- 1Ministry of Education Key Laboratory for Ecology of Tropical Islands, Key Laboratory of Tropical Animal and Plant Ecology of Hainan Province, College of Life Sciences, Hainan Normal University, Haikou, China
- 2Chengdu Institute of Biology, Chinese Academy of Sciences, Chengdu, China
Chelonians are considered the least vocally active group of extant reptiles and known as “low-frequency specialists” with a hearing range of <1.0 kHz. As they are ectothermic organisms, most of their physiological and metabolic processes are affected by temperature, which may include the auditory system responses. To investigate the influence of temperature on turtle hearing, Trachemys scripta elegans was chosen to measure the peripheral hearing sensitivity at 10, 20, 30, and 40°C (close to the upper limit of heat resistance) using the auditory brainstem response (ABR) test. An increase in temperature (from 10 to 30°C) resulted in improved hearing sensitivity (a wider hearing sensitivity bandwidth, lower threshold, and shorter latency) in T. scripta elegans. At 40°C, the hearing sensitivity bandwidth continued to increase and the latency further shortened, but the threshold sensitivity reduced in the intermediate frequency range (0.5–0.8 kHz), increased in the high-frequency range (1.0–1.3 kHz), and did not significantly change in the low-frequency range (0.2–0.4 kHz) compared to that at 30°C. Our results suggest that although the hearing range of turtles is confined to lower frequencies than that in other animal groups, turtle hearing showed exceptional thermal regulation ability, especially when the temperature was close to the upper limit of heat resistance. Temperature increases that are sensitive to high frequencies imply that the males turtles’ auditory system adapts to a high-frequency sound environment in the context of global warming. Our study is expected to spur further research on the high-temperature plasticity of hearing sensitivity in diverse taxa or in the same group with different temperature ranges. Moreover, it facilitates forecasting the adaptive evolution of the auditory system to global warming.
Introduction
Global warming forces animals to face a constantly changing thermal environment, and it has profound and diverse effects on organisms (Sinervo et al., 2010; Duarte et al., 2012; Luo et al., 2014; Laloë et al., 2017). Many behavioral and physiological processes of ectothermal animals are directly affected by ambient temperature, and relatively high temperatures can improve and maintain behavioral and physiological processes (Sollid et al., 2005; Du et al., 2011; Llusia et al., 2013; Ospina et al., 2013; Whitford et al., 2020; Sun et al., 2022). The associations among reptile behavior, breeding, and other life-history characteristics with temperature have attracted attention in terms of how global warming may affect the adaptability of the behavioral and physiological processes of species in the future.
The auditory system is the main way to receive acoustic signals, which play an important role in the life history of an organism (Gerhardt and Huber, 2002; Köppl et al., 2014). The auditory system of animals can produce neurophysiological responses based on a variety of physiological (Higgs, 2002; Parks et al., 2004) or external environmental factors (Kastak and Schusterman, 1999; Lovelace et al., 2003; Christensen-Dalsgaard et al., 2012). The effect of temperature on the auditory system has been studied in several animal taxa, such as insects (Oldfield, 1988; Pires and Hoy, 1992; Franz and Ronacher, 2002), fishes (Fay and Ream, 1992; Wysocki et al., 2009; Maiditsch and Ladich, 2014), frogs (Gans and Wever, 1974; Stiebler and Narins, 1990; Sun et al., 2019), mammals (Gold et al., 1985), and humans (Stockard et al., 1978). In reptiles, similar research has been conducted in some clades such as Squamata [lizards (Campbell, 1969; Werner, 1972, 1976), gecko (Eatock and Manley, 1981), snakes (Dooling et al., 2000)], and turtles (Patterson et al., 1968), and Crocodilia (Smolders and Klinke, 1984). However, the hearing range of animals in these studies was considerably wide.
Compared with that of other vertebrate classes, the chelonians’ hearing range is confined to low frequencies (mostly under 1 kHz), and they usually have higher auditory thresholds (Christensen-Dalsgaard et al., 2012; Martin et al., 2012; Ferrara et al., 2014). As turtles are ectothermic, they can more easily adapt to temperature variations than birds and mammals by adjusting their behavioral and physiological processes. However, the influence of temperature on the turtle auditory system is poorly understood compared with that in other animal taxa. The red-eared slider (Trachemys scripta elegans) is an eurythermal species, preferring 25–30°C (Hutchison et al., 1966). The thermal tolerance of T. scripta elegans hatchling is approximately 42°C (Zhang et al., 2003). The minimum tolerable water temperature for this species is 10°C (Hutchison et al., 1966; Ernst and Lovich, 2009). T. scripta elegans feeds when the water temperature is above 18°C and ceases feeding when the water temperature exceeds 37°C (Parmenter and Avery, 1990). Our previous study showed that the hearing sensitivity of this species is sexually dimorphic (females are more sensitive than males) (Wang et al., 2019), and female turtles show frequency-segmented development of hearing (Wang et al., 2021). As T. scripta elegans is known for its ability to tolerate a wide range of temperatures and as its hearing sensitivity is well studied, it is appropriate to study auditory adaptation under a wide temperature range, especially when the temperature is close to the upper limit of heat resistance.
The scalp-recorded response to activation of the nerve fibers in the auditory nerve and brainstem is called the auditory brainstem response (ABR). ABR is a minimally invasive and useful method for studying hearing sensitivity as well as the function of the auditory system (Capranica and Moffat, 1983; Schrode et al., 2014). In this study, we employed the ABR test to assess the hearing of T. scripta elegans at 10, 20, 30, and 40°C by focusing on the hearing sensitivity bandwidth, ABR threshold, and latency. The hearing sensitivity bandwidth means the range of acoustic frequency that the auditory system of an animal could accept. Latency is the time between stimulus onset and the first waveform valley of an ABR waveform. The objective of this study was to investigate the effect of temperature (especially at temperatures close to the upper limit of species heat resistance) on the functional auditory response of turtles. This study is the first to investigate the effects of temperature on the auditory responses of turtles to a wide range of temperature. Our study contributes to the understanding of the adaptive response of the auditory system of turtles under different temperatures, especially high temperatures. The results will provide a basis for studies on the adaptive evolution of animal auditory systems in response to global warming.
Materials and Methods
Experimental Animals
All T. scripta elegans individuals were purchased from the same farm in Hainan Province, maintained in aquaria under natural conditions, and fed an artificial diet (5#; Dolphin Aquarium Co., Ltd., Guangdong, China). Thirty-two 1-year old male T. scripta elegans were used to measure hearing sensitivity at 10°C (n = 7), 20°C (n = 7), 30°C (n = 6), and 40°C (n = 12). The temperature treatment was conducted according to the turtles’ temperature tolerance range. During the experiment, we used a cold light artificial climate box (ZRG–600C–L; Shanghai Binglin Electronic Technology Co., Ltd., Shanghai, China) to achieve different acclimation temperatures. In our preliminary experiments, the cloacal temperature was close to the acclimation temperature when the turtles were placed in the artificial climate box for approximately 2.5 h. Therefore, before the electrophysiology experiment, each turtle was placed in an artificial climate box to acclimatize for 3 h. All electrophysiological recordings were conducted in an adjustable-temperature soundproof booth lined with an echo-attenuating acoustic foam (3.5 m × 1.5 m × 2 m, length × width × height). Before electrode placement, each turtle was deeply anesthetized with a solution of 0.5% pelltobarbitalum natricum (CAS No. 57-33-0, Xiya Reagents, Shandong, China) dissolved in 0.9% sodium chloride. The anesthetic dose and method are described in detail elsewhere (Wang et al., 2019). All turtles were sacrificed after electrode placement in each temperature group to obtain blood for hormone measurement. All procedures were approved by the Animal Research Ethics Committee of Hainan Provincial Education Centre for Ecology and Environment, Hainan Normal University (HNECEE-2018-001) and carried out in strict accordance with the institutional guidelines.
Measurement of Morphological Data
Before recording the ABR, the body mass of each turtle was measured using an electronic balance [SE–3001FZH, 0.1 g; Ohaus International Trading (Shanghai) Co., Ltd., Shanghai, China], and the carapace length was measured using a Mitutoyo digital caliper (500-196-30, 0.01 mm; Mitutoyo Corp., Kawasaki-shi, Kanagawa Prefecture, Japan).
Measurement of Temperature
Before placing the turtles in the artificial climate box, we measured the ambient temperature (the temperature of the animals’ housing) using a handheld weather station (Sportstar; Shenzhen Healthy Mouse Technology Co., Ltd., Guangdong, China). The cloacal temperatures were recorded in the pre- and post-electrophysiological experiments using a digital thermometer (UT321; UNIT-T group Co., Ltd., Guangdong, China).
Measurement of Auditory Brainstem Response
Acoustic signals were played through a portable amplified field speaker with a frequency response ranging from 55 to 20 kHz (JBL GT7-6; Harman International Industries, Inc., United States). The distance between the speaker and the tip of the turtle jaw was fixed at 6 cm. ABR recordings were acquired using a TDT RA4LI low-impedance digital headstage and an RA4PA Medusa PreAmp with three needle electrodes (27 ga, 13 mm in length; Rochester Electro-Medical, Inc., Lutz, FL, United States), inserted subdermally above the tympanum (recording electrode) on top of the head under the frontal scale (reference electrode) and in the ipsilateral front leg (ground electrode). Electrode impedance was less than 3 kΩ. The responses were amplified (20 ×), filtered (0.03–3 kHz), and averaged using BioSig software and the System III hardware (TDT) data-acquisition system (Wang et al., 2019).
Stimulus presentation, ABR acquisition, equipment control, and data management procedures have been previously described (Wang et al., 2019). ABR stimulus levels were calibrated using a sensor signal conditioner (model 480C02; PCB Piezotronics, Inc., Depew, NY, United States) with a 1/4 ″ microphone (model 426B03; PCB Piezotronics). In our previous study, we found that a high sound level (90 dB) can’t elicit ABR response. Stimuli were synthesized digitally from 0.2 to 1.5 kHz with 100 Hz increments (9-ms duration, 2-ms rise/fall time, 24,414-Hz sampling rate, with alternating polarity) at a rate of 4/s, attenuated in 5-dB steps from 85 to 30 dB sound pressure level (SPL). Clicks were of 0.1-ms duration with a 249-ms interstimulus interval, attenuated in 5-dB steps from 85 to 30 dB SPL and presented at a rate of 4/s. ABR latencies were recorded as the time from the stimulus onset to the first negative waveform valley. The ABR threshold, latency, and ABR amplitude were determined via visual inspection (Brittan-Powell et al., 2002; Bierman et al., 2014; Scott et al., 2017). Thresholds were defined by the lowest stimulus level confirmed by two trained observers. The temperatures were maintained using a real-time temperature control pad (78 w, Superior heat mats, Guangzhou Reptile Structure Pet Products Co., Ltd., China) or ice box during ABR experiment.
Data Analysis and Statistics
Auditory brainstem response threshold and latency of T. scripta elegans individuals were obtained using their response to tone and click stimuli. Results were collated and analyzed using SPSS 22.0 (IBM Corp., Chicago, IL, United States). Before the statistical analysis, the assumptions of normality and homogeneity of variance in the data were examined using Shapiro–Wilk and Levene tests, respectively. Data of the body mass and carapace length among the temperature groups were analyzed using one-way ANOVA. An independent sample t-test was used to test the difference of cloacal temperatures in pre- and post-experiments in each group. A repeated-measures factorial ANOVA was used to determine the significance of the ABR threshold and latency at each stimulus frequency among the temperature groups. Differences in the ABR threshold at the same stimulus frequency among the temperature groups were analyzed using the multivariate analysis of the general linear model followed by a least significant difference (LSD) post-hoc test. Differences in the latency or ABR amplitude for click responses at the same stimulus intensity among the temperature groups were analyzed using the multivariate analysis of the general linear model followed by an LSD post-hoc test. Results are expressed as mean ± SD. The significance level was set at P < 0.05.
Results
Morphological Data
There was no significant difference in body mass (F = 1.66, P = 0.20) and carapace length among the temperature groups (F = 1.52, P = 0.23) (Table 1).

Table 1. Comparison of the body mass and carapace length of Trachemys scripta elegans among the temperature groups.
Temperature
The ambient temperature is shown in Table 2. The cloacal temperature of T. scripta elegans showed slight variations before and after the electrophysiological experiments (Table 2). No significant difference was found of cloacal temperatures in pre- and post-electrophysiological experiments in each group (P > 0.05), except for the group of 10°C.

Table 2. Changes in the cloacal temperature of Trachemys scripta elegans in pre- and post-electrophysiological experiments.
Auditory Brainstem Response Wave Morphology
The effect of the stimuli was identified using ABR waves evoked to click stimuli, which were characterized using valley–peak waveforms (Figure 1). Figures 1A–D shows a typical ABR response level series measured in a male T. scripta elegans in different temperature groups, for which the thresholds of 80 (A), 75 (B), 65 (C), and 60 dB SPL (D).
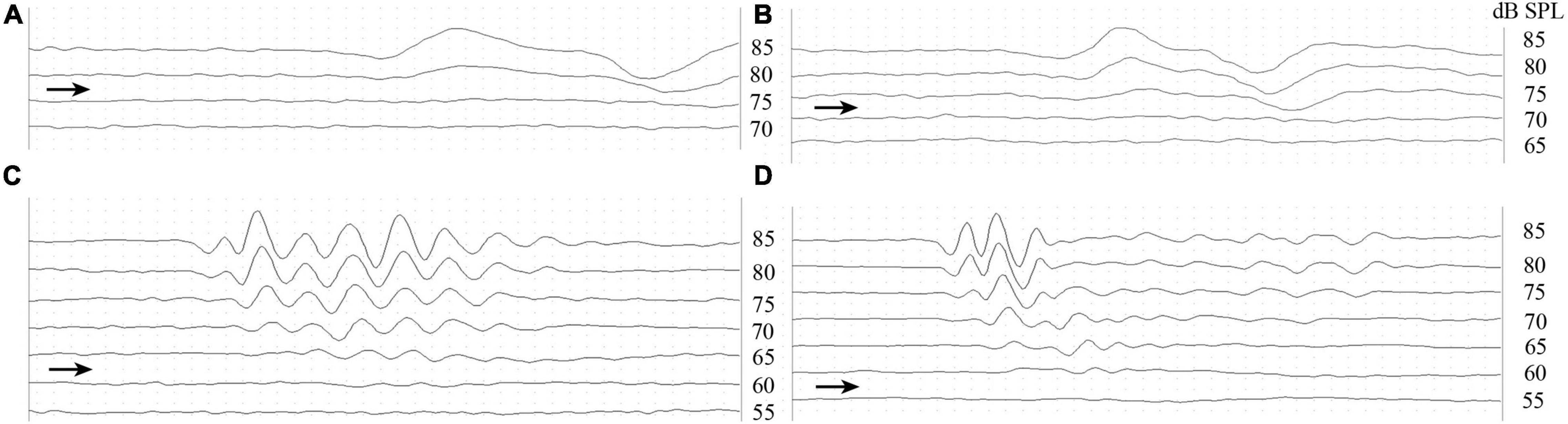
Figure 1. Valley–peak waveforms of click stimuli from a male Trachemys scripta elegans at 10°C (A), 20°C (B), 30°C (C), and 40°C (D). The right-pointing arrowheads indicate the visually detected thresholds for stimulus frequency.
Hearing Sensitivity Bandwidth and Auditory Brainstem Response Threshold
We measured the hearing sensitivity bandwidth and ABR threshold of individual turtles at all predetermined stimulus frequencies. Thereafter, the hearing sensitivity bandwidth and ABR threshold of each stimulus frequency were compared among different temperature groups. The hearing sensitivity bandwidths were 0.2–0.5, 0.2–1.1, 0.2–1.3, and 0.2–1.5 kHz in the temperature groups of 10, 20, 30, and 40°C, respectively (Figure 2). Trachemys scripta elegans had U-shaped ABR-derived audiograms (except that of the 10°C group) and a threshold above 40 dB at all stimulus frequencies (Figure 2).
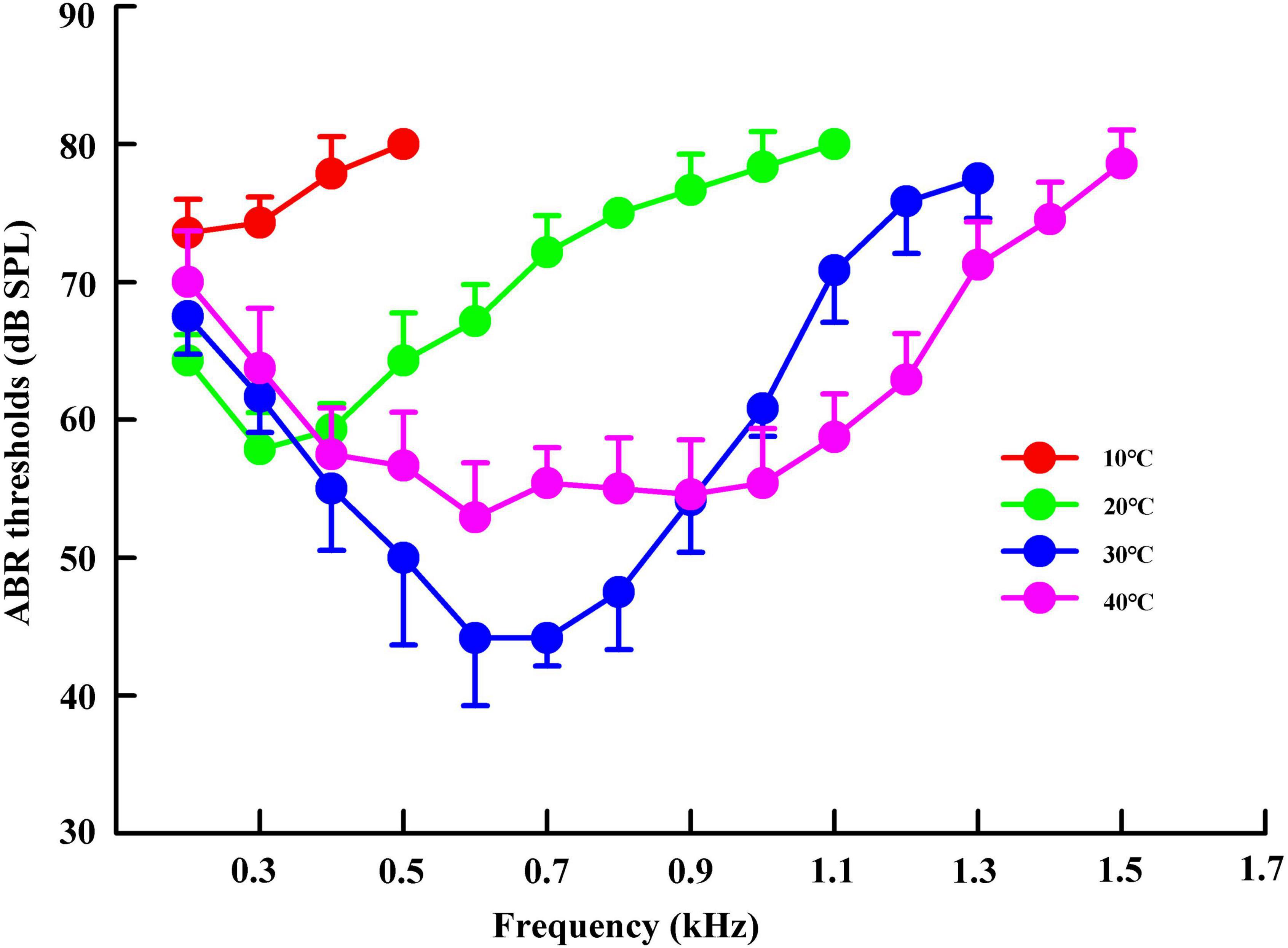
Figure 2. Variations in the auditory brainstem response (ABR) threshold of Trachemys scripta elegans at a stimulus frequency in different temperature groups. The plotted points represent the thresholds for tone bursts (mean ± standard deviation).
The lowest ABR thresholds were found at 0.2, 0.3, 0.6–0.7, and 0.6 kHz under 10, 20, 30, and 40°C, respectively. The ABR threshold decreased with increasing temperature (from 10 to 30°C), with significant differences among the temperature groups (F = 315.94, P < 0.05). The ABR threshold was significantly lower under 20°C than under 10°C, 30°C, and 40°C at the frequency of 0.3 kHz; significantly higher under 20°C than under 30°C and 40°C at 0.4–1.1 kHz (P < 0.05); significantly lower under 30°C than under 40°C at 0.5–0.8 kHz; and significantly higher under 30°C than under 40°C at 1.0–1.3 kHz (Table 3).
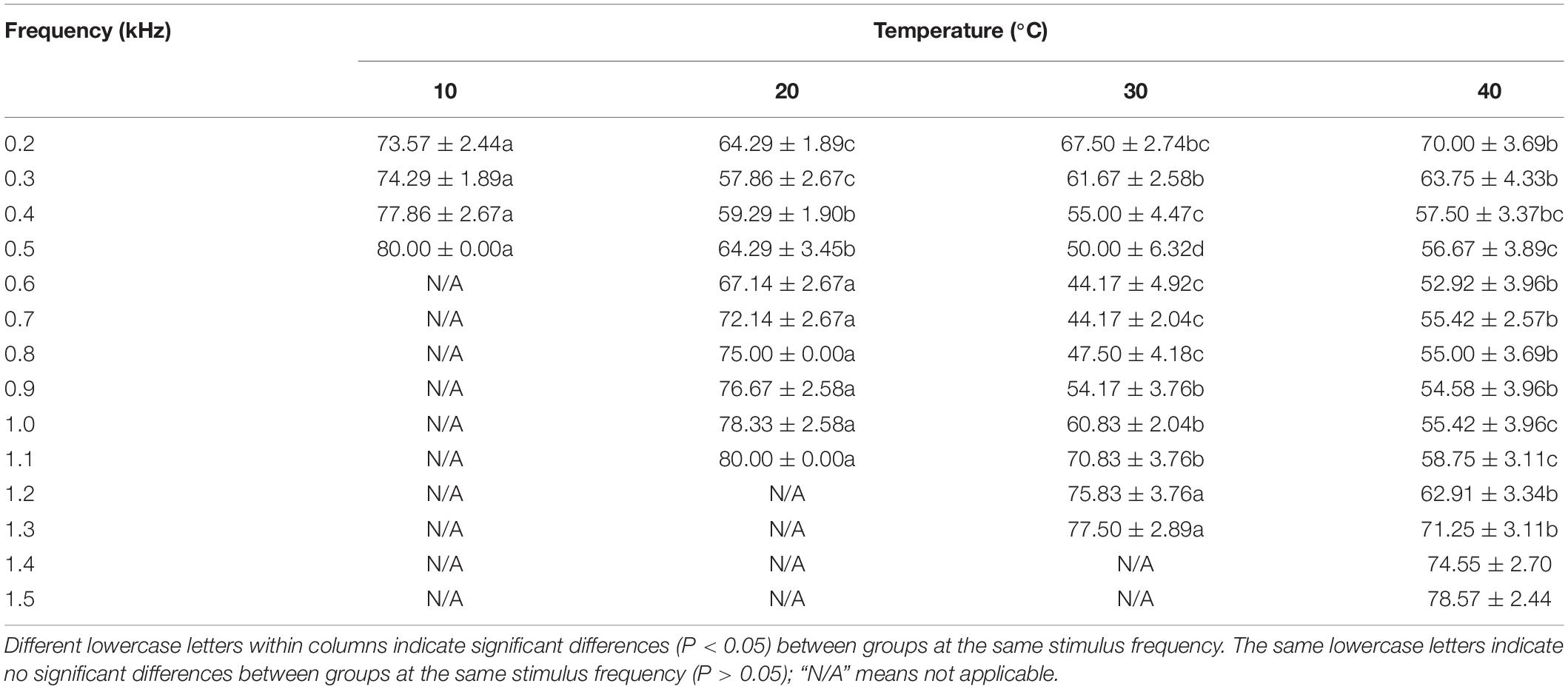
Table 3. Differences in the auditory brainstem response (ABR) threshold of Trachemys scripta elegans at each stimulus frequency among the temperature groups.
Latency
The latency was significantly longer at 20°C than at 30 and 40°C for intensities in the range of 75–80 dB (P < 0.05) (Table 4). The latency was significantly longer at 30°C than at 40°C for intensities in the 65–85 dB range (P < 0.05) (Table 4).
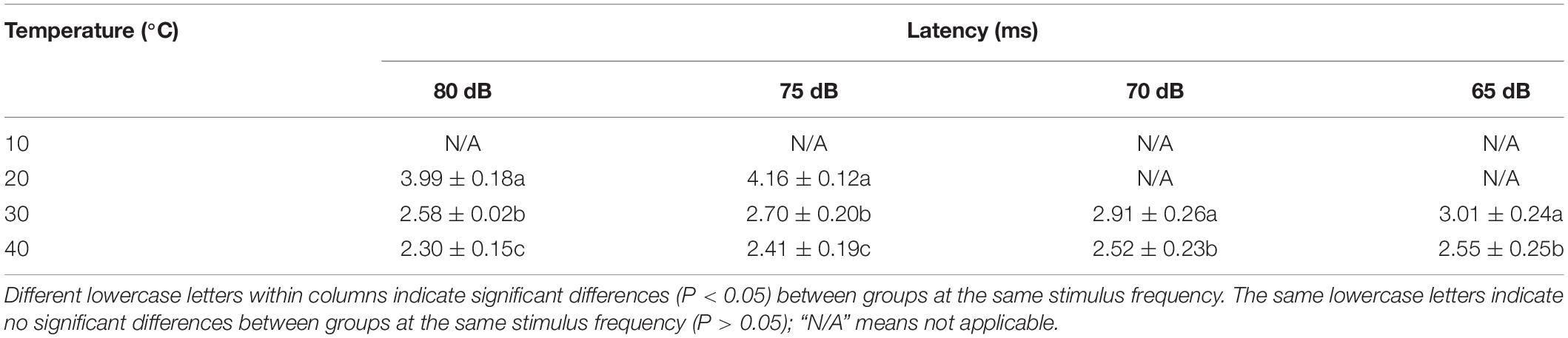
Table 4. Differences in latency in Trachemys scripta elegans at each stimulus intensity among the temperature groups.
Auditory Brainstem Response Amplitude
The ABR amplitude was significantly shorter at 40°C for 65-dB intensity (P < 0.05) (Table 5).

Table 5. Differences in the auditory brainstem response (ABR) amplitude in Trachemys scripta elegans at each stimulus intensity among the temperature groups.
Discussion
In contrast to most previous studies on the influence of temperature on the auditory system of reptiles (Patterson et al., 1968; Campbell, 1969; Werner, 1972, 1976), our study answered the questions on how temperature affects the physiological response of the auditory system in turtles with a poor hearing ability (hearing range mostly below 1 kHz). The temperature levels used in our study ranged from 10 to 40°C, and the temperature of 40°C was close to the upper limit of heat resistance of T. scripta elegans. We found that although the hearing range of turtles is confined to a low frequency compared with that of other animal groups, hearing showed exceptional regulation ability, especially when the temperature was close to the upper limit of heat resistance. To our knowledge, this is the first report on turtle hearing that showed frequency sensitivity differences when the temperature was close to the upper limit of heat resistance.
The temperature dependence of hearing sensitivity has been reported is several animal taxa. In fish, Wysocki et al. (2009) showed that the hearing threshold of the channel catfish Ictalurus punctatus decreased with increasing temperature (from 10 to 26°C) at frequencies of 0.1–4.0 kHz, more notably at higher frequencies. In anurans, by recording multiple-unit activity in the torus semicircularis, Mohneke and Schneider (1979) showed that the hearing threshold and latency of a male yellow-bellied toad (Bombina vs. variegata) decreased with increasing temperature from 5 to 20°C. The hearing sensitivity bandwidth was 0.1–2.0 kHz at 5°C, and 0.1–3.0 kHz at 20°C; however, the hearing sensitivity did not further increase with increasing temperature from 20 to 28°C (Mohneke and Schneider, 1979). In lizards, the hearing threshold of Sceloporus magister decreased with increasing temperature (from 16 to 26°C), decreased in the frequency range of 0.5–6.0 kHz, and increased in the frequency range of 8–10 kHz with temperature increasing from 26 to 35°C. The hearing sensitivity bandwidth was 0.6–10 kHz at 16°C, 0.1–10 kHz at 26°C, and 0.5–10 kHz at 35°C (Campbell, 1969). The hearing threshold of Dipsosaurus dorsalis decreased in the frequency from 0.8 to 10.0 kHz, but did not visibly change in frequency from 0.1 to 0.6 kHz with increasing temperature from 20 to 38°C (Werner, 1972). Similar patterns of change in hearing threshold with increasing temperature has also been found in Uma scoparia (Werner, 1972). In another lizard species (e.g., Cnemidophorus tigris), the hearing threshold decreased at 0.5–10 kHz, and no significant difference was observed at 0.1–0.4 kHz with temperature increasing from 21 to 35°C (Campbell, 1969). Turtles showed a trend similar to that of most vertebrate classes; an increase in temperature from 10 to 30°C resulted in improved hearing sensitivity. However, when the temperature was close to the upper limit of heat resistance, the threshold sensitivity of T. scripta elegans showed exceptional frequency sensitivity differences; it increased only in the high-frequency range (1.0–1.3 kHz), decreased in the intermediate frequency range (0.5–0.8 kHz), and did not significantly change in the low-frequency range (0.2–0.4 kHz). The hearing sensitivity bandwidth continued to change, and the latency further decreased with increasing temperature from 30 to 40°C when the upper sound level limit of stimulus was 85 dB SPL. Therefore, our results suggest that although the hearing range of turtle is confined to low frequencies compared with that of other animal groups, their hearing system has exceptional regulation ability, especially when the temperature is close to the upper limit of the heat resistance.
Studies in humans (Kaga et al., 1979) and other mammals (Doyle and Fria, 1985; Gold et al., 1985) have demonstrated that reduced body temperature results in a decrease in the amplitude of the auditory nerve action potential and prolongs latency. In anurans, temperature changes considerably affect the threshold of auditory nerve fibers (Stiebler and Narins, 1990; van Dijk et al., 1990). Eatock and Manley (1981) found that the whole tuning curve shifted reversibly up in frequency with warming and down with cooling in the Tokay gecko. Therefore, insensitive hearing of T. scripta elegans at low temperatures may be caused by the low temperatures that result in a higher threshold of auditory nerve fibers and prolong the nerve conduction time. In the inner ear of turtles, the auditory papilla is small and, similar to all amniote papillae, organized tonotopically, such that higher-frequency sounds excite the hair cells at the base and lower frequencies at the apex (Crawford and Fettiplace, 1980). Therefore, temperature may have differential effects on various parts of the auditory papilla in T. scripta elegans. Further research is needed to determine the physiological mechanisms underlying the exception frequency sensitivity differences when the temperature is close to the upper limit of heat resistance.
Most studies have shown that the relatively high body temperature of reptiles can promote the maintenance and regulation of behavioral and physiological functions (Huey and Kingsolver, 1989; Huey and Berrigan, 1996; Angilletta et al., 2002). Gu et al. (2016) found that the metabolism and activity increased with increasing acclimation temperature in T. scripta elegans (Gu et al., 2016). Ma (2013) reported that T. scripta elegans prefers 25–30°C, with temperatures of approximately 30°C during reproduction (Ma, 2013). Therefore, the more sensitive neurophysiological response of the turtle auditory system, accompanied by a relatively high metabolism level, as well as higher activity and other physiological and metabolic processes, promote increased fitness under relatively high ambient temperatures. In this study, we showed that the hearing sensitivity bandwidth remained elevated and latency decreased further at 40°C. Moreover, a high temperature increased the sensitivity to high frequency, which implies that the turtle auditory system can adapt to the high-frequency sounds environment in the context of global warming.
Some studies have shown that the influence of temperature on the auditory system may differ depending on whether a species is physiologically adjusted to tolerate a wide or narrow temperature range (Wysocki et al., 2009). Our study is expected spur further research on the high-temperature plasticity of hearing sensitivity in diverse taxa or in the same group with different temperature ranges. Our results have the potential to facilitate the forecast of the adaptive evolution of the auditory system to global warming.
Data Availability Statement
The original contributions presented in the study are included in the article/Supplementary Material, further inquiries can be directed to the corresponding author.
Ethics Statement
Animal Research Ethics Committee of Hainan Provincial Education Centre for Ecology and Environment, Hainan Normal University (HNECEE-2018-001).
Author Contributions
TW participated in data analysis and draft the manuscript. HL carried out the lab work and participated in data analysis. BC helped carry out the statistical analyses. JC and HS participated in the design of the study and critically revised the manuscript. JW conceived of the study, designed the study, and co-ordinated the study. All authors contributed to the article and approved the submitted version.
Funding
This work was supported by the National Natural Science Foundation of China (31860608 JW).
Conflict of Interest
The authors declare that the research was conducted in the absence of any commercial or financial relationships that could be construed as a potential conflict of interest.
Publisher’s Note
All claims expressed in this article are solely those of the authors and do not necessarily represent those of their affiliated organizations, or those of the publisher, the editors and the reviewers. Any product that may be evaluated in this article, or claim that may be made by its manufacturer, is not guaranteed or endorsed by the publisher.
Acknowledgments
We would like to thank Xiaofei Zhai, Chunhua Zhou, and Xintong Li for their assistance during this study.
Supplementary Material
The Supplementary Material for this article can be found online at: https://www.frontiersin.org/articles/10.3389/fevo.2022.856660/full#supplementary-material
References
Angilletta, M. J. Jr., Niewiarowski, P. H., and Navas, C. A. (2002). The evolution of thermal physiology in ectotherms. J. Therm. Biol. 27, 249–268. doi: 10.1016/S0306-4565(01)00094-8
Bierman, H. S., Thornton, J. L., Jones, H. G., Koka, K., Young, B. A., Brandt, C., et al. (2014). Biophysics of directional hearing in the American alligator (Alligator mississippiensis). J. Exp. Biol. 217, 1094–1107. doi: 10.1242/jeb.092866
Brittan-Powell, E. F., Dooling, R. J., and Gleich, O. (2002). Auditory brainstem responses in adult budgerigars (Melopsittacus undulatus). J. Acoust. Soc. Am. 112, 999–1008. doi: 10.1121/1.1494807
Campbell, H. W. (1969). The effects of temperature on the auditory sensitivity of Lizards. Physiol. Zool. 42, 183–210.
Capranica, R. R., and Moffat, A. J. M. (1983). “Neurobehavioral correlates of sound communication in anurans,” in Advances in Vertebrate Neuroethology, eds J.-P. Ewert, R. R. Capranica, and D. Ingle (CityplaceNew York, StateNY: Plenum Press), 701–730.
Christensen-Dalsgaard, J., Brandt, C., Willis, K. L., Christensen, C. B., Ketten, D., Edds-Walton, P., et al. (2012). Specialization for underwater hearing by the tympanic middle ear of the turtle, Trachemys scripta elegans. P. Roy. Soc. B. 279, 2816–2824. doi: 10.1098/rspb.2012.0290
Crawford, A., and Fettiplace, R. (1980). The frequency selectivity of auditory nerve fibres and hair cells in the cochlea of the turtle. J. Physiol. 306, 79–125. doi: 10.1113/jphysiol.1980.sp013387
Dooling, R. J., Fay, R. R., and Popper, A. N. (2000). Comparative Hearing: Birds and Reptiles. CityplaceNew York, StateNY: Springer.
Doyle, W. J., and Fria, T. J. (1985). The effects of hypothermia on the latencies of the auditory brain-stem response (ABR) in the rhesus monkey. Electroencephalogr. Clin. Neurophysiol. 60, 258–266. doi: 10.1016/0013-4694(85)90040-9
Du, W. G., Zhao, B., Chen, Y., and Shinec, R. (2011). Behavioral thermoregulation by turtle embryos. Proc. Natl. Acad. Sci. U S A. 108, 9513–9515. doi: 10.1073/pnas.1102965108
Duarte, H., Tejedo, M., Katzenberger, M., Marangoni, F., Baldo, D., Beltrán, J. F., et al. (2012). Can amphibians take the heat? vulnerability to climate warming in subtropical and temperate larval amphibian communities. Global Change Biol. 18, 412–421. doi: 10.1111/j.1365-2486.2011.02518.x
Eatock, R. A., and Manley, G. A. (1981). Auditory nerve fibre activity in the Tokay gecko: II. temperature effect on tuning. J. Comp. Physiol. 142, 219–226. doi: 10.1007/BF00605740
Ernst, C. H., and Lovich, J. E. (2009). Turtles of the United States and Canada. CityBaltimore, StateMD: PlaceNameplaceJohus PlaceNameHopkins PlaceTypeUniversity Press.
Fay, R. R., and Ream, T. J. (1992). The effects of temperature change and transient hypoxia on auditory nerve fiber response in the goldfish (Carassius auratus). Hear. Res. 58, 9–18. doi: 10.1016/0378-5955(92)90003-6
Ferrara, C. R., Vogt, R. C., Giles, J. C., and Kuchling, G. (2014). “Chelonian vocal communication,” in Biocommunication of Animals, ed. W. Guenther (CityplaceDordrecht: Springer), 261–274.
Franz, A., and Ronacher, B. (2002). Temperature dependence of temporal resolution in an insect nervous system. J. Comp. Physiol. A 188, 261–271. doi: 10.1007/s00359-002-0298-6
Gans, C., and Wever, E. G. (1974). Temperature effects on hearing in two species of Amphisbaenia. Nature 250, 79–80. doi: 10.1038/250079a0
Gerhardt, H. C., and Huber, F. (2002). Acoustic Communication in Insects and Anurans. CityChicago, StateIL: PlaceTypeplaceUniversity of PlaceNameChicago Press.
Gold, S., Cahani, M., Sohmer, H., Horowitz, M., and Shahar, A. (1985). Effects of body temperature elevation on auditory nerve-brain-stem evoked responses and EEGs in rats. Electroencephalogr. Clin. Neurophysiol. 60, 146–153. doi: 10.1016/0013-4694(85)90021-5
Gu, C. J., Jin, J. Y., Shangguan, F. G., Mao, L. N., Zhou, H. B., and Zhang, Y. P. (2016). Influence of temperature acclimation on selected body temperature, thermal tolerance, and antioxidant enzyme activity of juvenile red-eared slider turtles (Trachemys scripta elegans). Acta Ecol. Sinica 36, 1737–1745.
Higgs, D. M. (2002). Development of the fish auditory system: how do changes in auditory structure affect function? Bioacoustics 12, 180–183. doi: 10.1080/09524622.2002.9753688
Huey, R. B., and Kingsolver, J. G. (1989). Evolution of thermal sensitivity of ectotherm performance. Trends Ecol. Evol. 4, 131–135. doi: 10.1016/0169-5347(89)90211-5
Huey, R., and Berrigan, D. (1996). “Testing evolutionary hypotheses of acclimation,” in Animals and Temperature: Phenotypic and E Volutionary Adaptation. Society for E xperimental Biology Seminar Series, eds I. A. Johnston and A. F. Bennett (CityplaceCambridge: Cambridge University Press).
Hutchison, V. H., Vinegar, A., and Kosh, R. J. (1966). Critical thermal maxima in turtles. Herpetological 22, 32–41. doi: 10.1016/0300-9629(73)90085-6
Kaga, K., Takiguchi, T., Myokai, K., and Shiode, A. (1979). Effects of deep hypothermia and circulatory arrest on the auditory brain stem responses. Arch. Oto-rhino-laryngol. 225, 199–205. doi: 10.1007/BF00455255
Kastak, D., and Schusterman, R. (1999). In-air and underwater hearing sensitivity of a northern elephant seal (Mirounga angustirostris). country-regionplaceCan. J. Zool. 77, 1751–1758.
Köppl, C., Manley, G. A., Popper, A. N., and Fay, R. R. (2014). Insights from Comparative Hearing Research. StateplaceBerlin: Springer.
Laloë, J. O., Cozens, J., Renom, B., Taxonera, A., and Hays, G. C. (2017). Climate change and temperature-linked hatchling mortality at a globally important sea turtle nesting site. Global Change Biol. 23, 4922–4931. doi: 10.1111/gcb.13765
Llusia, D., Márquez, R., Beltrán, J. F., Benítez, M., and Do Amaral, J. P. (2013). Calling behaviour under climate change: geographical and seasonal variation of calling temperatures in ectotherms. Global Change Biol. 19, 2655–2674. doi: 10.1111/gcb.12267
Lovelace, C. T., Stein, B. E., and Wallace, M. T. (2003). An irrelevant light enhances auditory detection in humans: a psychophysical analysis of multisensory integration in stimulus detection. Cogn. Brain Res. 17, 447–453. doi: 10.1016/s0926-6410(03)00160-5
Luo, J., Koselj, K., Zsebok, S., Siemers, B. M., and Goerlitz, H. R. (2014). Global warming alters sound transmission: differential impact on the prey detection ability of echolocating bats. J. R. Soc. Interface 11:20130961. doi: 10.1098/rsif.2013.0961
Ma, K. (2013). Activity Rhythm and Home Range of Exotic Species Red-Eared Turtle in Wanquan River, Hainan Island, China. Master thesis. Haikou: Hainan Normal University.
Maiditsch, I. P., and Ladich, F. (2014). Effects of temperature on auditory sensitivity in eurythermal fishes: common carp Cyprinus carpio (Family Cyprinidae) versus CityplaceWels catfish Silurus glanis (Family Siluridae). PLoS One 9:e108583. doi: 10.1371/journal.pone.0108583
Martin, K. J., Alessi, S. C., Gaspard, J. C., Tucker, A. D., Bauer, G. B., and Mann, D. A. (2012). Underwater hearing in the loggerhead turtle (Caretta caretta): a comparison of behavioral and auditory evoked potential audiograms. J. Exp. Biol. 215, 3001–3009. doi: 10.1242/jeb.066324
Mohneke, R., and Schneider, H. (1979). Effect of temperature upon auditory thresholds in two anuran species, Bombina v. variegata and Alytes o. obstetricans (Amphibia, Discoglossidae). J. Comp. Physiol. A. 130, 9–16. doi: 10.1007/BF02582969
Oldfield, B. P. (1988). The effect of temperature on the tuning and physiology of insect auditory receptors. Hear. Res. 35, 151–158. doi: 10.1016/0378-5955(88)90114-1
Ospina, O. E., Villanueva-Rivera, L. J., Corrada-Bravo, C. J., and Aide, T. M. (2013). Variable response of anuran calling activity to daily precipitation and temperature: implications for climate change. Ecosphere 4:art47. doi: 10.1890/ES12-00258.1
Parks, T. N., Rubel, E. W., Fay, R. R., and Popper, A. N. (2004). Plasticity of the Auditory System. CityplaceNew York, StateNY: Springer.
Parmenter, R., and Avery, H. (1990). “The feeding ecology of the slider turtle,” in Life History and Ecology of the Slider Turtle, ed. J. W. Gibbons (CityplaceWashington, StateDC: Smithsonian Institution Press), 257–265.
Patterson, W. C., Evering, F. C., and McNall, C. L. (1968). The relationship of temperature to the cochlear response in a poikilotherm. J. Aud. Res. 8, 439–448.
Pires, A., and Hoy, R. (1992). Temperature coupling in cricket acoustic communication. I. field and laboratory studies of temperature effects on calling song production and recognition in Gryllus firmus. J. Comp. Physiol. A 171, 69–78. doi: 10.1007/BF00195962
Schrode, K. M., Buerkle, N. P., Brittan-Powell, E. F., and Bee, M. A. (2014). Auditory brainstem responses in Cope’s gray treefrog (Hyla chrysoscelis): effects of frequency, level, sex and size. J. Comp. Physiol. A 200, 221–238. doi: 10.1007/s00359-014-0880-8
Scott, L., Brecht, E., Philpo, A., Iyer, S., Wu, N., Mihic, S., et al. (2017). A novel BK channel-targeted peptide suppresses sound evoked activity in the mouse inferior colliculus. Sci. Rep. 7:42433. doi: 10.1038/srep42433
Sinervo, B., Méndez-de-la-Cruz, F., Miles, D. B., Heulin, B., Bastiaans, E., Villagrán-Santa Cruz, M., et al. (2010). Erosion of lizard diversity by climate change and altered thermal niches. Science 328, 894–899. doi: 10.1126/science.1184695
Smolders, J. W. T., and Klinke, R. (1984). Effects of temperature on the properties of primary auditory fibres of the spectacled caiman, Caiman crocodilus (L.). J. Comp. Physiol. A 155, 19–30. doi: 10.1007/BF00610927
Sollid, J., Weber, R. E., and Nilsson, G. E. (2005). Temperature alters the respiratory surface area of crucian carp Carassius carassius and goldfish Carassius auratus. J. Exp. Biol. 208, 1109–1116. doi: 10.1242/jeb.01505
Stiebler, I. B., and Narins, P. M. (1990). Temperature-dependence of auditory nerve response properties in the frog. Hear. Res. 46, 63–81. doi: 10.1016/0378-5955(90)90140-k
Stockard, J. J., Sharbrough, F. W., and Tinker, J. A. (1978). Effects of hypothermia on the human brainstem auditory response. Ann. Neurol. 3, 368–370. doi: 10.1002/ana.410030416
Sun, B., Williams, C. M., Li, T., Speakman, J. R., and Jin, Z. (2022). Higher metabolic plasticity in temperate compared to tropical lizards suggests increased resilience to climate change. Ecol. Monogr. 92:e1512. doi: 10.1002/ecm.1512
Sun, X., Zhao, L., Chen, Q., Wang, J., and Cui, J. (2019). Auditory sensitivity changes with diurnal temperature variation in little torrent frogs (Amolops torrentis). Bioacoustics 29, 684–696.
van Dijk, P., Lewis, E. R., and Wit, H. P. (1990). Temperature effects on auditory nerve fiber response in the American bullfrog. Hear. Res. 44, 231–240. doi: 10.1016/0378-5955(90)90083-2
Wang, J. C., Li, H. D., Wang, T. L., Chen, B., Cui, J. G., and Shi, H. T. (2021). Ontogenetic development of hearing sensitivity to airborne sound in the female red-eared slider, Trachemys scripta elegans. J. Acoust. Soc. Am. 149, 819–824. doi: 10.1121/10.0003359
Wang, T. L., Li, H. D., Cui, J. G., Zhai, X. F., Shi, H. T., and Wang, J. C. (2019). Auditory brainstem responses in the red-eared slider Trachemys scripta elegans (Testudoformes: Emydidae) reveal sexually dimorphic hearing sensitivity. J. Comp. Physiol. A 205, 847–854. doi: 10.1007/s00359-019-01372-y
Werner, Y. L. (1972). Temperature effects on inner-ear sensitivity in six species of iguanid lizards. J. Herpetol. 6, 147–177.
Werner, Y. L. (1976). Optimal temperatures for inner-ear performance in gekkonoid lizards. J. Exp. Zool. 195, 319–351. doi: 10.1002/jez.1401950302
Whitford, M. D., Freymiller, G. A., Higham, T. E., and Clark, R. W. (2020). The effects of temperature on the defensive strikes of rattlesnakes. J. Exp. Biol. 223:jeb223859. doi: 10.1242/jeb.223859
Wysocki, L. E., Montey, K., and Popper, A. N. (2009). The influence of ambient temperature and thermal acclimation on hearing in a eurythermal and a stenothermal otophysan fish. J. Exp. Biol. 212, 3091–3099. doi: 10.1242/jeb.033274
Keywords: auditory response, peripheral hearing sensitivity, temperature, Trachemys scripta elegans, adaptation
Citation: Wang T, Li H, Chen B, Cui J, Shi H and Wang J (2022) Effect of Temperature on the Plasticity of Peripheral Hearing Sensitivity to Airborne Sound in the Male Red-Eared Slider Trachemys scripta elegans. Front. Ecol. Evol. 10:856660. doi: 10.3389/fevo.2022.856660
Received: 17 January 2022; Accepted: 16 May 2022;
Published: 09 June 2022.
Edited by:
Lin Zhang, Hubei University of Chinese Medicine, ChinaCopyright © 2022 Wang, Li, Chen, Cui, Shi and Wang. This is an open-access article distributed under the terms of the Creative Commons Attribution License (CC BY). The use, distribution or reproduction in other forums is permitted, provided the original author(s) and the copyright owner(s) are credited and that the original publication in this journal is cited, in accordance with accepted academic practice. No use, distribution or reproduction is permitted which does not comply with these terms.
*Correspondence: Jichao Wang, d2pjQGhhaW5udS5lZHUuY24=