- 1Department of Plants and Crops, Faculty of Bioscience Engineering, Ghent University, Ghent, Belgium
- 2Department of Evolutionary and Population Biology, Institute for Biodiversity and Ecosystem Dynamics, University of Amsterdam, Amsterdam, Netherlands
Host plant specialisation can promote evolutionary divergence between herbivore populations associated with different plant species. While the mechanisms by which specialist species exploit their hosts have been studied widely across taxa, less is known about the mechanisms that allow intraspecific variants to arise and to be maintained across spatial and temporal scales. To understand whether adaptations to plant defences against herbivory contribute to the co-existence of genetically distinct populations of an herbivore, we investigate the interaction between honeysuckle (Lonicera periclymenum) and sympatric specialist and generalist populations of the spider mite Tetranychus urticae. We found that mite folivory induces the production of sticky droplets on honeysuckle, which have a defensive role: they increase mite mortality directly, and potentially indirectly by increasing the arrestment of a predator. We show that droplet induction and the preference to feed on honeysuckle depend on mite genotype, where the generalist avoids this host and the specialist suppresses droplet production. These traits are heritable and dominant in F1 hybrids between generalists and specialists. Selection pressure from honeysuckle and differences in host preference likely reduce the opportunity of mating encounters on this host. We propose that the interplay between selection from host plant defences and ecological barriers to hybridisation contribute to the persistence of genetically distinct populations of a single species in sympatry.
Introduction
Identifying the role of natural selection in the formation and maintenance of biodiversity is a central goal in evolutionary biology. Selection acting on phenotypes that confer a fitness advantage in one particular habitat, but not in another, can result in a shift in the allele frequencies of selected loci in the populations adapted to each local habitat (Levene, 1953). The maintenance of locally adapted populations in an environment will largely depend on the balance between the strength of selection and the extent of gene flow experienced between populations (Felsenstein, 1981). Reproductive isolation due to the physical separation of locally adapted populations facilitates the fixation of different variants across the landscape (Wright, 1946). However, spatial mosaics of genetically distinct populations can also exist at small spatial distances, despite prevalent gene flow (Dobzhansky, 1941; Levin, 1995; Shafer and Wolf, 2013; Richardson et al., 2014). Adaptation to ecological factors can promote barriers to hybridisation between co-existing populations, for example, when decreasing the chance of mating encounters, or when selection acts against migrants or against hybrids that are less fit than their parents (Funk et al., 2006, 2011; Egan and Funk, 2009; Shafer and Wolf, 2013; Villa et al., 2019). A large portion of the empirical evidence demonstrating that natural selection and barriers to gene flow shape evolutionary divergence across phylogenetic lineages comes from studies of herbivorous arthropods (Hawthorne and Via, 2001; Via and West, 2008; Nyman et al., 2010; Funk, 2012; Nosil et al., 2012; Gompert et al., 2014; Roesti et al., 2015; Riesch et al., 2017; Doellman et al., 2019). Yet, the ecological and molecular mechanisms that contribute to the persistence of genetic variation within herbivorous arthropod species in nature remain poorly characterised, particularly for non-insect arthropods (Gloss et al., 2016).
Plant defences exert a strong selective pressure on herbivores. Defences can act directly on the attacking herbivore to deter its feeding or hamper its metabolism, or indirectly by attracting natural enemies of the herbivore (Sabelis et al., 2001; Kessler and Baldwin, 2002). Consequently, herbivores are under pressure to evolve mechanisms in order to cope with plant defences. These antagonistic interactions can drive the evolution of host plant specialisation, potentially resulting in host races with varying degrees of reproductive isolation (Futuyma and Peterson, 1985; Zandt and Mopper, 1998; Dres and Mallet, 2002). For example, natural selection promotes reproductive barriers between host races of Neochlamisus leaf beetles by acting against hybrids and migrants that cannot exploit or find non-native hosts (Funk, 2012). Similarly, variations in mouthpart morphology between host races of rhopalid bugs have a genetic basis, and selection acts against migrants and hybrids from non-native hosts, since they cannot access their food source due to morphological impairments (Carroll et al., 2001, 2005). Host races are thought to be prevalent in herbivorous mites (Helle and Sabelis, 1985; Magalhães et al., 2007). This is because most herbivorous mite species mate on the hosts on which they feed, so host preference has a major role in mate choice (Helle and Sabelis, 1985; Gotoh et al., 1993; Gomi and Gotoh, 1996). In addition, intrinsic genetic incompatibilities that result in reproductive isolation between mite populations are common (de Boer, 1980; Helle and Sabelis, 1985; Villacis-Perez et al., 2021).
To understand how selection from plant defences can promote the persistence of genetic variation in herbivores, we study populations of the two-spotted spider mite Tetranychus urticae. T. urticae is considered to be an extreme generalist that can be found in many plant species (Migeon et al., 2010), yet there is evidence that T. urticae is actually a cryptic complex of host-associated races (Gotoh et al., 1993; Hurtado et al., 2008; Villacis-Perez et al., 2021). A T. urticae race that has specialized on honeysuckle (Lonicera periclymenum) co-exists in the Dutch dunes with generalist conspecifics with larger host ranges, thus forming a persistent pattern of intraspecific genetic variation in the field. Genetic incompatibilities result in incomplete reproductive isolation between these mite populations, and the survival of generalists is lower than the host race on honeysuckle (Villacis-Perez et al., 2021). Upon herbivory, honeysuckle can increase herbivore mortality by producing droplets composed of a viscous, sticky liquid via foliar unicellular trichomes, similar to nectar-producing trichomes in the petals of Lonicera (Fahn, 1979; Fox, 1996; Weryszko-Chmielewska and Bozek, 2008). In addition, Lonicera species have a rich secondary metabolite profile, comprised predominantly of iridoid-glucosides and other glucoside derivatives that are known to deter insect herbivores (Calis et al., 1984; Calis and Sticher, 1984; Gardener and Gillman, 2002; Cipollini et al., 2008; Lieurance and Cipollini, 2013; Lieurance et al., 2015). Here, we investigate whether interactions with honeysuckle contribute to the co-existence of genetically distinct mite populations.
To understand how distinct mite populations can co-exist at such small spatial scales within an ecosystem we ask two main questions: First, does mite genotype influence the production of the droplets produced by honeysuckle? And second, do the droplets have a direct or indirect defensive role against mite herbivory? To answer these questions, we quantify the production of sticky droplets upon herbivory by generalist mites and by the honeysuckle race, and characterise the biochemical composition of the droplets produced. We also quantify droplet production upon treatments with jasmonic acid (JA) and salicylic acid (SA), since these phytohormones often regulate the production of sugary exudates (Heil et al., 2001; Pieterse et al., 2012). We manipulate the presence of sticky droplets on attacked honeysuckle leaves to investigate whether they play a defensive role against mites, either directly by increasing mite mortality, or indirectly by increasing the arrestment time of the omnivore mite Amblyseius andersoni, a natural enemy of spider mites also found along the Dutch dunes (Sabelis et al., 1999; van Rijn and Tanigoshi, 1999; Wäckers et al., 2007). We investigate whether the honeysuckle race suppresses droplet production, and the preference of generalists and the honeysuckle race to settle and oviposit on either honeysuckle or on another widespread plant species in the Dutch dunes, spindle tree (Euonymus europaeus). Finally, to investigate the genetic basis of droplet suppression and host preference, we quantify these traits in F1 hybrid females between generalist and honeysuckle race individuals.
Materials and Methods
Plants and Mites
Honeysuckle (L. periclymenum) plants from a local garden centre were defoliated, re-potted, and allowed to regrow inside individual mite-proof cages in the greenhouse of the University of Amsterdam set to 20°C, 60% relative humidity, and 16 h Light:8 h Dark period (from here on referred to as “standard conditions”). Prior to each experiment, leaves were collected from three to five different plants out of a batch of 14 plants, pooled together and then used randomly to create experimental arenas. Each arena consisted of a honeysuckle twig cut above and below the node of one pair of opposing leaves, flattened on wet cotton wool; an area of 2 × 3 cm was delimited with wet paper tissue on each leaf. The basal and apical ends of each stalk were embedded in the wet cotton wool. Spindle tree (E. europaeus) leaves were collected from plants growing in the vicinity of the University of Amsterdam. Shortly prior to experiments, spindle tree leaves without signs of herbivory were collected from two to three different plants, pooled together and then used randomly for the experimental replicates. Common bean (Phaseolus vulgaris, cultivar “Speedy”) plants were sown at the University of Amsterdam in standard conditions.
Virgin T. urticae females were originally collected from dune ecosystems near Castricum and Meijendel, The Netherlands in 2015 and used to start iso-female lines. These lines were since then maintained on detached bean leaves surrounded by wet cotton wool under standard conditions. Each iso-female was classified as one of three genetically distinct populations of T. urticae commonly found in the Dutch dunes; following the nomenclature of Villacis-Perez et al. (2021), in which Cx represents the mitochondrial haplotype and Nx the nuclear genotype, population 1 is the honeysuckle race (lines C1N1a, C1N1b, and C1N1d), population 2 (lines C2N3a, C2N2b, and C2N2d), and population 3 (lines C3N3a, C3N3b, and C3N3d) are generalist populations occurring in the same ecosystem. For simplicity, lines from the honeysuckle race will be referred to as “specialists” and lines from the generalist populations as “generalists” from hereon.
A colony of the omnivore A. andersoni was obtained from Koppert Biological Systems. The colony was maintained on a plastic surface surrounded by wet paper tissue inside a mite-proof cage and maintained on a diet of Typha spp. pollen under standard conditions. One hour prior to experiments, adult A. andersoni females were starved by isolating them on a glass Petri dish surrounded by a water barrier without food.
Production of Sticky Droplets on Honeysuckle Leaves
We randomly selected one line of the specialist and generalist populations (lines C1N1a and C2N3a, respectively) and quantified the number of droplets produced when they fed on honeysuckle leaves. Experimental arenas (n = 6) exposing the abaxial surface and the adaxial surface were infested with five adult females of each line; a non-infested control was also included. The number of droplets produced was counted every day for five consecutive days. Females that died during the experiment were replaced with females from the same line. Since the droplets remained on the leaf surface throughout the duration of the experiment, we quantified the total number of droplets produced over the 5-day experiment. The total number of droplets between mite lines was compared using a linear model (command aov in R v3.6.1) with iso-female line as fixed factor, square-root transformed to improve model fit. Significant differences between groups were further analysed using a generalised linear hypothesis test with a Tukey correction for multiple testing (comman glht in R v.3.6.1).
Sticky Droplets as Direct and Indirect Defence Mechanisms of Honeysuckle
Effect of Sticky Droplets on Juvenile Spider Mite Survival
We quantified the effect of the presence of droplets on juvenile survival to adulthood of generalist line C2N3a in two experimental blocks. For each block, we infested the abaxial surface of experimental arenas with five adult female mites and let them feed and oviposit. After 48 h, we removed eggs, web, and droplets and allowed adult females to further feed and oviposit. Twenty-four hours later, we counted the newly formed droplets, removed the adult females, and reduced the number of eggs to a total of 12 eggs per arena. At this point, we divided the arenas into two treatments: one with sticky droplets and one without them, where droplets were removed manually with a fine brush daily (n = 6 replicates per treatment, per block). The proportion of juvenile survival was quantified every 2 days across five consecutive time points, spanning from egg hatching on day five, until reaching adulthood by day 13. To analyse the differences in juvenile survival we fit a Cox proportional hazards model with a log-rank test (package survival v. 3.2-7), with treatment as main factor and experimental block as a covariate.
Effect of Sticky Droplets on the Arrestment Time of A. andersoni
To infer the indirect defensive role of the droplets, we quantified the arrestment time of the predatory mite A. andersoni (van Rijn and Sabelis, 1990). We quantified the proportion of predators present on honeysuckle with and without sticky droplets. Each replicate consisted of an arena made from a honeysuckle leaf with the abaxial surface facing up, infested with either ten adult specialist females (line C1N1a) or with five adult generalist females (line C2N3a). The numbers of generalist and specialist spider mites used were different because the number of droplets produced after herbivory by specialists is lower than those produced after herbivory by generalists (see section “Results”). After 48 h, we removed adult mites and counted the number of spider mite eggs and droplets. The droplets from half of the experimental arenas were manually removed with a brush, creating a total of four treatments: arenas where generalist mites fed, with and without droplets, and arenas where specialist mites fed, with and without droplets (n = 12 replicates per treatment across two experimental blocks). Then, five starved adult A. andersoni females were introduced to each arena. Every hour for a total of 9 h, the number of A. andersoni females present in each arena was counted. To analyse the differences in arrestment time we fit a Cox proportional hazards model with a log-rank test (package survival v. 3.2-7), with treatment as main factor and experimental block as a covariate.
Spider Mite Adaptations to Honeysuckle
Suppression of Droplet Production by Specialists
To investigate whether the specialists suppress the production of sticky droplets, rather than fail to induce it, we compared the total number of droplets produced in four treatments. First, experimental arenas with one leaf exposing the abaxial surface were each infested with either five adult specialist or with five generalist females (lines C1N1a and C2N3a, respectively). After 2 days, females, eggs, web, and droplets were removed from the arenas and each was re-infested with five adult females in order to create four treatments: (1) generalist mites fed first and were replaced with generalist mites; (2) generalist mites fed first and were replaced with specialist mites; (3) specialist mites fed first and were replaced with generalist mites; and (4) specialist mites fed first and were replaced with specialist mites (n = 6 replicate for each treatment). Dead and missing females were replaced daily. We compared the total number of droplets by day four between treatments using a linear model (package aov in R v3.6.1; square-root transformed to improve model fit) with treatment as fixed factor. Significant differences were further analysed using a generalised linear hypothesis test with a Tukey correction for multiple testing.
Quantification of Host Preference by Spider Mites
We quantified mite preference for feeding and ovipositing on a particular host in a two-choice experiment. First, we synchronised the age of experimental individuals by collecting approximately 200 adult females from the specialist and generalist lines C1N1a and C2N3a, and transferring them to separate, detached bean leaves. Females were transferred to fresh bean leaves every other day to ensure a steady supply of age-synchronised mite cohorts. Then, honeysuckle and spindle tree leaf discs (15 mm diameter) were cut in half. Each experimental unit consisted on two half discs exposing the abaxial surface of the leaf, touching each other through the straight edge so mites could walk freely to either side. We embedded each pair of half discs on wet cotton wool. Ten adult female mites from the synchronised cohorts were first transferred to a small plastic square, which was then placed on top of the separation between the two half discs. We counted the number of females on each side of the disc after 1 h and the number of eggs on each half disc after 24 h. We created four different treatments: (1) honeysuckle vs. spindle tree (n = 30 pairs of discs per line) to assess host preference; (2) honeysuckle leaves on which specialist mites had fed on for at least 48 h vs. honeysuckle without previous herbivory (n = 10); (3) honeysuckle leaves on which generalist mites had fed on for at least 48 h vs. honeysuckle leaves without previous herbivory (n = 10); and (4) honeysuckle leaves on which specialist mites had fed on for at least 48 h vs. honeysuckle leaves on which generalist mites had fed on for at least 48 h (n = 10) to investigate whether host preference was altered by previous mite feeding. We compared the proportion of individuals and eggs present on honeysuckle relative to spindle tree to a 0.5:0.5 distribution using a chi-square test for given probabilities (package stats in R v3.6.1).
Quantification of Reproductive Performance per Host Plant Species
We isolated individuals in the last moulting stage before adulthood from specialist line C1N1a and generalist line C2N3a on bean leaves. Mites hatched after 24 h, and 48 h later, we transferred five adult virgin females to a leaf disc of either honeysuckle or spindle tree exposing the abaxial surface and embedded in wet cotton wool (n = 6 per plant species, per mite line). Every 24 h we scored the number of dead females. After a total of 4 days, we counted the total number of eggs on each disc. We compared the mean number of eggs per mite alive per day within each mite line using a linear model (package aov in R v3.6.1, square-root transformed to meet model requirements) with host species as fixed factor.
Inheritance of Adaptive Traits in Hybrid Offspring Between Generalists and Specialists
Sticky Droplet Production Upon Herbivory by F1 Hybrids
To determine whether suppression of droplet production in these mites is genetically determined, we compared the number of droplets produced upon feeding by specialist and generalist females to the amounts produced by their F1 hybrid offspring. Recently hatched parental and F1 hybrid virgin females from crosses between lines C1N1a and C2N3a were isolated on separate bean leaf discs for 48 h before placing them on honeysuckle arenas. Experimental arenas with one leaf exposing the abaxial surface were either infested with five adult females from the specialist line, the generalist line, or from the two reciprocal F1 hybrids. We compared the total number of droplets produced by day four using a linear mixed-effects model (package lme4 in R v3.6.1), square-root transformed to improve model fit with mite genotype as fixed factor, and the number of females alive by the end of the experiment as a random factor. Because the number of F1 females obtained was low due to innate genetic incompatibilities between specialists and generalists (Villacis-Perez et al., 2021), dead females were not replaced daily in any of the treatments. A p-value was obtained using a Satterthwaite’s approximation (package lmerTest in R v3.6.1).
Quantification of Host Preference in F1 Hybrids
To determine whether host preference in these mites is genetically determined, preference for ovipositing by F1 hybrid females was quantified. F1 hybrid females were collected from controlled crosses between lines C1N1a and C2N3a. Both F1 hybrid and parental juvenile females in the last quiescent stage before hatching were isolated on separate bean leaf discs for 48 h before the experiments. Because F1 hybrid females of specialists and generalists produce fewer eggs than the parental lines (Villacis-Perez et al., 2021), the number of eggs was counted after 48 h instead of 24 h, like the parental lines. Host preference and the proportion of eggs present on each host were analysed as described in the section “Quantification of Host Preference by Spider Mites.”
Results
Production of Sticky Droplets on Honeysuckle Leaves
The number of droplets produced by honeysuckle leaves differed significantly after feeding by specialist lines, generalist lines and controls (F2,15 = 13.70, p < 0.001). Specialists induced a much lower number of droplets than generalists, while controls without mites did not produce droplets (Figure 1). Close to mite feeding spots, a burst of an aqueous substance appears under the epidermis and expands through the leaf laminae across contiguous areas delimited by the leaf’s veins. The aqueous burst in the leaf lamina eventually resides, but the droplet remains on the leaf surface (Supplementary Videos 1, 2). Performing the experiment with more lines belonging to the mite populations found in the dunes yielded similar results, where mite population had a significant effect on the number of droplets produced (F5,5.7 = 6.11, p = 0.026). Specialist lines induced significantly fewer droplets than lines from the two generalist populations; neither thrips larvae nor controls induced droplet production (Supplementary Figure 1). Increasing the densities of mites feeding on honeysuckle leaves increased droplet production, but this effect was dependent on the mite line, as inferred by the significantly different slopes of the regression lines (interaction between mite density and mite line: F1,44 = 17.37, p < 0.001; Supplementary Figure 2). Honeysuckle twigs embedded in either JA or SA produced a significantly higher number of droplets than control twigs embedded in a mock solution, both upon feeding by specialist mites (F2,12 = 8.19, p = 0.006) and upon feeding by generalist mites (F2,12 = 9.97, p < 0.003; Supplementary Figure 3). The droplets contain fructose, glucose and sucrose, and at least 17 out of 20 amino acids tested (Supplementary Table 2).
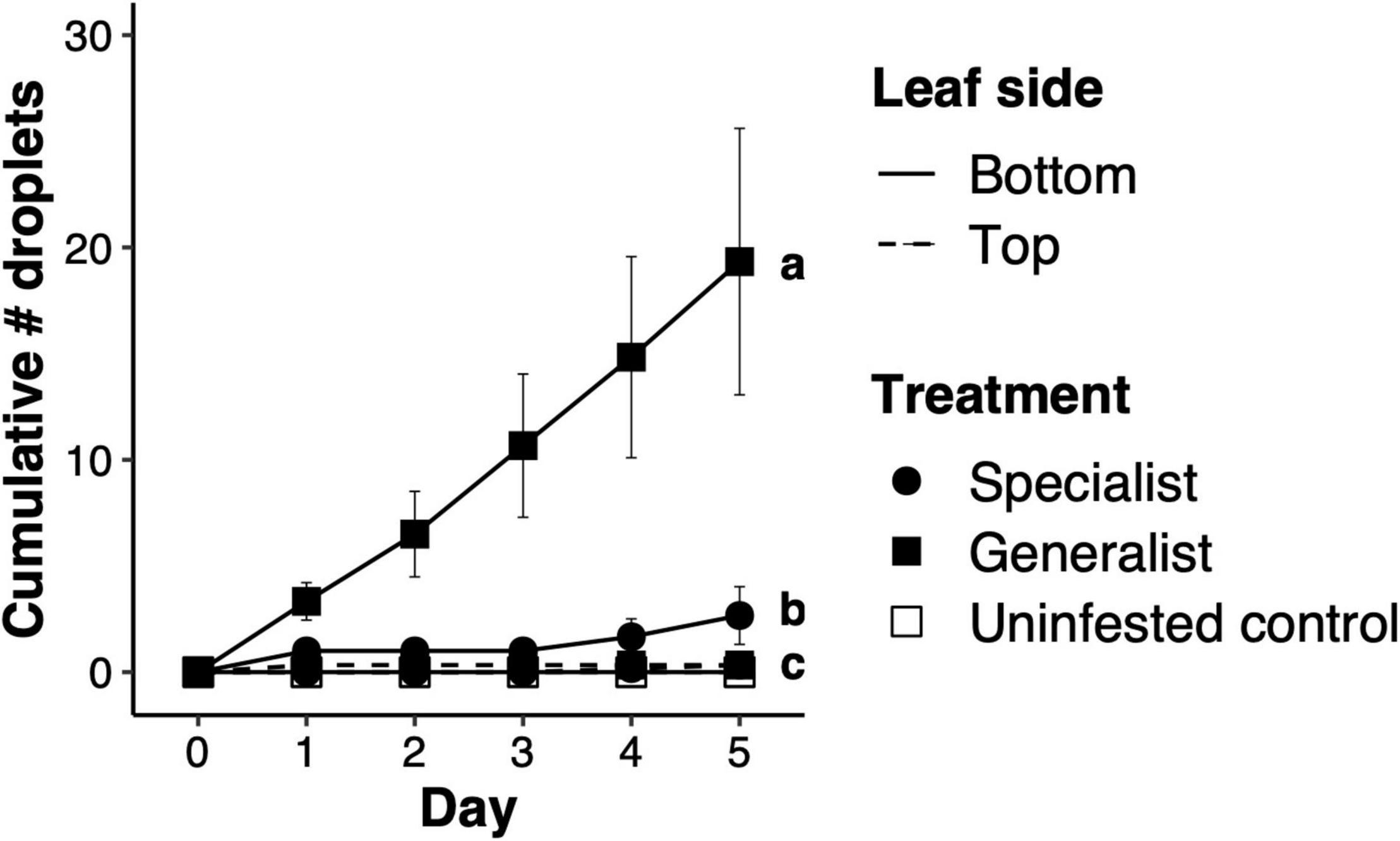
Figure 1. Production of sticky droplets on honeysuckle leaves upon spider mite herbivory. Total number of sticky droplets [mean ± one standard error of the mean (SEM)] exuded on either surface of honeysuckle leaves per consecutive day. “Specialist” and “Generalist” are field-derived lines representing the honeysuckle race of Tetranychus urticae and a generalist conspecific, respectively. Leaves either exposed the adaxial surface (“Top”) or the abaxial surface (“Bottom”). Letters next to symbols on day five indicate significant differences between treatments.
Sticky Droplets as a Defence Mechanism of Honeysuckle
Effect of Sticky Droplets on Mite Survival
The mortality of generalist juveniles was significantly higher on leaves with sticky droplets than on leaves without droplets [χ2 = 39.9, degrees of freedom (df) = 2, p < 0.001; Figure 2A]. Juvenile mortality increased in replicates with droplets because they either got stuck on the droplets or because they got trapped in the wet barriers surrounding the experimental arenas.
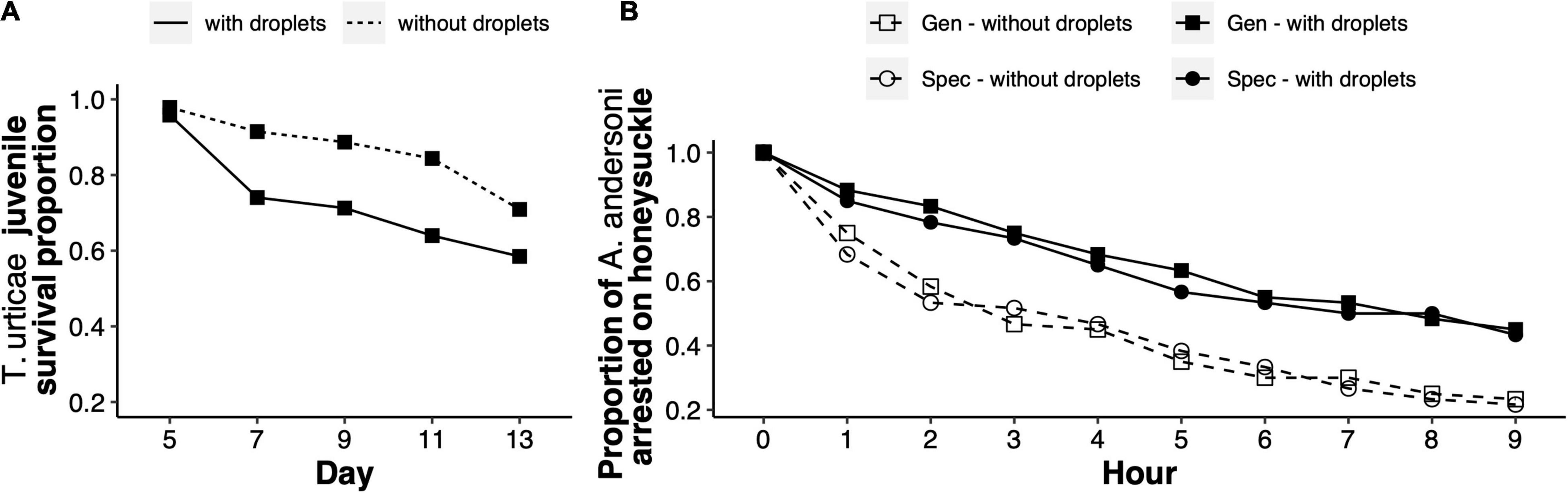
Figure 2. Sticky droplets on honeysuckle leaves as a direct and indirect defence mechanism. (A) Survival proportion of Tetranychus urticae generalist juveniles on honeysuckle leaves either with sticky droplets (solid line), or without sticky droplets (dashed line). Survival proportion was measured across five consecutive time points, spanning from egg hatching in day five, to reaching adulthood by day 13. (B) Proportion of Amblyseius andersoni adult females on honeysuckle leaves with previous herbivory from either generalist or specialist spider mites, and in which sticky droplets were either kept or removed manually (legend), across nine consecutive hours.
Interactions Between A. andersoni and Sticky Droplets
The arrestment time of A. andersoni on honeysuckle significantly increased with the presence of sticky droplets (experimental block 1: χ2 = 23.5, df = 3, p < 0.001; experimental block 2: χ2 = 51.8, df = 3, p < 0.001; Figure 2B). Arrestment time was significantly higher on leaves with droplets produced after generalist feeding (experimental block 1: χ2 = 14.0, df = 1, p < 0.001; experimental block 2: χ2 = 26.4, df = 1, p < 0.001), and on leaves with droplets produced after specialist feeding (experimental block 1: χ2 = 8.7, df = 1, p = 0.003; experimental block 2: χ2 = 25.2, df = 1, p < 0.001). Thus, predatory mites spend more time on honeysuckle leaves with sticky droplets than on leaves without droplets, but not because predators get stuck on the droplets, even though they do interact physically with them (Supplementary Video 3).
Spider Mite Adaptations to Honeysuckle
Suppression of Droplet Production by Specialist Mites
The total number of droplets produced by honeysuckle leaves upon herbivory by a mite genotype following herbivory by another mite genotype was significantly different between treatments (F3,112 = 27.85, p < 0.001; Figure 3). Replicates where the specialist mites fed before the generalist mites produced significantly fewer droplets than replicates upon which only generalist mites fed, while replicates in which the generalist mites fed first and then specialist mites fed, and in those were only the specialist fed, produced very few or nearly no droplets at all (Figure 3). Feeding by specialist mites impairs the production of droplets on honeysuckle leaves, even when droplet production is induced by generalist mites feeding either before or after specialist feeding.
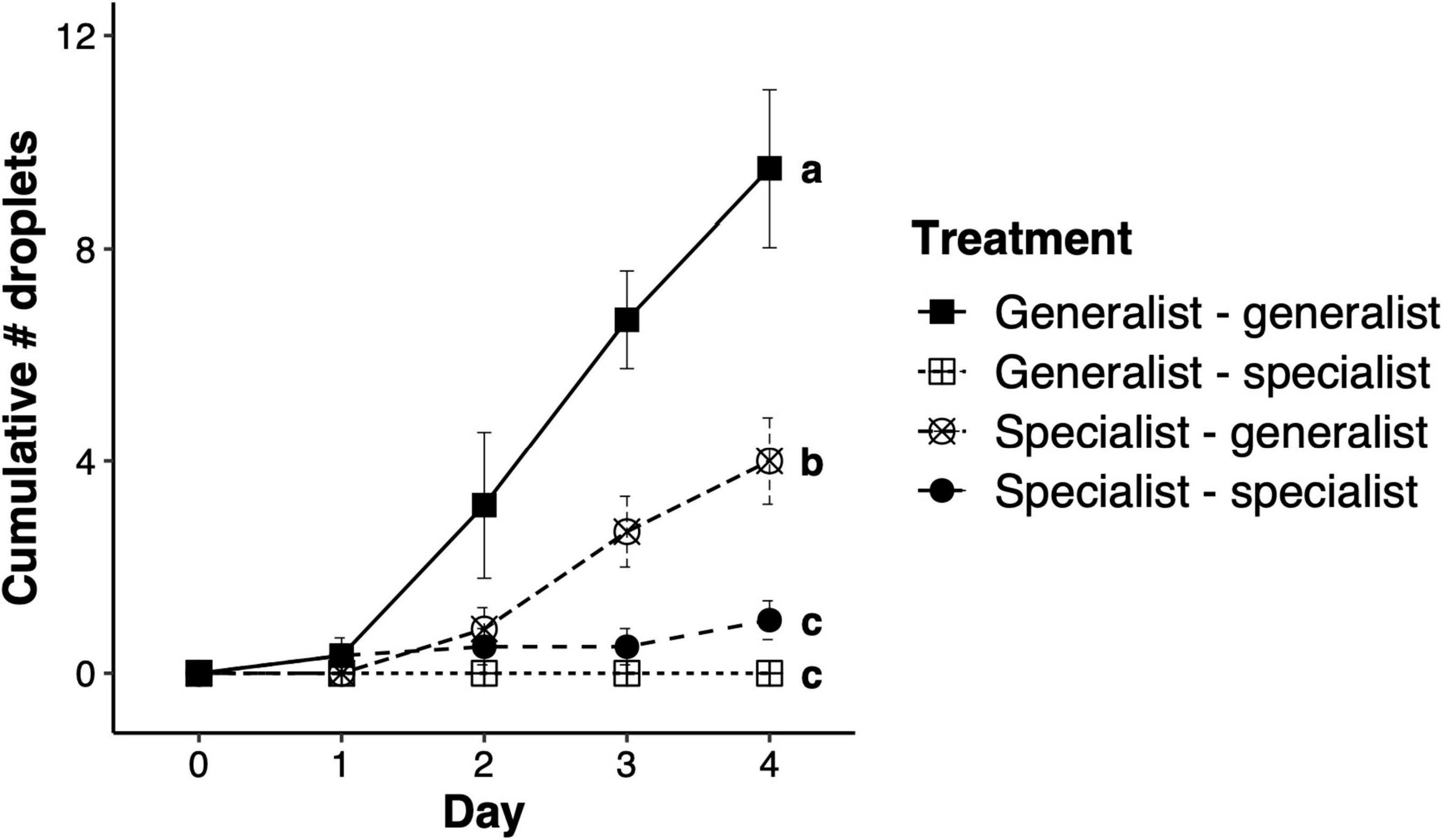
Figure 3. Suppression of sticky droplets on honeysuckle after specialist spider mite feeding. Total number of droplets (mean ± SEM) produced after spider mite herbivory by honeysuckle over four consecutive days. Treatments in the legend are based on the combination of mite herbivory on honeysuckle leaves 2 days before day 0 (by genotype left of the dash) and after day 0 (by genotype right of the dash). Letters next to data points at day four represent significant differences between treatments.
Mite Host Preference
The percentage of specialist mites feeding on honeysuckle 1 h after introduction to the experimental choice arena was not significantly different relative to spindle tree. However, a significantly lower percentage of generalist mites were found feeding on honeysuckle relative to spindle tree after 1 h (χ2 = 503.93, df = 1, p < 0.001; Figure 4A). Similarly, the percentage of eggs laid by specialist mites on honeysuckle did not differ from the percentage of eggs laid on spindle tree after 24 h, but the percentage of eggs laid by generalist mites on honeysuckle after 24 h was significantly lower than on spindle tree (χ2 = 32.32, df = 1, p < 0.001; Figure 4B). The percentage of eggs laid by specialist mites only differed significantly when laying a higher percentage of eggs on leaves where generalist mites fed on previously than on leaves without previous herbivory (χ2 = 4.2453, df = 1, p = 0.04; Supplementary Figure 4A). The percentage of eggs laid by generalist mites on honeysuckle leaves without previous herbivory was significantly higher than on leaves that had previously been used by specialist mites (χ2 = 14.107, df = 1, p < 0.001) or generalist mites (χ2 = 7.5, df = 1, p = 0.006). However, generalist mites laid as many eggs on leaves upon which either specialist or generalist mites fed upon previously (Supplementary Figure 4B). These data show that specialist mites did not prefer to feed or oviposit on either honeysuckle or spindle tree, but instead a large percentage of generalist mites avoid feeding and ovipositing on honeysuckle. Female mortality due to feeding on either host was not observed in our experiments, but some mortality did occur when mites got trapped on the wet cotton surrounding the experimental set-up (between 25 and 30% mortality). However, this cause of death was similar between the two lines, both 1 h and 24 h after introducing the mites to the experimental set up. The reproductive performance of the mite lines differed per plant species. Specialist mites laid a similar number of eggs on spindle tree and honeysuckle, but generalist mites laid significantly fewer eggs on honeysuckle than on spindle tree (generalist line: F2,15 = 67.004, p < 0.001; Figure 4C).
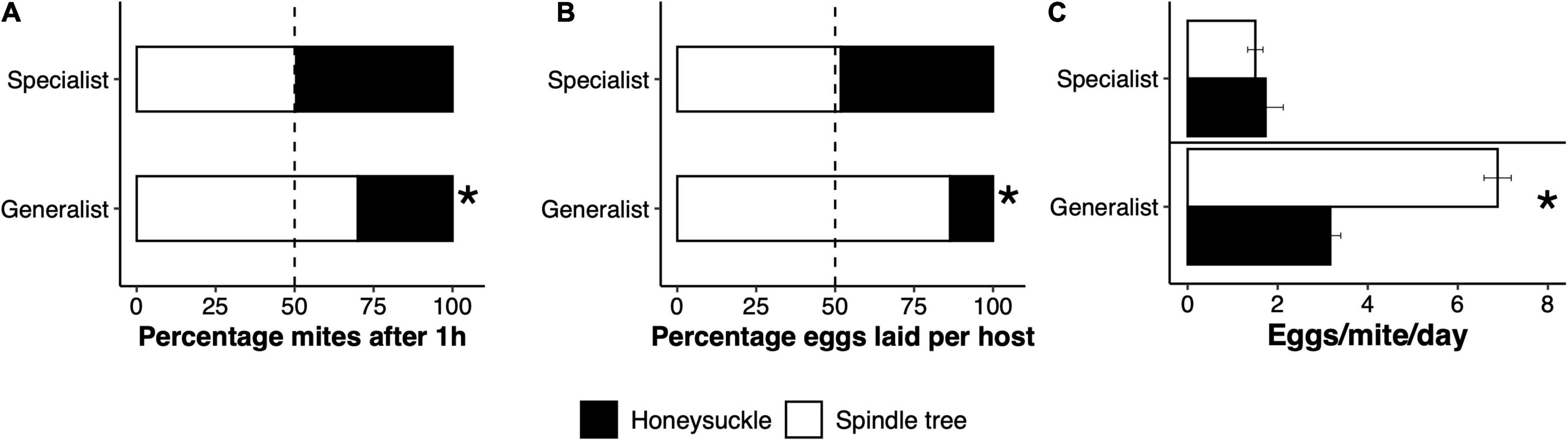
Figure 4. Adaptive avoidance of honeysuckle by generalist spider mites. In a two-choice experiment, generalist Tetranychus urticae females significantly preferred to feed on spindle tree after probing (A), and to oviposit most of their eggs on this host (B), rather than on honeysuckle. In a no-choice experiment, generalist mites laid significantly fewer eggs on honeysuckle compared to spindle tree (C). Honeysuckle specialist mites did not show a preference for either host, neither did they lay more eggs on any particular host. Stars represent a significant difference from a 50:50 probability in (A,B), and a significant difference in the mean egg production between hosts in (C).
Inheritance of Adaptive Traits in F1 Hybrids Between Generalists and Specialists
The effect of mite genotype resulted in an overall significant difference in the number of droplets produced on honeysuckle leaves (F3,12.78 = 8.65, p = 0.002). F1 hybrids and specialist mites induced a similar number of droplets upon herbivory on honeysuckle leaves, which was significantly lower than the amount induced by generalist mite feeding (Figure 5A). As for host preference, F1 hybrids oviposited a significantly lower proportion of eggs on honeysuckle relative to spindle tree in a two-choice experiment, similar to the generalist parents (generalist: χ2 = 9.45, df = 1, p = 0.002; specialist × generalist: χ2 = 4.30, df = 1, p < 0.001; and generalist × specialist: χ2 = 17.25, df = 1, p < 0.001; Figure 5B).
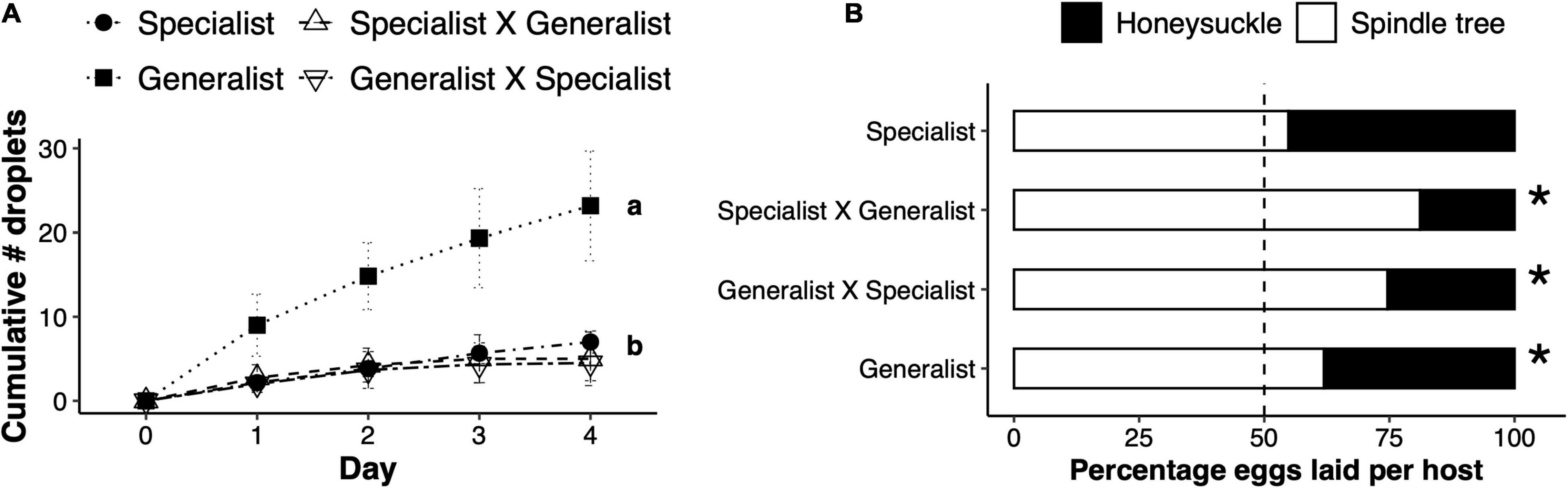
Figure 5. Inheritance of adaptive traits in F1 hybrid females. (A) Total number of sticky droplets (mean ± SEM) produced on honeysuckle leaves per day of herbivory by either honeysuckle specialist Tetranychus urticae females, generalist females, or their reciprocal F1 hybrids (female × male in the legend). Letters represent a significantly different number of droplets produced between treatments. (B) Percentage of the total number of eggs laid on honeysuckle or spindle tree (legend) by either specialist, generalist or F1 hybrid females. Stars next to a bar represent a significant difference from a 0.5:0.5 probability.
Discussion
We aimed to investigate the factors that allow genetically-distinct mite populations to co-exist at small spatial scales. We asked whether the production of sticky droplets on honeysuckle is a defence mechanism against spider mites, and whether spider mite genotypes that co-exist together in the Dutch dunes elicit different responses from honeysuckle.
Honeysuckle Produces Sticky Droplets as a Defence Mechanism Against Mite Herbivory
Spider mite herbivory induces the production of sticky droplets on the lamina of honeysuckle leaves (Figure 1 and Supplementary Videos 1, 2). We showed that sticky droplets are a direct defence mechanism of honeysuckle as it reduced the survival of generalist juveniles (Figure 2A). In addition, sticky droplets are probably an indirect defence mechanism, as they increased the arrestment time of a natural enemy of spider mites, A. andersoni (Figure 2B and Supplementary Video 3). Based on their sugar and amino acid content (Supplementary Tables 1, 2), sticky droplets could be a coveted food resource for omnivore predators (Krimmel and Pearse, 2013; Heil, 2015; Lopresti and Toll, 2017). Thus, sticky droplets are a defence mechanism of honeysuckle, reducing spider mite fitness directly and potentially also indirectly by arresting natural enemies.
Generalist and Specialist Mites Interact Differently With Honeysuckle
Since sticky droplets of honeysuckle reduce mite fitness directly and probably indirectly, mites feeding on this host would be under strong selection pressure to prevent droplet production. Indeed, we found that feeding by specialist mites resulted in much fewer droplets produced compared to generalist mites (Figure 1 and Supplementary Figure 1). We showed that the specialist has evolved the ability to suppress the production of sticky droplets (Figure 3). Suppression of droplet production is probably achieved via the manipulation of defence-associated pathways. Droplet production possibly involves regulating enzymes in charge of exuding sugary secretions, such as JA–induced cell wall invertases (Heil et al., 2001; Millán-Cañongo et al., 2014). We found that both JA and SA-treated leaves showed an enhanced droplet production after generalist feeding, while specialist feeding results in a low number of droplets produced for either phytohormone (Supplementary Figure 2). Suppression of droplet production is probably achieved via salivary effector molecules (Jonckheere et al., 2016; Villarroel et al., 2016; Blaazer et al., 2018). Alternatively, specialists could prevent droplet production, for example, by feeding stealthily from open stomata or by reducing the frequency of feeding events. Although we cannot rule out these possibilities entirely, they seem unlikely for at least two reasons. First, if specialist mites would feed stealthily from honeysuckle leaves, subsequent feeding by generalists on those leaves would have resulted in a similar number of droplets being produced compared to leaves in which only generalists fed, which was not the case (Figure 3). Second, not even the highest density of specialist individuals per leaf induced droplet production to the extent that a few generalists did (Supplementary Figure 3).
Herbivores that do not suppress honeysuckle defences might evolve an aversion to feed on honeysuckle and to prefer alternative plant species (Gripenberg et al., 2010). The generalist, whose fitness is negatively affected from inducing droplet production, avoids honeysuckle. The specialist, who suppresses droplet production, does not seem to have a preference for honeysuckle or for an alternative host (Figure 4). These results challenge the idea that specialists prefer to feed and oviposit on their native hosts (Gotoh et al., 1993; Gomi and Gotoh, 1996; Gripenberg et al., 2010). Instead, our data suggests that the honeysuckle race behaves as an “estranged generalist”: it does not have a preference for honeysuckle over other available host, and its fitness is similar across hosts (Villacis-Perez et al., 2021). Yet, it is associated strictly with honeysuckle in the field, partly because other genotypes avoid to feed on honeysuckle. It remains to be investigated if and which chemoreceptors, particularly gustatory receptors, underlie the differences in honeysuckle preference between generalists and specialists (Ngoc et al., 2016).
Co-existence of Specialist and Generalist Mite Populations in the Field
We found that intraspecific variation in host defence suppression and host plant preference are heritable traits. The defence suppression trait from the specialist parent and the host preference trait from the generalist parent are dominant in F1 hybrid females (Figure 5). This suggests that these are mendelian phenotypes, but further information about the genetic basis of these traits would require investigating the F2 generation, which is difficult to obtain due to strong genetic incompatibilities between mite genotypes (Villacis-Perez et al., 2021). Experimental evolution assays have revealed that the genomic structure of mite adaptation to tomato is likely polygenic and complex (Wybouw et al., 2019).
In nature, specialist and generalist co-occur at small physical distances, often on hosts species within metres or even touching each other (Villacis-Perez et al., 2021). Thus, there is ample opportunity for gene flow between these populations. However, because mites mate where they feed, traits related to host use that reduce the occurrence of mating encounters will have a large impact on their assortative mating (Helle and Sabelis, 1985). Given that specialists have no preference for honeysuckle, they could disperse to other plant species in the proximity, where the chances to meet with generalist individuals would be high. On hosts other than honeysuckle, the specialist is likely outcompeted by generalists, which have a higher reproductive performance across multiple hosts (Villacis-Perez et al., 2021). We show that when given the choice, generalists avoid honeysuckle, and that if they arrive on honeysuckle, selection by sticky droplet production would act against them. Thus, successful mating encounters are expected to be less frequent on honeysuckle relative to other hosts. If matings between generalist and specialist parents would occur on honeysuckle, most viable hybrids would avoid this host, despite their ability to suppress droplet production (Figure 5). Specialist traits would thus be diluted through introgression with the generalist population on other hosts or lost due to hybrid breakdown (Villacis-Perez et al., 2021). Therefore, the interplay between suppression of plant defences and host plant preferences potentially underlies the co-existence of distinct spider mite genotypes that colonise different hosts.
Data Availability Statement
The datasets used to produce the main figures, and the videos generated in this study are publicly available. These original contributions can be found here: https://doi.org/10.21942/uva.c.5617300.v1. Further queries should be directed to the corresponding author.
Author Contributions
EV-P, JB, and JA conceptualized the experimental design. JC and ZL collected data under the supervision of EV-P. EV-P conducted the experiments, data analyses, and drafted the article. JA, TV, and JB provided the critical comments and supervision. All authors contributed to the article and approved the submitted version.
Funding
This work was supported by the Institute for Biodiversity and Ecosystem Dynamics (IBED, TVL starters- grant), the European Research Council (ERC) under the European Union’s Horizon 2020 research and innovation program (ERC consolidator grant 772026- POLYADAPT to TV and 773902-SuperPests to TV).
Conflict of Interest
The authors declare that the research was conducted in the absence of any commercial or financial relationships that could be construed as a potential conflict of interest.
Publisher’s Note
All claims expressed in this article are solely those of the authors and do not necessarily represent those of their affiliated organizations, or those of the publisher, the editors and the reviewers. Any product that may be evaluated in this article, or claim that may be made by its manufacturer, is not guaranteed or endorsed by the publisher.
Acknowledgments
We thank Arne Janssen for revising earlier versions of the manuscript, and to the two reviewers for their constructive comments.
Supplementary Material
The Supplementary Material for this article can be found online at: https://www.frontiersin.org/articles/10.3389/fevo.2022.819894/full#supplementary-material
References
Blaazer, C. J. H., Villacis-Perez, E. A., Chafi, R., Van Leeuwen, T., Kant, M. R., and Schimmel, B. C. J. (2018). Why do herbivorous mites suppress plant defenses? Front. Plant Sci. 9:1057–1057. doi: 10.3389/fpls.2018.01057
Calis, I., Lahloub, M. F., and Sticher, O. (1984). Loganin, loganic acid and periclymenoside, a new biosidic ester iridoid glucoside from Lonicera periclymenum L.(Caprifoliaceae). Helvet. Chim. Acta 67, 160–165. doi: 10.1002/hlca.19840670119
Calis, I., and Sticher, O. (1984). Secoiridoid glucosides from Lonicera periclymenum. Phytochemistry 23, 2539–2540. doi: 10.1016/s0031-9422(00)84094-7
Carroll, S. P., Dingle, H., Famula, T. R., and Fox, C. W. (2001). Genetic architecture of adaptive differentiation in evolving host races of the soapberry bug, Jadera haematoloma. in Microevolution Rate, Pattern, Process. New York, NY: Springer, 257–272.
Carroll, S. P., Loye, J. E., Dingle, H., Mathieson, M., Famula, T. R., and Zalucki, M. P. (2005). And the beak shall inherit–evolution in response to invasion. Ecol. Lett. 8, 944–951. doi: 10.1111/j.1461-0248.2005.00800.x
Cipollini, D., Stevenson, R., Enright, S., Eyles, A., and Bonello, P. (2008). Phenolic metabolites in leaves of the invasive shrub, Lonicera maackii, and their potential phytotoxic and anti-herbivore effects. J. Chem. Ecol. 34, 144–152. doi: 10.1007/s10886-008-9426-2
de Boer, R. (1980). Genetic affinities between spider mite Tetranychus urticae (Acarina:Tetranychidae) populations in a non-agricultural area. Entomol. Exp. Appl. 28, 22–28. doi: 10.1111/j.1570-7458.1980.tb02983.x
Doellman, M. M., Egan, S. P., Ragland, G. J., Meyers, P. J., Hood, G. R., Powell, T. H. Q., et al. (2019). Standing geographic variation in eclosion time and the genomics of host race formation in Rhagoletis pomonella fruit flies. Ecol. Evol. 9, 393–409. doi: 10.1002/ece3.4758
Dres, M., and Mallet, J. (2002). Host races in plant-feeding insects and their importance in sympatric speciation. Philosoph. Trans. R. Soc. Lond. B 357, 471–492. doi: 10.1098/rstb.2002.1059
Egan, S. P., and Funk, D. J. (2009). Ecologically dependent postmating isolation between sympatric host forms of Neochlamisus bebbianae leaf beetles. Proc. Natl. Acad. Sci. 106, 19426–19431. doi: 10.1073/pnas.0909424106
Fahn, A. (1979). Ultrastructure of nectaries in relation to nectar secretion. Am. J. Bot. 66, 977–985. doi: 10.1002/j.1537-2197.1979.tb06309.x
Felsenstein, J. (1981). Skepticism towards Santa Rosalia, or why are there so few kinds of animals? Evolution 1981, 124–138. doi: 10.1111/j.1558-5646.1981.tb04864.x
Fox, B. W. (1996). Alternative foraging strategies of the White Admiral butterfly (Ladoga camilla L.) and the Broad Bordered Bee Hawk moth (Hemaris fuciformis L.) on Honeysuckle (Lonicera periclymenum L.). Univ. Southampt. Dep. Biol. 1996:235.
Funk, D. J. (2012). Of “host forms” and host races: terminological issues in ecological speciation. Internat. J. Ecol. 2012, 1–8. doi: 10.1155/2012/506957
Funk, D. J., Egan, S. P., and Nosil, P. (2011). Isolation by adaptation in Neochlamisus leaf beetles: host-related selection promotes neutral genomic divergence. Mole. Ecol. 20, 4671–4682. doi: 10.1111/j.1365-294X.2011.05311.x
Funk, D. J., Nosil, P., and Etges, W. J. (2006). Ecological divergence exhibits consistently positive associations with reproductive isolation across disparate taxa. Proc. Natl. Acad. Sci. 103, 3209–3213. doi: 10.1073/pnas.0508653103
Futuyma, D. J., and Peterson, S. C. (1985). Genetic variation in the use of resources by insects. Annu. Rev. Entomol. 30, 217–238. doi: 10.1146/annurev.en.30.010185.001245
Gardener, M. C., and Gillman, M. P. (2002). The taste of nectar–a neglected area of pollination ecology. Oikos 98, 552–557. doi: 10.1034/j.1600-0706.2002.980322.x
Gloss, A. D., Groen, S. C., and Whiteman, N. K. (2016). A genomic perspective on the generation and maintenance of genetic diversity in herbivorous insects. Annu. Rev. Ecol. Evol. Syst. 47, 165–187. doi: 10.1146/annurev-ecolsys-121415-032220
Gomi, K., and Gotoh, T. (1996). Host plant preference and genetic compatibility of the Kanzawa spider mite, Tetranychus kanzawai Kishida (Acari: Tetranychidae). Appl. Entomol. Zool. 31, 417–425. doi: 10.1303/aez.31.417
Gompert, Z., Comeault, A. A., Farkas, T. E., Feder, J. L., Parchman, T. L., Buerkle, C. A., et al. (2014). Experimental evidence for ecological selection on genome variation in the wild. Ecol. Lett. 17, 369–379. doi: 10.1111/ele.12238
Gotoh, T., Bruin, J., Sabelis, M., and Menken, S. B. J. (1993). Host race formation in Tetranychus urticae: genetic differentiation, host plant preference, and mate choice in a tomato and a cucumber strain. Entomol. Exp. Appl. 68, 171–178. doi: 10.1111/j.1570-7458.1993.tb01700.x
Gripenberg, S., Mayhew, P. J., Parnell, M., and Roslin, T. (2010). A meta-analysis of preference–performance relationships in phytophagous insects. Ecol. Lett. 13, 383–393. doi: 10.1111/j.1461-0248.2009.01433.x
Hawthorne, D. J., and Via, S. (2001). Genetic linkage of ecological specialization and reproductive isolation in pea aphids. Nature 412, 904–907. doi: 10.1038/35091062
Heil, M. (2015). Extrafloral nectar at the plant-insect interface: a spotlight on chemical ecology, phenotypic plasticity, and food webs. Annu. Rev. Entomol. 60, 213–232. doi: 10.1146/annurev-ento-010814-020753
Heil, M., Koch, T., Hilpert, A., Fiala, B., Boland, W., and Linsenmair, K. E. (2001). Extrafloral nectar production of the ant-associated plant, Macaranga tanarius, is an induced, indirect, defensive response elicited by jasmonic acid. Proc. Natl. Acad. Sci. 98, 1083–1088. doi: 10.1073/pnas.98.3.1083
Helle, W., and Sabelis, M. W. (1985). Spider mites: their biology, natural enemies and control. Amsterdam: Elsevier Science Publishers B.V.
Hurtado, M. A., Ansaloni, T., Cros-Arteil, S., Jacas, J. A., and Navajas, M. (2008). Sequence analysis of the ribosomal internal transcribed spacers region in spider mites (Prostigmata: Tetranychidae) occurring in citrus orchards in Eastern Spain: use for species discrimination. Ann. Appl. Biol. 153, 167–174.
Jonckheere, W., Dermauw, W., Zhurov, V., Wybouw, N., Van den Bulcke, J., Villarroel, C. A., et al. (2016). The salivary protein repertoire of the polyphagous spider mite Tetranychus urticae: a quest for effectors. Mol. Cell Prot. 15, 3594–3613. doi: 10.1074/mcp.M116.058081
Kessler, A., and Baldwin, I. T. (2002). Plant responses to insect herbivory: the emerging molecular analysis. Annu. Rev. Plant Biol. 53, 299–328. doi: 10.1146/annurev.arplant.53.100301.135207
Krimmel, B. A., and Pearse, I. S. (2013). Sticky plant traps insects to enhance indirect defence. Ecol. Lett. 16, 219–224. doi: 10.1111/ele.12032
Levene, H. (1953). Genetic equilibrium when more than one ecological niche is available. Am. Natural. 87, 331–333. doi: 10.1086/281792
Levin, D. A. (1995). Metapopulations: an arena for local speciation. J. Evol. Biol. 8, 635–644. doi: 10.1046/j.1420-9101.1995.8050635.x
Lieurance, D., Chakraborty, S., Whitehead, S. R., Powell, J. R., Bonello, P., Bowers, M. D., et al. (2015). Comparative herbivory rates and secondary metabolite profiles in the leaves of native and non-native Lonicera species. J. Chem. Ecol. 41, 1069–1079. doi: 10.1007/s10886-015-0648-9
Lieurance, D., and Cipollini, D. (2013). Exotic Lonicera species both escape and resist specialist and generalist herbivores in the introduced range in North America. Biolog. Invas. 15, 1713–1724. doi: 10.1007/s10530-012-0402-y
Lopresti, E. F., and Toll, K. (2017). The three criteria for resistance by plant carrion-provisioning: insect entrapment and predator enrichment on Mimulus bolanderi. Ecol. Entomol. 42, 230–234. doi: 10.1111/een.12377
Magalhães, S., Forbes, M. R., Skoracka, A., Osakabe, M., Chevillon, C., and McCoy, K. D. (2007). Host race formation in the Acari. Exp. Appl. Acarol. 42, 225–238. doi: 10.1007/s10493-007-9091-0
Migeon, A., Nouguier, E., and Dorkeld, F. (2010). Spider Mites Web: a comprehensive database for the Tetranychidae in: Trends in Acarology. New York, NY: Springer, 557–560.
Millán-Cañongo, C., Orona-Tamayo, D., and Heil, M. (2014). Phloem sugar flux and jasmonic acid-responsive cell wall invertase control extrafloral nectar secretion in Ricinus communis. J. Chem. Ecol. 40, 760–769. doi: 10.1007/s10886-014-0476-3
Ngoc, P. C. T., Greenhalgh, R., Dermauw, W., Rombauts, S., Bajda, S., Zhurov, V., et al. (2016). Complex evolutionary dynamics of massively expanded chemosensory receptor families in an extreme generalist chelicerate herbivore. Gen. Biol. Evol. 8, 3323–3339. doi: 10.1093/gbe/evw249
Nosil, P., Parchman, T. L., Feder, J. L., and Gompert, Z. (2012). Do highly divergent loci reside in genomic regions affecting reproductive isolation? A test using next-generation sequence data in Timema stick insects. BMC Evol. Biol. 12:164. doi: 10.1186/1471-2148-12-164
Nyman, T., Vikberg, V., Smith, D. R., and Boevé, J.-L. J. B. E. B. (2010). How common is ecological speciation in plant-feeding insects? A ‘Higher’. Nemat. Perspect. 10:266. doi: 10.1186/1471-2148-10-266
Pieterse, C. M., Van der Does, D., Zamioudis, C., Leon-Reyes, A., and Van Wees, S. C. (2012). Hormonal modulation of plant immunity. Annu. Rev. Cell Dev. Biol. 28, 489–521. doi: 10.1146/annurev-cellbio-092910-154055
Richardson, J. L., Urban, M. C., Bolnick, D. I., and Skelly, D. K. (2014). Microgeographic adaptation and the spatial scale of evolution. Trends Ecol. Evol. 29, 165–176. doi: 10.1016/j.tree.2014.01.002
Riesch, R., Muschick, M., Lindtke, D., Villoutreix, R., Comeault, A. A., Farkas, T. E., et al. (2017). Transitions between phases of genomic differentiation during stick-insect speciation. Nat. Ecol. Evol. 1:0082. doi: 10.1038/s41559-017-0082
Roesti, M., Kueng, B., Moser, D., and Berner, D. (2015). The genomics of ecological vicariance in threespine stickleback fish. Nat. Comm. 6:8767.
Sabelis, M. W., Janssen, A., Bruin, J., Bakker, F. M., Drukker, B., Scutareanu, P., et al. (1999). Interactions between arthropod predators and plants: a conspiracy against herbivorous arthropods? in: Ecology and Evolution of the Acari. New York, NY: Springer, 207–229.
Sabelis, M. W., Janssen, A., and Kant, M. R. (2001). The enemy of my enemy is my ally. Science 291, 2104–2105. doi: 10.1126/science.1059939
Shafer, A. B. A., and Wolf, J. B. W. (2013). Widespread evidence for incipient ecological speciation: a meta-analysis of isolation-by-ecology. Ecol. Lett. 16, 940–950. doi: 10.1111/ele.12120
van Rijn, P. C., and Tanigoshi, L. K. (1999). The contribution of extrafloral nectar to survival and reproduction of the predatory mite Iphiseius degenerans on Ricinus communis. Exp. Appl. Acarol. 23, 281–296.
van Rijn, P. C. J., and Sabelis, M. W. (1990). Pollen availability and its effect on the maintenance of populations of Amblyseius cucumeris, a predator of thrips. Mededelingen van de Faculteit Landbouwwetenschappen, Rijksuniversiteit Gent, Vol. 55. Noida: OILB, 335–341.
Via, S., and West, J. (2008). The genetic mosaic suggests a new role for hitchhiking in ecological speciation. Mole. Ecol. 17, 4334–4345. doi: 10.1111/j.1365-294X.2008.03921.x
Villa, S. M., Altuna, J. C., Ruff, J. S., Beach, A. B., Mulvey, L. I., Poole, E. J., et al. (2019). Rapid experimental evolution of reproductive isolation from a single natural population. Proc. Natl. Acad. Sci. 116, 13440–13445. doi: 10.1073/pnas.1901247116
Villacis-Perez, E., Snoeck, S., Kurlovs, A. H., Clark, R. M., Breeuwer, J. A. J., and Van Leeuwen, T. (2021). Adaptive divergence and post-zygotic barriers to gene flow between sympatric populations of a herbivorous mite. Comm. Biol. 4:853. doi: 10.1038/s42003-021-02380-y
Villarroel, C. A., Jonckheere, W., Alba, J. M., Glas, J. J., Dermauw, W., Haring, M. A., et al. (2016). Salivary proteins of spider mites suppress defenses in Nicotiana benthamiana and promote mite reproduction. Plant J. 86, 119–131. doi: 10.1111/tpj.13152
Wäckers, F. L., Romeis, J., and Rijn, P. V. (2007). Nectar and pollen feeding by insect herbivores and implications for multitrophic interactions. Annu. Rev. Ent. 52, 301–323. doi: 10.1146/annurev.ento.52.110405.091352
Weryszko-Chmielewska, E., and Bozek, M. (2008). Structure of trichomatous nectaries in flowers of Lonicera kamtschatica [Sevast.] Pojark. Acta Agrobot. 2008:61.
Wright, S. (1946). Isolation by distance under diverse systems of mating. Genetics 31, 39–59. doi: 10.1093/genetics/31.1.39
Wybouw, N., Kosterlitz, O., Kurlovs, A. H., Bajda, S., Greenhalgh, R., Snoeck, S., et al. (2019). Long-term population studies uncover the genome structure and genetic basis of xenobiotic and host plant adaptation in the herbivore Tetranychus urticae. Genetics 211, 1409–1427. doi: 10.1534/genetics.118.301803
Keywords: intraspecific genetic variation, sympatry, isolation by ecology, plant-herbivore interactions, host specialisation, plant defence suppression
Citation: Villacis-Perez E, Alba JM, Cotte J, van Loon Z, Breeuwer JAJ and Van Leeuwen T (2022) Interactions With Plant Defences Isolate Sympatric Populations of an Herbivorous Mite. Front. Ecol. Evol. 10:819894. doi: 10.3389/fevo.2022.819894
Received: 22 November 2021; Accepted: 25 March 2022;
Published: 29 April 2022.
Edited by:
Dennis Murray, Trent University, CanadaReviewed by:
George Broufas, Democritus University of Thrace, GreeceFreerk Molleman, Adam Mickiewicz University, Poland
Copyright © 2022 Villacis-Perez, Alba, Cotte, van Loon, Breeuwer and Van Leeuwen. This is an open-access article distributed under the terms of the Creative Commons Attribution License (CC BY). The use, distribution or reproduction in other forums is permitted, provided the original author(s) and the copyright owner(s) are credited and that the original publication in this journal is cited, in accordance with accepted academic practice. No use, distribution or reproduction is permitted which does not comply with these terms.
*Correspondence: Ernesto Villacis-Perez, RXJuZXN0by5WaWxsYWNpc1BlcmV6QFVHZW50LmJl; Juan Manuel Alba, Si5NLkFsYmFDYW5vQHV2YS5ubA==