- 1ARC Centre of Excellence for Coral Reef Studies and School of Biological Sciences, The University of Queensland, St. Lucia, QLD, Australia
- 2Centre for Ecology and Conservation, College of Life and Environmental Sciences, University of Exeter, Penryn, United Kingdom
- 3Department of Fish and Wildlife Conservation, Virginia Polytechnic Institute and State University, Blacksburg, VA, United States
The effects of fishing on the demography and population ecology of sex-changing fishes are largely unknown, despite the fact that their fisheries provide important economic and nutritional resources in coastal communities throughout the tropics, especially in Latin America. Species with female-first sex change often have naturally skewed sex ratios in the adult population, and fishing pressure can alter this natural bias, limiting egg production and fertilization success. How fishing alters demography and population vital rates depends on which sizes and sexes are selected. We consider two types of fishery selectivity “asymptotic (selecting the largest fish) and plate-sized (selecting fish between in a narrow, but small, size range)” that to represent fisheries for two important fish species of the Tropical Eastern Pacific, the Pacific goliath grouper (Epinephelus quinquefasciatus) and the endemic sailfin grouper (Mycteroperca olfax) of the Galapagos Islands, known locally as bacalao. Each of these large, long-lived species support small scale fisheries of significant value, but there is limited information on fishing effort, selectivity, or population trends. Using a population model, we estimate how the biology of these species contributes to their risk of overexploitation under different possible scenarios of fishing and reproductive biology. Specifically, we consider how variation in growth rates and fertilization rates interact with selectivity to affect age structure and sex ratios. We compare two metrics of population status: the spawning potential ratio (SPR), and the relative standing biomass after fishing (BF/B0). In our modeled populations, when fertilization rates were reduced, fishing rapidly decreased the spawning potential of both species, but did not affect biomass at moderate levels of fishing mortality. However, we predict low fertilization success, fast somatic growth, and asymptotic selectivity of fisheries for sex-changing species decreases both spawning potential and biomass, even at low levels of exploitation, suggesting these factors can cause rapid depletion of sex-changing species. Our findings highlight key gaps in our knowledge of spawning behavior and fertilization success of sex-changing fishes that must be filled if we are to sustainably manage these culturally and economically significant fisheries.
1. Introduction
Across Latin America more than 2 million people rely on fishing activities as their primary source of income and nutrition (FAO, 2000; Salas et al., 2007). Small-scale fisheries contribute 74% of total catches in the region, a greater contribution than other regions, such as Asia and Oceania (70%) or Europe (24%) (Chuenpagdee et al., 2019). Small-scale fishers often operate a variety of fishing gears, and select a range of sizes, depending on the species targeted and ecosystems fished (de Oliveira Leis et al., 2019). These complex fisheries characteristics, in combination with high uncertainty about total catch and fishing effort, make evaluations of the cumulative impact of fishing on many exploited fish species extremely challenging (Chuenpagdee et al., 2011).
Groupers (Epinephelidae) are among the most commercially valuable group of demersal fishes sought by small-scale fisheries in Latin America (e.g. Begossi et al., 2019; Castellanos-Galindo and Zapata Padilla, 2019). These species are large-bodied predators, which often fetch high market prices due to the high quality of their flesh (Sadovy de Mitcheson et al., 2013). Groupers are also at high risk of overfishing due to their particular life history characteristics (Sadovy de Mitcheson et al., 2020): they are long-lived species (Bullock et al., 1992) that form predictable spawning aggregations (Sala et al., 2003), and have high site fidelity (Koenig et al., 2011). In addition, many species of groupers are protogynous (female-first) hermaphrodites (Sadovy and Colin, 1995). Fishing pressure on sex-changing species with female-first life histories can alter population sex ratios, decrease fertilization rates, and hinder population growth (Alonzo et al., 2008; Kindsvater et al., 2017). For species that aggregate to spawn their eggs into the water column, a few large males can fertilize the eggs of many females, and female-biased sex ratios are common. However, little is known about the effects of fishing on this mating system and the potential consequences for fishery sustainability. This is especially true for tropical species that are targeted in small-scale fisheries that select for fish of different sizes, depending on the local fishing practices and seasonal fluctuations in demand. Concerns of overfishing have been raised for several protogynous grouper species along the Tropical Eastern Pacific, including the Pacific goliath grouper (Epinephelus quinquefasciatus) and the sailfin grouper (Mycteroperca olfax) (Sala et al., 2004; Usseglio et al., 2016), but there is little scientific guidance for their management or conservation.
The goliath grouper (Epinephelus itajara) was historically reported as widely distributed throughout the Tropical Atlantic and Eastern Pacific (Heemstra and Randall, 1993), until a genetic study by Craig et al. (2009) identified the population of the Eastern Pacific as a separate and distinct species, the Pacific goliath grouper (Epinephelus quinquefasciatus, hereafter PGG). Despite the Atlantic and Pacific species being genetically distinct species, no morphological differences were identified (Craig et al., 2009). Given its recognition as a new species, a regional assessment by Erisman et al. (2018) found severe population declines of PGG since the 1990s due to overfishing throughout is distribution (from Mexico to Peru). Despite signs of severe overfishing, the IUCN Red List categorizes the PGG as “Data Deficient” due to minimal species-specific data on fishing intensity and a complete lack of fishery-independent biological and ecological data (Sadovy de Mitcheson et al., 2013). An active fishery for the species has been documented in Pacific Colombia (Castellanos-Galindo et al., 2018). In Buenaventura (main fish market in Pacific Colombia), the fishery is characterized by the targeting of “mero especial” (“special grouper,”) which are groupers between 2.5 and 25 kg. These small fish often fetch higher commercial values (US$ 3.7 per kilo) than groupers >25 kg (US$1.8 per kilo) (Baos et al., 2015; Castellanos-Galindo et al., 2018). In most northern areas (north of Cabo Corrientes), the prices per kilo of PGG (US$ 2 per kilo) are the same regardless of the size (Castellanos-Galindo et al., 2018). Although fishing pressure for PGG in Pacific Colombia has been speculated to be less severe than in other countries (Sala et al., 2004; Castellanos-Galindo et al., 2018), there is scant information on the overall health of the population, including whether or not overfishing is occurring.
Unlike the widely distributed PGG, the geographic distribution of the sailfin grouper (Mycteroperca olfax) is restricted to three offshore island systems (i.e., Galapagos, Cocos Islands, and Malpelo Island) in the Tropical Eastern Pacific, with the majority of its population occurring in the Galapagos (Erisman and Craig, 2018). In the Galapagos, the species is locally known as bacalao, and is of great economic and cultural value (Nicolaides et al., 2002). Bacalao occupies a high trophic level (Okey et al., 2004) and is a protogynous hermaphrodite, resulting in a highly female-skewed sex ratio (0.009 male:female) (Coello and Grimm, 1993; Usseglio et al., 2015). Traditionally, this species is sold in dried form during Easter for a traditional dish called “fanesca” (Nicolaides et al., 2002). Commonly targeted by hand-line (i.e., hook-and-line), the bacalao fishery occurs year-around, peaking between the months of October and April (Nicolaides et al., 2002) in preparation for the Easter holiday. In addition, seafood markets commonly offer plate-sized fish to supply the local and tourism sectors demand for whole fresh fish. Similar to the PGG, a regional assessment for bacalao suggested population declines of 30% over the past four decades (Erisman and Craig, 2018). Given the species' limited geographical distribution and evidence of severe population declines, bacalao are currently categorized as Vulnerable in the IUCN Red List (Erisman and Craig, 2018). However, there is no research on how different levels of fishing pressure and fishing selectivities interact with their sex-changing life history to affect bacalao's demography and population productivity.
For both species, there is concern that overfishing may be occurring, as dramatic declines and fishery collapses have been documented in similar species with slow growth and protogynous sex change (de Mitcheson and Yin, 2015). However, there is limited information on the status of each population and the utility of different management and conservation actions for their fisheries. To inform management priorities, it is useful to calculate standard metrics of fishery health, known as reference points. These metrics quantify the effect of different fishing practices on the size-structure (biomass) and annual population productivity (measured as the size of the larval year class, called the “spawning potential”) (Goodyear, 1993). When the fished population spawning potential and biomass are compared to historic estimates, the resulting ratios (known in the fisheries literature as the spawning potential ratio, SPR, and the biomass reference point, BF/B0), are standardized indices of population health, or conversely, how overfished a population is. These metrics vary according to species' somatic growth and natural mortality rates, as well as the sizes of fish that are caught in the fishery (i.e., the fishery selectivity) (Beverton and Holt, 1957).
A sustainable fishery relies on a population's natural ability to compensate for fishing mortality. However, a population with truncated size structure (due to fishing) that faces a combination of poor environmental conditions and limited spawning potential can collapse suddenly because its natural ability to compensate for poor conditions has been eroded. The degree to which fishing has decrease a population's compensatory capacity can be quantified with the SPR and the biomass reference point. The SPR is especially useful for understanding how fishing can undermine a population's ability to buffer environmental change (Kindsvater et al., 2016). A common rule of thumb is that a fished population with values of SPR at 0.4 is fully exploited; an SPR lower than this threshold indicates the population is at risk of overfishing and potential collapse (Wiedenmann and Mangel, 2006).
In protogynous species, fishing can affect the spawning potential directly by removing females (reducing eggs) and by reducing the number of males, which could limit fertilization success (Alonzo and Mangel, 2004; Chuenpagdee et al., 2011). Fishing can also have indirect consequences for spawning potential, if the removal of large males induces sex change in smaller individuals. In other words, sex change from female to male can occur at smaller sizes in populations where large individuals are rare, as the population social structure adapts to fishing (Kindsvater et al., 2017; Pavlowich et al., 2018). This can lead to a situation where egg production decreases due to the loss of females to the male class, as well as to fishing. The ultimate consequences of fishing for the size structure of males and females will depend on the overall intensity of fishing mortality, the selectivity of the fishery, and the biology of the protogynous species (Alonzo and Mangel, 2004, 2005; Heppell et al., 2006; Provost and Jensen, 2015; Kindsvater et al., 2017).
The economic and cultural significance of the fisheries for Pacific goliath grouper and bacalao means severe population declines could jeopardize people's livelihoods, as well as cause cascading effects through nearshore marine ecosystems (Stallings, 2008; Estes et al., 2011). Understanding how varying levels of fishing selectivity can affect population trends and resilience to future changes of sex-changing fish is critical for assessing the economic and ecological sustainability of their fisheries (Provost and Jensen, 2015). We adapt a population model of sex-changing fish (Kindsvater et al., 2017) to simulate the population demography and dynamics of both PGG and bacalao under alternative fishing scenarios to evaluate interactions between sex change and fishery selectivity. We consider how variation in egg production and fertilization rates arising from the biology of each sex-changing species affect size and age structure after fishing. In addition, we contrast two scenarios of fishery selectivity, one where the largest individuals are preferred, and one targeting smaller (plate-sized) individuals. For each fishing selectivity scenario we estimate and compare two biological reference points which are used in fisheries management to evaluate population status: the spawning potential ratio (SPR), and relative biomass (BF/B0). This analysis allows us to identify the critical biology influences sex-changing species' resilience to fishing pressure, such as plasticity in size at sex change. We provide fisheries management recommendations, given what is currently known or can be inferred about these sex-changing species.
2. Methods
Species-specific life history information for Pacific goliath grouper and bacalao were sourced from published research. Biological data on age and growth, length at maturity, and mating and spawning behavior were deficient for PGG. Thus, life history parameters were inferred from its closely-related Atlantic counterpart (AGG, Epinephelus itajara), based on the assumption that PGG and AGG share similar growth rates and have a female-first reproductive strategy. Conversely, extensive information on life history characteristics such as age, growth, and reproduction exist for bacalao in the Galapagos Islands (Usseglio et al., 2015, 2016).
2.1. Demographic Modeling Framework
We adapted a modeling framework predicting age-structured population dynamics for sex-changing species (Kindsvater et al., 2017) to address the demographic consequences of fishing for PGG and bacalao, given their life histories and the specifics of their fisheries in the tropical eastern Pacific. The model structure is very similar to the general age-structured model used in fisheries stock assessments (Mangel, 2006; Haddon, 2011), but, unlike standard demographic models, follows the fates of males in addition to females. We used the published values of life history parameters for each species from our literature review, or inferred parameters from ecological principles or related species (Table 1). Individual length and age are related by data on species' growth, allowing us to model their vital rates - maturation, sex-change, fecundity, and fishing mortality—as a function of both size and age. For each species, we used discrete versions of the von Bertalanffy growth curve, which depend on its maximum length L∞ and growth coefficient k:
This function provides the length-at-age, given a length recruitment (a = 1), until the end of life (Amax).
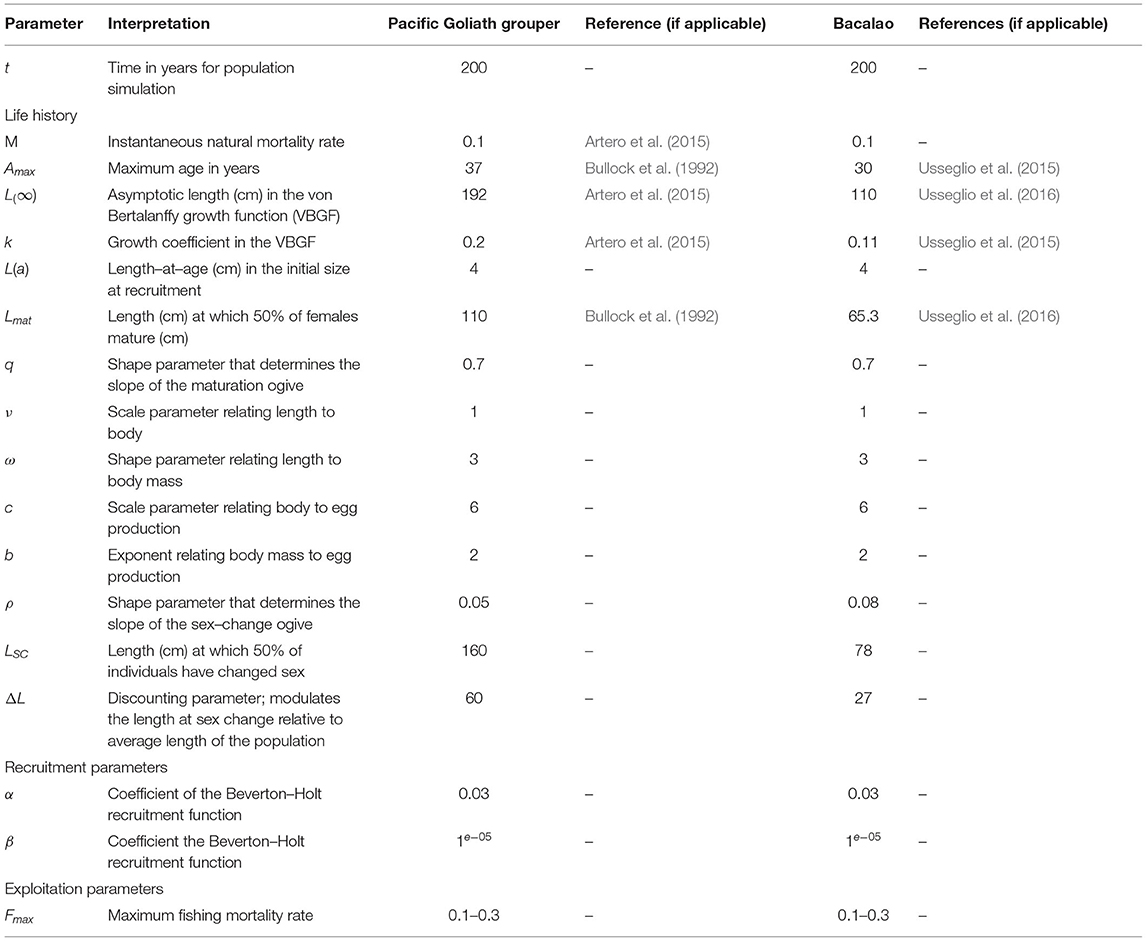
Table 1. Life history parameters for Pacific goliath grouper (Epinephelus quinquefasciatus) and Bacalao (Matertera olfax).
2.2. Maturation
For both species, we assumed all individuals were born and mature as females. It is possible that some individuals mature as males, but the evidence for this is lacking and has only been shown definitively for a few grouper species (Provost et al., 2017). We assumed that maturation is a function of length (which in turn is a function of age), where the probability an individual is mature at each size, pmat, is a logistic curve (ogive) (Figures 1C,D). The curve depends on the steepness parameter q and the parameter Lmat, which determines the length at which half of females of that length are mature (the inflection point):
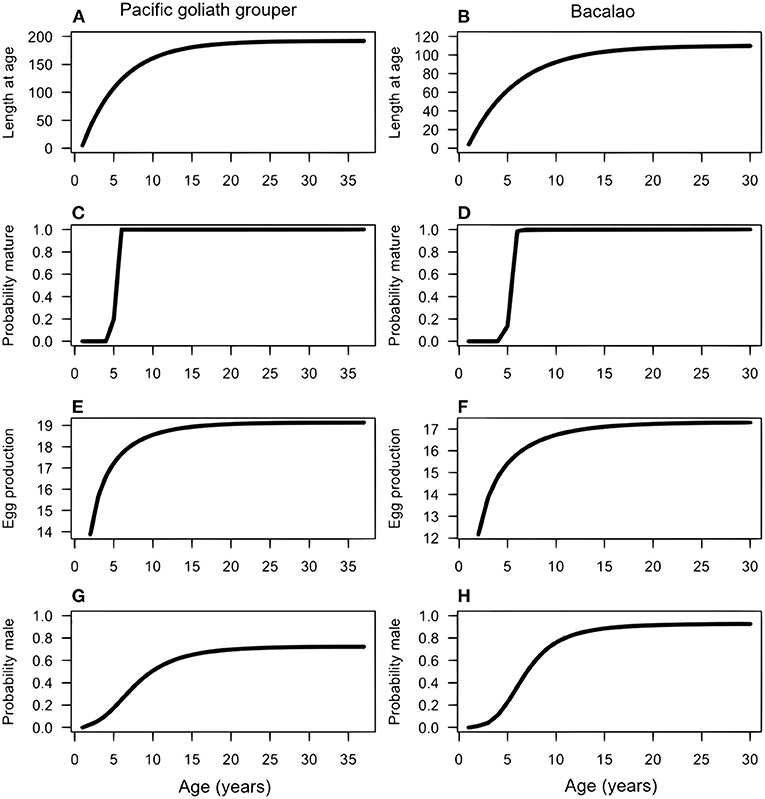
Figure 1. Life history information for the Pacific goliath grouper (left columns) and bacalao (right column). Length at age (A,B); maturation (C,D); fecundity (E,F); probability of sex-change (G,H).
2.3. Sex Change
Mature females transition to the terminal male class according to their length, relative to the average length of individuals in the population. We again represent the probability of sex change pSC with a logistic function of length, which depends on a steepness parameter ρ, and the mean length of individuals in the population, . Because the mean length incorporates immature individuals, we also included an offset parameter, ΔL, which adjusts the sensitivity of the sex change function to changes in mean size:
These assumptions mean that the probability an individual changes sex varies according to its length relative to the length of other mature individuals in the population. The probability an individual transitions at smaller sizes increases if large individuals are rare, and can vary as a function of time t. While little is known about the degree of plasticity in sex change rules in general, for many species there is evidence that individuals adapt to fishing by decreasing their size at sex change (Alonzo and Mangel, 2005). We also assumed that the probability to transition from female to male never exceeds the threshold of 0.8 (Figures 1G,H), so that some large individuals remain female.
2.4. Fecundity, Fertilization, and Recruitment
We assumed that egg production is related to female mass. As is commonly assumed in fisheries science, individual mass M is related to length with a simple cubic function M(a) = vL(a)3, where v scales the mass to the appropriate units (in this case, kilograms). We can then use another power function to relate mass to egg production E at each age, with a shape coefficient c and exponent b that can be adjusted according to measurements for each species. The number of viable eggs is further dependent on the specific fertilization probability f (where f is a number between 0 and 1):
Parameter values for Equation (4) can be found in Table 1. The parameter f is of interest because it allows us to address different rates of fertilization, representing potential scenarios of male depletion. Fertilization success is difficult to study in natural populations of sex-changing species, due to our limited knowledge of their spawning behaviors. The key unknown is how changes in the sex ratio of adults affect the chance that there are enough males, or large enough males, to fertilize all eggs produced in a spawning aggregation. The numbers and sizes of adult females and males change due to direct removal by fishing, as well as indirect effects on sex change (males could transition earlier as large individuals become more rare) and female egg production (fewer eggs are produced as large females are fished out or transition to males).
The pool, or year class, of larvae P in year t can be calculated as the sum of all individuals alive at time t, multiplied by the probability they are mature, their size-specific egg production, fertilization rates, and the probability they spawn as females or transition to males (which depends on the size structure of the spawning population).
Survival from the larval stage to “recruitment” to the age-1 year class N(1) in year t+1 depends on population density. As is customary in fish population models, we assumed a non-linear relationship between larval abundance and recruitment that was characterized by the Beverton-Holt recruitment function (Beverton and Holt, 1957; Kindsvater et al., 2017). This saturating function is characterized by the parameter α, which determines the slope near the origin, and β, which, along with α, determines the asymptote (Kindsvater et al., 2016):
Because there is very little empirical information on values of α and β, we varied the values of these parameters in sensitivity analyses, but we found they did not have a strong effect on reference points.
2.5. Natural and Fishing Mortality
For simplicity, after age one, we modeled an average natural mortality rate μ that applied to all ages. While it is certain that natural mortality rates vary with size, for these long-lived species natural mortality is low overall, so we approximate it with a constant rate (Kindsvater et al., 2017). As very little is known about fishing effort (e.g., number of fishers or boats), and because fishing effort certainly varies spatially for these species, we considered a range of fishing scenarios, S. The overall intensity of fishing varied with the coefficient Fmax, but was distributed across sizes (and ages) according to a selectivity function F[L(a)], which were chosen to represent different types of size-selective fishing scenarios. We focused on two scenarios, based on published information about fisheries for PGG and bacalao. First, we considered a preference for the largest individuals, known as “asymptotic” selectivity and second, a fishery with “plate-sized” selectivity, where fishers targeted only small fish, between 59 and 90 cm for PGG (Baos et al., 2015), and between 35 and 55 cm for bacalao [derived from Usseglio et al. (2016) and personal observations]. The asymptotic selectivity function was:
Note the parameter j is the steepness of curve and D determines the inflection point of the asymptotic selectivity function for each species. The parameters were chosen as follows: j = 0.05 and D = 90 for PGG and j = 0.1, D = 60 for bacalao. The selectivity functions (Figure 2) were adjusted by eye to match the distribution of sizes observed in fish markets (Usseglio et al., 2016; Castellanos-Galindo et al., 2018). By comparing the demographic structure of populations in each fishing scenario, and calculating the respective biological reference points, we are able to characterize the relative effects of fishing intensity and selectivity on population biomass and spawning potential.
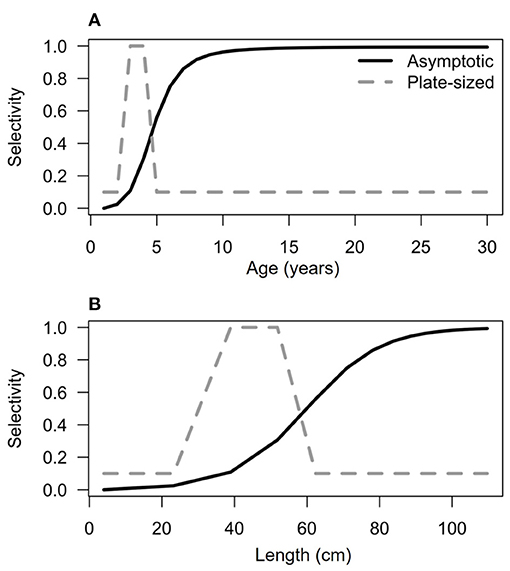
Figure 2. Selectivity functions at age (A) and length (B) for bacalao (similar functions for PGG are not shown). Asymptotic selectivity (solid black line) has a greater probability of capturing individuals larger than 60 cm in total length. Plate-sized selectivity (dotted gray line) has a greater probability of targeting individuals between 25 and 60 cm in total length.
2.6. Population Dynamics
We simulated the population dynamics through time, given the above functions governing births, recruitment, sex change, and mortality. The population N was initialized at an arbitrary starting size. The dynamics of each age class, in annual time steps, followed
We simulated populations until they reached a steady state, from which we were able to calculate the stable age distribution. We then “fished” our simulated population until it re-equilibrated to a new steady state. The unfished steady state (the carrying capacity) of the population emerges from the balance of births, deaths, and our assumptions about density-dependent recruitment. As very little information is known about recruitment function in tropical fisheries, an alternative is to focus on the relative effects of fishing on population biomass and larval production (spawning potential) under different fishing and fertilization scenarios (Kindsvater et al., 2016). Therefore, we calculated the spawning potential ratio (SPR) for each fishing scenario, S and rate of fertilization success, f.
The spawning potential of a cohort of females is equivalent to the expected lifetime larval production of a female in a population at equilibrium (O'Farrell and Botsford, 2005). The larval production is given in Equation (5). To calculate the spawning potential ratio, the larval production of a population that has reached a fished steady state is compared to the larval production of a population that is unfished.
We also calculated the biomass reference point, where in each fertilization scenario f, the steady-state fished biomass BS, f is compared with the unfished biomass B0, f. The steady-state biomass in each case is the product of the number of individuals in each age class a multiplied by their mass.
This ratio reflects the degree to which the population has been depleted by fishing, assuming constant recruitment and mortality rates, which represent average conditions over the long term. It is a useful metric that integrates changes in abundance and size structure of each sex caused by direct fishing mortality and the indirect effects of fishing on sex change.
3. Results
3.1. Literature Survey of Life History Information
Our literature review revealed that documented growth rates [the parameter k in Equation (1)] differed within and between our focal species. The reported growth rate for goliath grouper varied across its range. We assumed that growth rates among Atlantic and Pacific species would be similar at the same latitude. Therefore, we used the estimate of k = 0.2 from Artero et al. (2015) for AGG from French Guiana (approx. latitude 4° S) as a proxy for the growth rate of PGG from Pacific Colombia (approx. latitude 4.5° S). For bacalao, growth was estimated as k = 0.11. Goliath groupers had larger reported asymptotic lengths [L∞ = 192 cm, Artero et al. (2015)] than bacalao L∞ = 110 cm (Usseglio et al., 2015, 2016).
Maximum age for PGG was again estimated from studies of AGG to be approximately 37 years (Bullock et al., 1992). For bacalao, the maximum age was estimated to be 30 years (Usseglio et al., 2015). Figures 1A,B shows the resulting von Bertalanffy growth function (Equation 1) for PGG and bacalao, respectively. As these species both live longer than 30 years, natural mortality is likely to be relatively low. We assumed an average mortality rate of M = 0.1 for both species, after recruitment, which is similar to estimates used in other studies of long-lived teleosts (Kindsvater et al., 2016).
We found no explicit studies of PGG reproductive biology and spawning behavior. Hence, we used the estimated Lmat = 110 cm from Bullock et al. (1992) in the Gulf of Mexico for AGG. For bacalao, Usseglio et al. (2015) found that 50% of the female population reaches sexual maturity at approximately 65.4 cm in total length (Figures 1C,D). In terms of sex-change, the probability of females transitioning to males increases as a function of length, as seen in Equation (3). In the absence of explicit information on sex change for both PGG and bacalao [e.g., only one male found in Usseglio et al. (2016)], we manually adjusted the parameters Lc and ρ until the function approximated observations on male size structure in fish markets (Figures 1G,H).
In summary, we compiled parameters derived from empirical reports and inferred from related species to produce comprehensive descriptions of growth, maturation, egg production, and sex change as a function of age for each species (Figure 1).
3.2. Age Structure and Sex-Ratio
We next evaluated the effects of two types of fishing selectivity (i.e., asymptotic and plate-sized selectivity) on the age-structure and sex ratio of PGG and bacalao (Figure 3), given our parameters for the life history of each species. In the unfished scenario, for both species females vastly outnumbered males, a common pattern in female-first fish populations (Allsop and West, 2004). When assessing the effects of fishing (Fmax = 0.3) on the population age structure, asymptotic selectivity had a greater effect, depleting the largest and oldest individuals on the population for both species (Figures 3B,F). Targeting larger individuals of PGG significantly reduced the number of fish older than 15 years in the population (Figure 3B). As in PGG, a fishery with asymptotic selectivity also depleted the oldest individuals of the bacalao population, with fewer individuals reaching ages above 20 years old (Figure 3F). As expected, fishing that targeted large and old individuals resulted in populations characterized by younger individuals and a highly female-biased sex-ratio. All else being equal, the plate-sized selectivity scenario had less effect on the steady-state population structure for both species (Figures 3C,F). Targeting individuals only within size-specific slots (PGG, 59–90 cm TL; bacalao, 35–55 cm TL) allowed some old (large) males to persist. In both selectivity scenarios, due to plasticity in the size threshold for sex change, females transitioned to males earlier in life.
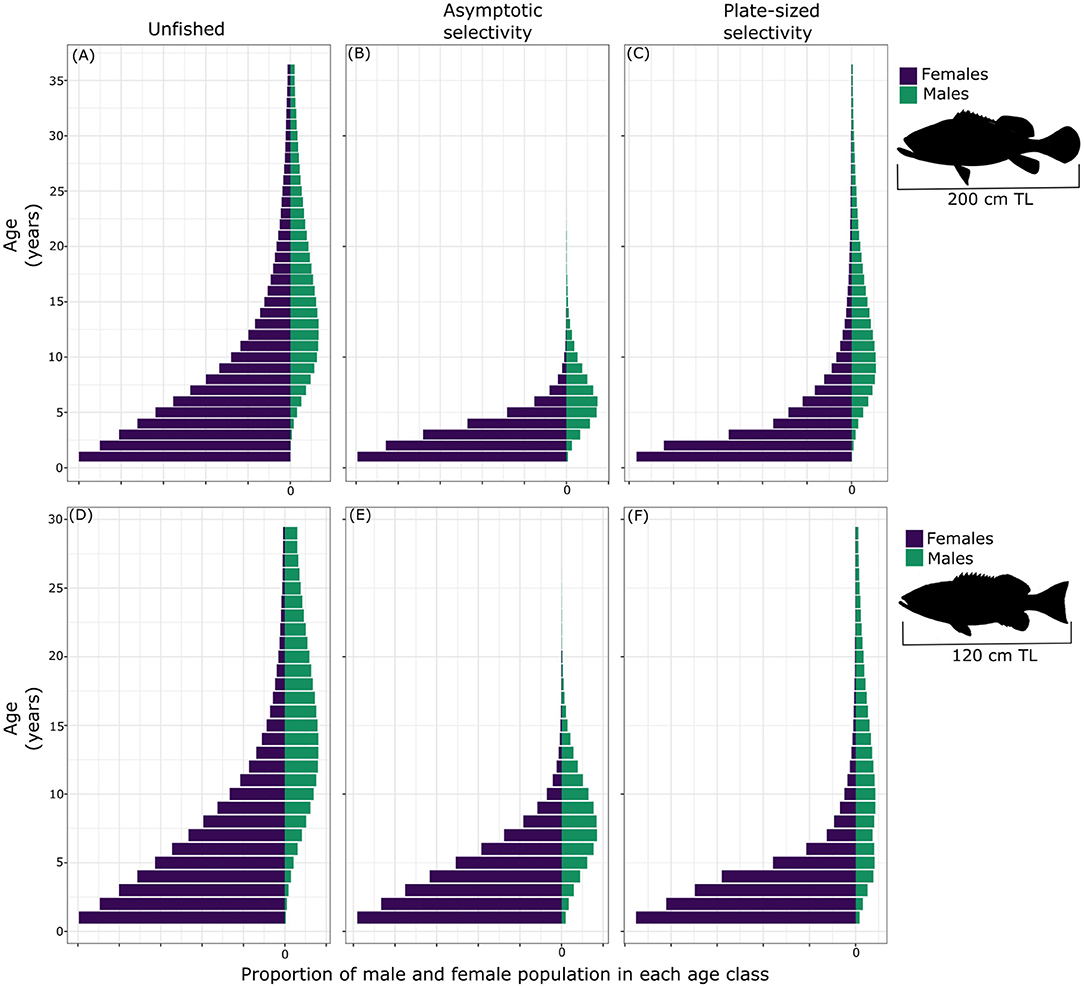
Figure 3. Stable age distribution of unfished (A), with asymptotic selectivity (B) and with plate-sized selectivity (C) for Pacific goliath grouper and of unfished (D), with asymptotic selectivity (E) and with plate-sized selectivity (F) of bacalao, with fishing mortality, F = 0.3 and fertilization success, f = 100%.
3.3. Biological Reference Points
We assessed the effects of varying levels of fishing mortality (Fmax) and fertilization success (f) on the spawning potential ratio (SPR) and relative biomass (BF/B0), for PGG and bacalao populations in each selectivity and fertilization scenario (Figure 4). We also varied overall fishing effort. Across our scenarios, targeting the largest individuals (asymptotic selectivity) had the greatest impact on the biological reference points (both SPR and BF/B0 were lowest) for populations of both species. Reduced fertilization success affected larval production: when fertilization rates dropped from 100 to 50% the SPR values declined by half regardless of the fishing selectivity. By contrast, the relative biomass (BF/B0) of both species was not affected (Figure 4), because of density-dependent compensation in larval recruitment. This result would likely change with stochastic variation in the recruitment function and warrants further investigation.
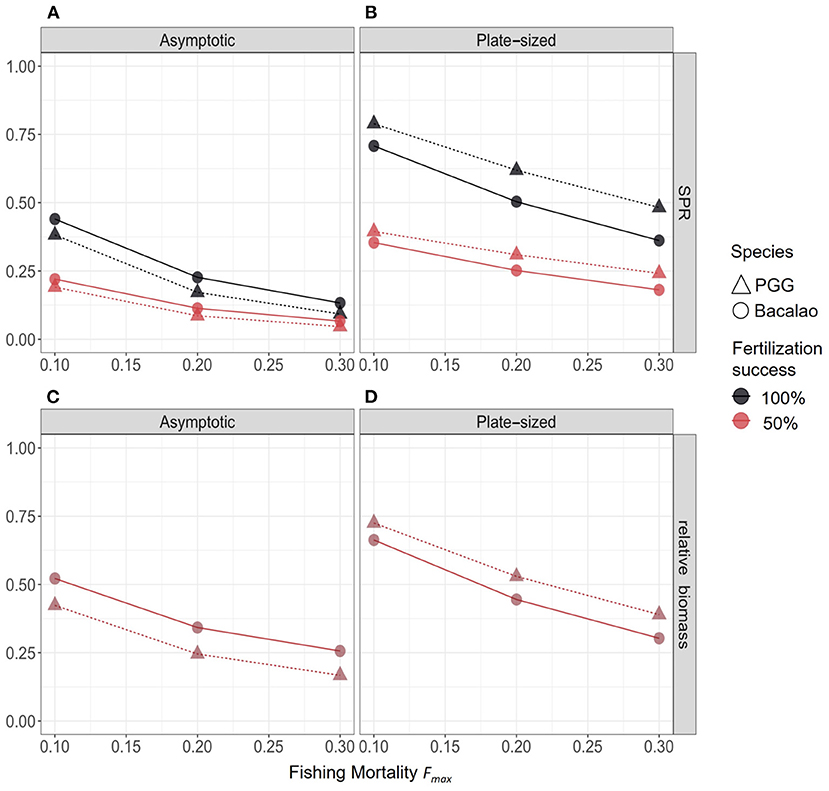
Figure 4. Biological reference points for PGG (triangles) and bacalao (circles), with different selectivity and fertilization scenarios. The top row (A,B) shows the SPR for each selectivity scenario, and bottom row (C,D) shows the biomass reference point. Colors represent fertilization success; note that for biomass, the two colors are on top of one another.
4. Discussion
For many small-scale fisheries in Latin American countries, fisheries -dependent and independent data is often limited in quantity and quality, making assessments of commercially, culturally, and ecologically valuable fish species extremely challenging (Chuenpagdee et al., 2011). Additional challenges arise when exploited fish populations are subject to multiple types of fishing selectivity. Depending on the species targeted and demand, fishing selectivity can vary across locations. Here, we considered two types of fishing selectivity for our population model, the asymptotic and plate-sized selectivity for PGG and bacalao, two sex-changing groupers of the Tropical Eastern Pacific. Traditionally, targeting the largest fish of the population often yields higher economic revenue for fishers. While this holds for certain species, there are growing market demands driven by people's needs, such as plate-sized fish, where fish within a specific size range yield higher prices than the largest individuals of the population. Accounting for particularities in fishing selectivity can help understand the effects of fishing on the sustainability of vulnerable fish populations.
We used species' life history characteristics to characterize vital rates in our population model, and evaluated how variation in growth rates, maturation, and fertilization success interact with different scenarios of intensity and selectivity of fishing to subsequently affect the age structure and sex ratio of PGG and bacalao. We demonstrated that changes in fishing selectivity can affect the population structure of PGG and bacalao differently.
4.1. Common Patterns of Vulnerability to Overfishing in Both Species
Despite the lack of species-specific life history information for PGG and limited data for bacalao, we inferred realistic biological and fishery parameters based on ecological principles and field observations of both species. We found the relative vulnerability of both of these species populations depended primarily on fishery selectivity. The PGG and bacalao populations were the most vulnerable to fishing scenarios targeting large individuals. Asymptotic selectivity led to age structure truncation, heavily skewed sex ratios, and severe declines in both reference points (SPR and relative biomass).
When we considered management scenarios that could improve the sustainability of fisheries for sex-changing species, plate-size selectivity was less detrimental (given the reference points of both species) when fishing effort was low or moderate (Figures 4B,D). This suggests that fisheries management that concentrates harvest on individuals within a limited size range could allow females to survive long enough to change sex and maintain sex ratios ensuring all eggs are fertilized. However, we still do not have sufficient information for either species to gauge what sex ratio is sufficient to maintain fertilization rates. Our model predicted that a fishery targeting smaller individuals will still lead to rapid overfishing (SPR < 0.4) if fertilization success rates are reduced by 50%, for either species (Supplementary Tables 1, 2). Furthermore, in contrast to the asymptotic selectivity scenario, the slower growing bacalao had a lower SPR and relative biomass than PGG in fishing scenarios with plate-sized selectivity. This is because slower growing fish (such as bacalao) spent more time at sizes vulnerable to fishing mortality, while fast growing fish (such as PGG) outgrow this range more quickly. This is an underappreciated source of variation in the response-of species to size-based management strategies. For example, Kindsvater et al. (2017) predicted that plate-sized selectivity that targeted sub-adults decreased the spawning potential of large sex-changing species more than other types of harvest selectivity. The differences between that analysis and the results of this paper likely arise from the particular biology of each species, including the somatic growth rate, the fishery selectivity relative to maturation size, and the details of the sex change function. The analyses presented here and in Kindsvater et al. (2017) are in agreement that management actions that preserve population size-structure are critically important for sex-changing species.
4.2. Contributions of Fishery Selectivity to Status of PGG Across Its Range
Our model predicted rapid overfishing of PGG (SPR < 0.4) when fishing selects the largest individuals of the population, even at relatively low levels of fishing mortality. This prediction is consistent with observed dynamics of goliath grouper fisheries. Similar population trends have been documented for the Atlantic goliath grouper (AGG, Epinephelus itajara) and Red Hind grouper (Epinephelus guttatus), where sport fisheries targeting the largest individuals (i.e., trophy fish) of the population contributed to sperm limitation and ultimately led to reproductive failure and population collapse (Bullock et al., 1992; Beets and Friedlander, 1999; Provost and Jensen, 2015). Across most of the range of PGG, there is little to no fisheries-dependent or independent data on population trends over time (Castellanos-Galindo et al., 2018; Erisman et al., 2018). However, in Pacific Panama and Ecuador, where large individuals are routinely harvested, it is likely that PGG have experienced severe disruption in their ability to recruit, due to low numbers of spawning males in the older cohorts.
The potential for a plate-sized fishery to be sustainable for PGG is consistent with the fact that in Pacific Colombia, where “plate-size” fish fetch a premium price and thus are selectivity targeted by fishers, larger individuals of PGG are still seen (Castellanos-Galindo et al., 2018). These larger fish potentially contribute to the pool of viable gametes, sustaining recruitment. Additional factors that are unique to this area might contribute to the health of the PGG population, including low human population density, relatively low fishing pressure, and the existence of large mangrove areas providing suitable nursery habitat for juvenile PGG (Castellanos-Galindo et al., 2018).
4.3. Suggested Improvements to Current Fishing Practices for Bacalao
Our model predicted when bacalao were fished with low intensity and with asymptotic selectivity, the estimated SPR value was close to the fully exploited threshold (SPR = 0.44) at the lowest level of fishing mortality (assuming all eggs are fertilized; Supplementary Table 2). In reality, the bacalao fishery in the Galapagos Islands occurs year-round and targets a wide range of sizes, including the largest individuals (Nicolaides et al., 2002). We do not know the level of fishing effort. Usseglio et al. (2016) found a highly skewed female population, and signs of overfishing (SPR < 0.5). These findings are consistent with our predictions, and suggests that if depletion of large individuals persists, reproductive failure and collapse can occur even at low levels of fishing mortality (Alonzo and Mangel, 2004).
Currently there are no catch size limits for any finish species in the Galapagos Islands, including bacalao. In the Galapagos National Park jurisdiction, fisheries management strategies include a zonation scheme (e.g., no-take zones), gear restrictions, and a licensing system (Castrejn et al., 2014). Research has suggested the potential benefits of marine reserves for protogynous species populations (e.g, Alonzo and Mangel, 2004; Heppell et al., 2006; Molloy et al., 2008; Easter et al., 2020) if they successfully protect enough of the male population to maintain healthy sex ratios (Allsop and West, 2004). However, the time it takes to observe population recovery will depend on the demography of the fished population and whether the population is demographically closed or open [i.e., the larval pool comes from inside or outside the protected area (Easter et al., 2020)].
4.4. Fisheries Management of Protogynous Species and Knowledge Gaps
Evidence has shown that protogynous hermaphrodite fish species are more susceptible to overfishing than gonochoristic species (Provost and Jensen, 2015; Kindsvater et al., 2017). In a sex-changing population, fishing mortality can disproportionately affect one sex, whereas in a gonochoristic population it is expected that fishing will impact both sexes equally (Provost and Jensen, 2015). When fishing mortality affects one sex more than the other, it can induce instability in the sex ratio, growth, and fertilization success of the targeted population (Alonzo et al., 2008). Therefore, sustaining exploited protogynous populations requires specific fisheries management strategies that address the details of sex-changing behaviors (Alonzo et al., 2008; Provost and Jensen, 2015).
We presented evidence suggesting that fisheries management targeting small (plate-sized) fish would be more sustainable for PGG and bacalao than current fishing practices. However, it is essential to understand the exact sex-changing rules governing these species to inform species-specific management strategies. In particular, further investigations on the size or age at which sex change in the population occurs, natural mortality, and recruitment dynamics are needed to improve model accuracy. Our population model was sensitive to our assumptions regarding natural mortality and the likelihood of transition (size at which females change sex to males). We assumed a constant natural mortality across all ages for each species, as is common in fisheries population models, but it is likely that natural mortality varies across different age groups and between sexes. Moreover, maturation and transition probabilities are size-specific and may vary among populations and locations (Alonzo and Mangel, 2004).
Finally, complex social structures of hermaphroditic fish can affect a species' susceptibility to fishing in ways that we could not address in our model (Alonzo and Mangel, 2004; Provost and Jensen, 2015). For example, during spawning aggregation events, a single large dominant male can fertilize the eggs of multiple females. Therefore, the removal of large males during a spawning period can disrupt complex social structures, causing fertilization failure (Alonzo and Mangel, 2004). However, there is no information about the mating behaviors of PGG and bacalao, and whether their reproductive patterns would differ from other protogynous grouper species (e.g., Red Hind; Sadovy et al., 1994). We recommend further investigation into the complex social structures, mating behaviors, and age-specific changes in survival and fecundity for bacalao and PGG to provide particular fisheries management strategies that would allow the recovery of the populations.
4.5. Modeling Approach for Data-Poor Fisheries
While PGG and bacalao represent commercially and culturally valuable fish species in Pacific Colombia and the Galapagos islands, there is little empirical information on the sex-changing rules governing these populations. Our modeling framework provided theoretical insights into the relative vulnerability to overfishing of these two data-poor fisheries, using life history characteristics gathered from the literature. This modeling framework has the flexibility to be applied to other fish species (i.e., protogynous and gonochoristic fish) of the Tropical Eastern Pacific, and to a variety of fishing scenarios. This approach presents an opportunity to prioritize species and fisheries for which sustainable management could be improved in developing, data-limited fisheries. For the fisheries considered here, addressing knowledge gaps with further investigation into the sex-changing rules of these species will improve our understanding of the potential consequences of fishing on population dynamics, and inform the best management strategies for the sustainability of these valuable fisheries.
Data Availability Statement
Analysis script and data reported in this article are archived through Zenodo at https://doi.org/10.5281/zenodo.6342753.
Author Contributions
CC-M and HKK conceived the idea. CC-M compiled the data and adapted and analyzed code initially written by HKK. Both authors wrote the manuscript and approved of its final contents.
Funding
This work was supported by NSF DEB-1556779 to HKK and by National Geographic Society Europe: Conservation grant (GEFNEY 152-15) to CC-M. The authors acknowledge the Virginia Tech Open Access Support Fund.
Conflict of Interest
The authors declare that the research was conducted in the absence of any commercial or financial relationships that could be construed as a potential conflict of interest.
Publisher's Note
All claims expressed in this article are solely those of the authors and do not necessarily represent those of their affiliated organizations, or those of the publisher, the editors and the reviewers. Any product that may be evaluated in this article, or claim that may be made by its manufacturer, is not guaranteed or endorsed by the publisher.
Supplementary Material
The Supplementary Material for this article can be found online at: https://www.frontiersin.org/articles/10.3389/fevo.2022.850006/full#supplementary-material
References
Allsop, D. J., and West, S. A. (2004). Sex-ratio evolution in sex changing animals. Evolution 58, 1019–1027. doi: 10.1111/j.0014-3820.2004.tb00435.x
Alonzo, S. H., Ish, T., Key, M., MacCall, A. D., and Mangel, M. (2008). The importance of incorporating protogynous sex change into stock assessments. Bull. Marine Sci. 83, 163–179.
Alonzo, S. H., and Mangel, M. (2004). The effects of size-selective fisheries on the stock dynamics of and sperm limitation in sex-changing fish. Fish. Bull. 102, 1–13.
Alonzo, S. H., and Mangel, M. (2005). Sex-change rules, stock dynamics, and the performance of spawning-per-recruit measures in protogynous stocks. Fish. Bull. 103, 229–246.
Artero, C., Murie, D. J., Koenig, C. C., Berzins, R., Bouchon, C., and Lampert, L. (2015). Age, growth, and mortality of the atlantic goliath grouper epinephelus itajara in french guiana. Endangered Species Res. 28, 275–287. doi: 10.3354/esr00691
Baos, R., Castellanos-Galindo, G. A., Zapata, L. A., and Craig, M. (2015). “Market-driven size-selective fishing can jeopardize pacific goliath grouper (Epinephelus quinquefasciatus) survival in the colombian pacific coast,” in Manejo de pesquerias Latinoamerica, 1. Available online at: https://www.scinapse.io/papers/1889416878
Beets, J., and Friedlander, A. (1999). Evaluation of a conservation strategy: a spawning aggregation closure for red hind, Epinephelus guttatus, in the u.s. virgin islands. Environ. Biol. Fishes 55, 91–98. doi: 10.1023/A:1007404421518
Begossi, A., Salyvonchyk, S., Glamuzina, B., de Souza, S. P., Lopes, P. F. M., Priolli, R. H. G., et al. (2019). Fishers and groupers (epinephelus marginatus and e. morio) in the coast of brazil: integrating information for conservation. J. Ethnobiol. Ethnomed. 15, 53. doi: 10.1186/s13002-019-0331-2
Beverton, R. J., and Holt, S. J. (1957). On the Dynamics of Exploited Fish Populations. London: Fishery Investigation Series.
Bullock, L. H., Murphy, M. D., Godcharles, M. F., and Mitchell, M. E. (1992). Age, growth, and reproduction of jewfish epinephelus itajara in the eastern gulf of mexico. Fish. Bull. 90, 243–249.
Castellanos-Galindo, G. A., Chong-Montenegro, C., Baos E, R. A., Zapata, L. A., Tompkins, P., Graham, R. T., et al. (2018). Using landing statistics and fishers traditional ecological knowledge to assess conservation threats to pacific goliath grouper in colombia. Aquatic Conserv. Marine Freshwater Ecosyst. 28, 305–314. doi: 10.1002/aqc.2871
Castellanos-Galindo, G. A., and Zapata Padilla, L. A. (2019). “Small-scale fisheries on the pacific coast of colombia: historical context, current situation, and future challenges,” in Viability and Sustainability of Small-Scale Fisheries in Latin America and The Caribbean, eds Salas, S., Barragn-Paladines, M. J., and Chuenpagdee, R., 79–100. Springer International Publishing, Cham.
Castrejn, M., Defeo, O., Reck, G., and Charles, A. (2014). “Fishery science in galapagos: from a resource-focused to a social ecological systems approach,” in The Galapagos Marine Reserve: A Dynamic Social-Ecological System, eds J. Denkinger, and L. Vinueza (Cham: Springer International Publishing), 159–185.
Chuenpagdee, R., Salas, S., and Barragn-Paladines, M. J. (2019). “Big questions about sustainability and viability in small-scale fisheries,” in Viability and Sustainability of Small-Scale Fisheries in Latin America and The Caribbean, eds S. Salas, M. J. Barragn-Paladines, and R. Chuenpagdee (Cham: Springer International Publishing), 3–13.
Chuenpagdee, R., Salas, S., Charles, A., and Seijo, J. (2011). “Assessing and managing coastal fisheries of latin america and the caribbean: underlying patterns and trends,” in Coastal Fisheries of Latin America and the Caribbean, eds R. Chuenpagdee, A. Charles, and J. Seijo (Rome: Food and Agriculture Organization of the United Nations), 385–401.
Coello, S., and Grimm, A. (1993). The reproductive biology of Mycteroperca olfax (jenyns)(pisces: Serranidae): protogyny and breeding season. Revista de Ciencias del Mar y Limnologia 3, 115–128.
Craig, M. T., Graham, R. T., Torres, R. A., Hyde, J. R., Freitas, M. O., Ferreira, B. P., et al. (2009). How many species of goliath grouper are there? cryptic genetic divergence in a threatened marine fish and the resurrection of a geopolitical species. Endangered Species Res. 7, 167–174. doi: 10.3354/esr00117
de Mitcheson, Y. S., and Yin, X. (2015). Cashing in on coral reefs: the implications of exporting reef fishes. Ecology of Fishes on Coral Reefs. Cambridge: Cambridge University Press, 166.
de Oliveira Leis, M., Barragn-Paladines, M. J., Saldaa, A., Bishop, D., Jin, J. H., Kerei, V., et al. (2019). “Overview of small-scale fisheries in latin america and the caribbean: Challenges and prospects,” in Viability and Sustainability of Small-Scale Fisheries in Latin America and The Caribbean, eds S. Salas, M. J. Barragn-Paladines, and R. Chuenpagdee (Cham: Springer International Publishing), 15–47.
Easter, E. E., Adreani, M. S., Hamilton, S. L., Steele, M. A., Pang, S., and White, J. W. (2020). Influence of protogynous sex change on recovery of fish populations within marine protected areas. Ecol. Appl. 30, e02070. doi: 10.1002/eap.2070
Erisman, B. E., Bertoncini, A. A., and Craig, M. T. (2018). Epinephelus quinquefasciatus. the iucn red list of threatened species 2018: e.t195292a100555967. Report, International Union for Conservation of Nature.
Erisman, B. E., and Craig, M. T. (2018). Mycteroperca olfax. the iucn red list of threatened species 2018: e.t14051a100466495. Report, International Union for Conservation of Nature.
Estes, J. A., Terborgh, J., Brashares, J. S., Power, M. E., Berger, J., Bond, W. J., et al. (2011). Trophic downgrading of planet earth. Science 333, 301–306. doi: 10.1126/science.1205106
FAO (2000). Informe del taller sobre manejo y asignacion de recursos pesqueros a pescadores artesanales en america latina. Report, Food and Agriculture Organization.
Goodyear, C. P.. (1993). Spawning stock biomass per recruit in fisheries management: foundation and current use. Can. Special Publ. Fish. Aquatic Sci. 67–82.
Heemstra, P., and Randall, J. (1993). Vol.16. groupers of the world (family serranidae, subfamily epinephelinae) an annotated and illustrated catalogue of the grouper, rockcod, hind, coral grouper and lyretail species known to date. Rome: FAO Fisheries Synopsis.
Heppell, S. S., Heppell, S. A., Coleman, F. C., and Koenig, C. C. (2006). Models to compare management options for a protogynous fish. Ecol. Appl. 16, 238–249. doi: 10.1890/04-1113
Kindsvater, H. K., Mangel, M., Reynolds, J. D., and Dulvy, N. K. (2016). Ten principles from evolutionary ecology essential for effective marine conservation. Ecol. Evol. 6, 2125–2138. doi: 10.1002/ece3.2012
Kindsvater, H. K., Reynolds, J. D., Sadovy de Mitcheson, Y., and Mangel, M. (2017). Selectivity matters: rules of thumb for management of plate-sized, sex-changing fish in the live reef food fish trade. Fish Fish. 18, 821–836. doi: 10.1111/faf.12208
Koenig, C. C., Coleman, F. C., and Kingon, K. (2011). Pattern of recovery of the goliath grouper epinephelus itajara population in the southeastern us. Bull. Marine Sci. 87, 891–911. doi: 10.5343/bms.2010.1056
Mangel, M.. (2006). The Theoretical Biologist's Toolbox: Quantitative Methods for Ecology and Evolutionary Biology. Cambridge: Cambridge University Press.
Molloy, P. P., Reynolds, J. D., Gage, M. J. G., Mosqueira, I., and Ct, I. M. (2008). Links between sex change and fish densities in marine protected areas. Biol. Conserv. 141, 187–197. doi: 10.1016/j.biocon.2007.09.023
Nicolaides, F., Murillo, J., Toral, V., and Reck, G. (2002). “Bacalo,” in Reserva marina de Galapagos: linea base de la biodiversidad., eds E. Danulat, and G. Edgar (Galapagos: Fundacion Charles Darwin/Servicio Parque Nacional Galapagos), 146–165.
O'Farrell, M. R., and Botsford, L. W. (2005). Estimation of change in lifetime egg production from length frequency data. Can. J. Fish. Aquatic Sci. 62, 1626–1639. doi: 10.1139/f05-064
Okey, T. A., Banks, S., Born, A. F., Bustamante, R. H., Calvopia, M., Edgar, G. J., et al. (2004). A trophic model of a galpagos subtidal rocky reef for evaluating fisheries and conservation strategies. Ecol. Model. 172, 383–401. doi: 10.1016/j.ecolmodel.2003.09.019
Pavlowich, T., Webster, D. G., and Kapuscinski, A. R. (2018). Leveraging sex change in parrotfish to manage fished populations. Elementa Sci. Anthropocene 6, 63. doi: 10.1525/elementa.318
Provost, M. M., and Jensen, O. P. (2015). The impacts of fishing on hermaphoditic and treatment of sex change in stock assessments. Fisheries 40, 536–545. doi: 10.1080/03632415.2015.1093471
Provost, M. M., Jensen, O. P., and Berlinsky, D. L. (2017). Influence of size, age, and spawning season on sex change in black sea bass. Marine Coastal Fish. 9, 126–138. doi: 10.1080/19425120.2016.1274696
Sadovy de Mitcheson, Y., Craig, M. T., Bertoncini, A. A., Carpenter, K. E., Cheung, W. W. L., Choat, J. H., et al. (2013). Fishing groupers towards extinction: a global assessment of threats and extinction risks in a billion dollar fishery. Fish Fisheries 14, 119–136. doi: 10.1111/j.1467-2979.2011.00455.x
Sadovy de Mitcheson, Y., Linardich, C., Barreiros, J. P., Ralph, G. M., Aguilar-Perera, A., Afonso, P., et al. (2020). Valuable but vulnerable: over-fishing and under-management continue to threaten groupers so what now? Marine Policy 116, 103909. doi: 10.1016/j.marpol.2020.103909
Sadovy, Y., and Colin, P. L. (1995). Sexual development and sexuality in the nassau grouper. J. Fish Biol. 46, 961–976.
Sadovy, Y., Rosario, A., and Romn, A. (1994). “Reproduction in an aggregating grouper, the red hind, epinephelus guttatus,” in Women in Ichthyology: An Anthology in Honour of ET, Ro and Genie, eds E. K. Balon, M. N. Bruton, and D. L. G. Noakes (Dordrecht: Springer), 269–286.
Sala, E., Aburto-Oropeza, O., Paredes, G., and Thompson, G. (2003). Spawning aggregations and reproductive behavior of reef fishes in the gulf of california. Bull. Marine Sci. 72, 103–121.
Sala, E., Aburto-Oropeza, O., Reza, M., Paredes, G., and Lpez-Lemus, L. G. (2004). Fishing down coastal food webs in the gulf of california. Fisheries 29, 19–25. doi: 10.1577/1548-8446(2004)29[19:FDCFWI]2.0.CO;2
Salas, S., Chuenpagdee, R., Seijo, J. C., and Charles, A. (2007). Challenges in the assessment and management of small-scale fisheries in latin america and the caribbean. Fish. Res. 87, 5–16. doi: 10.1016/j.fishres.2007.06.015
Stallings, C. D.. (2008). Indirect effects of an exploited predator on recruitment of coral-reef fishes. Ecology 89, 2090–2095. doi: 10.1890/07-1671.1
Usseglio, P., Friedlander, A. M., DeMartini, E. E., Schuhbauer, A., Schemmel, E., and de Lon, P. S. (2015). Improved estimates of age, growth and reproduction for the regionally endemic galapagos sailfin grouper Mycteroperca olfax (jenyns, 1840). PeerJ 3, e1270. doi: 10.7717/peerj.1270
Usseglio, P., Friedlander, A. M., Koike, H., Zimmerhackel, J., Schuhbauer, A., Eddy, T., et al. (2016). So long and thanks for all the fish: overexploitation of the regionally endemic galapagos grouper Mycteroperca olfax (jenyns, 1840). PLOS ONE 11, e0165167. doi: 10.1371/journal.pone.0165167
Keywords: data-poor, hermaphroditism, groupers, Latin America, sex change, Epinephelus quinquefasciatus, Mycteroperca olfax
Citation: Chong-Montenegro C and Kindsvater HK (2022) Demographic Consequences of Small-Scale Fisheries for Two Sex-Changing Groupers of the Tropical Eastern Pacific. Front. Ecol. Evol. 10:850006. doi: 10.3389/fevo.2022.850006
Received: 07 January 2022; Accepted: 28 February 2022;
Published: 30 March 2022.
Edited by:
Amanda Wilson Carter, The University of Tennessee, Knoxville, United StatesReviewed by:
Jorge Paramo, University of Magdalena, ColombiaSimon Nicol, Pacific Community (SPC), New Caledonia
Copyright © 2022 Chong-Montenegro and Kindsvater. This is an open-access article distributed under the terms of the Creative Commons Attribution License (CC BY). The use, distribution or reproduction in other forums is permitted, provided the original author(s) and the copyright owner(s) are credited and that the original publication in this journal is cited, in accordance with accepted academic practice. No use, distribution or reproduction is permitted which does not comply with these terms.
*Correspondence: Carolina Chong-Montenegro, Yy5jaG9uZ21vbnRlbmVncm9AdXEubmV0LmF1