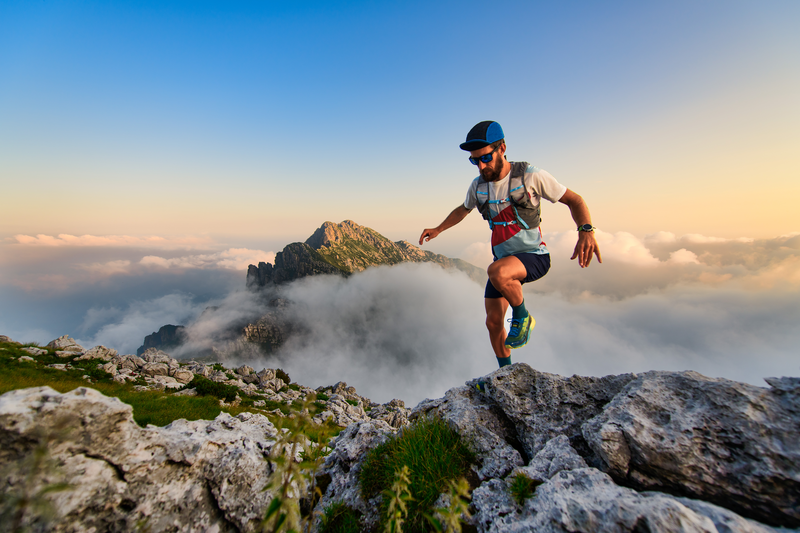
95% of researchers rate our articles as excellent or good
Learn more about the work of our research integrity team to safeguard the quality of each article we publish.
Find out more
ORIGINAL RESEARCH article
Front. Ecol. Evol. , 24 March 2022
Sec. Behavioral and Evolutionary Ecology
Volume 10 - 2022 | https://doi.org/10.3389/fevo.2022.824474
Pollinators are facing declines at a global level. One of the main factors driving this decline is insufficient access to floral resources due to habitat loss and degradation that can affect both diet generalist species as well as those with more restricted floral preferences. Here we evaluated the effect of a novel mitigation strategy in agricultural ecosystems, Farming with Alternative Pollinators (FAP) on the pollen diet of crop pollinators. The approach dedicates 25% of the cropped area to Marketable Habitat Enhancement Plants (MHEP) that attract pollinators, natural enemies of the crops, and provide farmers with income. We assessed the effect of the approach on pollen diet of faba bean (Vicia faba) and pumpkin (Cucurbita maxima) flower visitors in four different regions in Morocco during 2018 and 2019 by comparing control fields (monoculture) and FAP fields in 13 trials and 101 sites. Results from 25 wild bee species show that almost two-thirds of the species carrying or collecting pollen when visiting pumpkin flowers and half of the species carrying or collecting pollen when visiting faba bean flowers gathered this pollen from two or more host plants (i.e., MHEP, main crop, and/or wild plants) and displayed a wide dietary breadth. Pollen grains from the main crops were poorly represented on the female scopae, indicating that crops were mainly visited for nectar. Hence, crop flower visitors may require alternative pollen sources to meet their nutritional needs. The number of pollen genera collected by flower visitors and the dietary breadth of crop flower visitors did not show a significant increase in response to FAP management. Among the selected MHEP, sunflower (Helianthus annuus) was the pollen resource for pumpkin flower visitors. In faba bean, flower visitors collected pollen from coriander (Coriandrum sativum) and canola (Brassica napus). Our study sheds light on the importance of characterizing the pollen diet and the foraging behavior of crop pollinators to identify appropriate plant species that complement their food, maintain and conserve their populations.
Pollinators provide vital and free ecosystem services that benefit human well-being, including the pollination of 75% of the most important global food crops (Klein et al., 2007). However, both wild and domesticated pollinators are showing global declines (Goulson, 2019). Several anthropogenic and environmental stressors are driving pollinator decline, including agricultural intensification, invasive species (Aizen et al., 2009, 2019; Gill et al., 2016; Duchenne et al., 2020), climate change (Gérard et al., 2020; Martinet et al., 2020) and habitat loss and fragmentation (Potts et al., 2010).
Scarcity in floral resources due to habitat loss and agricultural intensification is negatively affecting generalist and specialist pollinators, but to varying extents (Scheper et al., 2014). Some pollinator groups with narrow dietary breadth have shown a greater relative decline (Kleijn and Raemakers, 2008; Wood et al., 2019), as they might struggle to switch to other alternative plants when their main host plants are scarce. In contrast, pollinators with wide dietary breadth display a higher stability in response to these external pressures (Chacoff et al., 2018), as they interact potentially with a greater number of species of the local plant community and can shift on the expanding ones (Martín González et al., 2010; Roger et al., 2017). However, lack of diversity in floral resources may restrict their dietary breadth, and thus induce nutritional shortage in quality and quantity (Carvell et al., 2006; Goulson et al., 2015; Vaudo et al., 2020).
Managed flower-rich habitats have been one of the most commonly suggested mitigation strategies applied to curb pollinator decline in intensively managed agro-ecosystems (Grass et al., 2016; IPBES, 2016; Albrecht et al., 2020). Flower strips support diverse pollinating species (Haaland et al., 2011; Albrecht et al., 2020) and have been found to provide a majority of the pollen and nectar collected by visiting species in experimental studies (Ouvrard et al., 2018). Furthermore, several studies have demonstrated an increase in pollinator abundance and species richness in crops adjacent to nearby flower strips (Blaauw and Isaacs, 2014; Feltham et al., 2015). However, wild-flower strips are not self-sustaining and farmers dislike WFS even if they gain financial compensation (Kleijn et al., 2019), and their effectiveness at supporting sustainably pollinator diversity has been reconsidered (Klein et al., 2003; Kleijn et al., 2019) due to the high implementation costs (Batáry et al., 2015). Indeed, pollinator-protection schemes should be economically self-sustaining and feasible for all nations (Christmann, 2020).
Farming with Alternative Pollinators (FAP) (Christmann and Aw-Hassan, 2012) is an economically self-sustaining agricultural approach (Christmann et al., 2017, 2021a,b) that aims to increase income per surface through pollinator-friendly habitat enhancement as an incentive for farmers to contribute to pollinator protection. Within this framework, farmers dedicate 25% of their field area to seed Marketable Habitat Enhancement Plants (MHEP) that provide pollinators with floral rewards and farmers with additional income.
Farming with Alternative Pollinators fields provides, in addition to MHEP, nesting and water support made out of local materials. The approach demonstrated its effectiveness in increasing crop yield and reducing pest abundance (Christmann et al., 2017, 2021a,b), and significantly boosting pollinator abundance and diversity in agro-ecosystems (Christmann et al., 2021a,b; Sentil et al., 2021).
One mechanistic explanation of the population boost could be the inclusion of additional pollen sources in the diet. However, to date, no study has assessed the contribution of the FAP approach on crop pollinator dietary breadth using pollen analyses, and no detailed research investigating the effect of flowering strips on crop pollinator dietary breadth has been conducted either. Most previous studies have assessed the effect of flower strips on crop pollinator abundance and species richness using floral visits (Blaauw and Isaacs, 2014; Garibaldi et al., 2014; Feltham et al., 2015). Floral visitation records do not provide comprehensive information regarding the dietary pollen niches of crop flower visitors, and thus lack information about the co-flowering plants that crop flower visitors use to complement and balance their diets. Floral visitation alone is therefore not sufficient for assessing the effect of marketable habitat enhancement plants on the diet or the health of crop pollinators.
In this study, we aim to describe the pollen diet and the foraging behavior of pumpkin and faba bean flower visitors and investigate whether the FAP approach is effective in supporting the flower visitors of the two crops by extending their pollen diet. More specifically, we ask the following questions: 1. Do crop flower visitor species collect or carry pollen from a single or from multiple host plants (i.e., MHEP, main crop, and/or wild plants)? 2. Do crop flower visitors visit crops for pollen or nectar? 3. Does the FAP approach increase the dietary breadth and the number of pollen genera of crop flower visitors?
We expect that: (1) crop flower visitors collect pollen from either single or multiple host plants, depending on the flower visitor species; (2) given the physical and the chemical defenses of pumpkin pollen (Lundgren, 2009) and the high-quality faba bean pollen (Goulson and Darvill, 2004), we expect that pumpkin and faba bean will be visited for nectar and pollen, respectively; and (3) the FAP approach increases the dietary breadth and the number of pollen genera collected by crop flower visitors.
The experiments were carried out in four regions in Morocco (Errachidia, Settat, Sefrou, and Kenitra), representing different climate and landscape characteristics. The landscape in Settat is homogenous and dominated by intensive agriculture (90% of the arable land). The major agricultural activity in this region is cereal production. Unlike Settat, the landscape in Kenitra is characterized by diversified agricultural crop production (33%), forest plains, and several other semi-natural habitat types. In Sefrou, arable land does not exceed 34%, the remaining landscape is covered by forest and other semi-natural areas (e.g., grasslands and pastures). In contrast to the other regions, 37% of the land surface of Errachidia is covered by Hamadas, consisting of high, largely barren, hard, and rocky plateaus. The major agricultural activities in these oases are date palm, cereal, and legume production (Ministry of Agriculture, Fisheries, Rural Development, Water, and Forests, 2020).
Two crops were selected: faba bean (Vicia faba, Fabaceae) and pumpkin (Cucurbita maxima, Cucurbitaceae). These crops are two of the leading food crops in Morocco and they are broadly cultivated in the four studied regions. Besides that, they show contrasting phenologies and floral traits (Ali et al., 2014; Murphy-Bokern et al., 2017) and rely on insect pollination to a differing extent, with pumpkin being essentially dependent on insect pollinators and faba bean being a moderately pollinator-dependent crop (Klein et al., 2007). Crops were planted in the four regions within two years (2018 and 2019). Faba bean and pumpkin were not planted in Errachidia in 2018 and pumpkin was not planted in Kenitra in 2018, resulting in 13 trials (i.e., three faba bean trials and two pumpkin trials in 2018 and four faba bean trials and four pumpkin trials in 2019). For each trial, eight fields of 300 m2 were selected (i.e., five FAP fields and three control fields), totaling 104 FAP and control fields (Supplementary Table 1). Three fields were excluded because of their low maintenance, resulting in 101 fields. For each trial, eight MHEP were chosen among the following plants: Fabaceae (cultivated lupines Lupinus albus, wild lupins Lupinus luteus, grass pea Lathyrus sativus, alfalfa Medicago sativa, white clover Trifolium repens), Apiaceae (anise Pimpinella anisum, celery Apium graveolens, coriander Coriandrum sativum, and cumin Cuminum cyminum), Cucurbitaceae (melon Cucumis melo and zucchini Cucurbita pepo), Asteraceae (sunflower Helianthus annuus), Brassicaceae (canola Brassica napus and arugula Eruca sativa), Lamiaceae (chia Salvia hispanica), and Malvaceae (okra Abelmoschus esculentus). The number of MHEP was restricted to 4, 5, or 6 MHEP in some trials depending on the crop phenology and the MHEP that can be sown during the blooming of the main crop. Within each trial, the same 4–8 MHEP were used for all FAP fields.
Farming with Alternative Pollinators and control fields were separated from each other by a minimum distance of 200 m, which corresponds to the average or the maximum foraging distance of the main wild pollinator groups (Gathmann and Tscharntke, 2002; Wratten et al., 2003; Elliott, 2009). Within FAP fields, the main crop was sown in 75% of the field area surrounded by four to eight MHEP strips in 25% of the field area. In contrast, the main crop in control fields occupied the entire field area (Figure 1). MHEP strips were all one meter wide and five to 30 m long depending on the number of selected MHEP (Figure 1 and all experimental designs in Supplementary Figures 1–4). MHEP were chosen depending on soil and climatic conditions of the regions and based on their attractiveness to insect pollinators (i.e., diverse floral traits, including color, morphology, bloom phenology, and flower rewards) and farmers. Crops were managed in a similar way under the supervision of the research team. Fields were irrigated by drip irrigation. Farmers applied manure as an organic fertilizer to improve the soil fertility and pulled out weeds manually by hand.
Figure 1. Experimental designs of FAP and control fields. On the left, FAP field (the main crop in 75% of the field surrounded by marketable habitat enhancement plant species in the remaining 25% area). On the right, control field (the main crop only). The main crop corresponds to either faba bean or pumpkin. For each trial, four to eight marketable habitat enhancement plant species were selected among the following species: cultivated lupinus, grass pea, alfalfa, canola, coriander, arugula, flax, chia, canola, wild lupinus, celery, sunflower, zucchini, vetch, clover, white clover, anise, melon and okra. The two arrows correspond to the two transects for pollinator survey.
Flower visitors of each trial were surveyed two times when the main crops and MHEP were in bloom and when climatic conditions were favorable for insect activity (i.e., sunny days). In each insect sampling, we surveyed the 75% zone (i.e., the main crop in FAP and in control fields). We observed the main crop in FAP and control fields for 10 min within two transect corridors (i.e., 5 min per transect corridor) that were 4 m wide (i.e., 2 m from each side of the transect) and 28 m long (Figure 1). The insects that were observed visiting flowers were caught using a net or hand vacuum, anesthetized with ethyl acetate and transferred into cyanide vials. They were then pinned and sent to specialists for identification to the species level using either comparative collections or identification keys (Priesner, 1957; Brooks, 1988; Osten, 2000; Michez et al., 2004; Bogusch and Straka, 2012). The remaining flower visitors were identified by the authors to the lowest taxonomic level feasible using entomological publications (Borror and White, 1991).
All flower visitors recorded on faba bean and pumpkin flowers (322 and 499 flower visitors, respectively) were examined under a microscope. Pollen grains were also removed under a microscope. Flies, wasps, butterflies, and wild bee males were excluded, because they do not collect pollen and they were not carrying pollen on their bodies, resulting in 118 bee individuals carrying or collecting pollen grains after this exclusion (76 in pumpkin and 42 in faba bean). Apis mellifera, Xylocopa pubescens, and Bombus terrestris were not examined, because they were identified during insect surveys and not captured.
Before removing pollen, bees were classified as nectar collectors (those carrying pollen in a disorderly manner on their bodies, but not on their scopae) or pollen foragers (those collecting pollen on their scopae). We extracted pollen from both categories: pollen foragers and nectar collectors. For the pollen foragers, we removed pollen from the bee scopae, and for the nectar collectors, we used an entomological pin to brush the pollen off of their bodies (e.g., head and thorax) onto a microscope slide. Pollen preparation and identification were conducted using the protocol described by Wood and Roberts (2017). The size of pollen loads was visually estimated relative to the size of the bee, ranging from a full load (4/4) to a one-fourth load (1/4). After the relaxation of the pinned specimens in a sealed container filled with boiling water, we removed pollen from the scopa or the body of captured insects using an entomological pin. The pollen grains were placed in a drop of water on a glass slide to allow rehydration. After gentle heating to allow evaporation, a 2 mm3 cube of fuchsin jelly was added. The cube was then melted and covered with a microscope cover slip to create a layer of stained pollen. Pollen was identified to morphological groups, largely corresponding to genera, using an own unpublished reference collection of Mediterranean plant species. The proportion of each pollen genus in the load was estimated along three randomly selected lines across the cover slip at a ×400 magnification. The proportion of the load by volume was estimated by the relative area of the slide occupied by each pollen genera, rather than the absolute number of grains, in order to better reflect the total volume of pollen collected. This is an important correction in mixed loads where pollen grains of different plant species often differ widely in size (Cane and Sipes, 2006). Species representing <2% of the load were excluded.
Moreover, we took into account the level of filling of the scopa with pollen. Pollen genera percentages were weighted based on the overall estimated size of each scopal load. For instance, a full load (4/4) comprised 40% of Brassica sp. and 60% of Sinapis sp. would give a final weight of 40 for Brassica sp. and a final Sinapis sp. weight of 60, while a quarter load (1/4) comprised of 40% Brassica sp. and 60% Sinapis sp. would receive a final Brassica sp. weight of 10 and a final Sinapis sp. weight of 15.
As we do not have a sufficiently large sample size to calculate dietary breadth using more established techniques (e.g., Wood and Roberts, 2017; Wood et al., 2019), a novel method was taken to calculate comparative dietary breadth. The method is based on the Berger–Parker’s dominance index (BPI). For each species, the weighted percentage of each collected pollen genus was calculated, and the highest percentage was taken as the measure of dietary breadth. For example, a species collecting pollen from a single pollen genus only (100%) would receive a score of 100, indicating a narrow dietary breadth. A species collecting five different pollen genera at 20% each would receive a score of 20, indicating a comparatively wider dietary breadth (see Supplementary Tables 2, 3). We calculated the inverse of BPI (i.e., 100-BPI) to render the values of Berger–Parker’s dominance index, much more intuitive (smaller value = narrower diet). To consider the number of pollen genera, as well as the abundance of each pollen genus we computed another index for each bee species: the Shannon’s diversity index (H′), using the following formula: H′ = −∑ [pi × log(pi)], in which pi is the proportion of a pollen of a specific plant genus compared to all pollen grains collected by that species.
As test assumptions of normality and homogeneity were not met, statistical analyses using generalized linear models (GLM) (stats package) with a Quasi-Poisson error structure were performed to compare both indices of dietary breadth: Shannon’s diversity index (H′) and Berger–Parker’s dominance index between the main crop in FAP and control fields. It was not possible to conduct Generalized Linear Mixed Models (GLMM) with the region as a random affect for dietary breadth, because the variable was computed at the species level, and the individuals of some flower visitor species were collected in more than one region. The number of pollen genera of the characterized flower visitor individuals were compared between crops, and between FAP and control fields using GLMM with a negative binomial error distribution (lme4 package). The equality of variances for dietary breadth and number of pollen genera were assessed using Levene’s test (Car package; Fox and Weisberg, 2020) and the normality was tested numerically using a Shapiro test (Mvnorm test package; Jarek, 2012). The statistical tests were conducted in R software (version 3.6.3; R Development Core Team, 2020). To verify whether the number of pollen genera was modulated by the number of MHEP species used in each trial, Spearman’s correlation (r) test was performed using a significance levels of 5%. The result of this correlation shows that there is no significant correlation between the two variables. Contrary to the number of pollen genera, it was not possible to test this correlation for dietary breadth, because this variable was computed at the species level, and some species included individuals from different trials.
Overall, 821 flower visitors were collected during surveys, 322 and 499 in pumpkin and faba bean, respectively. Wild bees were by far the main flower visitors of pumpkin and faba bean with 93 and 76% of the total flower visitors recorded in each crop, respectively (Figure 2A). Regarding wild bees, most of pumpkin flower visitors were Halictidae (89%), followed by Apidae (6%), while Andrenidae, Apidae, and Halictidae represented 37, 36, and 21% of the flower visitors recorded in faba bean, respectively (Figure 2B).
Figure 2. (A) Proportional abundance of flower visitor taxa in faba bean and pumpkin. (B) Relative abundance of wild bee family in faba bean and pumpkin. The numbers indicate the proportion of flower visitor taxa and bee family expressed as a percentage.
Among all the insect individuals caught foraging on faba bean and pumpkin (i.e., 821 flower visitors), 86% failed to carry or collect pollen, whereas only 14% (i.e., 76 and 42 flower visitors recorded on pumpkin and faba bean flowers, respectively) carried or collected pollen of crops, MHEP and/or wild plants on their bodies or on their scopae. The 118 flower visitors carrying or collecting pollen, belong to 25 bee species. In total, 20 pollen genera belonging to 10 families were identified (Supplementary Tables 2, 3).
Among the bee species that were carrying or collecting pollen in pumpkin and faba bean, 33 and 43%, respectively, carried or collected the pollen of a single plant genera, whilst the remaining bee species (67 and 57% in pumpkin and faba bean, respectively) had pollen of two or more host plants (i.e., crop, MHEP, and/or wild plants).
For dietary breadth, a similar trend was seen. A total of 67 and 44% of bee species with pollen recorded in pumpkin and faba bean, respectively, had dietary breadth with (100-BPI) values higher than 10%, and 56 and 38% of species with pollen recorded in pumpkin and faba bean, respectively, had dietary breadth with H′ values higher than 0.2, indicating that these species had a comparatively wide dietary breadth.
Although species visiting pumpkin carried or collected more pollen taxa and showed a broader dietary breadth in comparison to faba bean, there were no significant differences between crops, neither in number of pollen genera carried or collected (GLM, p = 0.321) nor in flower visitor species dietary breadth (Shannon’s diversity index; GLM, p = 0.19) (Berger–Parker’s dominance index; GLM, p = 0.352).
Among the pumpkin and faba bean flower visitors that were carrying or collecting pollen of crops, MHEP and/or wild plants either on their bodies or on their scopae, 95 and 10%, respectively, were carrying or collecting conspecific pollen. These results imply that only 22 and 1% of all flower visitors caught foraging on pumpkin and faba bean, respectively, were carrying or collecting the pollen of these crops.
Considerable variation in wild bee foraging behavior between pumpkin and faba bean was observed. Indeed, only 8% of the pollinators with conspecific pumpkin pollen, were collecting the pollen of this crop on their scopae, whereas the high proportion (92%) were carrying the pumpkin pollen in a disorderly manner on their bodies. In contrast to pumpkin, all the pollinators with conspecific pollen of faba bean were collecting the crop pollen on their scopae. These results imply that among the 76 and 42 flower visitors with pollen, recorded in pumpkin and faba bean, respectively, just 8 and 10% (i.e., 2 and 1% of all the flower visitors collected) were using the crops as pollen host plants (i.e., pollen foragers) and the remaining individuals were seeking nectar.
The FAP approach did neither impact the overall dietary breadth of bee species (Shannon’s diversity index; GLM, p = 0.089; Figure 3A) (Berger–Parker’s dominance index; GLM, p = 0.107; Figure 3B) nor the number of pollen carried or collected by bee individuals (GLMM, p = 0.238; Figure 3C).
Figure 3. Box plots comparing wild pollinator dietary breadth and the number of pollen genera between FAP fields (the main crop in 75% of the field surrounded by marketable habitat enhancement plant species in the remaining 25% area) and control fields (the main crop only). (A) Wild pollinator species dietary breadth in FAP and control fields using Shannon’s diversity index. (B) Wild pollinator species dietary breadth in FAP and control fields using Berger–Parker’s dominance index. (C) Number of pollen genera of wild pollinator individuals in FAP and control fields. Boxes represent the lower and upper quartiles, the solid line the median, outliers (circles) are values being more than 1.5 times box length from upper and lower edge of respective box.
The MHEP (i.e., coriander, canola, and sunflower) were substantial pollen sources for bees visiting the main crops in FAP fields (Figures 4, 5). The pollen of MHEP was recorded in the pollen loads of 35% of pumpkin flower visitors and 36% of faba bean visitors recorded in FAP fields. Wild bees recorded in pumpkin carried or collected pollen exclusively from sunflower. In faba bean, bees carried or collected pollen from coriander (27%) and canola (9%).
Figure 4. The proportional contribution of plant genera to the pollen diet of the bee species recorded in pumpkin (76 bees belonging to 9 species). Each column illustrates the proportions of plant genera in the pollen load of the bee species. The number between brackets indicates the number of individuals characterized per species.
Figure 5. The proportional contribution of plant taxa to the pollen diet of the characterized bee species recorded in faba bean (42 bees belonging to 16 species). Each column illustrates the proportions of plant taxa in the pollen load of the bee species. The number between brackets indicates the number of individuals characterized per species.
Our study shows that almost two-thirds of the species carrying or collecting pollen when visiting pumpkin flowers and half of the species carrying or collecting pollen when visiting faba bean flowers gathered this pollen from two or more host plants and a great proportion of the characterized bee species displayed a wide dietary breadth. Among all visitors recorded in pumpkin and faba bean (322 and 499, respectively), 22 and 1%, respectively were carrying or collecting the crop pollen, and only 2 and 1%, respectively were using crops as pollen host plants, with by far the greatest proportion seeking nectar. The FAP approach did not show an effect on the overall dietary breadth and the number of pollen genera carried or collected by flower visitors.
In line with previous findings, pumpkin attracts more generalist than specialist bee species (Brochu, 2018), whereas faba bean is mainly visited by bees specialized on Fabaceae (Marzinzig et al., 2018). The deep and curved nectar tube of faba bean corolla (Stoddard and Bond, 1987) restricts pollinator species and allows only pollinators with long tongues to really reach floral resources (Goulson and Darvill, 2004). Thus, bee species with long tongues such as Eucera can be more specialized in their choices of host plants than short-tongued species (Michez et al., 2019). Though we recorded a high proportion of faba bean flower visitor species with narrow dietary breadths, the proportion of visitor species with wide dietary breadth is not negligible (44% of the studied species).
Pumpkin provides abundant nectar rewards and displays accessible flowers (Vidal et al., 2006). Through the showy open flowers and the large amount of nectar, pumpkin attracts a wide array of flower visitors (Burgett, 2000), including pollinators with wide dietary breadth (Nicodemo et al., 2009; Artz and Nault, 2011; Ali et al., 2014; Pande and Verma, 2016). The important proportion of species with wide dietary breadth visiting pumpkin and faba bean underline the importance of providing diverse flowering plants to conserve healthy crop pollinators and other pollinators living in this area or farm. Pollinator-dependent crops provide a time-limited floral resource (Guzman et al., 2019). Outside this short crop blooming period, pollinators can experience a deficiency in nutritional resources in intensified agricultural landscapes (Rundlöf et al., 2014). The direct consequences of nutritional deficiency or unbalanced intakes may result in a decrease in offspring production and fitness (Di Pasquale et al., 2013; Vanderplanck et al., 2018). Indeed, access to diverse pollen diets was found to enhance resistance to diseases, tolerance to pesticides, and immunity to parasites and pathogens in polylectic bee species (Wilson-Rich et al., 2008; Alaux et al., 2010; Colwell et al., 2017; Zhang et al., 2020). For example, Di Pasquale et al. (2013) demonstrated that when parasitized, bees feed with a poly-floral blend lived longer than bees fed with mono-floral pollen.
We acknowledge that our sample size was small (e.g., Lasioglossum subhirtum was represented by a single specimen). As the sample size affects directly the number of pollen taxa collected or carried by flower visitor species, we assume that some bee species would be regarded as more generalized if a larger and more representative sample size of species with pollen was available.
Growing evidence suggests that insects recorded in visitation data do not necessarily carry pollen. Generally, in most bee collections, only a minority of specimens have pollen. Also, pollen grains could be lost from specimens while capturing and handling the flower visitors. For example, in the study of Popic et al. (2013), the proportion of bees not carrying pollen was high (72%). Therefore, the small percentage of specimens having pollen (i.e., 14%) among the examined flower visitors (i.e., 821 individuals) was expected. Additionally, the abundant species: A. mellifera and B. terrestris were not assessed for pollen grains, as they were identified visually during the surveys and not captured. This might shape the findings of the foraging behavior of crop flower visitors and also their dietary breadth, as these species are large-bodied, polylectic, and forage from a wide range of flowering plant species.
The low proportion of bees with crop pollen (in our case, 22 and 1% in pumpkin and faba bean, respectively) may result from differences in insect behaviors interacting with plant morphology and chemistry. For instance, faba bean visitors display three types of feeding behaviors; those visiting the front of the flowers (honest visitors), species visiting the base of the flowers (nectar robbers) and those using extrafloral nectaries (i.e., positioned underneath the stipules) (Tasei, 1976). Based on these categories, only bees visiting the front of flowers are exhibiting legitimate flower visits and could potentially pick up pollen (Vaudo et al., 2015).
In contrast to faba bean, pumpkin does not display any morphological adaptations to limit flower visitors (Vidal et al., 2006). Consequently, visitors of male flowers that are either seeking nectar or pollen may potentially pick up pollen grains, which explains the greater proportion of individuals carrying or collecting pumpkin pollen (22%). However, the proportion of individuals using pumpkin as a pollen host plant (i.e., collecting pumpkin pollen on their scopae) was still low (2%). The open shallow flowers of pumpkin and the high amount of nectar it produces attract a wide array of flower visitor species, but to protect pollen from over collection, pumpkin uses mechanical and chemical pollen defenses (Brochu, 2018). Cucurbit pollen is large, heavy, spiky, difficult to handle, has a sticky pollenkitt, and contains many compounds that may act as defenses to discourage generalist pollinators (Lundgren, 2009). For instance, reduced pollen consumption and reproduction leading to increased mortality were observed in Bombus impatiens colonies fed exclusively on cucurbit pollen (Brochu et al., 2020). Therefore, most studied bees in this experiment had pumpkin pollen on their bodies but were not intentionally collecting it.
These results emphasize the importance of considering pollen analyses and foraging behavior as important factors for developing appropriate marketable habitat enhancement plants that complement the floral resources provided by agricultural crops (Vaudo et al., 2015), rather than simply using floral visits that only partially predict pollinator pollen host plants (Bosch et al., 2009; Popic et al., 2013).
Our results show that the FAP approach displayed a neutral effect on crop flower visitor dietary breadth and the number of pollen genera carried or collected by crop flower visitors. These findings are in agreement with previous research that has found that wildflowers strips, hedgerows, and MHEP do not support crop flower visitor abundance, richness, and pollen diet (Sardiñas and Kremen, 2015; Sardiñas et al., 2016; Wood et al., 2017; Zamorano et al., 2020; Sentil et al., 2021). In contrast, flower strips are also known to benefit the pollen diet of common bees (e.g., honeybees and bumblebees), but not necessarily of wild solitary bees (Carvell et al., 2007; Wood et al., 2017). In the case of our research, the common bees (i.e., honeybees and bumblebees) were not included in the study, which could have changed the results in favor of the FAP approach, especially that the p-value of flower visitor dietary breadth (Shannon’s diversity index) was close to significance (p = 0.08). Adding to this, pollen load compositions of faba bean and pumpkin flower visitors show that wild flowering plants (e.g., Centaurea sp., Sinapis sp., and Papaver sp.) were clearly offering supplementary pollen resources. Indeed, 87 and 55% of the flower visitor species of faba bean and pumpkin with pollen, respectively carried or collected pollen from wild plants (Supplementary Tables 1, 2). These results are in line with the study contacted by Wood et al. (2017) that demonstrated that the majority of solitary bee species collect most of their pollen from the existing native wild flowering plant species and not necessarily from the provided floral resources, even in areas where sown flowering plants represent the highest proportion of available floral resources.
We acknowledge that the four studied regions have contrasting landscape context (e.g., Sefrou has more semi-natural areas compared to Settat), which could potentially affect the results of the impact of the FAP approach on flower visitor dietary breadth; however, it was not possible to include the region as a random effect in our models.
In our trials, wild bees recorded in pumpkin gathered pollen exclusively from sunflower, whereas in faba bean, bees carried or collected pollen from coriander and canola. Mass-flowering crops such as sunflower, canola, and coriander are known to attract abundant and diverse wild bees (Nderitu et al., 2008; Ali et al., 2011; Sharma and Meena, 2019), as they produce abundant pollen and nectar resources (Nicolson and Human, 2013; Adamchuk et al., 2017; Thom et al., 2018), which may explain the occurrence of their pollen grains on bees visiting the crops. The occurrence of the pollen of specific MHEP (i.e., sunflower, coriander, and canola) on the pollen loads of crop flower visitors is associated with the MHEP species planted surrounding faba bean and pumpkin. Thus, if other MHEP mixtures were provided, we would record the pollen of MHEP species from the new provided mixture. Pollen genera of the other provided MHEP were not recorded in pollen loads of the studied species. The possible explanations of this result may be that these MHEP have been visited for their nectar that was not assessed in this study and/or that they were not attractive to crop flower visitors. For instance, pollen of Cucurbitaceae (e.g., zucchini and melon) is sticky, large, heavy, and contains many chemical compounds that discourage bees from its consumption (Lundgren, 2009; Rodrigo Gómez et al., 2016; Brochu et al., 2020). However, the high amount of nectar they produce attracts a wide array of flower visitor species (Ali et al., 2014; Pande and Verma, 2016). Cultivated and wild lupinus are nectarless and are mainly visited for pollen (McGregor, 1976). In addition to that, grass pea, white clover, and spices (e.g., anise, coriander, and cumin) produce abundant pollen and nectar (Laxmikant and Devendra, 2014; Meena et al., 2015; Sharma and Meena, 2019). Nevertheless, their pollen grains were not recorded on crop flower visitors. Hence, further investigations on the foraging behavior of flower visitors on MHEP can contribute to better understanding of the foraging behavior of crop flower visitors.
Agricultural intensification at the expense of natural flowering vegetation is potentially affecting pollinator health (Naug, 2009), and bees using pollen from a smaller range of plants, or non-favorite host plants, are exposed to a higher risk of stress leading to decline (Rasmont, 1988; Kleijn and Raemakers, 2008; Vanderplanck et al., 2018; Wood et al., 2019). Plant diversification in farmlands may play an important role in curbing pollinator loss and maintaining healthy crop pollinators, in particular in countries unable to provide financial compensation for seeding wildflower strips.
Our results demonstrate that a great proportion of pumpkin and faba bean flower visitors had a wide dietary breadth and that the crop flowers were mainly visited for nectar. These results suggest that flower visitors of pumpkin and faba bean would benefit from more diverse pollen resources nearby. The FAP approach was not significantly effective in supporting the dietary breadth of crop flower visitors nor in increasing the number of pollen genera carried or collected by these visitors. Further studies on the impact of the FAP approach on the dietary breadth of other crops and using the common pollinators (i.e., honeybees and bumblebees) are needed. Additional investigations to characterize pollinator health in relation to dietary breadth (using pollen and nectar analyses) and their foraging behavior on other crops are required, in order to effectively tailor the MHEP that meet the food requirements of crop pollinators in farmlands.
We identified the contribution of MHEP to crop flower visitors’ diet. Nevertheless, from the pollinator conservation viewpoint, additional investigations on the contribution of the FAP approach to the diet of pollinators present in the agro-ecosystems rather than pollinators visiting the crops are needed. In fact, selecting only MHEP flowering at the same time as the main crop and benefiting only the pollinators of the main crop, would drive FAP into agricultural intensification, whereas it was developed to support a wide range of different pollinators by different types of MHEP flowers and by prolonging the flowering period beyond the period of the main crop (Christmann and Aw-Hassan, 2012; Christmann et al., 2021b; Sentil et al., 2021).
The raw data supporting the conclusions of this article will be made available by the authors, without undue reservation.
AS, TW, and DM conceived the structure of the manuscript. AS performed the statistical analyses and prepared the pollen. AS, PL, LH, IE, OI, and YB made the fieldwork. TW identified the pollen. PR made the data management. SC took charge of the project management. All authors have read the manuscript, participated in the revisions, and approved the final version.
This study is part of a project led by ICARDA and funded by the German Federal Ministry for the Environment, Nature Conservation and Nuclear Safety (BMU), within the International Climate Initiative (IKI). TW and DM were supported by the Research Foundation of Flanders (FWO) and Federation Wallonia – Brussels (FNRS) as part of the EOS project (CLiPS project 30947854) and “chargé de recherche” mandate.
The authors declare that the research was conducted in the absence of any commercial or financial relationships that could be construed as a potential conflict of interest.
All claims expressed in this article are solely those of the authors and do not necessarily represent those of their affiliated organizations, or those of the publisher, the editors and the reviewers. Any product that may be evaluated in this article, or claim that may be made by its manufacturer, is not guaranteed or endorsed by the publisher.
We would like to thank all specialists who contributed to the taxonomical expertise required for this work: Achik Dorchin (Tel Aviv University, Israel and UMons, Belgium) for Eucerini, Max Kasparek (Heidelberg, Germany) for Anthidiini, and Alain Pauly (Royal Belgian Institute of Natural History, Belgium) for Halictini. We acknowledge the contribution of Moulay Chrif Smaili (INRA Morocco), who worked on pests and natural enemies and cared for participatory pollinator-friendly pest control. We would like to thank Mohamed Chokri for his help with field sampling and data collection, and Sara Reverté for her assistance in statistical analyzes during revision. We also received a great help from Dimitri Evrard (UMons) for collection management and digitization.
The Supplementary Material for this article can be found online at: https://www.frontiersin.org/articles/10.3389/fevo.2022.824474/full#supplementary-material
BDI, Berger–Parker’s dominance index; FAP, Farming with Alternative Pollinators; MHEP, Marketable Habitat Enhancement Plants.
Adamchuk, L., Bilotserkivets, T., and Šimková, J. (2017). Nectar and pollen productivity of common chicory. Agrobiodiversity Improving Nutrition Health Life Qual. doi: 10.15414/agrobiodiversity.2017.2585-8246
Aizen, M. A., Aguiar, S., Biesmeijer, J. C., Garibaldi, L. A., Inouye, D. W., Jung, C., et al. (2019). Global agricultural productivity is threatened by increasing pollinator dependence without a parallel increase in crop diversification. Glob. Chang. Biol. 25, 3516–3527. doi: 10.1111/gcb.14736
Aizen, M. A., Garibaldi, L. A., Cunningham, S. A., and Klein, A. M. (2009). How much does agriculture depend on pollinators? lessons from long-term trends in crop production. Ann. Bot. 103, 1579–1588. doi: 10.1093/aob/mcp076
Alaux, C., Ducloz, F., Crauser, D., and Le Conte, Y. (2010). Diet effects on honeybee immunocompetence. Biol. Lett. 6, 562–565. doi: 10.1098/rsbl.2009.0986
Albrecht, M., Kleijn, D., Williams, N. M., Tschumi, M., Blaauw, B. R., Bommarco, R., et al. (2020). The effectiveness of flower strips and hedgerows on pest control, pollination services and crop yield: a quantitative synthesis. Ecol. Lett. 23, 1488–1498. doi: 10.1111/ele.13576
Ali, M., Saeed, S., Sajjad, A., and Bashir, M. A. (2014). Exploring the best native pollinators for pumpkin (Cucurbita pepo) production in Punjab, Pakistan. Pak. J. Zool. 46, 531–539.
Ali, M., Saeed, S., Sajjad, A., and Whittington, A. (2011). In search of the best pollinators for canola (Brassica napus L.) production in Pakistan. Appl. Entomol. Zool. 46, 353–361. doi: 10.1007/s13355-011-0051-0
Artz, D. R., and Nault, B. A. (2011). Performance of Apis mellifera, Bombus impatiens, and Peponapis pruinosa (Hymenoptera?: Apidae) as pollinators of pumpkin. J. Econ. Entomol. 104, 1153–1161. doi: 10.1603/EC10431
Batáry, P., Dicks, L. V., Kleijn, D., and Sutherland, W. J. (2015). The role of agri-environment schemes in conservation and environmental management. Conserv. Biol. 29, 1006–1016. doi: 10.1111/cobi.12536
Blaauw, B. R., and Isaacs, R. (2014). Flower plantings increase wild bee abundance and the pollination services provided to a pollination-dependent crop. J. Appl. Ecol. 51, 890–898. doi: 10.1111/1365-2664.12257
Bogusch, P., and Straka, J. (2012). Review and identification of the cuckoo bees of central Europe (Hymenoptera: Halictidae: Sphecodes). Zootaxa 3311, 1–41. doi: 10.11646/ZOOTAXA.3311.1.1
Borror, D. J., and White, R. E. (1991). Les Insectes de l’Amérique du Nord (au nord du Mexique). Zurich: Laprairie.
Bosch, J., Martín González, A. M., Rodrigo, A., and Navarro, D. (2009). Plant-pollinator networks: adding the pollinator’s perspective. Ecol. Lett. 12, 409–419. doi: 10.1111/j.1461-0248.2009.01296.x
Brochu, K. (2018). Differential Impacts of Pollen Quality and Microbial Communities on Generalist and Specialist Bees Visiting a Shared Food Resource. New York, NY: Cornell University.
Brochu, K. K., Van Dyke, M. T., Milano, N. J., Petersen, J. D., McArt, S. H., Nault, B. A., et al. (2020). Pollen defenses negatively impact foraging and fitness in a generalist bee (Bombus impatiens: Apidae). Sci. Rep. 10:3112. doi: 10.1038/s41598-020-58274-2
Brooks, R. W. (1988). Systematics and phylogeny of the Anthophorine bees (Hymenoptera Anthophoridae; Anthophorini). University Kansas Bull. 53, 436–575.
Burgett, D. M. (2000). Pollination and Pollinators of Pumpkin and Squash (Cucurbita maxima Duchesne) Grown for Seed Production in the Willamette Valley of Western Oregon. Oregon: Oregon State University.
Cane, J. H., and Sipes, S. S. (2006). Characterizing Floral Specialization by Bees: Analytical Methods and a Revised Lexicon for Oligolecty, Plant–pollinator Interactions: From Specialization to Generalization. London: The university of Chicago press.
Carvell, C., Meek, W. R., Pywell, R. F., Goulson, D., and Nowakowski, M. (2007). Comparing the efficacy of agri-environment schemes to enhance bumble bee abundance and diversity on arable field margins. J. Appl. Ecol. 44, 29–40. doi: 10.1111/j.1365-2664.2006.01249.x
Carvell, C., Roy, D. B., Smart, S. M., Pywell, R. F., Preston, C. D., and Goulson, D. (2006). Declines in forage availability for bumblebees at a national scale. Biol. Conserv. 132, 481–489. doi: 10.1016/j.biocon.2006.05.008
Chacoff, N. P., Resasco, J., and Vázquez, D. P. (2018). Interaction frequency, network position, and the temporal persistence of interactions in a plant–pollinator network. Ecology 99, 21–28. doi: 10.1002/ecy.2063
Christmann, S. (2020). Pollinator protection strategies must be feasible for all nations. Nat. Ecol. Evol. 4, 896–897. doi: 10.1038/s41559-020-1210-x
Christmann, S., and Aw-Hassan, A. A. (2012). Farming with alternative pollinators (FAP)-An overlooked win-win-strategy for climate change adaptation. Agric. Ecosyst. Environ. 161, 161–164. doi: 10.1016/j.agee.2012.07.030
Christmann, S., Aw-Hassan, A., Rajabov, T., Khamraev, A. S., and Tsivelikas, A. (2017). Farming with alternative pollinators increases yields and incomes of cucumber and sour cherry. Agron. Sustain. Dev. 37:24. doi: 10.1007/s13593-017-0433-y
Christmann, S., Aw-Hassan, A., Güler, Y., Cumhur, H., Bernard, M., et al. (2021a). Two enabling factors for farmer-driven pollinator protection in low- and middle-income countries. Int. J. Agric. Sustain. 20, 54–67. doi: 10.1080/14735903.2021.1916254
Christmann, S., Bencharki, Y., Anougmar, S., Rasmont, P., Smaili, M. C., et al. (2021b). Farming with alternative pollinators benefits pollinators, natural enemies, and yields, and offers transformative change to agriculture. Sci. Rep. 11:18206. doi: 10.1038/s41598-021-97695-5
Colwell, M. J., Williams, G. R., Evans, R. C., and Shutler, D. (2017). Honey bee-collected pollen in agro-ecosystems reveals diet diversity, diet quality, and pesticide exposure. Ecol. Evol. 7, 7243–7253. doi: 10.1002/ece3.3178
Di Pasquale, G., Salignon, M., Le Conte, Y., Belzunces, L. P., Decourtye, A., et al. (2013). Influence of pollen nutrition on honey bee health: do pollen quality and diversity matter? PLoS One 8:e72016. doi: 10.1371/journal.pone.0072016
Duchenne, F., Thébault, E., Michez, D., Gérard, M., Devaux, C., et al. (2020). Long-term effects of global change on occupancy and flight period of wild bees in Belgium. Glob. Chang. Biol. 26, 6753–6766. doi: 10.1111/gcb.15379
Elliott, S. E. (2009). Subalpine bumble bee foraging distances and densities in relation to flower availability. Environ. Entomol. 38, 748–756. doi: 10.1603/022.038.0327
Feltham, H., Park, K., Minderman, J., and Goulson, D. (2015). Experimental evidence that wildflower strips increase pollinator visits to crops. Ecol. Evol. 5, 3523–3530. doi: 10.1002/ece3.1444
Garibaldi, L. A., Carvalheiro, L. G., Leonhardt, S. D., Aizen, M. A., Blaauw, B. R., et al. (2014). From research to action: enhancing crop yield through wild pollinators. Front. Ecol. Environ. 12:439–447. doi: 10.1890/130330
Gathmann, A., and Tscharntke, T. (2002). Foraging ranges of solitary bees. J. Anim. Ecol. 71, 757–764. doi: 10.1046/j.1365-2656.2002.00641.x
Gérard, M., Vanderplanck, M., Wood, T., and Michez, D. (2020). Global warming and plant – pollinator mismatches. Emerg. Top. Life Sci. 4, 77–86. doi: 10.1042/ETLS20190139
Gill, R. J., Baldock, K. C. R., Brown, M. J. F., Cresswell, J. E., Dicks, L. V., et al. (2016). Protecting an ecosystem service: approaches to understanding and mitigating threats to wild insect pollinators. Adv. Ecol. Res. 54, 135–206. doi: 10.1016/bs.aecr.2015.10.007
Goulson, D. (2019). The insect apocalypse, and why it matters. Curr. Biol. 29, R967–R971. doi: 10.1016/j.cub.2019.06.069
Goulson, D., and Darvill, B. (2004). Niche overlap and diet breadth in bumblebees; are rare species more specialized in their choice of flowers? Apidologie 38, 67–76. doi: 10.1051/apido
Goulson, D., Nicholls, E., Botías, C., and Rotheray, E. L. (2015). Bee declines driven by combined stress from parasites, pesticides, and lack of flowers. Science 347:1255957. doi: 10.1126/science.1255957
Grass, I., Albrecht, J., Jauker, F., Diekötter, T., Warzecha, D., et al. (2016). Much more than bees-wildflower plantings support highly diverse flower-visitor communities from complex to structurally simple agricultural landscapes. Agric. Ecosyst. Environ. 225, 45–53. doi: 10.1016/j.agee.2016.04.001
Guzman, A., Chase, M., and Kremen, C. (2019). On-farm diversification in an agriculturally-dominated landscape positively influences specialist pollinators. Front. Sustain. Food Syst. 3:87. doi: 10.3389/fsufs.2019.00087
Haaland, C., Naisbit, R. E., and Bersier, L. F. (2011). Sown wildflower strips for insect conservation: a review. Insect Conserv. Divers 4, 60–80. doi: 10.1111/j.1752-4598.2010.00098.x
IPBES (2016). Individual Chapters and their Executive Summaries of the Thematic Assessment on Pollinators, Pollination and Food Production (deliverable 3(a)). Germany: IPBES.
Jarek, S. (2012). Mvnormtest: Normality Test for Multivariate Variables. R Package Version 0.1–9. Vienna: R Found. Stat. Comput.
Kleijn, D., and Raemakers, I. (2008). A retrospective analysis of pollen host plant use by stable and declining bumble bee species. Ecology 89, 1811–1823. doi: 10.1890/07-1275.1
Kleijn, D., Bommarco, R., Fijen, T. P. M., Garibaldi, L. A., Potts, S. G., and van der Putten, W. H. (2019). Ecological Intensification: bridging the gap between science and practice. Trends Ecol. Evol. 34, 154–166. doi: 10.1016/j.tree.2018.11.002
Klein, A. M., Steffan-Dewenter, I., and Tscharntke, T. (2003). Fruit set of highland coffee increases with the diversity of pollinating bees. Proc. R. Soc. B Biol. Sci. 270, 955–961. doi: 10.1098/rspb.2002.2306
Klein, A. M., Vaissière, B. E., Cane, J. H., Steffan-Dewenter, I., Cunningham, S. A., et al. (2007). Importance of pollinators in changing landscapes for world crops. Proc. R. Soc. B Biol. Sci. 274, 303–313. doi: 10.1098/rspb.2006.3721
Laxmikant, B., and Devendra, M. (2014). Melittopalynological investigation of winter honeys collected from Apis dorsata hives of Mul tahsil of Chandrapur District of Maharashtra State (India). Int. Res. J. Sci. Eng. 2, 112–118.
Lundgren, J. G. (2009). Relationships of Natural Enemies and Non-Prey Foods. New York, NY: Springer.
Martín González, A. M., Dalsgaard, B., and Olesen, J. M. (2010). Centrality measures and the importance of generalist species in pollination networks. Ecol. Complex 7, 36–43. doi: 10.1016/j.ecocom.2009.03.008
Martinet, B., Zambra, E., Przybyla, K., Lecocq, T., Anselmo, A., Nonclercq, D., et al. (2020). Mating under climate change: impact of simulated heatwaves on the reproduction of model pollinators. Funct. Ecol. 35, 739–752. doi: 10.1111/1365-2435.13738
Marzinzig, B., Brünjes, L., Biagioni, S., Behling, H., and Link, W. (2018). Bee pollinators of faba bean (Vicia faba L.) differ in their foraging behaviour and pollination efficiency. Agric. Ecosyst. Environ. 264, 24–33. doi: 10.1016/j.agee.2018.05.003
McGregor, S. E. (1976). Insect Pollination of Cultivated Crop Plants. Washington, DC: US Department of Agriculture.
Meena, N. K., Singh, B., Kant, K., Meena, R. D., and Solanki, R. K. (2015). Role of insect pollinators in pollination of seed spices-a review. Int. J. Seed Spices 5, 1–17.
Michez, D., Rasmont, P., Terzo, M., and Vereecken, N. J. (2019). Bees of Europe. Hymenoptera of Europe. France: N.A.P edition.
Michez, D., Terzo, M., and Rasmont, P. (2004). Révision des espèces ouest-paléarctiques du genre Dasypoda Latreille 1802 (Hymenoptera, Apoidea, Melittidae). Linz. Biol. Beitr. 36, 847–900.
Murphy-Bokern, D., Stoddard, F. L., and Watson, C. A. (2017). Legumes in Cropping Systems. Wallingford: CABI Publishing.
Naug, D. (2009). Nutritional stress due to habitat loss may explain recent honeybee colony collapses. Biol. Conserv. 142, 2369–2372. doi: 10.1016/j.biocon.2009.04.007
Nderitu, J., Nyamasyo, G., Kasina, M., and Oronje, M. L. (2008). Diversity of sunflower pollinators and their effect on seed yield in Makueni District Eastern Kenya. Spanish J. Agric. Res. 6, 271–278. doi: 10.5424/sjar/2008062-318
Nicodemo, D., Helena, R., Couto, N., Malheiros, E. B., and De Jong, D. (2009). Honey bee as an effective pollinating agent of pumpkin. Sci. Agric. 66, 476–480. doi: 10.1590/S0103-90162009000400007
Nicolson, S. W., and Human, H. (2013). Chemical composition of the “low quality” pollen of sunflower (Helianthus annuus, Asteraceae). Apidologie 44, 144–152. doi: 10.1007/s13592-012-0166-5
Osten, T. (2000). Die scoliiden des mittelmeer-gebietes und angrenzender regionen (Hymenoptera). Ein Bestimmungsschlüssel. Linz. Biol. Beitr. 32, 537–593.
Ouvrard, P., Transon, J., and Jacquemart, A. L. (2018). Flower-strip agri-environment schemes provide diverse and valuable summer flower resources for pollinating insects. Biodivers. Conserv. 27, 2193–2216. doi: 10.1007/s10531-018-1531-0
Pande, R., and Verma, V. K. (2016). Performance of hymenopteran insects as pollinators of pumpkin in Meghalaya. J. Appl. Nat. Sci. 8, 1806–1810. doi: 10.31018/jans.v8i4.1044
Popic, T. J., Wardle, G. M., and Davila, Y. C. (2013). Flower-visitor networks only partially predict the function of pollen transport by bees. Austral Ecol. 38, 76–86. doi: 10.1111/j.1442-9993.2012.02377.x
Potts, S. G., Biesmeijer, J. C., Kremen, C., Neumann, P., Schweiger, O., and Kunin, W. E. (2010). Global pollinator declines: trends, impacts and drivers. Trends Ecol. Evol. 25, 345–353. doi: 10.1016/j.tree.2010.01.007
Priesner, H. (1957). A review of the Anthophora-species of Egypt (Hymenoptera: Apidae). Bull. Soc. Entomol. 41, 1–115. doi: 10.11646/zootaxa.4511.1.1
R Development Core Team (2020). R: A Language and Environment for Statistical Computing. Vienna: R Foundation for Statistical Computing.
Rasmont, P. (1988). Monographie Écologique et Zoogéographique des Bourdons de France et de Belgique (Hymenoptera, Apidae, Bombinae). Belgique: Faculté des Sciences agronomiques de l’État, Gembloux.
Rodrigo Gómez, S., Ornosa, C., Selfa, J., Guara, M., and Polidori, C. (2016). Small sweat bees (Hymenoptera: Halictidae) as potential major pollinators of melon (Cucumis melo) in the Mediterranean. Entomol. Sci. 19, 55–66. doi: 10.1111/ens.12168
Roger, N., Moerman, R., Carvalheiro, L. G., Aguirre-Guitiérrez, J., Jacquemart, A. L., et al. (2017). Drift in distribution and quality of host-plant resources in common bumblebees. Glob. Change Biol. 23, 68–76. doi: 10.1111/gcb.13373
Rundlöf, M., Persson, A. S., Smith, H. G., and Bommarco, R. (2014). Late-season mass-flowering red clover increases bumble bee queen and male densities. Biol. Conserv. 172, 138–145. doi: 10.1016/j.biocon.2014.02.027
Sardiñas, H. S., and Kremen, C. (2015). Pollination services from field-scale agricultural diversification may be context-dependent. Agric. Ecosyst. Environ. 207, 17–25. doi: 10.1016/j.agee.2015.03.020
Sardiñas, H. S., Tom, K., Ponisio, L. C., Rominger, A., and Kremen, C. (2016). Sunflower (Helianthus annuus) pollination in California’s Central Valley is limited by native bee nest site location. Ecol. Appl. 26, 438–447. doi: 10.1890/15-0033
Scheper, J., Reemer, M., Van Kats, R., Ozinga, W. A., Van Der Linden, G. T. J., et al. (2014). Museum specimens reveal loss of pollen host plants as key factor driving wild bee decline in the Netherlands. Proc. Natl. Acad. Sci. U S A. 111, 17552–17557. doi: 10.1073/pnas.1412973111
Sentil, A., Lhomme, P., Michez, D., Reverté, S., Rasmont, P., and Christmann, S. (2021). Farming with Alternative Pollinators” approach increases pollinator abundance and diversity in faba bean fields. J. Insect Conserv. doi: 10.1007/s10841-021-00351-6
Sharma, K., and Meena, N. K. (2019). Diversity of insect pollinators in coriander (Coriandrum sativum Linn.) VAR. ACR-1 under Semi-Arid region of Rajasthan. J. Pharmacogn. Phytochem. 8, 198–201.
Stoddard, F. L., and Bond, D. A. (1987). The pollination requirements of the faba bean. Bee World 68, 144–152. doi: 10.1080/0005772X.1987.11098923
Tasei, J. N. (1976). Les insectes pollinisateurs de la féverole d’hiver (Vicia Faba Equina L.) et la pollinisation des plantes mâle-stérile en production de semence hybride. Apidologie 7, 1–28. doi: 10.1051/apido:19760101
Thom, M. D., Eberle, C. A., Forcella, F., Gesch, R., and Weyers, S. (2018). Specialty oilseed crops provide an abundant source of pollen for pollinators and beneficial insects. J. Appl. Entomol. 142, 211–222. doi: 10.1111/jen.12401
Vanderplanck, M., Decleves, S., Roger, N., Decroo, C., Caulier, G., et al. (2018). Is non-host pollen suitable for generalist bumblebees? Insect Sci. 25, 259–272. doi: 10.1111/1744-7917.12410
Vaudo, A. D., Tooker, J. F., Grozinger, C. M., and Patch, H. M. (2015). Bee nutrition and floral resource restoration. Curr. Opin. Insect Sci. 10, 133–141. doi: 10.1016/j.cois.2015.05.008
Vaudo, A. D., Tooker, J. F., Patch, H. M., Biddinger, D. J., Coccia, M., et al. (2020). Pollen protein: lipid macronutrient ratios may guide broad patterns of bee species floral preferences. Insects 11:132. doi: 10.3390/insects11020132
Vidal, M. D. G., De Jong, D., Wien, H. C., and Morse, R. A. (2006). Nectar and pollen production in pumpkin (Cucurbita pepo L.). Rev. Bras. Bot. 29, 267–273. doi: 10.1590/S0100-84042006000200008
Wilson-Rich, N., Dres, S. T., and Starks, P. T. (2008). The ontogeny of immunity: development of innate immune strength in the honey bee (Apis mellifera). J. Insect Physiol. 54, 1392–1399. doi: 10.1016/j.jinsphys.2008.07.016
Wood, T. J., and Roberts, S. P. M. (2017). An assessment of historical and contemporary diet breadth in polylectic Andrena bee species. Biol. Conserv. 215, 72–80. doi: 10.1016/j.biocon.2017.09.009
Wood, T. J., Gibbs, J., Graham, K. K., and Isaacs, R. (2019). Narrow pollen diets are associated with declining Midwestern bumble bee species. Ecology 100:e02697. doi: 10.1002/ecy.2697
Wood, T. J., Holland, J. M., and Goulson, D. (2017). Providing foraging resources for solitary bees on farmland: current schemes for pollinators benefit a limited suite of species. J. Appl. Ecol. 56, 323–333. doi: 10.1111/1365-2664.12718
Wratten, S. D., Bowie, M. H., Hickman, J. M., Evans, A. M., Sedcole, J. R., and Tylianakis, J. M. (2003). Field boundaries as barriers to movement of hover flies (Diptera: Syrphidae) in cultivated land. Oecologia 134, 605–611. doi: 10.1007/s00442-002-1128-9
Zamorano, J., Bartomeus, I., Grez, A. A., and Garibaldi, L. A. (2020). Field margin floral enhancements increase pollinator diversity at the field edge but show no consistent spillover into the crop field: a meta-analysis. Insect Conserv. Divers. 13, 519–531. doi: 10.1111/icad.12454
Keywords: nectar, pumpkin, faba bean, wild bees, foraging behavior
Citation: Sentil A, Wood TJ, Lhomme P, Hamroud L, El Abdouni I, Ihsane O, Bencharki Y, Rasmont P, Christmann S and Michez D (2022) Impact of the “Farming With Alternative Pollinators” Approach on Crop Pollinator Pollen Diet. Front. Ecol. Evol. 10:824474. doi: 10.3389/fevo.2022.824474
Received: 29 November 2021; Accepted: 28 February 2022;
Published: 24 March 2022.
Edited by:
Isabel Marques, University of Lisbon, PortugalReviewed by:
Stefan Dötterl, University of Salzburg, AustriaCopyright © 2022 Sentil, Wood, Lhomme, Hamroud, El Abdouni, Ihsane, Bencharki, Rasmont, Christmann and Michez. This is an open-access article distributed under the terms of the Creative Commons Attribution License (CC BY). The use, distribution or reproduction in other forums is permitted, provided the original author(s) and the copyright owner(s) are credited and that the original publication in this journal is cited, in accordance with accepted academic practice. No use, distribution or reproduction is permitted which does not comply with these terms.
*Correspondence: Ahlam Sentil, YWhsYW0uc2VudGlsQHVtb25zLmFjLmJl
Disclaimer: All claims expressed in this article are solely those of the authors and do not necessarily represent those of their affiliated organizations, or those of the publisher, the editors and the reviewers. Any product that may be evaluated in this article or claim that may be made by its manufacturer is not guaranteed or endorsed by the publisher.
Research integrity at Frontiers
Learn more about the work of our research integrity team to safeguard the quality of each article we publish.