- 1Laboratory of Avian Ecology and Evolution, Department of Zoology, Federal University of Pernambuco, Recife, Brazil
- 2Graduate Program in Animal Biology, Department of Zoology, Federal University of Pernambuco, Recife, Brazil
- 3Graduate Program in Zoology, Federal University of Amazonas, Manaus, Brazil
- 4Department of Natural History, Royal Ontario Museum, Toronto, ON, Canada
- 5Department of Ecology and Evolutionary Biology, University of Toronto, Toronto, ON, Canada
Amazonian rivers represent known barriers for avian dispersal, reducing gene flow and enhancing differentiation. Despite the importance of rivers in the avian evolutionary process, we have made only minor advances in understanding the limitations imposed by rivers on flying birds. To fill that gap, we conducted dispersal-challenge experiments over water, assessing the flying capabilities of 84 tropical bird species of 22 different avian families. We mist-netted and released 484 birds from a stationary boat on the Rio Branco, northern Amazonia, at increasing distances from the shore, including 249 individuals at 100; 219 at 200; 8 at 300; and 5 at 400 m. A successful trial was represented by a bird reaching the riverbank, whereas a failure would refer to birds not reaching the shore and landing on the water, when they were rescued by our team. Our main goal was to understand if the outcome in the experiments could be predicted by (i) phylogenetic constraints, (ii) morphology (body mass and wing shape), (iii) flight speed, (iv) ecological preferences (stratum, habitat, and river-island specialization), and (v) psychological reluctance to fly. Nearly two thirds of the individuals (332) were successful in reaching the riverbank, whereas 152 failed. We found significant differences among lineages. Whereas seven avian families succeeded in all of the trials, two families (antbirds and wrens) were particularly bad dispersers (<40% success). The hand-wing index (HWI) was the single most powerful predictor of trial success. Flying speed was also a significant predictor of success. Overall, ecological attributes had a low explanatory power. Only forest stratum preference had a significant, although weak, effect on dispersal ability: canopy- and ground-dwellers performed better than understory birds. However, we found no effect of habitat preference or river-island specialization on dispersal ability. Our speed estimates for 64 bird species are among the first produced for the tropics and suggest slower flying speeds than those reported from temperate migratory birds. Although birds showed behavioral differences when presented with the opportunity to fly away from the boat, we found no evidence that their reluctance to fly could predict the outcome in the experiments. This represents the first experimental study evaluating the riverine effect through dispersal ability of Amazonian birds, providing important insights to better understand dispersal limitations provided by riverine barriers.
Introduction
Given their ability to fly, birds are considered the most vagile class of terrestrial vertebrates, evidenced by their capacity to colonize even the most remote oceanic islands (Lees and Gilroy, 2014). However, it is well known that many avian taxa have river-bounded distributions, particularly in the Amazon basin, where dozens of avian lineages have morphologically and genetically distinct close relatives separated by rivers (Haffer, 1992; Naka et al., 2012). In fact, Amazonian rivers are known to represent important biogeographical boundaries (Haffer, 1969) and are traditionally used to delimit areas of endemism (Cracraft, 1985). First described for primates and birds (Wallace, 1852; Ayres and Clutton-Brock, 1992), the riverine effect on biodiversity has now been documented in other groups, such as butterflies (Brown, 1982; Rosser et al., 2021), frogs (Fouquet et al., 2012), lizards (Ávila-Pires, 1995; Pirani et al., 2019), and plants (Nazareno et al., 2021). However, it is among birds that this phenomenon is best known and best documented. River-bounded pairs of taxa are often represented by different subspecies in polytypic species or by allospecies in species complexes. The riverine effect on birds was first described for mighty Amazonian rivers such as the Amazon itself and its larger tributaries, like the Madeira, or the Negro, but we now know that even relatively small rivers, such as the Branco, the Aripuanã, and the Jiparanã also define the distributions of several pairs of avian taxa (Naka, 2011; Fernandes et al., 2014).
Although the role of rivers as primary or secondary barriers in the avian evolutionary process remains controversial (Naka and Brumfield, 2018), the fact that rivers do somehow limit dispersal and gene flow is well accepted (Haffer, 1992; Musher et al., 2022). In the last three decades, we have advanced considerably in gathering genetic evidence showing gene flow restrictions across rivers (Capparella, 1988, 1991; Ribas et al., 2012), but we had made only minor advances in understanding the real limitations that current rivers impose on flying birds.
Dispersal is one of the main forces of evolution and a key factor for understanding current and historic patterns of gene flow and differentiation. Dispersal also may affect community structure and composition of species assemblages. Therefore, understanding the capacity of species to move through the landscape can have direct implications for both evolutionary biology and ecology, and can be used to inform models of differentiation, community ecology, and biological conservation. For instance, high dispersal ability may provide the means to conquer new areas, stimulating peripatric speciation, but it could also facilitate gene flow and population homogenization, preventing species differentiation (Claramunt et al., 2012). Dispersal ability can also define which species colonize islands (MacArthur and Wilson, 1967; Lees and Gilroy, 2014) or which species are able to persist in fragmented landscapes (Burkey, 1989; Moore et al., 2008; Lees and Peres, 2009). Likewise, population connectivity in fragmented landscapes depends on species dispersal ability, which are mostly unreported for most tropical bird species (Hartfelder et al., 2020; Tourinho et al., 2022; Claramunt et al., 2022).
Given the flying capacity of birds, it is often assumed that features other than their flying apparatus should be involved in limiting species dispersal (Diamond, 1981). For instance, forest-interior species could be less prone to cross a river than open habitat birds due to their inexperience in dealing with heavily illuminated environments. In fact, some forest-interior Amazonian species exhibit higher dispersal abilities than non-specialized birds. Finally, behavioral reticence or reluctance to even attempt gap-crossing flights may also play an important role (Diamond, 1981; Laurance et al., 2004). From a bird’s perspective, crossing an open gap of water may represent more than a physical challenge, it may also mean venturing into a harsh, hostile environment.
On the other hand, recent studies have shown that flight capabilities and long-distance flight efficiency likely represent a key aspect for avian dispersal and gap-crossing tendencies (Moore et al., 2008; Ibarra-Macias et al., 2011; Claramunt et al., 2012; Claramunt, 2021; Claramunt et al., 2022). Therefore, flight speed could also represent an important variable, as faster birds may be more prone to successfully cross a riverine barrier than slower birds. Unfortunately, data on avian flying speed are not only rare, but mostly restricted to temperate migratory non-passerines (Alerstam et al., 2007; Pennycuick et al., 2013), and are virtually non-existent for tropical birds. Therefore, while multiple factors may be involved in determining a species ability to cross an inhospitable barrier, it is important to evaluate both physical, ecological, and behavioral constraints.
Moore et al. (2008) conducted one of the first experiments of dispersal limitation in tropical birds, assessing the ability of 10 forest-dwelling species to fly over a known distance in a lake in Panama. In that study, mist-netted birds were released at different distances from the shore, and the trial outcomes (success or failure) were correlated with species extinctions and colonization in forest fragments. This novel experimental approach has provided empirical evidence on the flight capabilities and limitations of tropical forest birds.
In this study, we used dispersal-challenge experiments over water to assess the barrier crossing capabilities of 84 tropical bird species widely distributed throughout the avian tree of life. Specifically, we aimed at understanding if success or failure in the dispersal experiments could be predicted by (i) phylogenetic constraints, (ii) morphology (body mass and wing shape), (iii) flight speed, (iv) habitat and microhabitat preferences, and (v) psychological reluctance to fly. To answer these questions, we mist-netted wild birds in riparian habitats along the Rio Branco, a medium-sized river in northern Amazonia, and released them from an anchored boat at known distances to the shore. We assessed success or failure in the experiments, and measured flight speed and an estimation of an individual’s reluctance to fly once they were released. We described the experimental results, evaluating differences among species and families, and correlated those results with the potential effect of dispersal ability on the evolutionary process. This represents the first experimental study to evaluate the effect of a riverine barrier through the dispersal ability of Amazonian birds and provides important insights to better understand the limitations provided by riverine barriers in the tropics.
Materials and Methods
Study Area
This study was conducted along the Rio Branco, located in the Brazilian state of Roraima, northern Amazonia. Despite its relatively short length (∼550 km), the Rio Branco represents the largest tributary of the Rio Negro (Goulding et al., 2003). The Rio Branco has a complicated hydrological setting, with blackwater, whitewater and clearwater tributaries, but generally it is considered a sediment-rich white-water river, particularly during the rainy season (Ríos-Villamizar et al., 2013). Geologically and biogeographically, the Rio Branco can be divided in the lower and upper sections, which host different avifaunas (Naka et al., 2007, 2020). All of our sampling was conducted on riparian habitats on the lower Rio Branco, where tall flooded forests, Cecropia-dominated riverine forests, and sandbar scrub are all intermingled throughout the landscape (Naka et al., 2020).
With over 430 bird species recorded, the avifauna of the Rio Branco is possibly the best documented for any Amazonian river (Naka et al., 2006, 2007, 2020; Laranjeiras et al., 2014, 2019, 2021). The river has been referred to as a biogeographical and ecological hotspot, due to the diversity of habitats along its margins and the powerful biogeographical effect that this river has on the non-flooded terra-firme forest avifauna (Naka et al., 2020). Phenotypic and genotypic studies have shown that the river represents a biogeographical barrier for at least 40 pairs of avian taxa (Naka, 2011; Naka et al., 2012; Naka and Brumfield, 2018), offering a unique opportunity to test avian dispersal abilities in a real biogeographical scenario.
Dispersal Experiments
We conducted dispersal experiments during two dry seasons (October 2013 and September–October 2014) using 511 birds (157 and 354, respectively). We adopted the ‘dispersal challenge’ approach used by Moore et al. (2008), where birds were captured using mist-nets and released from a stationary boat at specified distances from the shore (100, 200, 300, and 400 m). Only species that succeeded at 100 m were challenged to cross 200 m and so on. Only two species were used in trials at 300 and 400 m. These distances (100–400 m) are conservative, as large Amazonian rivers are generally much wider, but may account for the use of river islands as stepping-stones to cross a riverine barrier.
We mist-netted birds in various riparian habitats, including sandbar scrubs, Cecropia-dominated riverine forests, tall flooded (várzea) forests, and tall transitional forest. We minimized bird handling time to a minimum, checking mist-nets every 30 min in shaded tall forests and every 15 min on more exposed river islands. Upon capture, birds were identified, photographed, and visually aged and sexed, when possible. Birds were manipulated only to obtain photographs and measurements of body mass and wingspan, and then placed in individual cloth bags. Birds were marked by partially clipping the tip of one of the rectrices to avoid using the same bird in subsequent trials. Birds were transported to our release station (an anchored boat) within 30 min of capture. Prior to being released, birds were held in a box for 1 min, and then were allowed to fly back to the river bank. Upon release, at least two people followed the birds with binoculars and a third member of the team recorded the duration of flight with a digital stopwatch. Birds usually flew straight to the closest bank. When this was not the case, and birds covered longer distances, we measured the actual distance flown with the aid of a GPS. A second (non-anchored boat) was always ready to rescue birds that “failed” the dispersal trial and landed on the water. Birds that showed obvious signs of stress (weak, panting) were not used in the experiments. We measured wing speed prior to each release session using a Instrutemp Icel anemometer (model 3010), avoiding releasing birds under strong wind conditions (> 3 m/sec). We measured flight speed by quantifying flight duration over a known distance. For the speed estimates, we excluded birds that (i) did not fly at all, (ii) landed on the water, (iii) did not fly to the shore’s nearest point, (iv) did not fly in a straight line (performed zigzags or detours), (v) performed circles around the boat, or (vi) did not go to the shore (for ex., swallows). Birds were captured and released under a Research License granted to LNN by the Chico Mendes Institute for Biodiversity Conservation (ICMBio) from the Brazilian Ministry of the Environment (license no. 30112-1 and subsequent renewals).
Morphological Predictors
We obtained morphological measurements exclusively from museum specimens. The overwhelming majority of specimens measured for this study were collected from the Rio Branco, minimizing geographical variation within species. Most of these specimens are currently held at the Universidad Federal de Pernambuco’s ornithological collection. To avoid potential biases, a single person (BC) was responsible for measuring all birds. In a few cases, for species with a single sample, we included measurements from specimens held at the Royal Ontario Museum, which were measured by SC and collaborators.
From these specimens we estimated the hand-wing index (HWI), which reflects the degree of elongation of the distal portion of the wing (Kipp, 1959; Claramunt and Wright, 2017). The HWI is a proxy for the aspect ratio of the wing, and thus reflects the energetic efficiency of forward flight in birds (Norberg, 1990; Pennycuick, 2008). We calculated the HWI as 100*K/WL, where K is Kipp’s distance, the distance between the tip of the longest primary and the tip of secondary 1 on the closed wing, and WL is the traditional wing length measured from the carpal joint to the tip of the longest primary (Claramunt and Wright, 2017). Alternatively, we estimated K as WL – S1, where S1 is the distance between the carpal joint and the tip of the first secondary feather, the most distal flight feather of the forearm, which produces very similar results (Claramunt and Wright, 2017).
Ecological Predictors
We tested as ecological predictors: (i) foraging stratum, (ii) habitat preference, and (iii) river-island specialization. Species categorizations presented in Table 1 were obtained from our own experience on riparian habitats on the Rio Branco, which we have studied for over a decade (Naka et al., 2020). We understand that in a few cases, these may diverge from what species do elsewhere in Amazonia, but to the best of our knowledge, they represent the best categorization for this avifauna. We obtained data on habitat preference from hundreds of point counts conducted on the Rio Branco and its tributaries, which is available in the Supplementary Material of our recent publication (Naka et al., 2020).
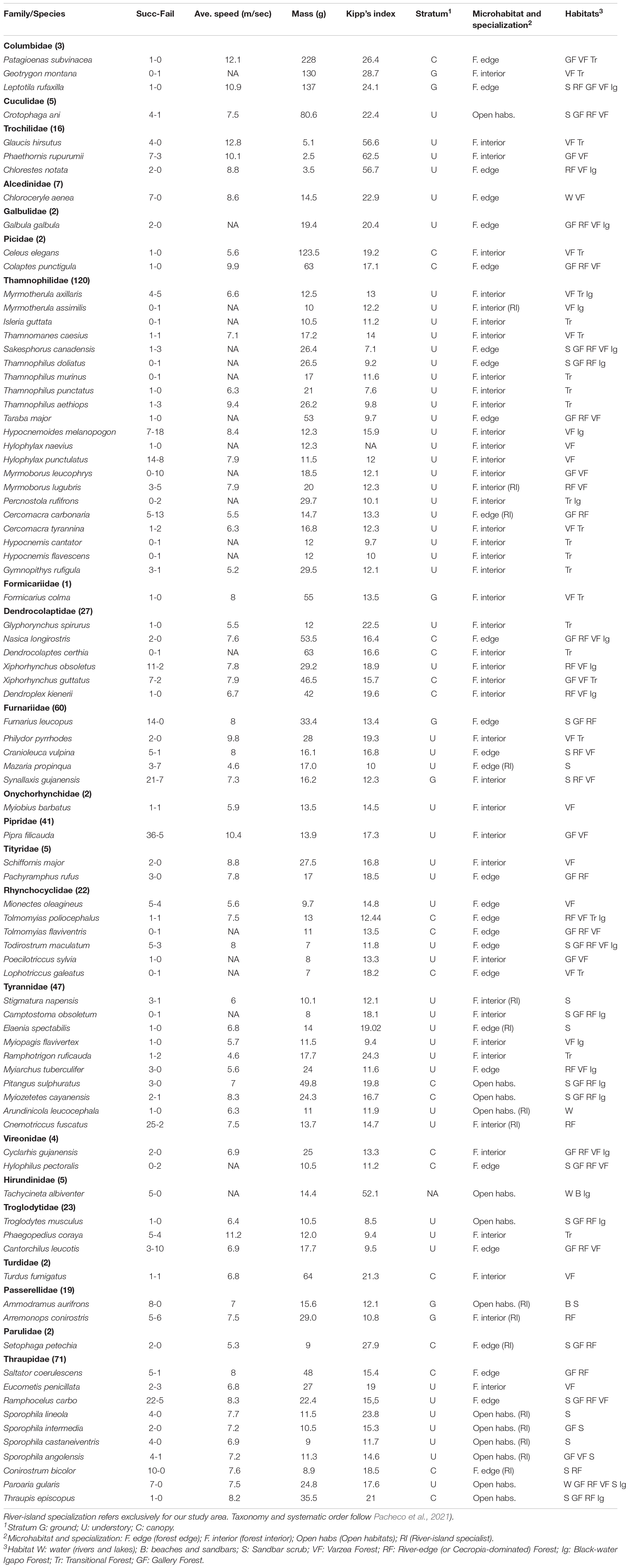
Table 1. Eighty-four (84) bird species included in the dispersal experiments, including rates of success and failure, average speed, and main habitats, microhabitat and stratum used based by each species (based on Naka et al., 2020). Numbers in parentheses after family names indicate the number of experiments per family.
Psychological Predictors
Upon release (i.e., opening the concealing box), not all birds flew immediately. Whereas some birds flew immediately upon the opening of the box, other individuals took their time. The time that each bird took to fly off the boat differed among individuals. We considered this waiting period as the reluctance to fly (measured in seconds). This period ranged from 0 to 5 min. When a bird refuse to take off after the 5-min period, the dispersal experiment was canceled, and the bird was taken back to the shore and released in its natural habitat.
Statistical Analyses
We estimated the phylogenetic signal or phylogenetic inertia in variables using the phylosig function in the phytools 0.7 library (Revell, 2012). Phylogenetic signal is assessed through a “lambda” transformation of internal branch lengths (Pagel, 1999). Lambda approximates zero when there is no or low phylogenetic signal and approximates to 1 when there is a high phylogenetic signal, showing Brownian motion-like phylogenetic inertia. We estimated lambda via maximum likelihood and present tests of whether lambda is equal to 0, using likelihood ratio tests.
We analyzed the relationship between morphological and ecological predictors and river-crossing success during the dispersal experiments using phylogenetic logistic regression models, which were implemented in the generalized estimating equation function compar.gee (Paradis and Claude, 2002) in the ape 5.4 R package (Paradis and Schliep, 2019). We modeled the frequency of success and failure by specifying a binomial distribution for the response and used a phylogenetic correlation structure to model phylogenetic non-independence among species.
The species phylogeny was obtained from birdtree.org using the Hackett et al. (2008) backbone topology and the V2.iii calibrations (Jetz et al., 2014). The final, maximum clade credibility tree, was then obtained from the sample of 1000 trees using the function maxCladeCred in phangorn 2.6.3 (Schliep, 2011).
Phylogenetic non-independence was taken into account by correlation structures that model the evolution of residuals along the branches of the phylogeny. We evaluated the performance of four phylogenetic correlation structures available in the ape library, including Brownian, Lambda, Martins, and Grafen (Grafen, 1989; Martins and Hansen, 1997; Pagel, 1999; Freckleton et al., 2002). We chose the correlation structure that minimized the quasi-likelihood information criterion (QIC, Paradis and Claude, 2002). We also used QIC to evaluate the relative fit of different models. To evaluate whether predictor coefficients were different from zero, we used t tests with phylogenetically adjusted degrees of freedom (Paradis and Claude, 2002). As a measure of absolute model fit, we estimated coefficients of determination based on squared correlation coefficients between the observed frequency of success and the predicted probabilities estimated by the models (Agresti, 2007), with weights determined by the number of experiments done for each species.
For our speed estimates, we only used experiments at 100 and 200 m from the coast, which represented more than 95% of the sample. Given that the average flight speeds were nearly identical at 100 and 200 m, we lumped those results, obtaining a single speed estimate per species, which is presented in Table 1.
Results
Dispersal Experiments
We conducted dispersal experiments on 484 individuals, representing 84 species, belonging to six avian orders and 22 families (Table 1). We captured an additional 27 birds, which were excluded from the analyses for various reasons (explained in section Materials and Methods), but mostly because they refused to fly off the boat. Most of our sample (85%) was composed of passerine birds of 16 different families. Six of these families (Thamnophilidae, 130 spp; Thraupidae, 76 spp.; Furnariidae, 63 spp.; Tyrannidae, 48 spp.; Pipridae, 41 spp. and Dendrocolaptidae, 28 spp.) accounted for 75% (386 ind.) of the trials. On average, we sampled 6.6 birds per species (± 7.8), with a median of 4 birds. Fifteen species were represented by singletons and 14 by doubletons, whereas 30 species had at least 5 replicates, and 5 species participated in more than 20 experiments (Table 1). A total of 249 birds of 68 species were released 100 m from the shore, 219 of 58 species at 200 m, 8 of 2 species (Pipra filicauda and Ramphocelus carbo) at 300 m and 4 individuals of a single species (Pipra filicauda) at 400 m (Supplementary Table S1).
About two thirds of the birds (172 individuals of 56 species) were successful in reaching the river bank at 100 m, whereas the other third (77 individuals of 29 species) failed to do so. An almost identical rate of success was apparent on the 200 m trials, where 146 individuals of 42 species managed to cross the water gap and 73 of 30 species failed. All 13 birds that were released at 300 and 400 m were successful, but they only represented two species. We found a clear variation in trial success among species; a total of 36 bird species (43%) succeeded in all their challenges, whereas 14 species (17%) failed in all of theirs (Table 1). On the other hand, 34 species (40%) had both successes and failures in the experiments. This variation was also evident at the family level (Figure 1). Seven of the 22 avian families tested, succeeded in 100% of the trials (Columbidae, Alcedinidae, Galbulidae, Picidae, Formicariidae, Tytiridae, Hirundinidae, and Parulidae), although two of these included less than 5 trials (Formicariidae and Tytiridae). Seven families succeeded in 75–90% of the trials (Cuculidae, Trochilidae, Dendrocolaptidae, Furnariidae, Tyrannidae, Pipridae, and Thraupidae). Another six families succeeded in 50–70% of the trials (Onychorhynchidae, Rhynchocyclidae, Columbidae, Vireonidae, Turdidae, and Passerellidae), although the last four families had fewer than 5 samples. Finally, the antbirds (Thamnophilidae) and the wrens (Troglodytidae) were particularly bad dispersers, attaining only 38 and 39% of success, respectively. Phylogenetic signal in river-crossing success rate was relatively low (lambda = 0.34) but significantly different from zero (Likelihood ratio = 12.8, p = 0.0003).
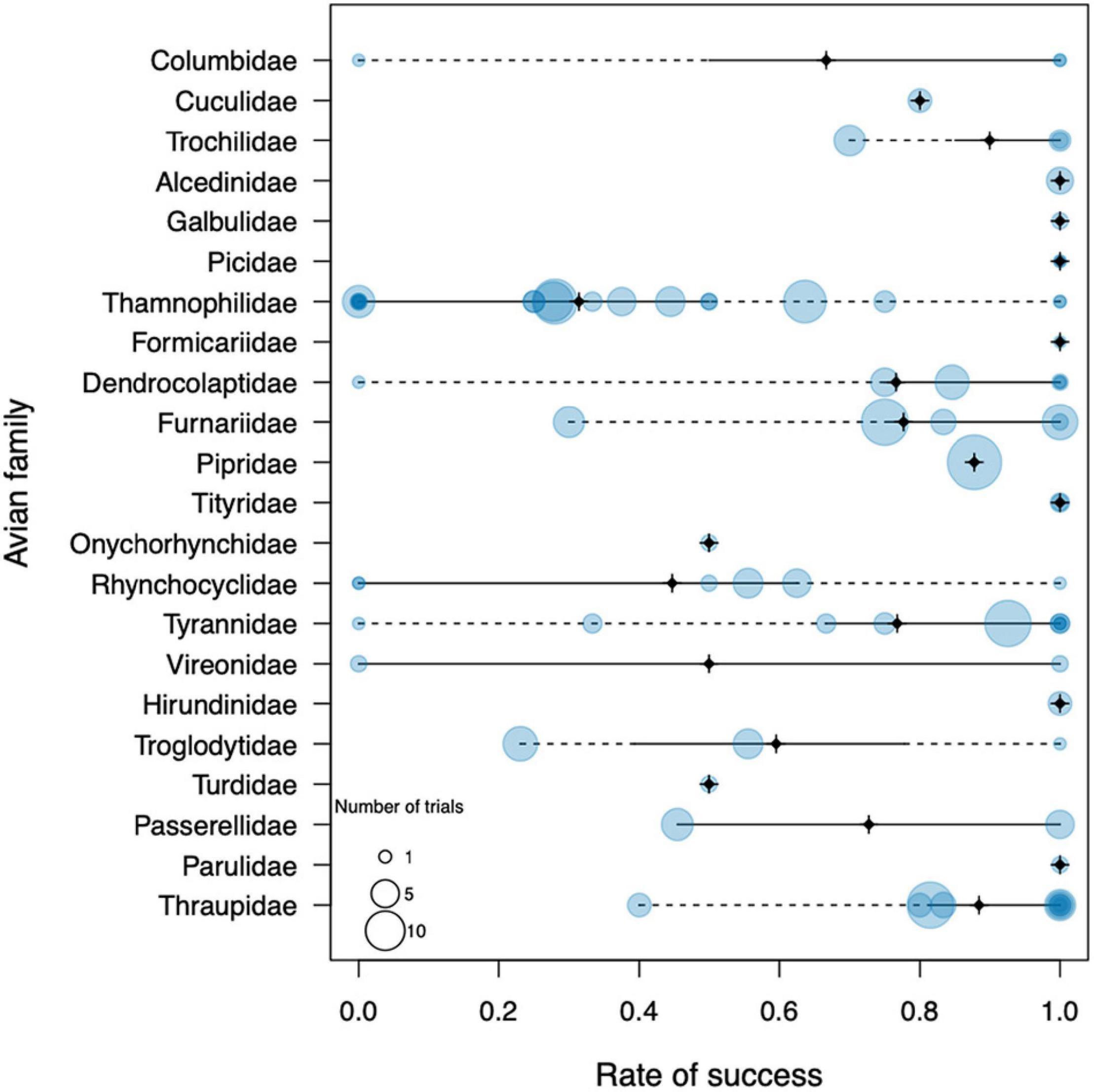
Figure 1. General results of the dispersal trial experiments conducted in the Rio Branco in northern Amazonia, grouped per family. Each bubble represents one species within the family, whereas the size of the bubble represents the number of individuals used in the experiments. The black cross marks the family median, solid lines span the interquartile range, and dotted lines span the total range.
Morphological Predictors
We evaluated two morphological predictors of dispersal success: the hand-wing index (HWI) and body mass. The HWI ranged from 7.1 (Sakesphorus canadensis) to 62.5 (Phaethornis rupurumii), and had a very strong phylogenetic signal (lambda = 0.99), significantly different from zero (Likelihood ratio = 111.8, p < 0.001), suggesting a Brownian motion mode of evolution. The HWI was a significant predictor of trial success, both at the species level (R2 = 0.29, t = 3.5, p = 0.0019; Figure 2A) and at the family level (R2 = 0.60; F = 22.5; p = 0.0004; Figure 2B), for which the predictive power was particularly strong. In general, families with HWIs higher than 15 were successful in more than 80% of the trials, whereas those with lower values fared worse in the dispersal experiments.
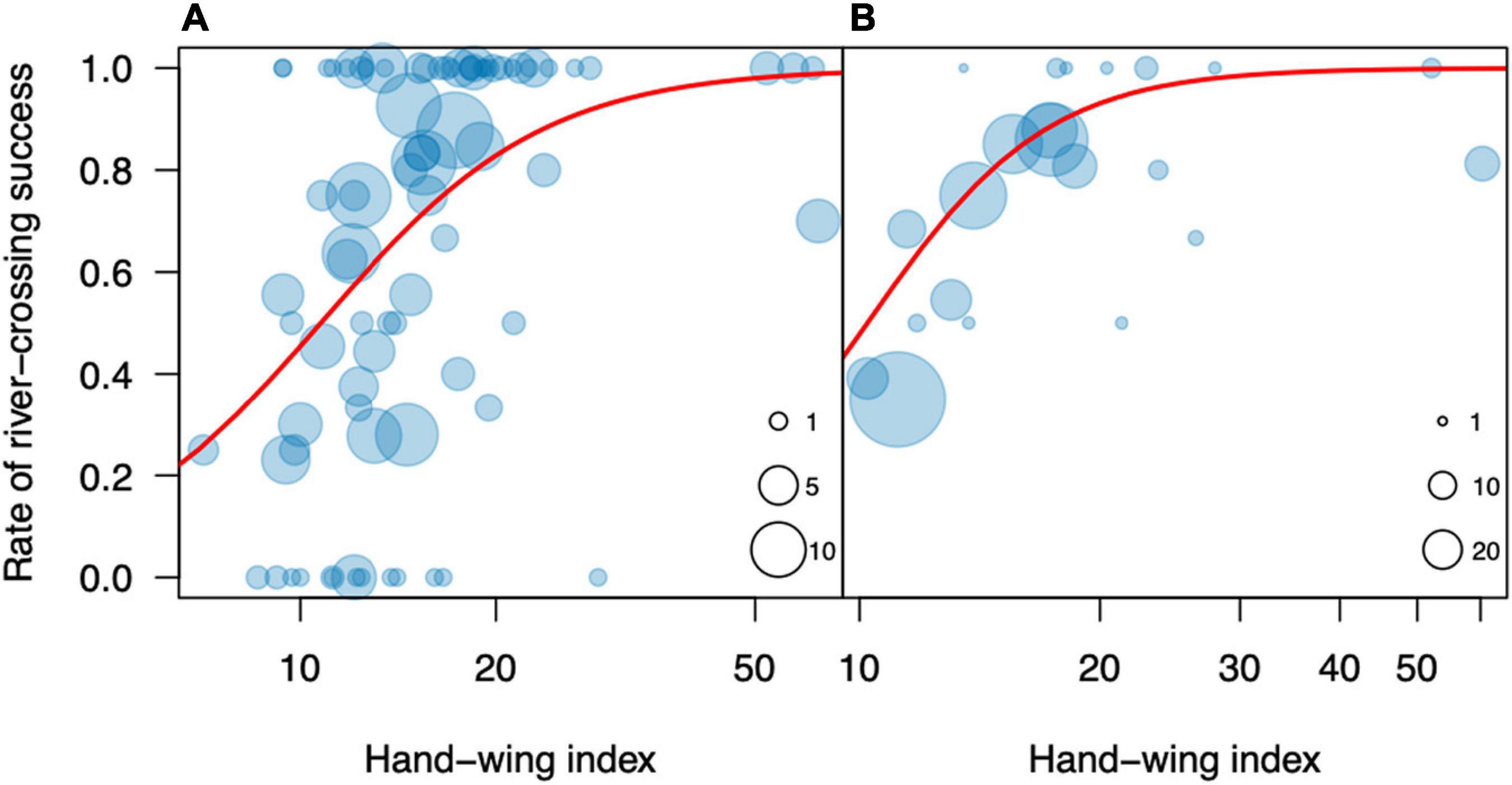
Figure 2. Rate of river-crossing success in the dispersal experiments on the Rio Branco in relation to the Hand-wing index at the species (A) and family (B) levels. In panel (A), each circle represents one species, and its diameter represents the number of individuals for that species. In panel (B), each circle represents one family, and its diameter represents the number of species for that family.
Body mass ranged from 2.5 g (Phaethornis rupurumii) to 228 g (Patagioenas subvinacea), with a median of 16.9 g (Table 1). In contrast to the HWI, we found no significant effect of mass on trial success (R2 = 0.001, t = 0.36, p = 0.72); smaller birds had the same chance of success, compared to larger ones, in the experimental trials (Figure 3A).
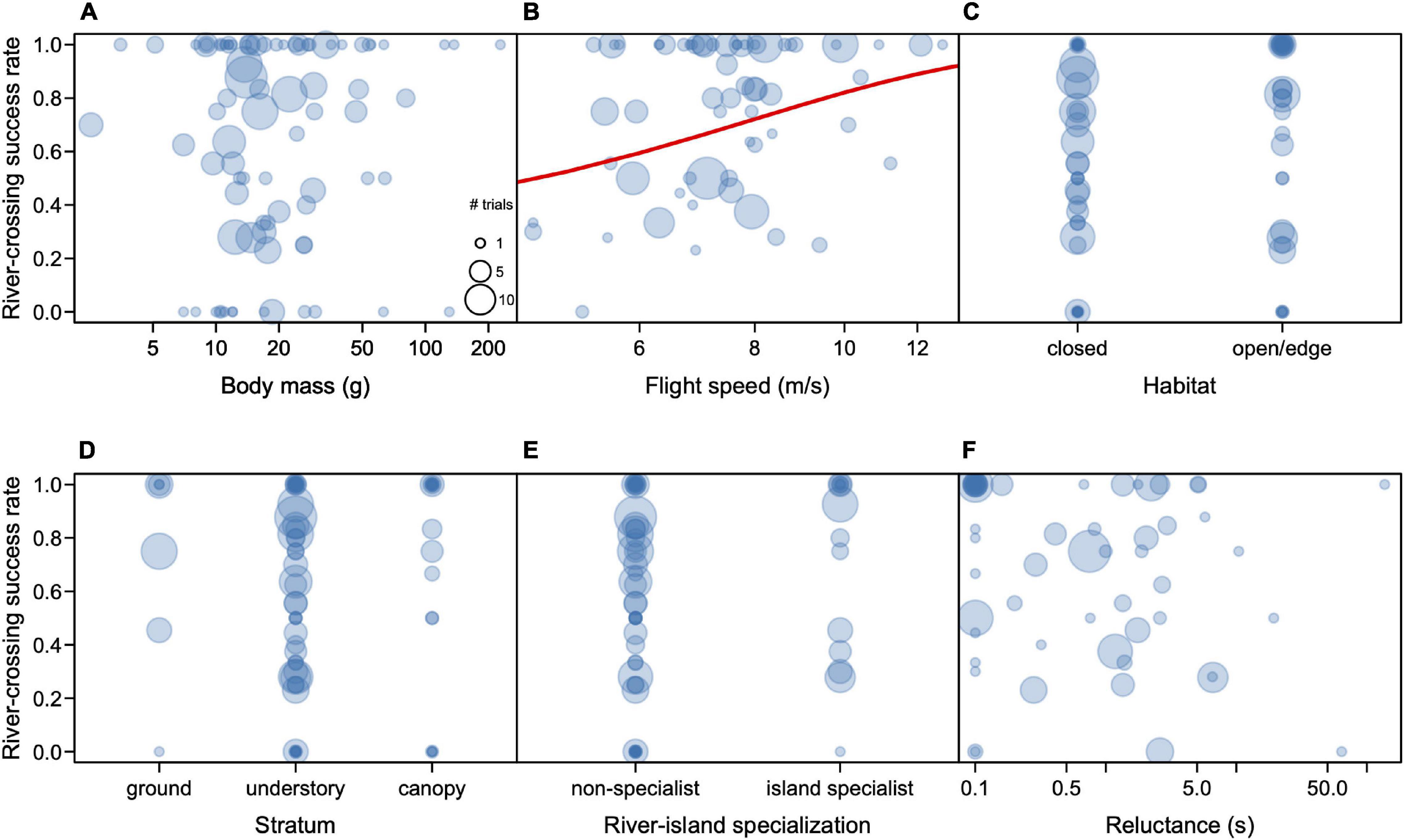
Figure 3. Rate of success in the dispersal experiments on the Rio Branco in relation to body mass (A), flight speed (B), habitat (C), Stratum (D), river island specialization (E), and reluctance to fly (F). Each circle represents one species, and its diameter represents the number of individuals for that species.
Speed
We obtained flight speed estimates of 269 individuals of 64 bird species (Table 1 and Supplementary Table S1). There was a medium phylogenetic signal in speed (lambda = 0.53), which was significantly different from zero (Likelihood ratio = 9.08, p = 0.0002). Average flight speeds were nearly identical at 100 and 200 m, averaging 7.6 m/sec (Table 2). The two species that were exposed to longer distances flew faster when crossing 300 and 400 m (Table 2). The slowest species in our sample that managed to cross the river gap presented in the experiments were the White-bellied Spinetail (Mazaria propinqua) and the Rufous-tailed Flatbill (Ramphotrigon ruficauda) which flew at 4.6 m/s, both of which had more failures than successes (3–7 and 1–2, respectively). On the other hand, the fastest species was a hummingbird, the Rufous-breasted Hermit (Glaucis hirsutus), which flew at 12.8 m/s (Table 2). For the one species for which we had trials at all distances, the Wire-tailed Manakin (Pipra filicauda), we found a significant and positive effect on distance over speed (R2 = 0.23; F = 9.54, p = 0.004), flying faster when covering longer distances. We found that flight speed is a significant predictor of trial success (R2 = 0.138; t = 2.62; p = 0.017, Figure 3B), with the caveat that speed was only measured for species that successfully crossed the riverine gap at least once.

Table 2. Summary of flight speed estimates obtained during the dispersal experiments on the Rio Branco.
Ecological Predictors
Most species involved in the experiments were from the understory (57 species), followed by the canopy (19 species), the ground (7 species), and the open airspace (1 species) (Table 1). About half (42) of the species were typical of open habitats (20 species) or forest edge (22 species) and the other half (40 species) was represented by forest interior birds (Table 1). At least 15 of the 84 species subjected to the experiments can be considered river-island specialists at our study site (Table 1). We found a significant effect of forest stratum preference on river-crossing success (R2 = 0.052, F = 4.2, p = 0.029) (Figure 3D), but not on habitat preferences (R2 = 0.027, t = 1.5, p = 0.15) (Figure 3C), or river-island specialization (R2 < 0.001, t = 0.23, p = 0.81) (Figure 3E). Contrary to our predictions, birds from open habitats and the forest edge, and river-island specialists did not perform better in the trials compared to forest-interior and non-specialists of islands.
Psychological Predictors
We measured the reluctance to fly (measured in seconds) in 354 individuals of 65 species. Most individuals (256 or 72%) of 29 species flew immediately upon release, but nearly 30% of the individuals took longer to fly or did not fly at all. Although we didn’t find an effect of the time taken to take off and the rate of success (R2 = 0.006, t = 0.67, p = 0.51, Figure 3F), we observed that certain families where more reluctant to fly than others. For example, individuals of eight species refused to take off from the boat (staying for more than 5 min), six of which were antbirds (Family Thamnophilidae), including the Dot-backed Antbird (Hylophylax punctulatus, 2 ind.), the Black-chinned Antbird (Hypocnemoides melanopogon, 2 ind.), the Guianan Warbling Antbird (Hypocnemis cantator, 2 ind.), the White-browed Antbird (Myrmoborus leucophrys, 3 ind.), the Ash-breasted Antbird (Myrmoborus lugubris, 1 ind.), and the Black-headed Antbird (Percnostola rufifrons, 1 ind.). The two other individuals that refused to fly belonged to the Ochre-bellied Flycatcher (Mionectes oleagineus (1 ind.) and the Coraya Wren (Pheugopedius coraya, 1 ind.).
Discussion
In this study, we present experimental evidence showing that riverine barriers may represent significant obstacles to avian dispersal. Our results are novel in several ways, representing the first dispersal experiments on birds in Amazonia, the first empirical dataset showing differences in the dispersal abilities of species and families, and the first estimates of avian flight speeds reported from the tropics. Despite the many confounding factors that are inheritably present in controlled experiments in the wild, several conclusions can be drawn from this study. First and most importantly, we have documented differences in the ability of bird species to cross a body of water, where even relatively narrow gaps (100 m) may represent an unsurmountable barrier for many species of birds. This differential ability seems to be related to flight performance, rather than body size or ecological and psychological factors. Specifically, we found significant effects of wing shape and flight speed on the ability of species to succeed in the dispersal challenges, both of which have a significant phylogenetic component. Among the ecological attributes tested, only stratum preference was a significant, albeit weak, predictor, whereas habitat preference and river island specialization did not prove to be important factors. Behavioral reluctance to fly is harder to evaluate in experimental conditions but deserves further investigation.
Morphological Predictors of Cross-River Dispersal
We assessed the effect of the hand-wind index (also known as Kipp’s Index) and body mass in predicting species outcomes in the dispersal experiments. The hand-wind index, which has a very strong phylogenetic signal, was the strongest predictor found in this study, both at the species and family levels. These results are consistent with theoretical expectations of increased long-distance flight efficiency with higher aspect ratio wings (Norberg, 1990; Pennycuick, 2008) and adds to the mounting evidence of the usefulness of the hand-wing index as a proxy for avian dispersal ability (Claramunt et al., 2012, 2022; Claramunt and Wright, 2017; Sheard et al., 2020; Claramunt, 2021).
On the other hand, we found no effect of body mass on dispersal capacity within our sample. Prior expectations were ambiguous. On the one hand, there are some empirical reasons to expect that larger birds could have better dispersal abilities than smaller birds, as predation and range size have been reported to be size-dependent (Suhonen et al., 1994; Ottaviani et al., 2006). Although some studies reported significant effects of body size on the capacity of tropical birds to move through fragmented landscapes (Lees and Peres, 2008, 2009; Neuschulz et al., 2013) these studies did not analyze indicators of flight efficiency, potentially confounding these two factors. On the other hand, recent phylogenetically controlled comparisons found no effect of body mass on dispersal distances in Holarctic birds (Claramunt, 2021) or in movements across habitat gaps in Amazonian birds (Claramunt et al., 2022). It is possible that our result is sample-dependent, as our sample lacks some large birds such as raptors and parrots, which are expected to be good flyers. But, we also lacked large nearly flightless species such as tinamous and trumpeters that are expected to have poor dispersal capabilities. Ultimately, dispersal abilities in birds depend on their flight abilities, and long-distance flight performance depends more on the morphology of the flight apparatus than on body size. Strong-flying and mobile species can be found along the entire spectrum of body sizes in birds from the smallest species (hummingbirds, swifts) to the largest (albatrosses, condors), and the same is true for nearly flightless sedentary species.
Flight Velocity and Dispersal Ability
Flight velocity was a significant predictor of species success in the dispersal challenges. Faster species had a higher probability of succeeding in the dispersal experiments. As with the HWI, flight velocity can be considered a measurement of flight efficiency, and both variables seem to be important in defining which species were able to cross open gaps of water, suggesting that species performance is directly related to their morphology.
Being the first large dataset on flight speeds ever produced for tropical birds, there is little data warranting direct comparisons. The best datasets available include those gathered using ornithodolites on 38 non-passerine migratory birds in Sweden (Pennycuick, 1982; Pennycuick et al., 2013) and radars on 138 species on migration in the Western Palearctic (Bruderer and Boldt, 2001; Alerstam et al., 2007). Our flight speed estimates seem low compared to those reported there, which ranged from 11 to 22 m/s in Sweden (Pennycuick et al., 2013) and from 8 to 23 m/s in the Western Palearctic (Bruderer and Boldt, 2001; Alerstam et al., 2007). Our mean values were similar to the slowest speeds in those studies, and our slowest values (4.6 m/s) were nearly half the slowest species in the temperate region. These values could indicate that flight speeds among tropical species is comparatively low, but most species in available datasets included many large non-passerine species such as ducks, swans, cranes, and waders and only a handful of passerines. The genus Turdus, however, was sampled both in the Palearctic and the Rio Branco, and allows a direct comparison. The average speed of four species of Palearctic thrushes averaged 12.3 m/s, whereas our only flight speed estimate of a thrush (Turdus fumigatus) was nearly half that (6.8 m/s).
These datasets, however, are not completely comparable, as Bruderer and Boldt’s (2001) measurements were obtained on migrating birds flying at constant speeds and using tracking radar. Migratory flight speeds are inherently faster than short distance flights, even for temperate birds. Furthermore, our estimates include the acceleration phase, which is composed of more powerful strokes, yet slower speeds, which would necessarily bias our estimates to slower speed estimates. However, having very similar estimates of 100 and 200 m suggests that this acceleration phase does not account for such large differences. Quite interestingly, Bruderer and Boldt (2001) mentioned that released birds were apparently reluctant to depart with migratory speed, flying at considerably lower speeds than migrating conspecifics. These authors showed that whereas migrating passerines fly at around 10–15 m/s, most released birds flew at a speed of 5 to 10 m/s, which is quite close to our estimated ranges.
Our method for measuring flight speed relied on measuring flight time over a known distance (i.e., shortest distance to the riverbank). Despite representing a direct measurement, our estimates are not devoid of potential biases. Although we avoided releasing birds under strong winds (> 3 m/s), even mild winds could influence speed estimates (Nilsson et al., 2014). Furthermore, although we measured wind speed prior to each release session, we did not note wind direction, which is an obvious aspect to take into consideration. Despite these potential biases, our measurements are quite consistent across species and families. For instance, hummingbirds ranged from 8.8 to 12.8 m/s and the four species of seedeaters tested, had very similar flying speeds (6.9 to 7.7 m/s).
Ecological Traits Associated With Dispersal
The role of ecological attributes on dispersal remains elusive. Despite having clear predictions in terms of species success in the dispersal experiments in relation to stratum and habitat preference, and river-island specialization, none of the attributes included in this study showed a strong effect of ecology. Favoring one of our predictions, we found a significant, although weak, effect of foraging stratum on the rate of success in the dispersal experiments. Both canopy and ground-dwelling species were more likely to succeed in reaching the river bank than understory birds. This result is concordant with previous studies showing lower genetic differentiation across rivers (Burney and Brumfield, 2009) and higher demographic connectivity across habitat gaps (Bradfer-Lawrence et al., 2018) in canopy birds compared to those from the understory. That ground dwelling birds may have higher flight capabilities than understory birds is an interesting result. This result is at odds with the Barro Colorado experience, where many ground-dwelling species have disappeared from islands and their populations have not been replenished (Willis, 1974). Although ground dwelling birds do not fly much during foraging, strong flight capabilities may be important for escaping predators and making larger-scale movements within the forest, such as that documented for Geotrygon montana (Stouffer and Bierregaard, 1993).
Empirical evidence suggests that forest-edge and open habitat birds are more likely to cross forest gaps than forest-interior species (Laurance et al., 2004; Lees and Peres, 2009). Birds of the forest edge, which are often compared to birds from open-habitats due to the high levels of solar irradiation (Foggo et al., 2001), are also expected to have enhanced mobility, and were expected to perform well in our dispersal experiments. However, we found no effect of habitat preference (forest interior vs. forest-edge and open areas) on crossing capabilities. This result is likely due to the large variation found among forest-edge/open areas birds, which includes some excellent flyers but also species such as the Black-crested Antshrike (Sakesphorus canadensis), the Plain-crowned Spinetail (Synallaxis gujanensis), and the Buff-breasted Wren (Cantorchilus leucotis), which fared poorly in our experiments likely because of their low flight performance.
Similarly, we hypothesized that river-island specialists should demonstrate higher dispersal capabilities than birds that do not need to relocate to other environments on a seasonal basis due to the ephemeral nature of river islands (Rosenberg, 1990; Zimmer and Leisler, 2003). However, we found no significant effect of this kind of specialization in relation to their success in the experiments. Recent ecological data on some of these river-island flooded forests specialists suggests that birds do not necessarily relocate to unflooded forests. Quite the contrary, they seem to perform vertical displacements along the vegetation, making use of the available space during the flooding period, allowing them year-round territoriality without major habitat shifts (Rowedder et al., 2021). These results do agree with recent genetic data that found stronger population structure among river island specialists, when compared to floodplain specialists (Choueri et al., 2017; Thom et al., 2018), which included some of our relatively bad dispersers (e.g., Myrmoborus lugubris).
Psychological Constraints
Empirical evidence suggests that the dispersal ability of birds may be limited, at least in part, by psychological constraints. Data from Amazonian forest fragments indicate that the dispersal of forest-interior birds may be limited by tree-fall gaps, clearings or relatively narrow roads (Stouffer and Bierregaard, 1995; Laurance et al., 2004), a distance that is unlikely to represent a physical challenge for a bird. Whether these limitations are related to the “fear of flying” (Diamond, 1981) or a physical limitation due to their sensory system (Ausprey et al., 2021) remains to be tested. However, flying over an open gap of water represents a very different challenge than crossing a forest gap or even a road, as birds need to cover the entire distance in a single bout, without pausing or resting along the way. We hypothesized that birds that were more reluctant to fly would have a lower success in the dispersal experiments. Although the time taken by each bird to fly was unrelated to individual success, our empirical data suggests that most species that refused to even attempt crossing the water gap were represented by either antbirds or other low success species. Those birds preferred to remain in their boxes rather than venturing into the unknown. We suspect that under natural circumstances, these species will likely avoid crossing a river, which may have long-term effects on the genetic structure of these populations. In fact, river crossing is clearly not devoted of risks, even for those that can cross the gap of water successfully. Among these dangers, we witnessed aerial attacks on some species by White-winged Swallows (Tachycineta albiventer), as birds were allegedly invading their aerial space, and a predation event where a Rufous-throated Antbird (Gymopithys rufigula) was attacked in flight and caught by a Bat Falcon (Falco rufigularis) during what otherwise looked like a potentially successful river crossing.
Dispersal Ability of Amazonian Birds
Results from experimental studies on dispersal could be readily applied in at least two different fields, including biological conservation and evolutionary biology. Recent studies have successfully explored links between dispersal capacity and fragment recolonization and extinctions (Bates, 2002; Moore et al., 2008), but little has been done to bridge the gap between dispersal ability and the avian evolutionary process, particularly in Amazonia, where riverine barriers define the distribution of multiple avian lineages. In fact, establishing a link between dispersal ability and avian distribution patterns in Amazonia has proven to be a challenging task. For example, nearly a third of the species that occur in the terra-firme forests of the Guiana Shield in northern Amazonia have taxon replacements across the lower Rio Negro, and those include groups generally considered to be good flyers, such as hummingbirds and parrots (Naka et al., 2012). Furthermore, the woodcreepers (Dendrocolaptidae), which responded very well to the dispersal experiments with > 80% of success rate, have more taxon replacements across the lower Rio Negro (73% of the species have an allospecies or different subspecies) than the antbirds (Thamnophilidae), which fared poorly in the experiments with a low success rate (< 40%), but have a lower (58%) taxon replacement across that same river (Naka and Brumfield, 2018).
There are few potential explanations behind these apparent contradictions. On the one hand, our results are highly conservative, because 100 or even 400 m represent a much lower distance than most Amazonian rivers currently impose on birds. On the other hand, at an evolutionary scale, the presence of river islands as well as rare seasonal droughts or even reductions in river discharge during dry Glacial cycles could make it easier for poor dispersers to manage to cross a river gap. However, simulations have shown that reduced dispersal across rivers can maintain two species in allopatry for many generations, despite repeated river crossings by few individuals (Santorelli et al., 2022).
It is important to note that the riverine barrier effect is best documented for terra-firme birds, which inhabit upland forests that never get flooded by the seasonal flood pulse of Amazonian rivers. These species demonstrate greater genetic diversity and levels of divergence across the landscape than floodplain species (Harvey et al., 2017). Therefore, although it could be argued that our sample obtained in riparian habitats may not be representative of the terra-firme forest avifauna, we believe our results are important for this discussion. First, nearly a third of our sample is represented by species which also occur in terra-firme forests and are readily found on tall transitional forests. Second, given the strong effect of morphology on dispersal success and the pervasive effect of the phylogeny on species dispersal abilities, it seems reasonable to expect similar results when including a larger sample of terra-firme forest species. In fact, our results seem to downplay the role of ecology on dispersal, suggesting that avian morphology may be more important than being a terra-firme or a flooded forest species. Finally, although it is possible that bird handling had an effect on flight capacity (mist-netted birds may be subject to temporary wing-strain) which might explain some failures in species with low sample sizes, we believe our large sample and consistent results at the species and family levels are strong enough to show a clear underlining pattern.
Final Conclusion
Our data shows that dispersal abilities are not homogeneous across the avian tree of life, and some lineages are more likely to successfully cross a riverine barrier than others. So far, variables associated with flight performance, such as the hand-wing index and flight speed, seem to be the best predictors of success in dispersal-challenge experiments. Our results also show that although there are good reasons for ecological attributes to have an effect, they do not seem to have a strong predictive power, suggesting that flight efficiency may represent a much more direct proxy to estimate species’ dispersal capabilities. We foresee that experimental data on dispersal abilities can shed light onto important aspects of avian ecology and evolution, including patterns of gene flow and genetic differentiation, as well as patterns of functional landscape connectivity in fragmented habitats. These two different aspects of avian biology will broaden our understanding on the role of riverine barriers in the evolution of the Amazonian biota and the effect of dispersal in species occupancy and recolonization in fragmented habitats.
Data Availability Statement
The raw data supporting the conclusions of this article are included in the article or as Supplementary Material (Supplementary Table S1).
Ethics Statement
We followed standard ethical guidelines for conducting research on wild birds (Fair et al., 2010). Procedures for capturing wild birds were approved by the Chico Mendes Institute for Biodiversity Conservation (ICMBio), Brazilian Ministry of the Environment (Research License no. 30112-1 granted to LN and subsequent renewals).
Author Contributions
LN and BC envisioned and designed this study. BC, GL, and LN conducted the experiments. LN, SC, and BC wrote the manuscript. SC, LN, and BC analyzed the data. All authors contributed to the article and approved the submitted version.
Funding
This research was funded by grants provided to LN by the Fundação Boticário de Proteção á Natureza (934-2012.1) and the Brazilian Research Council, CNPq (484219/2011-1 and 480496/2012-9). LN is currently funded by a CNPq Research Productivity Fellowship. SC work was supported by the Natural Sciences and Engineering Research Council of Canada (NSERC) Discovery Grant RGPIN-2018-06747.
Conflict of Interest
The authors declare that the research was conducted in the absence of any commercial or financial relationships that could be construed as a potential conflict of interest.
Publisher’s Note
All claims expressed in this article are solely those of the authors and do not necessarily represent those of their affiliated organizations, or those of the publisher, the editors and the reviewers. Any product that may be evaluated in this article, or claim that may be made by its manufacturer, is not guaranteed or endorsed by the publisher.
Acknowledgments
We are grateful to the many people involved in our field expeditions, including several field assistants and fellow researchers. Among these, we would like to thank the efforts of Hamilton de Melo, Daniele Mariz, and Thiago Orsi laranjeiras. Several people from the Chico Mendes Institute of Biodiversity Conservation (ICMBio) were key to the success of our trips on the Rio Branco, including Beatriz Ribeiro Lisboa, Antonio Lisboa, Erica Tieko Fijisaki, Inara Santos, and Romerio Briglia. Their help included offering logistical support, including speed boats, motors, and lodging. GL and LN are grateful to Maria Gracimar Pacheco, former Coordinator of the Graduate Program on Biological Diversity at the Universidade Federal do Amazonas. As graduate student GL received a fellowship from Fundação de Amparo á Pesquisa do Estado do Amazonas (FAPEAM). Lisa Davenport kindly reviewed an earlier version of the manuscript, providing many comments and editions. We are particularly grateful to the two reviewers whose comments and suggestions greatly improved the final version of this manuscript.
Supplementary Material
The Supplementary Material for this article can be found online at: https://www.frontiersin.org/articles/10.3389/fevo.2022.846975/full#supplementary-material
References
Agresti, A. (2007). An introduction to categorical data analysis. Second edition. Hoboken, NJ: John Wiley & Sons. doi: 10.1002/0470114754
Alerstam, T., Rosen, M., Beackman, J., Ericson, P. G. P., and Hellgren, O. (2007). Flight speeds among bird species: allometric and phylogenetic effects. PLoS Biol. 5:e197. doi: 10.1371/journal.pbio.0050197
Ausprey, I. J., Newell, F. L., and Robinson, S. K. (2021). Adaptations to light predict the foraging niche and disassembly of avian communities in tropical countrysides. Ecology 102:e03213. doi: 10.1002/ecy.3213
Ayres, J. M., and Clutton-Brock, T. H. (1992). River boundaries and species range size in Amazonian primates. Am. Nat. 140, 531–537. doi: 10.1086/285427
Ávila-Pires, T. C. S. (1995). Lizards of Brazilian Amazonia (Reptilia: squamata). Zool. Verh. 299, 1–706.
Bates, J. M. (2002). The genetic effects of forest fragmentation on five species of Amazonian birds. J. Avian Biol. 33, 276–294. doi: 10.1034/j.1600-048X.2002.330310.x
Bradfer-Lawrence, T., Gardner, N., and Dent, D. H. (2018). Canopy bird assemblages are less influenced by habitat age and isolation than understory bird assemblages in Neotropical secondary forest. Ecol. Evol. 8, 5586–5597. doi: 10.1002/ece3.4086
Brown, K. S. (1982). “Paleoecology and regional patterns of evolution in Neotropical forest butterflies,” in Biological diversification in the tropics, ed. G. T. Prance (New York: Columbia University Press), 255–308.
Bruderer, B., and Boldt, A. (2001). Flight characteristics of birds: i. Radar measurements of speeds. IBIS 143, 178–204. doi: 10.1111/j.1474-919X.2001.tb04475.x
Burkey, T. V. (1989). Extinction in nature reserves: the effect of fragmentation and the importance of migration between reserve fragments. Ecography 55, 75–81. doi: 10.2307/3565875
Burney, C. W., and Brumfield, R. T. (2009). Ecology Predicts Levels of Genetic Differentiation in Neotropical Birds. Am. Nat. 174, 358–368. doi: 10.1086/603613
Capparella, A. P. (1988). “Genetic Variation in Neotropical Birds:Implications for the Speciation Process,” in Acta XIX Congressus Internationalis Ornithological, ed. H. Ouellet (Ontario: University of Ottawa Press), 1658–1664.
Capparella, A. P. (1991). “Neotropical avian diversity and riverine barriers,” in Proceedings 20th International Ornithological Congress, ed. B. D. Bell (Wellington: Congressional Trust Board).
Choueri, E. L., Gubili, C., Borges, S. H., Thom, G., Sawakuchi, A. O., Soares, E. A., et al. (2017). Phylogeography and population dynamics of Antbirds (Thamnophilidae) from Amazonian fluvial islands. J. Biogeogr. 44, 2284–2294. doi: 10.1111/jbi.13042
Claramunt, S. (2021). Flight efficiency explains differences in natal dispersal distances in birds. Ecology. 102:e03442. doi: 10.1002/ecy.3442
Claramunt, S., Derryberry, E. P., Remsen, J. V. Jr., and Brumfield, R. T. (2012). High dispersal ability inhibits speciation in a continental radiation of passerine birds. Proc. R. Soc. B. 279, 1567–1574. doi: 10.1098/rspb.2011.1922
Claramunt, S., Hong, M., and Bravo, A. (2022). The effect of flight efficiency on gap-crossing ability in Amazonian forest birds. Biotropica. doi: 10.1111/btp.13109
Claramunt, S., and Wright, N. A. (2017). “Using museum specimens to study flight and dispersal,” in Emerging Frontiers in Collections-based Ornithological research: the Extended Specimen. Studies in Avian Biology 50, ed. M. S. Webster (Chicago: American Ornithological Society).
Cracraft, J. (1985). Historical biogeography and patterns of differentiation within the South American avifauna: areas of endemism. Ornithol. Monogr. 36, 49–84. doi: 10.2307/40168278
Diamond, J. M. (1981). Flightlessness and fear of flying in island species. Nature 293, 507–508. doi: 10.1038/293507a0
Fair, J., Paul, E., and Jones, J. (eds) (2010). Guidelines to the Use of Wild Birds in Research. Washington, DC: Ornithological Council.
Fernandes, A. M., Cohn-Haft, M., Hrbek, T., and Farias, I. P. (2014). Rivers acting as barriers for bird dispersal in the Amazon. Rev. Bras. Ornitol. 22, 361–371. doi: 10.1111/mec.14142
Foggo, A., Ozanne, C. M., Speight, M. R., and Hambler, C. (2001). “Edge effects and tropical forest canopy invertebrates,” in Tropical Forest Canopies: Ecology and Management, eds K. E. Linsenmair, A. J. Davis, B. Fiala, and M. R. Speight (Dordrecht: Springer), 347–359. doi: 10.1007/978-94-017-3606-0_28
Fouquet, A., Ledoux, J. B., Dubut, V., Noonan, B. P., and Scotti, I. (2012). The interplay of dispersal limitation, rivers, and historical events shapes the genetic structure of an Amazonian frog. Biol. J. Linn. Soc. 106, 356–373. doi: 10.1111/j.1095-8312.2012.01871.x
Freckleton, R. P., Harvey, P. H., and Pagel, M. (2002). Phylogenetic analysis and comparative data: a test and review of evidence. Am. Nat. 160, 712–726. doi: 10.1086/343873
Goulding, M., Barthem, R., and Ferreira, E. J. G. (2003). The Smithsonian atlas of the Amazon. Washington, DC: Smithsonian Institution Press.
Grafen, A. (1989). The phylogenetic regression. Philosophical Transactions of the Royal society of London. Series B. Biol. Sci. 326, 119–157. doi: 10.1098/rstb.1989.0106
Hackett, S. J., Kimball, R. T., Reddy, S., Bowie, R. C. K., Braun, E. L., Braun, M. J., et al. (2008). A phylogenomic study of birds reveals their evolutionary history. Science 320, 1763–1768. doi: 10.1126/science.1157704
Haffer, J. (1969). Speciation in Amazonian forest birds: most species probably originated in forest refuges during dry climatic periods. Science 165, 131–137. doi: 10.1126/science.165.3889.131
Haffer, J. (1992). On the “river effect” in some forest birds of southern Amazonia. Bol. Mus. Para. Emilio Goeldi. Sér.Zool. 8, 217–245.
Hartfelder, J., Reynolds, C., Stanton, R. A., Sibiya, M., Monadjem, A., McCleery, R. A., et al. (2020). The allometry of movement predicts the connectivity of communities. Proc. Natl. Acad. Sci. U. S. A. 117, 22274–22280. doi: 10.1073/pnas.2001614117
Harvey, M. G., Aleixo, A., Ribas, C. C., and Brumfield, R. T. (2017). Habitat association predicts genetic diversity and population divergence in Amazonian birds. Am. Nat. 190, 631–648. doi: 10.1086/693856
Ibarra-Macias, A., Robinson, W. D., and Gaines, M. S. (2011). Experimental evaluation of bird movements in a fragmented Neotropical landscape. Biol. Conserv. 144, 703–712. doi: 10.1016/j.biocon.2010.08.006
Jetz, W., Thomas, G. H., Joy, J. B., Hartmann, K., Redding, D., and Mooers, A. O. (2014). Distribution and conservation of global evolutionary distinctness in birds. Curr. Biol. 24, 919–930. doi: 10.1016/j.cub.2014.03.011
Laranjeiras, T. O., Naka, L. N., Bechtoldt, C. L., Costa, T. V. V., Andretti, C. B., Cerqueira, M. C., et al. (2014). The avifauna of Viruá National Park, Roraima: megadiversity in northern Amazonia. Rev. Bras. Ornitol. 22, 138–171. doi: 10.1007/BF03544242
Laranjeiras, T. O., Naka, L. N., and Cohn-Haft, M. (2019). Using river color to predict Amazonian floodplain forest avifaunas in the world’s largest blackwater river basin. Biotropica 51, 330–341. doi: 10.1111/btp.12650
Laranjeiras, T. O., Naka, L. N., Leite, G. A., and Cohn-Haft, M. (2021). Effects of a major Amazonian river confluence on the distribution of floodplain forest avifauna. J. Biogeogr. 48, 847–860. doi: 10.1111/jbi.14042
Laurance, S. G. W., Stouffer, P. C., and Laurance, W. F. (2004). Effects of road clearings a movement patterns of understory rainforest birds in central Amazonia. Conserv. Biol. 18, 1099–1109. doi: 10.1111/j.1523-1739.2004.00268.x
Lees, A. C., and Gilroy, J. J. (2014). Vagrancy fails to predict colonization of oceanic islands. Glob. Ecol. Biogeogr. 23, 405–413. doi: 10.1111/geb.12129
Lees, A. C., and Peres, C. A. (2008). Avian life-history determinants of local extinction risk in a hyper-fragmented neotropical forest landscape. Anim. Conserv. 11, 128–137. doi: 10.1111/j.1469-1795.2008.00162.x
Lees, A. C., and Peres, C. A. (2009). Gap-crossing movements predict species occupancy in Amazonian forest fragments. Oikos 118, 280–290. doi: 10.1111/j.1600-0706.2008.16842.x
MacArthur, R. H., and Wilson, E. O. (1967). The Theory of Island Biogeography. Princeton: Princeton University Press.
Martins, E. P., and Hansen, T. F. (1997). Phylogenies and the comparative method: a general approach to incorporating phylogenetic information into the analysis of interspecific data. Am. Nat. 149, 646–667. doi: 10.1086/286013
Moore, R. P., Robinson, W. D., Lovette, I. J., and Robinson, T. R. (2008). Experimental evidence for extreme dispersal limitation in tropical forest birds. Ecol. Lett. 11, 960–968. doi: 10.1111/j.1461-0248.2008.01196.x
Musher, L. J., Giakoumis, M., Albert, J., Del-Rio, G., Rego, M., Thom, G., et al. (2022). River network rearrangements promote speciation in lowland Amazonian birds. Sci. Adv. 8:eabn1099. doi: 10.1126/sciadv.abn1099
Naka, L. N. (2011). Avian distribution patterns in the Guiana Shield: implications for the delimitation of Amazonian areas of endemism. J. Biogeogr. 38, 681–696. doi: 10.1111/j.1365-2699.2010.02443.x
Naka, L. N., Bechtoldt, C. L., Henriques, L. M. P., and Brumfield, R. T. (2012). The Role of Physical Barriers in the Location of Avian Suture Zones in the Guiana Shield. Northern Amazonia. Am. Nat. 179, E115–E132. doi: 10.1086/664627
Naka, L. N., and Brumfield, R. T. (2018). The dual role of Amazonian rivers in the generation and maintenance of avian diversity. Sci. Adv. 4:eaar8575. doi: 10.1126/sciadv.aar8575
Naka, L. N., Cohn-Haft, M., Mallet-Rodrigues, F., Santos, M. P. D., and Torres, M. F. (2006). The Avifauna of the Brazilian state of Roraima: bird distribution and biogeography in the Rio Branco basin. Rev. Bras. Ornitol. 14, 191–232.
Naka, L. N., Cohn-Haft, M., Whittaker, A., Mazar Barnet, J., and Torres, M. F. (2007). Avian biogeography of Amazonian flooded forest in the Rio Branco basin Brazil. Wilson J. Ornithol. 119, 439–449. doi: 10.1676/06-062.1
Naka, L. N., Laranjeiras, T. O., Lima, G. R., Plaskievicz, A. C., Mariz, D., Costa, B. M. S., et al. (2020). The Avifauna of the Rio Branco, an Amazonian evolutionary and ecological hotspot in peril. Bird. Conserv. Int. 30, 21–39. doi: 10.1017/S0959270919000133
Nazareno, A. G., Knowles, L. L., Dick, C. W., and Lohmann, L. G. (2021). By Animal, Water, or Wind: can Dispersal Mode Predict Genetic Connectivity in Riverine Plant Species? Front. Plant Sci. 12:626405. doi: 10.3389/fpls.2021.626405
Neuschulz, E. L., Brown, M., and Farwig, N. (2013). Frequent bird movements across a highly fragmented landscape: the role of species traits and forest matrix. Anim. Conserv. 16, 170–179. doi: 10.1111/j.1469-1795.2012.00582.x
Nilsson, C., Bäckman, J., and Alerstam, T. (2014). Seasonal modulation of flight speed among nocturnal passerine migrants: differences between short-and long-distance migrants. Behav. Ecol. Sociobiol. 68, 1799–1807. doi: 10.1007/s00265-014-1789-5
Norberg, U. M. (1990). Vertebrate Flight. Mechanics, Physiology, Morphology, Ecology and Evolution. Germany: Springer-Verlag. doi: 10.1007/978-3-642-83848-4
Ottaviani, D., Cairns, S. C., Oliverio, M., and Boitani, L. (2006). Body mass as a predictive variable of home-range size among Italian mammals and birds. J. Zool. 269, 317–330. doi: 10.1111/j.1469-7998.2006.00060.x
Pacheco, J. F., Silveira, L. F., Aleixo, A., Agne, C. E., Bencke, G. A., Bravo, G. A., et al. (2021). Annotated checklist of the birds of Brazil by the Brazilian Ornithological Records Committee—second edition. Ornithol. Res. 29, 94–105. doi: 10.1007/s43388-021-00058-x
Pagel, M. (1999). Inferring the historical patterns of biological evolution. Nature 401, 877–884. doi: 10.1038/44766
Paradis, E., and Claude, J. (2002). Analysis of comparative data using generalized estimating equations. J. Theor. Biol. 218, 175–185. doi: 10.1006/jtbi.2002.3066
Paradis, E., and Schliep, K. (2019). ape 5.0: an environment for modern phylogenetics and evolutionary analyses in R. Bioinformatics 35, 526–528. doi: 10.1093/bioinformatics/bty633
Pennycuick, C. J. (1982). The ornithodolite: an instrument for collecting large samples of bird speed measurements. Philos. Trans. R. Soc. Lond. Ser. B. 300, 61–73. doi: 10.1098/rstb.1982.0157
Pennycuick, C. J. (2008). Modeling the Flying Bird. Burlington.: Academic Press Theoretical Ecology Series.
Pennycuick, C. J., Åkesson, S., and Hedenström, A. (2013). Air speeds of migrating birds observed by ornithodolite and compared with predictions from flight theory. J. R. Soc. Interface 10:20130419. doi: 10.1098/rsif.2013.0419
Pirani, R. M., Werneck, F. P., Thomaz, A. T., Kenney, M. L., Sturaro, M. J., Ávila-Pires, T. C., et al. (2019). Testing main Amazonian rivers as barriers across time and space within widespread taxa. J. Biogeogr. 46, 2444–2456. doi: 10.1111/jbi.13676
Revell, L. J. (2012). phytools: an R package for phylogenetic comparative biology (and other things). Methods Ecol. Evol. 3, 217–223. doi: 10.1111/j.2041-210X.2011.00169.x
Ribas, C. C., Aleixo, A., Nogueira, A. C. R., Miyaki, C. Y., and Cracraft, J. (2012). A palaeobiogeographic model for biotic diversification within Amazonia over the past three million years. Proc. Royal Soc. Lond. Series B Biol. Sci. 279, 681–689. doi: 10.1098/rspb.2011.1120
Ríos-Villamizar, E. A., Piedade, M. T. F., Da Costa, J. G., Adeney, J. M., and Junk, W. J. (2013). Chemistry of different Amazonian water types for river classification: a preliminary review. Trans. Ecol. Environ. 178, 17–28. doi: 10.2495/WS130021
Rosenberg, G. H. (1990). Habitat specialization and foraging behavior by birds of Amazonian river islands in northeastern Peru. Condor 92, 427–443. doi: 10.2307/1368240
Rosser, N., Shirai, L. T., Dasmahapatra, K. K., Mallet, J., and Freitas, A. V. (2021). The Amazon river is a suture zone for a polyphyletic group of co-mimetic heliconiine butterflies. Ecography 44, 177–187. doi: 10.1111/ecog.05282
Rowedder, A. R. P., Laranjeiras, T. O., Haugaasen, T., Gilmore, B., and Cohn-Haft, M. (2021). Response of understory avifauna to annual flooding of Amazonian floodplain forests. Forests 12:1004. doi: 10.3390/f12081004
Santorelli, S. Jr., Magnusson, W. E., de Deus, C. P., and Keitt, T. H. (2022). Neutral processes and reduced dispersal across Amazonian rivers may explain how rivers maintain species diversity after secondary contact. Perspect. Ecol. Conserv. in press doi: 10.1016/j.pecon.2021.12.004
Schliep, K. P. (2011). Phangorn: phylogenetic analysis in R. Bioinformatics 27, 592–593. doi: 10.1093/bioinformatics/btq706
Sheard, C., Neate-Clegg, M. H. C., Alioravainen, N., Jones, S. E. I., Vincent, C., MacGregor, H. E. A., et al. (2020). Ecological drivers of global gradients in avian dispersal inferred from wing morphology. Nat. Commun. 11, 1–9. doi: 10.1038/s41467-020-16313-6
Stouffer, P. C., and Bierregaard, R. O. Jr. (1995). Use of Amazonian forest fragments by understory insectivorous birds. Ecology 76, 2429–2443. doi: 10.2307/2265818
Stouffer, P. C., and Bierregaard, R. O. Jr. (1993). Spatial and temporal abundance patterns of Ruddy Quail-Doves (Geotrygon montana) near Manaus, Brazil. Condor 95, 896–903. doi: 10.2307/1369427
Suhonen, J., Norrdahl, K., and Korpimaki, E. (1994). Avian predation risk modifies breeding bird community on a farmland area. Ecology 75, 1626–1634. doi: 10.2307/1939623
Thom, G., Amaral, F. R. D., Hickerson, M. J., Aleixo, A., Araujo-Silva, L. E., Ribas, C. C., et al. (2018). Phenotypic and genetic structure support gene flow generating gene tree discordances in an Amazonian floodplain endemic species. Syst. Biol. 67, 700–718. doi: 10.1093/sysbio/syy004
Tourinho, L., Prevedello, J. A., Carvalho, B. M., Rocha, D. S., and Vale, M. M. (2022). Macroscale climate change predictions have little influence on landscape-scale habitat suitability. Perspect. Ecol. Conserv. 20, 29–37. doi: 10.1016/j.pecon.2021.10.003
Willis, E. O. (1974). Populations and local extinctions of birds on Barro Colorado Island, Panama. Ecol. Monogr. 44, 153–169. doi: 10.1126/science.179.4075.759
Keywords: avian flight, riverine barriers, dispersal experiments, flight speed, ecological predictors
Citation: Naka LN, Costa BMS, Lima GR and Claramunt S (2022) Riverine Barriers as Obstacles to Dispersal in Amazonian Birds. Front. Ecol. Evol. 10:846975. doi: 10.3389/fevo.2022.846975
Received: 31 December 2021; Accepted: 18 May 2022;
Published: 04 July 2022.
Edited by:
Daniel de Paiva Silva, Goiano Federal Institute (IFGOIANO), BrazilReviewed by:
Mariana M. Vale, Federal University of Rio de Janeiro, BrazilAlexander Charles Lees, Manchester Metropolitan University, United Kingdom
Copyright © 2022 Naka, Costa, Lima and Claramunt. This is an open-access article distributed under the terms of the Creative Commons Attribution License (CC BY). The use, distribution or reproduction in other forums is permitted, provided the original author(s) and the copyright owner(s) are credited and that the original publication in this journal is cited, in accordance with accepted academic practice. No use, distribution or reproduction is permitted which does not comply with these terms.
*Correspondence: Luciano N. Naka, THVjaWFuby5uYWthQHVmcGUuYnI=