- 1Departamento de Genetica e Biologia Evolutiva, Instituto de Biociências, Universidade de São Paulo, São Paulo, Brazil
- 2Red de Interacciones Multitróficas, Instituto de Ecologia A.C., Xalapa, Mexico
- 3Departamento de Ecologia Evolutiva, Estación Biológica de Doñana, Consejo Superior de Investigaciones Científicas, Seville, Spain
- 4Departamento de Fisiologia, Instituto de Biociências, Universidade de São Paulo, São Paulo, Brazil
Within the Neotropical genus Anastrepha, the nominal species Anastrepha fraterculus is widely distributed from Mexico through northern Argentina. Currently it is believed to comprises a complex of at least eight cryptic species—known as the Anastrepha fraterculus complex (AF complex)—three of which occur in Brazil: A. sp.1 aff. fraterculus, A. sp.2 aff. fraterculus, and A. sp.3 aff. fraterculus. In this study, we present the results of a broad integrated analysis of multiple biological attributes in samples of the three species collected in sympatric areas. Analyses of the mitotic chromosomes confirm that all of them differ in sex chromosomes, and that the relative frequency of the distinct karyotypes is associated with variation in altitude. In these sympatric areas, a single female hybrid karyotype was detected within a significant sample of individuals. Population samples were analyzed for the ribosomal transcribed spacer ITS1, confirming that the three species have specific sequence types. Observations of reproductive behavior under laboratory conditions revealed that A. sp.1 and A. sp.2 mate early in the morning, while A. sp.3 mates in the middle of the day. A bimodal distribution of mating time was observed in the laboratory for hybrids, obtained between A. sp.1 and A. sp.3. In a mating choice experiment, most of the mating pairs were homospecific. In addition, through a list of the most frequent hosts associated with geographical occurrence, a bioclimatic model of their potential distribution was generated. The set of data allowed for the construction of explanatory hypothesis about the observed geographical pattern and the differential use of host fruits. Morphometric analyses of wings clearly demonstrated differences among the three species, for both males and females. Based on a wing image of the A. fraterculus (Wiedemann, 1830) type specimen, the morphometric analysis indicated that the type specimen would correspond to a male of A. sp.2 aff. fraterculus. The information provided by this report is not only useful for taxonomic purposes, but also reveals aspects to be considered in any reconstruction of an evolutionary scenario of the Anastrepha fraterculus complex.
Introduction
In the search for understanding the origin and history of biological diversity, discussion about the origin of species is recurrent. However, according to Templeton (1989) there is a fundamental question that precedes the investigation of the processes involved in its origins: What is a species? The matter was earlier investigated with in more detail when Dobzhansky (1935, 1937) published a critique of the species concept, stressing that the core problem was to understand how a continuous process—that is, changes in allele frequencies—could produce distinct genetic and morphological groups living in a certain habitat. Aspects of natural history and biogeography were added to the genetic perspective by Mayr (1942), who stated that, when intercrossing populations become reproductively isolated, they become independent evolutionary units. Although there are currently numerous concepts of species (De Queiroz, 2007), it is undoubtedly desirable to adopt operational criteria in order to recognize taxonomic units. However, this task is particularly difficult for species that are morphologically similar: the so-called “cryptic species.” Cryptic species are widespread within the huge biodiversity of the Neotropics (see De Souza et al., 2015; Hernández-Triana, 2015; Vacher et al., 2019), and have been supposed to occur in lineages that have fast rates of evolution but have also remained quite morphologically invariant, in relation to ancient lineages (see Derryberry et al., 2011). Cryptic species complexes generate additional difficulties, especially in agricultural pest insects, for which a fine understanding of evolutionary units is relevant to generate not only theoretical knowledge, but also information that can be applied to their management. The fruit flies of the family Tephritidae include several species complexes spread among different genera (White and Elson-Harris, 1992), some of which are considered among the most devastating insect pests. They cause direct damage to commercial production by reducing yields, and indirect damage by disrupting national and international trade, causing economic losses on the order of billions of US dollars every year (Rendón and Enkerlin, 2021).
As tephritid fruit flies are a global problem, the recognition and characterization of distinct taxonomic units are of paramount importance for the development of more efficient and sustainable pest control methods (Aluja and Mangan, 2008; Cladera et al., 2014). In this context, FAO/IAEA (based in Vienna, Austria) promoted and coordinated a broad project, in which around 50 researchers from 20 countries from all continents participated. The aim was to identify and characterize the distinct biological entities within economically important cryptic species complexes of tephritid fruit flies, applying a methodology defined by the concept of integrative taxonomy (DeMeyer et al., 2015; Hendrichs et al., 2015). Such methodology not only facilitates the recognition of limits and naming the species, but also allows for a better understanding of the processes behind their origins (Schlick-Steiner et al., 2010; Schutze et al., 2017).
In the genus Anastrepha, which is endemic to the Neotropical Region, the species Anastrepha fraterculus (Wiedemann, 1830)—the South American fruit fly—is an important fruticulture pest, widely distributed from Mexico to northern Argentina (White and Elson-Harris, 1992). In an early taxonomic revision of the genus based on morphology, Stone (1942) identified some “geographical races” within this nominal species; however, over time, analyses of several biological parameters made it evident that, in reality, a complex of cryptic species existed under this name and eventually it has become recognized as Anastrepha fraterculus complex (AF complex) (see the review in Hendrichs et al., 2015).
The first successful attempt to use correlated analysis of multiple biological attributes in the study of the AF complex allowed for the recognition of two putative species in Brazil, which were provisionally named Anastrepha sp.1 affinis fraterculus and A. sp.2 aff. fraterculus (Selivon and Perondini, 1998; Selivon et al., 1999, 2005a). Later, the existence of a third putative species in Brazil, A. sp.3 aff. fraterculus was also proposed (Selivon et al., 2004). In the present report, these are denoted by A. sp.1, A. sp.2, and A. sp.3, respectively.
Further studies employing morphometrical analysis have distinguished eight morphotypes in the AF complex, over its wide geographical distribution in the Neotropical Region (Hernández-Ortiz et al., 2004, 2012, 2015). Thus, the “Mexican morphotype” occurs in Mexico and Central America; the “Venezuelan” morphotype in the Caribbean and lowlands of Venezuela; the “Andean” morphotype in the highlands of Venezuela and Colombia; the “Peruvian” morphotype in the Pacific coastal lowlands and inter-Andean valleys of Peru and Ecuador; and the “Ecuadorian” morphotype in the Andean-highlands of Peru and Ecuador. In addition, there are three morphotypes found in Brazil, named “Brazilian-1” (=A. sp.1 aff. fraterculus) occurring also in Argentina, “Brazilian-2” (=A. sp.2 aff. fraterculus) and “Brazilian-3” (=A. sp.3 aff. fraterculus).
The recognition of the existence of different species in the AF complex estimulated the genetic, morphological as well as reproductive behavior analyses of the complex. These analyses have been made in general employing laboratory colonies and just a few using samples from natural populations of A. sp.1 (rev. Dias et al., 2015; Giardini et al., 2015, 2020; Hendrichs et al., 2015; Vaníèková et al., 2015) and A. sp.3 (Manni et al., 2015; Roriz et al., 2017, 2018). Moreover, there are scarce information about the A. sp.2, as well as about the geographical occurrence and host fruit use by the three Brazilian species. Besides, the existence of sympatric regions in which the three species coexist (Selivon et al., 2005a; Sánchez et al., 2018; Prezotto et al., 2019) provides a unique opportunity to verify whether there is free gene flow in natural conditions.
One additional difficulty for the formal description of the fraterculus complex species is underpinned by the Brazilian species, due to the description of A. fraterculus (Wiedemann, 1830) having been based on a single male type specimen (currently housed in the Naturhistorisches Museum of Vienna, Austria), which was collected in Brazil without further information. Another difficulty for determining the application of the name fraterculus to the Brazilian species is that much of the taxonomy of the genus, and particularly within the fraterculus species group, relies on the female genitalia (Stone, 1942).
Herein, is presented a set of chromosomic, genetic, morphometric, and mating activity data on the AF complex species from samples of natural populations from regions of Eastern Brazil–specially in Southeastern and South areas–but also including data from Northeast and Central regions of the country. A more detailed description of the karyotypes–especially the sex chromosomes– previously analyzed of the three species (Selivon et al., 2004, 2005a; Goday et al., 2006) was included. Analysis of the mitotic chromosomes were then employed for screening sympatric regions allowing detection of the species and the presence of eventual hybrids in natural conditions. Additionally, is presented for the first time, a comparative analysis of mating activities for the three species employing individuals sampled from natural populations. Employing information on 107 collection sites and based on climatic characteristics associated with their known geographic distribution, a model of their potential distribution was generated. Moreover, based on a morphometric analysis of male wings, a method to solve the identification of type specimen of Anastrepha fraterculus described by Wiedemann in 1830 is presented, in the hope of contributing to the taxonomic resolution not only for the Brazilian species, but also for the other morphotypes of the AF complex.
Materials and Methods
Source of Samples
Specimens of the A. fraterculus complex were sampled from infested fruits collected in 22 localities along the inland plateau and coastal plains of southern areas of eastern Brazil (Table 1) during the fruit seasons (usually January–April) in a period of 2002–2016 (Supplementary Figure 1). The infested host fruits were taken to the laboratory and maintained under standard conditions (Selivon et al., 2005a). From these fruits, larvae samples were used for chromosomal analysis to confirm the species identification (Goday et al., 2006), while others were kept to complete development to the adult stage. After emergence and identification, the adults were employed in molecular, morphometric, and mating activity analyses, as well as to obtain hybrid progenies under laboratory conditions. Supplementary Table 1 shows an additional 85 collection localities where fruits were sampled and the AF complex species recovered from the records of Laboratory of Evolutionary Biology of Fruit Flies, Instituto de Biociências, USP, coordinated by D. Selivon.
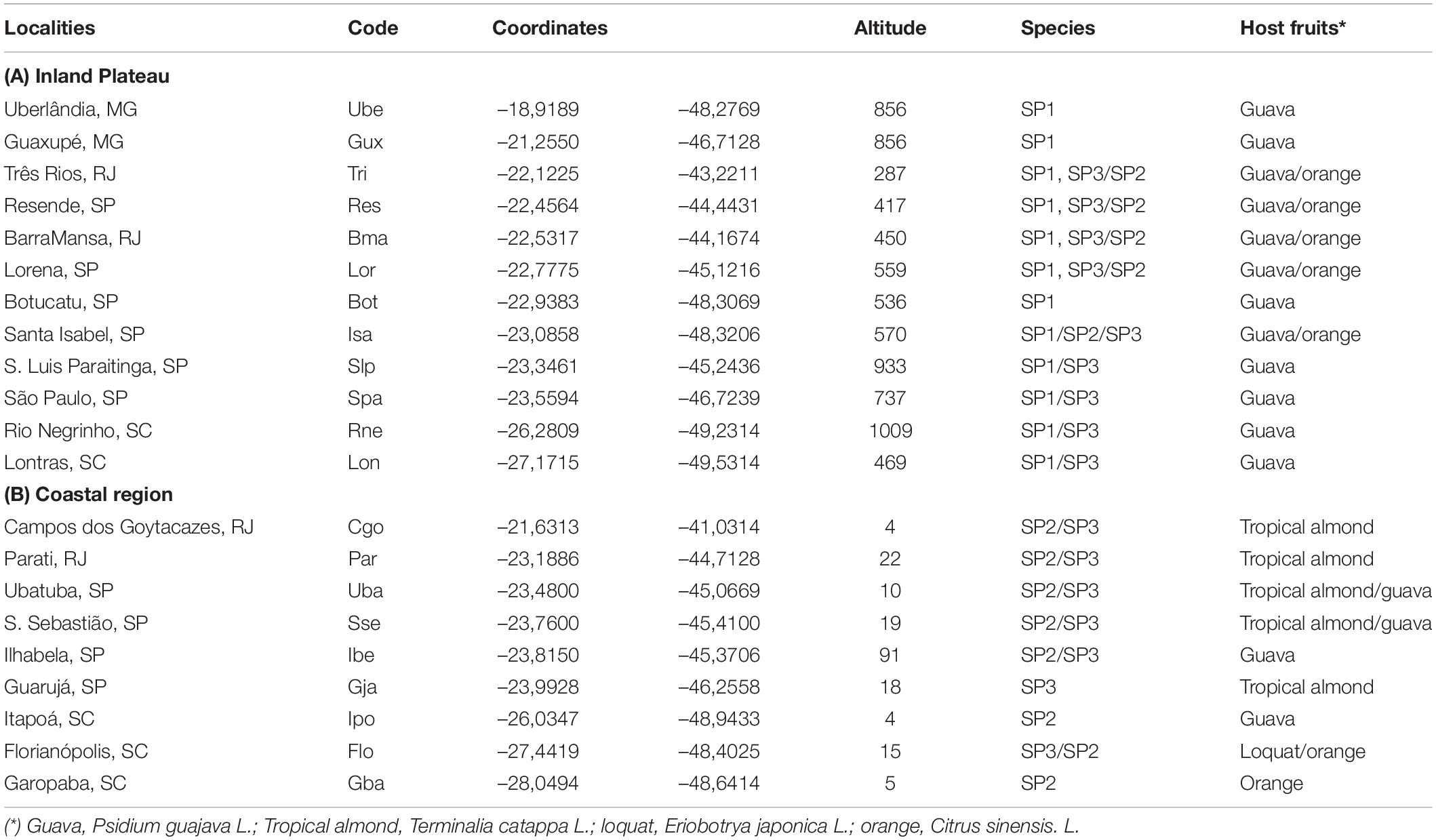
Table 1. Sites of collection, geographic coordinates, altitude, and host fruits of species of the AF complex.
Identification of the adults was based on previous information described in Selivon (1996); Santos et al. (2001), and Selivon et al. (2004, 2005a). As A. sp.3 adults present a differentiated mesonotum (Selivon et al., 2004) they were promptly isolated from A. sp.1 and A. sp.2 after the imagoes emerged. Since the differences between these two species are subtle (Santos et al., 2001), their host fruit and locality of collection were considered following information from Selivon (1996) and Selivon et al. (2005a). After this preliminary identification, karyotype analysis from mitotic chromosomes of the gonads of 5–6 adults were made (Solferini and Morgante, 1987) confirming the identification. For analysis involving hybrids between these species, recovered adults from the fruits were crossed accordingly the methodology previously described by Selivon et al. (1999, 2005a) using papaya (Carica papaya L.) as substrate for oviposition and larval development.
Karyotype Analysis
Chromosomes of mitotic cells of the three species were employed in two series of analyses: “Traits of sex chromosomes” and “Karyotype analysis in sympatric zones.” In the first, special features of the sex chromosomes were further described, as chromosomes length, size and location of heterochromatic blocks, including the chromosomes of hybrid specimens generate in the laboratory. In a second series, chromosome preparations made from larvae retrieved from fruits collected from natural populations (Table 1) allowed the identification of the species as well as the detection of eventual hybrids in the sampled localities. The number of larvae and of metaphases analyzed in each series are shown in the Results for the sake of clarity.
For chromosomal analysis the larvae were dissected, and neural ganglia and associated imaginal disks were isolated to obtain mitotic chromosome preparations, as described for Anastrepha species (Selivon et al., 2005a; Goday et al., 2006). Briefly, the imaginal disks and neural ganglia were immersed in 0.2% sodium citrate for 13 min, fixed in a solution of methanol, acetic acid, and water (11:11:2 v/v), and squashed between a slide and coverslip in 45% aqueous solution of acetic acid. After removal of the coverslip by freezing in liquid nitrogen, the preparations were fixed in 100% ethanol at –20°C, air-dried, and stored at 6°C in a sealed box with silica gel. Just before staining, the chromosome preparations were incubated in 2 × SSC for 45 min at 73°C, washed in ethanol, air-dried, treated for 3 min in 0.07 N NaOH, rinsed in ethanol, and air-dried again. The chromosomes were stained with DAPI (4′,6-diamidino-2-phenylindole) at a concentration of 0.5 μg/mL in 2 × SSC, rinsed in phosphate buffer (PBS), and mounted in PBS + glycerin (1:1) containing the anti-fade n-propyl-gallate. After analysis and image acquisition, the preparations were rinsed in buffer and the chromosomes were stained with Chromomycin A3 (CMA3) at 25 μg/mL in PBS buffer, rinsed in buffer, and mounted in PBS + glycerin (1:1) containing the anti-fade n-propyl-gallate (Goday et al., 2006). Following microscopic analysis and washing in water, the chromosomes were stained with Giemsa (Selivon and Perondini, 1997).
The chromosomal preparations were examined under an Olympus BX60 epifluorescence microscope using appropriate filters, and the images were digitalized using a Leica DC100 camera coupled to the microscope. The digital images were edited using Adobe Photoshop 7.0 software.
Chromosomes were measured using the IPWIN 32 software and the length was registered as the percent values relative to the total length of the diploid complement, according to Robertson (1957). Statistical comparison of chromosome length was made by a one-way ANOVA after the arc-sin transformation of the percent data. Size of the heterochromatic blocks of the X-chromosomes of A. sp.1 and A. sp.3 were compared by a t-test. The statistical tests were made employing the Statistica 7.0 (StatSoft Inc, Tursa, United States) software.
Analysis of ITS-1 Sequences
DNA extraction, PCR amplification, and analysis of the amplified fragments followed the methodology described by Prezotto et al. (2019). The amplified fragments (of about 900 bp) were cloned into Escherichia coli (One-Shot E. coli cells) using a Topo TA Cloning kit (Invitrogen and Applied Biosystems, Walthan, United States). Plasmid DNA was extracted using a Perfectprep Plasmid Mini Kit (Eppendorf, Hamburg, Germany). Fragments of each sample were sequenced using a BigDye reaction kit in an ABI Prism Automatic Sequencer (Applied Biosystems). The amplified fragments contained a complete ITS-1 and, after comparison with the ITS-1 sequence of nominal A. fraterculus (GenBank#AY775552), the partial sequences of the flanking genes 18S and 5.8S were trimmed off. Alignment of the sequence set was performed using the Clustal Omega software (Sievers et al., 2011), and genetic parameters were estimated by DnaSP 6.0 (Librado and Rozas, 2009) and MEGA 6.0 (Tamura et al., 2013). Relationships among the sequences were evaluated by phylogenetic inference using the maximum likelihood methodology (ML; Neil and Kumar, 2000), based on the best substitution model determined by the tool “Search the best Model” implemented in MEGA 6.0. The best model was GTR (general time reversible), according to Akaike information criteria (corrected). The initial trees for the heuristic search were obtained by automatic application of the bioNJ method to a matrix of pairwise distance estimated by the maximum composite likelihood method and selecting the tree topology with the superior log likelihood value. The phylogenetic ML tree was built using MEGA 6.0, complete deletion option and 1000 replications for the bootstrap test. ITS-1 sequences from A. obliqua (present paper) and A. ludens (EU305670) were employed as outgroups. The sampled populations are identified in the phylogenetic tree, and the amplified ITS1 sequences were deposited in GenBank under the codes JN223381, JN223384, JN223386, JN223387, JN223388, JN223389, JN223391, plus the sequences employed previously by Prezotto et al. (2019), JN223376-JN223380, JN223382, JN223383, JN223385, and JN223390.
Analysis of Mating Activities
The observations were aimed exclusively to investigate the mating time, as this component of reproductive behavior frequently differ among Anastrepha species, being the lighting intensity the extrinsic factor that determines their mating time (Aluja et al., 1999) whatever the observation conditions, laboratory, semi-natural (field cage) or natural (field) (Selivon, 1991).
The analyses were carried out with adult flies from samples identified in Table 1: A. sp.1 collected in guavas (Isa and Spa), A. sp.2 from orange (Isa) and tropical almond (Sse) and A. sp.3 from guava (Isa) and tropical almond (Sse). Recently emerged males and females from each natural population samples of each species were employed in the analysis. After identification, males and females were isolated until sexual maturity (ca. 15 days for males and 20 days for females). Then, they were employed for analysis of daily patterns of mating activity and mate partner recognition assays under laboratory conditions. All mating observations were conducted in a room with controlled temperature (25 ± 1°C) and relative humidity (60 ± 3%), in glass cages (10 cm × 10 cm × 20 cm) maintained near a wide window which allowed natural photoperiod conditions. The cages were supplied with water and food (3:1 mixture of sugar, corn protein hydrolyzate) according to Selivon et al. (2005a).
Daily Mating Time
For each sample, five replicas were analyzed, each one containing five mating pairs (10 individuals in the total), resulting in 25 mating pairs per sample. The assays were carried out over two consecutive days, from 06:00 to 19:00 h. After dusk the cages were kept in the dark until dawn the next day. The same procedure was adopted for analysis of daily time of mating pairs of hybrids individuals (A. sp.1 vs. A. sp.3) obtained as described above. The time the mating pairs remained engaged was registered for each species. Comparison of mating time (blocks of 06:00–09:00 h, 10:00–14:00 h, and 15:00–18:00 h) and duration of mating pairs between the species, were made by one-way ANOVA and Tukey test after arc-sin transformation of percent data. Statistical analysis was performed with the Statistica 7.0 (StatSoft. Inc) software.
Mate Partner Recognition
The experiments were made with the same protocol above described. In these assays, different pairs of species were confronted: A. sp.1 vs. A. sp.2, A. sp.1 vs. A. sp.3, and A. sp.2 vs. A. sp.3. A total of five trials were conducted for each pair. At dusk on the day before the assay, five sexually mature virgin males and five virgin females from each species in each trial were isolated and the mesonotum was marked by a non-toxic ink of different color for each species. They were placed in glass cages (10 cm × 10 cm × 20 cm). The assays were examined at hourly intervals, from 06:00 to 19:00 h and the number of observed mating pairs was scored in two categories, conspecific (1 × 1, 2 × 2, or 3 × 3) or inter-specific (e.g., 1 × 2, etc.) in these cases regardless of which species the male or female fly belonged to. The frequencies of mating pairs between the crosses in these tests were analyzed by one-way ANOVA, and the Tukey test for multiple comparisons after the Arc-sin transformation of the data. Statistical analysis was performed with the Statistica 7.0 (StatSoft. Inc) software.
Wing Morphometry
Morphometric analyses were performed on 13 population samples from eight localities in Brazil (Bot, Ibe, Pir, Lon, Spa, Sse, Uba, and Ube), all of which previously identified as described below. In some cases, samples coming from a single locality comprised more than one species living in sympatry (Table 1).
The right wing of each specimen was cut from its base, further dehydrated by a gradual alcohol series (50, 70, and 100%; holding them for 20 min at each step), placed in xylene for 2–3 min, and immediately mounted with Canada Balsam. Digital images were captured with a digital camera (Olympus C5050) adapted to a stereomicroscope (Zeiss, 2000, Jena, Germany). Permanent slides of the studied samples were deposited in the Laboratory of Evolutionary Biology of Fruit Flies at the Instituto de Biociências, Universidade de São Paulo (Brazil).
Information on the wing shape of both males and females was extracted through Generalized Procrustes Analysis (GPA), in order to remove non-shape variation (Goodall, 1991). Eighteen homologous landmarks (Supplementary Figure 2) were digitized on the wing of each specimen, identified by the intersection or ending of veins according to Hernández-Ortiz et al. (2015). The coordinates were then subjected to Principal Component Analysis (PCA), to sort data by major axes based on the measured variables. In addition, we a Canonical Variates Analysis (CVA) whose covariance matrices were previously screened for normality and homogeneity using the Shapiro–Wilk (Jarek, 2012) and Box’s M tests (Fox et al., 2020), further performing leave-one-out cross-validation, using the MASS package (Venables and Ripley, 2002). The statistical significance among groups was assessed by running a MANOVA followed by pairwise comparisons, setting p-values using the Benjamini and Yekutieli method with Multcomp package (Hothorn et al., 2008). Sex differentiation and centroid size variation among samples were estimated by a t-test, relying on 10,000 permutations using the RVAideMemoire package (Hervé, 2020).
To assess the identity of the male type specimen of the nominal species Anastrepha fraterculus (Wiedemann), an image of its wing was obtained from a photograph taken at the Naturhistorisches Museum of Vienna, Austria, provided by Dr. Carlos R. Vilela (Departamento de Genética e Biologia Evolutiva, Instituto de Biociências, Universidade de São Paulo). The wing landmarks used to distinguish the species currently identified in Brazil were scanned (Hernández-Ortiz et al., 2012; Prezotto et al., 2019). Then, a classification trial, using the wing Procrustes coordinates together with those of males coming from the three species surveyed was conducted, employing the “predict” function of the MASS package, thus yielding the probability that a new sighting belonged to any particular group (Venables and Ripley, 2002). This function sorts multivariate observations of an object X into the appropriate class, such that the weighted mean of the centroids in the group is at the origin. All statistical analyses were performed using the R program (R Core Team, 2020), and figures were drawn using the ggplot2 package (Wickham, 2016).
Bioclimatic Analysis
Herein, we aim to model the potential geographic distributions of the species across Brazil, and identify the bioclimatic variables that best predict their presence and the ones along which these species separate. After filtering the geographic records to leave a maximum of one record per raster cell for each species, our database (Supplementary Table 1, see above) is composed of 67 records for A. sp.1, 37 for A. sp.2, and 46 for A. sp.3. We applied the MaxEnt algorithm in R version 3.6.3 (R Core Team, 2020) to these occurrences and a subset of the bioclimatic variables provided by the WorldClim 2 database (Fick and Hijmans, 2017, 30 arc seconds cell resolution). Bioclimatic variables describe annual climatic trends for each raster cell (Supplementary Table 21).
Before modeling each species’ distribution, we cropped these variables to the full extent of Brazil, to which all of the species would be later projected. To avoid the overfitting of models due to have too many variables, we used the Variance Inflation Factor to retain only variables with up to 10 VIF units, using the package SSDM (Naimi and Araújo, 2016). This procedure led to retaining the following bioclimatic variables: Mean Diurnal Range, Isothermality, Mean Temperature of Wettest Quarter, Mean Temperature of Driest Quarter, Precipitation of Wettest Month, Precipitation of Driest Month, Precipitation Seasonality, Precipitation of Warmest Quarter, Precipitation of Coldest Quarter. Then, we followed the protocol described in the vignette of the R package dismo to model each fly species distributions using the subset of variables (Hijmans et al., 2017). These procedures included a four-fold partition of data into training and evaluation subsets, using 10,000 random background pseudoabsences (following Barbet-Massin et al., 2012). For 10,000 background pseudoabsences, a single iteration has been found to be enough to evaluate variables’ importance (Barbet-Massin et al., 2012). We did not filter occurrences to avoid spatial autocorrelation of records because these records come from inventories that would have detected any of the three species of flies in this study. Thus, we consider that the spatial aggregation of records for each species is natural, and not an artifact.
MaxEnt finds the variables with highest predictive ability of species’ occurrences based on their Permutation importance. The first, measures the increase in regularized gain contributed by the corresponding variable, in each iteration of the training algorithm. This measure of importance represents the percentual drop in training AUC when the variable is not included in the final model. These procedures allow for avoidance of the potential biases in the Area Under the receiver operating characteristic Curve (AUC) (identified in Lobo et al., 2008; Peterson et al., 2008). The AUC assesses the accuracy of model predictions, discriminating between records where the species is present and those where it is absent. AUC values over 0.5 indicate discriminations better than random (Hanley and McNeil, 1982). Finally, we used the fitted models to project the potential distribution of each species across the whole background region, Brazil. Besides, for each species, the three variables with highest contribution to the models were identified and presented in the results.
In order to find the bioclimatic variables along which the three Anastrepha species segregate, we visually assessed how the bioclimatic variables changed across the sites occupied by the three species. After choosing these variables, a Principal Component Analysis (Pearson, 1901) was applied to represent them. In this PCA, we also included altitude, latitude, and longitude. In this way, we could represent how the incidence of flies (i.e., the proportion of observations of each species for each segment of each geo-climatic axis) diverged in their use of climatic space, in a geographically explicit manner.
Results
Description of Karyotypes
Traits of Sex Chromosomes
The karyotypes of the three species of the fraterculus complex occurring in Brazil—A. sp.1, A. sp.2, and A. sp.3—have been previously described but chromosome length has been only compared between A. sp.1 and A. sp.2 (Selivon, 1996; Selivon et al., 2004, 2005a). Hence, herein a comparative analysis between A. sp.1 and A. sp2 with that of A. sp.3 was added. The analysis was based on chromosome preparations from five larvae from each species and five metaphase plates per larvae were analyzed. No interspecific differences were found in the length of autosomes and the most pronounced differences were observed for sex chromosomes (X and Y), which had unique characteristics for each species (Supplementary Table 3). As shown in Figures 1A–C, the sex chromosomes of A. sp.2 differed from those of A. sp1 and A. sp.3, in terms of the length, size, and position of the heterochromatic blocks: The X and Y chromosomes of A. sp.2 were longer than those of the other two species, while the sex chromosomes of A. sp.3 are slightly longer than those of A. sp.1 (Supplementary Table 3). The differences were more evident when the sex chromosomes of hybrids obtained in the laboratory were examined. The hybrid karyotypes are shown in Figures 1D,E. Additionally, a more detailed analysis of the size of the heterochromatic blocks of the X chromosomes of A. sp.1 and A. sp.3 was made. In A. sp.1, the heterochromatic block at the distal end was described as “slightly larger” than that in A. sp.3 (Goday et al., 2006). It was observed that in the hybrid females one of the X chromosomes had a more evident distal heterochromatic block than the corresponding one on the other chromosome (Figure 1D). From 5 to 6 hybrid females, 18 metaphases were analyzed, and the t-test showed that there was no significant difference between the proximal blocks of these chromosomes (t = –1.069, df = 17, p = 0.30). In contrast, the differences between the distal blocks were highly significant (t = 7.04, df = 17, P < 0.001**). These results clearly demonstrated that the X chromosomes of A. sp.1 and A. sp.3 can be distinguished by the size of the distal heterochromatic block, with that of A. sp.1 being larger than that of A. sp.3.
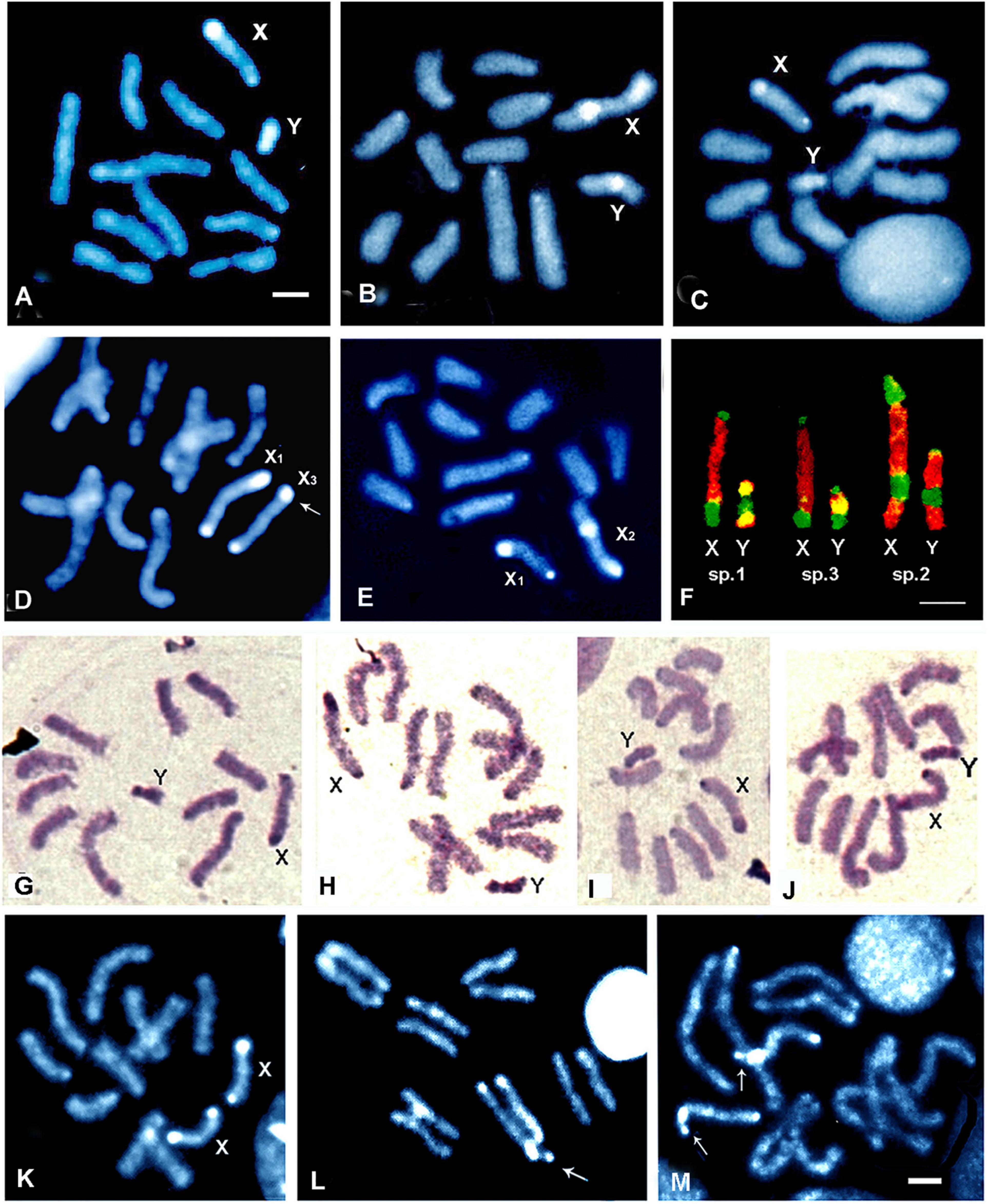
Figure 1. Karyotypes of A. sp.1 (A), A. sp.2 (B), and A. sp.3 (C) stained by DAPI. Note the heterochromatic blocks in the sex chromosomes; (D,E) Chromosomes of hybrid females obtained in laboratory and stained by DAPI. The arrow in (D) indicate the difference in size of the distal heterochromatic blocks of X1 and X3. (F) Chromosomes are stained sequentially by CMA3 and DAPI. Note the difference between the Y chromosomes of A. sp.1 and A. sp.3 in relation to the heterochromatin blocks (green, AT reach; yellowish, rich in AT + GC) and the larger size of the chromosomes of A. sp.2; (G–J) C-banded mitotic chromosomes of a A. sp.1 male showing the distinct structural configurations of the heterochromatic blocks of the Y chromosome; (K–M) The three genetic forms for the variant X chromosome in A. sp.1 females, (K) standard homozygous, (L) heterozygous, and (M) homozygous for the variant X. The variant X possess a larger distal heterochromatic block which is divided by a secondary constriction (arrows). Scale bar = 10 μm.
In addition, the karyotypes of A. sp.1 and A. sp.3 can be clearly distinguished by the peculiar characteristics of the Y chromosome, revealed by staining with the fluorochromes DAPI and CMA3 (Goday et al., 2006). As shown in Figure 1F, the Y chromosome of A. sp.1 has heterochromatic blocks rich in AT + GC (stained by DAPI and CMA3) at both ends and a block rich in AT (stained by DAPI) in the median region. In contrast, the Y chromosome of A. sp.3 shows an opposite structure: blocks rich in AT at the ends, and an intermediate block rich in AT + GC.
Thus, the morphological characteristics of sex chromosomes can unequivocally be used to identify the three species, as well as to detect eventual hybrids in population analyses based on karyotypes. The results of this analysis are described below.
Karyotype Analysis in Sympatric Zones
Based on differences existing among the sex chromosomes of the three Brazilian species of the AF complex, it was possible—by analysis of mitotic chromosomes—to infer the frequencies of different species in the sympatric zones in the Eastern and Southeastern regions of Brazil, as well as to detect eventual hybrids (Table 2). Chromosome preparations were made from 478 larvae sampled from collected fruits, 423 of which belonged to the fraterculus complex, while the other 55 did not have mitotic divisions or were derived from other fruit flies, such as Anastrepha obliqua and A. sororcula, whose karyotypes have previously been described (Selivon et al., 2005b).
In the Eastern Region of the inland plateau (valley of Paraiba do Sul River), out of 149 screened specimens, 88 were identified as A. sp.1, 35 as A. sp.2, and 26 as A. sp.3 This river descends from near the locality of Santa Isabel (Isa ≈ 800–900 m) toward its delta at the Atlantic Ocean, north of Rio de Janeiro (Campos dos Goytacazes, Cog). Additional collection along the valley, shows that the frequency of A. sp.1 decreased not being detected at sea level, while those of A. sp.2 and A. sp.3 increased at lower elevations (Figure 2). In the Southern Region of the inland plateau, among 52 screened larvae, A. sp.1 was found in sympatry with A. sp.3 in the two sampled localities, while A. sp.2 was not found in the sampled fruits (guavas and oranges) from those localities (Table 2).
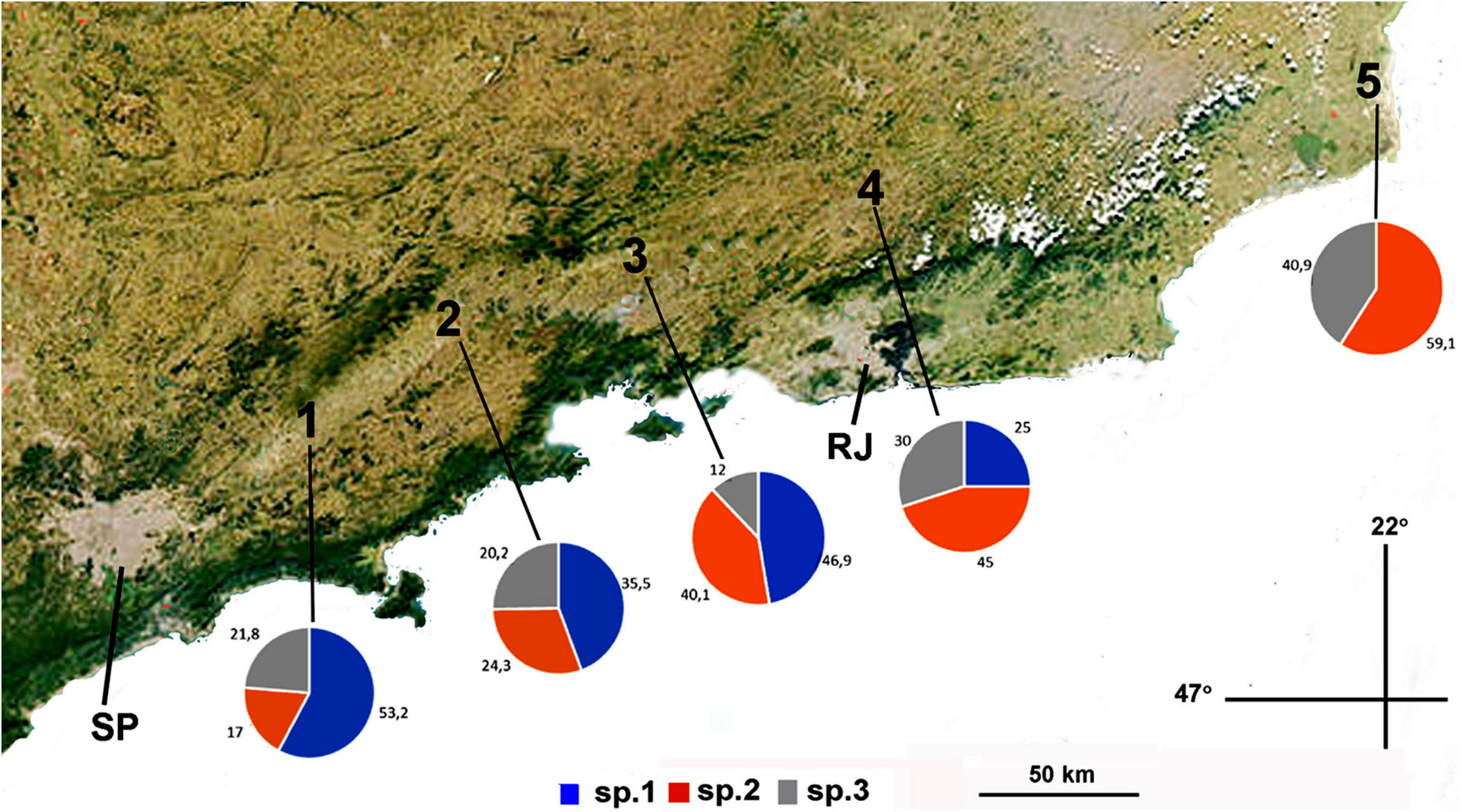
Figure 2. Frequencies of A. sp.1, A. sp.2, and A. sp.3 along the valley of Paraíba do Sul River. Identification of the species was determined by the chromosomic analysis. (1) Areas of Santa Isabel (800 m); (2) Lorena (550 m); (3) Resende (450 m); (4) Três Rios (280 m), and (5) Campos dos Goitacazes (sea level). SP, RJ, cities of São Paulo and Rio de Janeiro.
In the samples of the coastal region, from northern Rio de Janeiro (Cgo sample) throughout the most southern sample in Santa Catarina (Garopaba, Gba) (Table 2), A. sp.1 was not found, while A. sp.2 and A. sp.3 were found in sympatry in the majority of samples surveyed (n = 224 larvae).
It must be noted that only one female karyotypic hybrid exhibiting a small X of A. sp.1 and a large X of A. sp.2 (configuration of Figure 1E) was found in the sympatric zone of the Paraíba do Sul valley. Assuming that the sample size must be representative (123 larvae of A. sp.1 and A. sp.2), the estimated hybridization frequency was low in the area (0.81%). On the other hand, no hybrid karyotypes between A. sp.1 and A. sp.3 were found among the 164 specimens analyzed from the sympatric areas in the inland plateau, either of the Southeastern or South regions. Similarly, no hybrids were detected among the 224 individuals of A. sp.2 and A. sp.3 screened from the coastal samples in the Eastern or Southeastern regions (Table 2).
In the present study, variations on the structure of the Y chromosome of A. sp.1 and A. sp.3 were observed in preparations stained with the fluorochromes, and clearly seen in preparations submitted to C-banding. In mitotic divisions obtained from preparations of single individual larvae, different structural aspects were clearly observed in the C-band pattern of the Y chromosome. Figures 1G–J shows, as an example, the four metaphase plates of one A. sp.1 male, showing a distinct configuration of heterochromatic blocks in the Y chromosome, as well as the length of the chromosome. The analysis of chromosomes in A. sp.3 showed similar results, in terms of different “C-band morphologies” of the Y chromosome of single individuals.
A variant form of the X chromosome of A. sp.1 was found among the 43 specimens analyzed from the locality of Lorena (Lor) in the valley of the Paraiba do Sul River (Figures 1K–M). The X-variant had a distal heterochromatic block larger than the standard form, and usually appeared to be divided by a secondary constriction (Figures 1L,M). Among the 43 analyzed specimens, there were 24 females and 19 males. Among the 19 males, 3 (15.8%) were found bearing the variant X chromosome, while, within the 24 females, three karyotypes were found to exhibit the three genetic forms in relation to the X variant: 3 females (12.5%) were homozygous for the variant chromosome, 5 (20.8%) were heterozygotes, and 16 (66.7%) were homozygous for the standard chromosome.
Analysis of ITS-1
The present analysis includes samples from five collection sites from Brazil that had not been employed by Prezotto et al. (2019). The ITS-1 amplified fragments from 30 A. sp.1 adults (Tri, Slp, Gux), 10 of A. sp.2 (Slp), and 10 of A. sp.3 (Ibe), ranged from 540–547 bp. The sequences showed structural characteristics similar to those described for other sampled localities from Brazil, all belonging to sequence type TI variants (TIb, TIc, TId) (Prezotto et al., 2019). The ITS-1 fragments were rich in AT (70–84%) and structurally complex. The ITS-1 sequences had polymeric sub-regions rich in A or T, as well as other highly variable regions interspersed with conserved sub-sequences and with singular nucleotide variants (SNPs). Singular variations of nucleotides found in homopolymeric regions, but which occurred in all individuals of the samples, were considered as true nucleotide substitutions and not as amplification artifacts. When samples contained few individuals exhibiting one or two nucleotide substitutions—a variation that corresponds to a similarity equal to or greater than 99%—these sequences were considered equal and the sample was represented by only one sequence, the most frequent one, as previously demonstrated (Prezotto et al., 2019). For the following analysis, the obtained sequences were compared to the sequences described by Prezotto et al. (2019), A. sp.1: Uberlândia (Ube), Botucatu (Bot), Santa Isabel (Isa); A. sp.2: Santa Isabel (Isa), Três Rios (Tri), Ubatuba (Uba); A. sp.3: Ilhabela (Ibe), thus comprising a total of 60 A. sp.1 individuals, 40 A. sp.2 individuals, and 40 A. sp.3 individuals. Unique gaps and indels of different lengths were introduced in the sequences for alignment, resulting in sequences with 554 nucleotide positions. Thus, nine variable sequences were found for the AF complex in Brazil, indicating indels and/or nucleotide substitution at 46 sites along the fragment (Figure 3A). The singularities in the ITS-1 sequences of the species described by Prezotto et al. (2019) were also found in the samples herein analyzed: sequence types TIb for A. sp.1, TIc for A. sp.2 and TId for A. sp.3. The variability of ITS-1 was low within each species (Supplementary Table 4), and the pairwise comparison between the species revealed a small variability in nucleotide substitutions and genetic distance, but significant differences in population structure (Fst) (Table 3). In addition, we observed striking fixed differences (Hilton et al., 1994) between the species samples, 1.8–2.4% (Table 3 and Figure 3A).
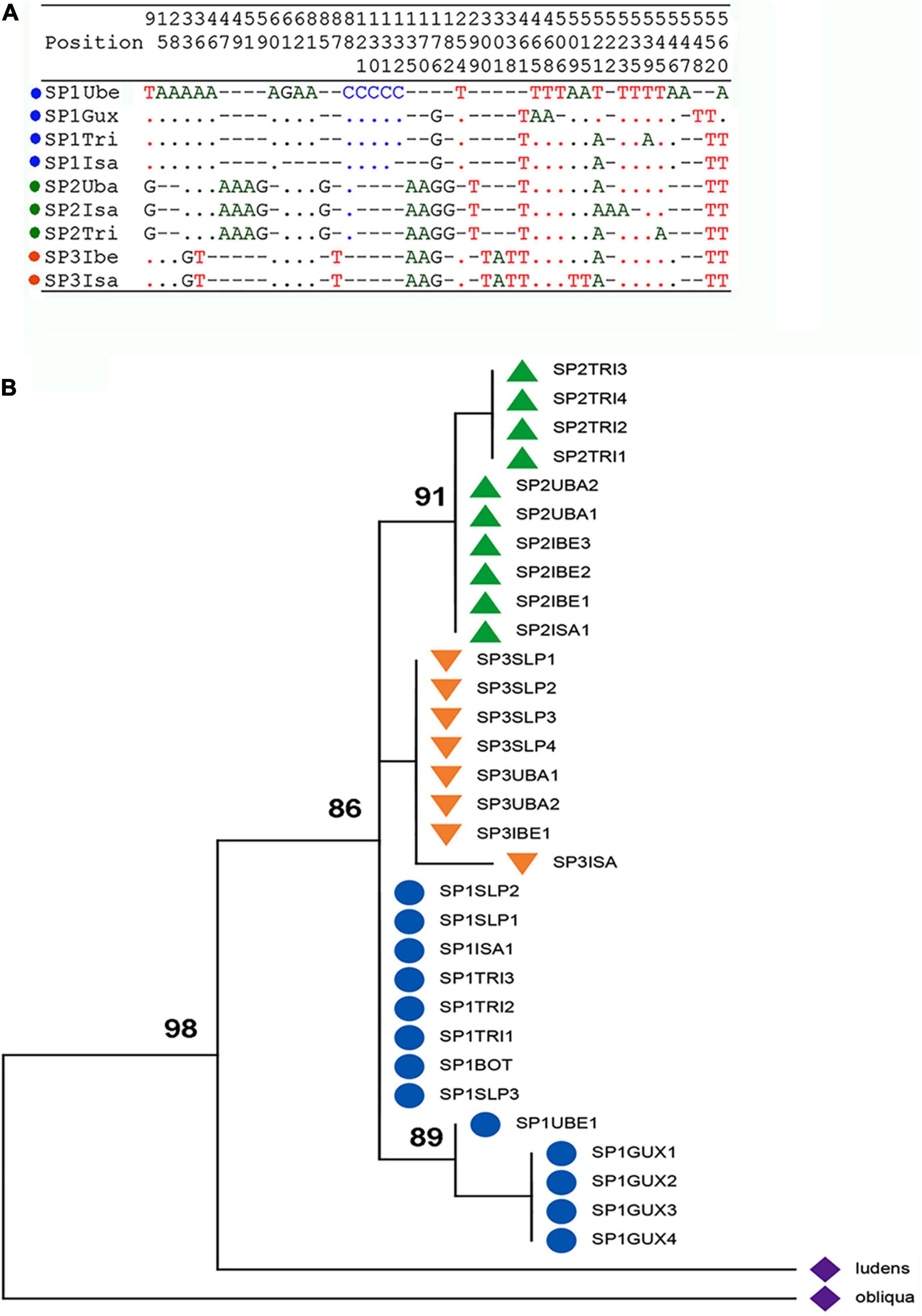
Figure 3. Analysis of ITS-1 sequence of the Anastrepha fraterculus complex from Brazil. (A) Polymorphic sites of the nine representative ITS-1 sequences; similarities are shown by dots and differences among the sequences by letters and gaps (–). (B) Phylogenetic relationships of the ITS1 sequences of the AF complex from Brazil. The evolutionary history was inferred using the maximum likelihood method based on the general time reversible model. The numbers at the nodes are bootstrap values (1,000 replications). Sequences of A. ludens and A. obliqua were used as outgroups.
Phylogenetic inferences between the sequences were obtained using the Maximum Likelihood methodology (Neil and Kumar, 2000). The topology of the ML tree (Figure 3B) shows that the AF complex sequences were gathered in a large clade that was isolated from the sequences of two other species—Anastrepha ludens and A. obliqua—that belong to the same infrageneric taxonomic group that is the fraterculus group (Norrbom et al., 1999). Although sub-divisions did exist within each species, it was clear that the sequences of A. sp.2 and A. sp.3 were grouped into distinct sub-clades, in relation to the sequences of A. sp.1. It must be noted that the different ITS-1 sequence types were clustered into distinct sub-clades, even those from sympatric samples: A. sp.2 and A. sp3 from Ubatuba (Uba) and Ilhabela (Ibe), A. sp.1 and A. sp2 from Três Rios (T), and all three species from Santa Isabel (Isa).
Mating Activities
As there was evidence of rare hybrid individuals between the species in the sympatric zone, we investigated daily mating activity by scoring the frequency of mating pairs in A. sp.1, A. sp.2, and A. sp.3. Since no significant differences were observed between the samples of two localities of each species as well as between the replicas, the data within each species were joined. Figure 4A shows the mean frequencies of mating pairs along the day. Mating pairs of A. sp.1 and A. sp.2 occurred preferentially between 06:00–09:00 h (94 and 90%, respectively) while for A. sp.3, mating activity occurred between 10:00 and 14:00 h (82%), with no mating activities before 10:00 h (Figure 4A). A one-way ANOVA test, based on arc-sin transformation of the data, was employed for the comparisons of the three blocks relative to mating time (06:00–09:00 h, 10:00–14:00 h, and 15:00–18:00 h) of the three species. The test showed significant differences (F = 8.123, p < 0.001), and the Tukey test indicate that there are no differences between mating time of A. sp.1 and A. sp.2 but both are distinct from mating time of A. sp.3. The time the couple remained engaged was significantly longer in A. sp.1 than in A. sp.2 and A. sp.3, while there were no significant differences between the two latter species (Supplementary Table 5).
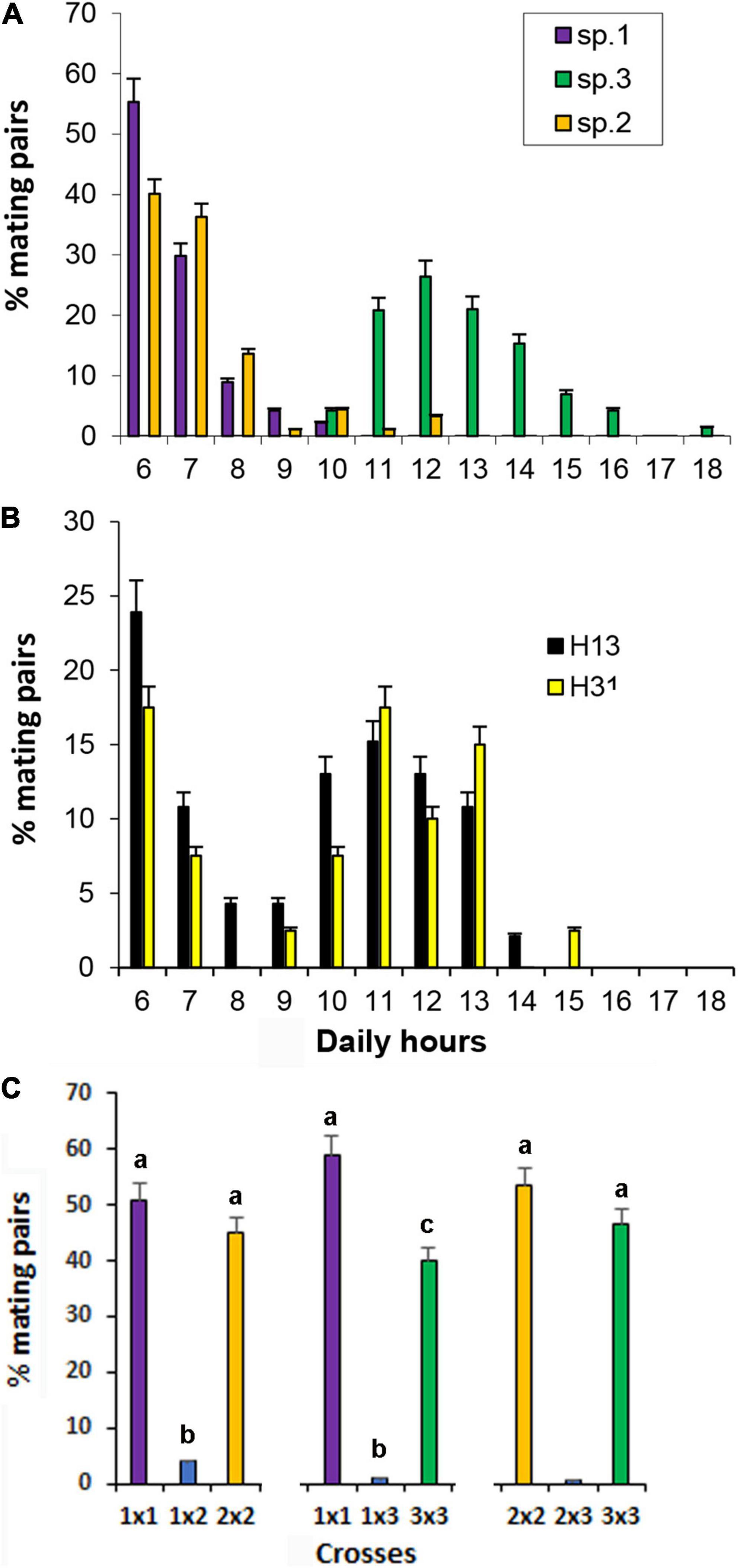
Figure 4. Mating activities. (A) Mating activities of the three specie and (B) daily mating activities of laboratory F1 hybrids from A. sp.1 and A. sp.3 crosses; (C) percent of homospecific and heterospecific mating pairs.
The mating time was scored also for the two types of F1 hybrids: H13 (female sp.1 vs. male sp.3) and the reciprocal ones, H31. The other possible hybrids—sp.2 vs. sp.3 and sp.1 vs. sp.2—were not analyzed as they occurred in insufficient numbers for analysis. The data indicated that the hybrids did not show the prevalent mating time of either of the parental species: Pair mating were observed to occur from 06:00 to 15:00 h, but according to a bimodal distribution, with two peaks, one from 06:00 to 09:00 h and another from 10:00 to 13:00 h for both reciprocal hybrid individuals (Figure 4B), with no significant differences between them (ANOVA, F = 0.386, p = 0.546). The time the couple remained engaged did not significantly differ between the two reciprocal hybrids (Supplementary Table 5).
In the mating partner recognition essays, a total of 55 mating pairs were registered in trials of A. sp.1 vs. A. sp.2, 68 in trials of A. sp.1 vs. A. sp.3, and 58 in trials of A. sp.2 vs. A. sp.3. The mean frequencies of mating pairs of the different crosses are shown in Figure 4C and indicate that the vast majority of mating pairs were conspecific and interspecific mating pairs were rare. Statistical analysis of arc-sin transformed data showed significant difference between the crosses (ANOVA, F = 1.112, p = 40.17). Tukey test showed that significant differences occurred between the homotypic mating pairs and the correspondent heterotypic crosses and that there are no differences between homotypic crosses of A. sp.1 vs. A. sp.2, and of A. sp.2 vs. A. sp.3 but lower mating pairs of homotypic A. sp.3 in relation to homotypic A. sp1.
Morphometry and Differentiation of Brazilian Species
Analysis of Male and Female Wings
The outcomes of the MANOVA were used to assess the variability between Brazilian species based on wing shape which proved to be helpful for discriminating against both males (F = 129.41, Wilk’s λ = 0.0666; DF = 2/91; P < 0.0001) and females (F = 236.37, Wilk’s λ = 0.0246; DF = 2/89; P < 0.0001), including all Hotelling pairwise comparisons, which were also significant (P < 0.0001; Supplementary Table 6).
Furthermore, the independent CVAs to assess the separation between males and females for each species disclosed substantial differences in all cases: A. sp.1 (t = 17,645, P < 0.001), A. sp.2 (t = 16.62, P < 0.001), and A. sp.3 (t = 15.53, P < 0.001). This means that wing shape enables discrimination not only between species, but also between sexes for each one of them (Figure 5A). The classification of the specimens, in accordance with our predictions, showed that females exhibited a higher rate of individuals accurately classified (90.9%), in comparison with that for males (81.4%; see Table 4).
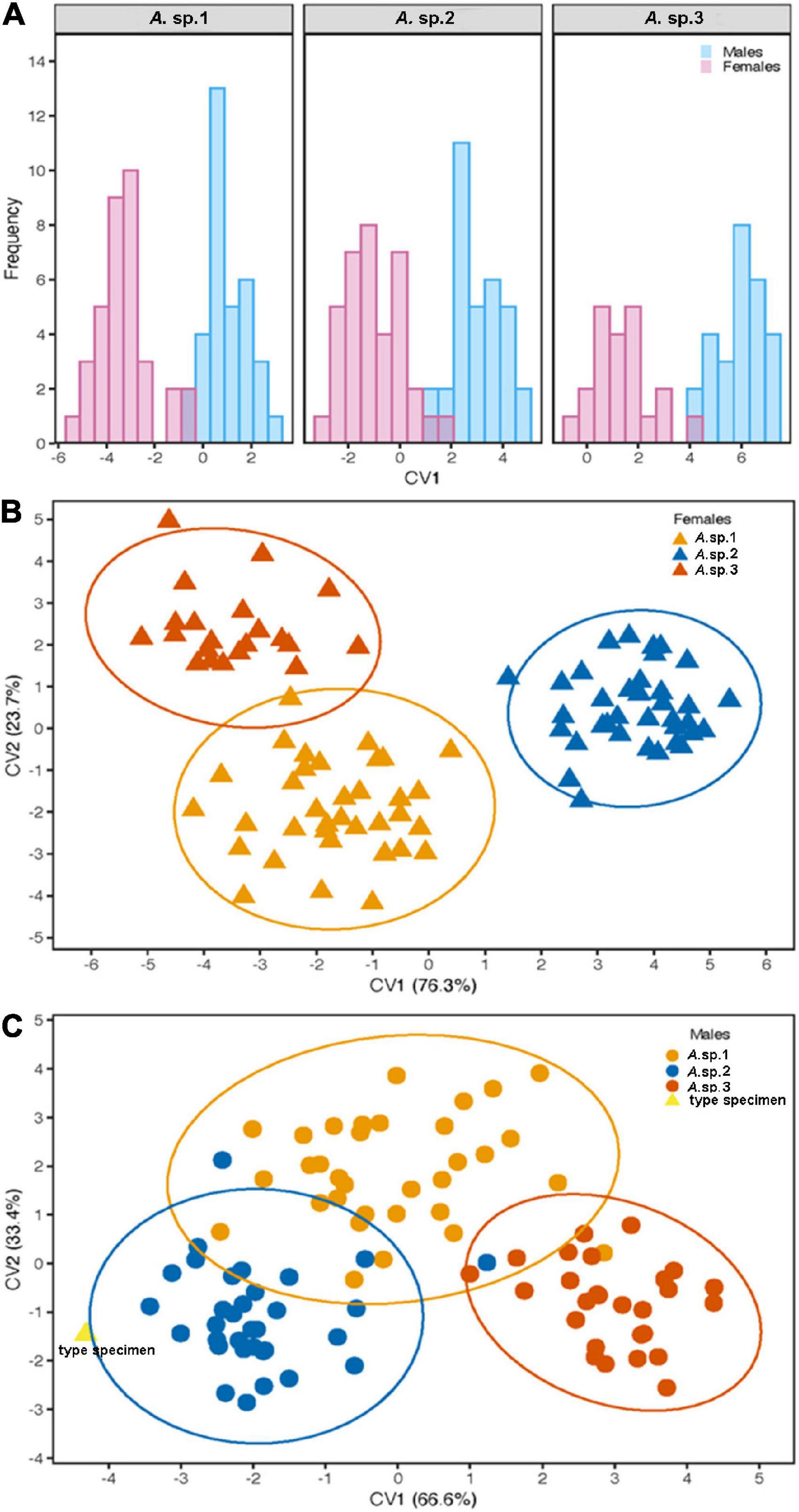
Figure 5. Wing shape of the AF complex. (A) Canonical Variate Analysis based on the wing shape, revealing the separation between males and females for each species, using the scores of the CV1. (B,C) Scatterplot of the specimens, as results of the CVA used to segregate three morphotypes found in Brazil: (B) female discrimination; (C) male discrimination, including the type specimen near A. sp.2 according to the predictive model. Circles indicate confidence intervals at 95%.
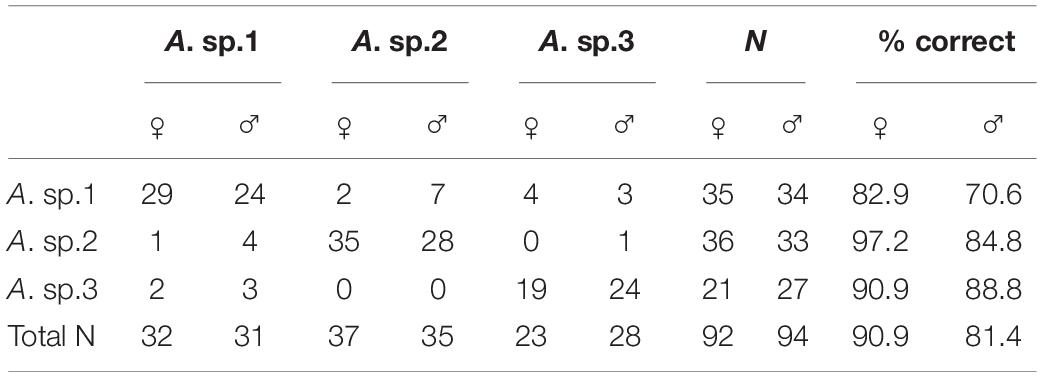
Table 4. Classification matrix for leave-one-out cross validation of individuals of the Brazilian species based on sexes.
To assess the overall wing size between species, we calculated the centroid size, leading to significant variations in males (F2.91 = 7.85, P < 0.001), as the wing of A. sp.2 turned out to be smaller than that of A. sp.1 (P = 0.001). Furthermore, comparisons among females also revealed statistically significant variations in centroid size (F2.89 = 8.39, P < 0.001), as specimens of the A. sp.3 displayed larger wing size than A. sp.1 and A. sp.2 (P = 0.002 and P = 0.001, respectively).
Predictive Classification of Type Specimen of Anastrepha fraterculus
Given the information that the origin of the Anastrepha fraterculus type specimen (Figure 6). was Brazil (Wiedemann, 1830), the specimen should be representative of one of the species occurring in this country. Our results demonstrated significant differences in wing shape among the three species of the fraterculus complex in Brazil which allow for the recognition of these species. This included differences in the wings of the males, which could thus reveal the identity of the type specimen. To explore the specimen identity, we used the CVA scores gained from male-based species segregation. The predictive method applied stated that the highest likelihood of accurate classification for the specimen (0.999) would be within A. sp.2 (Figure 5C). Meanwhile, the likelihood classification in the two other species, A. sp.1 and A. sp.3, was nearly zero (p < 0.0001).
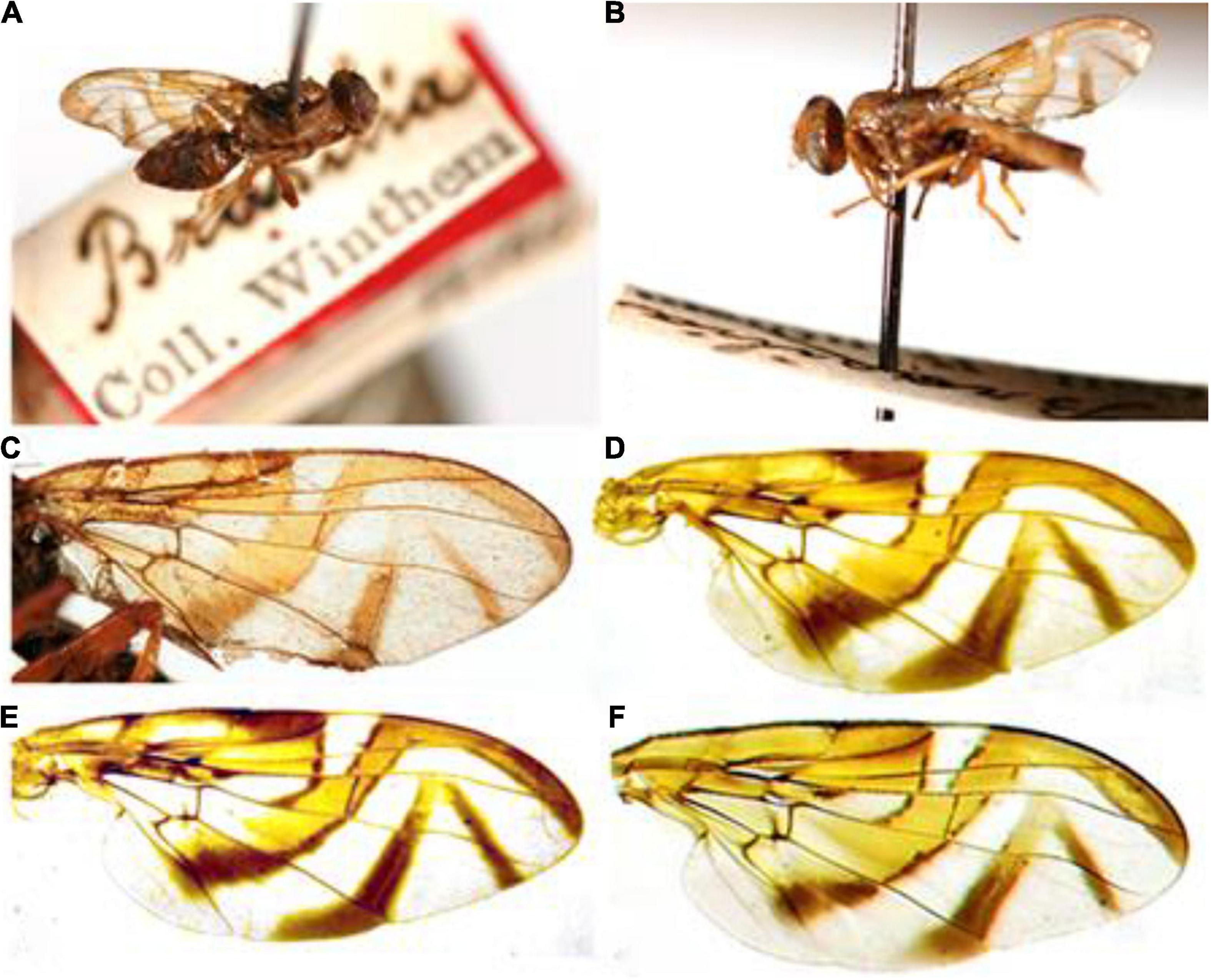
Figure 6. The Anastrepha fraterculus type specimen. (A,B) Micrographs of the male type specimen of Anastrepha fraterculus housed in the Naturhistorisches Museum Wien (NMW) (photos by Carlos Ribeiro Vilela); (C) right wing of the type specimen used for morphometric examination; (D) wing of A. sp.1; (E) wing of A. sp.2; (F) wing of A. sp.3.
Bioclimate Characteristics of Species
The occurrences that supported the bioclimatic analyses are shown in Table 1 and Supplementary Table 1, comprising a total of 107 collection sites in Brazil. The AUC values of our geographic models were all over 0.9, indicating a high capacity of bioclimatic variables to discern inhabited localities from random background locations (Table 5). The precipitation of the warmest quarter, isothermality (an index of thermal seasonality) and the mean diurnal range were the most important variables to predict the presence for all the three species (Table 5). The produced maps, which include the models of potential geographic distribution and occurrences, suggest that A. sp.1 reaches further off the coast, off the equator, and up to higher altitudes than the other two species. Instead, A. sp.2 and A. sp.3 were mostly distributed across the Brazilian coast (Figure 7).
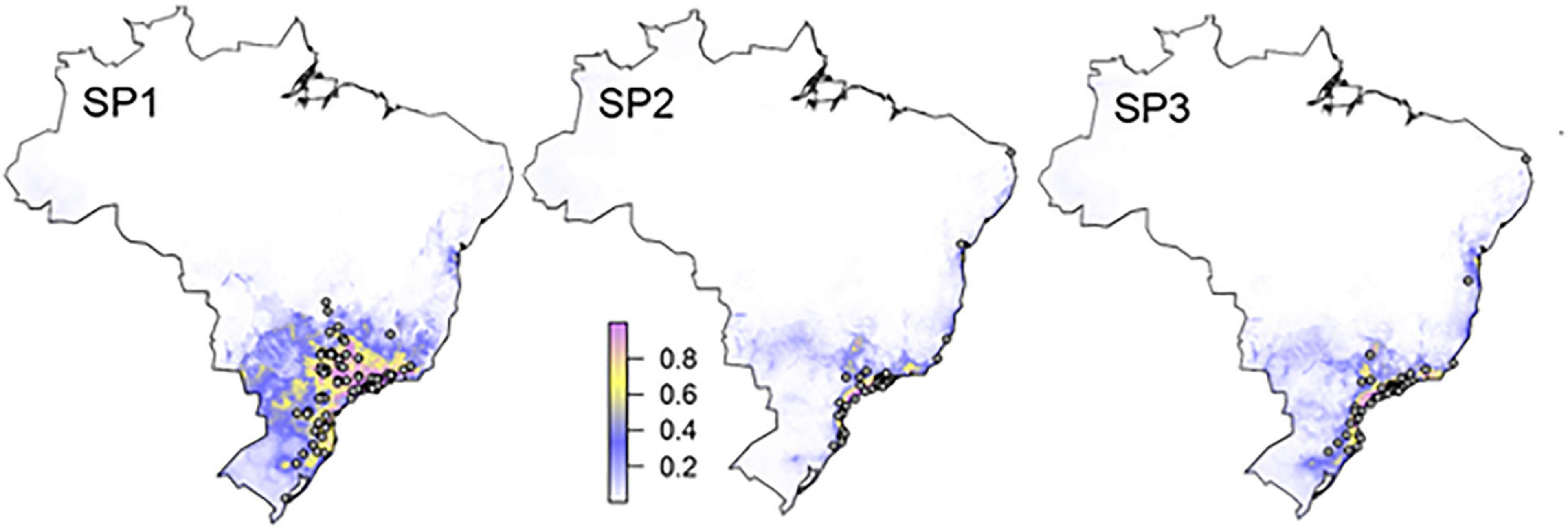
Figure 7. Distribution of three species of Anastrepha from the fraterculus complex in Brazil. Points represent known localities and colors toward the green indicate higher probability of occurrence, according to the MaxEnt model which best discriminate presence localities from a random set of localities, based on 14 bioclimatic variables, taken from WorldClim 2.
Geographic and bioclimatic variables across the known localities of species demonstrated that A. sp.1 extended over higher altitudes and further away from the equator and coast, thus experiencing higher exposure to daily and seasonal thermal variation and pluviometric seasonality, as well as drier sites, particularly during winter. Instead, A. sp.2 and A. sp.3 were more similar in geographic and bioclimatic variables. Figure 8 shows the incidence of each species, with respect to the three principal axes of variation in geographic and climatic variables, across Anastrepha species. In this analysis, PC1 describes increases in the seasonality of precipitation (bioclimatic variable Bio 15; loading = 0.82), distance from the coast (represented by longitude; loading = 0.75), and distance from equator (latitude; loading = 0.92). PC2 describes increases in the diurnal and annual temperature range (Bio 2, loading = 0.88; Bio7, loading = 0.90, respectively). PC3 represents a decrease in annual precipitation (Bio 12, loading = –0.90) and increasing altitude (loading = 0.68). Thus, A. sp.1 dominance increased with PC1 and PC3 and presented a more irregular pattern with respect to PC2. The other two species followed similar patterns, in a manner complementary to A. sp.1 (Supplementary Figure 3). Apart from extending across a wider climatic niche and geographic space, A. sp.1 appeared to be more general than A. sp.2 and A. sp.3, in relation to the number of host fruits.
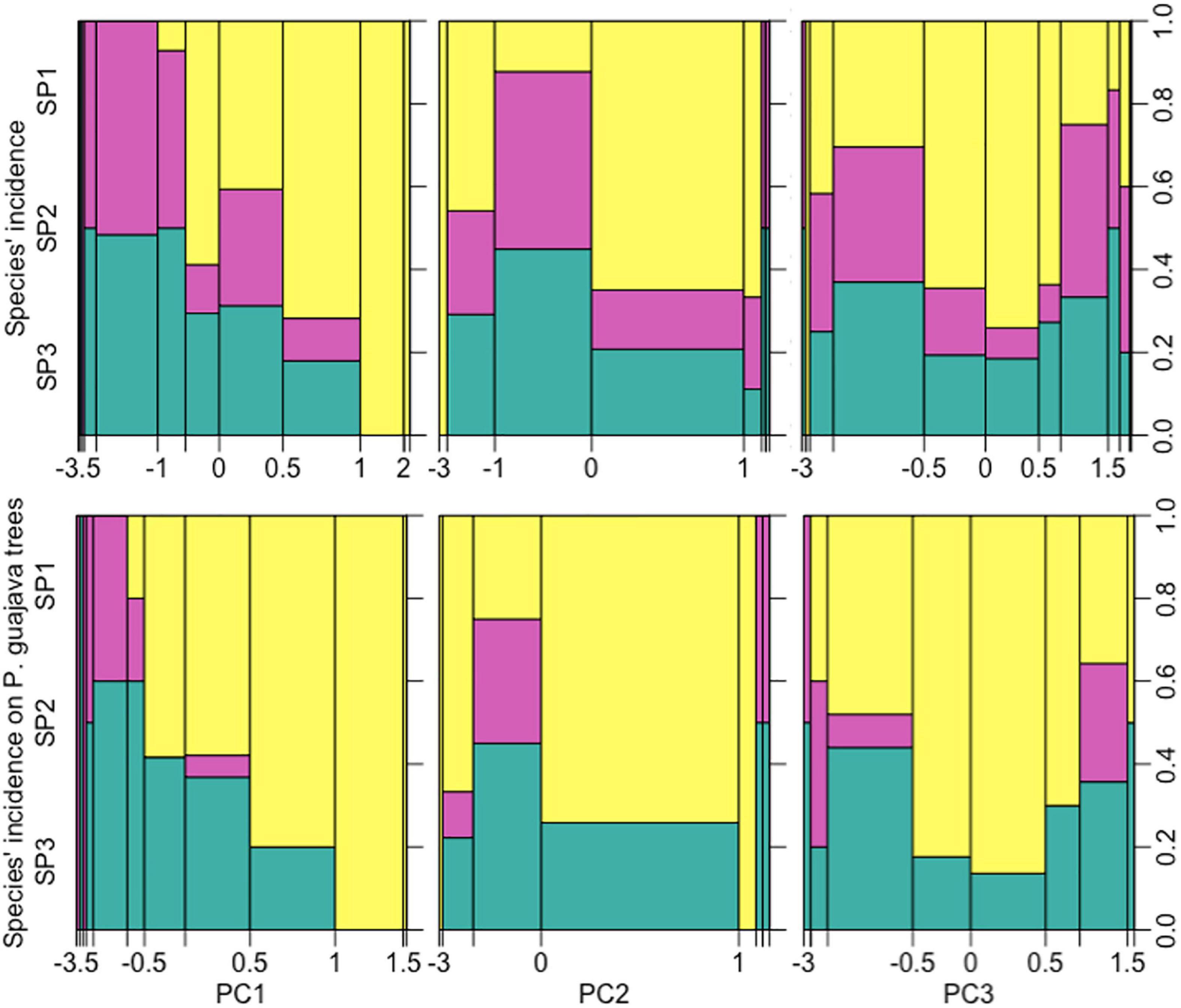
Figure 8. Changes in the relative frequency of A. sp.1 (yellow), A. sp.2 (purple), and A. sp.3 (green) across equally distributed segments of principal components representing climate and geography. Wider segments indicate that more records were made across the respective PC ranks. The length of each colored column represents the proportion of each species in records for a given segment in conditions. PC1 represents increases in precipitation seasonality and distance from the coast and increasing proximity to equator. PC2 depicts increases in the diurnal and annual temperature range. PC3 represents decrease in annual precipitation and increasing altitude.
Discussion
Karyotypes and ITS-1 Sequences
Chromosomal analyses were conducted in accordance with previous karyotype descriptions of the three species (Selivon et al., 2004, 2005a; Goday et al., 2006). In the present study, we demonstrated that these karyotypes are, in fact, representative of the different species, as they were shown to be constant over a much larger number of specimens as well as of sampled areas. From the karyotype analyses carried out in the eastern Brazilian coastal and inland regions, several relevant conclusions emerged: (1) No polymorphism in the Y chromosome was detected in the samples of the three species. The observed variation of the heterochromatic blocks in the Y chromosomes of A. sp.1 and A. sp.3 occurred in different metaphase plates of the same individual, indicating that they are related to different stages of the chromosome condensation process during mitosis of different cells. In samples of A. sp.1 from Argentina, six different forms of Y chromosome have been described (Basso et al., 2003, 2019) but an extensive analysis of mitotic chromosomes of Argentinean A. sp.1 from natural population samples demonstrated that only two forms of the Y chromosome appears to occur (Giardini et al., 2015, 2020); (2) An X chromosome variant in A. sp.1 was found in South Brazil (Selivon, 1996) and in Argentina (Basso et al., 2003; Giardini et al., 2015, 2020). In the present analysis, this variant chromosome X has been detected also in a population sample of A. sp.1 from the Paraiba do Sul River valley (Lorena, SP); however, it must be noted that, in both geographical areas—Argentina (Giardini et al., 2020) and Brazil (present data)—the frequency of males bearing the variant X chromosome was lower than among the females. According to Giardini et al. (2020), the additional satellite heterochromatic block could be the result of a translocation of the heterochromatic block at the proximal end of the X-chromosome to its distal end. Assuming that the translocated block might also contain parts of euchromatin, the lower frequency of males bearing the X-variant in Argentina as well as in the sample described herein, could be the result of differential silencing of euchromatic genes near the translocated heterochromatic blocks, resulting in the lower survival of a fraction of flies, a mechanism of heterochromatinization known in several organisms (Elgin and Reuter, 2013); (3) Species frequencies were related to altitudinal transects. It is notable that the karyotype analysis showed that A. sp.1 was the most frequent in higher altitude regions, decreasing in regions of lower altitude, and not detected in the coastal zone. A. sp1 seems to differ from A. sp.2 and A. sp.3 in this respect. Some environmental factor(s) that may have a differential effect on A. sp.1, relative to the other two species, as has been previously suggested by Sánchez et al. (2018), were also invoked in the present biogeographic analysis. Genetic differentiation related to altitudinal differences among populations of A. sp.1 and A. sp.3 from Brazil, has also been reported by Manni et al. (2015) in a population genetic approach using Simple Sequence Repeat (SSR); (4) In sympatric regions, hybrid karyotypes were not detected in a significant sample of individuals, except for one female who had an X chromosome of A. sp.1 and another of A. sp.2. This indicates that there exists no free gene flow between the species, even though they were sympatric and even syntopic. Similarly, no hybrids were detected between A. sp.2 and A. sp.3 in the coastal region. This fact, therefore, leads to the conclusion that reproductive barriers (sensu Mayr, 1963) or robust recognition systems (sensu Paterson, 1985) do exist between these species.
The present ITS-1 analysis of samples of the Brazilian species showed that, although the nucleotide variability was low, the sequences from the three species showed structural differences, including SNPs, indels, and other fixed differences, as previously described (Prezotto et al., 2019). ITS-1 sequences have been an important marker to identify species within complexes in several types of organisms, and were also adopted as the molecular marker to distinguish two species of the Bactrocera dorsalis complex by the International Plant Protection Convention (Barr et al., 2021).
Moreover, the genetic divergence estimated in present analysis indicated a recent divergence of the AF complex in accordance with previous studies carried out with different markers of the AF complex and the fraterculus species group (Morgante et al., 1980; Smith-Caldas et al., 2001; Selivon et al., 2005a; Segura et al., 2011; Prezotto et al., 2019). In a recent phylogenomic analysis of some species of the fraterculus group from Brazil, Congrains et al. (2021) also suggested a recent evolutionary origin and rapid diversification for the fraterculus species group and concluded that there are three evolutionary lineages among their A. fraterculus samples. However, the authors were not able to associate all of these lineages with specific ITS-1 sequences. Indeed, the two sequences the authors described as being similar with type TII, which is found in the Mexican morphotype, did not have the main attributes (indels) that characterize this sequence type. In fact, these two sequences had characteristics of sequence type TI, accordingly to the description by Sutton et al. (2015) and Prezotto et al. (2019). The meaning of these discrepancies may not be clarified with the available information.
The phylogenetic analysis of ITS1 sequences revealed a tree topology in which the three Brazilian species clustered in distinct sub-clades. Heterogeneities were observed within each sub-clade, but all sequences contained the major peculiarities defining the sequence type of each species (Prezotto et al., 2019), the heterogeneities comprising small differences in nucleotide positions.
Mating Activities
The rarity or absence of hybrid karyotypes in the samples from sympatric zones indicated that there exist strong reproductive barriers between the three Brazilian species of the AF complex, impeding or reducing gene flow between them. Reproductive barriers can be pre- or postzygotic and it is assumed that the prezygotic barriers are established when hybrid fitness is reduced, according to the classical genetic model of speciation (Dobzhansky, 1937, 1970); hence, reproductive isolation can be useful as an operational criterion for the identification of evolutionary independence between distinct lineages, as the rarity or absence of free gene flow provides stronger evidence of independence in organisms with sexual reproduction. Eventually, some difficulties do exist; for example, if populations do not overlap, there is no way of knowing whether they are reproductively isolated. This is not the case reported here, as there were regions in which the three Brazilian species so far detected have been found in sympatry. But which sort of mechanisms would assure reproductive isolation in sympatric areas? One of the most frequent prezygotic barriers is ethological isolation (Mayr, 1963). In fact, the daily pattern of reproductive activities is distinct for many Anastrepha species (Aluja et al., 1999), including different species of the fraterculus complex (Rull et al., 2013; Abraham et al., 2014; Devescovi et al., 2014; Roriz et al., 2017). Analyses of the daily pattern of reproductive activities revealed significant differences between A. sp.3 and the other two Brazilian species. Similar differences were also reported between A. sp.1 and A. sp.3, by Roriz et al. (2017) and also in pheromones of A. sp.1 (Roriz et al., 2018). However, it should be noted that the daily pattern herein observed did not agree with that of those authors. They reported two peaks of mating activity for both species, one in the early morning, and another in the middle of the day as well as for hybrids between the two species. In the current study, no reproductive activities of A. sp.3 were observed before 10 am, nor for A. sp.1 in the middle of the day. Nonetheless, our results agreed with other studies on the reproductive behavior of A. sp.1, conducted with laboratory colonies (Vera et al., 2006; Cáceres et al., 2009) and with samples from natural populations (Petit-Marty et al., 2004). We could attribute such discrepancies to the fact that our tests were conducted with samples from natural populations while Roriz et al. (2017) used laboratory colonies. In fact, the patterns described by Roriz et al. (2017) for the two species and for the hybrids were similar with the mating time of the hybrids described in the present analysis. The maintenance of two activity peaks in the mating time in the reciprocal hybrid individuals, each peak characteristic of the parental species, indicates that there is no dominance relationship in the expression of this phenotypic character. Hence, the discrepancies relative to the experiments of Roriz et al. (2017, 2018), remain elusive with the available information. Despite these differences, the present data and the results of Roriz et al. (2017) showed that mating times for pairs of A. sp.1 spent a longer time coupled than those of A. sp.3. Actually, longer mating pairs of A. sp.1 have also been observed in comparison with pairs of the Mexican morphotypes (Rull et al., 2013), but their mating times were shorter compared to the Andean morphotype (Devescovi et al., 2014). The differences in mating duration involving homotypic or heterotypic crosses seem not to affect the reproductive success of flies (Costa et al., 2012; Abraham et al., 2014; Roriz et al., 2017), suggesting—based on Sivinski et al. (1999)—that other factor may be involved in the mating duration of species from the AF complex. The hybrids H13 and H31 obtained in the present analysis spent a similar duration coupled but differed from the data of hybrid H31 described by Roriz et al. (2017), showing longer copula than hybrid H13.
Considering that hybrids between A. sp.1 and A. sp.2 were rare in the analyzed samples, that previous reproductive isolation tests revealed the existence of postzygotic incompatibility between these species (Selivon et al., 1999, 2005a) and the preference of conspecific mating partners in the mating choice tests (present data), indicated that prezygotic barriers exist between them. In these cases, reproductive barriers possibly reside in other components than the daily mating time since reproductive behavior involves an intricate visual and chemical signaling system (e.g., involving pheromone and acoustic emissions) in several Anastrepha species (Aluja et al., 1999; Sivinski et al., 1999), including species of the AF complex (Dias et al., 2015; Vaníèková et al., 2015; Roriz et al., 2017, 2018).
The choosing mating partners essays carried out between species revealed a high frequency of intraspecific choices. This reinforces the hypothesis that the prezygotic isolation between A. sp.1 and A. sp.2 might be determined by another reproductive component than the mating time. A high degree of discrimination between mating partners would explain the absence of or the rarity of hybridization events in sympatric areas revealed by chromosomal analysis. Another additional factor preventing gene flow between species of the AF complex could be host fruit usage as will be discussed in another section.
Bioclimatic Analysis
Since the AF complex involves pests of fruitcultures, identifying their potential distribution is relevant for guiding sampling strategies on areas of interest for further investigations. Likewise, the best explaining variables should also help identifying natural restrictors to their invasive potential, so as to subsidize control pest strategies. Although our knowledge on the distribution of Anastrepha species and their requirements for survival is still limited and restricts the ability of our Bioclimatic analysis of predicting their occurrence, the modeling algorithm that we used has been proved to perform well across samples of localities of different sampling sizes (Wisz et al., 2008). Our bioclimatic analysis suggests that A. sp.1 could be more likely to become an invasive species as it occupies a wider set of potential areas, including ones with higher seasonality in temperatures, as long as rains happen during the hot period of the year. A. sp.2 and A. sp.3 could still invade at least areas with a climate similar to the Brazilian coast.
Nonetheless, since species ranges can be restricted by purely historical reasons (i.e., time for dispersal, presence of biogeographical barriers), further laboratorial investigations of their thermal physiological tolerance are needed to set the true climatic boundaries for their survival, as has recently been achieved for A. sp.1 (Saruhashi, 2019), and other species of fruit flies (Gutierrez et al., 2021). Given the differences in the climatic niche of A. sp.1 in relation to the other two species, it is necessary to point out that in a scenario of climate change, global warming can profoundly impact the geographic occurrence of the AF complex in Brazil, for example, promoting the expansion of A. sp.2 and A. sp.3 on the Inland plateau. Apart from differences in climatic niche and geographic space, A. sp.1 also diverges from A. sp.2 and A. sp.3, in relation to the breadth of exploitation of host fruit, being apparently more generalist than the other two species as A. sp.1 was registered in 13 distinct host fruits while A. sp.2 in five and A. sp.3 in four, as shown in Table 1 and Supplementary Table 1. However, further studies are needed to disentangle host usage patterns from changes in the geographic availability of host plants.
Hypotheses on Geographical Patterns and Host Fruits Exploitation
Taking into account that A. sp.1 was detected in majority of fruits sampled in the Inland Plateau, including guavas, a native host, and that it was not detected in the samples from Coastal region (Table 1 and Supplementary Table 1), it is reasonable to suppose that this species would be resident in inland plateau. On the other hand, since A. sp.2 was detected in several samples from the Coastal region, including the native guavas (Supplementary Table 1), it is reasonable to consider that it would be resident in that region. Hence, the data are suggestive that their evolutionary histories may have occurred in an allopatric scenario, as was proposed by Prezotto et al. (2019). The similarities on mating time activities between these two species (present data) reinforce this hypothesis.
Notwithstanding, A. sp.3 was detected in both regions, Inland Plateau and Coast, but the present data show it overlap with A. sp.2 in its bioclimatic space being them distinct from A. sp.1 (Figure 7). Hence, we may suppose that A. sp.3, would also be resident in the coastal region. The distinctiveness in the daily mating time between A. sp.2 and A. sp.3 (Figure 4A) could be an indicative of this supposition. However, the possibility that the contemporary sympatry between A. sp.2 and A. sp.3 observed in the Coast, have been secondarily established may not be discarded and their evolutionary histories could be associated with the historical forest refugia of the Atlantic Forest through the Pleistocene, as there is strong evidence that the refuges occurred in this region and promoted the diversification of several taxa (Carnaval and Moritz, 2008). An association of Pleistocene refuges and AF complex history was also suggested by Dias et al. (2015). However, our analysis depicted a strong congruence of the current location of the AF complex species with the geographic historical forest refugia (Figures 2, 3 in Carnaval and Moritz, 2008). Indeed, it is important to keep in mind that an observed pattern is an inference based on a correlation and this is distinct from causality. Even so, recognition of patterns may be useful to construct hypotheses which can be submitted to falsifiability tests that effectively would lead to advances in the knowledge of the AF complex.
The present data also make clear that the region of the Valley of Paraiba do Sul River, in the inland plateau (Figure 2), the three species occur in sympatry, but the contact among them could have been established secondarily after an earlier divergence, considering the arguments presented above. However, in this region, the species present distinct preferences in the host fruit use: A. sp.2 infests preferentially the exotic oranges while A. sp.1 and A. sp.3 were detected infesting the native guavas as well as A. sp.3 was not detected so far attacking oranges (Supplementary Table 1 and Figure 9).
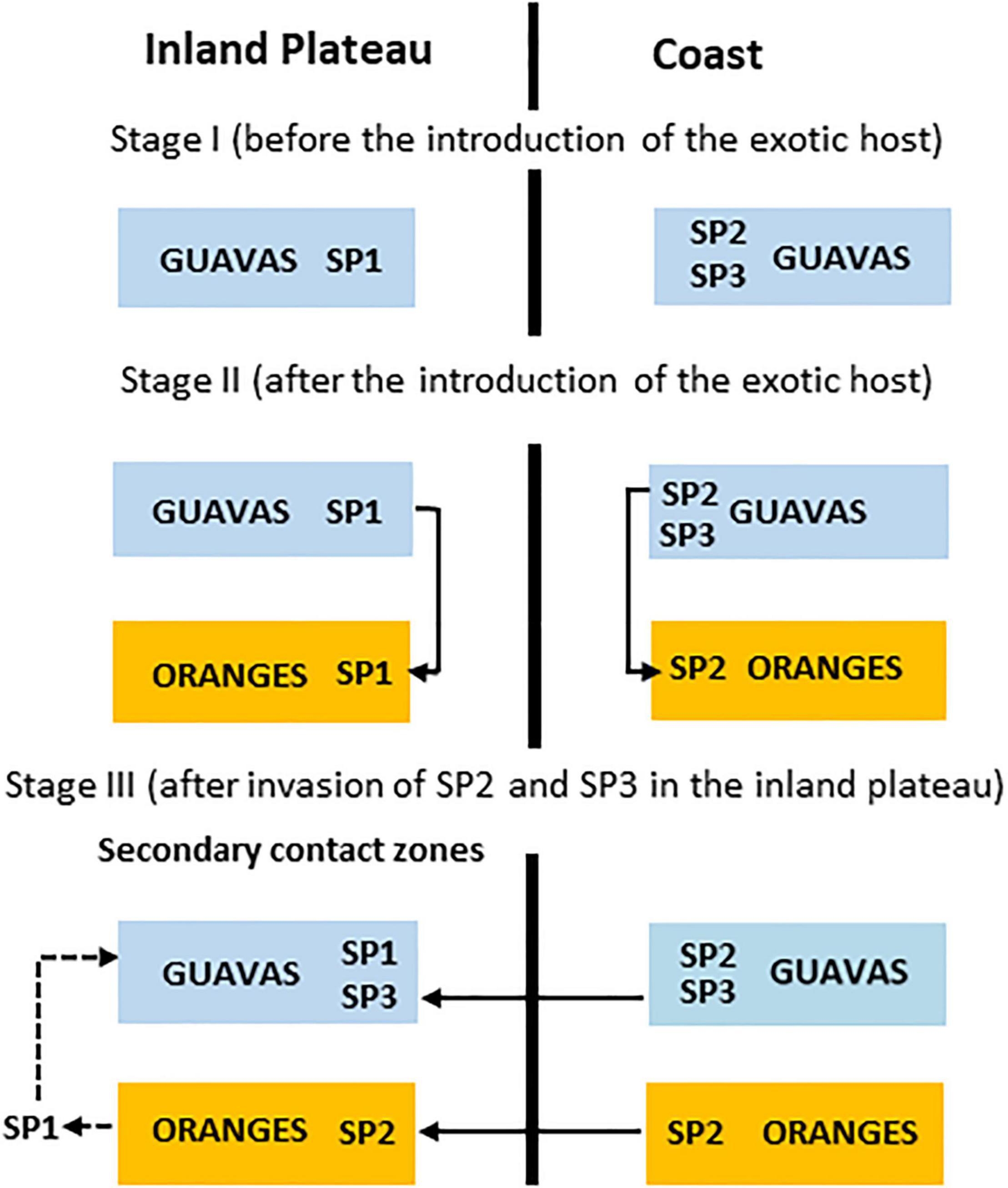
Figure 9. Hypothesis on the contemporary pattern of host fruit usage by the AF complex species in eastern Brazil. In stage I, A. sp.1 is the resident species in Inland Plateau while A. sp.2 and A. sp.3 are residents at the Coast; Stage II, the colonization of exotic fruit by A. sp.1, A. sp.2 and A. sp.3; Stage III, the secondary contact zone for the three species after invasion of populations from the coast to the inland plateau.
An earlier chromosomal analysis on fruit flies infesting oranges and loquat collected in 1947, in the inland plateau, State of São Paulo (Mendes, 1958), revealed that the exotic fruits were infested by A. fraterculus (s.l.). The described chromosomes are compatible to A. sp.1 (resident in inland plateau) but not to A. sp.2. In our analysis, we also detected infestation of loquat by A. sp.1 in the inland plateau samples from the state of São Paulo (Supplementary Table 1). However, the orange samples were infested by A. sp.2 and not by A. sp.1. Even though the factors shaping host plants use are highly diverse (Aluja and Mangan, 2008), a simplistic but plausible explicative hypothesis is presented in Figure 9. After the introduction of exotic orange, during the Portuguese colonization in the middle of the XIV century (Silva and Tassara, 2005), the fruits would have been colonized by the resident A. sp.1 in the inland plateau and by the resident A. sp.2 in the Coastal region; A. sp.3, seems uncapable to explore this host, since it was not found infesting oranges in our samples, neither in inland plateau nor in the coast. After a putative invasion (range expansion) of coastal residents A. sp.2 and A. sp.3 in the inland plateau, either naturally or by anthropic activities, A. sp.2 could have displaced A. sp.1 from oranges throughout a competitive displacement process while A. sp.3 have explored guavas with A. sp.1. Also in agreement with this hypothesis is the fact that in Argentina, where A. sp.2 seems not to occurs, oranges are infested by A. sp.1 (Rull et al., 2012).
Indeed, examples of a rapid human-induced evolution of insect-host association are well known, as described for the checkerspot butterfly, Euphydryas editha, that in less than 100 years changed the female oviposition preference from native to an introduced host plant (Singer et al., 1993), the colonization of apples by Rhagoletis pomonella (Bush, 1969; Feder et al., 2003, 2005), the Lonicera spp. an exotic fruit colonized by a hybrid species of Rhagoletis (Schwarz et al., 2005), and the apples colonization by A. fraterculus in Brazil that occurred in about three decades (Sugayama et al., 1997).
Diverse conditions are known which might lead to a competitive displacement in tephritid fruit flies, some of them related to the interactions between larvae within the same fruit: for example, the species whose larvae had the fastest developmental rate would have an advantage (Fitt, 1989). In fact, it has been found that A. sp.2 presents a developmental rate, faster than A. sp.1 (Ometto, 1997). Further experimental studies would be necessary to explore this possibility. Besides, previous data on reproductive isolation in allopatric and sympatric populations of A. sp.1 and A. sp.2, revealed the existence of stronger reproductive isolation between sympatric populations than between allopatric ones (Selivon et al., 2005a) what would agree with the model of Dobzhansky-Muller in an allopatric speciation process although alternative explanations do exist (Butlin, 1989).
It is assumed that cryptic species complexes evidenced a rapid radiation of species (Clarke et al., 2005). A rapid diversification of the fraterculus group as well as the fraterculus complex was postulated initially by Morgante et al. (1980) and also considered in other studies (e.g., Segura et al., 2011; Dias et al., 2015; Congrains et al., 2021). The prevalence of speciation by homoploid hybridization, a mechanism that originate independent lineages of hybrid individuals (Mallet, 2007), has been invoked to explain the rapid diversification of this group, based on the observation of hybrid individuals from a laboratory colony, assortative mating between hybrids (Segura et al., 2011; Dias et al., 2015), and introgression signals into the genomes have been described (Congrains et al., 2021). However, hybridization and introgression events can also occur during divergence processes. Indeed, if we consider even an allopatric scenario followed by the establishment of a secondary contact zone, it is possible that hybridization could initially occur as well as introgression, if robust reproductive barriers have not yet emerged as by-products of local adaptive process. The fate of the biological entities in this process will depend on hybrid fitness (Endler, 1977; Barton and Hewitt, 1985). Additionally, secondary contact zones could be promoters of higher diversification if we consider the basic principle that hybridization and introgression could lead to a higher level of genetic variance, improving the raw material for further diversification (Reiseberg and Wendell, 1993). It is important to keep in mind that data are always based on sampling, but at present no natural hybrid populations in the AF complex were detected, and there is so far no evidence of a hybrid species in Anastrepha. It is possible to consider a similar scenario for the fraterculus species group whose evolutionary history may be linked to past biogeographic events in which after the isolation in the Pleistocene refuges, secondary zones may have been established. In favor of this hypothesis it was estimated that the split of A. fraterculus, A. sorocula, and A. obliqua from their common ancestor occurred at ∼2.6 MYA (Diaz et al., 2018).
Species Status and Hypothesis on the Type Specimen Identity
As mentioned in the Introduction, there are alternative concepts and definitions of what a species is. Despite divergent opinions on this subject, there is a consensus that species are groups of populations following different evolutionary paths (De Queiroz, 1998, 2007). In fact, the divergences among different species concepts are determined by the criteria that are considered to recognize the point at which different lineages become irreversibly compromised with distinct paths, since speciation is a continuous process during which differences in distinct biological properties accumulate over time (Templeton, 1989; De Queiroz, 2007). The present data make clear that the differences among distinct biological entities within the AF complex in Brazil rely on criteria derived from different species concepts. The previous hypothesis of the existence of at least three distinct species in the AF complex in Brazil was not refuted by the present analysis. Even if these different biological entities from Brazil did not meet any criteria to reach species status, contrary to what was suggested by Diaz et al. (2018), they could no longer be treated as a monolithic entity, in biological terms and in regard to population control strategies, since they differed in multiple biological attributes.
The taxonomy of the genus Anastrepha has largely advanced based on the morphological traits of the female terminalia. For this reason, the identity of the type specimen of A. fraterculus, (Wiedemann, 1830), a male, has been uncertain in regard to the three Brazilian cryptic species of the complex. Furthermore, there are currently 10 other specific names considered synonyms of A. fraterculus (Hernández-Ortiz et al., 2016), six of which were described based on specimens from various regions of Brazil (e.g., São Paulo, SP; Vicosa, MG; Itajuba, MG; Agua Preta, BA; Itatiaia, RJ; and Santa Catarina) (Stone, 1942). The existence of three species in Brazil represents an enormous challenge to establish their identity. But considering that the three species characterized herein would be the only or the most frequent ones in Brazil, and as they can be recognized by morphometric analysis of both male and female flies, it was possible to recognize which of the three species correspond to the type specimen of A. fraterculus. The analysis indicated that the species Anastrepha sp.2 aff. fraterculus would correspond to the type specimen described by Wiedemann (1830; Figure 5C).
Conclusion
A substantial data set provided support for the recognition of three distinct species of the AF complex in Brazil, which presented differences in multiple biological properties. The extensive data on the geographical occurrence of the species and their associated host plants are crucial for inferences about the processes that gave rise to them. The rarity of hybrids in sympatric regions clearly demonstrated that robust reproductive barriers exist among them in natural conditions. Based on male wing geometric morphometry, it was possible to identify the A. fraterculus type specimen as A. sp.2 aff. fraterculus. Finally, our study portraits A. sp.1, a known fruit pest, as more capable of reaching areas with a higher thermal seasonality and daily thermal oscillations. The present report illustrates that an integrated analysis of multiple traits can furnish fundamental information to reconstruct evolutionary scenarios for fruit fly species. In addition, as the AF complex involves pest species, besides the academic interest, the presented results may have relevant economic implications, and could be useful to improve programs aimed at the population control of these pest insects.
Data Availability Statement
The datasets presented in this study can be found in online repositories. The names of the repository/repositories and accession number(s) can be found below: https://www.ncbi.nlm.nih.gov/genbank/, JN223376 to JN223391.
Author Contributions
DS, AP, VH-O, and FG: conceptualization. DS, AP, VH-O, AC, FdV, and LP: methodology. DS, AP, VH-O, and AC: formal analysis. DS and VH-O: funding acquisition. DS, AP, VH-O, AC, and FG: writing sections of the manuscript and review and editing. All authors: read and agreed to the submitted manuscript.
Funding
This work was supported by grants from the São Paulo State Research Foundation (FAPESP, 2003/02698-3; 2016/00782-7) to DS and (2014/16320-7) to FG. VH-O thank funding support for this investigation by the International Atomic Energy Agency (Vienna, Austria), under research contract IAEA No. 16080 (CRP—“Resolution of cryptic species complexes of Tephritid pests to overcome constraints to SIT application and international trade”), and research contract IAEA No: 22457 (CRP 040045 “SIT and Related Technologies to Manage Major Insect Plant Pests”). FG was a research fellow from the Brazilian Conselho Nacional de Desenvolvimento Cientıfico ì e Tecnoloìgico (CNPq) #304530/2019-0.
Conflict of Interest
The authors declare that the research was conducted in the absence of any commercial or financial relationships that could be construed as a potential conflict of interest.
Publisher’s Note
All claims expressed in this article are solely those of the authors and do not necessarily represent those of their affiliated organizations, or those of the publisher, the editors and the reviewers. Any product that may be evaluated in this article, or claim that may be made by its manufacturer, is not guaranteed or endorsed by the publisher.
Acknowledgments
The authors thank Carlos R. Vilela for ceding the photographs of the type specimen, Georgina Elzi dos Santos, Carlos Eduardo Lopes, and Fabiana Sipula for laboratory technical support (USP) during the project development. The authors are also grateful for the valuable comments and suggestions from the editor and reviewers that greatly improved the manuscript.
Supplementary Material
The Supplementary Material for this article can be found online at: https://www.frontiersin.org/articles/10.3389/fevo.2022.836608/full#supplementary-material
Footnotes
References
Abraham, S., Rull, J., Mendoza, M., Liendo, M. C., Devescovi, F., Roriz, A. K. P., et al. (2014). Differences in sperm storage and remating propensy between adult females of two morphotypes of the Anastrepha fraterculus (Diptera: Tephritidae) cryptic species complex. Bull. Entomol. Res. 104, 376–382. doi: 10.1017/S0007485314000200
Aluja, M., and Mangan, R. L. (2008). Fruit fly (Diptera: Tephritidae) status determination: critical conceptual, methodological, and regulatory considerations. Annu. Rev. Entomol. 53, 473–502. doi: 10.1146/annurev.ento.53.103106.093350
Aluja, M., Piñero, J., Jacome, I., Diaz-Fletcher, F., and Sivinski, J. (1999). “Behavior of flies in genus Anastrepha (Trypetinae: Toxotrypanini),” in Fruit Flies Tephritidae):Phylogeny and Evolution of Behavior, eds M. Aluja and A. L. Norrbom (Boca Raton, FL: CRC Press), 375–406. doi: 10.1201/9781420074468.ch15
Barbet-Massin, M., Jiguet, F., Albert, H., and Thuiller, W. (2012). Selecting pseudo-absences for species distribution models: How, where and how many? Methods Ecol. Evol. 3, 327–338. doi: 10.1111/j.2041-210x.2011.00172.x
Barr, N. B., Hauser, M., Belcher, J., Salinas, D., Schuenzel, E., Kerr, P., et al. (2021). Use of ITS-1 to identify Bactrocera dorsalis and Bactrocera occipitalis (Diptera: Tephritidae): a case study using flies trapped in California from 2008-2018. Fla. Entomol. 104, 96–106.
Barton, N. H., and Hewitt, G. M. (1985). Analysis of hybrid zones. Annu. Rev. Ecol. Syst. 16, 113–148.
Basso, A., Pereyra, A., and Bartoloni, N. (2019). Chromosome-site interaction in the South-American fruit fly Anastrepha fraterculus (Wied). J. Appl. Biotechnol. Bioeng. 6, 57–61. doi: 10.15406/jab.2019.06.00175
Basso, A., Sonvico, A., Quesada-Allue, L. A., and Manso, F. (2003). Karyotypic and molecular identification of laboratory stocks of the South American fruit fly Anastrepha fraterculus (Wied) (Diptera: Tephritidae). J. Econ. Entomol. 96, 1237–1244. doi: 10.1093/jee/96.4.1237
Bush, G. L. (1969). Sympatric host race formation and speciation in frugivorous flies of genus Rhagoletis (Diptera, Tephritidae). Evolution 23, 237–251. doi: 10.1111/j.1558-5646.1969.tb03508.x
Butlin, R. (1989). “Reinforcement of premating isolation,” in Speciation and Its Consequences, eds D. Otto and J. A. Endler (Sunderland, MA: Sinauer Associates), 158–179.
Cáceres, C., Segura, D. F., Vera, M. T., Wornoayporn, V., Cladera, J. L., Teal, P., et al. (2009). Incipient speciation revealed in Anastrepha fraterculus (Diptera, Tephritidae) by studies on mating compatibility, sex pheromones, hybridization and cytology. Biol. J. Linn. Soc. 97, 152–165. doi: 10.1111/j.1095-8312.2008.01193
Carnaval, A. C., and Moritz, C. (2008). Historical climate modelling predicts patterns of current biodiversity in the Brazilian Atlantic forest. J. Biogeogr. 35, 1187–1201. doi: 10.1111/j.1365-2699.01870.x
Cladera, J. L., Vilard, J. C., Juri, M., Paulin, L. E., Giardini, M. C., Gómez-Cendra, P. V., et al. (2014). Genetics and biology of Anastrepha fraterculus: research supporting the use of the sterile insect technique (SIT) to control this pest in Argentina. BMC Genet. 15(Suppl. 2):S12. doi: 10.1186/1471-2156-15-S2-S12
Clarke, A. R., Armstrong, K. F., Carmichael, A. E., Milne, J. R., Raghu, S., Roderick, G. K., et al. (2005). Invasive phytophagous pests arising through a recent tropical evolutionary radiaiition: the Bactrocera dorsalis complex of fruit flies. Annu. Rev. Entomol. 50, 293–319. doi: 10.1146/annurev.ento.50.071803.130428
Congrains, C., Zucchi, R. A., and Brito, R. A. (2021). Phylogenomic approach reveals strong signatures of introgression in the rapid diversification of neotropical true fruit flies (Anastrepha: Tephritidae). Mol. Phylogenet. Evol. 162:107200. doi: 10.1016/j.ympev.2021.107200
Costa, A. M., Anjos-Duarte, C. S., Roriz, A. K. P., Dias, V. D., and Joachin-Bravo, I. S. (2012). Male diet and age influence to inhibit females remating in Ceratitis capitata (Diptera: Tephritidae). J. Appl. Entomol. 136, 446–463. doi: 10.1111/j.1439-0418.2011.01669.x
De Queiroz, K. (1998). “The general lineage concept of species, species criteria, and the process of speciation: a conceptual unification and terminological recommendations,” in Endless Forms: Species and Speciation, eds D. J. Howard and S. H. Berlocher (New York, NY: Oxford University Press), 57–75.
De Queiroz, K. (2007). Species concepts and species delimitation. System. Biol. 56, 879–886. doi: 10.1080/10635150701701083
De Souza, T. O., dos Santos, T. A., Beasley, C. R., Lopes, L. R., de Simone, L. R. L., Marques-Silva, N. S., et al. (2015). Population structure and identification of two matrilinear and one patrilinear mitochondrial lineages in the mussel Mytella charruana. Estuar. Coast. Shelf Sci. 156, 165–174. doi: 10.1016/j.ecss.2014.11.009
DeMeyer, M., Clarke, A. R., Vera, M. T., and Hendrichs, J. (2015). Editorial. Zookeys 540, 1–3. doi: 10.3897/zookeys.540.6506
Derryberry, E. P., Claramunt, S., Derryberry, G., Chesser, R. T., Cracraft, J., Aleixo, A., et al. (2011). Lineage diversification and morphological evolution during an exceptional continental radiation, the Neotropical ovenbirds and woodcreepers (Aves: Furnariidae). Evolution 65, 2973–2986. doi: 10.1111/j.1558-5646.2011.01374.x
Devescovi, F., Abraham, S., Roriz, A. K., Nolazco, N., Castañeda, R., Tadeo, E., et al. (2014). Ongoing speciation within the Anastrepha fraterculus cryptic species complex: the case of the Andean morphotype. Entomol. Exp. Appl. 152, 238–247. doi: 10.1111/eea.12219
Dias, V., Silva, J. G., Lima, K. M., Pettinga, C. S. C. D., Hernández-Ortiz, V., Laumann, R. A., et al. (2015). An integrative multidisciplinary approach to understanding cryptic divergence in Brazilian species of the Anastrepha fraterculus complex (Diptera: Tephritidae). Biol. J. Linn. Soc. 117, 725–746. doi: 10.1111/bij.12712
Diaz, F., Lima, A. L. A., Nakamura, A. M., Fernandes, F., Sobrinho, I., and de Brito, R. A. (2018). Evidence for introgression among three species of Anastrepha fraterculus group, a radiating species complex of fruit flies. Front. Genet. 9:359. doi: 10.3389/fgene.2018.00359
Dobzhansky, T. (1935). A critique of the species concept in biology. Philos. Sci. 2, 344–355. doi: 10.1086/286379
Dobzhansky, T. (1970). Genetics of the Evolutionary Process. New York, NY: Columbia University Press.
Elgin, S. C. R., and Reuter, G. (2013). Position-effect variegation, heterochromatin formation, and gene silencing in Drosophila. Cold Spring Harb. Perspect. Biol. 5:a017780. doi: 10.1101/cshperspect.a017780
Endler, J. A. (1977). Geographic Variation, Speciation and Clines. Princeton, NJ: Princeton University Press.
Feder, J. K. L., Berlocher, S. H., Roethele, J. B., Dambroski, H., Smith, J. J., Perry, W. L., et al. (2003). Allopatric genetic origins for sympatric host plant shifts and race formation in Rhagoletis. Proc. Natl. Acad. Sci. U.S.A. 100, 10314–10319. doi: 10.1073/pnas.1730757100
Feder, J. K. L., Xien, X., Rull, J., Velez, S., Forbes, A., Leung, B., et al. (2005). Mayr, Dobzhansky, and Bush and the complexities of sympatric speciation in Rhagoletis. Proc. Natl. Acad. Sci. U.S.A. 102(Suppl. 1), 6573–6580. doi: 10.1073/pnas.0502099102
Fick, S. E., and Hijmans, R. J. (2017). WorldClim2: new 1-km spacial resolution climate surfaces for global land áreas. Int. J. Clim. 37, 4302–4315. doi: 10.1002/joc.5086
Fitt, G. P. (1989). “The role of interspecific interactions in the dynamics of tephritid populations,” in Fruit Lies, Their Biology, Natural Enemies and Control 3B, eds A. S. Robinson and G. Hooper (New York, NY: Elsevier), 281–300.
Fox, J., Friendly, M., and Monette, G. (2020). Vusialing Tests in Multivariate Linear Models (heplots). R package version 1.3-7. Available online at: http://CRAN.R-project.org/package=heplots (accessed October 1, 2022).
Giardini, M. C., Milla, F. H., Lanzavecchia, S., Nieves, M., and Cladera, J. L. (2015). Sex chromosome in mitotic and polytene tissues of Anastrepha fraterculus (Diptera: Tephritidae) from Argentina: a review. Zookeys 540, 83–94. doi: 10.3897/zookeys.540.6058
Giardini, M. C., Nieves, M., Scannapieco, A. C., Conte, C. A., Milla, F. H., Schapovaloff, M. E., et al. (2020). Geographic distribution of sex chromosome polymorphism in Anastrepha fraterculus sp.1 from Argentina. BMC Genet. 21(Suppl. 2):149. doi: 10.1186/s12863-020-00944-1
Goday, C., Selivon, D., Perondini, A. L. P., Greciano, P. G., and Ruiz, M. F. (2006). Cytological characterization of sex chromosomes and ribosomal DNA location in Anastrepha species (Diptera,Tephritidae). Cytogen. Gen. Res. 114, 70–76. doi: 10.1159/000091931
Goodall, C. (1991). Procrustes methods in the statistical analysis of shape. J. R. Stat. Soc. B 53, 285–321. doi: 10.1111/j.2517-6161.1991.tb01825.x
Gutierrez, A. P., Ponti, L., Neteler, M., Suckling, D. M., and Cure, J. R. (2021). Invasive potential of tropical fruit flies in temperate regions under climate change. Commun. Biol. 4:1141. doi: 10.1038/s42003-021-02599-9
Hanley, J. A., and McNeil, B. J. (1982). The meaning and use of the area under a receiver operating characteristic (ROC) curve. Radiology 143, 29–36. doi: 10.1148/radiology.143.1.7063747
Hendrichs, J., Vera, M. T., DeMeyer, M., and Clarke, A. K. (2015). Resolving cryptic species complexes of major tephritid pests. Zookeys 540, 5–39. doi: 10.3897/zookeys.540.9656
Hernández-Ortiz, V., Bartolucci, A., Morales-Valles, P., Frias, D., and Selivon, D. (2012). Cryptic species of the Anastrepha fraterculus complex (Diptera, Tephritidae): a multivariate approach for the recognition of South American morphotypes. Ann. Entomol. Soc. Am. 105, 305–318. doi: 10.1603/AN11123
Hernández-Ortiz, V., Canal, N. A., Salas, J. O. T., Ruíz-Hurtado, F. M., and Dzul-Cauich, J. F. (2015). Taxonomy and phenotypic relationships of the Anastrepha fraterculus complex in the Mesoamerican and Pacific Neotropical dominions (Diptera, Tephritidae). Zookeys 540, 95–124. doi: 10.3897/zookeys.540.6027
Hernández-Ortiz, V., Gomez-Anaya, J. A., Sanchez, A., McPheron, B. A., and Aluja, M. (2004). Morphometric analysis of Mexican and South American populations of the Anastrepha fraterculus complex (Diptera: Tephritidae) and recognition of a distinct Mexican morphotype. Bull. Entomol. Res. 94, 487–499. doi: 10.1079/BER2004325
Hernández-Ortiz, V., Vaccaro, N., and Basso, A. (2016). Diagnostic Protocol 9: Genus Anastrepha Schiner. International Standard for Phytosanitary Measures 27. (Rome: Food and Agriculture Organization of the United Nations), 30.
Hernández-Triana, L. M. (2015). DNA barcoding of Neotropical black flies (Diptera: Simuliidae): Species identification and discovery of cryptic diversity in Mesoamerica. Zootaxa 3936, 93–114. doi: 10.11646/zootaxa.3936.1.5
Hervé, M. (2020). RVAideMemoire: Testing and Plotting. Procedures for Biostatistics. R package version 0.9-75. Available online at: https://cran.r-project.org/web/packages/RVAideMemoire/RVAideMemoire.pdf (accessed April 9, 2020).
Hijmans, R. J., Phillips, S., Leathwick, J., Elith, J., and Hijmans, M. R. J. (2017). Package ‘dismo’. Circles 9, 1–68.
Hilton, H., Kliman, M., and Hey, J. (1994). Using hitchhiking genes to study adaptation and divergence during speciation within the Drosophila melanogaster species complex. Evolution 48, 1900–1913. doi: 10.1111/j.1558-5646.1994.tb02222.x
Hothorn, T., Bretz, F., and Westfall, P. (2008). Simultaneous inference in general parametric models. Biom. J. 50, 346–363. doi: 10.1002/bimj.200810425
Jarek, J. (2012). Normality Test for Multivariate Variables (mvnormtest). R package version 0.1-9. Available online at: https://cran.r-project.org/web/packages/mvnormtest/mvnormtest.pdf (accessed February 3, 2022).
Kovalesky, A., Uramoto, K., Sugayama, R., Canal, N. A., and Malavasi, A. (1999). A survey of Anastrepha Schiner (Diptera: Tephritidae) in the apple growing area of the state of Rio Grande do Sul, Brazil. Rev. Brasil. Entomol. 43, 229–234.
Librado, P., and Rozas, J. (2009). DnaSP v5: a software for comprehensive analysis of DNA polymorphism. Bioinformatics 25, 1451–1452. doi: 10.1093/bioinformatics/btp187
Lobo, J. M., Jiménez-Valverde, A., and Real, R. (2008). AUC: a misleading measure of the performance of predictive distribution models. Glob. Ecol. Biogeogr. 17, 145–151. doi: 10.1111/j.1466-8238.2007.00358.x
Manni, M., Lima, K. M., Guglielmino, C. R., Lanzavechia, S. B., Juri, M., Vera, M. T., et al. (2015). Relevant differentiation among Brazilian populations of Anastrepha fraterculus (Diptera: Tephritidae). Zookeys 540, 157–173. doi: 10.3897/zookeys.540.6713
Mendes, L. O. T. (1958). Observações citológicas em “moscas-das-frutas”. Bragantia 17, 29–39. doi: 10.1590/S0006-87051958000100034
Morgante, J. S., Malavasi, A., and Bush, G. L. (1980). Biochemical systematics and evolutionary relationships of Neotropical Anastrepha. Ann. Entomol. Soc. Am. 73, 622–630. doi: 10.1093/aesa/73.6.622
Naimi, B., and Araújo, M. B. (2016). sdm: a reproducible and extensible R platform for species distribution modelling. Ecography 39, 368–375. doi: 10.1111/ecog.01881
Neil, M., and Kumar, S. (2000). Molecular Evolution and Phylogeny. New York, NY: Oxford University Press.
Norrbom, A. L., Zucchi, R. A., and Herrnández-Ortiz, V. (1999). “Phylogeny of the genera Anastrepha and Toxotrypana (Trypetinae: Toxotrypanini) based on morphology,” in Fruit flies (Tephritidae): Phylogeny and Evolution of Behavior, eds M. Aluja and A. L. Norrbom (Boca Ratón, FL: CRC Press), 299–342. doi: 10.1201/9781420074468.ch12
Ometto, A. C. F. (1997). Estudo Demográfico em Anastrepha Fraterculus (Wiedemann) (Diptera:Tephritidae): Comparações de Co-Ortes Procedentes De Diferentes Hospedeiros. Master’s thesis. São Paulo: Universidade de São Paulo.
Paterson, H. E. H. (1985). “The recognition concept of species,” in: Species and Speciation, ed. E. S. Vrba (Pretoria: Transvaal Museum), 21–29.
Pearson, K. (1901). LIII. On lines and planes of closest fit to systems of points in space. Philosoph. Magaz. J. Sci. 2, 559–572. doi: 10.1080/14786440109462720
Perre, P. (2017). Utilização Diferencial de Frutos Hospedeiros por Anastrepha sp.1 aff. Fraterculus (Diptera, Tephritidae): Aspectos Morfológicos e Reprodutivos. Ph.D. dissertation. São Paulo: University of São Paulo.
Peterson, A. T., Papes, M., and Soberón, J. (2008). Rethinking receiver operating characteristic analysis applications in ecological niche modeling. Ecol. Model. 213, 63–72. doi: 10.1016/j.ecolmodel.2007.11.008
Petit-Marty, N., Vera, M. T., Calcagno, G., Cladera, J. L., Segura, D. F., Allinghi, A., et al. (2004). Sexual behavior and mating compatibility among four populations of Anastrepha fraterculus (Diptera, Tephritidae) from Argentina. Ann. Entomol. Soc. Am. 97, 1320–1327. doi: 10.1603/0013-8746(2004)097[1320:sbamca]2.0.co;2
Prezotto, L. F., Perondini, A. L. P., Hernández-Ortiz, V., Frías, D., and Selivon, D. (2019). What can integrate analysis of morphological and genetic data still reveal about the Anastrepha fraterculus (Diptera: Tephritidae) cryptic species complex? Insects 10:408. doi: 10.3390/insects10110408
Prezotto, L. F., Perondini, A. L. P., Hernández-Ortiz, V., Marino, C. L., and Selivon, D. (2017). Wolbachia strains in cryptic species of the Anastrepha fraterculus complex (Diptera, Tephritidae) along the Neotropical Region. Syst. Appl. Microbiol. 40, 59–67. doi: 10.1016/j.syapm.2016.11.002
R Core Team (2020). R: A Language and Environment for Statistical Computing. Vienna: R Foundation for Statistical Computing.
Reiseberg, L. H., and Wendell, J. F. (1993). “Introgression and its consequences in plants,” in Hybrid Zones and the Evolutionary Process, ed. R. G. Harisson (New York, NY: Oxford University Press), 70–109.
Rendón, P., and Enkerlin, W. (2021). “Area-wide fruit fly in Latin America,” in Area-Wide Integrated Pest Management Development and Field Application, eds J. Hendrichs, R. Pereira, and M. J. B. Vreysen (Boca Ratón, FL: CRC Press), 161–195. doi: 10.1002/ps.4585
Robertson, J. G. (1957). Somatic metaphase chromosomes in geographic isolates of the carrot rust fly, Chamaepsila rosae (F.) (Diptera:Psilidae). Can. J. Zool. 35, 453–458. doi: 10.1139/z57-037
Roriz, A. K. P., Japyassú, H. F., Cáceres, C., Vera, M. T., and Joachim-Bravo, I. S. (2018). Pheromone emission patterns and courtship sequences across distinct populations within Anastrepha fraterculus (Diptera, Tephritidae) cryptic species complex. Bull. Entomol. Res. 109, 408–417. doi: 10.1017/S0007485318000846
Roriz, A. K. P., Japyassú, H. F., and Joachin-Bravo, I. S. (2017). Incipient speciation in the Anastrepha fraterculus cryptic species complex: reproductive compatibility between A. sp.1 aff. fraterculus and A. sp.3 aff. fraterculus. Entomol. Exp. Appl. 162, 346–357. doi: 10.1111/eea.12526
Rull, J., Abraham, S., Kovaleski, A., Segura, D. F., Islam, A., Wornoayporn, V., et al. (2012). Random mating and reproductive compatibility among Argentinean and southern Brazilian populations of Anastrepha fraterculus complex (Diptera, Tephritidae). Bull. Entomol. Res. 102, 435–443. doi: 10.1017/S0007485312000016
Rull, J., Abraham, S., Kovaleski, A., Segura, D. F., Mendonza, M., Liendo, M. C., et al. (2013). Relative importance of prezygotic and postzygotic barriers to gene flow among three cryptic species within the Anastrepha fraterculus complex. Entomol. Exp. Appl. 148, 213–222. doi: 10.1111/eea.12094
Sánchez, L., Perondini, A. L. P., and Selivon, D. (2018). Understanding the dynamics underpinning the geographical distribution of the Anastrepha fraterculus complex in Eastern Brazil. Trends Entomol. 14, 13–31. doi: 10.31300/TENT.14.2018.17-31
Santos, P., Uramoto, K., and Matioli, S. R. (2001). Experimental hybridization among Anastrepha species (Diptera: Tephritidae): production and morphological characterization of F1 hybrids. Ann. Entomol. Soc. Am. 94, 717–725. doi: 10.1603/0013-8746(2001)094[0717:ehaasd]2.0.co;2
Saruhashi, S. (2019). Sensibilidade e Intolerância Térmica na Mosca-Das-Frutas Anastrepha sp.1 Affinis Fraterculus (Diptera Tephritidae). Master’s thesis. São Paulo: University of São Paulo.
Schlick-Steiner, B. C., Steiner, F. M., Stauffer, C., Christian, E., and Crozier, R. H. (2010). Integrative taxonomy: a multisource approach to exploring biodiversity. Annu. Rev. Entomol. 55, 421–438. doi: 10.1146/annurev-ento-112408-085432
Schutze, M. K., Virgilio, M., Norrbom, A., and Clarke, A. R. (2017). Tephritid integrative taxonomy: where we are now, with a focus on the resolution of three tropical fruit fly species complexes. Annu. Rev. Entomol. 62, 147–164. doi: 10.1146/annurev-ento-031616-035518
Schwarz, D., Matta, B. M., Shakir-Botteri, N., and McPheron, B. A. (2005). Host shift to an invasive plant triggers rapid animal hybrid speciation. Nature 436, 546–549. doi: 10.1038/nature03800
Segura, D. F., Vera, M. T., Rull, J., Wornoayporn, V., Islam, A., and Robinson, A. S. (2011). Assortative mating among Anastrepha fraterculus (Diptera, Tephritidae) hybrids as a possible route to radiation of the fraterculus cryptic species complex. Biol. J. Linn. Soc. 102, 346–354. doi: 10.1111/j.1095-8312.2010.01590x
Selivon, D. (1991). Some Aspects of the Behavior of Anastrepha striata SCHINER and Anastrepha bistrigata Bezzi (Diptera, Tephritidae). MS dissertation. São Paulo: University of São Paulo.
Selivon, D. (1996). Estudo Sobre a Diferenciação Populacional em Anastrepha fraterculus (Wiedemann) (Diptera: Tephritidae). Ph.D. thesis. São Paulo: University of São Paulo.
Selivon, D., and Perondini, A. L. P. (1997). Evaluation of techniques for C and ASG banding of the mitotic chromosomes of Anastrepha fraterculus (Diptera: Tephritidae). Braz. J. Genet. 20, 651–654. doi: 10.1590/S0100-84551997000400015
Selivon, D., and Perondini, A. L. P. (1998). Eggshell morphology in two cryptic species of Anastrepha fraterculus (Diptera: Tephritidae). Ann. Entomol. Soc. Am. 91, 473–478. doi: 10.1093/aesa/91.4.473
Selivon, D., Perondini, A. L. P., and Morgante, J. S. (1999). Haldane’s rule and other aspects of reproductive isolation observed in the Anastrepha fraterculus complex (Diptera, Tephritidae). Genet. Mol. Biol. 22, 507–510. doi: 10.1590/S1415-47571999000400007
Selivon, D., Perondini, A. L. P., and Morgante, J. S. (2005a). A genetic-morphological characterization of two cryptic species of the Anastrepha fraterculus complex (Diptera, Tephritidae). Ann. Entomol. Soc. Am. 98, 367–381. doi: 10.1603/0013-8746(2005)098[0367:agcotc]2.0.co;2
Selivon, D., Perondini, A. L. P., and Rocha, L. S. (2005b). Karyotype characterization of Anastrepha fruit flies (Diptera: Tephritidae). Neotrop. Entomol. 34, 273–279. doi: 10.1590/S1519-566X2005000200015
Selivon, D., Vretos, C., Fontes, L., and Perondini, A. L. P. (2004). “New variant forms in the Anastrepha fraterculus complex,” in Proceedings of the 6th International Fruit Flies Symposium, ed. B. Barnes (Irene: Isteg Scientific Publications), 253–258.
Sievers, F., Wilm, A., Dinneen, D., Gibson, T. J., Karplus, K., Li, W., et al. (2011). Fast, scalable generation of high-quality protein multiple sequence alignments using Clustal Omega. Mol. Syst. Biol. 7:539. doi: 10.1038/msb.2011.75
Singer, M. C., Thomas, C. D., and Parmesan, C. (1993). Rapid human-induced evolution of insect-host associations. Nature 366, 681–683. doi: 10.1038/366681a0
Sivinski, J., Aluja, M., Dodson, G., Freidberg, A., Headrick, D., Kaneshiro, K., et al. (1999). “Topics in the evolution of sexual behavior in the Tephritidae,” in Fruit Flies (Tephritidae): Phylogeny and Evolution of Behavior, eds M. Aluja and A. L. Norrbom (Boca Ratón, FL: CRC Press), 751–792. doi: 10.1201/9781420074468.ch28
Smith-Caldas, M. R. B., McPheron, B. A., Silva, J. G., and Zucchi, R. A. (2001). Phylogenetic relationships among species of the fraterculus group (Anastrepha: Diptera: Tephritidae) inferred from DNA sequences of mitochondrial cytochrome oxidase I. Neotrop. Entomol. 30, 565–573. doi: 10.1590/S1519-566X2001000400009
Solferini, V. N., and Morgante, J. S. (1987). Karyotype study of eight species of Anastrepha (Dipetra, Tephritidae). Caryologia 40, 229–241. doi: 10.1080/00087114.1987.10797826
Stone, A. (1942). The Fruit Flies of the Genus Anastrepha. (Washington, DC: USDA), 1–112. doi: 10.1007/978-1-4757-2278-9_2
Sugayama, R. L., Branco, E. S., Malavasi, A., Kovalesky, A., and Nora, I. (1997). Oviposition behavior of Anastrepha fraterculus in apple and diel pattern of activity in an apple orchard in Brazil. Entomol. Exp. Appl. 83, 239–245. doi: 10.1046/j.1570-7458.1997.00178.x
Sutton, B. D., Steck, G. J., Norrbom, A. L., Rodriguez, E. J., Srivastava, P., Alvarado, N. N., et al. (2015). Nuclear ribosomal internal transcribed spacer 1 (ITS1) variation in the Anastrepha fraterculus cryptic species complex (Diptera, Tephritidae) of the Andean region. Zookeys 540, 175–191. doi: 10.3897/zookeys.540.6147
Tamura, K., Stecher, G., Peterson, D., Filipski, A., and Kumar, S. (2013). MEGA 6: molecular evolutionary genetics analysis version 6.0. Mol. Biol. Evol. 30, 2725–2729. doi: 10.1073/pnas.044206101
Vacher, J.-P., Chave, J., Gentile, F., Sommeria-Klein, G., Tao, S., Thébaud, C., et al. (2019). Large-scale DNA-based survey of frogs in Amazonia suggests a vast underestimation of species richness and endemism. J. Biogeog. 47, 1781–1791. doi: 10.1111/jbi.13847
Vaníèková, L., Hernández-Ortiz, V., Joachin-Bravo, I. S., Dias, V., Roriz, A. K. P., Laumann, R. A., et al. (2015). Current knowledge of the species complex Anastrepha fraterculus (Diptera: Tephritidae) in Brazil. Zookeys 540, 211–237. doi: 10.3897/zookeys.9791
Venables, W. N., and Ripley, B. D. (2002). Modern Applied Statistics with S. New York, NY: Springer.
Vera, M. T., Cáceres, C., Wornoyapor, V., Islam, A., Robinson, A. S., De La Veja, M. H., et al. (2006). Mating incompatibility among populations of the South American fruit fly Anastrepha fraterculus (Diptera, Tephritidae). Ann. Entomol. Soc. Am. 99, 387–397. doi: 10.1603/0013-8746(2006)099[0387:miapot]2.0.co;2
White, I. M., and Elson-Harris, M. M. (1992). Fruit Flies of Economic Significance: Their Identification and Bionomics. Wallingford: CAB International.
Wiedemann, C. R. W. (1830). Dacus fraterculus. Available online at: https://citeseerx.ist.psu.edu/viewdoc/download?doi=10.1.1.614.4961&rep=rep1&type=pdf (accessed October 2, 2022).
Keywords: South American fruit fly, Tephritidae, competitive exclusion, secondary contact zone, MaxEnt, allopatry, sympatry
Citation: Selivon D, Perondini ALP, Hernández-Ortiz V, doVal FC, Camacho A, Gomes FR and Prezotto LF (2022) Genetical, Morphological, Behavioral, and Ecological Traits Support the Existence of Three Brazilian Species of the Anastrepha fraterculus Complex of Cryptic Species. Front. Ecol. Evol. 10:836608. doi: 10.3389/fevo.2022.836608
Received: 15 December 2021; Accepted: 29 March 2022;
Published: 27 April 2022.
Edited by:
Isabel Marques, University of Lisbon, PortugalReviewed by:
Alzira Kelly Roriz, Federal University of Bahia, BrazilAllen Norrbom, Systematic Entomology Laboratory (USDA-ARS), United States
Copyright © 2022 Selivon, Perondini, Hernández-Ortiz, doVal, Camacho, Gomes and Prezotto. This is an open-access article distributed under the terms of the Creative Commons Attribution License (CC BY). The use, distribution or reproduction in other forums is permitted, provided the original author(s) and the copyright owner(s) are credited and that the original publication in this journal is cited, in accordance with accepted academic practice. No use, distribution or reproduction is permitted which does not comply with these terms.
*Correspondence: Denise Selivon, ZHNlbGl2b25AaWIudXNwLmJy