- USDA Forest Service, Athens, GA, United States
Invasive non-native woody shrubs pose a major threat to forested ecosystems in many parts of the world and there is an urgent need for research on the restoration and recovery of these areas. We studied patterns of tree growth and regeneration 13 years after the experimental eradication (by chainsaw or mulching machine followed by herbicide treatments) of Ligustrum sinense Lour. (Chinese privet) from riparian forests in Georgia, United States. We also followed the recovery of bee and butterfly populations using sites with no history of privet invasion as a reference. By the end of the study, the basal area of restored plots was 24% greater, on average, than still-invaded control plots. Because tree growth rates did not differ among treatments, this increase is attributable to the 60% increase in the number of regenerating native stems (dominated by Acer negundo L.) following privet removal. The benefits of privet removal on pollinators were immediate and long-lasting with the richness and abundance of bees and butterflies being consistently higher in restored plots than in control plots. The diversity, abundance, and composition of bees in restored and reference (i.e., never invaded) plots were comparable by the end of the study. This was less true for butterflies, however, possibly due to the legacy effects of privet invasion on plant communities. Our results demonstrate the long-term benefits of removing privet on forest regeneration and pollinator communities. Indeed, without such efforts, it is probable that forest cover will gradually thin and ultimately disappear from privet-invaded areas as overstory trees die without replacement.
Introduction
Invasive non-native species are considered one of the biggest threats to biodiversity globally and continue to alter the structure and composition of ecosystems in profound ways. While the effects of many non-native taxa are benign or even beneficial (Schlaepfer et al., 2011), others have the potential to displace entire ecosystems. Some of the most problematic species invading forested systems involve trees and shrubs that have become naturalized or invasive across their non-native range (Fagan and Peart, 2004; Hartman and McCarthy, 2007; Richardson and Rejmánek, 2011). By preventing tree regeneration, for instance, dense thickets formed by certain invasive shrubs have the potential to convert native forests into invasive shrublands (Cash et al., 2020; Lázaro-Lobo et al., 2021; Turner et al., 2022). While the loss of forest cover will be gradual, playing out over many decades as overstory trees die, the invasion of such shrubs will have more immediate effects on other species as well as the ecosystem services they provide. There is thus an urgent need for research on how to preserve forest cover in such areas while also conserving diverse assemblages of native species. This is a major and growing challenge facing land managers worldwide (Richardson and Rejmánek, 2011).
Among the most ecologically consequential invasive shrubs in North America and beyond is Ligustrum sinense Lour. (i.e., Chinese privet, hereafter “privet”) an Asian species that was intentionally introduced as an ornamental plant to countries throughout the world beginning in the mid nineteenth century (Cash et al., 2020). This semi-evergreen species is now naturalized widely where it often forms a dense midstory layer up to about 10 m in height, especially in riparian forests (Figure 1A). Although privet is already considered one of the worst invasive plants in many regions (Batianoff and Butler, 2003; Drake et al., 2003), the problem is expected to get much worse as the species continues to spread into new areas. For example, Wang et al. (2016) estimated that the total area invaded by privet in Alabama and Mississippi will increase from 1.36% to nearly one third of total forest area within the next two decades. Because the survival and growth of native tree seedlings is greatly reduced beneath privet (Greene and Blossey, 2012), and privet quickly fills in gaps created by recently fallen trees (Turner et al., 2022), there are real concerns about the future of forest cover in areas invaded by this shade-tolerant species (Cash et al., 2020).
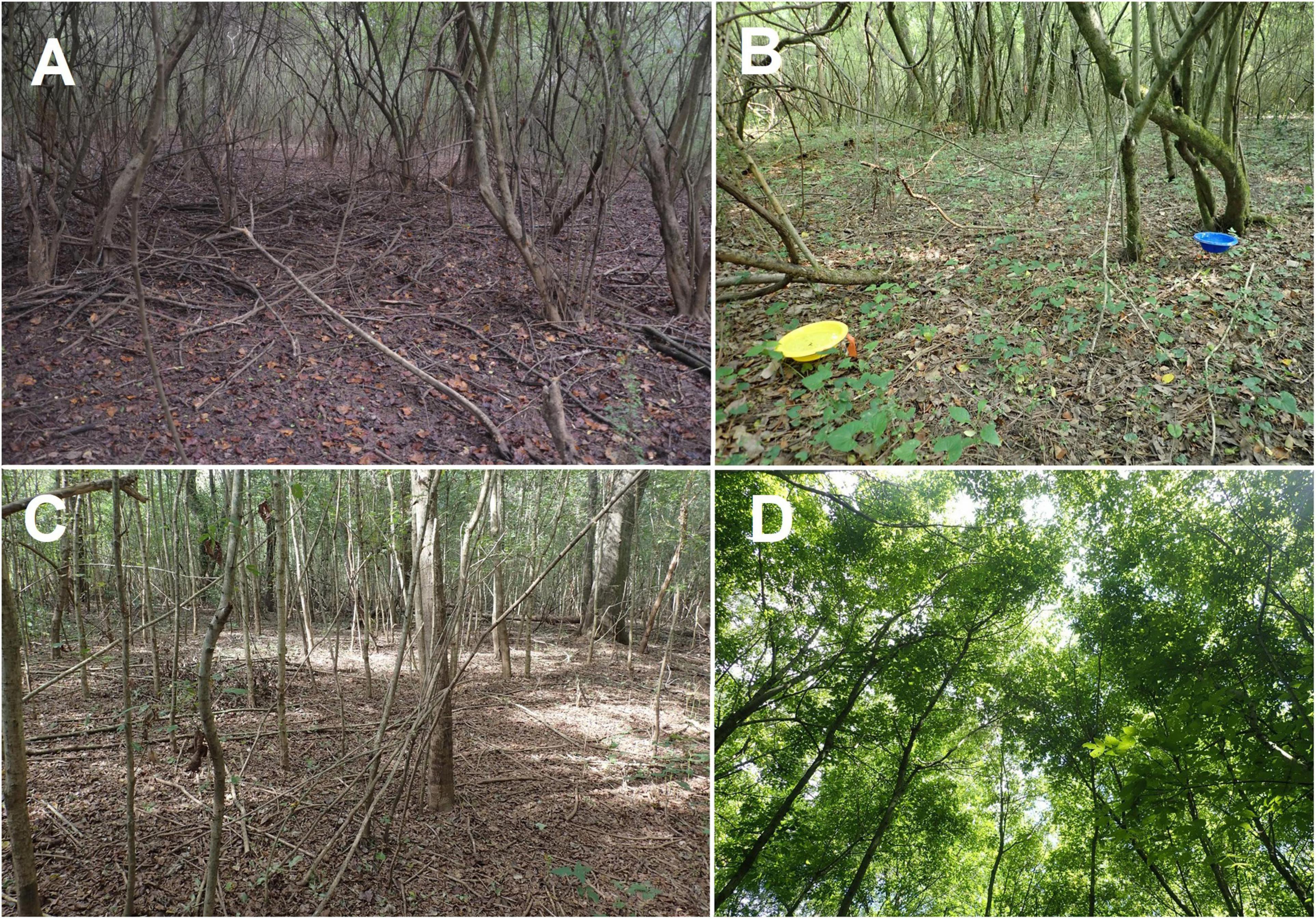
Figure 1. Control plot showing thicket of privet stems overshadowing the forest floor (A); Pair of colored pan traps for sampling pollinators in one of the control subplots (B); Regenerating saplings in a mulch plot, 2019 (C); canopy cover consisting entirely of boxelder saplings that grew after privet removal, 2019 (D).
Among the many species likely to be impacted by privet invasion are pollinating insects. Bees and other flower-visitors are critically important to the reproductive success of many plants in both natural and agricultural systems (Ollerton et al., 2011) and there is a growing appreciation for the importance of forests to these insects. In the northeastern United States, for example, about a third of all bee species are considered forest-associated (Smith et al., 2021). Forests provide a wide variety of floral resources including the flowers of both wind- and animal-pollinated trees (Splitt et al., 2021) as well as those of shrubs and herbs in the understory. Forests also provide critical nesting resources to bees and other taxa that may be rare or absent elsewhere. These include dead wood, cavities created by wood-boring beetles and patches of undisturbed soil. Pollinator populations are in decline (Goulson et al., 2015; Powney et al., 2019; Wepprich et al., 2019) in part due to invasive plants (Cunningham-Minnick and Crist, 2020; Mathiasson and Rehan, 2020) that, like privet, can exclude herbaceous plants from the forest floor (Merriam and Feil, 2003; Hanula et al., 2009; Figure 1A). The removal of such invaders is recommended for improving habitat quality for pollinators (Hanula et al., 2016; Mathiasson and Rehan, 2020) but the long-term benefits of such interventions are not well understood. There is also little known about the potential benefits of privet to pollinators, e.g., as a floral resource.
In 2005, a study aimed at investigating the ecological effects of privet eradication was initiated at four forested locations in northern Georgia, United States (Hanula et al., 2009). Although numerous benefits to insects were reported within the first 5 years after eradication (Hanula and Horn, 2011a,b; Hudson et al., 2013), particularly near the forest floor (Ulyshen et al., 2010a,2020), questions remained about whether these would continue into the future. For example, the presence of privet in the midstory prevented the regeneration of trees that would otherwise have replaced trees lost from the overstory. Once privet was removed from these forests, the canopy was much more open than what is typical for mature forests of the region (Hanula et al., 2009, MDU personal observation) and it is possible these openings alone may have been driving the increase in insect abundance following removal. Here we analyze patterns of native tree/shrub regeneration and pollinator diversity after 13 years of recovery when these stands had begun to resemble the closed-canopy forests more typical of the region. Regarding tree regeneration, we predicted that stem number, basal area and tree growth would be significantly higher in restored plots cleared of privet than in still-invaded control plots. As for pollinators, we predicted that bees and butterflies would still be significantly more abundant and species rich in restored plots than in control plots 13 years after privet removal and that these communities would be comparable to those occurring in reference stands with no history of privet invasion. To better understand the value of privet to pollinators as a floral resource, we also report the results from an effort to sample bees from privet flowers near our study areas.
Materials and Methods
Study Areas
This study took place in the Piedmont ecoregion of northern Georgia, United States, a transitional zone between the Appalachian mountains to the north and the Coastal Plain to the south. A Chinese privet eradication study, initiated in 2005 (Hanula et al., 2009), was replicated at four locations heavily invaded by privet within the Oconee River watershed (Figure 2). These were Sandy Creek Nature Center (SC) and the Georgia State Botanical Gardens (BG), both near the city of Athens, and Scull Shoals Experimental Forest (SS) and Watson Springs Forest (WS) in nearby Oglethorpe and Greene counties, respectively. Forests were hardwood-dominated at all four locations, with common genera including Quercus, Acer, Fraxinus, Liquidambar, Populus, Platanus, etc. There was also a minor pine component at all sites, largely consisting of Pinus taeda L. All locations were situated near rivers or streams and experienced seasonal (winter or early spring) flooding.
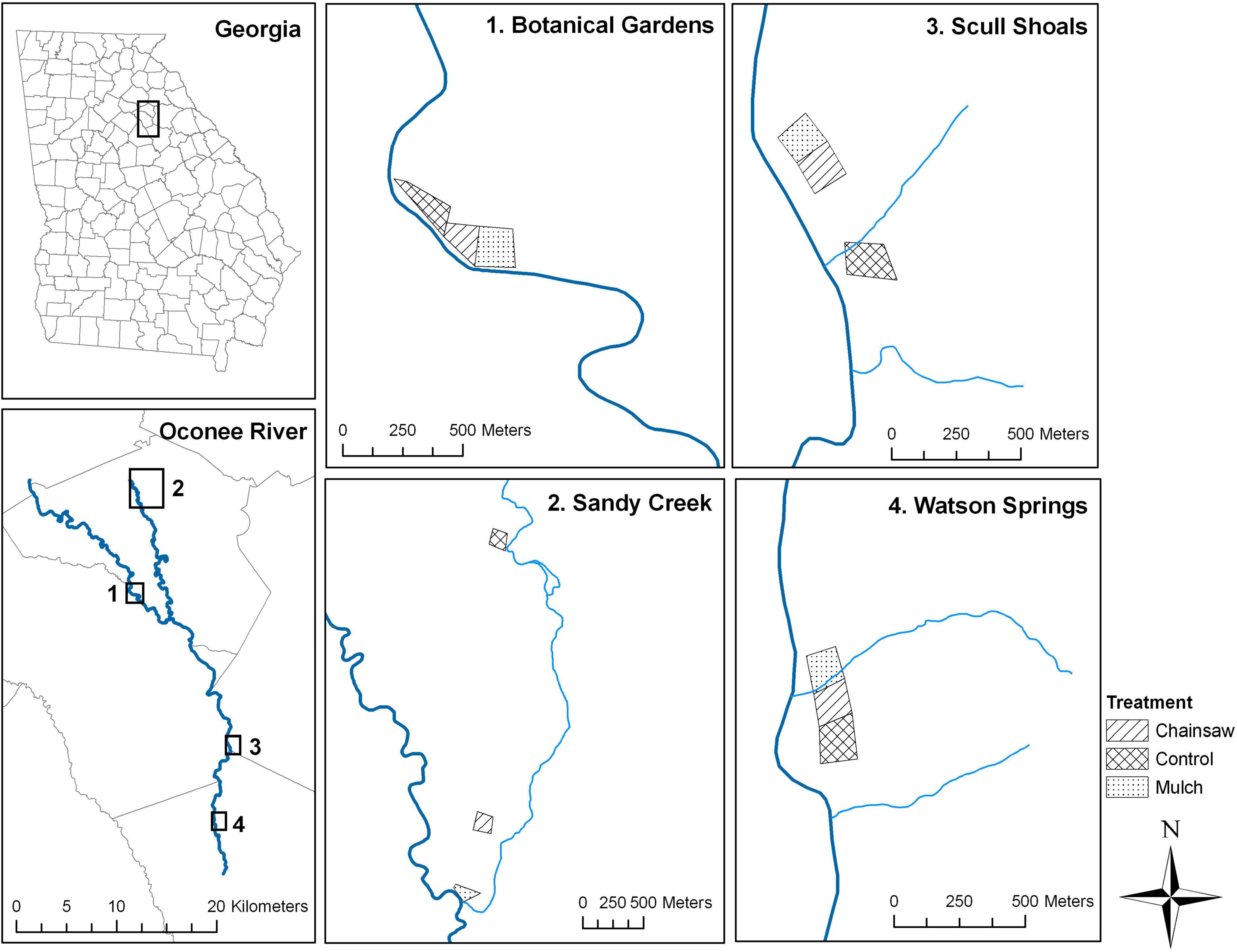
Figure 2. Map showing the locations of the privet eradication study in northern Georgia, United States.
Three ∼2 ha plots, roughly square in shape, were established at each of the riparian forest locations (Figure 2). Two plots at each location were assigned to one of two privet-eradication treatments. In the “chainsaw” treatment, hand tools were used to fell all privet stems, leaving a layer of debris ≤1 m high on the forest floor. The “mulch” treatment involved using a low-impact tracked machine to grind privet into a fine mulch (Klepac et al., 2007). These treatments were applied in October 2005 and were immediately followed by a herbicide treatment [30% triclopyr (Garlon 4) or 30% glyphosate (Foresters’)] to all remaining stumps. A follow-up foliar treatment of 2% glyphosate was conducted in December 2006 to eliminate all surviving privet sprouts. The eradication treatments had no effect on non-privet shrubs or saplings relative to the control plots (Hanula et al., 2009). No further treatments were applied until 2017 when herbicide was applied at the same rates as the initial effort to cut stumps and foliage in the chainsaw and mulch treatments. Privet was left undisturbed in the third treatment which served as the experimental “control”. Pollinator and tree data were collected (see below for details) in five subplots within each plot: one in the center and the other four situated 30–50 m away from the center, approximately half-way between the center and each plot corner. Due to the unanticipated partial clearance of privet within the control plot at BG in the fall and winter of 2018, we did not collect pollinator data from that plot in 2019. We did collect tree data from that plot, however.
To better assess the recovery of pollinator communities following the removal of privet, we also sampled in three riparian forest locations near our plots that had never been colonized by privet. These plots are refered to as the “reference” condition. Two were located along the Apalachee River and Harris Creek in Greene County and one along Falling Creek in Oglethorpe County. Although pollinator data were collected at these reference plots, we did not collect tree data at these locations.
Tree Data Collection
In 2019, more than 13 years after the study began, we measured all native trees and woody shrubs greater than 2.5 cm diameter in the 0.1 ha subplots described above (0.5 ha per plot). We measured the diameter at breast height (1.4 m) of each tree using calipers. Any tree on the edge of a plot was included only if more than half of its stem was inside the plot boundary. The same methods were used 7 years previously (2012) to record the diameters of trees >4 cm within 0.04 ha areas centered on the same sublots (Hudson et al., 2014). These trees were individually tagged, allowing us to compare tree growth between treatments over the intervening seven-year period.
Pollinator Data Collection
Pollinators were sampled repeatedly following privet eradication beginning in 2006 (the season immediately following eradication) and ending in 2019. Sampling involved using colored pan traps consisting of blue and yellow plastic bowls filled with soapy water (530 ml capacity) (Figure 1B). While pan traps are known to be more effective for some taxa than others (Cane et al., 2000), and can under-sample bees when flowers are abundant (Baum and Wallen, 2011), they represent an inexpensive and highly standardized method for sampling a wide range of flower-visiting insect taxa (Campbell and Hanula, 2007; Ulyshen et al., 2021). Although such traps have been reported to be ineffective at sampling butterflies in previous studies (Vrdoljak and Samways, 2012), large captures in this study led us to begin recording butterfly data in addition to bee data in 2007. Each bowl was suspended about 30 cm above the ground on a wire stand (Figure 1B). We placed one trap of each color at each of the five subplots within each plot for a total of ten traps per plot. The two traps in each subplot were separated by 2 m. We operated the traps for a total of seven, seven-day periods (March, April, May, June, July, August, and October) each year. All samples from each plot and sampling period were combined and stored in ethanol. The same methodology was used to sample pollinators in the reference plots beginning in 2007 although the five subplots were arranged in a linear transect at those locations with ∼30–50 m spacing. We used printed (Mitchell, 1960, 1962; Glassberg et al., 2000; Gibbs, 2011; Gibbs et al., 2013) and online (https://www.discoverlife.org) keys along with an established reference collection to identify all bees and butterflies to species.
In addition to sampling with colored bowls, we sampled directly from privet flowers to determine the value of privet to bees as a floral resource. We spent a total of 22.5 person-hours sampling from privet growing in open areas near our sampling locations as well as additional sites in Athens, Georgia in 2004, 2005, 2006, and 2020. Voucher specimens are held in the personal research collection of MDU.
Analysis
After pooling tree data across subplots, we calculated the total number of stems ≥2.5 cm per ha, the total basal area of stems ≥2.5 cm per ha, and the change in basal area of the tagged subset of trees that were measured both in 2012 and 2019. These response variables are hereafter referred to as stem number, basal area, and growth, respectively. Only stems that remained the same or increased in diameter (n = 576) from 2012 to 2019 were included in our analysis of growth. We used the mixed procedure of SAS (SAS Institute, 2013) to compare these metrics among treatments with treatment and location as fixed and random effects, respectively. No transformations were necessary to meet model assumptions. Because an initial analysis found no significant differences of least square means between the chainsaw and mulch treatments for stem number (t = 0.01, P = 0.99), basal area (t = 0.45, P = 0.67) or tree growth (t = 0.85, P = 0.43), we treated these as a single “restored” treatment in the final analysis. Because different tree genera are likely to grow at different rates, we accounted for differences in tree composition among plots in our model of tree growth. To do this, we performed non-metric multidimensional scaling (NMDS) in PC-ORD (McCune and Mefford, 2011) using a matrix consisting of all tree genera included in our tree growth measurements. We used total basal area of remeasured trees in 2012 to reflect the relative abundance of each tree genus per plot. Genera present in fewer than three plots were dropped prior to analysis. This analysis yielded a one-dimensional solution (final stress = 35.7) and the resulting coordinates were included in the model of growth as a covariate. Correlations between this axis and the various tree genera are given in Supplementary Table 1. Finally, to compare the number of stems per ha between restored and control plots, the Wilcoxon Two-Sample Test was performed for each 5-cm diameter increment separately using the npar1way procedure of SAS.
Bee and butterfly data were also pooled across subplots and sampling periods for each plot and year of sampling. Because bee identifications were made by three different people over 13 years, an effort was made to make the datasets as consistent as possible by updating the nomenclature and combining some potentially confused species into single taxa (e.g., L. hitchensi/weemsi, etc.) rather than treating them separately. Out of caution, and because data were not collected at all sites every year (e.g., BG control not sampled in 2019), we chose to analyze each year separately. We calculated the total number of individuals (abundance), species (richness) and Shannon’s diversity for each plot and year and used the mixed procedure of SAS to compare these metrics among treatments with treatment and location as fixed and random effects, respectively. As a preliminary initial analysis found the abundance, richness, and diversity of bees and butterflies to be comparable between chainsaw and mulch treatments (Supplementary Table 2 and Supplementary Figure 1), we treated these as a single “restored” treatment in the final analysis. When assumptions were violated, the npar1way procedure of SAS was used to perform the Wilcoxon Two-Sample Test. To confirm the conclusions from this analysis, we used the iNEXT package (Hsieh et al., 2016) of R to compare 2019 bee and butterfly diversity among treatments based on the rarefaction and extrapolation sampling curves of Hill numbers. Hill numbers are a mathematically unified family of diversity indices (Chao et al., 2014) where the value of q determines how much weight is given to species based on their abundance. While q = 0 (richness) gives rare and abundant species equal weight, q = 1 (Shannon’s diversity) gives more weight to abundant species and q = 2 (Simpson’s diversity) counts only the dominant species (Hsieh et al., 2016). For both bees and butterflies, we analyzed sample-based (incidence) data (because sampling effort varied among control, reference, and restored treatments) and compared diversity at the base sample size which in this case was 8, the maximum reference sample size (Chao et al., 2014). Differences are considered significant when 95% confidence intervals do not overlap at the base sample size.
To compare the composition of bee and butterfly communities among the four treatments (i.e., the chainsaw and mulch treatments were not combined for this analysis) for each year separately, we used the multi-response permutation procedure (MRPP) with pairwise comparisons in PC-ORD (McCune and Mefford, 2011). We then tested if any of the bee or butterfly species collected in 2019 were strongly associated with one or more of the four treatments using the function multipatt (multilevel pattern analysis) in the R package indicspecies (Cáceres and Legendre, 2009). Indicator values range from 0 (no association) to 1 (complete association).
Finally, to better understand the diversity of bees visiting privet flowers, we calculated the Chao1 estimator using the rareNMtests package in R (Cayuela and Gotelli, 2014) which estimates the total number of species in a community and 95% confidence intervals based on a list of species with abundance data.
Results
Trees
In 2019 we measured a total of 4487 trees and woody shrubs >2.5 cm DBH (Supplementary Table 3). There were 33 genera represented in all, with five genera accounting for over 75% of the total basal area: Liquidambar (25.1%), Quercus (14.4%), Acer (13.2%), Platanus (12.8%) and Pinus (10.3%). Four genera accounted for over 75% of the number of stems: Acer (41.1%), Liquidambar (19.2), Ulmus (12.0) and Fraxinus (6.4). On average, the restored plots had nearly 60% more stems per ha than the control plots (mean ± SE = 854.5 ± 97.8 and 534.5 ± 84.5, respectively), a significant difference (Figure 3). This difference was driven primarily by trees ≤5 cm in diameter which were nearly four times more abundant in restored than control plots, a significant difference (Z = −2.13, P = 0.03) (Figure 4). In restored plots, this regenerating cohort of trees consisted largely of Acer which accounted for 80% of all stems ≤5 cm in diameter (Figures 1C,D). Acer was the most common genus among stems in this diameter class at all four locations, accounting for 45.6, 70.2, 77.3, and 90.7% of stems in the restored plots at SC, SS, WS, and BG, respectively. Three species of Acer were measured in this study but almost all of them (98%) were Acer negundo L. The other two species of Acer, A. leucoderme Small and A. rubrum L., were much less common.
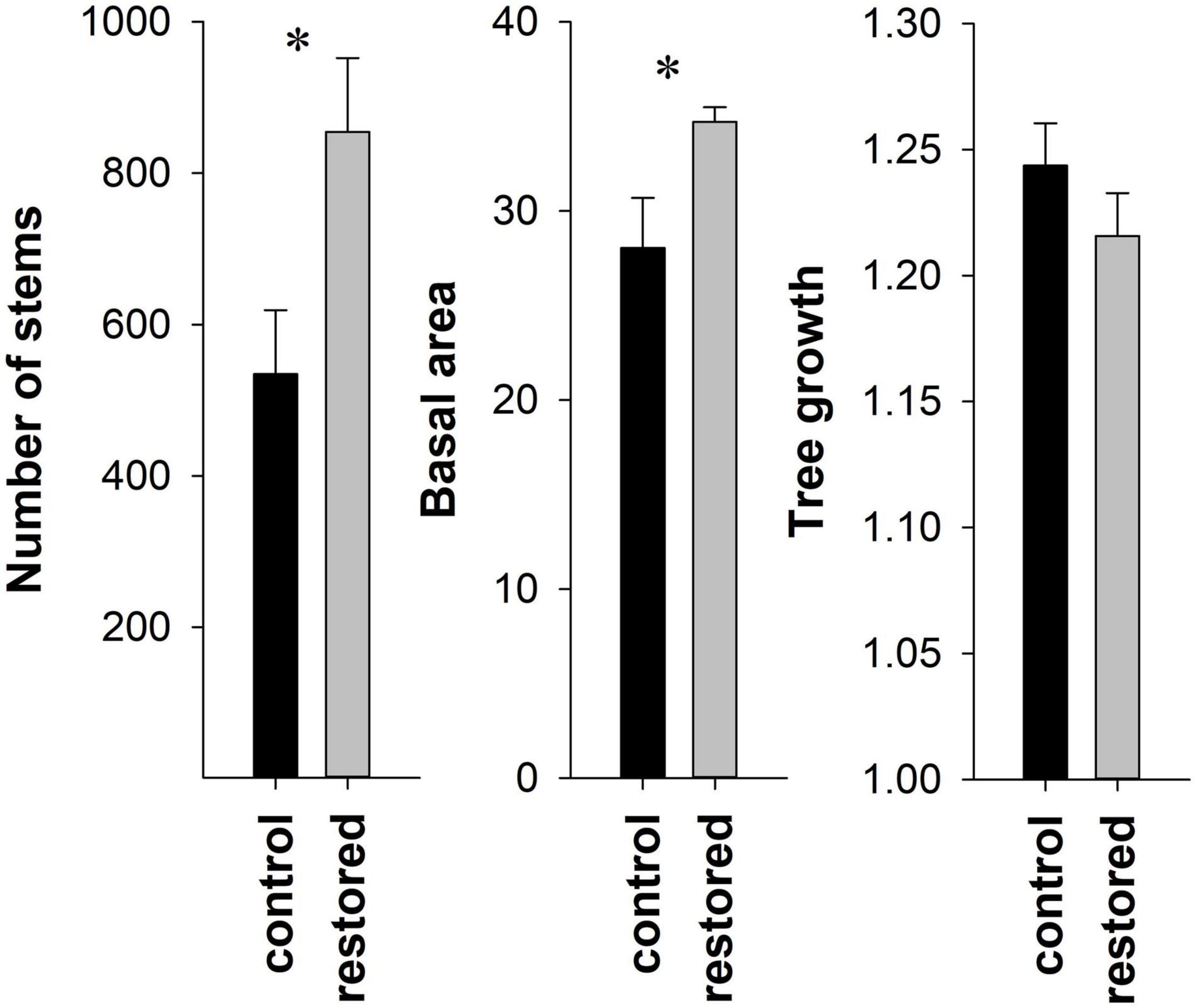
Figure 3. Mean ± SE number of native tree/shrub stems (≥2.5 cm diameter) per ha, basal area (m2) per ha (all stems ≥2.5 cm diameter), and tree growth in control (n = 4, black) and restored (n = 8, gray) plots. Asterisks denote significant differences.
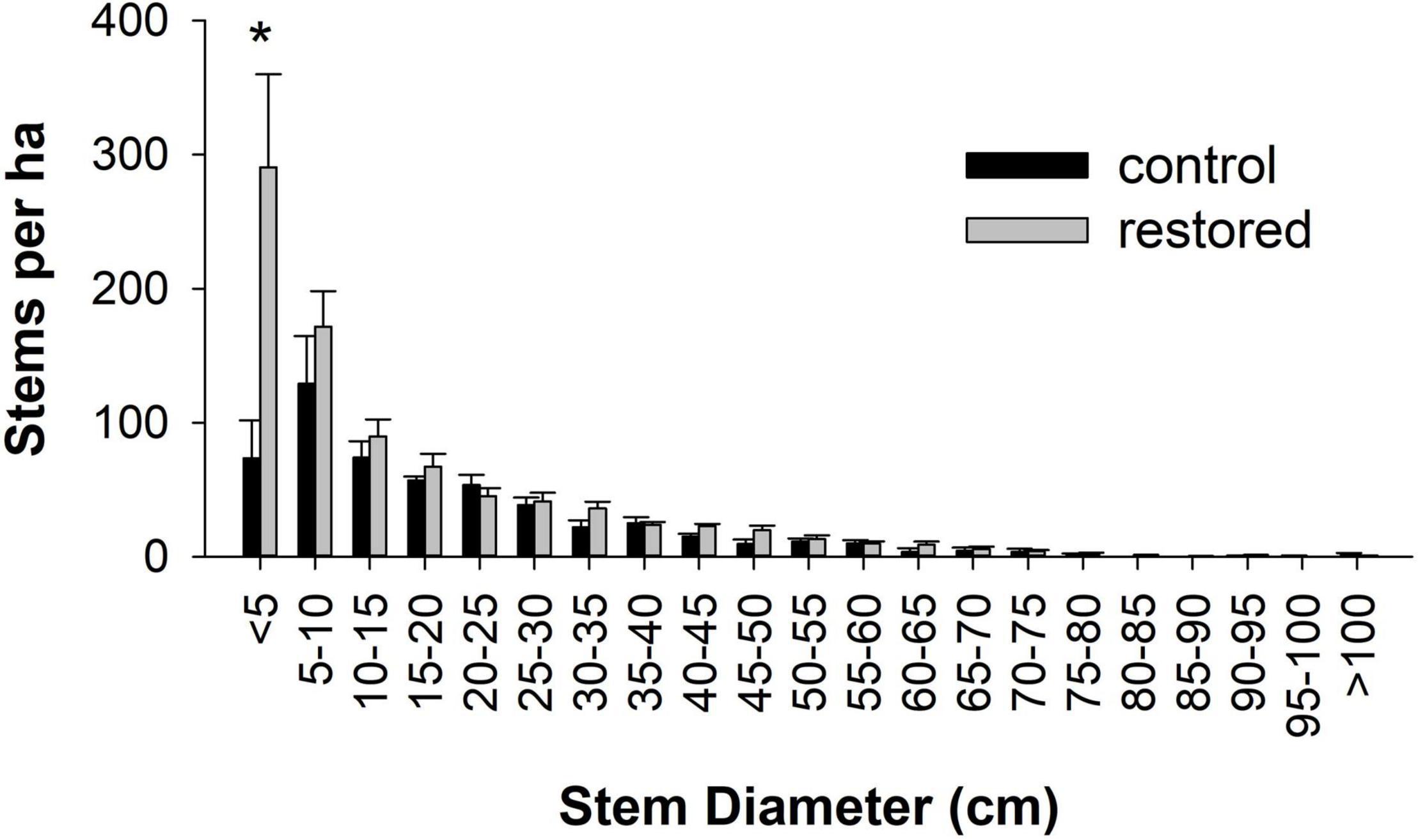
Figure 4. Mean ± SE number of stems per ha by diameter class in control (n = 4, black) and restored (n = 8, gray) plots. Asterisks denote significance based on the Wilcoxon rank-sum test.
Similar to the patterns for stem number, native basal area was nearly 24% higher, on average, in the restored plots than in the control plots (mean ± SE = 34.73 ± 0.76 and 28.05 ± 2.66, respectively), also a significant difference (Figure 3). However, based on the subset of trees measured both in 2012 and 2019, tree growth over this seven-year period did not differ between treatments (Figure 3).
Pollinators
Over the 5 years of sampling, we collected a total of 144 bee species from 14,511 individuals and 55 butterfly species from 5,027 individuals (Supplementary Table 4). Bee richness, diversity and abundance were significantly higher in the restored plots than the control plots across all years (Figure 5 and Table 1). The rarefaction and extrapolation curves of Hill numbers support these conclusions: species richness, Shannon’s diversity, and Simpson’s diversity were all significantly higher in the restored plots compared to the control plots at the end of the study (Supplementary Figure 2). Bee abundance and richness were particularly high in the restored plots the 2nd year (2007) after privet removal, even relative to the reference plots, but numbers gradually decreased thereafter until 2019 when they became comparable to the reference plots (Figure 5). Bee richness was highest in the restored and reference plots in March and then consistently lower thereafter based on 2019 data, a seasonal pattern consistent with observations in other temperate deciduous forests (Harrison et al., 2018). No such seasonality was observed in the control plots, however, where bee richness was consistently low, and lower than in the other treatments, throughout the year (Supplementary Figure 3). This difference remained significant even when data from March were excluded from the analysis (Supplementary Table 5). Butterfly richness and abundance were also significantly higher in the restored plots than the control plots in all five sample years (Figure 5 and Table 1), a pattern confirmed by rarefaction and extrapolation curves (Supplementary Figure 2). The same pattern was true for butterfly diversity in 2012 and 2019 but not in 2007 or 2011. Unlike bees, butterfly numbers were not dramatically higher than in the reference plots soon after privet removal. By contrast, butterfly richness, diversity, and abundance were comparatively higher in the reference plots and this remained true even during the last year of sampling. Unlike bees, there was no clear seasonal trend for butterfly richness based on 2019 data (Supplementary Figure 3).
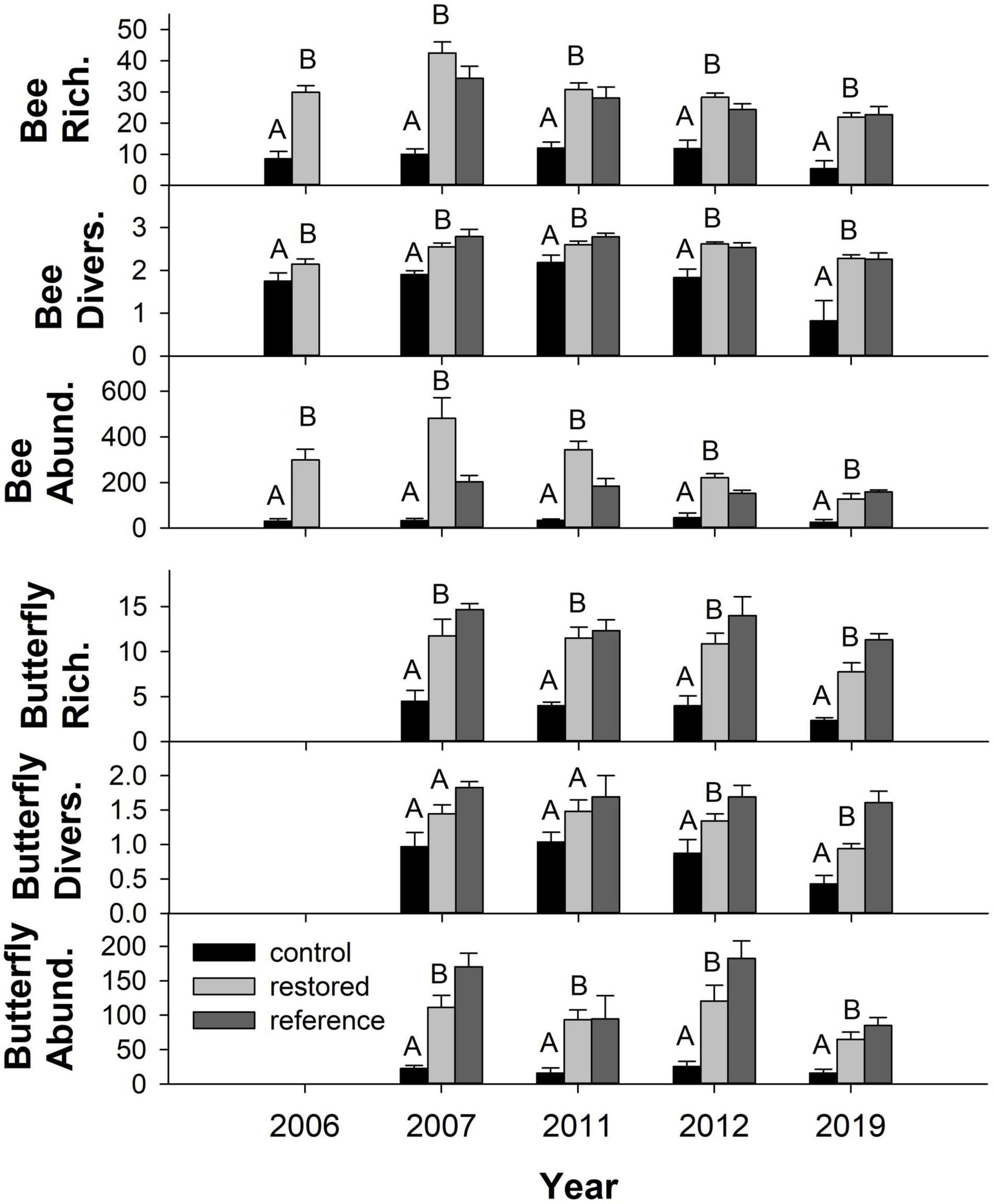
Figure 5. Mean ± SE species richness, abundance and diversity of bees (top) and butterflies (bottom) in control (i.e., privet-invaded, n = 3–4, black) and restored (i.e., privet eradicated, n = 8, light gray) plots as well as in reference (n = 3, dark gray) plots with no history of privet invasion. For each year, bars with different letters are significantly different. Note that the reference plots were not included in this analysis as they were not part of the blocked design.
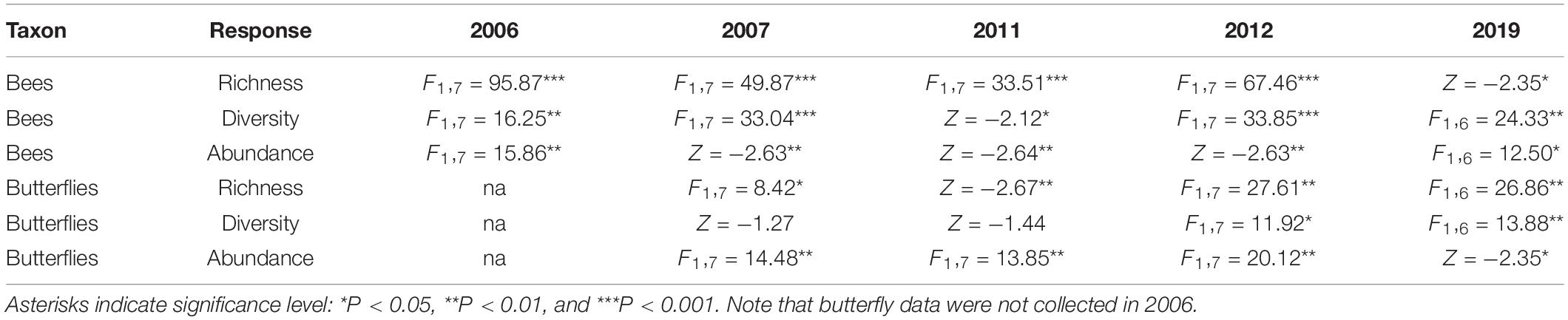
Table 1. Results from mixed models (F values) or the Exact Wilcoxon Two-Sample Test (Z values) comparing bee and butterfly abundance, diversity and richness between control and restored plots for each year separately.
The composition of bee and butterfly communities differed significantly between control plots and both privet-eradication treatments in all years (Table 2). The only difference in community composition between eradication treatments was observed for bees in 2006. There were no differences in community composition between eradication treatments and the reference plots after 2011 for bees or after 2007 for butterflies (Table 2). Three bee and two butterfly species were strongly associated with one or more of the treatments at the end of the study (2019) based on indicator species analysis. The bees Lasioglossum hitchensi/weemsi and Augochlora pura (Say) were both significantly associated with chainsaw, mulch and reference treatments (IV = 0.995, P = 0.003 and IV = 0.980, P = 0.002, respectively) and Lasioglossum imitatum (Smith) was associated with the mulch and reference treatments (IV = 1.0, P = 0.001). Among butterflies, Poanes zabulon (Boisduval and Le Conte) was associated with the chainsaw, mulch and reference treatments (IV = 0.994, P = 0.002) while Calycopis cecrops (Fabricius) was associated with the reference plots (IV = 1.0, P = 0.006). Notably, no species was significantly associated with the control treatment.
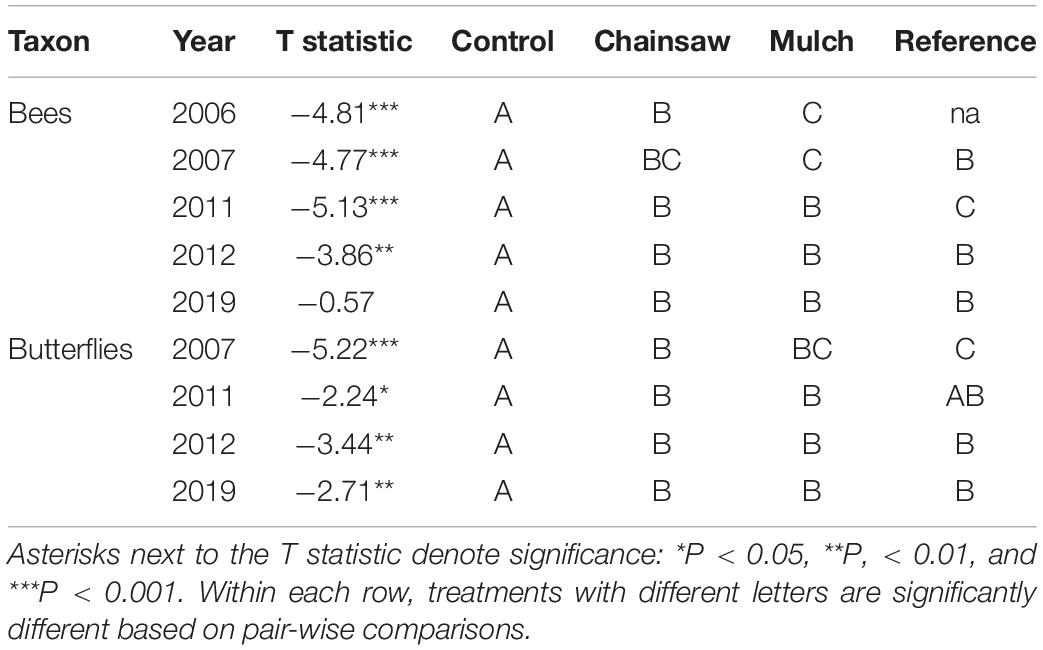
Table 2. Results from MRPP analysis comparing bee and butterfly composition among the four treatments.
Our efforts to sample bees directly from privet flowers yielded 240 specimens from 28 species (Supplementary Table 6). Xylocopa virginica (Linnaeus) and Apis mellifera L. accounted for over 70% of specimens while three species of Bombus (bimaculatus Cresson, griseocollis (De Geer) and impatiens Cresson) accounted for a further 13%. The other species collected were much less common, with 16 species being singletons. Our Chao1 estimate for the total number of species visiting privet blooms was 53.49 with a 95% confidence interval of 35.11–119.38.
Discussion
Our results demonstrate the long-term ecological benefits of eradicating Chinese privet from invaded forests. After 13 years, the restored plots had about 60% more stems and 24% greater basal area than the control plots. Because we observed no differences in existing growth rates among treatments, this dramatic increase in basal area can be attributed to the growth of new stems which established following the first privet eradication treatments in 2005. These results highlight the devastating effect privet invasion has on tree regeneration. Indeed, regeneration is so suppressed in privet-invaded sites that it is reasonable to expect forest cover to gradually disappear as overstory trees die without replacement.
Although woody shrubs in other systems are known to reduce overstory tree growth (Hartman and McCarthy, 2007), and this has been shown specifically for privet in past studies (Foard et al., 2016), we found no evidence for this in the current study. While it is possible that 7 years were insufficient to detect differences in growth rates between treatments, previous dendrochronological work by Hudson et al. (2014) supports the conclusion that privet does not suppress overstory tree growth in our plots. Despite contrasting results from other locations, this may not be surprising considering the seasonal flooding experienced annually at our study sites. The deposition of nutrient-rich sediments during these events may prevent competition between privet and established trees.
The benefits of privet removal on pollinators near the forest floor were immediate and long-lasting with the richness and abundance of bees and butterflies being consistently higher in restored plots than in control plots. These patterns are likely due to a combination of enhanced trap visibility and increased floral resource availability (although this was not measured in this study) in the restored plots. For bees, the most dramatic difference was observed during the second season post eradication when average abundance was 14.8 and 2.38 times higher in restored plots than in control and reference plots, respectively. Richness was similarly 4.25 and 1.24 times higher in restored plots than in the control and reference plots, respectively. Interestingly, this contrast between restored and reference sites weakened over time and disappeared by 2019, the last year of sampling. These patterns along with the compositionally similar bee communities at restored and reference sites suggest that bee communities have fully recovered within the timespan of this study. As more open forest canopies are known to favor bees and other pollinators (Hanula et al., 2016; Viljur and Teder, 2016; Roberts et al., 2017), we suspect that as the trees grew and filled in the existing canopy gaps, the canopy gradually became more closed (Figure 1D) and this likely depressed numbers of bees captured over time until they matched the reference conditions. Despite this canopy closure, bees remained significantly more species rich, diverse, and abundant in the restored plots than the control plots by the end of the study. Although 2019 bee richness in the restored and reference plots peaked in March, coinciding with the spring bloom period as in other temperate deciduous forests (Harrison et al., 2018), it remained significantly higher in restored plots than in control plots throughout the season (Supplementary Table 5 and Supplementary Figure 3).
Unlike bees, butterflies were comparatively less numerous in the restored plots than at the reference sites and this pattern was observed throughout the study. These results suggest that butterflies did not respond as strongly to the dramatic increase in canopy openness following privet removal and were still incompletely recovered in the restored plots by the end of the study. Although we are not aware of any formal comparisons of host specificity between butterflies and bees, we suspect butterflies may be more host specific than bees in our study area given that over 90% of bee species in eastern North America are polylectic (Fetridge et al., 2008). If true, butterflies may be more sensitive to the legacy effects of privet invasion on plant communities (Corbin and D’Antonio, 2012). While we did not collect data on herbaceous plants in 2019, plant communities differed considerably between restored and reference plots in 2006, 2007, and 2012 (Hanula et al., 2009; Hudson et al., 2014) and studies on other invasive shrubs suggest plant recovery may require many decades post-removal (Maclean et al., 2018). Butterfly recovery may mirror such patterns. Based on 2019 data, only one pollinator species, the butterfly Calycopis cecrops, was found to be specifically associated with reference plots compared to the other treatments. As this species feeds on rotting leaves (Brock and Kaufman, 2003), a resource likely to be found in all of the treatments, the reason for this association is not clear.
We collected a total of 28 bee species from privet flowers based on 22.5 h of sampling across 4 years. Whereas carpenter bees (Xylocopa virginica), honey bees (Apis mellifera) and bumble bees (Bombus spp.) accounted for most specimens captured, 16 species were represented by a single specimen. These results suggest that privet flowering in open areas may provide a source of nectar or pollen for some bee species during its late-spring blooming period. Because privet rarely blooms beneath the forest canopy (MDU, personal observation), however, these benefits are unlikely to be felt by bees within an invaded forest. Indeed, based on indicator species analysis of 2019 data, no species of bee or butterfly was found to be strongly associated with the control plots. By contrast, three bee species and two butterfly species were significantly associated with one or more of the other treatments.
Despite the dramatic differences in pollinator numbers reported in this study, it is important to note that sampling took place only near the forest floor. Previous studies suggest the negative influence of privet on bees and other insects may not extend into the canopy above the privet layer (Ulyshen et al., 2010a,2020). While it remains unclear why the canopies of temperate deciduous forests harbor such a diversity of bees (Urban-Mead et al., 2021), a pattern that extends even after trees have finished flowering (Ulyshen et al., 2010b), bees within the canopy appear to be unaffected by privet in the midstory (Ulyshen et al., 2020). Thus, by sampling only near the forest floor in this study, our results may exaggerate the negative influence of privet on pollinators. Regardless, it is clear from this long-term study that removing privet from invaded forests will greatly benefit both native plants and pollinators near the forest floor.
Conclusion
Privet removal allowed tree regeneration to recommence, helping to ensure the continuity of forest cover into the future. It also greatly increased the numbers of bees and butterflies near the forest floor, thus promoting the conservation of these essential insects. By the end of our study, bee communities, if not also butterflies, appeared to be fully recovered relative to reference sites with no history of privet invasion. The method of privet removal (i.e., chainsaw vs. mulching machine) did not matter for tree regeneration or pollinator recovery, suggesting that either method will achieve good results. Despite the success of this restoration effort, privet will eventually return to pretreatment level without further intervention (Hudson et al., 2014). The long-term recovery of areas invaded by this and similar shrubs will require a large and sustained commitment of resources (Benez-Secanho et al., 2018) over increasingly large areas (Wang et al., 2016) or the development of alternative control strategies such as biocontrol (Shaw et al., 2018).
Data Availability Statement
The original contributions presented in the study are included in the article/Supplementary Material, further inquiries can be directed to the corresponding author.
Author Contributions
MU and SH collected 2019 data and identified the bees and butterflies from 2019, respectively. JH identified, or oversaw the identification, of bees in previous years. MU analyzed the data and wrote the manuscript with inputs from the other authors. All authors contributed to the article and approved the submitted version.
Funding
This study was funded by the USDA Forest Service Special Technology Development Program (initially and after 5 years) and by the USDA Forest Service, Southern Research Station.
Conflict of Interest
The authors declare that the research was conducted in the absence of any commercial or financial relationships that could be construed as a potential conflict of interest.
Publisher’s Note
All claims expressed in this article are solely those of the authors and do not necessarily represent those of their affiliated organizations, or those of the publisher, the editors and the reviewers. Any product that may be evaluated in this article, or claim that may be made by its manufacturer, is not guaranteed or endorsed by the publisher.
Acknowledgments
We thank Jacob Hudson for help with field work and bee identification in 2011–2012 and three reviewers for comments that greatly improved the manuscript.
Supplementary Material
The Supplementary Material for this article can be found online at: https://www.frontiersin.org/articles/10.3389/fevo.2022.832268/full#supplementary-material
References
Batianoff, G. N., and Butler, D. W. (2003). Impact assessment and analysis of sixty-six priority invasive weeds in south-east Queensland. Plant Prot. Q. 18, 11–17.
Baum, K. A., and Wallen, K. E. (2011). Potential bias in pan trapping as a function of floral abundance. J. Kans. Entomol. 84, 155–159. doi: 10.2317/jkes100629.1
Benez-Secanho, F. J., Grebner, D. L., Ezell, A. W., and Grala, R. K. (2018). Financial trade-offs associated with controlling Chinese privet (Ligustrum sinense Lour.) in Forestlands in the Southern USA. J. For. 116, 236–244.
Brock, J. P., and Kaufman, K. (2003). Kaufman Field Guide to Butterflies of North America. Singapore: Hillstar Editions L.C.
Cáceres, M. D., and Legendre, P. (2009). Associations between species and groups of sites: indices and statistical inference. Ecology 90, 3566–3574. doi: 10.1890/08-1823.1
Campbell, J. W., and Hanula, J. L. (2007). Efficiency of malaise traps and colored pan traps for collecting flower visiting insects from three forested ecosystems. J. Insect Conserv. 11, 399–408.
Cane, J. H., Minckley, R. L., and Kervin, L. J. (2000). Sampling bees (Hymenoptera: Apiformes) for pollinator community studies: pitfalls of pan-trapping. J. Kans. Entomol. 73, 225–231.
Cash, J. S., Anderson, C. J., and Gulsby, W. D. (2020). The ecological effects of Chinese privet (Ligustrum sinense) invasion: a synthesis. Invasive Plant Sci. Manag. 13, 3–13. doi: 10.1017/inp.2020.4
Cayuela, L., and Gotelli, N. J. (2014). rareNMtests: Ecological and Biogeographical Null Model Tests for Comparing Rarefaction Curves. R Package v1. Vienna: The R Foundation for Statistical Computing.
Chao, A., Gotelli, N. J., Hsieh, T. C., Sander, E. L., Ma, K. H., Colwell, R. K., et al. (2014). Rarefaction and extrapolation with Hill numbers: a framework for sampling and estimation in species diversity studies. Ecol. Monogr. 84, 45–67. doi: 10.1890/13-0133.1
Corbin, J. D., and D’Antonio, C. M. (2012). Gone but not forgotten? invasive plants’ legacies on community and ecosystem properties. Invasive Plant Sci. Manag. 5, 117–124. doi: 10.1614/ipsm-d-11-00005.1
Cunningham-Minnick, M. J., and Crist, T. O. (2020). Floral resources of an invasive shrub alter native bee communities at different vertical strata in forest-edge habitat. Biol. Invasions 22, 2283–2298. doi: 10.1007/s10530-020-02248-y
Drake, S. J., Weltzin, J. F., and Parr, P. D. (2003). Assessment of non-native invasive plant species on the United States department of energy oak ridge national environmental research park. Castanea 68, 15–30.
Fagan, M. E., and Peart, D. R. (2004). Impact of the invasive shrub glossy buckthorn (Rhamnus frangula L.) on juvenile recruitment by canopy trees. For. Ecol. Manag. 194, 95–107. doi: 10.1016/j.foreco.2004.02.015
Fetridge, E. D., Ascher, J. S., and Langellotto, G. A. (2008). The bee fauna of residential gardens in a Suburb of New York City (Hymenoptera: Apoidea). Ann. Entomol. Soc. Am. 101, 1067–1077. doi: 10.1603/0013-8746-101.6.1067
Foard, M., Burnette, D. J., Burge, D. R. L., and Marsico, T. D. (2016). Influence of river channelization and the invasive shrub, Ligustrum sinense, on oak (Quercus spp.) growth rates in bottomland hardwood forests. Appl. Veg. Sci. 19, 401–412. doi: 10.1111/avsc.12240
Gibbs, J. (2011). Revision of the metallic Lasioglossum (Dialictus) of eastern North America (Hymenoptera: Halictidae: Halictini). Zootaxa 3073, 1–216.
Gibbs, J., Packer, L., Dumesh, S., and Danforth, B. N. (2013). Revision and reclassification of Lasioglossum (Evylaeus), L.(Hemihalictus) and L.(Sphecodogastra) in eastern North America (Hymenoptera: Apoidea: Halictidae). Zootaxa 3672, 1–117.
Glassberg, J., Minno, M. C., and Calhoun, J. V. (2000). Butterflies Through Binoculars. Oxford: Oxford University Press.
Goulson, D., Nicholls, E., Botías, C., and Rotheray, E. L. (2015). Bee declines driven by combined stress from parasites, pesticides, and lack of flowers. Science 347:1255957. doi: 10.1126/science.1255957
Greene, B. T., and Blossey, B. (2012). Lost in the weeds: Ligustrum sinense reduces native plant growth and survival. Biol. Invasions 14, 139–150. doi: 10.1007/s10530-011-9990-1
Hanula, J. L., and Horn, S. (2011a). Removing an exotic shrub from riparian forests increases butterfly abundance and diversity. Forest Ecol. Manag. 262, 674–680.
Hanula, J. L., and Horn, S. (2011b). Removing an invasive shrub (Chinese privet) increases native bee diversity and abundance in riparian forests of the Southeastern United States. Insect Conserv. Divers. 4, 275–283.
Hanula, J. L., Horn, S., and Taylor, J. W. (2009). Chinese privet (Ligustrum sinense) removal and its effect on native plant communities of riparian forests Invas. Plant Sci. Manag. 2, 292–300. doi: 10.1614/ipsm-09-028.1
Hanula, J. L., Ulyshen, M. D., and Horn, S. (2016). Conserving pollinators in North American forests: a review. Nat. Area. J. 36, 427–439.
Harrison, T., Gibbs, J., and Winfree, R. (2018). Forest bees are replaced in agricultural and urban landscapes by native species with different phenologies and life-history traits. Glob. Change Biol. 24, 287–296. doi: 10.1111/gcb.13921
Hartman, K. M., and McCarthy, B. C. (2007). A dendro-ecological study of forest overstorey productivity following the invasion of the non-indigenous shrub Lonicera maackii. Appl. Veg. Sci. 10, 3–14.
Hsieh, T., Ma, K., and Chao, A. (2016). iNEXT: an R package for rarefaction and extrapolation of species diversity (H ill numbers). Methods Ecol. Evol. 7, 1451–1456. doi: 10.1111/2041-210x.12613
Hudson, J. R., Hanula, J. L., and Horn, S. (2013). Removing Chinese privet from riparian forests still benefits pollinators five years later. Biol. Conserv. 167, 355–362.
Hudson, J. R., Hanula, J. L., and Horn, S. (2014). Impacts of removing Chinese privet from riparian forests on plant communities and tree growth five years later. For. Ecol. Manag. 324, 101–108. doi: 10.1016/j.foreco.2014.04.013
Klepac, J., Rummer, R. B., Hanula, J. L., and Horn, S. (2007). Mechanical Removal of Chinese Privet. Asheville: USDA Forest Service Southern Research Station.
Lázaro-Lobo, A., Lucardi, R. D., Ramirez-Reyes, C., and Ervin, G. N. (2021). Region-wide assessment of fine-scale associations between invasive plants and forest regeneration. For. Ecol. Manag. 483:118930.
Maclean, J. E., Mitchell, R. J., Burslem, D. F. R. P., Genney, D., Hall, J., and Pakeman, R. J. (2018). Understorey plant community composition reflects invasion history decades after invasive Rhododendron has been removed. J. Appl. Ecol. 55, 874–884. doi: 10.1111/1365-2664.12973
Mathiasson, M. E., and Rehan, S. M. (2020). Wild bee declines linked to plant-pollinator network changes and plant species introductions. Insect Conserv. Divers. 13, 595–605. doi: 10.1111/icad.12429
McCune, B., and Mefford, M. J. (2011). PC-ORD. Multivariate Analysis of Ecological Data, Version 6. Gleneden Beach: MjM Software.
Merriam, R. W., and Feil, E. (2003). The potential impact of an introduced shrub on native plant diversity and forest regeneration. Biol. Invasion 4, 369–373.
Mitchell, T. B. (1960). Bees of the Eastern United States, Volume I. Salisbury: The North Carolina Agricultural Experiment Station.
Mitchell, T. B. (1962). Bees of the Eastern United States, Volume II. Salisbury: The North Carolina Agricultural Experiment Station.
Ollerton, J., Winfree, R., and Tarrant, S. (2011). How many flowering plants are pollinated by animals? Oikos 120, 321–326.
Powney, G. D., Carvell, C., Edwards, M., Morris, R. K. A., Roy, H. E., Woodcock, B. A., et al. (2019). Widespread losses of pollinating insects in Britain. Nat. Commun. 10:1018. doi: 10.1038/s41467-019-08974-9
Richardson, D. M., and Rejmánek, M. (2011). Trees and shrubs as invasive alien species – a global review. Divers. Distrib. 17, 788–809.
Roberts, H. P., King, D. I., and Milam, J. (2017). Factors affecting bee communities in forest openings and adjacent mature forest. For. Ecol. Manag. 394, 111–122. doi: 10.1016/j.foreco.2017.03.027
SAS Institute (2013). SAS System for Windows, Version 9.4. Cary: Statistical Analysis Systems Institute.
Schlaepfer, M. A., Sax, D. F., and Olden, J. D. (2011). The potential conservation value of non-native species. Conserv. Biol. 25, 428–437.
Shaw, R. H., Cock, M., and Evans, H. C. (2018). The natural enemies of privets (Ligustrum: Oleaceae): a literature review, with particular reference to biological control. CAB Rev. 13, 1–24.
Smith, C., Harrison, T., Gardner, J., and Winfree, R. (2021). Forest-associated bee species persist amid forest loss and regrowth in eastern North America. Biol. Conserv. 260:109202. doi: 10.1016/j.biocon.2021.109202
Splitt, A., Skórka, P., Strachecka, A., Borański, M., and Teper, D. (2021). Keep trees for bees: pollen collection by Osmia bicornis along the urbanization gradient. Urban For. Urban Green. 64:127250. doi: 10.1016/j.ufug.2021.127250
Turner, T. N., Dean, T. J., and Kuehny, J. S. (2022). Long-term suppression of hardwood regeneration by Chinese privet (Ligustrum sinense). Front. For. Glob. Change 4:725582. doi: 10.3389/ffgc.2021.725582
Ulyshen, M. D., Hiers, J. K., Pokswinksi, S. M., and Fair, C. (2021). Pyrodiversity promotes pollinator diversity in a fire-adapted landscape. Front. Ecol. Environ. doi: 10.1002/fee.2436
Ulyshen, M. D., Horn, S., and Hanula, J. L. (2010a). Response of beetles (Coleoptera) at three heights to the experimental removal of an invasive shrub, Chinese privet (Ligustrum sinense), from floodplain forests. Biol. Invasion 12, 1573–1579.
Ulyshen, M. D., Soon, V., and Hanula, J. L. (2010b). On the vertical distribution of bees in a temperate deciduous forest. Insect Conserv. Divers. 3, 222–228.
Ulyshen, M. D., Horn, S., and Hanula, J. L. (2020). Effects of Chinese privet on bees and their vertical distribution in riparian forests. For. Sci. 66, 416–423.
Urban-Mead, K. R., Muñiz, P., Gillung, J., Espinoza, A., Fordyce, R., van Dyke, M., et al. (2021). Bees in the trees: diverse spring fauna in temperate forest edge canopies. For. Ecol. Manag. 482:118903.
Viljur, M., and Teder, T. (2016). Butterflies take advantage of contemporary forestry: clear-cuts as temporary grasslands. For. Ecol. Manag. 376, 118–125. doi: 10.1016/j.foreco.2016.06.002
Vrdoljak, S. M., and Samways, M. J. (2012). Optimising coloured pan traps to survey flower visiting insects. J. Insect Conserv. 16, 345–354. doi: 10.1007/s10841-011-9420-9
Wang, H.-H., Wonkka, C. L., Grant, W. E., and Rogers, W. E. (2016). Range expansion of invasive shrubs: implication for crown fire risk in forestlands of the southern USA. AoB Plants 8:plw012. doi: 10.1093/aobpla/plw012
Keywords: Apoidea, biodiversity, flower visitors, insects, Lepidoptera, restoration, pollination
Citation: Ulyshen MD, Horn S and Hanula JL (2022) Decadal Patterns of Forest and Pollinator Recovery Following the Eradication of an Invasive Shrub. Front. Ecol. Evol. 10:832268. doi: 10.3389/fevo.2022.832268
Received: 09 December 2021; Accepted: 03 February 2022;
Published: 22 February 2022.
Edited by:
Daniel de Paiva Silva, Goiano Federal Institute (IFGOIANO), BrazilReviewed by:
Keng-Lou Hung, University of Toronto, CanadaEdina Török, Institute of Ecology and Botany, Centre for Ecological Research, Hungarian Academy of Sciences, Hungary
Karen Goodell, Ohio State University, United States
Copyright © 2022 Ulyshen, Horn and Hanula. This is an open-access article distributed under the terms of the Creative Commons Attribution License (CC BY). The use, distribution or reproduction in other forums is permitted, provided the original author(s) and the copyright owner(s) are credited and that the original publication in this journal is cited, in accordance with accepted academic practice. No use, distribution or reproduction is permitted which does not comply with these terms.
*Correspondence: Michael D. Ulyshen, Michael.d.ulyshen@usda.gov