- 1Institut de Recherche sur la Biologie de l’Insecte (UMR 7261), CNRS – University of Tours, Tours, France
- 2Department of Entomology, Texas A&M University, College Station, TX, United States
Social life is usually associated with enhanced propagule pressure, which increases the chance of introducing several individuals during a single introduction event. Social insects are therefore among the most successful invasive species, benefiting from rapid establishment and increased foundation success in new habitats. In termites, propagule pressure may also be increased by the development of reproductive individuals from a small group of foraging workers. This suggests that enhanced exploration activity may increase propagule pressure through an elevated chance of transporting isolated groups of foragers. Here, we analyzed the exploration behavior of three termite species of the Reticulitermes genus, comparing the invasive species Reticulitermes flavipes (testing both native and introduced populations) to the native species Reticulitermes grassei and Reticulitermes lucifugus. Different features representative of the exploration capacity were measured during 48 h, including: the number of tunnels, the length of tunnels, the number of foragers, and the interindividual distance of foragers in a straight line or through tunnels. Our results show that compared to the native Reticulitermes species, R. flavipes foragers from both populations dug more tunnels with a longer total length, and individuals were more spatially dispersed and covered a larger exploration zone. These findings suggest that the enhanced exploration ability of R. flavipes may have played a role in its invasion success, by increasing its propagule pressure through a higher chance of human-mediated transport. In addition, the absence of differences between the native and introduced populations of R. flavipes suggests that the exploration behaviors facilitating the worldwide invasion of this species originated in its native range.
Introduction
Biological invasions are a serious global environmental threat (Walther et al., 2009) and have economic repercussions due to their impairment of ecosystem services and destruction of human infrastructure (Scanes, 2018). The spread of an invasive species occurs in three stages: introduction of propagules (i.e., small group of individuals), establishment, and proliferation (Allendorf and Lundquist, 2003). An increased propagule pressure, which corresponds to larger propagule sizes (number of individuals involved in an invasion event) and higher propagule numbers (number of release events), impacts these three stages and is therefore a key element of species invasiveness (Britton and Gozlan, 2013). Specific life history traits (longevity, fecundity, or number of generations) can increase invasiveness by not only accelerating the development of introduced colonies (Lockwood et al., 2005; Fahrner and Aukema, 2018), but also by enhancing the success of dispersal events.
Social insects are among the most successful species at invading new habitats, with 57% of invasive insects being social (Bertelsmeier, 2021). The invasive success of Hymenoptera (ants, bees, and wasps) and Isoptera (termites) is primarily attributed to their social life, whereby the cooperation of many individuals may buffer stressful environmental conditions in their novel environment(s). Furthermore, social life may increase propagule pressure by increasing the probability of introducing several individuals during a single introduction event (Duncan et al., 2014). In addition to social life, invaders can also exhibit specific life history traits that increase their propagule pressure and thus enhance their invasiveness (Chapman and Bourke, 2001; Hanna et al., 2013; Evans, 2021; Eyer and Vargo, 2021). For example, the presence of numerous queens per colony (i.e., polygyny) and colony foundation by fission increase propagule pressure by increasing the foundation success of new colonies (Yang et al., 2012), which results in lower dispersal costs (Rust and Su, 2012; Hanna et al., 2013). In subterranean termites, several biological factors are known to promote invasiveness. First, these termite species nest in wood or soil, which are globally traded materials. This feature increases the number of propagules dispersed, as it favors their chance of being introduced through unintended transport. The invasive success of many termite species is also enhanced by the development of neotenic reproductives from nymphs or workers, which can transform a small group of workers into a viable propagule (Eyer and Vargo, 2021). Both of these features increase their propagule pressure, as every transported wood/soil material containing a colony fragment may represent an invasive threat (Evans et al., 2013). The development of numerous reproductives in mature colonies also augments the reproductive capacity of the colony (Perdereau et al., 2015). In subterranean termites, colonies are often composed of multiple satellite nests and feeding sites connected by underground tunnels. The large number of workers per colony increases their capacity to exploit food through tunneling [sometimes over 100 m (Dronnet et al., 2005)]. In addition to representing a significant threat to human infrastructure (Patel et al., 2020; Shults et al., 2021), high foraging activity in hidden environments may enhance propagule pressure through an elevated chance of accidentally transporting isolated groups of workers. Notably, the ability of an invasive species to associate itself with human activity and survive transport may also favor the successful establishment of its propagules (Su, 2013). Moreover, higher exploratory behavior may facilitate discovery of favorable environment allowing to increase survival success. Interestingly, a variation in foraging strategy is often present between different species of the same genus (Mizumoto et al., 2020), sometimes even between colonies of the same species (Mizumoto and Matsuura, 2013). These variations in foraging strategy may therefore differentially influence invasion success. For example, in drywood termites of the Cryptotermes genus, invasion proficiency is associated with the construction of longer tunnels and a foraging preference for small pieces of wood, which increases human-assisted dispersion (note that the one-piece genus Cryptotermes could not be directly compared to the multiple-piece genus Reticulitermes). Understanding the mechanisms driving the exploration behaviors of different termite species is therefore essential to better control their spread and reduce the associated economic costs.
Among subterranean termites, the genus Reticulitermes is one of the most costly pests, inflicting heavy damage upon wooden structures worldwide (Vargo and Husseneder, 2009; Evans et al., 2013; Buczkowski and Bertelsmeier, 2017). Reticulitermes flavipes (Kollar, 1837) is well established in France, after it was introduced from the eastern United States during the 18th century with the expansion of trade shipment (Vargo and Husseneder, 2009; Evans et al., 2013; Buczkowski and Bertelsmeier, 2017). Interestingly, differences in colony structure have been observed between native and introduced populations of this species. In the native populations, most colonies are headed by a couple of primary reproductives, whereas introduced colonies are several orders of magnitude larger and composed of hundreds of neotenic reproductives (Vargo and Husseneder, 2009; Baudouin et al., 2017). In its introduced range, R. flavipes is present in several urban areas, as well as in pine forests along the Atlantic coast. Concerning Reticulitermes grassei (Clément, 1978) and Reticulitermes lucifugus (Rossi, 1792), they are both considered as native in Europe. Interestingly, most R. flavipes populations occur in sympatry with R. grassei (Baudouin et al., 2018; Perdereau et al., 2019). In these populations, R. flavipes is dominant and outcompetes R. grassei, particularly in urban areas (Perdereau et al., 2011), which could also enhance dispersion by increasing chance of human mediated transport. Overall, the differences in ecological dominance between the different species, together with drastic changes in colony structure and colony size between the native and invasive ranges of R. flavipes, suggest that these species and populations potentially exhibit strong divergences in their exploration behaviors after propagule introductions.
In this study, we aimed at determining whether invasiveness is influenced by a shift in exploration behavior in the Reticulitermes genus after introduction of a propagule in a new environment. Using three species of this genus (R. flavipes, R. grassei, and R. lucifugus), we determined whether small groups of workers display differences in their exploration behaviors. These small groups were composed of thirty workers – the initial number of individuals required to form a viable propagule (Pichon et al., 2007). Due to the previously observed variations between species within this genus, we hypothesized that different species of Reticulitermes exhibit differences in their exploration behaviors. For the invasive species R. flavipes, we also compared these behaviors between native and introduced populations. We hypothesized that the changes in social organization observed between native and invasive populations may underlie differences in exploration behaviors between the two populations. Finally, we predicted that the two populations of R. flavipes possess higher exploration efficiency compared to the two native species (R. grassei and R. lucifugus), whereby high foraging activity potentially drives invasive success via enhanced propagule pressure.
Materials and Methods
Study Species and Laboratory Conditions
Fifty-three colonies of three different species of the Reticulitermes genus were collected in the field. Two populations of R. flavipes were collected in 2019, including sixteen colonies from Oléron (Charente-Maritime, France) for the invasive French population (called R. flavipes FR), and thirteen colonies from Lake Bryan (TX, United States) for the native American population (called R. flavipes US). Fourteen colonies of R. grassei were collected in 2019 from Oléron (Charente-Maritime, France), and ten colonies of R. lucifugus were collected in 2020 from Sainte-Maxime (Var, France). For each species, all colonies were sampled at least 300 m apart to ensure that distinct colonies were collected (Perdereau et al., 2010). Colonies were maintained under standard lab conditions (26 ± 1°C and >95% RH) within black individual plastic boxes (Starpack) containing ultrapure paper (47 mm diameters; Whatman, grade 42 Ashless), moistened sand, and pine wood sawdust (Lucas et al., 2018).
Experimental Design
Behavioral observations were performed using a glass sheet design (Brossette et al., 2017). A hole (15 mm diameter) was drilled in the center of one of the two glass sheets to allow the introduction of termites into the exploration area. A diamond drill bit was used to drill the hole in the center to prevent the glass from cracking. The two glass sheets (220 mm × 220 mm) were separated by two spacers (100 mm × 10 mm × 1.4 mm) located on each side, to obtain a 200 mm × 200 mm exploration area. Sand was introduced between the two glass sheets and moistened with Milli-Q water 24 h before the introduction of individuals, and no food was added. One hour before observations, 30 workers were randomly selected per colony and placed in plastic boxes (50 mm diameter; Starpack Cat#04913) containing moistened pure cellulose paper (47 mm diameter; Whatman, GE Healthcare). Individuals were sorted under CO2 then placed in a 1.5 ml tube to facilitate their introduction into the arena. The glass hole was plugged with plexiglass and covered with a glass blade to prevent escape. Measurements were carried out for 48 h using cameras (Basler acA1300 – 60gc) driven by the software Labview (v16.0). Cameras were fixed to a rail above the arenas. Pictures of the arena were taken at three observation times: 6, 24, and 48 h. For each species and population, five factors were investigated: the number of tunnels (NT), the total length of the tunnels, the number of foragers (NF) (individuals dispersed away from the introduction area), and the shortest distances between individuals (distance in a straight line and distance through tunnels). Data were recorded and analyzed blindly regarding the treatments (Penn and Frommen, 2010).
Spatial Data Analyses
The cartography of tunnels was analyzed using QGIS (v3.10.2). Pictures of the two-glass systems for each time were implemented as raster files. The RGF93/Lambert93 EPSG:2154 coordinate system was derived to scale the pictures (Bech et al., 2017). The spatial distribution was analyzed, using a point shape (on the neck) to spot each forager (individual dispersed away from the introduction area), as well as to calculate the shortest distance between foragers. Tunnels were counted and drawn to obtain an overall tunnel layout, thereby allowing the total length of the tunnels to be summed. Distances between foragers through tunnels were calculated using the previous tunnel layouts. Note that termites could block unused tunnels; however, in our analyses, such tunnels were not counted anymore. Therefore, the total length of the tunnels could sometimes decrease over time.
Statistical Analyses
The number and the length of tunnels, the interindividual distances (in a straight line and through tunnels) and the number of foragers were analyzed using linear mixed models (LMM). To fit with a normal distribution of residuals and homoscedasticity, all variables were log (+1)-transformed except the number of foragers. Two-way ANOVAs were performed for each variable and the explanatory factors were the observation time and the species. Colony IDs and observation times were used as random factors. The models were first tested with interactions between variables, which were removed when not significant (p > 0.05). To analyze significance across the different species for each observation time (if applicable) we performed Tukey’s HSD all-pairwise comparisons tests.
Edge effects of the arena were tested by comparing colonies with tunnels hitting the edges to colonies without tunnels hitting the edges. Edge effects were calculated for the five variables and for each species/population. We performed either a Student’s t-test or Wilcoxon test depending on normality of the data. Data which did not fit a normal distribution were the NT for R. flavipes FR and R. lucifugus, as well as the NF for R. lucifugus.
At the end of the experiments, termites were extracted to count the total number of alive individuals. No mortality effect was found (all p > 0.20); with a mortality range between 0 and 5 individuals per colony (1.2 deads on average). Over the 53 tested colonies, 19 colonies showed no mortality and only 2 colonies showed 5 deads.
To infer the relationships between the five variables, we conducted a Principal Component Analysis (PCA) for each observation time. These analyses provided five orthogonal principal components (PCs), out of which we retained the first two PCs (total variances explained >83.9; Table 1). We extracted loadings of each variable on the two PCs (Table 1) to analyze its contribution to the overall PCA, and therefore to determine its influence in driving the difference between species. We applied Mardia’s principle, which states that a PC is loaded by a variable when the value of the loading is higher than 0.8 (Mardia et al., 1979). We then extracted projection values of colonies on the two PCs of the PCA. These values were used as explanatory variables in three linear models (one for each observation time), in which the species was entered as fixed factor. We conducted one-way ANOVAs to compare PC values across species (one for each PC). When applicable, pairwise comparisons between species were tested using Tukey’s post-hoc tests.
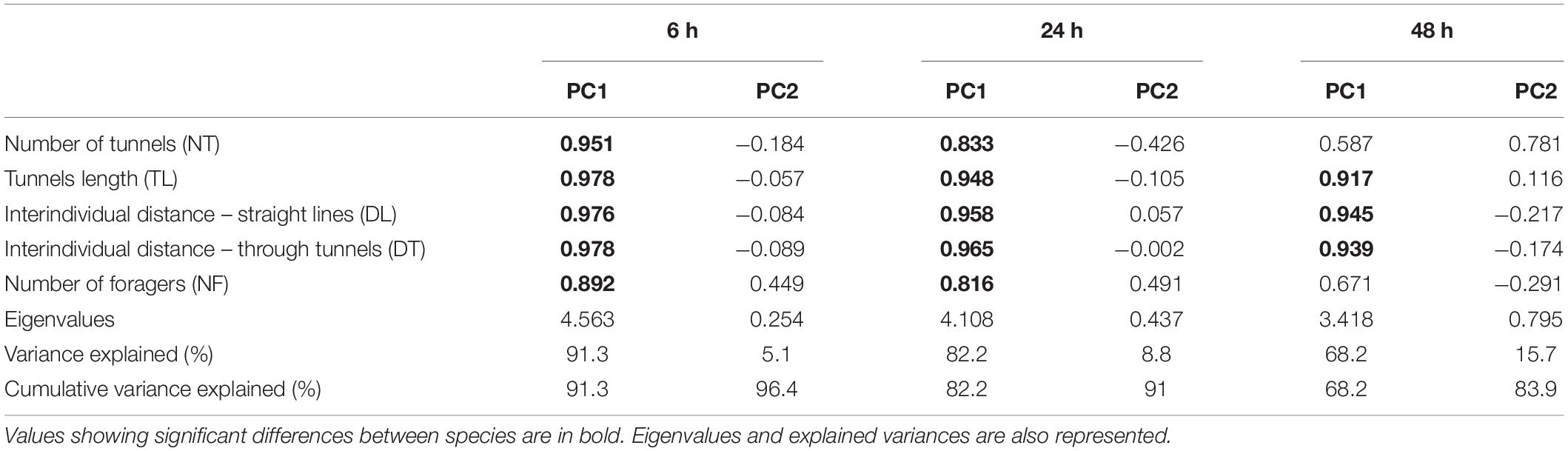
Table 1. Loadings of each variable on the two principal components (PC1 and PC2) for each observation time (6, 24, and 48 h).
All analyses and graphs were performed using the lme4 (Bates et al., 2015), car (Fox et al., 2013), emmeans (Lenth, 2020), FactoMineR (Lê et al., 2008), sciplot (Morales, 2017), and factoextra (Kassambara and Mundt, 2020) packages in R v3.6.1.1
Results
The five variables showed the same pattern (Figure 1). The number of tunnels (ANOVA, F6,53 = 25.49, p < 0.001) and the total length of the tunnels (ANOVA, F6,53 = 70.05, p < 0.0001), the interindividual distances in a straight line (ANOVA, F6,53 = 117.43, p < 0.0001) and through tunnels (ANOVA, F6,53 = 45.26, p < 0.0001), and the number of foragers (ANOVA, F6,53 = 28.05, p < 0.0001) were dependent on the interaction between the species and the observation time. No difference between species/populations were observed at 48 h, regardless of the variable considered (Tukey’s multiple comparisons test, all p > 0.0794).
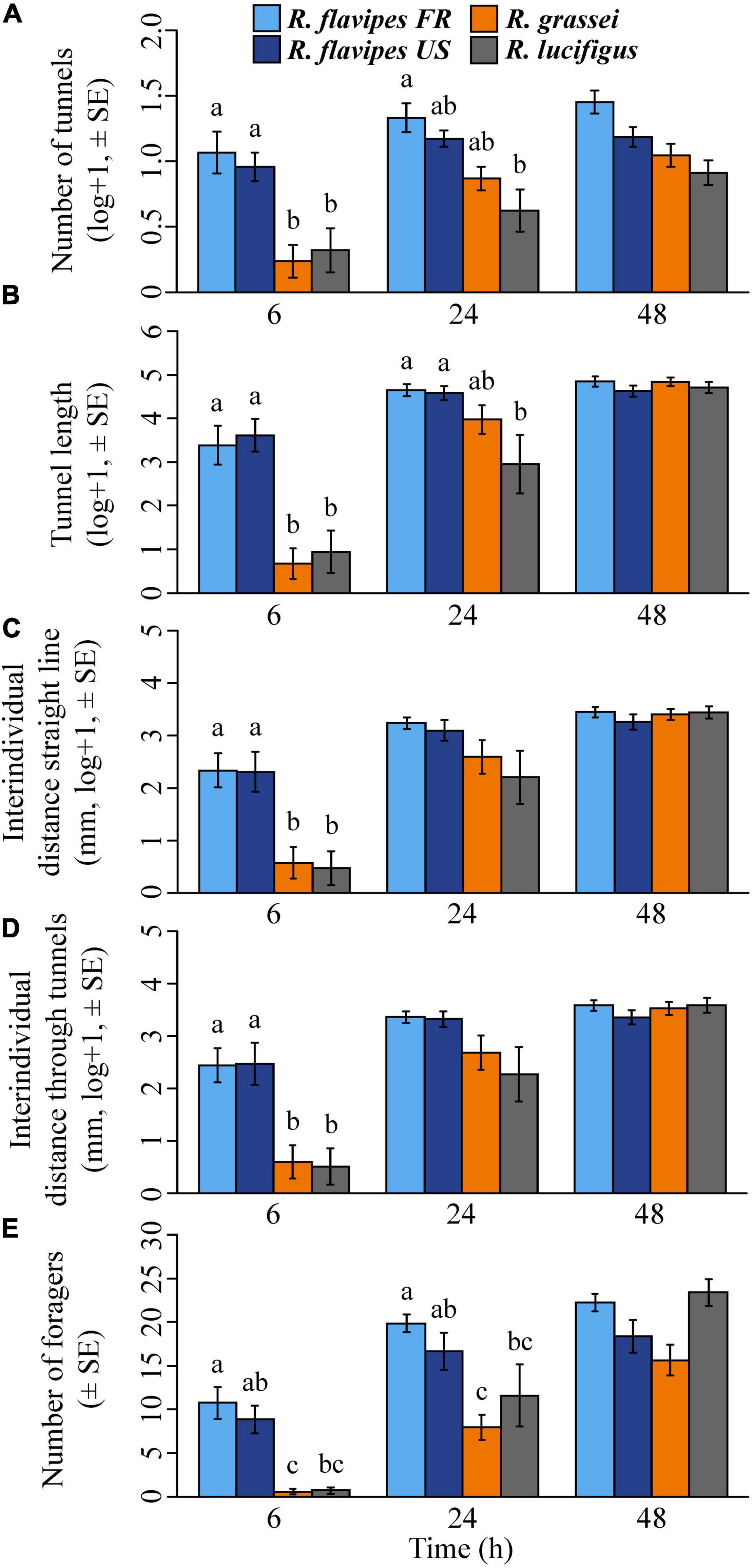
Figure 1. The five variables measured at 6, 24, and 48 h. Number of tunnels (A), total length of the tunnels (B), distance between individuals in a straight line (C), distance between individuals through tunnels (D), and number of foragers (individuals dispersed away from the introduction area) (E). Significant differences between species are indicated by different letters (p < 0.05).
The number of tunnels at 6 h was higher in the two populations of R. flavipes than in R. grassei and R. lucifugus (Tukey’s multiple comparisons test, all p < 0.024; Figure 1A). But at 24 h, R. flavipes FR constructed more tunnels than only R. lucifugus (Tukey’s multiple comparisons test, p = 0.004). The total length of the tunnels at 6 h for R. flavipes FR and R. flavipes US was higher than R. grassei and R. lucifugus (Tukey’s multiple comparisons test, all p < 0.0001; Figure 1B). But at 24 h, tunnels of R. flavipes FR and R. flavipes US were longer than only R. lucifugus (Tukey’s multiple comparisons test, all p < 0.042). Both interindividual distances were higher for R. flavipes FR and R. flavipes US compared to R. grassei and R. lucifugus, 6 h after introduction (in a straight line, Tukey’s multiple comparisons test, all p < 0.0001; Figure 1C and through tunnels, Tukey’s multiple comparisons test, all p < 0.01; Figure 1D). However, no significant differences were observed between species/populations after 24 or 48 h. At 6 and 24 h (Figure 1E), the number of foragers was higher for R. flavipes FR compared to R. grassei (Tukey’s multiple comparisons test, both p < 0.001) and R. lucifugus (Tukey’s multiple comparisons test, both p < 0.038). The number of foragers for R. flavipes US was significantly higher compared to R. grassei (Tukey’s multiple comparisons test, both p < 0.023), but not compared to R. lucifugus (Tukey’s multiple comparisons test, both p > 0.067) at 6 or 24 h. No edge effect was found between species for the five variables (all p > 0.067). Nevertheless, it must be noted that colonies were rarely observed with tunnels hitting edges, as only four colonies of R. flavipes FR, one colony of R. flavipes US and three colonies of R. lucifugus made it to the edge (and none for R. grassei). Overall, these results revealed a strong difference in exploration strategies between the two populations of R. flavipes and the two other Reticulitermes species at 6 h, and that this difference subsequently faded over time (Figure 2). Additionally, no difference was ever observed between the native and invasive populations of R. flavipes, and no difference was observed between the two non-invasive species, R. grassei and R. lucifugus.
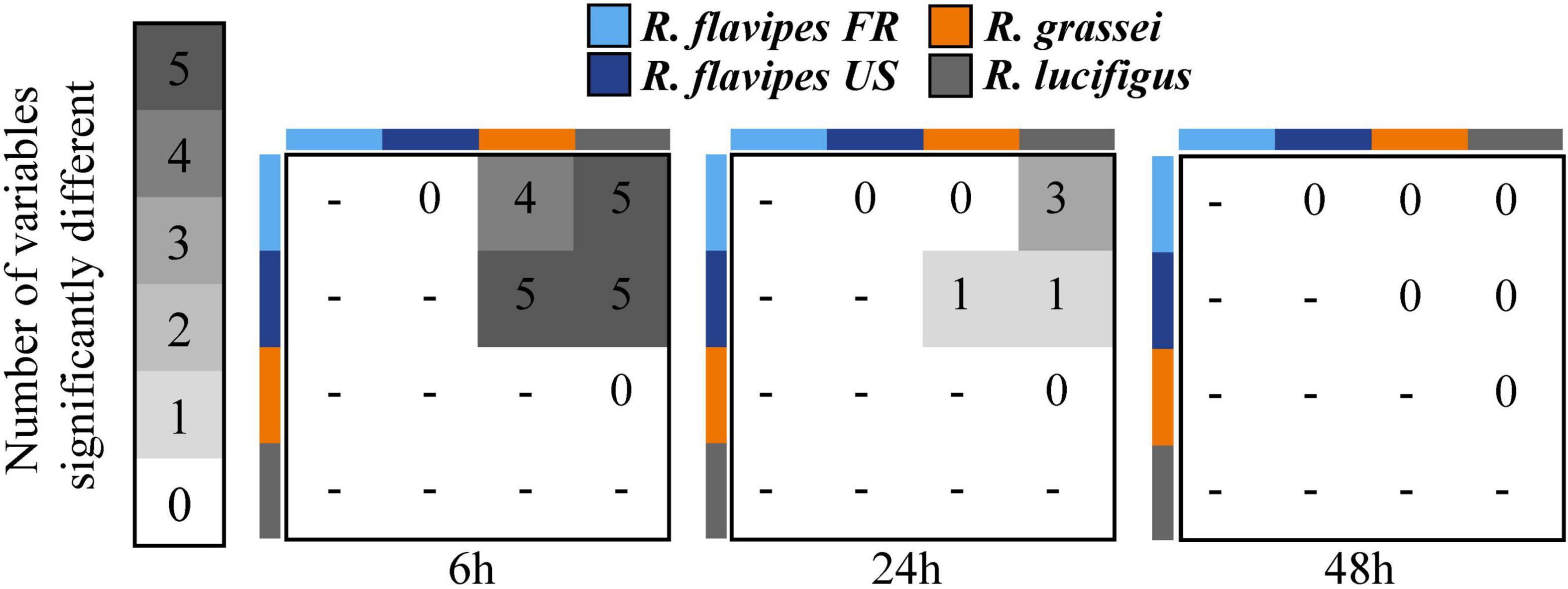
Figure 2. Summary of the differences between the three species, reflecting the variations in the five measured variables at 6, 24, and 48 h.
The differences in exploration behavior between species at 6 h were highlighted on the PCA, which segregated the two populations of R. flavipes from the two other species. This segregation mostly results from a difference between species on the first component (PC1) (Figure 3A; ANOVA, F3,53 = 12.8, p < 0.01), which explained 91.29% of the variation observed. Consistent with our results above, significant differences were observed between R. flavipes and the two non-invasive species, while no difference was observed between the native and invasive populations of R. flavipes, and no difference was observed between R. grassei and R. lucifugus. At 6 h, PC1 was positively loaded by all five variables (Table 1), which were all correlated together, thereby reflecting the importance of all variables in explaining the differences between species (Figure 3B and Table 1; all the loadings >0.816). However, no difference between species was observed at 6 h on PC2 (Figure 3A; ANOVA, F3,53 = 1.27, p = 0.29) and no variable was loaded on PC2; but it is important to note that this component only slightly explained the observed variance (5.09%).
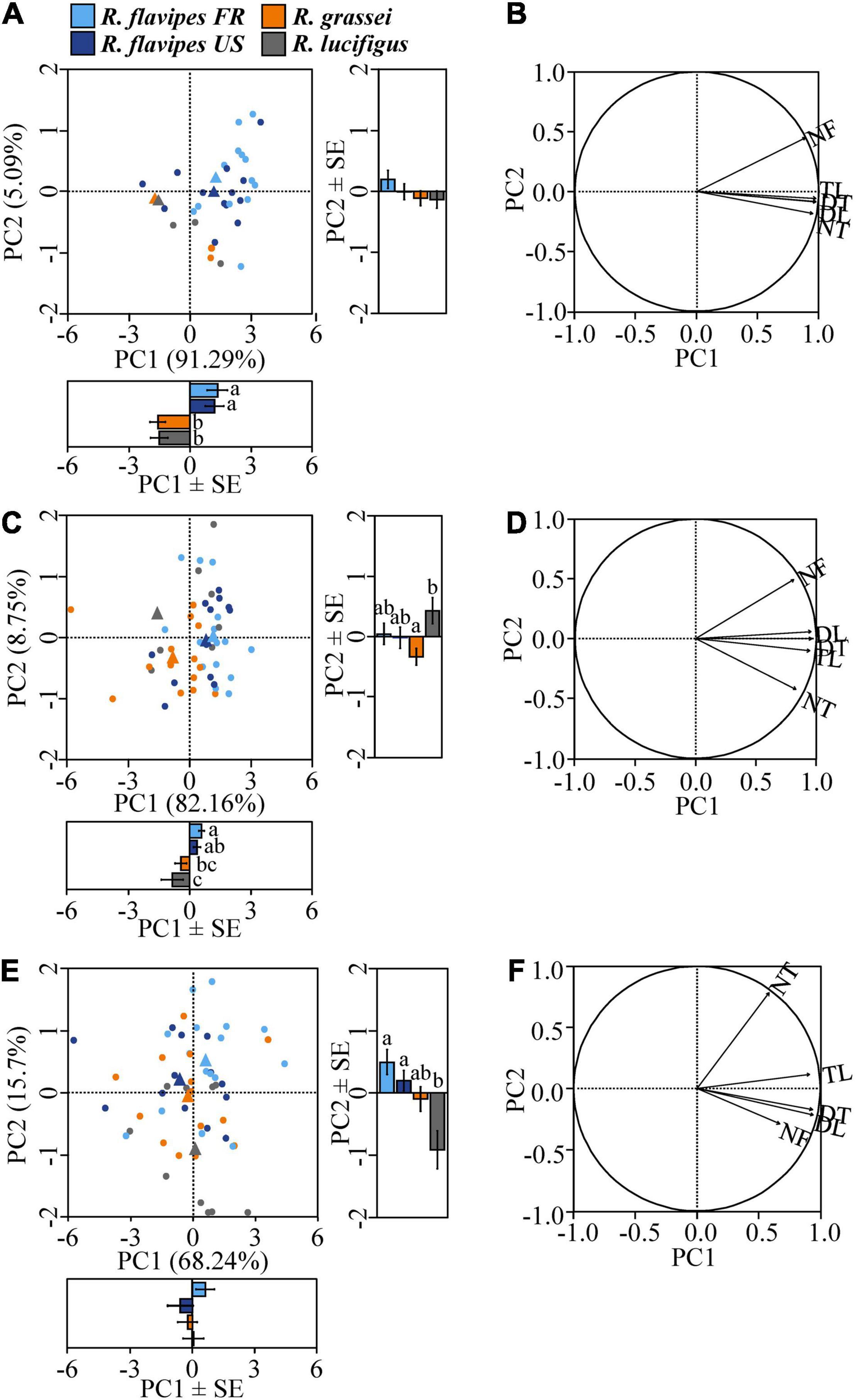
Figure 3. Principal component analysis (PCA) of the colonies of the different species/populations at each observation time. PCA of the five variables and the effect of species/populations on PC1 and PC2 at 6 h (A), 24 h (C), and 48 h (E). For each species/population, dots represent colonies and triangles represent centroids. Significant differences between species are indicated by different letters (p < 0.05). The two PC eigenvectors for the five variables at 6 h (B), 24 h (D), and 48 h (F). The x-axis represents principal component 1 (PC1) while the y-axis represents principal component 2 (PC2) of the PCAs. NF, number of foragers; TL, tunnel length; DT, distance through tunnels; DL, distance in a straight line; NT, number of tunnels. Note that colonies which did not start digging, present identical PCA coordinates. It results in hidden points in (A) (11, 7, 3, and 1 colonies for R. grassei, R. lucifugus, and R. flavipes FR and R. flavipes US, respectively) and in (C) (1 colony for R. grassei and 3 for R. lucifugus).
At 24 h, although the PCA only slightly segregated the different species studied, PC1 was able to discriminate the two populations of R. flavipes from R. lucifugus (Figure 3C, Tukey’s multiple comparisons test, both p < 0.03), as well as R. flavipes FR and R. grassei (Figure 3C; Tukey’s multiple comparisons test, p < 0.04). Similarly, PC1 was positively loaded by all five variables (Table 1). Interestingly, PC2 significantly segregated R. grassei and R. lucifugus. This difference primary stemmed from the number of tunnels and the number of foragers, as these variables were more loaded on PC2 (Figure 3D) than the three other variables, despite their loading values being lower than the threshold value of 0.8 (Table 1). At 24 h, R. grassei was characterized by a higher NT, while R. lucifugus was characterized by a higher NF.
At 48 h, the PCA was unable to discriminate the three species, as all colonies studied randomly segregated along each axis, regardless of their species or population of origin. Accordingly, there was no difference between species/populations at 48 h on PC1 (Figure 3E; ANOVA, F3,53 = 5.59, p = 0.35), which explained most of the variance observed (68.24%). At 48 h, only the tunnel length (TL) and the two interindividual distances (DL, DT) were associated with PC1 (Figure 3F and Table 1; all the loadings >0.917). Interestingly, PC2 at 48 h gave the same result as PC1 at 24 h, with it slightly segregating R. flavipes and the two non-invasive species, despite only the difference between R. flavipes FR and R. lucifugus being significant. Following Mardia’s principle (Mardia et al., 1979), PC2 at 48 h was only marginally loaded by the number of tunnels (Figure 3F; loadings = 0.781).
Discussion
The three species of Reticulitermes studied exhibited differences in their exploration behaviors for all five observed variables. Six hours after introduction, the two populations of R. flavipes (US and FR) showed a greater exploration ability compared to R. grassei and R. lucifugus. Foragers of R. flavipes were able to dig more tunnels with a longer total length, with individuals being more spatially dispersed and covering a larger exploration zone. Interestingly, the differences observed between species at 6 h almost disappear at 24 h, and are equal at 48 h, suggesting that R. flavipes more rapidly reaches adequate gallery size. These findings suggest that the enhanced exploration ability of R. flavipes may have played a role in its invasion success. The rapid construction of long tunnels may increase propagule pressure through a higher chance of human-mediated transport. Surprisingly, both the native and invasive population of R. flavipes have similar exploration capacities, suggesting that these exploration behaviors likely originated in its native range.
Both native and introduced populations of R. flavipes exhibit high exploration abilities for all five variables. Similar, yet different, tunneling patterns have been reported in invasive subterranean termites of the Coptotermes genus (Mizumoto et al., 2020). During experiments with food, Coptotermes formosanus constructs a low number of long tunnels, while Coptotermes gestroi constructs a high number of short tunnels (Grace et al., 2004; Lee et al., 2007). Longer tunnels are potentially associated with higher propagule pressure. This ability to colonize many pieces of wood also increases the chance of human-mediated transportation (Evans et al., 2011). Our data show that both populations of R. flavipes are characterized by a high number of long tunnels, 6 h after the introduction of foragers. These traits could represent advantages in terms of exploration capacities, food detection and foraging strategies, as described in termites and ants (Traniello, 1989; Hölldobler and Wilson, 1990; Traniello and Leuthold, 2000). Interestingly, the differences between species decrease over time and completely disappear at 48 h. The lack of differences between species at 48 h could be explained by the reduced size of the arena. Other experiments with similar two-dimension designs showed that the maximum tunneling distances are dependent on the size of the arena (Nobre et al., 2007; Li et al., 2010; Chouvenc et al., 2011), as well as the group size (Su and Lee, 2009). However, our findings reveal that R. flavipes reaches the maximum/adequate gallery size faster than the two other Reticulitermes species, confirming the superior exploration ability of this species. Additionally, in our experimental design, colonies rarely built tunnels that met the edge of the arena, even if we cannot exclude that they detected the edges. Indeed, termites are able to use vibrational cues to estimate the size of a piece of wood (Evans et al., 2005). Nevertheless, removing the few colonies that hit the edge did not affect the results for any of the five variables. Another factor influencing foraging patterns is the caste ratio, since the presence of soldiers increases the survival of workers by reducing the stress caused by competitors (Tian et al., 2017). It could be interesting to test the group size/composition effect on the exploration behaviors in these different species, with a suitable design for a larger number of individuals.
This higher short-term exploration capacity for R. flavipes FR compared to R. grassei and R. lucifugus may also reflect differences in their life history traits. Indeed, R. flavipes and R. grassei are sympatric in the sampling area of the current study (Oléron) where they display differences in their life history traits. Both species exhibit extended families (i.e., the presence of multiple neotenic reproductives), but they differ in traits like parental care (Brossette et al., 2019), colony foundation (Brossette et al., 2017) and aggressiveness (Perdereau et al., 2011; Duarte et al., 2018). Feeding at multiple sites is a common trait for R. flavipes, while less than half of the colonies of R. grassei exhibit this trait in the studied population (Deheer et al., 2005). Exploiting multiple feeding sites leads to an increase in distance between individuals, as observed in R. flavipes in this study. Moreover, in accordance with our findings, introduced populations of R. flavipes have been previously found to inhabit larger foraging areas (up to 90,000 m2) and to construct linear foraging tunnels (sometimes up to 320 m) compared to R. grassei (up to 70 m, respectively) (Deheer et al., 2005; Dronnet et al., 2005). Overall, R. flavipes exhibits a higher survival rate, a higher production of individuals, along with a higher total number of individuals during colony foundation compared to R. grassei (Brossette et al., 2017). Similar results have been observed in the eastern United States where R. flavipes and Reticulitermes virginicus are sympatric species. R. flavipes has higher foraging activities and is now considered as invasive in other United States locations, while R. virginicus remains endemic to eastern United States (Pitts-Singer and Forschler, 2000; Janowiecki and Vargo, 2021). As shown here, R. flavipes also possesses a higher short-term exploration rate compared to the two native Reticulitermes species. Altogether, these results may explain both the dominance of R. flavipes during interspecific competition and its invasive success. For R. lucifugus, no study has investigated its social structure or life history traits during colony foundation. Our study represents the first report on the exploration capacities of this understudied species despite its large geographical distribution in Europe (Kutnik et al., 2020). As shown in the results, differences between species are present at different time points after propagule introduction.
The native United States and the invasive French populations of R. flavipes exhibit similar exploration features, suggesting that the introduction of this species did not alter its foraging strategy. The establishment of an invasive species in a new environment is often facilitated by certain biological traits. Sometimes, such traits are already present in the native population and may represent a pre-disposition to invasion, as was described in plants for tetraploidy or biomass production (Henery et al., 2010; Van Kleunen et al., 2011). On the other hand, introduced populations sometimes exhibit post-introduction phenotypic changes in morphology, behavior, and/or life history traits. Finally, in some cases, successful invaders may simply occupy a vacant ecological niche within their novel environment(s), without exhibiting phenotypic changes when compared to native populations or greater competitive ability compared to native species. Specific traits favoring invasive success have also been described in social insects (Eyer and Vargo, 2021). For example, the perennial colony cycle and the low discrimination toward non-nestmates already present in the native ranges of Vespula wasps may have facilitated queen recruitment and the formation of highly polygyne nests in their invasive ranges (Kasper et al., 2008; Hanna et al., 2013). In the ant Brachyponera chinensis, the occurrence of highly inbred colonies in native populations may have acted as a pre-adaptive trait for invasiveness, mitigating the detrimental effect of inbreeding that introduced colonies commonly experience after a bottleneck event (Eyer et al., 2018). Here, the rapid and efficient exploration strategy pre-existing in the United States population of R. flavipes may have promoted its invasive success, by enabling the development of spatially expansive colonies spreading across multiple sites. In addition, it may favor invasiveness by increasing its ecological dominance and monopolization of resources. In its native range, R. flavipes exhibits a smaller foraging area (up to 800 m2) and linear foraging tunnels (sometimes up to 76 m) compared to the introduced population (Vargo and Husseneder, 2009). Finally, this feature may increase its propagule pressure, by increasing the likelihood of human-mediated transport of distinct colony fragments. Moreover, in the Reticulitermes genus, workers are able to differentiate into neotenic reproductives (Myles, 1999), meaning that every piece of transported wood or soil with workers can become an independent functional colony (Evans et al., 2013). Interestingly, a recent molecular analysis suggests that extensive human-mediated jump dispersal is common in both the native and introduced ranges of R. flavipes (Eyer et al., 2021), which is consistent with the high exploration abilities observed in both of its ranges here. However, the widespread admixture within and across native and introduced populations through repeated introductions and potential re-invasion of the native range from the French population results in a lack of differentiation between native and introduced ranges (Eyer et al., 2021). It could therefore explain why no differences in exploration strategy were observed between the two tested populations of R. flavipes. Future experiments on different populations of R. flavipes in the United States will enable the investigation of possible adaptative traits favoring exploration to different environments.
Overall, this study provides new knowledge to better understand the establishment of R. flavipes in France, as well as the exploration characteristics that favor its invasive success. R. flavipes is prevalent in urban areas, while the two other species are mostly present in non-anthropized environments (Perdereau et al., 2019). Anthropized environments are subject to drastic changes caused by human activities. The rapid exploration of surrounding areas could represent a key factor in the establishment of R. flavipes colonies, and therefore a major advantage for its invasive success in urban areas. Our results therefore emphasize the need for early detection to prevent damages and to control expansion of R. flavipes. R. flavipes exhibits enhanced colony foundation characteristics compared to R. grassei, such as a significantly higher survival rate of alates (adultoid reproductive) and a higher production of eggs, larvae and workers (Brossette et al., 2017). Overall, our study highlights the greater short-term exploration capacity of the invasive species, R. flavipes, compared to the two other Reticulitermes species studied. Together with its capacity for developing numerous reproductives, reduced intraspecific aggression, rapid colony foundation, and elevated interspecific competitive ability (Perdereau et al., 2011; Brossette et al., 2017, 2019), this enhanced exploration activity may promote its invasiveness. Greater exploration activity not only increases species dominance and facilitates the monopolization of resources, but also increases propagule pressure, which is an essential component of dispersal.
Data Availability Statement
The original contributions presented in the study are publicly available. This data can be found here: doi: 10.5281/zenodo.6023265.
Author Contributions
CL, LP, AG, and FR designed the experiments. CL, LP, and FR coordinated the data collection. LP and AM collected the data. LP and CL performed the data analysis. LP, CL, and P-AE wrote the manuscript with help of FR. All authors contributed to the article and approved the submitted version.
Funding
This work was supported by the European Union’s Horizon 2020 Research and Innovation Program (grant agreement 773324) and the Région Centre-Val de Loire (APR-IR 2017-00117111 BioControleTermite; APR-IA 2012).
Conflict of Interest
The authors declare that the research was conducted in the absence of any commercial or financial relationships that could be construed as a potential conflict of interest.
Publisher’s Note
All claims expressed in this article are solely those of the authors and do not necessarily represent those of their affiliated organizations, or those of the publisher, the editors and the reviewers. Any product that may be evaluated in this article, or claim that may be made by its manufacturer, is not guaranteed or endorsed by the publisher.
Acknowledgments
We are grateful to Miguel Pineirua for the video monitoring scripts and for his help to develop the recording design, and to Esteban Moyer for his help to install the video monitoring setup. We also thank Simon Dupont, Carole Labrousse, and Esteban Moyer for their help with field collection and rearing; and Alexander Blumenfeld for helpful comments on previous drafts of this manuscript.
Footnotes
References
Allendorf, F. W., and Lundquist, L. L. (2003). Introduction: population biology, evolution, and control of invasive species. Conserv. Biol. 17, 24–30. doi: 10.1046/j.1523-1739.2003.02365.x
Bates, D., Mächler, M., Bolker, B., and Walker, S. (2015). Fitting linear mixed-effects models using lme4. J. Stat. Softw. 67, 1–47.
Baudouin, G., Bech, N., Bagnères, A.-G., and Dedeine, F. (2018). Spatial and genetic distribution of a north American termite, Reticulitermes flavipes, across the landscape of Paris. Urban Ecosyst. 21, 751–764. doi: 10.1007/s11252-018-0747-9
Baudouin, G., Dedeine, F., Bech, N., Bankhead-Dronnet, S., Dupont, S., and Bagnères, A.-G. (2017). An american termite in Paris: temporal colony dynamics. Genetica 145, 491–502. doi: 10.1007/s10709-017-9991-9
Bech, N., Depeux, C., Durand, S., Debenest, C., Lafitte, A., and Beltran-Bech, S. (2017). When GIS zooms in: spatio-genetic maps of multipaternity in Armadillidium vulgare. Genetica 145, 503–512. doi: 10.1007/s10709-017-9992-8
Bertelsmeier, C. (2021). Globalization and the anthropogenic spread of invasive social insects. Curr. Opin. Insect Sci. 46, 16–23. doi: 10.1016/j.cois.2021.01.006
Britton, J. R., and Gozlan, R. E. (2013). How many founders for a biological invasion? Predicting introduction outcomes from propagule pressure. Ecology 94, 2558–2566. doi: 10.1890/13-0527.1
Brossette, L., Bagnères, A.-G., Millot, A., Blanchard, S., Dupont, S., and Lucas, C. (2017). Termite’s royal cradle: does colony foundation success differ between two subterranean species? Insectes Soc. 64, 515–523. doi: 10.1007/s00040-017-0571-x
Brossette, L., Meunier, J., Dupont, S., Bagnères, A.-G., and Lucas, C. (2019). Unbalanced biparental care during colony foundation in two subterranean termites. Ecol. Evol. 9, 192–200. doi: 10.1002/ece3.4710
Buczkowski, G., and Bertelsmeier, C. (2017). Invasive termites in a changing climate: a global perspective. Ecol. Evol. 7, 974–985. doi: 10.1002/ece3.2674
Chapman, R. E., and Bourke, A. F. G. (2001). The influence of sociality on the conservation biology of social insects. Ecol. Lett. 4, 650–662. doi: 10.1046/j.1461-0248.2001.00253.x
Chouvenc, T., Bardunias, P., Li, H.-F., Elliott, M. L., and Su, N.-Y. (2011). Planar arenas for use in laboratory bioassay studies of subterranean termites (Rhinotermitidae). Florida Entomol. 94, 817–826. doi: 10.1653/024.094.0413
Deheer, C. J., Kutnik, M., Vargo, E. L., and Bagnères, A.-G. (2005). The breeding system and population structure of the termite Reticulitermes grassei in Southwestern France. Heredity 95, 408–415. doi: 10.1038/sj.hdy.6800744
Dronnet, S., Chapuisat, M., Vargo, E. L., Bagnères, A.-G., and Lohou, C. (2005). Genetic analysis of the breeding system of an invasive subterranean termite. Reticulitermes santonensis, in urban and natural habitats. Mol. Ecol. 14, 1311–1320. doi: 10.1111/j.1365-294X.2005.02508.x
Duarte, S., Nobre, T., Borges, P. A. V., and Nunes, L. (2018). Symbiotic flagellate protists as cryptic drivers of adaptation and invasiveness of the subterranean termite Reticulitermes grassei Clément. Ecol. Evol. 8, 5242–5253. doi: 10.1002/ece3.3819
Duncan, R. P., Blackburn, T. M., Rossinelli, S., and Bacher, S. (2014). Quantifying invasion risk: the relationship between establishment probability and founding population size. Methods Ecol. Evol. 5, 1255–1263.
Evans, T. A. (2021). Predicting ecological impacts of invasive termites. Curr. Opin. Insect Sci. 46, 88–94. doi: 10.1016/j.cois.2021.03.003
Evans, T. A., Forschler, B. T., and Grace, J. K. (2013). Biology of invasive termites: a worldwide review. Annu. Rev. Entomol. 58, 455–474. doi: 10.1146/annurev-ento-120811-153554
Evans, T. A., Inta, R., and Lai, J. C. S. (2011). Foraging choice and replacement reproductives facilitate invasiveness in drywood termites. Biol. Invasions. 13, 1579–1587.
Evans, T. A., Lai, J. C. S., Toledano, E., McDowall, L., Rakotonarivo, S., and Lenz, M. (2005). Termites assess wood size by using vibration signals. Proc. Natl. Acad. Sci. U.S.A. 102, 3732–3737. doi: 10.1073/pnas.0408649102
Eyer, P., and Vargo, E. L. (2021). Breeding structure and invasiveness in social insects. Curr. Opin. Insect Sci. 46, 24–30. doi: 10.1016/j.cois.2021.01.004
Eyer, P., Blumenfeld, A. J., Johnson, L. N. L., Perdereau, E., Shults, P., Wang, S., et al. (2021). Extensive human-mediated jump dispersal within and across the native and introduced ranges of the invasive termite Reticulitermes flavipes. Mol. Ecol. 30, 3948–3964. doi: 10.1111/mec.16022
Eyer, P., Matsuura, K., Vargo, E. L., Kobayashi, K., Yashiro, T., Suehiro, W., et al. (2018). Inbreeding tolerance as a pre-adapted trait for invasion success in the invasive ant Brachyponera chinensis. Mol. Ecol. 27, 4711–4724. doi: 10.1111/mec.14910
Fahrner, S., and Aukema, B. H. (2018). Correlates of spread rates for introduced insects. Glob. Ecol. Biogeogr. 27, 734–743.
Fox, J., Friendly, M., and Weisberg, S. (2013). Hypothesis Tests for Multivariate Linear Models Using the car Package. R J. Available online at: https://journal.r-project.org/archive/2013/RJ-2013-004/index.html (accessed July 30, 2021).
Grace, J. K., Aihara-Sasaki, M., and Yates, J. R. (2004). Differences in tunneling behavior of Coptotermes vastator and Coptotermes formosanus (Isoptera: Rhinotermitidae). Sociobiology 43, 153–158.
Hanna, C., Cook, E. D., Thompson, A. R., Dare, L. E., Palaski, A. L., Foote, D., et al. (2013). Colony social structure in native and invasive populations of the social wasp Vespula pensylvanica. Biol. Invasions. 16, 283–294.
Henery, M. L., Bowman, G., Mráz, P., Treier, U. A., Gex-Fabry, E., Schaffner, U., et al. (2010). Evidence for a combination of pre-adapted traits and rapid adaptive change in the invasive plant Centaurea stoebe. J. Ecol. 98, 800–813.
Janowiecki, M., and Vargo, E. L. (2021). Seasonal activity. spatial distribution, and physiological limits of subterranean termites (Reticulitermes Species) in an east texas forest. Insects 12:86. doi: 10.3390/insects12020086
Kasper, M. L., Reeson, A. F., and Austin, A. D. (2008). Colony characteristics of Vespula germanica (F.) (Hymenoptera. Vespidae) in a Mediterranean climate (southern Australia). Aust. J. Entomol. 47, 265–274.
Kassambara, A., and Mundt, F. (2020). Factoextra: Extract and Visualize the Results of Multivariate Data Analyses. Available online at: https://cran.r-project.org/web/packages/factoextra/index.html (accessed July 30, 2021).
Kutnik, M., Paulmier, I., Ansard, D., Montibus, M., and Lucas, C. (2020). “Update on the distribution of termites and other wood-boring insects in Europe, p. IRG/WP 20-10960, in Proceedings IRG Annual Meeting, 1–15.
Lê, S., Josse, J., and Husson, F. (2008). FactoMineR: an R package for multivariate analysis. J. Stat. Softw. 25, 253–258.
Lee, S.-H., Bardunias, P., and Su, N.-Y. (2007). Optimal length distribution of termite tunnel branches for efficient food search and resource transportation. Biosystems 90, 802–807. doi: 10.1016/j.biosystems.2007.04.004
Lenth, R. (2020). Emmeans: Estimated Marginal Means, aka Least-Squares Means. Available online at: https://cran.r-project.org/web/packages/emmeans/index.html (accessed July 30, 2021).
Li, H.-F., Yang, R.-L., and Su, N.-Y. (2010). Interspecific competition and territory defense mechanisms of Coptotermes formosanus and Coptotermes gestroi (Isoptera: Rhinotermitidae). Environ. Entomol. 39, 1601–1607. doi: 10.1603/EN09262
Lockwood, J. L., Cassey, P., and Blackburn, T. (2005). The role of propagule pressure in explaining species invasions. Trends Ecol. Evol. 20, 223–228. doi: 10.1016/j.tree.2005.02.004
Lucas, C., Brossette, L., Lefloch, L., Dupont, S., Christidès, J.-P., and Bagnères, A.-G. (2018). When predator odour makes groups stronger: effects on behavioural and chemical adaptations in two termite species. Ecol. Entomol. 43, 513–524. doi: 10.1111/een.12529
Mizumoto, N., and Matsuura, K. (2013). Colony-specific architecture of shelter tubes by termites. Insectes Soc. 60, 525–530.
Mizumoto, N., Bardunias, P. M., and Pratt, S. C. (2020). Complex relationship between tunneling patterns and individual behaviors in termites. Am. Nat. 196, 555–565. doi: 10.1086/711020
Morales, M. (2017). Sciplot: Scientific Graphing Functions For Factorial Designs. Available online at: https://cran.r-project.org/web/packages/sciplot/index.html (accessed July 30, 2021).
Myles, T. G. (1999). Review of secondary reproduction in termites (Insecta: Isoptera) with comments on its role in termite ecology and social evolution. Sociobiology 33, 1–43.
Nobre, T., Nunes, L., and Bignell, D. E. (2007). Tunnel geometsry of the subterranean termite Reticulitermes grassei (Isoptera: Rhinotermitidae) in response to sand bulk density and the presence of food. Insect Sci. 14, 511–518.
Patel, J. S., Lee, S.-B., Chouvenc, T., and Su, N.-Y. (2020). Inferring termite colony size using wood consumption in subterranean termites (Blattodea: Rhinotermitidae) in laboratory-rearing conditions. J. Econ. Entomol. 113, 905–910. doi: 10.1093/jee/toz353
Penn, D. J., and Frommen, J. G. (2010). “Kin recognition: an overview of conceptual issues, mechanisms and evolutionary theory,” in Anim. Behav. Evol. Mech, ed. P. Kappeler (Berlin: Springer), 55–85.
Perdereau, E., Bagnères, A.-G., Dupont, S., and Dedeine, F. (2010). High occurrence of colony fusion in a European population of the American termite Reticulitermes flavipes. Insectes Soc. 57, 393–402.
Perdereau, E., Bagnères, A.-G., Vargo, E. L., Baudouin, G., Xu, Y., Labadie, P., et al. (2015). Relationship between invasion success and colony breeding structure in a subterranean termite. Mol. Ecol. 24, 2125–2142. doi: 10.1111/mec.13094
Perdereau, E., Baudouin, G., Bankhead-Dronnet, S., Chevalier, Z., Zimmermann, M., Dupont, S., et al. (2019). Invasion dynamics of a termite. reticulitermes flavipes, at different spatial scales in France. Insects 10:30. doi: 10.3390/insects10010030
Perdereau, E., Dedeine, F., Christidès, J.-P., Dupont, S., and Bagnères, A.-G. (2011). Competition between invasive and indigenous species: an insular case study of subterranean termites. Biol. Invasions. 13, 1457–1470. doi: 10.1007/s10530-010-9906-5
Pichon, A., Kutnik, M., Leniaud, L., Darrouzet, E., Châline, N., Dupont, S., et al. (2007). Development of experimentally orphaned termite worker colonies of two Reticulitermes species (Isoptera: Rhinotermitidae). Sociobiology 50, 1015–1034.
Pitts-Singer, T. L., and Forschler, B. T. (2000). Influence of guidelines and passageways on tunneling behavior of Reticulitermes flavipes (Kollar) and R. virginicus (Banks) (Isoptera: Rhinotermitidae). J. Insect Behav. 13, 273–290.
Rust, M. K., and Su, N.-Y. (2012). Managing social insects of urban importance. Annu. Rev. Entomol. 57, 355–375. doi: 10.1146/annurev-ento-120710-100634
Scanes, C. G. (2018). “Invasive Species,” in Anim. Hum. Soc, eds C. G. Scanes and S. R. Toukhsati (New York, NY: Elsevier), 413–426.
Shults, P., Richardson, S., Eyer, P., Chura, M., Barreda, H., Davis, R. W., et al. (2021). Area-Wide elimination of subterranean termite colonies using a novaluron bait. Insects 12, 1–12. doi: 10.3390/insects12030192
Su, N.-Y., and Lee, S.-H. (2009). Tunnel volume regulation and group size of subterranean termites (Isoptera: Rhinotermitidae). Ann. Entomol. Soc. Am. 102, 1158–1164. doi: 10.1603/008.102.0626
Tian, L., Preisser, E. L., Haynes, K. F., and Zhou, X. (2017). Social buffering in a eusocial invertebrate: termite soldiers reduce the lethal impact of competitor cues on workers. Ecology 98, 952–960. doi: 10.1002/ecy.1746
Traniello, J. F. A., and Leuthold, R. H. (2000). “Behavior and ecology of foraging in termites,” in Termit. Evol. Soc. Symbioses, Ecol, eds T. Abe, D. E. Bignell, and M. Higashi (Dordrecht: Springer Netherlands), 141–168.
Van Kleunen, M., Schlaepfer, D. R., Glaettli, M., and Fischer, M. (2011). Preadapted for invasiveness: do species traits or their plastic response to shading differ between invasive and non-invasive plant species in their native range? J. Biogeogr. 38, 1294–1304.
Vargo, E. L., and Husseneder, C. (2009). Biology of subterranean termites: insights from molecular studies of Reticulitermes and Coptotermes. Annu. Rev. Entomol. 54, 379–403. doi: 10.1146/annurev.ento.54.110807.090443
Walther, G. R., Roques, A., Hulme, P. E., Sykes, M. T., Pyšek, P., Kühn, I., et al. (2009). Alien species in a warmer world: risks and opportunities. Trends Ecol. Evol. 24, 686–693. doi: 10.1016/j.tree.2009.06.008
Keywords: exploration behaviors, propagule pressure, termites, social insects, invasive species
Citation: Pailler L, Matte A, Groseiller A, Eyer P-A, Ruhland F and Lucas C (2022) High Exploration Behavior of Termite Propagules Can Enhance Invasiveness. Front. Ecol. Evol. 10:840105. doi: 10.3389/fevo.2022.840105
Received: 20 December 2021; Accepted: 03 February 2022;
Published: 04 March 2022.
Edited by:
Solange Del Carmen Issa Ponce, Simón Bolívar University, VenezuelaReviewed by:
Paulo A. V. Borges, University of the Azores, PortugalThomas Chouvenc, University of Florida, United States
Grant Terral Kirker, Forest Products Laboratory, United States Forest Service (USDA), United States
Copyright © 2022 Pailler, Matte, Groseiller, Eyer, Ruhland and Lucas. This is an open-access article distributed under the terms of the Creative Commons Attribution License (CC BY). The use, distribution or reproduction in other forums is permitted, provided the original author(s) and the copyright owner(s) are credited and that the original publication in this journal is cited, in accordance with accepted academic practice. No use, distribution or reproduction is permitted which does not comply with these terms.
*Correspondence: Christophe Lucas, Y2hyaXN0b3BoZS5sdWNhc0B1bml2LXRvdXJzLmZy