- 1Lolldaiga Hills Research Programme, Nanyuki, Kenya
- 2Sustainability Research Institute, School of Earth and Environment, University of Leeds, Leeds, United Kingdom
- 3Space for Giants, Cape Chestnut, Nanyuki, Kenya
- 4National Zoo and Smithsonian Conservation Biology Institute, Conservation Ecology Center, Front Royal, VA, United States
- 5Mpala Research Centre, Nanyuki, Kenya
- 6Department of Natural Resources, Karatina University, Karatina, Kenya
- 7Department of Natural Resources, Egerton University, Egerton, Kenya
- 8USDA–ARS Rangeland Resources Research Unit, Fort Collins, CO, United States
- 9Department of Environment and Geography, University of York, York, United Kingdom
- 10Department of Plant Sciences and Ecology Graduate Group, University of California, Davis, Davis, CA, United States
Over a quarter of the world’s land surface is grazed by cattle and other livestock, which are replacing wild herbivores and widely regarded as drivers of global biodiversity declines. The effects of livestock presence versus absence on wild herbivores are well documented. However, the environmental context-specific effects of cattle stocking rate on biodiversity and livestock production are poorly understood, precluding nuanced rangeland management recommendations. To address this, we used a long term exclosure experiment in a semi-arid savanna ecosystem in central Kenya that selectively excludes cattle (at different stocking rates), wild mesoherbivores, and megaherbivores. We investigated the individual and interactive effects of cattle stocking rate (zero/moderate/high) and megaherbivore (>1,000 kg) accessibility on habitat use (measured as dung density) by two dominant wild mesoherbivores (50–1,000 kg; zebra Equus quagga and eland Taurotragus oryx) across the “wet” and “dry” seasons. To explore potential tradeoffs or co-benefits between cattle production and wildlife conservation, we tested for individual and interactive effects of cattle stocking rate and accessibility by wild mesoherbivores and megaherbivores (collectively, large wild herbivores) on the foraging efficiency of cattle across both seasons. Eland habitat use was reduced by cattle at moderate and high stocking rates across both dry and wet seasons and regardless of megaherbivore accessibility. We observed a positive effect of megaherbivores on zebra habitat use at moderate, but not high, stocking rates. Cattle foraging efficiency (g dry matter step–1 min–1) was lower in the high compared to moderate stocking rate treatments during the dry season, and was non-additively reduced by wild mesoherbivores and high cattle stocking rates during the wet season. These results show that high stocking rates are detrimental to wild mesoherbivore habitat use and cattle foraging efficiency, while reducing to moderate stocking rates can benefit zebra habitat use and cattle foraging efficiency. Our findings demonstrate that ecosystem management and restoration efforts across African rangelands that involve reducing cattle stocking rates may represent a win-win for wild herbivore conservation and individual performance of livestock.
Introduction
Over a quarter of the world’s land surface is grazed by cattle and other livestock (Asner et al., 2004), which now comprise 60% of mammalian biomass globally (Bar-On et al., 2018), and underpin the livelihoods of millions of people. This livestock grazing is having large impacts on global biodiversity (Reid et al., 2014). Considering that the current network of protected areas is insufficient in size and too fragmented to ensure the future of many large herbivore species (Craigie et al., 2010), a better understanding of how wildlife can coexist with people and their livestock outside of protected areas is critical for ensuring the socio-ecological integrity of global rangelands. This is particularly true of arid and semi-arid regions of Africa (Tyrrell et al., 2017), which are home to the most diverse and threatened large mammal communities (Ceballos et al., 2015). In these regions, cattle, sheep and goats account for >90% of herbivore biomass (Hempson et al., 2017), and livestock are the primary source of food, livelihoods, and cultural value for local communities (Homewood, 2009).
A global review has indicated that livestock and wildlife interactions are generally negative (Schieltz and Rubenstein, 2016). Livestock can impact wild herbivore populations mainly via indirect exploitation competition and, to a smaller extent, direct interference competition (Loft et al., 1991; Stewart et al., 2002; Madhusudan, 2004). Exploitation competition occurs due to alteration to forage quantity and/or quality (Ranglack et al., 2015; Kimuyu et al., 2017; Keesing et al., 2018), fire regimes and vegetation structure (Kimuyu et al., 2014; Hempson et al., 2017; Odadi et al., 2017a), water availability (Connolly et al., 2021), predation risk (Ng’weno et al., 2019), and/or disease transmission (Keesing et al., 2018). However, moderate levels of grazing by livestock and wildlife can also improve pasture quality and facilitate coexistence in certain agro-ecological and climatic contexts (Brown et al., 2010; Odadi et al., 2011; McLaren et al., 2018; Young et al., 2018).
The spectrum from competitive to facilitative interactions in herbivore communities may depend on the temporal dynamics of resource availability (Veblen, 2008). In a Kenyan savanna, for instance, cattle and wild herbivores compete for forage during the dry season, but facilitation can occur during wet periods when grazing promotes the growth of higher quality grass (Odadi et al., 2011). The competition and facilitation processes can be mediated by the presence or absence of megaherbivores (>1,000 kg). There is evidence that elephants Loxodonta africana can dampen the negative effects of cattle on plains zebras Equus quagga (Kimuyu et al., 2017), by competing with cattle for forbs (Odadi et al., 2009, 2013; Coverdale et al., 2016) and facilitating zebras by altering the cover of grasses and woody vegetation (Wells et al., 2021a). Forbs can play a particularly important role in herbivore interactions because they are an important source of protein (Odadi et al., 2013).
A limitation to our current understanding of the interactions between livestock and wild herbivores is that virtually all evidence comes from simply comparing livestock to non-livestock conditions (Briske et al., 2011; Schieltz and Rubenstein, 2016). A gradient of livestock stocking rate may govern competition and facilitation with wild herbivores across seasons. The ecosystem effects along this gradient are poorly understood and, in African rangelands, may be mediated by the presence of megaherbivores. Moreover, how wildlife and season mediate the intraspecific competition among cattle at different stocking rates is also poorly understood. In this experimental study, we sought to fill this knowledge gap within the context of an African savanna rangeland in central Kenya. Specifically, we investigated: (i) the individual and interactive effects of cattle stocking rate (zero/moderate/high) and megaherbivore accessibility (presence/absence) on habitat use (measured as dung density) by the two dominant wild mesoherbivores (50–1,000 kg; zebra Equus quagga and eland Taurotragus oryx), across “wet” and “dry” seasons, and (ii) the underlying individual and interactive effects of cattle stocking rate and accessibility by wild mesoherbivores and megaherbivores (collectively, large wild herbivores) on the foraging efficiency of cattle across the wet and dry seasons. Cattle may compete more with eland (which, like cattle, are ruminants) than with zebra, which are non-ruminants. Indeed, previous work has shown that cattle at moderate stocking rates supress eland more so than zebra (Kimuyu et al., 2017). We therefore predicted that eland will be more negatively affected by increasing cattle stocking rates than zebra.
Materials and Methods
Study Site
We conducted this study in the Kenya Long-term Exclosure Experiment (KLEE) at Mpala Research Centre (0°17′N, 36°52′E, 1,800 m.a.s.l.) in Laikipia, Kenya. Mpala Research Centre is managed for both wildlife conservation and livestock production, where cattle are the main domestic animal. Rainfall at KLEE is weakly trimodal with a pronounced dry season December-March. From 2001 to 2019, annual rainfall averaged 613 mm yr–1 (range: 421–1,009 mm yr–1, interannual coefficient of variation: 27%). Soils are poorly drained vertisols with high clay content (>40%) known as “black cotton.” Black cotton soils are widespread across Africa and with other vertisols cover >100 million hectares across the continent (Ahmad, 1996). The overstory of this savanna ecosystem is dominated by Acacia drepanolobium (syn. Vachellia drepanolobium, 97% of the canopy; Young et al., 1997), while five perennial grass species (Brachiaria lachnantha, Themeda triandra, Pennisetum stramineum, P. mezianum, and Lintonia nutans) comprise 85% of herbaceous understory cover at KLEE (Porensky et al., 2013). The above ground net primary productivity (ANPP) of the study site is comparable to the productivity of Serengeti grasslands with comparable rainfall (Sala et al., 2012) and somewhat higher than herbaceous ANPP estimates from an adjacent, less productive soil type (Augustine and McNaughton, 2006). Wild herbivore species at KLEE include: plains zebra, eland, oryx (Oryx beisa), hartebeest (Alcelaphus buselaphus), buffalo (Syncerus caffer), Grant’s gazelle (Nanger granti), Grevy’s zebra (E. grevyi), common duiker (Sylvicapra grimmia), steenbok (Raphicerus campestris), elephant (Loxodonta africana), and giraffe (Giraffa camelopardalis).
Experimental Design
The KLEE plots were established in 1995 and use barriers to control access to 200 m2 × 200 m2 (4-ha) treatment plots by three herbivore types – wild mesoherbivores (50–1,000 kg), wild megaherbivores (>1,000 kg; elephant and giraffe), and cattle – in different combinations. KLEE consists of three replicate blocks of six herbivore treatments: (1) accessible only to cattle; (2) accessible only to wild mesoherbivores; (3) accessible only to wild mesoherbivores and megaherbivores; (4) accessible only to cattle and wild mesoherbivores; (5) accessible to cattle, wild mesoherbivores, and megaherbivores; and (6) excluding cattle, wild mesoherbivores, and megaherbivores. Here, we excluded the final treatment because it was not relevant to addressing our research questions. The treatment plots accessible to cattle are typically grazed by 100–120 mature Boran cows Bos indicus (sometimes with calves and/or bulls) for 2–3 days (2 hrs day–1) within a 2-week period, 3–4 times per year (i.e., moderate stocking rate). The timing and number of grazing days depends on forage availability and reflects typical grazing regimes of private ranches in the region, wherein cattle graze in an area for several days before being moved to allow that area to recover.
The three treatments accessible to cattle each contain a 50 m2 × 50 m2 (0.25-ha) subplot (established in 2008), in which the same cattle herd is grazed for a further 30 min following the initial 2-h grazing period in the wider plot, to achieve an approximately fourfold increase in cattle stocking rate compared to the wider plot (Supplementary Figures 1, 2). The five herbivore treatments plus the three high cattle stocking rate treatments make a total of eight treatments. For the five main treatments, we selected one of four 50 m2 × 50 m2 subplots within the central hectare of each of the fifteen 4-ha treatment plots for wildlife monitoring. In plots accessible to cattle the subplot closest to the high cattle stocking rate subplots was selected to be more comparable with the moderate cattle stocking rate plots, while subplots were randomly selected in plots excluding cattle (experimental layout illustrated in Supplementary Figure 2).
Because cattle only access individual plots a few times per year, responses of wildlife are unlikely to be due to direct interaction with cattle or herders. Fire has not been used as a management tool in this ecosystem for over 50 years and is rarely used by other ranches in the region. Natural-ignition fires have not occurred in decades. See Young et al. (1997) and Young et al. (2018) for further details of the experimental design.
Habitat Use by Wild and Domestic Herbivores
To assess habitat use by wild and domestic herbivores we conducted four dung surveys. Two surveys were conducted during the wet seasons of 2019 and 2020 and two during the dry seasons of 2020 and 2021. Dung surveys are a robust metric of habitat use by wild herbivores (Kimuyu et al., 2017; Wells et al., 2021a). In each survey, we counted dung along six 4 m2 × 40 m2 belt transects in all 24 0.25-ha subplots (three replicates of eight treatments). We crushed recorded dung to prevent recounting in subsequent surveys. We also assessed megaherbivore dung densities, because a change in megaherbivore habitat use between treatments may influence the effect of the megaherbivore-accessible treatment. Because we used dung densities as a metric of species-specific relative habitat use, we avoid issues relating to estimating population densities from dung, the decay rates of which vary across species and seasons (Nchanji and Plumptre, 2001; Rivero et al., 2004).
We modeled treatment effects on wild mesoherbivore habitat use by employing Gaussian linear mixed-effect models (LMMs) using glmmTMB package version 1.0.1 (Brooks et al., 2017) in R version 3.6.3 (R Core Team, 2020). To evaluate the treatment effects on wild mesoherbivore habitat use we restricted our analysis to the two most common species, zebra and eland, the combined dung of which comprised >80% of the total wild mesoherbivore dung recorded. All other wild mesoherbivore species were data limited, being recorded in fewer than two-thirds of treatments and each species accounting for <6% of total wild mesoherbivore dung. We pooled dung piles across the two temporal replicates within each season by calculating species-specific dung density per survey for each treatment plot for wet and dry seasons. This avoids issues of temporal autocorrelation associated with repeated measurements. To evaluate the individual and interactive effects of cattle stocking rate and megaherbivores on wild mesoherbivore habitat use, we coded cattle (none/moderate/high), megaherbivores (accessible/inaccessible) and the interaction between them as the fixed effect and coded block as a random effect. To evaluate the effects of cattle stocking rate on megaherbivores we coded cattle (none/moderate/high) as the fixed effect and coded block as a random effect. Due to the restricted sample size, we fit separate models for wet and dry seasons. We visually checked the normality and homoscedasticity of the residuals. We performed Tukey’s post hoc tests for treatment comparisons using the emmeans package version 1.7 (Lenth et al., 2021).
Cattle Foraging Behaviour
To assess cattle foraging behaviour we followed Odadi et al. (2017a). We monitored the frequency, size, and plant species composition of bites, as well as step frequency in all 18 subplots accessible to cattle (three replicates of six treatments). For each trial we randomly selected five steers as test animals to use in all experimental plots. The order in which the treatment plots were surveyed was randomised, such that on each sampling day one randomly selected main treatment subplot and its corresponding high cattle stocking rate subplot were sampled. The animals were allowed a 7-day adjustment period prior to each trial, during which they became accustomed to being herded together and observed by researchers at close range. To evaluate seasonal variation we conducted surveys during one “wet” (May 2020) and one “dry” (February 2021) period, selecting a different group of steers for each season. The ten steers (five animals in both wet and dry periods) aged 30–40 months and averaged 311 kg (±15 SE).
Bite and step frequencies were recorded twice in each treatment subplot. On each sampling day, the five steers were moved to a designated experimental plot (containing one moderate and one high cattle stocking rate subplot) at 08:00–09:00 hours and removed 1–2 hours later. The steers were allowed approximately 10 minutes to settle prior to observations at the start of each day. While in the experimental plot, the steers were herded in the two subplots sequentially. To avoid biases associated with time of day, this was repeated in the same sequence such that we observed foraging behaviour in both subplots twice during the same morning. Whether the moderate or high cattle stocking rate subplot was visited first was randomly determined. While in each subplot, we observed each animal for 2-min periods, during which all bites taken and steps moved were recorded on two separate tally counters. A “bite” represents removal of a part of a plant or the whole plant, while a “step” represents the forward displacement of either front limb. Bites and steps were recorded when the focal animals were actively foraging. Focal animals were considered to be actively foraging when searching for food or eating appeared to be a primary priority. On the rare occasion that an animal being observed did not eat or move during the entire focal period, bites and steps were recorded as zero. All observations were made at a distance of less than 4 m from the focal animal, by two experienced observers. Observer identity had no detectable effect on foraging efficiency estimates in either the wet season (Z = −0.74, p = 0.46) or the dry season (Z = 0.45, p = 0.65). In total, we recorded 720 min of foraging behaviour (5 animals, each observed for two 2-min periods in 18 subplots during two seasons). For further details see Odadi et al. (2017a).
To evaluate cattle foraging efficiency, we estimated bite size in each subplot for each focal animal between the first and second rounds of bite and step counts. We followed the focal animal while it took five consecutive bites, picking a handful of plants that represented the amount consumed by the animal during each bite. We combined the plant matter collected for all five animals (totalling 25 bites) and dried it in a dessicator, weighing it every day until it reached a stabilised dry mass. In total, we recorded 50 bites (five bites from each of the 5 animals during two seasons).
We modelled treatment effects on cattle foraging behaviour by implementing Gaussian linear mixed-effects models (LMMs) using glmmTMB package version 1.0.1 (Brooks et al., 2017) in R version 3.6.3 (R Core Team, 2020). We calculated foraging efficiency as: bite size × bites × time–1 × steps–1. To evaluate the individual and interactive effects of cattle stocking rate and large wild herbivores on cattle foraging efficiency, we coded as fixed effects cattle (moderate/high), wild mesoherbivores (accessible/inaccessible), megaherbivores (accessible/inaccessible), the interaction between cattle and wild mesoherbivores and the interaction between cattle and megaherbivores, while coding block as a random effect. Again, we fit separate models for wet and dry seasons, due to the restricted sample size. We visually checked the normality and homoscedasticity of the residuals and tested for treatment comparisons using Tukey’s post hoc test.
Results
Cattle at High Stocking Rates Suppress Dominant Mesoherbivore Habitat Use
For both dry and wet seasons, and regardless of megaherbivore accessibility, eland habitat use was similarly reduced by cattle at both moderate (dry: −66%, Z = −3.8, p < 0.001; wet: −66%, Z = −4.9, p < 0.001) and high stocking rates (dry: −69%, Z = −5.6, p < 0.001; wet: −65%, Z = −4.9, p < 0.001; Figure 1).
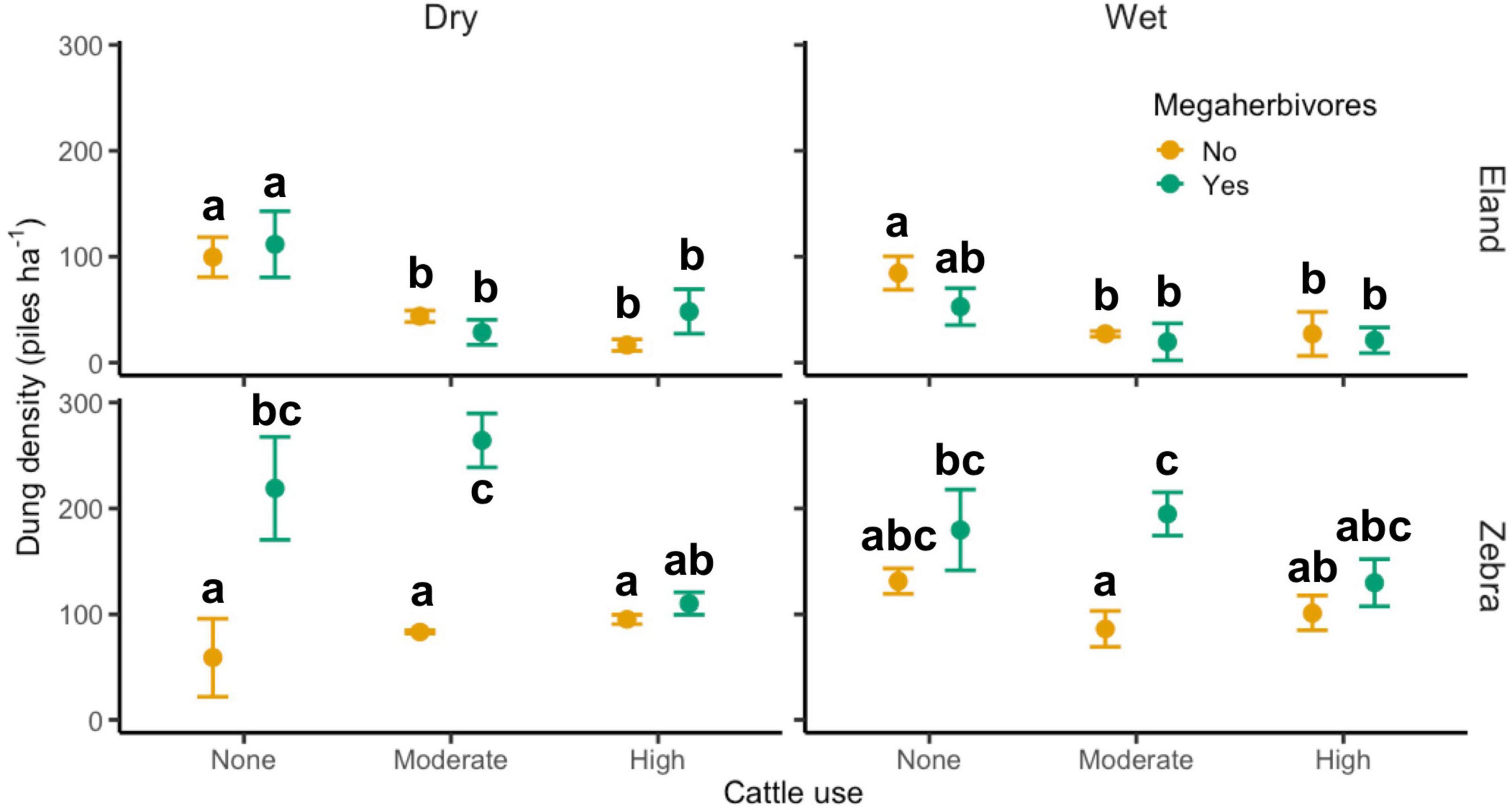
Figure 1. Treatment effects on the habitat use of the two dominant wild mesoherbivore species (M ± 1 SE). Within mesoherbivore species, treatments not sharing letters are significantly different (p < 0.05) based on Tukey’s post hoc tests. The three cattle stocking rates equate to: 0, ∼2, and ∼10 kg hr m– 2 yr– 1.
During the dry season, high cattle stocking rates reduced zebra habitat use in megaherbivore-accessible plots, but not in megaherbivore-excluding plots (megaherbivore × cattle interaction, high: Z = −3.2, p = 0.001; see Tukey’s test results in Figure 1). However, this effect was not observed at moderate cattle stocking rates (p = 0.64). There was a positive effect of megaherbivores on zebra habitat use at moderate cattle stocking rates (Z = 5.1, p < 0.001), which was negated at high stocking rates. We observed broadly similar patterns during the wet season, although not statistically significant (megaherbivore x cattle interaction, moderate: Z = 1.6, p = 0.10, high: Z = −0.5, p = 0.60).
Cattle at moderate and high stocking rates significantly reduced elephant habitat use during the dry season (moderate: −35%, Z = −2.5, p = 0.01; high: −35%, Z = −2.5, p = 0.01; but see Tukey’s test results in Figure 2), but not during the wet season (moderate: 8%, Z = 0.2, p = 0.86; high: −42%, Z = −1.0, p = 0.33; Figure 2). Giraffe habitat use was unaffected by cattle stocking rate in both seasons (all p-values > 0.15).
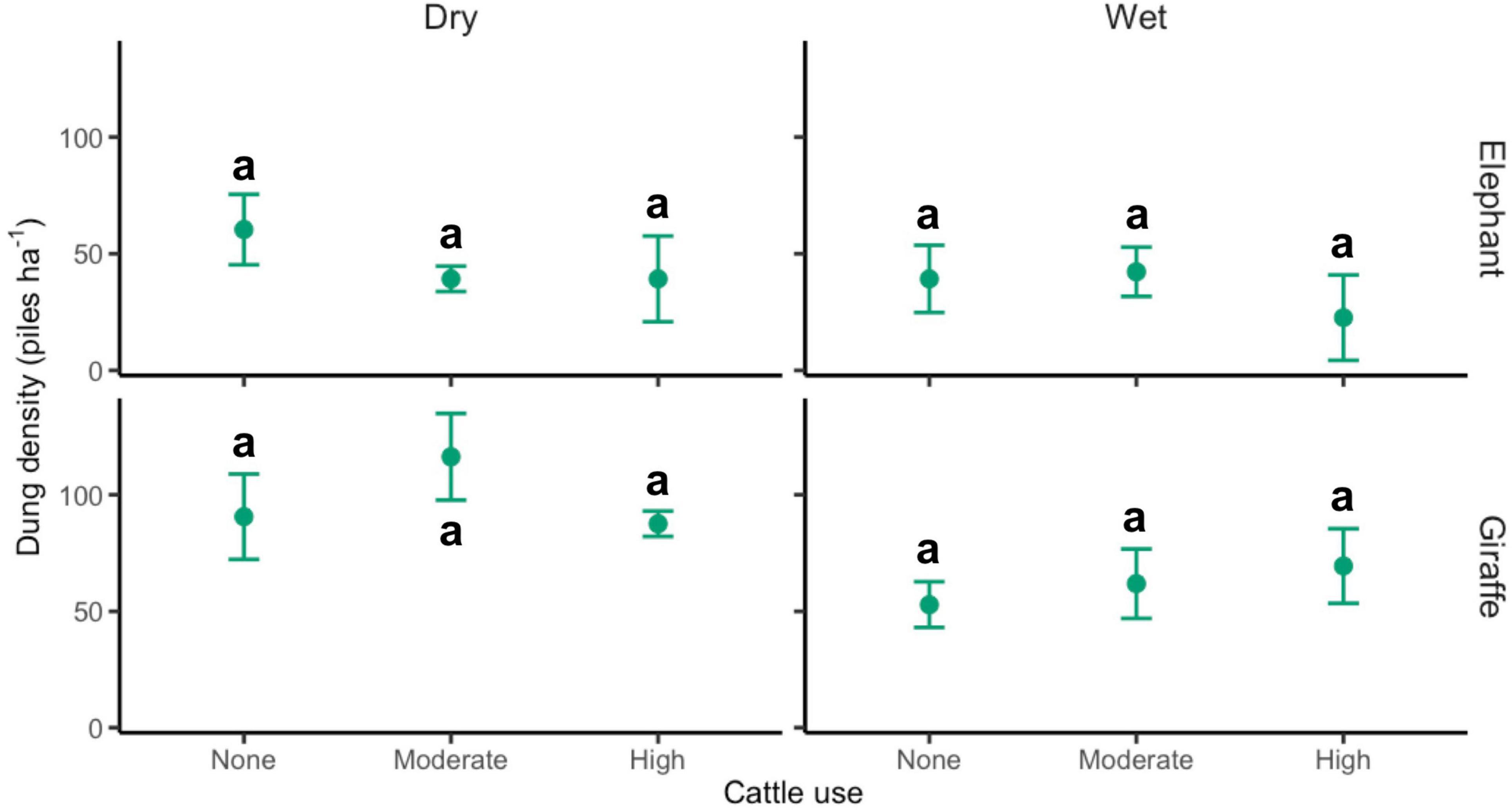
Figure 2. Effects of cattle stocking rate on wild megaherbivore habitat use (M ± 1 SE). Within megaherbivore species, treatments not sharing letters are significantly different (p < 0.05) based on Tukey’s post hoc tests.
Cattle at High Stocking Rates Reduce Cattle Foraging Efficiency, Particularly in the Dry Season
During the dry season, cattle foraging efficiency (dry g step–1 min–1) was 66% lower under high compared to moderate cattle stocking rates (Z =−4.5, p < 0.001), except in plots accessible to megaherbivores (megaherbivore x cattle interaction, moderate: Z = 1.9, p = 0.06, high: Z = −0.2, p = 0.83; see Tukey’s test results in Figure 3). During the wet season, however, cattle foraging efficiency was lower under high compared to moderate cattle stocking rates only in plots accessible to wild mesoherbivores but excluding megaherbivores (megaherbivore x cattle interaction, moderate: Z = 1.4, p = 0.15, high: Z = −3.0, p = 0.002; see Tukey’s test results in Figure 3).
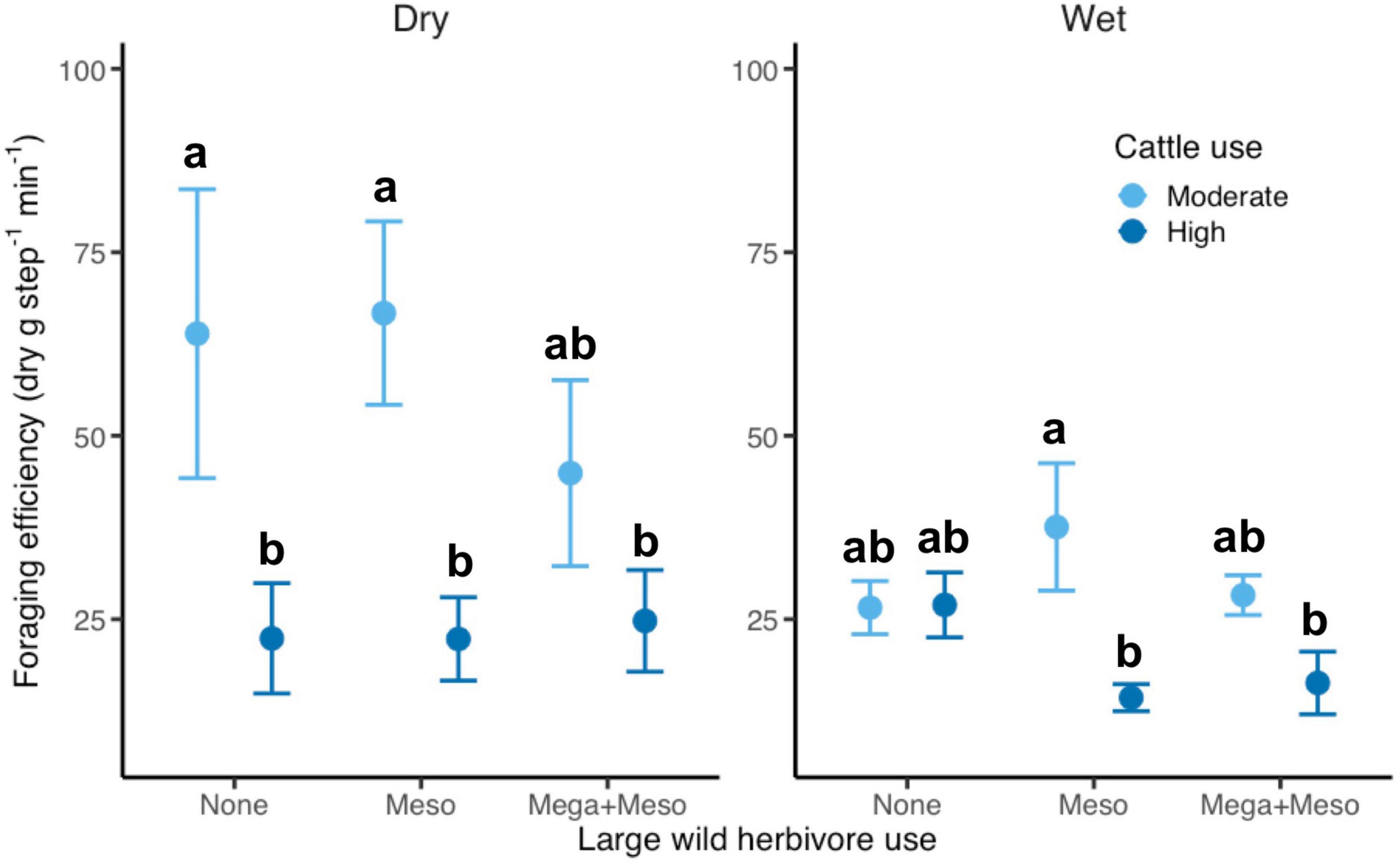
Figure 3. Treatment effects on cattle foraging efficiency across seasons (M ± 1 SE). Within each season, treatments not sharing letters are significantly different (p < 0.05) based on Tukey’s post hoc tests.
Discussion
The effects of cattle stocking rate on wild herbivores remains poorly understood, in part because most studies simply compare cattle presence versus absence (Briske et al., 2011; Schieltz and Rubenstein, 2016). As yet, the potential tradeoffs or co-benefits between livestock production and wildlife conservation are rarely investigated. Our results show that, overall, high cattle stocking rates were detrimental to both wild mesoherbivore habitat use and cattle foraging efficiency. We also found that megaherbivores increase zebra habitat use, but that this effect is negated at high cattle stocking rates. These findings are important to guide ecosystem management and restoration efforts across African rangelands by providing novel insights into the tradeoffs between, and potential win-wins for, cattle production and wild herbivore conservation.
Wild Mesoherbivore Habitat Use Responses to Cattle Stocking Rate
Our experimental results demonstrate that cattle at both moderate and high stocking rates suppress habitat use by eland, while zebra appeared to be more responsive to megaherbivores than to cattle. The negative effect of cattle on eland corroborates the largely negative effects of livestock presence versus absence on wild herbivores reported in other rangelands globally (Keesing and Young, 2014; Schieltz and Rubenstein, 2016). The strong negative response of eland to cattle stocking rates may be, in part, due to competition over forbs. Forbs are a vital source of protein for cattle (Odadi et al., 2013) and are heavily relied upon by eland (68% forbs in diet; Kartzinel and Pringle, 2020), while rarely consumed by zebra (2% forbs in diet; Kartzinel et al., 2019; Kartzinel and Pringle, 2020).
Our results confirm that zebras are facilitated by megaherbivores in this system (Kimuyu et al., 2017; Wells et al., 2021a), due to tree density reductions (Guldemond and Van Aarde, 2008) and/or facilitation of grasses (Coverdale et al., 2016). However, we show that this facilitation effect is negated at high cattle stocking rates, expressed by a sharp reduction on zebra habitat use, particularly during the dry season. This builds on previous research suggesting that megaherbivores temper the negative effects of cattle at moderate stocking rates on zebras (Young et al., 2005; Kimuyu et al., 2017). Moreover, previous studies in this system suggest that the increasing grass cover by megaherbivores that facilitates zebra, is also a result of the former reducing cattle foraging efficiency. It has been suggested that this is due to the reduction of forb cover by elephants, which translates to cattle spending more time moving in search of patches with forbs (Odadi et al., 2009, 2013). Our results suggest that zebra habitat use is reduced at high cattle stocking rates in megaherbivore-accessible plots, because the heavy grazing (mostly by cattle) overwhelms the positive effects that reduced cattle foraging efficiency (by megaherbivores and intraspecific competition with other cattle) has on grass cover.
The diversity in body sizes and feeding strategies of wild savanna herbivores precludes confident generalisations from the responses of zebra and eland to other wild mesoherbivores. However, other equids, such as Grevy’s zebra Equus grevyi, as well as predominantly grazing bovids, such as hartebeest Alcelaphus buselaphus (89% grass in diet; Kartzinel and Pringle, 2020), may respond in similar ways to zebras. Likewise, the responses of eland may reflect those of species with comparable feeding strategies, such as Grant’s gazelle Nanger granti and impala Aepyceros melampus (Kartzinel et al., 2019; Kartzinel and Pringle, 2020). Cattle grazing may also facilitate smaller-bodied short grass specialists, such as Thomson’s gazelle Eudorcas thomsoni and warthog Phacochoerus africanus (Bhola et al., 2012; Ogutu et al., 2014; Crego et al., 2020; Wells et al., 2021a).
Our results provide further evidence that species interactions can be complex and mediated by different factors, such as season and changes in vegetation structure due to megaherbivores (Odadi et al., 2009; Kimuyu et al., 2017). Further experimental research in other grassland systems testing different stocking rates can help elucidate the nature of livestock-wildlife interactions, which livestock producers generally assume to be competitive, despite the lack of evidence (Ranglack et al., 2015; Traba et al., 2017). Such information can be important to find novel management strategies that can promote coexistence between wild and domestic species (Pozo et al., 2021).
Cattle Foraging Efficiency Responses to Cattle Stocking Rate
Increasing cattle stocking rate had a greater negative impact on cattle foraging efficiency (dry g step–1 min–1) than did the presence of large wild herbivores, particularly in the dry season. This suggests that high cattle stocking rates (at greater numbers than large wild herbivores) increase intraspecific competition among cattle during drier periods and is a more important factor in reducing foraging efficiency than interspecific competition. The stronger influence of cattle stocking rate on cattle foraging efficiency, compared to season and large wild herbivore accessibility, highlights the importance of stocking rates in determining individual performance of domestic animals noted in other rangelands (Briske et al., 2003; Smart et al., 2010; Fang et al., 2014; Raynor et al., 2021).
Among the large wild herbivores, megaherbivores had a stronger effect on cattle foraging efficiency than mesoherbivores, possibly due to more intense competition over protein-rich forbs (Odadi et al., 2009, 2013). The effects of cattle stocking rate and megaherbivores on cattle foraging efficiency were absent or weaker during the wet season (Figure 3). This may be because grass and forb availability is less limited, as evidenced by differences between treatments being smaller due to grazing-induced compensatory plant growth (McNaughton et al., 1983; Charles et al., 2017; Wells et al., 2021b). However, high cattle stocking rates and wild mesoherbivores suppressed cattle foraging efficiency more than additively in the wet season (Figure 3). This statistically significant interaction suggests that there is a threshold number of animals (domestic and wild) foraging in a plot at which the herbivory-enhanced plant productivity is reversed and competition between herbivores intensifies. The trend toward facilitative effects of wild mesoherbivores on cattle performance at moderate stocking rates, although lacking strong statistical support (Figure 3), mirrors results of previous research in this system (Odadi et al., 2011). Crucially, our results show that this facilitation shifts to competition at high cattle stocking rates. The interactive effect of cattle and wild mesoherbivores was not observed in the dry season, possibly because the suppression of cattle foraging efficiency is dominated by the negative effects of cattle stocking rate and, to a lesser extent, megaherbivores.
Implications for Wildlife Conservation and Cattle Management in Rangelands
In African rangelands, pastoral societies have shared livestock foraging areas and migratory routes with wildlife for thousands of years and domestic animals remain embedded in the cultural identity and livelihoods of many pastoral communities today (Homewood, 2009; Fynn et al., 2016). Historically, wildlife co-existed in high numbers alongside pastoral communities and still do under certain conditions today (Tyrrell et al., 2017; Russell et al., 2018; Crego et al., 2020; Kiffner et al., 2020), but this relationship has deteriorated in recent decades (Reid et al., 2008; Løvschal et al., 2019). Reduced pastoralist mobility and increased livestock numbers has led to continuous grazing over the same areas, which, at high stocking rates, can result in land degradation that is detrimental to both livestock production and wildlife populations (Western et al., 2009). Our results confirm that higher stocking rates negatively affect large wild herbivores. Results also show that in the presence of megaherbivores, lowering cattle stocking rate to moderate can benefit wild mesoherbivores by alleviating interspecific competition. Moderate stocking rates were also shown to improve cattle foraging efficiency by mitigating intraspecific competition. This opens the possibility for a win-win scenario for both people and wildlife across Africa. However, herd-level cattle productivity can increase with cattle stocking rate, even while individual-level performance declines (Smart et al., 2010; Fang et al., 2014; Raynor et al., 2021). This would lead to a tradeoff between herd-level cattle productivity and wildlife conservation that requires further investigation.
Stocking rate is an important aspect of livestock grazing practices (Briske et al., 2003), but there are other factors that may influence the effects of domestic animals on native wild herbivores, such as the timing, duration, and intensity of grazing, as well as herd composition (Schieltz and Rubenstein, 2016; Odadi et al., 2017b). One of the limitations of our study was that we only investigated one rotational grazing regime at different stocking rates. Although this is representative of privately managed ranches in the areas surrounding the study site, it contrasts the largely continuous grazing in many communally managed rangelands that is linked to reduced mobility (Letai and Lind, 2013). However, our results may be representative of certain communally managed rangelands, where rotational grazing practices are becoming increasingly common (e.g., Odadi et al., 2017b). Comparisons of different grazing regimes while maintaining constant overall stocking rates would provide insights into how rangeland managers can adapt grazing practices to minimize competition with native wild herbivores (Augustine et al., 2020).
Because our study focused on cattle, it may be challenging to draw inference from our results to rangeland systems with other domestic species with differing foraging characteristics. Similar research on other domestic livestock species with different diets will be critical to complement our study, as sheep, goats, and camels are becoming more prevalent in rangelands across Africa (Ogutu et al., 2016; Løvschal et al., 2019; Volpato and King, 2019) and other continents (Bainbridge, 2007). It will also be important to investigate how observed climatic changes (Schmocker et al., 2016) will affect interactions between cattle and wild herbivores due to variations in vegetation productivity.
Data Availability Statement
The raw data supporting the conclusions of this article will be made available by the authors, without undue reservation.
Author Contributions
HW, LS, AD, WO, DK, and TY conceived and designed the methodology. HW, JE, and MN collected the data. HW and RC analysed the data and led the writing of the manuscript. All authors contributed critically to drafts and approved publication.
Funding
The KLEE plots were built and maintained by grants from the James Smithson Fund of the Smithsonian Institution, The National Geographic Society (Grants 4691-91, 9106-12, and 9986-16), and the National Science Foundation (LTREB DEB 97-07477, 03-16402, 08-16453, 12-56004, 12-56034, and 19-31224). Funding for field equipment was received by HW from The Rufford Foundation (Grant 27451-1) and the University of Leeds Centre for Climate’s Climate Research Bursary Award. Additional financial support was provided through the Leeds Doctoral Scholarship to HW.
Conflict of Interest
The authors declare that the research was conducted in the absence of any commercial or financial relationships that could be construed as a potential conflict of interest.
Publisher’s Note
All claims expressed in this article are solely those of the authors and do not necessarily represent those of their affiliated organizations, or those of the publisher, the editors and the reviewers. Any product that may be evaluated in this article, or claim that may be made by its manufacturer, is not guaranteed or endorsed by the publisher.
Acknowledgments
We thank Mpala Research Centre and its staff for logistical support. This research complies with the current laws of the country in which it was conducted (Kenya).
Supplementary Material
The Supplementary Material for this article can be found online at: https://www.frontiersin.org/articles/10.3389/fevo.2022.825689/full#supplementary-material
References
Ahmad, N. (1996). Occurrence and distribution of vertisols. Dev. Soil Sci. 24, 1–41. doi: 10.1016/S0166-2481(96)80003-1
Asner, G. P., Elmore, A. J., Olander, L. P., Martin, R. E., and Harris, A. T. (2004). Grazing systems, ecosystem responses, and global change. Ann. Rev. Environ. Resour. 29, 261–299. doi: 10.1146/annurev.energy.29.062403.102142
Augustine, D. J., Derner, D. J., Fernández-Giménez, M. E., Porensky, L. M., Wilmer, H., and Briske, D. (2020). Adaptive, multipaddock rotational grazing management: a ranch-scale assessment of effects on vegetation and livestock performance in semiarid rangeland. Rangel. Ecol. Manag. 73, 796–810. doi: 10.1016/j.rama.2020.07.005
Augustine, D. J., and McNaughton, S. J. (2006). Interactive effects of ungulate herbivores, soil fertility, and variable rainfall on ecosystem processes in a semi-arid savanna. Ecosystems 9, 1242–1256. doi: 10.1007/s10021-005-0020-y
Bainbridge, D. A. (2007). A Guide for Desert & Dryland Restoration: New Hope for Arid Lands. Washington, DC: Island Press, 391.
Bar-On, Y. M., Phillips, R., and Milo, R. (2018). The biomass distribution on earth. Proc. Natl. Acad. Sci. U.S.A. 115, 6506–6511. doi: 10.1073/pnas.1711842115
Bhola, N., Ogutu, J. O., Piepho, H.-P., Said, M. Y., Reid, R. S., Hobbs, N. T., et al. (2012). Comparative changes in density and demography of large herbivores in the Masai Mara Reserve and its surrounding human-dominated pastoral ranches in Kenya. Biodivers. Conserv. 21, 1509–1530. doi: 10.1007/s10531-012-0261-y
Briske, D. D., Derner, J. D., Milchunas, D. G., and Tate, K. W. (2011). “An evidence-based assessment of prescribed grazing practices,” in Conservation Benefits of Rangeland Practices: Assessment, Recommendations and Knowledge gaps, ed. D. D. Briske (Washington, DC: Natural Resources Conservation Service), 21–47.
Briske, D. D., Fuhlendorf, S. D., and Smeins, F. E. (2003). Vegetation dynamics on rangelands: a critique of the current paradigms. J. Appl. Ecol. 40, 601–614. doi: 10.1046/j.1365-2664.2003.00837.x
Brooks, M. E., Kristensen, K., van Bentham, K. J., Magnusson, A., Berg, C. W., Nielsen, A., et al. (2017). glmmTMB balances speed and flexibility among packages for zero-inflated generalized linear mixed. R J. 9, 378–400. doi: 10.32614/rj-2017-066
Brown, N. A., Ruckstuhl, K. E., Donelon, S., and Corbett, C. (2010). Changes in vigilance, grazing behaviour and spatial distribution of bighorn sheep due to cattle presence in Sheep River Provincial Park, Alberta. Agric. Ecosyst. Environ. 135, 226–231. doi: 10.1016/j.agee.2009.10.001
Ceballos, G., Ehrlich, P. R., Barnosky, A. D., García, A., Pringle, R. M., and Palmer, T. M. (2015). Accelerated modern human–induced species losses: entering the sixth mass extinction. Sci. Adv. 1:e1400253. doi: 10.1126/sciadv.1400253
Charles, G. K., Porensky, L. M., Riginos, C., Veblen, K. E., and Young, T. P. (2017). Herbivore effects on productivity vary by guild: cattle increase mean productivity while wildlife reduce variability. Ecol. Appl. 27, 143–155. doi: 10.1002/eap.1422
Connolly, E., Allan, J., Brehony, P., Aduda, A., Western, G., Russell, S., et al. (2021). Coexistence in an African pastoral landscape: evidence that livestock and wildlife temporally partition water resources. Afr. J. Ecol. 59, 696–711. doi: 10.1111/aje.12869
Coverdale, T. C., Kartzinel, T. R., Grabowski, K. L., Shriver, R. K., Hassan, A. A., Goheen, J. R., et al. (2016). Elephants in the understory: opposing direct and indirect effects of consumption and ecosystem engineering by megaherbivores. Ecology 97, 3219–3230. doi: 10.1002/ecy.1557
Craigie, I. D., Baillie, J. E. M., Balmford, A., Carbone, C., Collen, B., Green, R. E., et al. (2010). Large mammal population declines in Africa’s protected areas. Biol. Conserv. 143, 2221–2228. doi: 10.1016/j.biocon.2010.06.007
Crego, R. D., Ogutu, J. O., Wells, H. B. M., Ojwang, G. O., Martins, D. J., Leimgruber, P., et al. (2020). Spatiotemporal dynamics of wild herbivore dpecies richness and occupancy across a savannah rangeland: implications for conservation. Biol. Conserv. 242:108436. doi: 10.1016/j.biocon.2020.108436
Fang, Q. X., Andales, A. A., Derner, J. D., Ahuja, L. R., Ma, L., Bartling, P. N. S., et al. (2014). Modeling weather and stocking rate effects on forage and steer production in northern mixed-grass prairie’. Agric. Syst. 129, 103–114. doi: 10.1016/j.agsy.2014.05.011
Fynn, R. W. S., Augustine, D. J., and Peel, M. J. S. de Garine-Wichatitsky, M. (2016). Strategic management of livestock to improve biodiversity conservation in African savannahs: a conceptual basis for wildlife-livestock coexistence. J. Appl. Ecol. 53, 388–397. doi: 10.1111/1365-2664.12591
Guldemond, R., and Van Aarde, R. (2008). A meta-analysis of the impact of African elephants on savanna vegetation. J. Wildl. Manag. 72, 892–899. doi: 10.2193/2007-072
Hempson, G. P., Archibald, S., and Bond, W. J. (2017). The consequences of replacing wildlife with livestock in Africa. Sci. Rep. 7:17196. doi: 10.1038/s41598-017-17348-4
Homewood, K. (2009). Ecology of African Pastoral Societies. Oxford: Oxford and the Ohio University Press, 320.
Kartzinel, T. R., Hsing, J. C., Musili, P. M., Brown, B. R. P., and Pringle, R. M. (2019). Covariation of diet and gut microbiome in African megafauna. Proc. Natl. Acad. Sci. U.S.A. 116, 23588–23593. doi: 10.1073/pnas.1905666116
Kartzinel, T. R., and Pringle, R. M. (2020). Multiple dimensions of dietary diversity in large mammalian herbivores. J. Anim. Ecol. 89, 1482–1496. doi: 10.1111/1365-2656.13206
Keesing, F., Ostfeld, R. S., Okanga, S., Huckett, S., Bayles, B. R., Chaplin-Kramer, R., et al. (2018). Consequences of integrating livestock and wildlife in an African savanna. Nat. Sustain. 1, 566–573. doi: 10.1038/s41893-018-0149-2
Keesing, F., and Young, T. P. (2014). Cascading consequences of the loss of large mammals in an African savanna. Bioscience 64, 487–495. doi: 10.1093/biosci/biu059
Kiffner, C., Kioko, J., Baylis, J., Beckwith, C., Brunner, C., Burns, C., et al. (2020). Long-term persistence of wildlife populations in a pastoral area. Ecol. Evol. 10, 10000–10016. doi: 10.1002/ece3.6658
Kimuyu, D. M., Sensenig, R. L., Riginos, C., Veblen, K. E., and Young, T. P. (2014). Native and domestic browsers and grazers reduce fuels, fire temperatures, and Acacia ant mortality in an African savanna. Ecol. Appl. 24, 741–749. doi: 10.1890/13-1135.1
Kimuyu, D. M., Veblen, K. E., Riginos, C., Chira, R. M., Githaiga, J. M., and Young, T. P. (2017). Influence of cattle on browsing and grazing wildlife varies with rainfall and presence of megaherbivores. Ecol. Appl. 27, 786–798. doi: 10.1002/eap.1482
Lenth, R. V., Buerkner, P., Herve, M., Love, J., Riebl, H., and Singmann, H. (2021). Package ‘emmeans’ https://cran.microsoft.com/snapshot/2019-08-15/web/packages/emmeans/index.html.
Loft, E. R., Menke, J. W., and Kie, J. G. (1991). Habitat shifts by mule deer: the influence of cattle grazing. J. Wildl. Manag. 55, 16–26. doi: 10.2307/3809236
Letai, J., and Lind, J. (2013). “Squeezed from all sides: changing resource tenure and pas- toralist innovation on the Laikipia plateau, Kenya,” in Pastoralism and Development in Africa: Dynamic Change at the Margins, eds A. Catley, J. Lind, and I. Scoones (London: Routledge), 164–176.
Løvschal, M., Håkonsson, D. D., and Amoke, I. (2019). Are goats the new elephants in the room? Changing land-use strategies in Greater Mara, Kenya. Land Use Policy 80, 395–399. doi: 10.1016/j.landusepol.2018.04.029
Madhusudan, M. D. (2004). Recovery of wild large herbivores following livestock decline in a tropical Indian wildlife reserve. J. Appl. Ecol. 41, 858–869. doi: 10.1111/j.0021-8901.2004.00950.x
McLaren, B. E., MacNearney, D., and Siavichay, C. A. (2018). Livestock and the functional habitat of vicuñas in Ecuador: a new puzzle. Ecosphere 9:e02066. doi: 10.1002/ecs2.2066
McNaughton, S. J., Wallace, L. L., and Coughenour, M. B. (1983). Plant adaptation in an ecosystem context: effects of defoliation, Nitrogen, and water on growth of an African C4 sedge. Ecology 64, 307–318. doi: 10.2307/1937078
Nchanji, A. C., and Plumptre, A. J. (2001). Seasonality in elephant dung decay and implications for censusing and population monitoring in South-Western Cameroon. Afr. J. Ecol. 39, 24–32.
Ng’weno, C. C., Buskirk, S. W., Georgiadis, N. J., Gituku, B. C., Kibungei, A. K., Porensky, L. M., et al. (2019). Apparent competition, lion predation, and managed livestock grazing: can conservation value be enhanced? Front. Ecol. Evol. 7:123. doi: 10.3389/fevo.2019.00123
Odadi, W. O., Fargione, J., and Rubenstein, D. I. (2017a). Vegetation, wildlife, and livestock responses to planned grazing management in an African pastoral landscape: planned grazing enhances pastoral rangeland productivity. Land Degrad. Dev. 28, 2030–2038. doi: 10.1002/ldr.2725
Odadi, W. O., Karachi, M. K., Abdulrazak, S. A., and Young, T. P. (2011). African wild ungulates compete with or facilitate cattle depending on season. Science 333, 1753–1755. doi: 10.1126/science.1208468
Odadi, W. O., Karachi, M. K., Abdulrazak, S. A., and Young, T. P. (2013). Protein supplementation reduces non-grass foraging by a primary grazer. Ecol. Appl. 23, 455–463. doi: 10.1890/12-0878.1
Odadi, W. O., Kimuyu, D. M., Sensenig, R. L., Veblen, K. E., Riginos, C., and Young, T. P. (2017b). Fire-induced negative nutritional outcomes for cattle when sharing habitat with native ungulates in an African savanna. J. Appl. Ecol. 54, 935–944. doi: 10.1111/1365-2664.12785
Odadi, W. O., Okeyo-Owuor, J. B., and Young, T. P. (2009). Behavioural responses of cattle to shared foraging with wild herbivores in an East African rangeland. Appl. Anim. Behav. Sci. 116, 120–125. doi: 10.1016/j.applanim.2008.08.010
Ogutu, J. O., Piepho, H.-P., Said, M. Y., Ojwang, G. O., Njino, L. W., Kifugo, S. C., et al. (2016). Extreme wildlife declines and concurrent increase in livestock numbers in Kenya: what are the causes? PLoS One 11:e0163249. doi: 10.1371/journal.pone.0163249
Ogutu, J. O., Reid, R. S., Piepho, H.-P., Hobbs, N. T., Rainy, M. E., Krushka, R. S., et al. (2014). Large herbivore responses to surface water and land use in an East African savanna: implications for conservation and human-wildlife conflicts. Biodivers. Conserv. 23, 573–596. doi: 10.1007/s10531-013-0617-y
Porensky, L. M., Wittman, S. E., Riginos, C., and Young, T. P. (2013). Herbivory and drought interact to enhance spatial patterning and diversity in a savanna understory. Oecologia 173, 591–602. doi: 10.1007/s00442-013-2637-4
Pozo, R. A., Cusack, J. J., Acebes, P., Malo, J. E., Traba, J., Iranzo, E. C., et al. (2021). Reconciling livestock production and wild herbivore conservation: challenges and opportunities. Trends Ecol. Evol. 36, 750–761. doi: 10.1016/j.tree.2021.05.002
R Core Team (2020). R: A Language and Environment for Statistical Computing. Vienna: R Foundation for Statistical Computing.
Ranglack, D. H., Durham, S., and du Toit, J. T. (2015). Competition on the range: science vs. perception in a bison–cattle conflict in the Western USA. J. Appl. Ecol. 52, 467–474. doi: 10.1111/1365-2664.12386
Raynor, E. J., Derner, J. D., Baldwin, T., Ritten, J. P., and Augustine, D. A. (2021). Multidecadal directional shift in shortgrass stocking rates. Rangel. Ecol. Manag. 74, 72–80. doi: 10.1016/j.rama.2020.09.005
Reid, R. S., Fernández-Giménez, M. E., and Galvin, K. A. (2014). Dynamics and resilience of rangelands and pastoral peoples around the globe. Annu. Rev. Environ. Resour. 39, 217–242. doi: 10.1146/annurev-environ-020713-163329
Reid, R. S., Galvin, K. A., and Kruska, R. S. (2008). “Global significance of extensive grazing lands and pastoral societies: an introduction,” in Fragmentation in Semi-Arid and Arid Landscapes: Consequences for Human and Natural Systems, eds K. A. Galvin, R. S. Reid, R. H. Behnke Jr., and N. T. Hobbs (London: Springer), 1–24. doi: 10.1007/978-1-4020-4906-4_1
Rivero, K., Rumiz, D. I., and Taber, A. B. (2004). Estimating brocket deer (Mazama Gouazoubira and M. americana) abundance by dung pellet counts and other indices in seasonal Chiquitano forest habitats of Santa Cruz, Bolivia. Eur. J. Wildl. Res. 50, 161–167. doi: 10.1007/s10344-004-0064-x
Russell, S., Tyrrell, P., and Western, D. (2018). Seasonal interactions of pastoralists and wildlife in relation to pasture in an African savanna ecosystem. J. Arid Environ. 154, 70–81. doi: 10.1016/j.jaridenv.2018.03.007
Sala, O. E., Gherardi, L. A., Reichmann, L., Jobbaìgy, E., and Peters, D. (2012). Legacies of precipitation fluctuations on primary production: theory and data synthesis. Philos. Trans. R. Soc. B 367, 3135–3144. doi: 10.1098/rstb.2011.0347
Schieltz, J. M., and Rubenstein, D. I. (2016). Evidence based review: positive versus negative effects of livestock grazing on wildlife. What do we really know? Environ. Res. Lett. 11:113003. doi: 10.1088/1748-9326/11/11/113003
Schmocker, J., Liniger, H. P., Ngeru, J. N., Brugnara, Y., Auchmann, R., and Brönnimann, S. (2016). Trends in mean and extreme precipitation in the Mount Kenya region from observations and reanalyses. Int. J. Climatol. 36, 1500–1514. doi: 10.1002/joc.4438
Smart, A. J., Derner, J. D., Hendrickson, J. R., Gillen, R. L., Dunn, B. H., Mousel, E. M., et al. (2010). Effects of grazing pressure on efficiency of grazing on North American Great Plains rangelands. Rangel. Ecol. Manag. 63, 397–406. doi: 10.2111/REM-D-09-00046.1
Stewart, K. M., Bowyer, R. T., Kie, J. G., Cimon, N. J., and Johnson, B. K. (2002). Temporal distributions of elk, mule deer, and cattle: resource partitioning and competitive displacement. J. Mammal. 83, 229–244. doi: 10.1644/1545-15422002083<0229:TDOEMD<2.0.CO;2
Traba, J., Iranzo, E. C., Carmona, C. P., and Malo, J. E. (2017). Realised niche changes in a native herbivore assemblage associated with presence of livestock. Oikos 126, 1400–1409. doi: 10.1111/oik.04066
Tyrrell, P., Russell, S., and Western, D. (2017). Seasonal movements of wildlife and livestock in a heterogenous pastoral landscape: implications for coexistence and community based conservation. Glob. Ecol. Conserv. 12, 59–72. doi: 10.1016/j.gecco.2017.08.006
Veblen, K. E. (2008). Season and herbivore-dependent competition and facilitation in a semiarid savanna. Ecology 89, 1532–1540. doi: 10.1890/07-0973.1
Volpato, G., and King, E. G. (2019). From cattle to camels: trajectories of livelihood adaptation and social-ecological resilience in a Kenyan pastoralist community. Reg. Environ. Change 19, 849–865. doi: 10.1007/s10113-018-1438-z
Wells, H. B. M., Crego, R. D., Opedal, ØH., Khasoha, L. M., Alston, J. M., Reed, C. G., et al. (2021a). Experimental evidence that effects of megaherbivores on mesoherbivore space use are influenced by species’ traits. J. Anim. Ecol. 90, 2510–2522. doi: 10.1111/1365-2656.13565
Wells, H. B. M., Kimuyu, D. M., Odadi, W. O., Dougill, A. J., Stringer, L. C., and Young, T. P. (2021b). Wild and domestic savanna herbivores increase smaller vertebrate diversity, but less than additively. J. Appl. Ecol. 58, 953–963. doi: 10.1111/1365-2664.13843
Western, D., Groom, R., and Worden, J. (2009). The impact of subdivision and sedentarisation of pastoral lands on wildlife in an African savanna ecosystem. Biol. Conserv. 142, 2538–2546. doi: 10.1016/j.biocon.2009.05.025
Young, T. P., Okello, B. D., Kinyua, D., and Palmer, T. M. (1997). KLEE: a long-term multi-species herbivore exclusion experiment in Laikipia, Kenya. Afr. J. Range For. Sci. 14, 94–102. doi: 10.1080/10220119.1997.9647929
Young, T. P., Palmer, T. M., and Gadd, M. E. (2005). Competition and compensation among cattle, zebras, and elephants in a semi-arid savanna in Laikipia, Kenya. Biol. Conserv. 122, 351–359. doi: 10.1016/j.biocon.2004.08.007
Keywords: elephant, competition, facilitation, foraging efficiency, livestock-wildlife interactions, rangeland, savanna
Citation: Wells HBM, Crego RD, Ekadeli J, Namoni M, Kimuyu DM, Odadi WO, Porensky LM, Dougill AJ, Stringer LC and Young TP (2022) Less Is More: Lowering Cattle Stocking Rates Enhances Wild Herbivore Habitat Use and Cattle Foraging Efficiency. Front. Ecol. Evol. 10:825689. doi: 10.3389/fevo.2022.825689
Received: 30 November 2021; Accepted: 13 January 2022;
Published: 11 February 2022.
Edited by:
Karsten Wesche, Senckenberg Museum of Natural History Görlitz, GermanyReviewed by:
Teowdroes Kassahun Teka, Crop Research Institute, CzechiaFrancesca Parrini, University of the Witwatersrand, South Africa
Copyright © 2022 Wells, Crego, Ekadeli, Namoni, Kimuyu, Odadi, Porensky, Dougill, Stringer and Young. This is an open-access article distributed under the terms of the Creative Commons Attribution License (CC BY). The use, distribution or reproduction in other forums is permitted, provided the original author(s) and the copyright owner(s) are credited and that the original publication in this journal is cited, in accordance with accepted academic practice. No use, distribution or reproduction is permitted which does not comply with these terms.
*Correspondence: Harry B. M. Wells, harrybmwells@gmail.com