- 1Department of Biological Sciences, Auburn University, Auburn, AL, United States
- 2Department of Ecology and Evolutionary Biology, University of Colorado, Boulder, Boulder, CO, United States
- 3Department of Biology, Tennessee Technological University, Cookeville, TN, United States
- 4Research School of Biology, The Australian National University, Canberra, ACT, Australia
Maternal nesting behavior in oviparous species strongly influences the environmental conditions their embryos experience during development. In turn, these early-life conditions have consequences for offspring phenotypes and many fitness components across an individual’s lifespan. Thus, identifying the evolutionary and ecological causes and effects of nesting behavior is a key goal of behavioral ecology. Studies of reptiles have contributed greatly to our understanding of how nesting behavior shapes offspring phenotypes. While some taxonomic groups have been used extensively to provide insights into this important area of biology, many groups remain poorly studied. For example, the squamate genus Anolis has served as a model to study behavior, ecology, and evolution, but research focused on Anolis nesting behavior and developmental plasticity is comparatively scarce. This dearth of empirical research may be attributed to logistical challenges (e.g., difficulty locating nests), biological factors (e.g., their single-egg clutches may hinder some experimental designs), and a historical focus on males in Anolis research. Although there is a gap in the literature concerning Anolis nesting behavior, interest in nesting ecology and developmental plasticity in this group has grown in recent years. In this paper, we (1) review existing studies of anole nesting ecology and developmental plasticity; (2) highlight areas of anole nesting ecology that are currently understudied and discuss how research in these areas can contribute to broader topics (e.g., maternal effects and global change biology); and (3) provide guidelines for studying anole nesting in the field. Overall, this review provides a foundation for establishing anoles as models to study nesting ecology and developmental plasticity.
Introduction
Nesting is an important reproductive behavior that can have lasting impacts on maternal and offspring fitness (Refsnider and Janzen, 2010). Because embryos are highly sensitive to environmental perturbations, even short-term exposure to different conditions can have far-reaching consequences across subsequent life stages (West-Eberhard, 2003; Uller, 2008; While et al., 2018). For example, brief exposure to temperature or pH during development can permanently affect offspring sex in some animals (Cook, 2002; Valenzuela, 2004) and have lasting individual- and population-level consequences (Warner and Shine, 2008a; Grayson et al., 2014). Because successful early-life development is a prerequisite for future reproduction, natural selection on maternal nesting behaviors should be particularly strong. These points illustrate the importance of nesting ecology, which is a topic that broadly incorporates aspects of maternal behaviors (e.g., choice of nest location and level of parental care), nest environment (e.g., biotic and abiotic variables around and within the nest), embryo or neonatal development (e.g., physiological tolerances and developmental plasticity), and a variety of other factors that influence the nest and its surrounding environment (e.g., soil composition, predators, and parasites) (Doody et al., 2021). Overall, understanding an organism’s nesting ecology can provide insight into a range of behavioral and physiological traits (Angilletta et al., 2009; Hall and Warner, 2021), answer basic questions about ecology and evolution (Resetarits, 1996; Refsnider and Janzen, 2010), and solve problems in conservation and management (Hare et al., 2002, 2004; Mazaris et al., 2017).
Nest-site selection is particularly important for oviparous species that lack post-nesting parental care because developing offspring have little opportunity to adjust to changing environmental conditions (but see Warkentin, 2011; Shine and Du, 2018). For example, extended parental attendance in fish, amphibians and birds can reduce the risk of nest predation (e.g., Sargent, 1988; Komduer and Kats, 1999; Lehtinen et al., 2014; Schulte et al., 2020), whereas unattended eggs may be considerably more vulnerable in species without parental care depending on where mothers place their nests. Additionally, nest temperatures of some species (e.g., birds) are relatively stable and predictable due to parental incubation (Deeming and Reynolds, 2015; Refsnider, 2015), but temperatures fluctuate widely in nests of most non-avian reptiles (henceforth “reptiles”) (Warner and Shine, 2008b; Du et al., 2010). Reptiles have been useful models for studies of nesting ecology, largely due to their considerable variation in nest environments across both large and small spatial and temporal scales. Indeed, maternal choice of microhabitats for nesting is well documented in reptiles, and nests can vary considerably in thermal and hydric conditions among and within species (Janzen and Morjan, 2001; Doody et al., 2006; Refsnider, 2015). As such, embryos vary across species with respect to environmental tolerances (Andrews and Schwarzkopf, 2012; Hall and Sun, 2021) and respond to incubation environments in ways that influence fitness-related morphological, physiological, behavioral, and life-history traits of offspring (i.e., developmental plasticity; Noble et al., 2018; Warner et al., 2018; While et al., 2018). The long-term effects of developmental plasticity on fitness and their potential adaptive significance are important components of nesting ecology, but this topic is poorly studied (Mitchell et al., 2018). Indeed, filling in this knowledge gap would provide important insight into the factors that shape maternal nesting behaviors.
While reptile nesting ecology and developmental plasticity have been active subjects of study for several decades (Warner et al., 2018), we have a poor understanding of these topics in many otherwise well-studied clades. The lizard genus Anolis is an excellent example. This group consists of approximately 400 species found across the Caribbean and the mainland from the southern United States through Central and South America. Additionally, many species are colonizers and have become invasive across the globe (Losos, 2009; Latella et al., 2011). Anoles have served as important models for testing foundational hypotheses in ecology, evolution, behavior, and developmental biology (Losos, 2009; Sanger and Kircher, 2017; Feiner, 2019). Yet relatively little is known about their nesting ecology despite its potential importance for individual fitness and population dynamics (Andrews, 1988). Several factors contribute to the deficiency of empirical work on anole nesting. For example, the single-egg clutch of anoles can complicate experimental designs for studies of egg incubation because eggs from a single clutch cannot be allocated among different treatments. Their single-egg clutch also makes observing nesting in the wild challenging because the time required for nesting is short. Relatedly, the small eggs and inconspicuous nesting behavior of anoles hinders our ability to detect nests in complex habitats. Finally, the historical precedent of research on male morphology and behavior (e.g., charismatic dewlaps and display behaviors) has diverted research attention away from important aspects of female biology (Kamath and Losos, 2017). These factors present roadblocks for empirical work on nesting ecology of anoles. Establishing methodologies that overcome these logistical difficulties is essential.
Studies on nesting in anoles can provide new insight into many well-studied aspects of their biology. For example, nest environments shape fitness-related phenotypes of offspring (e.g., body size and locomotor performance) that may relate to resource competition (Pearson and Warner, 2018); this could inform work on niche partitioning and community ecology (Roughgarden, 1995). Important eco-morphological traits that exhibit considerable convergence across species (e.g., limb length and body size) may be developmentally sensitive to early-life conditions (Losos et al., 2000; Downes and Hoefer, 2007; but see Warner et al., 2012); this could illuminate the role of developmental plasticity in convergent evolution and adaptive radiation (Losos, 2009; but see Feiner et al., 2020). Availability and location of suitable nest sites may influence the spatial distribution of females and nest predators in ways that influence mate competition among males and population dynamics (Andrews, 1988; Chalcraft and Andrews, 1999; Angilletta et al., 2009); this could inform studies of territorial behaviors, mating systems, and population biology. Lastly, methodology and protocols that are developed for studying Anolis nesting can be applied to other taxa, and therefore be useful tools for providing broader information about nesting ecology and reproductive behaviors in many other oviparous reptiles. These are just a few examples of how studies of nesting ecology in anoles could enhance their usefulness as models for a range of topics.
Anoles have several features that make them useful models for studies of nesting ecology. First, several species are conspicuous members of their ecological communities and occur in high densities. This facilitates large sample sizes for observational and experimental studies and indicates that nests/eggs are abundant in the field during the reproductive season. Second, anoles are somewhat unique among reptile clades because all species are constrained to produce a single egg-clutch every 1–3 weeks over a relatively long reproductive season; while a single-egg clutch could hinder experimental designs (noted above), this potentially facilitates repeated observations of nesting, which could benefit some laboratory experiments of nesting behavior. Third, many species have a relatively short life span (1–2 years), which makes studies of the life-long effects of developmental environments feasible. Fourth, captive husbandry and protocols for captive breeding are well established and logistically feasible (Sanger et al., 2008a). Moreover, the small body size of most species means that large numbers of captive individuals can be housed in relatively small areas; these are welcome features for most research programs where animal-housing space and funds are limited. Indeed, studies on anole nesting and development have even been conducted in a secondary school classroom (Reedy et al., 2013). Finally, because anoles have been studied extensively for other purposes, there is a wealth of knowledge concerning their life-history, reproductive physiology, evolutionary relationships, and general ecology (Losos, 2009). Such background knowledge provides critical context for observations of nesting behavior and its effects on lifetime fitness.
In this review, we aim to achieve three major goals. First, we provide an overview of the current state of knowledge on anole nesting ecology and developmental plasticity. Second, we identify knowledge gaps in anole nesting ecology and developmental plasticity and discuss how filling these gaps will contribute to broader topics. Third, we establish useful guidelines for finding nests in the field, which will help future anole biologists address some of the gaps we identify. Overall, by addressing these objectives, we hope to bring more attention to anoles as models for studying nesting ecology and developmental plasticity.
An Overview of Nesting Ecology in Anoles
Most descriptions of anole nesting are based on anecdotal observations of nest microhabitats, but some laboratory and field experiments have provided key insights into nest-site selection and oviposition behavior (Table 1 and Supplementary Table 1). Collectively, these anecdotes and experiments indicate that females nest in a wide range of microhabitats while seeking conditions that have important developmental consequences for offspring. Much of the variation in nest microhabitats is likely explained by differences among species, local environments, seasonal timing of nesting, and habitat availability. Here we summarize the broad range of behaviors associated with oviposition, as well as general trends concerning maternal choice of nest microhabitats in anoles.
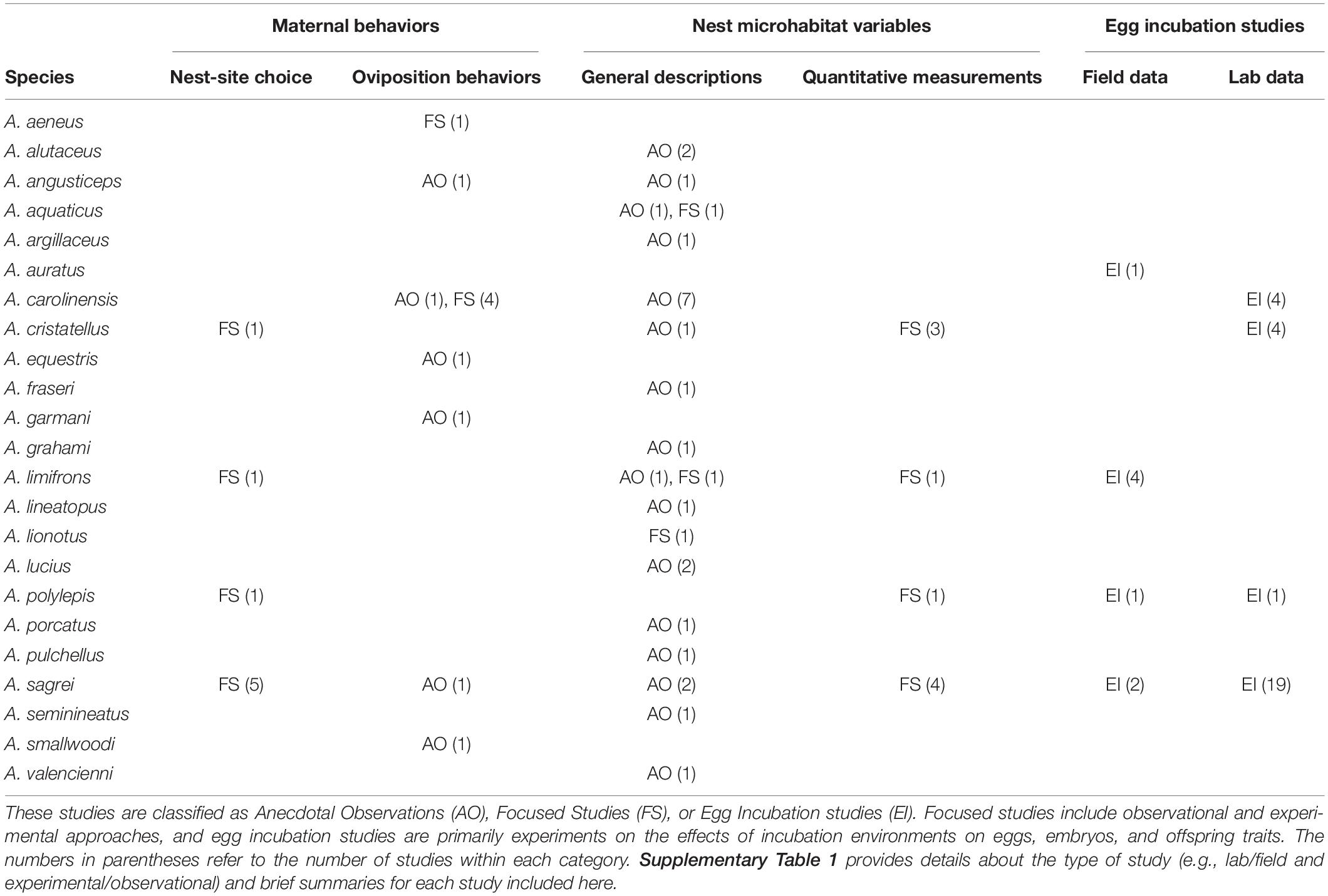
Table 1. Summary of studies that provide information on nesting ecology for different Anolis species.
Anecdotal Reports
Direct observations of nesting in the wild are rare, because nest sites and nesting behavior are inconspicuous. For example, Anolis carolinensis females dig a nest, oviposit, and cover the egg in 11–26 min (Propper et al., 1991), which is much shorter than in many reptiles (e.g., turtles – nesting can take several hours). Consequently, most reports of anole nest sites involve serendipitous discoveries of eggs in the field, and several researchers have made the most of these discoveries by describing various aspects of the immediate nest microenvironment (e.g., Allen and Slatten, 1945; Alfonso et al., 2012; Delaney et al., 2013; Supplementary Table 1A). These observations indicate that anoles typically nest beneath cover objects or within cryptic spaces (Rand, 1967; Tiatragul et al., 2019). For example, eggs of several species have been found under rocks (A. carolinensis, Carr, 1940; Allen and Slatten, 1945; Anolis aquaticus, Swierk et al., 2019; Anolis sagrei, Pruett et al., 2020), within leaf litter (Anolis limifrons, Andrews, 1988; A. cristatellus, Tiatragul et al., 2019), under or within plants (Anolis argillaceus, Alfonso et al., 2012; Anolis lionotus, Montgomery et al., 2011), and above ground within tree holes (Anolis angusticeps, Robinson et al., 2014; A. limifrons, Andrews, 1988). In some cases, eggs have been found buried in substrate underneath a cover object (Allen and Slatten, 1945; Delaney et al., 2013), but eggs are often unburied (Tiatragul et al., 2019). Interestingly, eggs of the Cuban anole, Anolis lucius, have been discovered stuck to the walls of caves (Dunn, 1926) with numerous eggshells on the cave floor, indicating that eggshells fall during or after hatching (Hardy, 1957); although eggs of A. lucius are exposed to ambient air, the humid cave environment might reduce the risk of egg desiccation. Similar observations have been made in several Asian geckos (Somaweera, 2009; Kalaimani, 2015), and this relatively unique choice of oviposition site warrants further investigation in Anolis.
Observations of multiple eggs within a single nest are common, indicating that females nest communally and/or return to the same site to oviposit. In some cases, anoles may use the same nest site as other species. For example, eggs of A. argillaceus were found with eggs of two gecko species (Alfonso et al., 2012), and A. carolinensis eggs have been found within alligator nests (Kushlan and Kushlan, 1980) and in the upper chambers of ant mounds (Kwapich, 2021). Evidence of communal nesting (i.e., between 4–24 eggs in a single nest) in the field has also been reported for A. angusticeps (Robinson et al., 2014), A. lionotus (Montgomery et al., 2011), A. aquaticus (Márquez and Márquez, 2009), A. sagrei (Pruett et al., 2020) and A. carolinensis (Godfrey et al., 2018; García-Padrón, 2021). Given these observations, communal nesting may be relatively widespread across anoles. Importantly, communal nesting behavior may be a function of several factors, such as limited suitable habitat for nest sites (resulting in aggregations of eggs in specific locations) or due to females actively seeking eggs as cues for nest-site choice. Additionally, given that many anoles continuously produce an egg almost every week, these communal nests may reflect the same female returning to a nest site to lay subsequent eggs. Little is known about the developmental consequences for eggs incubating in communal versus solitary environments; however, communal nesting is generally common across reptiles (Doody et al., 2009) and anoles could make excellent models to unearth the evolutionary impetus and ecological effects of this behavior (Warner and Chapman, 2011; Dees et al., 2020).
Although field observations of nest sites exist for a diversity of species, lab observations of oviposition behavior have been reported only for A. carolinensis. Captive A. carolinensis have been observed using their forelimbs and snout to create a nest hole in Spanish moss or in soil substrate (Greenberg and Noble, 1944; Gordon, 1956). Females often place their cloaca directly over the hole for oviposition. If eggs do not fall directly into the hole, females will push the egg in with their snout. This process usually takes about 1 min (likely why nesting is rarely observed) but can last up to about 26 min. Females use their forelimbs to push substrate over the eggs, and the snout is used to pack the substrate around the egg (Greenberg and Noble, 1944). Detailed descriptions of these behaviors are in Tokarz and Jones (1979) and Propper et al. (1991). Intriguingly, several female A. sagrei have been observed in the laboratory and field carrying an egg in their mouth (Delaney et al., 2021); whether the egg was produced by that same female is unknown, but this behavior raises exciting questions about maternal care in anoles (e.g., do females move their eggs among different locations?).
These anecdotal observations are critical in understanding the types of habitat females use for nesting, and for generating new questions and hypotheses about nesting behavior. Generally, these reports suggest that females select nest microhabitats that are relatively cool, moist, and thermostable as well as sites that are hidden from predators. While these reports are largely descriptive and often do not provide quantitative microhabitat measurements (e.g., temperature and moisture levels), they can form the basis for focused observational and experimental work needed to obtain such data. Of course, conclusions about anole nesting that are drawn from anecdotal observations must be done cautiously, as these observations rarely involve systematic approaches to finding nests and can inevitably create a biased perspective on nesting ecology. Therefore, such observations are most useful when further tested by experimental studies.
Experimental Studies
While focused experimental studies of anole nesting are rare, these few laboratory and field studies provide critical insight into maternal nest-site choice and its adaptive significance (Supplementary Table 1B). Field studies have been particularly useful in assessing the fitness consequences of maternal nesting behavior under ecologically-meaningful conditions. Unlike field studies, however, laboratory experiments can hold specific variables constant so that we can pinpoint critical components of the micro-environment that females choose for nesting and their effects on development. Both laboratory and field studies can also be designed to examine the consequences of maternal nest site choice on egg survival. For example, laboratory studies can quantify egg survival across a range of incubation conditions that include and exceed those chosen by females. Whereas field studies can compare egg survival and environmental variables between actual nests (those chosen by females) and putative nests (those chosen and constructed by researchers in randomly or non-randomly selected locations or microhabitats). Overall, by integrating laboratory and field studies we can combine the power of controlled experiments with the realism of natural environments and make ecologically-relevant conclusions about nesting ecology. Here, we review the current state of knowledge about Anolis nesting ecology based on experimental approaches in the lab and field.
Focused studies of anole nesting aim primarily to quantify choice of nest microhabitat by females and consequences of the nest environment on offspring. In many cases, results are generally consistent with the anecdotal observations above: anoles seek relatively cool, moist microhabitats for nesting, and often lay eggs under some type of cover (e.g., leaf litter, rocks, and logs). Laboratory experiments on Anolis polylepis and A. sagrei, which provided females with nest pots that vary in moisture levels, demonstrate that females choose relatively moist substrates when nesting (Socci et al., 2005; Reedy et al., 2013). Moist nesting conditions facilitate egg hatching success because eggs must absorb water during incubation for proper development (Warner et al., 2011; Reedy et al., 2013). Moreover, A. sagrei choose relatively moist nest sites at the time of oviposition even when the hydric conditions of those nest sites fluctuate unpredictably toward lethally dry conditions (Warner et al., 2021). These lab-based results are consistent with observations where nesting behavior is stimulated by artificially watering substrate that simulates rainfall (A. carolinensis, Gordon, 1956; Anolis aeneus, Stamps, 1976; A. sagrei, Brown and Sexton, 1973), and parallels results of field studies showing that substrates of maternally-chosen nest sites have greater moisture than those of randomly-chosen sites (Pruett et al., 2020; Tiatragul et al., 2020).
Focused studies of anole nesting also corroborate the anecdotal reports concerning communal nesting. Searches for A. lionotus eggs along a river in Panama revealed that all nests were communal and always covered with vegetation on downstream sides of rocks surrounded by water (Montgomery et al., 2011). Communal nesting in this species was evident for active nests that contained unhatched eggs (mean egg number per nest was 11 eggs, range 4–24), and for inactive nests of hatched eggs (mean = 6.3 eggs, range 0–16). In a laboratory experiment with A. sagrei, Dees et al. (2020) examined nest-site choice based on the presence or absence of hatched eggshells, as well as the use of fresh soil versus soil previously used by nesting females to determine if these were important cues that facilitate communal nesting. Females chose nest sites that contained hatched eggshells more frequently than sites without eggshells. Moreover, females preferred nesting in previously-used soil to fresh, unused soil. These results indicate that (1) both visual and olfactory cues play a role in nest-site choice, and (2) communal nesting may be a function of females actively seeking cues of conspecifics, rather than just aggregating eggs in a limited number of suitable oviposition sites.
The thermal environment also correlates with nest-site choice, as successful development can only proceed over a limited range of temperatures (Sanger et al., 2018; Pruett and Warner, 2021). Because putative nest temperatures can vary considerably among microhabitats and across the nesting season (Schlaepfer, 2003; Pearson and Warner, 2016, 2018), gravid females face important challenges when choosing suitable nest habitats. Indeed, field data from an island population of A. sagrei in Florida, United States, show that females choose shadier nest microhabitats with temperatures that rarely reach lethal extremes compared to open sites that are also available for nesting (Pruett et al., 2020); this pattern remains consistent across the season even with temporal changes in air and ground temperatures. Similar trends were observed in a field study of A. cristatellus across suburban and forested locations in Miami, Florida (Tiatragul et al., 2019, 2020). In forested sites, nest temperatures are nearly identical to temperatures of randomly selected areas; thus, there is little opportunity for females to choose microhabitats based on temperature due to the high thermal homogeneity in this heavily shaded habitat. In contrast, females in suburban areas nest close to trees, and consequently, their nests have greater canopy cover and lower temperatures than what is generally available across the suburban landscape. These field studies also examined the consequences of maternally-chosen nest environments on egg survival by either placing eggs in putative nest sites in the field (Pruett et al., 2020) or by incubating eggs in the lab (Tiatragul et al., 2020). Both studies demonstrate that maternal choice of nest habitat is adaptive because eggs had greater survival under the relatively cool conditions chosen by females than under the warmer conditions readily available across the landscape. This tendency for anoles to select relatively cool sites is in contrast with other lizards which utilize warmer areas for nesting (e.g., Shine and Harlow, 1996; Warner and Shine, 2008a; Angilletta et al., 2009) and may relate to potentially high thermal variation of relatively shallow nests (discussed below).
Predators are also an important component of nesting ecology (Martin, 1993; Spencer and Thompson, 2003), and a few experiments demonstrate their influence on nest success, maternal behaviors, and population biology in anoles. Predation by invertebrates plays a major role in nest success of A. limifrons in Panama (Andrews, 1982); in this study, anole eggs produced by a breeding colony were placed in putative nest locations at two different sites and eggs were monitored for 50 days. Predation by Solenopsis ants and snails was the most common known source of egg mortality (57–77% egg mortality), and egg survival was positively associated with leaf litter abundance. Not surprisingly, after systematically searching for eggs across different habitat types (including several ground and above ground sites), Andrews (1988) estimated that 99.5% of eggs are laid on the ground under leaf litter. However, 60% of all eggs were depredated by Solenopsis ants regardless of whether nests were on the ground or above ground (Andrews, 1988). This predator-prey relationship between ants and anole eggs was further explored by simulating rainfall across different experimental plots and examining its impact on egg predation (Chalcraft and Andrews, 1999). This study revealed that ant density increased under conditions that simulated wet years, and in turn, resulted in greater egg predation than under conditions that simulated dry years. Similar interactions between predator density and microhabitat features have been shown for A. sagrei nests in Florida. In this study, DeSana et al. (2020) manipulated the density of terrestrial marsh crabs (Armases cinereum) and habitat type (open, leaf litter, palm fronds) within replicate experimental field enclosures. After placing eggs in these microhabitats, they found that egg predation was greatest in the high-density crab treatment, and significantly fewer eggs were depredated if they were buried under leaf litter compared to the other locations (DeSana et al., 2020). Together, results from these experiments in Panama and Florida indicate that the use of leaf litter for nesting would be favored by natural selection when invertebrate predators are abundant. Of course, the tendency to nest in cryptic spaces may benefit maternal survival as well as offspring.
Most studies of adaptive nest-site choice in reptiles focus on the fitness consequences of eggs/offspring rather than on the risks that mothers take to find suitable nesting habitat. However, theory predicts that natural selection will shape traits (e.g., nesting behavior) depending on how they influence maternal fitness, rather than that of individual offspring (Godfray and Parker, 1991; Roff, 1992; Delaney and Janzen, 2020). This issue was addressed in a recent study on the predator-prey relationship between the ground-dwelling curly-tailed lizard (Leiocephalus carinatus) and its arboreal prey, A. sagrei. In this study, Pruett (2021) provided female A. sagrei with ground and arboreal nest sites in large outdoor enclosures and showed that females prefer to nest on the ground. After 2 weeks, L. carinatus were introduced into half the enclosures, and females shifted to using arboreal nest sites, and did so more quickly than those in the non-predator control treatment. This pattern is consistent with field studies in the Bahamas where A. sagrei exhibits a shift toward high perches accompanied by a reduction in female survival when this natural predator is present (Lapiedra et al., 2018); although not acknowledged in that study, the reduction in female survival might be associated with maternal nesting behaviors and a lack of arboreal nest sites. Overall, given the diverse range of potential anole predators (e.g., birds, snakes, and lizards), this threat on maternal fitness likely plays an important role in shaping nesting behaviors as well as population dynamics (i.e., recruitment into juvenile or adult age classes). This understudied aspect of anole nesting ecology is ripe with questions waiting to be explored.
Egg Incubation Studies of Developmental Plasticity
The last decade has witnessed a proliferation of studies of reptile developmental plasticity that more closely replicate real-world nest environments in the laboratory (Carter and Janzen, 2021). Anolis lizards have been important models in this new frontier (Hall and Warner, 2018; Pearson and Warner, 2018; Sanger et al., 2018; Tiatragul et al., 2020; Hall et al., 2021) for several reasons. Methods for laboratory collection of anole eggs and embryos are established (Sanger et al., 2008a), and an embryo staging series is available (Sanger et al., 2008b). Such tools serve as a foundation for studies of development and are necessary to explore the underlying mechanisms that regulate embryo interactions with the environment (e.g., Sanger et al., 2018). Additionally, basic measures of ecological (egg survival and hatchling phenotypes) and physiological (water uptake, developmental rate, and oxygen consumption) responses to important nest variables like temperature and moisture are available for some species (e.g., Warner et al., 2012; Reedy et al., 2013; Sanger et al., 2018; Hall and Warner, 2019, 2021; Pruett and Warner, 2021). Thus, we have an understanding of embryo tolerances to nest conditions which is vital for designing ecologically relevant laboratory experiments and interpreting ecological data from nests in the wild (e.g., nest temperature; Hall and Warner, 2021). Finally, important environmental data, like canopy cover, temperature, and soil moisture, have been measured in and around nests (Sanger et al., 2018; Tiatragul et al., 2019; Pruett et al., 2020). Such studies provide ecological context to laboratory studies that assess how embryos respond to environmental conditions (e.g., Sanger et al., 2018; Hall and Warner, 2021). Although most of the aforementioned studies have been conducted on a few species (most commonly, A. sagrei), these studies can serve as models for other species, allowing for important cross-species comparisons. Ultimately, the unification of these tools and available data make anoles well positioned to serve as an important model for determining how natural nest environments influence embryo development, egg survival, and hatchling phenotypes (Hall et al., 2021).
Most studies of developmental plasticity in anoles focus on effects of temperature and moisture during development (Supplementary Table 1C), with comparatively few studies measuring the effects of other aspects of the nest environment (e.g., substrate type; Hall et al., 2021). Generally, studies of anoles are in congruence with those of other reptiles: a range of temperature and moisture allows for successful development and fitness-relevant phenotypes are compromised at extremes. Regarding moisture, all anoles studied have pliable-shelled eggs that must absorb water from the nest environment for successful development. As such, anoles have provided insight into relationships between water availability during development and important aspects of embryo physiology. Due to their small size and consequently large surface-area-to-volume ratio, anole eggs quickly desiccate in relatively dry incubation media (Socci et al., 2005; Hall et al., 2021; Warner et al., 2021). Some studies find that egg survival increases with moisture availability (e.g., Reedy et al., 2013) but most observe an optimum level of moisture for egg survival (Andrews and Sexton, 1981; Socci et al., 2005; Hall et al., 2021). Indeed, eggs rapidly desiccate in dry conditions, but excessively moist environments lead to fungal infection and embryo death (Andrews and Sexton, 1981; Socci et al., 2005). Additionally, greater water availability during development results in relatively large hatchling body size by enhancing the efficiency of conversion of yolk to hatchling somatic tissue (Warner et al., 2012; Hall et al., 2021). This water uptake by anole eggs is a passive process early in development but actively regulated later in development (Warner et al., 2011). Results from these studies have implications for maternal nesting behavior: wetter substrates enhance hatching success and hatchling body size, demonstrating the fitness benefits of anoles’ tendency to nest in moist microhabitats.
The relationship between nesting behavior and results from incubation temperature experiments is less straightforward than those of moisture experiments. Warmer temperatures speed developmental rates and enhance locomotor performance for A. sagrei (Pearson and Warner, 2016, 2018; Hall and Warner, 2018), but cooler temperatures enhance growth rates in A. carolinensis (Goodman, 2008). In the wild, females typically select nest sites that are cooler than what is generally available for nesting (Pruett et al., 2020; Tiatragul et al., 2020). Therefore, nesting behavior related to temperature probably represents a trade-off between enhancing fitness-relevant traits of hatchings and maximizing egg hatching success. This is illustrated by a recent study demonstrating that embryos and hatchlings have different thermal optima with respect to incubation temperature (Pruett and Warner, 2021): egg survival is greatest at relatively cool temperatures while hatchling survival is greatest for those incubated at warmer temperatures. The different optimal incubation temperatures for embryos vs. hatchlings might be related to the relatively shallow nests that anoles construct. As a result, anole nests exhibit extreme, diurnal variation in temperature which often exposes eggs to stressfully warm temperatures for a few hours each day (Sanger et al., 2018; Hall and Warner, 2021). Therefore, nest sites must be warm enough to enhance important hatchling traits but cool enough to avoid lethal extremes. Many incubation experiments demonstrate that heat stress during incubation (even exposure for <1h) have morphological, physiological, and ecological consequences (Hall and Warner, 2018, 2019, 2021; Pearson and Warner, 2018; Sanger et al., 2018; Tiatragul et al., 2020). For example, eggs of the crested anole (A. cristatellus) have greater survival when incubated under relatively cool temperatures that females select compared to incubation temperatures that reach high extremes that are rarely experienced in maternally-selected nests (Tiatragul et al., 2020).
In addition to numerous effects of abiotic factors, two studies have considered the biotic factor of egg aggregation due to communal nesting on egg survival and embryo development (Warner and Chapman, 2011; Dees et al., 2020). Most reptiles lay multi-egg clutches potentially generating competition for moisture or oxygen among eggs or altering nest temperatures via metabolic heating. However, the single-egg clutch of anoles may eliminate such effects. Conversely, in the wild, anole eggs are often found in communal nests which may indicate a shortage of acceptable nest sites (i.e., constraint hypothesis) or some advantage to laying eggs together (i.e., adaptive hypothesis). Warner and Chapman (2011) incubated A. sagrei eggs alone, paired with a live egg, and paired with an artificial egg. They found no adverse effects on egg survival and embryo development when eggs incubated adjacent to a live egg or an artificial egg, but eggs that incubated next to an egg that eventually died experienced reduced water uptake and hatchling body size. Dees et al. (2020) incubated A. sagrei eggs alone or in groups of 4 or 9 eggs. Incubation in groups reduced water uptake by eggs and body condition of hatchings. Indeed, in the 9-egg aggregation, water uptake was negatively related to the number of eggs that each egg in the cluster was touching, implicating competition among eggs for water. These studies demonstrate there are potential costs for egg-aggregation in anoles, thus, communal nesting in this group may be due to constraint rather than some adaptive advantage.
Comparative studies of egg incubation across populations or species can provide important insights into the evolution of embryo tolerances and plasticity. However, despite a substantial upward trend in the number of incubation studies conducted with anoles (Figure 1), most recent studies focus on a single species, A. sagrei, likely due to its availability, high fecundity, and hardiness in captivity. Of the nearly 400 species of anoles only six have been used in incubation studies. Although studies abound comparing morphological, ecological, and physiological traits of adult anoles across species or populations, only four incubation studies have made similar comparisons. For example, latitudinal comparisons of the North American A. carolinensis show that the effects of egg incubation temperature on offspring growth varies among populations (Goodman, 2008), and that thermal plasticity of offspring cell size (erythrocytes and epithelial cells) is greater in southern vs. northern populations (Goodman and Heah, 2010). In studies of species comparisons, eggs of A. auratus exhibit greater water storage and desiccation resistance under dry incubation conditions compared to those of A. limifrons, which may relate to habitat-specific adaptations in eggshell morphology (Andrews and Sexton, 1981). Additionally, A. sagrei embryos are more tolerant of heat stress than those of A. cristatellus, potentially reflecting physiological adaptation to species-specific nesting habitats (Hall and Warner, 2019). Given the results of these studies, the diversity of nesting habitats used across anoles, and the general tendency for adult anoles to differ in morphology and physiology, there is great potential for habitat-specific adaptations of embryo responses to nest conditions.
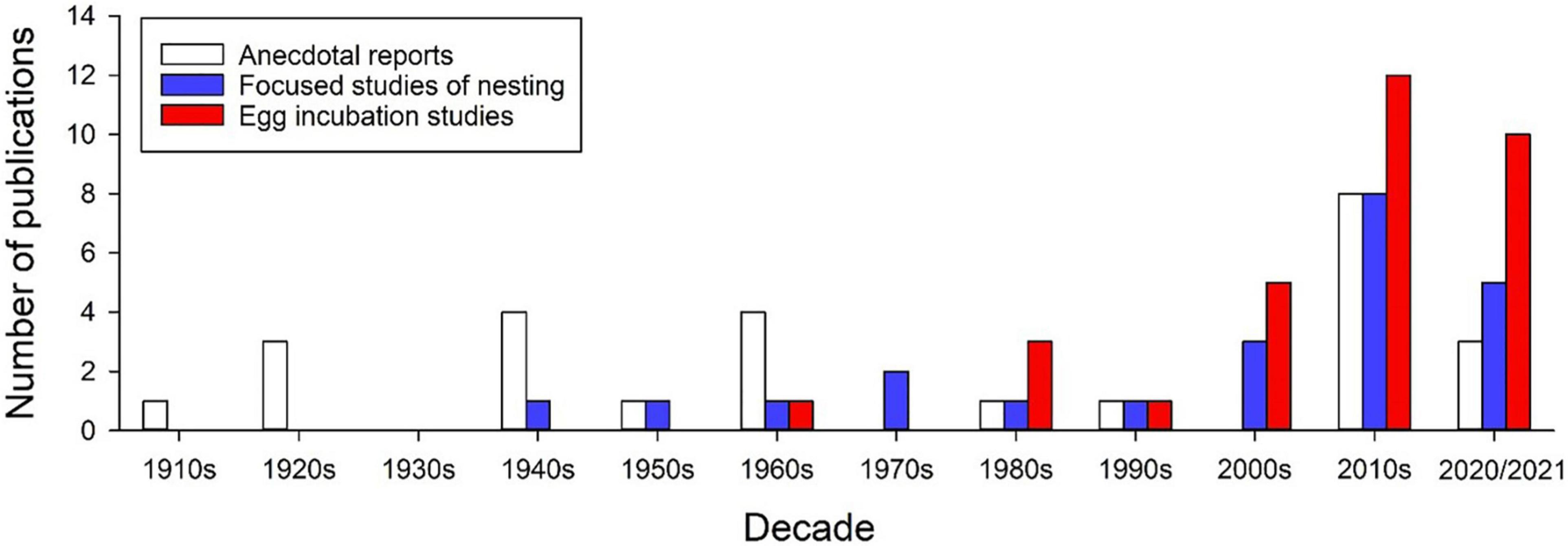
Figure 1. Number of publications over the past century on aspects of nesting ecology in Anolis lizards. These publications include anecdotal reports, focused studies of maternal nesting behaviors and descriptions of nest environments (observational and experimental), and egg incubation studies (lab and field) of the effects of incubation environments on egg survival, embryo development and offspring phenotypes. Note that the upward trend will likely continue through the 2020 decade, which is currently represented by only 2 years. Supplementary Table 1 provides details of each study in these categories.
Knowledge Gaps and Future Directions
The number of studies that have examined anole nesting and the effects of egg incubation environments has increased in the past decade (Figure 1), but there are several notable knowledge gaps. Research programs that address these topics would greatly advance our understanding of anole biology, and ecology and evolution more generally. Here, we have identified four topics that need more research attention and explain how addressing these knowledge gaps can contribute to broader areas of research.
Studying Developmental Plasticity in Real Nest Environments
While laboratory incubation studies are useful for quantifying developmental plasticity and for making predictions of microhabitats that females “should” use for nesting, there are several limitations concerning the utility of lab results. For example, treatments used in many incubation studies do not simulate the complex nest environment in the wild. That is, real nest environments are composed of a range of variables (e.g., substrate composition and chemistry, hydric and thermal conditions, oxygen, micro-organisms, and nest predators) that fluctuate over the incubation period. These variables can also include soil pollutants that are commonly used in ecotoxicology studies; to our knowledge, no studies have examined effects of chemical pollutants on embryo development in anoles. Decades of research demonstrate that variation in these incubation conditions results in a myriad of phenotypic effects on reptile embryos and hatchlings (Warner et al., 2018). Thus, while several authors of laboratory incubation studies make inferences about embryo plasticity and nesting behavior in the wild (including ourselves), we must be cautious in our interpretations of lab results. Indeed, most experiments poorly reflect natural nest environments but have served as a foundation for our current understanding and future work (Hall and Warner, 2020). These concerns highlight a major gap in our understanding of the effects of the multifaceted nest environment, and creative designs for field and laboratory studies are needed to quantify the interactive effects of multiple environmental factors (e.g., factorial experiments of moisture and temperature) to better understand the consequences of developmental plasticity and nesting behavior. Fortunately, because anoles have several characteristics that make them suitable models for studies of developmental plasticity (discussed above), filling this knowledge gap is achievable.
Predicting Effects of Global Change
Several attributes make anoles particularly useful to study effects of global change on nesting behavior and egg survival. Females construct relatively shallow nests which exhibit wide variation in temperature and moisture. For example, nest temperatures can vary greatly across spatial scales (e.g., differences in canopy cover; Pearson and Warner, 2016), across temporal scales during a long reproductive season (Pearson and Warner, 2018), or due to both spatiotemporal effects (Pruett et al., 2020). In particular, the relatively broad reproductive season of anoles and their propensity to colonize a diversity of habitats (e.g., urban vs. natural areas) means that nest conditions can change markedly throughout the breeding season and across habitats resulting in eggs experiencing an array of environmental conditions. As such, developmental tolerances of embryos are broad with respect to moisture and temperature (Reedy et al., 2013; Pruett and Warner, 2021) and nesting behavior is highly plastic, since females are capable of locating acceptable nest sites across a diversity of conditions (Hall et al., 2021).
Seasonal changes in precipitation due to climate change will likely influence nesting behavior and egg survival in complex ways. For example, Chalcraft and Andrews (1999) simulated rainfall in both wet and dry years and observed greater egg depredation of A. limifrons by ants in wet vs. dry plots, indicating an interaction between precipitation and depredation. This system could be used to understand how changing patterns of rainfall due to climate change will alter species interactions. Additionally, nests exhibit wide spatiotemporal variation in temperature, resulting in variable egg survival (Pruett et al., 2020). Anolis embryos routinely encounter stressful temperatures in the wild under current climate conditions (Sanger et al., 2018; Hall and Warner, 2021); therefore, future changes in mean and maximum nest temperatures due to climate warming will require mitigation via changes in nesting behavior, embryo thermal physiology, or both. Anoles could be useful models to understand how nesting behavior may mitigate adverse effects of climate change on offspring.
Finally, human-caused habitat changes (e.g., agriculture and urbanization) have context-dependent effects on embryo development and egg survival in anoles. For example, nests in urban and suburban habitats exhibit higher mean and maximum nest temperatures compared to forested areas (Tiatragul et al., 2017, 2019), potentially reducing hatching success and hatchling viability (Hall and Warner, 2018). However, the harmful effects of these extreme temperatures vary among species (Hall and Warner, 2019). Alternatively, Schlaepfer (2003) found that anole eggs incubating in agricultural fields exhibit faster development and higher survival than those in adjacent forest interior or edge habitat. Thus, habitat alteration may enhance survival for species whose eggs are well-adapted to disturbed conditions but reduce survival for others. Given the broad variation in habitat preference across Anolis, the high density of many species in both natural and disturbed areas, and the abundance of eggs due to high fecundity, research on anoles can provide novel insight into how nesting ecology relates to habitat disturbance and other aspects of global change.
Evolutionary Potential of Nesting Behavior and Developmental Plasticity
While several of the studies described above provide evidence of adaptive nesting behavior, we lack a strong grasp of the evolutionary potential of this important reproductive trait. Quantifying the evolutionary potential of nesting behavior and embryo plasticity will provide insight into basic questions about the evolution of maternal effects and phenotypic plasticity. Moreover, nesting behavior may also place constraints on the evolution of embryo plasticity in that maternal nest-site choice could buffer embryos from environmental variation (Tiatragul et al., 2020) and reduce the strength of selection on embryo reaction norms. In addition to these important conceptual topics in ecology and evolution, an understanding of the evolutionary potential of these traits will inform predictions of how these animals respond to environmental change. Moreover, given the abundance of invasive anoles across the planet, research on this topic will provide key insight into their capacity to successfully establish in novel habitat outside their native range.
To address these knowledge gaps, we need to quantify the strength and form of natural selection acting on nesting behaviors and embryo reaction norms, as well as estimates of their heritability. Studies that address these topics are challenging, particularly in species that lay single egg clutches over long reproductive seasons, such as anoles. In particular, researchers would need to identify a suitable proxy for maternal fitness (i.e., hatching success of some or all the eggs produced by an individual) and examine its relationship with some aspect of nesting behavior (e.g., choice of nest habitat). Although this is inherently difficult with anoles due to the numerous nesting events of individuals throughout a reproductive season, these challenges can still be addressed with well-designed field or laboratory experiments that investigate selection on maternal nesting behaviors and embryo reaction norms for relevant traits. Additionally, lab-based quantitative genetics experiments that span generations could be designed to quantify the degree of heritability in maternal nest-site choice and embryo reaction norms. Such experiments have been conducted for thermal traits in anoles (Logan et al., 2018), and could be extended to traits associated with nesting ecology. Of course, such experiments are challenging, but creative experimental designs, dedicated research programs, and recent advances in quantitative genetic methods will help advance this poorly understood aspect of anole nesting ecology.
Comparative Studies of Embryo Physiology
Anolis has been used extensively in studies of comparative morphology and physiology; however, similar studies of embryos are lacking (but see Sanger et al., 2008b; Hall and Warner, 2019). Moreover, Anolis lizards are considered a model system for adaptive radiation, but studies never consider that embryo plasticity and egg survival may be an important driver of evolution (Kolbe et al., 2012a). This is important because egg survival can determine population cycles for anoles (Andrews, 1988), and likely plays a vital role in population viability, survival, and colonization success (Losos et al., 2003). Additionally, the anole radiation is characterized by multiple, independent dispersal events, often from and to small islands throughout the Caribbean (Poe et al., 2018; Huie et al., 2021). Although key innovations, phenotypic plasticity, niche expansion and other processes are considered important in such dispersals, these processes are typically evaluated from the perspective of adult phenotypes. Successful embryo development, however, is required for persistence in every environment. Comparative studies of embryo physiology and developmental plasticity would illuminate the importance of embryo adaptation in colonizing novel environments (e.g., urban landscapes) and responding to environmental perturbations caused by global change (e.g., climate change). Currently, data on nest conditions and embryo physiology are only available for a few species. More studies of nesting ecology and embryo development are needed for a variety of species from across the phylogenetic tree. Such comparative studies (which are currently underway, Muell et al., 2022) will enable phylogenetically-informed analyses of the macroevolution of developmental plasticity of anoles, and have the potential to provide novel insights into the role of nest environments in shaping the Anolis radiation.
Methods for Studying Anole Nesting
Quantitative data on anole nests in the field are needed to design laboratory experiments and interpret their results in an ecological context. Yet, this information remains largely unavailable for most species. Perhaps the primary reason why Anolis nesting ecology is poorly studied is due to the difficulty of finding nests in the field. Here we describe methods we have used to find nests in habitats that are commonly used in evolutionary and ecological studies of anoles: urbanized habitat (Winchell et al., 2016; Battles et al., 2019), densely forested habitat (Leal and Fleishman, 2002, 2004), and small islands (Schoener and Schoener, 1980; Losos et al., 1997; Campbell and Echternacht, 2003; Calsbeek and Cox, 2010; Kolbe et al., 2012b; Stuart et al., 2014). Importantly, anole biologists could incorporate our methods into their work to enhance understanding of Anolis ecology and evolution more generally. Our approaches were developed for studies of invasive anoles in Florida (A. sagrei and A. cristatellus), but our methods could be applied or modified for other species or in different habitats. Searching for small eggs is a daunting task, but to make searches manageable we limited the coverage to several 1 m2 quadrats at each location and used spoons to turn up 1–5 cm of soil and comb through the substrate. Although this protocol was used for both approaches, we describe below important differences in methodology in our different habitat types.
Nest Searching on Spoil Islands
Spoil islands have been important settings for Anolis research for several reasons (geographic separation, relatively easy population manipulations, etc.) (Campbell and Echternacht, 2003; Stuart et al., 2014; Pearson and Warner, 2018; Kahrl et al., 2021). Our study was conducted on a spoil island within the Guana Tolomato Matanzas National Estuarine Research Reserve in the Intracoastal Waterway near Palm Coast, Florida (Figure 2A). The island is approximately 90 m long and 60 m wide at its longest/widest points with a canopy of palm trees and red cedar trees (∼30 m in diameter) in the central part of the island, surrounded by low brush and dirt patches, and a periphery of needlerush (Juncus roemerianus) near the shoreline. This island contains a dense population of A. sagrei, but A. carolinensis is present in lower numbers. We installed a permanent 9 × 11 grid system across the island using PVC stakes (spaced 5 m from each other), resulting in eighty 5 m × 5 m square grids (Figure 2). Stakes along the eastern shoreline were occasionally swept away when parts of the island eroded due to waves. Such a grid system is useful for studies of nesting in anoles as well as studies of population ecology (Andrews, 1988). We credit Robin Andrews for the original use of such a system to study anole nesting.
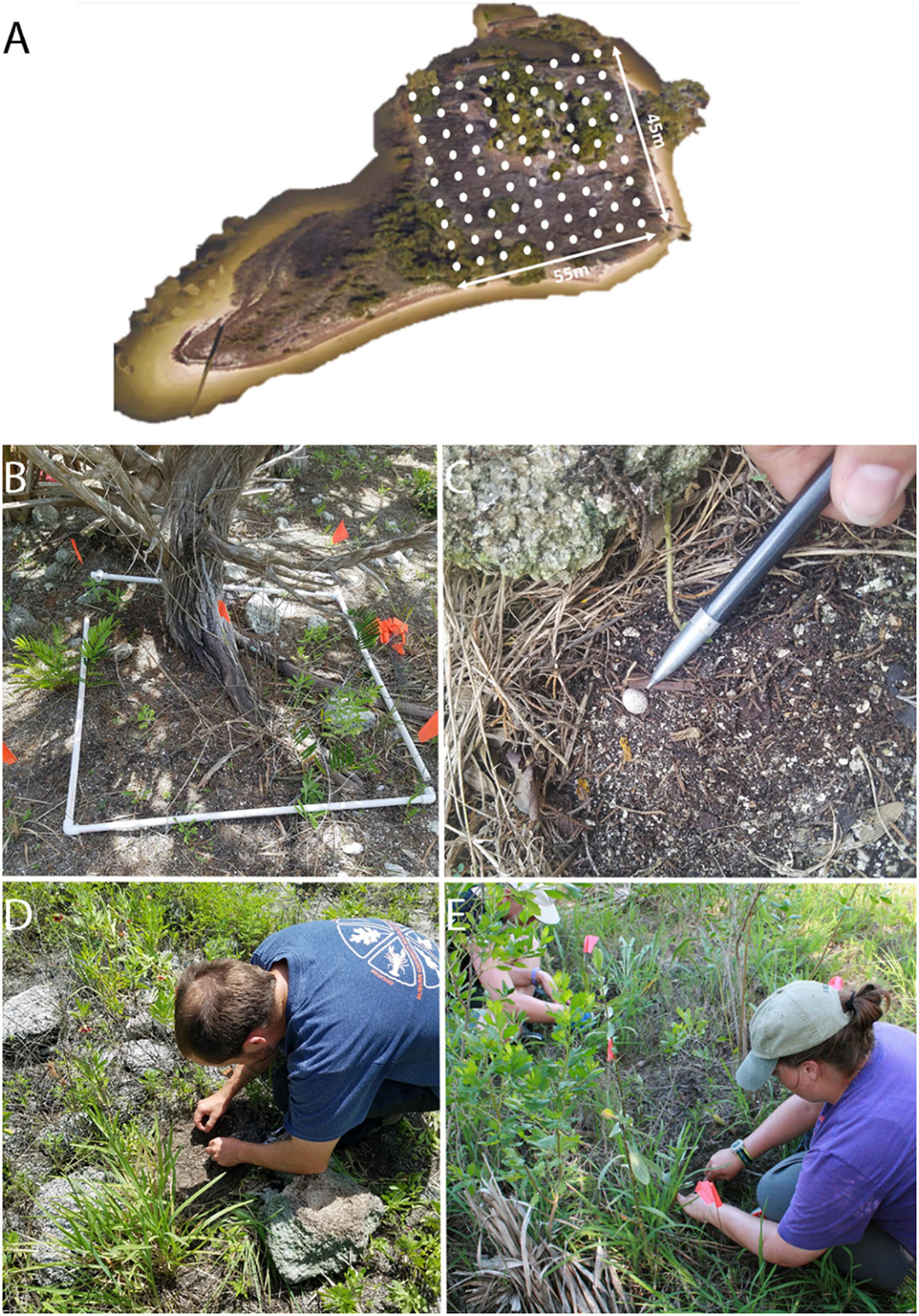
Figure 2. (A) An aerial view of the spoil island used for studying nesting behavior. Each white dot represents a stake in the grid system. (B–E) Photos from the island field site where we searched for anole nests. The 1 m2 quadrat that we used for searching (B), a brown anole egg found under a rock (C), a targeted search being conducted under rocks (D), and a random search being conducted in dense vegetation (E).
At this site, we used three separate search methods to reduce biased searching among microhabitats while ensuring sufficient sample sizes. These included selecting randomly-located quadrats (i.e., random searches), an intermediate method that combined random and non-random components (i.e., targeted-random searches), and non-randomly selecting quadrats (i.e., targeted searches). The random searches were performed to eliminate potential bias by covering as much of the island as possible and searching across all microhabitats. We used a random number generator to select a stake in the grid system, and then used the random number generator to select a distance (0–500 cm) and direction (0–359°) from each stake. We placed a 1 m2 quadrat over the resulting location and searched the square thoroughly, removing all potential cover objects and leaf litter. While this method reduces bias and covers all microhabitats, much of the landscape is not used for nesting and this method resulted in finding few nests. Thus, additional search methods were used.
For the targeted-random search method, we randomly selected a grid and then placed the 1 m2 quadrat in a location within the grid that we thought was most likely to contain an egg (based on a priori knowledge of where we find eggs; i.e., beneath cover objects in relatively shaded, moist areas). This method ensured that we covered a significant portion of the island and the various habitat types, but greatly increased sample size. For the targeted searches, we did not use the grid system, but searched the island for sites that looked suitable for egg incubation based on previous knowledge. The specific microhabitats that we targeted were based on anecdotal reports (e.g., in leaf litter, under cover objects, in moist areas, see review above and Supplementary Table 1). When an egg was located using the targeted search method, we placed the 1 m2 quadrat over the nest (with the egg in the middle) and searched the entire quadrat for eggs. We conducted 20 of each search type in each sampling period to get a thorough sampling of the island.
This combination of approaches was necessary to successfully locate nests while minimizing biased search effort. For instance, had we used only the random and random-targeted approaches, we would have found only 7 and 19 nests, respectively; this low sample size would not have given an accurate assessment of the range of microhabitats used for nesting. However, by including the targeted searches (by definition, a biased method), we found 131 nests with comparatively little effort. Additionally, nests found via targeted searches can be compared to those found via randomized searches to ensure that targeted searching does not introduce substantial bias. Moreover, microhabitat data (e.g., temperature, moisture, and canopy cover) should be collected from all sites, so that nest microhabitats can be compared with what is generally available across the island (e.g., comparing sites with and without nests; Tiatragul et al., 2020) and across search methods. Thus, we advocate for using a combination of approaches to (1) quantify the general characteristics of microhabitats available for nesting and (2) determine how these microhabitats compare to those selected by females.
Nest Searching in Suburban and Forested Habitats
Anoles have been a model species for studying urban ecology and evolution (Kolbe et al., 2012b, 2016; Winchell et al., 2016, 2018; Chejanovski et al., 2017; Lapiedra et al., 2017; Battles and Kolbe, 2018; Campbell-Staton et al., 2020; Lailvaux, 2020; Narváez et al., 2020) but few studies address aspects of nesting ecology (but see Tiatragul et al., 2017, 2019, 2020). The following protocol for nest searches was used in suburban and forested habitat in Miami-Dade County, Florida, United States (Figure 3). Our two sites included several species of anoles, but A. cristatellus and A. sagrei were most abundant (Battles and Kolbe, 2018; Tiatragul et al., 2019). The suburban site was along a 1-km stretch of a two-lane road (State Road 959, also known as “Red Road”) running parallel to a canal. The habitat consisted of mowed lawn, sparsely planted trees (including Ficus citrifolia, Ficus aurea, Sabal spp.) and many human-made structures like lamp posts, houses, bus stops, and guardrails. This area receives frequent vehicle and pedestrian traffic during the day and night. Searchable areas were also fragmented by roads, paved footpaths, and a canal. Additionally, because we were working next to a residential area, we limited our searches to public areas. As such, setting up a permanent grid system (as above) in this area was not preferable. The forested habitat was at Matheson Hammock Park which is a fragment of dense forest consisting of fig trees intermixed with smaller shrubs; this habitat had no human-made structures except for narrow walking trails. Since our studies (Tiatragul et al., 2019, 2020) aimed to compare characteristics of sites used by females for nesting to those not used by females, we decided that a limited random search was the best way to obtain an unbiased representation of maternal choice of nest microhabitats.
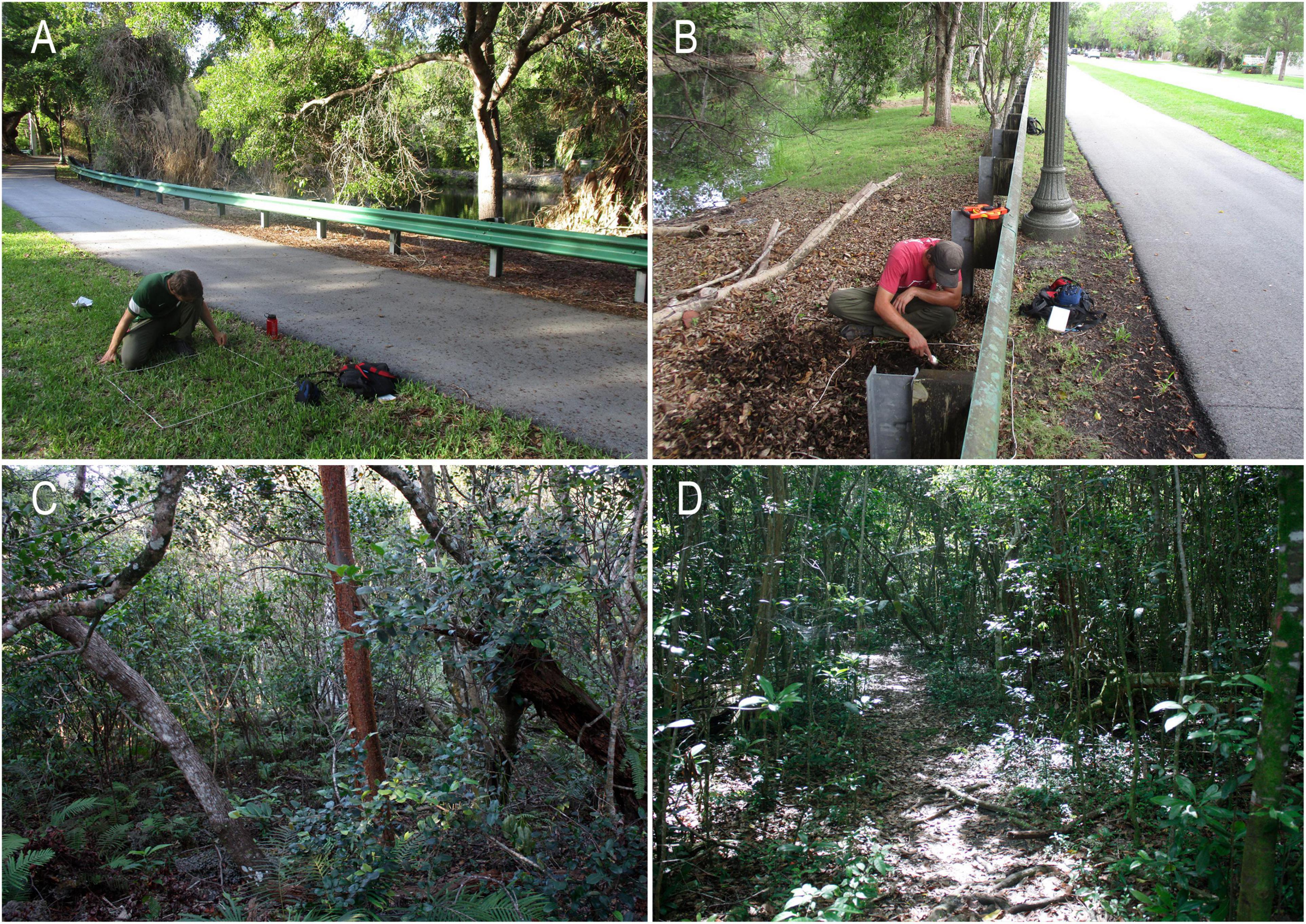
Figure 3. Photographs of suburban (A,B) and forested (C,D) habitats where anole nesting ecology was studied in Miami-Dade County, FL, United States. Note that the suburban site includes mowed lawn, roads, and a walking path. The same egg search methods were used in both habitats.
We chose four blocks within each habitat (suburban vs. forest) and searched 10 randomly selected 1 m2 plots in each block (Figure 4). We used four blocks because this number captured a representative area (with statistical replication) that anoles use throughout our habitats, but researchers may increase the number of blocks depending on other factors (e.g., size of the field site; diversity of microhabitat across the study site). Additionally, researchers could also include targeted searches (as described above) to increase representation of sites used for nesting if nests are difficult to find in some habitats (see Tiatragul et al., 2020).
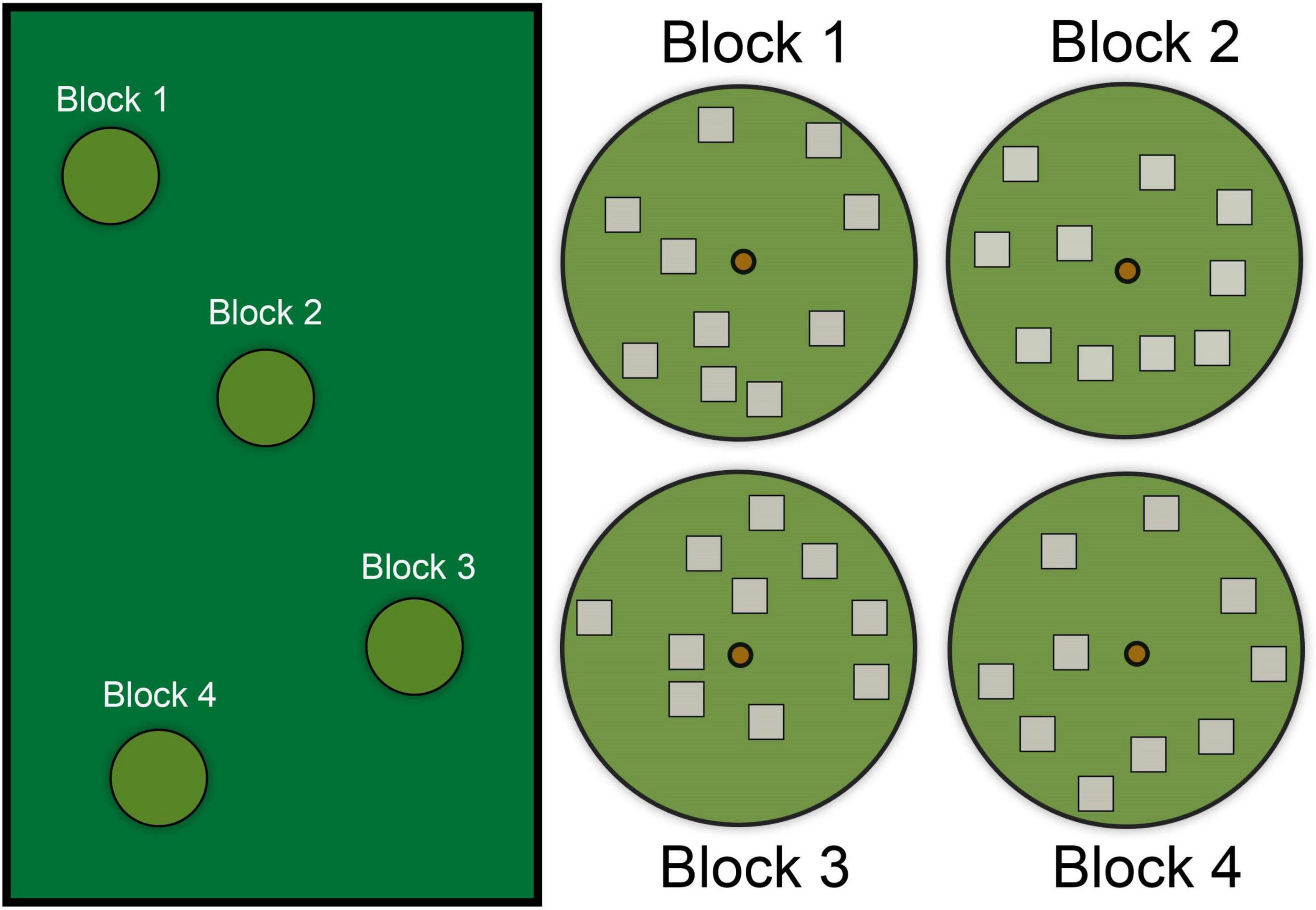
Figure 4. Diagram showing four hypothetical random search “blocks” within a larger habitat. Each “block” contains ten randomly distributed 1 m2 “plots.” The location of each block is selected within the larger habitat area based on the presence of a tree inhabited by anoles. The location of each plot is determined by random distance and direction (with or without constraints) from the center anchor (i.e., small circle in the center, which was a tree in our study). In this scenario, the circular block represents the limit of the radius from the anchor. Each plot is then searched thoroughly for anole eggs.
The location of each block was determined by the abundance of anoles to ensure that plots are sampled where nests are likely to occur. This was most critical in the suburban habitat where extensive areas were not suitable for nests (e.g., roads and buildings) or are not searchable (private property), but our block locations in the forest were not constrained because anoles were continuously abundant. Because A. cristatellus was the target species and they prefer relatively large, broad canopy trees, we chose four large trees in each habitat as the “anchor” point for each block. Thus, each block was a circle about the tree with radius of 20 m. Plots were selected using a random number generator to select a distance (1–20 m) and direction (0 – 359°) from the central tree. We created a 1 m2 quadrat at each plot and searched it for eggs as described previously (i.e., removing all cover, sifting through the soil with a spoon). We counted hatched and unhatched eggs. Hatched eggs are a good proxy for where females nest, as heavy rain and flooding did not wash away hatched eggshells indicating that eggshells likely remain in the location where females oviposited. However, we still recommend observing or experimentally determining whether hatched eggs are good proxies at other field sites. Overall, we found 44 plots with eggs (n = 31 in forest, n = 13 in suburb) and 36 plots (n = 9 in forest, n = 27 in suburb) without eggs (Tiatragul et al., 2020).
These search methods provide a promising avenue for future research. Finding anole eggs in the field outside of chance encounters was previously considered logistically prohibitive, but the work outlined here shows otherwise. We were able to successfully locate anole nests in three different habitats, and our methods produced sample sizes large enough to perform informative statistical analyses.
Limitations and Recommendations
To maximize the chance of locating eggs, researchers should align searches with reproductive cycles since eggs are not found in equal frequencies across the year. Moreover, the random, targeted-random, and targeted searches were not equally productive, and the methods researchers employ will depend on the questions asked and may vary among species. We recommend using a randomized search method to accompany targeted searching when seeking to draw conclusions about nest site choice as this will reduce bias while allowing for sufficient sample sizes. However, if the goal is simply to locate as many eggs as possible (e.g., measuring specific habitat features like temperature or sourcing eggs for lab experiments), using targeted searches would be expedient. Additionally, because anoles occasionally nest above ground, a systematic method for searching above-ground nest sites may be required in some habitats (see Andrews, 1988). Finally, our methods are likely most effective for species that are highly fecund and occur in high densities (e.g., A. sagrei). Although eggs could still be found using these methods for species that occur in lower densities, sample sizes would likely be much smaller and effort much greater. For example, although the crown giant, Anolis equestris, is relatively abundant at Matheson Hammock, we only found one egg of this species during our study.
Conclusion
The environmental conditions that females select for nesting have important effects on embryo development, egg survival, and fitness-related phenotypes of hatchlings. These consequences of the nest environment indicate that maternal nesting behavior should be under strong selection, and likely varies across species and populations depending upon several factors (e.g., local habitat and evolutionary history). As such, nesting behavior is an important aspect of reptile ecology, evolution and natural history. While anole nesting ecology is relatively understudied compared to other aspects of their biology, we argue that this group of lizards has several features that could advance knowledge of nesting behavior and developmental plasticity. Indeed, we highlight several recent studies of anoles that make progress toward understanding their nesting ecology, but much work remains. We hope that future studies will consider this radiation of lizards as a useful taxonomic group for research related to nesting ecology and evolution as well as developmental plasticity. With established protocols for locating and monitoring nests in the wild, breeding anoles in captivity, and incubating eggs and staging embryos in the laboratory, we have a complete toolkit to illuminate broader trends in ecological developmental biology, global change research, comparative embryo physiology, and the evolution of nesting behavior in vertebrates.
Author Contributions
JMH and DAW conducted comprehensive reviews of the literature on nesting and developmental plasticity. JEP, ST, and DAW developed nest searching methodology. JEP, JMH, and DAW contributed equally to writing the first draft of the manuscript. All authors contributed critically to the subsequent drafts and gave final approval for publication.
Funding
Financial support during the preparation of this manuscript was provided by the National Science foundation (CAREER Award DEB-1942145 to DAW; Graduate Research Fellowship Program and Postdoctoral Research Fellowship in Biology to JEP).
Conflict of Interest
The authors declare that the research was conducted in the absence of any commercial or financial relationships that could be construed as a potential conflict of interest.
Publisher’s Note
All claims expressed in this article are solely those of the authors and do not necessarily represent those of their affiliated organizations, or those of the publisher, the editors and the reviewers. Any product that may be evaluated in this article, or claim that may be made by its manufacturer, is not guaranteed or endorsed by the publisher.
Acknowledgments
We are grateful to members of the Warner and Wolak Labs for comments on early drafts of this manuscript.
Supplementary Material
The Supplementary Material for this article can be found online at: https://www.frontiersin.org/articles/10.3389/fevo.2022.821115/full#supplementary-material
References
Alfonso, Y. U., Charruau, P., Fajardo, G., and Estrada, A. R. (2012). Interspecific communal oviposition and reproduction of three lizard species in Southeastern Cuba. Herpetol. Notes 5, 73–77.
Allen, E. S., and Slatten, R. (1945). A herpetological collection from the vicinity of Key West, Florida. Herpetologica 3, 25–26.
Andrews, R. M. (1982). Spatial variation in egg mortality of the lizard Anolis limifrons. Herpetologica 38, 165–171.
Andrews, R. M. (1988). Demographic correlates of variable egg survival for a tropical lizard. Oecologia 76, 376–382. doi: 10.1007/BF00377032
Andrews, R. M., and Schwarzkopf, L. (2012). Thermal performance of squamate embryos with respect to climate, adult life history, and phylogeny. Biol. J. Linn. Soc. 106, 851–864. doi: 10.1111/j.1095-8312.2012.01901.x
Andrews, R. M., and Sexton, O. J. (1981). Water relations of the eggs of Anolis auratus and Anolis limifrons. Ecology 62, 556–562. doi: 10.2307/1937721
Angilletta, M. J. Jr., Sears, M. W., and Pringle, R. M. (2009). Spatial dynamics of nesting behavior: lizards shift microhabitats to construct nests with beneficial thermal properties. Ecology 90, 2933–2939. doi: 10.1890/08-2224.1
Battles, A. C., Irschick, D. J., and Kolbe, J. J. (2019). Do structural habitat modifications associated with urbanization influence locomotor performance and limb kinematics in Anolis lizards? Biol. J. Linn. Soc. 127, 100–112. doi: 10.1093/biolinnean/blz020
Battles, A. C., and Kolbe, J. J. (2018). Miami heat: urban heat islands influence the thermal suitability of habitats for ectotherms. Glob. Change Biol. 25, 562–576. doi: 10.1111/gcb.14509
Brown, K. M., and Sexton, O. J. (1973). Stimulation of reproductive activity of female Anolis sagrei by moisture. Physiol. Zool. 46, 168–172. doi: 10.1086/physzool.46.2.30155597
Calsbeek, R., and Cox, R. (2010). Experimentally assessing the relative importance of predation and competition as agents of selection. Nature 465, 613–616. doi: 10.1038/nature09020
Campbell, T. S., and Echternacht, A. C. (2003). Introduced species as moving targets: changes in body sizes of introduced lizards following experimental introductions and historical invasions. Biol. Invasions 5, 193–212. doi: 10.1023/A:1026172314139
Campbell-Staton, S. C., Winchell, K. M., Rochette, N. C., Fredette, J., Maayan, I., Schweizer, R. M., et al. (2020). Parallel selection on thermal physiology facilitates repeated adaptation of city lizards to urban heat islands. Nat. Ecol. Evol. 4, 652–658. doi: 10.1038/s41559-020-1131-8
Carr, A. (1940). A Contribution to the Herpetology of Florida, Vol. 3. Gainesville, FL: The University of Florida, 1–118.
Carter, A. L., and Janzen, F. J. (2021). Predicting the effects of climate change on incubation in reptiles: methodological advances and new directions. J. Exp. Biol. 224(Suppl._1):jeb236018. doi: 10.1242/jeb.236018
Chalcraft, D. R., and Andrews, R. M. (1999). Predation on lizard eggs by ants: species interactions in a variable physical environment. Oecologia 119, 285–292. doi: 10.1007/s004420050788
Chejanovski, Z. A., Avilés-Rodríguez, K. J., Lapiedra, O., Preisser, E. L., and Kolbe, J. J. (2017). An experimental evaluation of foraging decisions in urban and natural forest populations of Anolis lizards. Urban Ecosyst. 20, 1011–1018. doi: 10.1007/s11252-017-0654-5
Cook, J. M. (2002). “Sex determination in invertebrates,” in Sex Ratios: Concepts and Research Methods, ed. I. C. W. Hardy (Cambridge: Cambridge University Press), 178–194. doi: 10.1046/j.1432-1327.1999.00442.x
Croshaw, D. A., and Scott, D. E. (2005). Experimental evidence that nest attendance benefits female marbled salamanders (Ambystoma opacum) by reducing egg mortality. Am. Midl. Nat. 154, 398–411. doi: 10.1674/0003-0031(2005)154[0398:eetnab]2.0.co;2
Deeming, D. A., and Reynolds, S. J. (2015). Nests, Eggs, and Incubation: New Ideas about Avian Reproduction. New York, NY: Oxford University Press.
Dees, A., Wilson, K., Reali, C., Pruett, J. E., Hall, J. M., Brandt, R., et al. (2020). Communal egg-laying behaviour and the consequences of egg aggregation in the brown anole. Ethology 126, 751–760. doi: 10.1111/eth.13028
Delaney, D. M., Brandt, R., Beaumont, C. M., and Warner, D. A. (2021). Anolis sagrei (brown anole). Egg-carrying behavior. Herpetol. Rev. 52, 642–643.
Delaney, D. M., and Janzen, F. J. (2020). Risk-sensitive maternal investment: an evaluation of parent-offspring conflict over nest site choice in the wild. Anim. Behav. 163, 105–113. doi: 10.1016/j.anbehav.2020.03.004
Delaney, D. M., Reedy, R. M., Mitchell, T. S., Durso, A. M., Morrison, E. J., and Warner, D. A. (2013). Anolis sagrei (brown anole). Nest-site choice. Herpetol. Rev. 44:314.
DeSana, A., Fargevieille, A., and Warner, D. A. (2020). Survival of lizard eggs varies with microhabitat in the presence of an invertebrate nest predator. Evol. Ecol. 34, 483–499. doi: 10.1007/s10682-020-10058-w
Doody, J. S., Freedberg, S., and Keogh, J. S. (2009). Communal egg-laying in reptiles and amphibians: evolutionary patterns and hypotheses. Q. Rev. Biol. 84, 229–252. doi: 10.1086/605078
Doody, J. S., Guarino, E., Georges, A., Corey, B., Murray, G., and Ewert, M. (2006). Nest site choice compensates for climate effects on sex ratios in a lizard with environmental sex determination. Evol. Ecol. 20, 307–330. doi: 10.1007/s10682-006-0003-2
Doody, J. S., Soennichsen, K. F., James, H., McHenry, C., and Clulow, S. (2021). Ecosystem engineering by deep-nesting monitor lizards. Ecolgy 102:e03271. doi: 10.1002/ecy.3271
Downes, S., and Hoefer, A.-M. (2007). An experimental study of the effects of weed invasion on lizard phenotypes. Oecologia 153, 775–785. doi: 10.1007/s00442-007-0775-2
Du, W.-G., Ye, H., Zhao, B., Warner, D. A., and Shine, R. (2010). Thermal acclimation of heart rates in reptilian embryos. PLoS One 5:e15308. doi: 10.1371/journal.pone.0015308
Feiner, N. (2019). Evolutionary lability in Hox cluster structure and gene expression in Anolis lizards. Evol. Lett. 3, 474–484. doi: 10.1002/evl3.131
Feiner, N., Jackson, I. S. C., Munch, K. L., Radersma, R., and Uller, T. (2020). Plasticity and evolutionary convergence in the locomotor skeleton of Greater Antillean Anolis lizards. eLife 9:e57468. doi: 10.7554/eLife.57468
García-Padrón, L. Y. (2021). Cuban green anoles (Anolis porcatus): communal nesting in bromeliads. Reptiles Amphib. 28, 44–46. doi: 10.17161/randa.v28i1.15304
Godfray, H. C. J., and Parker, G. A. (1991). Clutch size, fecundity and parent-offspring conflict. Philos. Trans. R. Soc. Lond. B Biol. Sci. 332, 67–79. doi: 10.1098/rstb.1991.0034
Godfrey, S. T., Duberstein, J. A., Mota, J., and Moore, L. (2018). Anolis carolinensis (green anole). Nest sites and communal nesting. Herpetol. Rev. 49:115.
Goodman, R. M. (2008). Latent effects of egg incubation temperature on growth in the lizard Anolis carolinensis. J. Exp. Zool. 309A, 525–533. doi: 10.1002/jez.483
Goodman, R. M., and Heah, T. P. (2010). Temperature-induced plasticity at cellular and organismal levels in the lizard Anolis carolinensis. Integr. Zool. 5, 208–217. doi: 10.1111/j.1749-4877.2010.00206.x
Gordon, R. E. (1956). The Biology and Biodemography of Anolis carolinensis carolinensis Voigt. Ph.D. Dissertation. New Orleans, LA: Tulane University.
Grayson, K. L., Mitchell, N. J., Monks, J. M., Keall, S. N., Wilson, J. N., and Nelson, N. J. (2014). Sex ratio bias and extinction risk in an isolated population of tuatara (Sphenodon punctatus). PLoS One 9:e94214. doi: 10.1371/journal.pone.0094214
Greenberg, B., and Noble, G. K. (1944). Social behavior of the American chameleon (Anolis carolinensis Voigt). Physiol. Zool. 17, 392–439. doi: 10.1086/physzool.17.4.30151738
Hall, J. M., Miracle, J., Scruggs, C. D., and Warner, D. A. (2021). Natural nest substrates influence squamate embryo physiology but have little effect on hatchling phenotypes. Integr. Zool. [Online ahead of print]. doi: 10.1111/1749-4877.12553
Hall, J. M., and Sun, B. J. (2021). Heat tolerance of reptile embryos: current knowledge, methodological considerations, and future directions. J. Exp. Zool. 335, 45–58. doi: 10.1002/jez.2402
Hall, J. M., and Warner, D. A. (2018). Thermal spikes from the urban heat island increase mortality and alter physiology of lizard embryos. J. Exp. Biol. 221:jeb181552. doi: 10.1242/jeb.181552
Hall, J. M., and Warner, D. A. (2019). Thermal tolerance in the urban heat island: thermal sensitivity varies ontogenetically and differs between embryos of two sympatric ectotherms. J. Exp. Biol. 222:jeb210708. doi: 10.1242/jeb.210708
Hall, J. M., and Warner, D. A. (2020). Ecologically relevant thermal fluctuations enhance offspring fitness: biological and methodological implications for studies of thermal developmental plasticity. J. Exp. Biol. 223:jeb231902.
Hall, J. M., and Warner, D. A. (2021). Thermal sensitivity of lizard embryos indicates a mismatch between oxygen supply and demand at near-lethal temperatures. J. Exp. Zool. A 335, 72–85. doi: 10.1002/jez.2359
Hardy, J. D. (1957). Observations on the life history of the Cuban lizard, Anolis lucius. Herpetologica 13, 241–245.
Hare, K. M., Daugherty, C. H., and Cree, A. (2002). Incubation regime affects juvenile morphology and hatching success, but not sex, of the oviparous lizard Oligosoma suteri (Lacertilia: Scincidae). N. Z. J. Zool. 29, 221–229. doi: 10.1080/03014223.2002.9518306
Hare, K. M., Longson, C. G., Pledger, S., Daugherty, C. H., and Beaupre, S. J. (2004). Size, growth, and survival are reduced at cool incubation temperatures in the temperate lizard Oligosoma suteri (Lacertilia: Scincidae). Copeia 2004, 383–390. doi: 10.1643/CP-03-084R2
Huie, J. M., Prates, I., Bell, R. C., and de Queiroz, K. (2021). Convergent patterns of adaptive radiation between island and mainland Anolis lizards. Biol. J. Linn. Soc. 134, 85–110. doi: 10.1093/biolinnean/blab072
Hulbert, A. C., Hall, J. M., Mitchell, T. S., and Warner, D. A. (2020). Use of human-made structures, not thermal adaptation facilitates survival of a non-native ectotherm. Biol. Invasions 22, 2017–2031. doi: 10.1007/s10530-020-02236-2
Janzen, F. J., and Morjan, C. L. (2001). Repeatability of microenvironment-specific nesting behavior in a turtle with environmental sex determination. Anim. Behav. 62, 73–82. doi: 10.1006/anbe.2000.1732
Kahrl, A. F., Kustra, M. C., Reedy, A. M., Bhave, R. S., Seears, H. A., Warner, D. A., et al. (2021). Selection on sperm count, but not on sperm morphology or velocity, in a wild population of Anolis lizards. Cells 10:2369. doi: 10.3390/cells10092369
Kalaimani, A. (2015). Notes on egg laying sites of Calodactylodes aureus (Beddome, 1870) in Tirupattur forest division, Southern India. Herpetol. Bull. 131, 24–25.
Kamath, A., and Losos, J. (2017). The erratic and contingent progression of research on territoriality: a case study. Behav. Ecol. Sociobiol. 71:89. doi: 10.1007/s00265-017-2319-z
Kolbe, J. J., Leal, M., Schoener, T. W., Spiller, D. A., and Losos, J. B. (2012a). Founder effects persist despite adaptive differentiation: a field experiment with lizards. Science 335, 1086–1089. doi: 10.1126/science.1209566
Kolbe, J. J., VanMiddlesworth, P. S., Losin, N., Dappen, N., and Losos, J. B. (2012b). Climatic niche shift predicts thermal trait response in one but not both introductions of the Puerto Rican lizard Anolis cristatellus to Miami, Florida, USA. Ecol. Evol. 2, 1503–1516. doi: 10.1002/ece3.263
Kolbe, J. J., VanMiddlesworth, P., Battles, A. C., Stroud, J. T., Buffum, B., Forman, R. T. T., et al. (2016). Determinants of spread in an urban landscape by an introduced lizard. Landsc. Ecol. 31, 1795–1813.
Komduer, J., and Kats, R. K. H. (1999). Predation risk affects trade-off between nest guarding and foraging in Seychelles warblers. Behav. Ecol. 10, 648–658. doi: 10.1093/beheco/10.6.648
Kushlan, J. A., and Kushlan, M. S. (1980). Everglades alligator nests: nesting sites for marsh reptiles. Copeia 1980, 930–932. doi: 10.2307/1444493
Kwapich, C. L. (2021). Green anole (Anolis carolinensis) eggs associated with nest chambers of the trap jaw ant, Odontomachus brunneus. EcoEvoRxiv [Preprint] doi: 10.32942/osf.io/jw7rn
Lailvaux, S. P. (2020). It’s not easy being green: behavior, morphology, and population structure in urban and natural populations of green anole (Anolis carolinensis) lizards. Front. Ecol. Evol. 8:570810. doi: 10.3389/fevo.2020.570810
Lapiedra, O., Chejanovski, Z., and Kolbe, J. J. (2017). Urbanization and biological invasion shape animal personalities. Glob. Change Biol. 23, 592–603. doi: 10.1111/gcb.13395
Lapiedra, O., Schoener, T. W., Leal, M., Losos, J. B., and Kolbe, J. J. (2018). Predator-driven natural selection on risk-taking behavior in anole lizards. Science 360, 1017–1020. doi: 10.1126/science.aap9289
Latella, I. M., Poe, S., and Giermakowski, J. T. (2011). Traits associated with naturalization in Anolis lizards: comparison of morphological, distributional, anthropogenic, and phylogenetic models. Biol. Invasions 13, 845–856. doi: 10.1007/s10530-010-9873-x
Leal, M., and Fleishman, L. J. (2002). Evidence for habitat partitioning based on adaptation to environmental light in a pair of sympatric lizard species. Proc. R. Soc. Lond. B Biol. Sci. 269, 351–359. doi: 10.1098/rspb.2001.1904
Leal, M., and Fleishman, L. J. (2004). Differences in visual signal design and detectability between allopatric populations of Anolis lizards. Am. Nat. 163, 26–39. doi: 10.1086/379794
Lehtinen, R. M., Green, S. E., and Pringle, J. L. (2014). Impacts of paternal care and seasonal change on offspring survival: a multiseason experimental study of a Caribbean frog. Ethology 120, 400–409. doi: 10.1111/eth.12215
Logan, M. L., Curlis, J. D., Gilbert, A. L., Miles, D. B., Chung, A. K., McGlothlin, J. W., et al. (2018). Thermal physiology and thermoregulatory behaviour exhibit low heritability despite genetic divergence between lizard populations. Proc. R. Soc. B Biol. Sci. 285:20180697. doi: 10.1098/rspb.2018.0697
Losos, J., Warheitt, K., and Schoener, T. (1997). Adaptive differentiation following experimental island colonization in Anolis lizards. Nature 387, 70–73. doi: 10.1038/387070a0
Losos, J. B. (2009). Lizards in an Evolutionary Tree: Ecology and Adaptive Radiation of Anoles. Berkeley, CA: University of California Press.
Losos, J. B., Creer, D. A., Glossip, D., Goellner, R., Hampton, A., Roberts, G., et al. (2000). Evolutionary implications of phenotypic plasticity in the hindlimb of the lizard Anolis sagrei. Evolution 54, 301–305. doi: 10.1111/j.0014-3820.2000.tb00032.x
Losos, J. B., Schoener, T. W., and Spiller, D. A. (2003). Effect of immersion in seawater on egg survival in the lizard Anolis sagrei. Oecologia 137, 360–362. doi: 10.1007/s00442-003-1363-8
Martin, T. E. (1993). Nest predation and nest sites: new perspectives on old patterns. BioScience 43, 523–532. doi: 10.2307/1311947
Márquez, C. M., and Márquez, L. D. (2009). Reproductive biology in the wild and in captivity of Anolis aquaticus (Sauria: Polychrotidae) in Costa Rica. Bol. Técnico Ser. Zool. 8, 50–73.
Mazaris, D., Schofield, G., Gkazinou, C., Almpanidou, V., and Hays, G. C. (2017). Global sea turtle conservation successes. Sci. Adv. 3:e1600730. doi: 10.1126/sciadv.1600730
Mitchell, T. S., Janzen, F. J., and Warner, D. A. (2018). Quantifying the effects of embryonic phenotypic plasticity on adult phenotypes in reptiles: a review of current knowledge and major gaps. J. Exp. Zool. 329, 203–214. doi: 10.1002/jez.2187
Montgomery, C. E., Griffith Rodreguez, E. J., Ross, H. L., and Lips, K. R. (2011). Communal nesting in the Anoline lizard Norops lionotus (Polychrotidae) in central Panama. Southwest. Nat. 56, 83–88. doi: 10.1894/pas-16.1
Muell, M. R., Oaks, J. R., and Warner, D. A. (2022). The evolution of thermal developmental plasticity across Anolis lizards – a comparative approach. Integr. Comp. Biol.
Narváez, A. E., Ghia, T., Moretta-Urdiales, M. M., and Moreira, N. M. (2020). Feeding habits of Anolis sagrei, an introduced species, in urban ecosystems of Guayas Province. Urban Ecosyst. 23, 1371–1376. doi: 10.1007/s11252-020-00979-3
Noble, D. W. A., Stenhouse, V., and Schwanz, L. E. (2018). Developmental temperatures and phenotypic plasticity in reptiles: a systematic review and meta-analysis: incubation temperature and plasticity. Biol. Rev. 93, 72–97. doi: 10.1111/brv.12333
Pearson, P. R., and Warner, D. A. (2016). Habitat-and season-specific temperatures affect phenotypic development of hatchling lizards. Biol. Lett. 12:20160646. doi: 10.1098/rsbl.2016.0646
Pearson, P. R., and Warner, D. A. (2018). Early hatching enhances survival despite beneficial phenotypic effects of late-season developmental environments. Proc. R. Soc. B Biol. Sci. 285:20180256. doi: 10.1098/rspb.2018.0256
Poe, S., de Oca, A. N., Torres-Carvajal, O., de Queiroz, K., Velasco, J. A., Truett, B., et al. (2018). Comparative evolution of an archetypal adaptive radiation: innovation and opportunity in Anolis lizards. Am. Nat. 191, E185–E194. doi: 10.1086/697223
Propper, C. R., Jones, R. E., Rand, M. S., and Austin, H. (1991). Nesting behavior of the lizard Anolis carolinensis. J. Herpetol. 25, 484–486. doi: 10.2307/1564774
Pruett, J. E. (2021). Nesting Ecology of Reptiles: Effects of Maternal Nesting Behavior and Egg Incubation Environments on Offspring Phenotypes and Fitness. Ph.D. Dissertation. Auburn, AL: Auburn University.
Pruett, J. E., Fargevieille, A., and Warner, D. A. (2020). Temporal variation in maternal nest choice and its consequences on lizard embryos. Behav. Ecol. 31, 902–910. doi: 10.1093/beheco/araa032
Pruett, J. E., and Warner, D. A. (2021). Spatial and temporal variation in phenotypes and fitness in response to developmental thermal environments. Funct. Ecol. 35, 2635–2646. doi: 10.1111/1365-2435.13910
Reedy, A. M., Zaragoza, D., and Warner, D. A. (2013). Maternally chosen nest sites positively affect multiple components of offspring fitness in a lizard. Behav. Ecol. 24, 39–46. doi: 10.1093/beheco/ars133
Refsnider, J. M. (2015). Nest-site choice and nest construction in non-avian reptiles: evolutionary significance and ecological implications. Avian Biol. Res. 9, 76–88. doi: 10.3184/175815516x14490631289752
Refsnider, J. M., and Janzen, F. J. (2010). Putting eggs in one basket: ecological and evolutionary hypotheses for variation in oviposition-site choice. Annu. Rev. Ecol. Evol. 41, 39–57. doi: 10.1146/annurev-ecolsys-102209-144712
Resetarits, W. J. (1996). Oviposition site choice and life history evolution. Am. Zool. 36, 205–215. doi: 10.1093/icb/36.2.205
Robinson, C. D., Kircher, B. K., and Johnson, M. A. (2014). Communal nesting in the Cuban twig anole (Anolis angusticeps) from South Bimini, Bahamas. Reptiles Amphib. 21, 71–72. doi: 10.17161/randa.v21i2.13993
Roff, D. A. (1992). The Evolution of Life Histories: Theory and Analysis. New York, NY: Chapman and Hall.
Roughgarden, J. (1995). Anolis Lizards of the Caribbean: Ecology, Evolution, and Plate Tectonics. Oxford: Oxford University Press.
Sanger, T. J., Hime, P. M., Johnson, M. A., Diani, J., and Losos, J. B. (2008a). Laboratory protocols for husbandry and embryo collection of Anolis lizards. Herpetol. Rev. 39, 58–63.
Sanger, T. J., Losos, J. B., and Gibson-Brown, J. J. (2008b). A developmental staging series for the lizard genus Anolis: a new system for the integration of evolution, development, and ecology. J. Morphol. 269, 129–137. doi: 10.1002/jmor.10563
Sanger, T. J., and Kircher, B. K. (2017). Model clades versus model species: Anolis lizards as an integrative model of anatomical evolution. Methods Mol. Biol. 1650, 285–297. doi: 10.1007/978-1-4939-7216-6_19
Sanger, T. J., Kyrkos, J., Lachance, D. J., Czesny, B., and Stroud, J. T. (2018). The effects of thermal stress on the early development of the lizard Anolis sagrei. J. Exp. Zool. A 329, 244–251. doi: 10.1002/jez.2185
Sargent, R. C. (1988). Paternal care and egg survival both increase with clutch size in the fathead minnow, Pimephales promelas. Behav. Ecol. Sociobiol. 23, 33–37. doi: 10.1007/bf00303055
Schlaepfer, M. A. (2003). Successful lizard eggs in a human-disturbed habitat. Oecologica 137, 304–311. doi: 10.1007/s00442-003-1338-9
Schoener, T. W., and Schoener, A. (1980). Densities, sex ratios, and population structure in four species of Bahamian Anolis lizards. J. Anim. Ecol. 49, 19–53. doi: 10.2307/4276
Schulte, L. M., Ringler, E., Rojas, B., and Stynoski, J. L. (2020). Developments in amphibian parental care research: history, present advances, and future perspectives. Herpetol. Monogr. 34, 71–97. doi: 10.1655/herpmonographs-d-19-00002.1
Shine, R., and Du, W. G. (2018). How frequent and important is behavioral thermoregulation by embryonic reptiles? J. Exp. Zool. 329, 215–221. doi: 10.1002/jez.2153
Shine, R., and Harlow, P. S. (1996). Maternal manipulation of offspring phenotypes via nest-site selection in an oviparous lizard. Ecology 77, 1808–1817. doi: 10.2307/2265785
Socci, A. M., Schlaepfer, M. A., and Gavin, T. A. (2005). The importance of soil moisture and leaf cover in a female lizard’s (Norops polylepis) evaluation of potential oviposition sites. Herpetologica 61, 233–240. doi: 10.1655/04-67.1
Somaweera, R. (2009). Reproductive ecology of the Kandyan day gecko, Cnemaspis kandiana, in Gannoruwa forest reserve. J. Nat. Sci. Found. 37, 13–22. doi: 10.4038/jnsfsr.v37i1.453
Spencer, R.-J., and Thompson, M. B. (2003). The significance of predation in nest site selection of turtles: an experimental consideration of macro- and microhabitat preferences. Oikos 102, 592–600. doi: 10.1034/j.1600-0706.2003.12436.x
Stamps, J. A. (1976). Egg retention, rainfall and egg laying in a tropical lizard Anolis aeneus. Copeia 1976, 759–764. doi: 10.2307/1443460
Stuart, Y. E., Campbell, T. S., Hohenlohe, P. A., Reynolds, R. G., Revell, L. J., and Losos, J. B. (2014). Rapid evolution of a native species following invasion by a congener. Science 346, 463–466. doi: 10.1126/science.1257008F
Swierk, L., Carriere, A., Delfin, M., Fondren, A., Lopera, D., and Putnam, B. J. (2019). Anolis aquaticus (=Norops aquaticus) (Water Anole), egg size and oviposition site. Herpetol. Rev. 50, 360–362.
Tiatragul, S., Hall, J. M., Pavlik, N. G., and Warner, D. A. (2019). Lizard nests differ between suburban and forested habitats. Biol. J. Linn. Soc. 126, 392–403. doi: 10.1093/biolinnean/bly204
Tiatragul, S., Hall, J. M., and Warner, D. A. (2020). Nestled in the city heat; urban nesting behavior enhances embryo development of an invasive lizard. J. Urban. Ecol. 6:juaa001.
Tiatragul, S., Kurniawan, A., Kolbe, J. J., and Warner, D. A. (2017). Embryos of non-native anoles are robust to urban thermal environments. J. Therm. Biol. 65, 119–124. doi: 10.1016/j.jtherbio.2017.02.021
Tokarz, R. R., and Jones, R. E. (1979). A study of egg-related maternal behavior in Anolis carolinensis (Reptilia, Lacertilia, Iguanidae). J. Herpetol. 13, 283–288. doi: 10.2307/1563320
Uller, T. (2008). Developmental plasticity and the evolution of parental effects. Trends Ecol. Evol. 23, 432–438. doi: 10.1016/j.tree.2008.04.005
Valenzuela, N. (2004). “Temperature-dependent sex determination,” in Reptilian Incubation: Environment, Evolution and Behaviour, ed. C. Deeming (Nottingham: Nottingham University Press), 2004.
Warkentin, K. M. (2011). Environmentally cued hatching across taxa: embryos respond to risk and opportunity. Integr. Comp. Biol. 51, 14–25. doi: 10.1093/icb/icr017
Warner, D. A., and Chapman, M. N. (2011). Does solitary egg incubation enhance water uptake and offspring quality in a lizard that lays single-egg clutches? J. Exp. Zool. A 315, 149–155. doi: 10.1002/jez.660
Warner, D. A., Du, W. G., and Georges, A. (2018). Introduction to the special issue—developmental plasticity in reptiles: physiological mechanisms and ecological consequences. J. Exp. Zool. 329, 153–161. doi: 10.1002/jez.2199
Warner, D. A., Moody, M. A., and Telemeco, R. S. (2011). Is water uptake by reptilian eggs regulated by physiological processes of embryos or a passive hydraulic response to developmental environments? Comp. Biochem. Physiol. A Mol. Integr. Physiol. 160, 421–425. doi: 10.1016/j.cbpa.2011.07.013
Warner, D. A., Moody, M. A., Telemeco, R. S., and Kolbe, J. J. (2012). Egg environments have large effects on embryonic development, but have minimal consequences for hatchling phenotypes in an invasive lizard. Biol. J. Linn. Soc. 105, 25–41. doi: 10.1111/j.1095-8312.2011.01778.x
Warner, D. A., Pruett, J. E., Fargevieille, A., and Klabacka, R. (2021). Do female lizards choose nest sites based on the predictability of substrate moisture? Soc. Integr. Comp. Biol. 61, E960–E961.
Warner, D. A., and Shine, R. (2008a). Maternal nest-site choice in a lizard with temperature-dependent sex determination. Anim. Behav. 75, 861–870. doi: 10.1016/j.anbehav.2007.07.007
Warner, D. A., and Shine, R. (2008b). The adaptive significance of temperature-dependent sex determination in a reptile. Nature 451, 566–568. doi: 10.1038/nature06519
West-Eberhard, M. J. (2003). Developmental Plasticity and Evolution. Oxford: Oxford University Press.
While, G. M., Noble, D. W. A., Uller, T., Warner, D. A., Riley, J. L., Du, W.-G., et al. (2018). Patterns of developmental plasticity in response to incubation temperature in reptiles. J. Exp. Zool. 329, 162–176. doi: 10.1002/jez.2181
Winchell, K. M., Carlen, E. J., Puente-Rolón, A. R., and Revell, L. J. (2018). Divergent habitat use of two urban lizard species. Ecol. Evol. 8, 25–35.
Keywords: nesting, Anolis, oviparous, development, plasticity
Citation: Pruett JE, Hall JM, Tiatragul S and Warner DA (2022) Nesting in Anolis Lizards: An Understudied Topic in a Well-Studied Clade. Front. Ecol. Evol. 10:821115. doi: 10.3389/fevo.2022.821115
Received: 23 November 2021; Accepted: 15 March 2022;
Published: 09 May 2022.
Edited by:
J. Sean Doody, University of South Florida, United StatesReviewed by:
Matthew Gifford, University of Central Arkansas, United StatesElizabeth Addis, Gonzaga University, United States
Copyright © 2022 Pruett, Hall, Tiatragul and Warner. This is an open-access article distributed under the terms of the Creative Commons Attribution License (CC BY). The use, distribution or reproduction in other forums is permitted, provided the original author(s) and the copyright owner(s) are credited and that the original publication in this journal is cited, in accordance with accepted academic practice. No use, distribution or reproduction is permitted which does not comply with these terms.
*Correspondence: Jenna E. Pruett, amVubmEucHJ1ZXR0QGNvbG9yYWRvLmVkdQ==