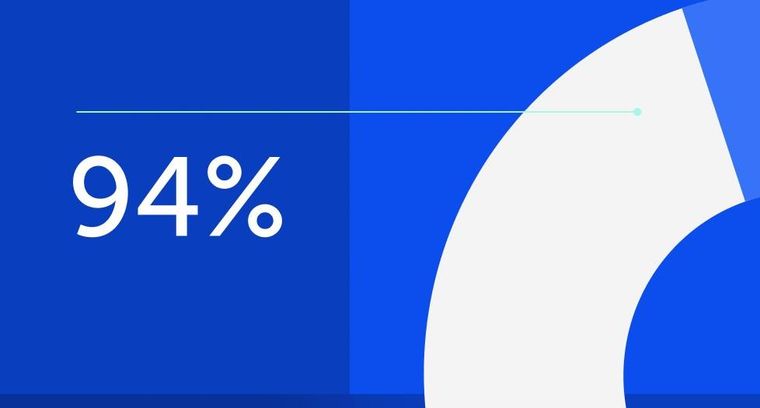
94% of researchers rate our articles as excellent or good
Learn more about the work of our research integrity team to safeguard the quality of each article we publish.
Find out more
PERSPECTIVE article
Front. Ecol. Evol., 29 March 2022
Sec. Population, Community, and Ecosystem Dynamics
Volume 10 - 2022 | https://doi.org/10.3389/fevo.2022.845560
This article is part of the Research TopicNew Perspectives and Emerging Directions in Predator–Prey Functional Response Research: Hommage to C.S. Holling (1930–2019)View all 18 articles
For simplicity and to minimize variation, functional response experiments frequently use environments of simple physical structure and small size. Less attention is paid to similarity of the experimental environment to the natural environment where predation occurs. Assumptions about predator and prey use of space are often implied in the choice of experimental environment. We illustrate how these assumptions may affect conclusions with an experiment testing how arena size affects a functional response. Toxorhynchites rutilus preyed upon larval Culex restuans in containers differing in volume by 15x, but spanning a similar range of prey/liter. The most plausible Type II model included attack rates that were statistically indistinguishable, but in the larger volume, had handling time that was lower by > 30x compared to the smaller volume, suggesting a major change in predator behavior with container volume. When we altered our assumption that predation scales with prey/liter, assuming instead that aggregation causes predation to scale with prey/area of surface or bottom, the conclusions changed: neither attack rate nor handling time differed with container size. Thus, our assumption about how predator and prey used space altered the conclusions of the experiment. We then summarize recently published experiments showing that spatial context affects estimated functional responses. We suggest that functional response experiments would be improved by using larger experimental spaces that represent physical complexity of environments where predation occurs. Greater spatial extent and complexity are likely to cause aggregation of predation. Effects of more realistic spatial context are likely to yield more complete understanding of quantitative aspects of predation.
Since Holling’s thorough development of the quantitative treatment of the functional response of a predator to the density of its prey (Holling, 1959, 1965, 1966), functional responses have remained one of the most prevalent concepts in biological study of consumer-resource interactions. The quantitative relationship of consumption vs. resource density figures prominently in many investigations in biology, ecology, and behavior. Functional response models are commonly used in investigations of animal behavior, in attempts to understand the behavioral processes limiting feeding by predators and mortality of prey (e.g., Juliano, 1989; Jeschke et al., 2002). Functional responses also form the basis of theory of foraging behavior predicting choices made by consumers while foraging (e.g., Stephens and Krebs, 1986; Cressman et al., 2014) and in resource-based models of competition and predation (e.g., Grover, 1997; Chase and Leibold, 2003). Empirically determined functional responses have been used in developing predictive models of predator-prey interactions for biological control (e.g., Madadi et al., 2011), predictions of potential for introduced species to become problematic invasive species (e.g., Dick et al., 2017; McCard et al., 2021), quantification of how multiple predators impact prey populations (e.g., McCoy et al., 2012; Hossie and Murray, 2016; Sentis and Boukal, 2018), and in evaluations of stability of species interactions and their effects on community diversity (e.g., Buxton et al., 2020; Kratina et al., 2021). A central idea in all the uses of functional responses is that individual behavioral interactions of consumers and their resources can be scaled up across different levels of biological/ecological organization, from behavioral choices and interactions of individuals, to population dynamics of consumers and victims, to community level interactions as they effect coexistence and diversity. Scaling up requires that functional responses are quantified in ways that are meaningful and predictive of processes occurring at higher levels of organization. This creates a need to measure functional responses in spatial contexts that are appropriate for the organisms and representative of the environments in which individual interactions of consumer and resource occur, and in which those individual processes influence population dynamics and community organization.
For simplicity and to minimize experimental variation, experimental environments used in functional response experiments are frequently simple in physical structure, small in size, and otherwise designed for ease of data collection. Less attention is paid to the similarity of the experimental environment to the natural environment about which predictions will be made (Griffen, 2021). These simplifications of the environment can have consequences for the results of the experiment, and are often based on assumptions about how both predator and prey perceive and use their environment.
The purpose of this perspective is: (1) To describe a case study of how assumptions about animal behavior in different spatial contexts can affect conclusions of functional response experiments; we do this via a simple example manipulating spatial context, and alternative analyses of the data under different assumptions about how predator and prey aggregate in the space in which the experiment was run. (2) To summarize recent examples of how different spatial contexts, and what is assumed about how predator and prey use experimental space, can change the conclusions and predictions of functional response experiments, often because of aggregation.
The functional response experiment used the predatory Toxorhynchites rutilus larvae and Culex restuans larvae as prey. Both mosquitoes are native to North America, and are commonly found in man-made (e.g., tires) and natural (e.g., tree holes) water filled containers. Toxorhynchites spp. are obligate predators on other invertebrates in water filled containers (Steffan and Evenhuis, 1981). Culex restuans is a member of the Culex pipiens complex, and is primarily a filter feeder (Merritt et al., 1992). Culex restuans were collected in Normal IL as egg rafts and placed individually in small vials. After egg hatch, larvae from each raft were identified as either C. restuans or C. pipiens (Darsie and Ward, 2005) and C. restuans were placed into a tray holding ∼300-400 larvae. Toxorhynchites rutilus were from a laboratory colony originating at Tyson Research Center, Eureka MO.
The functional response experiment tested the hypothesis that the size of the experimental arena (water-filled container) affects the functional response. We used first instar T. rutilus as predators and first instar C. restuans as prey. Water-filled containers were plastic Tripour® beakers of two sizes: 50 ml beakers holding 28 ml of water and 1, 2, 5, 7, and 12 prey, replicated 3, 2, 3, 4, and 4 times, respectively, or 1000 ml tripour beakers holding 420 ml of water and 11, 37, 75, 112, and 187 prey, replicated 5, 3, 3, 4, and 4 times, respectively, each with one T. rutilus. The combinations of these volumes and prey numbers were designed to produce a similar range of densities of prey/L in both arena sizes. Both prey and predators were 1-2 d post hatch. Predators had not been fed mosquito prey before the experiment, and each predator was used only once. The experiment ran at 25°C for 6 h after which the predator was removed, and surviving prey larvae counted. Number eaten was quantified as the difference between the initial number and number surviving.
We chose to design this experiment using prey/L as the quantification of density as this has been the implicit assumption of functional response experiments with Toxorhynchites (e.g., Livdahl, 1979; Russo, 1983; Juliano, 2001; Griswold and Lounibos, 2005). An alternative assumption is that prey/dm2 surface or bottom is the best way to quantify density. This quantification arises because both predator and prey are air-breathing insect larvae that must come to the water’s surface (Clements, 1992). Culex species often filter feed while hanging at the surface (Yee et al., 2004; Skiff and Yee, 2014). For T. rutilus, some investigators have found that prey capture occurs primarily at the bottom (Russo, 1986; Juliano and Reminger, 1992), and others have suggested that captures occur primarily at the surface (Linley, 1995; Focks, 2007). Either case would result predation being spatially aggregated in approximately two dimensions, although behavioral studies show that captures do occur in all parts of the water (Juliano and Reminger, 1992). Thus, we also analyzed the functional responses expressing prey density as number/area (dm2) based on the area of the surface of the water in the experimental arena, which also approximates the area of the arena bottom.
Although past tests for Toxorhynchites functional response have found Type II functional responses are most common (Juliano, 2001; Griswold and Lounibos, 2005), we used logistic regressions of proportion of prey eaten (Juliano, 2001) to test whether a Type II or Type III functional response was more appropriate. That analysis (Supplementary Material) confirmed that Type II functional responses were best for all analyses. Data were analyzed by non-linear regressions of number of prey eaten vs. prey density expressed as either number/L or number/dm2 of surface area, using the implicit function method (Juliano, 2001) to fit a Type II functional response accounting for prey depletion (Rogers, 1972):
Where Ne = number of prey eaten, N0 = initial density of prey, a = attack rate, Th = handling time per prey eaten, and T = duration of the experiment (= 6 h). Parameters estimated and compared between the two sized experimental arenas are a and Th. An indicator variable (values of 0 or 1) was used to distinguish the two arena sizes (Juliano, 2001). Models tested for differences in both a and Th, only a, only Th, or neither a nor Th, and were evaluated by AICc (Motulsky and Christopoulos, 2004; Anderson, 2008), which is a useful way to evaluate models of similar geometric complexity (Novak and Stouffer, 2021) such as those compared here. Model fits also yielded hypothesis tests for differences of parameters between the two container sizes. All analyses were conducted using PROC NLIN SAS Statistical Software version 9.13.
AICc for this analysis (Figure 1A) led to the conclusion that the most plausible model has the same attack rate (a) in both experimental arena sizes, but different handling times (Th) in each arena size. For this model, handling times differed significantly (Figure 1A), with the handling time in small experimental area considerably greater than that in the large experimental arena. This is evident in the plot of the predicted values of number eaten Ne vs. initial density/L N0 (Figure 1A).
Figure 1. Number of prey eaten per predator per 6 h experiment vs. prey density based on (A). water volume and (B). surface or bottom area. Points are observed values and lines are the predicted functions for the most plausible model in each analysis. For comparisons of the different models: k is the number of parameters, wi is the Akaike weight for each model, and E is the evidence ratio (Anderson, 2008). Estimated parameters (95% confidence interval): (A). Volume: Equal a, Th differ: a = 0.0066 (0.0040, 0.0092) L/h, Th(small) = 0.6302 (0.2852, 0.9753) h, Th(large) -Th(small) = −0.6088 (−0.9039, −0.3138) h. (B). Area: Equal a, equal Th: a = 0.0133 (0.0091, 0.0176) dm2/h, Th = 0.0558 (−0.0748, 0.1864) h.
AICc for this analysis (Figure 1B) led to a different conclusion: that the most plausible model has the same attack rate (a) and handling time (Th) in both arena sizes. In this analysis, handling times were also not significantly different (Th(large)-Th(small) = −0.098 h, 95% CI = [−0.484, 0.288] h). The plot of the predicted values of number eaten Ne vs. initial density/dm2 N0 from the most plausible model suggests little difference in the functional responses in the different experimental arena sizes (Figure 1B).
Thus, what is assumed about how the predator and prey use space affects the conclusion about the effects of container size on predation. Under the assumption of interactions over volume, one might conclude predator behavior changes with container size. Predator processing time of victims appears to decrease greatly in the large experimental arena, yielding a prediction that does not reach an asymptote (Figure 1A). Uiterwaal et al., 2019 suggested that arena size is most likely to affect apparent attack rates, rather than handling time, when prey and predator are non-randomly distributed in space (see also Table 1). Aggregation of predator and prey would have the effect rendering our prey densities expressed per liter inaccurate and suggested to us an alternative assumption of interactions over area may be more appropriate.
Table 1. Summary of recent functional response experiments investigating the effects of spatial context on functional response type and parameter estimates.
Under the assumption that predator-prey interactions are aggregated in two dimensions, our analysis suggests that there is no change in predator behavior. The conclusion of no difference in handling time might be criticized as a product of low statistical power, but power was adequate to detect a significant difference in handling time under the assumptions of the alternative analysis using volume. Resolving these issues would require additional experiments, e.g., experiments at different water volumes in the same containers, so that surface area remains the same and determining if the functional responses remain consistent as volume changes. A factorial experiment manipulating surface area and volume independently and quantifying functional responses in all combinations of surface and volume would also be useful. Direct observations of T. rutilus hunting and capturing mosquito prey would also be useful to determine if predators, prey, and captures are aggregated, either at the bottom, at the water’s surface, or in another location. Nevertheless, this simple experiment illustrates how the spatial context and assumptions about how predator and prey interact in space can have a major effect on the interpretation of predator functional response data.
We chose published empirical studies of functional responses of invertebrate predators, comparing different spatial contexts in two clear categories: effects of experimental arena size; and effects of environmental complexity, including arena shape, physical structure, and prey spatial heterogeneity. Effects of spatial context on functional response parameters are common in published work, changing one or both parameters of Type II functional responses, or changing the form of the functional response from Type II to Type III (summarized in Table 1). These effects occur in both terrestrial (largely 2 dimensional) systems and in aquatic (potentially 3 dimensional systems).
Uiterwaal et al. (2019) reported the most thorough analysis of the effect of arena size because they showed convincingly in several experiments that their spider predator and its prey do not uniformly occupy two-dimensional arenas of various sizes, but rather show positive thigmotaxis, aggregating near the walls. This can be interpreted as the animals using 1 dimensional space (i.e., linear, but circular wall of the arena), even though the arena is 2 dimensional. Similar aggregation in space at walls in experimental arenas of different sizes was demonstrated for mysid shrimp preying on cladocerans, and this aggregation affected the attack rate by concentrating encounters of predator and prey (Bergström and Englund, 2004). The difference in functional responses in the two analyses of our experiment is consistent with similar aggregation of predator and prey, perhaps at the surface or bottom. A different mechanism was postulated for attack rates of damselflies that increase with arena size (Uiterwaal et al., 2017): damselfly behavior and search were inhibited in small aquatic environments that do not represent the typical habitats occupied by these predators. A previous review of functional responses of 23 coccinellid beetle predators on multiple different types of prey (Uiterwaal and DeLong, 2018) also showed a general pattern of arena size having a consistently strong positive effect on attack rate, but not on handling time, though arena size often interacted with predator size or predator stage to affect both attack rate and handling time. Aggregation imposed by investigators also can change functional responses from hyperbolic with uniformly distributed prey to sigmoid with aggregated prey (Hossie and Murray, 2016). Greater arena size, and associated greater spatial heterogeneity, are likely to result in greater prey aggregation, and thus may generally shift functional responses from Type II to Type III (Griffen, 2021). Experiments have found effects of arena size without considering the behavioral mechanism involved (e.g., Yaşar and Özgar, 2005). Explicit consideration of arena size in experiments testing for effects of predator-predator interactions on the functional response is also likely to be important, as arena size is postulated to affect how predators interact (e.g., Cuthbert et al., 2020).
Environmental complexity in the form of physical structures within aquatic and terrestrial environments has multiple potential effects, some of which are likely related to aggregation of predation. There were complex effects on Type II functional responses of Aeshna to the physical structure of the experimental aquatic environment, with most plausible models postulating some combination of quadratic changes in handling time, first declining, then increasing with habitat complexity, and stepwise increases in attack rate from no to some structural complexity (Mocq et al., 2021). Behavioral data implicated behavioral change of the predator in the presence of habitat complexity, but the authors also postulated that aggregation of pelagic prey in open-water spaces as another mechanism that may contribute to altered functional responses (Mocq et al., 2021). Effects of habitat complexity on predation by notonectids (Wasserman et al., 2016; Buxton et al., 2020) show a trend of decreasing predation at highest habitat complexity, which they attributed to changes in predator behavior (Buxton et al., 2020). Buxton et al. (2020) also showed that habitat complexity could impact predator dependence in functional responses, enhancing multipredator effects that increase predation for some predators (Anisops) and having no impact on multipredator effects for others (Einithares). Fractal complexity of walls of two-dimensional arenas reduced attack rates of predatory thrips and was interpreted to be a result of escape from predation by thrips prey occupying vertices in complex walls which served as a refuge from predation (Hoddle, 2003). This implies that habitat complexity may stimulate aggregation of prey in refuges, aggregating successful predation away from those refuges. Similar hypotheses were stated to account for effects of structural complexity of host plants, which can change predator functional responses from Type II to Type III (Messina and Hanks, 1998) or produce differences in the parameters of the Type II functional response for some predators (Timms et al., 2008; Madadi et al., 2011). But greater structural complexity may alter the type of functional response by changing predator hunting efficiency or strategy, rather than by providing a prey refuge (Hossie and Murray, 2010). A similar interpretation for the effect of physical structure on functional response parameters regarded structures as obstacles that provide no refuge for prey but reduce predator movement rate (Hauzy et al., 2010), and thus reduce attack rate, and also reduce predator-predator interference. More complex effects arise for nematode predators hunting nematode prey in environments with vs. without physical structure (Kreuzinger-Janik et al., 2019), where structure affected Type III attack rate for small prey, but handling time for large prey, and mechanisms behind these changes were unclear. Adding habitat complexity in the form of leaves altered the functional response of centipedes to density of Collembola (Kalinkat et al., 2013). This change was associated with the increased two-dimensional surface area of the arena due to the dual surfaces of the leaves creating more two-dimensional space for both predator and prey, effectively reducing prey density per unit space (Kalinkat et al., 2013). As in our experiment, Kalinkat et al. (2013) conducted alternative data analyses incorporating different assumptions about how their predator and prey used the experimental environment to reach their conclusions.
What is clear from this brief review is that the spatial context used in experiments, including arena size and spatial complexity, often affects the functional response. Often the spatial context may alter the functional response through aggregation of predation away from prey refuges, in areas preferred by predator, or on edges of arenas. Functional response experiments are likely to be improved by incorporating complexity of the environment in which predator and prey interact and by considering behavioral processes that are affected by spatial context, and that result in aggregation of predation even in simple experimental arenas. Previous theoretical treatments of functional responses have included predator and prey aggregation in models (e.g., Fryxell et al., 2007; Fortin et al., 2015), often in the context of social organization of prey. But aggregation may arise even in organisms with little or no social structure when environments are spatially heterogeneous, and organisms have preferences for particular physical situations (e.g., edges vs. open spaces). Spatial extent and heterogeneity, and how predator and prey use heterogeneous space are therefore likely to be generally important for a wide array of predator-prey systems. In nature, spatial context may alter functional responses in still other ways, for example, by fostering coexistence of multiple prey species of different vulnerabilities, which can reduce predation rates and stabilize community food webs (e.g., Hammill et al., 2015).
Our perspective is that several practical recommendations for experiments on functional responses arise from this review: (1) Small arenas, relative to organism sizes, should be avoided, as smaller arenas necessarily have greater edge relative to area or volume, and many organisms seem to respond to arena edges. (2) Including experimental structural complexity in ways that represent the natural environment in which focal predators and prey interact is likely to yield more accurate predictions of predation in nature, as virtually all predator-prey interactions, with the possible exception of those of planktonic animals, occur in environments with physical structures. (3) Quantifying how animals use space in functional response arenas can help to identify spatial processes that affect functional responses, and contribute to better predictions.
The raw data supporting the conclusions of this article will be made available by the authors, without undue reservation.
SJ and GO designed the experiment and revised the manuscript. JG and GO conducted the experiment and collected experimental data. SJ analyzed the data and reviewed the literature on functional responses. JG wrote the first draft. All authors contributed to the final written version.
This research was supported by NIAID grants 1R15AI094322-01A1 and 1R15AI124005-01 to SJ. The experiment was part of a Student Inquiry and Research project for the Illinois Math and Science Academy.
The authors declare that the research was conducted in the absence of any commercial or financial relationships that could be construed as a potential conflict of interest.
All claims expressed in this article are solely those of the authors and do not necessarily represent those of their affiliated organizations, or those of the publisher, the editors and the reviewers. Any product that may be evaluated in this article, or claim that may be made by its manufacturer, is not guaranteed or endorsed by the publisher.
We thank Dennis Murray and Thomas John Hossie for the invitation to contribute to this Research Topic on functional response research, and Jaclyn Everly, Victoria Borowicz, and two referees for helpful comments.
The Supplementary Material for this article can be found online at: https://www.frontiersin.org/articles/10.3389/fevo.2022.845560/full#supplementary-material
Anderson, D. R. (2008). Model Based Inference in the Life Sciences: A Primer on Evidence. New York, NY: Springer Science & Business Media.
Bergström, U., and Englund, G. (2004). Spatial scale, heterogeneity and functional responses. J. Anim. Ecol. 73, 487–493.
Buxton, M., Cuthbert, R. N., Dalu, T., Nyamukondiwa, C., and Wasserman, R. J. (2020). Predator density modifies mosquito regulation in increasingly complex environments. Pest Manage. Sc. 76, 2079–2086. doi: 10.1002/ps.5746
Chase, J. M., and Leibold, M. A. (2003). Ecological Niches: Linking Classical and Contemporary Approaches. Chicago, IL: University of Chicago Press.
Clements, A. N. (1992). The Biology of Mosquitoes: Development, Nutrition and Reproduction, Vol. 1. London: Chapman and Hall.
Cressman, R., Køivan, V., Brown, J. S., and Garay, J. (2014). Game-Theoretic methods for functional response and optimal foraging behavior. PLoS One 9:e88773. doi: 10.1371/journal.pone.0088773
Cuthbert, R. N., Callaghan, A., Sentis, A., Dalal, A., and Dick, J. T. A. (2020). Additive multiple predator effects can reduce mosquito populations. Ecol. Entomol. 45, 243–250. doi: 10.1111/een.12791
Darsie, R. F., and Ward, R. A. (2005). Identification and Geographical Distribution of the Mosquitoes of North America, North of Mexico. Gainesville, FL: University Press of Florida.
Dick, J. T. A., Alexander, M. E., Ricciardi, A., Laverty, C., Downey, P. O., Xu, M., et al. (2017). Functional responses can unify invasion ecology. Biol. Invas. 19, 1667–1672. doi: 10.1007/s10530-016-1355-3
Focks, D. A. (2007). Toxorhynchites as biocontrol agents. J. Am. Mosquito Contr. Assoc. 23(Suppl. 2) 118–128. doi: 10.2987/8756-971X(2007)23[118:TABA]2.0.CO;2
Fortin, D., Buono, P. L., Schmitz, O. J., Courbin, N., Losier, C., St-Laurent, M. H., et al. (2015). A spatial theory for characterizing predator–multiprey interactions in heterogeneous landscapes. Proc. R. Soc. B 282:1812. doi: 10.1098/rspb.2015.0973
Fryxell, J. M., Mosser, A., Sinclair, A. R. E., and Packer, C. (2007). Group formation stabilizes predator–prey dynamics. Nature 449, 1041–1043. doi: 10.1038/nature06177
Griffen, B. D. (2021). Considerations when applying the consumer functional response measured under artificial conditions. Front. Ecol. Evol. 9:713147. doi: 10.3389/fevo.2021.713147
Griswold, M. W., and Lounibos, L. P. (2005). Does differential predation permit invasive and native mosquito larvae to coexist in Florida? Ecol. Entomol. 30, 122–127. doi: 10.1111/j.0307-6946.2005.00671.x
Gunn, J. C., Clements, S. J., Kansman, J. T., Cianci-Gaskill, J. A., and Anderson, T. L. (2021). Effects of habitat complexity on giant water bug (Belostoma) functional response to rams-horn snail prey (Helisoma). Hydrobiologia 848, 4585–4597. doi: 10.1007/s10750-021-04663-1
Hammill, E., Kratina, P., Vos, M., Petchey, O. L., and Anholt, B. R. (2015). Food web persistence is enhanced by non-trophic interactions. Oecologia 178, 549–556. doi: 10.1007/s00442-015-3244-3
Hauzy, C., Tully, T., Spataro, T., Paul, G., and Arditi, R. (2010). Spatial heterogeneity and functional response: an experiment in microcosms with varying obstacle densities. Oecologia 163, 625–636. doi: 10.1007/s00442-010-1585-5
Hoddle, M. S. (2003). The effect of prey species and environmental complexity on the functional response of Franklinothrips orizabensis: a test of the fractal foraging model. Ecol. Entomol. 28, 309–318.
Holling, C. S. (1959). Some characteristics of simple types of predation and parasitism. Can. Entomol. 91, 385–398.
Holling, C. S. (1965). The functional response of predators to prey density. Mem. Entomol. Soc. Can. 45, 1–60.
Holling, C. S. (1966). The functional response of invertebrate predators to prey density. Mem. Entomol. Soc. Can. 48, 1–86.
Hossie, T. J., and Murray, D. L. (2010). You can’t run but you can hide: refuge use in frog tadpoles elicits density-dependent predation by dragonfly larvae. Oecologia 163, 395–404. doi: 10.1007/s00442-010-1568-6
Hossie, T. J., and Murray, D. L. (2016). Spatial arrangement of prey affects the shape of ratio-dependent functional response in strongly antagonistic predators. Ecology 97, 834–841. doi: 10.1890/15-1535.1
Jeschke, J. M., Kopp, M., and Tollrian, T. (2002). Predator functional responses: discriminating between handling and digesting prey. Ecol. Monogr. 72, 95–112.
Juliano, S. A. (1989). Queueing models of predation and the importance of contingent behavioural choices for optimal foragers. Anim. Behav. 38, 757–770.
Juliano, S. A. (2001). “Non-linear curve fitting: predation and functional response curves,” in Design and Analysis of Ecological Experiments, 2 Edn, eds S. M. Scheiner and J. Gurevitch (Oxford: Oxford Univ. Press), 178–216. doi: 10.1186/s40462-021-00282-6
Juliano, S. A., and Reminger, L. (1992). The relationship between vulnerability to predation and behavior: geographic and ontogenetic differences in larval Treehole mosquitoes. OIKOS 63, 465–476.
Kalinkat, G., Brose, U., and Rall, B. C. (2013). Habitat structure alters top-down control in litter communities. Oecologia 172, 877–887. doi: 10.1007/s00442-012-2530-6
Kratina, P., Rosenbaum, B., Gallo, B., Horas, E. L., and O’Gorman, E. J. (2021). The combined effects of warming and body size on the stability of predator-prey interactions. Front. Ecol. Evol. 9:772078. doi: 10.3389/fevo.2021.772078
Kreuzinger-Janik, B., Brüchner-Hüttemann, H., and Traunspurger, W. (2019). Effect of prey size and structural complexity on the functional response in a nematode-nematode system. Nat. Sci. Rep. 9:5696. doi: 10.1038/s41598-019-42213-x
Linley, J. R. (1995). Behavior on approach to surface prey by larvae of Toxorhynchites amboinensis and T. brevipalpis (Diptera: Culicidae). J. Med. Entomol. 32, 53–65. doi: 10.1093/jmedent/32.1.53
Livdahl, T. P. (1979). Evolution of handling time: the functional response of a predator to the density of sympatric and allopatric strains of prey. Evolution 33, 765–768. doi: 10.1111/j.1558-5646.1979.tb04728.x
Madadi, H., Parizi, E. M., Allahyari, H., and Enkegaard, A. (2011). Assessment of the biological control capability of Hippodamia variegata (Col.: Coccinellidae) using functional response experiments. J. Pest Sci. 84, 447–455. doi: 10.1007/s10340-011-0387-9
McCard, M., South, J., Cuthbert, R. N., Dickey, J. W. E., McCard, M., and Dick, J. T. A. (2021). Pushing the switch: functional responses and prey switching by invasive lionfish may mediate their ecological impact. Biol. Invas. 23, 2019–2032. doi: 10.1007/s10530-021-02487-7
McCoy, M. W., Stier, A. C., and Osenberg, C. W. (2012). Emergent effects of multiple predators on prey survival: the importance of depletion and the functional response. Ecol. Lett. 15, 1449–1456. doi: 10.1111/ele.12005
Merritt, R. W., Dadd, R. H., and Walker, E. D. (1992). Feeding behavior, natural food, and nutritional relationships of larval mosquitoes. Annu. Rev. Entomol. 37, 349–376. doi: 10.1146/annurev.en.37.010192.002025
Messina, F. J., and Hanks, J. B. (1998). Host plant alters the shape of the functional response of an aphid predator (Coleoptera: Coccinellidae). Environ. Entomol. 27, 1196–1202. doi: 10.1093/ee/27.5.1196
Mocq, J., Soukup, P. R., Näslund, J., and Boukal, D. S. (2021). Disentangling the nonlinear effects of habitat complexity on functional responses. J. Anim. Ecol. 90, 1525–1537. doi: 10.1111/1365-2656.13473
Motulsky, H., and Christopoulos, A. (2004). Fitting Models to Biological Data Using Linear and Nonlinear Regression: A Practical Guide to Curve Fitting. New York, NY: Oxford University Press.
Novak, M., and Stouffer, D. B. (2021). Geometric complexity and the information-theoretic comparison of functional-response models. Front. Ecol. Evol. 9:740362. doi: 10.3389/fevo.2021.740362
Russo, R. (1983). The functional response of Toxorhynchites rutilus rutilus (Diptera: Culicidae), a predator on container-breeding mosquitoes. J. Med. Entomol. 20, 585–590.
Russo, R. (1986). Comparison of predatory behavior in five species of Toxorhynchites (Diptera: Culicidae). Ann. Entomol. Soc. Am. 79, 715–722.
Sentis, A., and Boukal, D. S. (2018). On the use of functional responses to quantify emergent multiple predator effects. Nat. Sci. Rep. 8:11787. doi: 10.1038/s41598-018-30244-9
Skiff, J. J., and Yee, D. A. (2014). Behavioral differences among four co-occurring species of container mosquito larvae: effects of depth and resource environments. J. Med. Entomol. 51, 375–381. doi: 10.1603/ME13159
Steffan, W. A., and Evenhuis, N. L. (1981). Biology of Toxorhynchites. Ann. Rev. Entomol. 26, 159–181.
Stephens, D. W., and Krebs, J. R. (1986). Foraging Theory. Princeton, NJ: Princeton University Press.
Timms, J. E., Oliver, T. H., Straw, N. A., and Leather, S. R. (2008). The effects of host plant on the coccinellid functional response: is the conifer specialist Aphidecta obliterata (L.) (Coleoptera: Coccinellidae) better adapted to spruce than the generalist Adalia bipunctata (L.) (Coleoptera: Coccinellidae)? Biol. Control 47, 273–281. doi: 10.1016/j.biocontrol.2008.08.009
Uiterwaal, S. F., and DeLong, J. P. (2018). Multiple factors, including arena size, shape the functional responses of ladybird beetles. J. Appl. Ecol. 55, 2429–2438. doi: 10.1111/1365-2664.13159
Uiterwaal, S. F., Dell, A. I., and DeLong, J. P. (2019). Arena size modulates functional responses via behavioral mechanisms. Behav. Ecol. 30, 483–489. doi: 10.1093/beheco/ary188
Uiterwaal, S. F., Mares, C., and DeLong, J. P. (2017). Body size, body size ratio, and prey type influence the functional response of damselfly nymphs. Oecologia 185, 339–346. doi: 10.1007/s00442-017-3963-8
Wasserman, R. J., Alexander, M. E., Weyl, O. L. F., Barrios-O’Neill, D., Froneman, P. W., and Dalu, T. (2016). Emergent effects of structural complexity and temperature on predator–prey interactions. Ecosphere 7:e01239. doi: 10.1002/ecs2.1239
Yaşar, B., and Özgar, S. (2005). Functional response of Oenopia conglobata (L.) (Coleoptera: Coccinellidae) on Hyalopterus pruni (Geoffroy) (Homoptera: Aphididae) in three different size arenas. Türk. Entomol. Derg. 29, 91–99.
Keywords: aggregation, area, edge effects, environmental complexity, predation, prey density, spatial dimensions, volume
Citation: Juliano SA, Goughnour JA and Ower GD (2022) Predation in Many Dimensions: Spatial Context Is Important for Meaningful Functional Response Experiments. Front. Ecol. Evol. 10:845560. doi: 10.3389/fevo.2022.845560
Received: 29 December 2021; Accepted: 07 March 2022;
Published: 29 March 2022.
Edited by:
Dennis Murray, Trent University, CanadaReviewed by:
Pavel Kratina, Queen Mary University of London, United KingdomCopyright © 2022 Juliano, Goughnour and Ower. This is an open-access article distributed under the terms of the Creative Commons Attribution License (CC BY). The use, distribution or reproduction in other forums is permitted, provided the original author(s) and the copyright owner(s) are credited and that the original publication in this journal is cited, in accordance with accepted academic practice. No use, distribution or reproduction is permitted which does not comply with these terms.
*Correspondence: Steven A. Juliano, c2FqdWxpYW5AaWxzdHUuZWR1
†Present address: Jonah A. Goughnour, Beckenham Dr., Bloomington, IL, United States; Geoffrey D. Ower, Illinois Natural History Survey, Prairie Research Institute, University of Illinois at Urbana-Champaign, Champaign, IL, United States
Disclaimer: All claims expressed in this article are solely those of the authors and do not necessarily represent those of their affiliated organizations, or those of the publisher, the editors and the reviewers. Any product that may be evaluated in this article or claim that may be made by its manufacturer is not guaranteed or endorsed by the publisher.
Research integrity at Frontiers
Learn more about the work of our research integrity team to safeguard the quality of each article we publish.