- 1Pearl River Fisheries Research Institute, Chinese Academy of Fishery Sciences, Guangzhou, China
- 2Guangzhou Scientific Observing and Experimental Station of National Fisheries Resources and Environment, Guangzhou, China
- 3Key Laboratory of Aquatic Animal Immune Technology of Guangdong Province, Guangzhou, China
Energy allocation is an important component of the reproductive cycle, and as such, it will affect survival, migration, and reproductive success. To reduce the risk of reproductive migration failure and to optimally allocate a limited amount of energy, it is vital to first understand the trade-off between reproduction and somatic growth in migrating fish. In this study, we chose Megalobrama terminalis, an endemic species residing in the Pearl River basin with relatively high migratory potential, as a candidate to investigate energy accumulation and allocation strategy during reproductive migration. The analysis used a quantitative assessment of biochemical composition and energy density in somatic and visceral tissues of M. terminalis females during the reproductive cycle. The results indicated that stage III to stage IV of M. terminalis was the vital migration-launching period. The asynchrony of development was confirmed in energetic relationships in muscle and ovary. Specifically, there was a regulatory mechanism for allocating lipids to each tissue reasonably during the breeding migratory preparation period (stage III). The significant change in lipid content of the ovary is considered as a crucial physiological index, which reflects the readiness for breeding migration of M. terminalis. In addition, the negative energy density relationship between somatic and reproductive tissues indicated a trade-off between maximum metabolic ability and energy efficiency before migration launching in M. terminalis. The present findings provide effective information for initiating further research on the ecological adaptation of migrating fish species.
Highlights
- Current study is the first demonstration of energy accumulation and allocation strategy in Megalobrama terminalis during the breeding migration.
- The remarkable change of lipid content in ovary is considered as a crucial physiological index reflecting the readiness for breeding migration of M. terminalis.
- A regulatory mechanism to allocate the lipid of each tissue reasonably in the breeding migratory preparation period of M. terminalis has been found in our research.
Introduction
Almost half of all vertebrate species are fish, and they encompass a wide spectrum of physiological and ecological adaptations. Generally speaking, fish life-history theory predicts that organisms will balance their energy allocation among maintenance, storage, growth, migration, and reproduction to maximize their fitness (Roff, 1983). As a vital aspect of the life history, reproductive behavior is highly relevant to optimal energy accumulation and allocation (Alonso-Fernández and Saborido-Rey, 2012; Villegas-Ríos et al., 2014). Specifically, females are recognized as allocating more energy to gonad development than males (Saborido–Rey et al., 2004). In addition, fish reproductive migration is a highly energy-consuming activity. In the course of reproductive migration, fish are likely to face various risks, for example, less effective avoidance of predation and energy shortages. Therefore, fish regulate the types and levels of energy stored in various organs to meet the energy requirements of reproductive migration (Caudill et al., 2007). The previous research has indicated that body size, osmotic pressure regulation, swimming speed, and sexual maturity contribute to fish migratory preparation (McCormick et al., 1998; Saborido–Rey et al., 2004). Usually, the migrating fish with large body size and high fertility rates have a clear “profit” as concerning the consumption of energy during reproduction migration (Wysujack et al., 2010; Barneche et al., 2018). Moreover, it has been hypothesized that storing sufficient energy may be necessary for migratory fish reproduction migratory (Jonsson and Jonsson, 2003; Bureau et al., 2007).
Barbour (1985) proposed the hypothesis that fish need to reserve enough energy for migration during the migratory preparation period. Certain relevant research points supported this hypothesis (Thorpe et al., 1998). Nevertheless, the preparation period that differs among individuals within a population is considered discrepantly (Jonsson and Jonsson, 2003). Relevant research has indicated that individuals with higher energy initiated the migration earlier (Colombier et al., 2007). The contribution of energy molecules stored in different fish tissues is unevenly distributed during reproductive migration (Kiessling et al., 2004), although information related to the fish migratory preparation process is lacking. Energy density is identified as one of the most important indexes used to assess the stored energy capacity of fish, because it depends on the contents carrying molecules such as proteins, fat, and carbohydrates (Mourente et al., 2002; Penney and Moffitt, 2014). In the recent years, energetics related to fish migration has become a focus in fish ecology (Penney and Moffitt, 2014; Thomas and Johan, 2018; Lennox et al., 2019; Tamario et al., 2019). However, to the best of our knowledge, a very few studies have been conducted on the mechanism of energy reallocation in fish tissues in relation to digestion, growth, reproduction, and other major functions during the maturing process. Thus, the study of the biochemical composition of wild fish populations is an important approach for understanding the variation in energy allocation among life-history processes (Connell, 1975).
The black Amur bream (Megalobrama terminalis) is a migratory species inhabiting the lower reaches of the Pearl River. The species is regarded as a good candidate for studying the energy allocation of migratory fish (Chen et al., 2020). During spawning seasons, M. terminalis migrate nearly 250 km upstream from a drainage network to the spawning grounds (Luopangjiang and Qingpeitang). Owing to the continuous enhancement of human activities (e.g., water conservancy projects, waterway dredging, water pollution, and overfishing), serious decreases in wild populations of M. terminalis have been reported in the Pearl River basin during this decade (Li et al., 2014, 2018). In our previous research, it was demonstrated that the M. terminalis spawning migration occurs from late June to mid-July, a pattern that is different from historical records (Liu et al., 2021b). In the recent studies, M. terminalis was deemed to be an omnivorous fish with strong ecological adaptability (Liu et al., 2020, Liu et al., 2021b). The amount of energy allocated between growth and reproduction is deemed to be the critical factors for the survival and reproduction of M. terminalis in natural ecosystems. Related research suggests that there is a shift in the diet of black Amur bream during the gonad development period, with different food preferences of both immature and mature individuals (Xia et al., 2017, 2020). Further research has provided evidence that M. terminalis regulates activities of the gut microbiome and degradation enzymes to digest foods with higher nutrition to supply energy for the spawning migration (Liu et al., 2021a). However, the overall effect on variation in energy allocating during M. terminalis reproduction migratory has been difficult to evaluate.
To date, most previous research on M. terminalis has been related to larval resources, feeding habits, ecological investigation of spawning grounds, and digestive function (Tan et al., 2009; Wang et al., 2010; Xia et al., 2017; Liu et al., 2020), whereas a very few studies have reported the pattern of energy accumulation and reproductive investment for M. terminalis in the course of the reproduction cycle. To fill this knowledge gap, we attempted to investigate the utilization efficiency of energy storage, energetic trade-offs between somatic and reproductive growth and differences in energy accumulation, and allocation for gonadal maturation and spawning migration of M. terminalis females. A quantitative assessment method that involves biochemical composition and energy density was utilized to analyze somatic and visceral tissues of M. terminalis females in the spawning ground, the fattening ground, and migration routes during the reproductive cycle. The aim was to characterize the process of energy accumulation and allocation during reproductive cycle of M. terminalis. In parallel, through analysis of fine-scale variation in proximate composition and energy content in the course of gonad development, the study aimed to provide data supporting further exploration of fitness in wild population of M. terminalis. Additionally, this study also collects data relevant to energetic ecology of fish migration.
Materials and Methods
Ethics Approval
The methods that involve animals in this study were conducted in accordance with the Laboratory Animal Management Principles of China. All experimental protocols were approved by the Ethics Committee of the Pearl River Fisheries Research Institute, Chinese Academy of Fishery Sciences.
Fish Sampling
A total of 350 female specimens of black Amur bream from five localities were collected using circular cast nets (16 m diameter, mesh size 3 cm) in the Pearl River during 2019. The sampling variables that include date of collection, location, and environment information are provided in Table 1. According to the corresponding sampling sites and sampling times, sampling in sites S1–S2 was conducted from July 9 to 21. Sampling in sites S3–S5 was conducted from June 18 to July 8 to ensure the distinction between migratory and non-migratory M. terminalis (Table 1). The sampling sites are shown in Figure 1. For each sample, total body weight in g (Wt, measured to the nearest 1 g) and standard length (SL, to the nearest 1 mm), eviscerated weight (EW, to the nearest 1 g), and gonad and liver weights (GW and LW, respectively, to the nearest 0.01 g) were measured. The sex and stage of sexual maturity were identified for all individuals based on the morphological characteristics, as described by Nikolsky (1963): I = immature, II = developing, III = maturing, IV = mature, V = spawning and post-spawning. The gonadosomatic index (GSI = 100 × GW/EW) was estimated as an indicator of the fish reproductive period. The hepatosomatic index (HSI = 100 × LW/EW) and fatness (K = 100 × Wt/SL3) were measured as bioenergetic indices to evaluate fish conditions.
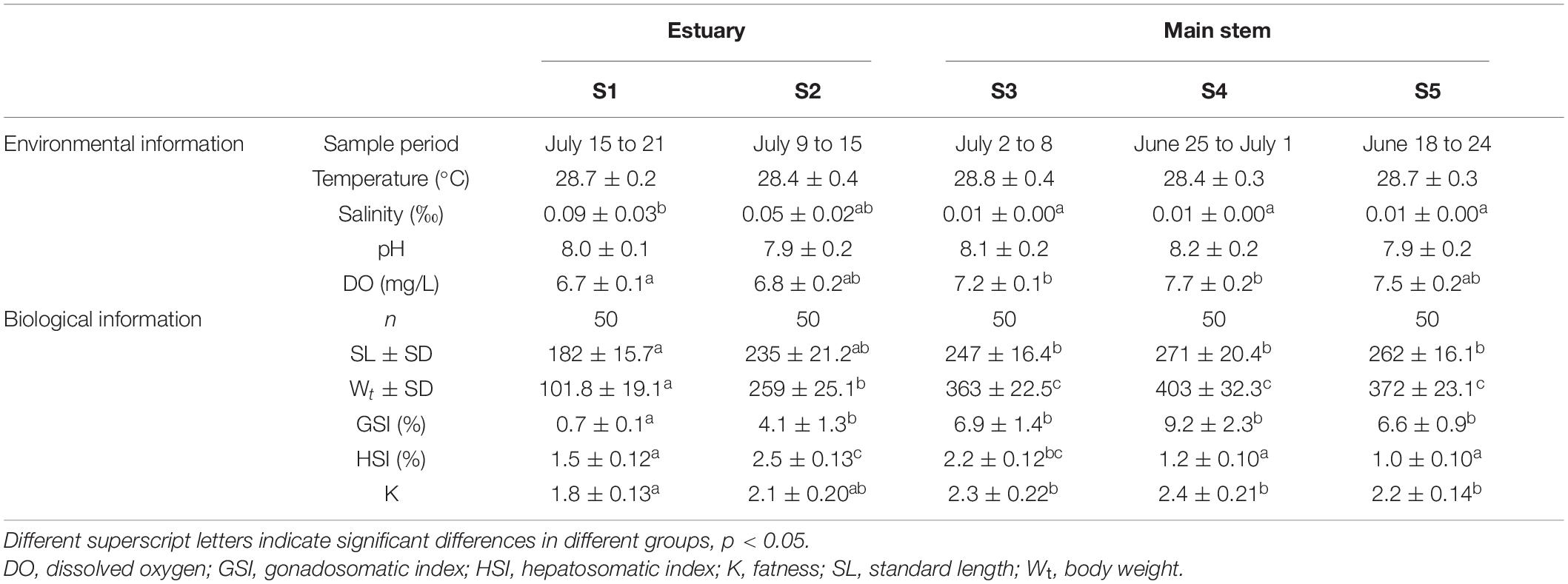
Table 1. Basic environmental information and biological information of gut microbial community pertaining for the different groups studied of Megalobrama terminalis.
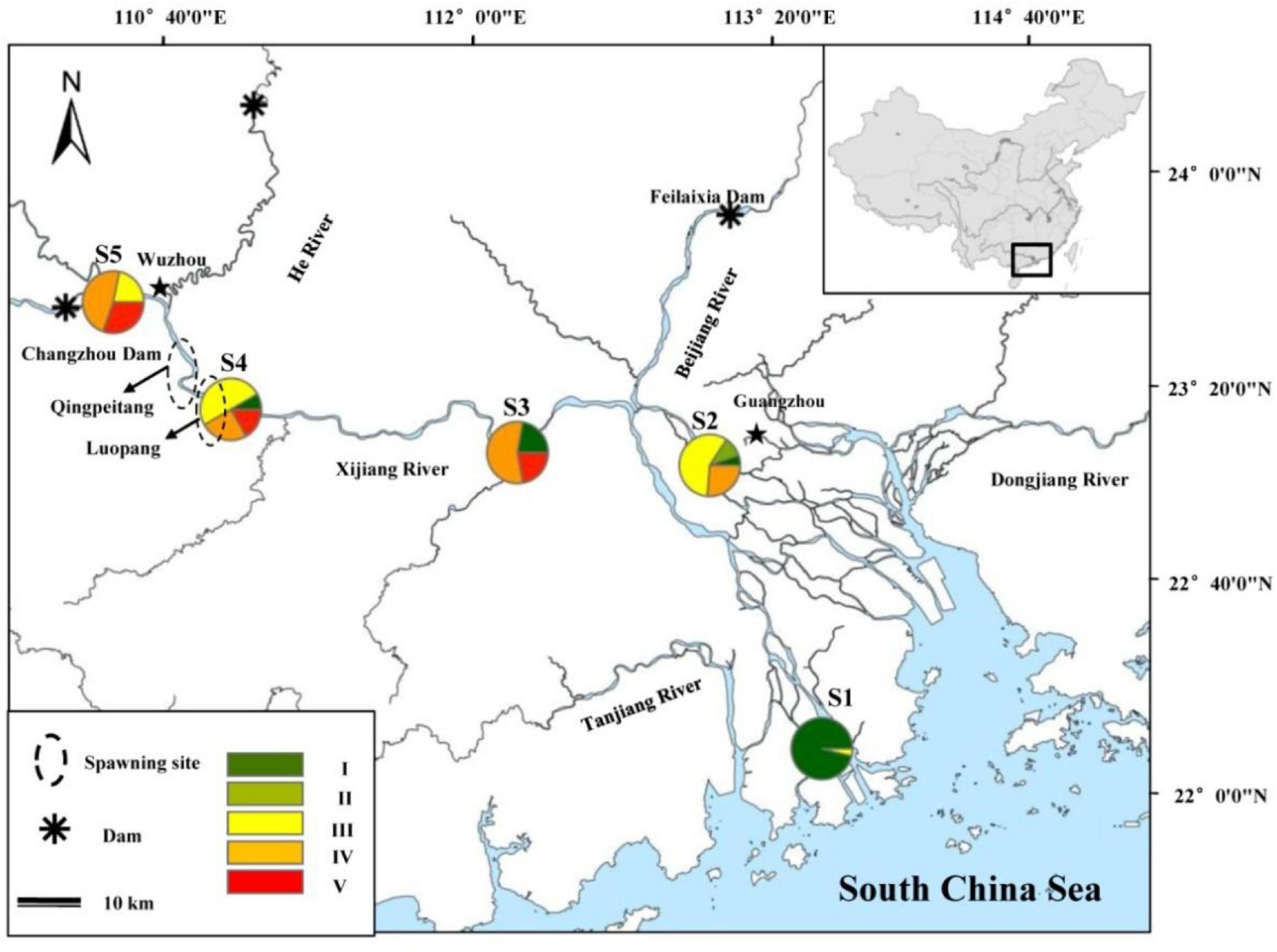
Figure 1. Sampling map showing the sample locations of Megalobrama terminalis. Rate of each ovary development stage of M. terminalis in sample sites was shown during spawning migration period. Black circles were shown as spawning ground of M. terminalis in Pearl River.
Histological Observations
Fish selected in different reproductive periods were anesthetized using MS-222 (0.2 g L–1 MS-222 + 0.2 g L–1 NaHCO3) and then stunned and quickly decapitated. For histological analysis, the left ovaries of fish were dissected out and fixed with Bouin’s fixative for 24 h at°C and embedded in paraffin wax, and then, the tissues were cut to 5-mm thicknesses sections and stained with hematoxylin–eosin (H&E).
Biochemical Assays
To investigate the fish biochemical composition and energy density, 120 fish tissue samples were randomly selected for analysis. Each specimen was dissected, and organs and muscles (without skin) were removed from a location posterior to the insertion of the dorsal fin. The liver and ovaries were taken from each fish stored in plastic bags on ice and frozen (–80°C) until processing. For biochemical analysis, each tissue of each sampled fish was homogenized and freeze-dried for 24 h at –80°C to a constant weight. The resulting dry tissue was cooled and weighed, and the moisture percentage was calculated as (100–%dry tissue). Determination of crude protein content was measured by the Kjeldahl method (Hach et al., 1985); determination of crude lipid content was measured by the chloroform–methanol extraction method (Folch et al., 1957); ash content was determined by the method of Penney and Moffitt (2014). The energy value was measured by a Phillipson Microbomb Calorimeter (Gentry Instruments Inc., Aiken, SC, United States). All biochemical analyses were performed in triplicate.
Statistical Analysis
The data were analyzed using STATISTICA 6.0 (StatSoft, Inc., Tulsa, OK, United States). The normality of the data and homogeneity of variance were assessed with the Kolmogorov–Smirnov test and Levene’s test, respectively. The GSI, HSI, fatness, composition, and energy density were analyzed by one-way ANOVA to evaluate the differences among groups. All data were expressed as means ± SD, and Tukey’s post-hoc test was used as necessary. A p-value below 0.05 was used to determine statistical significance. Principal component analysis (PCA) was used to obtain principal coordinates and their visualization from the complex biochemical composition of the ovary. To better understand the relationship between biochemical content of the fish ovary and the habitat environment and biological indices associated with different groups, a redundancy analysis (RDA) was conducted. Here, we used the R implementation of the procedure (version 1.1.3).
Results
Changes During Reproductive Migration
The biochemical composition changes in ovary of M. terminalis during the reproductive migration period are shown in Figure 1. The proportion of sexually mature (stages IV and V) individuals increased significantly in the main stem (S3, S4, and S5), whereas immature ovaries were dominant in the estuary (S1 and S2). The ovary of M. terminalis gradually matures during reproductive migration. Lipid constituents of the ovary of the black Amur bream rose from the ovary from S1 to S5, whereas water content showed an opposite pattern of variability (Supplementary Figure 1). To compare the similarity of proximate composition among different sample sites, PCA ordination revealed a clear separation of the proximate composition of the ovary between estuary and main stem populations. The proximate composition of ovary between the S1 and S2 groups on PCA scores showed greater similarity, separated from the proximate composition of the ovary in the S4 and S5 groups that formed a cluster (Figure 2A). Samples in the S3 group were located between non-migrating (S1, S2) and migrating populations (S4, S5) (Figure 2A). The proximate composition of ovary in each group was closely related to their habitat and biological index (Figure 2B). The proximate composition of S4 and S5 groups was more closely related to dissolved oxygen, fatness, and GSI. In contrast, the proximate composition of S1 and S2 was correlated with salinity and HSI.
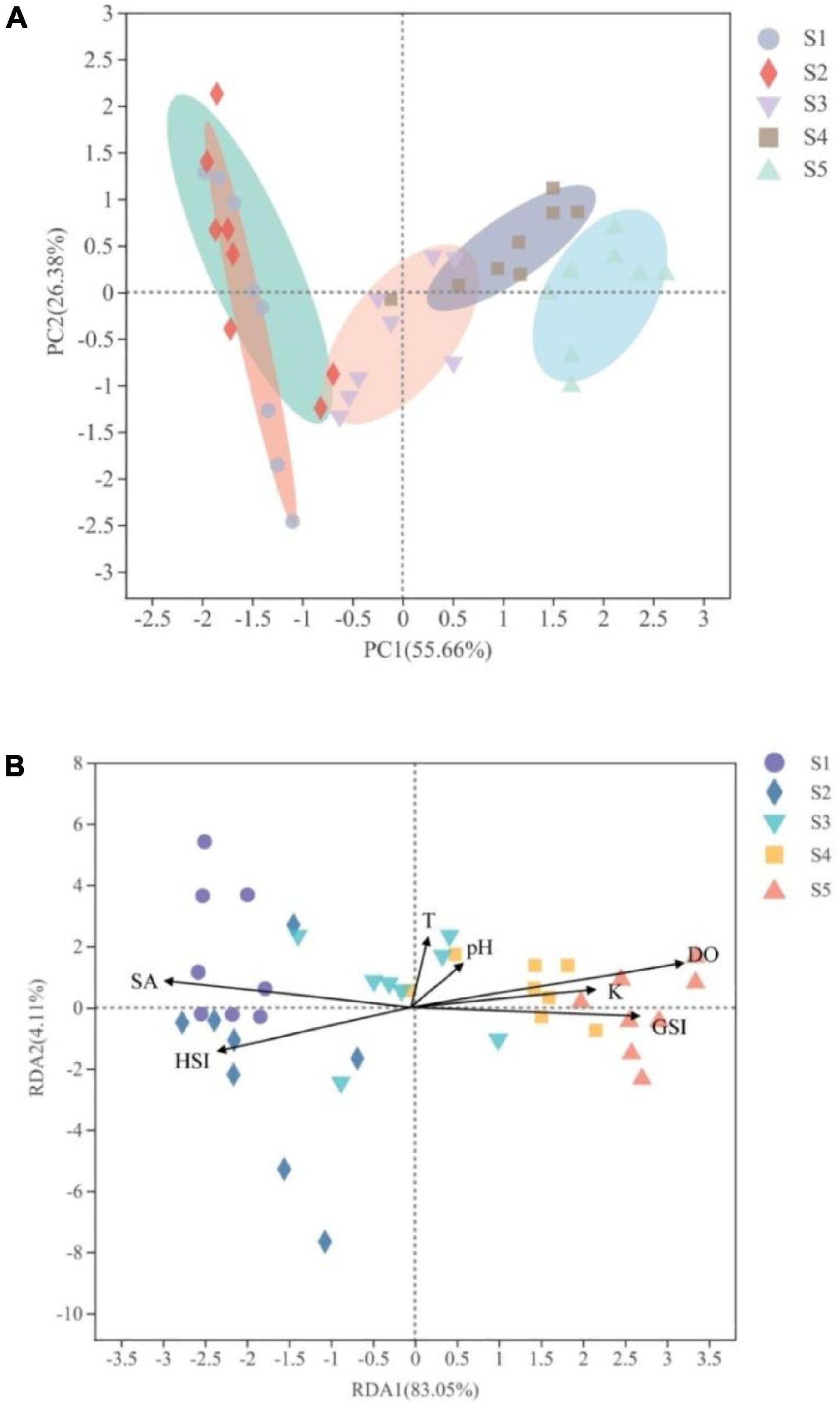
Figure 2. Comparison of the main biochemical compositions in the different groups studied of Megalobrama terminalis. (A) Principal coordinate analysis (PCA) of main biochemical compositions in M. terminalis ovary from different groups. The individual samples are color-coordinated according to the different locations. (B) Redundancy analysis (RDA) showed the correlation between the biochemical compositions of the different groups studied of M. terminalis and their biological indices and habitat environment factors.
Morphometry and Histology
The distribution of the morphometrical parameters across maturity stages is shown in Figure 3. It is clear that there were increases in length and weight from the immature stage to the mature stage (Figures 3A,B). Although this increase was gradual throughout the maturation for the somatic measurements, the GSI showed a sudden peak at stage IV (Figure 3C). However, HSI showed a peak at stage II and then gradually decreased (Figure 3D). Histological observations of the M. terminalis ovary during different developmental stages are shown in Figure 4. Oocytes varied markedly with ovary development, and the description of histological changes in M. terminalis is given shown in Table 2.
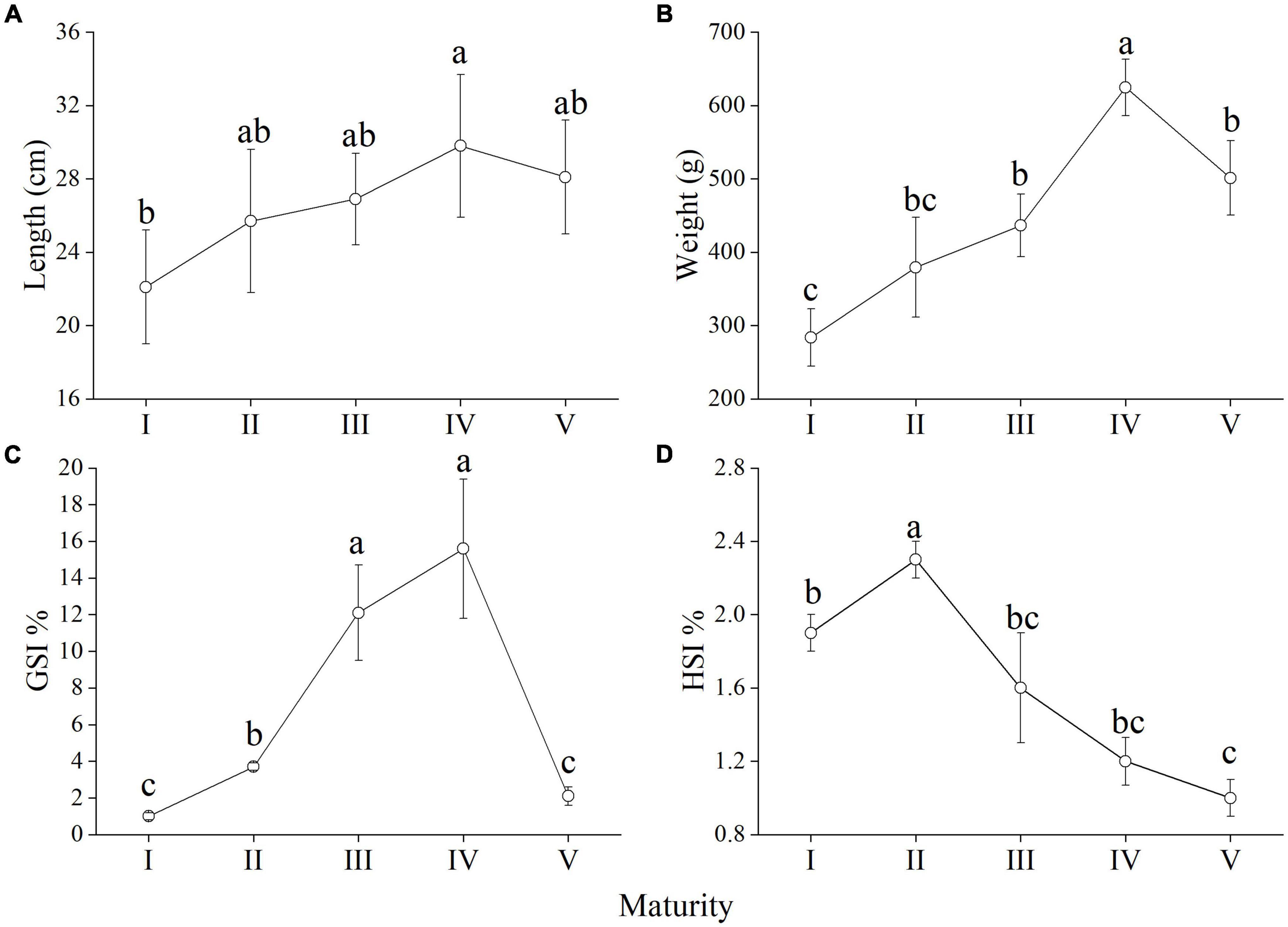
Figure 3. Line chart showing the distribution of (A) body length, (B) body length data across Megalobrama terminalis maturity stages. Shown are also the patterns in (C) gonadosomatic index (GSI), and (D) hepatosomatic index (HSI) of M. terminalis. Different superscript letters indicate significant differences of component in different maturity stage, p < 0.05.
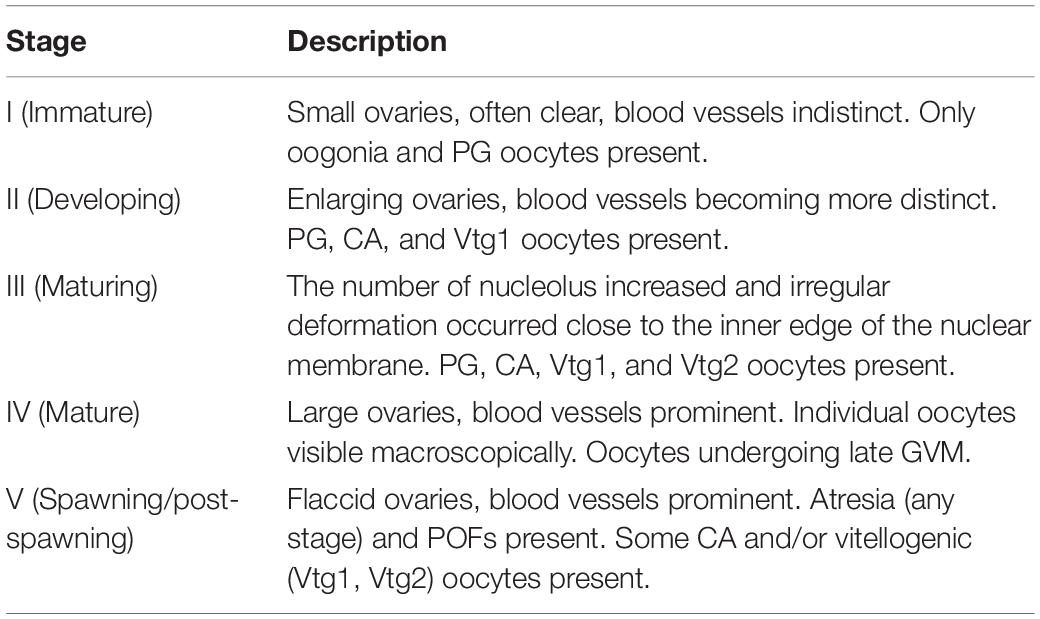
Table 2. Histological descriptions of the stages in the reproductive cycle of female Megalobrama terminalis.
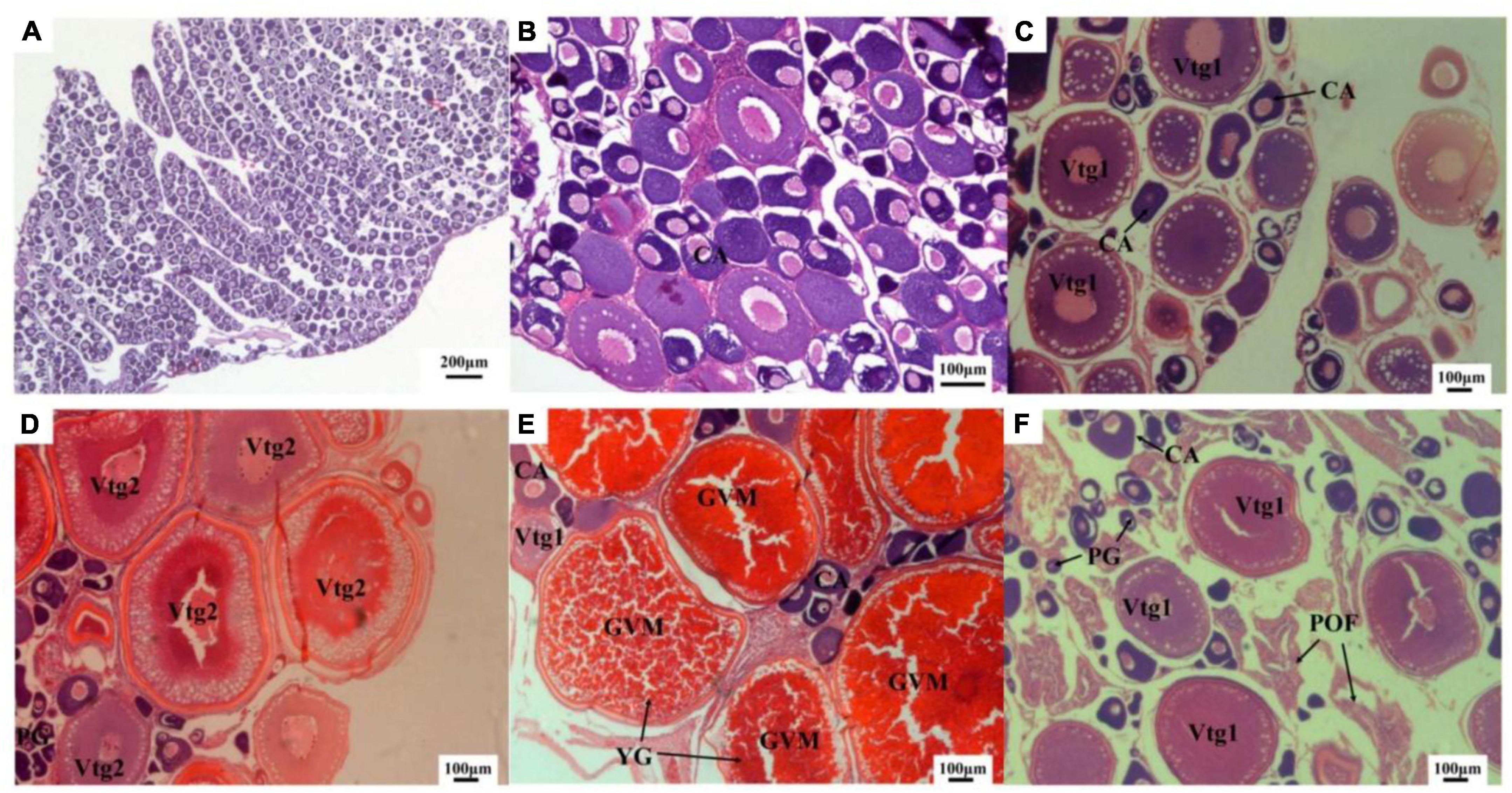
Figure 4. Transverse sections of ovary of the black Amur bream (A,B) immature female ovary; (C) developing female ovary; (D) maturing female ovary; (E) mature female ovary; (F) spawning and post-spawning female ovary; CA, cortical alveolar oocyte; GVM, germinal vesicle migration; PG, primary growth oocyte; POF, post-ovulatory follicle; Vtg1, primary vitellogenic oocyte; Vtg2, secondary vitellogenic oocyte.
Proximate Composition: Variability in Different Tissues
Biochemical changes across different maturity stages in each tissue of female M. terminalis are presented in Figure 5. During sexual maturation, water contents in the ovary and liver decreased from stages I to III and then increased from stages III to V (Figures 5A,C). In the contrast, water content in muscle had no obvious changes from stages I to V (Figure 5B). For total ash, a slight downward trend was observed in ovary tissues from stages I and II (Figure 5D), whereas muscle and liver tissues remained practically unaffected (Figures 5E,F). Concerning protein content, this constituent increased in the ovary from stages I to III and dropped significantly in stage IV, which shows an opposite pattern compared to water content in ovary tissue (Figure 5G). In the muscle tissue, total protein content declined from stages III to V, whereas less variation was observed in the liver tissue during maturation (Figures 5H,I). Lipid content in the ovary and liver gradually went up from stages I to IV and then dropped off significantly in spawning and post-spawning (stage V) (Figures 5J,L). Similarly, lipid content in the muscle tissues increased and subsequently decreased during maturation with a maximum value in stage III (Figure 5K).
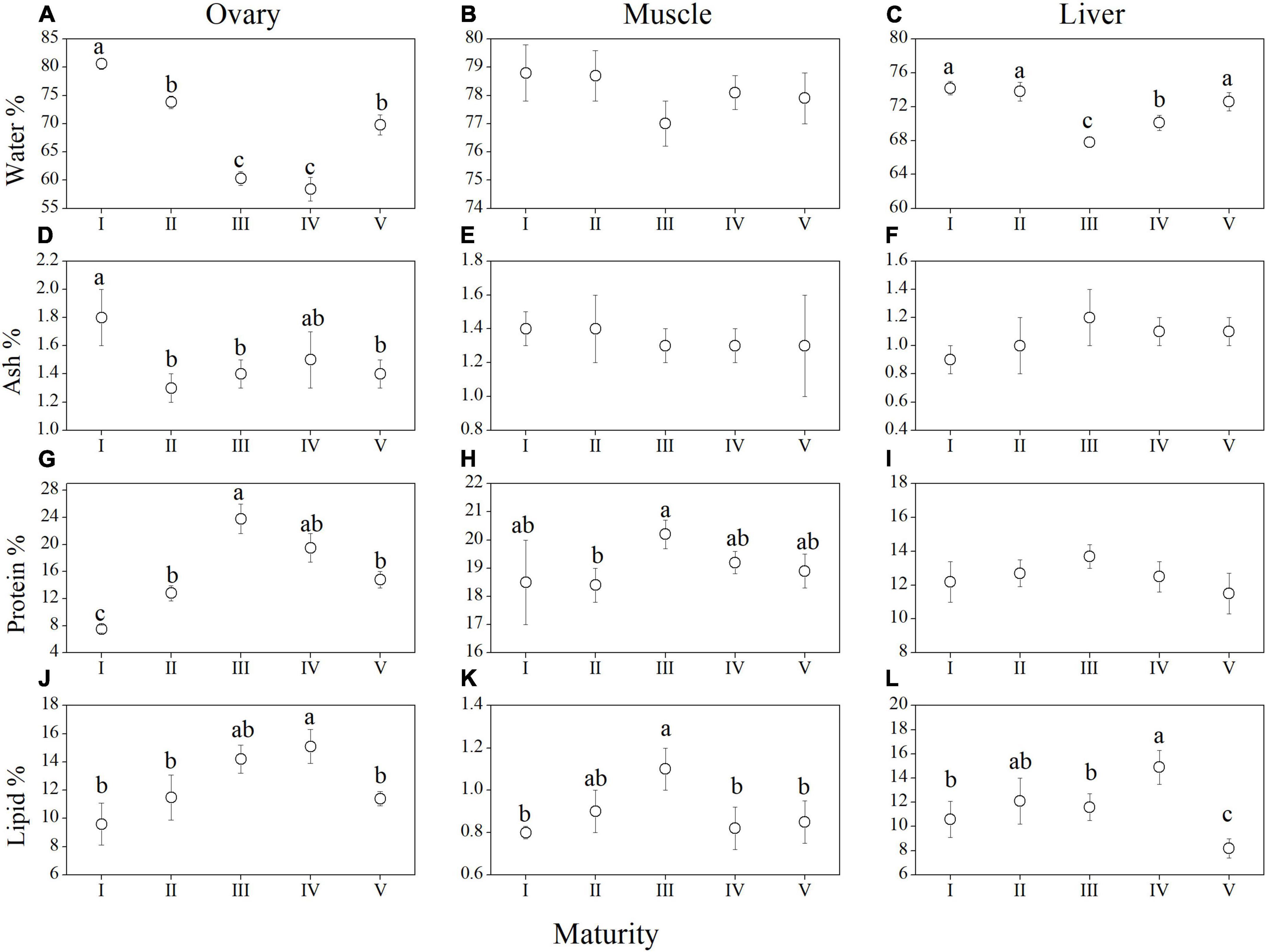
Figure 5. Four main biochemical constituent proportion of different tissues in female Megalobrama terminalis from maturity stages I to V. Water content in the ovary (A), in the muscle (B), and in the liver (C); ash content in the ovary (D), in the muscle (E), and in the liver (F); protein content in the ovary (G), in the muscle (H), and in the liver (I); lipid content in the ovary (J), in the muscle (K), and in the liver (L). Different superscript letters indicate significant differences of component in different maturity stage, p < 0.05.
Energy Accumulation and Allocation
The weights of trunk, ovary, and liver were positively correlated with body mass from stages III to IV (Figures 6A–C and Supplementary Table 1). The rate of increase of ovary mass rose sharply from stages III to IV, whereas the rate of increase of trunk mass declined. The proportions of protein and lipid in muscle were positively correlated (R2 = 0.152; p < 0.01) (Figure 6D), and the proportions of proteins and lipids were negatively related in the ovary and liver tissues from stages III to IV (Rovary2 = 0.312; Povary < 0.01; R2liver = 0.284; pliver < 0.01) (Figures 6E,F). The variation in energy density across maturity stages for each tissue is shown in Figures 7A–C. In the muscle, no significant change in energy density was perceived from stages I to V (Figure 7A). In contrast, there was a clear pattern related to reproduction, since maxima were observed in ovary and liver at female stage IV (Figures 7B,C), and there was a sharp drop reaching a minimum of energy density at stage V. Some statistically significant negative relationships were observed between ovary and muscle energy density from stages III to IV (R2 = 0.215; p < 0.01) (Figure 7D). The muscle energy density decreased rapidly, whereas the ovary increased slowly in stage III. In contrast, energy density of muscle dropped slightly and ovarian energy density rose dramatically in stage IV. Ovary and liver energy density showed a positive relationship (R2 = 0.246; p < 0.01) (Figure 7E). By contrast, muscle and liver energy density had a negative relationship from stages III to IV (R2 = 0.193; p < 0.05) (Figure 7F).
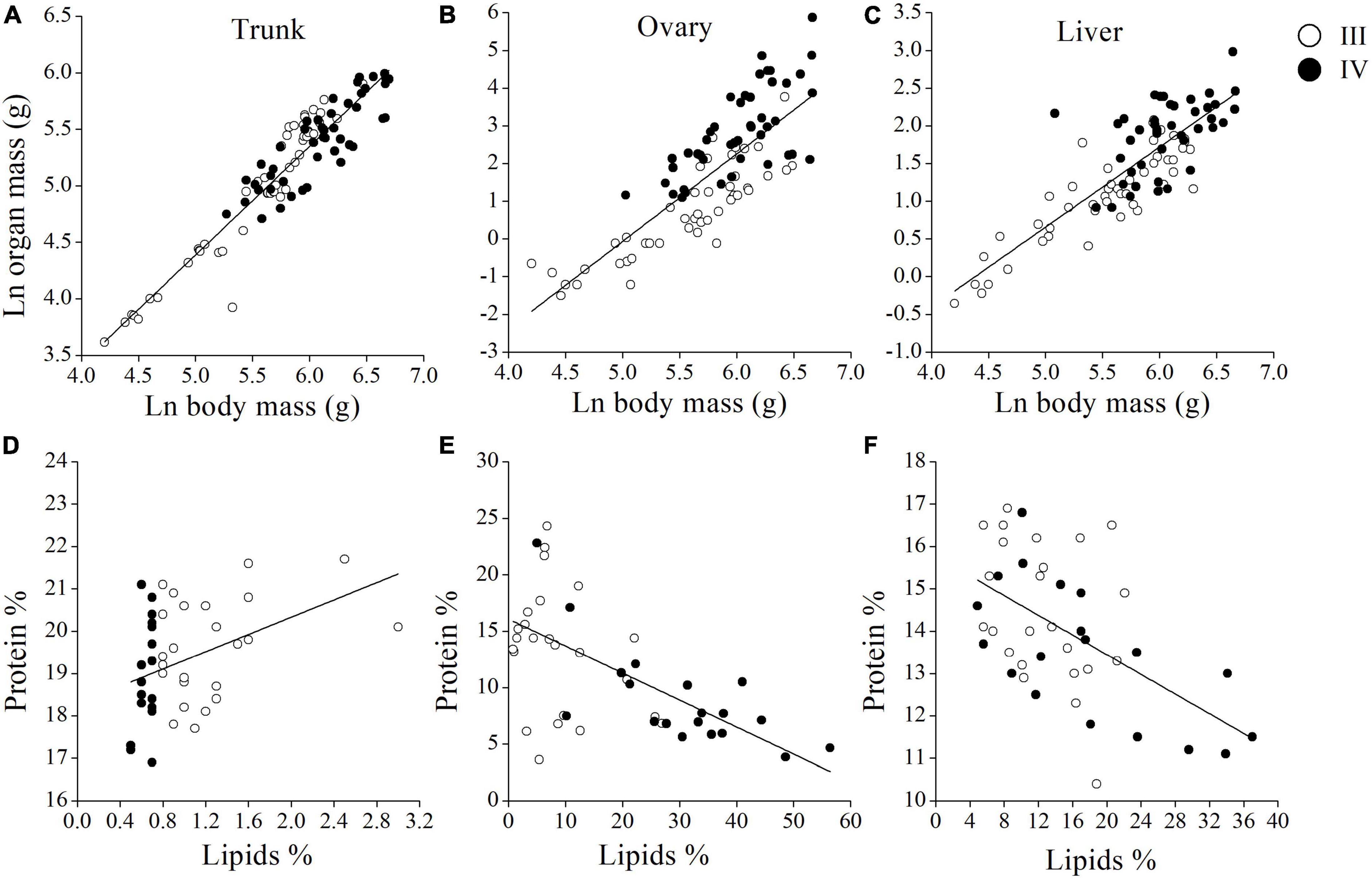
Figure 6. Scatter plots indicating the relationships between the organ and body mass for maturity stage III and IV of Megalobrama terminalis female in the trunk (A), in the ovary (B), and in the liver (C). Scatter plots showing the relationships between the proportion of total lipids and proteins for M. terminalis female in maturity stage III and IV in the muscle (D), in the ovary (E), and in the liver (F). Lines show regression fits with the exceptions.
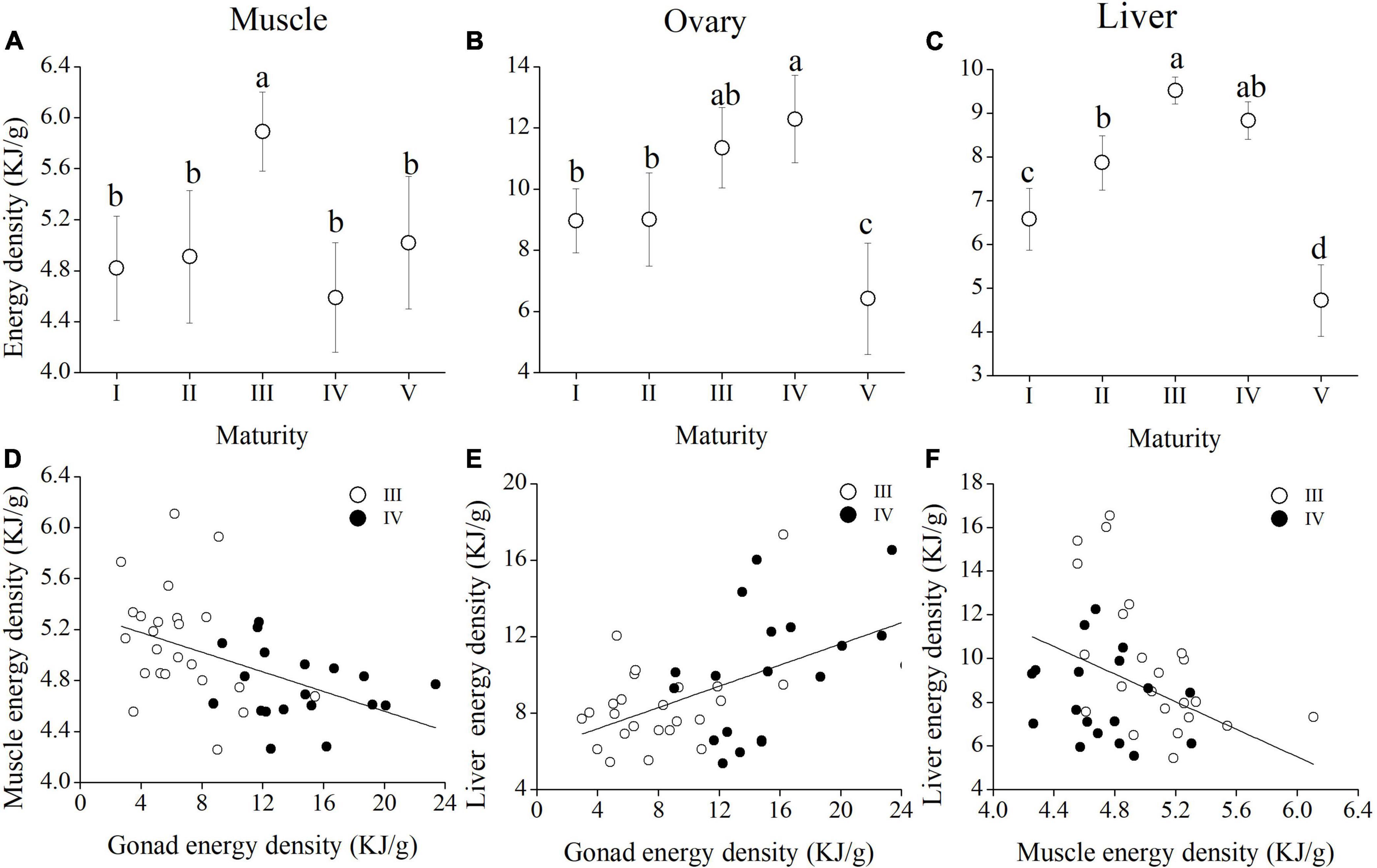
Figure 7. Changes in energy density across Megalobrama terminalis female maturity stages in the muscle (A), ovary (B), and liver (C). Scatter plots indicating the relationships of energy density in specific tissues [muscle (D), ovary (E), and liver (F) of M. terminalis female in maturity stages III and IV. Different superscript letters indicate significant differences of energy density in different maturity stages, p < 0.05.
Discussion
In this study, we found that the ovary of migrating black Amur bream gradually matured during movement from the estuary (fattening ground) to the main stem (spawning ground) during the breeding season (Figure 1). Maturity stages III to IV of the black Amur bream were observed as a vital migration-launching period. Meanwhile, significant differences in environmental factors and biological indices were observed between estuary and main stem populations, especially in salinity, dissolved oxygen, GSI, HSI, and fatness (Table 1). A clear separation of the proximate composition of the ovary was detected between estuary and main stem populations (Figure 2A). Secor (1999) indicated that not all individuals of migratory populations participated in the breeding migration. Non-migrating and migrating individuals demonstrated significant differences in maturity and energy reserves of fish reproductive organs. It is widely recognized that lipids and proteins are high energy substances for fish storage (Zaboukas et al., 2006; Sieiro et al., 2020). The recent study has illustrated that migratory individuals are evolving toward larger body size and higher fertility than non-migratory individuals (Burns and Bloom, 2020). In this study, we found that migrating population in the main stem had larger body size and higher GSI than that of non-migrating population (Table 1). The proximate composition of ovary in the groups S4 and S5 had a close relationship with biological indices, especially in fatness and GSI (Figure 2B). Lipid content in the ovary of the black Amur bream gradually increased from the estuary to the main stem, which indicates that migrating population stored more energy for ovary development. The high lipid content of tissues also signified the need for high energy biomolecules to perform the energy-consuming locomotion (Zaboukas et al., 2006). Relevant studies have pointed out that fish utilize lipids to regulate their energy expenditure for gonad development (Takama et al., 1985). Thus, the remarkable change in lipid content of the ovary might be considered as a crucial physiological index, which reflects the readiness for breeding migration of M. terminalis.
In order to make an effective assessment of M. terminalis ovary development, it is imperative to describe the process of energy accumulation and oocyte recruitment during ovary development. According to our study, it appears that M. terminalis follows a similar pattern of oocyte developmental stages as in most one-time spawning type fish species (Murua and Saborido–Rey, 2003; Costa et al., 2015). The variation tendon of the proximate composition in muscle and ovary tissues was due to increases in the protein content from stages I to III (Figures 5G,H). The protein content is essential to maintaining major body organic structure and function of migrating fish during reproductive migration (Lin et al., 2017; Serrat et al., 2019). In addition, somatic tissue is regarded as primarily location of fish energy reserves, and this is commonly determined by the protein content throughout reproduction (Lin et al., 2019). Lipid content in the ovary gradually increased during the migration-launching period, whereas that in the muscle slightly declined. This indicated that energetic investment of M. terminalis concentrated on reproduction, whereas there was a more intense reduction in the lipid contents of the somatic tissues (Zaboukas et al., 2006). Furthermore, decreases in the lipid contents of fish somatic tissues are mainly devoted to supplying energy for reproductive migration (Doucett et al., 1999; Kiessling et al., 2004). During the migration-launching period, the accumulation of lipids in the ovary proceeds until the onset of vitellogenesis; this is interpreted as initiation of the synthesis of yolk-forming molecules. The production of abundant eggs during the relatively short period of reproduction is very energy intensive (Tocher, 2003). In the course of this period, the proportions of protein and lipid in the ovary showed a negative correlation, which suggests that lipids were more important than proteins in the M. terminalis ovary during advanced maturity stages. Studies present have shown that lipids are important in the ovaries of marine fish during maturity stages (Rosa et al., 2002; Serrat et al., 2019). Related research reveals that lipids predominate in the liver and ovary in the common octopus (Sieiro et al., 2006). Despite lipid content of somatic and reproductive tissue being of vital importance, the lipid content in tissues of M. terminalis rose to a certain level and remained stable instead of increasing blindly during the migratory reproductive preparation period (stage III). Relevant research has indicated that excessive lipids limited fish swimming speed during migration (Slotte et al., 2000). Consequently, lipids may have diverse effects in different tissues of the black Amur bream, and there may be a regulatory mechanism for allocating the lipids to each tissue to reduce the risk of migration failure in the breeding migratory preparation period.
This study demonstrated that ovary mass gain is accelerated, while somatic mass grows more slowly during the vital migration-launching period. Relevant studies have indicated that the relationship between organ and body size can be applied to explain the allometric variation at the metabolic level (Oikawa and Itazawa, 1992). Some studies have manifested that the somatic tissues accumulated energy continuously until the physiologically maturing stage, whereas the reproductive organs had a drastic energy accumulation throughout the sexual maturation period (Lahti et al., 2001, 2002). The energy density in M. terminalis somatic tissues increased initially after declining owing to fluctuating contents of total protein and lipid during the maturation process. More specifically, the rise in the energy density of somatic tissues was due to the body growth of M. terminalis from stages I to III. Rapid body growth in fish results from intensive protein synthesis and low protein degradation (Houlihan et al., 1990; Sieiro et al., 2020). Owing to the high level of protein in the somatic tissues, the energy metabolism is mainly protein-based (Navarro et al., 2014). However, a negative energy relationship between somatic and reproductive tissues was observed in M. terminalis during the vital migration-launching period. The downtrend of energy density in somatic tissues after stage III was related to the decline of lipid content. The previous research has suggested that somatic tissues of migrating fish are applied to provide energy for the migration process (Kiessling et al., 2004). Moreover, lipids are crucial energy substances with high energy efficiency in fish somatic tissues (Hinch et al., 2002). Higher variability of energy density in the ovary and liver was observed during a vital migration-launching phase due to enhanced lipid contents. This ascent of energy density in ovary associated with yolk accumulation in stage IV has been found in some fish species (Fernández et al., 2009; Wu et al., 2017). Specifically, rapid synthesis of yolk materials in the developing oocytes during the migration-launching phase is conducive to the energy substances being rapidly synthesized in the later physiological maturity stages (Alonso-Fernández and Saborido-Rey, 2012; Lin et al., 2017). Not all individuals in migratory fish populations participate in the migration (Secor, 1999). Non-migrating and migrating individuals presented obvious discrepancies in body size and lipid storage status. Our previous results suggested that lipid metabolism in migrating population was higher than that in non-migrating population, which provides a line of evidence for that M. terminalis consuming lipids to supply energy for spawning migration (Liu et al., 2021a). Similarly, it has been proposed that Atlantic salmon maturation was linked with growth rate and lipid metabolism (Herbinger and Friars, 1991). Barneche et al. (2018) revealed that larger females disproportionately reproduce more than smaller females whether measured by fecundity or by total reproductive energy. Larger females had a higher metabolic ability factor that is significant for fish survival, energy consumption, and migration (Priede, 1985; Hinch and Rand, 2000). The higher metabolic ability requires a more complex organizational structure, which inevitably leads to higher energy consumption and lower energy efficiency (Weiner, 1993; Chappell et al., 1999; Hinch and Rand, 2000). In fact, energy accumulation is closely linked to the co-evolved life-history traits favored for optimal energetic allocation (Lin et al., 2017). Therefore, there may be a trade-off between maximum metabolic ability and energy efficiency before migration launching in the black Amur bream.
Conclusion
Above all, stages III to IV of the black Amur bream were observed as a crucial migration-launching period. The asynchrony of development was confirmed in energetic relationships in somatic and ovary tissues. In addition, the negative energy relationship between somatic and reproductive tissues clarified a trade-off between maximum metabolic ability and energy efficiency before the migration launching of M. terminalis. The lipid content displayed diverse effects in different tissues of M. terminalis, and there was a regulatory mechanism for allocating the lipid content of each tissue reasonably to reduce the risk of migration failure during the breeding migratory preparation period. The results demonstrated that there is a dependency between somatic and reproductive tissue growth in M. terminalis females during migratory preparation that serves to improve fitness. Finally, these findings illustrate that the high energy metabolism demands of reproduction of M. terminalis are regarded as a key factor driving the variation in energy accumulation and allocation. The findings facilitate further research on ecological adaptations of migrating fish during the reproductive cycle.
Data Availability Statement
The original contributions presented in the study are included in the article/Supplementary Material, further inquiries can be directed to the corresponding author.
Ethics Statement
The methods involving animals in this study were conducted in accordance with the Laboratory Animal Management Principles of China. All experimental protocols were approved by the Ethics Committee of the Pearl River Fisheries Research Institute, Chinese Academy of Fishery Sciences. Written informed consent was obtained from the owners for the participation of their animals in this study.
Author Contributions
YaL: conceptualization, data curation, and writing–original draft. XL: funding acquisition. JL: funding acquisition and supervision. YuL: formal analysis, writing, reviewing, and editing. All authors contributed to the article and approved the submitted version.
Funding
This study was funded by Guangdong Basic and Applied Basic Research Foundation, grant number: 2019B1515120064; National Key R&D Program of China, grant numbers: 2018YFD0900902 and 2018YFD0900903; Open Fund of Key Lab of Freshwater Biodiversity Conservation, Ministry of Agriculture and Rural Affairs of China, grant number: LFBC1006; Open Fund Project of Fishery Resources and Environmental Science Experimental Station of The Upper-Middle Reaches of Yangtze River Ministry of Agriculture, grant number: 0202020017.
Conflict of Interest
The authors declare that the research was conducted in the absence of any commercial or financial relationships that could be construed as a potential conflict of interest.
Publisher’s Note
All claims expressed in this article are solely those of the authors and do not necessarily represent those of their affiliated organizations, or those of the publisher, the editors and the reviewers. Any product that may be evaluated in this article, or claim that may be made by its manufacturer, is not guaranteed or endorsed by the publisher.
Acknowledgments
We are grateful to Weitao Chen and Shuli Zhu for assistances in collecting specimens. We thank LetPub (www.letpub.com) for its linguistic assistance during the preparation of this manuscript.
Supplementary Material
The Supplementary Material for this article can be found online at: https://www.frontiersin.org/articles/10.3389/fevo.2022.848228/full#supplementary-material
References
Alonso-Fernández, A., and Saborido-Rey, F. (2012). Relationship between energy allocation and reproductive strategy in Trisopterus luscus. J. Exp. Mar. Biol. Ecol. 416–417, 8–16. doi: 10.1016/j.jembe.2012.02.001
Barbour, S. E. (1985). Variation in Life History, Ecology and Resource Utilization by Arctic charr (Salvelinus alpinus L.) in Scotland. [Ph.D thesis]. Edinburgh: University of Edinburgh.
Barneche, D. R., Robertson, D. R., White, C. R., and Marshall, D. J. (2018). Fish reproductive–energy output increases disproportionately with body size. Science 360, 642–645. doi: 10.1126/science.aao6868
Bureau, D., Colombier, S. B. D., Bolliet, V., Lambert, P., and Bardonnet, A. (2007). Energy and migratory behavior in glass eels (Anguilla Anguilla). Physiol. Behav. 92, 684–690.
Burns, M. D., and Bloom, D. D. (2020). Migratory lineages rapidly evolve larger body sizes than non- migratory relatives in ray- finned fishes. Proc. Royal Soc. B 287:20192615. doi: 10.1098/rspb.2019.2615
Caudill, C. C., Daigle, W. R., Keefer, M. L., Boggs, C. T., Jepson, M. A., Burke, B. J., et al. (2007). Slow dam passage in adult Columbia River salmonids associated with unsuccessful migration: delayed negative effects of passage obstacles or condition–dependent mortality? Can. J Fish Aquat. Sci. 64, 979–995. doi: 10.1139/f07-065
Chappell, M., Bech, C., and Buttemer, W. (1999). The relationship of central and peripheral organ masses to aerobic performance variation in house sparrows. J. Exp. Biol. 202, 2269–2279. doi: 10.1242/jeb.202.17.2269
Chen, W., Li, C., Chen, F., Li, Y., Yang, J., Li, J., et al. (2020). Phylogeographic analyses of a migratory freshwater fish (Megalobrama terminalis) reveal a shallow genetic structure and pronounced effects of sea-level changes. Gene 737:144478. doi: 10.1016/j.gene.2020.144478
Colombier, S. B. D., Bolliet, V., Lambert, P., and Bardonnet, A. (2007). Energy and migratory behavior in glass eels (Anguilla anguilla). Physiol. Behav. 92, 684–690. doi: 10.1016/j.physbeh.2007.05.013
Costa, E. F., Dias, J. F., and Murua, H. (2015). Reproductive strategy and fecundity of the keystone species Paralonchurus brasiliensis (Teleostei, Sciaenidae): an image processing techniques application. Environ. Biol. Fish. 98, 2093–2108. doi: 10.1007/s10641-015-0432-2
Doucett, R. R., Booth, R. K., Power, G., and Mckinley, R. S. (1999). Effects of the spawning migration on the nutritional status of anadromous Atlantic salmon (Salmo salar): insights from stable isotope analysis. Can. J. Fish. Aquat. Sci. 56, 2172–2180. doi: 10.1139/f99-147
Fernández, D. A., Lattuca, M. E., Boy, C. C., Pérez, A. F., Ceballos, S. G., Vanella, F. A., et al. (2009). Energy density of sub–Antarctic fishes from the Beagle Channel. Fish. Physiol. Biochem. 35, 181–188. doi: 10.1007/s10695-008-9234-1
Folch, J. M., Lees, M., and Sloane–Stanley, G. H. (1957). A simple method for the isolation and purification of total lipid from animal tissue. J. Biol. Chem. A 226, 497–509. doi: 10.1016/s0021-9258(18)64849-5
Hach, C. C., Scott, V. B., and Kopelove, A. B. (1985). A powerful Kjeldahl nitrogen method using peroxymonosulfuric acid. J. Agricul. Food. Chem. 33, 1117–1123. doi: 10.1021/jf00066a025
Herbinger, C. M., and Friars, G. W. (1991). Correlation between condition factor and total lipid content in Atlantic salmon, salmo salar l.parr. Aquac. Fish. Mana. 22, 527–529. doi: 10.1111/j.1365-2109.1991.tb00766.x
Hinch, S. G., and Rand, P. S. (2000). Optimal swim speeds and forward–assisted propulsion: energy–conserving behaviours of upriver–migrating adult salmon. Can. J. Fish. Aquat. Sci. 57, 2470–2478. doi: 10.1139/f00-238
Hinch, S. G., Standen Emily, M., Healey Michael, C., and Farrell Anthony, P. (2002). Swimming patterns and behaviour of upriver-migrating adult pink (Oncorhynchus gorbuscha) and sockeye (O. nerka) salmon as assessed by EMG telemetry in the Fraser River, British Columbia, Canada. Hydrobiologia 483, 147–160. doi: 10.1007/978-94-017-0771-8_17
Houlihan, D. F., McMillan, D. N., Agnisola, C., Genoino, I. T., and Foti, L. (1990). Protein synthesis and growth in Octopus vulgaris. Mar. Biol. 106, 251–259.
Jonsson, N., and Jonsson, B. (2003). Energy allocation among developmental stages, age groups, and types of Atlantic salmon (Salmo salar) spawners. Can. J Fish Aquat. Sci. 60, 506–516. doi: 10.1139/f03-042
Kiessling, A., Lindahl-Kiessling, K., and Kiessling, K. (2004). Energy utilization and metabolism in spawning migrating early start sockeye salmon (Oncorhynchus nerka): the migratory paradox. Can. J. Fish. Aquat. Sci. 61, 452–465. doi: 10.1139/f04-006
Lahti, K., Huusknen, H., Laurila, A., and Piironen, J. (2002). Metabolic rate and aggressiveness between brown trout populations. Func. Ecol. 16, 167–174. doi: 10.1046/j.1365-2435.2002.00618.x
Lahti, K., Laurila, A., Enberg, K., and Piironen, J. (2001). Variation in aggressive behaviour and growth rate between populations and migratory forms in the brown trout, Salmo trutta. Anim. Behav. 62, 935–944. doi: 10.1006/anbe.2001.1821
Lennox, R. J., Paukert, C. P., Aarestrup, K., Auger-Méthé, M., Baumgartner, L., Birnie-Gauvin, K., et al. (2019). One Hundred Pressing Questions on the Future of Global Fish Migration Science, Conservation, and Policy. Front. Ecol. Evol. 7:286. doi: 10.3389/fevo.2019.00286
Li, Y., Li, C., Zhu, S., Yang, J., Xia, Y., and Li, X. (2018). Exploitation status of Megalobrama terminalis based on analysis of SBR and YPR models in Xijiang river. Acta Hydrobiol. Sin. 42, 975–983.
Li, Y., Li, X., Yang, J., Sovan, L., Shuai, F., and Li, J. (2014). Effect of Pearl River closed fishing on Megalobrama terminalis recruitment stock. J. Fish. China. 38, 503–509.
Lin, D., Chen, X., Wei, Y., and Chen, Y. (2017). The energy accumulation of somatic tissue and reproductive organs in post–recruit female Illex argentines and the relationship with sea surface oceanography. Fish. Res. 185, 102–114. doi: 10.1016/j.fishres.2016.09.023
Lin, D., Han, F., Xuan, S., and Chen, X. (2019). Fatty acid composition and the evidence for mixed income–capital breeding in female Argentinean short–fin squid Illex argentinus. Mar. Biol. 166, 90.
Liu, Y., Chen, W., Li, Y., Li, J., and Li, X. (2020). Growth and ontogenetic development of digestive functionality in black Amur bream (Megalobrama terminalis). Aquac. Res. 51, 3593–3601. doi: 10.1111/are.14697
Liu, Y., Li, X., Li, Y., Li, J., and Zhu, S. (2021b). Reproductive biology and strategy of black Amur bream (Megalobrama terminalis) of Xijiang River. J. Lake Sci. 33, 232–241. doi: 10.18307/2021.0117
Liu, Y., Li, X., Li, J., and Chen, W. (2021a). The gut microbiome composition and degradation enzymes activity of black Amur bream (Megalobrama terminalis) in response to breeding migratory behavior. Ecol. Evol. 11, 5150–5163. doi: 10.1002/ece3.7407
McCormick, S. D., Hansen, L. P., Quinn, T. P., and Saunders, R. L. (1998). Movement, migration, and smolting of Atlantic salmon (Salmo salar). Can. J. Fish. Aquat. Sci. 55, 77–92. doi: 10.1139/d98-011
Mourente, G., Megina, C., and Díaz-Salvago, E. (2002). Lipids in female northern bluefin tuna (Thunnus thynnus thynnus L.) during sexual maturation. Fish. Physiol. Biochem. 24, 351–363.
Murua, H., and Saborido–Rey, F. (2003). Female reproductive strategies of marine fish of the North Atlantic. J. Northw. Atl. Fish. Sci 33, 23–31. doi: 10.2960/j.v33.a2
Navarro, J. C., Monroig, Ó, and Sykes, A. V. (2014). “Nutrition as a key factor for cephalopod aquaculture,” in Cephalopod Culture, eds J. Iglesias, L. Fuentes, and R. Villanueva (Netherlands: Springer), 77–95. doi: 10.1007/978-94-017-8648-5_5
Oikawa, S., and Itazawa, Y. (1992). Relationship between metabolic rate in vitro and body mass in a marine teleost, porgy pagrus major. Fish Physiol. Biochem. 10, 177–182. doi: 10.1007/BF00004511
Penney, Z. L., and Moffitt, C. M. (2014). Proximate Composition and Energy Density of Stream-Maturing Adult Steelhead during Upstream Migration, Sexual Maturity, and Kelt Emigration. Tran. Am. Fish. Soc. 143, 399–413. doi: 10.1080/00028487.2013.862184
Priede, I. (1985). “Metabolic scope in fishes,” in Fish Energetics, eds Tytler P. Calow (Dordrecht: Springer), 33–64. doi: 10.1007/978-94-011-7918-8_2
Roff, D. A. (1983). An allocation model of growth and reproduction in fish. Can. J. Fish. Aquat. Sci. 40, 1395–1404. doi: 10.1139/f83-161
Rosa, R., Nunes, M. L., and Sousa Reis, C. (2002). Seasonal changes in the biochemical composition of Octopus vulgaris, Cuvier, 1797, from three areas of the Portuguese coast. Bull. Mar. Sci. 71, 739–751.
Saborido–Rey, F., Garabana, D., Stransky, C., Melnikov, S., and Shibanov, V. (2004). Review of the population structure and ecology of S. mentella in the Irminger sea and adjacent waters. Rev. Fish Biol. Fish. 14, 455–479. doi: 10.1007/s11160-005-3585-9
Secor, D. H. (1999). Specifying divergent migrations in the concept of stock: the contingent hypothesis. Fish. Res. 43, 13–34. doi: 10.1016/s0165-7836(99)00064-8
Serrat, A., Saborido-Rey, F., García Fernández, C., Muñoz Frigola, M., Lloret Romañach, J., Thorsen, A., et al. (2019). New insights in oocyte dynamics shed light on the complexities associated with fish reproductive strategies. Sci. Rep. 9:18411. doi: 10.1038/s41598-019-54672-3
Sieiro, P., Aubourg, S. P., and Rocha, F. (2006). Seasonal study of the lipid composition in different tissues of the common octopus (Octopus vulgaris). Eur. J. Lipid Sci. Tech. 108, 479–487. doi: 10.1002/ejlt.200500322
Sieiro, P., Otero, J., and Aubourg, S. P. (2020). Biochemical Composition and Energy Strategy Along the Reproductive Cycle of Female Octopus vulgaris in Galician Waters (NW Spain). Front. Physiol. 11:760. doi: 10.3389/fphys.2020.00760
Slotte, A., Johannessen, A., and Kjesbu, O. S. (2000). Effects of fish size on spawning time in Norwegian spring-spawning herring. J. Fish Biol. 56, 295–310. doi: 10.1111/j.1095-8649.2000.tb02107.x
Takama, K., Love, R. M., and Smith, G. L. (1985). Selectivity in mobilisation of stored fatty acids by maturing cod, Gadus morhua L. Comp. Biochem. Physiol. B. 80, 713–718. doi: 10.1016/0305-0491(85)90450-x
Tamario, C., Sunde, J., Petersson, E., Tibblin, P., and Forsman, A. (2019). Ecological and Evolutionary Consequences of Environmental Change and Management Actions for Migrating Fish. Front. Ecol. Evol. 7:271. doi: 10.3389/fevo.2019.00271
Tan, X., Li, X., Lin, J., Zhou, D., Gao, X., and Li, J. (2009). Ecological differentiation between two breeding populations of Megalobrama terminalis in the Pearl River based on hydro–acoustic research. Acta Ecol. Sin. 29, 1756–1762.
Thomas, A., and Johan, B. (2018). Ecology of animal migration. Cur. Biol. 28, 968–972. doi: 10.1016/j.cub.2018.04.043
Thorpe, J. E., Mangel, M., Metcalfe, N. B., and Huntingford, F. A. (1998). Modelling the proximate basis of salmonid life-history variation, with application to Atlantic salmon, Salmo salar L. Evol. Ecol. 12, 581–599. doi: 10.1023/a:1022351814644
Tocher, D. (2003). Metabolism and functions of lipids and fatty acids in teleost fish. Rev. Fish. Sci. 11, 107–184. doi: 10.1080/713610925
Villegas-Ríos, D., Alonso-Fernández, A., Domínguez-Petit, R., and Saborido-Rey, F. (2014). Energy allocation and reproductive investment in a temperate protogynous hermaphrodite, the ballan wrasse Labrus bergylta. J. Sea Res. 86, 76–85. doi: 10.1016/j.seares.2013.11.010
Wang, C., Li, X. H., Lai, Z. N., Tan, X., Li, J., Li, Y., et al. (2010). The study on community structure difference of phytoplankton in two Megalobrama terminalis spawning grounds of Xijiang River. Guan. Agri. Sci. 37, 156–160.
Weiner, J. (1993). Physiological limits to sustainable energy budgets in birds and mammals: ecological implications. Trends Ecol Evol. 7, 384–388. doi: 10.1016/0169-5347(92)90009-Z
Wu, L., Tang, W., and Zhang, Y. (2017). Research on the differences of anadromous migratory distance between Coilia mystus and Coilia nasus based on the transfer process of body lipid. J. Fish. Chin. 41, 212–220.
Wysujack, K., Greenberg, L. A., Bergman, E., and Olsson, I. C. (2010). The role of the environment in partial migration: food availability affects the adoption of a migratory tactic in brown trout Salmo trutta. Ecol. Freshw. Fish. 18, 52–59. doi: 10.1111/j.1600-0633.2008.00322.x
Xia, Y., Li, J., Li, Y., Zhu, S., Huang, Y., Wu, Z., et al. (2017). Small–subunit ribosomal DNA sequencing analysis of dietary shifts during gonad maturation in wild black Amur bream (Megalobrama terminalis) in the lower reaches of the Pearl River. Fish. Sci. 83, 955–965. doi: 10.1007/s12562-017-1123-z
Xia, Y., Li, Y., Zhu, S., Li, J., Li, S., and Li, X. (2020). Individual dietary specialization reduces intraspecific competition, rather than feeding activity, in black amur bream (Megalobrama terminalis). Sci. rep. 10:17961. doi: 10.1038/s41598-020-74997-8
Keywords: reproduction, energy allocation, migration, Megalobrama terminalis, proximate composition
Citation: Liu Y, Li X, Li J and Li Y (2022) Insights Into Energy Accumulation and Allocation Strategy of Reproductive Migration of Black Amur Bream (Megalobrama terminalis) in the Pearl River Basin, China. Front. Ecol. Evol. 10:848228. doi: 10.3389/fevo.2022.848228
Received: 04 January 2022; Accepted: 25 February 2022;
Published: 26 April 2022.
Edited by:
Chuanbo Guo, Institute of Hydrobiology (CAS), ChinaReviewed by:
Xiuming Li, Chongqing Normal University, ChinaXu Pang, Southwest University, China
Yang Liu, Chinese Academy of Fishery Sciences, China
Copyright © 2022 Liu, Li, Li and Li. This is an open-access article distributed under the terms of the Creative Commons Attribution License (CC BY). The use, distribution or reproduction in other forums is permitted, provided the original author(s) and the copyright owner(s) are credited and that the original publication in this journal is cited, in accordance with accepted academic practice. No use, distribution or reproduction is permitted which does not comply with these terms.
*Correspondence: Jie Li, lijie1561@163.com