- 1Conservation Ecology Center, Smithsonian Conservation Biology Institute, Front Royal, VA, United States
- 2Smithsonian Migratory Bird Center, Smithsonian Conservation Biology Institute, Washington, DC, United States
In grassland ecosystems, grazing by large herbivores is a highly influential process that affects biodiversity by modifying the vegetative environment through selective consumption. Here, we test whether restoration of bison is associated with increased bird diversity and cervid occupancy in networks of riparian habitat within a temperate grassland ecosystem, mixed-grass prairie in northcentral Montana, United States. We used a long time-series of remote sensing imagery to examine changes in riparian vegetation structure in stream networks within bison and cattle pastures. We then assessed how vegetation structure influenced diversity of bird communities and detection rates of mammals in these same riparian networks. We found that percent cover of woody vegetation, and native grasses and forbs increased more rapidly over time in bison pastures, and that these changes in vegetation structure were associated with increased bird diversity and cervid occupancy. In conclusion, bison reintroduction appears to function as a passive riparian restoration strategy with positive diversity outcomes for birds and mammals.
Introduction
Restoration of evolutionary grazing processes, those that replicate or mimic effects of native herbivores, is a common goal of restoration efforts in temperate grassland systems (Freese et al., 2014; Fuhlendorf et al., 2018). Grazing is a ubiquitous natural process that creates and maintains habitat for myriad grassland species (Milchunas et al., 1988; Gao and Carmel, 2020). Whereas native grazers are the preferred option for restoration, in nearly all temperate grassland systems, native grazers have been replaced with cattle (Bos taurus) (van Zanten et al., 2016), which are raised for milk, meat or other animal products. When managed sustainably, cattle grazing can provide the disturbance regimes and vegetation heterogeneity necessary for diverse grassland systems (Milchunas et al., 1998; Porensky et al., 2020; Boyce et al., 2021). Furthermore, sustainable cattle grazing maintains healthy soils and resilient plant communities, resulting in more intact ecosystems than row-crop agriculture, which is the primary alternative land use in many grasslands (Krausman et al., 2009; da Silva et al., 2015). Restoration efforts in temperate grassland systems in North America have often focused on the re-introduction of the native megaherbivore; plains bison (Bison bison bison) (Freese et al., 2014). There is some evidence for biodiversity benefits of bison reintroduction, including increased diversity in plants (McMillan et al., 2018) and increased abundance of some grassland obligate songbirds (Boyce et al., 2021) but opportunities to evaluate its biodiversity impacts are rare (but see Allred et al., 2013; Nickell et al., 2018). Furthermore, the bison reintroduction process is both expensive (Carbyn and Watson, 2001) and controversial (Ranglack et al., 2015), so it is critical to evaluate whether these efforts are resulting in increasingly diverse and resilient ecosystems.
Most comparisons between bison and cattle have focused on differences in biodiversity or vegetation structure in upland grasslands (Greibel et al., 1998; Lueders et al., 2006; Moran, 2014; McMillan et al., 2018; Nickell et al., 2018). However, the largest behavioral differences between these species is their use of wetlands and associated woody vegetation (Kohl et al., 2013). There are several ecological and physiological differences between bison and cattle that support the hypothesis that their divergent grazing patterns and habitat preferences will differentially affect riparian systems. Cattle are known to degrade riparian areas (Kauffman and Krueger, 1984; Fleischner, 1994) due to damage or removal of riparian woody vegetation; effects that cascade into degradation of water quality and large fluctuations in stream temperatures and biogeochemistry (Larson et al., 2019). In contrast, bison are more drought and heat-tolerant, allowing them to graze farther from water, especially in hot conditions (Allred et al., 2013; Kohl et al., 2013). Compared with cattle, bison select against areas with woody vegetation and standing water, spend less time browsing, and specialize more on grasses, as opposed to forbs or woody vegetation (Peden et al., 1974; Knapp et al., 1999; Steuter and Hidinger, 1999; Allred et al., 2011; Kohl et al., 2013; Ranglack and du Toit, 2015).
The above differences predict replacing cattle with bison will have net positive impacts on quantity and complexity of riparian vegetation, but this hypothesis is largely untested. Furthermore, we predict that increases in riparian vegetation will be associated with increased diversity of bird communities (MacArthur and MacArthur, 1961; Macarthur, 1964; Cooper et al., 2020). We also predict that deer use will increase with higher shrub and tree cover after accounting for distance to the Missouri River drainage, a forested landscape that serves as a source population for cervids. Here we combine contemporary data on vegetation structure, bird community diversity and ungulate occupancy, with a long-term remotely sensed vegetation dataset to test whether bison reintroduction in a mixed-grass prairie ecosystem has resulted in higher quality riparian habitat in comparison with areas seasonally grazed by cattle.
Materials and Methods
Study Area
We studied vegetation and animal communities associated with ephemeral streams on the northwest glaciated plains subregion of the Northern Great Plains ecosystem (Forrest et al., 2004). Our study area included parts of Blaine, Phillips and Valley counties bounded by the Milk River in the north, the Missouri River in the south and the western boundary of the Fort Belknap Indian Reservation on the west (Figure 1). Land ownership is a mix of private lands concentrated in the vicinity of permanent water and alluvial soils, with large blocks of public land composed of mixed-grass prairie or sage steppe. The Charles M. Russell National Wildlife Refuge is an exception, as it contains nearly 1 million acres of rugged “breaks” canyons with conifer (Pinus ponderosa and Juniperus scopulorum) savannah and includes the extensive riparian bottomlands of the Missouri River. In the uplands, conversion from native grassland to row-crop agriculture is ongoing, and conversion of riparian vegetation to hay fields for cattle forage is widespread (Gage et al., 2016).
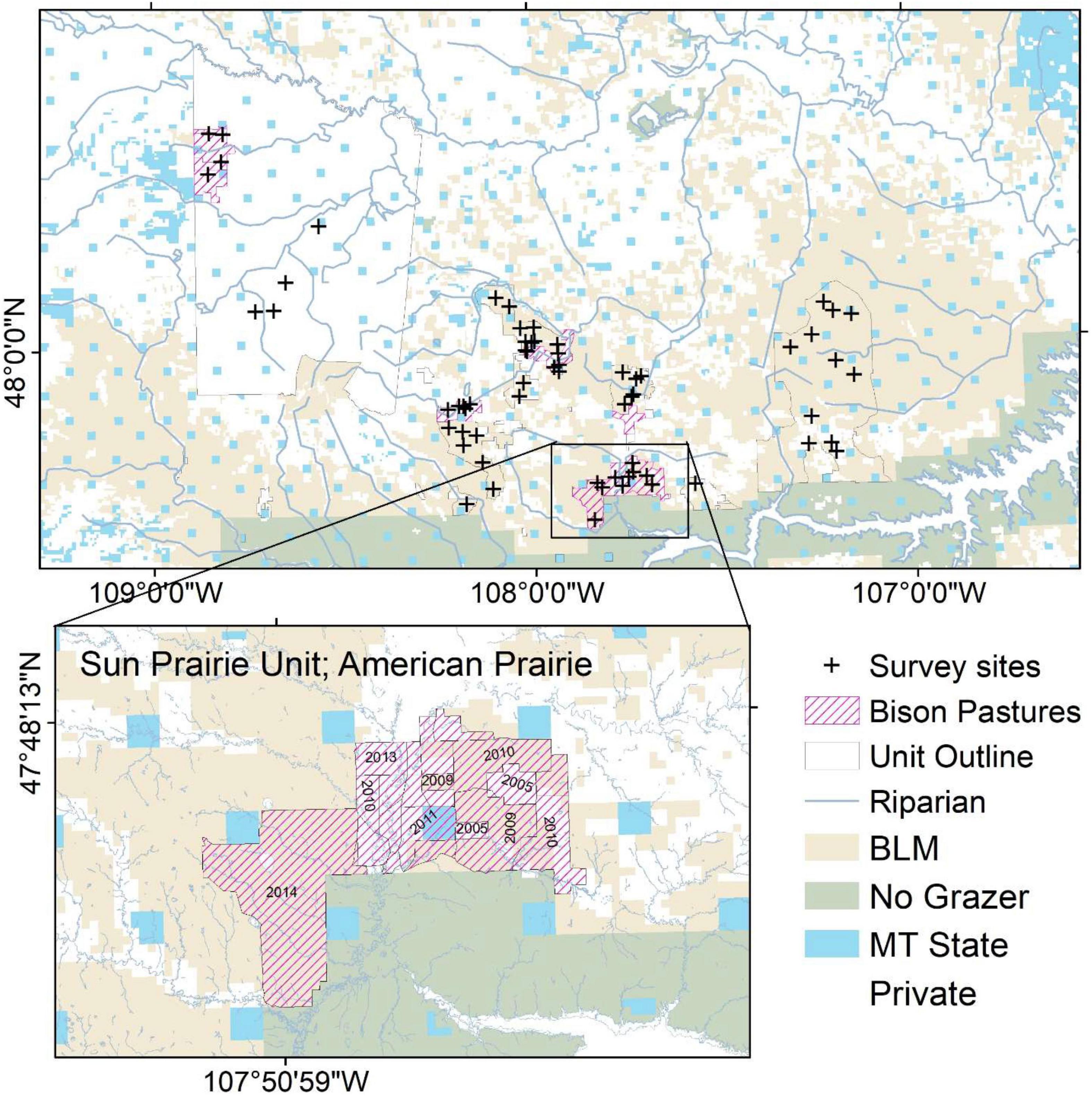
Figure 1. Survey sites. Unit outlines include Fort Belknap Indian Reservation and American Prairie lands.
The study area contains many waterways, from small ephemeral streams which innervate the expansive uplands, to the Missouri River, one of the largest in North America. The small seasonal or ephemeral streams are isolated strands of riparian vegetation amidst large expanses of grassland or sage steppe. Typical riparian vegetation in the region includes woody shrubs like common snowberry (Symphoricarpos albus), sandbar willow (Salix exigua), and wild rose (Rosa spp.). Common tree species are eastern cottonwood (Populus deltoides), box elder (Acer negundo), and peach-leaf willow (Salix amygdaloides). In heavily grazed areas, woody vegetation can be entirely absent, with low-growing grasses and forbs found up to the channel edge.
American Prairie is a private non-governmental organization with the mission to create the largest nature reserve in the lower 48 states1. American Prairie owns 423 km2 of private land and holds the grazing leases for an additional 1,275 km2 acres of public land as of 2021. The goal of American Prairie is to manage its lands as a fully-functioning grassland ecosystem complete with keystone grazers (bison and black-tailed prairie-dogs Cynomys ludovicianus) fulfilling their ecological role (Knapp et al., 1999; Freese et al., 2018). From 2005 through 2021, American Prairie has reintroduced bison to three large parcels of either private land or a mixture of private land and leased grazing allotments managed by the Bureau of Land Management (BLM; Freese et al., 2018; Boyce et al., 2021). Bison herds vary in size; approximately 150, 200, and 400 animals within 2,349, 2,963, and 10,909 ha pastures as of 2021. These herds have corresponding stocking rates of 0.77, 0.81, and 0.44 AUMs per hectare, which correspond to normal-year precipitation estimates calculated by BLM staff for public grazing allotments and by a private contractor (EMPSi Inc., Boulder, CO, United States), and conform to NRCS methodology for private parcels. Bison populations are regulated by public hunting opportunities, donations of animals to other conservation herds, and temporary chemical contraception (Freese et al., 2018). The Aaniiih and Nakoda peoples, who live at Fort Belknap Community, also maintain a large conservation herd of bison (∼900 individuals) on its 8,903 ha Snake Butte pasture. This pasture has a substantially higher stocking rate (1.21 AUMs per hectare) as those managed by American Prairie and bison were introduced nearly 30 years ago (Shamon et al., 2022). All bison herds are managed via continuous grazing, in which bison move freely within each grazing allotment throughout the year.
Cattle pastures within our study area were managed via deferred rotation grazing (Rhodes, 2020, BLM, personal communication). Deferred rotation refers to a management regime where allotments are divided into 3–4 pastures using barbed-wire fence, and cattle are moved sequentially through all pastures over the course of each grazing season (March–November). During winter, cattle are removed from public lands grazing allotments and sold or fed overwinter on private lands. Because private lands experience winter grazing, we separated BLM and private (Non-BLM) cattle pastures for our analysis. Cattle pastures in the region have been managed consistently using this protocol for 10+ years (Rhodes, 2020, BLM, personal communication). Cattle stocking rates on BLM lands are dictated by the same NRCS methodology used to determine bison stocking rates on both private and BLM lands. Specific stocking rates are variable over time in response to precipitation and across space in response to small differences in soil productivity. Because bison and cattle stocking rates are determined by the same methodology, we consider the effective stocking rates as similar, with the key difference that bison numbers per unit area are lower because their AUMs are distributed across a 12-month period as opposed to a shorter growing season grazing period in cattle. There are two exceptions to this principle within our study area. First, bison stocking rate on the Snake Butte pasture on the Fort Belknap Indian Reservation is substantially higher than on American Prairie bison pastures and BLM cattle pastures (Boyce et al., 2021; Shamon et al., 2022). Second, cattle stocking rates on private lands are variable depending on economic decisions by individual ranchers and details are not publicly available.
Vegetation
Current Differences in Vegetation Cover Amongst Treatments
We compared current vegetation cover proportions (2019) between treatments using a logistic regression with logit-link function; f(vegcover)∼Treatment (Warton and Hui, 2011). Intercept was set to BLM cattle, the most common form of management in the study region. We ran 8 model combinations (2 distance categories × 4 vegetation types). Current vegetation cover estimates were derived from Range Analysis Platform (RAP) Vegetation Cover Dataset 2019 at 30 m resolution (Allred et al., 2021).
We assessed riparian vegetation structure across four general treatments: BLM cattle, private lands cattle, bison, and no bovine grazers. Bison pastures were then subcategorized according to the year at which bison were reintroduced to the pasture (2005, 2009, 2010, 2011, 2012, 2013, 2014), creating 10 total grazing treatments. First, we identified riparian segments using the National Hydrography Dataset (Moore et al., 2019). Next, we created two distance category buffers around streams: (1) 0 m from stream (e.g., within streambed); (2) <30 m from stream edge. Distance categories were chosen at 30 m scale which matches the resolution of the vegetation cover data used for the analysis. Distance categories highlight the gradient effects of grazing treatment on vegetation. Next, we extracted vegetation cover percentages for each pixel centroid that fell within the two distance categories of a streams using Google Earth Engine (Gorelick et al., 2017).
Long-Term Vegetation Trends
We assessed the direction and rate of change of vegetation cover using RAP Vegetation Cover Dataset 2000–2019 time-series (Allred et al., 2021). We computed a pixel-wise Mann–Kendall rank correlation and estimated Theil-Sen’s slope for four vegetation types. Calculations were done using the “rkt” package (Marchetto, 2017) available from CRAN (R Core, 2018). Mann–Kendall rank correlation is a non-parametric test that is considered resilient to outliers and combined with Theil-Sen’s slope has proven to be a successful predictor for time-series analysis such as vegetation and climate trends (Gocic and Trajkovic, 2013; Li et al., 2013). Mann–Kendall test provides an assessment of slope estimate uncertainty, and the slope indicates the rate of change and the direction of the trend where positive slope means increase in vegetation cover and negative slope means reduction. Overall, we calculated vegetation tends for 80 combinations of grazing treatment (10) × distance category (2) × vegetation type (4). Vegetation types included: (1) annual forbs and grasses; (2) perennial forbs and grasses; (3) shrubs; and (4) tree cover.
We modeled current vegetation cover and time-series slope estimates to determine differences between BLM cattle and the other nine treatments. The analysis was conducted at two distance categories, and four vegetation types separately. We only used estimates with significance level of <0.05 in this analysis. Slope estimates were used as a response variable against treatment categories using a generalized linear model framework (GLM); “stats” R base package (R Core, 2018). Intercept was set to BLM cattle grazed (most common form of management in the study region) and we ran 8 model combinations (2 distance categories × 4 vegetation types).
Vertebrate Surveys
Occupancy of Grazers and Browsers
We detected ungulates with camera traps (model Reconyx HyperFire 2) during two growing seasons (July–October 2018, May–September 2019). We deployed camera traps at 78 riparian sites. We placed three cameras in each survey site spaced 250–400 m apart. Cameras were set at 50 cm above ground, facing north to minimize false triggers induced by direct sun exposure. Images were sorted, identified to species, and stored in the eMammal repository (Shamon, 2021). We collected habitat data at each camera location to assess detection bias. These data included the percentage of ground cover vegetation (bare ground, grass, forb, and shrub), percentage of canopy cover, mean shrub height within 5 m in front of each camera, distance at which the camera sensor was triggered in response to an approaching human, and whether or not the camera was set on an obvious animal trail. Percent cover class and mean shrub height were estimated visually. Riparian sites were used to model cervid occupancy in relation to vegetation cover and structure, and these data were used to compare cattle and bison activity with the riparian area. We only used camera deployments which functioned for ≥7 days, which resulted in 213 deployments in the riparian area for deer species. For the bison and cattle models, we eliminated deployments from areas where no cattle were present in the pasture due to rotation to other sub-pastures or removal from the pasture entirely, resulting in 198 deployments (107 bison and 91 cattle).
To compare between bison and cattle activity at streams we used detections counts for each species. Detection counts were modeled using N-mixture models, a family of models that can estimate counts while accounting for imperfect detection (Royle, 2004; Joseph et al., 2009; Zhou and Carin, 2015). Cattle and bison densities in the tested area remained steady during the study period, therefore we do not expect bias due to population fluctuations. Model calculations were done in a two-step process where first we identified the variables that affect detection probability (binominal distribution; see detection covariates above). Detection models were ranked by Akaike information criterion (AIC) and the best fitting model was used in each N-mixture model combination (count model using Poisson distribution). We modeled both species together using “species” as a categorical covariate to learn about the difference in activity by streams, and bison was set as an intercept. We assumed detection probability would be the same for bison and cattle given the similarity of body size and the consistency of camera setups, therefore detection was modeled for both species together.
We estimated occupancy for three deer species (mule deer; Odocoileus hemionus, white-tailed deer; Odocoileus virginianus, and elk; Cervus canadensis) in riparian habitats (MacKenzie et al., 2002). We used presence-absence data instead of detection counts to avoid bias of population fluctuations between years. Model calculations were done in a two-step process where first we identified the variables that affect detection probability (binominal distribution; see detection covariates above). Detection models were ranked by AIC and the best fitting model was used in each occupancy model. Second, we modeled deer presence-absence data against proportion of vegetation cover within 100 m buffer around the camera location derived from both the RAP vegetation cover dataset and the distance from major rivers. Different combinations were tested and competing models were ranked by AIC score for each species and we considered models with <2 delta AIC as equivalent.
Bird Diversity
To characterize riparian bird communities we conducted 10-min 200 m fixed-radius point counts laid out along ephemeral or seasonal streams such that the center points of each point count were at least 500 m from the nearest neighbor point count location to avoid double-counting (Hutto et al., 1986). Each cell was visited once per field season between May 30 and July 3, in 2018 and in 2019. All birds seen or heard were recorded and estimated distance and bearing to each individual was recorded to help prevent double-counting. Point counts began at 30 min before sunrise and all counts were completed prior to 8 am to minimize variation in detectability related to time of day. Following Hutto et al. (1986), point counts were not conducted during strong wind or precipitation. We aimed to describe communities of birds associated with riparian habitats, so we removed grassland obligate species under the assumption they were detected in adjacent grassland or sage steppe. We also removed species only detected as flyovers which we could not safely assume were using habitat in the point count area (primarily raptors and waterfowl).
We used three diversity metrics to describe the bird community at each survey location: species richness (SR), Faith’s phylogenetic diversity (Faith, 1992; hereafter PD), and functional richness (Mason et al., 2005; Villéger et al., 2008, hereafter FRic). Species richness is simply the number of species detected at a given site. PD describes the total phylogenetic breadth of a community, calculated as the sum of all branch lengths in a phylogenetic tree including all species in a community. Under the general assumption that ecological roles are phylogenetically conserved, PD should be a proxy for overall niche space encompassed by a given community. FRic is analogous to PD, but distance among species is not defined by their phylogenetic relatedness, but by how similar or different they are based on a variety of functional traits related to a species’ ecology (Villéger et al., 2008).
To facilitate calculation of PD, we downloaded a subset of 1,000 trees from www.birdtree.org (Jetz et al., 2012) containing all riparian species detected on our counts. We produced a consensus tree and estimated all branch lengths using the “consensus.edges” function in the phytools package (Revell, 2012). To estimate FD, we compiled seven functional traits for each species from the literature. Six of seven traits were morphological measurements linked to locomotion and diet: wing chord, tail length, tarsus length, bill length, hand-wing index, and body mass (Miles and Ricklefs, 1984, 1994; Pigot et al., 2020). We also compiled information on the primary diet of each species, classified into one of six categories: invertebrates, vertebrates, seeds, fruits, plants, and omnivores. Hand-wing index, body mass, and diet data were sampled from a comprehensive dataset compiled by Sheard et al. (2020). All other morphological traits were compiled from a large dataset of passerine morphology (Ricklefs, 2017), Cornell’s Birds of the World (Billerman et al., 2020) or references therein. Body mass values were log-transformed before analyses and all analyses were conducted in R v. 3.5.1 “Feather Spray” (R Core Team, 2015).
Phylogenetic diversity and species richness were calculated using the “pd” function in the package “picante” (Kembel et al., 2010). PD can only be estimated for communities of at least two species, so we assigned a PD value of 0 to communities of only one species. FRic was calculated using the “dbFD” function in the package “FD” (Laliberte and Legendre, 2010). FRic can only be estimated for communities containing at least three species, so we assigned a FRic value of zero to all communities with fewer than three species (1 site).
To describe associations between riparian vegetation and bird communities we combined data from the remotely sensed RAP platform (Allred et al., 2021) with our riparian point-count data. We fitted generalized linear models with each diversity metric (SR, PD, and FRic) as the response variable, and three of the four vegetation metrics (tree cover, shrub cover, perennial grass/forb cover) as explanatory variables. Since percentages must add to 100, the fourth category, annual grass/shrub, was excluded from the model as redundant and the parameter estimated for each included category are in comparison to annual grass/forb. Models explaining variation in raw species richness values were fit using a Poisson distribution while all other models used a Gaussian distribution.
Results
Contemporary Differences in Vegetation
We found perennial grass/forb cover was significantly higher in private cattle grazed pastures and bison pastures in areas at, or closer to, the stream (category 0 m and <30 m; excluding 2014 bison pastures; Figure 2). In contrast, annual grass/forb cover was significantly higher at BLM cattle streams; consistent for categories 0 m and <30 m (Figure 2). Differences in shrub cover were variable between BLM cattle and bison pastures but were significantly lower at private cattle pastures and higher in the no-grazer area (Figure 2). Differences in tree cover were variable between BLM cattle and bison pastures with a tendency to be higher at bison pastures and were significantly higher at private cattle pastures and the no-grazer area (Figure 2).
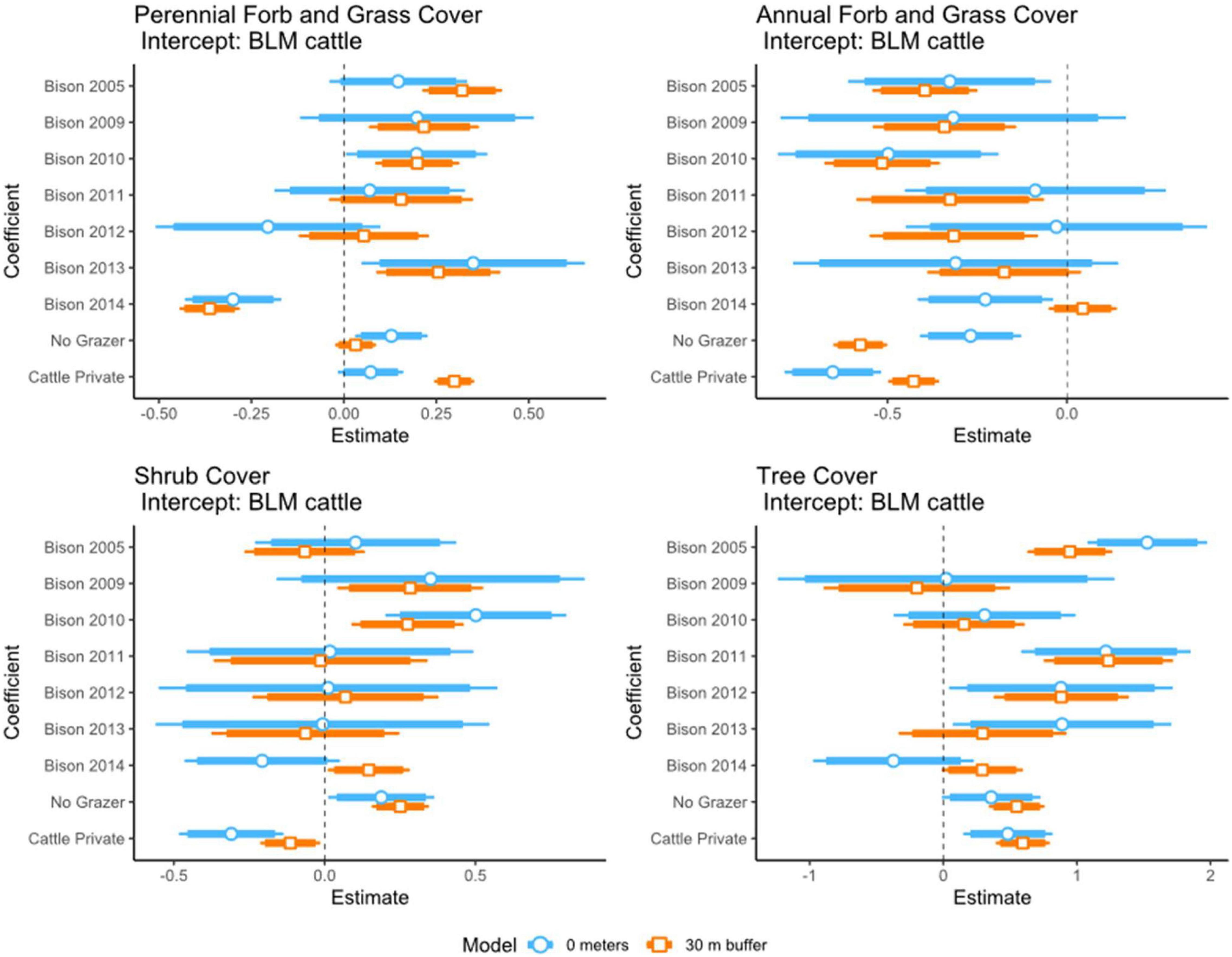
Figure 2. 2019 percentage of vegetation cover in relation to grazing treatment. Intercept: BLM cattle grazed streams. Vegetation: perennial forbs and grasses, annual forbs and grasses, shrub cover, and tree cover. Treatment: BLM cattle, Bison (2005, 2009, 2010, 2011, 2012, 2013, 2014), no grazers, private lands cattle. Models were run for two distance categories: (1) 0 m from stream; (2) <30 m from stream edge.
Long-Term Vegetation Trends
We found perennial grass/forb cover trends were significantly higher in bison pastures at both distance categories and no-grazer areas for distance categories <30 m (Figure 3). At stream (0 m) results were variable with a tendency to be higher than BLM cattle (Figure 4); the opposite trend was observed for private cattle pastures. Annual grass/forb cover trends were variable with a tendency to be higher for 0 m at bison pasture and lower for <30 m at bison and no-grazer areas (Figure 3). Annual grass/forb cover trends were significantly higher at private cattle pastures. Differences in shrub cover trends were variable between BLM cattle and bison pastures but were significantly lower at private cattle pastures and higher in no-grazer areas (Figure 3). Differences in tree cover trends were variable between BLM cattle and bison pastures with tendency to be higher at bison pastures and were significantly higher at private cattle pastures and no-grazer areas (Figure 3).
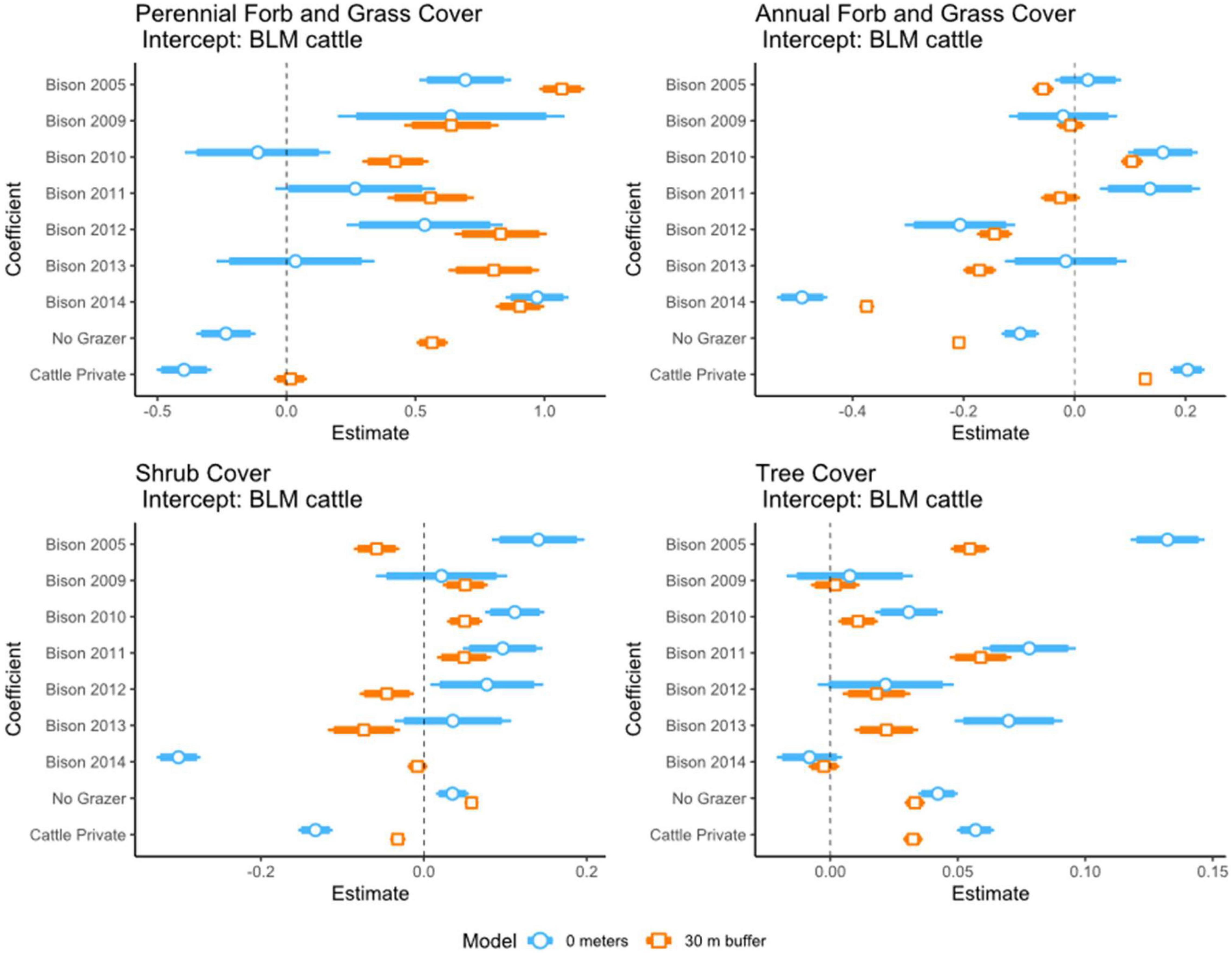
Figure 3. Long-term vegetation trend estimates (slope) in relation to grazing treatment. Intercept: BLM cattle grazed streams. Vegetation: perennial forbs and grasses, annual forbs and grasses, shrub cover, and tree cover. Treatment: BLM cattle, Bison (2005, 2009, 2010, 2011, 2012, 2013, 2014), no grazers, private lands cattle. Models were run for two distance categories: (1) 0 m from stream; (2) <30 m from stream edge.
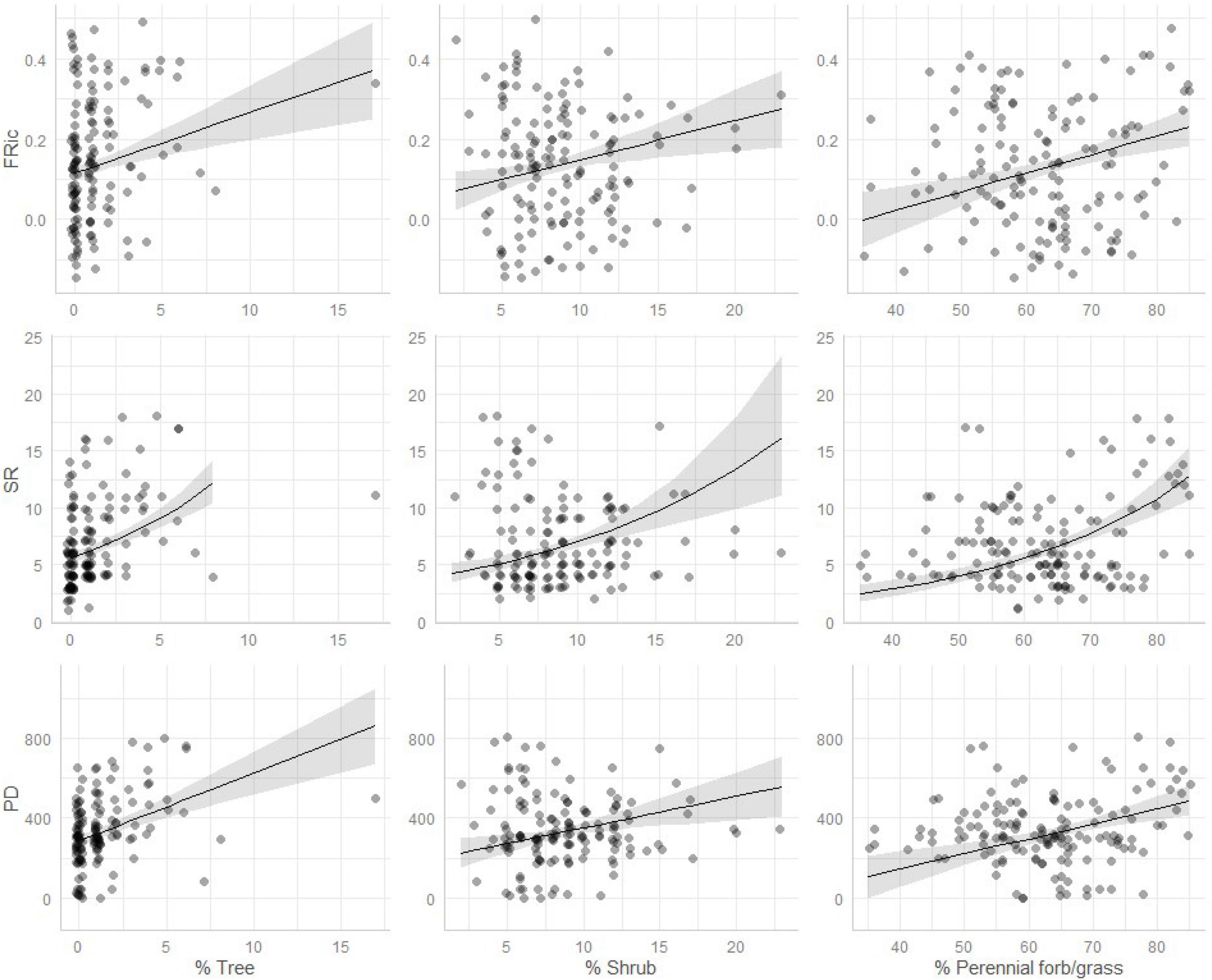
Figure 4. Marginal relationships between three components of remotely sensed vegetative cover and three metrics riparian bird community diversity; functional richness (FRic), species richness (SR), and Faith’s phylogenetic diversity (PD). Dots represent individual samples, lines and shading represent predicted relationships and associated 95% confidence intervals. All three vegetative components shown here are increasing in percent cover most rapidly in areas grazed by bison and areas with no grazers. Vegetation cover data are estimated from the RAP platform.
Ungulate Occupancy
Comparison of Cattle and Bison Detection Counts
The mean and standard deviation of cattle and bison detections per 100 camera nights at riparian cameras were 106.8 ± 376.7 and 40.0 ± 107.9, respectively (number of camera deployments: ncattle = 91 and nbison = 107). Because cattle graze seasonally and are rotated among multiple pastures, they were not always present during camera surveys. Accordingly, the data were subset to include only times when cattle were present, resulting in mean of 255.7 ± 553.2 cattle detections per 100 camera nights (number of camera deployments: n = 38). Modeling the full dataset that included times when cattle were not present at the pasture show there are no significant differences between bison and cattle overall activity in riparian areas (Table 1). However, modeling the dataset that only contained deployments when cattle were present in the pasture containing the camera deployment revealed that cattle are significantly more active in riparian areas than bison (coefficient = 1.4 ± 0.1 where bison category was set as the intercept; Table 1A).
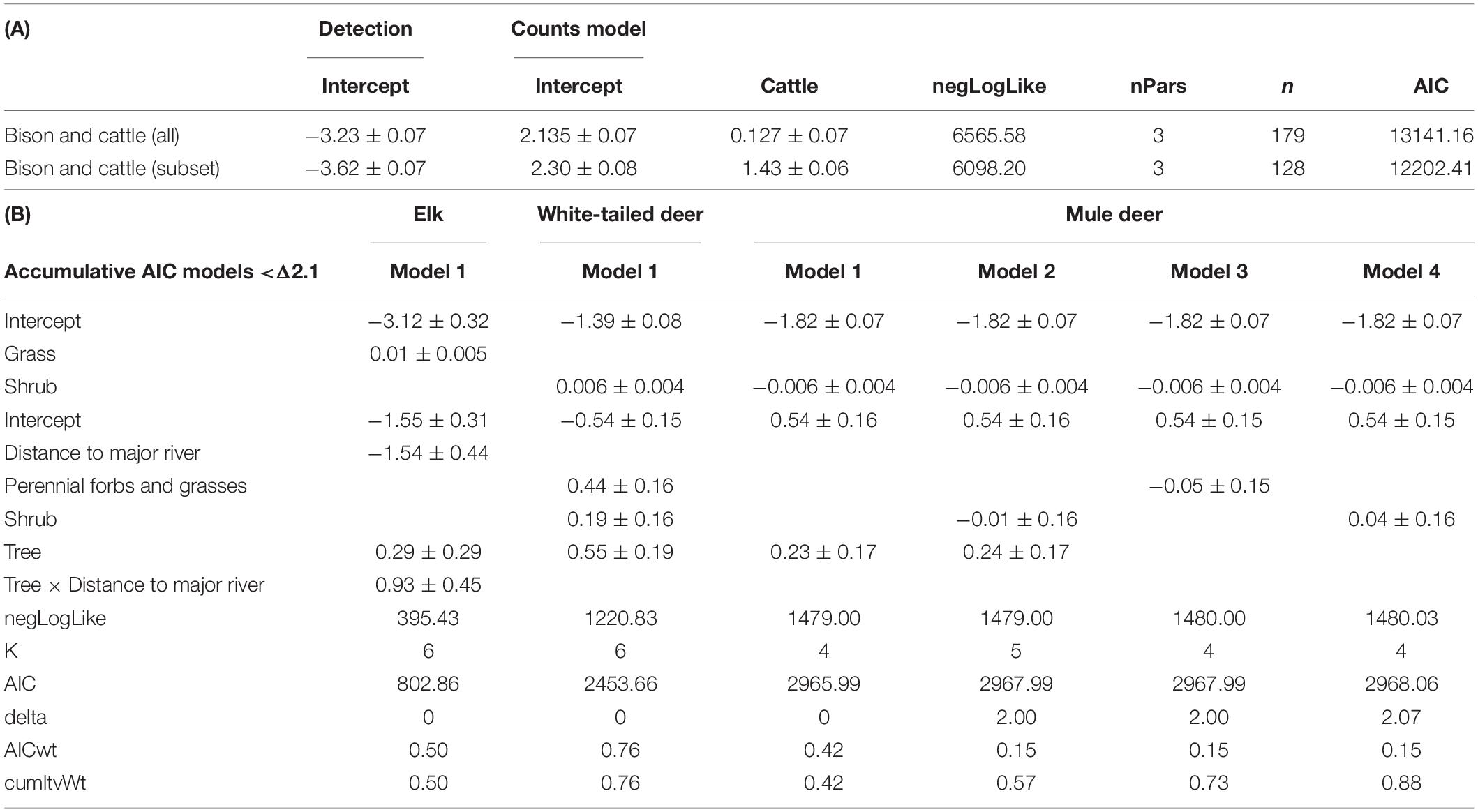
Table 1. (A) Bison and cattle count model including the full dataset (all) and a subset that only includes deployments when cattle were active. (B) Occupancy estimation for deer species in relation to vegetation cover within a 100 m buffer around a camera and distance to major rivers.
Deer Occupancy in Relation to Riparian Vegetation Cover
Elk occupancy was primarily determined by distance to major rivers and the interaction between woody cover and distance to major rivers (Table 1B). Mule deer occupancy was unrelated to distance to rivers and did not show any specific relationship with vegetation cover (Table 1B). White-tailed deer occupancy increased with higher tree cover and higher perennial grass/forb cover (Table 1B).
Bird Diversity
We conducted 147 point-counts along prairie streams (Figure 1) and detected 59 species (mean species richness per count = 6.9; range 1–18; Supplementary Material). All three metrics of riparian bird community diversity (SR, PD, and FRic) increased with increasing tree cover, shrub cover, and perennial grass/forb cover in comparison with annual grass cover (Table 2 and Figure 4). Across all three metrics tree cover had the largest positive effect on diversity, followed by woody shrub cover and finally perennial grass/forb (Table 2 and Figure 4).
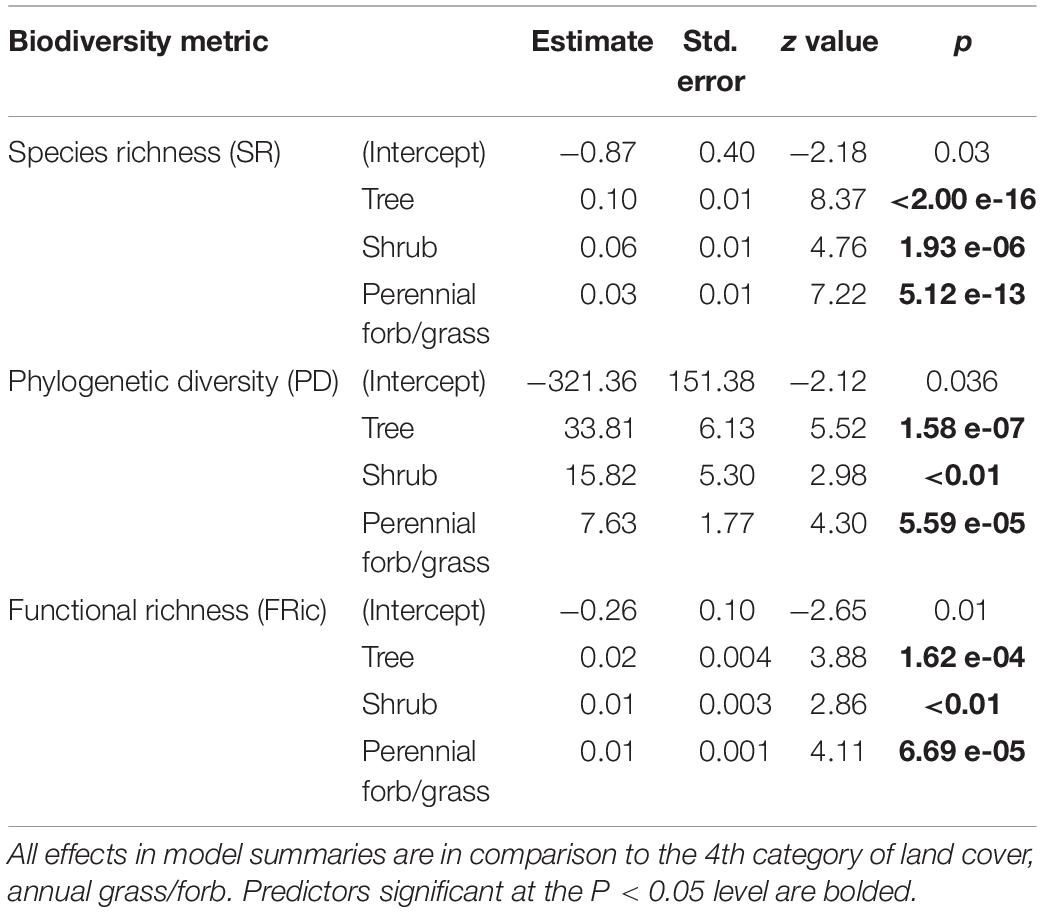
Table 2. Models describing the relationship between raw and fitted values of avian community diversity and remotely sensed vegetation metrics from the Rangeland Analysis Platform (Allred et al., 2021).
Discussion
Reintroduction of bison and replacement of cattle with bison significantly impacted riparian systems in our study area. We found that streams in pastures grazed year-round by bison had a faster rate of increase of perennial grass/forb cover, as well as shrub and woody cover, when compared to streams in seasonally grazed cattle pastures. These trends are supported by camera trap records in streams that show cattle are detected significantly more within streams than bison and that activity is concentrated in time due to the rotation schedule. These results are consistent with previous studies showing that bison select against low-elevations and woody vegetation compared with cattle, and that bison forage farther from water (Knapp et al., 1999; Allred et al., 2011; Kohl et al., 2013; Ranglack and du Toit, 2015). We used remote sensing to document riparian area changes, but a ground-based study in the same area comparing vegetation plots within bison and cattle pastures found similar results of increased plant diversity and increased shrub cover within riparian areas of bison pastures (Yu, 2021). Furthermore, we show that streams with more trees and woody shrubs, and higher percent cover of perennial grasses and forbs relative to non-native annuals are associated with more diverse breeding bird communities and have higher rates of occupancy for two of three species of native ungulates (the exception was mule deer). The idea that greater amounts and complexity of vegetation drives increased bird diversity is hardly a new result (see Macarthur, 1964), but taken together, our results suggest that bison reintroduction, and a minimal intervention grazing management strategy, is associated with positive biodiversity outcomes in riparian habitats found within the Northern Great Plains.
Negative impacts of cattle grazing on riparian systems are well documented, particularly intense in arid regions (Fleischner, 1994; Belsky et al., 1999), negatively impact imperiled species (Ohmart, 1994; Wilcove et al., 1998; USFWS, 2002, 2020; Dettenmaier et al., 2017), and may increase in severity with climate change (Allred et al., 2013). Cattle grazing has been shown to have negative effects on riparian breeding bird communities in Montana (Fletcher and Hutto, 2008) and across the west (Tewksbury et al., 2002). In forested systems, removal of domestic grazers may present a suitable remedy for this issue since forest succession does not rely on intense grazing by bovines as a primary source of disturbance (Hessburg et al., 2019). Because of their ecological and physiological coevolution with arid grassland systems, bison are ideally suited to grassland ecosystems because they provide disturbance and thus heterogeneity in upland habitats (Gibson, 1989; McMillan et al., 2018), but have reduced impacts on riparian habitats, at least at agency standard stocking rates. Whether bison are reintroduced and managed as wild animals for ecosystem benefits, cultural benefits, or meat production, they are likely to provide ecosystem benefits if managed in a way that facilitates natural grazing patterns (Shamon et al., 2022).
Restoration in the Northern Great Plains will not only involve the introduction of large herbivores but also large carnivores. Due to their great mobility large carnivores can repopulate areas once given adequate movement corridors. Riparian systems are important movement corridors and refugia for dispersing, migrating, or resident wildlife (Machtans et al., 1996; Skagen et al., 2005). Linear features such as streams are used by both predator and prey species (Dickie et al., 2020) and forested riparian areas are key dispersal corridors for large mammals including species recolonizing grassland ecosystems in North America (Morrison et al., 2015; Gigliotti et al., 2019). Specifically, riparian corridors are used by black bears (Ursus americanus) for movement across grasslands in south-central United States (Gantchoff and Belant, 2017). Grizzly bears (Ursus arctos) use riparian areas to move through mixed use areas in British Columbia, Canada (McLellan and Hovey, 2001). Finally, mountain lions (Felis concolor) use riparian corridors to disperse between forest fragments (LaRue and Nielsen, 2008) and use riparian forest for dispersal through an agricultural/grassland matrix. The passive restoration of riparian corridors via bison reintroduction has the potential to increase landscape connectivity for large predators including grizzly bears and mountain lions which are actively recolonizing the Northern Great Plains, and a conservation network of riparian systems would benefit the movement of multiple mammal species (Fremier et al., 2015).
In addition to movement corridors, riparian areas can serve as seasonal habitat for many species, as evidenced by our breeding bird surveys. Intact riparian and mesic areas within a grassland or sage steppe matrix are of critical importance for sage grouse brood-rearing (Aldridge and Boyce, 2007; Donnelly et al., 2016), but are subject to damage via cattle grazing (Beck and Mitchell, 2000). Sage grouse conservation is of critical importance to multiple stakeholders in the western United States (Duvall et al., 2017), but habitat manipulations for their benefit do not always improve overall biodiversity measures (Carlisle et al., 2018). To this end, grazing with bison has the potential for positive conservation outcomes for sage grouse via reduced damage to wet habitats within a grassland or sage-steppe matrix.
Intact riparian systems in grasslands also buffer temperature extremes and may serve as thermal refugia during increasingly frequent and severe heat waves (Turunen et al., 2021). Increased native plant cover along stream banks increased stream stability in North Dakota grasslands (Hecker et al., 2019). Grassland streams with forested riparian buffers have increased abundance of aquatic insects (Wahl et al., 2013). A high diversity stream in a tall grass prairie system in Illinois saw some improvements (and no degradation) in stream quality following bison restoration (Vandermyde, 2017). In this way, year-round bison grazing in large pastures and with appropriate stocking rates, may facilitate increased climate resilience in grassland ecosystems.
Despite the potential ecological benefits of year-round bison grazing in comparison to seasonal cattle grazing, bison are not a singular solution to grassland conservation or restoration of the system. Bison constrained to small pastures or maintained at high stocking rates can certainly have negative effects on grassland biodiversity (Beschta et al., 2020) or individual species (Powell, 2006). Furthermore, upland bird communities are similar between seasonally grazed cattle and year-round bison (Boyce et al., 2021), meaning that exclusion of cattle from riparian zones within a grassland matrix may result in similar overall ecosystem health compared with bison pastures. True restoration efforts of prairie riparian areas must include restoration of beaver (Castor canadensis) as major engineers of riparian systems (Pollock et al., 1995; Hood and Bayley, 2008).
Domestic livestock grazing has negative biodiversity effects across trophic levels (Filazzola et al., 2020), but paradoxically, grassland ecosystems require disturbance from grazers to produce the diverse vegetative niches required for maximal biodiversity (Becerra et al., 2017). Bison appear to resolve this paradox due to reduced preference for riparian habitats and vegetation, along with increased heat and drought tolerance that facilitates grazing far from water resources (Allred et al., 2013; Kohl et al., 2013). The use of bison as a restoration tool may therefore be particularly effective as northern grasslands become increasingly hot and dry, such that their role as a keystone grazer not only supports biodiversity, but also increases resilience to climate change in one the world’s most imperiled ecosystems.
Data Availability Statement
The raw data supporting the conclusions of this article will be made available by the authors, without undue reservation.
Author Contributions
AB, HS, and WM formulated the idea. AB and HS conducted the fieldwork, performed the analyses, and wrote the first draft of the manuscript. All authors contributed to revisions of the manuscript.
Funding
This work was made possible by the generous support of John and Adrienne Mars.
Conflict of Interest
The authors declare that the research was conducted in the absence of any commercial or financial relationships that could be construed as a potential conflict of interest.
The authors declare that this study received funding from John and Adrienne Mars. The funder was not involved in the study design, collection, analysis, interpretation of data, the writing of this article or the decision to submit it for publication.
Publisher’s Note
All claims expressed in this article are solely those of the authors and do not necessarily represent those of their affiliated organizations, or those of the publisher, the editors and the reviewers. Any product that may be evaluated in this article, or claim that may be made by its manufacturer, is not guaranteed or endorsed by the publisher.
Acknowledgments
We would like to thank Curt Freese, Kyran Kunkel Daniel Kinka, and Randy Matchett for historical information and thoughtful input on study design. We thank the people of the Nakoda and Aaniiih Tribes for allowing us to work and study on their lands. We would like to thank Damien Austin at American Prairie, B. J. Rhodes with the BLM Malta Field Office, Harold Main from Fort Belknap Fish and Wildlife Department, and Randy Matchett at the Charles M. Russell NWR for assistance throughout the project. We would also like to thank Hunter Balog, Emily Card, Courtney Check, Andrew Dreelin, Janna Freeman, Alex Hahn, Andrew Hallberg, Randall Holmes, Elizabeth Opheim, Zoe Paraskevopolou, Antonia Prudholm, Dan Stoken, and Johnathan Stutzman for their expertise and perseverance during fieldwork.
Supplementary Material
The Supplementary Material for this article can be found online at: https://www.frontiersin.org/articles/10.3389/fevo.2022.821822/full#supplementary-material
Footnotes
References
Aldridge, C. L., and Boyce, M. S. (2007). Linking occurrence and fitness to persistence: habitat-based approach for endangered greater sage-grouse. Ecol. Appl. 17, 508–526. doi: 10.1890/05-1871
Allred, B. W., Bestelmeyer, B. T., Boyd, C. S., Brown, C., Davies, K. W., Duniway, M. C., et al. (2021). Improving Landsat predictions of rangeland fractional cover with multitask learning and uncertainty. Methods Ecol. Evol. 12, 841–849. doi: 10.1111/2041-210X.13564
Allred, B. W., Fuhlendorf, S. D., and Hamilton, R. G. (2011). The role of herbivores in great plains conservation: comparative ecology of bison and cattle. Ecosphere 2, 1–17. doi: 10.1890/ES10-00152.1
Allred, B. W., Fuhlendorf, S. D., Hovick, T. J., Elmore, R. D., Engle, D. M., and Joern, A. (2013). Conservation implications of native and introduced ungulates in a changing climate. Glob. Chang. Biol. 19, 1875–1883. doi: 10.1111/gcb.12183
Becerra, T. A., Engle, D. M., Fuhlendorf, S. D., and Elmore, R. D. (2017). Preference for grassland heterogeneity: implications for biodiversity in the great plains. Soc. Nat. Resour. 30, 601–612. doi: 10.1080/08941920.2016.1239293
Beck, J. L., and Mitchell, D. L. (2000). Influences of livestock grazing on sage grouse habitat. Wildl. Soc. Bull. 28, 993–1002. doi: 10.1016/j.jenvman.2019.01.085
Belsky, A. J., Matzke, A., and Uselman, S. (1999). Survey of livestock influences on stream and riparian ecosystems in the western United States. J. Soil Water Conserv. 54, 419–431.
Beschta, R. L., Ripple, W. J., Kauffman, J. B., and Painter, L. E. (2020). Bison limit ecosystem recovery in northern Yellowstone. Food Webs. 23:e00142. doi: 10.1016/j.fooweb.2020.e00142
Billerman, S. M., Keeney, B. K., Rodewald, P. G., and Schulenberg, T. S. (2020). Birds of the World. Ithaca, NY: Cornell Lab of Ornithology.
Boyce, A. J., Shamon, H., Kunkel, K., and McShea, W. J. (2021). Grassland bird diversity and abundance in the presence of native and non-native grazers. Avian Conserv. Ecol. 16:13.
Carbyn, L. N., and Watson, D. (2001). “Translocation of plains bison to wood buffalo national park: economic and conservation implications,” in Large Mammal Restoration: Ecological And Sociological Considerations On The 21st Century, eds D. S. Maehr, R. F. Noss, and J. L. Larkin (Washington D.C: Island Press), 189–205.
Carlisle, J. D., Chalfoun, A. D., Smith, K. T., and Beck, J. L. (2018). Nontarget effects on songbirds from habitat manipulation for greater sage-grouse: implications for the umbrella species concept. Condor 120, 439–455. doi: 10.1650/CONDOR-17-200.1
Cooper, W. J., McShea, W. J., Forrester, T., and Luther, D. A. (2020). The value of local habitat heterogeneity and productivity when estimating avian species richness and species of concern. Ecosphere 11:e03107. doi: 10.1002/ecs2.3107
da Silva, T. W., Dotta, G., and Fontana, C. S. (2015). Structure of avian assemblages in grasslands associated with cattle ranching and soybean agriculture in the Uruguayan savanna ecoregion of Brazil and Uruguay. Condor 117, 53–63. doi: 10.1650/CONDOR-14-85.1
Dettenmaier, S. J., Messmer, T. A., Hovick, T. J., and Dahlgren, D. K. (2017). Effects of livestock grazing on rangeland biodiversity: a meta-analysis of grouse populations. Ecol. Evol. 7, 7620–7627. doi: 10.1002/ece3.3287
Dickie, M., McNay, S. R., Sutherland, G. D., Cody, M., and Avgar, T. (2020). Corridors or risk? Movement along, and use of, linear features varies predictably among large mammal predator and prey species. J. Anim. Ecol. 89, 623–634. doi: 10.1111/1365-2656.13130
Donnelly, J. P., Naugle, D. E., Hagen, C. A., and Maestas, J. D. (2016). Public lands and private waters: scarce mesic resources structure land tenure and sage-grouse distributions. Ecosphere 7:e01208. doi: 10.1002/ecs2.1208
Duvall, A. L., Metcalf, A. L., and Coates, P. S. (2017). Conserving the greater sage-grouse: a social-ecological systems case study from the California-nevada region. Rangel. Ecol. Manag. 70, 129–140. doi: 10.1016/j.rama.2016.08.001
Faith, D. P. (1992). Conservation evaluation and phylogenetic diversity. Biol. Conserv. 61, 1–10. doi: 10.1016/0003-2697(75)90168-2
Filazzola, A., Brown, C., Dettlaff, M. A., Batbaatar, A., Grenke, J., Bao, T., et al. (2020). The effects of livestock grazing on biodiversity are multi-trophic: a meta-analysis. Ecol. Lett. 23, 1298–1309. doi: 10.1111/ele.13527
Fleischner, T. L. (1994). Ecological costs of livestock grazing in Western North America. Conserv. Biol. 8, 629–644. doi: 10.1046/j.1523-1739.1994.08030629.x
Fletcher, R. J., and Hutto, R. L. (2008). Partitioning the multi-scale effects of human activity on the occurrence of riparian forest birds. Landsc. Ecol. 23, 727–739. doi: 10.1007/s10980-008-9233-8
Forrest, S., Strand, H., Haskins, W. H., Freese, C., Proctor, J., and Dinerstein, E. (2004). Ocean of Grass: A Conservation Assessment for the Northern Great Plains. Bozeman, MT: Northern Plains Conservation Network.
Freese, C. H., Fuhlendorf, S. D., and Kunkel, K. (2014). A management framework for the transition from livestock production toward biodiversity conservation on great plains rangelands. Ecol. Restor. 32, 358–368. doi: 10.3368/er.32.4.358
Freese, C. H., Kunkel, K. E., Austin, D., and Holder, B. (2018). Bison Management Plan. Available online at: https://www.americanprairie.org/sites/default/files/APR_BisonPlan_062018.pdf.
Fremier, A. K., Kiparsky, M., Gmur, S., Aycrigg, J., Craig, R. K., Svancara, L. K., et al. (2015). A riparian conservation network for ecological resilience. Biol. Conserv. 191, 29–37. doi: 10.1016/j.biocon.2015.06.029
Fuhlendorf, S. D., Davis, C. A., Elmore, R. D., Goodman, L. E., and Hamilton, R. G. (2018). Perspectives on grassland conservation efforts: should we rewild to the past or conserve for the future? Philos. Trans. R. Soc. B Biol. Sci. 373:20170438. doi: 10.1098/rstb.2017.0438
Gage, A. M., Olimb, S. K., and Nelson, J. (2016). Plowprint: tracking cumulative cropland expansion to target grassland conservation. Gt. Plains Res. 26, 107–116. doi: 10.1353/gpr.2016.0019
Gantchoff, M. G., and Belant, J. L. (2017). Regional connectivity for recolonizing American black bears (Ursus americanus) in southcentral USA. Biol. Conserv. 214, 66–75. doi: 10.1016/j.biocon.2017.07.023
Gao, J., and Carmel, Y. (2020). A global meta-analysis of grazing effects on plant richness. Agric. Ecosyst. Environ. 302:107072. doi: 10.1016/j.agee.2020.107072
Gibson, D. J. (1989). Effects of animal disturbance on tallgrass prairie. Am. Midl. Nat. 121, 144–154. doi: 10.2307/2425665
Gigliotti, L. C., Matchett, M. R., and Jachowski, D. S. (2019). Mountain lions on the prairie: habitat selection by recolonizing mountain lions at the edge of their range. Restor. Ecol. 27, 1032—1040. doi: 10.1111/rec.12952
Gocic, M., and Trajkovic, S. (2013). Analysis of changes in meteorological variables using Mann-Kendall and Sen’s slope estimator statistical tests in Serbia. Glob. Planet. Change 100, 172–182. doi: 10.1016/j.gloplacha.2012.10.014
Gorelick, N., Hancher, M., Dixon, M., Ilyushchenko, S., Thau, D., and Moore, R. (2017). Google earth engine: planetary-scale geospatial analysis for everyone. Remote Sens. Environ. 202, 18–27. doi: 10.1016/j.rse.2017.06.031
Greibel, R. L., Winter, S. L., and Steuter, A. A. (1998). Grassland birds and habitat structure in sandhills prairie managed using cattle or bison plus fire. Gt. Plains Res. 8, 255–268.
Hecker, G. A., Meehan, M. A., and Norland, J. E. (2019). Plant Community Influences on Intermittent Stream Stability in the Great Plains. Rangel. Ecol. Manag. 72, 112–119. doi: 10.1016/j.rama.2018.08.002
Hessburg, P. F., Miller, C. L., Parks, S. A., Povak, N. A., Taylor, A. H., Higuera, P. E., et al. (2019). Climate, environment, and disturbance history govern resilience of western North American forests. Front. Ecol. Evol. 7:239. doi: 10.3389/fevo.2019.00239
Hood, G. A., and Bayley, S. E. (2008). Beaver (Castor canadensis) mitigate the effects of climate on the area of open water in boreal wetlands in western Canada. Biol. Conserv. 141, 556–567. doi: 10.1016/j.biocon.2007.12.003
Hutto, R. L., Pletschet, S. M., and Hendricks, P. (1986). A fixed-radius point count method for nonbreeding and breeding season use. Auk 103, 593–602. doi: 10.1093/auk/103.3.593
Jetz, W., Thomas, G. H., Joy, J. B., Hartmann, K., and Mooers, A. O. (2012). The global diversity of birds in space and time. Nature 491, 444–448. doi: 10.1038/nature11631
Joseph, L. N., Elkin, C., Martin, T. G., and Possingham, H. P. (2009). Modeling abundance using N-mixture models: the importance of considering ecological mechanisms. Ecol. Appl. 19, 631–642. doi: 10.1890/07-2107.1
Kauffman, J. B., and Krueger, W. C. (1984). Livestock impacts on riparian ecosystems and streamside management implications. A review. J. Range Manag. 37, 430–438. doi: 10.2307/3899631
Kembel, S. W., Cowan, P. D., Helmus, M. R., Cornwell, W. K., Morlon, H., Ackerly, D. D., et al. (2010). Picante: R tools for integrating phylogenies and ecology. Bioinformatics 26, 1463–1464. doi: 10.1093/bioinformatics/btq166
Knapp, A. K., Blair, J. M., Briggs, J. M., Collins, S. L., Hartnett, D. C., Johnson, L. C., et al. (1999). The keystone role of bison in North American tallgrass prairie. Bioscience 49, 39–50. doi: 10.2307/1313492
Kohl, M. T., Krausman, P. R., Kunkel, K., andWilliams, D. M. (2013). Bison versus cattle: are they ecologically synonymous. Rangel. Ecol. Manag. 66, 721–731. doi: 10.2111/REM-D-12-00113.1
Krausman, P. R., Naugle, D. E., Frisina, M. R., Northrup, R., Bleich, V. C., Block, W. M., et al. (2009). Livestock grazing, wildlife habitat, and rangeland values. Rangelands 31, 15–19. doi: 10.2111/1551-501X-31.5.15
Laliberte, E., and Legendre, P. (2010). A distance-based framework for measuring functional diversity from multiple traits. Ecology 91, 299–305. doi: 10.1890/08-2244.1
Larson, D. M., Dodds, W. K., and Veach, A. M. (2019). Removal of woody riparian vegetation substantially altered a stream ecosystem in an otherwise undisturbed grassland watershed. Ecosystems 22, 64–76. doi: 10.1007/s10021-018-0252-2
LaRue, M. A., and Nielsen, C. K. (2008). Modelling potential dispersal corridors for cougars in midwestern North America using least-cost path methods. Ecol. Modell. 212, 372–381. doi: 10.1016/j.ecolmodel.2007.10.036
Li, Z., Huffman, T., McConkey, B., and Townley-Smith, L. (2013). Monitoring and modeling spatial and temporal patterns of grassland dynamics using time-series MODIS NDVI with climate and stocking data. Remote Sens. Environ. 138, 232–244. doi: 10.1016/j.rse.2013.07.020
Lueders, A. S., Kennedy, P. L., and Johnson, D. H. (2006). Influences of management regimes on breeding bird densities and habitat in mixed-grass prairie: an example from north dakota. J. Wildl. Manage. 70, 600–606. doi: 10.2193/0022-541x(2006)70[600:iomrob]2.0.co;2
Macarthur, R. H. (1964). Environmental factors affecting bird species diversity. Am. Nat. 98, 387–397.
Machtans, C. S., Villard, M., and Hannon, S. J. (1996). Use of riparian buffer strips as movement corridors by forest birds. Conserv. Biol. 10, 1366–1379. doi: 10.1046/j.1523-1739.1996.10051366.x
MacKenzie, D. I., Nichols, J. D., Lachman, G. B., Droege, S., Andrew Royle, J., and Langtimm, C. A. (2002). Estimating site occupancy rates when detection probabilities are less than one. Ecology 83, 2248–2255. doi: 10.1890/0012-9658(2002)083[2248:esorwd]2.0.co;2
Marchetto, A. (2017). Rkt: Mann-Kendall Test, Seasonal and Regional Kendall Tests. Available online at: https://cran.r-project.org/package=rkt.
Mason, N. W. H., Mouillot, D., Lee, W. G., and Wilson, J. B. (2005). Functional richness, functional evenness and functional divergence: the primary components of functional diversity. Oikos 111, 112–118. doi: 10.1111/j.0030-1299.2005.13886.x
McLellan, B. N., and Hovey, F. W. (2001). Habitats selected by grizzly bears in a multiple use landscape. J. Wildl. Manage. 65, 92–99. doi: 10.2307/3803280
McMillan, N. A., Kunkel, K. E., Hagan, D. L., and Jachowski, D. S. (2018). Plant community responses to bison reintroduction on the Northern Great Plains, United States: a test of the keystone species concept. Restor. Ecol. 27, 379–388. doi: 10.1111/rec.12856
Milchunas, D. G., Lauenroth, W. K., and Burke, I. C. (1998). Livestock grazing: animal and plant biodiversity of shortgrass steppe and the relationship to ecosystem function. Oikos 83, 65–74. doi: 10.2307/3546547
Milchunas, D. G., Sala, O. E., and Lauenroth, W. K. (1988). A generalized model of the effects of grazing by large herbivores on grassland community structure. Am. Nat. 132, 87–106.
Miles, D. B., and Ricklefs, R. E. (1984). The correlation between ecology and morphology in deciduous forest passerine birds. Ecology 65, 1629–1640.
Miles, D. B., and Ricklefs, R. E. (1994). “Ecological and evolutionary inferences from morphology: an ecological perspective,” in Ecological Morphology: Integrative Organismal Biology, eds P. C. Wainwright and S. M. Reilly (Chicago: University of Chicago Press), 13–41.
Moore, R. B., McKay, L. D., Rea, A. H., Bondelid, T. R., Price, C. V., Dewald, T. G., et al. (2019). User’s Guide For The National Hydrography Dataset Plus (NHDPlus) High Resolution. Reston, VA: US Geological Survey.
Moran, M. D. (2014). Bison grazing increases arthropod abundance and diversity in a tallgrass prairie. Environ. Entomol. 43, 1174–1184. doi: 10.1603/EN14013
Morrison, C. D., Boyce, M. S., and Nielsen, S. E. (2015). Space-use, movement and dispersal of sub-adult cougars in a geographically isolated population. PeerJ 2015, 1–24. doi: 10.7717/peerj.1118
Nickell, Z., Varriano, S., Plemmons, E., and Moran, M. D. (2018). Ecosystem engineering by bison (Bison bison) wallowing increases arthropod community heterogeneity in space and time. Ecosphere 9:e02436. doi: 10.1002/ecs2.2436
Ohmart, R. D. (1994). The effects of human-induced changes on the avifauna of western riparian habitats. Stud. Avian Biol. 15, 273–285.
Peden, D. G., Van Dyne, G. M., Rice, R. W., and Hansen, R. M. (1974). The trophic ecology of Bison bison L. on shortgrass plains. J. Appl. Ecol. 11, 489–497. doi: 10.2307/2402203
Pigot, A. L., Sheard, C., Miller, E. T., Bregman, T. P., Freeman, B. G., Roll, U., et al. (2020). Morphological form to ecological function in birds. Nat. Ecol. Evol. 4, 230–239. doi: 10.1038/s41559-019-1070-4
Pollock, M. M., Naiman, R. J., Erickson, H. E., Johnston, C. A., Pastor, J., and Pinay, G. (1995). “Beaver as engineers: influences on biotic and abiotic characteristics of drainage basins,” in Linking Species & Ecosystems, eds G. JonesJohn and H. Lawton (Boston, MA: Springer), 117–126.
Porensky, L. M., McGee, R., and Pellatz, D. W. (2020). Long-term grazing removal increased invasion and reduced native plant abundance and diversity in a sagebrush grassland. Glob. Ecol. Conserv. 24:e01267. doi: 10.1016/j.gecco.2020.e01267
Powell, A. F. L. A. (2006). Effects of prescribed burns and bison (Bos bison) grazing on breeding bird abundances in tallgrass prairie. Auk 123, 183–197.
R Core (2018). R: A Language and Environment for Statistical Computing (Version 3.5.2, R Foundation for Statistical Computing, Vienna, Austria, 2018).
R Core Team (2015). R: A Language And Environment For Statistical Computing. Available online at: https://www.r-project.org.
Ranglack, D. H., Durham, S., and du Toit, J. T. (2015). Competition on the range: science vs. perception in a bison-cattle conflict in the western USA. J. Appl. Ecol. 52, 467–474. doi: 10.1111/1365-2664.12386
Ranglack, D., and du Toit, J. T. (2015). Habitat selection by free-ranging bison in a mixed grazing system on public land. Rangel. Ecol. Manag. 68, 349–353. doi: 10.1016/j.rama.2015.05.008
Revell, L. J. (2012). Phytools: an R package for phylogenetic comparative biology (and other things). Methods Ecol. Evol. 3, 217–223. doi: 10.1111/j.2041-210X.2011.00169.x
Ricklefs, R. E. (2017). Passerine morphology: external measurements of approximately one-quarter of passerine bird species. Ecology 98:1472. doi: 10.1002/ecy.1783
Royle, J. A. (2004). N-mixture models for estimating population size from spatially replicated counts. Biometrics 60, 108–115. doi: 10.1111/j.0006-341X.2004.00142.x
Shamon, H. (2021). Smithsonian Grassland Ecology. Washington, DC: eMammal; Smithsonian Institution. doi: 10.25571/9G7Y-E537
Shamon, H., Cosby, O., Andersen, C. L., BearCub, S., and Bresnan, C. (2022). The potential of bison restoration as an ecological approach to future tribal food sovereignty on the northern great plains. Front. Ecol. Evol. 10:82682. doi: 10.3389/fevo.2022.826282
Sheard, C., Neate-clegg, M. H. C., Alioravainen, N., Jones, S. E. I., Vincent, C., Macgregor, H. E. A., et al. (2020). Dispersal inferred from wing morphology. Nat. Commun. 11:2463. doi: 10.1038/s41467-020-16313-6
Skagen, S. K., Hazlewood, R., and Scott, M. L. (2005). The Importance And Future Condition Of Western Riparian Ecosystems As Migratory Bird Habitat. Albany, CA: U.S. Dept. of Agriculture.
Steuter, A. A., and Hidinger, L. (1999). Comparative ecology of Bison and cattle on mixed-grass prairie. Gt. Plains Res. 9, 329–342.
Tewksbury, J. J., Black, A. E., Nur, N., Saab, V. A., Logan, B. D., and Dobkin, D. S. (2002). Effects of anthropogenic fragmentation and livestock grazing on western riparian bird communities. Stud. Avian Biol. 25, 158–202.
Turunen, J., Elbrecht, V., Steinke, D., and Aroviita, J. (2021). Riparian forests can mitigate warming and ecological degradation of agricultural headwater streams. Freshw. Biol. 66, 785–798. doi: 10.1111/fwb.13678
USFWS (2002). Final Recovery Plan Southwestern Willow Flycatcher (Empidonax traillii extimus). 18085–18530. Available online at: https://www.fws.gov/carlsbad/SpeciesStatusList/RP/20020830_RP_SWWF.pdf (accessed January 25, 2022).
USFWS (2020). Endangered and threatened wildlife and plants: revised designation of critical habitat for the western distinct population segment of the yellow-billed cuckoo. Fed. Regist. 85, 11458–11594. doi: 10.1016/0196-335x(80)90058-8
van Zanten, H. H. E., Mollenhorst, H., Klootwijk, C. W., van Middelaar, C. E., and de Boer, I. J. M. (2016). Global food supply: land use efficiency of livestock systems. Int. J. Life Cycle Assess. 21, 747–758. doi: 10.1007/s11367-015-0944-1
Vandermyde, J. M. (2017). Establish Long-Term Stream Monitoring At Nachusa Grasslands To Assess Effects Of Prairie Land Management Practices On Illinois Prairie Streams. Champaign, IL: Illinois Environmental Protection Agency.
Villéger, S., Mason, H., and Mouillot, D. (2008). New multidimensional functional diversity indices for a multifaceted framework in functional ecology. Ecology 89, 2290–2301. doi: 10.1890/07-1206.1
Wahl, C. M., Neils, A., and Hooper, D. (2013). Impacts of land use at the catchment scale constrain the habitat benefits of stream riparian buffers. Freshw. Biol. 58, 2310–2324. doi: 10.1111/fwb.12211
Warton, D. I., and Hui, F. K. C. (2011). The arcsine is asinine: the analysis of proportions in ecology. Ecology 92, 3–10. doi: 10.1890/10-0340.1
Wilcove, D. S., Rothstein, D., Dubow, J., Phillips, A., and Losos, E. (1998). Quantifying threats to imperiled species in the United States: assessing the relative importance of habitat destruction, alien species, pollution, overexploitation, and disease. Bioscience 48, 607–615. doi: 10.2307/1313420
Yu, S. W. (2021). American Bison Impacts On Riparian And Wallow Vegetation Communities. Dissertation, Clemson University.
Keywords: grassland, songbirds, buffalo, cattle, conservation, ungulates, rewilding, American Prairie
Citation: Boyce AJ, Shamon H and McShea WJ (2022) Bison Reintroduction to Mixed-Grass Prairie Is Associated With Increases in Bird Diversity and Cervid Occupancy in Riparian Areas. Front. Ecol. Evol. 10:821822. doi: 10.3389/fevo.2022.821822
Received: 24 November 2021; Accepted: 11 February 2022;
Published: 18 March 2022.
Edited by:
Karsten Wesche, Senckenberg Museum of Natural History Görlitz, GermanyReviewed by:
Miguel Nuno Bugalho, University of Lisbon, PortugalPerry S. Barboza, Texas A&M University, United States
Bethanne Bruninga-Socolar, University of Minnesota, Twin Cities, United States
Copyright © 2022 Boyce, Shamon and McShea. This is an open-access article distributed under the terms of the Creative Commons Attribution License (CC BY). The use, distribution or reproduction in other forums is permitted, provided the original author(s) and the copyright owner(s) are credited and that the original publication in this journal is cited, in accordance with accepted academic practice. No use, distribution or reproduction is permitted which does not comply with these terms.
*Correspondence: Andy J. Boyce, Ym95Y2VhQHNpLmVkdQ==
†These authors have contributed equally to this work