- Faculty of Biological Sciences, Institute for Ecology, Evolution, and Diversity, Goethe University Frankfurt, Frankfurt am Main, Germany
Understanding how species relate mechanistically to their environment via traits is a central goal in ecology. Many macroecological rules were found for macroorganisms, however, whether they can explain microorganismal macroecological patterns still requires investigation. Further, whether macroecological rules are also applicable in microclimates is largely unexplored. Here we use fruit body-forming fungi to understand both aspects better. A recent study showed first evidence for the thermal-melanism hypothesis (Bogert’s rule) in fruit body-forming fungi and relied on a continental spatial scale with large grid size. At large spatial extent and grid sizes, other factors like dispersal limitation or local microclimatic variability might influence observed patterns besides the rule of interest. Therefore, we test fungal assemblage fruit body color lightness along a local elevational gradient (mean annual temperature gradient of 7°C) while considering the vegetation cover as a proxy for local variability in microclimate. Using multivariate linear modeling, we found that fungal fruiting assemblages are significantly darker at lower mean annual temperatures supporting the thermal-melanism hypothesis. Further, we found a non-significant trend of assemblage color lightness with vegetation cover. Our results support Bogert’s rule for microorganisms with macroclimate, which was also found for macroorganisms.
Introduction
Coloration in organisms functions during thermoregulation (Caro, 2017; Cuthill et al., 2017; Delhey, 2019). Studying the relationship between organism traits and their thermal environment is traditionally an integral part of ecology and has led to formulating many universal rules (Bergmann, 1847; Allen, 1877; Rensch, 1929). Yet, understanding community shifts and identifying which functional traits respond to changes in the thermal environment remains important in times of global warming (McGill et al., 2006). One such rule, the thermal-melanism hypothesis (TMH)—Bogert’s rule—assumes an advantage of dark-pigmented ectotherms in cold habitats (Bogert, 1949). Macroecological patterns supporting this rule have been found in macroorganisms like insects (Zeuss et al., 2014; Heidrich et al., 2018), reptiles (Clusella-Trullas et al., 2008; Castella et al., 2013; Broennimann et al., 2014), and also in microorganisms such as yeasts (Cordero et al., 2018; Pinkert and Zeuss, 2018) and fruit-body-forming fungi (Krah et al., 2019). Fungal fruit body assemblages, recorded across 40 years, were darker at colder macroclimatic temperatures on a continental scale (Krah et al., 2019), supporting the thermal-melanism hypothesis. Despite these insights, we still require more studies investigating the macroecological rules for microorganisms, as a recent review suggested (Dickey et al., 2021). Further, besides cold environments via macroclimate, closed vs. open vegetation cover can result in cold habitats, with potential effects on fruit body color. Macroecological studies typically cannot account for the local variability due to their large grid size—100 km × 100 km (Rahbek, 2005; Chown and Gaston, 2016). Thus, we do not know whether macroecological rules can also explain trait responses in local thermal variability, e.g., mediated via vegetation cover. Finally, on macroecological scales, other processes, than climate, may produce the observed pattern, e.g., dispersal limitation (MacArthur and MacArthur, 1961; Leibold et al., 2004; Vellend, 2010; Peay et al., 2012). Therefore, we investigated Bogert’s rule for fruit body-forming fungi along a macroclimatic elevational gradient and assessed vegetation cover as a proxy for local microclimatic variability.
To our knowledge, changes in color lightness along an elevational gradient have not been studied before in fruit body-forming fungi. Diez et al. (2020) demonstrated that many species fruited at higher elevations across a 50 years dataset, likely in response to global warming, but we currently lack a mechanistic understanding of the driving processes involved. On a latitudinal scale, Krah et al. (2019) found that assemblages are darker in colder macroclimates. Although fruit bodies only represent an ephemeral stage in fungal development, sessile fruit body-forming fungi are dependent on the dispersal of spores released by their sexual reproductive organ (Halbwachs et al., 2016). Suboptimal dispersal of spores would decrease the chance of reaching new habitats or escaping unfavorable conditions (Norros et al., 2015), lowering fecundity and fungal genetic diversity (Lee et al., 2010). According to the thermal-melanism hypothesis, darker pigmentation may be advantageous because it allows for more rapid heating through solar radiation (Clusella-Trullas et al., 2008; Castella et al., 2013; Broennimann et al., 2014; Krah et al., 2019). Although a detailed understanding of the physiological processes is currently lacking, there is evidence that miotic recombination (step after karyogamy, when typically four haploid spores are produced) is enhanced by a higher temperature of the fruit body (Kües, 2000). Fruit body temperatures above the ambient is thus assumed to benefit the fruit body-forming fungus by faster or enhanced spore development. Other mechanisms may also lead to darker fruit bodies in higher elevations. Precipitation increases with the elevational gradient, leading to more humid environments. Such moist habitats may correspond with higher pathogen pressure (cf. Yin et al., 2016) and pigments exhibit antimicrobial properties (Cordero and Casadevall, 2017). Thus, darker fruit body assemblages with increasing precipitation would be plausible. We thus expect darker fruit body assemblages with higher precipitation.
Besides macroclimate (Tedersoo et al., 2014a; Vìtrovskı et al., 2019), fungal fruiting community composition is also influenced by the microclimate (Krah et al., 2018; Müller et al., 2020). The vegetation cover strongly drives microclimate in forest ecosystems: beneath a dense tree vegetation cover, macroclimatic mean conditions can be buffered, whereas the loss of vegetation cover leads to increased solar radiation and thus higher temperatures, UV radiation, and vapor pressure deficit (Scharenbroch and Bockheim, 2007; Thom et al., 2020; Frenne et al., 2021). Depending on the vegetation cover, the microclimatic variability can even exceed the variability of the macroclimate (Zellweger et al., 2020). Although in deciduous forests with completely closed vegetation cover, less than 10% of direct beam and diffuse radiation reaches the forest floor, they still experience diurnal fluctuations in near-ground temperature (Reifsnyder et al., 1971; Hutchison and Matt, 1977; Boehnke, 2021). Further, the loss of vegetation canopy has increased with climate warming and is likely to increase further due to enhanced disturbances and tree die-off due to climate change (Schelhaas et al., 2003; Seidl et al., 2014). Vegetation canopy cover has thus frequently been used as proxy for stand microclimate (Scharenbroch and Bockheim, 2007; Seibold et al., 2016; Krah et al., 2018; Müller et al., 2020; Thom et al., 2020; Vogel et al., 2020). We have no prior knowledge of fruit body coloration in microclimates; thus, different responses are theoretically possible. First, dark forest stands with high vegetation cover are colder than those under open vegetation cover. Since darker forest stands are not free of radiation (Reifsnyder et al., 1971; Hutchison and Matt, 1977), we would expect even darker fruit bodies under high vegetation cover if the thermal-melanism hypothesis can explain their pattern. Further, when considering the harsher conditions under open vegetation cover—higher maximum temperatures, drought, radiation—both more light-colored or more dark-colored fruit bodies could be expected under open vegetation cover. Under open vegetation cover, dark fruit bodies may heat up rapidly and overheat and thus weak pigmentation may reduce heat stress [“thermal-melanism hypothesis” (Bogert, 1949)]. However, strong pigmentation of fruit bodies may also lower the damaging effect of radiation and desiccation under open canopies because pigments can reduce oxidative stress (Cordero and Casadevall, 2017) [“melanism-desiccation hypothesis” (Law et al., 2020) and “photo-protection hypothesis” (Rensch, 1929; Law et al., 2020)].
Here we thus test the response of the assemblage fruit body color lightness, calculated as the assemblage mean, along with a local elevational gradient (mean annual temperature difference ca. 7°C between highest and lowest plot) and a vegetation cover gradient as proxy for microclimate (Figure 1). We hypothesize the following pattern: (1) the assemblage fruit body color lightness decreases with macroclimatic temperature; (2) The assemblage fruit body color lightness decreases with higher vegetation coverage (lower microclimatic temperature); however, alternative patterns are also possible, as outlined above by the melanism-desiccation hypothesis or photo-protection hypothesis. These hypotheses further relate to whether macroecological rules are similar between macro- and microorganisms and whether they can also apply to microclimatic temperature.
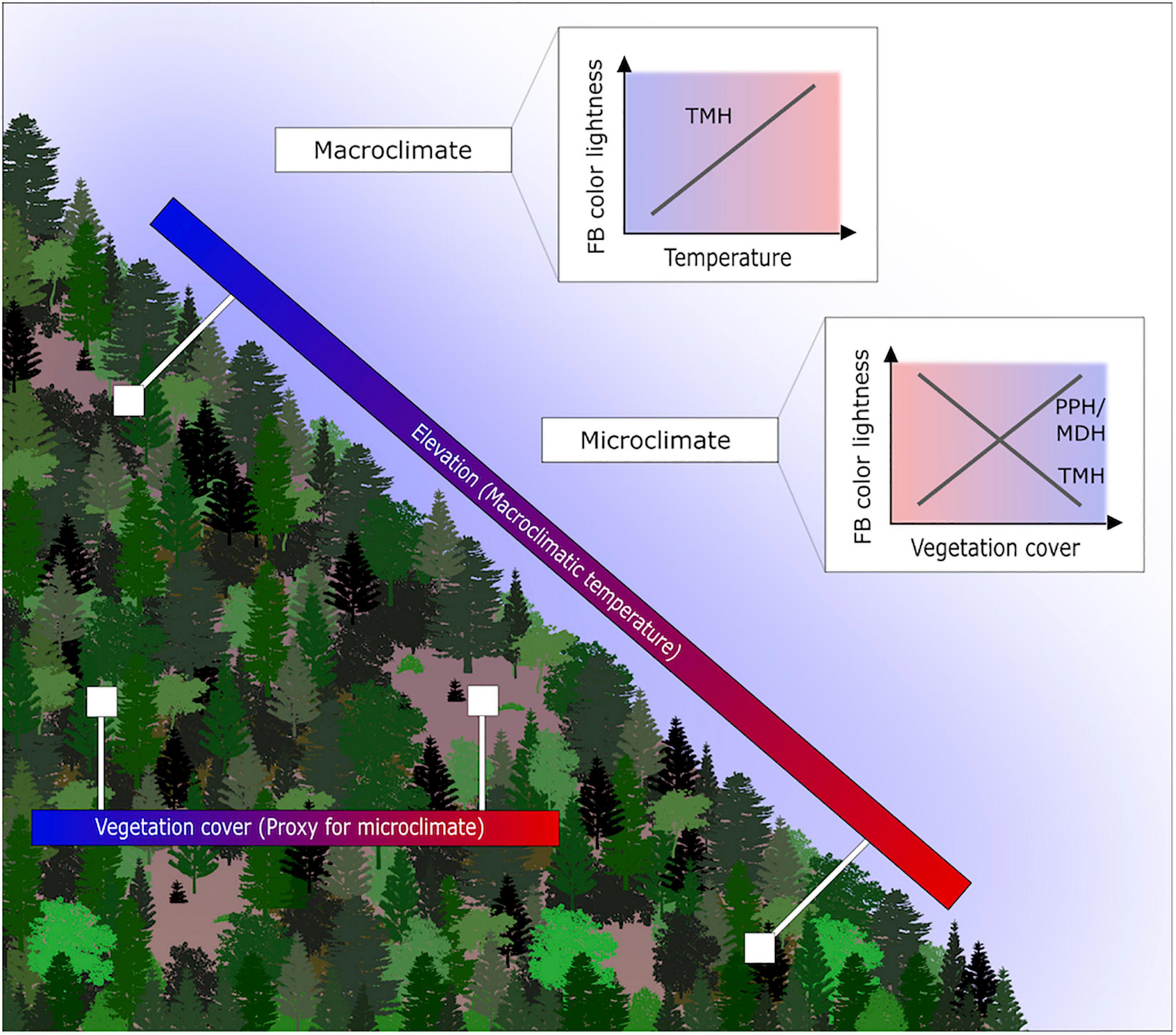
Figure 1. Study setting, gradients and expected pattern of assemblage fruit body color lightness. Displayed are the elevational gradient reflecting the macroclimatic temperature gradient. Further, displayed is the closed and open vegetation cover, which we use as a proxy for the microclimate. We investigate whether the pattern of the assemblage fruit body color lightness follows predictions of the thermal-melanism hypothesis (TMH), which states darker pigmented individuals in colder environments. Alternative hypotheses for the microclimate include the photo-protection hypothesis (PPH; vegetation cover as a proxy for UV radiation) and the melanism-desiccation hypothesis (MDH; vegetation cover as a proxy for drought).
Materials and Methods
Study Site and Design
Our data set was assembled within the BIOKLIM-project, which was installed to investigate the dependency of various taxa on the environmental factors and the expected effects of climate change on mountain range forest ecosystems in Central Europe (Bässler et al., 2009). The BIOKLIM-project is situated both inside and outside the boundaries of the Bavarian-Forest National Park, located in southeastern Germany (48°54′ N, 13°29′ E) along the border to the Czechia. The National Park covers an area of approximately 242 km2 of the Bohemian massif. In conjunction with the adjacent Czechia Šumava National Park, it forms one of the most extensive forest landscapes in Central Europe (Bässler et al., 2010a). It is characterized by montane and high montane areas with slopes facing predominantly southwest toward the Danube River. The elevational gradient of approximately 1,100 m (between 287 and 1,420 m a.s.l.) of the study site results in a difference in the mean annual air temperature of 10.5°C in valleys near the Danube River and 3.8°C in higher montane zones as well as the total yearly precipitation ranging from 760 to 1,340 mm in the year 2016. This precipitation occurs as snowfall with snow cover persisting for 7–8 months at high elevations and 5–6 months in valleys, respectively (Cailleret et al., 2014). The forested area of the National Park, which accounts for 98% of its surface (Elling et al., 1987), mainly consists of tree species such as European Beech (Fagus sylvatica) and Norway Spruce (Picea abies). At higher elevations, the montane forest is dominated by Norway Spruce (Bässler et al., 2015b).
Following the elevational gradient, 157 plots were established along four transects. The arrangement of the plots ensures to encompass the main vegetation- and management types available and map the elevational gradient well with several replications.
Fruit Body Inventory
The sampling process of fungal fruit bodies followed the Alberta Biodiversity Monitoring Program (Stokland and Sippola, 2004) conducted from August to October 2016. Here, a total time of 2 h per plot was set for sampling an area of 0.1 ha, in which fungal fruit bodies from a minimum of 15 dead-wood objects representative for each plot were collected. For the remainder of the time, fungi from as many objects as possible are sampled. For further information regarding the sampling process also see Bässler et al. (2009) and Bässler et al. (2010b). Species were identified either in the field or microscopically if necessary. The nomenclature follows that of MycoBank (Crous et al., 2004). To study the response of assemblage mean fruit body color lightness to components of the thermal environment, we standardized our dataset to a group of fungal species with comparable traits due to similar lifestyles. Wood-decaying fungi are of utmost importance for forest ecosystems due to their role in circulating nutrients and providing habitats for other organisms (Lonsdale et al., 2008). Retaining diversity among this group is crucial for a forest’s regeneration and ultimately the associated ecosystem services it provides. Like other saproxylic organisms, however, wood-decaying fungi are threatened by removing deadwood through silviculture management and general loss of old growth forests (Lindhe et al., 2004; Lonsdale et al., 2008). This threat highlights the need to understand species composition through trait-based assembly processes better. We selected saprotrophs within the dataset based on an existing genus-coding (Tedersoo et al., 2014b). Ultimately, 1,771 samples of wood-decaying fungi from 144 fungal species were considered in our study (Supplementary Table 1), with an average of 14 ± 5 species per plot (mean ± SD). Please note that we also performed statistical analyses (see below) separately for both fruit body types and retrieved highly similar results (data not shown) and therefore analyzed both fruit body types together.
Environmental Variables
To address our hypotheses, we investigated the response of fungal fruit body color lightness to macro- and microclimate. We used macroclimatic variables based on an ArcEGMO terrain model including information on 14 main climate stations, 88 rain gauges, and 13 temperature and humidity-logger, and 30 data loggers placed on representative plots (Becker et al., 2002). The relevant environmental variables could then be extracted from the model for each plot (Bässler et al., 2010a). For further details of the modeled macroclimatic data please see Müller et al. (2009) and Bässler et al. (2010a; 2010b,2015b; 2016). Based on this macroclimatic modeled data we calculated the mean annual temperature of the vegetation period and the summed precipitation of the vegetation period. We have chosen the vegetation period as the frame for our environmental variables as this is also the main time for mycelial activity and fruit body formation in fungi (cf. Andrew et al., 2016; Bässler et al., 2016). Further, we quantified vegetation cover as the proxy for microclimatic temperature (Seibold et al., 2016; Krah et al., 2018; Frenne et al., 2019; Vogel et al., 2020), which was individually estimated per plot during the fruit body sampling. The degree of vegetation coverage represents the microclimatic conditions of the mushroom fruiting bodies, encompassing multiple aspect of the microclimate such as temperature, amount of UV-radiation, and vapor pressure deficit (Scharenbroch and Bockheim, 2007; Thom et al., 2020). The vegetation cover is defined here as the sample area shaded by the horizontal projection of the overlaying layers of tree foliage (upper, middle, and under layer) in percentage (Bässler et al., 2009). Due to multiple layers, this percentage can exceed 100%. The range of this environmental variable extends from completely open (0% vegetation cover) to covered by two layers of vegetation (200% vegetation cover) with a mean vegetation cover of 93.7% and a standard deviation of 48.1%. Additionally, we quantified variables associated with the host, including number of tree species (hosts), summed dead-wood surface area, and average decay stage per plot. These variables were recorded during the sampling. The number of tree species per plot derives from the dead-wood objects hosting the sampled fungi of each plot. The sampled dead-wood surface area was calculated as the sum of the surface of all sampled objects. The surface was approximated via the recorded length and diameter describing a cylindrical shape. To calculate the average decay stage, each dead-wood object was attributed a numerical value relating to the progress of decay [alive (0), freshly dead (1), bark loose or removed (2), splint already soft (3), and log shape disappeared (4); Albrecht, 1990] which was then averaged per plot.
Trait Data
To link the occurrence of species to their traits, we attributed a mean cap color lightness value to each species. We adopted a protocol previously developed and established to be accurate (Krah et al., 2019) to determine the lightness from the HSL (hue, saturation, lightness) color space model (van den Broek and van Rikxoort, 2004). Here, a representative and publicly available image for each species is selected based on the authors’ expertise to represent field conditions from different websites.1–6 The color values of nine different pixels (one in the center of the fruit body cap and two radiating outward in four orthogonal directions) are sampled using the program “pipette” (Stefan Trost Media7). The three components, hue, lightness, and saturation were averaged for each species, and the mean lightness was used for further analyses (Supplementary Figure 1). To ensure accurate data, we applied a quality control protocol, e.g., that the selected images are neither over- nor underexposed and the pixels are not sampled in areas with reflections from water or contaminations (e.g., leaves, dirt, animals) (Krah et al., 2019). We assumed variation in color lightness variation to be higher at the interspecific- than intraspecific-level.
Another thermally relevant trait included in our analysis was fruit body size (Bässler et al., 2021). The minimum and maximum fruit body cap diameter values were obtained from the literature (Knudsen and Vesterholt (eds), 2012) and online resources.8–10 We calculated the average fruit body cap diameter from these and measured fruit body size. In our analysis, we only considered Basidiomycetes with stipitate- and sessile-pileate fruit bodies to ensure that our samples share a comparable fruit body morphology, and hence be subjected to similar ecological constraints (Tóth and Feest, 2007; Bässler et al., 2015a). Fruit body types were coded based on Sánchez-García et al. (2020).
Preparation of Community Matrix and Null Model
We determined the assemblage fruit body color lightness at the plot level. Here, a community matrix with fungal species (Supplementary Table 1) and the sampled plots in presence-absence data was created. Next, we calculated assemblage means for the log10-transformed trait values of the species per plot. The minimum number of species per assemblage (plot) is important to ensure more meaningful communities and mean trait values. However, larger minimum number of species per plot removes plots from the analysis, thus reducing the elevational range and statistical power. To maximize the number of species per plot and the number of plots, we used at least five species per plot (n ≥ 5). The effects of the main results remained significant with lower and larger number of species per plot (n ≥ 3, n ≥ 7) (Supplementary Table 2). An object-based analysis was also considered, however, due to many fine woody debris objects harboring only one or two species, too many data points would have been lost from the analysis. Based on a threshold of five species per plot, 129 plots remained for the analysis with an elevation range from 287 to 1,420 m a.s.l., covering a mean temperature of 7.9–14.5°C.
The assemblage fruit body color lightness might be correlated to the species diversity of communities, which differs with vegetation cover (Krah et al., 2018), therefore potentially confounding results. Further, the trait pattern observed may also stem from a changed mycelium community, which may have differing fruit body morphology independently of microclimate (artifact). In this case, we would expect a random distribution of traits with microclimate. To account and test for both an uneven number of species and to test against the artifact, we used a null model (“independentswap,” Gotelli and Entsminger, 2003) that randomizes species occurrence across sites but fixes both marginal sums for sites (i.e., species richness of sites) and marginal sums for species (i.e., occupancy of logs across the plots). We used the function randomizeMatrix from the R package picante (Kembel et al., 2010). We calculated the observed logarithmic assemblage mean (OBS) as well as the standardized effect size (SES) based on Cohen (2013) with 10,000 randomizations. Here, the expected mean across the 10,000 randomizations is subtracted from the observed mean, and the difference is divided by the standard deviation of the randomizations for each plot.
Statistical Analysis
We used a multivariate linear model to test the response of assemblage fruit body color lightness to macro- and microclimate. We computed separate models with the observed assemblage mean (OBS) and the standardized effect sizes (SES) as the response variable. Our main goal was to test, whether the assemblage mean fruit body color lightness responds to the thermal environment. Therefore, we included temperature (mean annual temperature of the vegetation period) and vegetation cover as explanatory variables. We further added the summed precipitation during the vegetation period as predictor to the model.
Additional to the macroclimate, host-related parameters may further influence our analysis and therefore we considered the number of host species, the sampled surface and the decay stage as covariates: (i) The host tree species was found to influence certain traits of wood-inhabiting fungi (Purhonen et al., 2020). Although we did not expect a response regarding color lightness, we included the number of host tree species in the model to account for potential effects. (ii) To account for sampling bias and standardize each plot for sampling effort, the log10-transformed summed surface area of all sampled dead-wood objects was also incorporated. (iii) Further, the stage of decay is associated with water content and temperature stability of the dead-wood (Pouska et al., 2016). Therefore, certain decay stages could favor specific fruit body traits and ultimately affect fungal community composition (Rajala et al., 2012; Pouska et al., 2017). Therefore, we added it to the model. (iv) Fruit body size in fungi was recently a thermally relevant fruit body trait (Bässler et al., 2021). We accounted for potential correlations between color lightness and size traits in response to temperature by including fruit body size in our model.
Finally, collinearity amongst the predictor variables might affect the model performance. To avoid collinearity, Dormann et al. (2013) recommend that all pairwise correlation coefficients should not exceed the threshold of | r| < 0.7. The variables exhibit only weak collinearity (| r| < 0.6), and thus all could be used (Supplementary Figure 2). Especially, temperature and vegetation cover showed no substantial collinearity (r = 0.38). Thus, macroclimatic temperature was not substantially correlated to vegetation cover.
Results
Our multivariate linear model found that temperature was the most important predictor variable (highest effect size of the model predictors, Table 1). The model showed an explained variance of 29% (R2 = 0.29). Further, note that temperature alone had an adjusted R2 of 0.19 when considered as univariate predictor (Figure 2). Overall mean temperature positively affected the assemblage fruit body color lightness (t = 5.25, p < 0.001; Table 1 and Figure 2). Assemblage fruit body color lightness was further positively influenced by precipitation (t = 2.18, p = 0.031; Table 1) and negatively by average decay stage (t = −3.15, p = 0.002; Table 1). However, the assemblage mean color lightness was not significantly affected by vegetation cover (t = −0.95, p = 0.345; Table 1 and Figure 2). The observed assemblage fruit body lightness responses were consistent with the responses of the standardized effect sizes of the assemblage lightness based on the null model (Table 1 and Figure 3).
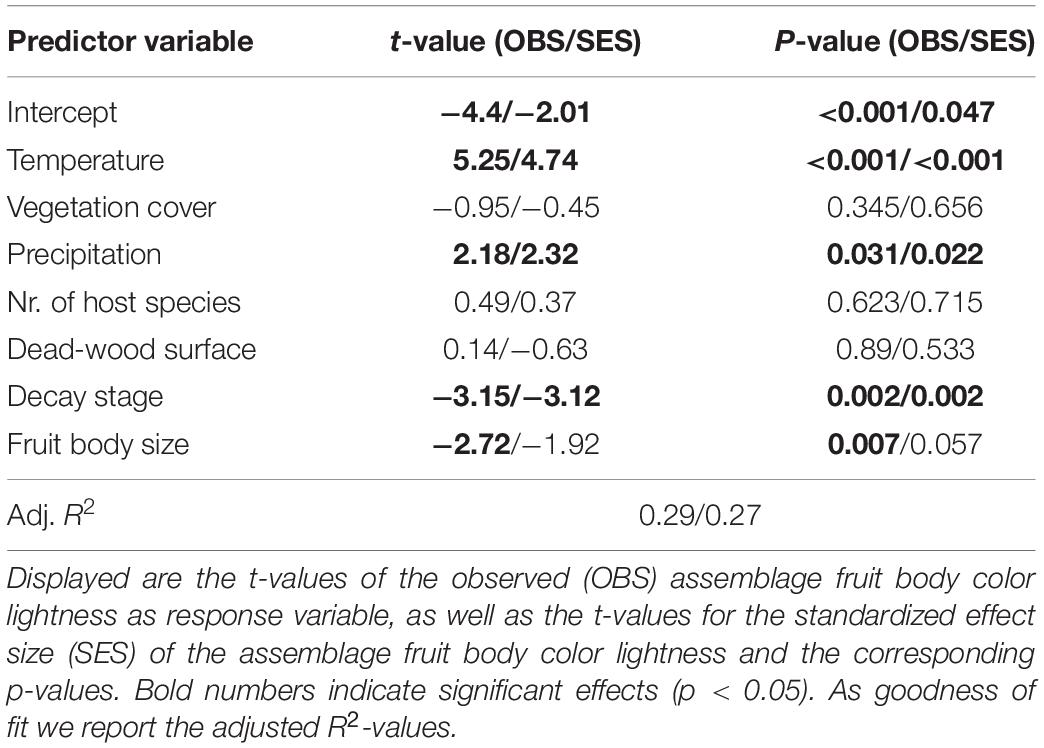
Table 1. Multivariate linear model testing the effect of temperature on assemblage fruit body color lightness.
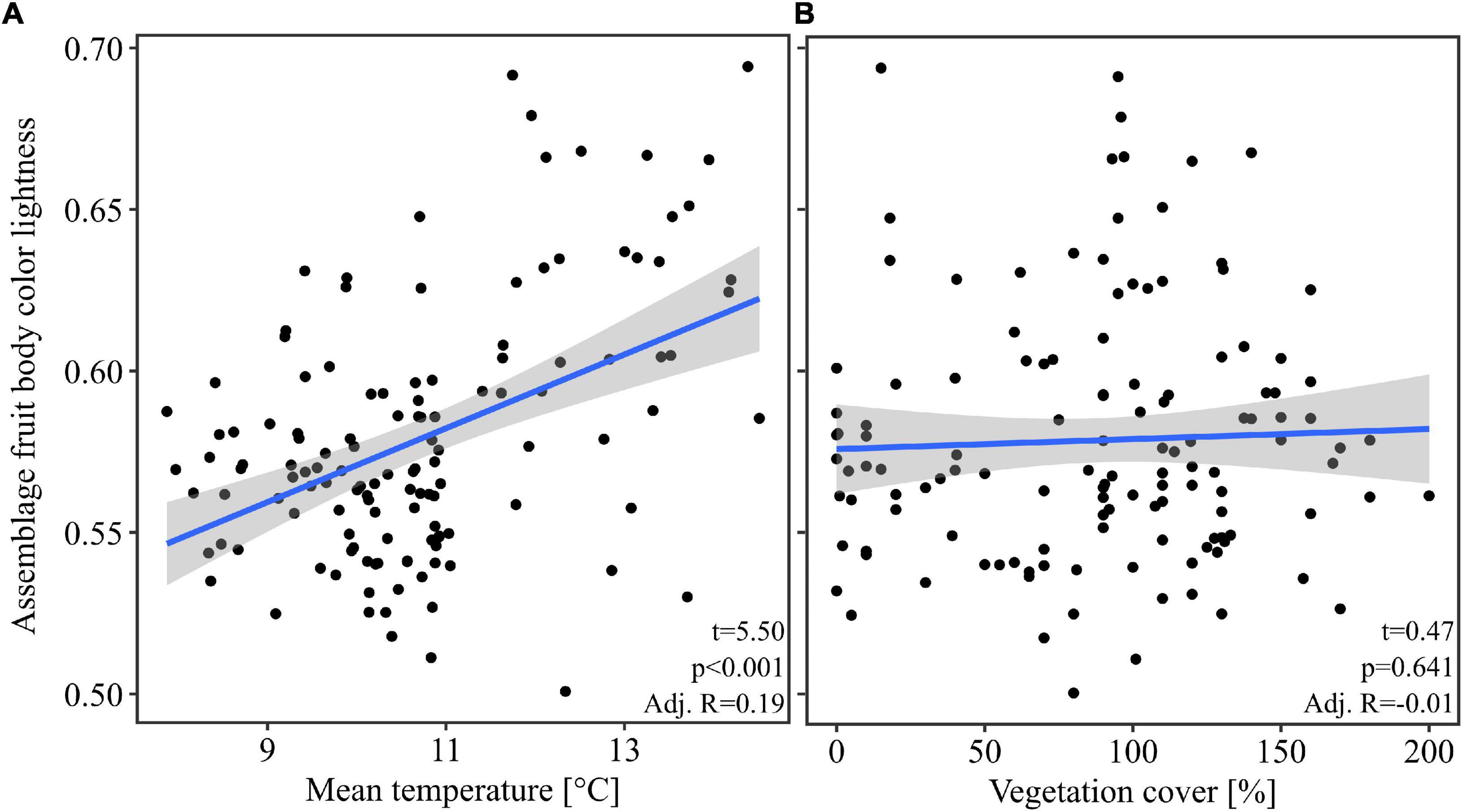
Figure 2. Response of the assemblage fruit body color lightness. (A) Response of the assemblage fruit body color lightness with the mean temperature. (B) Response of the assemblage fruit body color lightness with the vegetation cover. The blue line indicates the univariate linear regression line with the standard error of the mean (shaded area). Statistics within plots are from univariate linear models. For multivariate statistics see Table 1.
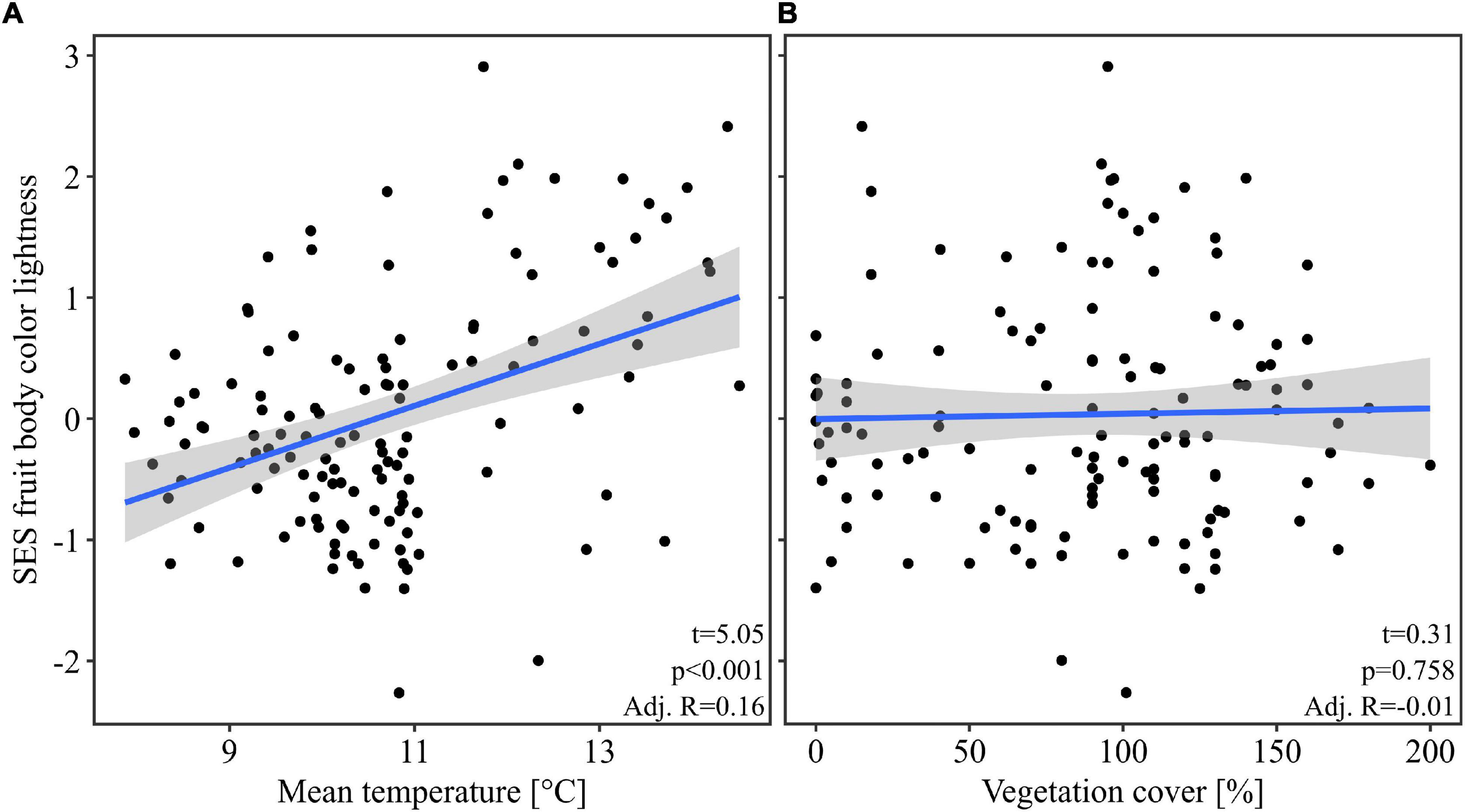
Figure 3. Response of the standardized effect size of the assemblage fruit body color lightness. Here we used the null model “independentswap.” Response of the standardized effect size (SES) of the assemblage mean fruit body color lightness (A) to the mean temperature, and (B) to the vegetation cover per plot. The blue line indicates the univariate linear regression line with the standard error of the mean (shaded area). For multivariate statistics see Table 1.
Discussion
We tested patterns predicted by the thermal-melanism hypothesis (Bogert’s rule) for fruit body-forming fungi on a local macro- and microclimatic gradient. We found significantly darker fungal assemblages in colder macroclimatic temperatures and lower precipitation. However, we did not find a significant effect of the vegetation cover (proxy for microclimate) on fungal color lightness. Our findings thus support the thermal-melanism hypothesis for ectotherm macrofungi. However, fruit body coloration of wood-inhabiting fungi does not seem to explain fruiting community changes between microclimates.
Multiple macroecological studies investigating color lightness of different species groups along macroclimatic latitudinal gradients, have found broad support of the thermal-melanism hypothesis (Lindgren et al., 2014; Zeuss et al., 2014; Heidrich et al., 2018; Stelbrink et al., 2019). Besides latitudinal gradients, darker coloration has also been found at higher elevations in ants, bees, flies, wasps, and moths (Bishop et al., 2016; McCabe et al., 2019; de Souza et al., 2020; Heidrich et al., 2021). Thus, currently most macroecological support comes from macroorganisms (Dickey et al., 2021). We can add such a local pattern for microorganismal fruit body-forming fungi with this study. Thus, accumulating evidence suggests the generality of darker organisms in colder climates. However, according to Cordero et al. (2018), three elements should be fulfilled upon which the thermal-melanism hypothesis could be confirmed: (1) spatial trait patterning with temperature, (2) elevated heating of dark-colored individuals under same conditions, and (3) a fitness advantage of dark-colored individuals in cold climates. For fruit body-forming fungi, there is now support for (1), via our findings and one previous study (Krah et al., 2019). Further, Krah et al. (2019) also could show more rapid heating of darker fruit bodies. However, it is currently unclear whether darker fruit body coloration leads to a fitness advantage in colder climates than light-colored fruit bodies. Further studies should thus add this missing link to the thermal-melanism hypothesis for fruit body-forming fungi, e.g., by measuring fecundity (fruit body biomass and number of viable spores) of differently light-colored species within experimental settings where temperature can be independently manipulated. Besides temperature, we also found darker fruit body assemblages with low precipitation (Table 1), which is in contrast to our expectation that moister habitats contain a higher pathogen pressure resulting in more defense via pigmentation. Our results thus suggest that drier conditions are related to pigmentation, however, please note that our elevational gradient is situated in an area with overall high precipitation and thus our study site is not able to resolve drought conditions as e.g., occurring in Spain.
Further, regarding microclimate, we did not find a significant response of assemblage fruit body color lightness of wood-inhabiting fungi with vegetation cover. Studies testing the coloration of assemblages in microclimates are otherwise rare. One study tested moth color along an elevational gradient and found 10–20% explained variation of vegetation cover but did not find marked differences in color between closed and open stands consistent with our findings (Heidrich et al., 2021). Another study investigated color lightness in ant communities within different tree strata and found that they are darker in the canopy than near the ground, which the authors attribute to increased UV radiation (Law et al., 2020). We can think of three possible ecological explanations for a lack of response of assemblage fruit body color lightness with microclimate (for data limitations, see below): First, we used mean species traits and although a significant change in community composition was found between microclimates (Krah et al., 2018; Müller et al., 2020), changes in traits may be more subtle. Thus, intraspecific trait variability of individuals occurring under closed and open canopies may explain trait change, which is not covered by species mean traits (Krah and Bässler, 2021). Second, fruit bodies may react to microclimate via biochemical rather than morphological traits (Krah et al., 2021), i.e., because responses need to happen rapidly and phenotypic changes may be too slow compared with biochemical responses such as heat shock proteins. Third, not only abiotic factors affect fruit body coloration along with environmental gradients. Further studies should thus also include biotic predictor variables, intraspecific trait variability and biochemical traits.
Finally, certain limitations arise from our data. First, we used presence/absence analyses because fruit body counts are not a good measure of abundances for fruit body-forming fungi since multiple fruit bodies can arise from a single or multiple mycelia. Thus individual-based abundances are not reliable when using fruit body counts. However, presence/absence analyses can be considered conservative in their interpretation. Second, we used vegetation cover as a proxy for the microclimatic conditions, an established proxy in the literature (Scharenbroch and Bockheim, 2007; Seibold et al., 2016; Krah et al., 2018; Müller et al., 2020; Thom et al., 2020; Vogel et al., 2020). However, more fine-scale measurements would allow a more detailed estimate of the microclimate, e.g., via data loggers. Manipulative canopy removal and the elevational gradient would further allow standardization of the microclimate (Krah et al., 2018). However, to account for additional effects of microclimate, we have included several explanatory variables in our model, which might influence the response of the fruiting community. Especially dead wood itself may influence the microclimate. Therefore, we added as covariates to the model, the number of host tree species, the sampled surface and the decay stage. We thus accounted for possible confounding effects of the host on microclimate. Only decay stage had a significant negative effect on the assemblage color lightness. Thus, with higher decay stage we found darker fruiting assemblages. Generally dead wood increases in water content over time (Pichler et al., 2012) and would thus be able to maintain cooler temperatures as compared with wood of lower water content. However, whether water content is the driving factor here remains speculative as other properties are also correlated to decay stage, e.g., C:N ratio or other organisms associated with wood decay (cf. Rajala et al., 2012; Pouska et al., 2016). Third, color lightness values were obtained by sampling representative images of fruit bodies for each species. This methodology of sampling color values using representative images has been used in previous studies (Zeuss et al., 2014; Heidrich et al., 2021). Concerning fungi, color values obtained through this approach have also been confirmed to accurately represent the average fruit body color (Krah et al., 2019). However, this approach is limited in the ecological interpretations of our results. Ideally the color lightness should be measured for each fruit body during the sampling process in the field which might better reflected trait variations in response to microclimate. Fourth, our data is limited to understanding the underlying processes shaping fruiting communities. Environmental treatments may or may not affect the mycelium community. If the fruiting community only reflects the changes of the mycelium community, we would expect a random response of the community fruit body lightness with the gradients. We include a null model in our analysis to test against the random response of fruit body traits. We found consistent results of the observed and standardized effect sizes with our treatment (Table 1) and thus conclude that our effects do likely not simply reflect mycelium shifts. Nevertheless, null models cannot replace parallel investigations of the mycelium and fruiting community to understand how climate affects the fungal organism.
Conclusion
In conclusion, we found significantly darker fungal fruit body assemblages in colder macroclimates, following predicted pattern of the thermal-melanism hypothesis (Bogert’s rule). However, microclimate did not significantly affect assemblage fruit body color lightness in our study. Our results thus add support for the thermal-melanism hypotheses which seems to be applicable for macro- and microorganisms, however, more research is needed on how microclimate affects macrofungal traits. Studies should investigate intraspecific trait variability, fecundity-color relationships, and biotic factors to understand coloration in fungi better.
Data Availability Statement
The original contributions presented in the study are included in the article/Supplementary Material, further inquiries can be directed to the corresponding author.
Author Contributions
F-SK had the initial idea of the study, conceptualized the analysis, and helped to write the manuscript. HO conducted statistical analysis and wrote the first draft of the manuscript. Both authors contributed to the article and approved the submitted version.
Funding
This research was supported by the Bavarian Forest National Park and the Bavarian State Ministry of the Environment and Consumer Protection.
Conflict of Interest
The authors declare that the research was conducted in the absence of any commercial or financial relationships that could be construed as a potential conflict of interest.
Publisher’s Note
All claims expressed in this article are solely those of the authors and do not necessarily represent those of their affiliated organizations, or those of the publisher, the editors and the reviewers. Any product that may be evaluated in this article, or claim that may be made by its manufacturer, is not guaranteed or endorsed by the publisher.
Acknowledgments
We thank Lothar Krieglsteiner, Peter Karasch, Andreas Gminder, and Frank Dämmrich for help with species identification. We further thank Jörg Müller, Roland Brandl, Linda Seifert, and Claus Bässler for initializing the BIOKLIM project and Claus Bässler for discussions.
Supplementary Material
The Supplementary Material for this article can be found online at: https://www.frontiersin.org/articles/10.3389/fevo.2022.829981/full#supplementary-material
Footnotes
- ^ fungi-without-borders.eu
- ^ 123pilze.de
- ^ mycokey.com
- ^ mykoweb.com
- ^ mushroomobserver.org
- ^ mushroomexpert.com
- ^ http://www.sttmedia.com
- ^ http://www.fungi-without-borders.eu
- ^ http://www.pilze-ammersee.de
- ^ https://www.mycoquebec.org
References
Albrecht, L. (1990). Grundlagen, Ziele und Methodik der waldökologischen Forschung in Naturwaldreservaten. Munich: Bayerisches Staatsministerium für Ernährung Landwirtschaft und Forsten.
Allen, J. A. (1877). The influence of physical conditions in the genesis of species. Radic. Rev. 1, 108–140.
Andrew, C., Heegaard, E., Halvorsen, R., Martinez-Peña, F., Egli, S., Kirk, P. M., et al. (2016). Climate impacts on fungal community and trait dynamics. Fungal Ecol. 22, 17–25. doi: 10.1016/j.funeco.2016.03.005
Bässler, C., Brandl, R., Müller, J., Krah, F. S., Reinelt, A., and Halbwachs, H. (2021). Global analysis reveals an environmentally driven latitudinal pattern in mushroom size across fungal species. Ecol. Lett. 24, 658–667. doi: 10.1111/ele.13678
Bässler, C., Förster, B., Moning, C., and Müller, J. (2009). The BIOKLIM project: biodiversity research between climate change and wilding in a temperate montane forest–The conceptual framework. Waldökol. Landschaftsforsch. Naturschutz 7, 21–34.
Bässler, C., Halbwachs, H., Karasch, P., Holzer, H., Gminder, A., Krieglsteiner, L., et al. (2016). Mean reproductive traits of fungal assemblages are correlated with resource availability. Ecol. Evol. 6, 582–592. doi: 10.1002/ece3.1911
Bässler, C., Seifert, L., and Müller, J. (2015b). The BIOKLIM Project in the National Park Bavarian Forest: lessons from a biodiversity survey. Silva Gabreta 21, 81–93.
Bässler, C., Heilmann-Clausen, J., Karasch, P., Brandl, R., and Halbwachs, H. (2015a). Ectomycorrhizal fungi have larger fruit bodies than saprotrophic fungi. Fungal Ecol. 17, 205–212. doi: 10.1016/j.funeco.2014.06.005
Bässler, C., Müller, J., and Dziock, F. (2010a). Detection of climate-sensitive zones and identification of climate change indicators: a case study from the bavarian forest national park. Folia Geobot. 45, 163–182. doi: 10.1007/s12224-010-9059-4
Bässler, C., Müller, J., Dziock, F., and Brandl, R. (2010b). Effects of resource availability and climate on the diversity of wood-decaying fungi. J. Ecol. 98, 822–832. doi: 10.1111/j.1365-2745.2010.01669.x
Becker, A., Klöcking, B., Lahmer, W., and Pfützner, B. (2002). “The hydrological modeling system ArcEGMO,” in Mathematical Models of Large Watershed Hydrology, ed. V. P. Singh (Littleton, CO: Water Resources Publications), 321–384.
Bergmann, C. (1847). Ueber die Verhältnisse der Wärmeökonomie der Thiere zu ihrer Grösse. Gott. Stud. 3, 595–708.
Bishop, T. R., Robertson, M. P., Gibb, H., van Rensburg, B. J., Braschler, B., Chown, S. L., et al. (2016). Ant assemblages have darker and larger members in cold environments. Glob. Ecol. Biogeogr. 25, 1489–1499. doi: 10.1111/geb.12516
Boehnke, D. (2021). Exploring the thermal microcosms at the forest floor—a case study of a temperate forest. Atmosphere 12:503. doi: 10.3390/atmos12040503
Bogert, C. M. (1949). Thermoregulation in reptiles, a factor in evolution. Evolution 3, 195–211. doi: 10.2307/2405558
Broennimann, O., Ursenbacher, S., Meyer, A., Golay, P., Monney, J.-C., Schmocker, H., et al. (2014). Influence of climate on the presence of colour polymorphism in two montane reptile species. Biol. Lett. 10:20140638. doi: 10.1098/rsbl.2014.0638
Cailleret, M., Heurich, M., and Bugmann, H. (2014). Reduction in browsing intensity may not compensate climate change effects on tree species composition in the bavarian forest national park. For. Ecol. Manage. 328, 179–192. doi: 10.1016/j.foreco.2014.05.030
Caro, T. (2017). Wallace on Coloration: contemporary perspective and unresolved insights. Trends Ecol. Evol. 32, 23–30. doi: 10.1016/j.tree.2016.10.003
Castella, B., Golay, J., Monney, J.-C., Golay, P., Mebert, K., and Dubey, S. (2013). Melanism, body condition and elevational distribution in the asp viper. J. Zool. 290, 273–280. doi: 10.1111/jzo.12037
Chown, S. L., and Gaston, K. J. (2016). Macrophysiology – progress and prospects. Funct. Ecol. 30, 330–344. doi: 10.1111/1365-2435.12510
Clusella-Trullas, S., Terblanche, J. S., Blackburn, T. M., and Chown, S. L. (2008). Testing the thermal melanism hypothesis: a macrophysiological approach. Funct. Ecol. 22, 232–238. doi: 10.1111/j.1365-2435.2007.01377.x
Cordero, R. J., and Casadevall, A. (2017). Functions of fungal melanin beyond virulence. Fungal Biol. Rev. 31, 99–112. doi: 10.1016/j.fbr.2016.12.003
Cordero, R. J. B., Robert, V., Cardinali, G., Arinze, E. S., Thon, S. M., and Casadevall, A. (2018). Impact of yeast pigmentation on heat capture and latitudinal distribution. Curr. Biol. 28, 2657–2664.e3. doi: 10.1016/j.cub.2018.06.034
Crous, P. W., Gams, W., Stalpers, J. A., Robert, V., and Stegehuis, G. (2004). MycoBank: an online initiative to launch mycology into the 21st century. Stud. Mycol. 50, 19–22.
Cuthill, I. C., Allen, W. L., Arbuckle, K., Caspers, B., Chaplin, G., Hauber, M. E., et al. (2017). The biology of color. Science 357:eaan0221. doi: 10.1126/science.aan0221
Delhey, K. (2019). A review of Gloger’s rule, an ecogeographical rule of colour: definitions, interpretations and evidence. Biol. Rev. 94, 1294–1316. doi: 10.1111/brv.12503
Dickey, J. R., Swenie, R. A., Turner, S. C., Winfrey, C. C., Yaffar, D., Padukone, A., et al. (2021). The utility of macroecological rules for microbial biogeography. Front. Ecol. Evol. 9:633155. doi: 10.3389/fevo.2021.633155
Diez, J., Kauserud, H., Andrew, C., Heegaard, E., Krisai-Greilhuber, I., Senn-Irlet, B., et al. (2020). Altitudinal upwards shifts in fungal fruiting in the Alps. Biol. Sci. 287:20192348. doi: 10.1098/rspb.2019.2348
Dormann, C. F., Elith, J., Bacher, S., Buchmann, C., Carl, G., Carré, G., et al. (2013). Collinearity: a review of methods to deal with it and a simulation study evaluating their performance. Ecography 36, 27–46. doi: 10.1111/j.1600-0587.2012.07348.x
Elling, W. (1987). Klima und Böden: Waldstandorte, 2nd Edn. Grafenau: Nationalparkverwaltung Bayerischer Wald.
Frenne, P., Lenoir, J., Luoto, M., Scheffers, B. R., Zellweger, F., Aalto, J., et al. (2021). Forest microclimates and climate change: importance, drivers and future research agenda. Glob. Change Biol. 27, 2279–2297. doi: 10.1111/gcb.15569
Frenne, P., Zellweger, F., Rodríguez-Sánchez, F., Scheffers, B. R., Hylander, K., Luoto, M., et al. (2019). Global buffering of temperatures under forest canopies. Nat. Ecol. Evol. 3, 744–749. doi: 10.1038/s41559-019-0842-1
Gotelli, N. J., and Entsminger, G. L. (2003). Swap algorithms in null model analysis. Ecology 84, 532–535. doi: 10.1890/0012-9658(2003)084[0532:sainma]2.0.co;2
Halbwachs, H., Simmel, J., and Bässler, C. (2016). Tales and mysteries of fungal fruiting: how morphological and physiological traits affect a pileate lifestyle. Fungal Biol. Rev. 30, 36–61. doi: 10.1016/j.fbr.2016.04.002
Heidrich, L., Friess, N., Fiedler, K., Brändle, M., Hausmann, A., Brandl, R., et al. (2018). The dark side of lepidoptera: colour lightness of geometrid moths decreases with increasing latitude. Glob. Ecol. Biogeogr. 27, 407–416. doi: 10.1111/geb.12703
Heidrich, L., Pinkert, S., Brandl, R., Bässler, C., Hacker, H., Roth, N., et al. (2021). Noctuid and geometrid moth assemblages show divergent elevational gradients in body size and color lightness. Ecography 44, 1169–1179. doi: 10.1111/ecog.05558
Hutchison, B. A., and Matt, D. R. (1977). The distribution of solar radiation within a deciduous forest. Ecol. Monogr. 47, 185–207. doi: 10.2307/1942616
Kembel, S. W., Cowan, P. D., Helmus, M. R., Cornwell, W. K., Morlon, H., Ackerly, D. D., et al. (2010). Picante: R tools for integrating phylogenies and ecology. Bioinformatics 26, 1463–1464. doi: 10.1093/bioinformatics/btq166
Knudsen, H., and Vesterholt, J. (eds) (2012). Funga Nordica: Agaricoid, Boletoid, Clavarois, Cyphelloid, and Gastroid. Copenhagen: Nordsvamp.
Krah, F.-S., and Bässler, C. (2021). What can intraspecific trait variability tell us about fungal communities and adaptations? Mycol. Prog. 20, 905–910. doi: 10.1007/s11557-021-01708-6
Krah, F.-S., Büntgen, U., Schaefer, H., Müller, J., Andrew, C., Boddy, L., et al. (2019). European mushroom assemblages are darker in cold climates. Nat. Commun. 10:2890. doi: 10.1038/s41467-019-10767-z
Krah, F.-S., Hess, J., Hennicke, F., Kar, R., and Bässler, C. (2021). Transcriptional response of mushrooms to artificial sun exposure. Ecol. Evol. 11, 10538–10546. doi: 10.1002/ece3.7862
Krah, F.-S., Seibold, S., Brandl, R., Baldrian, P., Müller, J., and Bässler, C. (2018). Independent effects of host and environment on the diversity of wood-inhabiting fungi. J. Ecol. 106, 1428–1442. doi: 10.1111/1365-2745.12939
Kües, U. (2000). Life history and developmental processes in the basidiomycete Coprinus cinereus. Microbiol. Mol. Biol. Rev. 64, 316–353. doi: 10.1128/MMBR.64.2.316-353.2000
Law, S. J., Bishop, T. R., Eggleton, P., Griffiths, H., Ashton, L., and Parr, C. (2020). Darker ants dominate the canopy: testing macroecological hypotheses for patterns in colour along a microclimatic gradient. J. Anim. Ecol. 89, 347–359. doi: 10.1111/1365-2656.13110
Lee, S. C., Ni, M., Li, W., Shertz, C., and Heitman, J. (2010). The evolution of sex: a perspective from the fungal kingdom. Microbiol. Mol. Biol. Rev. 74, 298–340. doi: 10.1128/MMBR.00005-10
Leibold, M. A., Holyoak, M., Mouquet, N., Amarasekare, P., Chase, J. M., Hoopes, M. F., et al. (2004). The metacommunity concept: a framework for multi-scale community ecology. Ecol. Lett. 7, 601–613. doi: 10.1111/j.1461-0248.2004.00608.x
Lindgren, J., Sjövall, P., Carney, R. M., Uvdal, P., Gren, J. A., Dyke, G., et al. (2014). Skin pigmentation provides evidence of convergent melanism in extinct marine reptiles. Nature 506, 484–488. doi: 10.1038/nature12899
Lindhe, A., Åsenblad, N., and Toresson, H.-G. (2004). Cut logs and high stumps of spruce, birch, aspen and oak – nine years of saproxylic fungi succession. Biol. Conserv. 119, 443–454. doi: 10.1016/j.biocon.2004.01.005
Lonsdale, D., Pautasso, M., and Holdenrieder, O. (2008). Wood-decaying fungi in the forest: conservation needs and management options. Eur. J. For. Res. 127, 1–22. doi: 10.1007/s10342-007-0182-6
MacArthur, R. H., and MacArthur, J. W. (1961). On bird species diversity. Ecology 42, 594–598. doi: 10.2307/1932254
McCabe, L. M., Cobb, N. S., and Butterfield, B. J. (2019). Environmental filtering of body size and darker coloration in pollinator communities indicate thermal restrictions on bees, but not flies, at high elevations. PeerJ 7:e7867. doi: 10.7717/peerj.7867
McGill, B. J., Enquist, B. J., Weiher, E., and Westoby, M. (2006). Rebuilding community ecology from functional traits. Trends Ecol. Evol. 21, 178–185. doi: 10.1016/j.tree.2006.02.002
Müller, J. Bässler, C. Strätz, C. Klöcking, B., and Brandl, R. (2009). Molluscs and climate warming in a low mountain range national park. Malacologia 51, 89–109. doi: 10.4002/040.051.0106
Müller, J., Ulyshen, M., Seibold, S., Cadotte, M., Chao, A., Bässler, C., et al. (2020). Primary determinants of communities in deadwood vary among taxa but are regionally consistent. Oikos 129, 1579–1588. doi: 10.1111/oik.07335
Norros, V., Karhu, E., Nordén, J., Vähätalo, A. V., and Ovaskainen, O. (2015). Spore sensitivity to sunlight and freezing can restrict dispersal in wood-decay fungi. Ecol. Evol. 5, 3312–3326. doi: 10.1002/ece3.1589
Peay, K. G., Schubert, M. G., Nguyen, N. H., and Bruns, T. D. (2012). Measuring ectomycorrhizal fungal dispersal: macroecological patterns driven by microscopic propagules. Mol. Ecol. 21, 4122–4136. doi: 10.1111/j.1365-294X.2012.05666.x
Pichler, V., Homolák, M., Skierucha, W., Pichlerová, M., Ramírez, D., Gregor, J., et al. (2012). Variability of moisture in coarse woody debris from several ecologically important tree species of the temperate zone of Europe. Ecohydrology 5, 424–434. doi: 10.1002/eco.235
Pinkert, S., and Zeuss, D. (2018). Thermal biology: melanin-based energy harvesting across the tree of life. Curr. Biol. 28, R887–R889. doi: 10.1016/j.cub.2018.07.026
Pouska, V., Macek, P., and Zíbarová, L. (2016). The relation of fungal communities to wood microclimate in a mountain spruce forest. Fungal Ecol. 21, 1–9. doi: 10.1016/j.funeco.2016.01.006
Pouska, V., Macek, P., Zíbarová, L., and Ostrow, H. (2017). How does the richness of wood-decaying fungi relate to wood microclimate? Fungal Ecol. 27, 178–181. doi: 10.1016/j.funeco.2016.06.006
Purhonen, J., Ovaskainen, O., Halme, P., Komonen, A., Huhtinen, S., Kotiranta, H., et al. (2020). Morphological traits predict host-tree specialization in wood-inhabiting fungal communities. Fungal Ecol. 46:100863. doi: 10.1016/j.funeco.2019.08.007
Rahbek, C. (2005). The role of spatial scale and the perception of large-scale species-richness patterns. Ecol. Lett. 8, 224–239. doi: 10.1111/j.1461-0248.2004.00701.x
Rajala, T., Peltoniemi, M., Pennanen, T., and Mäkipää, R. (2012). Fungal community dynamics in relation to substrate quality of decaying Norway spruce (Picea abies L Karst.) logs in boreal forests. FEMS Microbiol. Ecol. 81, 494–505. doi: 10.1111/j.1574-6941.2012.01376.x
Reifsnyder, W. E., Furnival, G. M., and Horowitz, J. L. (1971). Spatial and temporal distribution of solar radiation beneath forest canopies. Agric. Meteorol. 9, 21–37. doi: 10.1016/0002-1571(71)90004-5
Rensch, B. (1929). Das Prinzip Geographischer Rassenkreise und das Problem der Artbildung. Berlin: Gebruder Borntraeger.
Sánchez-García, M., Ryberg, M., Khan, F. K., Varga, T., Nagy, L. G., and Hibbett, D. S. (2020). Fruiting body form, not nutritional mode, is the major driver of diversification in mushroom-forming fungi. Proc. Natl. Acad. Sci. U.S.A. 117, 32528–32534. doi: 10.1073/pnas.1922539117
Scharenbroch, B. C., and Bockheim, J. G. (2007). Impacts of forest gaps on soil properties and processes in old growth northern hardwood-hemlock forests. Plant Soil 294, 219–233. doi: 10.1007/s11104-007-9248-y
Schelhaas, M.-J., Nabuurs, G.-J., and Schuck, A. (2003). Natural disturbances in the European forests in the 19th and 20th centuries. Glob. Chang Biol. 9, 1620–1633. doi: 10.1046/j.1365-2486.2003.00684.x
Seibold, S., Bässler, C., Brandl, R., Büche, B., Szallies, A., Thorn, S., et al. (2016). Microclimate and habitat heterogeneity as the major drivers of beetle diversity in dead wood. J. Appl. Ecol. 53, 934–943. doi: 10.1111/1365-2664.12607
Seidl, R., Schelhaas, M.-J., Rammer, W., and Verkerk, P. J. (2014). Increasing forest disturbances in Europe and their impact on carbon storage. Nat. Clim. Change 4, 806–810. doi: 10.1038/nclimate2318
Souza, A. R., Mayorquin, A. Z., and Sarmiento, C. E. (2020). Paper wasps are darker at high elevation. J. Therm. Biol. 89:102535. doi: 10.1016/j.jtherbio.2020.102535
Stelbrink, P., Pinkert, S., Brunzel, S., Kerr, J., Wheat, C. W., Brandl, R., et al. (2019). Colour lightness of butterfly assemblages across North America and Europe. Sci. Rep. 9:1760. doi: 10.1038/s41598-018-36761-x
Stokland, J. N., and Sippola, A.-L. (2004). Monitoring protocol for wood-inhabiting fungi in the Alberta Biodiversity Monitoring Program. Available online at: https://ftp-public.abmi.ca/home/publications/documents/336_Stokland_etal_2004_SuggestedProtocolsForFungiMonitoring_ABMI.pdf (accessed May 21, 2021).
Tedersoo, L., Bahram, M., Põlme, S., Kõljalg, U., Yorou, N. S., Wijesundera, R., et al. (2014a). Fungal biogeography. global diversity and geography of soil fungi. Science 346:1256688. doi: 10.1126/science.1256688
Tedersoo, L., Bahram, M., Ryberg, M., Otsing, E., Kõljalg, U., and Abarenkov, K. (2014b). Global biogeography of the ectomycorrhizal/sebacina lineage (Fungi, Sebacinales) as revealed from comparative phylogenetic analyses. Mol. Ecol. 23, 4168–4183. doi: 10.1111/mec.12849
Thom, D., Sommerfeld, A., Sebald, J., Hagge, J., Müller, J., and Seidl, R. (2020). Effects of disturbance patterns and deadwood on the microclimate in European beech forests. Agric. For. Meteorol. 291:108066. doi: 10.1016/j.agrformet.2020.108066
Tóth, B. B., and Feest, A. (2007). A simple method to assess macrofungal sporocarp biomass for investigating ecological change. Can. J. Bot. 85, 652–658. doi: 10.1139/B07-068
van den Broek, E. L., and van Rikxoort, E. M. (2004). “Evaluation of color representation for texture analysis,” in Proceedings of the 16th Belgium–Netherlands Conference on Artificial Intelligence, Groningen, 51–58.
Vellend, M. (2010). Conceptual synthesis in community ecology. Q. Rev. Biol. 85, 183–206. doi: 10.1086/652373
Vìtrovskı, T., Kohout, P., Kopeckı, M., Machac, A., Man, M., Bahnmann, B. D., et al. (2019). A meta-analysis of global fungal distribution reveals climate-driven patterns. Nat. Commun. 10:5142. doi: 10.1038/s41467-019-13164-8
Vogel, S., Gossner, M. M., Mergner, U., Müller, J., and Thorn, S. (2020). Optimizing enrichment of deadwood for biodiversity by varying sun exposure and tree species: an experimental approach. J. Appl. Ecol. 57, 2075–2085. doi: 10.1111/1365-2664.13648
Yin, H., Shi, Q., Shakeel, M., Kuang, J., and Li, J. (2016). the environmental plasticity of diverse body color caused by extremely long photoperiods and high temperature in Saccharosydne procerus (Homoptera: Delphacidae). Front. Physiol. 7:401. doi: 10.3389/fphys.2016.00401
Zellweger, F., Frenne, P., Lenoir, J., Vangansbeke, P., Verheyen, K., Bernhardt-Römermann, M., et al. (2020). Forest microclimate dynamics drive plant responses to warming. Science 368, 772–775. doi: 10.1126/science.aba6880
Keywords: color, community mean, climate, traits, thermal-melanism hypothesis, Bogert’s rule
Citation: Oechler H and Krah F-S (2022) Response of Fruit Body Assemblage Color Lightness to Macroclimate and Vegetation Cover. Front. Ecol. Evol. 10:829981. doi: 10.3389/fevo.2022.829981
Received: 06 December 2021; Accepted: 04 February 2022;
Published: 24 February 2022.
Edited by:
Mario R. Moura, Federal University of Paraíba, BrazilReviewed by:
Sylvain Pincebourde, UMR7261 Institut de Recherche sur la Biologie de l’Insecte (IRBI), FranceMari Jönsson, Swedish University of Agricultural Sciences, Sweden
Copyright © 2022 Oechler and Krah. This is an open-access article distributed under the terms of the Creative Commons Attribution License (CC BY). The use, distribution or reproduction in other forums is permitted, provided the original author(s) and the copyright owner(s) are credited and that the original publication in this journal is cited, in accordance with accepted academic practice. No use, distribution or reproduction is permitted which does not comply with these terms.
*Correspondence: Franz-Sebastian Krah, a3JhaEBiaW8udW5pLWZyYW5rZnVydC5kZQ==; orcid.org/0000-0001-7866-7508