- 1Department of Biology, College of Staten Island CUNY, New York, NY, United States
- 2Biology Doctoral Program, Graduate Center CUNY, New York, NY, United States
European gulls Chroicocephalus ridibundus, Larus canus, and L. graellsii have dispersed to North America and C. ridibundus and L. graellsii have bred or attempted to breed. North American gulls L. delawarensis, Leucophaeus atricilla, Leucophaeus pipixcan, and Chroicocephalus philadelphia have dispersed to Europe, although no successful breeding by non-hybrid pairs has yet occurred. We hypothesized that as gull population sizes increase, the number of birds exploring potential new breeding sites also increases. To test our hypothesis, we compared the number of transatlantic vagrants to the population size on the previous year using generalized linear models. We found an increasing number of transatlantic vagrants moving in both directions, which suggests that vagrancy is not a random phenomenon driven by strong winds nor caused by reverse migration. Population size predicted transatlantic vagrancy in four of the seven species. However, our hypothesis that increases in population size drive increases in vagrancy was only supported in two of these instances. We further looked at sub-populations of L. delawarensis in North America and tested our hypothesis for each subpopulation. We found partial support for our hypothesis for these data. Even within one species, we observed multiple relationships between vagrancy and population size. Our results showed that size or trend in source population size—in some circumstances—is clearly a driver of vagrancy, but other factors must play an important role too. As anthropogenic development continues, and high-quality habitats become farther apart, it is important that we continue to investigate all drivers of vagrancy because the persistence of a species may depend crucially on its longest-distance dispersers.
Introduction
The long-distance movement of individuals that disperse considerably outside the margins of their current species’ range is called vagrancy (Grinnell, 1922; Baker, 1978). As the size of populations increases, these margins change, thus, vagrancy is an incessant process (Veit et al., 2021). On the other hand, migration is a dispersal that occurs annually, usually either toward a breeding or a non-breeding location (Veit et al., 2021). To distinguish whether a dispersing individual is a “migrant” or a “vagrant” is impossible because vagrants are just the extremes of dispersal (DeSante and George, 1994). Thus, all individuals disperse, those with the most consistent orientations are “migrants,” while those that disperse farther than the population average are “vagrants” (Veit, 1997; Howell et al., 2014; Lees and Gilroy, 2014; Veit et al., 2021). The majority of vagrants are pre-breeders, usually hatching year but can be much older in seabirds like gulls that do not breed until approximately 5 years old (Baird et al., 1958; Kadlec and Drury, 1968; Veit and Lewis, 1996; Veit, 2000; Newton, 2008). It is possible that only especially physically fit individuals can bear the high energetic cost of larger than usual dispersions typical of vagrancy events (Davis and Watson, 2018). Because of its exploratory nature, vagrancy is related to range expansions and distribution shifts that may be critical for an efficient adaptive response of species to the current human-induced climate change (Jiguet and Barbet-Massin, 2013). Additionally, vagrants contribute to the genetic mix of populations and reduce competitive pressures in the source population (Ronce, 2007). Thus, understanding the mechanism underlying vagrancy has important implications for effective conservation planning to mitigate population losses.
In the case of birds, there have been several proposed explanations of vagrancy including wind drift hypothesis, reverse migration hypothesis and increase of population size hypothesis. According to the wind drift hypothesis, birds are swept by strong prevailing winds and end up at “wrong” locations during migration (Mclaren, 1981). However, vagrant birds have been recorded in years without strong anticyclones (Pfeifer et al., 2007; Alfrey et al., 2018). Additionally, Baker (1980) argued that vagrant birds are not helplessly carried by winds, but they use winds to their advantage when performing long-distance exploration, which minimizes the energetic cost of the travel. The reverse migration hypothesis states that the internal navigational mechanisms of “defective birds” mistake north for south making them migrate in the “wrong” direction, with a 180° rotation of the usual migratory route of the species (Rabøl, 1969; Thorup, 2004). Nevertheless, moving in the opposite direction or sideways from the standard migratory route does not imply a malfunction in the migratory mechanism since this behavior is characteristic of the exploratory nature of birds (Baker, 1980). The population size hypothesis, on the other hand, does not assume differences in behavior between vagrant and non-vagrant birds, and states that vagrancy occurs as a result of a large reproductive outcome in the populations, that is, a larger number of young produced and, consequently, population sizes increased with time (Veit, 1997, 2000). Thus, as the population increases, the carrying capacity of the currently occupied habitat can be surpassed and competitive pressures increased, conditions that will lead to increases in dispersal and vagrancy events (DeSante, 1983) when immature birds move farther to explore, and potentially find higher-quality habitats (DeBenedictis, 1971; Mclaren, 1981; Lowe, 2009). Support for this hypothesis has been found for several passerine birds (Veit, 2000; Zawadzki et al., 2019) and in raptors (Ławicki and Perlman, 2017). These hypotheses are not mutually exclusive, for example, strong winds may contribute to vagrancy events in populations increasing their size. However, studies considering both winds and population size have found little support for winds to explain vagrancy increases, with increasing population being vagrancy’s main driver (Zawadzki et al., 2019).
The phenomenon of vagrancy is well documented in birds, although research has been mainly focused on landbirds (Veit, 1997, 2000; Thorup, 2004; Mclaren et al., 2006; Pfeifer et al., 2007; Bloom et al., 2011; Zawadzki et al., 2019). In seabirds, on the other hand, the potential causes of vagrancy are still poorly understood (but see Zawadzki et al., 2021 on Lesser Black-backed Gull source populations, and Veit et al., 2021 on elegant tern vagrancy; both in this special issue). Among seabirds, gulls are feeding and flight generalists with energy efficient flight modes that allow them to fly over water or land and to cover long distances, finding suitable food in almost any habitat (Klaassen et al., 2012). These advantageous characteristics can contribute to the gulls’ successful long-distance trips and make them an appealing group for studying vagrancy mechanisms. For example, Lesser Black-backed Gull individuals perform long-distance pre- and post-migratory round-trip movements, sometimes crossing European seas, presumably to explore potential feeding or wintering habitats (Klaassen et al., 2012). Additionally, several North American and European gull species have been recorded annually on the side of the Atlantic opposite to their original populations since the fifties (American Birding Association and National Audubon Society, 2019; British Birds Rarities Committee, 2019). Furthermore, gulls have experienced changes in population sizes with time (Grandgeorge et al., 2008; Morris et al., 2011; Camphuysen, 2013; Nisbet et al., 2013; Giroux et al., 2016). On one hand, the placement of protection laws, and the affiliation of some gull species to garbage dumps and fishery wastes in which they find nesting sites and food have been related to increases in gull population sizes (Grandgeorge et al., 2008). On the other hand, after 1990, population sizes of some species decreased markedly due to landfill closures, the collapse of offshore fisheries, and management measures like presence of raptors, nest removal and egg oiling (Morris et al., 2011; Camphuysen, 2013; Nisbet et al., 2013; Giroux et al., 2016). These documented changes in population sizes enhance the appeal of gulls as a study group. Despite these facts, to our knowledge, studies exploring the drivers of vagrancy in gulls are scarce (Vinicombe, 1985; Boertmann, 2009), most studies being dedicated to describing their typical migratory patterns and mechanisms (Kilpi and Saurola, 1984; Bledsoe and Sibley, 1985; Belant and Dolbeer, 1993; Pedersen et al., 2000; Helberg et al., 2009; Marques et al., 2010; Stenhouse et al., 2012; Bustnes et al., 2013; Baert et al., 2018).
In this article we focus specifically on seven gull species that breed on one side of the North Atlantic Ocean and are found as vagrants on the opposite side of the Atlantic. These species have been increasing their vagrancy records, they are very visible and easily identified. We test the hypothesis that the annual population size in the source area predicts vagrancy to the receiving area. The rationale behind this hypothesis is that increases in population size, based on the number of nesting pairs in the breeding season or the number of individuals in the wintering season, imply that large numbers of young are being produced. Thus, as the population increases, more young birds need to disperse and may become vagrants in the following year. Therefore, we expect that the number of transatlantic vagrants will increase in both directions in proportion to increases in the source population size as the immature birds explore potential new breeding sites.
Materials and Methods
Species Selection
To test the hypothesis that the annual population size in the source area predicts vagrancy to the receiving area, we selected seven North Atlantic gull species that have reported vagrants on the side of the Atlantic opposite to their original populations. The European species are Chroicocephalus ridibundus (Black-headed Gull), Larus canus (Common Gull), and L. graellsii (Lesser Black-backed Gull). The American gulls are Larus delawarensis (Ring-billed Gull), Leucophaeus atricilla (Laughing Gull), Leucophaeus pipixcan (Franklin’s Gull), and Chroicocephalus philadelphia (Bonaparte’s Gull). We used the total number of vagrants per species recorded from 1970 to 2010 as a criterion to include gull species in our study, since some species occur very infrequently on the other side of the Atlantic. In the specific case of Herring Gull, although there is a substantial data set, they were excluded from the analysis because it is very difficult to differentiate birds from different source locations (Europe, North America) based on morphology in the field (Olsen, 2018). Otherwise, all North Atlantic gulls with substantial vagrant records are used in our analyses, being Franklin’s Gull the species with the smallest number of vagrants recorded, with a total number of 87 records across the study period.
Population Size Data
European Gulls
To estimate the annual population size of the European gulls, we considered as source areas Scotland, England, Northern Ireland, Wales, and the Republic of Ireland. We obtained estimates of the species’ breeding population sizes for these regions from the British Breeding Seabird Censuses from 1969 to 2009 (BBSC; Joint Nature Conservation Committee [JNCC], 2019). During these censuses, stretches of coastline are divided into sites where species counts are conducted (Joint Nature Conservation Committee [JNCC], 2019). We included in our study only sites that were censused in all years of the study period, for a total of 28 sites (Supplementary Table 1). For our analysis, we considered the counts of apparent occupied nests, apparent occupied sites, and apparent occupied territories, adjusted to represent the number of breeding pairs. We estimated annual population size for each species as the total number of breeding pairs across sites. We checked for statistically significant temporal trends in population size using a non-parametric Spearman rank correlation coefficient (rs) between the observations and time for each period (“pastecs” R package).
North American Gulls
For the American species, we considered Canada and the United States as the source areas (latitudes between 24.597° and 81.825°, longitudes between −176.635° and −52.678°). To estimate the annual size of the populations of Franklin’s Gull, Laughing Gull and Ring-billed Gull, we extracted data from the Breeding Bird Survey from 1969 to 2009 (BBS, Pardieck et al., 2020). The BBS is a transect survey performed annually between May and June with routes measuring 39.2 km. Each route is divided in 50 stops, and, at each stop, every bird seen or heard is recorded. We included in the analysis only BBS routes that were sampled in all years of the study period, for a total of 115 routes (Supplementary Table 2). In the case of Bonaparte’s Gull, there were not enough annual BBS data to warrant our analysis. Bonaparte’s Gull nests in trees in habitats remote from human settlements making their detection difficult in surveys like the BBS (Burger and Gochfeld, 2020). In winter, however, Bonaparte’s Gull is found in large flocks in coastal areas close to human activity (Burger and Gochfeld, 2020). Therefore, for this species, we used instead population data extracted from the Christmas Bird Count (CBC) from 1969 to 2009 (National Audubon Society, 2019). The CBC is an annual survey performed at the beginning of the winter season in circles of about 24 km diameter in which abundances of all birds seen or heard within the circle are recorded. We included in the analysis only CBC circles that were sampled in all years of the study period and that had reported a sampling effort (i.e., number of party hours) greater than 0 h, for a total of 351 circles (Supplementary Table 3). We recognize that the numbers reported in the CBC are not an exact estimate of these species’ total population size, however, an increase in the total breeding population size will lead to an increase in the number of birds overwintering in the United States and Canada, which will be reflected in the gull’s numbers recorded during the CBC. Additionally, the CBC gulls’ numbers represent individuals that stay close to breeding ranges instead of migrating south in winter, individuals that might be, in turn, more likely to continue the eastward movement and cross the Atlantic Ocean, which makes this data set appropriate for testing our hypothesis for Bonaparte’s Gull. The fact that we used wintering populations for this species did not bias our results because our objective was not to compare populations between seasons. We consistently estimated population changes in the source area from year to year for each species and compared these changes with the number of vagrants to the receiving area. In any case, an increase in population due to reproductive output will be reflected in both wintering and breeding populations.
We estimated annual population size for Franklin’s Gull, Laughing Gull, and Ring-billed Gull as the total number of individuals across BBS routes. For Bonaparte’s Gull, we estimated annual population size as the total number of individuals across CBC circles divided by party hours, to account for sampling effort. For all the species, we checked for statistically significant temporal trends in population size as described above.
Vagrants’ Data
To estimate the annual number of European gulls’ transatlantic vagrants, we considered the East of North America (i.e., Northeastern Maritime Region, Atlantic Canada Region, New England Region, Hudson Delaware Region, Middle Atlantic Coast Region, Southern Atlantic Coast Region, and Florida Region) as the receiving area. We compiled vagrancy data reported in North American Birds journals for these species in these regions during fall and winter (i.e., August 1st to February 28th next year) from 1970 to 2010 (American Birding Association and National Audubon Society, 2019).
For the North American transatlantic vagrant gulls, we considered as receiving areas the United Kingdom, the Republic of Ireland and Iceland. These are obviously not the only areas which might receive vagrant individuals, but they have a long history of substantially sampled areas. To estimate the annual number of transatlantic vagrants reported in these areas from 1970 to 2010, we extracted vagrancy data for Laughing Gull, Franklin’s Gull and Bonaparte’s Gull from the rare birds reports published by the Birds Rarities Committees in these countries (British Birds Rarities Committee, 2019; Icelandic Institute of Natural History and Icelandic Rarities Committee [IINHIRC], 2020; Irish Rare Birds Committee [IRBC], 2020). For the Ring-billed Gull’s transatlantic vagrants, the data were taken exclusively from the reports on scarce migrant birds in Britain (Fraser and Rogers, 2002; White and Kehoe, 2017) and the Icelandic records (Icelandic Institute of Natural History and Icelandic Rarities Committee [IINHIRC], 2020). All these reports include vagrants sighted across all the seasons in a given year. We did not include Ring-billed Gull vagrants’ data from Ireland because the Irish Rare Birds Committee stopped assessing Ring-billed Gull records in 1994 as Ring-billed Gulls were so regularly occurring that they no longer met the criteria of being a rare bird (Colum Flynn, Irish Rare Birds Committee, personal communication). Therefore, including Irish records for this species only up to 1994 would have biased our vagrancy estimates.
We checked for statistically significant temporal trends in vagrancy as described above.
Within Species Analysis: Ring-Billed Gull’ Data
Because results from our transatlantic analysis might be due to interspecific differences in ecology, we assessed local variation in individual vagrancy within a single species. We chose Ring-billed Gull for its abundant data, and easily delimited regions within a widespread breeding range. We divided the species range into four regions: West (California, Washington, Oregon, Nevada, and British Columbia), West Central (Idaho, Alberta, Montana, Colorado, and Saskatchewan), East Central (Manitoba, North Dakota, South Dakota, and Minnesota), and East (Michigan, Illinois, Indiana, Ontario, Quebec, Newfoundland, New Brunswick, Nova Scotia, Maine, Vermont, New Hampshire, New York, Pennsylvania, and Ohio), and used these regional counts as indices of total population size within each region.
We utilized BBS data for breeding population estimates, and band recovery data from the Bird Banding Lab (USGS Bird Banding Laboratory, 2019) for the period 1960–2017. Since band recovery data informs about how far species disperse, we assessed vagrancy by using the number of recaptures with dispersal distances greater than zero as the response variable and breeding population size (estimated as the number of individuals divided by the number of routes sampled each year) as the explanatory variable. Correcting breeding population sizes by number of routes allowed comparison across years, in case there were differing numbers of routes where Ring-billed Gull were detected, across years (Supplementary Figure 1). For vagrancy numbers, we were better able to quantify sampling effort. As a measure of annual total effort per region, we analyzed the temporal trend of total bander days (number of days that a bander spent banding, summed over all banders in a given year and region). Bander effort significantly declined in East (p < 0.001) and West Central (p = 0.009) regions but did not change significantly in East Central or West regions (Supplementary Figure 2). Based on these results, we did not correct our recapture numbers for sampling effort because this probably did not bias the changes observed with time.
Statistical Analyses
To assess whether the total number of transatlantic vagrant gulls was increasing in both directions, we checked for statistically significant temporal trends in both the annual total number of European vagrants reported in the east of North America and the annual total number of North American vagrants recorded in the European receiving areas as described above. Additionally, we performed a correlation analysis between the annual total number of European vagrants reported in the east of North America and the annual total number of North American vagrants recorded in the European receiving areas. To calculate the annual total number of vagrants, we added all vagrant records of species coming from each source area for each year.
Statistical Models
The data for both population size and vagrancy were not normally distributed (Supplementary Table 4), and the variance of our response variable was greater than its mean in all the species (Supplementary Table 5). Thus, in our analysis we utilized generalized linear models (GLM, base R package) parameterized with the log link function and Negative Binomial family for the distribution of errors, as has been shown for seabirds in other studies (Veit and Manne, 2015). The fitted models related the source population size in a given year as explanatory variable of the number of transatlantic vagrants in the following year. To assess the GLM performance, we included a null model (no predictor) for each species. Of each pair of models per species, we selected as the best model the one with the lowest Akaike’s Information Criterion adjusted for small sample sizes (AICc; Burnham and Anderson, 2002; function aictab R package AICcmodavg; Mazerolle, 2020). Models with difference in AICc values lower than two units were considered as equivalent (Burnham and Anderson, 2002). In addition, we conducted Generalized Additive Models (GAMs) which, in most cases, showed AICc values very similar to the GLMs (Supplementary Table 6).
To test the hypothesis that the relationship between vagrancy and population size may be particular to local circumstances, we conducted the same analyses for four subpopulations of Ring-billed Gull (region definitions as above). As described above, we fitted a GLM (base package in R) for Ring-billed Gull in each geographic region (family = Negative Binomial and link function = “log”). In this case, the explanatory variable was the breeding population size and the response variable was the number of recaptures with dispersal distances greater than zero.
All statistical analyses were performed using R software (R Core Team, 2020).
Results
Transatlantic Vagrancy in Both Directions
The total number of European vagrants reported in east North America showed a significant increase with time (Figure 1, Spearman rank correlation rs = 0.86, p < 0.001). Likewise, the total number of North American vagrants reported in the selected European areas showed an increase through time (Figure 1, Spearman rank correlation rs = 0.68, p < 0.001). Additionally, these two estimates were strongly positively correlated to each other (Spearman rank correlation rs = 0.63, p < 0.001). Taken together, these results indicate that transatlantic vagrancy has increased in both directions between 1970 and 2010.
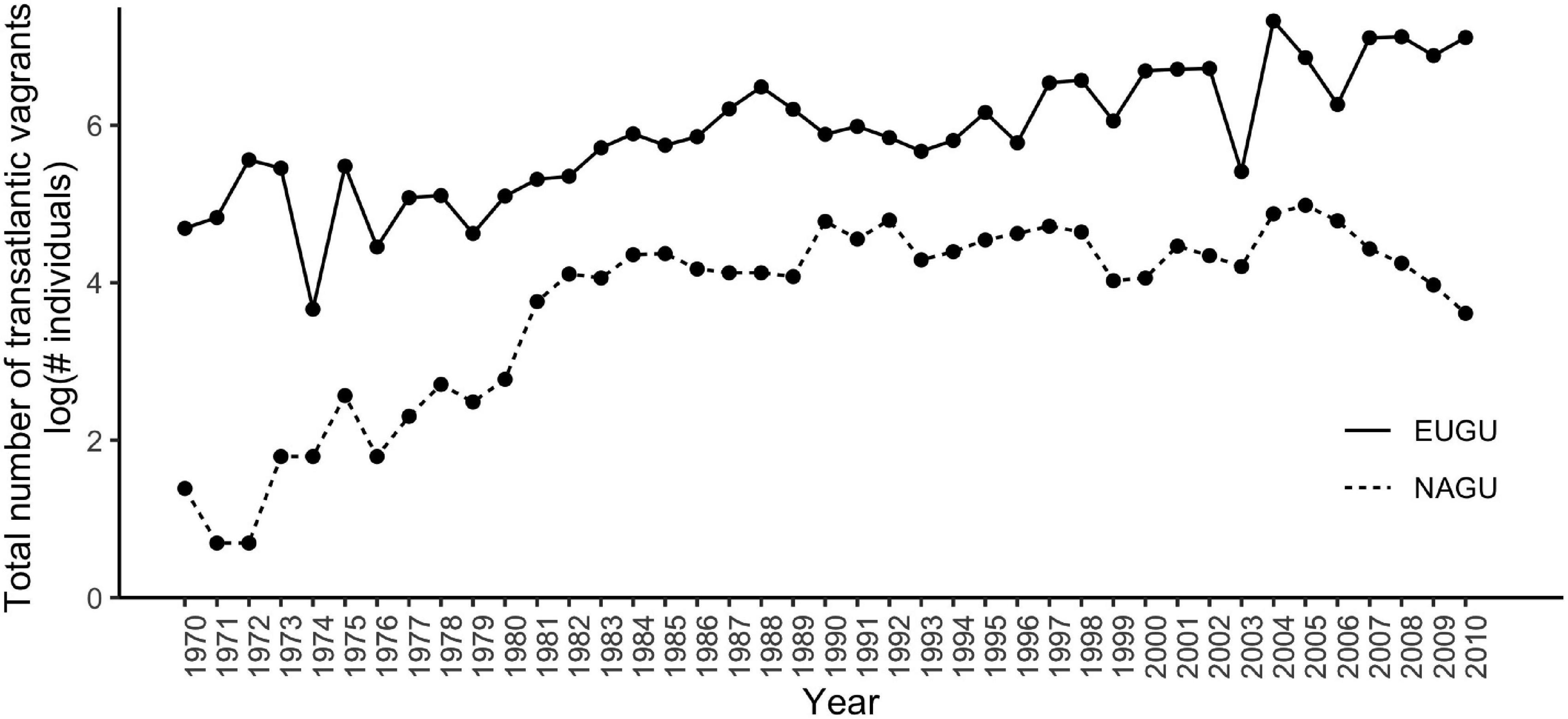
Figure 1. Annual total number of transatlantic vagrants from Europe to eastern North America (EUGU, solid line) and from North America to Europe (NAGU, dashed line) during 1970–2010. There was a strong positive correlation between the annual number of European and North American transatlantic vagrants (Spearman rank correlation rs = 0.63, p = 1.04 × 10–05).
Among the European species, Lesser Black-backed Gull had the highest number of vagrants reported for the study period, with a maximum per year of 1194 vagrants, while Common Gull had the lowest annual vagrant numbers, with never more than 24 vagrants in one year. Of the North American species, Ring-billed Gull had the highest number of vagrants throughout the study with maximum of 109 individuals sighted per year, while Franklin’s Gull demonstrated the lowest annual vagrant numbers with never more than 11 vagrants in one year.
Between 1970 and 2010, vagrancy increased significantly with time for all species except for Black-headed Gull and Ring-billed Gull (Figures 2, 3 right panels, Supplementary Table 7). For Black-headed Gull (Figure 2, top right), we did not detect a significant temporal trend in vagrancy. Interestingly, for Ring-billed Gull (Figure 3, bottom right), we found that transatlantic vagrant numbers significantly increased before 1990 (p < 0.001, Supplementary Table 7), while after this year, the number of birds reported in the European receiving areas significantly declined with time (p = 0.002, Supplementary Table 7).
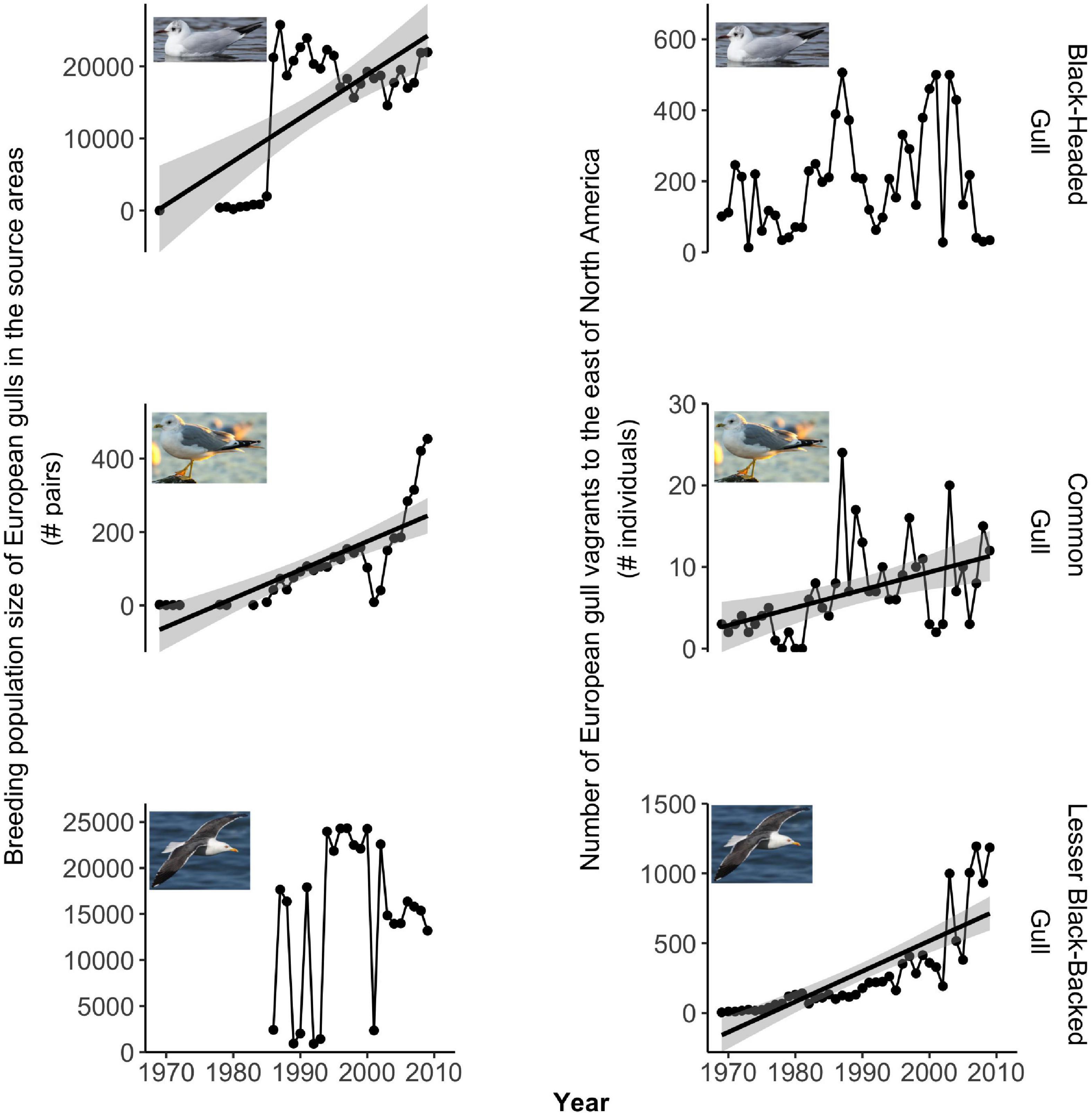
Figure 2. Temporal trends for the European gulls. Left column: Breeding population size (1969–2009). Right column: Number of vagrants reported in eastern North America (1970–2010). Note that the y-axes have different scales.
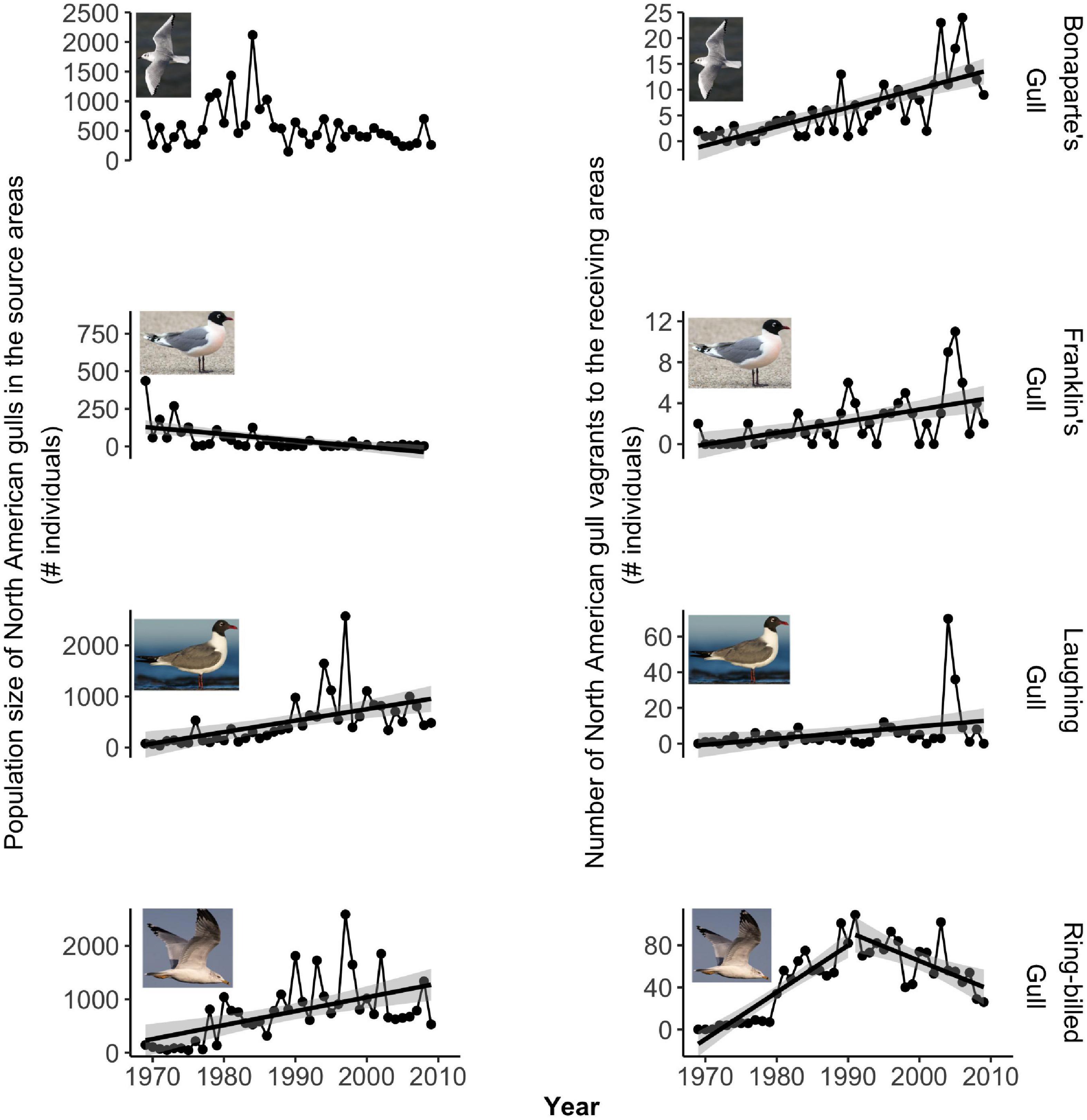
Figure 3. Temporal trends for the North American gulls. Left column: Population size (1969–2009). Right column: Number of vagrants reported in the European receiving areas (1970–2010). Note that the y-axes have different scales.
Population Size as Driver of Transatlantic Vagrancy
Population size increased significantly with time for Black-headed Gull, Common Gull, and Laughing Gull, while it significantly declined for Franklin’s Gull, and it did not significantly change for Lesser Black-backed Gull and Bonaparte’s Gull (Figures 2, 3 left panels, Supplementary Table 7). For Ring-billed Gull, population size significantly increased before 1990 and after this year it started to decline, although this negative trend was not statistically significant (Figure 3 bottom left and Supplementary Table 7).
Our GLMs predicted a statistically significant positive relationship between annual population size in the source areas and vagrancy to the receiving areas in the following year for Common Gull (Figure 4 and Table 1), Laughing Gull, and Ring-billed Gull (Figure 5 and Table 1). Thus, vagrancy tended to increase with increases in population size for these three species. In the case of Laughing Gull, the GLM including the predictor (full model) was statistically significant, however, its performance (as measured by the AICc) was not different from the null model (Table 1). For Bonaparte’s Gull the relationship between vagrancy and population size was significantly negative (Figure 5 and Table 1). Thus, higher numbers in population size were associated with lower numbers in transatlantic vagrancy.
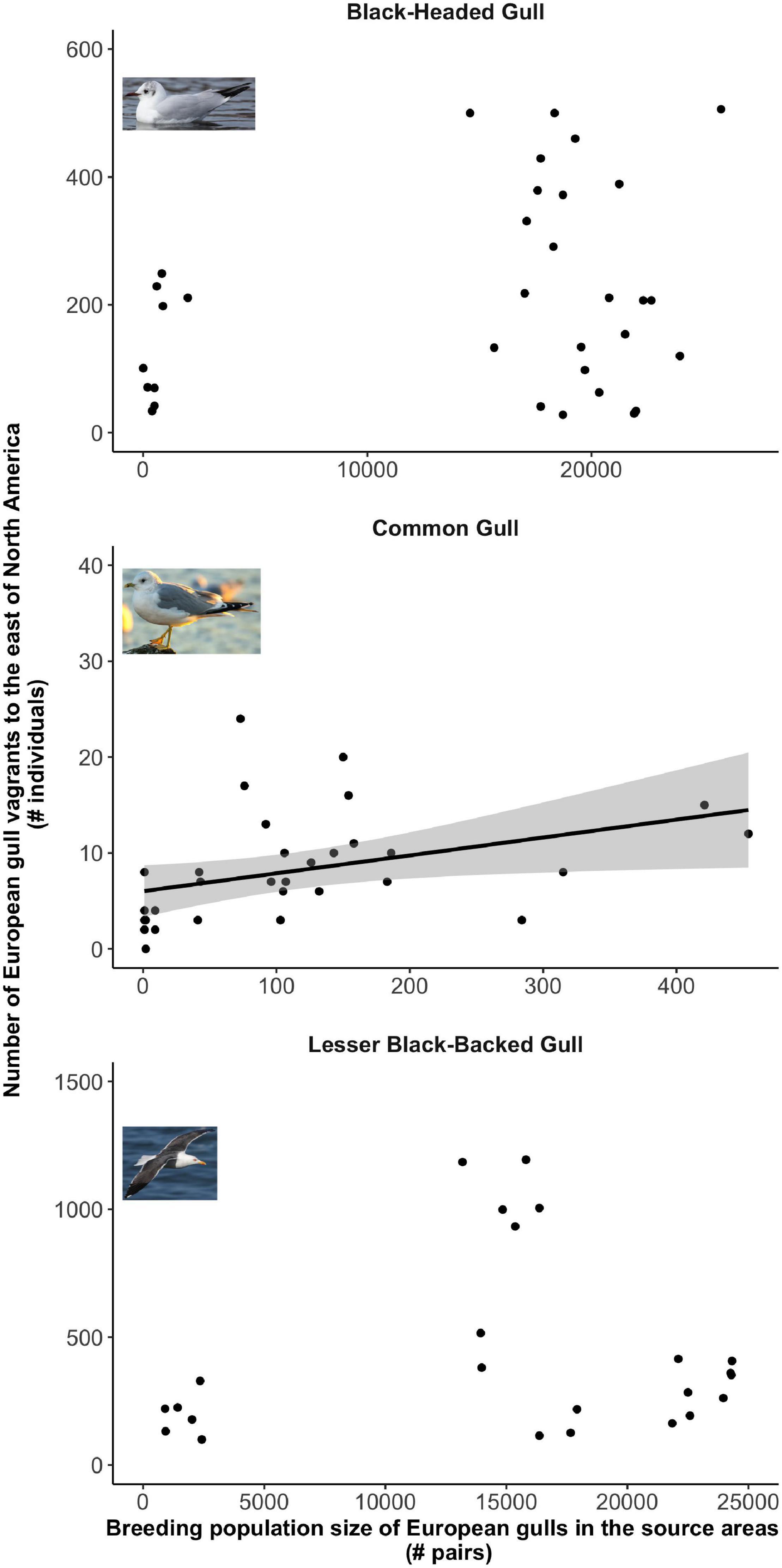
Figure 4. Relationship between the number of European gull vagrants reported in eastern North America in a given year and the size of their source populations in the previous year. Only Common Gull showed a statistically significant relationship between these two variables. Note that both axes have different scales.
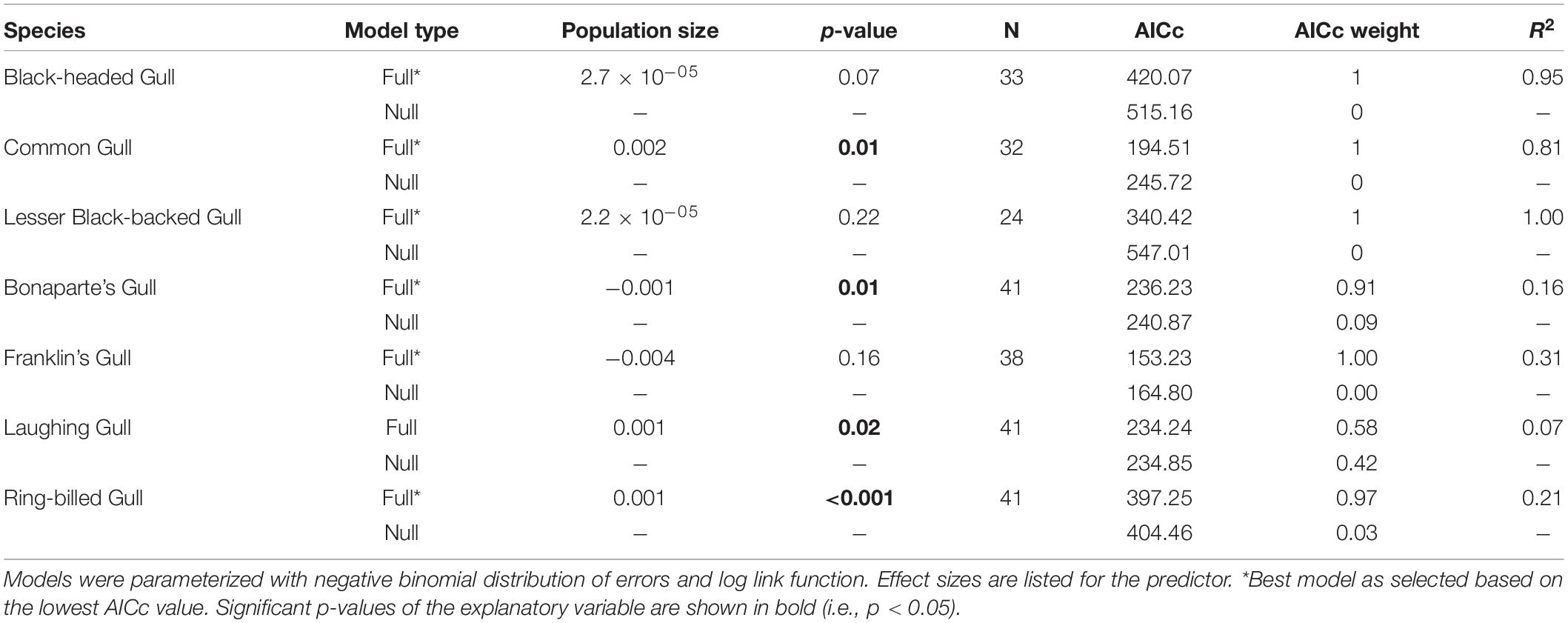
Table 1. Parameter estimates for Generalized Linear Models of the relationship between population size in the source area (explanatory variable) and the number of transatlantic vagrants in the receiving area in the following year (response variable).
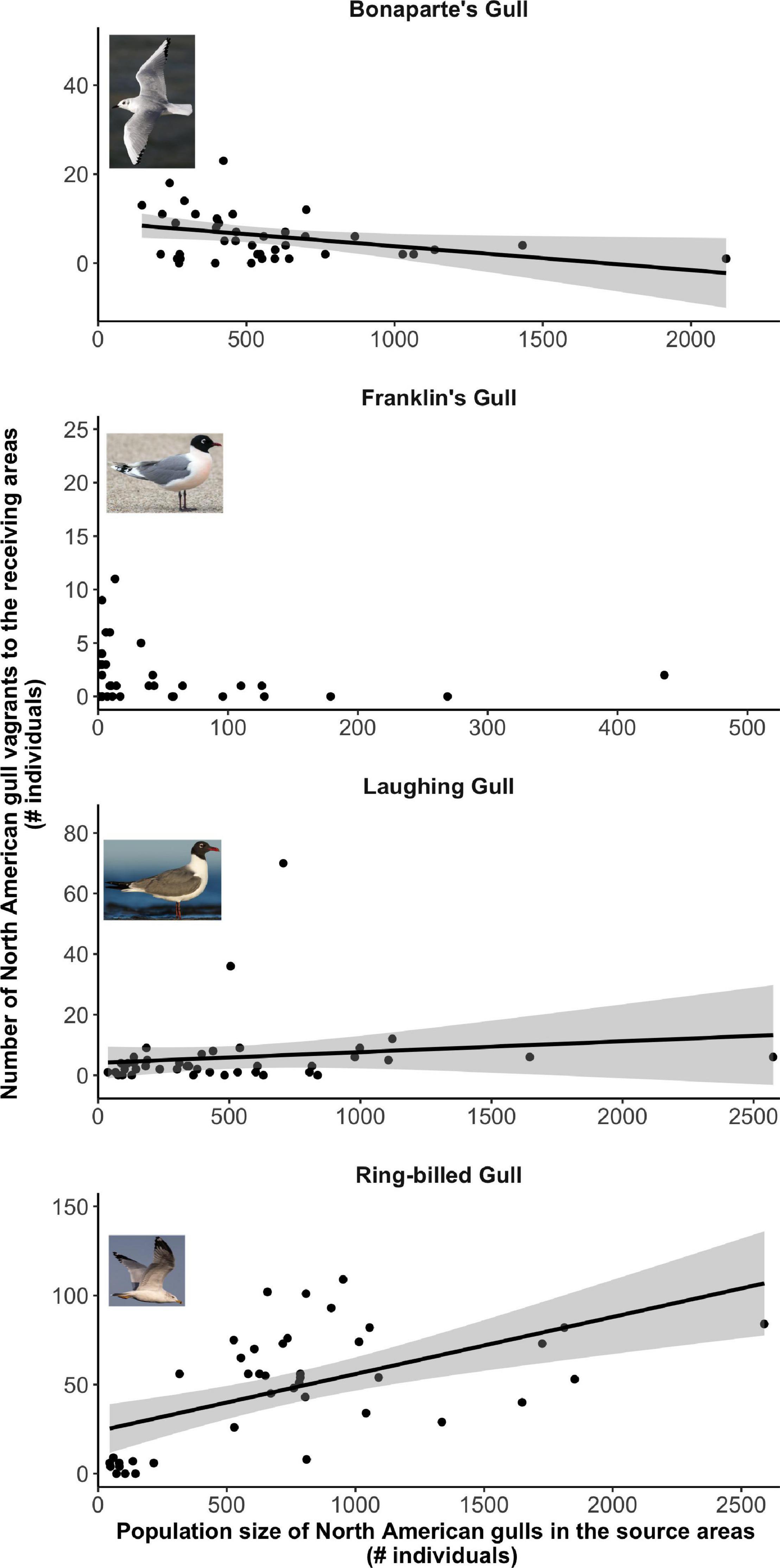
Figure 5. Relationship between the number of North American gull vagrants reported in the European receiving areas in a given year and the size of their population of origin in the previous year. Only Franklin’s Gull did not show a statistically significant relationship between these two variables. Note that both axes have different scales.
For Black-headed Gull, Lesser Black-baked Gull and Franklin’s Gull, our models did not predict a statistically significant relationship between population size and vagrancy (Figures 4, 5, Table 1). In the case of the temporal trend of Black-headed Gull’s population size, we detected an increase in the number of breeding pairs from 1,991 to 21,235 pairs (Figure 2). To further investigate the effect of these low sizes in our results, we repeated the models considering only data after 1,985, that showed breeding sizes above 21,235 pairs. We did not detect a statistically significant relationship between population size and vagrancy in this instance either.
Within Species Analysis: Ring-Billed Gull
In all regions except the East Central region, we detected a sharp change in the temporal trends of the subpopulations’ size after 1990 (Figure 6). Before 1990, the subpopulations in the West, West Central and East regions increased significantly with time, while after this year the number of gulls per BBS route significantly declined. However, for the West and West Central regions, we did not have enough non-zero distance band recovery records to justify analyses of two time periods. Therefore, for all regions except the East region we performed the analyses for the full study period (i.e., 1960–2017). For the East region we present our results for two periods: before and after 1990.
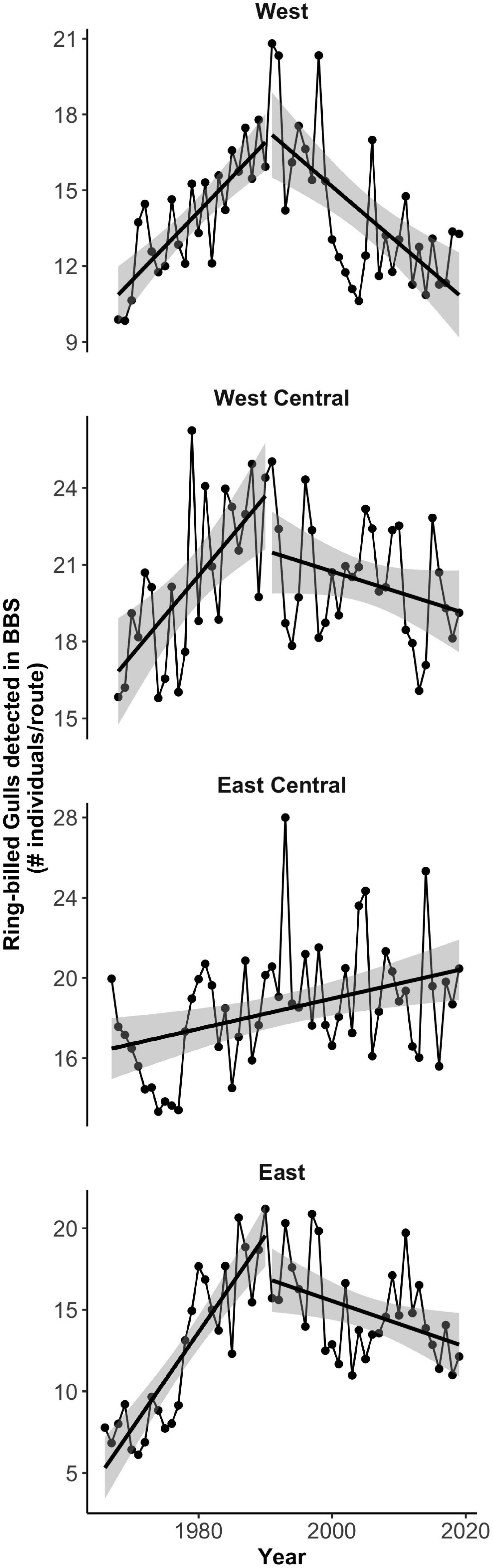
Figure 6. Ring-billed Gull breeding size in time for four subpopulations of North America (1960–2017). Population size increased in the West (top), West Central (top center), and East (bottom) regions prior to 1990 and decreased after 1990. In the East Central region, the population size increased throughout the whole study period (bottom center). Note that the y axes have different scales and do not start at zero.
The four subpopulations (regions) behaved differently with respect to if and how vagrancy related to population size (Table 2). For the West region, the GLM predicted a significantly negative relationship between number of recoveries and population size (p = 0.02, Figure 7 top and Table 2). For the two subpopulations in the center of North America, our models did not detect a statistically significant relationship between number of recoveries and population size (Table 2). For the East region, as described above, population size increased significantly prior to 1990 and significantly decreased after 1990 (Figure 6 bottom panel). However, these changes in population numbers prior to 1990 were not positively associated with our index of vagrancy (Table 2). Figure 7 (bottom left panel) shows that recaptures decreased with increasing population size (p < 0.001) during this earlier period, contrary to our expectation. After 1990, recaptures significantly increased with increasing population size (p = 0.004, Figure 7 bottom right panel and Table 2).
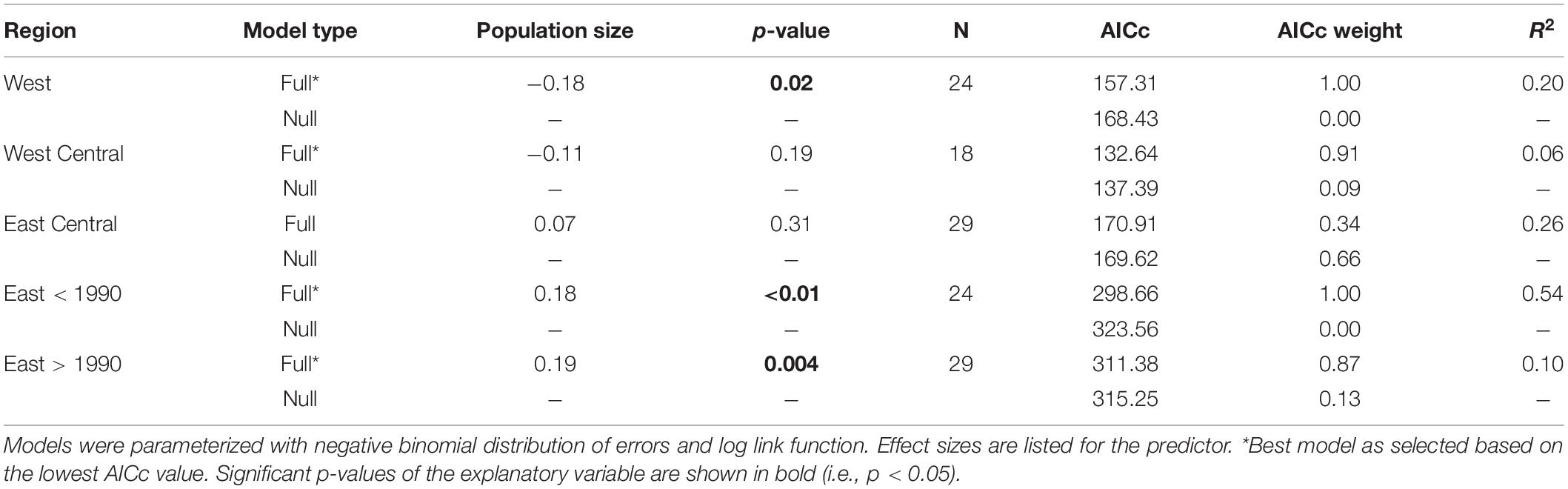
Table 2. Parameter estimates for Generalized Linear Models of the relationship between population size (explanatory variable) and the number of non-zero distance band recoveries (response variable) in four North American sub-populations of Ring-billed Gull.
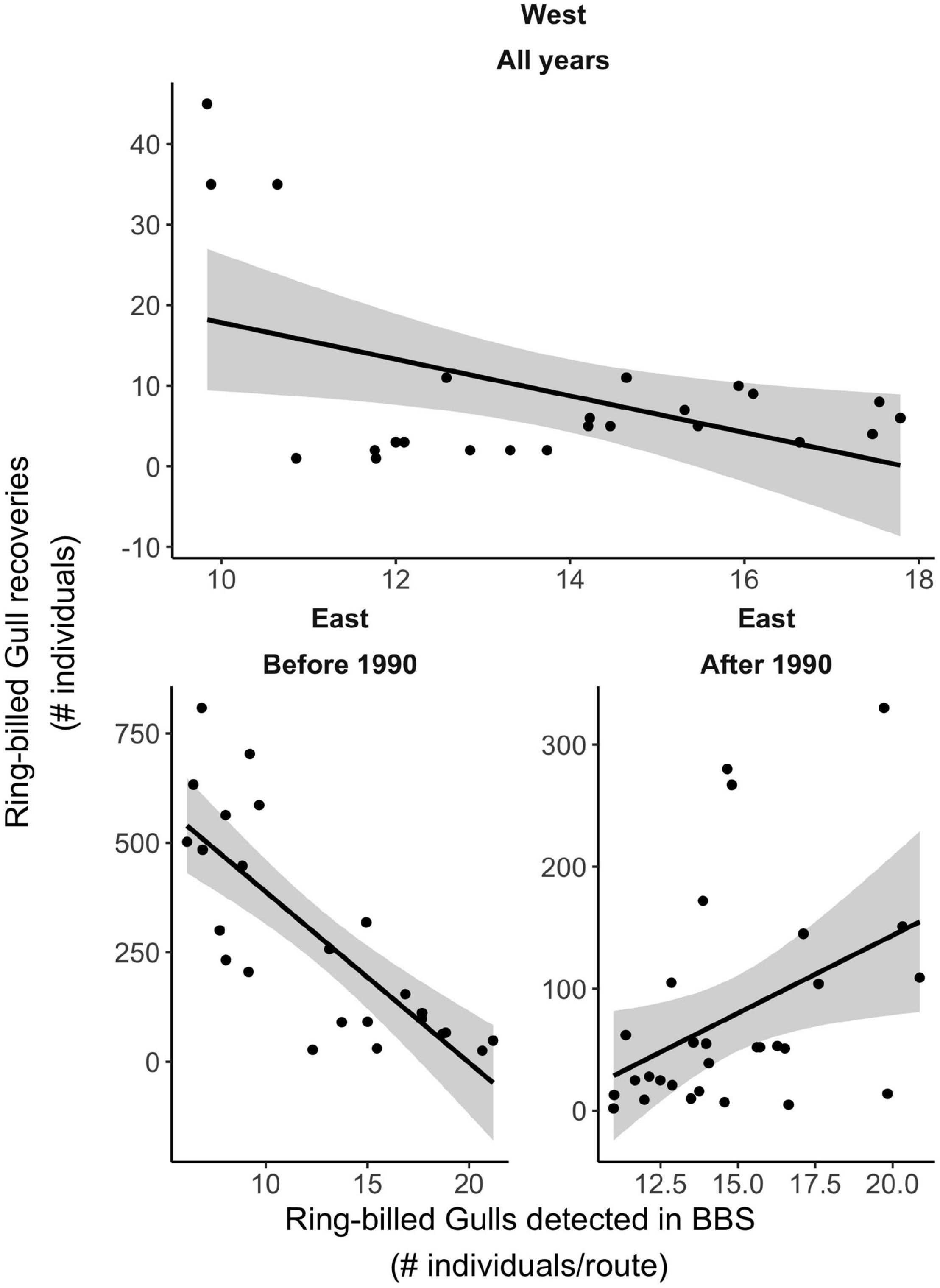
Figure 7. The association between Ring-billed Gull population size and band recoveries was significantly negative in the West region (top). Declines and increases in bird recoveries with increasing size (Eastern birds, pre- and post-1990, respectively) (bottom), no relationship between recoveries and size evident for East Central and West Central regions (not shown).
Discussion
In this analysis, we found that there was a strong positive correlation between the total number of transatlantic vagrants in both directions. According to our models, the population size in the source area predicted changes in transatlantic vagrancy for four of the seven species analyzed. However, in one of these four species, increases in population size were associated with decreases in the number of vagrants, contrary to our expectation. Consequently, population size in the source area as a driver of vagrancy to the receiving area may be related to the particular species analyzed, or the particular conditions a species is experiencing (i.e., food availability, nesting sites and intraspecific or interspecific competition). These results are supported by the within species analyses for Ring-billed Gull since different subpopulations showed different patterns of the relationship between population size and recaptured birds. Thus, the existence of a statistical relationship between population size and vagrancy inside the United States and Canada also depends on the particularities of each region and on the time period examined.
When using long-term survey data for analyses, as in our study, there is always the possibility of increases in observer effort biasing the results. For the BBSC, at each site, every individual or pair of a species is counted. For the BBS, at each route only one citizen scientist highly skilled in avian identification performs the count. Thus, for these two data sets, we minimized the probability of observer effort bias by estimating population size in the areas of origin using only sites that were sampled in all the 41 years of the study period (1969–2009). In the specific case of Bonaparte’s Gull, in which we used CBC data, we minimized the probability of observer effort bias by using only CBC circles that were sampled in all the 41 years of the study period (1969–2009) and by accounting for sampling effort at each circle (dividing population size estimates by the number of party hours). In the case of the vagrant’s temporal trends detected in our study, we consider that observer effort was unlikely to be strongly biasing the changes observed with time. For example, for the European gulls, while Common and Lesser Black-backed Gull were increasing vagrancy with time, Black-headed Gull did not show any significant trend. In the case of the American gulls, Bonaparte’s, Franklin’s, and Laughing Gull increased their vagrant number in time, while for Ring-billed Gull vagrancy increased before 1990 but significantly declined after this year. Observer effort cannot explain all these opposite trends at the same time. Additional evidence of opposing temporal trends in vagrancy exists for Asian warbler species recorded in Britain between 1990 and 2019 (ex., Phylloscopus inornatus, P. proregulus, Acrocephalus dumetorum, and Hippolais polyglotta, White and Kehoe, 2018, 2021), and for northern-breeding warblers and vireo species recorded in California, North America (see Patten and Marantz, 1996). These opposite trends found by us in gull species on both sides of the Atlantic, as well as the reports for Passerine species, do not support the idea that observer effort results in increasing vagrancy records.
Other possible biases of these data sets include limited access to data. Particularly for the Seabird Breeding censuses of Britain and Ireland, there were only 28 sites that met our inclusion criteria. This database contains valuable long-term information but is also limited because survey coverage has increased in more recent censuses and the intensity of monitoring has been higher in some locations and periods than others in Great Britain (White and Kehoe, 2018, 2021). Additionally, in the specific case of Ring-billed Gull, we were not able to include vagrant records from Ireland, which can have affected our results for this species. Data limitation also precluded us to conduct an intra-population analysis for Common Gull similar to the one conducted for Ring-billed Gull. Common Gull is an appealing candidate to conduct subregional analysis since its range includes most of Eurasia, however, we did not have access to breeding data for this species outside of the United Kingdom.
Below we treat each analysis in turn.
Transatlantic Vagrancy in Both Directions
We found that the annual total number of vagrants for both European and North American gull species was significantly increasing with time. Thus, the strong positive correlation found between the annual total numbers of European and North American vagrants was expected. In conjunction, these results showed that vagrancy across the Atlantic Ocean was increasing both eastwards toward Europe and westward toward North America. In addition, when analyzed on a per-species basis, the number of vagrant gulls significantly and consistently increased with time for all the species studied except for Black-headed Gull and for Ring-billed Gull after 1990. These results align with the idea of vagrancy being an adaptive response and are not consistent with the notion that vagrants are predominantly blown off course by extreme weather events. Wind-driven vagrancy should have had a pattern of peaks of higher than usual number of vagrants only in some years -the storm-occurring years- and not the consistent increase or decrease we observed on the species that have a significant temporal trend in vagrancy. Moreover, under the wind-driven hypothesis, prevailing winds in the north Atlantic, which have a west to east direction, may have promoted vagrancy increases from North America to Europe but not vice versa, which partially contradict our results, particularly for Ring-billed Gull, a North American species in which vagrancy started to significantly decline after 1990. It is important to note that we did not include a weather predictor in our analyses, therefore, we cannot categorically exclude this factor as a driver of vagrancy. However, the results presented above are difficult to interpret under the wind-driven hypothesis and point toward population size in the source area as a better explanation of the increases in transatlantic vagrancy. Additionally, gulls are generalist species that use different flight strategies and can rest on water and find food potentially everywhere (Klaassen et al., 2012), therefore, weather conditions may not play such an important role as a driver of transatlantic vagrancy in this group. Our findings are also difficult to explain under the reverse migration hypothesis which is based on a “defective bird” with a 180° shift in orientation from the typical migration direction of its species. For gulls, this idea is uninterpretable since virtually all species studied show a broad range of orientations, so it is not evident what a 180° shift means [i.e., 180° from what? see Newton (2008), Phillips (2000)].
Population Size as Driver of Transatlantic Vagrancy
The models of population size in the source areas as a predictor of vagrancy across the Atlantic showed multiple relationships between vagrancy and population size. For four of the seven gulls studied, our models predicted a statistically significant relationship between these variables.
However, our hypothesis of a positive association between increasing bird population size and vagrancy was supported only for two species: Common Gull and Ring-billed Gull. The results for these species are consistent with the propensity of immature birds from burgeoning populations more frequently dispersing farther distances and exploring potential new breeding sites. Particularly gulls take 3–6 years to reach reproductive maturity, so they might undergo explorative behavior over this entire period (Olsen and Larsson, 2004). This extended period of immaturity in these long-lived birds might in part account for the direct correspondence between the number of birds born last summer and vagrancy the following year. Significant correlations between vagrancy and population size have been also reported for several landbird species (Veit, 1997, 2000; Zawadzki et al., 2019). For example, Zawadzki et al. (2019) found that the effect of population size on the number of Ash-throated Flycatcher vagrants on the East Coast of North America had a larger effect on vagrancy than measures of reproductive success or prevailing winds. In general, increasing populations not only increase the number of young individuals that are potential vagrants but also increase selective pressures (i.e., competition, inbreeding) that can in turn favor vagrancy as an adaptive strategy. Thus, vagrancy may have an evolutionary value since, through exploration of new habitats, it confers to birds the ability to expand their distributional ranges, to escape conspecific competition over limited resources and to adapt to anthropogenic climate change by avoiding deteriorating conditions in the source population areas (Ronce, 2007).
In the case of Laughing Gull, although both population size and vagrancy were increasing in time and the GLM showed a significantly positive relationship between them, this GLM was no different from the null model. Thus, we consider that our hypothesis was not supported in this instance. In fact, the positive temporal trend in the number of vagrants was driven by two outlier years in which an unusually high number of vagrants were reported. Once these outliers were removed, vagrancy did not statistically change with time (p = 0.06).
For Bonaparte’s Gull, although our models showed a statistically significant relationship between vagrancy and population size, our hypothesis was not supported since higher population size in the source areas was associated with lower transatlantic vagrant numbers. Bonaparte’s Gull population size showed a decline in time (although not statistically significant, p = 0.11). These results may reflect the susceptibility of these gulls to human disturbance and to the loss of nesting sites in prairie marshes (Burger and Gochfeld, 2020). Therefore, even if their reproductive output is sufficient to maintain populations (Southern, 1980) it might not be enough to drive transatlantic vagrancy.
For Franklin’s Gull, Black-headed Gull, and Lesser Black-backed Gull we did not find statistical support for our hypothesis. This might be a result of the particularities of the temporal trends in either the population size or the number of transatlantic vagrants. For example, in Franklin’s Gull population size was low throughout the study period (maximum size below 450 individuals) and showed a statistically significant decline with time. Thus, these levels of population size might not be high enough to support vagrancy. For Black-headed Gull, neither the population size after 1985 nor the temporal trend in the number of transatlantic vagrants had significantly changed, thus, local factors such as food availability, predation or competition may be influencing vagrancy in this species. In the case of Lesser Black-backed Gull, although population size in the source areas did not significantly change with time, vagrants to eastern North America consistently increased their numbers across the study period. This species is known for its colonization of Greenland during the twentieth century, and the range expansion to this novel habitat has been attributed to vagrancy events promoted by the growth of source populations from Iceland and northwest Europe (Boertmann, 2009). Thus, we propose that the increasing influx of Lesser Black-backed Gull’ vagrants to East North America may have come from the closer colonies in Greenland instead of from Europe (Zawadzki et al., 2021). Our lab is currently investigating this latter hypothesis with Icarus satellite trackers deployed on Lesser Black-backed Gull, in order to understand where they typically travel.
The differences that we found in the statistical significance of our models indicate variability in species-specific responses and the changes in local conditions with time. These results suggest that increases in population size may be necessary but not sufficient to drive transatlantic vagrancy. Thus, for these species, further research exploring factors not accounted for in this study may shed light on other drivers of vagrancy.
Within Species Analysis: Ring-Billed Gull
Variability in vagrancy as a response to changes in the size of the source population was not only found among species but also among populations of a single species at the local level. While for the East Central and West Central breeding colonies of Ring-billed Gull increases in the size of the source population were not related to changes in the number of recaptures (and thus possible vagrancy events), for West populations increasing population sizes were generally related to decreasing numbers of recaptures. On the other hand, Eastern populations changed their relationship between population sizes and the number of recaptures with time. Before 1990, increases in population sizes were related to declines in the number of recaptures. We propose two possible explanations for these findings. First, suitable environmental conditions may have existed before 1990. In this period there was high food availability linked to the existence of landfills and the existence of protection laws (Grandgeorge et al., 2008). Thus, these factors may have promoted not only increases in the population size but also likely reduced movement of young birds which might, in turn, result in lower numbers of recaptured gulls. Second, this result may be an indication that before 1990 the young gulls were traveling farther, contributing to the number of transatlantic vagrants, and decreasing the number of recaptures inside the United States and Canada, since we found that before 1990 the number of Ring-billed Gull transatlantic vagrants significantly increased. After 1990, Eastern population size and number of recaptures significantly decreased, and there was a significant positive relationship between these variables. Consistent with these trends, the number of transatlantic vagrants declined significantly with time after 1990. In this case, the general decline in vagrancy and in the number of recaptured birds was likely driven by declines in the source population size.
Conclusion
The larger interpretation of what we have shown here is that vagrancy in several gull species increased on both sides of the Atlantic, a result not easily explained by reverse orientation or wind-drift. Our results showed that size or trend in source population size is clearly a partial driver of vagrancy, but for some species other factors may affect transatlantic vagrancy. These results were mirrored at the species level, since subpopulations of the same species showed a wide variety of relationships between vagrancy and population size. Thus, vagrancy in a single species can be driven by increasing population sizes, but increasing population may be necessary, but not sufficient to drive increasing vagrancy. Other factors must be necessary as well, reflecting local conditions and particular species tolerances. As anthropogenic development continues, and high-quality habitats become farther apart, it is relevant that we continue to investigate drivers of vagrancy because the persistence of a species may depend crucially on its longest-distance dispersers.
Data Availability Statement
The data analyzed in this study is subject to the following licenses/restrictions: some of the data was provided to us from Rare Birds Committees, and we do not have permission to make it publicly available. Requests to access these datasets should be directed to MAA, macostaalamo@gradcenter.cuny.edu. Data from the Joint Nature Conservation Committee, the North American Breeding Bird Survey, and the North American Christmas Bird Count are publicly available from their webpages.
Ethics Statement
Ethical review and approval was not required for the animal study because we used previously collected data (which did not require animal contact to collect).
Author Contributions
LM conceived of the idea. MAA, LM, and RV carried out analyses, wrote, and edited the manuscript. All authors contributed to the article and approved the submitted version.
Funding
We would like to thank PSC-CUNY for funding of MAA (grant #62794-00 50).
Conflict of Interest
The authors declare that the research was conducted in the absence of any commercial or financial relationships that could be construed as a potential conflict of interest.
Publisher’s Note
All claims expressed in this article are solely those of the authors and do not necessarily represent those of their affiliated organizations, or those of the publisher, the editors and the reviewers. Any product that may be evaluated in this article, or claim that may be made by its manufacturer, is not guaranteed or endorsed by the publisher.
Acknowledgments
We thank the North American Breeding Bird Survey, Christmas Bird Count and National Audubon Society, Joint Nature Conservation Committee, British Birds Rarities Committee, Danny Bystrak of Bird Banding Lab, Colum Flynn of Irish Rare Bird Committee, Brian Burke, Niall Keogh and Josh Jones for additional Irish records, Yann Kolbeinsson of Icelandic Rarities Committee, Peter Alfrey, Director of Little Oak; and all the volunteers for all these citizen science surveys that make this large-scale analysis possible. Special thank you to Blair Dudeck, Darren Clark, Joseph Bailey, Dorian Anderson, US Geological Survey (Robert Dusek), and Thorfinnur Sigurgeirsson for granting us permission to use their gull pictures.
Supplementary Material
The Supplementary Material for this article can be found online at: https://www.frontiersin.org/articles/10.3389/fevo.2022.850577/full#supplementary-material
References
Alfrey, P., David, M., and Vincent, L. (2018). Nearctic Vagrants on Corvo, Azores, in 2005-17. Dutch Bird. 40, 297–317.
American Birding Association and National Audubon Society (2019). North American Birds. Colorado: Colorado Springs. 1999–2020.
Baert, J. M., Stienen, E. W. M., Heylen, B. C., Kavelaars, M. M., Buijs, R. J., Shamoun-Baranes, J., et al. (2018). High-Resolution GPS Tracking Reveals Sex Differences in Migratory Behaviour and Stopover Habitat Use in the Lesser Black-Backed Gull Larus Fuscus. Nat. Sci. Rep. 8, 1–11. doi: 10.1038/s41598-018-23605-x
Baird, J., Robbins, C. S., Bagg, A. M., and Dennis, J. V. (1958). ’Operation Recovery’–the Atlantic Coastal Netting Project. Bird Band. 29, 137–168. doi: 10.2307/4510699
Baker, R. R. (1978). Evolutionary Ecology of Animal Migration. New York, NY: Holmes and Meier Publishers.
Baker, R. R. (1980). The Significance of the Lesser Black-Backed Gull to Models of Bird Migration. Bird Study 27, 41–50. doi: 10.1080/00063658009476655
Belant, J. L., and Dolbeer, R. A. (1993). Migration and Dispersal of Laughing Gulls in the United States. J. Field Ornithol. 64, 557–565.
Bledsoe, A. H., and Sibley, D. (1985). A Bird Better Knownas a Vagrantthan on Its Normal Winter Range AnthonyH. Bledsoeand David Sibley. Am. Birds 39, 219–228.
Bloom, P. H., Scott, J. M., Papp, J. M., Thomas, S. E., and Kidd, J. W. (2011). Vagrant Western Red-Shouldered Hawks: Origins, Natal Dispersal Patterns, and Survival. Condor 113, 538–546. doi: 10.1525/cond.2011.100052
Boertmann, D. (2009). The Lesser Black-Backed Gull, Larus Fuscus, in Greenland. Arctic 61, 129–133. doi: 10.14430/arctic17
British Birds Rarities Committee (2019). Available online at: https://www.bbrc.org.uk/main-information/statistics (accessed November 2, 2019).
Burger, J., and Gochfeld, M. (2020). “Lesser Black-Backed Gull (Larus Fuscus), Version 1.0” in Birds of the World, ed. A. F. Poole (USA: Cornell Lab of Ornithology). 2020. doi: 10.2173/bow.fragul.01
Burnham, K. P., and Anderson, D. R. (2002). Model Selection and Multimodel Inference: a Practical Information-Theoretic Approach. 2nd Edn. New York, NY: Springer.
Bustnes, J. O., Moe, B., and Phillips, R. A. (2013). Rapid Long-Distance Migration in Norwegian Lesser Black-Backed Gulls Larus Fuscus Fuscus along Their Eastern FLyway. IBIS 155, 402–406. doi: 10.1111/ibi.12022
Camphuysen, C. J. (2013). A Historical Ecology of Two Closely Related Gull Species (Laridae): Multiple Adaptations to a Man-made Environment. Groningen: University of Groningen.
Davis, R. A., and Watson, D. M. (2018). Vagrants as Vanguards of Range Shifts in a Dynamic World. Biol. Conserv. 224, 238–241. doi: 10.1016/j.biocon.2018.06.006
DeBenedictis, P. (1971). Wood Warblers and Vireos in California: The Nature of the Accidental. California Birds 2, 111–128.
DeSante, D. F. (1983). Annual Variability in the Abundance of Migrant Landbirds on Southeast Farallon Island, California. Auk 100, 826–852. doi: 10.1093/auk/100.4.826
DeSante, D. F., and George, T. L. (1994). Population Trends in the Landbirds of Western North America. Stud. Avian Biol. 15, 173–190.
Fraser, P. A., and Rogers, M. J. (2002). Report on Scarce Migrant Birds in Britain in 2002. 16. British Birds
Giroux, J. F., Patenaude-Monette, M., Lagarde, F., Thiériot, E., Brousseau, P., and Molina, P. (2016). The Rise and Fall of Ring-Billed Gulls (Larus Delawarensis) in Eastern North America. Waterbirds 39, 87–98. doi: 10.1675/063.039.sp101
Grandgeorge, M., Wanless, S., Dunn, T. E., Maumy, M., Beaugrand, G., and Grémillet, D. (2008). Resilience of the British and Irish Seabird Community in the Twentieth Century. Aquat. Biol. 4, 187–199. doi: 10.3354/ab00095
Helberg, M., Systad, G. H., Birkeland, I., Lorentzen, N. H., and Bustnes, J. O. (2009). Migration Patterns of Adult and Juvenile Lesser Black-Backed Gulls Larus Fuscus from Northern Norway. Ardea 97, 281–286. doi: 10.5253/078.097.0303
Howell, S. N. G., Lewington, I., and Russell, W. C. (2014). Rare Birds of North America. Princeton: Princeton University Press.
Icelandic Institute of Natural History and Icelandic Rarities Committee [IINHIRC] (2020). Bliki: journal on Icelandic Birdlife. Available online at: https://en.ni.is/resources/publications/bliki-journal-icelandic-birdlife (accessed April 25, 2020).
Irish Rare Birds Committee [IRBC] (2020). Irish Rare Birds Committee. 2020. Available online at: http://www.irbc.ie (accessed December 15, 2020).
Jiguet, F., and Barbet-Massin, M. (2013). Climate Change and Rates of Vagrancy of Siberian Bird Species to Europe. Edited by R. Norris. Ibis 155, 194–198. doi: 10.1111/ibi.12001
Joint Nature Conservation Committee [JNCC] (2019). Available onlijne at: https://webarchive.nationalarchives.gov.uk/20190301135521/http://jncc.defra.gov.uk/page-4460-theme=default (accessed January 21, 2020).
Kadlec, J. A., and Drury, W. H. (1968). Structure of the New England Herring Gull Population. Ecology 49, 644–676. doi: 10.2307/1935530
Kilpi, M., and Saurola, P. (1984). Migration and Wintering Strategies of Juvenile and Adult Larus Marinus, L. Argentatus and L. Fuscus from Finland. Ornis Fenn. 61, 1–8.
Klaassen, R. H. G., Ens, B. J., Judy, S. B., Klaus-Michael, E., and Franz, B. (2012). Migration Strategy of a Flight Generalist, the Lesser Black-Backed Gull Larus Fuscus. Behav. Ecol. 23, 58–68. doi: 10.1093/beheco/arr150
Ławicki, Ł, and Perlman, Y. (2017). Black-Winged Kite in the WP: Increase in Breeding Population, Vagrancy and Range. Dutch Bird. 39:13.
Lees, A. C., and Gilroy, J. J. (2014). Vagrancy Fails to Predict Colonization of Oceanic Islands: Avian Island Vagrancy. Global Ecol. Biogeogr. 23, 405–413. doi: 10.1111/geb.12129
Lowe, W. H. (2009). What Drives Long-Distance Dispersal? A Test of Theoretical Predictions. Ecology 90, 1456–1462. doi: 10.1890/08-1903.1
Marques, P. A. M., Sowter, D., and Jorge, P. E. (2010). Gulls Can Change Their Migratory Behavior during Lifetime. Oikos 119, 946–951. doi: 10.1111/j.1600-0706.2009.18192.x
Mazerolle, M. J. (2020). AICcmodavg: Model Selection and Multimodel Inference Based On (Q)AIC(c). R Package Version 2.3-1. Available online at: https://CRAN.R-project.org/package=AICcmodavg (accessed February 5, 2022).
Mclaren, I. A., Lees, A. C., Field, C., and Collins, K. J. (2006). Origins and Characteristics of Nearctic Landbirds in Britain and Ireland in Autumn: A Statistical Analysis: A Statistical Analysis of Nearctic Landbirds in Britain and Ireland. IBIS 148, 707–726. doi: 10.1111/j.1474-919X.2006.00574.x
Morris, R. D., Weseloh, D. V., Wires, L. R., Pekarik, C., Cuthbert, F. J., and Moore, D. J. (2011). Population Trends of Ring-Billed Gulls Breeding on the North American Great Lakes, 1976 to 2009. Waterbirds 34, 202–212. doi: 10.1675/063.034.0209
National Audubon Society. (2019). The Christmas Bird Count Historical Results. 1960–2015. Manhattan: National Audubon Society.
Nisbet, I. C. T., Veit, R. R., Auer, S. A., and White, T. P. (2013). The Marine Birds of the Eastern United States and the Bay of Fundy: Distributions, Numbers, Trends, Threats, and Management. Cambridge: Nuttall Ornithological Club.
Olsen, K. M. (2018). Gulls of the World: A Photographic Guide. Princeton: Princeton University Press.
Olsen, K. M., and Larsson, H. (2004). Gulls of North America, Europe and Asia. Princeton: Princeton University Press.
Pardieck, K. L., Ziolkowski, D. J.Jr., Lutmerding, M., Aponte, V. I., and Hudson, M. A. R. (2020). North American Breeding Bird Survey Dataset 1966 - 2019: U.S. Geological Survey Data Release. United States: U.S. Geological Survey. doi: 10.5066/P9J6QUF6
Patten, M. A., and Marantz, C. A. (1996). Implications of Vagrant Southeastern Vireos and Warblers in California. Auk 113, 911–923. doi: 10.2307/4088868
Pedersen, K. T., Fritze, E. B., and Kharitonov, S. P. (2000). Migration Patterns of Common Gulls Larus Canus Ringed in the Non-breeding Season in Copenhagen and the Surrounding Area. Ring. Migrat. 20, 97–106. doi: 10.1080/03078698.2000.9674230
Pfeifer, R., Stadler, J., and Brandl, R. (2007). Birds from the Far East in Central Europe: A Test of the Reverse Migration Hypothesis. J. Ornithol. 148, 379–385. doi: 10.1007/s10336-007-0140-6
Phillips, J. (2000). Autumn Vagrancy: ‘Reverse Migration’ and Migratory Orientation. Ring. Migrat. 20, 35–38. doi: 10.1080/03078698.2000.9674226
R Core Team. (2020). R: a Language and Environment for Statistical Computing. Vienna: R Foundation for Statistical Computing.
Rabøl, J. (1969). Reversed Migration as the Cause of Westward Vagrancy by Four Phylloscopus Warblers. Br. Birds 62, 89–92.
Ronce, O. (2007). How Does It Feel to Be Like a Rolling Stone? Ten Questions About Dispersal Evolution. Annu. Rev. Ecol. Evolut. Syst. 38, 231–253. doi: 10.1146/annurev.ecolsys.38.091206.095611
Southern, W. E. (1980). “Comparative Distribution and Orientation of North American Gulls” in Behavior of Marine Animals: Current Perspectives in Research. Marine Birds, eds J. Burger, B. L. Olla, and H. E. Winn (Boston: Springer), 449–498. doi: 10.1007/978-1-4684-2988-6_11
Stenhouse, I. J., Egevang, C., and Phillips, R. A. (2012). Trans-Equatorial Migration, Staging Sites and Wintering Area of Sabine’s Gulls Larus Sabini in the Atlantic Ocean: Sabine’s Gull Migration. IBIS 154, 42–51. doi: 10.1111/j.1474-919X.2011.01180.x
White, S., and Kehoe, C. (2018). Report on Scarce Migrant Birds in Britain in 2016. Part 2, Passerines. Br. Birds 111, 519–542.
White, S., and Kehoe, C. (2021). Report on Scarce Migrant Birds in Britain in 2019. Br. Birds 114, 443–464.
Thorup, K. (2004). Reverse Migration as a Cause of Vagrancy. Bird Study 51, 228–238. doi: 10.1080/00063650409461358
USGS Bird Banding Laboratory (2019). 1966-2019. North American Bird Banding and Band Encounter Data Set. Laurel: Patuxent Wildlife Research Center.
Veit, R. R. (1997). Long-Distance Dispersal and Population Growth of the Yellow-Headed Blackbird Xanthocephalus Xanthocephalus. Ardea 85, 135–143.
Veit, R. R. (2000). Vagrants as the Expanding Fringe of a Growing Population. Auk 117, 242–246. doi: 10.1093/auk/117.1.242
Veit, R. R., and Lewis, M. A. (1996). Dispersal, Population Growth, and the Allee Effect: Dynamics of the House Finch Invasion of Eastern North America. Am. Nat. 148, 255–274. doi: 10.1086/285924
Veit, R. R., and Manne, L. L. (2015). Climate and Changing Winter Distribution of Alcids in the Northwest Atlantic. Front. Ecol. Evol. 3:38. doi: 10.3389/fevo.2015.00038
Veit, R. R., Velarde, E., Horn, M. H., and Manne, L. L. (2021). Population Growth and Long-Distance Vagrancy Leads to Colonization of Europe by Elegant Terns Thalasseus Elegans. Front. Ecol. Evol. 9:13. doi: 10.3389/fevo.2021.725614
White, S., and Kehoe, C. (2017). Report on Scarce Migrant Birds in Britain in 2015. Br. Birds 110, 493–552.
Zawadzki, L. C., Hallgrimsson, G. T., Veit, R. R., Rasmussen, L. M., Boertmann, D., Gillies, N., et al. (2021). Predicting Source Populations of Vagrants Using Breeding Population Data: A Case Study of the Lesser Black-Backed Gull (Larus Fuscus). Front. Ecol. Evol. 9:11.
Keywords: seabirds, gulls, vagrancy, population size, transatlantic
Citation: Acosta Alamo M, Manne LL and Veit RR (2022) Does Population Size Drive Changes in Transatlantic Vagrancy for Gulls? A Study of Seven North Atlantic Species. Front. Ecol. Evol. 10:850577. doi: 10.3389/fevo.2022.850577
Received: 07 January 2022; Accepted: 09 March 2022;
Published: 25 April 2022.
Edited by:
Brett K. Sandercock, Norwegian Institute for Nature Research (NINA), NorwayReviewed by:
Edward James Raynor, Agricultural Research Service (USDA), United StatesFloyd Hayes, Pacific Union College, United States
Copyright © 2022 Acosta Alamo, Manne and Veit. This is an open-access article distributed under the terms of the Creative Commons Attribution License (CC BY). The use, distribution or reproduction in other forums is permitted, provided the original author(s) and the copyright owner(s) are credited and that the original publication in this journal is cited, in accordance with accepted academic practice. No use, distribution or reproduction is permitted which does not comply with these terms.
*Correspondence: Marlen Acosta Alamo, macostaalamo@gradcenter.cuny.edu