- 1Institute of Geography and Geology, University of Greifswald, Greifswald, Germany
- 2Institute of Earth Sciences, University of Iceland, Reykjavík, Iceland
- 3Institute of Geophysics and Geology, University of Leipzig, Leipzig, Germany
- 4Universalmuseum Joanneum, Department for Geology and Palaeontology, Graz, Austria
- 5Institute of Earth Sciences, NAWI Graz Geocenter, University of Graz, Graz, Austria
Body-size variability results from a variety of extrinsic and intrinsic factors (environmental and biological influences) underpinned by phylogeny. In ostracodes it is assumed that body size is predominantly controlled by ecological conditions, but investigations have mostly focused on local or regional study areas. In this study, we investigate the geographical size variability (length, height, and width) of Holocene and Recent valves of the salinity-tolerant ostracode species Cyprideis torosa within a large geographical area (31°–51° latitude, and 12°–96° longitude). It is shown that distant local size clusters of Cyprideis torosa are framed within two large-scale geographical patterns. One pattern describes the separation of two different size classes (i.e., morphotypes) at around ∼42° N. The co-occurrence of both size morphotypes in the same habitats excludes an environmental control on the distribution of the morphotypes but rather could point to the existence of two differentiated lineages. Generally, correlations between valve size and environmental parameters (salinity, geographical positions) strongly depend on the taxonomic resolution. While latitude explains the overall size variability of C. torosa sensu lato (i.e., undifferentiated for morphotypes), salinity-size correlations are restricted to the morphotype scale. Another large-scale pattern represents a continuous increase in valve size of C. torosa with latitude according to the macroecological pattern referred as Bergmann trend. Existing explanations for Bergmann trends insufficiently clarify the size cline of C. torosa which might be because these models are restricted to intraspecific levels. The observed size-latitude relationship of C. torosa may, therefore, result from interspecific divergence (i.e., size ordered spatially may result from interspecific divergence sorting) while environmental influence is of minor importance. Our results imply that geographical body-size patterns of ostracodes are not straightforward and are probably not caused by universal mechanisms. Consideration of phylogenetic relationships of ostracodes is therefore necessary before attempting to identify the role of environmental controls on body size variability.
Introduction
Body size is a master trait of an organism, because it influences nearly every aspect of the biology of an organism (Verberk et al., 2021). It is determined by and/or responds to extrinsic environmental factors (abiotic and biotic) and intrinsic (biological) influences that reflect direct functional and/or evolutionary effects, all further underpinned by phylogeny (Hart and Bychek, 2011). These common underlying causes and processes result in consistent large-scale patterns across temporal, spatial or taxonomical scales. One of the longest and best-known ecological generalizations is that large body size is typically found at higher latitudes, or in colder climates as first described by Bergmann (1847)) for mammals. Bergmann’s rule has also been demonstrated to apply to some groups of ectotherms, but observed patterns and possibly underlying mechanisms remain elusive (Ashton and Feldman, 2003; Blanckenhorn et al., 2006; Tesche and Hodges, 2015; Maszczyk and Brzeziński, 2018).
Within the crustaceans, ostracodes are exceptionally well-suited for the investigation of Bergmann clines due to their high taxonomic diversity (ca. 2000 extant non-marine species; Martens et al., 2008), an extensive fossil record (Horne, 2005; Martens et al., 2008), and an extraordinary diversity of life-history strategies (Chaplin et al., 1994; Butlin et al., 1998; Geiger et al., 1998). This, in combination with their relatively short generation time, denote ostracodes as valuable model organisms to study fundamental evolutionary questions (Fernandes Martins et al., 2009). Moreover, the ubiquitous distribution of ostracodes in nearly all freshwater and marine habitats, but also interstitial and semi-terrestrial environments (Horne et al., 2002) allows to investigate geographical variation of body-size traits in various environments (e.g., marine vs. freshwater; tropical vs. temperate).
However, ostracodes (and crustaceans in general) were neglected in research of macroecological patterns so far. Therefore, there is poor knowledge on effects of geographic variation on overall ostracode size. Actually, ostracode-body size was mainly investigated with respect to ecophenotypical responses which are mostly based on local or small regional scales and/or related to single environmental parameters (e.g., Yin et al., 2001). These studies have shown that ostracode size is influenced by salinity (Boomer et al., 2017), temperature (Martens, 1985), as well as pH and CaCO3 concentration (Kim et al., 2015). Hitherto, Bergmann trends were detected only in deep-sea species (Hunt and Roy, 2006; Hunt et al., 2010).
In this study, we investigate geographical size variation of an ostracode taxon generally assigned to the species Cyprideis torosa. Cyprideis torosa was chosen because it has a large geographical range which covers the Eurasian realm and Africa (Wouters, 2017; Figure 1) where it occurs in freshwater to hypersaline habitats and is characterized by tolerance of a wide temperature range and various oxygen and substrate conditions (De Deckker and Lord, 2017). In addition, high abundance of valves in Quaternary deposits (e.g., West et al., 1994; Pint et al., 2012) enables examination of body-size traits on different temporal scales.
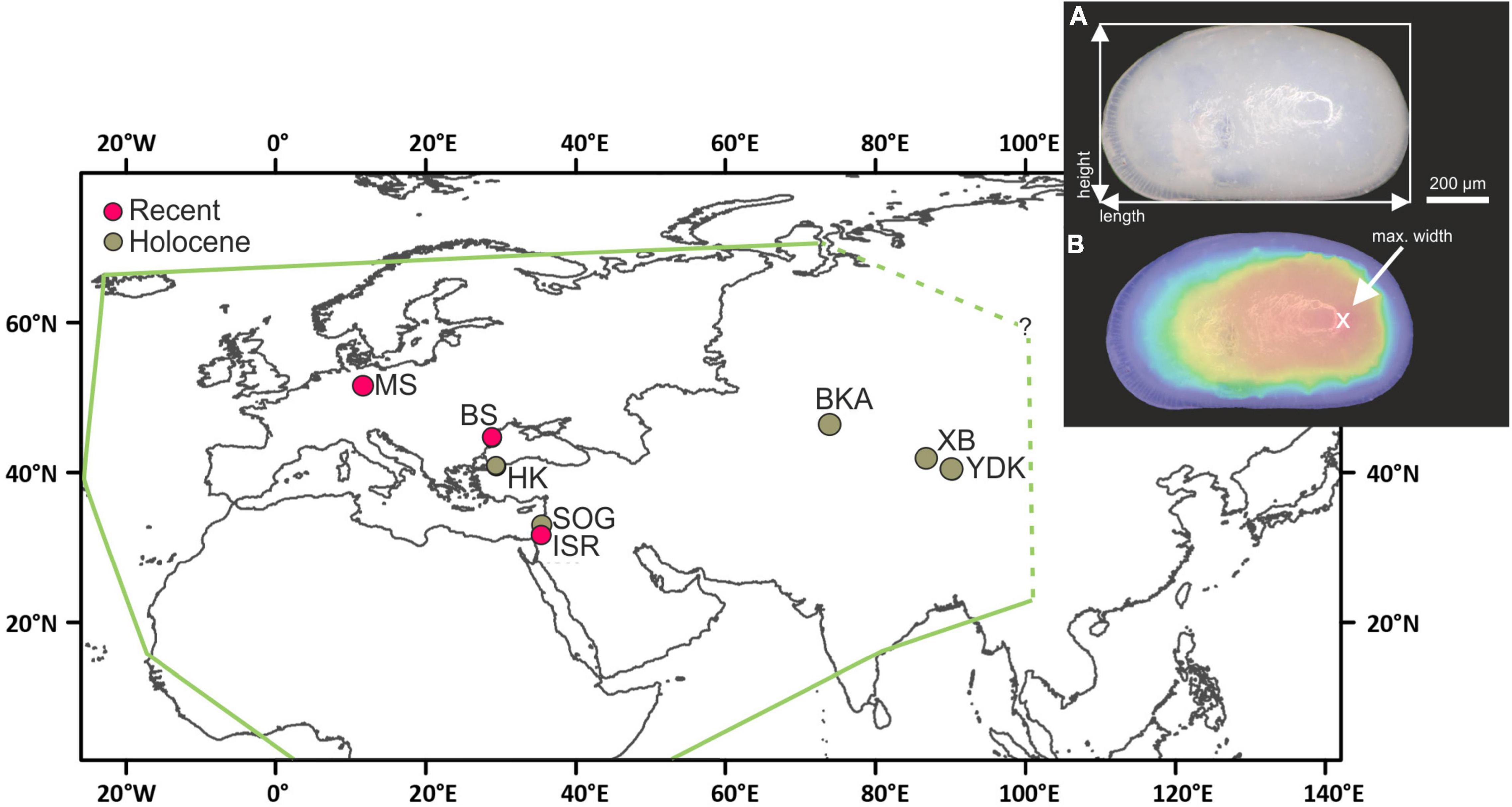
Figure 1. Map of the Eurasian realm, showing the localities (YDK, Tarim Basin, China; XB, Lake Bosten, China; BKA, Lake Balkhash, Kazakhstan; ISR, Dead Sea, Israel; SOG, Sea of Galilee, Israel; BS, Danube Delta, Romania; HK, Hersek lagoon, Turkey; MS, Mansfeld Lakes, Germany) of investigated Cyprideis valves and the geographical range of Cyprideis torosa according to Wouters (2017) (green line). Inset displays measured size traits on Cyprideis valves. Length and height were measured as maximum extension of the valve outline (A). Valve width was obtained as maximum height of focus-stacked photographs (B). Displayed are female left valves, measurements were, however, identical for male left and right valves.
We investigate valve-size variability of C. torosa along a 20° latitudinal range to address questions related to evolution of body-size clines in non-marine ostracodes. In order to understand if and how specific these relationships are, we compare the new information with a dataset of the Neotropical species Cytheridella ilosvayi (Wrozyna et al., 2016, 2018, 2019). Questions addressed include: (1) How does size vary within C. torosa’s geographical range and the Holocene timescale? (2) Is there a clinal variation in body size (valve length, height, and width) of C. torosa across latitude? (3) Is there a size variation in the species according to salinity?
Materials and Methods
Sampling Sites
Our study area was chosen with respect to cover inland occurrences and combination of fossil and Recent material. The study area includes nine locations and represents a wide geographical range from ca. 31° to 51° N and 12° to 96° E (Germany, Romania, Turkey, Israel, Kazakhstan, China), coinciding roughly with the known geographical range of the species in Europe and Asia (Wouters, 2017; Figure 1).
The included ostracod material covers a stratigraphic range from Middle Pleistocene to Recent (Table 1). Majority of the localities represents distinct inland occurrences. Only coastal lakes [Hersek lagoon (HK) and Danube Delta (BS)] probably have at least a temporary connection to marine conditions. Some localities are represented by more than one sample either from different water bodies within a small geographical region or from different stratigraphic levels of a sedimentary sequence (Table 1). Since the foremost question of this study addresses the variation between populations and/or localities, all samples from one locality or nearby locations where habitat connectivity is theoretically possible (e.g., the ISR samples from the wetland Enot Zuqim, Israel; Table 1) were pooled. Recent Cyprideis valves are derived from bio- and taphocenoses.
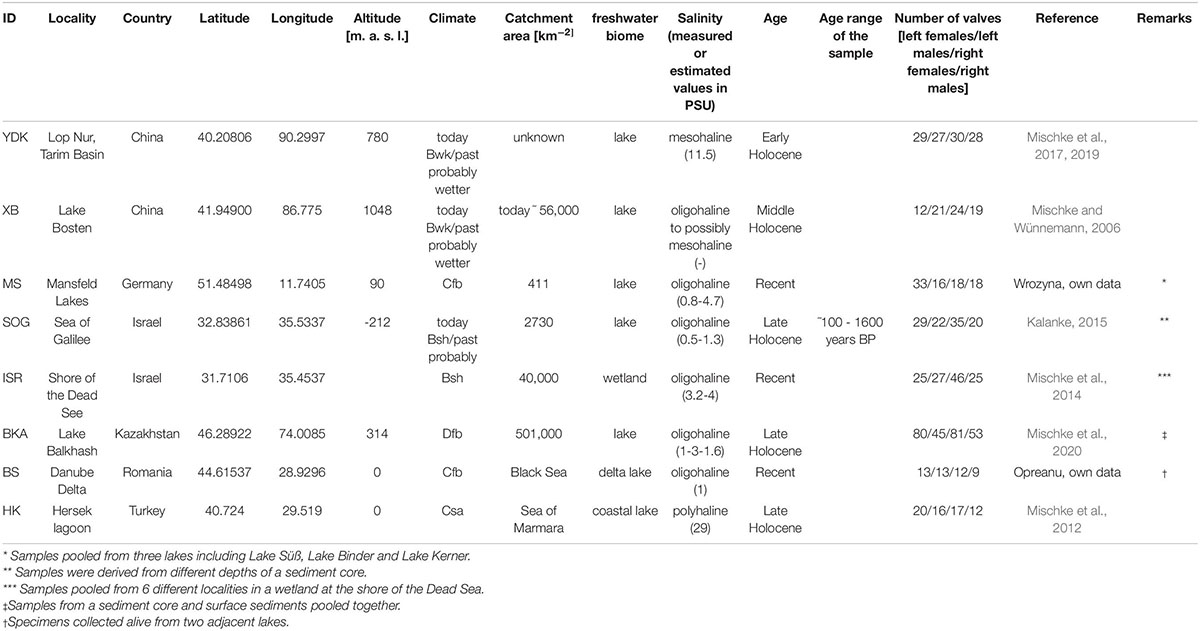
Table 1. Sample-locality information for the investigated valves of Cyprideis torosa. Classification of the climate follows the Köppen system.
Salinity data of modern populations of Mansfeld Lakes (MS) and Enot Zuqim (ISR) were measured in situ in the field with hand-held probes. Estimation of paleo-specific conductivity for the fossil localities of Lake Balkhash (BKA), Sea of Galilee (SOG), and Tarim Basin (YDK) were derived by the application of ostracode-based transfer functions established for the Near East and the Tibetan Plateau. These transfer functions are calibrated with modern quantitative relationships between species compositions and related conductivity values. Applied by different statistical approaches (e.g., weighted-averaging partial least squares regression) to fossil species assemblages, reliable estimates of past conductivity can be derived (Kalanke, 2015; Mischke et al., 2019, 2020). Since conductivity values are calculated for each stratigraphic layer based on relative abundances of ostracode species, assemblage variations in a sedimentary sequence of a specific locality typically result in variations of the calculated conductivity values. Variation ranges are, however, small varying between 0.5 and 1.3 PSU for samples of Sea of Galilee (SOG) and 1.5 and 1.6 PSU for Lake Balkhash (BKA).
Hersek lagoon (HK) is located at the eastern Marmara Sea. The fossil record continuously indicates polyhaline conditions. Samples were derived from the upper part of a sediment core dating back to ∼1200 years BP. Since the composition of the ostracode fossils did not change during the considered time a salinity value of today (29 PSU; Mischke et al., 2012) was assumed as reliable estimate of the paleo-salinity. A salinity estimate for the modern samples from the Danube Delta (BS) was taken from the literature (van de Velde et al., 2019). Samples from Lake Bosten (XB) were excluded from size-salinity regression analyses since no salinity data were available. Recorded specific conductivity data were converted to salinity for comparison with published salinity data using the factor of 0.65 (Hem, 1982; Table 1).
Morphological Variables
All adult specimens were sorted under a binocular microscope and photographed with a digital Keyence VHX-950F microscope. Photographs of left and right valves of females and males representatives for each locality are provided in the Supplementary Figure 1. Measurements of maximum length, height, and width were completed directly via the Keyence software (VHX-7000 Series Software) on screen (Figures 1A,B). Valve-width measurements were obtained as “highest points” on focus-stacked photographs which were automatically merged by the software of the microscope. This approach for the measurement of valve width was not yet used for ostracodes. Due to dimorphism, both sexes and both left and right valves were investigated.
Statistical Analyses
Prior to statistical analyses, size, salinity, and geographical data were log-transformed and all analyses were done separately for the four sub-data sets of female and male left and right valves. Site-specific size variability was tested for normal distribution by Shapiro Wilk’s tests separately for length, height and width. Multivariate normality was tested on overall data sets (length, height, width) by Mardia test of multivariate skewness and kurtosis followed by Doornik and Hansen omnibus tests. Principal component analyses were used to explore the overall size variability in relation to morphotypes distribution. Classification of the specimens according to a morphotype group was based on histograms of length. Threshold values for the differentiation of the morphotypes were derived from the areas (i.e., bins) with minimal overlapping of both size groups in the histograms. As a Permutational Multivariate Analysis of Variance (PerMANOVA) assumes no (normal) distribution it was selected for testing of significant differences between groups (i.e., morphotypes, sites) based on all size traits (length, height, width). Pairwise comparisons (i.e., post hoc analyses) were done by pairwise Hoteling’s tests of each site. Relationships between salinity and size traits were investigated by linear regressions on log-transformed salinity and size data. Redundancy analyses (RDA) were performed to assess which of the investigated potential explanatory variables, geographical region (i.e., latitude and longitude) or salinity, provide largest explanatory power on the size data (length, height, width) of C. torosa. All statistical analyses were done with the software PAST (version 4.09; Hammer et al., 2001).
Results
Size Variability
In total 875 valves composed of 504 females and 371 males were investigated. Minimum sample sizes of the different sites range from 12 and 13 valves of females (left and right valves, respectively) and 12 and 9 male valves (left and right, respectively) to maximum samples sizes of 80 and 81 female valves (left and right, respectively) and 46 and 53 male valves (left and right, respectively). The mean number of investigated valves per sample is 109 (left and right males and females together). With a total of 259 valves, Lake Balkhash provided largest total sample size (161 female valves and 98 male valves). Lowest valve numbers were provided by the sample of the Danube Delta (BS) with a total of 47 valves (25 females and 22 males; Table 1).
The valve length of adult left and right valves of female C. torosa varies from 742 to 1087 μm and 723 to 1049 μm, respectively. Male specimens show ranges from 861 to 1182 μm and 795 to 1155 μm for left and right valves, respectively. The width of left and right valves of female specimens is in a range from 166 to 307 μm and 140 to 287 μm, respectively. Male valves vary between 121 and 299 μm (left valves) and 127 to 302 μm (right valves). Generally, right valves of both males and females are smaller and less vaulted outside (Figures 2A,B).
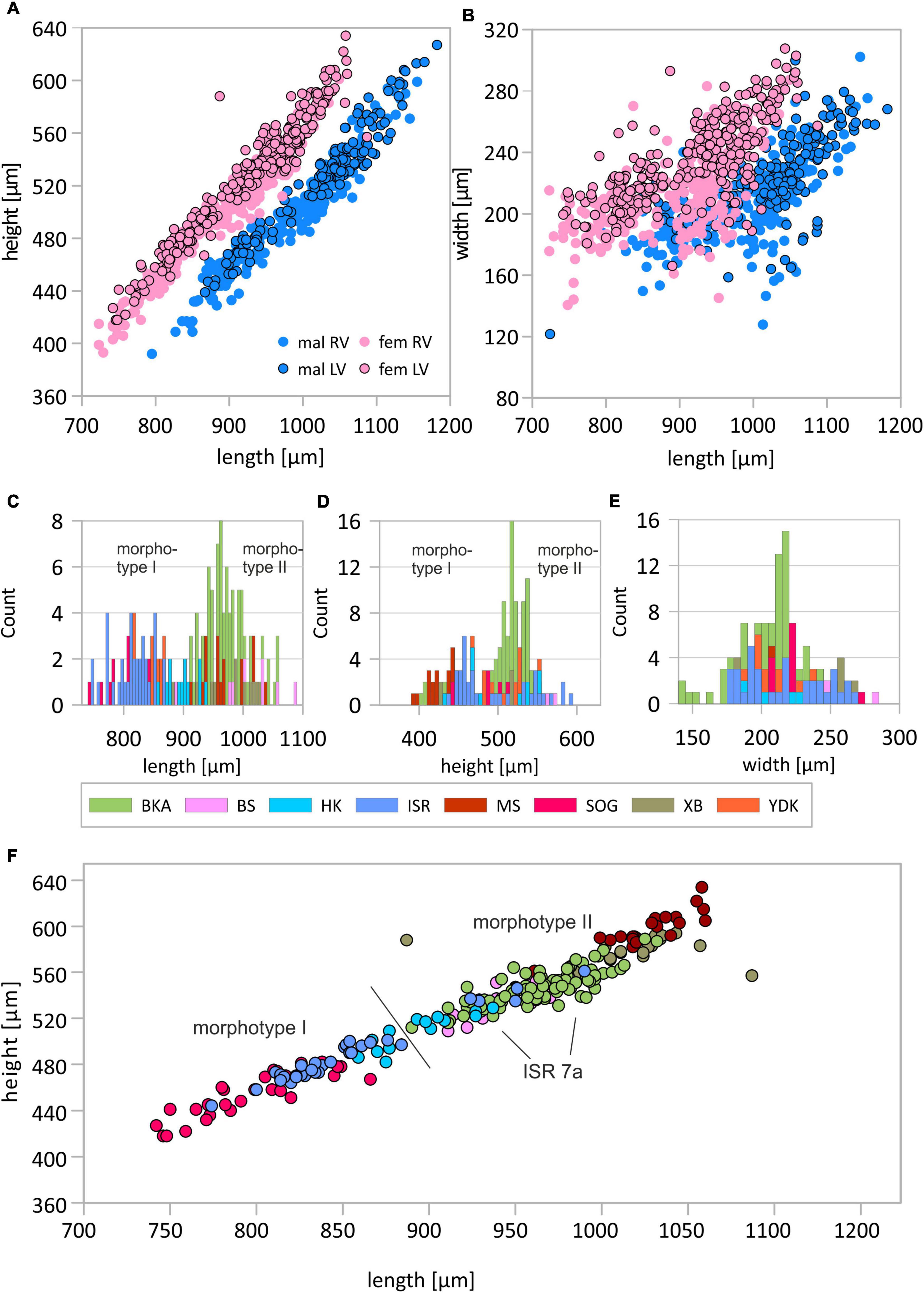
Figure 2. Length-height (A) and length-width (B) plots for female and male left and right valves (LV and RV, respectively). Distribution of size data presented as histograms of valve length (C), valve height (D), valve width (E), and length-height plot in which populations are indicated (F). Population abbreviations are: YDK, Tarim Basin, China; XB, Lake Bosten, China; BKA, Lake Balkhash, Kazakhstan; ISR, Dead Sea, Israel; SOG, Sea of Galilee, Israel; BS, Danube Delta, Romania; HK, Hersek lagoon, Turkey; MS, Mansfeld Lakes, Germany. Details about the localities are provided in Table 1. Displayed are data of female left valves, other datasets are available in the Supplementary Material.
According to a Shapiro–Wilk test most traits and samples exhibit normal distribution (α = 0.05; Table 2). Size traits differ with respect to their normal distribution. While length and height display normal distributions for the majority of samples the number of normal distributed samples is lower for valve width. Not normally distributed samples are majorly from Enot Zuqim (ISR) and Lake Balkhash (BKA; Table 2). Mardia tests for multivariate normality indicate that the overall data sets of left and right females and left and right males are not multivariate normally distributed (female LV: skewness: 2.683, p ≤ 0.001; kurtosis: 29.86, p = 0; Doornik and Hansen omnibus E = 128.2, p ≤ 0.001; female RV: skewness: 1.935, p ≤ 0.001; kurtosis: 18.24, p ≤ 0.001; Doornik and Hansen omnibus E = 26.17, p ≤ 0.001; male LV: skewness: 3.835, p ≤ 0.001; kurtosis: 21.4, p ≤ 0.001; Doornik and Hansen omnibus E = 40.37, p ≤ 0.001; male RV: skewness: 1.935, p ≤ 0.001; kurtosis: 18.24, p ≤ 0.001; Doornik and Hansen omnibus E = 26.17, p ≤ 0.001).
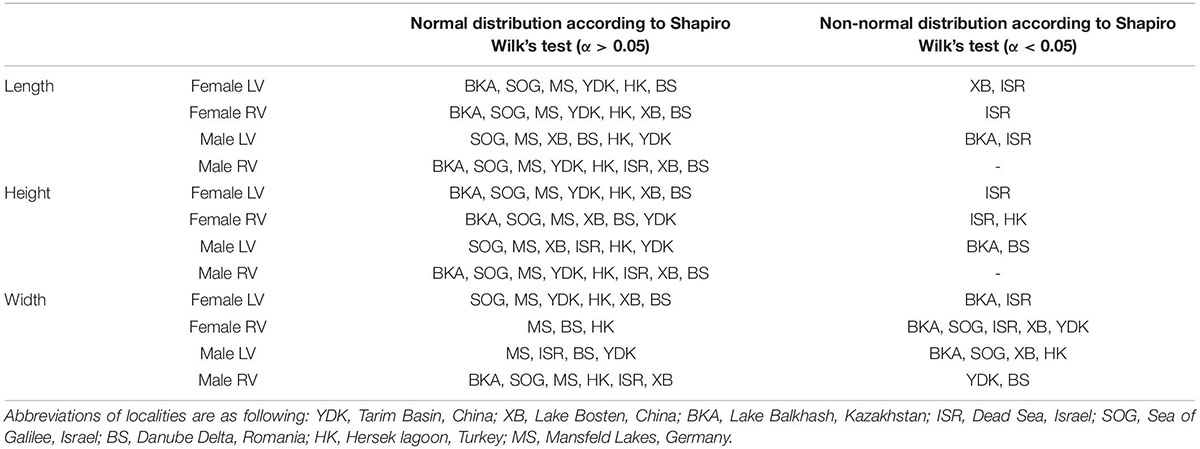
Table 2. Overview of Shapiro Wilk’s tests for normal distribution separately for length, height, and width of female and male left (LV) and right (RV) valves.
While there is a distinct linear relationship between length and height (r-values for all relationships ≥ 0.96, p ≤ 0.001), the regression relationship is slightly reduced between length and width (r-values between 0.63 and 0.75; p ≤ 0.001).
Although variance becomes generally smaller with larger sample sizes, there are exceptions as for instance in samples of Enot Zuqim (ISR) in which large variation ranges are caused by presence of two unevenly distributed size morphotypes (Figure 2F). In both length-height and length-width plots, it is indicated that C. torosa represents two size classes or morphotypes (Figure 2). The existence of two morphotypes is also expressed in bimodal distributions of length and height data (Figure 2). Means of the morphotypes’ length differ significantly with the smaller morphotype I providing for females left and right valves 829 and 803 μm, and for male left and right valves 929 and 897 μm (also Figure 2; Supplementary Tables 1–4). The larger morphotype II is characterized by mean length of female left and right valves of 952 and 937 μm, and male left and right valves display mean lengths of 1040 and 1023 μm. Valve width generally does not support a separation in two or more size classes (Figure 2E). Samples from Enot Zuqim (ISR) and Sea of Galilee (SOG) show in the width data an ambiguous pattern slightly following a bimodal distribution as length and height. Regression lines of length-height relationships are similar for both morphotypes. Divergence occurs by proportional differences in valve width-length relationships. Morphotype I is, thus, characterized by slightly larger width relative to the valve length if compared to the larger morphotype II. Also, correlation coefficients for the width-length relationship are higher for morphotype II (Table 3).
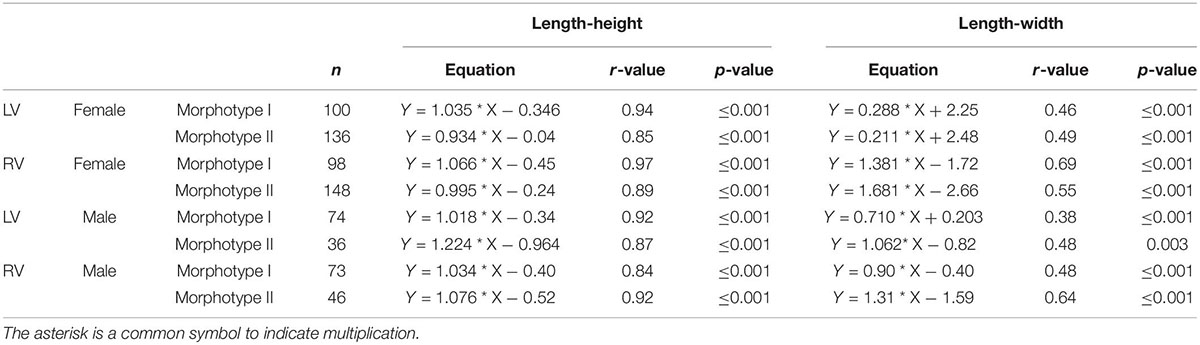
Table 3. Differences of length-height regression coefficients and slopes of both Cyprideis morphotypes indicated for both sexes and left (LV) and right (RV) valves.
The first two axes of the PCAs performed on all size traits explain in all sub-data sets >98% of the total variance (Figure 3). PC 1 explains between 80.8% (female right valves) and 87.9% (female left valves; 83.2% male left valves; 81.9% male right valves) and is in all analyses evenly high correlated with length, height, and width (r-values range for length between 0.87 and 0.94, for height between 0.88 and 0.95, and for width between 0.89 and 0.93). Scatter plots disclose the disjunct size variability of the morphotypes with morphotype I displaying negative scores on PC 1 and morphotype II having positive scores on PC 1. As indicated by histograms and length-height plots valves from Hersek lagoon (HK) provide an intermediate position in the scatter plots caused by co-occurrence of both morphotypes. PerMANOVA results showed that morphotypes are significantly different (females RV: total sum of squares = 1.419, within-group sum of squares = 0.695, F = 257.0, p < 0.001; females LV: total sum of squares = 1.375, within-group sum of squares = 0.534, F = 391.4, p < 0.001; males RV: total sum of squares = 0.978, within-group sum of squares = 0.551, F = 138.6, p < 0.001; males LV: total sum of squares = 0.844, within-group sum of squares = 0.404, F = 198.7, p < 0.001).
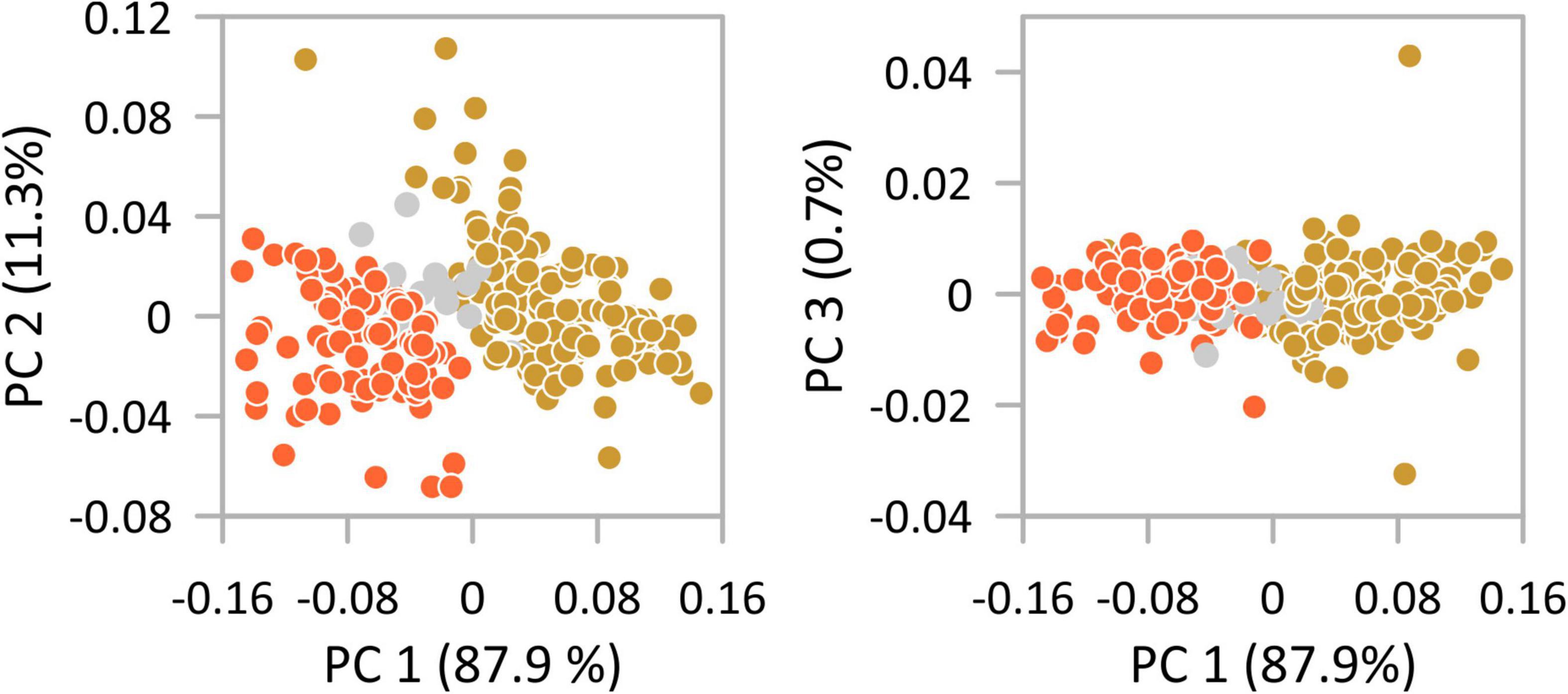
Figure 3. Principal component analysis of the variability of size traits (length, height, width) of females left valves of Cyprideis torosa. Indicated is the small morphotype I (orange) and the larger morphotype II (gold). Valves of Hersek lagoon (HK) where both morphotypes co-occur are highlighted in gray.
Latitudinal Variability of Size
Generally, largest valves occur in Mansfeld Lakes (MS) followed by Lake Bosten (XB), Lake Balkhash (BKA), Black Sea (BS), and Hersek lagoon (HK). Smallest valves occur in Enot Zuqim (ISR), Tarim Basin (YDK), and Sea of Galilee (SOG; Supplementary Figures 2, 3). Thereby, the cluster of the smaller valve sizes (i.e., morphotype I) includes all valves from Sea of Galilee (SOG) and Tarim Basin (YDK). Valves of Hersek lagoon and Enot Zuqim (ISR) contribute to both clusters. While valve sizes for Hersek lagoon can be considered intermediate, probably including both morphotypes, the data for Enot Zuqim represent two distinctly separated size classes in which only one locality contains solely valves of the larger size cluster while the other localities include only the valves sizes according to the smaller cluster (Figure 2F).
Within-sample variability of length shows similar ranges for males and females between 59 and 178 μm and 45 to 220 μm, respectively. Small sample sizes generally provide lower differences (e.g., XB). Maximum length differences are recorded for HK (males) and XB (females). Absolute differences for valve width range for males and females between 59 and 178 μm and 32 and 121 μm, respectively. ISR provides the largest within-sample variability for width of male and female valves (Supplementary Figures 2B, 3).
PerMANOVA results showed that the sites are significantly different (females RV: total sum of squares = 1.419, within-group sum of squares = 0.570, F = 51.24, p < 0.001; females LV: total sum of squares = 1.375, within-group sum of squares = 0.388, F = 87.86, p < 0.001; males RV: total sum of squares = 0.997, within-group sum of squares = 0.284, F = 62.60, p < 0.001; males LV: total sum of squares = 0.844, within-group sum of squares = 0.243, F = 62.24, p < 0.001). Pairwise comparisons (i.e., post hoc tests) confirmed that valve size is different between the sites. Exceptions represent sample pairs BKA and BS, and ISR and YDK, which are not significantly different in terms of their size characteristics (Supplementary Figure 2).
Size in terms of length, height and width of morphotypes is moderately to not correlated to latitude. The r-values are very variable ranging between r = 0.02 and r = 0.64 with a mean of r = 0.25 (Table 4). In contrast, considering length and height of C. torosa valves as one group they are correlated with latitude (Figure 4), with correlation coefficients of r ≥ 0.77 for all data sets (female and male left and right valves; Table 4). Width displays lower and more heterogeneous r-values between males and females. While r-values of latitude-width for males are almost identical (r = 0.43 and r = 0.49, respectively) females provide a relatively large range around the values of the males. Thus, the highest correlation is exhibited by left female valves (r = 0.57) in contrast to a relatively low r-value shown by right female valves (r = 0.34).
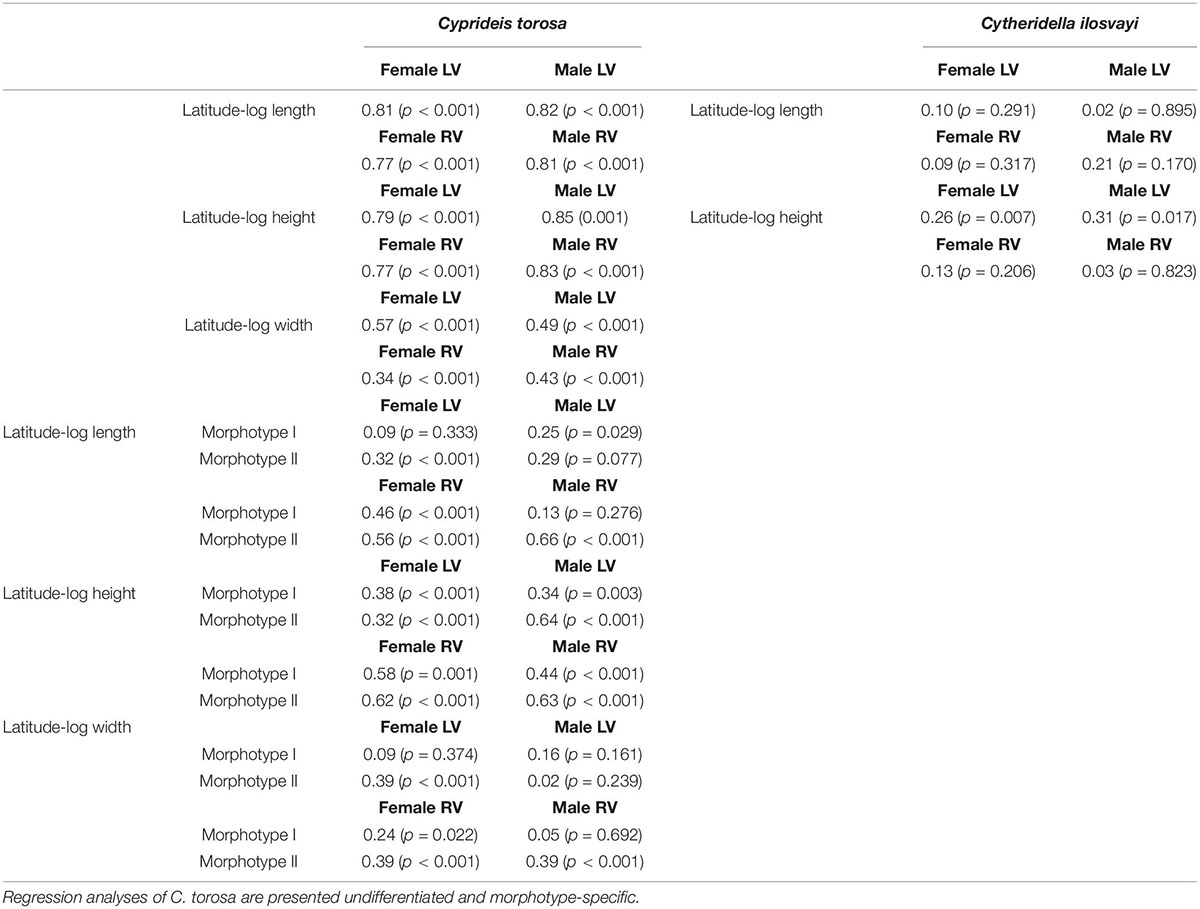
Table 4. Regression coefficients of log-transformed size-latitude relationships for Cyprideis torosa and Cytheridella ilosvayi separated for sexes and left and right valves (LV and RV, respectively).
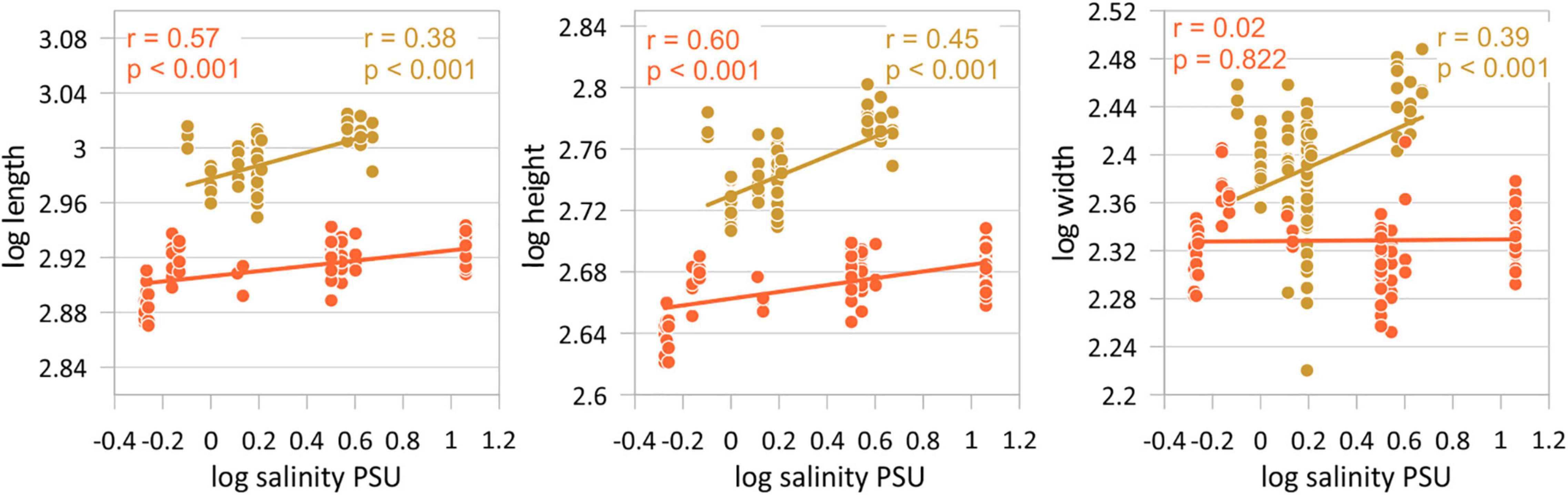
Figure 4. Correlations between valve length, height, and width and salinity separately for both morphotypes (orange = morphotype I, gold = morphotype II). Displayed are female left valves. Results of the other correlation analyses are given in Table 4.
Size-Salinity Relationships
Variation of valve size in relation to salinity reveals an opposite relationship to that observed in the latitude-size relationship, but this also depends on whether morphotypes are considered or not. Correlation coefficients between valve size traits and salinity are low if C. torosa is considered undifferentiated for morphotypes (r-values range between 0 and 0.16) of both female and male valves. Correlation coefficients are distinctly higher if the relationships are considered separately for both morphotypes. Thus, size traits of both morphotypes of C. torosa are positively correlated with salinity. This is particularly true for length and height. Valve width displays only for morphotype II a weak positive relationship with salinity (Figure 5 and Table 5).
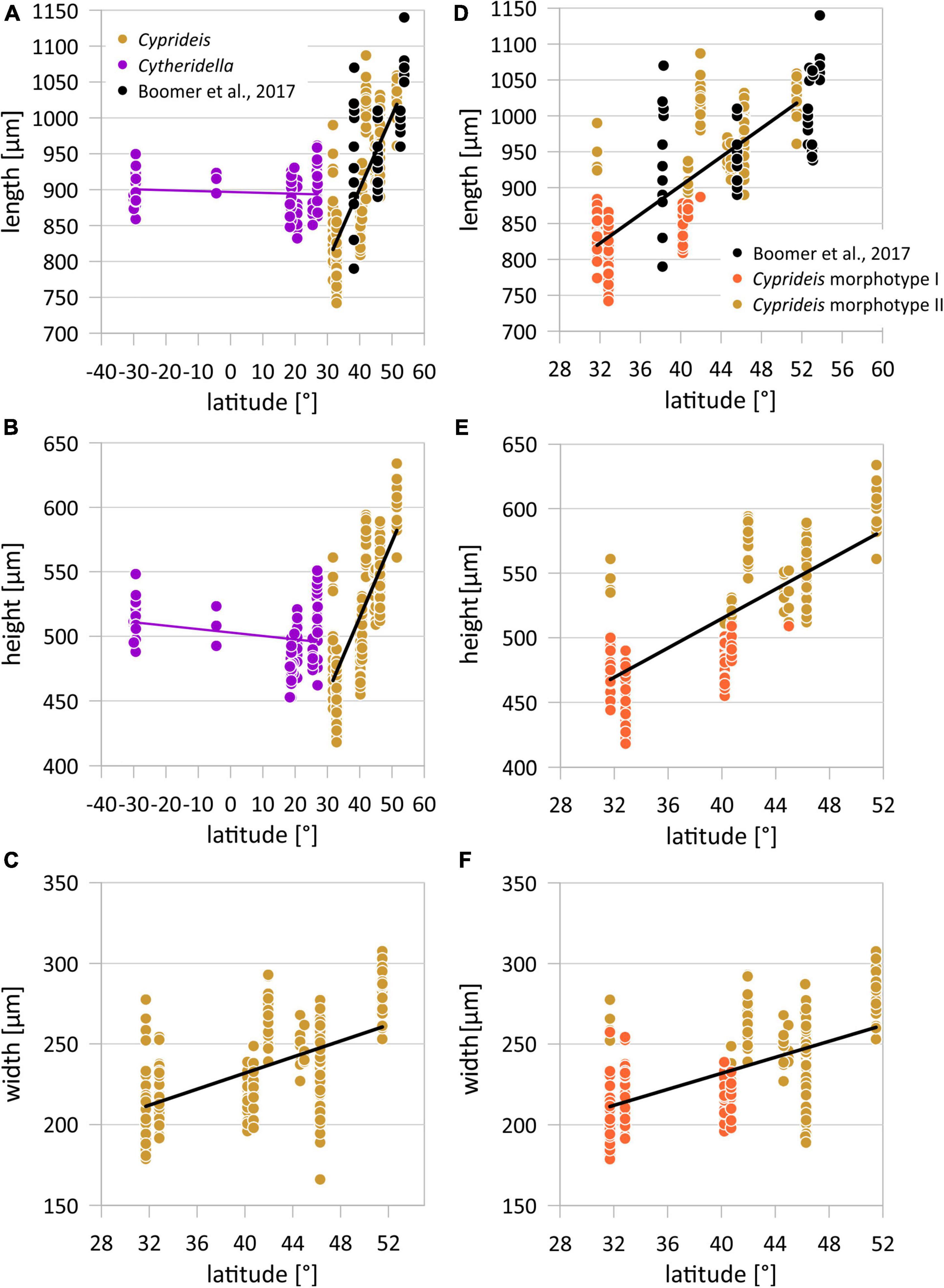
Figure 5. Latitudinal variation of valve length, height, and width of Cyprideis torosa and length and height of Cytheridella ilosvayi (A–C). Latitudinal distribution of Cyprideis size traits differentiated for morphotype I and II (D–F).
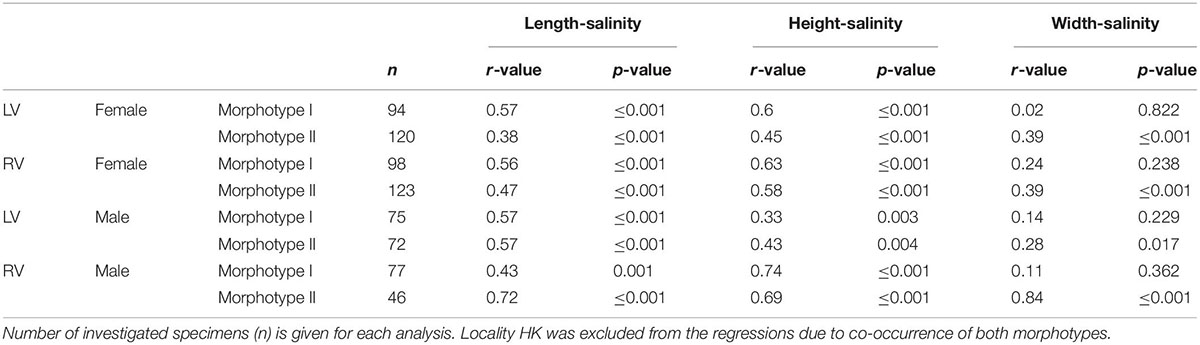
Table 5. Regression coefficients of log-transformed size and salinity data for females and males left (LV) and right valves (RV).
The RDA explains between 37.5% (female right valves) and 49.9% (female left valves; 44.4% male left valves; 46.4% male right valves) of the variability in the size data. Variabilities explained by the RDA axes 1–3 were highly significant at p = 0.001. The explanatory percentages of the axes differ greatly with very low contributions of the second and third axes to the overall variability (<0.6% and <0.8% of left female and male valves; and 2 and 2.2% of right female and male valves), respectively. According to the RDA biplots, latitude has the strongest influence on valve length and height while salinity and longitude are not relevant for the overall variability in size data. Valve width is not controlled by geographical area and salinity (Figure 6).
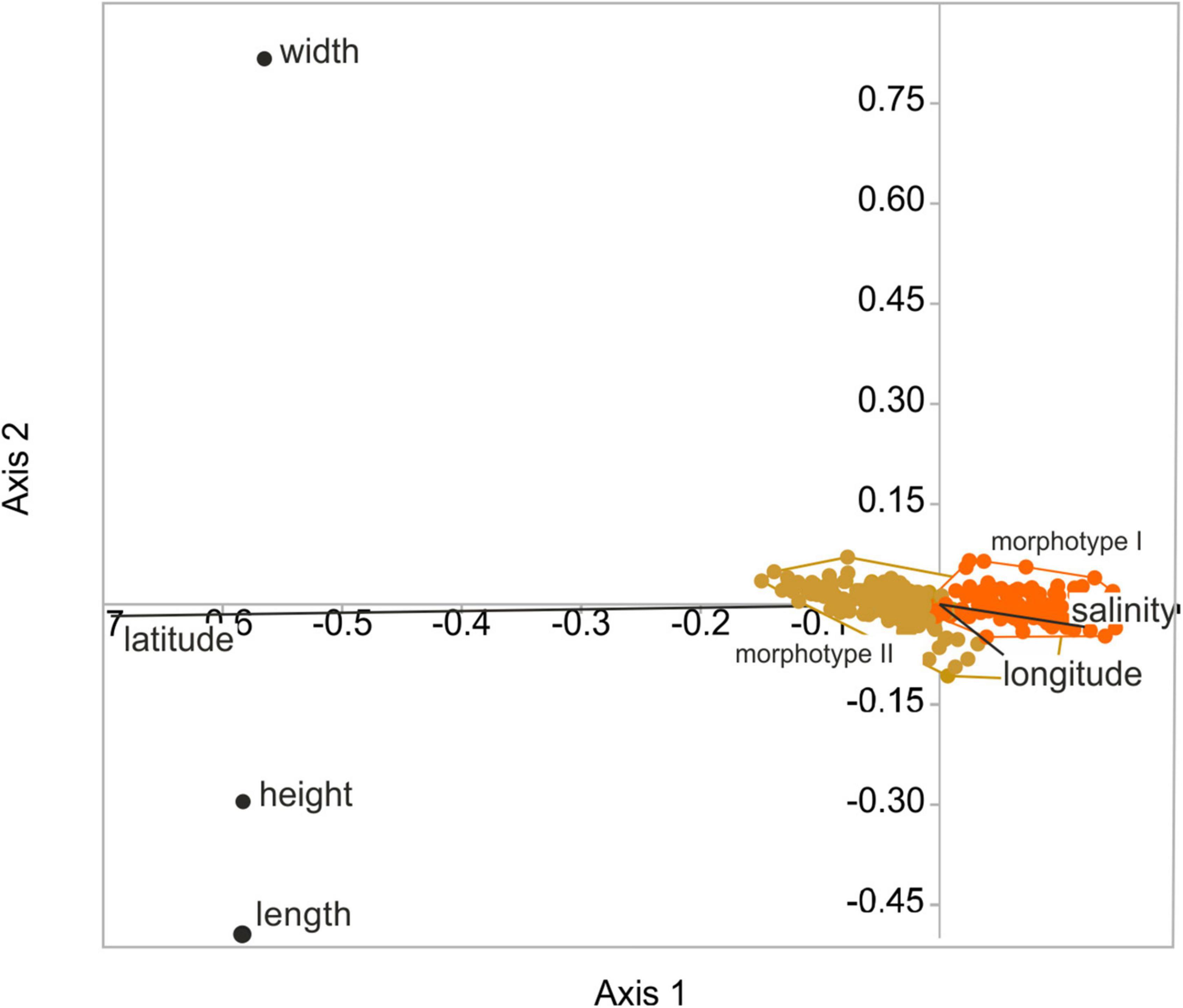
Figure 6. Results of the redundancy analysis (RDA) of valve size (length, height, width) and geographical area (latitude, longitude) displayed for female left valves differentiated for size morphotypes (orange = morphotype I, gold = morphotype II; adjusted r2 = 0.50).
Discussion
Valve-Size Variability
Cyprideis torosa s.s. occurs at the beginning of the Calabrian Stage (middle Early Pleistocene) (Gliozzi et al., 2017) and persists until present day without known speciation as documented from African Cyprideis (Wouters and Martens, 1994, Wouters and Martens, 2001; Schön et al., 2000). Therefore, all fossil and Recent records are considered the same species and it is not reasonable to treat them differently. Additionally, our results show no pattern in valve size related to Holocene temperature conditions (see below), so the combination of Recent and fossil records is not expected to promote any of the patterns found in the dataset.
The observed size-variation ranges within samples as well as the overall data set of C. torosa fits very well to known size-variability patterns of modern C. torosa (Boomer et al., 2017) and fossil Cyprideis species (Gitter et al., 2015). It is somewhat surprising that the length variation detected by Berndt et al. (2019) of 770–1036 μm for females and 903–1106 μm for males, respectively, almost completely matches our data range although these data are derived from a single sediment-core location covering the last ∼7800 years. This similarity highlights not only the large local variation in size over time, but also that (large) spatial and (long) temporal variations can have similar amplitudes. Actually, it is assumed that geographical and temporal changes in body size are manifestations of the same drivers (Yom-Tov and Geffen, 2011).
As shown by the length-width plot (Figure 2B), morphotypes are differentiated not only by length but also by proportions in valve length and width expressed by differences in the regression slopes (Table 3). Morphotype I displays, thus, slightly larger width relative to the valve length compared to morphotype II. This indicates that valve shape could be also different.
Size-Latitude Relationships
So far, data sets of geographical size variability of ostracode species are mostly restricted to relatively small (i.e., regional) scales. Our size data revealed two large-scale geographical patterns. The first is the almost complete separation in two size classes at around ∼42° N (Figures 5A–C), and the second is that clinal variation of body size of C. torosa is consistent to the Bergmann trend.
While the Bergmann trend describes a successive increase of valve length with latitude, Cyprideis also shows a differentiation between smaller and larger size populations (i.e., morphotypes I and II) (Figures 2,3,6). This could be an additional size cline representing, e.g., the adaptation to different environmental conditions as observed in other organisms (Bai et al., 2016). However, localities Enot Zuqim (ISR) and Hersek lagoon (HK) contain both morphotypes, which refuses an ecological control.
Co-occurrence of two size groups can result from different generations per year which is in turn affected by length of the warm season. Thus, populations in warmer areas with a longer summer period produce two generations per year, and oppositely, only one generation per year occurs due to a shorter warm period in colder regions. This seasonal differentiation is often accompanied by different body sizes related to differences in the developmental time (Aguilar-Alberola and Mesquita-Joanes, 2014; Wȩsławski et al., 2020). Actually, life history and/or seasonal population structure of C. torosa is ambiguous. While Mediterranean populations clearly show a bivoltine (Mezquita et al., 2000) reproduction pattern, northern occurrences provide evidence for both univoltine (Belgium; Heip, 1976) and bivoltine (United Kingdom; Roberts et al., 2020) patterns. Nonetheless, a bivoltine reproduction would result in a slightly overlapping but double peaked distribution of size data at local scales. Enot Zuqim (ISR) samples show such a pattern. Samples were derived from different but possibly connected sites within a wetland. This indicates that morphotypes have a disjunct distribution even within a small geographical area. Only Hersek lagoon (HK) provides both morphotypes. The co-occurrence of different size groups as a result from bivoltine reproduction is therefore only for Hersek lagoon (HK) possible.
Although body-size evolution results from diverse factors, divergence of size can be consistent with phylogenetical patterns (Suatoni et al., 2006; Haye et al., 2019). It has been shown that interspecific size distribution is not random but instead probably organized through interspecific interactions. Two processes are known that lead to population-wide patterns of interspecific body-size variations. The first is adjustment (i.e., character displacement) which is an evolutionary response of species in sympatry and allopatry. The other one represents size assortment according to ecological interaction among species in which different-sized species can successfully colonize the same locality together (Sota et al., 2000).
In contrast, intraspecific morphological divergence may result from phenotypical plasticity, where the influence of abiotic or biotic selective forces could lead to the expression of alternative phenotypes between populations from a single genotype, or divergent selection drives local adaptation and heritable evolutionary differences in phenotypes on account of genetic differentiation (Bower et al., 2018). The bimodal size-pattern of C. torosa (Figures 2C,D) contradicts assumptions on the expression of phenotypical plasticity, which is usually associated with gradual successions in morphological traits (Emlen et al., 2007; Lafuente and Beldade, 2019). The observed body-size patterns may, therefore, reflect interspecific size differentiation.
Although extensive investigations of morphological and/or genetical divergence of C. torosa are missing, there are hints pointing to spatial variations in morphology (Wouters, 2017) and genetics (Sywula et al., 1995). In our dataset, the occurrence of distinct morphotypes with little geographical overlap could point to the existence of at least two different species. Nonetheless, integrated approaches combining examinations of morphological traits, preferably by quantitative and genetic data, are therefore needed to evaluate not only the importance of divergence in size of Cyprideis but also to achieve a more complete understanding of spatial morphological variability and underlying causes.
Body size of crustaceans (marine and freshwater) generally display Bergmann trends as reported in the review by Maszczyk and Brzeziński (2018). It is, however, not clear whether these patterns result from direct effects of temperature or from the interaction of temperature with other extrinsic factors. Actually, it is stated that temperature-dependent oxygen limitation (Huston and Wolverton, 2011; Horne et al., 2015; Rollinson and Rowe, 2018) is a major controlling factor for aquatic invertebrates beside a number of other factors (e.g., sexual selection, predation) that could cause correlations between body size and latitude (Verberk et al., 2011).
It has been documented that rate of growth and size of ostracodes is affected by water temperature. Accordingly, valves of the same species will reach a larger size under lower temperature (Martens, 1985). Later, a strong temperature effect was noted on inter-molt time but not on growth factors (Liberto et al., 2014) indicating other or additional factors (e.g., genetical) that control the ultimate valve size. The explanatory power of these studies is, however, limited since they do not provide hints on side effects of temperature changes or related changes of other parameters such as oxygen availability. By this, a secondary dependence of temperature on the valve size could be related to the influence on the development time.
Hunt and Roy (2006) and Hunt et al. (2010) provide the only studies on ostracode size with respect to latitudinal patterns which showed that body size of deep-sea ostracode species has increased greatly over the past 40 million years mirroring the trajectory of bottom-water temperature that can be translated into a latitudinal cline of size according to the Bergmann trend.
Accepting that size clines are related to parameters connected to latitude such as temperature, climate change will lead to changes in body size (Teplitsky and Millien, 2014). Our data cover a relatively large temporal range from Early Holocene to modern day. Populations lived under warmer and colder periods relative to mean temperature variations of the late 20th century (Figure 7). If temperature is a major control on body size of Cyprideis, the distribution of size should be different from what we actually found with largest valves occurring under coldest temperatures and vice versa. However, there is no pattern in valve size in relation to Holocene temperature conditions revealed.
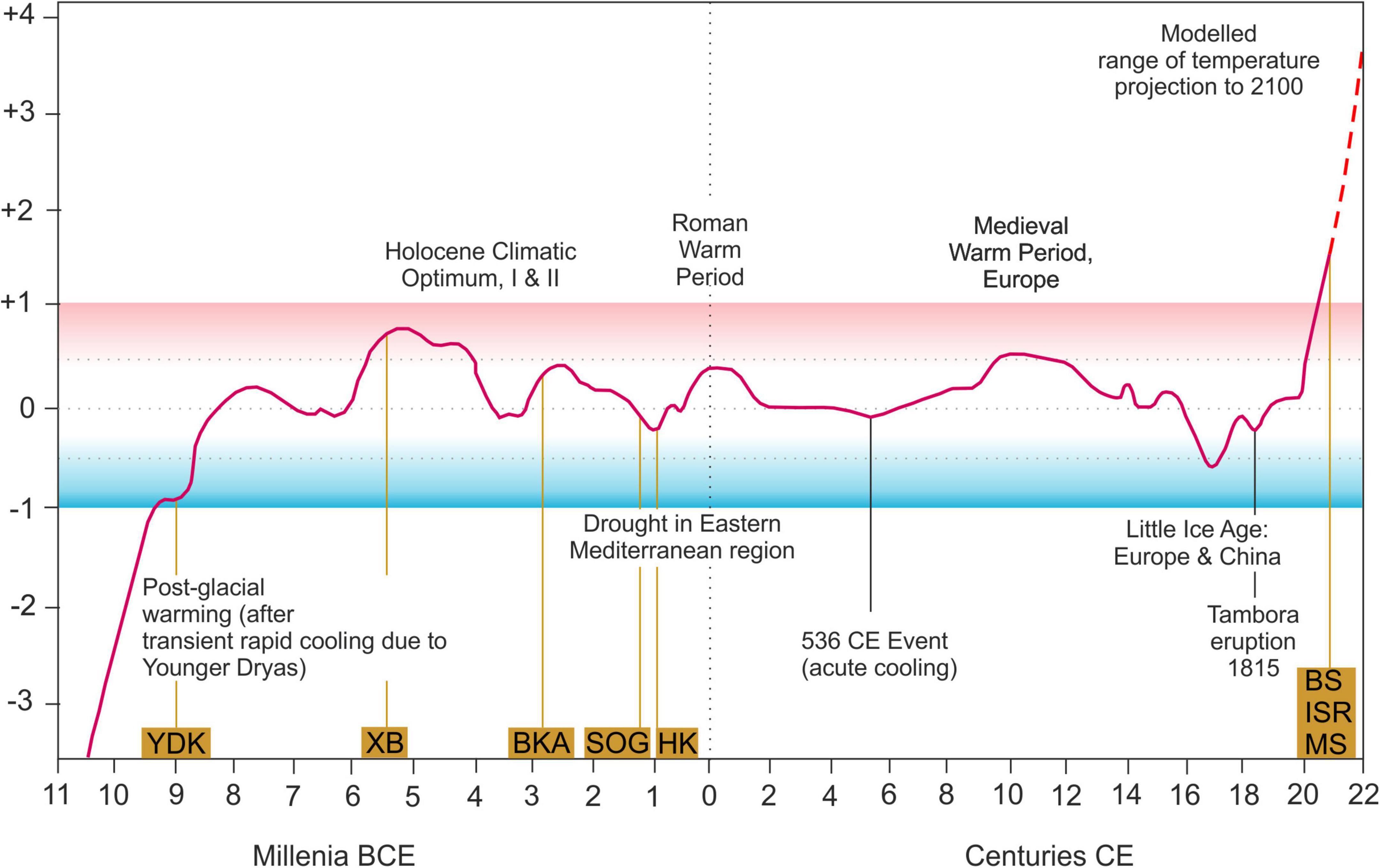
Figure 7. Temporal distribution of the samples in relation to northern hemisphere temperature fluctuations during the Holocene, °C (relative to mean temperature during 1960–1980), averaged from multiple sources [modified after McMichael (2012)].
This implies that size clines in non-marine ostracodes or at least (temperate) Cyprideis are probably not (directly) controlled by temperature. Another objective of this study was the question how species and latitude-specific size-latitude trends in non-marine ostracodes are expressed. A similar data set that enables the comparison of C. torosa with another species is available from the Neotropical ostracode species Cytheridella ilosvayi (Wrozyna et al., 2016, 2018, 2019). Both species belong to the same superfamily Cytheroidea, which is characterized by a great variation in size and valve shape and comprises marine, brackish, and freshwater species (Meisch, 2000). Cytheridella ilosvayi is a common freshwater species occurring in a variety of aquatic habitats in the entire Neotropical range. The size data (length, height) of C. ilosvayi were derived from modern populations sampled between 30°S and 26°N.
Despite relatively large gaps in the size data of Cytheridella, no or only a weak relationship between valve size and latitude is displayed by the available data (correlation coefficients of r < 0.3) (Figures 5A,B and Table 4). Assuming this as a general trend for the entire geographical range, it implies that tropical non-marine ostracodes might not develop latitudinal-size clines. However, more data from underrepresented regions and other species are needed to verify this hypothesis.
An explanation why Bergmann trends are possibly not exhibited by Cytheridella could be the missing or low temperature gradient and stable resources within its tropical to subtropical geographical range as it is suggested for some tropical vertebrates which provide little support for Bergmann trends (Reed, 2003; Read et al., 2018).
The preceding discussion has shown that existing models on causes and mechanisms of Bergmann trends only insufficiently explain latitude-size relationship of C. torosa. Actually, it is accepted that intra- and interspecific gradients in body size may represent different phenomena (Blackburn et al., 1999). Although Bergmann’s rule was originally defined inter-specifically, it has been commonly applied to populations within a single species. The expected mechanisms involved in size-latitude trends, are primarily limited to intraspecific scales and no general mechanism accounting for interspecific size-latitude patterns has been suggested (Berke et al., 2013). Thus, the observed size clines in C. torosa may result from interspecific divergence (i.e., size-ordered spatial sorting; Muñoz et al., 2014). Approaches that parse out intra- and interspecific variations on size-environment relationships revealed that patterns apparent at the level of clades may be (almost) absent at the level of species, and vice versa. This could be also displayed by C. torosa where the strong correlation with latitude is displayed only when size data are used undifferentiated and are strongly diminished for both morphotypes (Table 4).
Meiri and Thomas (2007) recommended to consider phylogenetic structures within taxa in order to elucidate whether patterns in the geography of size result from turnover within or between taxonomic levels. From a growing number of organism groups inconsistent (interspecific) latitudinal-size clines are reported suggesting that there may be no universal interspecific trend in latitudinal variation of body size, even among closely related groups (Pallarés et al., 2019). This is reflected by Cyprideis and Cytheridella. Accordingly, their geographical body size patterns are neither straightforward nor universally transferable to ostracodes in general. The consideration of different phylogenetic scales and relationships is therefore important before any reliable conclusion on size-environment relationships can be drawn.
Control of Salinity on Cyprideis torosa Size
Boomer et al. (2017) investigated valve size of C. torosa in relation to salinity. They identified a two-fold pattern in which valve size is largest and relatively constant at salinities between 0.7 and 8‰ and gradually decreases under higher salinities. The present study not only reveals an opposite trend to that reported by Boomer et al. (2017) with smallest valves at lower and lowest salinities, but also provides implications for the scale dependency of the salinity influence. As clearly shown by the RDA (Figure 6), latitude contributes most explanatory power on the overall size variability of C. torosa superimposing the salinity control. This agrees well with observations that community patterns and intraspecific trait variability depend on the spatial and ecological scales of investigations (Evangelista et al., 2019), and are often structured by a hierarchy of environmental factors (Hirschfeld et al., 2021). Also, our data underline the importance of the taxonomic resolution (e.g., morphotypes) for inferences on the sensitivity of a species toward environmental (i.e., salinity) conditions. Disregarding the morphotypes and supposing all occurrences as one Cyprideis species no relationship between size data and salinity is exhibited. In contrast, considering the morphotypes separately, significant (morphotype-specific) positive correlations between valve size (length and height) and salinity are revealed. Moreover, only morphotype II shows a weak correlation between width and salinity, while there is no correlation displayed by the other morphotype. These slight but obvious differences point to an ecological differentiation of the morphotypes possibly representing another indication for the presence of at least two species currently considered as C. torosa.
Thus, our investigations suggest that salinity is not a primary control on the size of C. torosa sensu lato as it is also assumed for other Cyprideis species (Gitter et al., 2015; Meyer et al., 2017). The discrepancies in salinity-size relationships of Boomer et al. (2017) and our data might result from integration of several unperceived Cyprideis species.
Nonetheless, considering the geographical distribution of the size data set of Boomer et al. (2017) the same latitudinal size trend as in our data is reflected and even extends the latitudinal size cline of Cyprideis (Figure 4).
Salinity values used for the investigations of salinity-size relationships were derived from different sources. Paleo-salinity of the fossil occurrences of C. torosa for most sites was inferred by the application of ostracode-based transfer functions. These are based on the significant and independent contribution of electrical conductivity (i.e., salinity) to the total variance in ostracode species distribution and provide very reliable salinity estimates (Mischke et al., 2007, 2010, 2014). Although there have been probably no fundamental changes in salinity, sites where salinity was estimated due to similarity of fossil and Recent ostracode species distribution (Hersek lagoon), can provide only rough estimates of salinity. Verification of our findings and interpretations should be based on investigations of living specimens and in situ measurements of salinity conditions.
Conclusion
In conclusion, the investigation of distant Recent and Holocene populations of C. torosa shows that there is a large variation in valve-size traits (length, height, width). Distinct local size clusters are superimposed by two large-scale geographical patterns. One represents a differentiation in two significantly different size classes, which separates southern populations from northern counterparts at around ∼42°N. The second large-scale pattern is the continuous increase of valve size of C. torosa with latitude according to a Bergmann trend. Contrary, the available size data of the tropical species Cytheridella ilosvayi do not display this trend.
The co-occurrence of both size morphotypes of C. torosa in a wetland close to the Dead Sea (Enot Zuqim) and in the Turkish Hersek lagoon precludes an environmental control on this geographical pattern. Additionally, size-salinity relationships are morphotype-specific. A possible explanation of this could be the existence of at least two different Cyprideis species.
Majority of explanations for Bergmann trends relate to ecological processes and are, thus, restricted to intraspecific phylogenetic levels. These models only insufficiently contribute to the understanding of the observed size-cline of C. torosa. Our results imply that size of Cyprideis is predominantly controlled by phylogeny, rather than environmental factors (e.g., salinity).
By comparing both species (Cyprideis torosa and Cytheridella ilosvayi) it is indicated that size-clines in ostracodes might be taxon-specific and no general pattern or underlying causes for ostracodes exist. Therefore, it is important to consider phylogenetic relationships and possible existence of more species than anticipated before attempting to explain size variability solely by environmental influences.
Data Availability Statement
The original contributions presented in the study are included in the article/Supplementary Material, further inquiries can be directed to the corresponding author.
Author Contributions
CW and WP designed and conceived the study and wrote the manuscript. SM, MG, and MH provided sample material and additional environmental data. MG and SM edited the manuscript. All authors commented and agreed on the final version of the manuscript.
Funding
Financial support was provided by the German Science Foundation (DFG; project WR 169/2-1).
Conflict of Interest
The authors declare that the research was conducted in the absence of any commercial or financial relationships that could be construed as a potential conflict of interest.
Publisher’s Note
All claims expressed in this article are solely those of the authors and do not necessarily represent those of their affiliated organizations, or those of the publisher, the editors and the reviewers. Any product that may be evaluated in this article, or claim that may be made by its manufacturer, is not guaranteed or endorsed by the publisher.
Acknowledgments
Christian Burmeister is thanked for his assistance in the preparation of the study-area map. Special thanks go to AW and the reviewer who enhanced the quality of the manuscript.
Supplementary Material
The Supplementary Material for this article can be found online at: https://www.frontiersin.org/articles/10.3389/fevo.2022.857499/full#supplementary-material
Supplementary Figure 1 | External views of female and male left and right valves of Cyprideis torosa from the studied localities. YDK, Tarim Basin, China; XB, Lake Bosten, China; MS, Mansfeld Lakes, Germany; SOG, Sea of Galilee, Israel; ISR, Dead Sea, Israel; BKA, Lake Balkhash, Kazakhstan; BS, Danube Delta, Romania; HK, Hersek lagoon, Turkey.
Supplementary Figure 2 | Variability of valve length (a) and width (b) of populations. Displayed are variability ranges of female valves. Boxplots of male valves are provided in the Supplementary Figure 3. Numbers indicate sample sizes. Population abbreviations are: YDK, Tarim Basin, China; XB, Lake Bosten, China; BKA, Lake Balkhash, Kazakhstan; ISR, Dead Sea, Israel; SOG, Sea of Galilee, Israel; BS, Danube Delta, Romania; HK, Hersek lagoon, Turkey; MS, Mansfeld Lakes, Germany. Details about the localities are provided in Table 1. Tables display the pairwise comparisons of the post hoc test results of the PerMANOVAs for each data set [female right (RV; c) and left (LV; d) valves, and male right (e) and left valves (f).
Supplementary Figure 3 | Variability of valve length (a) and width (b) of populations. Displayed are variability ranges of male valves. Numbers indicate sample sizes. Population abbreviations are: YDK, Tarim Basin, China; XB, Lake Bosten, China; BKA, Lake Balkhash, Kazakhstan; ISR, Dead Sea, Israel; SOG, Sea of Galilee, Israel; BS, Danube Delta, Romania; HK, Hersek lagoon, Turkey; MS, Mansfeld Lakes, Germany. Details about the localities are provided in Table 1.
Supplementary Table 1 | Size data of female left valves. Salinity data referred to the original publications. See Table 1 for details.
Supplementary Table 2 | Size data of female right valves. Salinity data referred to the original publications. See Table 1 for details.
Supplementary Table 3 | Size data of male left valves. Salinity data referred to the original publications. See for Table 1 details.
Supplementary Table 4 | Size data of male right valves. Salinity data referred to the original publications. See Table 1 for details.
References
Aguilar-Alberola, J. A., and Mesquita-Joanes, F. (2014). Breaking the temperature-size rule: thermal effects on growth, development and fecundity of a crustacean from temporary waters. J. Therm. Biol. 42, 15–24. doi: 10.1016/j.jtherbio.2014.02.016
Ashton, K. G., and Feldman, C. R. (2003). Bergmann’s rule in non-avian reptiles: turtles follow it, lizards and snakes reverse it. Evolution 57, 1151–1163. doi: 10.1111/j.0014-3820.2003.tb00324.x
Bai, Y., Dong, J.-J., Guan, D.-L., Xie, J.-Y., and Xu, S.-Q. (2016). Geographic variation in wing size and shape of the grasshopper Trilphidia annulata (Orthoptera: Oedipodidae): morphological trait variations follow an ecogeographical rule. Sci. Rep. 6:32680. doi: 10.1038/srep32680
Bergmann, C (1847). Ueber die Verhältnisse der Wärmeökonomie der Thiere zu ihrer Größe. Göttinger Stud. 3, 595–708.
Berke, S. K., Jablonski, D., Krug, A. Z., Roy, K., and Tomasovych, A. (2013). Beyond Bergmann’s rule: size-latitude relationships in marine Bivalvia world-wide. Glob. Ecol. Biogeogr. 22, 173–183. doi: 10.1111/j.1466-8238.2012.00775.x
Berndt, C., Frenzel, P., and Çiner, A. (2019). Intraspecific length variation and shell thickness of the ostracod Cyprideis torosa (Jones, 1850) as a potential tool for palaeosalinity characterization. Geosciences 9:83. doi: 10.3390/geosciences9020083
Blackburn, T. M., Gaston, K. J., and Loder, N. (1999). Geographic gradients in body size: a clarification of Bergmann’s rule. Divers. Distrib. 5, 165–174.
Blanckenhorn, W. U., Stillwell, R. C., Young, K. A., Fox, C. W., and Ashton, K. G. (2006). When Rensch meets Bergmann: does sexual size dimorphism change systematically with latitude? Evolution 60, 2004–2011. doi: 10.1111/j.0014-3820.2006.tb01838.x
Boomer, I., Frenzel, P., and Feike, M. (2017). Salinity-driven size variability in Cyprideis torosa (Ostracoda, Crustacea). J. Micropalaeontol. 36, 63–69. doi: 10.1144/jmpaleo2015-043
Bower, L. M., Jawad, L. A., Gnohossou, P. M., and Tossou, A. G. (2018). Intraspecific morphological divergence in two Cichlid species from Benin. Afr. J. Aquat. Sci. 43, 385–392. doi: 10.2989/16085914.2018.1517079
Butlin, R., Schön, I., and Martens, K. (1998). Asexual reproduction in nonmarine ostracods. Heredity 81, 473–480. doi: 10.1046/j.1365-2540.1998.00454.x
De Deckker, P., and Lord, A. (2017). Cyprideis torosa: a model organism for the Ostracoda? J. Micropalaeontol. 36, 3–6. doi: 10.1144/jmpaleo2016-100
Emlen, D. J., Corley Lavine, L., and Ewen-Campen, B. (2007). On the origin and evolutionary diversification of beetle horns. PNAS 104, 8661–8668.
Evangelista, C., Olden, J. D., Lecerf, A., and Cucherousset, J. (2019). Scale-dependent patterns of intraspecific trait variations in two globally invasive species. Oecologia 189, 1083–1094. doi: 10.1007/s00442-019-04374-4
Fernandes Martins, M. J., Vandekerkhove, J., Mezquita, F., Schmit, O., Rueda, J., Rossetti, G., et al. (2009). Dynamics of sexual and parthenogenetic populations of Eucypris virens (Crustacea: Ostracoda) in three temporary ponds. Hydrobiologia 636, 219–232. doi: 10.1007/s10750-009-9952-0
Geiger, W., Otero, M., and Rossi, V. (1998). “Clonal ecology diversity,” in Sex and Parthenogenesis: Evolutionary Ecology of Reproductive Modes in Non-Marine Ostracods, ed. K. Martens (Leiden: Backhuys Publishers), 243–256.
Gitter, F., Gross, M., and Piller, W. E. (2015). Sub-decadal resolution in sediments of Late Miocene Lake Pannon reveals speciation of Cyprideis (Crustacea, Ostracoda). PLoS One 10:e0109360. doi: 10.1371/journal.pone.0109360
Gliozzi, E., Rodriguez-Lazaro, J., and Pipik, R. (2017). The Neogene Mediterranean origin of Cyprideis torosa (Jones, 1850). J. Micropalaeontol. 36, 80–93. doi: 10.1144/jmpaleo2016-029
Hammer, Ø, Harper, D. A. T., and Ryan, P. D. (2001). PAST: paleontological Statistics software package for education and data analysis. Palaeontol. Electron. 4:9.
Hart, R. C., and Bychek, E. A. (2011). Body size in freshwater planktonic crustaceans: an overview of extrinsic determinants and modifying influences of biotic interactions. Hydrobiologia 668, 61–108. doi: 10.1007/s10750-010-0400-y
Haye, P. A., Segovia, N. I., Varela, A. I., Rojas, R., Rivadeneira, M. M., and Thiel, M. (2019). Genetic and morphological divergence at a biogeographic break in the beach-dwelling brooder Excirolana hirsuticauda Menzies (Crustacea, Peracarida). BMC Evol. Biol. 19:118. doi: 10.1186/s12862-019-1442-z
Hem, J. D. (1982). “Conductance: A collective measure of dissolved ions,” in Water Analysis, Inorganic Species, Part 1, eds R. A. Minear and L. H. Keith (Cambridge: Academic Press), 137–161.
Hirschfeld, M. N. C., Maia, A. C. D., and Faria, L. R. R. (2021). The key is in variation: spatial-environmental structuring of the morphological variation of a widespread Neotropical bee (Eulaema nigrita). Zool. Anz. 293, 138–144. doi: 10.1016/j.cz.2021.05.012
Horne, C. R., Hirst, A. G., and Atkinson, D. (2015). Temperature-size responses match latitudinal-size clines in arthropods, revealing critical differences between aquatic and terrestrial species. Ecol. Lett. 18, 327–335. doi: 10.1111/ele.12413
Horne, D. J. (2005). “Ostracoda,” in Encyclopedia of Geology, eds R. C. Selley, L. R. M. Cocks, and I. R. Plimer (Amsterdam: Elsevier Academic), 453–463.
Horne, D. J., Cohen, A., and Martens, K. (2002). “Taxonomy, morphology and biology of Quaternary and living Ostracoda,” in The Ostracoda - Applications in Quaternary Research, Geophys Monogr, eds J. A. Holmes and A. R. Chivas (Washington, DC: American Geophysical Union), 5–36. doi: 10.1029/131GM02
Hunt, G., and Roy, K. (2006). Climate change, body size evolution, and Cope’s Rule in deep-sea ostracodes. PNAS 103, 1347–1352. doi: 10.1073/pnas.0510550103
Hunt, G., Wickasono, S. A., Brown, J. E., and Macleod, K. G. (2010). Climate-driven body-size trends in the ostracod fauna of the deep Indian Ocean. Palaeontologia 53, 1255–1268. doi: 10.1111/j.1475-4983.2010.01007.x
Huston, M. A., and Wolverton, S. (2011). Regulation of animal size by eNPP, Bergmann’s rule, and related phenomena. Ecol. Monogr. 81, 349–405.
Kalanke, J. (2015). Late Holocene History of the Sea of Galilee (Israel) Inferred from Ostracod Assemblages. M.Sc. thesis. Berlin: Free University of Berlin, 47.
Kim, Y., Mo, H., Son, J., Lee, Y.-S., Lee, S.-E., and Cho, K. (2015). Interactive effects of water pH and hardness levels on the growth and reproduction of Heterocypris incongruens (Crustacea: Ostracoda). Hydrobiologia 753, 97–109. doi: 10.1007/s10750-015-2199-z
Lafuente, E., and Beldade, P. (2019). Genomics of developmental plasticity in animals. Front. Genet. 10:720. doi: 10.3389/fgene.2019.00720
Liberto, R., César, I. I., and Mesquita-Joanes, F. (2014). Postembryonic growth in two species of freshwater Ostracoda (Crustacea) shows a size-age sigmoid model fit and temperature effects on development time, but no clear temperature-size rule (TSR) pattern. Limnology 15, 57–67. doi: 10.1007/s10201-013-0413-0
Martens, K. (1985). Effects of temperature and salinity on postembryonic growth in Mytilocypris henricae (Chapman) (Crustacea, Ostracoda). J. Crustac. Biol. 5, 258–272.
Martens, K., Schön, I., Meisch, C., and Horne, D. J. (2008). Global diversity of ostracods (Ostracoda, Crustacea) in freshwater. Hydrobiologia 595, 185–193. doi: 10.1007/s10750-007-9245-4
Maszczyk, P., and Brzeziński, T. (2018). “Body size, maturation size, and growth rate of crustaceans,” in Life Histories, eds G. A. Wellborn and M. Thiel (Oxford: Oxford University Press), 35–65.
McMichael, A. J. (2012). Insights from past millennia into climatic impacts on human health and survival. PNAS 109, 4730–4737. doi: 10.1073/pnas.1120177109
Meiri, S., and Thomas, G. H. (2007). The geography of body size – challenges of the interspecific approach. Glob. Ecol. Biogeogr. 16, 689–693. doi: 10.1111/j.1466-8238.2007.00343.x
Meyer, J., Wrozyna, C., Gross, M., Leis, A., and Piller, W. E. (2017). Morphological and geochemical variations of Cyprideis (Ostracoda) from modern waters of the northern Neotropics. Limnology 18, 251–273. doi: 10.1007/s10201-016-0504-9
Mezquita, F., Olmos, V., and Oltra, R. (2000). Population ecology of Cyprideis torosa (Jones, 1850) in a hypersaline environment of the Western Mediterranean (Santa Pola, Alacant) (Crustacea: Ostracoda). Ophelia 53, 119–130.
Mischke, S., Almogi-Labin, A., Al-Saqarat, B., Rosenfeld, A., Elyashiv, H., Boomer, I., et al. (2014). An expanded ostracod-based conductivity transfer function for climate reconstruction in the Levant. Quat. Sci. Rev. 93, 91–105. doi: 10.1007/s10933-009-9359-y
Mischke, S., Almogi-Labin, A., Ortal, R., Rosenfeld, A., Schwab, M. J., and Boomer, I. (2010). Quantitative reconstruction of lake conductivity in the Quaternary of the Near East (Israel) using ostracods. J Paleolim 43, 667–688.
Mischke, S., Herzschuh, U., Massmann, G., and Zhang, C. (2007). An ostracod-conductivity transfer function for Tibetan lakes. J. Paleolim 38, 509–523. doi: 10.1007/s10933-006-9087-5
Mischke, S., Liu, C., Zhang, J., Zhang, C., Zhang, H., Jiao, P., et al. (2017). The world’s earliest Aral-Sea type disaster: the decline of the Loulan Kingdom in the Tarim Basin. Sci. Rep. 7:43102. doi: 10.1038/srep43102
Mischke, S., Schudack, U., Bertrand, S., and Leroy, S. (2012). Ostracods from a Marmara Sea lagoon (Turkey) as tsunami indicators. Quat. Int. 261, 156–161.
Mischke, S., and Wünnemann, B. (2006). The Holocene salinity history of Bosten Lake (Xinjiang, China) inferred from ostracod species assemblages and shell chemistry: possible palaeoclimatic implications. Quat. Int. 15, 100–112.
Mischke, S., Zhang, C., Liu, C., Zhang, J., Jiao, P., and Plessen, B. (2019). The Holocene salinity history of Lake Lop Nur (Tarim Basin, NW China) inferred from ostracods, foraminifera, ooids and stable isotope data. Glob. Planet. Change 175, 1–12.
Mischke, S., Zhang, C., and Plessen, B. (2020). Lake Balkhash (Kazakhstan): recent human impact and natural variability in the last 2900 years. J. Great Lakes Res. 46, 267–276.
Muñoz, M., Wegener, J. E., and Algar, A. C. (2014). Untangling intra- and interspecific effects on body size clines reveals divergent processes structuring convergent patterns in Anolis lizards. Am. Nat. 184, 636–646. doi: 10.1086/678084
Pallarés, S., Lai, M., Abellán, P., Ribera, I., and Sánchez-Fernández, D. (2019). An interspecific test of Bergmann’s rule reveals inconsistent body size patterns across several lineages of water beetles (Coleoptera: Dytiscidae). Ecol. Entom. 44, 249–254.
Pint, A., Frenzel, P., Fuhrmann, R., Scharf, B., and Wennrich, V. (2012). Distribution of Cyprideis torosa (Ostracoda) in Quaternary athalassic sediments in Germany and its application for palaeoecological reconstructions. Int. Rev. Hydrobiol. 97, 330–355. doi: 10.1002/iroh.201111495
Read, Q. D., Baiser, B., Grady, J. M., Zarnetske, P. L., Record, S., and Belmaker, J. (2018). Tropical bird species have less variable body sizes. Biol. Lett. 14:20170453. doi: 10.1098/rsbl.2017.0453
Reed, R. N. (2003). Interspecific patterns of species richness, geographic range size, and body size among New World venomous snakes. Ecography 26, 107–117.
Roberts, L. R., Holmes, J. A., and Horne, D. J. (2020). Tracking the seasonal calcification of Cyprideis torosa (Crustacea, Ostracoda) using Mg/Ca-inferred temperatures, and its implications for palaeotemperature reconstruction. Mar. Micropal. 156:101838. doi: 10.1016/j.marmicro.2020.101838
Rollinson, N., and Rowe, L. (2018). Temperature-dependent oxygen limitation and the rise of Bergmann’s rule in species with aquatic respiration. Evolution 72, 977–988. doi: 10.1111/evo.13458
Schön, I., Verheyen, E., and Martens, K. (2000). Speciation in ancient lake ostracods: comparative analysis of Baikalian Cytherissa and Tanganyikan Cyprideis. SIL Proc. 27, 2674–2677. doi: 10.1080/03680770.1998.11898152
Sota, T., Takami, Y., Kubota, K., Ujiie, M., and Ishikawa, R. (2000). Interspecific body size differentiation in species assemblages of the carabid subgenus Ohomopterus in Japan. Popul. Ecol. 42, 279–291.
Suatoni, E., Vicario, S., Rice, S., Snell, T., and Caccone, A. (2006). An analysis of species boundaries and biogeographic patterns in a cryptic species complex: the rotifer–Brachionus plicatilis. Mol. Phylogenetics Evol. 41, 86–98. doi: 10.1016/j.ympev.2006.04.025
Sywula, T., Glazewska, I., Whatley, R. C., and Moguilevsky, A. (1995). Genetic differentiation in the brackish-water ostracod Cyprideis torosa. Mar. Biol. 121, 647–653.
Teplitsky, C., and Millien, V. (2014). Climate warming and Bergmann’s rule through time: is there any evidence? Evol. Appl. 7, 156–168. doi: 10.1111/eva.12129
Tesche, M. R., and Hodges, K. E. (2015). Rethinking biogeographic patterns: high local variation in relation to latitudinal clines for a widely distributed species. Oecol 179, 139–149. doi: 10.1007/s00442-015-3340-4
van de Velde, S., Jorissen, E. L., Neubauer, T. A., Radan, S., Pavel, A. B., Stoica, M., et al. (2019). A conservation palaeobiological approach to assess faunal response of threatened biota under natural and anthropogenic environmental change. Biogeosciences 16, 2423–2442. doi: 10.5194/bg-16-2423-2019
Verberk, W. C. E. P., Atkinson, D., Hoefnagel, N., Hirst, A. G., Horne, C. R., and Siepel, H. (2021). Shrinking body sizes in response to warming: explanations for the temperature–size rule with special emphasis on the role of oxygen. Biol. Rev. Cam. Philos. Soc. 96, 247–268. doi: 10.1111/brv.12653
Verberk, W. C. E. P., Bilton, D. T., Calosi, P., and Spicer, J. I. (2011). Oxygen supply in aquatic ectotherms: partial pressure and solubility together explain biodiversity and size patterns. Ecology 92, 1565–1572. doi: 10.1890/10-2369.1
Wȩsławski, J. M., Legeżyńska, J., and Włodarska-Kowalczuk, M. (2020). Will shrinking body size and increasing species diversity of crustaceans follow the warming of the Arctic littoral? Ecol. Evol. 10, 10305–10313. doi: 10.1002/ece3.6780
West, R. G., Knudsen, K. L., Penney, D. N., Preece, R. C., and Robinson, J. E. (1994). Palaeontology and taphonomy of Late Quaternary fossil assemblages at Somersham, Cambridgeshire, England, and the problem of reworking. J. Quat. Sci. 9, 357–366.
Wouters, K. (2017). On the modern distribution of the euryhaline species Cyprideis torosa (Jones, 1850) (Crustacea, Ostracoda). J. Micropalaeontol. 36, 21–30. doi: 10.1144/jmpaleo2015-021
Wouters, K., and Martens, K. (1994). Contribution to the knowledge of the Cyprideis flock (Crustacea: Ostracoda) of Lake Tananyika, with the description of three new species. Biologie 64, 111–128.
Wouters, K., and Martens, K. (2001). On the Cyprideis species flock (Crustacea, Ostracoda) in Lake Tanganyika, with the description of four new species. Hydrobiologia 450, 111–127.
Wrozyna, C., Meyer, J., Gross, M., Ramos, M. I. F., and Piller, W. E. (2018). Definition of regional ostracod (Cytheridella) morphotypes by use of landmark-based morphometrics. Freshw. Sci. 37, 573–592. doi: 10.1086/699482
Wrozyna, C., Meyer, J., Gross, M., Ramos, M. I. F., and Piller, W. E. (2019). Sexual, ontogenetic, and geographic variation of the Neotropical freshwater ostracod Cytheridella ilosvayi. BMC Zool. 4:4. doi: 10.1186/s40850-019-0042-0
Wrozyna, C., Neubauer, T. A., Meyer, J., and Piller, W. E. (2016). Shape Variation in Neotropical Cytheridella (Ostracoda) using semilandmarks-based Geometric Morphometrics: a methodological approach and possible biogeographical implications. PLoS One 11:e0168438. doi: 10.1371/journal.pone.0168438
Yin, Y., Li, W., Yang, X., Wang, S., Li, S., and Xia, W. (2001). Morphological responses of Limnocythere inopinata (Ostracoda) to hydrochemical environment factors. Sci. Chin. Ser. D-Earth Sci. 44, 316–323. doi: 10.1007/BF02912001
Keywords: body size, geographical variability, Bergmann’s rule, ostracodes, Cyprideis, morphometrics
Citation: Wrozyna C, Mischke S, Hoehle M, Gross M and Piller WE (2022) Large-Scale Geographic Size Variability of Cyprideis torosa (Ostracoda) and Its Taxonomic and Ecologic Implications. Front. Ecol. Evol. 10:857499. doi: 10.3389/fevo.2022.857499
Received: 18 January 2022; Accepted: 16 June 2022;
Published: 29 July 2022.
Edited by:
Paula Arribas, Institute of Natural Products and Agrobiology (CSIC), SpainReviewed by:
Anna Pint, Friedrich Schiller University Jena, GermanyAleksandra Walczyńska, Jagiellonian University, Poland
Copyright © 2022 Wrozyna, Mischke, Hoehle, Gross and Piller. This is an open-access article distributed under the terms of the Creative Commons Attribution License (CC BY). The use, distribution or reproduction in other forums is permitted, provided the original author(s) and the copyright owner(s) are credited and that the original publication in this journal is cited, in accordance with accepted academic practice. No use, distribution or reproduction is permitted which does not comply with these terms.
*Correspondence: Claudia Wrozyna, Y2xhdWRpYS53cm96eW5hQHVuaS1ncmVpZnN3YWxkLmRl