- 1Department of Biology, Hofstra University, Hempstead, NY, United States
- 2Department of Biological Sciences, Rutgers University Newark, Newark, NJ, United States
Wood turtles (Glyptemys insculpta) have been suffering range-wide population declines since the 1900s. Most monitoring efforts of these turtles involve population surveys to assess population size and viability but relatively few investigate rates of reproductive success. We collected four consecutive years (2013–2016) of wood turtle nesting data at a nesting site in northwestern New Jersey; population-level hatching success was unusually low. Furthermore, annual, intra-individual hatch rates and comparisons between natural and artificial incubation revealed that approximately half of all females usually produced clutches with low (<50%) hatch rates, regardless of incubation conditions. In contrast, the annual hatch rates of other females were either consistently high (>50%) or highly variable, ranging from 0 to 100%. Thus, some adult females are potentially making much larger contributions to the next generation than others. A repeatability analysis suggested that approximately 60% of the hatch rate variability observed in this population can be attributed to maternal identity. The remaining 40% may be attributed to the random environmental factors that are often theorized to be potential reasons for reduced hatch rates in turtle populations (e.g., unsuitable incubation conditions, flooding, desiccation, egg infertility, egg damage due to improper handling by researchers, root and insect predation, and microbial infection). The ultimate causes of this population’s hatching success variability are uncertain, but maternally-linked hatching failure in turtle populations could be associated with inbreeding, infertility, senescence, inadequate maternal diets, or environmental contamination. This study indicates that commonly suggested hypotheses for hatching failure, such as unsuitable incubation conditions or infertility, are unlikely to explain all of the hatch rate variability in some turtle populations. This study also reveals a cryptic conservation implication for vulnerable turtle populations: that the presence of many nesting females and nests does not necessarily assure high or even sustainable reproductive rates. When coupled with the high rates of nest predation and low juvenile survival rates that are common in most turtle populations, the exceedingly low hatch rates observed in this population suggest that recruitment in some turtle populations could be severely hindered even when nests are protected in the field or incubated in laboratory settings.
Introduction
In oviparous reptiles without parental care, the causes of nest failure and low hatching success are usually attributed to extrinsic (i.e., environmental) and/or intrinsic (i.e., genetic and non-genetic parental) factors (Bell et al., 2003; Rafferty et al., 2011; Noel et al., 2012). Extrinsic factors such as predation (e.g., Fowler, 1979; Congdon et al., 1983; Leslie et al., 1996; Kolbe and Janzen, 2002; Feinberg and Burke, 2003; Horne et al., 2003) and inundation by rising waters (e.g., Plummer, 1976; Leslie et al., 1996; Standing et al., 1999; Horne et al., 2003; Doody et al., 2004; Spradling et al., 2010; Pike et al., 2015) are major causes of visible nest failure and reduced hatching success in wild turtle populations. In studies involving protected or artificially-incubated nests, less conspicuous extrinsic factors, such as unfavorable thermal and hydric conditions, are often recognized as the primary reasons for reduced hatch rates (e.g., Packard et al., 1987; Spotila et al., 1994; Wilson, 1998; Standing et al., 1999; Congdon et al., 2000; Demuth, 2001). Even less perceptible than unfavorable incubation conditions are the various intrinsic reasons for nest failure and low hatching success; these include egg infertility (Blanck and Sawyer, 1981; Whitmore and Dutton, 1985; Wyneken et al., 1988), developmental failures attributed to maternal effects (i.e., non-genetic maternal influences on offspring phenotype; Wolf and Wade, 2009; Rafferty et al., 2011), and inbreeding depression (Ennen et al., 2010; Noel et al., 2012). Such factors are often overlooked, improperly diagnosed, and, for these reasons, poorly understood in wild reptile populations (Ewert, 1979).
Determining the true causes of hatching failure is vital to understanding reptile life histories and the development of conservation and management strategies for rare species, but making such determinations is difficult when the causes of hatching failure cannot be clearly linked to obvious extrinsic factors (e.g., predation, fungal infection, flooding, desiccation, unfavorable incubation temperatures, etc.). Intrinsic factors are almost always difficult to implicate as drivers of low hatch rates, especially in wild populations, but studying the inter- and intra-individual hatch rate variation within a population can provide insight into why some nests fail while others succeed (Bell et al., 2003; Wallace et al., 2007; Rafferty et al., 2011). To determine whether the reasons for hatching failure are primarily extrinsic or intrinsic, environmental/incubation conditions of nests and the annual hatch rates of multiple individual females can be investigated through multiple nesting seasons. Additionally, estimates of hatch rate repeatability can be compared among populations and used to formulate testable hypotheses for low hatch rates.
While overall, population-level hatch rates are often reported for multi-year turtle nesting studies, the variation among and within individuals is usually disregarded or simply not known (e.g., Congdon et al., 1983; Christens and Bider, 1987; Bobyn and Brooks, 1994; Standing et al., 1999; Horne et al., 2003; Litzgus and Mousseau, 2006; Walde et al., 2007; but see Buhlmann and Osborn, 2011). For any quantitative parameter (e.g., clutch size, hatching success, body mass, etc.), the total amount of variation that exists in a population is the sum of the parameter’s inter- and intra-individual variation within the population. The relative amount of inter-individual variation alone, is known as repeatability (Wolak et al., 2012). Estimates of repeatability are useful for assessing the reliability of repeated measurements on more than one individual, as well as the consistency of individual performance seen in a group of individuals after multiple observations (Lessells and Boag, 1987). Additionally, repeatability estimates can be used to differentiate between the effects of individual identity and random environmental factors on quantitative parameters such as clutch size and hatch rates (Van Noordijk et al., 1980). Using nesting turtles as an example, higher repeatability estimates (i.e., closer to 1.0) indicate that more of the observed variation in hatching success is attributable to maternal identity, while lower repeatability estimates (i.e., closer to 0) indicate that more of the observed variation is attributable to random environmental factors that are independent of the mother. Because repeatability tests estimate a parameter’s intra- and inter-individual variation, estimates of repeatability can only be obtained from repeated measurements on multiple individuals of known identity (Wolak et al., 2012). Consequently, obtaining repeatability estimates that pertain to the reproductive variability of turtle populations can be challenging because this requires collecting repetitive nesting data on the same individuals over multiple nesting runs or nesting seasons.
Wood turtles (Glyptemys insculpta) offer opportunities for obtaining hatch rate repeatability estimates and studying the causes of nest failure and low hatching success. Adults of this species are easily radio-tracked and females in some populations exhibit strong nesting site fidelity for easily monitored nesting sites that attract many turtles each year; therefore, several consecutive years of nesting data can be collected for individual females and both intra- and inter-individual variations in hatch rates can be observed.
Wood turtles currently face numerous conservation concerns; therefore, studies gathering critical details on wood turtle reproduction can inform strategies for their conservation and management. van Dijk and Harding (2013) estimated that wood turtles have experienced a range-wide population decline of more than 50% in the last century, warranting the current IUCN listing of “Endangered.” Wood turtles have a geographic range coinciding with the highly developed northeastern region of the U.S., the upper Midwest U.S., and neighboring regions in Canada (Ernst and Lovich, 2009) and a unique habitat usage cycle that makes them susceptible to anthropogenic population decline and extirpation. From late fall to early spring, wood turtles use rivers and streams as breeding habitats and refuges from freezing temperatures. During warmer months, they disperse away from riparian corridors to a variety of habitats, including upland fields and forests and low-lying fens and meadows. These diverse habitat preferences and unique habitat-usage cycles increase the likelihood that wood turtle populations will be negatively impacted by habitat degradation, fragmentation, and destruction (Jones and Willey, 2015); automobiles (Gibbs and Shriver, 2002; Steen et al., 2006; pers. obs.) and agricultural machinery (Saumure et al., 2006; Tingley et al., 2009; pers. obs.); subsidized predators (Brooks et al., 1992; Buhlmann and Osborn, 2011); and illegal collection for the live animal trade (Garber and Burger, 1995; McCollough, 1997; Levell, 2000). In addition to anthropogenic pressures, most wood turtle populations experience exceptionally low recruitment due to low reproductive frequencies (i.e., on average females typically lay one or less than one clutch per year; Jones and Willey, 2015), lack of suitable nesting habitats (Buech et al., 1997), nest predation (Brooks et al., 1992; Buhlmann and Osborn, 2011), and high juvenile mortality (Paterson et al., 2012, 2014; Dragon, 2014). A high degree of inter-population variation in overall hatching success has also been documented in wood turtles, with some populations experiencing much lower hatch rates than others (Table 1).
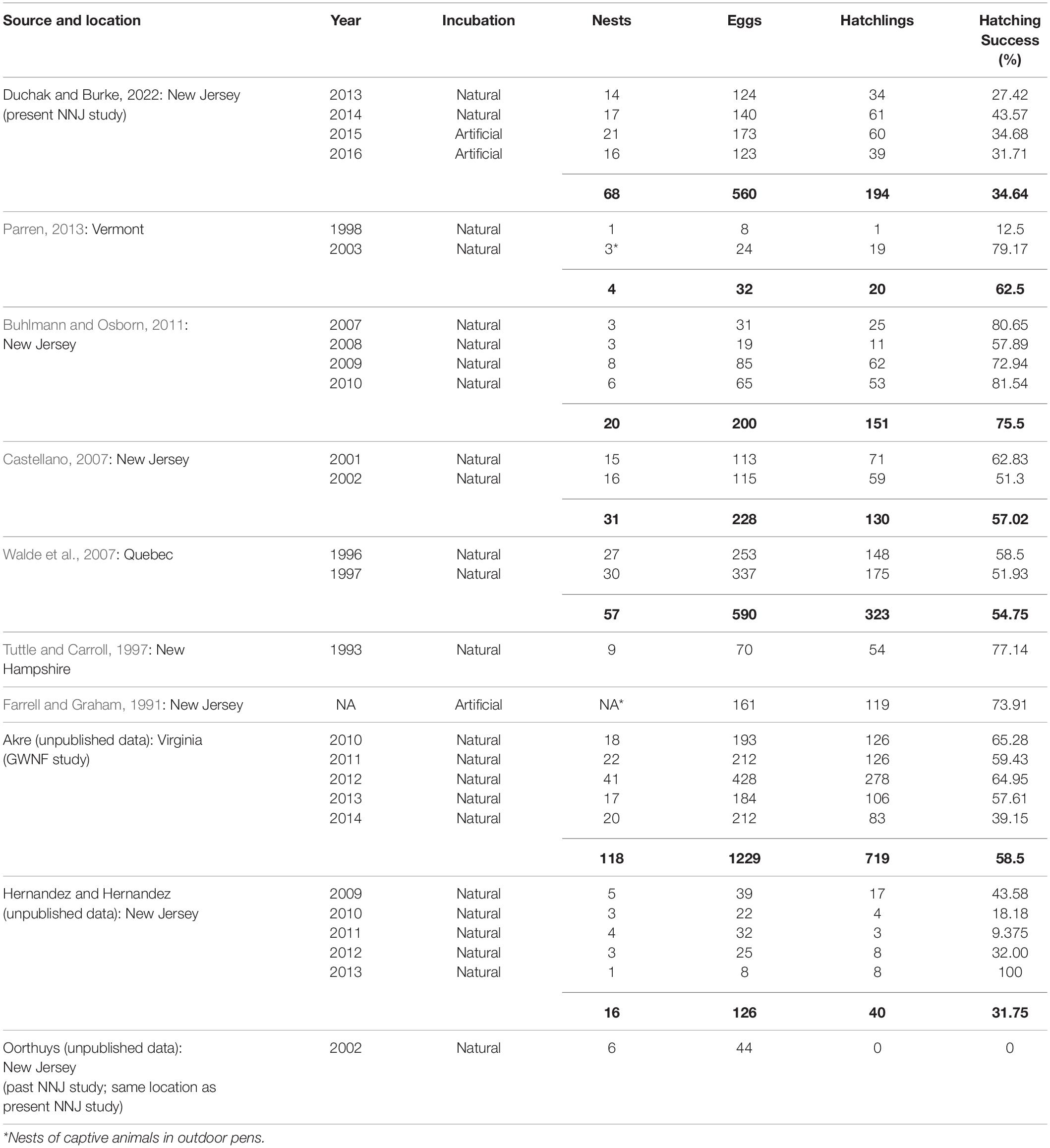
Table 1. Annual overall hatching success of naturally and artificially-incubated wood turtle (Glyptemys insculpta) nests in 10 nesting studies (excluding depredated nests). Overall hatching success and sums of nests, eggs, and hatchlings are shown in bold for studies that reported multi-year data.
In this study, our goal was to investigate the annual inter- and intra-individual hatch rate variation in wood turtle populations with different degrees of hatching success and to determine whether the hatch rates observed in these populations are primarily influenced by extrinsic or intrinsic factors. If annual hatch rates of individual females yielded relatively low repeatability estimates and improved under carefully monitored and controlled, artificial incubation conditions, then the hatch rates we observed were primarily influenced by extrinsic factors such as unfavorable thermal and hydric conditions. Alternatively, if annual hatch rates of individual females yielded relatively high repeatability estimates and remained relatively constant between natural and artificial incubation conditions, then the hatch rates we observed were primarily influenced by intrinsic factors and warrant further investigation.
Materials and Methods
Nesting Site
We conducted most of this study at a turtle nesting site in a relatively undisturbed area of northern New Jersey (NNJ), with no known history of unusual chemical use or pollution. To protect this population from illegal collection, we refrain from disclosing this site’s exact location and descriptive details of its surroundings. During the mid-1900s, the site was deforested and excavated for cobble, leaving a large patch of exposed, glacial till-laden sediment that has attracted nesting wood turtles, common snapping turtles (Chelydra serpentina) and painted turtles (Chrysemys picta) since at least the late 1990s. Prior to the 2013 nesting season, we remediated the site to facilitate turtle nesting and improve our ability to observe turtles from a distance. First, we tilled the entire nesting site to soften the ground and remove vegetation. Then, we excavated two 80 m2 rectangular patches of the site’s original, cobble-laden sediment and replaced them with sand from a nearby, eroding hillside. To minimize site disturbance, we left much of the site’s original sediment in place, even though the cobble within it frequently precludes females from digging suitable nest cavities. In subsequent years, we repeated pre-nesting season tilling operations to delay succession and keep soft sediments exposed.
Nesting Site Monitoring
During the nesting seasons (i.e., 20 May–20 June) from 2013 to 2016, we monitored the nesting area for female wood turtles every morning from 6:00 to 12:00 and every evening from 16:00 to 21:00. We observed the area from a distance with binoculars or from a hunting blind to avoid disturbing nesting females. We often continued nesting site monitoring beyond these time intervals until all wood turtles either finished nesting or retreated into the vegetation surrounding the site. We retrieved all females immediately after staging or nesting behavior concluded to verify identity (i.e., notch code) and, if oviposition occurred, pinpoint nest locations.
This nesting study was part of a long-term mark-recapture study of the surrounding area’s wood turtle population that we have been conducting since 1998. Throughout the study, we gave unique notch codes to most of the adult population and radio-tracked more than 20 adult turtles. Furthermore, an unpublished 2002 study conducted at the same nesting area revealed an exceedingly low overall hatch rate of 0% for six nests that were naturally-incubated in situ (i.e., where oviposited) and protected from predation with hardware cloth caging (Oorthuys, unpublished data; Table 1).
Egg Handling, Incubation, and Hatching
In 2013 and 2014, we incubated all nests in situ (i.e., where oviposited) under natural conditions and protected them from predation with hardware cloth caging; this type of predator excluder is unlikely to affect nest temperatures (Riley and Litzgus, 2013; Burke et al., unpublished data). Immediately after oviposition and prior to installing predator excluders, we carefully excavated all nests by hand, recorded clutch sizes, and promptly returned all eggs to their nest cavities. To ensure that all nests received similar treatment and to limit the possibility of embryonic mortality due to careless handling, we took great care when excavating and handling each egg; throughout the entire study, no eggs were handled or moved 6 h past oviposition, and no eggs were jarred, shaken, inverted, dropped, or visibly damaged in any way. Before reburying each nest, we programmed temperature loggers (Thermochron® iButtons) for hourly readings, sealed them in duct tape to waterproof them, and placed them among the eggs of each nest to record natural incubation temperatures.
During the 2013 and 2014 hatching seasons (1–20 August), we checked nest cages for hatchlings at least three times daily in the morning, afternoon, and evening to document hatchling emergence and prevent hatchling mortality from intense sun exposure. Since the objectives of this study were primarily concerned with hatch rates, recording hatching success (i.e., the percentage of hatchlings that hatched from each nest) took precedence over recording emergence success (i.e., the percentage of hatchlings that emerged from each nest). To obtain accurate records of hatching success, we excavated nests one day after hatchling emergence to recover unhatched eggs as well as any live hatchlings that failed to emerge.
In 2015 and 2016, we carefully excavated all nests by hand immediately after oviposition, recorded clutch sizes, and transported all clutches to a nearby laboratory where we incubated them at a constant temperature of 30°C. To prepare clutches for incubation and for transport from the nesting site to the laboratory, we equidistantly spaced the eggs of each clutch on beds of moistened vermiculite within separate “incubation containers” (i.e., plastic food storage containers). As in the previous years of this study (2013 and 2014), we took great care when excavating and handling all eggs. We also took great care when transporting all clutches via motor vehicle; transportation time never exceeded 15 min, and we held all incubation containers aloft to reduce vibrations during transport.
The care we took in excavating, handling, and transporting eggs may have been excessive as we always finished moving eggs within 6 h of oviposition. Although the sensitivity of turtle eggs to mechanical disturbance is poorly known for most species (Booth, 2004), it is generally accepted that inversion during the first few hours after oviposition and even during the first 12 h of incubation is unlikely to negatively affect embryonic survival in most species (Deeming, 1991). Furthermore, after inverting a total of 22 eggs belonging to four different species during the second to fourth day of incubation, Ewert (1979) reported both hatching success and failure in unspecified numbers of eggs, thus demonstrating that egg inversions do not always result in embryonic mortality, even when inversions occur days after oviposition. Additionally, Ewert (1979) also mentioned that eggs are rather tolerant of the vibrations they experience in natural nests positioned along active railroad tracks and during transportation in vehicles on rough roads. Lastly, a study of painted turtle (C. picta) nests found no difference in hatching success between eggs that were left completely undisturbed in natural nests throughout incubation and eggs that were excavated, transported via motor vehicle for an hour, cleaned, weighed, measured, and reburied within 24 h of oviposition (Samson et al., 2007). Despite all of the information suggesting that freshly laid eggs are relatively tolerant of moderate mechanical disturbances, we still handled all eggs with the utmost level of care to ensure that improper handling did not affect hatching success.
Two weeks after collecting the final clutches in 2015 and 2016, we visually examined all eggs for signs of “chalking” (i.e., eggshell whitening). To provide an initial conservative estimate of fertility, we divided the number of eggs that showed evidence of chalking by the number of eggs that showed no evidence of chalking. Chalking in turtle eggs has long been regarded as direct evidence of fertilization and subsequent embryonic development (Ewert, 1979, 1985; Deeming, 1991); however, the absence of chalking is an unreliable indicator of egg infertility because external examinations of eggs cannot distinguish between true infertility and early embryonic mortality (Croyle et al., 2016). Consequently, while external examinations of eggs may confirm fertility in eggs that show clear signs of development, they cannot confirm infertility in eggs that show no evidence of development.
We determined hatching success for each nest by dividing the number of pipped live hatchlings by the clutch size. Similarly, we determined overall hatching success for each year by dividing the total number of pipped live hatchlings counted in all nests during a given year by the total number of eggs counted in all nests during the same year. We released all hatchlings at their respective nest sites immediately after hatching/emergence (naturally-incubated nests from 2013 to 2014) or as soon as they absorbed their external yolk sacs (lab-incubated nests from 2015 to 2016).
Comparison Data
We obtained hatching success data from a multi-year wood turtle nesting study conducted within George Washington National Forest (GWNF) in northern Virginia. GWNF is the southern limit of the wood turtle’s range and is approximately 400 km southwest of the NNJ site. The GWNF dataset contained hatching success data from 118 nests laid by 55 females over five consecutive nesting seasons (2010–2014). All nests included in the GWNF dataset were incubated in situ under natural conditions and either unpredated or protected from predation with hardware cloth caging (Akre, unpublished data).
Data Analysis
To determine whether the NNJ hatch rates were different among years, we used a Kruskal Wallis test to compare the individual hatch rates of all nests from 2013 to 2016. To determine whether the NNJ hatch rates of eggs that were naturally-incubated in situ were different from the NNJ hatch rates of eggs that were artificially-incubated in the laboratory, we combined the hatch rates from 2013 to 2014 and compared them with the combined hatch rates of 2015 and 2016 using a two-tailed Mann–Whitney U test. We conducted this analysis once for all nests, then again including only “repeat nesters” (i.e., females who had more than one annual nest represented in the data) to determine whether the presence of multiple “single nesters” (i.e., females who only had one nest represented in the data) during some years influenced overall annual hatch rates.
To determine whether hatch rates were different between the NNJ and GWNF populations, we converted the overall hatch rates of all repeat nesters into counts in four categories: 0–24.99, 25–49.99, 50–74.99, and 75–100%. We then ran a Freeman-Halton extension of the Fisher’s exact probability test for a two-rows by four-columns contingency table comparing the overall hatch rates of repeat nesters between the NNJ and GWNF populations. To determine whether the overall NNJ hatch rates of repeat nesters were significantly lower than the overall GWNF hatch rates of repeat nesters, we used a two-tailed Mann–Whitney U test.
To determine the proportion of reproductive variability that is due to inter-individual variation within each population, we estimated hatching success repeatability for all repeat nesters in the NNJ and GWNF populations with the following equations from Wolak et al. (2012):
where MSgroups = mean sum of squares for groups in a random effects (Model 2) ANOVA and MSerror = mean sum of squares for error in a random effects (Model 2) ANOVA.
We obtained repeatability estimates and their associated 95% confidence intervals using the R package “ICC” developed by Wolak et al. (2012).
To investigate the possible effects of body size on hatching success in the NNJ population, we conducted a linear regression for each repeat nester’s straight line plastron length (SLPL) versus each repeat nester’s overall hatch rate. We also conducted linear regressions for each repeat nester’s SLPL and each repeat nester’s mean and maximum clutch sizes to determine whether body size was correlated with clutch size in the NNJ population. Lastly, to investigate the possible effects of clutch size on hatching success in the NNJ and GWNF populations, we conducted linear regressions for each repeat nester’s mean and maximum clutch sizes versus each repeat nester’s overall hatch rate.
Results
From 2013 to 2016, we recorded the hatch rates of 68 nests laid by 27 females at the NNJ site. The annual overall hatching success rates for 2013, 2014, 2015, and 2016 were 27.42, 43.57, 34.68, and 31.71%, respectively, and the hatching success rate for all 4 years combined was 34.64% (Table 1). We found that 70.92% of all NNJ eggs collected for incubation in 2015 and 2016 showed evidence of chalking. At the GWNF site, the annual, overall hatching success rates for 2010, 2011, 2012, 2013, and 2014 were 65.28, 59.43, 64.95, 57.61, and 39.15%, respectively, the overall hatching success rate for all 5 years combined was 58.5% (Akre, unpublished data; Table 1).
We found no significant differences among the 2013, 2014, 2015, and 2016 NNJ hatch rates (Kruskal Wallis test: H = 1.56, DF = 3, P = 0.716). Additionally, we found no significant difference between the hatch rates of all nests that were naturally-incubated in situ during 2013 and 2014 (N females = 19, N nests = 31, mean = 0.32) and the hatch rates of all nests that were artificially-incubated in the laboratory during 2015 and 2016 (N females = 25, N nests = 37, mean = 0.33; two-tailed Mann–Whitney U test: UA = 563.5, Z = 0.12, P = 0.9045). Similarly, we found no significant difference between the “natural” (N females = 17, N nests = 29, mean = 0.34) and “artificial” hatch rates (N females = 18, N nests = 31, mean = 0.38) of all repeat nests at the NNJ site (two-tailed Mann–Whitney U test: UA = 411.5, Z = 0.35, P = 0.7263).
Throughout the entire four-year NNJ study (2013–2016), 27.78% of repeat nesters failed to produce more than 1 hatchling, 38.89% produced at least 10 hatchlings, and 22.22% produced at least 20 hatchlings (Figure 1). Throughout the entire five-year GWNF study (2010–2014), all repeat nesters produced at least 3 hatchlings, 86.11% produced at least 10 hatchlings, and 33.33% produced at least 20 hatchlings (Figure 2). Fifty percent of all repeat nesters from NNJ had overall hatching success rates between 0 and 24.99%, 5.56% between 25 and 49.99%, 27.78% between 50 and 74.99%, and 16.67% between 75 and 100%. Approximately six percent of GWNF repeat nesters had overall hatching success rates between 0 and 24.99%, 27.78% between 24 and 49.99%, 38.89% between 50 and 74.99%, and 27.78% between 75 and 100%. We found that the overall NNJ hatch rates of repeat nesters (N females = 18, N nests = 59, mean = 0.37) were significantly different (Fisher’s exact probability test: DF = 4, P = 0.00466) and lower (two-tailed Mann–Whitney U test: UA = 194.5, Z = 2.37, P = 0.0178) than the overall GWNF hatch rates of repeat nesters (N females = 36, N nests = 99, mean = 0.60; Figure 3). Additionally, 33.33% of repeat nesters from NNJ always had annual hatch rates of 50% or greater, 22.22% had annual hatch rates that varied from less than 50 to 50% or greater, and 44.44% consistently produced hatch rates below 50%, whereas 36.11% of repeat nesters from GWNF always had annual hatch rates of 50% or greater, 58.33% had annual hatch rates that varied from less than 50 to 50% or greater, and only 5.56% consistently produced hatch rates below 50% (Figure 4).
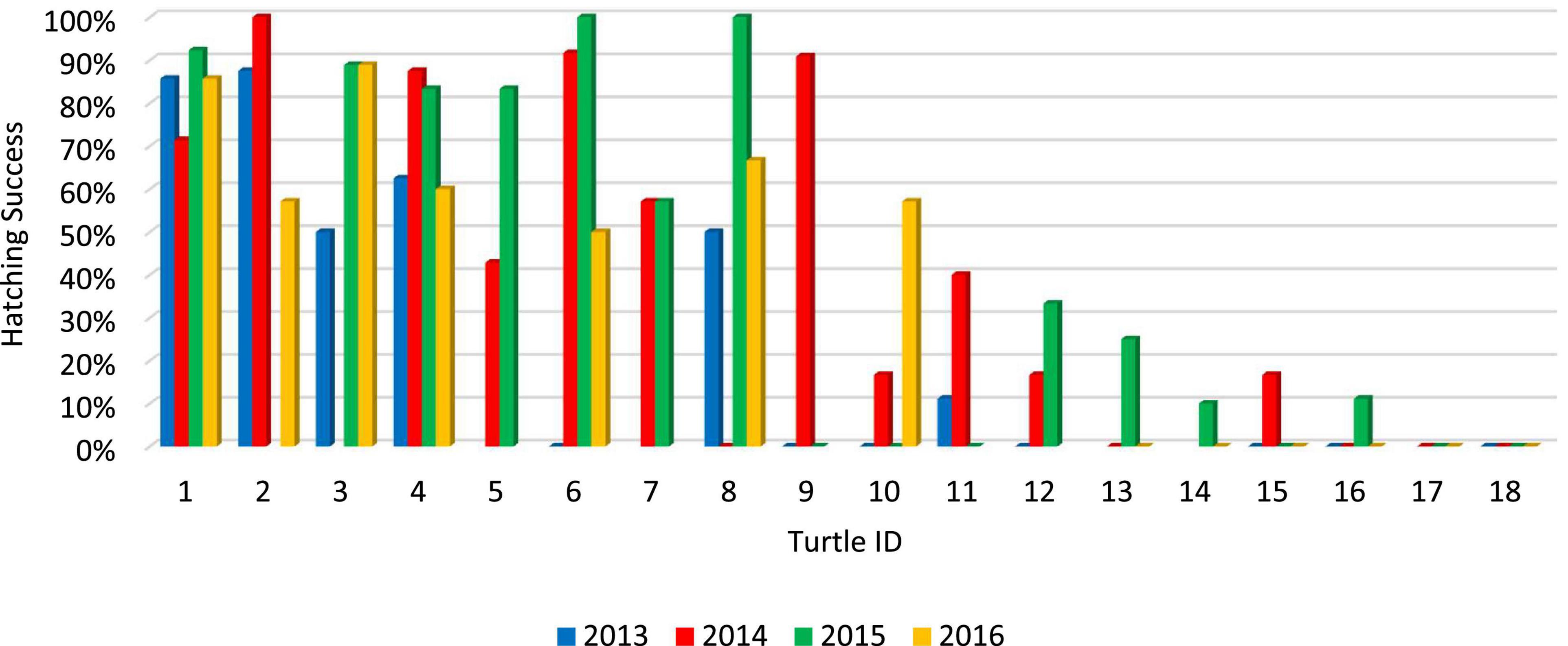
Figure 1. Annual hatching success rates of all “repeat nester” female wood turtles (Glyptemys insculpta) at the northern New Jersey (NNJ) site from 2013 to 2016. Individual females are ranked from 1 to 18 according to their average hatching success rates.
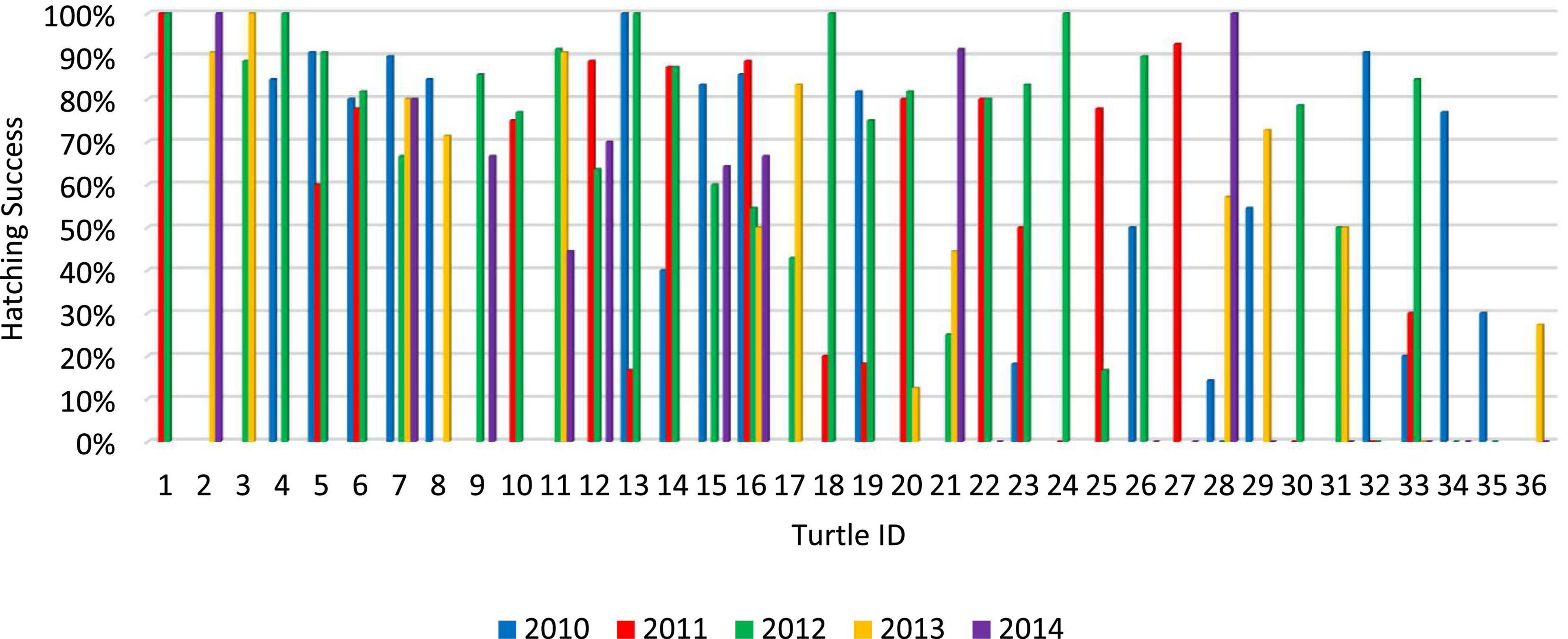
Figure 2. Annual hatching success rates of all “repeat nester” female wood turtles (Glyptemys insculpta) at the George Washington National Forest (GWNF) site from 2010 to 2014. Individual females are ranked from 1 to 36 according to their average hatching success rates.
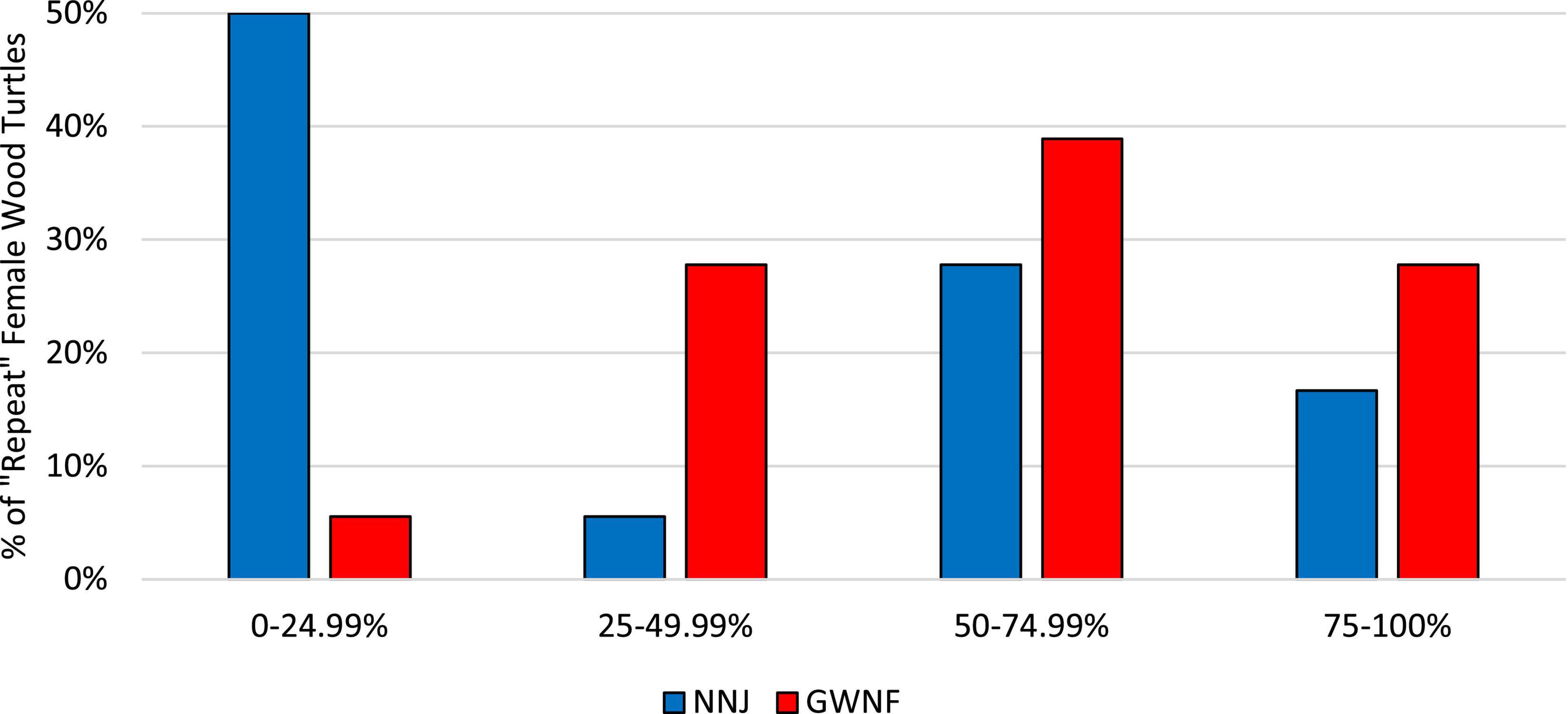
Figure 3. Percentages of “repeat nester” wood turtles (Glyptemys insculpta) with overall hatching success rates between 0 and 24.99%, 25 and 49.99%, 50 and 74.99%, 75 and 100% in the northern New Jersey (NNJ) and George Washington National Forest (GWNF) studies. The overall NNJ hatch rates of repeat nesters (N females = 18, N nests = 59, mean = 0.37) were significantly different (Fisher’s exact probability test: DF = 4, P = 0.00466) and lower (two-tailed Mann–Whitney U test: UA = 194.5, Z = 2.37, P = 0.0178) than the overall GWNF hatch rates of repeat nesters (N females = 36, N nests = 99, mean = 0.60).
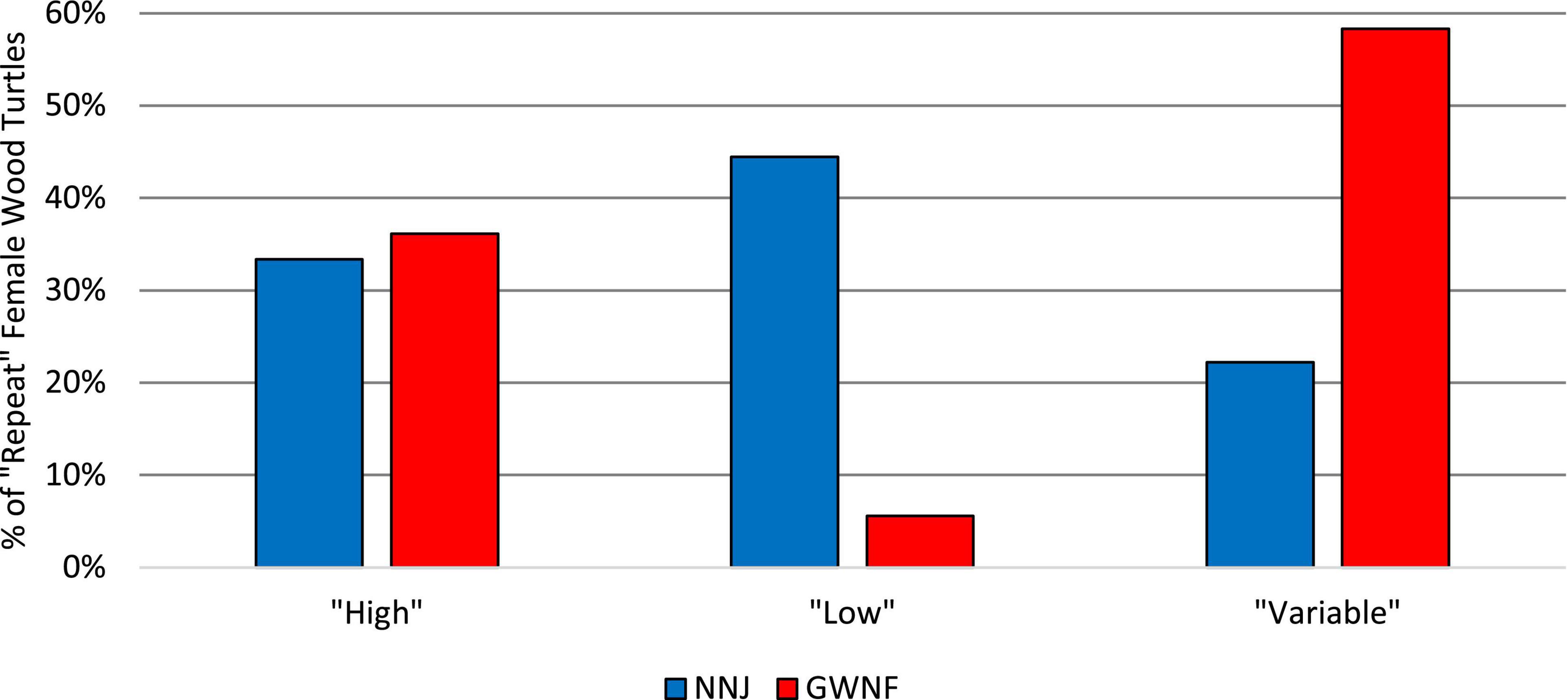
Figure 4. Proportions of “high hatch rate,” “low hatch rate,” and “variable hatch rate,” “repeat nester” wood turtles (Glyptemys insculpta) in the northern New Jersey (NNJ) and George Washington National Forest (GWNF) studies. “High hatch rate females” always had annual hatch rates of 50% or greater, “variable hatch rate females” had annual hatch rates that varied from less than 50 to 50% or greater, and “low hatch rate females” consistently produced hatch rates below 50%.
Our estimates of hatching success repeatability from the NNJ and GWNF datasets were 0.580 (N = 18; K = 3.268; lower CI: 0.328; upper CI: 0.792; CIW: 0.464) and 0.056 (N = 36; K = 2.771; lower CI: −0.162; upper CI: 0.274; CIW: 0.436), respectively. The 95% confidence intervals about our estimates do not overlap, indicating that the NNJ and GWNF hatching success repeatability estimates are significantly different from each other (Figure 5).
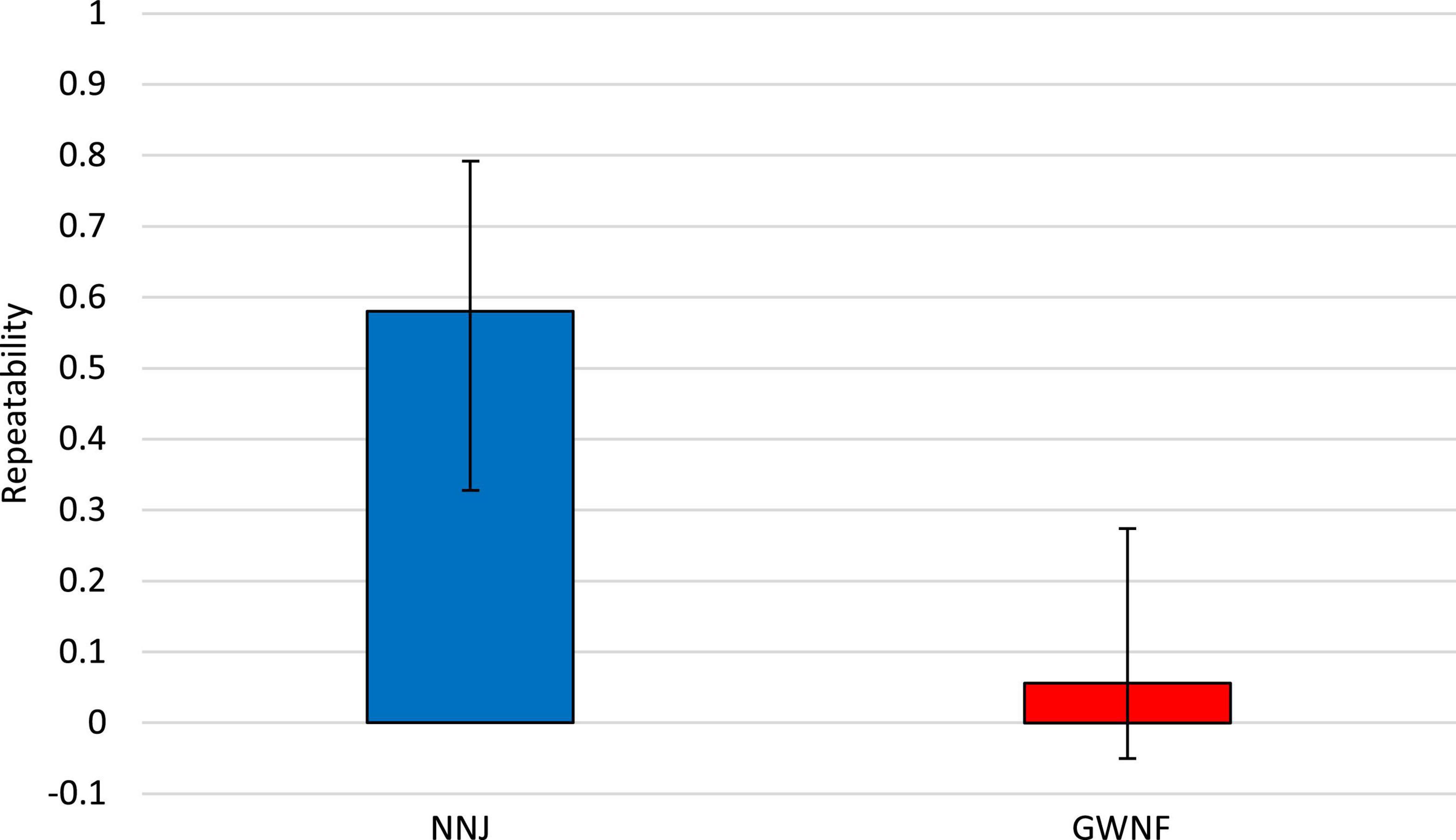
Figure 5. Wood turtle (Glyptemys insculpta) hatching success repeatability estimates with corresponding 95% confidence intervals for the northern New Jersey (NNJ) and George Washington National Forest (GWNF) studies. Estimates of hatching success repeatability from the NNJ and GWNF datasets were 0.580 (N = 18; K = 3.268; lower CI: 0.328; upper CI: 0.792; CIW: 0.464) and 0.056 (N = 36; K = 2.771; lower CI: -0.162; upper CI: 0.274; CIW: 0.436), respectively.
Linear regression revealed no significant association between SLPL and overall hatch rates among repeat nesters in the NNJ population (T = 0.889, DF = 16, R2 = 0.217, P = 0.3871); however, significant associations between SLPL and both mean (T = 3.782, DF = 16, R2 = 0.472, P = 0.0016) and maximum clutch sizes (T = 4.068, DF = 16, R2 = 0.508, P = 0.0009) were found among repeat nesters in the NNJ population. No significant effects of either mean (T = 0.062, DF = 16, R2 = 0, P = 0.9517) or maximum clutch sizes (T = -0.896, DF = 16, R2 = 0.048, P = 0.3836) were found on the overall hatch rates of repeat nesters in the NNJ population. Likewise, no significant effects of either mean (T = -0.304, DF = 34, R2 = 0.003, P = 0.7633) or maximum clutch sizes (T = -0.267, DF = 34, R2 = 0.002, P = 0.7913) were found on the overall hatch rates of repeat nesters in the GWNF population.
Discussion
In this study, our goal was to investigate the annual inter- and intra-individual hatch rate variation in wood turtle populations with different degrees of hatching success and to determine whether the hatch rates observed in these populations are primarily influenced by extrinsic or intrinsic factors. We initially revealed potential reproductive issues at the NNJ site when we found the population’s 2013 and 2014 hatch rates to be considerably lower than the hatch rates reported for seven other wood turtle populations (Table 1). As an initial attempt to increase hatching success and determine the cause of the low NNJ hatch rates, we artificially-incubated all NNJ clutches under identical conditions during 2015 and 2016. When extrinsic factors like predation, microbial infection, flooding, desiccation, and unfavorable incubation conditions are primarily responsible for low hatch rates in wild turtle populations, carefully-monitored and controlled, artificial incubation should improve overall hatching success rates and the hatching success rates of individual females (Noel et al., 2012); however, we found that the NNJ hatch rates of nests that were naturally-incubated in situ (2013–2014) were similar to the NNJ hatch rates of nests that were artificially-incubated in the laboratory (2015–2016). This indicates that unknown intrinsic factors were reducing the hatch rates of this population. Additionally, we found the overall NNJ hatch rates of repeat nesters to be significantly lower than the overall GWNF hatch rates of repeat nesters (Table 1), indicating that the unknown factors that reduced the reproductive potential of the NNJ population are not present in all wood turtle populations.
Our NNJ hatching success repeatability estimate indicated that approximately 60% of the observed variation in hatching success is attributed to maternal identity while the remaining 40% is attributed to random environmental factors. In contrast, our GWNF hatching success repeatability estimate was significantly lower than the NNJ estimate and indicated that approximately 5% of the observed variation in hatching success is attributed to maternal identity while the remaining 95% is attributed to random environmental factors. This low range repeatability estimate suggests that environmental factors influence hatching success variation more than maternal identity in GWNF (Figure 5). The higher NNJ hatching success repeatability estimate is certainly due to the many repeat nesters who consistently produced low hatch rates and the relatively smaller numbers of repeat nesters that produced either consistently high hatch rates or hatch rates that varied from year to year. Forty-four percent of repeat nesters in the NNJ study never had a clutch with a hatch rate of 50% or greater (i.e., “low hatch rate females”), 33.33% always had annual hatch rates of 50% or greater (i.e., “high hatch rate females”), and 22.22% had annual hatch rates that varied from less than 50 to 50% or greater (i.e., “variable hatch rate females”). In comparison, only 5.56% of repeat nesters in the GWNF study could be qualified as low hatch rate females, while the majority of repeat nesters could be qualified as either high hatch rate females (36.11%) or variable hatch rate females (58.33%; Figure 4). These contrasting results suggest that the reproductive capacity of the NNJ population is much more limited than that of the GWNF population and that this limitation is primarily due to a higher proportion of low hatch rate females in the NNJ population.
Similar to other wood turtle populations, we found significant associations between body size and clutch size in the NNJ population (e.g., Brooks et al., 1992; Walde et al., 2007; Jones, 2009); however, we found no significant associations between body size and hatching success. We also found no significant associations between clutch size and hatching success in either NNJ or GWNF populations. This indicates that parameters like body size and clutch size are not always reliable predictors of hatching success in turtle populations and that other factors are most likely causing maternally-linked hatching failure in the NNJ population.
It is difficult to conclude whether maternally-linked hatching failure is an abnormal feature of turtle populations because repeat nesting data are seldom reported or analyzed in scientific studies. Nevertheless, some studies documented maternally-linked hatching failure in turtles and crocodilians and suggested several hypotheses for its occurrence; among these are egg infertility (Heinz et al., 1991; Kuchling and Shunqing, 2015), inbreeding depression (Ennen et al., 2010), maternal senescence (Warner et al., 2016), inadequate maternal nutrition (Noble et al., 1993; Craven et al., 2008) and environmental contamination (Perrault et al., 2011).
Egg infertility is an unlikely explanation for maternally-linked hatching failure in NNJ wood turtles simply because 70.92% of all wood turtle eggs collected for incubation during 2015 and 2016 showed at least some evidence of “chalking” (i.e., eggshell whitening) and chalking in turtle eggs has long been regarded as direct evidence of fertilization and subsequent embryonic development (Ewert, 1979, 1985; Deeming, 1991). Furthermore, our long-term mark-recapture data indicate that there is no shortage of reproductive males in the NNJ population (Duchak and Burke, unpublished data). An abundance of males combined with the occurrence of female sperm storage (Figueras and Burke, 2017) and multiple paternity (Galbraith, 1991; Bouchard et al., 2016) in wood turtles make even sporadic occurrences of infertile nests especially unlikely in the NNJ and any other wild wood turtle populations of considerable size; unless contaminants that cause male infertility are prevalent (Heinz et al., 1991).
Maternally-linked hatching failure as a consequence of inbreeding is also unlikely at the NNJ site. Our mark-recapture efforts since 2012 have confirmed the existence of over 110 uniquely marked adult wood turtles that are not geographically isolated from neighboring wood turtle populations; relatively undisturbed stream corridors connect the NNJ population to adjacent upstream and downstream wood turtle populations. Moreover, Spradling et al. (2010) showed that an even smaller, geographically disjunct Iowa population was not inbred and actually exhibited a substantial amount of genetic diversity despite having significantly lower allelic richness and heterozygosity than a larger population in West Virginia. Additionally, Tessier et al. (2005) found high degrees of genetic variability among six Canadian wood turtle populations, some of which had experienced drastic declines in recent history. Tessier et al. (2005) and Spradling et al. (2010) theorized that declining turtle populations may be buffered from the effects of genetic drift by the presence of long-lived adults that maintain much of the population’s original genetic variability when they breed with each other or individuals of younger age classes. Considering these studies and the NNJ population’s size and connections to neighboring populations, the possibility of inbreeding reducing NNJ hatch rates seems implausible.
Maternal senescence is also an unlikely cause of the low NNJ hatch rates. Unlike many classes of vertebrates, female reptiles are not oocyte-limited and appear capable of reproduction until death (Kuchling, 1999; Jones, 2011); accordingly, chelonians were traditionally thought to exhibit only negligible senescence (Congdon et al., 2001, 2003; Miller, 2001). In contrast, Warner et al. (2016) showed that adult survivorship and hatching success decline with old age in painted turtles (C. picta); however, our long-term mark-recapture and morphometric datasets suggest that a diversity of age classes exist at the NNJ site and that some NNJ repeat nesters with consistently poor hatch rates are unlikely to be old. For example, the most unsuccessful repeat nester in the NNJ study laid four annual nests totaling 37 eggs from 2013 to 2016 but failed to produce a single hatchling (female #18; Figure 1). This turtle was first encountered in 1999 as a non-reproductive juvenile with a straight-line plastron length (SLPL) of 74 mm, indicating that this turtle was approximately 20 years old during the last year of the present NNJ study (2016). Twenty years is a relatively young age for a turtle species that reaches reproductive maturity during its teenage years and lives 50 years or more in the wild (Jones and Willey, 2015). Thus, it is unlikely that senescence accounts for all of the maternally-linked hatching failure observed in the NNJ population.
In contrast to egg infertility, inbreeding depression, and maternal senescence, differences in food resources and maternal diets may be a slightly more convincing hypothesis as to why some NNJ females have consistently low hatching success; however, this hypothesis may still be implausible, especially if the foraging sites of all NNJ females yield the necessary nutrients for producing healthy eggs. Turtle hatchlings receive either very limited or no parental care; therefore, maternal reproductive investment in turtles is largely represented by the allocation of dietary nutrients like lipids to the yolks of eggs (Congdon, 1989; Harms et al., 2005). Some of the fatty acid constituents of these lipids may be vital to embryonic development (Noble et al., 1993), and studies have shown associations of inadequate maternal diets with fatty acid deficiencies and reduced hatch rates in captive birds and reptiles (Noble et al., 1993, 1996; Craven et al., 2008). It may be possible that individuals of some species can exhibit hatch rate impairing dietary deficiencies in the wild, especially if a high degree of variation exists in a species’ habitat/resource use and feeding ecology (Graveland and Drent, 1997).
Wood turtles are opportunistic omnivores that forage in diverse habitats (see Jones and Willey, 2015 for reviews of diet and habitat use). Like many other wood turtle sites, the NNJ site is a highly heterogeneous mix of habitats (e.g., streams, riparian floodplains, marshes, swamps, fens, mixed hardwood forests, hemlock groves, pine forests, thickets, old fields, agricultural fields, etc.), and we observed a high degree of inter-individual habitat use variation and foraging site fidelity among a sample of more than 20 NNJ females during a multiyear radio-tracking study (Duchak and Burke, unpublished data). Because some females spent the majority of their activity seasons foraging in old fields while others spent much of their time foraging in hardwood forests or various wetlands, diets and possibly nutritional statuses of these females could differ markedly. Given the wood turtle’s highly variable ecology, one might expect that inter-individual variations in habitat use and, consequently, maternal diet could conceivably influence inter-individual variations in hatching success. Although, while dietary differences probably exist in wild wood turtle populations, it may be especially unlikely that any wild diets or foraging grounds are so nutritionally limited that they would repeatedly impair the annual hatch rates of multiple females in a given population; otherwise, we might expect maternally-linked hatching failure to be a common feature in many wood turtle populations. In any event, the extent to which maternal diet and the availability of key nutrients vary across wild wood turtle populations is not known, nor is how different diets affect hatching success.
Radio-tracking repeat nesters to determine whether major differences in summer foraging habitats exist between high and low hatch rate females would be an appropriate first step in examining what effect, if any, maternal diet has on hatching success. Tracking these females would also offer opportunities to collect regular fecal samples for dietary analyses and blood samples for health assessments that could examine the potential differences between high and low hatch rate females. If high and low hatch rate females are found to have dissimilar diets and blood chemistries, then assessing the lipid and fatty acid compositions of their freshly laid eggs might reveal different quantities of vital nutrients which could explain why some females consistently have less successful hatch rates than others. Alternatively, if no differences in diet or blood chemistry are found between high and low hatch rate females, then inadequate maternal diets would be an unlikely explanation for maternally-linked hatching failure in NNJ wood turtles.
While inadequate maternal diets may be a possible, yet perhaps unlikely hypothesis for maternally-linked hatching failure in NNJ wood turtles, environmental contamination is certainly a much more plausible one. Numerous studies link maternal accumulations of contaminants with reduced hatch rates or hatchling deformities in turtle populations (e.g., Bishop et al., 1998; Nagle et al., 2001; Bell et al., 2006; Perrault et al., 2011; Hopkins et al., 2013). At first glance, the NNJ site appears relatively free of environmental contamination, aside from a presumed history of past agricultural chemical use; however, closer investigation reveals that lead (Pb) from a long history (approximately 100 years or more) of intensive upland gamebird hunting has been and continues to be deposited at the NNJ site. Similar areas managed for upland gamebird hunting were estimated to hold several thousand lead pellets per hectare in the most superficial soil layers (Lewis and Legler, 1968; Esslinger and Klimstra, 1983; Keel et al., 2002). Spent lead pellets can take decades to completely disintegrate (Thomas, 1997), but they corrode rapidly and release particulate compounds, especially in acidic soils that are subjected to agricultural treatments like plowing or tilling (Jørgensen and Willems, 1987). These lead compounds tend to adsorb to detritus, making major wood turtle prey species such as earthworms and possibly slugs, carriers of high lead burdens at contaminated sites (Ma, 1982; Niederberger and Seidel, 1999; Jones and Sievert, 2009). Although no studies have investigated the impacts of lead shot deposition on turtles, many have documented the effects of lead shot deposition on upland birds (for reviews see Kendall et al., 1996; Fisher et al., 2006). In a controlled experiment, Edens et al. (1976) demonstrated that chronic dietary lead exposures can adversely affect hatching success rates in quail; the same could be true for terrestrial turtles. Given their small home range sizes, foraging site fidelity, and tendency to feed on invertebrates that can carry high lead loads, some wood turtles could be especially likely to accumulate lead concentrations high enough to affect the hatch rates of their clutches. Furthermore, the presumably heterogeneous distribution of lead shot throughout the landscape could explain why some female wood turtles always have lower hatch rates than others.
Measuring lead concentrations in the eggs of repeat nesters could potentially determine whether lead is influencing NNJ wood turtle hatch rates, but unfortunately, egg contaminant tests require the sacrifice of eggs and can also present analytical complications for researchers. Although less invasive than testing egg contents, testing eggshells alone and comparing lead levels between the shells of eggs that hatch and the shells of eggs that fail to hatch would still be problematic. In a study of slider turtles (Trachemys scripta), Burger and Gibbons (1998) found no correlation in lead concentrations, nor any other heavy metal concentrations, between egg contents and eggshells except for manganese. Lead concentrations were also significantly higher and, presumably, more readily detectable in egg contents than eggshells (Burger and Gibbons, 1998). In addition to correlations between egg contents and eggshells proving elusive, chemical compositions of eggshells and egg contents change throughout incubation with many elements decreasing in eggshells and increasing in egg contents as they are absorbed from eggshells by growing embryos (Yalçin-Ödilek et al., 2011; Orłowski et al., 2019). Moreover, eggshells and contents of embryonated eggs (i.e., fertile eggs with visible embryos) may have significantly higher heavy metal concentrations than eggshells and contents of non-embryonated eggs (i.e., presumably infertile eggs with no visible embryos). Therefore, to obtain objective comparisons of egg lead concentrations between high and low hatch rate females, it is advisable to: (1) measure lead concentrations in whole fresh eggs (i.e., undecomposed, homogenized eggshell and egg contents) of the same age or developmental stage and, if possible, (2) avoid including embryonated and non-embryonated eggs in the same analysis (Orłowski et al., 2016); however, effectively differentiating truly non-embryonated eggs (i.e., infertile eggs) from embryonated eggs that suffered early embryonic mortality is only possible via perivitelline membrane-bound sperm detection (Croyle et al., 2016), a technique that is not readily available to most turtle researchers and could potentially complicate contaminant analyses. Furthermore, any comparisons of lead concentrations between high and low hatch rate females would require adequate sample sizes to be conclusive, and sacrificing multiple eggs from multiple nesting females may not be permitted nor viewed as ethical in studies involving protected species. Therefore, prior to negotiating the complications associated with measuring lead concentrations in eggs, a much less invasive preliminary analysis should be performed to determine whether NNJ wood turtles even carry lead burdens high enough to justify testing their eggs.
Measuring lead concentrations in the scutes of repeat nester NNJ wood turtles would be the most conservative starting point for determining whether lead could be influencing their hatch rates. Since turtle shell scutes are composed of keratin, they provide a non-lethal, minimally invasive way to test turtles for heavy metal exposure (Overmann and Krajicek, 1995; Presti et al., 1999; Sakai et al., 2000; Blanvillain et al., 2007). Lead concentrations in hard, calcified materials like bone and keratin are good indicators of long-term accumulation (Overmann and Krajicek, 1995; Sakai et al., 2000) and should also be higher and more readily detectable than lead concentrations in eggs (Sakai et al., 2000), which represent short-term accumulation (Orłowski et al., 2016). Although it is entirely unknown whether wood turtle scute lead concentrations correlate with wood turtle egg lead concentrations, finding higher scute lead concentrations in NNJ wood turtles than those of other populations or other species would confirm that NNJ wood turtles indeed have a history of lead exposure that could be responsible for their low hatch rates. Because scute lead concentrations are better indicators of long-term rather than short-term exposure, such a result may not be sufficient to fully implicate lead as the main cause of the NNJ population’s low hatch rates, but it would certainly warrant sacrificing a limited number of eggs from each repeat nester in order to measure their egg lead concentrations. As egg lead concentrations are indicators of recent exposure, they may be better suited to directly determine whether lead could be impacting the hatch rates of NNJ wood turtles but only if the previously mentioned complications associated with measuring heavy metal concentrations in eggs are mitigated. If females with consistently lower hatch rates bear significantly higher lead burdens in their eggs than females with consistently higher or variable hatch rates, it is very likely that lead contamination is responsible for the reduced hatch rates in the NNJ population. However, additional tests will be necessary to determine the possible source(s) and pathway(s) of lead exposure. Radio-tracking females with high and low hatching success records to their summer foraging habitats and quantifying lead levels in the soils and food items of these areas will be necessary to implicate recreational hunting as a major source of contamination.
Conclusion
The potential causes of hatching failure are important, yet often overlooked aspects of reptile ecology and conservation. The commonly suggested hypotheses for hatching failure, such as unsuitable incubation conditions or infertility, are unlikely to explain all of the hatch rate variability in some populations. This study found that approximately 60% of the hatch rate variability observed in a wood turtle population with low hatching success can be attributed to maternal identity. The remaining 40% can be attributed to the random environmental factors that are usually theorized to be major reasons for reduced hatch rates in many turtle populations (e.g., unsuitable incubation conditions, flooding, desiccation, egg infertility, egg damage due to improper handling by researchers, root and insect predation, and fungal/microbial infection). We hypothesize that maternally-linked hatching failure in seemingly healthy wild turtle populations is most likely an indicator of environmental contamination.
This study reveals a cryptic but critical conservation concern for vulnerable turtle populations: that the presence of many nesting females does not necessarily guarantee high or even sustainable reproductive rates. The exceedingly low hatch rates we observed suggest that recruitment in some turtle populations could be severely hindered even when managers attempt to mitigate well-known factors that decrease recruitment such as nest predation and low juvenile survival. Although time-consuming and labor intensive, we recommend that all turtle population studies investigate hatching success and document at least one year of overall, population-level hatch rate data as hatching success is an important indicator of every population’s reproductive potential.
Data Availability Statement
The raw data supporting the conclusions of this article will be made available by the authors, without undue reservation.
Ethics Statement
The animal study was reviewed and approved by Hofstra University Institutional Animal Care and Use Committee.
Author Contributions
TD performed all field and lab work, performed all literature reviews, wrote most of the abstract, introduction, materials and methods, result, and discussion sections, and prepared all figures and tables. RB proposed and performed most analyses, contributed to portions of the materials and methods and result sections that are relevant to data analysis, and provided helpful edits and suggestions. Both authors contributed to the article and approved the submitted version.
Conflict of Interest
The authors declare that the research was conducted in the absence of any commercial or financial relationships that could be construed as a potential conflict of interest.
Publisher’s Note
All claims expressed in this article are solely those of the authors and do not necessarily represent those of their affiliated organizations, or those of the publisher, the editors and the reviewers. Any product that may be evaluated in this article, or claim that may be made by its manufacturer, is not guaranteed or endorsed by the publisher.
Acknowledgments
We sincerely thank the people and organizations that made this study possible. The New Jersey Division of Fish and Wildlife provided the necessary permits to conduct this study as well as continual assistance with nesting site remediation and maintenance operations. The New Jersey School of Conservation was integral to this study as it provided lab space and assistance with incubation monitoring. Tom Akre of the Smithsonian Conservation Biology Institute graciously provided valuable comparison data from his long-term wood turtle study in George Washington National Forest, Virginia. Lastly, Hofstra University’s Institutional Animal Care and Use Committee pre-approved this project.
References
Bell, B. A., Spotila, J. R., Paladino, F. V., and Reina, R. D. (2003). Low reproductive success of leatherback turtles, Dermochelys coriacea, is due to high embryonic mortality. Biol. Conserv. 115, 131–138. doi: 10.1016/s0006-3207(03)00102-2
Bell, B., Spotila, J. R., and Congdon, J. (2006). High incidence of deformity in aquatic turtles in the John Heinz National Wildlife Refuge. Environ. Pollut. 142, 457–465. doi: 10.1016/j.envpol.2005.10.020
Bishop, C. A., Ng, P., Pettit, K. E., Kennedy, S. W., Stegeman, J. J., Norstrom, R. J., et al. (1998). Environmental contamination and developmental abnormalities in eggs and hatchlings of the common snapping turtle (Chelydra serpentina serpentina) from the Great Lakes-St Lawrence River basin. Environ. Pollut. 101, 143–156. doi: 10.1016/s0269-7491(98)00005-0
Blanck, C. E., and Sawyer, R. H. (1981). Hatchery practices in relation to early embryology of the loggerhead sea turtle, Caretta caretta (Linné). J. Exp. Mar. Biol. Ecol. 49, 163–177. doi: 10.1016/0022-0981(81)90068-x
Blanvillain, G., Schwenter, J. A., Day, R. D., Point, D., Christopher, S. J., Roumillat, W. A., et al. (2007). Diamondback terrapins, Malaclemys terrapin, as a sentinel species for monitoring mercury pollution of estuarine systems in South Carolina and Georgia, USA. Environ. Toxicol. Chem. 26, 1441–1450. doi: 10.1897/06-532r.1
Bobyn, M. L., and Brooks, R. J. (1994). Interclutch and interpopulation variation in the effects of incubation conditions on sex, survival, and growth of hatchling turtles (Chelydra serpentina). J. Zool. 233, 233–257. doi: 10.1111/j.1469-7998.1994.tb08586.x
Booth, D. T. (2004). “Artificial incubation,” in Reptilian Egg Incubation: Environment, Evolution, and Behavior, ed. D. C. Deeming (Nottingham: Nottingham University Press), 253–263.
Bouchard, C., Tessier, N., and Lapointe, F. (2016). “You are the fathers: parentage analysis reveals extensive occurrences of multiple paternity in wood turtles [abstract],” in Proceedings of the 14th Annual Symposium on the Conservation and Biology of Tortoises and Freshwater Turtles, New Orleans, LA.
Brooks, R. J., Shilton, C. M., Brown, G. B., and Quinn, N. W. S. (1992). Body size, age distribution, and reproduction in a northern population of wood turtles (Clemmys insculpta). Can. J. Zool. 70, 462–469. doi: 10.1139/z92-070
Buech, R. R., Hanson, L. G., and Nelson, M. D. (1997). “Identification of wood turtle nesting areas for protection and management,” in Proceedingsof the Conservation, Restoration, and Management of Tortoises and Turtles-An International Conference, ed. J. Van Abbema (New York, NY: New York Turtle and Tortoise Society), 383–391.
Buhlmann, K. A., and Osborn, C. P. (2011). Use of an artificial nesting mound by wood turtles (Glyptemys insculpta): a tool for turtle conservation. North. Nat. 18, 315–334. doi: 10.1656/045.018.0305
Burger, J., and Gibbons, J. W. (1998). Trace elements in egg contents and egg shells of slider turtles (Trachemys scripta) from the Savanna River Site. Arch. Environ. Contam. Toxicol. 34, 382–386. doi: 10.1007/s002449900334
Castellano, C. M. (2007). Ecology and Conservation of the Wood Turtle, Glyptemys insculpta, at Delaware Water Gap National Recreation Area. Doctoral dissertation. Bronx, NY: Fordham University.
Christens, E., and Bider, J. R. (1987). Nesting activity and hatching success of the painted turtle (Chrysemys picta marginata) in southwestern Quebec. Herpetologica 43, 55–65.
Congdon, J. D. (1989). Proximate and evolutionary constraints on energy relations in reptiles. Physiol. Zool. 62, 356–373. doi: 10.1086/physzool.62.2.30156175
Congdon, J. D., Nagle, R. D., Kinney, O. M., and van Loben Sels, R. C. (2001). Hypothesis of aging in a long-lived vertebrate, Blanding’s turtle (Emydoidea blandingii). Exp. Gerontol. 36, 813–827. doi: 10.1016/s0531-5565(00)00242-4
Congdon, J. D., Nagle, R. D., Kinney, O. M., Ostentoski, M., Avery, H. W., van Loben Sels, R. C., et al. (2000). Nesting ecology and embryo mortality: implications for hatching success and demography of Blanding’s turtles (Emydoidea blandingii). Chelonian Conserv. Biol. 3, 569–579.
Congdon, J. D., Nagle, R. D., Kinney, O. M., van Loben Sels, R. C., Quinter, T., and Tinkle, D. W. (2003). Testing hypotheses of aging in long-lived painted turtles (Chrysemys picta). Exp. Gerontol. 38, 765–772. doi: 10.1016/s0531-5565(03)00106-2
Congdon, J. D., Tinkle, D. W., Breitenbach, G. L., and van Loben Sels, R. C. (1983). Nesting ecology and hatching success in the turtle Emydoidea blandingi. Herpetologica 39, 417–429.
Craven, K. S., Parsons, J., Taylor, S. A., Belcher, C. N., and Owens, D. W. (2008). The influence of diet on fatty acids in the egg yolk of green sea turtles, Chelonia mydas. J. Comp. Physiol. B 178, 495–500. doi: 10.1007/s00360-007-0242-8
Croyle, K., Gibbons, P., Light, C., Goode, E., Durrant, B., and Jensen, T. (2016). Chelonian perivitelline membrane-bound sperm detection: a new breeding management tool. Zoo Biol. 35, 95–103. doi: 10.1002/zoo.21273
Deeming, D. C. (1991). “Reasons for the dichotomy in egg turning in birds and reptiles,” in Egg Incubation: Its Effects on Embryonic Development in Birds and Reptiles, eds D. C. Deeming and M. W. J. Ferguson (Cambridge: Cambridge University Press), 307–323. doi: 10.1017/cbo9780511585739.020
Demuth, J. P. (2001). The effects of constant and fluctuating incubation temperatures on sex determination, growth, and performance in the tortoise Gopherus polyphemus. Can. J. Zool. 79, 1609–1620. doi: 10.1139/z01-120
Doody, J. S., Georges, A., and Young, J. E. (2004). Determinants of reproductive success and offspring sex in a turtle with environmental sex determination. Biol. J. Linn. Soc. 81, 1–16. doi: 10.1111/j.1095-8312.2004.00250.x
Dragon, J. (2014). Habitat Selection, Movement, and Survival of Hatchling Wood Turtles (Glyptemys insculpta) in an Atypical Habitat. MS thesis. Fairfax, VA: George Mason University.
Edens, F. W., Benton, E., Bursian, S. J., and Morgan, G. W. (1976). Effect of dietary lead on reproductive performance in Japanese quail, Coturnix coturnix japonica. Toxicol. Appl. Pharmacol. 38, 307–314. doi: 10.1016/0041-008x(76)90137-x
Ennen, J. R., Kreiser, B. R., and Qualls, C. P. (2010). Low genetic diversity in several gopher tortoise (Gopherus polyphemus) populations in the Desoto National Forest, Mississippi. Herpetologica 66, 31–38. doi: 10.1655/08-083.1
Ernst, C. H., and Lovich, J. E. (2009). Turtles of the United States and Canada. Baltimore, MD: Johns Hopkins University Press, 827.
Esslinger, C. G., and Klimstra, W. D. (1983). Lead shot incidence on a public goose hunting area in southern Illinois. Wildlife Soc. Bull. 11, 166–169.
Ewert, M. A. (1979). “The embryo and its egg: development and natural history,” in Turtles: Perspectives and Research, eds M. Harless and H. Morlock (New York, NY: John Wiley and Sons), 333–413.
Ewert, M. A. (1985). “Embryology of turtles,” in Biology of the Reptilia, Development A, Vol. 14, eds C. Gans, F. Billet, and P. Maderson (New York, NY: John Wiley and Sons), 75–267.
Farrell, R. F., and Graham, T. E. (1991). Ecological notes on the turtle Clemmys insculpta in Northwestern New Jersey. J. Herpetol. 25, 1–9. doi: 10.2307/1564787
Feinberg, J. A., and Burke, R. L. (2003). Nesting ecology and predation of diamondback terrapins, Malaclemys terrapin, at Gateway National Recreation Area, New York. J. Herpetol. 37, 517–526. doi: 10.1670/207-02a
Figueras, M., and Burke, R. (2017). Glyptemys insculpta (Wood Turtle). Long-term sperm storage. Herpetol. Rev. 48:175.
Fisher, I. J., Pain, D. J., and Thomas, V. G. (2006). A review of lead poisoning from ammunition sources in terrestrial birds. Biol. Conserv. 131, 421–432. doi: 10.1016/j.biocon.2006.02.018
Fowler, L. E. (1979). Hatching success and nest predation in the green sea turtle, Chelonia mydas, at Tortuguero, Costa Rica. Ecology 60, 946–955. doi: 10.2307/1936863
Galbraith, D. A. (1991). Studies of Mating Systems in Wood Turtles (Clemmys insculpta) and Snapping Turtles (Chelydra serpentina) using DNA Fingerprinting. Ph.D. dissertation. Kingston, ON: Queen’s University.
Garber, S. D., and Burger, J. (1995). A 20-yr study documenting the relationship between turtle decline and human recreation. Ecol. Appl. 5, 1151–1162. doi: 10.2307/2269362
Gibbs, J. P., and Shriver, W. G. (2002). Estimating the effects of road mortality on turtle populations. Conserv. Biol. 16, 1647–1652. doi: 10.1046/j.1523-1739.2002.01215.x
Graveland, J., and Drent, R. H. (1997). Calcium availability limits breeding success of passerines on poor soils. J. Anim. Ecol. 66, 279–288. doi: 10.2307/6028
Harms, H. K., Paitz, R. T., Bowden, R. M., and Janzen, F. J. (2005). Age and season impact resource allocation to eggs and nesting behavior in the painted turtle. Physiol. Biochem. Zool. 78, 996–1004. doi: 10.1086/432920
Heinz, G. H., Percival, H. F., and Jennings, M. L. (1991). Contaminants in American alligator eggs from Lake Apopka, Lake Griffin, and Lake Okeechobee, Florida. Environ. Monitor. Assess. 16, 277–285. doi: 10.1007/BF00397615
Hopkins, B. C., Willson, J. D., and Hopkins, W. A. (2013). Mercury exposure is associated with negative effects on turtle reproduction. Environ. Sci. Technol. 47, 2416–2422. doi: 10.1021/es304261s
Horne, B. D., Brauman, R. J., Moore, M. J. C., and Seigel, R. A. (2003). Reproductive and nesting ecology of the yellow-blotched map turtle, Graptemys flavimaculata: implications for concern and management. Copeia 2003, 729–738. doi: 10.1643/ha02-213.1
Jones, M. T. (2009). Spatial Ecology, Population Structure, and Conservation of the Wood Turtle, Glyptemys insculpta, in central New England. Ph.D. thesis. Amherst, MA: University of Massachusetts.
Jones, M. T., and Sievert, P. R. (2009). Glyptemys insculpta (wood turtle). Diet. Herpetol. Rev. 40, 433–434.
Jones, M. T., and Willey, L. L. (2015). Status and Conservation of the Wood Turtle in the Northeastern United States. Regional Conservation Needs Report, 288. New Salem, MA: American Turtle Observatory.
Jones, S. M. (2011). “Hormonal regulation and ovarian function in reptiles,” in Hormones and Reproduction of Vertebrates: Reptiles, Vol. Volume 3, eds D. O. Norris and K. H. Lopez (Amsterdam: Academic Press), 89–115. doi: 10.1016/b978-0-12-374932-1.00028-7
Jørgensen, S. S., and Willems, M. (1987). The fate of lead in soils: the transformation of lead pellets in shooting-range soils. Ambio 16, 11–15.
Keel, M. K., Davidson, W. R., Doster, G. L., and Lewis, L. A. (2002). Northern bobwhite and lead shot deposition in an upland habitat. Arch. Environ. Contam. Toxicol. 43, 318–322. doi: 10.1007/s00244-002-1212-5
Kendall, R. J., Lacher, T. E., Bunck, C., Daniel, B., Driver, C., Grue, C. E., et al. (1996). An ecological risk assessment of lead shot exposure in non-waterfowl avian species: upland game birds and raptors. Environ. Toxicol. Chem. 15, 4–20. doi: 10.1002/etc.5620150103
Kolbe, J. J., and Janzen, F. J. (2002). Spatial and temporal dynamics of turtle nest predation: edge effects. Oikos 99, 538–544. doi: 10.1034/j.1600-0706.2002.11853.x
Kuchling, G., and Shunqing, L. (2015). Rafetus swinhoei in China – still on the brink despite eight years of captive breeding attempts. Turtle Surv. 34–35.
Leslie, A. J., Penick, D. N., Spotila, J. R., and Paladino, F. V. (1996). Leatherback turtle, Dermochelys coriacea, nesting and nest success at Tortuguero, Costa Rica. Chelon. Conserv. Biol. 2, 159–168.
Lessells, C. M., and Boag, P. T. (1987). Unrepeatable repeatabilities: a common mistake. Auk 104, 116–121. doi: 10.2307/4087240
Levell, J. P. (2000). Commercial exploitation of Blanding’s turtle, Emydoidea blandingii, and the wood turtle, Clemmys insculpta, for the live animal trade. Chelon. Conserv. Biol. 3, 665–674.
Lewis, J. C., and Legler, E. (1968). Lead shot ingestion by mourning doves and incidence in soil. J. Wildlife Manag. 32, 476–482. doi: 10.2307/3798925
Litzgus, J. D., and Mousseau, T. A. (2006). Geographic variation in reproduction in a freshwater turtle (Clemmys guttata). Herpetologica 62, 132–140. doi: 10.1655/05-26.1
Ma, W. C. (1982). The influence of soil properties and worm-related factors on the concentration of heavy metals in earthworms. Pedobiologia 24, 109–119.
McCollough, J. P. (1997). “Status and conservation of turtles in Maine,” in Status and Conservation of Turtles of the Northeastern United States, ed. T. F. Tyning (Lanesboro, MN: Serpent’s Tail Natural History Books), 7–11. doi: 10.1126/sciadv.1603155
Miller, J. K. (2001). Escaping senescence: demographic data from the three-toed box turtle (Terrapene carolina triunguis). Exp. Gerontol. 36, 829–832. doi: 10.1016/s0531-5565(00)00243-6
Nagle, R. D., Rowe, C. L., and Congdon, J. D. (2001). Accumulation and selective maternal transfer of maternal contaminants in the turtle Trachemys scripta associated with coal ash deposition. Arch. Environ. Contam. Toxicol. 40, 531–536. doi: 10.1007/s002440010206
Niederberger, A. J., and Seidel, M. E. (1999). Ecology and status of a wood turtle (Clemmys insculpta) population in West Virginia. Chelon. Conserv. Biol. 3, 414–418.
Noble, R. C., McCartney, R., and Ferguson, M. W. J. (1993). Lipid and fatty acid compositional differences between eggs of wild and captive-breeding alligators (Alligator mississippiensis): an association with reduced hatchability? J. Zool. 230, 639–649. doi: 10.1111/j.1469-7998.1993.tb02712.x
Noble, R. C., Speake, B. K., McCartney, R., Foggin, C. M., and Deeming, D. C. (1996). Yolk lipids and their fatty acids in the wild and captive ostrich (Struthio camelus). Comp. Biochem. Physiol. 113B, 753–756. doi: 10.1016/0305-0491(95)02097-7
Noel, K. M., Qualls, C. P., and Ennen, J. R. (2012). A comparison of artificial incubation and natural incubation hatching success of gopher tortoise (Gopherus polyphemus) eggs in southern Mississippi. Herpetologica 68, 324–333. doi: 10.1655/herpetologica-d-11-00061.1
Orłowski, G., Hałupka, L., Pokorny, P., Klimczuk, E., Sztwiertnia, H., and Dobicki, W. (2016). The effect of embryonic development on metal and calcium content in eggs and eggshells in a small passerine. IBIS 158, 144–154. doi: 10.1111/ibi.12327
Orłowski, G., Merta, D., Pokorny, P., Łukaszewicz, E., Dobicki, W., Kobielski, J., et al. (2019). Eggshell resorption, and embryonic mobilization and accumulation of calcium and metals in eggs of wild and captive Capercaillies Tetrao urogallus. Environ. Pollut. 249, 152–162. doi: 10.1016/j.envpol.2019.03.010
Overmann, S. R., and Krajicek, J. J. (1995). Snapping turtles (Chelydra serpentina) as biomonitors of lead contamination of the Big River in Missouri’s Old Lead Belt. Environ. Toxicol. Chem. 14, 689–695. doi: 10.1897/1552-8618(1995)14[689:stcsab]2.0.co;2
Packard, G. C., Packard, M. J., Miller, K., and Boardman, T. J. (1987). Influence of moisture, temperature, and substrate on snapping turtle eggs and embryos. Ecology 68, 983–993. doi: 10.2307/1938369
Parren, S. G. (2013). A twenty-five year study of the wood turtle (Glyptemys insculpta) in Vermont: movements, behavior, injuries, and death. Herpetol. Conserv. Biol. 8, 176–190.
Paterson, J. E., Steinberg, B. D., and Litzgus, J. D. (2012). Revealing a cryptic life-history stage: differences in habitat selection and survivorship between hatchlings of two turtle species at risk (Glyptemys insculpta and Emydoidea blandingii). Wildlife Res. 39, 408–418. doi: 10.1071/wr12039
Paterson, J. E., Steinberg, B. D., and Litzgus, J. D. (2014). Effects of body size, habitat selection, and exposure on hatchling turtle survival. J. Zool. 294, 278–285. doi: 10.1111/jzo.12176
Perrault, J., Wyneken, J., Thompson, L. J., Johnson, C., and Miller, D. L. (2011). Why are hatching and emergence success low? Mercury and selenium concentrations in nesting leatherback sea turtles (Dermochelys coriacea) and their young in Florida. Mar. Pollut. Bull. 62, 1671–1682. doi: 10.1016/j.marpolbul.2011.06.009
Pike, D. A., Roznick, E. A., and Bell, I. (2015). Nest inundation from sea-level rise threatens sea turtle population viability. R. Soc. Open Sci. 2:150127. doi: 10.1098/rsos.150127
Plummer, M. V. (1976). Some aspects of nesting success in the turtle, Trionyx muticus. Herpetologica 32, 353–359.
Presti, S. M., Hidalgo, A. R. S., Sollod, A. E., and Seminoff, J. A. (1999). Mercury concentration in the scutes of black sea turtles, Chelonia mydas agassizii, in the Gulf of California. Chelon. Conserv. Biol. 3, 531–533.
Rafferty, A. R., Tomillo, P. S., Spotila, J. R., Paladino, F. V., and Reina, R. D. (2011). Embryonic death is linked to maternal identity in the leatherback turtle (Dermochelys coriacea). PLoS One 6:e21038. doi: 10.1371/journal.pone.0021038
Riley, J. L., and Litzgus, J. D. (2013). Evaluation of predator-exclusion cages used in turtle conservation: cost analysis and effects on nest environment and proxies of hatchling fitness. Wildlife Res. 40, 499–511. doi: 10.1071/wr13090
Sakai, H., Saeki, K., Ichihashi, H., Suganuma, H., Tanabe, S., and Tatsukawa, R. (2000). Species-specific distribution of heavy metals in tissues and organs of loggerhead turtle (Caretta caretta) and green turtle (Chelonia mydas) from Japanese coastal waters. Mar. Pollut. Bull. 40, 701–709. doi: 10.1016/s0025-326x(00)00008-4
Samson, J., Hughes, E. J., and Brooks, R. J. (2007). Excavation is a nondeleterious method for obtaining fecundity and morphometric data from small-sized eggs of freshwater turtles. Chelon. Conserv. Biol. 6, 255–259. doi: 10.2744/1071-8443(2007)6[255:eianmf]2.0.co;2
Saumure, R. A., Herman, T. B., and Titman, R. D. (2006). Effects of haying and agricultural practices on a declining species: the North American wood turtle, Glyptemys insculpta. Biol. Conserv. 135, 581–591.
Spotila, J. R., Zimmerman, L. C., Binckley, C. A., Grumbles, J. S., Rostal, D. C., List, A., et al. (1994). Effects of incubation on sex determination, hatching success, and growth of hatching desert tortoises, Gopherus agassizii. Herpetol. Monogr. 8, 103–116. doi: 10.2307/1467074
Spradling, T. A., Tamplin, J. W., Dow, S. S., and Meyer, K. J. (2010). Conservation genetics of a peripherally isolated population of the wood turtle (Glyptemys insculpta) in Iowa. Conserv. Genet. 11, 1667–1677. doi: 10.1007/s10592-010-0059-y
Standing, K. L., Herman, T. B., and Morrison, I. P. (1999). Nesting ecology of Blanding’s turtle (Emydoidea blandingii) in Nova Scotia, the northeastern limit of the species’ range. Can. J. Zool. 77, 1609–1614. doi: 10.1139/z99-122
Steen, D. A., Aresco, M. J., Beilke, S. G., Compton, B. W., Condon, E. P., Dodd, C. K., et al. (2006). Relative vulnerability of female turtles to road mortality. Anim. Conserv. 9, 269–273. doi: 10.1111/j.1469-1795.2006.00032.x
Tessier, N., Paquette, S. R., and Lapointe, F. J. (2005). Conservation genetics of the wood turtle (Glyptemys insculpta) in Quebec, Canada. Can. J. Zool. 83, 765–772. doi: 10.1139/z05-065
Thomas, V. G. (1997). The environmental and ethical implications of lead shot contamination of rural lands in North America. J. Agric. Environ. Ethics 10, 41–54.
Tingley, R., McCurdy, D. G., Pulsifer, M. D., and Herman, T. B. (2009). Spatio-temporal differences in the use of agricultural fields by male and female wood turtles (Glyptemys insculpta) inhabiting an agri-forest mosaic. Herpetol. Conserv. Biol. 4, 185–190.
Tuttle, S. E., and Carroll, D. M. (1997). Ecology and natural history of the wood turtle (Glyptemys insculpta) in southern New Hampshire. Chelonian Conserv. Biol. 2, 447–449.
van Dijk, P. P., and Harding, J. (2013). IUCN Red List of Threatened Species. Wood turtle (Glyptemys insculpta). Available online at: http://www.iucnredlist.org/details/4965/0 (accessed September 01, 2015).
Van Noordijk, A. J., Van Balen, J. H., and Scharloo, W. (1980). Heritability of ecological important traits in the great tit. Ardea 68, 193–203. doi: 10.1111/j.1365-2656.2011.01835.x
Walde, A. D., Bider, J. R., Masse, D., Saumure, R. A., and Titman, R. D. (2007). Nesting ecology and hatching success of the wood turtle, Glyptemys insculpta, in Québec. Herpetol. Conserv. Biol. 2, 49–60.
Wallace, B. P., Sotherland, P. R., Tomillo, P. S., Reina, R. D., Spotila, J. R., and Paladino, F. V. (2007). Maternal investment in reproduction and its consequences in leatherback turtles. Oecologia 152, 37–47. doi: 10.1007/s00442-006-0641-7
Warner, D. A., Miller, D. A. W., Bronikowski, A. M., and Janzen, F. J. (2016). Decades of field data reveal that turtles senesce in the wild. Proc. Natl. Acad. Sci. U.S.A. 113, 6502–6507. doi: 10.1073/pnas.1600035113
Whitmore, C. P., and Dutton, P. H. (1985). Infertility, embryonic mortality, and nest-site selection in leatherback and green sea turtles in Suriname. Biol. Conserv. 34, 251–272. doi: 10.1016/0006-3207(85)90095-3
Wilson, D. S. (1998). Nest-site selection: microhabitat variation and its effects on the survival of turtle embryos. Ecology 79, 1884–1892. doi: 10.1890/0012-9658(1998)079[1884:nssmva]2.0.co;2
Wolak, M. E., Fairbairn, D. J., and Paulsen, Y. R. (2012). Guidelines for estimating repeatability. Methods Ecol. Evol. 3, 129–137. doi: 10.1111/j.2041-210x.2011.00125.x
Wolf, J. B., and Wade, M. J. (2009). What are maternal effects (and what are they not)? Philos. Trans. R. Soc. Lond. B Biol. Sci. 364, 1107–1115. doi: 10.1098/rstb.2008.0238
Wyneken, J., Burke, T. J., Salmon, M., and Pederson, D. K. (1988). Egg failure in natural and relocated sea turtle nests. J. Herpetol. 22, 88–96. doi: 10.2307/1564360
Keywords: reproductive ecology, hatching success, multi-year turtle nesting study, repeatability, egg incompetence, maternal effects, egg infertility, ecotoxicology
Citation: Duchak TJ and Burke RL (2022) Hatching Failure in Wood Turtles (Glyptemys insculpta) Is Linked to Maternal Identity. Front. Ecol. Evol. 10:836800. doi: 10.3389/fevo.2022.836800
Received: 13 January 2022; Accepted: 05 April 2022;
Published: 20 June 2022.
Edited by:
Jeanine M. Refsnider, University of Toledo, United StatesReviewed by:
Andrew Badje, Wisconsin Department of Natural Resources, United StatesJeff Tamplin, The University of Iowa, United States
Copyright © 2022 Duchak and Burke. This is an open-access article distributed under the terms of the Creative Commons Attribution License (CC BY). The use, distribution or reproduction in other forums is permitted, provided the original author(s) and the copyright owner(s) are credited and that the original publication in this journal is cited, in accordance with accepted academic practice. No use, distribution or reproduction is permitted which does not comply with these terms.
*Correspondence: Thomas J. Duchak, VGhvbWFzLkouRHVjaGFrQGhvZnN0cmEuZWR1; VGhvbWFzLkouRHVjaGFrQHJ1dGdlcnMuZWR1