- 1Shandong Provincial Key Laboratory of Applied Microbiology, Ecology Institute, Qilu University of Technology (Shandong Academy of Sciences), Ji’nan, China
- 2State Key Laboratory of Water Environment Simulation, School of Environment, Beijing Normal University, Beijing, China
- 3Henan Key Laboratory of Ecological Environment Protection and Restoration of Yellow River Basin, Yellow River Institute of Hydraulic Research, Zhengzhou, China
Wetland drainage has been intensively implemented globally, and it has exerted significant effects on wetland ecosystems. The effects of wetland drainage on the soil fungal community remain to be clarified. Soil samples were collected at depths of 0–5 and 5–10 cm in freshwater Phragmites australis wetlands to investigate changes in the fungal community before and after drainage (termed FW and DFW, respectively) using high-throughput sequencing of the fungal-specific internal transcribed spacer 1 (ITS1) gene region. No significant differences in the α diversity of the soil fungal community were found in 0–10 cm soils between FW and DFW (p > 0.05), except for the abundance-based coverage estimator (ACE) and Chao1 indices in 5–10 cm soils. Significantly higher values of ACE and Chao1 in 5–10 cm soils in FW than in DFW indicated that wetland drainage may reduce fungal community richness in 5–10 cm soils. Ascomycota, Sordariomycetes, and Cephalothecaceae were the dominant fungal phylum, class, and family, respectively, in 0–5 and 5–10 cm soils of both FW and DFW, representing as high as 76.17, 58.22, and 45.21% of the fungal community in 5–10 FW soils, respectively. Saprotrophic fungi predominated in both FW and DFW. Drainage altered both the fungal community structure and some edaphic factors. Mantel tests and Spearman correlation analyses implied that edaphic factors [i.e., soil organic matter (SOM), electronic conductivity (EC), pH, and clay] also affected soil fungal community structure. Overall, wetland drainage altered the community structure of the fungal community in the freshwater wetlands.
Introduction
Due to anthropogenic activities (i.e., overexploitation), and the ecosystem services they provide have been strongly impacted (Jones et al., 2018). Specifically, extensive wetland drainage for agriculture or other land uses has been conducted throughout the world and has increased dramatically (Drexler et al., 2009; Cortus et al., 2011; Xue et al., 2020). Wetland drainage is one of the primary reasons for wetland loss, for example, causing the loss of more than 40% of prairie wetlands in Alberta (Canada), more than 90% of the wetland area in some states of the midwestern and eastern United States, and even turning 90.5% of fens into seasonally inundated wetlands (Cortus et al., 2011; Jones et al., 2018; Zhang et al., 2019). Wetland drainage will cause drastic changes in the structure and function of wetland soils and further lead to landscape modifications such as land-surface subsidence and loss of water storage capacity (Wiltermuth and Anteau, 2016; Jones et al., 2018). Consequently, the loss of soil mass, the changes in soil physiochemical properties, reduced soil fertility, and the reduction of ecosystem services will result from the drainage of wetlands (Drexler et al., 2009). Additionally, the oxidation of organic matter caused by drainage will result in secondary subsidence in wetlands (Drexler et al., 2009). Historical data also indicate that the landscape modifications by drainage have altered the exchange of energy and moisture at the surface, finally affecting local and regional climate (Schneider and Eugster, 2007). Undoubtedly, drainage induces inevitable variation in the water table, thereby changing hydraulic conductivity and hydrologic processes in wetlands (Brunet and Westbrook, 2012; Jones et al., 2018). However, drainage in depressional wetlands has turned temporarily flooded wetlands into permanently flooded areas with higher water levels (McCauley et al., 2015). It has been reported that the drainage accelerated wetland degradation in the Zoige Plateau (Li et al., 2012; Xue et al., 2020) as the stability of the hydrology maintains the structure and function of wetlands (Zedler, 2000). It is worth noting that the hydrologic modifications may further lead to changes in wetland sedimentation and nutrification as well as community composition (Wiltermuth and Anteau, 2016). Thus, the biota in wetlands, including vegetation and microbes, will certainly be affected by drainage (Brunet and Westbrook, 2012; McCauley et al., 2015).
Fungi are substantially involved in vital ecosystem services (i.e., nutrient cycling, production of clean water, pollutant degradation, compound transformations, suppression of disease-causing soil organisms, and ecological restoration), and they affect global biogeochemical cycles on a large scale due to their important roles as plant symbionts, primary decomposers of organic material, key regulators of soil carbon balance, and the meditation in phosphorus and nitrogen cycles through secreting extracellular enzymes (Treseder and Lennon, 2015; Xu et al., 2017; Guan et al., 2018; Xiao et al., 2020). Fungal biodiversity has been shown to essentially influence the maintenance of wetland ecosystems due to its functions involved in soil health, resilience to perturbations, and capacity for coevolution, interpreting biogeographic patterns, and screening natural products (Mueller and Schmit, 2007; Frąc et al., 2018; Xie et al., 2020). Moreover, the variation in soil fungal community composition can signify environmental changes (Xu et al., 2017). Dini-Andreote et al. (2016) highlighted the importance of fungal community composition in reflecting the successional stages of a marine environment being transformed into a terrestrial system. Onufrak et al. (2020) reported that the variations in fungal community structure contributed to wetland quality assessments with support from the Ohio Rapid Assessment Method (ORAM), and this will further facilitate better management of wetland ecosystems. In addition, Almeida et al. (2020) emphasized that the diversity and composition of the fungal community can be utilized to evaluate and improve restoration success. Thus, the important roles played by fungi necessitate identifying the important factors determining the diversity and composition of the fungal community. Therefore, more exploration of soil fungi is needed, as fungi still exist in a kind of “Earth’s dark matter” (Xu et al., 2017).
The effects of anthropogenic activities on the soil fungal community have been reported by numerous researchers. For example, Hui et al. (2018) found that reforestation of farmland restored the transitional fungal communities and decreased soil fungal diversity. Liu et al. (2020) demonstrated that afforestation of cropland altered functional groups of fungi and reduced fungal diversity. Xiao et al. (2020) found that freshwater restoration reduced the diversity of soil fungi but strengthened the network connectivity. However, how wetland drainage affects the soil fungal community has rarely been reported. As fungi are primarily aerobic heterotrophs and are sensitive to soil oxygen conditions, it can be predicted that the water table drawdown and the consequent increase in oxygen availability brought by wetland drainage will affect the soil fungal community (Asemaninejad et al., 2018; Bergsveinson et al., 2019; Xue et al., 2020). Previous studies have indicated that soil characteristics (i.e., salinity, pH, soil organic carbon, and soil moisture), geographic conditions, and vegetation types impact the diversity of the fungal community (Guan et al., 2018; Hui et al., 2018; Xie et al., 2020). Liu et al. (2018) reported that soil physicochemical characteristics could be reshaped by water table drawdown in Zoige peatlands. Therefore, this study focused on the changes in the diversity and community structure of the soil fungal community before and after drainage to investigate the effects of wetland drainage on the soil fungal community.
Materials and Methods
Sampling Sites, Soil Sample Collection, and Soil Analysis
Close to the north bank of the Yellow River, the studied freshwater Phragmites australis wetlands (FW) are located in the Nature Reserve of the Yellow River Delta. FW were created in 2002 to restore degraded salt marshes by channeling freshwater from the Yellow River. The soils of FW have been inundated by freshwater since then. However, FW has been drained for the construction project of artificial islands since November 2018. Consequently, no standing water exists where P. australis grows, but the soils were still saturated in December 2018.
Soil samples with three replicates were taken from 0–5 and 5–10 cm depths of FW before (October 2018) and after (December 2018) the wetland drainage. As we intended to investigate whether there were differences in the fungal community from 0–5 and 5–10 cm soils, we selected these two soil layers instead of just 0–10 cm soils. After drainage, the wetlands were named drained freshwater P. australis wetlands (DFW). Soils for sequencing were placed in an ice cooler box and stored in a refrigerator at −80°C after being brought to the laboratory. Sequencing and data processing were conducted by Beijing Novogene Co. Ltd. (Beijing, China). Soils for the determination of water content (WC) were dried using a drying oven. Soils for the measurement of other soil properties were air-dried and sieved through a 10-mesh sieve (soil particle analysis), a 20-mesh sieve [pH and electronic conductivity (EC)], or a 100-mesh sieve [including soil organic matter (SOM) and TN], respectively. The specific methods for the determination of soil properties can be found in Zhao et al. (2020).
DNA Extraction, Internal Transcribed Spacer Amplification, and Illumina MiSeq Sequencing
Soil DNA was extracted from approximately 0.5 g soil using a TIANGEN® Magnetic Soil and Stool DNA Kit (Qiagen, Valencia, CA, United States). The DNA concentration and purity were monitored on 1% agarose gels. According to the concentration, DNA was diluted to 1 ng/μl using sterile water. The internal transcribed spacer 1 (ITS1) region was amplified using the primer pair ITS1-1F-F (5′-CTTGG TCATTTAGAGGAAGTAA-3′) and ITS1-1F-R (5′-GCTGCGTT CTTCATCGATGC-3′). All PCR reactions were carried out in 30 μl reactions with 15 μl of Phusion® High-Fidelity PCR Master Mix (New England Biolabs, Ipswich, MA, United States); 0.2 μM of forward and reverse primers, and about 10 ng template DNA. Thermal cycling consisted of initial denaturation at 98°C for 1 min, followed by 30 cycles of denaturation at 98°C for 10 s, annealing at 50°C for 30 s, elongation at 72°C for 30 s, and final extension at 72°C for 5 min.
Equal volumes of 1X loading buffer (containing SYB green) were mixed with PCR products and subjected to electrophoresis on 2% agarose gels for detection. Samples with a bright main strip between 400 and 450 bp were chosen for further analysis. PCR products were mixed in equidensity ratios. Then, the PCR products were purified using the GeneJET Gel Extraction Kit (Thermo Scientific, Belmont, MA, United States). Sequencing libraries were generated using an Illumina TruSeq DNA PCR-Free Sample Preparation Kit (Illumina, San Diego, CA, United States) following the manufacturer’s recommendations, and index codes were added. The library quality was assessed on the Qubit@ 2.0 Fluorometer (Thermo Scientific, Belmont, MA, United States) and Agilent Bioanalyzer 2100 system. Finally, the library was sequenced on an Illumina NovaSeq 6000 platform and 250 bp paired-end reads were generated.
Sequence Processing and Taxonomic Assignment
Sequencing of purified amplicon products was conducted on the Illumina MiSeq PE300 platform in Beijing Novogene Biotech Co. Ltd. (Beijing, China). Paired-end reads were assigned to samples based on their unique barcode and truncated by cutting off the barcode and primer sequence. FLASH (version 1.2.71) was used to merge paired-end reads, and then raw tags were obtained. Quality filtering on the raw tags was performed under specific filtering conditions to obtain the high-quality clean tags according to the QIIME (Quantitative Insights into Microbial Ecology, version 1.9.12) quality control process. The tags were compared with the UNITE database (version 8.03) using the UCHIME algorithm (UCHIME4) to detect and remove chimera sequences. Then, the effective tags were finally obtained. Sequence analyses were performed using Uparse software (Uparse version 7.0.10015). Sequences with ≥ 97% similarity were assigned to the same OTUs. A representative sequence for each OTU was screened for further annotation. For each representative sequence, the UNITE Database6 was used based on the Mothur algorithm to annotate taxonomic information. The phylogenetic relationships of different OTUs and the difference in the dominant species in different samples (groups) of multiple sequence alignment were identified using the MUSCLE software (version 3.8.317). All raw sequencing data of this study are deposited into the NCBI database with the Short Read Archive (SRA) accession number PRJNA814347.
The α diversity indices, including observed species (Sobs), abundance-based coverage estimator (ACE), Chao1, and Shannon diversity, were calculated using QIIME. The β-diversity indices, including principal component analysis (PCA) and principal coordinate analysis (PCoA), were calculated using QIIME software to evaluate the differences in bacterial community composition before and after drainage. Both PCA and PCoA were assessed on the basis of the Bray–Curtis distance of OTUs.
Statistical Analyses
Student’s t-test was conducted to test the difference in soil properties and soil α-diversity indices between the same soil layer of different sites and different soil layers from the same site at p < 0.05 level. Sequencing data were processed on the cloud platform of Novegene. Based on the Bray–Curtis distance, the similarity percentage (SIMPER) analysis was performed using R software to evaluate the contribution of fungal species to the difference in the fungal community between FW and DFW. The Mantel test between the fungal communities and soil properties was conducted at the OTUs, phylum, and class levels based on the Bray–Curtis distance algorithm, to determine environmental variables that structure these communities. Spearman correlation analysis was used to identify the relationships between environmental variables and selected fungal taxa by calculating the correlation coefficients. Mantel test, and Spearman correlation analysis were performed using R software. The trophic model of the fungal community was categorized by subjecting the taxonomic data to the FUNGuild database8.
Results
The Changes in Edaphic Factors After Drainage
Soil properties in different soil layers before and after drainage are shown in Table 1. Soil EC, Mg2+, Ca2+, SO42–, and silt content in 0–5 cm soils were significantly lower in FW than in DFW (p < 0.05), while sand content in 0–5 cm soils was significantly higher in FW than in DFW (p < 0.05). In 5–10 cm soils, soil Mg2+ and SO42– were higher in DFW than in FW (p < 0.05), whereas soil pH was lower in DFW than in FW (p < 0.05). However, EC, Ca2+, SO42–, and clay content between FW and DFW showed significant differences (p < 0.05). Vertically, soil Na+, K+, Mg2+, Ca2+, SO42–, and WC in 0–5 cm soils were significantly higher than in 5–10 cm soils in FW (p < 0.05), while soil SOM, EC, Mg2+, Ca2+, and SO42– in 0–5 cm soils were significantly lower than those in 5–10 cm soils in DFW (p < 0.05).
The α and β Diversities of Soil Fungal Community Before and After Drainage
The comparison of α diversity indices of the fungal community between FW and DFW is shown in Table 2. The coverage index of the fungal communities in FW and DFW both exceeded 99%, indicating that the sequencing could reflect the fungal communities of both sites. The values of Sobs in 0–5 cm soils showed no significant differences between FW and DFW (p > 0.05). However, significantly higher values of Sobs in FW compared with DFW were observed in 5–10 cm soils (p < 0.05), with mean values of 416 and 272 in soils in FW and DFW, respectively. Similar to Sobs, the ACE and Chao1 showed significant differences in 5–10 cm soils between FW and DFW (p < 0.05), with higher values occurring in FW. As for the Shannon index, no significant differences between FW and DFW in 0–5 or 5–10 cm soils (p < 0.05) were found. Besides, no significant differences in Sobs, ACE, Chao1, or Shannon indices were observed between 0–5 and 5–10 cm soils in either FW or DFW.
The PCA and PCoA plots, based on the Bray-Curtis distance of OTUs between FW and DFW, are shown in Figures 1A,B, respectively. In the PCA plot, PC1 and PC2 explained 19.1 and 12.15% of the total variance, respectively. Fungi in the soils of DFW were distributed on the upper axis of PC2. However, fungi in soils of FW were scattered on the lower axis of PC2, except for fungi from one soil sample, which showed a closer relationship with samples of DFW. This indicated that the fungal communities between soils from FW and DFW can be separated to a great degree. Similar to PCA, the PCoA plot showed that the fungal communities of most soil samples from DFW can be distinguished from those of soil samples from FW (Figure 1B), as soil samples from FW and DFW are mainly distributed on the upper and lower axis of PCoA 2, respectively.
The Fungal Community Structure Before and After Drainage
The fungal community structure at phylum level in 0–5 and 5–10 cm soils of FW and DFW is illustrated in Figure 2A. More ITS reads in 0–10 cm soils of DFW (>40%) than in those of FW (<30%) could not be assigned to known fungal phyla. In total, 15 fungal phyla were identified in the soils of FW and DFW. Ascomycota was recognized as the most predominant fungal phylum in both 0–5 and 5–10 cm soils of FW and DFW, with the relative abundance exceeding 50% in 0–10 cm soils of FW and 5–10 cm soils of DFW. The highest relative abundance of Ascomycota was found in 5–10 cm soils of FW, reaching 76.17%. The rest of the 14 fungal phyla occupied less than 1% of the fungal community in 0–10 cm soils of both FW and DFW, except for Rozellomycota, Chytridiomycota, Basidiomycota, Mortierellomycota, and Glomeromycota. Kickxellomycota was not detected in FW, while Monoblepharomycota, Blastocladiomycota, Neocallimastigomycota, and Basidiobolomycota were only found in FW. Ascomycota and Mortierellomycota in both FW and DFW, Chytridiomycota in DFW, and Glomeromycota, Mucoromycota, Monoblepharomycota, Blastocladiomycota, Neocallimastigomycota, and Basidiobolomycota in FW showed higher abundances in 5–10 cm soils than in 0–5 cm soils. The SIMPER analysis showed that the change in abundance of Ascomycota contributed 43.67 and 43.66% to the dissimilarity in 0–5 and 5–10 cm soils between FW and DFW, respectively (Table 3). Additionally, Rozellomycota, Chytridiomycota, Basidiomycota, Mortierellomycota, and Glomeromycota each explained more than 1% of the dissimilarity in 0–5 and 5–10 cm soils between FW and DFW (Table 3). At the phylum level, the changes in abundance of these six taxa determined as high as about 61% of the dissimilarity between FW and DFW, among those with individual contributions greater than 1%.
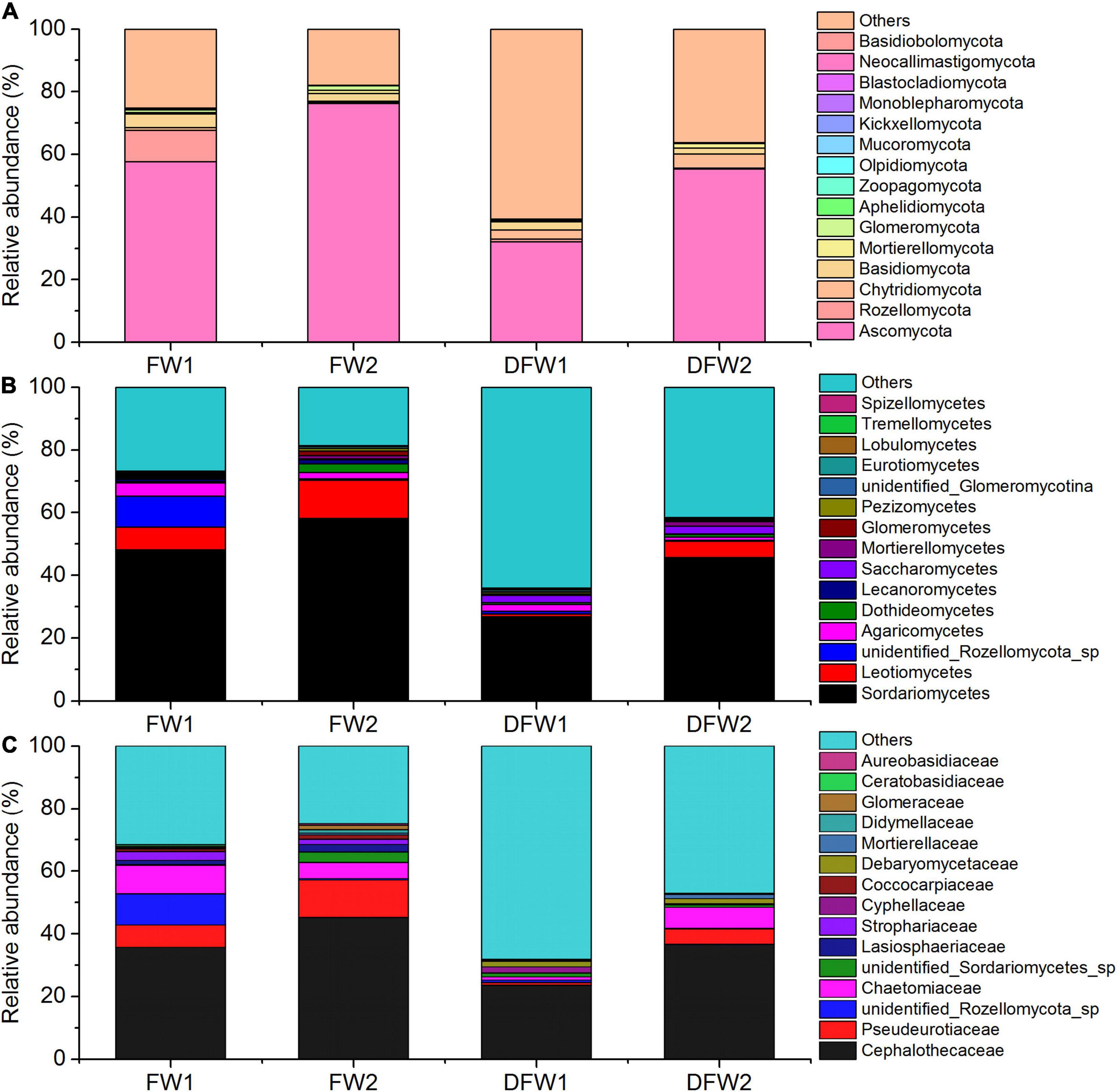
Figure 2. The fungal community structure in 0–5 and 5–10 cm soils of FW and DFW at phylum level (A), at class level (B), and at family level (C). (Note: 1 represents 0–5 cm soils and 2 represents 5–10 cm soils).
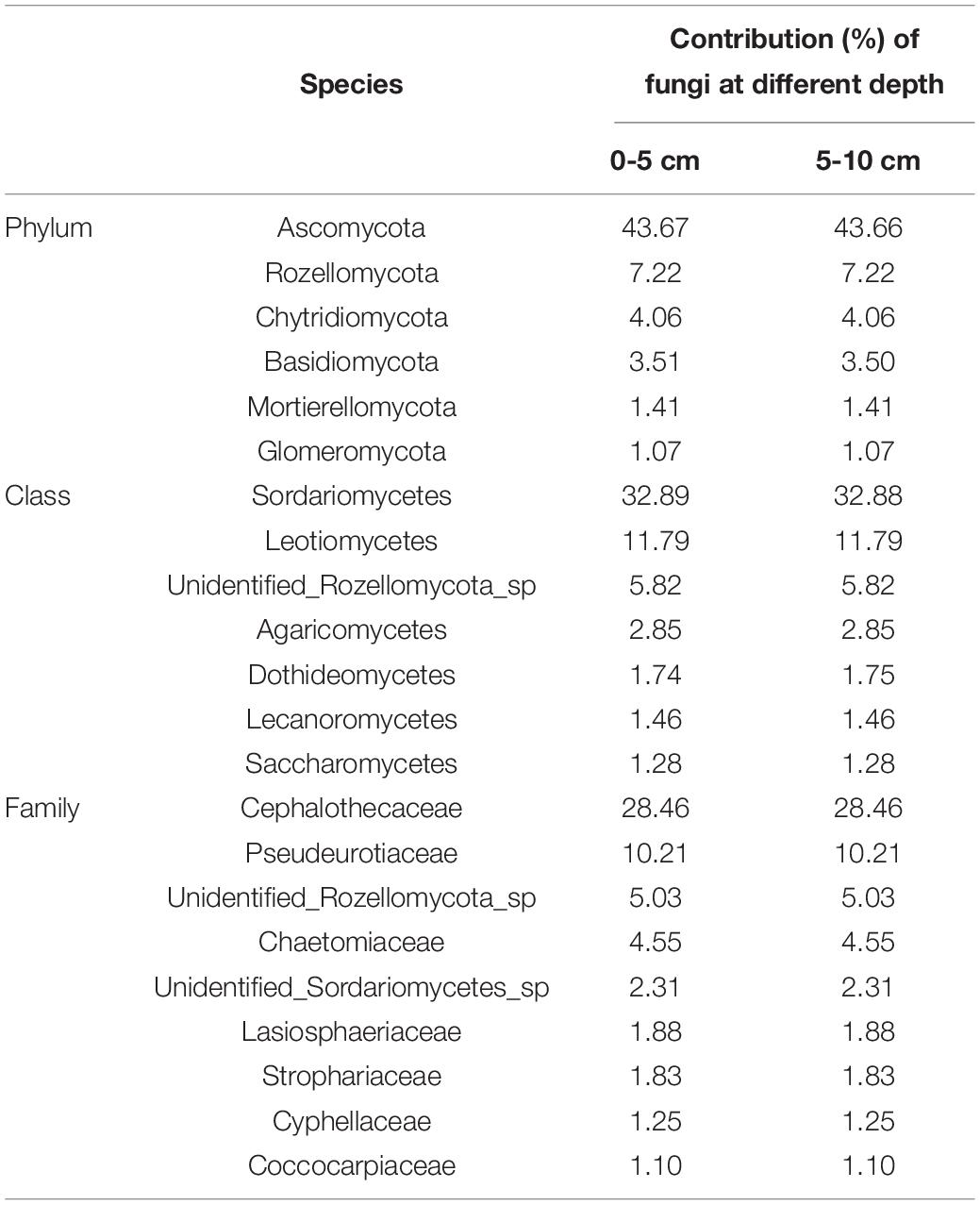
Table 3. The similarity percentage (SIMPER) analysis at phylum, class and family level between FW and DFW.
Figures 2B,C show the top 15 fungal classes and families in 0–5 and 5–10 cm soils of FW and DFW, respectively. The dominant soil fungal class was Sordariomycetes in 0–10 cm soils of both FW and DFW. The proportions occupied by Sordariomycetes in FW were 48.16% in 0–5 cm soils and 58.22% in 5–10 cm soils. However, the relative abundance of Sordariomycetes was lower in DFW than in FW, with values of 26.81% in 0–5 cm soils and 45.61% in 5–10 cm soils. Although Leotiomycetes was the second most abundant fungal class, the proportions of Leotiomycetes in the fungal community in 0–5 cm soils of FW and 0–10 cm soils of DFW were less than 10%. Sordariomycetes, Leotiomycetes, Dothideomycetes, Lecanoromycetes, Saccharomycetes, Mortierellomycetes, and Tremellomycetes in both FW and DFW; Glomeromycetes, Pezizomycetes, and Eurotiomycetes in FW; and unidentified Glomeromycotina in DFW showed increasing trends along with the soil profile, while unidentified Rozellomycota sp., Agaricomycetes, Lobulomycetes, and Spizellomycetes in FW and DFW; Glomeromycetes, Pezizomycetes, and Eurotiomycetes in DFW; and unidentified Glomeromycotina in FW were more enriched in 0–5 cm soils than in 5–10 cm soils. Simultaneously, the changes in the abundances of Sordariomycetes, Leotiomycetes, unidentified Rozellomycota sp., Agaricomycetes, Dothideomycetes, Lecanoromycetes, and Saccharomycetes contributed 61.34 and 61.36% of the dissimilarity in 0–5 and 5–10 cm soils between FW and DFW, respectively, among those with individual contributions greater than 1% (Table 3). The largest contribution was made by Sordariomycetes (32.89% in 0–5 cm soils and 32.88% in 5–10 cm soils), followed by Leotiomycetes (11.79% in 0–10 cm soils).
At the family level, the relative abundance of Cephalothecaceae was highest in 0–10 cm soils in both FW (35.72% in 0–5 cm soils and 45.20% in 5–10 cm soils) and DFW (23.62% in 0–5 cm soils and 36.62% in 5–10 cm soils), followed by Pseudeurotiaceae (Figure 2C). The relative abundance of Pseudeurotiaceae was less than 1% in 0–5 cm soils of DFW but reached 12.00% in 5–10 cm soils of FW. Notably, fungi belonging to Cyphellaceae and Debaryomycetaceae were not detected in 0–10 cm soils in FW. Cephalothecaceae, Pseudeurotiaceae, unidentified Sordariomycetes sp., Lasiosphaeriaceae, Coccocarpiaceae, Mortierellaceae, Didymellaceae, and Aureobasidiaceae in FW and DFW; Chaetomiaceae, Strophariaceae and Ceratobasidiaceae in DFW; and Glomeraceae in FW showed a lower relative abundance in 0–5 cm soils than in 5–10 cm soils, while the relative abundances of unidentified Rozellomycota sp. in FW and DFW; Chaetomiaceae, Strophariaceae, and Ceratobasidiaceae in FW; Cyphellaceae, Debaryomycetaceae, and Glomeraceae in DFW were higher in 0–5 cm soils than in 5–10 cm soils. According to the SIMPER analysis (Table 3), Cephalothecaceae and Pseudeurotiaceae made the highest (28.46%) and second-highest (10.21%) contributions to the dissimilarity in 0–10 cm soils between FW and DFW at the family level, respectively. Each of the abundances of unidentified Rozellomycota_sp., Chaetomiaceae, unidentified Sordariomycetes sp., Lasiosphaeriaceae, Strophariaceae, Cyphellaceae, and Coccocarpiaceae explained > 1% of the dissimilarity between FW and DFW, while the sum of the contributions made by these seven taxa reached 59.04% in both 0–5 and 5–10 cm soils.
The Trophic Characterization of Soil Fungal Community Before and After Drainage
The fungal OTUs in 0–10 cm soils of FW and DFW were categorized into eight trophic modes based on FUNGuild (Figure 3). The proportion of OTUs that could be classified by FUNGuild was higher in FW (>60%) than in DFW (<50%). Saprotroph was the dominant trophic mode in both 0–5 and 5–10 cm soils of FW and DFW, accounting for more than 54.26, 67.16, and 55.98% in 0–5 and 5–10 cm soils of FW and 5–10 cm soils of DFW, respectively. Although Saprotroph just occupied 27.08% of the fungal community in 0–5 cm soils of DFW, its abundance ranked the highest among the OTUs that could be assigned by FUNGuild at 92.79%. Among the remaining seven trophic modes, only the relative abundance of Symbiotroph in 0–10 cm soils of FW and Pathotroph-Saprotroph in 0–5 cm soils of DFW and 5–10 cm soils of FW were higher than 1%. Saprotroph, Pathotroph, Saprotroph-Pathotroph-Symbiotroph, Saprotroph-Symbiotroph, and Saprotroph-Symbiotroph in 0–5 and 5–10 cm soils of FW; Pathotroph-Saprotroph-Symbiotroph and Pathotroph-Symbiotroph in 0–5 cm soils of FW; and Pathotroph-Saprotroph in 5–10 cm soils of FW were more enriched than those of DFW, showing a decreasing trend after drainage.
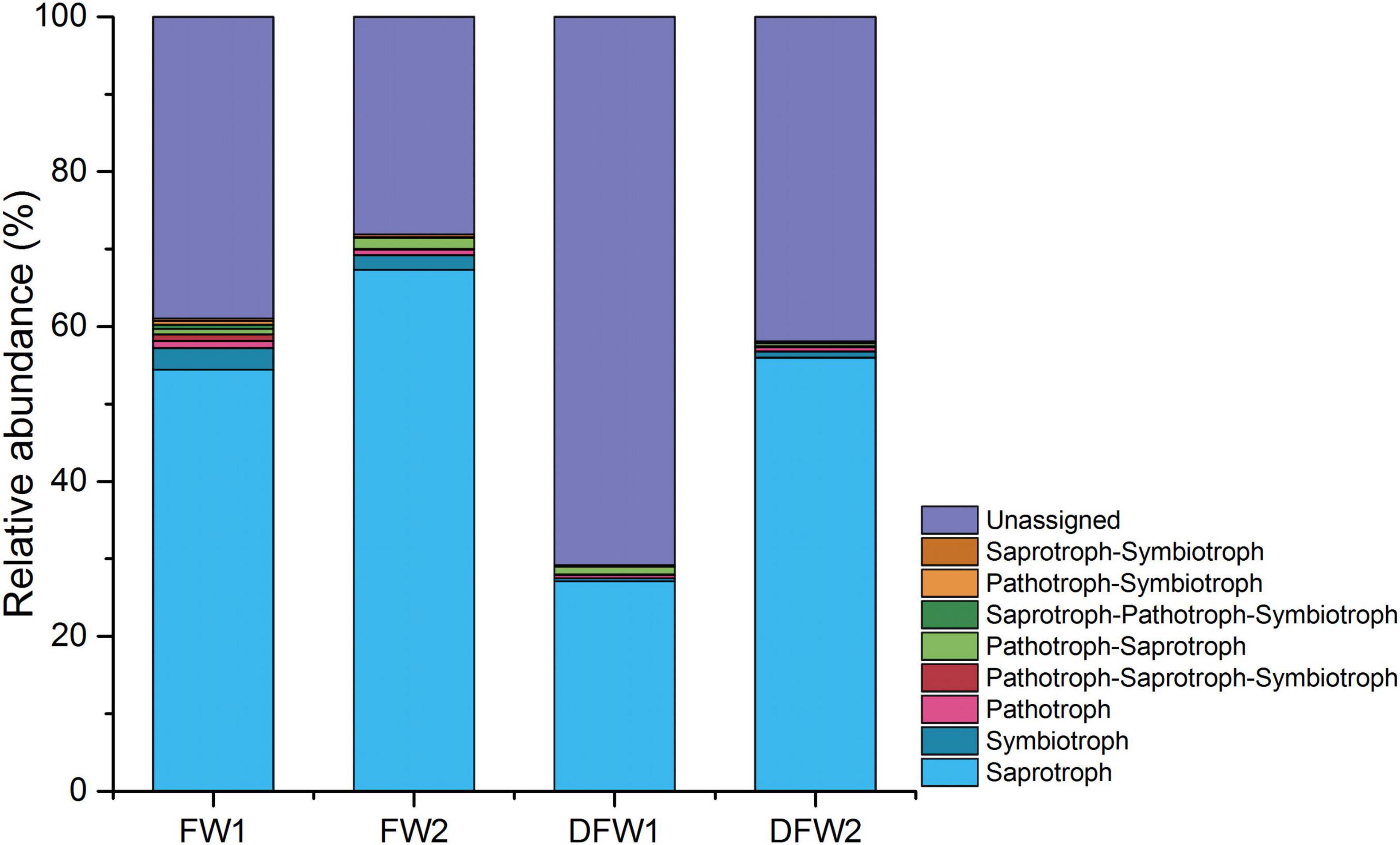
Figure 3. The relative abundance of fungal trophic modes in 0–5 and 5–10 cm soils of FW and DFW. (Note: 1 represents 0–5 cm soils and 2 represents 5–10 cm soils).
The Relationship Between the Fungal Community and Environmental Variables
The results of the Mantel tests between the fungal community and environmental variables at OTU, phylum, class, and family level are shown in Table 4. EC and pH showed significant correlations with the fungal community at OTU, phylum, class, and family levels (p < 0.05) with correlation coefficients ranging from 0.31 to 0.48. However, no significant relationships between the fungal community and other environmental variables were observed (p > 0.05).
The Spearman correlations between environmental variables and α diversity of the fungal community and fungal community at phylum, class, and family level are exhibited in Figures 4A–D, respectively. Soil clay and Cl– showed significantly negative and positive relationships with Sobs, ACE, and Chao1 indices (p < 0.05), respectively, while soil Ca2+ showed a significantly negative relationship with the Chao1 index (p < 0.05). At the phylum level, there were significant correlations between Ascomycota, Chytridiomycota, Mortierellomycota, Aphelidiomycota, Zoopagomycota, Olpidiomycota, Mucolomycota, Blastocladiomycota, and Neocallimastigomycota and all soil properties except for silt (p < 0.05, Figure 4B). At the class level, Dothideomycetes, Saccharomycetes, Mortierellomycetes, Glomeromycetes, Lobulomycetes, Tremellomycetes, and Spizellomycetes were significantly related to all soil properties except for silt and pH (p < 0.05, Figure 4C). At the family level, soil clay, Cl–, Ca2+, K+, Na+, pH, EC, C/N ratio, TN, and SOM were significantly related to Lasiosphaeriaceae, Strophariaceae, Cyphellaceae, Coccocarpiaceae, Debaryomycetaceae, Mortierellaceae, Didymellaceae, and Aureobasidiaceae (p < 0.05, Figure 4D).
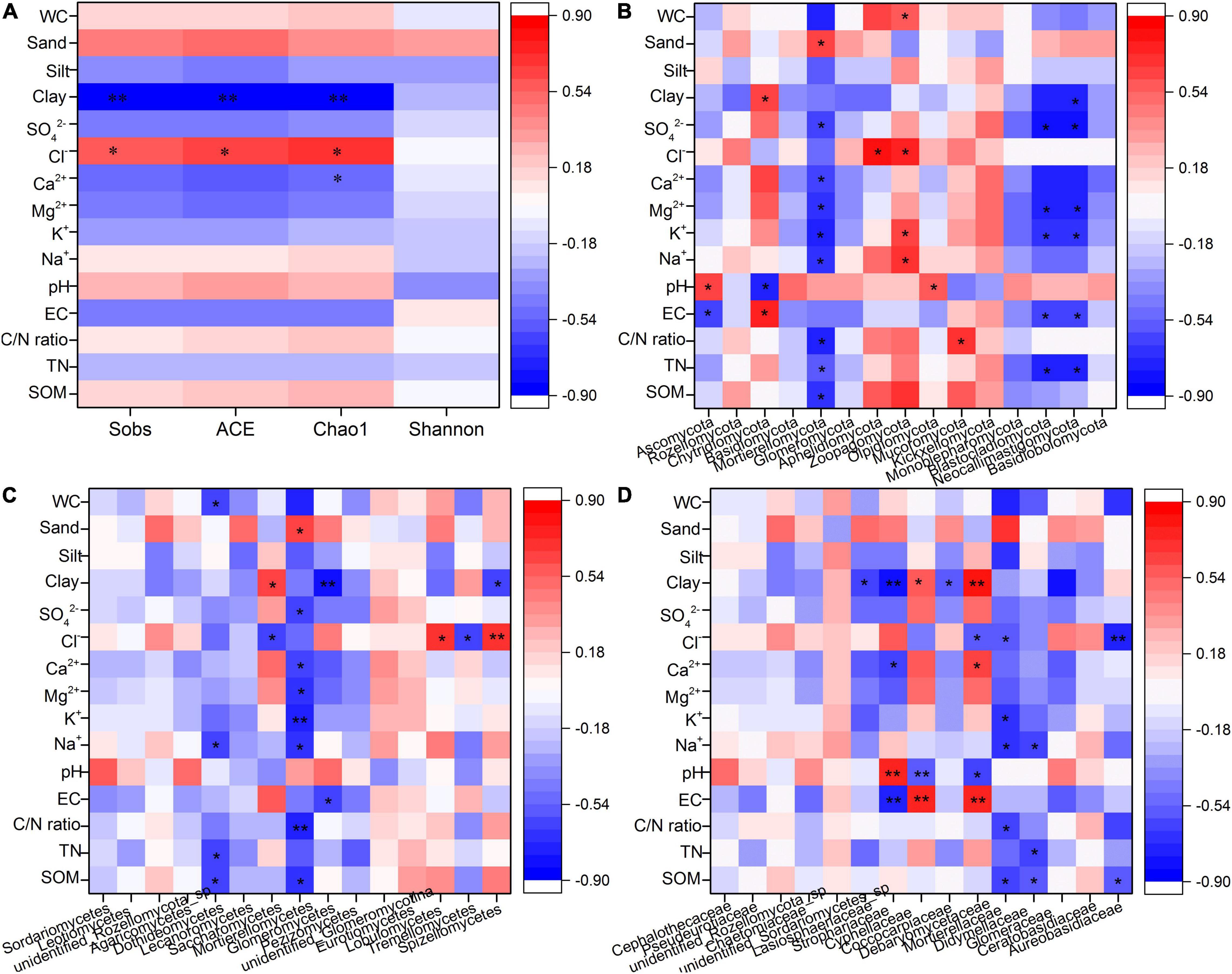
Figure 4. Spearman correlation analyses between environmental variables and α diversity indices (A), fungal community at phylum level (B), selected fungal classes (C), and selected fungal families (D).
Discussion
How Drainage Affects the Soil Fungal Community
Drainage caused the disappearance of standing water in FW, turning the long-term flooded soils into a saturated state and temporarily creating a more aerobic soil environment. Hydrology is one of the dominant controlling factors of fungal diversity and community composition, and water table drawdown could induce both positive and negative effects on fungal communities (Asemaninejad et al., 2017; Almeida et al., 2020). The increased oxygen availability by short-term water table drawdown favors the fungal cell energy metabolism (especially for saprotrophs), while long-term water table drawdown results in comprehensive changes in the soil environment (Asemaninejad et al., 2017). Microorganisms are sensitive to the availability of water and oxygen in wetland ecosystems (Xue et al., 2020). Fungi are also capable of compensating for unfavorable soil moisture conditions (Jassey et al., 2017). Soil moisture has been confirmed as one of the main drivers for changes in the fungal community (Zhao et al., 2019). Different oxygen requirements of different fungi in their life cycle possibly render fungal communities altered after drainage (Guan et al., 2018). Almeida et al. (2020) considered that the sensitivity of some fungi to dry conditions or the increased dispersal of spores to wetter sites could explain the decreased fungal diversity and shifted composition as the water level relatively lowered in the Everglades tree islands. Peltoniemi et al. (2009) reported that a short-term water-level drawdown induced an increase in fungal diversity in a boreal peatland in Finland. Tian et al. (2022) found that the composition and size of the fungal community in 0–25 cm soils in Zoige peatlands were not significantly affected by aerobic conditions. A decreasing trend in the richness of the soil fungal community was observed after drainage, but statistically significant differences were only detected for the Sobs, ACE, and Chao1 indices in 5–10 cm soils (Table 2). The diversity indicated by the Shannon index in 0–10 cm soils and the abundance of the fungal community in 0–5 cm soils showed no significant differences before and after drainage. The disparity in these studies might be caused by the initial in situ water table level, duration time of the water-level drawdown, or the wetland type (Peltoniemi et al., 2009; Tian et al., 2022).
Various studies have reported the influence of soil properties on soil fungal abundance and diversity (Guan et al., 2018; Zhao et al., 2019; Xue et al., 2020; Hou et al., 2021). According to Hou et al. (2021), soil Ca2+ was negatively correlated with the Chao1 index of soil fungi. In this study, Spearman correlation analysis showed that soil clay and Ca2+ negatively affected the abundance of the fungal community (Figure 4A). However, soil Ca2+ and clay positively responded to wetland drainage, indicating that drainage possibly causes the decreased abundance of the fungal community in the long run. Guan et al. (2018) found that soil organic carbon negatively affected the diversity of the fungal community due to the competition for organic matter and the increased decomposition ability. No significant differences in SOM were found in 0–10 cm soils of FW or DFW in this study, a factor that might explain the similar α diversity of fungal communities in FW and DFW. Salinity has been reported to negatively affect the fungal Shannon diversity in degraded grasslands (Wu et al., 2021). Xiao et al. (2020) found that EC explained 14.93% of the difference in soil fungal communities between freshwater restored wetlands and natural wetlands. Zhao et al. (2019) reported that salinity could predict changes in the fungal community. Although no significant correlations were observed between fungal α diversity indices or EC and salt ions, these salinity parameters selectively affected some fungal species as shown by the Spearman correlation analysis (Figure 4). Simultaneously, soil EC, Mg2+, Ca2+, and SO42– responded positively to wetland drainage. Thus, drainage possibly imposes a further decreasing effect on fungal community diversity by affecting soil salinity. Soil pH has been shown to significantly affect soil fungal OTU numbers and community structure, but no significant relationship between the abundance of the fungal community and pH was observed (Wang et al., 2015; Dini-Andreote et al., 2016). In this study, pH significantly affected the soil fungal community at various levels (p < 0.05, Table 4) and had significant relationships with the abundances of Ascomycota, Chytridiomycota, Olpidiomycota, Strophariaceae, Cyphellaceae, and Debaryomycetaceae (p < 0.05, Figure 4). Several studies have reported the structuring effect of both silt and sand content on the fungal community (Chen et al., 2017; Zhao et al., 2017; Dos Passos et al., 2021), a pattern that was confirmed in this study. Although a statistically significant difference was only observed in 0–5 cm soils, the silt and sand content increased and decreased after drainage in this study. Higher WC can stimulate an increase in the diversity of the fungal community (Xu et al., 2017). However, drainage did not have any significant effects on the soil WC (Table 1), which might be another reason for the similar diversity of the fungal community before and after drainage. Additionally, SOM, TN, C/N ratio, Na+, and WC significantly affected some fungal species despite the fact that there were no significant drainage effects on these factors. Thus, the associated changes caused by drainage in soil properties would alter the soil fungal community. Apart from soil properties, soil depth is considered a non-negligible influencing factor. The variations of the fungal community along the soil profile have been reported at both small and large spatial scales by several researchers. For example, Pagano et al. (2016) found that the abundance of the fungal community showed a clear decreasing tendency as soil depth increased in anthropic Amazonian dark soils. Li et al. (2020) reported that the community structure of soil fungi in paddy fields varied along with soil depth due to the variations of soil organic carbon and nitrogen content in three regions across China. In this study, the fungal community richness showed no significant changes along with the depth, but the community composition varied between 0–5 and 5–10 cm soil depths.
The Specific Effects of Drainage on Fungal Community Structure and the Possible Ecological Impact
The fungal community structure seemed to be more sensitive to drainage than the α diversity indices. The proportions of ITS reads that could not be assigned to any fungal phyla reached up to 60.62% in 0–5 cm soils and 36.24% in 5–10 cm soils of DFW, which were much higher than those in 0–10 cm soils of FW (25.16% for 0–5 cm soil layer and 17.92% for 5–10 cm soil layer). Dini-Andreote et al. (2016) also found that 49.5% of the fungal sequences from sediments of salt marshes with zero year of succession could not be affiliated with any fungal phylum in the database. Recently, Cheung et al. (2018) reported that about 23% of ITS reads cannot be recognized as known fungal phyla, and they considered that these unassigned fungi might be identified as novel early diverging lineages that could facilitate our understanding of the evolution of fungi.
Both compositional and functional shifts in the fungal community associated with water table level changes have been observed by Peltoniemi et al. (2012) and Asemaninejad et al. (2017). In this study, drainage decreased the relative abundances of Saprotroph, Pathotroph, Saprotroph-Pathotroph-Symbiotroph, Saprotroph-Symbiotroph, Saprotroph-Symbiotroph Pathotroph-Saprotroph-Symbiotroph, Pathotroph-Symbiotroph, and Pathotroph-Saprotroph in 0–5 or/and 5–10 cm soils in the freshwater wetlands. Jassey et al. (2017) found that saprotrophic fungi showed a gradually increasing trend as water level decreased, which collaborates with this study. Saprotrophic fungi can boost the process of carbon mineralization and potentially affect long-term carbon storage through accretion of soil recalcitrant C carbon (Treseder and Lennon, 2015). Moreover, increased saprotrophic fungi improved enzyme activity (Jassey et al., 2017). Thus, the changes in the relative abundance of saprotrophic fungi resulting from wetland drainage will consequently affect wetland carbon storage in the future. At the phylum level, the fungal species contributing more than 1% to the dissimilarity before and after drainage were Ascomycota, Rozellomycota, Chytridiomycota, Basidiomycota, Mortierellomycota, and Glomeromycota. However, the fungal class and family that contributed more than 1% to the difference between the soil fungal communities of FW and DFW belonged to three phyla (Ascomycota, Rozellomycota, and Basidiomycota). Among fungal species (at phylum, class, and family level) that made higher contributions to the dissimilarity before and after drainage, the relative abundances of most species in both 0–5 and 5–10 cm soils decreased after drainage except for Mortierellomycota, Chaetomiaceae, and unidentified Sordariomycetes sp. in 5–10 cm soils of FW, Dothideomycetes, and Saccharomycetes in 0–5 cm soils of FW and Cyphellaceae in both 0–5 and 5–10 cm soils of FW.
As saprotrophic fungal species and important decomposers of cellulose, Ascomycota have been reported as the dominant fungal phylum in various soil types, such as coastal wetlands sediments, alpine wetlands soils, paddy soils, subtropical bamboo forests, and degraded grasslands (Li et al., 2017, 2020; Cheung et al., 2018; Xie et al., 2020; Wu et al., 2021). Consistently, Ascomycota, the Sordariomycetes class, and the Cephalothecaceae family belonging to Ascomycota held the highest proportions in fungal communities in 0–5 and 5–10 cm soils in both FW and DFW in this study, respectively, demonstrating that cellulose-degrading species dominated in the fungal community of both FW and DFW (Li et al., 2017). Dothideomycetes have been reported as the largest class of Ascomycota with the highest ecological diversity (Hongsanan, 2020). Most species of freshwater Dothideomycetes are saprobic fungi, decomposing submerged woody debris and leaves in water (Dong et al., 2020). However, the relative abundance of Dothideomycetes in 0–10 cm soils of FW and DFW was quite low in this study. As the second-largest class of Ascomycota, Sordariomycetes are widely found in various environments and contain functionally diverse members, such as plant pathogens and saprobes (Maharachchikumbura et al., 2016). Freshwater Sordariomycetes contain diverse lignicolous freshwater fungi and thus participate in the functioning of ecosystems (Luo et al., 2019). Similar to Sordariomycetes, Leotiomycetes consist of a large number of non-lichenized ascomycetes fungi and are widely found in various biotopes, producing inoperculate, unitunicate asci in perithecial or apothecial ascomata (Zhang and Wang, 2015). Members of Chaetomiaceae are capable of producing a series of enzymes that possess significant applications in biotechnology and industry, and most members are renowned for breaking down cellulose (Ibrahim et al., 2021). The decrease in the relative abundances of Ascomycota, Sordariomycetes, and Cephalothecaceae after drainage indicated that drainage would affect cellulose decomposition (Li et al., 2017).
Rozellomycota consist of uniflagellated and unwalled zoospores with ready motility acquired from swimming (Dini-Andreote et al., 2016). Thus, the members of Rozellomycota may thrive more in the flooding soil environment of FW than in DFW, explaining the higher relative abundance of Rozellomycota in FW than in DFW. Besides, it has been reported that sand content positively affected the relative abundance of Rozellomycota (Dini-Andreote et al., 2016). The higher sand content in 0–10 cm soils of FW than that of DFW might partly explain why Rozellomycota was more enriched in FW.
Generally, Chytridiomycota (Chytrids) favor aquatic environments and terrestrial habitats with surface water (Peltoniemi et al., 2009). However, many Chytrids can endure short-term desiccation through producing resistant spores, and thus they can survive from water table drawdown for short periods (Asemaninejad et al., 2017). In this study, the relative abundance of Chytridiomycota increased after drainage, perhaps due to the high proportion of desiccation-resistant Chytrids in the freshwater.
Basidiomycota are also involved in carbon cycling through degrading organic substances (especially lignin), and members (especially in the class Agaricomycetes) are typical representatives of cellulose degraders (Treseder and Lennon, 2015; Li et al., 2017; Wu et al., 2021). Xue et al. (2020) reported that the relative abundance of Basidiomycota showed an enormously increasing trend as the water table lowered in drained peatlands of the Zoige Plateau. This might be associated with the differences in drainage duration observed in these two studies, as drainage duration affects the response of microbial communities to the decline of the water table (Xue et al., 2020). In line with the study of Fu et al. (2021), no environmental variables significantly affected the relative abundance of Basidiomycota. Agaricomycetes are the most abundant class within the phylum Basidiomycota are widely distributed in soils due to the high production of atmospheric fungal spores (50%) (Santillán et al., 2021). The relative abundance of Agaricomycetes also showed a negative response to wetland drainage in this study.
Mortierellomycota contain a large group of saprotrophs in the soil that can dissolve soil mineral phosphorus to enhance soil nutrient levels (Wang et al., 2020). The relative abundance of Mortierellomycota has been reported to be diminished by higher SOM and TN content (Qiu et al., 2020; Wu et al., 2021), which collaborates with the spearman correlation results of this study (Figure 4). However, SOM and TN weren’t significantly altered by drainage in this study, which might explain why only a quite small change was observed in the relative abundance of Mortierellomycota.
Glomeromycota are a globally distributed fungal phylum, and Glomeromycota fungi that can form arbuscular mycorrhiza are known as Arbuscular mycorrhizal fungi (AMF), importantly affecting plant growth and maintaining soil functions and the sustainability of the soil environment (Rivera-Becerril et al., 2017; Stürmer et al., 2018). Although no significant relationships between Glomeromycota and edaphic factors were observed, the relative abundance of Glomeromycota decreased after drainage.
Conclusion
This study has shed light upon the effects of drainage on the fungal community and soil properties in a freshwater wetland in the Yellow River Delta vegetated with P. australis. Wetland drainage lowered the water table in freshwater wetlands and turned the flooded soil environment into a saturated state, altering the soil fungal community as well as several edaphic factors. Drainage increased the contents of soil EC, Mg2+, Ca2+, SO42–, and silt but lowered sand content and pH in 0–5 or 5–10 cm soils. The predominant Ascomycota, Sordariomycetes, and Cephalothecaceae at phylum, class, and family level, respectively, all decreased after drainage and also made the highest contribution to the dissimilarity in the fungal community before and after drainage, as revealed by the SIMPER analysis. However, drainage increased the relative abundances of Chytridiomycota and Cyphellaceae in both 0–5 and 5–10 cm soils. Additionally, most of the remaining fungal species caused large changes (1%) in the fungal community before and after drainage exhibited a decrease after drainage in both 0–5 and 5–10 cm soils except for Mortierellomycota, Chaetomiaceae, unidentified Sordariomycetes sp., Dothideomycetes, Saccharomycetes, and Cyphellaceae. Multiple statistical analyses unraveled that SOM, TN, C/N ratio, EC, pH, Cl–, Na+, K+, Mg2+, Ca2+, SO42–, clay, and WC influenced fungal community structure at different levels. Thus, drainage also changed the soil fungal community through altering edaphic factors. The effects of drainage on the soil fungal community potentially change soil nutrient cycling, especially the carbon cycle in wetlands. Therefore, the possible influence of drainage on wetland ecosystems should be taken into consideration when trading off whether to conduct a drainage project.
Data Availability Statement
The original contributions presented in the study are included in the article/supplementary material, further inquiries can be directed to the corresponding author/s.
Author Contributions
QZ: methodology, investigation, analysis, draft writing, revision, and funding acquisition. JB: methodology and supervision. JJ: investigation, analysis, draft writing, and revision. GZ: investigation and analysis. JW and YG: literature search and funding acquisition. All authors contributed to the article and approved the submitted version.
Funding
This study was financially supported by the Shandong Provincial Natural Science Foundation (ZR2019BD007), Major Scientific and Technological Innovation Projects in Shandong Province (2021CXGC011201), Joint Funds of the National Natural Science Foundation of China (U1806217), and the National Key R&D Program of China (2019YFC1804103).
Conflict of Interest
The authors declare that the research was conducted in the absence of any commercial or financial relationships that could be construed as a potential conflict of interest.
Publisher’s Note
All claims expressed in this article are solely those of the authors and do not necessarily represent those of their affiliated organizations, or those of the publisher, the editors and the reviewers. Any product that may be evaluated in this article, or claim that may be made by its manufacturer, is not guaranteed or endorsed by the publisher.
Acknowledgments
We are grateful to the editor and two reviewers for their constructive feedback on our article.
Footnotes
- ^ http://ccb.jhu.edu/software/FLASH/
- ^ http://qiime.org/scripts/split_libraries_fastq.html
- ^ http://unite.ut.ee/
- ^ http://www.drive5.com/usearch/manual/uchime_algo.html
- ^ http://drive5.com/uparse/
- ^ http://www.arb-silva.de/
- ^ http://www.drive5.com/muscle/
- ^ http://www.stbates.org/guilds/app.php
References
Almeida, B. K., Ross, M. S., Stoffella, S. L., Sah, J. P., Cline, E., Sklar, F., et al. (2020). Diversity and Structure of Soil Fungal Communities across Experimental Everglades Tree Islands. Diversity 12:324. doi: 10.3390/d12090324
Asemaninejad, A., Thorn, R. G., Branfireun, B. A., and Lindo, Z. (2018). Climate change favours specific fungal communities in boreal peatlands. Soil Biol. Biochem. 120, 28–36. doi: 10.1016/j.soilbio.2018.01.029
Asemaninejad, A., Thorn, R. G., and Lindo, Z. (2017). Experimental climate change modifies degradative succession in boreal peatland fungal communities. Microbial. Ecol. 73, 521–531. doi: 10.1007/s00248-016-0875-9
Bergsveinson, J., Perry, B., Simpson, G., Yost, C. K., Schutzman, R. J., Hall, B. D., et al. (2019). Spatial analysis of a hydrocarbon waste-remediating landfarm demonstrates influence of management practices on bacterial and fungal community structure. Microb. Biotechnol. 12, 1199–1209. doi: 10.1111/1751-7915.13397
Brunet, N. N., and Westbrook, C. J. (2012). Wetland drainage in the Canadian prairies: Nutrient, salt and bacteria characteristics. Agric. Ecosyst. Environ. 146, 1–12. doi: 10.1016/j.agee.2011.09.010
Chen, Y., Xu, T., Veresoglou, S., Hu, H., Hao, Z., Hu, Y., et al. (2017). Plant diversity represents the prevalent determinant of soil fungal community structure across temperate grasslands in northern China. Soil Biol. Biochem. 110, 12–21. doi: 10.1016/j.soilbio.2017.02.015
Cheung, M. K., Wong, C. K., Chu, K. H., and Kwan, H. S. (2018). Community Structure, Dynamics and Interactions of Bacteria, Archaea and Fungi in Subtropical Coastal Wetland Sediments. Sci. Rep. 8:14397 doi: 10.1038/s41598-018-32529-5
Cortus, B. G., Jeffrey, S. R., Unterschultz, J. R., and Boxall, P. C. (2011). The Economics of Wetland Drainage and Retention in Saskatchewan. Can. J. Agric. Econ. 59, 109–126. doi: 10.1111/j.1744-7976.2010.01193.x
Dini-Andreote, F., Pylro, V. S., Baldrian, P., van Elsas, J. D., and Salles, J. F. (2016). Ecological succession reveals potential signatures of marine-terrestrial transition in salt marsh fungal communities. ISME J. 10, 1984–1997. doi: 10.1038/ismej.2015.254
Dong, W., Wang, B., Hyde, K. D., McKenzie, E. H. C., Raja, H. A., Tanaka, K., et al. (2020). Freshwater Dothideomycetes. Fungal Diver. 105, 319–575.
Dos Passos, J., Maia, L., de Assis, D., Da Silva, J., Oehl, F., and Da Silva, I. (2021). Arbuscular mycorrhizal fungal community structure in the rhizosphere of three plant species of crystalline and sedimentary areas in the Brazilian dry forest. Microb. Ecol. 82, 104–121. doi: 10.1007/s00248-020-01557-y
Drexler, J. Z., de Fontaine, C. S., and Deverel, S. J. (2009). The legacy of wetland drainage on the remaining peat in the Sacramento — San Joaquin Delta, California, USA. Wetlands 29, 372–386. doi: 10.1672/08-97.1
Frąc, M., Hannula, S. E., Bełka, M., and Jêdryczka, M. (2018). Fungal biodiversity and their role in soil health. Front. Microbiol. 9:707. doi: 10.3389/fmicb.2018.00707
Fu, B., Li, Z., Gao, X., Wu, L., Lan, J., and Peng, W. (2021). Effects of subsurface drip irrigation on alfalfa (Medicago sativa L.) growth and soil microbial community structures in arid and semi-arid areas of northern China. Appl. Soil Ecol. 159:103859. doi: 10.1016/j.apsoil.2020.103859
Guan, M., Pan, X., Wang, S., Wei, X., Zhang, C., Wang, J., et al. (2018). Comparison of fungal communities among ten macrophyte rhizospheres. Fungal Biol. 122, 867–874. doi: 10.1016/j.funbio.2018.05.001
Hongsanan, S. (2020). Refined families of Dothideomycetes: Dothideomycetidae and Pleosporomycetidae. Mycosphere 11, 1553–2107. doi: 10.5943/mycosphere/11/1/13
Hou, Y., Zeng, W., Hou, M., Wang, Z., Luo, Y., Lei, G., et al. (2021). Responses of the Soil Microbial Community to Salinity Stress in Maize Fields. Biology 10:1114. doi: 10.3390/biology10111114
Hui, N., Liu, X., Jumpponen, A., Setälä, H., Kotze, D. J., Biktasheva, L., et al. (2018). Over twenty years farmland reforestation decreases fungal diversity of soils, but stimulates the return of ectomycorrhizal fungal communities. Plant Soil 427, 231–244. doi: 10.1007/s11104-018-3647-0
Ibrahim, S. R. M., Mohamed, S. G. A., Sindi, I. A., and Mohamed, G. A. (2021). Biologically active secondary metabolites and biotechnological applications of species of the family Chaetomiaceae (Sordariales): an updated review from 2016 to 2021. Mycol. Prog. 20, 595–639. doi: 10.1007/s11557-021-01704-w
Jassey, V. E. J., Reczuga, M. K., Zielińska, M., Słowińska, S., Robroek, B. J. M., Mariotte, P., et al. (2017). Tipping point in plant–fungal interactions under severe drought causes abrupt rise in peatland ecosystem respiration. Glob. Change Biol. 24, 972–986. doi: 10.1111/gcb.13928
Jones, C. N., Evenson, G. R., Mclaughlin, D. L., Vanderhoof, M. K., Lang, M. W., Mccarty, G. W., et al. (2018). Estimating restorable wetland water storage at landscape scales. Hydrol. Proc. 32, 305–313. doi: 10.1002/hyp.11405
Li, M., Li, L., Liao, H., Huang, Z., Huang, Z., and Huang, W. (2012). The influence of drainage on wetland degradation in Zoige Plateau. Dis. Adv. 5, 659–666.
Li, X., Wang, H., Li, X., Li, X., and Zhang, H. (2020). Distribution characteristics of fungal communities with depth in paddy fields of three soil types in China. J. Microbiol. 58, 279–287. doi: 10.1007/s12275-020-9409-8
Li, Y., Li, Y., Chang, S. X., Liang, X., Qin, H., Chen, J., et al. (2017). Linking soil fungal community structure and function to soil organic carbon chemical composition in intensively managed subtropical bamboo forests. Soil Biol. Biochem. 107, 19–31. doi: 10.1016/j.soilbio.2016.12.024
Liu, J., Le, T. H., Zhu, H., Yao, Y., Zhu, H., Cao, Y., et al. (2020). Afforestation of cropland fundamentally alters the soil fungal community. Plant Soil 457, 279–292. doi: 10.1007/s11104-020-04739-2
Liu, L., Chen, H., Jiang, L., Hu, J., and Zhan, W. (2018). Water table drawdown reshapes soil physicochemical characteristics in Zoige peatlands. Catena 170, 119–128. doi: 10.1016/j.catena.2018.05.025
Luo, Z., Hyde, K., Liu, J., Maharachchikumbura, S., Jeewon, R., Bao, D., et al. (2019). Freshwater Sordariomycetes. Fungal Divers. 99, 451–660. doi: 10.1007/s13225-019-00438-1
Maharachchikumbura, S., Hyde, K., Jones, E., McKenzie, E. H. C., Bhat, J. D., Dayarathne, M. C., et al. (2016). Families of Sordariomycetes. Fungal Divers. 79, 1–317. doi: 10.1007/s13225-016-0369-6
McCauley, L. A., Anteau, M. J., van der Burg, M. P., and Wiltermuth, M. T. (2015). Land use and wetland drainage affect water levels and dynamics of remaining wetlands. Ecosphere 6, 1–22.
Mueller, G. M., and Schmit, J. P. (2007). Fungal biodiversity: what do we know? What can we predict? Biodivers. Conserv. 16, 1–5. doi: 10.1007/s10531-006-9117-7
Onufrak, A., Rúa, M. A., and Hossler, K. (2020). The Missing Metric: An Evaluation of Fungal Importance in Wetland Assessments. Wetlands 40, 825–838. doi: 10.1007/s13157-019-01228-w
Pagano, M., Ribeiro-Soares, J., Cançado, L., Falcão, N., Gonçalves, V., Rosa, L., et al. (2016). Depth dependence of black carbon structure, elemental and microbiological composition in anthropic Amazonian dark soil. Soil Till. Res. 155, 298–307. doi: 10.1016/j.still.2015.09.001
Peltoniemi, K., Fritze, H., and Laiho, R. (2009). Response of fungal and actinobacterial communities to water-level drawdown in boreal peatland sites. Soil Biol. Biochem. 41, 1902–1914. doi: 10.1016/j.soilbio.2009.06.018
Peltoniemi, K., Straková, P., Fritze, H., Iráizoz, P. A., Pennanen, T., and Laiho, R. (2012). How water-level drawdown modifies litter-decomposing fungal and actinobacterial communities in boreal peatlands. Soil Biol. Biochem. 51, 20–34. doi: 10.1016/j.soilbio.2012.04.013
Qiu, Y., Lv, W., Wang, X., Xie, Z., and Wang, Y. (2020). Long-term effects of gravel mulching and straw mulching on soil physicochemical properties and bacterial and fungal community composition in the Loess Plateau of China. Eur. J. Soil Biol. 98:103188. doi: 10.1016/j.ejsobi.2020.103188
Rivera-Becerril, F., van Tuinen, D., Chatagnier, O., Rouard, N., Béguet, J., Kuszala, C., et al. (2017). Impact of a pesticide cocktail (fenhexamid, folpel, deltamethrin) on the abundance of Glomeromycota in two agricultural soils. Sci. Total Environ. 577, 84–93. doi: 10.1016/j.scitotenv.2016.10.098
Santillán, J., López-Martínez, R., Aguilar-Rangel, E., Hernández-García, K., Vásquez-Murrieta, M., Cram, S., et al. (2021). Microbial diversity and physicochemical characteristics of tropical karst soils in the northeastern Yucatan peninsula, Mexico. Appl. Soil Ecol. 165:103969. doi: 10.1016/j.apsoil.2021.103969
Schneider, N., and Eugster, W. (2007). Climatic impacts of historical wetland drainage in Switzerland. Clim. Change 80, 301–321. doi: 10.1007/s10584-006-9120-8
Stürmer, S., Bever, J., and Morton, J. (2018). Biogeography of arbuscular mycorrhizal fungi (Glomeromycota): a phylogenetic perspective on species distribution patterns. Mycorrhiza 28, 587–603. doi: 10.1007/s00572-018-0864-6
Tian, J., Liu, L., Chen, H., Zhong, L., Zhou, X., Jiang, L., et al. (2022). Aerobic environments in combination with substrate additions to soil significantly reshape depth-dependent microbial distribution patterns in Zoige peatlands, China. Appl. Soil Ecol. 170:104252. doi: 10.1016/j.apsoil.2021.104252
Treseder, K. K., and Lennon, J. T. (2015). Fungal Traits That Drive Ecosystem Dynamics on Land. Microbiol. Mol. Biol. Rev. 79, 243–262. doi: 10.1128/MMBR.00001-15
Wang, J., Zheng, Y., Hu, H., Zhang, L., Li, J., and He, J. (2015). Soil pH determines the alpha diversity but not beta diversity of soil fungal community along altitude in a typical Tibetan forest ecosystem. J. Soils Sedim. 15, 1224–1232. doi: 10.1007/s11368-015-1070-1
Wang, S., Cheng, J., Li, T., and Liao, Y. (2020). Response of soil fungal communities to continuous cropping of flue-cured tobacco. Sci. Rep. 10:19911 doi: 10.1038/s41598-020-77044-8
Wiltermuth, M., and Anteau, M. (2016). Is consolidation drainage an indirect mechanism for increased abundance of cattail in northern prairie wetlands? Wetlands Ecol. Manag. 24, 533–544. doi: 10.1007/s11273-016-9485-z
Wu, X., Yang, J., Ruan, H., Wang, S., Yang, Y., Naeem, I., et al. (2021). The diversity and co-occurrence network of soil bacterial and fungal communities and their implications for a new indicator of grassland degradation. Ecol. Indic. 129:107989. doi: 10.1016/j.ecolind.2021.107989
Xiao, R., Guo, Y., Zhang, M., Pan, W., and Wang, J. J. (2020). Stronger network connectivity with lower diversity of soil fungal community was presented in coastal marshes after sixteen years of freshwater restoration. Sci. Total Environ. 744:140623. doi: 10.1016/j.scitotenv.2020.140623
Xie, F., Ma, A., Zhou, H., Liang, Y., Yin, J., Ma, K., et al. (2020). Revealing Fungal Communities in Alpine Wetlands through Species Diversity, Functional Diversity and Ecological Network Diversity. Microorganisms 8:632 doi: 10.3390/microorganisms8050632
Xu, F., Cai, T., Yang, X., and Sui, W. (2017). Soil fungal community variation by large-scale reclamation in Sanjiang plain, China. Ann. Microbiol. 67, 679–689. doi: 10.1007/s13213-017-1296-9
Xue, D., Chen, H., Zhan, W., Huang, X., and Liu, J. (2020). How do water table drawdown, duration of drainage and warming influence greenhouse gas emissions from drained peatlands of the Zoige Plateau? Land Degradat. Dev. 32, 3351–3364. doi: 10.1002/ldr.4013
Zedler, J. B. (2000). Progress in wetland restoration ecology. Trends Ecol. Evol. 15, 402–407. doi: 10.1016/s0169-5347(00)01959-5
Zhang, H., Yao, Z., Ma, L., Zheng, X., Wang, R., Wang, K., et al. (2019). Annual methane emissions from degraded alpine wetlands in the eastern Tibetan Plateau. Sci. Total Environ. 657, 1323–1333. doi: 10.1016/j.scitotenv.2018.11.443
Zhang, N., and Wang, Z. (2015). “3 Pezizomycotina: Sordariomycetes and Leotiomycetes,” in Systematics and Evolution. The Mycota (A Comprehensive Treatise on Fungi as Experimental Systems for Basic and Applied Research), eds D. McLaughlin and J. Spatafora (Berlin: Springer), doi: 10.1007/978-3-662-46011-5_3
Zhao, H., Li, X., Zhang, Z., Zhao, Y., Yang, J., and Zhu, Y. (2017). Species diversity and drivers of arbuscular mycorrhizal fungal communities in a semi-arid mountain in China. PeerJ 5:e4155. doi: 10.7717/peerj.4155
Zhao, Q., Bai, J., Gao, Y., Zhao, H., Zhang, G., and Cui, B. (2020). Shifts in the soil bacterial community along a salinity gradient in the Yellow River Delta. Land Degrad. Develop. 31, 2255–2267. doi: 10.1002/ldr.3594
Keywords: wetland drainage, fungal community, diversity, community structure, freshwater wetlands
Citation: Zhao Q, Bai J, Jia J, Zhang G, Wang J and Gao Y (2022) The Effects of Drainage on the Soil Fungal Community in Freshwater Wetlands. Front. Ecol. Evol. 10:837747. doi: 10.3389/fevo.2022.837747
Received: 17 December 2021; Accepted: 07 March 2022;
Published: 25 May 2022.
Edited by:
Haitao Wu, Northeast Institute of Geography and Agroecology (CAS), ChinaReviewed by:
Fei-Hai Yu, Taizhou University, ChinaMingxiang Zhang, Beijing Forestry University, China
Copyright © 2022 Zhao, Bai, Jia, Zhang, Wang and Gao. This is an open-access article distributed under the terms of the Creative Commons Attribution License (CC BY). The use, distribution or reproduction in other forums is permitted, provided the original author(s) and the copyright owner(s) are credited and that the original publication in this journal is cited, in accordance with accepted academic practice. No use, distribution or reproduction is permitted which does not comply with these terms.
*Correspondence: Qingqing Zhao, qingqingzhao@qlu.edu.cn; Jia Jia, jiajiast@126.com