- College of Life Science and Technology, Harbin Normal University, Harbin, China
The effects of warming temperatures on embryonic and hatchling development are critical for determining the vulnerability of species to climate warming. However, these effects have rarely been investigated in high-latitude oviparous species, particularly in their low-latitude margin populations. This study investigated the embryonic and hatchling development and fitness-related traits of a low-latitude margin population of a high-latitude lizard (Lacerta agilis). These traits were examined under present (24°C), moderate warming (27 and 30°C), and severe warming scenarios (33°C). Based on embryonic and hatchling responses to thermal variation, this study aimed to predict the vulnerability of the early life stages of low-latitude margin population of Lacerta agilis to climate warming. The incubation period of the low-latitude margin population of Lacerta agilis decreased as the temperature increased from 24 to 33°C. Hatching success was similar at 24, 27, and 30°C but decreased significantly at 33°C. No differences with temperature were observed for hatchling snout-vent length and hatchling body mass. The sprint speed was higher for hatchlings from temperatures of 24 and 33°C. The growth rate of hatchlings was highest at 30°C; however, the survival rate of hatchlings was not affected by the thermal environment. This study demonstrated that even for a low-latitude margin population of the high-latitude lizard, Lacerta agilis, moderate warming (i.e., 27 and 30°C) would benefit embryonic and hatchling development. This was indicated by the results showing higher hatching success, growth rate, and survival rate. However, if temperatures increase above 33°C, development and survival would be depressed significantly. Thus, low-latitude margin population of high-latitude species Lacerta agilis would benefit from climate warming in the near future but would be under stress if the nest temperature exceeded 30°C.
Introduction
Anthropic climate warming has imposed many negative effects on animals, including changes in phenology (e.g., Gibbs and Breisch, 2001; Sherry et al., 2007), inhibition of dispersal (e.g., Massot et al., 2008), contractive distribution (e.g., Thomas et al., 2006), decreasing population growth (e.g., Deutsch et al., 2008), and extinction (e.g., Harte et al., 2004; Jevanandam et al., 2013). Therefore, understanding how animals respond to thermal variations caused by climate warming is critical. It is particularly relevant to understand thermal adaptation for determining the vulnerability of animals to the effects of climate change (Huey et al., 2012). Although it is likely that the effects of climate warming are evidently negative for most animals (Dillon et al., 2010; Barnosky et al., 2011), the vulnerability of species across different latitudes is still controversial. The warming rate has been predicted to increase with higher latitude, supporting the likelihood of increased vulnerability of animals at higher latitudes (e.g., Root et al., 2003; Parmesan, 2006). However, higher sensitivities to warming for low-latitude animals indicated the opposite pattern, that animals from low latitudes, including tropical areas, have a higher vulnerability to warming temperatures from climate change (e.g., Deutsch et al., 2008; Dillon et al., 2010; Sunday et al., 2011). Therefore, the response of animals from different latitudes to climate warming has received increasing attention. In contrast to low latitudes, where animal responses to thermal variations and climate warming have been extensively investigated (e.g., Huey et al., 2009; Laurance and Useche, 2009; Sinervo et al., 2010; Logan et al., 2013; Tao et al., 2021), much less investigations with focus on the responses to climate warming of animals from high latitudes.
Increasing knowledge about physiological and life-history responses to warming temperatures is critical for evaluating potential vulnerabilities (Williams et al., 2008; Huey et al., 2012; Pacifici et al., 2015). As typical ectothermic vertebrates, oviparous reptiles are physiologically sensitive to thermal variations, particularly during the early stages of life (e.g., embryonic development). Therefore, reptiles have an important role in the context of investigating their physiological and life-history responses to thermal variations and climate warming (Huey et al., 2012). Reptiles have been found to be negatively affected by climate warming (e.g., Huey et al., 2010; Sinervo et al., 2010; Barnosky et al., 2011; Diele-Viegas et al., 2020; Taylor et al., 2021). Furthermore, without parental care, most reptile embryos face developmental challenges from the surrounding environment (Du and Shine, 2015; Noble et al., 2018a,b; Du et al., 2019). In addition, many environmental factors can affect embryonic and hatchling development, including hatching success, incubation period, body size and growth of hatchlings, and survival rates (e.g., Sun et al., 2014; Du and Shine, 2015; Zhang et al., 2016; Noble et al., 2018b; Li et al., 2020). Recent research has revealed that reptile embryos are more vulnerable to climate warming than adults because of their lower heat tolerance. Embryonic heat tolerance has thus been predicted to limit the distribution of species under climate warming (Angilletta et al., 2013; Levy et al., 2015; Carlo et al., 2018; Hall and Sun, 2021; Sun et al., 2021).
Reptiles from low latitudes have been predicted to migrate toward high latitudes. This will allow them to escape from the heat stress caused by climate warming (e.g., Massot et al., 2008; Boyle et al., 2016). In addition, the embryos of reptiles at high latitudes may benefit from climate warming by enhancing hatching success, hatchling growth, and survival rates (Andrews et al., 1999; Qualls and Andrews, 1999; Li et al., 2018; Liu et al., 2022). However, for reptile species that have a wide geographic distribution across high latitudes, the response to climate warming by its low-latitude margin population (i.e., population from low-latitude margin of its distribution area) is relatively unknown. Investigating the embryonic and hatchling responses of the low-latitude margin population of high-latitude species to warming temperatures (e.g., Olsson et al., 2011; Ljungström et al., 2015) would increase the understanding of species response to climate warming across latitudes.
This study focused on a low-latitude margin population (i.e., Burqin, Xinjiang, China) of the sand lizard (Lacerta agilis), which is a high-latitude species. The eggs were incubated at four different temperatures to mimic the present (i.e., 24°C), moderate warming (27 and 30°C), and severe warming (33°C) scenarios for the population according to predicted warming rate. The hatchlings were then reared at the present, moderate warming, and severe warming scenarios coherently. The increase in egg mass during incubation, hatching success, incubation period, hatchling body size, growth, and survival rates were then determined to reveal the thermal effects of different warming scenarios on embryonic and hatchling development. Furthermore, warming scenarios would benefit the embryonic and hatchling development of Lacerta agilis if the low-latitude margin population responded in the same way to the benefits of climate warming as that documented in other species from high latitudes (Andrews et al., 1999; Qualls and Andrews, 1999; Li et al., 2018). Alternatively, it was predicted that the warming scenarios would depress embryonic and hatchling development. This would have been the case if this population followed the consensus that warming temperatures have a negative effect on embryonic and hatchling development.
Materials and Methods
Study Species
The sand lizard (Lacerta agilis) is a medium-size oviparous lizard of up to 114 mm in snout-vent length (SVL). This species is widely distributed across northern Europe, Russia, Mongolia, and Xinjiang in China (61° N–43°N), which have cold climates with significant thermal fluctuations (Strijbosch and Creemers, 1988; Trakimas, 2005; Ekner et al., 2008). Sand lizard habitats were found in forest, shrubland, and grassland (Figure 1A). The sand lizard becomes active in the field in March after the end of hibernation then starts the reproductive season. During the reproductive season, females lay one or two clutches of 3–11 eggs per clutch (IUCN).1 Previous research on Lacerta agilis provided key background information, thereby making it a great study system for investigating the responses of high-latitude reptiles to thermal variations and climate warming (e.g., Olsson, 1994a,b; Olsson and Shine, 1996, 1997a,1997b; Madsen et al., 2000; Ljungström et al., 2015).
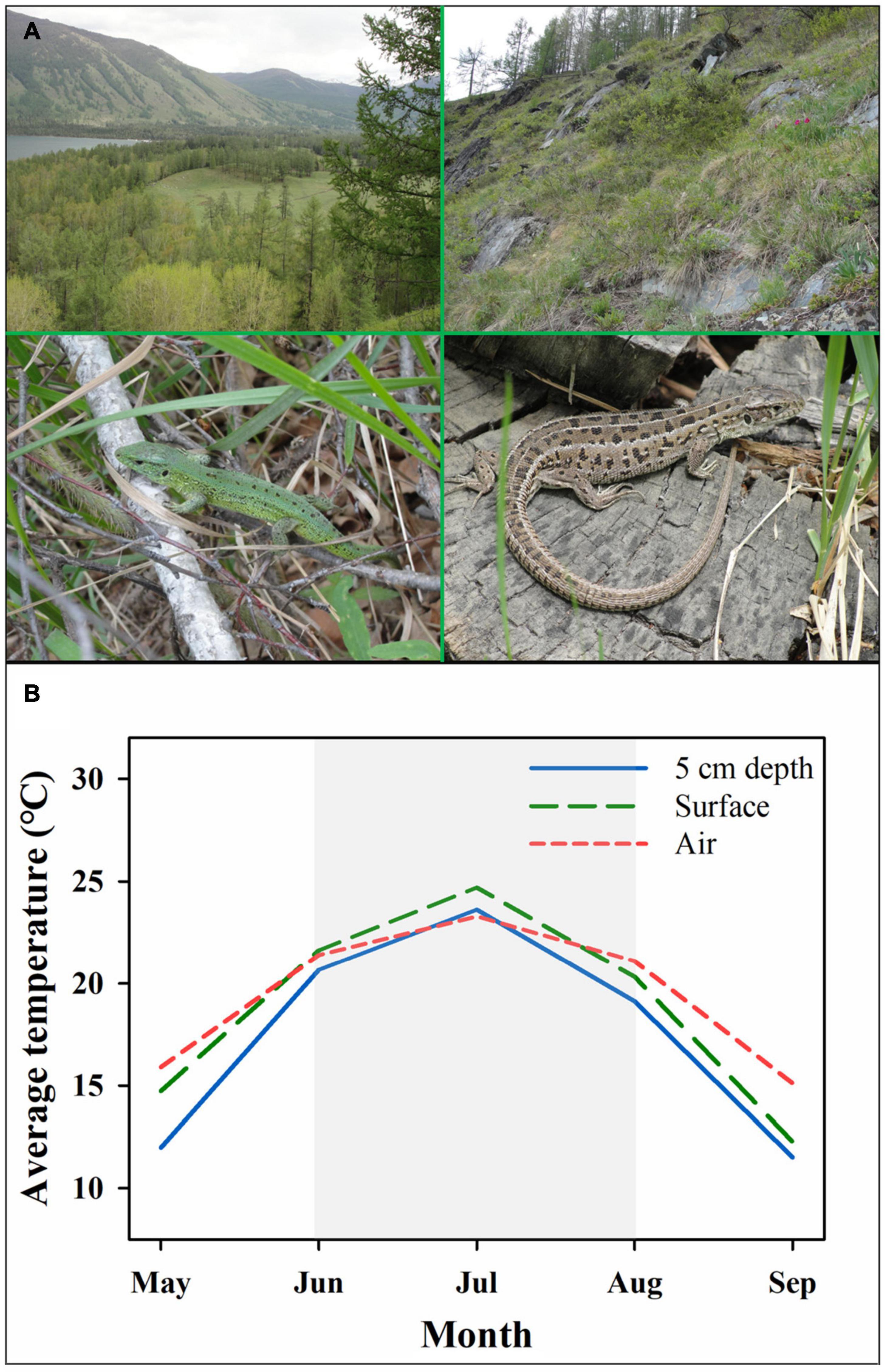
Figure 1. Natural habitats of (A) and thermal environments (B) for sand lizards (Lacerta agilis) from Burqin population. (A) The photographs indicate the natural habitats and demographic photographs for Lacerta agilis. (B) The blue solid, green long dash and red short dash lines indicate the temperatures of nest at depth of 5 cm underground, surface and air. The shade column indicates the reproductive season mainly for embryonic development. The data of temperatures were from China Meteorological Data Service Centre (http://data.cma.cn/en).
Animal Collection and Environmental Factors
Adult lizards were collected in early May of 2014 from Burqin, Xinjiang, China, (47°22′ N, 88°06′ E), which is almost the lowest latitude at which Lacerta agilis is found. The lowest monthly average temperature was –16°C in January, and the highest monthly average temperature was 21°C in July in Burqin.2 All the lizards were collected either by hand or using a lizard noose. The active body temperature of a subset of lizards (n = 16) was measured randomly during collection. The average body temperature for lizards in the field was 32.97 ± 0.62°C (28.2–36.3°C). After collection, the lizards were transferred to the laboratory at the Harbin Normal University. Six to eight lizards were housed in each terrarium (950 × 450 × 500 mm, length × width × height). These were placed in a temperature-controlled room at 18°C, with a photoperiod of 12 L: 12D (0700–1,900). A 100 W UVA + UVB heating lamb was suspended 50 mm above one end of each terrarium to provide a thermal gradient of 18–40°C for behavioral thermoregulation from 0800 to 1,600. Food (Larva of Tenebrio molitor and crickets dusted with mixed vitamins) and water were provided ad libitum.
Embryonic Development
The female lizards were palpated every other day to determine their gravid status. The female lizards with oviduct eggs were reared individually in plastic terraria (35 × 30 × 40 mm, length × width × height). The terraria were lined with 100 mm of moist soil, where the females laid their eggs. The terraria were checked three times per day for freshly laid eggs. Once found, the eggs were weighed, numbered, and randomly assigned to one of the four incubation temperatures. A total of 160 eggs from 43 females were incubated. A split clutch design was used for incubation, with eggs from each female being incubated at different temperatures. The present temperature scenario was set according to soil temperatures during June and July (Figure 1B). Moderate warming scenarios were set to mimic the maximum temperature increasing at RCP 4.5 and RCP 6.0 scenarios, while the severe warming scenario was set to that of RCP 8.5 (IPCC, 2013, 2021). The incubation temperatures were set at 24, 27, 30, and 33°C, which mimicked the thermal environments under present climates (24°C), moderate warming scenarios (27 and 30°C), and severe warming scenario (33°C). The eggs were half-buried in plastic boxes (160 × 115 × 40 mm) which were filled with moist vermiculite (–220 kPa). A subset of eggs (7, 10, 9, and 8 eggs for 24, 27, 30, and 33°C) were measured every 5 days, to determine the increase in egg mass during incubation. Water was added to the boxes every 5 days to maintain the moisture of the vermiculite until the hatchlings emerged. After hatching, the incubation period was recorded. The hatching success was calculated by the ratio of the number of hatchlings to the number of eggs incubated.
Hatchling Husbandry
The hatchlings were measured for SVL (± 0.001 mm) and weighed for body mass (BM, ± 0.001 g). Hatchlings were individually marked and reared under different thermal regimes. To detect the coherent effects of temperature on embryonic and hatchling development, the hatchlings were reared at the same average temperatures as those under incubation. These were 24 ± 3°C, 27 ± 3°C, 30 ± 3°C, and 33 ± 3°C for hatchings that incubated at 24, 27, 30, and 33°C, respectively. A total of 8–10 hatchlings were reared in each temperature-controlled chamber (450 L), with a light cycle of 12 L:12D (0700–1,900). Food and water were provided ad libitum.
Locomotion
One week after hatching, the locomotor performance of the hatchlings was started to be determined. Using the average body temperatures recorded in the field as a baseline (32.97°C), the locomotor performance tests were conducted at 33°C. Prior to the test, the hatchlings were acclimated to a temperature at 33°C for at least 1 h. Hatchlings were encouraged to run across a 1 m racetrack by touching their tails with a soft paintbrush. The process was recorded using a digital video camera (DCR-SR220E, Sony, Japan). Each hatchling was tested twice with an interval of 1 h between each test for resting. The videos were then analyzed using AVS Video Editor software (Online Media Technologies Ltd., United Kingdom). The fastest speed at 20 cm intervals was recorded. The average of the fastest speed out of the two tests was calculated as being the sprint speed. The maximum distance for each burst and the number of stops during each test were also recorded, and the average of two records were calculated as the maximum distance and number of stops, respectively.
Growth and Survival
Husbandry of all the hatchlings lasted for at least 40 days. The hatchlings were weighed and measured, with their survival status being checked every 10 days after hatching. SVL and BM were recorded to determine the growth rate. The growth rate was calculated as the difference in SVL and BM per day, expressed as mm/day and g/day, respectively. Survival rates were determined by the number of live individuals after 40 days of husbandry against the total number of hatchlings.
Statistical Analysis
IBM SPSS 21.0 was used for statistical analysis. Before the analysis, the normality of distributions and homogeneity was tested using the Kolmogorov–Smirnov test and Levene’s test, respectively. As the initial egg mass being the same but the embryonic processes were different on the same day across the incubation temperatures, the egg mass increase was analyzed using the final egg mass before hatching by one-way analysis of covariance (ANCOVA), with incubation temperature was the main factor, and initial egg mass was a covariate. The incubation period for the embryos, hatchling sprint speed, and the number of stops were analyzed by one-way ANOVA, with incubation temperature as the main factor. The hatchling SVL and BM and growth rate of hatchlings SVL and BM were analyzed by one-way ANCOVA with incubation temperatures, and husbandry temperature as the main factors, and egg mass, initial SVL, and BM as covariates, respectively. The hatchling SVL and hatchling BM before winter were analyzed by one-way ANOVA. The hatching success and hatchling survival rates were analyzed by the Chi-square test. The Tukey post hoc comparison was conducted once significant difference was detected across temperature regimes.
Results
Embryonic Development
The egg mass increase was affected by incubation temperatures across the incubation duration, with egg mass increasing the most at 24°C than at other incubation temperatures [F(3, 29) = 10.858, P < 0.0001]. The other incubation temperatures did not influence the egg mass increase (min P = 0.054) (Figure 2). The incubation period was influenced by the incubation temperature, which decreased from 24 to 33°C [F(3, 63) = 557.55, P < 0.0001] (Figure 3A). The hatching success was significantly different among incubation temperatures, which was lower at 33°C (8/40) than at 24°C (14/40), 27°C (21/40), and 30°C (24/40) (χ2 = 15.89, P < 0.01). However, the incubation temperatures of 24–30°C did not affect hatching success (all P > 0.05) (Figure 3B).
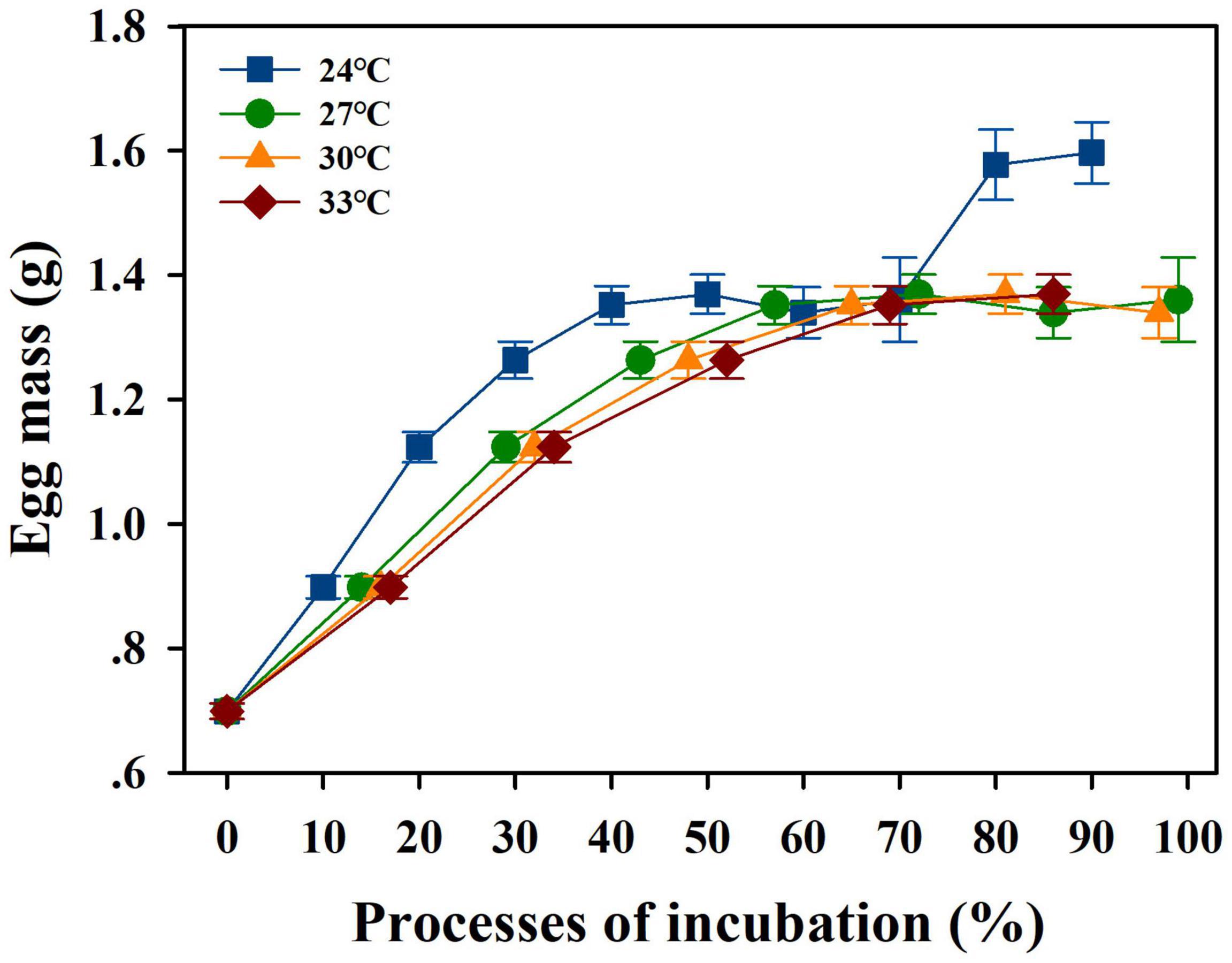
Figure 2. Egg mass increase during incubation. The blue, green, orange and red spot and lines indicate the eggs incubated under 24, 27, 30, and 33°C, respectively. The data are shown as mean ± SE.
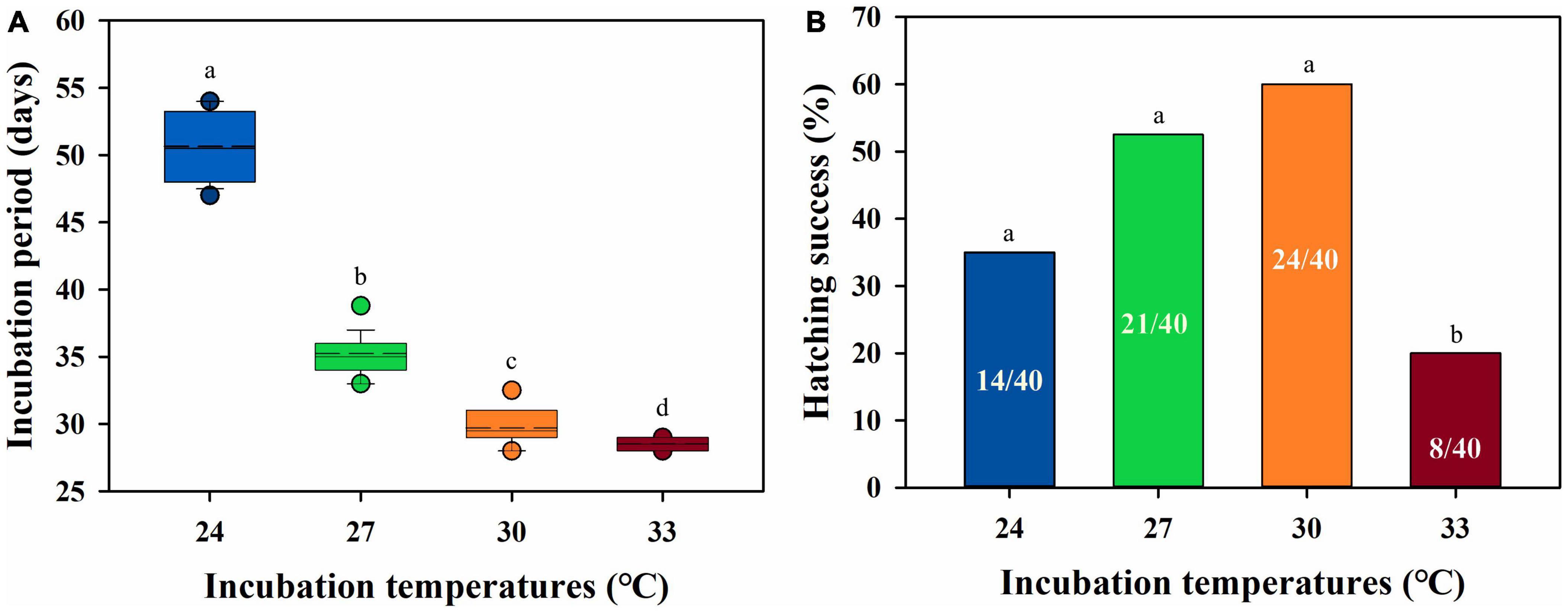
Figure 3. Incubation period (A) and hatching success (B) of embryos under 24, 27, 30, and 33°C. (A) The dash lines indicate the mean value of incubation period; the data are shown as mean ± SE. (B) The number in the columns indicate the sample size for each temperature. The different letters above the error bars indicate the significant difference between incubation temperatures.
Hatchling Body Size and Locomotion
Hatchling snout-vent length (HSVL) [F(3, 62) = 1.010, P = 0.394] and body mass (HBM) [F(3, 62) = 2.050, P = 0.116] were not affected by incubation temperatures (Table 1). The sprint speed of hatchlings was significantly affected by incubation temperature [F(3, 61) = 6.827, P < 0.001]. Hatchlings incubated at 24 and 33°C had higher sprint speeds than siblings at 27 and 30°C (Figure 4A). The number of stops for hatchlings at 30°C were significantly higher than the siblings at 27°C, along with those at 24 and 33°C [F(3, 61) = 3.838, P = 0.014] (Figure 4B). However, the maximum distance for each sprint test was not influenced by incubation temperature [F(3, 61) = 1.886, P = 0.141].
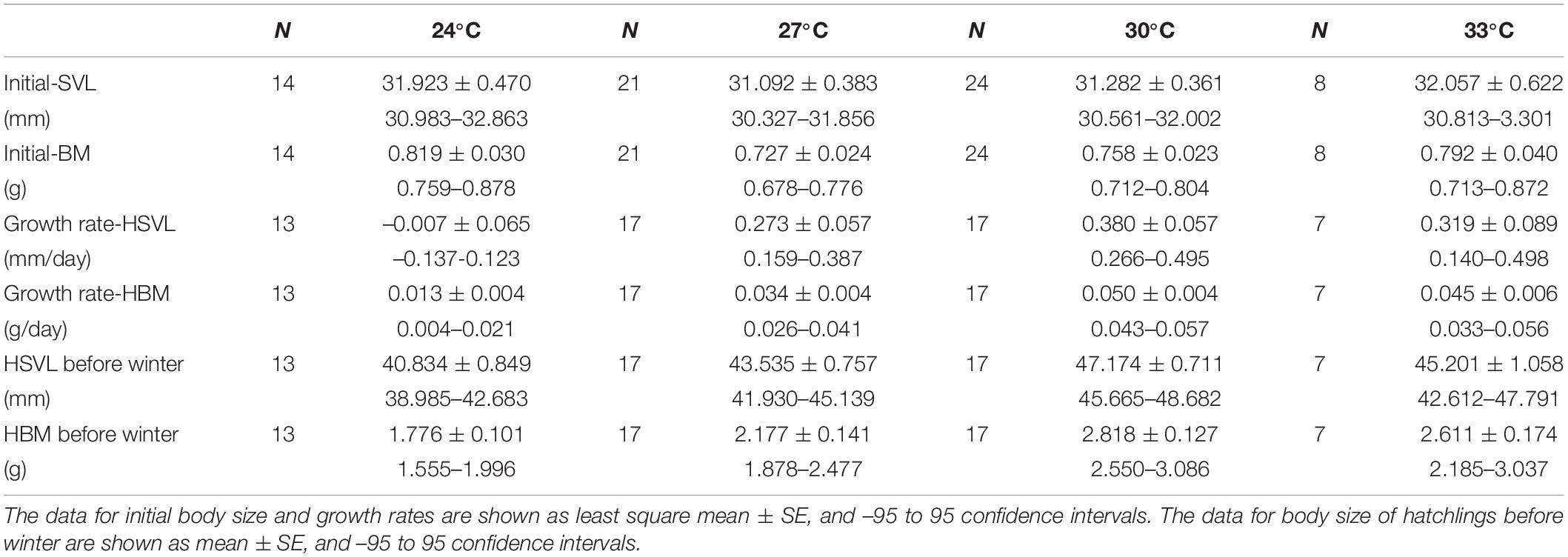
Table 1. Initial body size (SVL and BM), growth rate of hatchlings (SVL and BM), and hatchlings body size (SVL and BM) before winter under different temperatures.
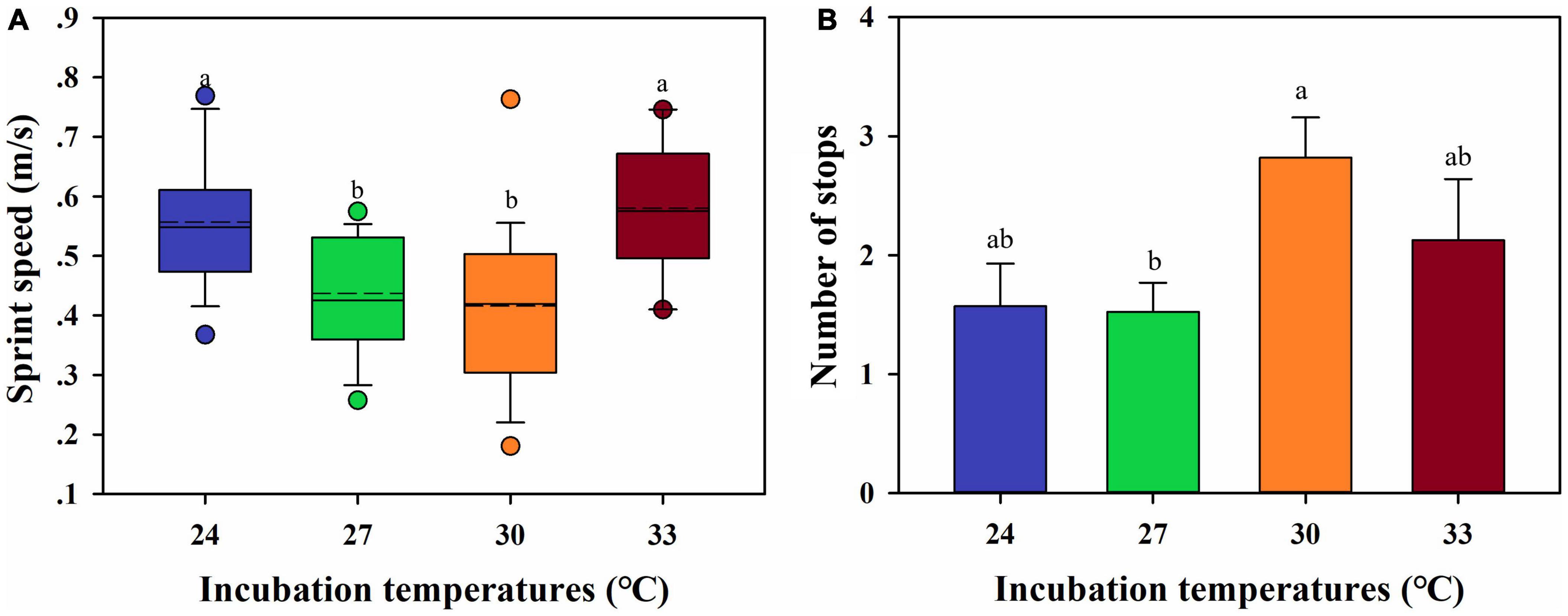
Figure 4. Sprint speed (A) and the number of stops (B) of hatchlings incubated at 24, 27, 30, and 33°C. (A) The dash lines indicate the mean value of sprint speed; the data are shown as mean ± SE. (A,B) The different letters above the bars indicate the significant differences between incubation temperatures.
Hatchling Development
The growth rates of HSVL and HBM were significantly affected by the thermal environment of the embryos and hatchlings. The growth rate of HSVL in hatchlings at 24°C was lower than siblings from temperatures of 27, 30, and 33°C [F(3, 49) = 7.234, P < 0.001; 30°C a > 33°C a > 27°C a > 24°C b] (Table 1). The growth rate of HBM was the lowest for hatchlings from 24°C, highest for hatchlings from 30°C, and in between for hatchlings from 27 and 33°C [F(3, 49) = 7.234, P < 0.001; 30°C a > 33°C ab > 27°C b > 24°Cc] (Table 1). Consequently, the SVL [F(3, 50) = 11.484, P < 0.0001; 30°C a > 33°C ab > 27°C b > 24°C c] and BM before winter [F(3, 50) = 11.834, P < 0.0001; 30°C a > 33°C ab > 27°C b >24°C c] were the highest for hatchlings at 30°C, and the lowest at 24°C, with those at 27 and 33°C in between (Table 1). However, the survival rates of hatchlings from 24 (10/14, 71.4%), 27 (17/21, 80.9%), 30 (17/24, 70.8%), and 33°C (7/8, 87.5%) were similar after 40 days of husbandry before winter (χ2 = 1.38, P > 0.05).
Discussion
The effects of climate warming on animals have been extensively investigated over the previous decades (Pacifici et al., 2015). Although the current consensus is that climate warming would have negative effects on animals at many biological hierarchies, additional research is required to comprehensively determine the latitudinal pattern of vulnerabilities (e.g., Root et al., 2003; Parmesan, 2006; Huey et al., 2009, 2012; Dillon et al., 2010; Sun et al., 2021). This study focuses on how the low-latitude margin population of a high-latitude species, Lacerta agilis, would respond to climate warming.
Although in this study, low incubation temperature (i.e., 24°C) enhanced the egg mass during incubation (Figure 2), they did not affect the hatchling body size (Table 1). In contrast, with shorter incubation periods and enhanced hatching success, embryonic development was found to be enhanced under moderate warming scenarios at 27 and 30°C (Figure 3). Furthermore, higher growth rates in SVL and BM indicated that moderate (30°C) and severe (33°C) warming temperatures were beneficial for hatchling development (Figure 4 and Table 1). In combination, similar to other high-latitude reptiles (e.g., Andrews et al., 1999; Qualls and Andrews, 1999; Li et al., 2018; Liu et al., 2022), moderate warming (i.e., 30°C) would benefit embryonic and hatchling development in the low-latitude margin population of a high-latitude lizard, Lacerta agilis.
Moderate Warming Enhanced the Embryonic Development
For oviparous species, a shortened incubation period has been considered an advantage for hatchlings. In this study, the moderate warming temperatures and severe warming temperature shortened the incubation period, potentially decreasing the possibility of embryos being preyed upon by predators (e.g., Torralvo et al., 2017) or having reduced survival rates due to extreme heat events (Levy et al., 2015; Ma et al., 2018b; Hall and Sun, 2021; Sun et al., 2021). Meanwhile, a shorter incubation period can allow a relatively long period for hatchling development. This potentially increases the chance of hatchlings having an increased body size to respond to the challenges of lower temperatures during the autumn and winter months (e.g., Sun et al., 2018a; Liu et al., 2022). The physiological and biochemical mechanisms underlying the shortened incubation period may be associated with enhanced mitochondrial functions and metabolic enzyme activities in embryos at high temperatures (e.g., Sun et al., 2015). By enhancing the mitochondrial metabolism, embryonic metabolism and thus embryonic development can be significantly increased. The incubation period can therefore be shortened (e.g., Du and Shine, 2010, 2015; Du et al., 2010a,b, 2011; Sun et al., 2013; Liu et al., 2022).
Hatching success is an indicator of the survival rate of embryos during development, which is a critical proxy for evaluating the effects of incubation environments on embryonic development (e.g., Du and Shine, 2015; Noble et al., 2018b). In this study, moderate warming temperatures increased hatching success, without any depress on hatchling SVL and BM (Table 1), indicating the presence of a higher hatchling recruitment for the population (e.g., Ma et al., 2018a,b; Liu et al., 2022). However, a severe warming incubation temperature of 33°C significantly decreased hatching success, with only 20% (8/40) of embryos hatched successfully. In combination, moderate warming temperatures of 27 and 30°C, respectively, led to higher hatching success and shortened incubation periods, both of which are advantages for embryonic development.
Hatchling Growths Are Enhanced by Warming Husbandry
For hatchling husbandry in this study, the same thermal regimes were consistently used for incubation temperatures, with a ± 3°C fluctuation. Although this limited the interpretation of the interactive effects of incubation and husbandry temperatures, which are normally employed in factory-manipulated investigations (e.g., Deeming and Ferguson, 1991; Taylor et al., 2021). The current thermal regime still met the need to evaluate the thermal effects on the development of hatchlings. With higher growth (Table 1) and equal survival rates, the hatchlings from moderate warming at 30°C and severe warming at 33°C both benefited in comparison with hatchlings from the present thermal environments of 24°C in this study. The higher growth rate of hatchlings allowed faster mass accumulation before winter, which improved resistance to cold weather during the winter months, which is a widespread adaptive strategy employed at high latitudes by many animals, including fish, reptile, and mammal (e.g., Festa-Bianchet et al., 1997; Larivée et al., 2010; Eto et al., 2018; Sun et al., 2018b; Lu et al., 2019). As a high-latitude species, Lacerta agilis faces the challenge of cold climatic conditions for a long time from October to May each year. Owing to their large body sizes, the hatchlings are predicted to have enhanced survival rates during winter (e.g., Litvak and Leggett, 1992; Steiger, 2013). In addition, larger body size for Lacerta agilis is a critical proxy for mating choice, as larger adults may have priority in mating, thereby enhancing reproduction (Olsson, 1993).
Implication for Vulnerability to Climate Warming
At the most sensitive life-history stage, understanding the role of physiological proxies of embryos and hatchlings, such as their hatching success, incubation period, hatchling growth, and body size, to thermal variations are fundamental and necessary to evaluate the vulnerabilities of species and their populations (e.g., Pike, 2014; Howard et al., 2015; Ma et al., 2018a,b). In this study, we mimicked the present climate and moderate and severe warming scenarios to reveal the responses of embryos and hatchlings according to different RCP scenarios (IPCC, 2013, 2021). Based on the responses of embryos and hatchlings, it was predicted that the low-latitude margin population of Lacerta agilis would not be vulnerable to moderate climate warming but would be severely vulnerable to severe warming scenarios.
This study revealed that moderate warming temperatures of 27 and 30°C enhanced embryonic development. Moderate warming at 30°C and severe warming temperatures of 33°C facilitated hatchling development. Based on the present findings, it is predicted that moderate warming scenarios for embryonic and hatchling development would be beneficial for Lacerta agilis. In contrast, severe warming temperatures also enhanced the growth of hatchlings but reduced hatching success at 33°C. This would be harmful to population recruitment (e.g., Spear and Nur, 1994; Tang et al., 1998). Similarly, extreme warming temperatures for embryonic development would also depress the hatchling body size, growth rates, and even survival rates in the brown anoles (Anolis sagrei) and fence lizards (Carlo et al., 2018; Hall and Warner, 2021).
In summary, with the low-latitude margin population of a high-altitude species, Lacerta agilis, we found that embryonic and hatchling development can be enhanced under moderate warming temperatures. The responses follow the increasingly demonstrated benefits of climate warming at high latitudes. This has indicated that Lacerta agilis would not be vulnerable to climate warming in the near future. Nonetheless, this study was limited by its failure to reveal the interactive effects of incubation and hatchling temperatures on embryonic and hatchling development (e.g., Sun et al., 2018a,b). Moreover, with a focus on embryonic development, the nesting behavior and maternal effects of females in this study were excluded, that can significantly affect embryonic and hatchling development (e.g., Refsnider and Janzen, 2010a,b; Meylan et al., 2012; Li et al., 2018). Further research is required to reveal the interactive effects of embryonic and hatchling developmental temperatures, as well as the effects of nesting behavior and maternal modifications.
Data Availability Statement
The original contributions presented in the study are included in the article/Supplementary Material, further inquiries can be directed to the corresponding author/s.
Ethics Statement
The animal study was reviewed and approved by Animal Ethics Committees of Harbin Normal University.
Author Contributions
LC, CY, WZ, and PL designed the studies. CY, LC, DZ, SL, and PL collected the data. LC, WZ, and PL analyzed the data and led the draft writing. All authors contributed critically to the drafts and gave final approval for publication.
Funding
This work was supported by the National Natural Science Foundation of China (Project No. 31172079) to WZ, the Natural Science Foundation of Heilongjiang, China (Project No. C2016035) to PL. LC was supported by the Doctoral Innovation Fund of Harbin Normal University (HSDBSCX2021-03).
Conflict of Interest
The authors declare that the research was conducted in the absence of any commercial or financial relationships that could be construed as a potential conflict of interest.
Publisher’s Note
All claims expressed in this article are solely those of the authors and do not necessarily represent those of their affiliated organizations, or those of the publisher, the editors and the reviewers. Any product that may be evaluated in this article, or claim that may be made by its manufacturer, is not guaranteed or endorsed by the publisher.
Acknowledgments
We thank Zhitao Liu, Hui Chen, Mengyan Zhu, Wanli Liu, and Yu Meng for their assistance in the field and Lab. We thank Baojun Sun for constructive comments that greatly improved the manuscript.
Supplementary Material
The Supplementary Material for this article can be found online at: https://www.frontiersin.org/articles/10.3389/fevo.2022.845309/full#supplementary-material
Footnotes
References
Andrews, R. M., Mathies, T., Qualls, C. P., and Qualls, F. J. (1999). Rates of embryonic development of Sceloporus lizards: Do cold climates favor the evolution of rapid development? Copeia 1999, 692–700. doi: 10.2307/1447601
Angilletta, M. J. Jr., Zelic, M. H., Adrian, G. J., Hurliman, A. M., and Smith, C. D. (2013). Heat tolerance during embryonic development has not diverged among populations of a widespread species (Sceloporus undulatus). Conserv. Physiol. 1:cot018. doi: 10.1093/conphys/cot018
Barnosky, A. D., Matzke, N., Tomiya, S., Wogan, G. O. U., Swartz, B., Quental, T. B., et al. (2011). Has the Earth’s sixth mass extinction already arrived? Nature 471, 51–57. doi: 10.1038/nature09678
Boyle, M., Schwanz, L., Hone, J., and Georges, A. (2016). Dispersal and climate warming determine range shift in model reptile populations. Ecol. Model. 328, 34–43. doi: 10.1016/j.ecolmodel.2016.02.011
Carlo, M. A., Riddell, E. A., Levy, O., and Sears, M. W. (2018). Recurrent sublethal warming reduces embryonic survival, inhibits juvenile growth, and alters species distribution projections under climate change. Ecol. Lett. 21, 104–116. doi: 10.1111/ele.12877
Deeming, D. C., and Ferguson, M. W. J. (1991). Egg Incubation: Its Effects on Embryonic Development in Birds and Reptiles. Cambridge: Cambridge University Press.
Deutsch, C. A., Tewksbury, J. J., Huey, R. B., Sheldon, K. S., Ghalambor, C. K., Haak, D. C., et al. (2008). Impacts of climate warming on terrestrial ectotherms across latitude. Proc. Natl. Acad. Sci. U.S.A. 105, 6668–6672. doi: 10.1073/pnas.0709472105
Diele-Viegas, L. M., Figueroa, R. T., Vilela, B., and Rocha, C. F. D. (2020). Are reptiles toast? A worldwide evaluation of Lepidosauria vulnerability to climate change. Clim. Change 159, 581–599. doi: 10.1007/s10584-020-02687-5
Dillon, M. E., Wang, G., and Huey, R. B. (2010). Global metabolic impacts of recent climate warming. Nature 467, 704–706. doi: 10.1038/nature09407
Du, W. G., and Shine, R. (2010). Why do the eggs of lizards (Bassiana duperreyi: Scincidae) hatch sooner if incubated at fluctuating rather than constant temperatures? Biol. J. Linn. Soc. 101, 642–650. doi: 10.1111/j.1095-8312.2010.01525.x
Du, W. G., and Shine, R. (2015). The behavioural and physiological strategies of bird and reptile embryos in response to unpredictable variation in nest temperature. Biol. Rev. 90, 19–30. doi: 10.1111/brv.12089
Du, W. G., Shine, R., Ma, L., and Sun, B. J. (2019). Adaptive responses of the embryos of birds and reptiles to spatial and temporal variations in nest temperatures. Proc. R. Soc. B Biol. Sci. 286:20192078. doi: 10.1098/rspb.2019.2078
Du, W. G., Ye, H., Zhao, B., Pizzatto, L., Ji, X., and Shine, R. (2011). Patterns of interspecific variation in the heart rates of embryonic reptiles. PLoS One 6:e29027. doi: 10.1371/journal.pone.0029027
Du, W. G., Zhao, B., and Shine, R. (2010a). Embryos in the fast lane: high-temperature heart rates of turtles decline after hatching. PLoS One 5:e9557. doi: 10.1371/journal.pone.0009557
Du, W. G., Warner, D. A., Langkilde, T., Robbins, T., and Shine, R. (2010b). The physiological basis of geographic variation in rates of embryonic development within a widespread lizard species. Am. Nat. 176, 522–528. doi: 10.1086/656270
Ekner, A., Majlath, I., Majlathova, V., Hromada, M., Bona, M., Antczak, M., et al. (2008). Densities and morphology of two co-existing lizard species (Lacerta agilis and Zootoca vivipara) in extensively used farmland in Poland. Folia Biol. 56, 165–171. doi: 10.3409/fb.56_3-4.165-171
Eto, T., Sakamoto, S. H., Okubo, Y., Tsuzuki, Y., Koshimoto, C., and Morita, T. (2018). Individual variation of daily torpor and body mass change during winter in the large Japanese field mouse (Apodemus speciosus). J. Comp. Physiol. B. 188, 1005–1014. doi: 10.1007/s00360-018-1179-9
Festa-Bianchet, M., Jorgenson, J. T., Bérubé, C. H., Portier, C., and Wishart, W. D. (1997). Body mass and survival of bighorn sheep. Can. J. Zool. 75, 1372–1379. doi: 10.1139/z97-763
Gibbs, J. P., and Breisch, A. R. (2001). Climate warming and calling phenology of frogs near lthaca, New York, 1900–1999. Conserv. Biol. 15, 1175–1178. doi: 10.1046/j.1523-1739.2001.0150041175.x
Hall, J. M., and Sun, B. J. (2021). Heat tolerance of reptile embryos: current knowledge, methodological considerations, and future directions. J. Exp. Zool. A 335, 45–58. doi: 10.1002/jez.2402
Hall, J. M., and Warner, D. A. (2021). Thermal sensitivity of lizard embryos indicates a mismatch between oxygen supply and demand at near-lethal temperatures. J. Exp. Zool. A 335, 72–85. doi: 10.1002/jez.2359
Harte, J., Ostling, A., Green, J. L., and Kinzig, A. (2004). Climate change and extinction risk. Nature 430, 34–34.
Howard, R., Bell, I., and Pike, D. A. (2015). Tropical flatback turtle (Natator depressus) embryos are resilient to the heat of climate change. J. Exp. Biol. 218, 3330–3335. doi: 10.1242/jeb.118778
Huey, R. B., Deutsch, C. A., Tewksbury, J. J., Vitt, L. J., Hertz, P. E., Álvarez Pérez, H. J., et al. (2009). Why tropical forest lizards are vulnerable to climate warming. Proc. R. Soc. B Biol. Sci. 276, 1939–1948. doi: 10.1098/rspb.2008.1957
Huey, R. B., Kearney, M. R., Krockenberger, A., Holtum, J. A., Jess, M., and Williams, S. E. (2012). Predicting organismal vulnerability to climate warming: roles of behaviour, physiology and adaptation. Philos. Trans. R. Soc. Lond. B Biol. Sci. 367, 1665–1679. doi: 10.1098/rstb.2012.0005
Huey, R. B., Losos, J. B., and Moritz, C. (2010). Are Lizards Toast? Science 328, 832–833. doi: 10.1126/science.1190374
IPCC (2013). Climate Change 2013:The Physical Science Basis. Contribution of Working Group I to the Fifth Assessment Report of the Intergovernmental Panel on Climate Change. Cambridge: Cambridge University Press.
IPCC (2021). “Summary for Policymakers,” in Climate Change 2021: The Physical Science Basis. Contribution of Working Group I to the Sixth Assessment Report of the Intergovernmental Panel on Climate Change, eds V. Masson-Delmotte, P. Zhai, A. Pirani, S. L. Connors, C. Péan, S. Berger, et al. (Cambridge: Cambridge University Press).
Jevanandam, N., Goh, A. G. R., and Corlett, R. T. (2013). Climate warming and the potential extinction of fig wasps, the obligate pollinators of figs. Biol. Lett. 9:20130041. doi: 10.1098/rsbl.2013.0041
Larivée, M. L., Boutin, S., Speakman, J. R., Mcadam, A. G., and Humphries, M. M. (2010). Associations between over-winter survival and resting metabolic rate in juvenile North American red squirrels. Funct. Ecol. 24, 597–607. doi: 10.1111/j.1365-2435.2009.01680.x
Laurance, W. F., and Useche, D. C. (2009). Environmental synergisms and extinctions of tropical species. Conserv. Biol. 23, 1427–1437. doi: 10.1111/j.1523-1739.2009.01336.x
Levy, O., Buckley, L. B., Keitt, T. H., Smith, C. D., Boateng, K. O., Kumar, D. S., et al. (2015). Resolving the life cycle alters expected impacts of climate change. Proc. R. Soc. B Biol. Sci. 282:20150837. doi: 10.1098/rspb.2015.0837
Li, S. R., Hao, X., Wang, Y., Sun, B. J., Bi, J. H., Zhang, Y. P., et al. (2018). Female lizards choose warm, moist nests that improve embryonic survivorship and offspring fitness. Funct. Ecol. 32, 416–423. doi: 10.1111/1365-2435.12995
Li, X. H., Wu, P. F., Ma, L., Huebner, C., Sun, B. J., and Li, S. R. (2020). Embryonic and post-embryonic responses to high-elevation hypoxia in a low-elevation lizard. Integr. Zool. 15, 338–348. doi: 10.1111/1749-4877.12441
Litvak, M. K., and Leggett, W. C. (1992). Age and size-selective predation on larval fishes: the bigger is better hypothesis revisited. Mar. Ecol. Prog. Ser. 81, 13–24. doi: 10.3354/meps081013
Liu, W. L., Liu, P., Cui, L. X., Meng, Y., Tao, S. A., Han, X. Z., et al. (2022). Moderate climate warming scenarios during embryonic and post-embryonic stages benefit a cold-climate lizard. Funct. Ecol. [Epub ahea of print]. doi: 10.1111/1365-2435.14032
Ljungström, G., Wapstra, E., and Olsson, M. (2015). Sand lizard (Lacerta agilis) phenology in a warming world. BMC Evol. Biol. 15:206. doi: 10.1186/s12862-015-0476-0
Logan, M. L., Huynh, R. K., Precious, R. A., and Calsbeek, R. G. (2013). The impact of climate change measured at relevant spatial scales: new hope for tropical lizards. Glob. Change Biol. 19, 3093–3102. doi: 10.1111/gcb.12253
Lu, D. L., Ma, Q., Wang, J., Li, L. Y., Han, S. L., Limbu, S. M., et al. (2019). Fasting enhances cold resistance in fish through stimulating lipid catabolism and autophagy. J. Physiol. 597, 1585–1603. doi: 10.1113/JP277091
Ma, L., Sun, B. J., Li, S. R., Hao, X., Bi, J. H., and Du, W. G. (2018b). The vulnerability of developing embryos to simulated climate warming differs between sympatric desert lizards. J. Exp. Zool. A 329, 252–261. doi: 10.1002/jez.2179
Ma, L., Sun, B. J., Cao, P., Li, X. H., and Du, W. G. (2018a). Phenotypic plasticity may help lizards cope with increasingly variable temperatures. Oecologia 187, 37–45. doi: 10.1007/s00442-018-4127-1
Madsen, T., Olsson, M., Wittzell, H., Stille, B., Gullberg, A., Shine, R., et al. (2000). Population size and genetic diversity in sand lizards (Lacerta agilis) and adders (Vipera berus). Biol. Conserv. 94, 257–262. doi: 10.1016/s0006-3207(99)00127-5
Massot, M., Clobert, J., and FerriÈre, R. (2008). Climate warming, dispersal inhibition and extinction risk. Glob. Change Biol. 14, 461–469. doi: 10.1111/j.1365-2486.2007.01514.x
Meylan, S., Miles, D. B., and Clobert, J. (2012). Hormonally mediated maternal effects, individual strategy and global change. Philos. Trans. R. Soc. B 367, 1647–1664. doi: 10.1098/rstb.2012.0020
Noble, D. W. A., Stenhouse, V., Riley, J. L., Warner, D. A., While, G. M., Du, W. G., et al. (2018a). A comprehensive database of thermal developmental plasticity in reptiles. Sci. Data 5:180138. doi: 10.1038/sdata.2018.138
Noble, D. W. A., Stenhouse, V., and Schwanz, L. E. (2018b). Developmental temperatures and phenotypic plasticity in reptiles: a systematic review and meta-analysis. Biol. Rev. 93, 72–97. doi: 10.1111/brv.12333
Olsson, M. (1993). Male preference for large females and assortative mating for body size in the sand lizard (Lacerta agilis). Behav. Ecol. Sociobiol. 32, 337–341.
Olsson, M. (1994a). Nuptial coloration in the sand lizard, Lacerta agilis: an intra-sexually selected cue to fighting ability. Anim. Behav. 48, 607–613. doi: 10.1006/anbe.1994.1280
Olsson, M. (1994b). Sperm competition in the sand lizard, Lacerta agilis. Anim. Behav. 48, 193–200. doi: 10.1006/anbe.1994.1226
Olsson, M., Schwartz, T., Wapstra, E., Uller, T., Ujvari, B., Madsen, T., et al. (2011). Climate change, multiple paternity and offspring survival in lizards. Evolution 65, 3323–3326. doi: 10.1111/j.1558-5646.2011.01387.x
Olsson, M., and Shine, R. (1996). Does reproductive success increase with age or with size in species with indeterminate growth? A case study using sand lizards (Lacerta agilis). Oecologia 105, 175–178. doi: 10.1007/bf00328543
Olsson, M., and Shine, R. (1997b). The seasonal timing of oviposition in sand lizards (Lacerta agilis): Why early clutches are better. J. Evol. Biol. 10, 369–381. doi: 10.1046/j.1420-9101.1997.10030369.x
Olsson, M., and Shine, R. (1997a). The limits to reproductive output: offspring size versus number in the sand lizard (Lacerta agilis). Am. Nat. 149, 179–188. doi: 10.1086/285985
Pacifici, M., Foden, W. B., Visconti, P., Watson, J. E. M., Butchart, S. H. M., Kovacs, K. M., et al. (2015). Assessing species vulnerability to climate change. Nat. Clim. Change 5, 215–225.
Parmesan, C. (2006). Ecological and evolutionary responses to recent climate change. Annu. Rev. Ecol. Evol. Syst. 37, 637–669. doi: 10.1146/annurev.ecolsys.37.091305.110100
Pike, D. A. (2014). Forecasting the viability of sea turtle eggs in a warming world. Glob. Change Biol. 20, 7–15. doi: 10.1111/gcb.12397
Qualls, C. P., and Andrews, R. M. (1999). Cold climates and the evolution of viviparity in reptiles: cold incubation temperatures produce poor-quality offspring in the lizard, Sceloporus virgatus. Biol. J. Linn. Soc. 67, 353–376. doi: 10.1111/j.1095-8312.1999.tb01939.x
Refsnider, J., and Janzen, F. (2010a). Can nest-site choice compensate for the effects of climate change on reptiles with temperature-dependent sex determination? Integr. Comp. Biol. 50:E145.
Refsnider, J. M., and Janzen, F. J. (2010b). Putting eggs in one basket: ecological and evolutionary hypotheses for variation in oviposition-site choice. Annu. Rev. Ecol. Evol. Syst. 41, 39–57. doi: 10.1146/annurev-ecolsys-102209-144712
Root, T. L., Price, J. T., Hall, K. R., Schneider, S. H., Rosenzweig, C., and Pounds, J. A. (2003). Fingerprints of global warming on wild animals and plants. Nature 421, 57–60. doi: 10.1038/nature01333
Sherry, R. A., Zhou, X., Gu, S., Arnone, J. A., Schimel, D. S., Verburg, P. S., et al. (2007). Divergence of reproductive phenology under climate warming. Proc. Natl. Acad. Sci. U.S.A. 104, 198–202. doi: 10.1073/pnas.0605642104
Sinervo, B., Mendez-De-La-Cruz, F., Miles, D. B., Heulin, B., Bastiaans, E., Cruz, M. V. S., et al. (2010). Erosion of lizard diversity by climate change and altered thermal niches. Science 328, 894–899. doi: 10.1126/science.1184695
Spear, L., and Nur, N. (1994). Brood Size, Hatching order and hatching date: effects on four life-history stages from hatching to recruitment in western gulls. J. Anim. Ecol. 63, 283–298. doi: 10.2307/5547
Steiger, S. (2013). Bigger mothers are better mothers: disentangling size-related prenatal and postnatal maternal effects. Proc. R. Soc. B Biol. Sci. 280:20131225. doi: 10.1098/rspb.2013.1225
Strijbosch, H., and Creemers, R. C. M. (1988). Comparative demography of sympatric populations of lacerta-vivipara and lacerta-agilis. Oecologia 76, 20–26. doi: 10.1007/BF00379595
Sun, B. J., Li, S. R., Xu, X. F., Zhao, W. G., Luo, L. G., Ji, X., et al. (2013). Different mechanisms lead to convergence of reproductive strategies in two lacertid lizards (Takydromus wolteri and Eremias argus). Oecologia 172, 645–652. doi: 10.1007/s00442-012-2524-4
Sun, B. J., Li, T., Gao, J., Ma, L., and Du, W. G. (2015). High incubation temperatures enhance mitochondrial energy metabolism in reptile embryos. Sci. Rep. 5:8861. doi: 10.1038/srep08861
Sun, B. J., Ma, L., Li, S. R., Williams, C. M., Wang, Y., Hao, X., et al. (2018a). Phenology and the physiological niche are co-adapted in a desert-dwelling lizard. Funct. Ecol. 32, 2520–2530. doi: 10.1111/1365-2435.13201
Sun, B. J., Wang, Y., Wang, Y., Lu, H. L., and Du, W. G. (2018b). Anticipatory parental effects in a subtropical lizard in response to experimental warming. Front. Zool. 15:51. doi: 10.1186/s12983-018-0296-3
Sun, B. J., Ma, L., Wang, Y., Mi, C. R., Buckley, L. B., Levy, O., et al. (2021). Latitudinal embryonic thermal tolerance and plasticity shape the vulnerability of oviparous species to climate change. Ecol. Monogr. 91:e01468.
Sun, B. J., Wang, T. T., Pike, D. A., Liang, L., and Du, W. G. (2014). Embryonic oxygen enhances learning ability in hatchling lizards. Front. Zool. 11:21. doi: 10.1186/1742-9994-11-21
Sunday, J. M., Bates, A. E., and Dulvy, N. K. (2011). Global analysis of thermal tolerance and latitude in ectotherms. Proc. R. Soc. B Biol. Sci. 278, 1823–1830. doi: 10.1098/rspb.2010.1295
Tang, K. W., Dam, H. G., and Feinberg, L. R. (1998). The relative importance of egg production rate, hatching success, hatching duration and egg sinking in population recruitment of two species of marine copepods. J. Plankton Res. 20, 1971–1987. doi: 10.1093/plankt/20.10.1971
Tao, S. A., Cheng, K. M., Li, X. H., Han, X. Z., Wang, J. C., Zheng, R. Q., et al. (2021). The thermal biology of Takydromus kuehnei indicates tropical lizards from high elevation have not been severely threatened by climate change. Front. Ecol. Evol. 9:767102. doi: 10.3389/fevo.2021.767102
Taylor, E. N., Diele-Viegas, L. M., Gangloff, E. J., Hall, J. M., Halpern, B., Massey, M. D., et al. (2021). The thermal ecology and physiology of reptiles and amphibians: a user’s guide. J. Exp. Zool. A 335, 13–44. doi: 10.1002/jez.2396
Thomas, C. D., Franco, A. M. A., and Hill, J. K. (2006). Range retractions and extinction in the face of climate warming. Trends Ecol. Evol. 21, 415–416. doi: 10.1016/j.tree.2006.05.012
Torralvo, K., Botero-Arias, R., and Magnusson, W. E. (2017). Temporal variation in black-caiman-nest predation in varzea of central Brazilian amazonia. PLoS One 12:e0183476. doi: 10.1371/journal.pone.0183476
Trakimas, G. (2005). Geographic distribution and status of sand lizard (Lacerta agilis) and common lizard (Lacerta (Zootoca) vivipara) in Lithuania. Acta Zool. Litu. 15, 372–375. doi: 10.1080/13921657.2005.10512703
Williams, S. E., Shoo, L. P., Isaac, J. L., Hoffmann, A. A., and Langham, G. (2008). Towards an integrated framework for assessing the vulnerability of species to climate change. PLoS Biol. 6, 2621–2626. doi: 10.1371/journal.pbio.0060325
Keywords: climate warming, embryonic development, hatchling development, temperature, high latitude, lizard, Lacerta agilis
Citation: Cui L, Yang C, Zhang D, Lin S, Zhao W and Liu P (2022) Beneficial Effects of Warming Temperatures on Embryonic and Hatchling Development in a Low-Latitude Margin Population of the High-Latitude Lizard Lacerta agilis. Front. Ecol. Evol. 10:845309. doi: 10.3389/fevo.2022.845309
Received: 29 December 2021; Accepted: 03 March 2022;
Published: 13 April 2022.
Edited by:
Lin Zhang, Hubei University of Chinese Medicine, ChinaCopyright © 2022 Cui, Yang, Zhang, Lin, Zhao and Liu. This is an open-access article distributed under the terms of the Creative Commons Attribution License (CC BY). The use, distribution or reproduction in other forums is permitted, provided the original author(s) and the copyright owner(s) are credited and that the original publication in this journal is cited, in accordance with accepted academic practice. No use, distribution or reproduction is permitted which does not comply with these terms.
*Correspondence: Wenge Zhao, zhaowenge311@126.com; Peng Liu, liupeng111111@163.com