- 1Department of Environment and Geography, University of Manitoba, Winnipeg, MB, Canada
- 2Department of Environmental Studies and Science and Biology, University of Winnipeg, Winnipeg, MB, Canada
The invasive emerald ash borer (Agrilus planipennis Fairmaire; Coleoptera: Buprestidae) has killed tens of millions of ash (Fraxinus spp.) trees across North America. A. planipennis was first detected in Winnipeg, Manitoba in 2017 and has the potential to become a serious threat to the city's ash canopy which accounts for ~30% of the public tree inventory. The goal of this study was to predict when adult A. planipennis emergence and peak activity would occur in Winnipeg to help logistical planning for the implementation of a city-wide management program. The management program would focus on detection and limiting the spread of the beetle with the objective of preserving ash trees as long as possible allowing for more proactive management of the EAB infestation. To predict adult emergence and peak activity of A. planipennis, we used local weather station data to calculate the number of degree-days accumulated in each year for the 1970–2019 period using three different degree-day accumulation models. Developmental thresholds for A. planipennis were derived from previous North American studies. The estimated mean emergence dates for the 50-year period were June 14 ± 8.5 days (double sine model), June 14 ± 8.5 days (single sine model), and June 19 ± 9.1 days (standard model) whereas the peak activity dates were July 16 ± 8.8 days (double sine model), July 17 ± 8.7 days (single sine model), and July 21 ± 9.4 days (standard model). Meteorological records indicate that temperatures in the Winnipeg region have increased over the study period. However, our predicted emergence dates do not significantly differ over the 50 years examined in the study, although estimated peak activity dates are significantly earlier, suggesting that EAB movement may benefit from climate change. The results from this study will provide managers with information regarding the temporal behavior of A. plannipennis in Winnipeg allowing for improved timing of control measures and monitoring, thereby extending the projected life span of a significant ash tree population within the Winnipeg urban region. The management model developed for Winnipeg could serve as an example for other locations in the prairie region of North America.
Introduction
The emerald ash borer (EAB; Agrilus planipennis Fairmaire; Coleoptera: Buprestidae) is an invasive wood boring beetle that was first detected in North America near Detroit, Michigan and Windsor, Ontario in 2002 (Haack et al., 2002; Poland and McCullough, 2006). Dendrochronological evidence suggests that EAB likely became established in North America in the early 1990s (Siegert et al., 2014) and has since killed tens of millions of ash (Fraxinus spp.) trees (McCullough et al., 2009a; Herms and McCullough, 2014). Within the current North American distribution of EAB, ash trees in forested sites have experienced as much as 100% mortality associated with significant economic and ecological cost (Flower et al., 2013; Herms and McCullough, 2014; Klooster et al., 2014, 2018). Complete mortality of affected urban ash stands is usually observed within 6 years of initial infestation (Knight et al., 2013); however, individual mature ash trees can die in 3–5 years after becoming infested (Wang et al., 2010). EAB is difficult to detect at low densities at the beginning of an infestation because infestations generally begin in the upper tree canopy and few visible symptoms are present (Cappaert et al., 2005). Locating newly infested trees and trees with low densities of EAB is also difficult because these trees do not exhibit the external symptoms that characterize heavily infested trees (McCullough et al., 2009b). Since its detection in 2002, EAB has killed ash trees in forest, riparian, and urban areas becoming one of the most destructive and costly forest insects to ever invade North America (Aukema et al., 2011).
EAB is native to northeastern China, the Korean peninsula, Japan, Mongolia, and eastern Russia (Baranchikov et al., 2008; Valenta et al., 2017). In its native range, EAB functions as a secondary pest colonizing and killing unhealthy ash trees (Liu et al., 2003). However, North American ash species have no evolutionary history with EAB and thus are very susceptible to the insect (Villari et al., 2016; Valenta et al., 2017). While unhealthy trees are preferred, EAB also attacks and kills healthy trees in North America meaning that native ash species could eventually be extirpated from North American forest systems (Herms and McCullough, 2014; Klooster et al., 2014). Six native ash species across North America are threatened by EAB. These include white ash (Fraxinus americana L.), green ash (Fraxinus pensylvanica Marsh.), black ash (Fraxinus nigra Marsh.), blue ash (Fraxinus quadrangulata Michx.), Carolina ash (Fraxinus caroliniana Mill.), and pumpkin ash (Fraxinus profunda (Bush) Bush). All native North American ash species within the EAB's current invasive range are known to be susceptible to EAB attack (Poland and McCullough, 2006) and the continued loss of mature ash trees from forest communities will present challenges for conserving genetic diversity in ash (Granger et al., 2020). The most abundant ash species in North America are white, green, and black ash (Herms and McCullough, 2014). These three species especially serve as potential hosts for EAB and face extirpation from forest communities (Villari et al., 2016). All three of these species are highly susceptible and are critically endangered because of the threat posed by EAB (Steiner et al., 2019; IUCN, 2021). Green, white, and black ash may experience functional extirpation to the point that they no longer provide significant ecosystem functions and services as EAB continues to spread (Flower et al., 2013). Since its introduction, EAB has expanded its range significantly and now threatens ash trees in 35 US states (USDA, 2021) and five Canadian provinces (Canadian Food Inspection Agency, 2021).
Ecologically, EAB also threatens the biological integrity of forests where ash is a major component thus significantly impacting the species that depend on them. Ash species provide cover and food for several small animal species (Brakie, 2013), many bird species use ash for habitat, food, nesting sites, and roosts (Twedt and Best, 2004), and ash trees provide browse, thermal cover, and protection for a variety of wildlife species (Liu, 2018). Wagner and Todd (2016) reported 21 species of ash-feeding moths which also depends on the survival of ash trees. In addition, 43 native arthropod species exclusively use ash for feeding or breeding purposes (Gandhi and Herms, 2010). Additionally, canopy space opened by the death of ash trees may be occupied over time by invasive plants which will lead to further habitat alteration, species displacement, and the loss of species diversity (Liu, 2018). The loss of ash due to EAB will likely result in native species diversity that is dependent on ash being reduced or lost (Schrader et al., 2021).
EAB was first detected in Winnipeg, Manitoba in December 2017 (Government of Canada, 2017) and threatens Winnipeg's extensive ash inventory. Based on the City of Winnipeg Public Tree Inventory (City of Winnipeg, 2017), there are 85,005 public green ash trees and 8,672 public black ash trees. The combination of green and black ash comprises ~30% of all public trees in Winnipeg (K. LaFrance, Pers. Comm., City of Winnipeg Urban Forestry Branch, August 5, 2020). Public trees in this study refer to those that are located on boulevards and city parks. In addition, there are ~240,857 green ash trees and 6,534 black ash trees on private property (K. LaFrance, Pers. Comm., City of Winnipeg Urban Forestry Branch, August 5, 2020). There are other ash cultivars in Winnipeg that are known to have various levels of susceptibility to EAB. These species include white ash, Northern treasure ash (Fraxinus x ‘Northern Treasure’), and Mancana Manchurian ash (Fraxinus mandshurica ‘Mancana’). However, given that these species account for 1.5% of Winnipeg's public tree inventory and 2.8% of Winnipeg's private ash inventory, the threat that EAB poses to Winnipeg's green and black ash canopy is of more serious concern.
The calculation of degree-day accumulations is often used to predict the timing of insect development stages and can be used to predict adult emergence dates of insects (Dearborn and Westwood, 2014). Degree-day calculations have been widely used to increase the precision of insecticide applications, and to improve integrated pest management techniques for both invasive and endemic insect pests (Baek et al., 2008; Mironidis et al., 2010; Thöming and Saucke, 2011; Dearborn and Westwood, 2014). The prediction of some or all of an insect's development using a degree-day model requires determination of the lower development threshold temperature for that species (Dearborn and Westwood, 2014). The lower development threshold temperature (LTT) is the temperature point at which an immature insect undergoes development and below which no development occurs. Thus, once the immature insect either begins or resumes development above the LTT, it begins accumulating degree-days. The LTT may vary within an individual species and across various life stages; however, a single threshold temperature has often been used for several larval instars, or several different developmental stages of the same species (O'Neal et al., 2011; Son et al., 2012). On the other hand, upper development threshold temperatures (UTT) are the point at which development slows due to excessive heat (O'Neal et al., 2011; Son et al., 2012).
Once the LTT and UTT have been determined, several methods are available to calculate degree-days. Most methods of calculation are based on the assumption that the relationship between insect development rate and temperature is linear (Johansen, 1997; University of California, 2016). In reality, the relationship between these two variables is usually non-linear, especially near the upper and lower developmental thresholds (Régnière et al., 2012; Son et al., 2012). Sine models generally better reflect the growth rates for insects as development may be more non-linear close to the upper and lower developmental thresholds (Herms, 2004; Murray, 2008). However, linear degree-day models have been demonstrated to work well-enough for practical purposes, such as timing of insect life stages for pest control (Johansen, 1997; O'Neal et al., 2011; University of California, 2016). A simple, but widely used method, involves taking the average daily temperature and subtracting the LTT of the specific development stage(s) of the species under study [(max daily temp + min daily temp)/2 – LTT]. This is called the standard model (Dearborn and Westwood, 2014). A more complex model fits the daily maximum and minimum temperatures to a sine wave and then calculates the area of the curve above the LTT, represented by a horizontal line, for each day of the insect's development. This can be done using the single sine or double sine method. The single sine method only considers the LTT and does not have a vertical cut-off whereas the double sine method considers both the LTT and a vertical cut-off. The vertical cut-off assumes that no development occurs above the UTT. Other cut-off methods include the horizontal cut-off which assumes development continues at a constant rate at temperatures above the UTT and the intermediate cut-off which assumes development slows, but does not stop, at temperatures above the UTT (University of California, 2016).
Several studies report the threshold temperatures and degree-day requirements for EAB. Adult EAB emergence generally begins in late spring at 230–260 degree-days base=LTT 10°C (DD10) and emergence continues throughout the summer (Cappaert et al., 2005; Poland et al., 2011; Gould et al., 2016; Herms et al., 2019). Herms et al. (2019) used a biofix date (the starting date for degree-day calculations) of January 1 while the other studies did not mention a biofix date. Peak adult flight activity (peak activity) generally occurs in late June or early July at 514–556 degree-days (DD10) and drops off sharply by the end of July at about 833 degree-days (DD10) as the beetles begin to die off and new emergence declines (Brown-Rytlewski and Wilson, 2004; McCullough et al., 2009b; Poland et al., 2011; Gould et al., 2016; Herms et al., 2019). EAB has a 1 or 2-year life cycle depending on geographical location and infestation stage (Liu, 2018). In unhealthy trees within the invasive range, nearly all EAB develop within 1-year with larvae feeding from mid-summer into autumn during which time they complete four instars before they overwinter as prepupae under the bark (Wang et al., 2010). Although EAB generally has a 1-year life cycle, it has been reported that 2 years may be required to complete development. A longer life cycle may be adaptive in northern locations with cooler climates, when EAB attack densities are low, hosts are vigorous, or when oviposition occurs in late summer (Cappaert et al., 2005; Tluczek et al., 2011; Tussey et al., 2018). In North America, a 1 and 2-year larval development cycle can occur in the same location and even on the same trees (McCullough et al., 2009b).
In this study, our objective was to determine when adult EAB emergence and peak activity thresholds would have been reached in Winnipeg using weather station data from the past 50 years. We developed estimates for a range of days that first adult emergence and peak adult activity would have occurred in Winnipeg based on development data from other jurisdictions. We wanted to determine if climate warming would have a significant impact on the emergence and peak activity periods in Winnipeg and if continued climate warming would make it necessary to update prediction estimates regularly. Prediction of first adult emergence and peak adult activity in Winnipeg will allow pest control managers to better plan and execute EAB control initiatives and develop long-term management strategies. Currently, it is unknown if EAB can complete its life cycle in 1 year in Winnipeg or if a second year is needed to complete adult development. If EAB requires 2 years to complete its life cycle, this would have significant implications for management of this species in Winnipeg.
Methods
Historic annual temperature records in Winnipeg were analyzed for the previous 50 years (1970–2019) to perform degree-day calculations that predict EAB adult emergence and peak activity using the LTTs and UTTs reported in the literature. This 50-year period was chosen so that potential decadal differences could be examined prior to some of the first reported cases of EAB in Winnipeg, and to determine if there are temperature trends that might influence emergence and peak activity dates predicted by the GDD model outcomes. Our prediction is that climate warming will change the emergence and peak activity dates in the future; therefore, determining temperature trends may become increasingly important for effective management. Temperature records from the Environment Canada weather station at the Richardson International Airport on the northwest edge of Winnipeg (49° 54' 21.59” N; −97° 14' 14.40” W) were analyzed for the years 1970–1999 and similar records from the Environment Canada weather station located in central Winnipeg (49° 53' 18” N; 97° 7' 47 W) were analyzed for the years 2000–2019. While the distance between the two weather stations is only 7.1 kilometers; the weather data from the central Winnipeg station was used whenever possible as this location better represents a more developed urban environment and associated temperature conditions in the heart of Winnipeg's urban forest where EAB has been found.
Each temperature dataset consisted of the daily minimum and maximum temperature for every day of the year. There were infrequent gaps in the meteorological record for Winnipeg and in these instances, temperatures from the Steinbach weather station (49.53°N, 96.69°W) were used. This station is located 51.5 km from central Winnipeg and 58.5 km from the Richardson International Airport weather stations. For data gaps involving all of the stations in this study, values were interpolated by averaging the temperatures for the days prior and following the missing day. In total over the 50 years used in this study, there were 73 days with missing data (see Supplementary Material). We used data for 57 of these days from the Steinbach weather station and the remaining 16 days were interpolated using the averaging method described above.
GDD Model Preparation
The double sine, single sine, and standard degree-day models were run for the 50-year study period. The double sine and single sine models were run using the degree-day calculator from the University of California Integrated Pest Management Program http://ipm.ucanr.edu/WEATHER/index.html. This calculator requires that certain parameters be fulfilled to run any model. These parameters include the temperature unit, the LTT, the UTT (optional), the method of calculation (model), and the cutoff method (optional). Degree-days were calculated using the double sine model with a LTT of 10°C (Brown-Rytlewski and Wilson, 2004; Poland et al., 2011; Herms et al., 2019) and a UTT of 37.8°C (Pest Prophet, 2021) with a vertical cut-off. The vertical cut-off assumes that no development occurs above the UTT (37.8°C). Degree-days were calculated using the single sine model with a LTT of 10°C and no UTT. The standard model was also calibrated based on a LTT of 10°C. The Julian date was recorded as well as the calendar date in which the GDD 250 and GDD 550 thresholds occurred in each year and were recorded for each model. The thresholds used in this study were chosen based on results published from jurisdictions closest geographically and climatologically to Winnipeg, including: (Brown-Rytlewski and Wilson, 2004; Cappaert et al., 2005; McCullough et al., 2009a; Poland et al., 2011). For these studies, the observed degree-day range for adult emergence was 230–260 degree-days (DD10) and the range for peak adult activity was 514–556 degree-days (DD10). We chose GDD 250 and GDD 550 as median threshold values based on mid-range activity ranges provided by these other studies to account for Winnipeg's more northern location. The biofix date selected was January 1.
Six degree-day model combinations were tested (GDD 250 double sine, GDD 550 double sine, GDD 250 single sine, GDD 550 single sine, GDD 250 standard, and GDD 550 standard) with each combination resulting in a degree-day indicator value for either first adult emergence or peak activity. We tested for significance between the decades by expressing adult emergence and peak activity as the number of days from January 1 (Julian date). Years were pooled by decade (1970–1979 to 1970s, 1980–1989 to 1980s, 1990–1999 to 1990s, 2000–2009 to 2000s, and 2010–2019 to 2010s) to determine if the model predictions were significantly different. The decadal breakdown is useful for a general comparison across the study period (50-years) especially for local managers. The blocking of decades also helps reduce the year to year variation.
Statistical Analysis
After the creation of the six degree-day model combinations, data for each variable were tested for departure from the normal distribution and Levene's test was used to ensure equality of variances between treatments (Dytham, 2011). An ANOVA test was used to compare degree-day accumulations for first emergence and peak activity between decades and when the ANOVA was significant, a Bonferroni (with correction) post-hoc test was used to test means (Dytham, 2011). Linear regressions were run for each of the six model combinations for the entire 50-year study period for the purpose of determining if there was a difference in threshold dates/trends over time. Prior to all statistical analyses, variables were tested for evidence of non-linearity and equality of variance. All analyses were conducted at the α < 0.05 level of significance. All tests were run in SPSS (2017).
Results
Comparison of Differences Between Degree-Day Models
Estimated emergence dates for EAB ranged from 23 May to 30 June for the 50-year period across all six model-threshold combinations. For the 1970s, estimated emergence dates ranged from 23 May to 30 June; for the 1980s, estimated emergence dates ranged from 25 May to 30 June; for the 1990s, estimated emergence dates ranged from 31 May to 29 June; for the 2000s, estimated emergence dates ranged from 3 June to 28 June; for the 2010s, estimated emergence dates ranged from 3 June to 22 June.
Mean number of GDD (in Julian date) and standard deviations were calculated for the 50-year period for each of the six model-threshold combinations (Table 1) and by decade (Table 2). The double sine and single sine models had almost identical results; however, the standard model accrued the most GDD in all combinations tested (Tables 1, 2).
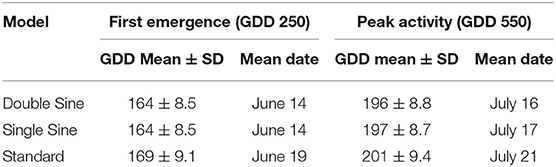
Table 1. Mean Growing Degree-Days (GDD) using the number of days from January 1 (Julian date) across the three models over the 50-year (1970–2019) period.
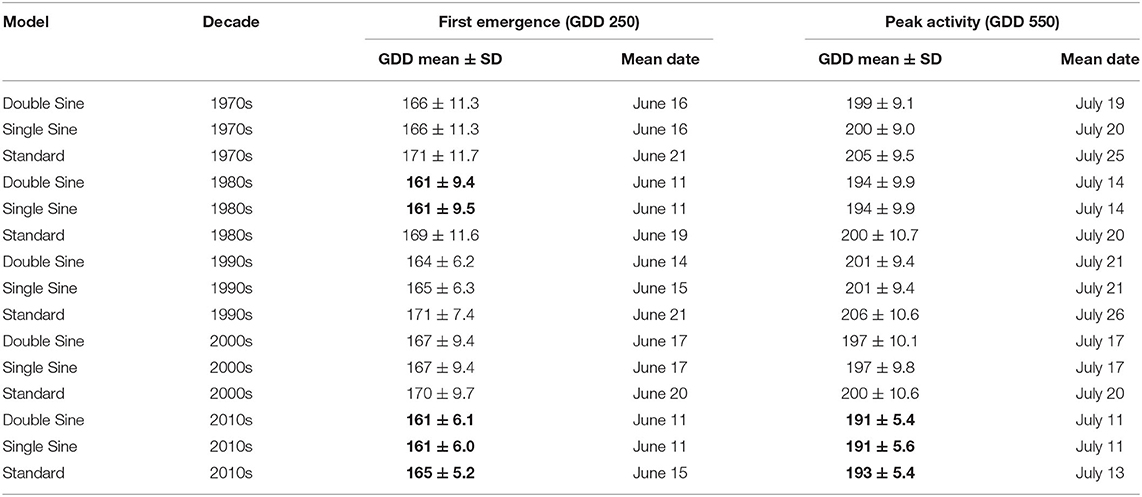
Table 2. Mean Growing Degree-Days (GDD) using the number of days from January 1 (Julian date) by decade across the three models (Bolded data are the lowest values in their respective threshold-model combinations which corresponds to the earliest emergence and peak activity dates).
Emergence (GDD 250) Results
The ANOVA of emergence degree-days between decades was not significant (GDD 250 double sine p = 0.479; GDD 250 single sine p = 0.488; GDD 250 standard p = 0.591).
The regression analysis of the full study period (50 years) showed for all models that there was no significant increase or decrease in the first emergence dates over the study period (GDD 250 double sine p = 0.685; GDD 250 single sine p = 0.677; GDD 250 standard p = 0.282) (Figure 1).
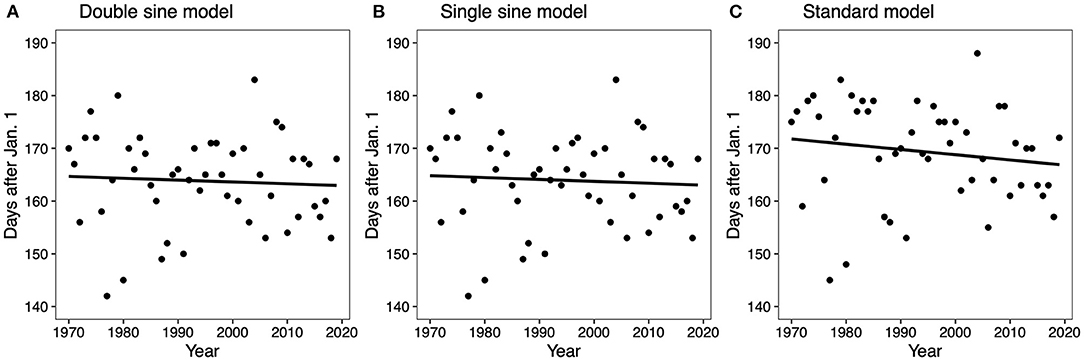
Figure 1. Linear regressions for the GDD 250 double sine model (A), the GDD 250 single sine model (B), and the GDD 250 standard model (C). All regressions were not significant (GDD 250 double sine F = 0.166, df = 49, p = 0.685, R2 = 0.003; GDD 250 single sine F =0.175, df = 49, p = 0.677, R2 = 0.004; GDD 250 standard F = 1.184, df = 49, p = 0.282, R2 = 0.024).
Peak Activity (GDD 550) Results
The ANOVA of peak activity degree-days between decades was significant for the GDD 550 standard model (p = 0.032) indicating difference between decades. The other models were not significant (GDD 550 double sine p = 0.097; GDD 550 single sine p = 0.097). Examination of the peak activity analysis showed that only the GDD 550 standard model accrued significantly more days (F = 2.90, df = 49, p = 0.032, η2 = 0.205). Post-hoc tests indicate that these differences are attributable to the 1990–1999 decade in comparison to the 2010–2019 decade (Table 3).
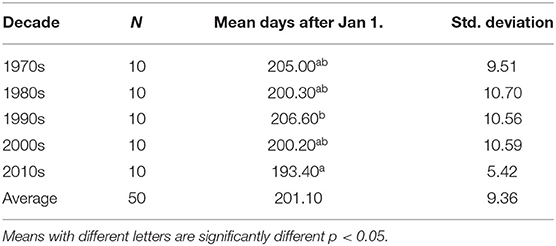
Table 3. The mean number of days from January 1 (Julian date) and standard deviations for each decade (group) for the GDD 550 standard model predicting adult peak activity.
The regression analysis of the full study period (50 years) showed a significant shift to an earlier date in peak activity for the GDD 550 standard model (R2 = 0.114, F = 6.16, p = 0.017) (Figure 2). The peak activity date for the GDD 550 standard model was July 25 (205 ± 9.5 days after January 1) in 1970–1979, and shifted to July 13 (193 ± 5.4 days after Jan. 1) in 2010–2019. The other models were not significant (GDD 550 double sine p = 0.09; GDD 550 single sine p = 0.095) (Figure 2).
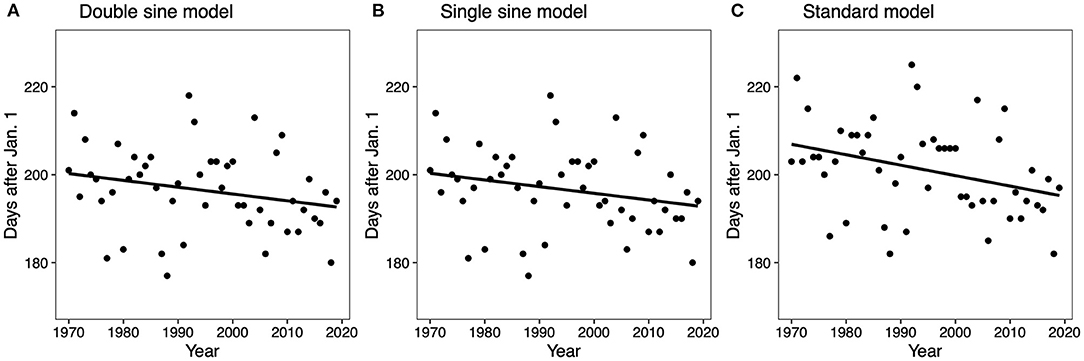
Figure 2. Linear regressions for the GDD 550 double sine model (A), the GDD 550 single sine model (B), and the GDD 550 standard model (C). The GDD 550 standard model was significant (F = 6.158, df = 49, p = 0.017, R2 = 0.114), while the other models were not significant (GDD 550 double sine F = 2.987, df = 49, p = 0.09, R2 = 0.059; GDD 550 single sine F = 2.899, df = 49, p = 0.095, R2 = 0.057).
Discussion
General Discussion
Using degree-day accumulations derived from weather station data to estimate EAB emergence and peak activity will help guide logistical planning for a city wide EAB management program in Winnipeg. We recommend using the results from the last decade analyzed (2010–2019) as at least one model showed a significant decline in the date for peak activity by EAB over the 50-year study period. Despite reports of increased temperatures and longer growing seasons in central North America (Liang and Fei, 2014; Cuddington et al., 2018) it appears that climate change that would affect EAB first emergence in the Winnipeg region are not profound enough to produce a significant change in first emergence data in most of the decades in this study. Our results did not show any significant changes in emergence dates and we did not detect increasing spring temperatures over the 50-year study period. Similarly, a study in eastern Manitoba that examined the effect of climate warming on butterfly phenology using degree-days found that there was no change in spring temperatures, but a significant increase in late summer and fall (Westwood and Blair, 2010). Given this result, first emergence predictions should be based on the most recent decadal data. Management activities that focus on early adult beetle detection (i.e., trap installation) are best initiated prior to the emergence date, which for Winnipeg, is June 11 ± 6.1 days (double sine model), June 11 ± 6.0 days (single sine model), June 15 ± 5.2 days (standard model). Management activities that could be implemented prior to the emergence date include setting artificial pheromone traps, branch sampling, and the establishment of trap trees by girdling them. It appears that the effect of climate change on the EAB peak activity range in Winnipeg over the 50-year study period is not consistent as only the GDD 550 standard model showed a significant earlier trend in the peak activity date. Given this result, the peak activity date should be monitored more closely in the future as peak activity dates may continue to trend earlier in the season with warming temperatures depending on the model used. The adult peak activity date could be used to better inform the planning of activities that aim to limit the spread of adult beetles, such as restrictions on ash, wood handling, and transport. Management activities that could be implemented with consideration of the peak activity date include conducting visual surveys for the beetles themselves, public outreach, and trap inspections.
Our analysis suggested that climate change in the Winnipeg region would not have significantly impacted the date of first emergence for EAB over the 50 years examined in the study had it been present. When comparing across the five decades, the earliest emergence dates for each model-threshold combination occurred in the 2010 decade. For the GDD 250 double and single sine models, the earliest date of emergence was at 161 Julian days (June 11), which occurred in both the 1980 and 2010 decades. The primary difference in these decades was slightly greater climate variability in the 1980s compared with the 2010s (double sine—std. 9.4 vs. 6.1; single sine—std. 9.5 vs. 6.0). The GDD 250 standard model's earliest emergence date occurred in the 2010 decade on June 15 (165 ± 5.2).
While there appears to be little change in emergence dates, the analysis suggested that GDD accumulations have been increasing during the summer in the Winnipeg region over time for one of the models. The earliest peak activity dates for each model-threshold combination also occurred in the 2010 decade (GDD 550 double sine model on July 11 191 ± 5.4; GDD 550 single sine model on July 11 191 ± 5.6; GDD 550 standard model on July 13 193 ± 5.4). The GDD 550 standard model was significant showing an earlier peak activity date. Although not significant, the double and single sine models also predict earlier peak activity dates. These findings suggest that summer temperatures have been increasing in the Winnipeg region and peak activity dates are becoming earlier as measured by at least one model. The trends that we observed in Winnipeg over the past 50 years are consistent with climatological data covering summer temperatures in the Winnipeg region (Prairie Climate Centre, 2018; Climate Atlas of Canada, 2019a). Other jurisdictions with confirmed EAB infestations have reported various levels of warming spring and summer temperatures including Detroit Michigan (GLISA, 2017); The Twin Cities, Minnesota (Minnesota Department of Health, 2021; Minnesota Pollution Control Agency, 2021); and London Ontario (Climate Atlas of Canada, 2019b).
The three models allowed us to develop three scenarios for adult emergence and peak activity dates. The models used to predict EAB emergence and peak activity periods in Winnipeg operate with different variables; therefore, comparisons between the models allows for the consideration of different biological factors that would control EAB immature development. However, it is difficult to determine which model is most accurate due to the lack of Winnipeg-specific EAB data. Further research should determine EAB's GDD thresholds (LTT and UTT) in Winnipeg which would help in resolving which model is more accurate.
Decadal analysis was used to determine if there were differences and trends within a 50-year period that includes the first cases of EAB in Winnipeg. By aggregating over decade periods, interannual variability was reduced. Additionally, given the distinct possiblitity of a 2-year life-cycle for EAB in areas with extreme and highly variable climate like Winnipeg, blocking these data over time was appropriate. Evaluation of management prescriptions will similarly need to consider how best to monitor over time considering the potential of a multi-year lag between infection and emergence. While we did not examine different temporal blocking strategies, the decadal analysis allowed for comparisons to determine how GDD for those parameters have historically changed in Winnipeg. The GDD 550 standard model showed statistically declining peak emergence dates but not the other models. This decreasing peak activity date is probably indicative of warmer summers. Westwood and Blair (2010) found that for the period 1971–2004, mean monthly temperatures in the autumn and winter increased significantly in southern Manitoba and the butterflies they were studying seemed to be responding to this warming trend over late summer and fall but did not find any changes in spring emergence. In another study, three decades (1982–2008) were analyzed to better understand seasonality shifts in the North American boreal forests (central Manitoba included) and they found that the region has experienced earlier springs especially in the western forests (Buermann et al., 2013). In our study we found earlier peak activity date estimates for the GDD 550 standard model but emergence date estimates and spring temperatures had no significant change.
Several studies have used GDD and/or air temperatures to predict life cycle timing of other wood-boring insects in North America. Mitton and Ferrenberg (2012) used temperature data from 1970 to 2008 to calculate annual and daily mean air temperatures for each year and degree-days were derived to predict development for the Mountain Pine Beetle (MPB, Dendroctonus ponderosae) in Colorado. They found that air temperatures have been increasing in Colorado over the previous two decades, and the flight season for the MPB occurred earlier in the year and was approximately twice as long as the historically reported season (Mitton and Ferrenberg, 2012). This is similar to our result for the GDD 550 standard model in that (peak) flight activity is becoming earlier in the year corresponding with increasing temperatures. Bentz et al. (2013) tested the influence of air temperature on the timing of adult emergence and flight for MPB in the western United States. They found that MPB life cycle timing is univoltine at warmer sites and a mix of univoltine and semivoltine at cooler sites. Other MPB studies have indicated that MPB populations have evolved local adaptations resulting in genetic differences in development times (Bentz et al., 2011; Bracewell et al., 2013). These studies on MPB suggest that increasing temperatures significantly impact the MPB's life cycle and flight activity, similar to what we found for EAB (GDD 550 standard model).
Kappel et al. (2017) predicted emergence and time to adult maturity for the Asian Longhorned Beetle (ALB; Anoplophora glabripennis) by studying the effects of temperature-dependent development and host species abundance throughout the contiguous United States. They found that the southern and eastern US states are at the greatest risk of infestation due to the warmer conditions which promote faster beetle maturation and population growth. Keena and Moore (2010) studied developmental thresholds for ALB by comparing degree-days, larval stages, and constant temperatures in the states of Illinois and New York, USA. The developmental thresholds used in this study were 10°C (LTT) and 30°C (UTT) which are very similar to the thresholds we used in our study. They found that temperature had a significant impact on ALB development as the relationship between temperature and development was linear between the LTT and UTT. We were unable to establish such a relationship in our study, but recommend that this be considered in future research. Duell et al. (2022) found that the extreme cold tolerance in Winnipeg EAB individuals is most likely due to phenotypic plasticity rather than genetic adaptation which allows the beetle to survive the harsh winter climate.
Poland et al. (2011) related the number of EAB captured (artificial traps) with GDD accumulations for various field sites in Michigan. GDD were calculated with an LTT of 10°C (DD10) in 2006–2008 to make recommendations for operational survey and trap program initiation to predict and manage EAB emergence and peak flight activities. McCullough et al. (2009b) studied how EAB attraction differs between girdled, wounded, or herbicide treated trees in Michigan. Cumulative GDD corresponding to the period of peak EAB activity were generally similar in each year (McCullough et al., 2009b). These studies demonstrated the usefulness of using GDD to predict EAB life cycle activites, and how the results can be used to assist EAB control and management measures.
1 vs. 2-Year Life Cycle
The results of this study summarize GDD accumulations in each year and do not consider the possibility of EAB larvae carrying accumulated GDD into a second year of development. Therefore, our results are reflective of a 1-year developmental life cycle for EAB in Winnipeg. Currently, it is unknown if EAB has a one or 2-year life cycle in southern Manitoba. Given the high level of winter cold tolerance by EAB in Manitoba (Duell et al., 2022), it will be important to determine the life cycle duration of this species in Winnipeg. To determine the probability of a 2-year life cycle, detailed sampling of infested trees is required over several years to understand how the immature stages are proportionally distributed within the population which was beyond the scope of our study. The duration of development varies from 1 to 2 years according to climatic conditions with most EAB developing in 1 year in regions with a warmer climate; developing in 2 years in regions with a colder climate; and in regions with an intermediate climate, a part of the population has a 1-year life cycle and another part has a 2-year life cycle (Orlova-Bienkowskaja and Bieńkowski, 2016; Duell et al., 2022). Ultimately, the speed of larval development is largely influenced by climate, primarily the duration of the warm period and the amount of heat that larvae receive in one season (Orlova-Bienkowskaja and Bieńkowski, 2016). In the southern part of its native range in China, EAB has a 1-year life cycle (Liu et al., 2007) with few larvae taking 2 years to complete development (Wang et al., 2010). In northern China, EAB's life cycle is almost always 2 years and larvae overwinter twice (Liu et al., 2007; Wei et al., 2007). In some locations, EAB populations may experience both 1 and 2-year life cycles such as Michigan (Cappaert et al., 2005). However, a 2-year cycle also appears to be more common in low-density EAB populations (Herms and McCullough, 2014; Orlova-Bienkowskaja and Bieńkowski, 2016).
Should evidence of a 2-year life cycle in Winnipeg be reported, the adult emergence and peak activity dates could differ from the results of this study. Overwintering larvae that require a second year of development will have already accumulated some GDD in their first year of development (Wang et al., 2010; Villari et al., 2016); and it is difficult to extrapolate the analysis to a second year without having local emergence and peak activity measurements to build upon. A 2-year life cycle may also have implications on how EAB management is carried out (Tluczek et al., 2011). The management approaches themselves would not change but rather the timelines for implementation would need to be adjusted as a 2-year cycle could result in different emergence and peak activity dates compared to our 1-year estimates.
Management Methods
There are several management tools that can help slow the spread and potential destruction of EAB. As previously mentioned, some methods are better undertaken prior to the adult emergence date such as setting artificial pheromone traps, branch sampling, the establishment of trap trees by girdling them, and visual surveys of EAB symptoms in the canopy. In operational survey programs, traps do not necessarily need to capture the first EAB beetles, but they should be in place before peak flight activity (Poland et al., 2011). If trapping surveys are to be implemented in Winnipeg, managers need to have traps in place before peak flight activity - July 11 (191 ± 5.4), July 11 (191 ± 5.6), or July 13 (193 ± 5.4) according to the double sine, single sine, and standard models, respectively. Managers in Winnipeg should have traps setup by late June to early July (340–460 DD10) to ensure that traps are in place for adult peak activity (Poland et al., 2011). Branch sampling is suitable for sampling open-grown ash in any landscape, but it is of particular value in urban areas with high-value ash trees (Ryall et al., 2011). Branch sampling can be performed at any time between September and May; however, because larvae continue to feed and grow in size in early fall, their galleries are easiest to see if branches are sampled after October (Ryall et al., 2011). Adult EAB beetles preferentially colonize unhealthy trees over healthy trees (Herms and McCullough, 2014; Klooster et al., 2014; McCullough, 2020); therefore, establishing trap trees by girdling them should be in place before peak activity to maximize trap catch. Conducting visual surveys to identify EAB-induced symptoms on ash trees is another tool that can be undertaken near emergence dates or in the late fall. Signs and symptoms of EAB infestation include crown thinning and dieback, epicormic shoots, woodpecker damage, bark splits, notched leaflets by adult feeding, D-shaped exit holes, and dead trees (Tluczek et al., 2011; Liu, 2018; City of Winnipeg, 2020). Some of these symptoms such as woodpecker damage, bark splitting, and exit holes are easiest to detect during the leaf-off period from approximately October – May (K. LaFrance, Pers. Comm., City of Winnipeg Urban Forestry Branch, August 5, 2020).
Alternatively, some methods are better undertaken just before or during the peak activity date such as visual surveys for adult beetles themselves, public outreach, and trap inspections. Conducting public outreach just prior to the peak activity date can raise public awareness of EAB symptoms and encourage vigilance and reporting which may assist managers in locating EAB problem areas. Additionally, increasing the frequency of trap (pheromone and girdled trees) inspections during peak activity may allow for more immediate detection and mitigation.
Limitations
Our study estimated GDD in a single calendar year. Our analyses and models estimated when the adult emergence and peak activity thresholds occur but did not estimate full life cycle GDD accumulation or within various developmental stages. Further research should concentrate on determining developmental thresholds and life stage GDD accumulations as well as determine life cycle length and possible proportion of the population that may take 2 years to develop to maturity which was beyond the scope of our study. A potential limitation to this study is it does not consider the impacts of winter minimums on EAB development and how extreme cold temperatures might influence the life cycle of EAB in Winnipeg. EAB has several mechanisms to cope with cold temperatures such as antifreeze agents, cuticular waxes, high concentrations of glycerol, resistance to external ice, and they do not dehydrate from the cold (Crosthwaite et al., 2011; Duell et al., 2022). EAB is a freeze avoidant insect meaning that it is killed by internal ice formation by temperatures around −30°C (Crosthwaite et al., 2011). A recent study found that complete (99%) mortality of EAB prepupae was predicted for temperatures at or colder than −35.4°C while 75% mortality at −30.6°C (Cuddington et al., 2018). Additionally, the lowest underbark temperatures recorded for the cities of Grand Rapids and St. Paul, Minnesota were −34° and −26.3°C, respectively and the overwintering mortality for EAB larvae was 50% for Grand Rapids and 20% for St. Paul (Tussey et al., 2018). EAB is also found in Sault Ste. Marie, Ontario and Thunder Bay, Ontario (Canadian Food Inspection Agency, 2021) where winter minimums have reached below −30°C (Government of Canada, 2019, 2021). Duell et al. (2022) conclude that increased cold tolerance is sufficient to allow EAB to survive extreme winter events in locations that previous research suggested they could not. However, areas with regular exposure to temperatures below −35°C may allow for the local persistence of native ash stands (Christianson and Venette, 2018). A key question for future research is whether EAB overwintering mortality events are sufficient to reduce the death rates of ash trees. There are other variables that may affect how EAB accumulates GDD in cold climates including snow cover, sun exposure, and underbark microclimate. Snow cover may provide insulation acting as a buffer for larvae (DeSantis et al., 2013); larvae found on the south side of trees will be exposed to more sunlight/heat accruing more GDD (Vermunt et al., 2012); and the underbark microclimate experiences different temperatures than direct air temperature (Vermunt et al., 2012). These variables were not accounted for in this study and they may impact how EAB accumulates GDD in Winnipeg.
Conclusion
This study suggested that climate change in the Winnipeg region has not significantly impacted the date of first emergence for EAB; however, it has significantly impacted the date of peak activity (GDD 550 standard model). When comparing across the five decades, the earliest emergence and peak activity dates for each model-threshold combination occurred in the 2010 decade. We did not directly analyze climatological data in this study to calculate the rate of change in temperatures and thus cannot compare to other published estimates. We were also unsure if the development rate thresholds (LTT = 10°C, UTT = 37.8°C) were valid for our region as these have not yet been verified for Winnipeg. Therefore, we recommend that the development thresholds be determined for our region as well as further investigations of snow cover, sun exposure, and the underbark microclimate on EAB development in Winnipeg.
The results of this study can better inform EAB management programs and decision-makers in Winnipeg and other Canadian cities with a similar climate to Winnipeg. We recommend that EAB managers in Winnipeg (and areas with a similar climate) consider the results of all three of our models representing the range of days from (June 11–June 15 for emergence; July 11–July 13 for peak activity) when choosing a time to implement EAB control measures based on the temperature data from the 2010 decade. Future spatial analysis research is underway to determine the susceptibility to infestation of different neighborhoods in Winnipeg, and how EAB might advance throughout the city, both of which would prove useful in future management decisions.
Data Availability Statement
Publicly available datasets were analyzed in this study. This data can be found here: “https://climate.weather.gc.ca/historical_data/search_histori_data_stations_e.html?searchType=stnName&timeframe=1&txtStationName=winnipeg+the+forks&searchMethod=contains&optLimit=yearRange&StartYear=1840&EndYear=2016&Year=2016&Month=5&Day=25&selRowPerPage=25”; “https://climate.weather.gc.ca/climate_data/daily_data_e.html?hlyRange=1953-01-01%7C2013-01-17&dlyRange=1938-01-01%7C2008-07-24&mlyRange=1938-01-01%7C2007-11-01&StationID=3698&Prov=MB&urlExtension=_e.html&searchType=stnName&optLimit=yearRange&StartYear=1970&EndYear=1999&selRowPerPage=25&Line=1&searchMethod=contains&Month=1&Day=30&txtStationName=Richardson&timeframe=2&Year=1970”; “https://climate.weather.gc.ca/climate_data/daily_data_e.html?hlyRange=1999-11-01%7C2022-05-30&dlyRange=1999-11-01%7C2022-05-30&mlyRange=1999-11-01%7C2007-11-01&StationID=28051&Prov=MB&urlExtension=_e.html&searchType=stnName&optLimit=yearRange&StartYear=1840&EndYear=2022&selRowPerPage=25&Line=0&searchMethod=contains&Month=1&Day=30&txtStationName=The+Forks&timeframe=2&Year=2000”.
Author Contributions
As part of the master thesis of BM. RB, AW, and DW conceived the project and provided advice throughout. BM designed the study, performed statistical analyses, and wrote the manuscript. All authors contributed to the article and approved the submitted version.
Funding
BM received the University of Manitoba's Faculty of Graduate Studies Research Completion Scholarship.
Conflict of Interest
The authors declare that the research was conducted in the absence of any commercial or financial relationships that could be construed as a potential conflict of interest.
Publisher's Note
All claims expressed in this article are solely those of the authors and do not necessarily represent those of their affiliated organizations, or those of the publisher, the editors and the reviewers. Any product that may be evaluated in this article, or claim that may be made by its manufacturer, is not guaranteed or endorsed by the publisher.
Acknowledgments
We extend our appreciation and acknowledgments to Kerienne LaFrance and the City of Winnipeg's Urban Forestry Branch for providing valuable feedback and information to this study. We want to acknowledge Katherine Dearborn, a postdoctoral fellow at the University of Winnipeg, for the creation of the figures.
Supplementary Material
The Supplementary Material for this article can be found online at: https://www.frontiersin.org/articles/10.3389/fevo.2022.846144/full#supplementary-material
References
Aukema, J. E., Leung, B., Kovacs, K., Chivers, C., Britton, K. O., Englin, J., et al. (2011). Economic impacts of Non-Native forest insects in the continental United States. PLoS ONE 6:e24587. doi: 10.1371/journal.pone.0024587
Baek, S., Cho, K., Song, Y. H., and Lee, J. H. (2008). Degree-day based models for forecasting the flight activity of adult Helicoverpa assulta (Lepidoptera: Noctuidae) in hot pepper fields. Int. J. Pest Manag. 54, 295–300. doi: 10.1080/09670870802203865
Baranchikov, Y., Mozolevskaya, E., Yurchenko, G., and Kenis, M. (2008). Occurrence of the emerald ash borer, Agrilus planipennis in Russia and its potential impact on European forestry. EPPO Bull. 38, 233–238. doi: 10.1111/j.1365-2338.2008.01210.x
Bentz, B., Vandygriff, J., Jensen, C., Coleman, T., Maloney, P., Smith, S., et al. (2013). Mountain pine beetle voltinism and life history characteristics across latitudinal and elevational gradients in the Western United States. For. Sci. 60, 434–449. doi: 10.5849/forsci.13-056
Bentz, B. J., Bracewell, R. R., Mock, K. E., and Pfrender, M. E. (2011). Genetic architecture and phenotypic plasticity of thermally-regulated traits in an eruptive species, Dendroctonus ponderosae. Evol. Ecol. 25, 1269–1288. doi: 10.1007/s10682-011-9474-x
Bracewell, R. R., Pfrender, M. E., Mock, K. E., and Bentz, B. J. (2013). Contrasting geographic patterns of genetic differentiation in body size and development time with reproductive isolation in Dendroctonus ponderosae (Coleoptera: Curculionidae, Scolytinae). Ann. Entomol. Soc. Am. 106, 385–391. doi: 10.1603/AN12133
Brakie, M. (2013). Plant Guide—Green Ash (Fraxinus Pennslyvanica Marsh). USDA – Natural Resources Conservation Service. Available online at: https://www.nrcs.usda.gov/Internet/FSE_PLANTMATERIALS/publications/etpmcpg11869.pdf
Brown-Rytlewski, D., and Wilson, M. (2004). “Tracking the emergence of emerald ash borer adults,” in Emerald Ash Borer Research and Technology Development Meeting, eds V. Mastro and R. Reardon (USDA – Forest Health Technology Enterprise Team), 13–14. Available online at:https://www.invasive.org/eab/eab2004.pdf
Buermann, W., Bikash, P. R., Jung, M., Burn, D. H., and Reichstein, M. (2013). Earlier springs decrease peak summer productivity in North American boreal forests. Environ. Res. Lett. 8:024027. doi: 10.1088/1748-9326/8/2/024027
Canadian Food Inspection Agency (2021). Areas regulated for the emerald ash borer. Available online at: https://inspection.canada.ca/plant-health/invasive-species/insects/emerald-ash-borer/areas-regulated/eng/1347625322705/1367860339942
Cappaert, D., Mccullough, D. G., Poland, T. M., and Siegert, N. W. (2005). Emerald ash borer in North America: a research and regulatory challenge. Am. Entomol. 51, 152–165. doi: 10.1093/ae/51.3.152
Christianson, L., and Venette, R. (2018). Modest effects of host on the cold hardiness of emerald ash borer. Forests 9:346. doi: 10.3390/f9060346
City of Winnipeg (2017). City of WinnipegOpen Data Services. Available online at: https://data.winnipeg.ca/Parks/Tree-Inventory/hfwk-jp4h
City of Winnipeg (2020). Emerald Ash Borer (EAB). Parks and Open Space. Available online at: https://winnipeg.ca/publicworks/parksopenspace/urbanforestry/EmeraldAsh.stm#18
Climate Atlas of Canada (2019a). Municipality: Winnipeg. Available online at: https://climateatlas.ca/data/city/465/plus30_2030_85/line
Climate Atlas of Canada (2019b). Municipality: London. Available online at: https://climateatlas.ca/data/city/452/plus30_2030_85/line
Crosthwaite, J. C., Sobek, S., Lyons, D. B., Bernards, M. A., and Sinclair, B. J. (2011). The overwintering physiology of the emerald ash borer, Agrilus planipennis Fairmaire (coleoptera: buprestidae). J. Insect Physiol. 51, 166–173. doi: 10.1016/j.jinsphys.2010.11.003
Cuddington, K., Sobek-Swant, S., Crosthwaite, J. C., Lyons, D. B., and Sinclair, B. J. (2018). Probability of emerald ash borer impact for Canadian cities and North America: a mechanistic model. Biol. Invasions 20, 2661–2677. doi: 10.1007/s10530-018-1725-0
Dearborn, K., and Westwood, R. (2014). Predicting adult emergence of Dakota skipper and Poweshiek skipperling (Lepidoptera: Hesperiidae) in Canada. J. Insect Conserv. 18, 875–884. doi: 10.1007/s10841-014-9695-8
DeSantis, R., Moser, K., Gormanson, D., Bartlett, M., and Vermunt, B. (2013). Effects of climate on emerald ash borer mortality and the potential for ash survival in North America. Agric. For. Meteorol. 178–179, 120–128. doi: 10.1016/j.agrformet.2013.04.015
Duell, M. E., Gray, M. T., Roe, A. D., MacQuarrie, C. J. K., and Sinclair, B. J. (2022). Plasticity drives extreme cold tolerance of emerald ash borer (Agrilus planipennis) during a polar vortex. Curr. Res. Insect Sci. 2:100031. doi: 10.1016/j.cris.2022.100031
Dytham, C. (2011). Choosing and Using Statistics: A Biologist's Guide, 3rd Edn. Chichester; West Sussex: John Wiley and Sons Inc.
Flower, C. E., Knight, K. S., and Gonzalez-Meler, M. A. (2013). Impacts of the emerald ash borer (Agrilus planipennis Fairmaire) induced ash (Fraxinus spp.) mortality on forest carbon cycling and successional dynamics in the eastern United States. Biol. Invasions 15, 931–944. doi: 10.1007/s10530-012-0341-7
Gandhi, K. J. K., and Herms, D. A. (2010). North American arthropods at risk due to widespread Fraxinus mortality caused by the alien Emerald ash borer. Biol. Invasions 12, 1839–1846. doi: 10.1007/s10530-009-9594-1
GLISA (2017). Historical Climatology: Detroit, Michigan. Great Lakes Integrated Sciences and Assessments Team, Michigan. Available online at: https://detroitenvironmentaljustice.org/wp-content/uploads/2017/10/2016-Detroit_MI_Climatology-3.pdf
Gould, J. S., Bauer, L. S., Duan, J. J., and Petrice, T. R. (2016). Emerald ash Borer Biological Control Release and Recovery Guidelines. USDA–APHIS–ARS-FS, Riverdale, Maryland. Available online at: http://treesearch.fs.fed.us/pubs/40545
Government of Canada (2017). Emerald ash Borer Confirmed in Winnipeg. Canadian Food Inspection Agency. Available online at: https://www.canada.ca/en/food-inspection-agency/news/2017/12/emerald_ash_borerconfirmedinwinnipeg.html
Government of Canada (2019). Daily Temperature Data for Sault Ste Marie, Ontario. Available online at: https://climate.weather.gc.ca/climate_data/daily_data_e.html?hlyRange=2012-03-20%7C2021-12-29anddlyRange=2012-03-20%7C2021-12-29andmlyRange=%7CandStationID=50092andProv=ONandurlExtension=_e.htmlandsearchType=stnNameandoptLimit=yearRangeandStartYear=1840andEndYear=2021andselRowPerPage=25andLine=2andsearchMethod=containsandtxtStationName=sault+ste+marieandtimeframe=2andtime=LSTandDay=29andYear=2019andMonth=1#
Government of Canada (2021). Daily Temperature Data for Thunder Bay, Ontario. Available online at: https://climate.weather.gc.ca/climate_data/daily_data_e.html?hlyRange=2012-04-12%7C2021-12-29anddlyRange=2018-10-30%7C2021-12-29andmlyRange=%7CandStationID=50132andProv=ONandurlExtension=_e.htmlandsearchType=stnNameandoptLimit=yearRangeandStartYear=1840andEndYear=2021andselRowPerPage=25andLine=1andsearchMethod=containsandtxtStationName=thunder+bayandtimeframe=2andtime=LSTandDay=29andYear=2021andMonth=2#
Granger, J. J., Zobel, J. M., and Buckley, D. S. (2020). Differential impacts of emerald ash borer (Agrilus planipennis Fairmare) on forest communities containing native ash (Fraxinus spp.) species in Eastern North America. For. Sci. 66, 38–48. doi: 10.1093/forsci/fxz063
Haack, R., Jendek, E., Liu, H., Marchant, K., Petrice, T. R., Poland, T. M., et al. (2002). The emerald ash borer: a new exotic pest in North America. J. Chem. Inf. Model. 47, 1–5. Available onine at: https://www.fs.usda.gov/treesearch/pubs/11858
Herms, D., (2004). “Using degree-days and plant phenology to predict pest activity,” in IPM (Integrated Pest Management) of Midwest Landscapes, eds V. Krischik, and J. Davidson (Regents of the University of Minnesota. Minnesota Agricultural Experiment Station Publication SB-07645), 49–59.
Herms, D., and McCullough, D. (2014). Emerald ash borer invasion of North America: history, biology, ecology, impacts, and management. Annu. Rev. Entomol. 59, 13–30. doi: 10.1146/annurev-ento-011613-162051
Herms, D., Mccullough, D., Smitley, D., Clifford, C., and Cranshaw, W. (2019). Insecticide Options for Protecting Ash Trees from Emerald Ash Borer Insecticide, 3rd Edn. North Central Center Bulletin, 1–16. Available online at: http://www.emeraldashborer.info/documents/Multistate_EAB_Insecticide_Fact_Sheet.pdf
IUCN (2021). The IUCN Red List of Threatened Species. IUCN Red List of Threatened Species. Available online at: https://www.iucnredlist.org/en
Johansen, N. S. (1997). Influence of temperature on development, fecundity and survival of the cabbage moth Mamestra brassicae (L.) (Lep., Noctuidae) in relation to the improvement of forecasting and control methods. J. Appl. Entomol. 121, 81–88. doi: 10.1111/j.1439-0418.1997.tb01375.x
Kappel, A. P., Trotter, R. T., Keena, M. A., Rogan, J., and Williams, C. A. (2017). Mapping of the Asian longhorned beetle's time to maturity and risk to invasion at contiguous United States extent. Biol. Invasions 19, 1999–2013. doi: 10.1007/s10530-017-1398-0
Keena, M. A., and Moore, P. M. (2010). Effects of temperature on Anoplophora glabripennis (Coleoptera: Cerambycidae) Larvae and Pupae. Environ. Entomol. 39, 1323–1335. doi: 10.1603/EN09369
Klooster, W. S., Gandhi, K. J. K., Long, L. C., Perry, K. I., Rice, K. B., and Herms, D. A. (2018). Ecological impacts of emerald ash borer in forests at the epicenter of the invasion in North America. Forests 9:250. doi: 10.3390/f9050250
Klooster, W. S., Herms, D. A., Knight, K. S., Herms, C. P., McCullough, D. G., Smith, A., et al. (2014). Ash (Fraxinus spp.) mortality, regeneration, and seed bank dynamics in mixed hardwood forests following invasion by emerald ash borer (Agrilus planipennis). Biol. Invasions 16, 859–873. doi: 10.1007/s10530-013-0543-7
Knight, K. S., Brown, J. P., and Long, R. P. (2013). Factors affecting the survival of ash (Fraxinus spp.) trees infested by emerald ash borer (Agrilus planipennis). Biol. Invasions 15, 371–383. doi: 10.1007/s10530-012-0292-z
Liang, L., and Fei, S. (2014). Divergence of the potential invasion range of emerald ash borer and its host distribution in North America under climate change. Clim. Change 122, 735–746. doi: 10.1007/s10584-013-1024-9
Liu, H. (2018). Under Siege: ash management in the wake of the emerald ash borer. J. Integr. Pest Manag. 9:5. doi: 10.1093/jipm/pmx029
Liu, H., Bauer, L. S., Gao, R., Zhao, T., Petrice, T. R., and Haack, R. A. (2003). Exploratory Survey for the Emerald Ash Borer, Agrilus planipennis (Coleoptera: Buprestidae), and Its Natural Enemies in China. Great Lakes Entomologist 36. Available online at: https://scholar.valpo.edu/tgle/vol36/iss2/11/
Liu, H., Bauer, L. S., Miller, D. L., Zhao, T., Gao, R., Song, L., et al. (2007). Seasonal abundance of Agrilus planipennis (Coleoptera: Buprestidae) and its natural enemies Oobius agrili (Hymenoptera: Encyrtidae) and Tetrastichus planipennisi (Hymenoptera: Eulophidae) in China. Biol. Control 42, 61–71. doi: 10.1016/j.biocontrol.2007.03.011
McCullough, D. G. (2020). Challenges, tactics and integrated management of emerald ash borer in North America. Forestry 93, 197–211. doi: 10.1093/forestry/cpz049
McCullough, D. G., Poland, T., and Cappaert, D. (2009b). Attraction of the emerald ash borer to ash trees stressed by girdling, herbicide treatment, or wounding. Can. J. For. Res. 39, 1331–1345. doi: 10.1139/X09-057
McCullough, D. G., Poland, T. M., Anulewicz, A. C., and Cappaert, D. (2009a). Emerald ash borer (Coleoptera: Buprestidae) attraction to stressed or baited ash trees. Environ. Entomol. 38, 1668–1679. doi: 10.1603/022.038.0620
Minnesota Department of Health (2021). Climate and Health in Minnesota. Available online at: https://www.health.state.mn.us/communities/environment/climate/climate101.html
Minnesota Pollution Control Agency (2021). Effects of climate change in Minnesota. Available online at: https://www.pca.state.mn.us/air/effects-climate-change-minnesota
Mironidis, G. K., Stamopoulos, D. C., and Savopoulou-Soultani, M. (2010). Overwintering Survival and Spring Emergence of Helicoverpa armigera (Lepidoptera: Noctuidae) in Northern Greece. Environ. Entomol. 39, 1068–1084. doi: 10.1603/EN09148
Mitton, J. B., and Ferrenberg, S. M. (2012). Mountain pine beetle develops an unprecedented summer generation in response to climate warming. Am. Nat. 179, 163–171. doi: 10.1086/665007
Murray, M. (2008). Using Degree Days to Time Treatments for Insect Pests. Utah State University Extension and Utah Plant Pest Diagnostic Laboratory. Available online at: https://climate.usu.edu/includes/pestFactSheets/degree-days08.pdf
O'Neal, M. J., Headrick, D. H., Montez, G. H., and Grafton-Cardwell, E. E. (2011). Temperature thresholds and degree-day model for Marmara gulosa (Lepidoptera: Gracillariidae). J. Econ. Entomol. 104, 1286–1293. doi: 10.1603/EC10438
Orlova-Bienkowskaja, M. J., and Bieńkowski, A. O. (2016). The life cycle of the emerald ash borer Agrilus planipennis in European Russia and comparisons with its life cycles in Asia and North America. Agric. For. Entomol. 18, 182–188. doi: 10.1111/afe.12140
Pest Prophet (2021). The Complete Guide to Emerald Ash Borer. Available online at: https://blog.pestprophet.com/emerald-ash-borers/
Poland, T. M., and McCullough, D. G. (2006). Emerald ash borer: Invasion of the urban forest and the threat to North America's ash resource. J. Forestry 104, 118–124. doi: 10.1093/jof/104.3.118
Poland, T. M., McCullough, D. G., and Anulewicz, A. C. (2011). Evaluation of double-decker traps for emerald ash borer (Coleoptera: Buprestidae). J. Econ. Entomol. 104, 517–531. doi: 10.1603/EC10254
Prairie Climate Centre (2018). Custom Climate Change Reports for Your Community: Municipality: Winnipeg. University of Winnipeg. Available online at: https://prairieclimatecentre.ca/2018/05/custom-climate-change-reports-for-your-community/
Régnière, J., Powell, J., Bentz, B., and Nealis, V. (2012). Effects of temperature on development, survival and reproduction of insects: Experimental design, data analysis and modeling. J. Insect Physiol. 58, 634–647. doi: 10.1016/j.jinsphys.2012.01.010
Ryall, K., Fidgen, J., and Turgeon, J. (2011). Detection of Emerald Ash Borer in Urban Environments Using Branch Sampling. Natural Resources Canada – Canadian Forest Service, Sault Ste. Marie. Available online at: https://epe.lac-bac.gc.ca/100/200/301/nrcan-rncan/frontline_forestry_research_applications-e/Fo123-1-111-eng.pdf
Schrader, G., Baker, R., Baranchikov, Y., Dumouchel, L., Knight, K. S., McCullough, D. G., et al. (2021). How does the emerald ash borer (Agrilus planipennis) affect ecosystem services and biodiversity components in invaded areas? EPPO Bull. 51, 216–228. doi: 10.1111/epp.12734
Siegert, N. W., Mccullough, D. G., Liebhold, A. M., and Telewski, F. W. (2014). Dendrochronological reconstruction of the epicentre and early spread of emerald ash borer in North America. Divers. Distributions 20, 847–858. doi: 10.1111/ddi.12212
Son, Y., Nadel, H., Baek, S., Johnson, M. W., and Morgan, D. J. W. (2012). Estimation of developmental parameters for adult emergence of Gonatocerus morgani, a novel egg parasitoid of the glassy-winged sharpshooter, and development of a degree-day model. Biol. Control 60, 233–240. doi: 10.1016/j.biocontrol.2011.04.008
Steiner, K. C., Graboski, L. E., Knight, K. S., Koch, J. L., and Mason, M. E. (2019). Genetic, spatial, and temporal aspects of decline and mortality in a Fraxinus provenance test following invasion by the emerald ash borer. Biol. Invasions 21, 3439–3450. doi: 10.1007/s10530-019-02059-w
Thöming, G., and Saucke, H. (2011). Key factors affecting the spring emergence of pea moth (Cydia nigricana). Bull. Entomol. Res. 101, 127–133. doi: 10.1017/S0007485309990642
Tluczek, A. R., Mccullough, D. G., and Poland, T. M. (2011). Influence of host stress on emerald ash borer (Coleoptera: Buprestidae) adult density, development, and distribution in Fraxinus pennsylvanica trees. Environ. Entomol. 40, 357–366. doi: 10.1603/EN10219
Tussey, D. A., Aukema, B. H., Charvoz, A. M., and Venette, R. C. (2018). Effects of adult feeding and overwintering conditions on energy reserves and flight performance of emerald ash borer (Coleoptera: Buprestidae). Environ. Entomol. 47, 755–763. doi: 10.1093/ee/nvy040
Twedt, D. J., and Best, C. (2004). Restoration of floodplain forests for the conservation of migratory landbirds. Ecol. Restor. 22, 194–203. doi: 10.3368/er.22.3.194
University of California. (2016). About Degree-Days. University of California Agriculture and Natural Resources – Statewide Integrated Pest Management Program. Available online at: http://ipm.ucanr.edu/WEATHER/ddconcepts.html
USDA (2021). Emerald Ash Borer. USDA – Animal and Plant Health Inspection Service. Available online at: https://www.aphis.usda.gov/aphis/ourfocus/planthealth/plant-pest-and-disease-programs/pests-and-diseases/emerald-ash-borer
Valenta, V., Moser, D., Kapeller, S., and Essl, F. (2017). A new forest pest in Europe: a review of emerald ash borer (Agrilus planipennis) invasion. J. Appl. Entomol. 141, 507–526. doi: 10.1111/jen.12369
Vermunt, B., Cuddington, K., Sobek-Swant, S., Crosthwaite, J. C., Barry Lyons, D., and Sinclair, B. J. (2012). Temperatures experienced by wood-boring beetles in the under-bark microclimate. For. Ecol. Manage. 269, 149–157. doi: 10.1016/j.foreco.2011.12.019
Villari, C., Herms, D. A., Whitehill, J. G. A., Cipollini, D., and Bonello, P. (2016). Progress and gaps in understanding mechanisms of ash tree resistance to emerald ash borer, a model for wood-boring insects that kill angiosperms. N. Phytol. 209, 63–79. doi: 10.1111/nph.13604
Wagner, D., and Todd, K. (2016). New ecological assessment for the emerald ash borer: a cautionary tale about unvetted host-plant literature. Am. Entomol. 62, 26–35. doi: 10.1093/ae/tmw005
Wang, X., Yang, Z.-Q., Gould, J. R., Zhang, Y.-N., Liu, G.-J., and Liu, E. (2010). The biology and ecology of the emerald ash borer, Agrilus planipennis, in China. J. Insect Sci. 10, 128. doi: 10.1673/031.010.12801
Wei, X., Wu, Y., Reardon, R., Sun, T.-H., Lu, M., and Sun, J.-H. (2007). Biology and damage traits of emerald ash borer (Agrilus planipennis Fairmaire) in China. Insect Sci. 14, 367–373. doi: 10.1111/j.1744-7917.2007.00163.x
Keywords: emerald ash borer, degree-days, management, Urban ecology, emergence, peak activity, conservation, invasive
Citation: MacDonald B, Baydack R, Westwood AR and Walker D (2022) Predicting Emerald Ash Borer Adult Emergence and Peak Flight Activity in Winnipeg, Manitoba, Canada. Front. Ecol. Evol. 10:846144. doi: 10.3389/fevo.2022.846144
Received: 30 December 2021; Accepted: 26 May 2022;
Published: 17 June 2022.
Edited by:
Steven Petersen, Brigham Young University, United StatesReviewed by:
Fredrik Schlyter, Czech University of Life Sciences Prague, CzechiaMichael Domingue, United States Department of Agriculture (USDA), United States
Copyright © 2022 MacDonald, Baydack, Westwood and Walker. This is an open-access article distributed under the terms of the Creative Commons Attribution License (CC BY). The use, distribution or reproduction in other forums is permitted, provided the original author(s) and the copyright owner(s) are credited and that the original publication in this journal is cited, in accordance with accepted academic practice. No use, distribution or reproduction is permitted which does not comply with these terms.
*Correspondence: Brett MacDonald, bWFjZG9uODRAbXl1bWFuaXRvYmEuY2E=; YnJldHRtYWMxMTE5QGdtYWlsLmNvbQ==