostered birds from 1997 to 1998 followed over their lifetimes (Roche et al., 2011). The current analysis of over 6,000 birds followed for at least 4 breeding years gives confidence in asserting that the heritability of colony size is approximately 0.4 for cliff swallows in western Nebraska.
The coefficient of variation can be used as one measure of repeatability (Dochtermann and Royauté, 2019). The average CV for all birds, 0.475, was slightly higher than the population repeatability as measured by the ICC, yet the CV had the advantage of being calculable for each individual and thus allowed us to investigate correlates associated with an individual’s consistency in colony size. Unlike for many behavioral traits in which repeatability diminishes as the time between measurements increases (Bell et al., 2009), we found no effect on the CV of how many years we knew an individual’s colony size (within the range of 4–10) or the length of time (number of years) between those measurements. Thus, the repeatability estimates provided here can be considered lifetime measures. We should emphasize that this study used only the subset of cliff swallows that had their breeding colonies known for at least 4 years. This restriction was necessary in order to avoid including birds with short, incomplete histories and to achieve relatively robust CVs, but the consequence was that birds living less than 4 years were excluded. However, other studies looked explicitly at colony-size choice among yearling cliff swallows and shorter-lived birds (Brown and Brown, 2000; Roche et al., 2011), and the results suggest similar repeatabilities for those age classes.
The functional repeatability of colony-size choice in cliff swallows may be greater than what we estimated. We used actual colony sizes to calculate ICCs and CVs, which would have treated, for example, colonies of 500 and 900 nests as quantitatively rather different from each other, when biologically there is relatively little difference in the birds’ behavior in colonies of those sizes. Birds in both exhibit traits of large-colony phenotypes, and predator attack rates, ectoparasite loads, and residents’ foraging tactics differ little in colonies of 500–900 nests (Brown and Brown, 1996). Partly for this reason, an earlier study used colony ranks in which the available colonies in a given year were ranked from smallest to largest and analyses based on those (Brown and Brown, 2000). We did not use that method in this study because it requires specifying a subset of colonies that are “available” to each individual, which then necessitates making spatial assumptions (Roche et al., 2011). Here, we avoid that problem by using actual colony sizes, but we should keep in mind that some differences in colony size are biologically irrelevant (especially at the upper end of the size range) and also that individuals are sometimes constrained in their choice of colony size by what is available in a given year (see below).
One of the hypotheses for the evolution and maintenance of colony-size variation is that individuals possessing differing life-history traits are optimized for different group sizes (Brown and Brown, 2000, 2018; Møller, 2002; Brown et al., 2003; Serrano and Tella, 2007; Spottiswoode, 2007, 2009; Brown, 2016). Cliff swallows occupying colonies of different sizes vary in traits such as stress hormone levels, testis and brain size, susceptibility to ectoparasites, and possibly propensity for vigilance and risk-taking (Brown and Brown, 1996, 2003; Brown et al., 2005, 2015; Roche and Brown, 2013; Hannebaum et al., 2019b; Wagnon and Brown, 2020). The life-history optimization hypothesis predicts heritability of colony size and thus that individuals would show measurable consistency throughout their lifetimes in choice of colony size. The motivation for this study was to document that repeatability.
Our results revealed that about 46% of birds showed relatively high consistency in choosing breeding colonies of particular sizes over their lifetimes (CVs < 0.4; Figure 3). These consistent individuals spanned the size range, meaning that apparent small-colony, intermediate-colony, and large-colony phenotypes existed in the population. Birds in the largest colonies seemed in general more likely to have the lowest CVs (Figure 5), indicating perhaps that large-colony phenotypes are the least likely to undertake drastic colony-size changes over their lives. Given the inherent variability in what colony sizes might be available in a given year, and that colony size also depends in part on other birds settling at or departing from a colony site (Brown and Brown, 1996; Russell and Rosales, 2010), it is unlikely that finer-grain phenotypic size preferences exist or would be possible to discriminate.
However, over 40% of birds exhibited relatively high variation in colony-size choice (CVs > 0.5), typically using both small and large colonies over their lifetimes. One possibility is that such individuals truly are “social generalists,” equally capable of performing in groups of any size. No evidence for this in cliff swallows or other species exists, although we really have not examined the issue rigorously. Another possibility is that colony-size inconsistency reflects external constraints on an individual’s ability to choose a given colony size. While cliff swallows probably have reliable information on a colony’s size at the time of settlement and before egg-laying (see above), sometimes other birds arrive at a site well after an individual has settled, inflating the size, or some residents may leave a site due to nest failure, reducing the colony size. Such cascades in colony-size shifts, brought about partly by individuals’ use of social information especially in the absence of direct knowledge of or experience at a site (Johst and Brandl, 1997; Russell and Rosales, 2010), may strand individuals in colony sizes they would not otherwise have chosen. Whether they abandon the site and search for a more suitable colony size may depend on their nesting stage, the time of year, physical proximity of alternative colony sites, or whether their close neighbors are among those who remain (Brown and Brown, 1996; Brown and Hannebaum, unpubl. data). In other cases, fluctuations in population size brought about by episodic weather-related mortality events (Brown and Brown, 1998, 2018) or vagaries in local site use (Brown et al., 2013) may restrict the choice of colonies to ones either smaller or larger than what an individual would prefer, leading to inconsistent size use.
Two other factors that affect cliff swallow breeding site choice are experience at a given colony site and the extent of ectoparasitism at a site, and here we investigated the effect of both on colony-size consistency. Perennially occupying the same colony site regardless of size improves an individual’s probability of surviving during the breeding season by ∼8% per month (Brown et al., 2008), probably because of experience with local foraging conditions and the habits of local predators. Our results showed that consistency in colony-size choice varied with whether an individual used the same site in multiple years, with colony size being more variable for those birds tending to use different sites each year (Figure 6A). Among the birds using different sites each year, however, colony-size consistency was markedly greater for individuals occupying the larger colonies in the size range and was most variable among birds using the smallest colonies (Figure 6A). There was less variation in CV across the size range for those always using the same site.
Thus, except for birds selecting the largest colonies, dispersing to a new colony site increases the likelihood that an individual will also change colony sizes. Whether an individual changes sizes because it disperses for other reasons, or disperses specifically to change colony sizes, is unclear. While some colony sites are predictable in size from year to year, many are not (Brown et al., 2013), so philopatry does not automatically result in use of the same colony size. However, the experience-related advantages associated with philopatry could partly compensate individuals that occupy the same site when it differs greatly in size between years and explain why some individuals have greater colony-size variation over their lifetimes than might be expected based on life-history optimization.
Cliff swallows respond to infestations of ectoparasites (primarily swallow bugs) when choosing colony sites and nests within sites (Brown and Brown, 1986, 1996, 2015). The experimental removal of these parasites (for other research, via fumigation) at certain colony sites within the study area allowed us to assess how consistency in an individual’s colony size may have been determined in part by the extent of ectoparasite infestation at a site. Among those birds never using a fumigated site (the majority of the population), the CV varied with mean colony size in the curvilinear pattern (Figure 6B) seen across all years (Figure 5), with the birds occupying the smallest and largest colonies being the most consistent in size choice. However, those always using a fumigated site increased in their size consistency across the size range, meaning that birds that sometimes used small fumigated sites were more variable in their size choice and that birds tending to use large fumigated sites were more likely to always use those large colonies (Figure 6B). The lack of parasites at large fumigated colonies likely makes those sites attractive: they offer the social advantages of large groups (easier foraging, better vigilance) without one of the major costs (ectoparasitism), and this alone may cause birds to perennially seek out large fumigated colonies (Brown et al., 2017). Interestingly, the small-colony phenotypes may be cueing on factors besides the lack of parasites at a fumigated site, explaining their higher CVs (Figure 6B). Perhaps parasites are less of an issue for small-colony birds because small colonies tend to have fewer ectoparasites to start with (Brown and Brown, 1986, 1996).
The interaction between the proportion of the same site being used and the proportion of fumigated sites (Figure 7) also suggested that birds perennially using the same fumigated sites exhibited less colony-size variation than ones not using the same fumigated site as often, whereas the reverse seemed to hold for birds never using fumigated sites. For those never using fumigated sites, birds using the same site repeatedly were slightly more likely to show variation in colony size (Figure 7). The contrasting results illustrate the influence that the absence of parasites at a site can have on settlement decisions and indicates that the extent of ectoparasitism at a site may contribute to individual variation in colony-size choice.
An earlier study that tracked a cohort of about 70 cliff swallows beyond their third breeding season (equivalent to the time frame of the current work) found some evidence that individuals showed a net shift toward using larger colonies as they got older (Roche et al., 2011). One measure of such a shift, which we used here, was the difference between a bird’s last known colony size and its first. For the 6,296 individuals combined across all years, there was no deviation from a mean difference of zero, suggesting that overall cliff swallows did not exhibit predictable net change in colony size over their lifetimes. This result would be consistent with no directional population-wide shift in colony-size distributions over time (Brown et al., 2013) and thus no change in environmental conditions favoring either small, medium, or large colonies over the 40 years of the study (Brown et al., 2016; Brown and Brown, 2018). Such a finding would also be predicted from life-history optimization when the different phenotypes have equal fitness (Brown, 2016). In addition, we detected no temporal change in yearly repeatability estimates (Figure 1), suggesting that conditions favoring consistency in size choice among phenotypes did not change, at least over the duration of our study.
However, when the analysis was done by year-cohort, we did find significant directional changes in colony size over an individual’s lifetime (Figure 9). In 23 of 27 years, the net size difference varied significantly from zero, being negative in some years and positive in others. The reason(s) for this annual variation are unclear but illustrate that using only 1 or 2 year-cohorts (e.g., Roche et al., 2011) will not capture the extent of temporal variation in colony-size selection. One possible interpretation for the net negative changes from 1989 to 1995 was that those cohorts endured an unusual weather-related mortality event in 1996 that reduced the population in the study area by 53% (Brown and Brown, 1998, 2018) and consequently led to smaller colony sizes on average until the population recovered in about 2002 (Brown et al., 2013). Thus, many of the birds in those cohorts would have reached their final breeding seasons during a time when smaller colony sizes were common. In addition, the net advantages associated with different colony sizes do fluctuate across years based partly on climatic conditions (Brown et al., 2016; Brown and Brown, 2018), and our results showing directional change in colony size for individuals could also reflect fluctuating selection on birds using certain colony sizes (and their removal from the population) in those particular years.
We also found that the difference between an individual’s final and initial colony sizes depended on the length of time between the measurements (Interval) and the extent to which an individual had used fumigated colony sites. As the Interval increased, individuals were more likely to shift to larger colonies. This may be an artifact of longer-interval birds necessarily being captured for the last time in the later years of the study, when net changes tended to be more positive (Figure 9). Birds using sites that were never fumigated tended to shift to smaller colonies over their lifetimes, although this pattern weakened during the study (Figure 10), possibly because the cost of ectoparasitism to cliff swallows has waned over time under natural conditions (Brown et al., 2021). In contrast, cliff swallows always using fumigated sites shifted to larger colonies over their lifetimes (Figure 10), again consistent with the amelioration of the cost of parasitism at fumigated sites that removes constraints on occupying large colonies there each year.
Our dataset of 6,296 individual cliff swallows followed over at least four breeding seasons illustrates that colony-size choice is significantly repeatable to a degree that is broadly equivalent to behavioral traits in other species for which repeatability or heritability has been measured. Some individuals clearly used mostly small colonies, others intermediate sized colonies, and still others mostly large colonies. However, at least 40% of birds varied widely in their choice of colony size over their lifetimes, and the reasons for the variation are still unclear. Cliff swallows probably integrate other cues into settlement decisions besides colony size (e.g., Brown et al., 2000). For example, we found that an individual’s past use of a site (probably leading to experience-related advantages) and the absence of ectoparasites at a site also affected the observed variation in a bird’s annual colony size. Constraints on achieving a given colony size (arrival or departure of other residents; Sibly, 1983; Pulliam and Caraco, 1984) can probably lead to use of non-preferred colony sizes at times; that many individuals come so close to achieving consistent colony sizes across their lifetimes is remarkable. Our results at least partly support life-history optimization based on an individual’s phenotypic characteristics, with colony-size choice moderately heritable. The results, however, also emphasize the importance of local ecological conditions (extent of parasitism) and past experience that may interact with phenotype to produce and maintain the colony-size variation seen in cliff swallows and other species (Brown, 2016).
The raw data supporting the conclusions of this article will be made available by the authors, without undue reservation.
This study was reviewed and approved by the Institutional Animal Care and Use Committee of the University of Tulsa.
CB designed the study, collected and analyzed the data, wrote the manuscript, and directed the project. SH analyzed the data and edited the manuscript. Both authors approved the submitted version.
Financial support was received from the National Science Foundation (BSR-8600608, BSR-9015734, DEB-9613638, IBN-9974733, DEB-0075199, DEB-0514824, DEB-1019423, DEB-1453971, IOS-1556356, and DEB-1930803), the National Institutes of Health (R01AI057569), the National Geographic Society, the Erna and Victor Hasselblad Foundation, the National Academy of Sciences, the Chapman Fund of the American Museum of Natural History, the American Philosophical Society, Sigma Xi, the University of Tulsa, Yale University, and Princeton University.
The authors declare that the research was conducted in the absence of any commercial or financial relationships that could be construed as a potential conflict of interest.
All claims expressed in this article are solely those of the authors and do not necessarily represent those of their affiliated organizations, or those of the publisher, the editors and the reviewers. Any product that may be evaluated in this article, or claim that may be made by its manufacturer, is not guaranteed or endorsed by the publisher.
Over 80 people participated in our mark-recapture work from 1982 to 2013, with Mary Bomberger Brown having a central role in data collection and data entry during most of those years. Amy Moore, Catherine Page, and Erin Roche also assisted with data management. We thank the Oren Clary family, Duane Dunwoody, Dave and Deb Knight, and Loren Soper for access to land, and the Cedar Point Biological Station of the University of Nebraska–Lincoln for use of facilities. Birds were banded and handled under permits from the U.S. Fish and Wildlife Service and Nebraska Game and Parks Commission.
The Supplementary Material for this article can be found online at: https://www.frontiersin.org/articles/10.3389/fevo.2022.860407/full#supplementary-material
Ainley, D. G., Ford, R. G., Brown, E. D., Suryan, R. M., and Irons, D. B. (2003). Prey resources, competition, and geographic structure of kittiwake colonies in Prince William Sound. Ecology 84, 709–723. doi: 10.1890/0012-9658(2003)084[0709:prcags]2.0.co;2
Avilés, L. (1997). “Causes and consequences of cooperation and permanent-sociality in spiders,” in The Evolution of Social Behavior in Insects and Arachnids, eds J. Choe and B. Crespi (Cambridge: Cambridge University Press), 476–498. doi: 10.1186/1471-2148-9-257
Bacon, P. J., and Andersen-Harild, P. (1987). Colonial breeding in mute swans (Cygnus olor) associated with an allozyme of lactate dehydrogenase. Biol. J. Linn. Soc. 30, 193–228. doi: 10.1111/j.1095-8312.1987.tb00297.x
Bell, A. M., Hankison, S. J., and Laskowski, K. L. (2009). The repeatability of behaviour: a meta-analysis. Anim. Behav. 77, 771–783. doi: 10.1016/j.anbehav.2008.12.022
Boake, C. R. B. (1989). Repeatability: its role in evolutionary studies of mating behavior. Evol. Ecol. 3, 173–182. doi: 10.1007/bf02270919
Brown, C. R. (1988). Enhanced foraging efficiency through information centers: a benefit of coloniality in cliff swallows. Ecology 69, 602–613. doi: 10.2307/1941009
Brown, C. R. (2016). The ecology and evolution of colony-size variation. Behav. Ecol. Sociobiol. 70, 1613–1632. doi: 10.1007/s00265-016-2196-x
Brown, C. R., and Brown, M. B. (1986). Ectoparasitism as a cost of coloniality in cliff swallows (Hirundo pyrrhonota). Ecology 67, 1206–1218. doi: 10.2307/1938676
Brown, C. R., and Brown, M. B. (1996). Coloniality in the Cliff Swallow: The Effect of Group Size on Social Behavior. Chicago, IL: University of Chicago Press.
Brown, C. R., and Brown, M. B. (1998). Intense natural selection on body size and wing and tail asymmetry in cliff swallows during severe weather. Evolution 52, 1461–1475. doi: 10.1111/j.1558-5646.1998.tb02027.x
Brown, C. R., and Brown, M. B. (2000). Heritable basis for choice of group size in a colonial bird. Proc. Natl. Acad. Sci. U.S.A. 97, 14825–14830. doi: 10.1073/pnas.97.26.14825
Brown, C. R., and Brown, M. B. (2001). Avian coloniality: progress and problems. Curr. Ornithol. 16, 1–82. doi: 10.1016/b978-0-12-249408-6.50010-8
Brown, C. R., and Brown, M. B. (2003). Testis size increases with colony size in cliff swallows. Behav. Ecol. 14, 569–575. doi: 10.1093/beheco/arg030
Brown, C. R., and Brown, M. B. (2004a). Empirical measurement of parasite transmission between groups in a colonial bird. Ecology 85, 1619–1626. doi: 10.1890/03-0206
Brown, C. R., and Brown, M. B. (2004b). Group size and ectoparasitism affect daily survival probability in a colonial bird. Behav. Ecol. Sociobiol. 56, 498–511. doi: 10.1007/s00265-004-0813-6
Brown, C. R., and Brown, M. B. (2015). Ectoparasitism shortens the breeding season in a colonial bird. R. Soc. Open Sci. 2:140508. doi: 10.1098/rsos.140508
Brown, C. R., and Brown, M. B. (2018). Extreme group sizes in a colonial bird favored during a rare climatic event. Ecosphere 9:e02428. doi: 10.1002/ecs2.2428
Brown, C. R., and Rannala, B. (1995). Colony choice in birds: models based on temporally invariant site quality. Behav. Ecol. Sociobiol. 36, 221–228. doi: 10.1007/s002650050143
Brown, C. R., Brown, M. B., and Brazeal, K. R. (2008). Familiarity with breeding habitat improves daily survival in colonial cliff swallows. Anim. Behav. 76, 1201–1210. doi: 10.1016/j.anbehav.2008.03.028
Brown, C. R., Brown, M. B., and Danchin, E. (2000). Breeding habitat selection in cliff swallows: the effect of conspecific reproductive success on colony choice. J. Anim. Ecol. 69, 133–142. doi: 10.1046/j.1365-2656.2000.00382.x
Brown, C. R., Brown, M. B., and Roche, E. A. (2013). Spatial and temporal unpredictability of colony size in cliff swallows across 30 years. Ecol. Monogr. 83, 511–530. doi: 10.1890/12-2001.1
Brown, C. R., Brown, M. B., Pyle, P., and Patten, M. A. (2020). “Cliff swallow (Petrochelidon pyrrhonota), version 1.0,” in Birds of the World, ed. P. G. Rodewald (Ithaca, NY: Cornell Lab of Ornithology). doi: 10.2173/bow.cliswa.01
Brown, C. R., Brown, M. B., Raouf, S. A., Smith, L. C., and Wingfield, J. C. (2005). Steroid hormone levels are related to choice of colony size in cliff swallows. Ecology 86, 2904–2915. doi: 10.1890/05-0246
Brown, C. R., Brown, M. B., Roche, E. A., O’Brien, V. A., and Page, C. E. (2016). Fluctuating survival selection explains variation in avian group size. Proc. Natl. Acad. Sci. U.S.A. 113, 5113–5118. doi: 10.1073/pnas.1600218113
Brown, C. R., Covas, R., Anderson, M. D., and Brown, M. B. (2003). Multistate estimates of survival and movement in relation to colony size in the sociable weaver. Behav. Ecol. 14, 463–471. doi: 10.1093/beheco/arg034
Brown, C. R., Hannebaum, S. L., O’Brien, V. A., Page, C. E., Rannala, B., Roche, E. A., et al. (2021). The cost of ectoparasitism in cliff swallows declines over 35 years. Ecol. Monogr. 91:e01446. doi: 10.1002/ecm.1446
Brown, C. R., Roche, E. A., and Brown, M. B. (2014). Variation in age composition among colony sizes in cliff swallows. J. Field Ornithol. 85, 289–300. doi: 10.1111/jofo.12068
Brown, C. R., Roche, E. A., and Brown, M. B. (2015). Parent-offspring resemblance in colony-specific adult survival of cliff swallows. Evol. Ecol. 29, 537–550. doi: 10.1007/s10682-015-9764-9
Brown, C. R., Roche, E. A., and Brown, M. B. (2017). Why come back home? Breeding-site fidelity varies with group size and parasite load in a colonial bird. Anim. Behav. 132, 167–180. doi: 10.1016/j.anbehav.2017.08.009
Brown, C. R., Sas, C. M., and Brown, M. B. (2002). Colony choice in cliff swallows: effects of heterogeneity in foraging habitat. Auk 119, 446–460. doi: 10.1093/auk/119.2.446
Brown, C. R., Stutchbury, B. J., and Walsh, P. D. (1990). Choice of colony size in birds. Trends Ecol. Evol. 5, 398–403. doi: 10.1016/0169-5347(90)90023-7
Brown, J. L. (1982). Optimal group size in territorial animals. J. Theor. Biol. 95, 793–810. doi: 10.1016/0022-5193(82)90354-x
Bukacińska, M., Bukacińska, D., and Jabłoński, P. (1993). Colonial and noncolonial great crested grebes (Podiceps cristatus) at Lake Łuknajno: nest site characteristics, clutch size and egg biometry. Colon. Waterbirds 16, 111–118.
Burger, J., and Gochfeld, M. (1990). The Black Skimmer: Social Dynamics of a Colonial Species. New York, NY: Columbia University Press.
Charmantier, A., Keyser, A. J., and Promislow, D. E. L. (2007). First evidence for heritable variation in cooperative breeding behaviour. Proc. R. Soc. B 274, 1757–1761. doi: 10.1098/rspb.2007.0012
Coulson, J. C., and White, E. (1956). A study of colonies of the kittiwake Rissa tridactyla (L.). Ibis 98, 63–79. doi: 10.1111/j.1474-919x.1956.tb03030.x
Coulson, J. C., and White, E. (1958). The effect of age on the breeding biology of the kittiwake Rissa tridactyla. Ibis 100, 40–51. doi: 10.1111/j.1474-919x.1958.tb00364.x
Danchin, E. (1992). The incidence of the tick parasite Ixodes uriae in kittiwake Rissa tridactyla colonies in relation to the age of the colony, and a mechanism of infecting new colonies. Ibis 134, 134–141. doi: 10.1111/j.1474-919x.1992.tb08390.x
Danchin, E., and Wagner, R. H. (1997). The evolution of coloniality: the emergence of new perspectives. Trends Ecol. Evol. 12, 342–347. doi: 10.1016/s0169-5347(97)01124-5
Danchin, E., Boulinier, T., and Massot, M. (1998). Conspecific reproductive success and breeding habitat selection: implications for the study of coloniality. Ecology 79, 2415–2428. doi: 10.2307/176832
Dardenne, S., Ducatez, S., Cote, J., Poncin, P., and Stevens, V. M. (2013). Neophobia and social tolerance are related to breeding group size in a semi-colonial bird. Behav. Ecol. Sociobiol. 67, 1317–1327. doi: 10.1007/s00265-013-1560-3
Davis, J. A., and Brown, C. R. (1999). Costs of coloniality and the effect of colony size on reproductive success in purple martins. Condor 101, 737–745. doi: 10.2307/1370060
Dochtermann, N. A., and Royauté, R. (2019). The mean matters: going beyond repeatability to interpret behavioural variation. Anim. Behav. 153, 147–150. doi: 10.1016/j.anbehav.2019.05.012
Dohm, M. R. (2002). Repeatability estimates do not always set an upper limit to heritability. Funct. Ecol. 16, 273–280. doi: 10.1046/j.1365-2435.2002.00621.x
Edelaar, P., Jovani, R., and Gomez-Mestre, I. (2017). Should I change or should I go? Phenotypic plasticity and matching habitat choice in the adaptation to environmental heterogeneity. Am. Nat. 190, 506–520. doi: 10.1086/693345
Evans, J. C., Votier, S. C., and Dall, S. R. X. (2016). Information use in colonial living. Biol. Rev. 91, 658–672. doi: 10.1111/brv.12188
Falconer, D. S., and Mackay, T. F. C. (1996). Introduction to Quantitative Genetics, 4th Edn. Harlow: Pearson Prentice Hall.
Forbes, L. S., Jajam, M., and Kaiser, G. W. (2000). Habitat constraints and spatial bias in seabird colony distributions. Ecography 23, 575–578. doi: 10.1111/j.1600-0587.2000.tb00176.x
Fretwell, S. D., and Lucas, H. L. Jr. (1970). On territorial behavior and other factors influencing habitat distribution in birds. I. Theoretical development. Acta Biotheor. 19, 1–36. doi: 10.1007/BF01601953
Gibbs, J. P., Woodward, S., Hunter, M. L., and Hutchinson, A. E. (1987). Determinants of great blue heron colony distribution in coastal Maine. Auk 104, 38–47. doi: 10.2307/4087230
Hannebaum, S. L., Brown, M. B., and Brown, C. R. (2019a). Ecological correlates of group integrity among dispersing cliff swallows. Ecosphere 10:e02913. doi: 10.1002/ecs2.2913
Hannebaum, S. L., Wagnon, G. S., and Brown, C. R. (2019b). Variation in neophobia among cliff swallows at different colonies. PLoS One 14:e0226886. doi: 10.1371/journal.pone.0226886
Höglund, J., Montgomerie, R., and Widemo, F. (1993). Costs and consequences of variation in the size of ruff leks. Behav. Ecol. Sociobiol. 32, 31–39. doi: 10.1007/bf00172220
Hoogland, J. L., Cannon, K. E., DeBarbieri, L. M., and Manno, T. G. (2006). Selective predation on Utah prairie dogs. Am. Nat. 168, 546–552. doi: 10.1086/507714
Jarman, P. J. (1974). The social organisation of antelope in relation to their ecology. Behaviour 48, 215–267. doi: 10.1163/156853974X00345
Johst, K., and Brandl, R. (1997). The effect of dispersal on local population dynamics. Ecol. Model. 104, 87–101. doi: 10.1016/s0304-3800(97)00112-9
Jovani, R., Lascelles, B., Garamszegi, L. Z., Mavor, R., Thaxter, C. B., and Oro, D. (2016). Colony size and foraging range in seabirds. Oikos 125, 968–974. doi: 10.1111/oik.02781
Kharitonov, S. P., and Siegel-Causey, D. (1988). Colony formation in seabirds. Curr. Ornithol. 5, 223–272. doi: 10.1007/978-1-4615-6787-5_5
Lessells, C. M., and Boag, P. T. (1987). Unrepeatable repeatabilities: a common mistake. Auk 104, 116–121. doi: 10.2307/4087240
Magurran, A. E., Seghers, B. H., Shaw, P. W., and Carvalho, G. R. (1995). The behavioral diversity and evolution of guppy, Poecilia reticulate, populations in Trinidad. Adv. Stud. Behav. 24, 155–202. doi: 10.1016/S0065-3454(08)60394-0
Martínez-Abraín, A., Oro, D., Forero, M. G., and Conesa, D. (2003). Modeling temporal and spatial colony-site dynamics in a long-lived seabird. Popul. Ecol. 45, 133–139. doi: 10.1007/s10144-003-0150-z
Matthiopoulos, J., Harwood, J., and Thomas, L. (2005). Metapopulation consequences of site fidelity for colonially breeding mammals and birds. J. Anim. Ecol. 74, 716–727. doi: 10.1111/j.1365-2656.2005.00970.x
Minias, P., Gach, K., Włodarczyk, R., Bartos, M., Drzewińska-Chańko, J., Rembowski, M., et al. (2020). Colony size as a predictor of breeding behaviour in a common waterbird. PLoS One 15:e0241602. doi: 10.1371/journal.pone.0241602
Mitchell, D. J., Dujon, A. M., Beckmann, C., and Biro, P. A. (2020). Temporal autocorrelation: a neglected factor in the study of behavioral repeatability and plasticity. Behav. Ecol. 31, 222–231. doi: 10.1093/beheco/arz180
Møller, A. P. (2002). Parent-offspring resemblance in degree of sociality in a passerine bird. Behav. Ecol. Sociobiol. 51, 276–281. doi: 10.1007/s00265-001-0438-y
Møller, A. P. (2010). Brain size, head size and behaviour of a passerine bird. J. Evol. Biol. 23, 625–635. doi: 10.1111/j.1420-9101.2009.01928.x
Natusch, D. J. D., Lyons, J. A., and Shine, R. (2017). Safety first: terrestrial predators drive selection of highly specific nesting sites in colonial-breeding birds. J. Avian Biol. 48, 1104–1113. doi: 10.1111/jav.01380
Nuechterlein, G. L., Buitron, D., Sachs, J. L., and Hughes, C. R. (2003). Red-necked grebes become semicolonial when prime nesting substrate is available. Condor 105, 80–94. doi: 10.1093/condor/105.1.80
Pulliam, H. R., and Caraco, T. (1984). “Living in groups: is there an optimal group size?,” in Behavioural Ecology, eds J. R. Krebs and N. B. Davies (Sunderlund, MA: Sinauer), 122–147.
Roche, E. A., and Brown, C. R. (2013). Among-individual variation in vigilance at the nest in colonial cliff swallows. Wilson J. Ornithol. 125, 685–695. doi: 10.1676/12-196.1
Roche, E. A., Brown, C. R., and Brown, M. B. (2011). Heritable choice of colony size in cliff swallows: does experience trump genetics in older birds? Anim. Behav. 82, 1275–1285. doi: 10.1016/j.anbehav.2011.09.005
Roche, E. A., Brown, C. R., Brown, M. B., and Lear, K. M. (2013). Recapture heterogeneity in cliff swallows: increased exposure to mist nets leads to net avoidance. PLoS One 8:e58092. doi: 10.1371/journal.pone.0058092
Runjaic, J., Bellovich, I. J., Page, C. E., Brown, C. R., and Booth, W. (2017). No detectable insecticide resistance in swallow bugs (Hemiptera: Cimicidae) following long-term exposure to naled (Dibrom 8). J. Med. Entomol. 54, 994–998. doi: 10.1093/jme/tjw230
Russell, G. J., and Rosales, A. (2010). Sociability leads to instability: site-switching cascades in a colonial species. Theor. Ecol. 3, 3–12. doi: 10.1007/s12080-009-0048-2
Rypstra, A. L. (1985). Aggregations of Nephila clavipes (L.) (Araneae, Araneidae) in relation to prey availability. J. Arachnol. 13, 71–78.
Safran, R. J., Doerr, V. A. J., Sherman, P. W., Doerr, E. D., Flaxman, S. M., and Winkler, D. W. (2007). Group breeding in vertebrates: linking individual- and population-level approaches. Evol. Ecol. Res. 9, 1163–1185. doi: 10.1086/698217
Scheiner, S. (2016). Habitat choice and temporal variation alter the balance between adaptation by genetic differentiation, a jack-of-all-trades strategy, and phenotypic plasticity. Am. Nat. 187, 633–646. doi: 10.1086/685812
Schippers, P., Stienen, E. W. M., Schotman, A. G. M., Snep, R. P. H., and Slim, P. A. (2011). The consequences of being colonial: allee effects in metapopulations of seabirds. Ecol. Model. 222, 3061–3070. doi: 10.1016/j.ecolmodel.2011.05.022
Serrano, D., and Tella, J. L. (2007). The role of despotism and heritability in determining settlement patterns in the colonial lesser kestrel. Am. Nat. 169, E53–E67. doi: 10.1086/510598
Sibly, R. M. (1983). Optimal group size is unstable. Anim. Behav. 31, 947–948. doi: 10.1016/S0003-3472(83)80250-4
Smith, L. C., Raouf, S. A., Brown, M. B., Wingfield, J. C., and Brown, C. R. (2005). Testosterone and group size in cliff swallows: testing the “challenge hypothesis” in a colonial bird. Horm. Behav. 47, 76–82. doi: 10.1016/j.yhbeh.2004.08.012
Spottiswoode, C. N. (2007). Phenotypic sorting in morphology and reproductive investment among sociable weaver colonies. Oecologia 154, 589–600. doi: 10.1007/s00442-007-0844-6
Spottiswoode, C. N. (2009). Fine-scale life-history variation in sociable weavers in relation to colony size. J. Anim. Ecol. 78, 504–512. doi: 10.1111/j.1365-2656.2008.01507.x
Ventura, L., Smith, D. R., and Lubin, Y. (2017). Crowding leads to fitness benefits and reduced dispersal in a colonial spider. Behav. Ecol. 28, 1384–1392. doi: 10.1093/beheco/arx106
Votier, S. C., Bearhop, S., Crane, J. E., Arcos, J. M., and Furness, R. W. (2007). Seabird predation by great skuas Stercorarius skua—intra-specific competition for food? J. Avian Biol. 38, 234–246. doi: 10.1111/j.2007.0908-8857.03893x
Wagnon, G. S., and Brown, C. R. (2020). Smaller brained cliff swallows are more likely to die during harsh weather. Biol. Lett. 16:20200264. doi: 10.1098/rsbl.2020.0264
Wilson, A. J. (2018). How should we interpret estimates of individual repeatability? Evol. Lett. 2, 4–8. doi: 10.1002/evl3.40
Wolak, M. E., Fairbairn, D. J., and Paulsen, Y. R. (2012). Guidelines for estimating repeatability. Methods Ecol. Evol. 3, 129–137. doi: 10.1111/j.2041-210X.2011.00125.x
Keywords: cliff swallow, coloniality, group size, Petrochelidon pyrrhonota, repeatability, social behavior
Citation: Brown CR and Hannebaum SL (2022) Birds of a Feather Flock Together: Extent of Long-Term Consistency of Colony-Size Choice in Cliff Swallows. Front. Ecol. Evol. 10:860407. doi: 10.3389/fevo.2022.860407
Received: 22 January 2022; Accepted: 23 February 2022;
Published: 24 March 2022.
Edited by:
Leticia Aviles, University of British Columbia, CanadaReviewed by:
Daniel Steven Cooper, Natural History Museum of Los Angeles County, United StatesCopyright © 2022 Brown and Hannebaum. This is an open-access article distributed under the terms of the Creative Commons Attribution License (CC BY). The use, distribution or reproduction in other forums is permitted, provided the original author(s) and the copyright owner(s) are credited and that the original publication in this journal is cited, in accordance with accepted academic practice. No use, distribution or reproduction is permitted which does not comply with these terms.
*Correspondence: Charles R. Brown, Y2hhcmxlcy1icm93bkB1dHVsc2EuZWR1
Disclaimer: All claims expressed in this article are solely those of the authors and do not necessarily represent those of their affiliated organizations, or those of the publisher, the editors and the reviewers. Any product that may be evaluated in this article or claim that may be made by its manufacturer is not guaranteed or endorsed by the publisher.
Research integrity at Frontiers
Learn more about the work of our research integrity team to safeguard the quality of each article we publish.