- College of Forest Resources and Environmental Science, Michigan Technological University, Houghton, MI, United States
It is widely accepted that predators disproportionately prey on individuals that are old, weak, diseased or injured. By selectively removing individuals with diseases, predators may play an important role in regulating the overall health of prey populations. However, that idea is seldom tested empirically. Here we assess the extent that wolves (Canis lupus) select adult moose (Alces alces) in Isle Royale National Park on the basis of age-class and osteoarthritis, a chronic, non-communicable disease. We also assess how temporal variation in kill rates (on moose by wolves) were associated with the subsequent incidence of osteoarthritis in the moose population over a 33-year period (1975–2007). Wolves showed strong selection for senescent moose and tended to avoid prime-aged adults. However, the presence of severe osteoarthritis, but not mild or moderate osteoarthritis, appeared to increase the vulnerability of prime-aged moose to predation. There was weak evidence to suggest that senescent moose with osteoarthritis maybe more vulnerable to wolves, compared to senescent moose without the disease. The incidence of osteoarthritis declined following years with higher kill rates–which is plausibly due to the selective removal of individuals with osteoarthritis. Together those results suggest that selective predation plays an important role in regulating the health of prey populations. Additionally, because osteoarthritis is influenced by genetic factors, these results highlight how wolf predation may act as a selective force against genes associated with developing severe osteoarthritis as a prime-aged adult. Our findings highlight one benefits of allowing predators to naturally regulate prey populations. The evidence we present for predation’s influence on the health of prey populations is also relevant for policy-related arguments about refraining from intensively hunting wolf populations.
Introduction
Selective predation occurs when a particular type of prey occurs more frequently in the predator’s diet than is expected based on the prey types frequency in the environment. Selective predation is believed to be common for coursing predators, such as wolves (Peterson, 1977; Wright et al., 2006; Hoy et al., 2021), and may also be common among stalking predators (Krumm et al., 2010; Heurich et al., 2016). Selection tends to be for individuals that are in some way easier or less risky for predators to capture because of differences in age, conspicuousness, behaviors or body size and condition (Temple, 1987; Magnhagen, 1991; Pierce et al., 2000; Berger-Tal et al., 2009). For example, senescent prey, and prey with diseases or parasites are thought to be easier for predators to catch because they are in substandard condition (Hudson et al., 1992; Krumm et al., 2010; Hoy et al., 2015, 2021).
Age-based selection can have important, if not readily anticipated, impacts on prey population dynamics. For example, prey population growth rates tend to be less impacted by predation when predators exhibit selection for juveniles and senescent adults because those age-classes have lower reproductive values than prime-aged adults (Wright et al., 2006; Gervasi et al., 2012; Hoy et al., 2015). Other effects of age-based selection are not so easily anticipated, such as sometimes making prey populations less resilient, reducing prey equilibrium values or having a destabilizing effect on predator-prey dynamics (Hoy et al., 2021).
Less well understood – but commonly speculated – is the notion that selection for prey with infectious or communicable diseases and parasites can result in healthier prey populations. For example, mathematical models predict that selection for infected individuals may reduce the prevalence and transmission rates of diseases or parasites under certain circumstances (Packer et al., 2003; Wild et al., 2011), but empirical assessments have been less forthcoming (Tanner et al., 2019). Even less well understood is whether the health of prey populations is affected by selective predation for non-communicable diseases with a genetic basis.
Here we assess the extent that wolves (Canis lupus) select adult moose (Alces alces) in Isle Royale National Park (IRNP) on the basis of both age-class and osteoarthritis, which is a chronic, non-communicable disease that is strongly influenced by genetic factors (Fernández-Moreno et al., 2008; Valdes and Spector, 2008). Osteoarthritis is a progressively crippling disease caused by degeneration of cartilage in the articulating surfaces of moveable joints. Osteoarthritis often becomes painful and limits mobility, which could increase vulnerability to wolf predation.
This analysis uses a database of necropsies, which includes information about the year-of-death, age-at-death, cause-of-death, and incidence of osteoarthritis for 1,571 moose dying over a 47-year period (1959–2007). We also assessed the extent that temporal variation in per capita kill rates (prey killed, per predator, per unit of time) was associated with the subsequent incidence of osteoarthritis in the moose population over a 33-year period (1975–2007).
Assessments of the extent that wolves selectively prey on individuals with osteoarthritis and the impact of selective predation on the incidence of osteoarthritis would contribute to better understanding of the ecological importance of wolves for maintaining healthy prey populations and the breath of ecosystem services that predators provide. Additionally, because osteoarthritis is a senescent-related disease, these assessments allow for disentangling the extent to which the basis for selective predation in this population is some generic consequences of senescence or more specifically osteoarthritis.
Materials and Methods
Study System
Isle Royale National Park is an archipelago in Lake Superior, North America (47°50′N, 89°00′W), comprised of a large island (544 km2) and dozens of smaller islets (most of which are< 2 km2). Isle Royale is also known as Minong by local indigenous communities and is under the stewardship of the Grand Portage Anishinaabe and U.S. National Park Service. Populations of wolves and moose have been continuously studied in IRNP since 1959 (Peterson et al., 2014). Moose are the primary prey for wolves, comprising∼90% of their kills, and wolves are the only predator of moose (Peterson et al., 1998). Neither the forest nor moose have been harvested for over a century, and wolves have been unaffected by human-caused mortality since their arrival in the mid-20th century.
Osteoarthritis is a senescent-related disease and its incidence increases with age (Peterson et al., 2010; Figure 1). Older individuals are also more likely to have severe forms of the disease (Figure 1). However, osteoarthritis can sometimes result from trauma or injury to joints (Lacourt et al., 2012; Rickey et al., 2012; Boyce et al., 2013; Jiménez et al., 2018). In humans and horses, the rate at which osteoarthritis progresses can vary greatly among individuals: in some cases, it may take several years before individuals develop severe forms of the disease; in other cases, rapid deterioration may occur in less than a year (Pivec et al., 2013; Driban et al., 2014; McCoy et al., 2020). The rate at which osteoarthritis progresses in moose may be similarly variable.
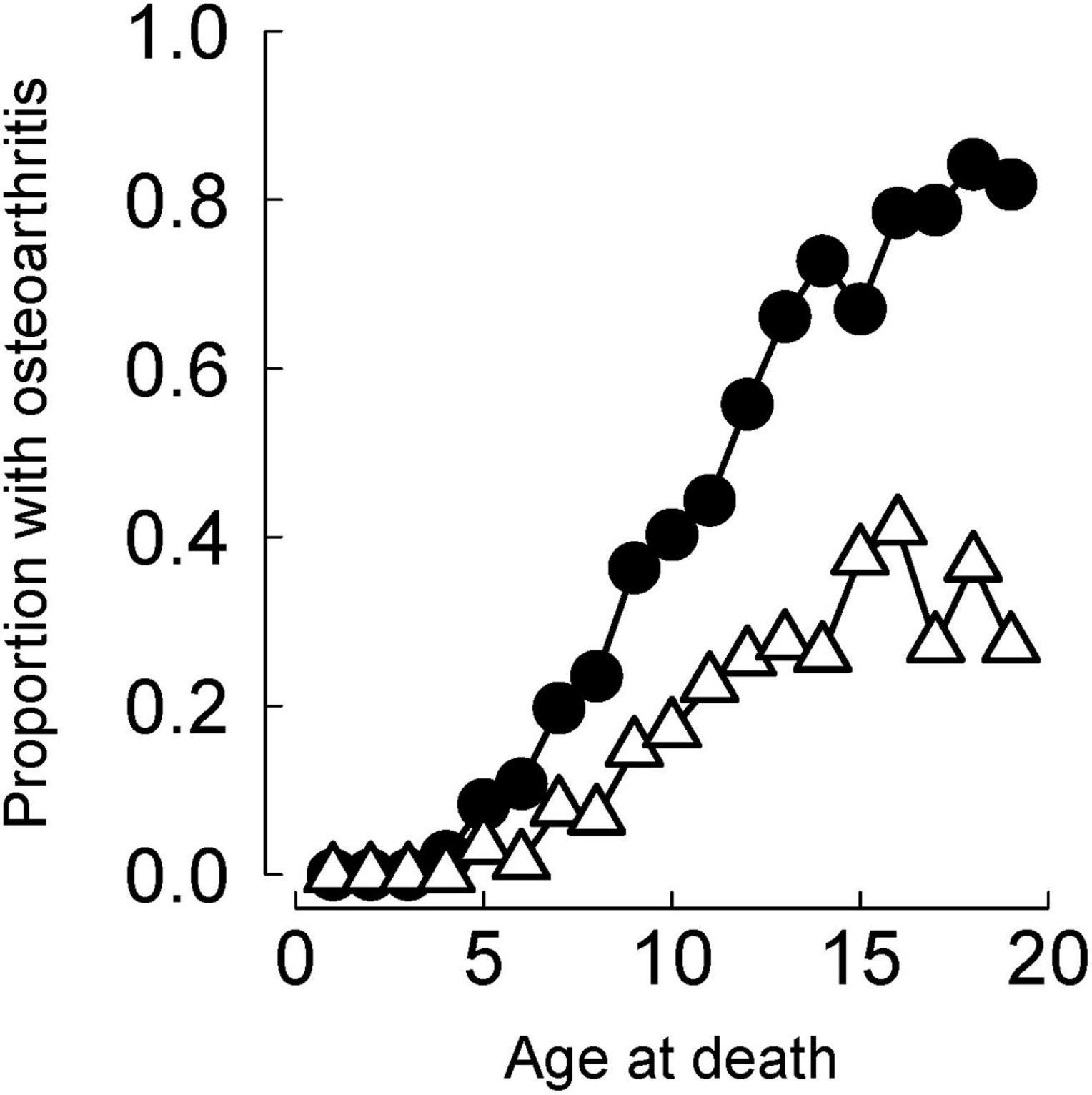
Figure 1. The proportion of moose exhibiting any sign of osteoarthritis (black circles) and severe osteoarthritis (white triangles) at the time of death increases with age in a population of moose in Isle Royale National Park. Dataset is based on necropsies of 1,571 moose that died between 1959–2007.
Data Collection
Each year between 1959–2007, we conducted intensive aerial surveys in winter (January–February) and ground surveys in summer (May–September) to locate the carcasses of moose that died during the previous year (Montgomery et al., 2014; Hoy et al., 2021). We necropsied carcasses to determine the individual’s cause-of-death, age-at-death and whether that individual had osteoarthritis. Moose died from various causes, primarily predation, starvation and accidents. We observed various types of accidental deaths including: moose falling on or through ice, falling down cliffs or abandoned mine-shafts, injuries sustained during the rut. We used field sign, such as blood on trees, signs of a chase as indicated by tracks, hair and blood in the snow and signs of struggle including broken branches to infer the cause-of-death as predation (Metz et al., 2012; Montgomery et al., 2014). If predation was not determined to be the cause of death, we used the condition of bone marrow in the femur to assess whether starvation/malnutrition was a likely cause of death (Peterson, 1977; Mech and Delgiudices, 1985). We estimated the age-at-death for yearlings through tooth eruption patterns and for adults by counting cementum lines of teeth (Peterson, 1977; Haagenrud, 1978; Rolandsen et al., 2008). We excluded calves from this analysis as previous studies have assessed wolf selection for calves (Wright et al., 2006; Hoy et al., 2021). Moreover, in most cases the carcasses of calves are too badly damaged to assess whether individuals had skeletal abnormalities or defects.
We searched for the presence of osteoarthritis throughout each skeleton. Osteoarthritis was most commonly observed in the lowest vertebrae (fifth lumbar and first sacral) and pelvic (coxofemoral) joint (Peterson et al., 2010). We classified instances of osteoarthritis as being slight, moderate, or severe. Specimens were classed as slight if we observed small osteophytes on vertebral edges or if the acetabular fossa of the pelvis was largely open but exhibited bone ingrowths. Specimens were classed as moderate if osteophytes had started to bridge gaps between vertebra or if bone growth had entirely closed the acetabular fossa and small areas of cartilage loss (sclerosis) were observed, but no other modifications of the acetabular joints were observed. Lastly, specimens were classed as severe if we observed any of these conditions: osteophytes extending over vertebral joints, vertebrae starting to fuse together, significant remodeling of joints in the pelvis, such as sclerosis, subchondral lesions (cavities) and osteophytes growing around the entire coxofemoral joint or dorsal migration of the joint. Figure 1 in Peterson et al. (2010) provides images which show the progressive deterioration of the coxofemoral joint associated with osteoarthritis. To ensure that individuals were assessed for osteoarthritis in a consistent manner, the severity of osteoarthritis was determined by the same observer (ROP) throughout the entire study period. It was not possible to determine how long individuals might have had osteoarthritis prior to death because the rate at which osteoarthritis progresses is known to be highly variable (Pivec et al., 2013; Driban et al., 2014; McCoy et al., 2020).
Statistical Analyses
All analyses were performed in Program-R version 4.0.5 (R Core Team, 2021). First, we assessed whether wolves selectively preyed on moose with osteoarthritis whilst also taking into consideration whether prey were prime-aged (aged 1–9 years old) or senescent (>10 years old). It is plausible that an individual’s vulnerability to predators may vary with the severity of the osteoarthritis, e.g., moose with more severe osteoarthritis may have more limited mobility than individuals with slight osteoarthritis. Therefore, we considered eight types of moose: prime-absent (n = 639), prime-slight (n = 33), prime-moderate (n = 30), prime-severe (n = 39), senescent-absent (n = 374), senescent-slight (n = 102), senescent-moderate (n = 194), and senescent-severe (n = 264).
Following Hoy et al. (2021), we estimated the strength of wolves’ selection for each of the eight moosetypes using the Manly-Chesson selection index, denoted α (Manly, 1974; Chesson, 1978, 1983). The Manly-Chesson index is a relative measure of selection and commonly used to assess wolf predation (Ståhlberg et al., 2017; Torretta et al., 2017; Hoy et al., 2021). It is calculated as:
where r_i is the proportion of prey item i in the diet (i.e., dietary frequency), e_i is the proportion of prey item i in the environment (environmental frequency), and m represents the number of prey types in the environment, where m = 8 in our case. Values of αi range from 0 (complete avoidance) to 1 (strongest possible selection). If predators exhibit no selection, then frequency in the diet matches the frequency in the environment and αi=1/m. In a formal sense, α is proportional to the probability that a predator attacks a prey type given an encounter. Additionally, α is also related to the attack rate in the functional response of a consumer-resource model (Chesson, 1978).
We estimated the environmental frequency of osteoarthritis by multiplying estimates of the age-specific incidence of moderate to severe osteoarthritis (Figure 1) by estimates of the average age-structure of the moose population between 1959–2007. The average age structure of the moose population was estimated from annual estimates of age-structure between 1959–2007, which were produced as part of an earlier study (Hoy et al., 2020, 2021). We do not assess whether selection for moose with osteoarthritis differed for bulls and cows because sex-specific estimates of age-structure are not available for this population. To estimate dietary frequency, we filtered our necropsy database to include only moose killed by wolves between 1959–2007 (same period as age-structure estimates) and then estimated the proportion of all wolf-killed moose belonging to each of the eight prey types.
Second, we assessed whether cause-specific mortality differed with the severity of osteoarthritis. To do so, we used generalized linear models (GLMs), with a binomial error structure, where the response variable was 1 or 0 depending on whether moose died from wolf predation or from other causes (i.e., starvation, accidents) respectively. If an individual’s cause of death could not be determined (due to inconclusive field evidence) we excluded it from this analysis. The predictor variable indicated whether osteoarthritis was absent, slight, moderate or severe. We assessed cause-specific mortality separately for prime-aged and senescent moose because wolves are known to show strong selection for senescent moose(Hoy et al., 2021).
Lastly, we used GLMs with a binomial error structure to assess the extent that interannual variation in per capita kill rate subsequently influenced the proportion of moose dying with osteoarthritis. To account for interannual variability in the number of individuals dying we carried out a weighted regression (annual sample sizes used as weights) where the response variable was the proportion of moose dying with osteoarthritis. For this analysis, we considered only two types of moose (those with and without osteoarthritis) because annual sample size was not large enough to support an analysis that takes account of the severity of osteoarthritis. We built models allowing for the possibility that kill rate’s effect on the incidence of osteoarthritis occurs after some time lag (up to 3 years). Time lags are a common feature of ecological interactions, including predation and disease (MacDonald, 1978). We estimated per capita kill rate from aerial surveys each surveys in Jan-Feb each year (Gasaway et al., 1986; Peterson and Page, 1988). We excluded data prior to 1975 because estimates of per capita kill rate (with 3-year lag) are not available for earlier years. Because the incidence of osteoarthritis is also likely influenced by fluctuations in the age structure of the moose population, we included an index of age structure (proportion of adults that were senescent, > 9 years old) as a predictor variable (Hoy et al., 2020).
To check assumptions of heteroskedasticity and normally distributed errors we visually inspected plots of model residuals and we formally tested for heteroskedasticity using Breusch-Pagan tests. We also checked for overdispersion and refitted models with a quasibinomial error structure if the dispersion factor was greater than 1.3. We estimated Cook’s distance to check whether any observations had unduly large leverage. Lastly, we estimated variance inflation factors (VIF) to check whether multicollinearity was a concern for each model.
Results
Osteoarthritis was detected in 38.3% of the 1,571 skeletons of moose that we examined whose age-at-death could be determined. Senescent moose accounted for 35.4% of individuals dying with no signs of osteoarthritis (n = 970), 74.0% of individuals dying with slight osteoarthritis (n = 127), 86.4% of individuals with moderate osteoarthritis (n = 214), and 86.9% of individuals with severe osteoarthritis (n = 260). Wolf predation accounted for 58.0% of the 1,416 dead moose whose cause-of-death could be determined, with prime-aged individuals accounting for 42.9% of moose killed by wolves.
Wolves showed strong selection for senescent moose, and avoidance of prime-aged adults. However, wolves showed weaker avoidance of prime-age moose with severe osteoarthritis (α = 0.08) compared to prime-aged moose without osteoarthritis or with only slight or moderate osteoarthritis (α = 0.04, Figure 2). Moreover, wolves exhibited weaker selection for senescent moose without osteoarthritis (α = 0.16) compared to senescent moose with slight, moderate or severe osteoarthritis (α = 0.21, α = 0.22, α = 0.20, respectively, Figure 2). For additional context, we would expect α = 0.13 if wolves exhibited no selection or no avoidance for a prey class (i.e., they killed prey types in proportion to their relative abundance).
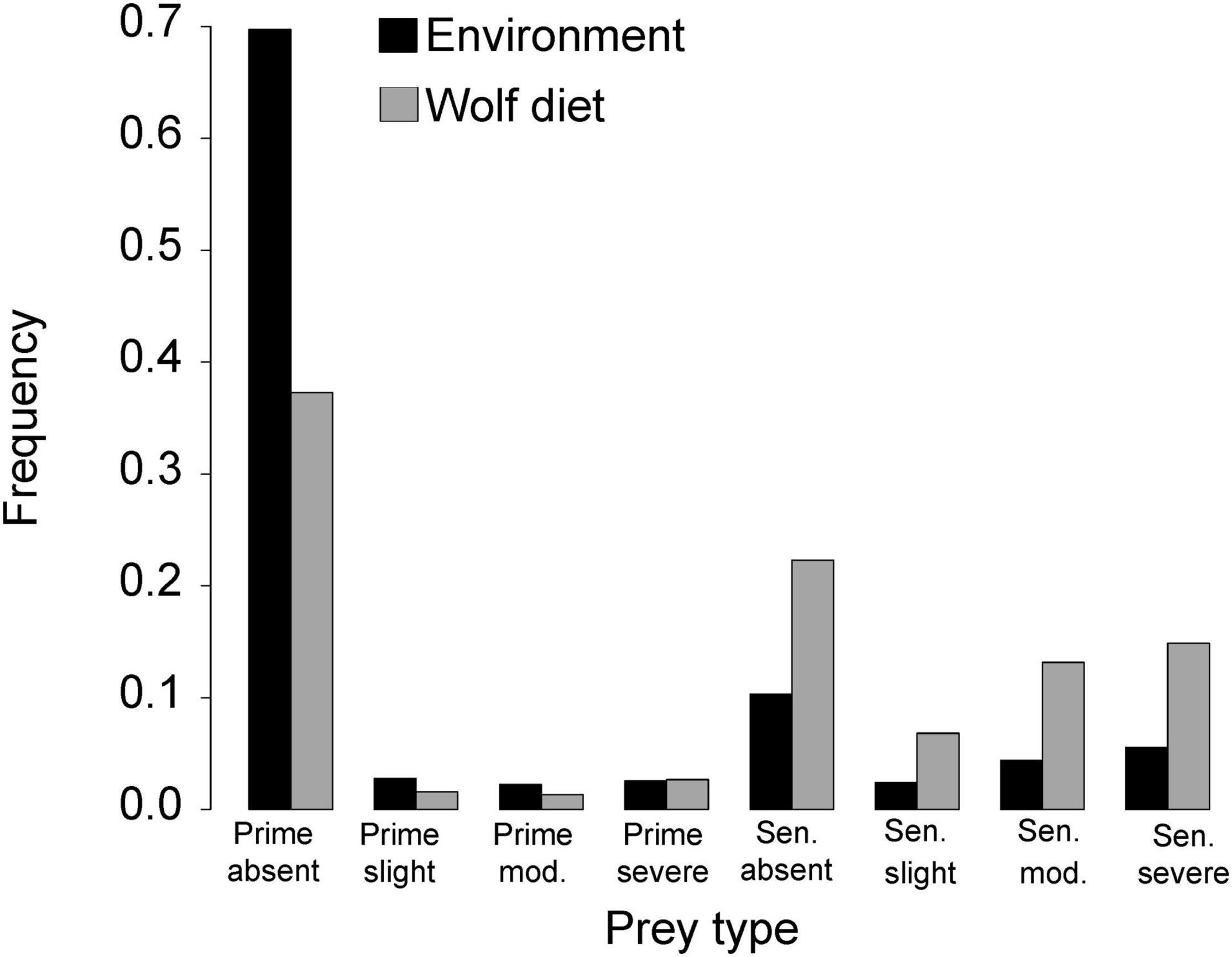
Figure 2. Frequency of different types of moose in the environment and in wolf diets in Isle Royale National Park, 1959–2007. Moose were grouped according to age [prime-aged (1–9 years old) or senescent (>9 years old, abbreviated “Sen”)] and severity of OA [absent, mild, moderate (abbreviated to mod.), severe]. Black bars indicate environmental frequency, averaged across years. Gray bars indicate dietary frequency, averaged across years.
Cause-specific mortality differed with the severity of osteoarthritis for prime-aged moose (see Figure 3A). More precisely, the probability of being killed by wolves was significantly higher for prime-aged moose with severe osteoarthritis compared to prime-aged moose with slight, moderate or no sign of osteoarthritis (p = 0.02). There was weak evidence to suggest that senescent moose without osteoarthritis were less likely to be killed by wolves than moose with slight, moderate or severe osteoarthritis; but the difference was not statistically significant (Figure 3B, p = 0.08).
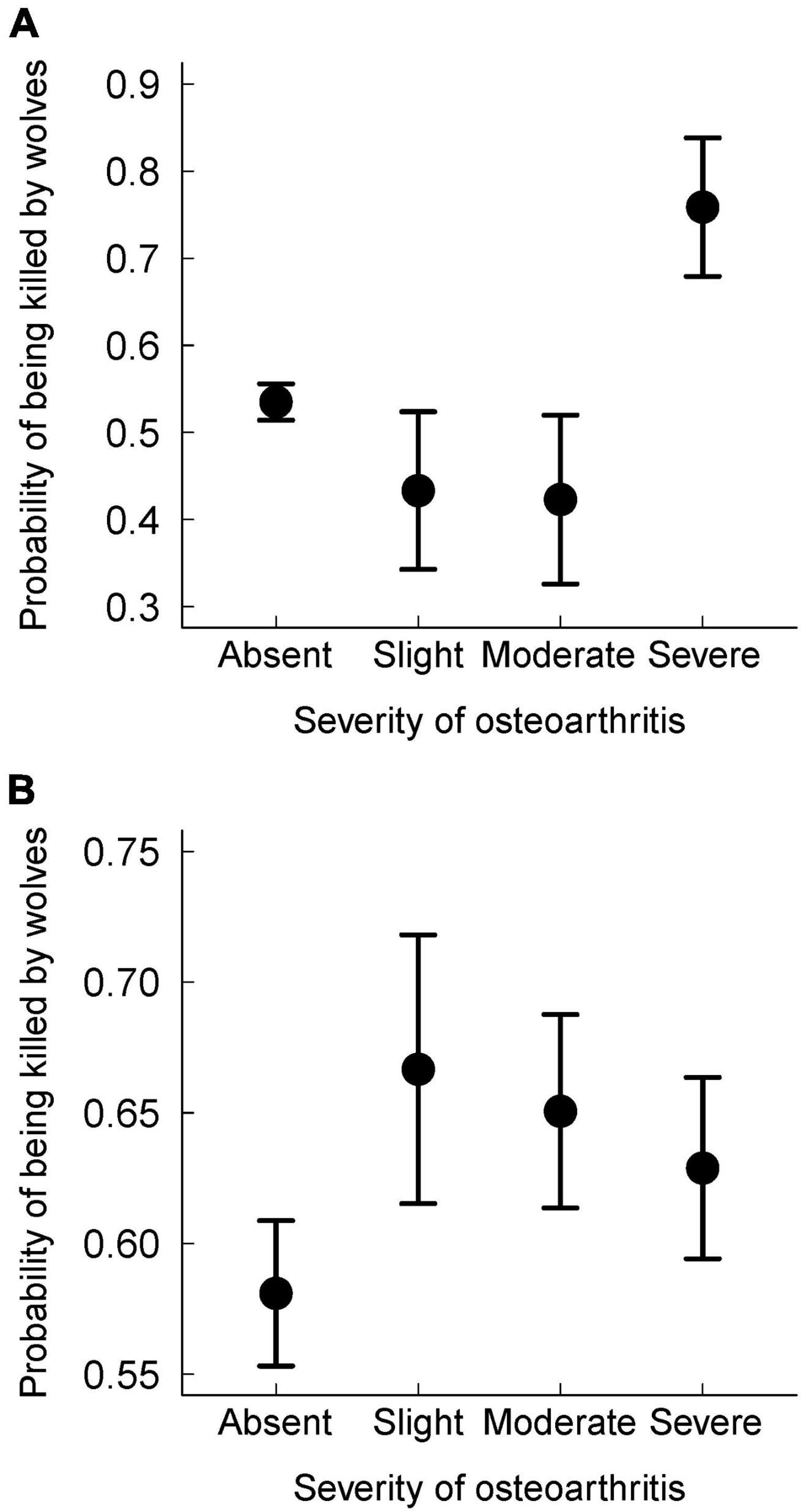
Figure 3. Proportion of moose killed by wolves (as opposed to dying from other causes, i.e., starvation or accidents) varies with the severity of osteoarthritis for prime-aged moose (A) but not for senescent moose (B). Moose were considered to be in their “prime” if they died between 1–9 years old or senescent if they died> 9 years old. Bars represent standard errors. Analysis is based on necropsies of 1,416 moose in Isle Royale National Park between 1959–2007 and for whom age-at-death, cause-of-death, and osteoarthritis status could be determined.
The incidence of osteoarthritis among dead moose was negatively correlated with kill rates following a 2–3-year lag (Table 1 and Figure 4) with the correlation being strongest for a 3-year lag. By contrast, temporal variation in the incidence of osteoarthritis among dead moose was not strongly correlated with the indicator of population age-structure (proportion of adults in the population that were senescent, Table 1).
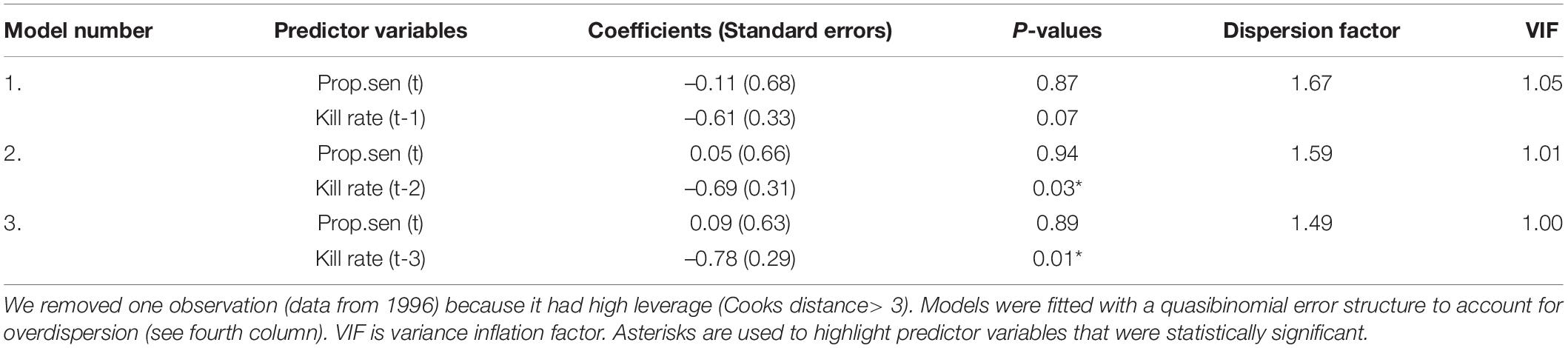
Table 1. Coefficients from bivariate models predicting temporal variation in the incidence of OA among dead moose over a 33-year period (1975–2007) from the proportion of adults in the populations that were senescent (Prop.sen, an indicator of population age structure) and kill rate by wolves (the number of moose killed, per wolf, each year) lagged up to 3 years.
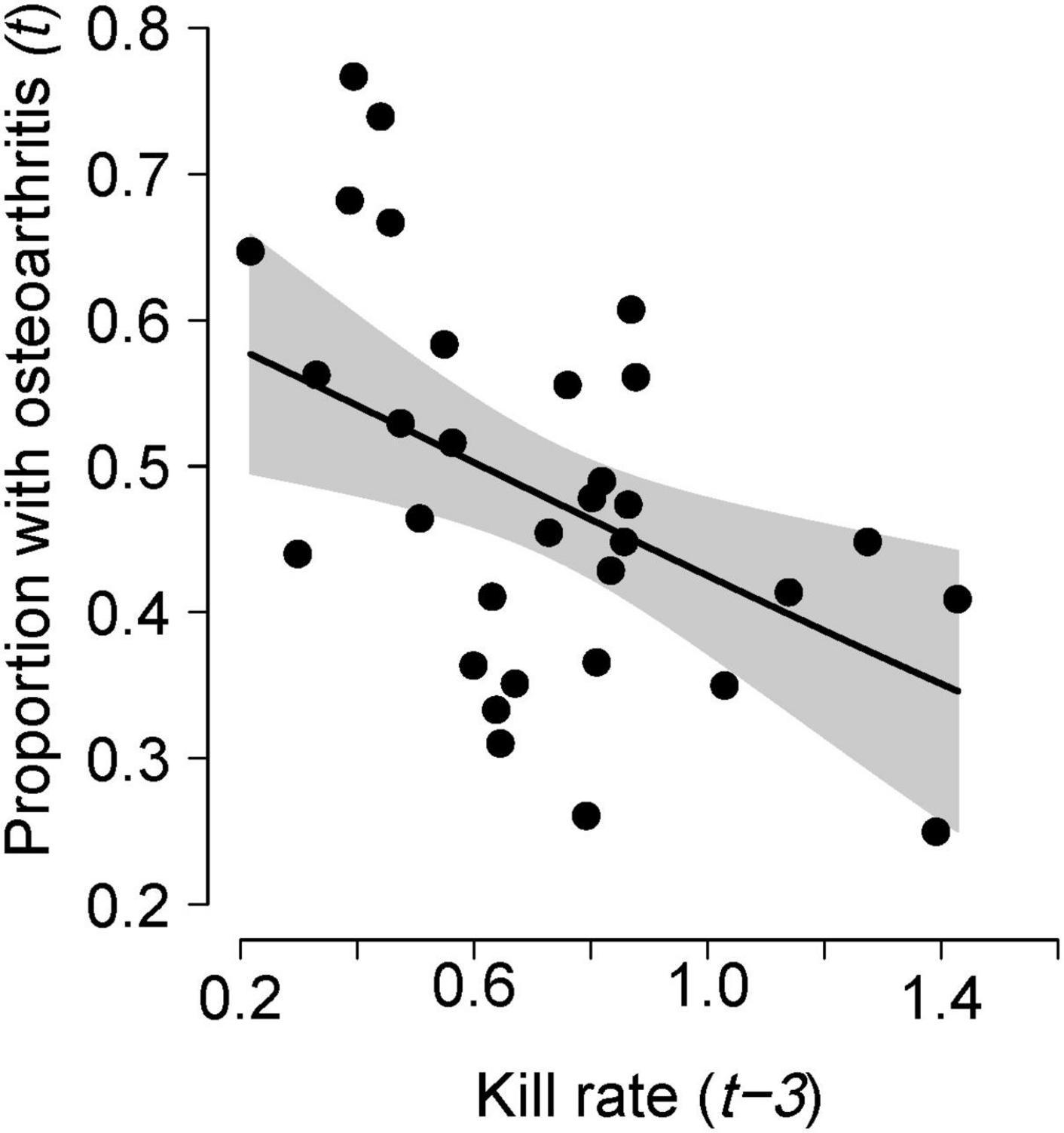
Figure 4. Proportion of moose exhibiting osteoarthritis that died (from all causes) in year t in relationship to the per capita kill rate by wolves in year t–3. Each point represents a year (t) between 1979–2010. The line indicates a predicted value from a generalized linear model with two predictor variables, kill rate (t-3) and proportion of senescent moose in the population (t), where the proportion of senescent moose was fixed at the median value observed during the 33-year study period. Gray areas indicate 95% confidence intervals. The relationship with the proportion of moose exhibiting osteoarthritis and kill rates (t-2) is similar to the one shown above.
Discussion
Wolves showed strong selection for senescent moose and tended to avoid prime-aged adults, which is consistent with previous research (Hoy et al., 2021). However, this research goes further by showing that selection for age-classes of moose may also be influenced by a chronic disease, osteoarthritis. More precisely, our results suggest that the presence of severe, but not mild or moderate osteoarthritis, increases the vulnerability of prime-aged moose to predation by wolves. Two results point to this conclusion. First, wolf predation was more likely the cause of death for prime-aged moose with severe osteoarthritis than for prime-aged moose without osteoarthritis or with mild or moderate osteoarthritis (Figure 3A). Second, wolves avoided prime-aged moose with severe osteoarthritis less intensely (α = 0.08) than prime-aged moose without the disease or with mild and moderate cases of osteoarthritis (α = 0.04, Figure 2).
There was weak evidence to suggest that senescent moose without osteoarthritis were less vulnerable to wolves, compared to senescent moose with the disease. Specifically, the estimated value of α for senescentmoose without osteoarthritis (α = 0.16) was close to the value that corresponds to no selection for a given prey type (α = 0.13). There was also some evidence to suggest that the probability of being killed by wolves is lower for senescent moose with no signs of osteoarthritis (Figure 3B), but that result was not statistically significant. Therefore, this study offers limited support for the idea that osteoarthritis is a more important basis for selection, rather than some other aspect(s) of being senescent.
Senescent adults may be more vulnerable to coursing predators, irrespective of whether they have osteoarthritis, due to some combination of the following factors. First, senescent mammals may be less able to detect nearby predators because of age-related declines in hearing, visual acuity, and cognition (Spear et al., 1994; Chapagain et al., 2018; Jayakody et al., 2018). Second, older adults tend to become more sedentary (Ingram, 2000; Froy et al., 2018) because of declines in muscle mass and strength, aerobic capacity or spatial memory (Barnes, 1988; Doherty, 2003; Short et al., 2005; Tanaka and Seals, 2008). Third, sedentariness may be accompanied by a tendency to spend more time in habitats where forage availability is high (Froy et al., 2018), even if doing so increases the likelihood of encountering predators. This pattern has been observed on Isle Royale, where senescent moose were more likely than prime-aged moose to be killed closer to the shorelines where forage availability is thought to be higher and where wolves tend to be more active (Montgomery et al., 2013). Thus, age-related changes in behaviors, such as habitat selection, in addition to physiological declines, may contribute to senescent moose being more vulnerable to predation, irrespective of whether they have osteoarthritis. For these reasons, age should also be an important consideration when evaluating the effect of disease on prey vulnerability.
The incidence of osteoarthritis tended to be higher following years with lower kill rates (Figure 4 and Table 1). Because predation is a major cause of mortality in this moose population (Vucetich et al., 2011; Figure 3), its plausible that arthritic individuals may live longer during periods when kill rates are low, leading to an increase in osteoarthritis in the population. Conversely, the incidence of osteoarthritis may lower following years with high kill rates because of the selective removal of moose with severe osteoarthritis. Because osteoarthritis is importantly influenced by genetic factors (Fernández-Moreno et al., 2008; Valdes and Spector, 2008) our results highlight the potential for wolf predation to act as an important selective force against genes that predispose individuals to developing severe osteoarthritis at a relatively young age. Wolves may also play an important role in regulating other chronic, non-communicable diseases in prey populations given that osteoarthritis is linked to (and may be an important risk factor for) other serious health conditions–at least in humans (Wang et al., 2016; Hawker and King, 2021). Therefore, we suggest that future studies assess how selective predation by wolves is influenced by prey having osteoarthritis as well as other chronic health conditions. Valuable insights might also be gained by future studies comparing how the incidence of osteoarthritis varies among prey populations that are subject to different levels of predation by wolves.
Temporal fluctuations in the incidence of osteoarthritis could also be caused by several other processes, in addition to wolf predation. First, the incidence of osteoarthritis may be related to processes which affect gene frequencies, such as genetic drift or inbreeding given that osteoarthritis is importantly influenced by genetic factors (Fernández-Moreno et al., 2008; Valdes and Spector, 2008). Any such effects of genetic drift and inbreeding on osteoarthritis may be more pronounced in Isle Royale moose than in mainland moose populations because the Isle Royale population is a relatively small and isolated. Second, the incidence of osteoarthritis has also been linked to fluctuations in nutritional condition for moose. Specifically, previous research suggests that moose which experienced poor nutritional conditions in early life are more likely to develop osteoarthritis in later life (Peterson et al., 2010). The nutritional condition of moose is importantly determined by interannual variation in weather [summer temperatures and snow depth, (Hoy et al., 2022)] because weather can affect physiological and energetic costs for moose, moose foraging behavior, the abundance of important parasites for moose, and the growth and quality of important forage species for moose. Nutritional conditions for moose may also fluctuate over time in response to changes in the level of intraspecific competition for food. However, there is no strong evidence linking fluctuations in nutritional condition to moose abundance (a common indicator of intraspecific competition) in this study system (Hoy et al., 2022). More importantly, fluctuations in moose abundance are largely driven by wolf predation in IRNP (Vucetich et al., 2011). Thus, even if changes in moose abundance have an important influence on nutrition and the incidence of osteoarthritis, then any such fluctuations are likely to ultimately trace back to changes in predation pressure. That observation highlights the complex interrelationships among processes influencing the incidence of osteoarthritis. We suggest future research focus on assessing the top-down and bottom-up processes causing temporal fluctuations in the incidence of osteoarthritis.
Management Implications
This research adds significant evidence for how selective predation may regulate the health of prey populations (Packer et al., 2003; Barber-Meyer et al., 2007; Wild et al., 2011; Tanner et al., 2019), which has implications for two management issues. First, the management of population health for ungulates has typically focused on the use of culls or recreational hunting to reduce the incidence of disease or parasites of concern (Mysterud et al., 2019; Debow et al., 2021). However, culls and harvests tend to be less selective for old and diseased individuals than predation (Wright et al., 2006; Krumm et al., 2010). Furthermore, killing healthy prime-aged adults is likely to be less effective at controlling diseases and may reduce ungulate populations to unnecessarily low densities. Indeed, previously published simulation analyses have indicated that selective predation is more effective at reducing disease prevalence and causes smaller declines in prey populations compared to a similar rate of culling or harvest that is non-selective (Wild et al., 2011). This strongly suggests that predation is largely compensatory to overall mortality, whereas culling and hunting is largely additive to overall mortality. Moreover, field evidence suggests that recreational hunting is typically not effective for limiting the incidence of disease in ungulate populations, even when regulations are designed to increase the efficacy of recreational hunters (Mysterud et al., 2019). Although culling can limit diseases more effectively than recreational hunting, culls tend to be highly controversial among hunters and the general public (Mysterud et al., 2019). Our work supports the view that natural predator populations represent a valuable alternative approach toward disease management (Tanner et al., 2019; Escobar et al., 2020).
Second, the evidence we present for predation’s influence on the health of prey populations is also relevant for policy arguments about hunting wolf populations. More precisely, a common policy-related argument is that reasons offered for intensive wolf hunting (i.e., to mitigate threats to human safety, livestock loss, opportunities to hunt ungulates) do not outweigh the reasons to refrain from intensive wolf hunting (Vucetich et al., 2017). Those reasons for refraining include moral considerations (Vucetich et al., 2015), ecological consequences of allowing wolves to naturally regulate prey populations (Ripple et al., 2014), evolutionary consequences of allowing wolves to naturally regulate prey populations (Coltman et al., 2003; Festa-Bianchet, 2013). Our work contributes to better understanding the robustness of that argument by providing evidence for how unharvested wolf populations may influence the incidence ofosteoarthritis, a genetically based non-communicable disease.
Data Availability Statement
The raw data supporting the conclusions of this article will be made available by the authors, without undue reservation.
Ethics Statement
The animal study was reviewed and approved by the Institutional Animal Care and Use Committee (IACUC) at Michigan Technological University (protocol number L0093R).
Author Contributions
SH, JV, and RP conceived the ideas for the study. RP and JV led data collection. SH carried out the analysis and led the writing of the manuscript. All authors contributed to editing drafts and gave final approval for publication.
Funding
This work was supported by the U.S. National Science Foundation (DEB-1453041 to JV), Isle Royale National Park (CESU Task Agreement No. P16AC00004 to JV), and a McIntire-Stennis Grant (USDA-Nifa#1014575 to JV). This work was also supported by private donations.
Author Disclaimer
The views expressed here do not necessarily reflect those of the U.S. National Park Service.
Conflict of Interest
The authors declare that the research was conducted in the absence of any commercial or financial relationships that could be construed as a potential conflict of interest.
Publisher’s Note
All claims expressed in this article are solely those of the authors and do not necessarily represent those of their affiliated organizations, or those of the publisher, the editors and the reviewers. Any product that may be evaluated in this article, or claim that may be made by its manufacturer, is not guaranteed or endorsed by the publisher.
Acknowledgments
We are grateful to the many individuals who contributed to the collection of field data. We are also grateful to the U.S. National Park Service for permitting us to conduct research in Isle Royale National Park and for facilitating field work activities.
References
Barber-Meyer, S. M., White, P. J., and Mech, L. (2007). Survey of selected pathogens and blood parameters of northern yellowstone elk: wolf sanitation effect implications - core reader. Am. Midl. Nat. 158, 369–381. doi: 10.1674/0003-0031(2007)158[369:sospab]2.0.co;2
Barnes, C. A. (1988). Aging and the physiology of spatial memory. Neurobiol. Aging 9, 563–568. doi: 10.1016/S0197-4580(88)80114-3
Berger-Tal, O., Mukherjee, S., Kotler, B. P., and Brown, J. S. (2009). Look before you leap: is risk of injury a foraging cost? Behav. Ecol. Sociobiol. 63, 1821–1827. doi: 10.1007/s00265-009-0809-3
Boyce, M. K., Trumble, T. N., Carlson, C. S., Groschen, D. M., Merritt, K. A., and Brown, M. P. (2013). Non-terminal animal model of post-traumatic osteoarthritis induced by acute joint injury. Osteoarthr. Cartil. 21:746. doi: 10.1016/J.JOCA.2013.02.653
Chapagain, D., Range, F., Huber, L., and Virányi, Z. (2018). Cognitive aging in dogs. Gerontology 64:165. doi: 10.1159/000481621
Chesson, J. (1978). Measuring preference in selective predation. Ecology 59, 211–215. doi: 10.2307/1936364
Chesson, J. (1983). The estimation and analysis of preference and its relationship to foraging models. Ecology 64, 1297–1304. doi: 10.2307/1937838
Coltman, D. W., O’Donoghue, P., Jorgenson, J. T., Hogg, J. T., Strobeck, C., and Festa-Bianchet, M. (2003). Undesirable evolutionary consequences of trophy hunting. Nature 426, 655–658. doi: 10.1038/nature02177
Debow, J., Blouin, J., Rosenblatt, E., Alexander, C., Gieder, K., Cottrell, W., et al. (2021). Effects of winter ticks and internal parasites on moose survival in vermont. USA. J. Wildl. Manage. 85, 1423–1439. doi: 10.1002/JWMG.22101
Doherty, T. J. (2003). Invited review: aging and sarcopenia. J. Appl. Physiol. 95, 1717–1727. doi: 10.1152/JAPPLPHYSIOL.00347.2003
Driban, J. B., Eaton, C. B., Lo, G. H., Barbe, M. F., Ward, R. J., Lu, B., et al. (2014). Greater age and body mass index at baseline are associated with the onset of rapid knee osteoarthritis: data from the osteoarthritis initiative. Osteoarthr. Cartil. 22, S381–S382. doi: 10.1016/J.JOCA.2014.02.713
Escobar, L. E., Pritzkow, S., Winter, S. N., Grear, D. A., Kirchgessner, M. S., Dominguez-Villegas, E., et al. (2020). The ecology of chronic wasting disease in wildlife. Biol. Rev. Camb. Philos. Soc. 95:393. doi: 10.1111/BRV.12568
Fernández-Moreno, M., Rego, I., Carreira-Garcia, V., and Blanco, F. J. (2008). Genetics in osteoarthritis. Curr. Genomics 9:542. doi: 10.2174/138920208786847953
Festa-Bianchet, M. (2013). “Why evolutionary biology is important for conservation,” in Ignoring Nature No More: The case for compassionate conservation, ed. M. Bekoff (Chicago: University of Chicago Press), 125–136. doi: 10.7208/CHICAGO/9780226925363.003.0009
Froy, H., Börger, L., Regan, C. E., Morris, A., Morris, S., Pilkington, J. G., et al. (2018). Declining home range area predicts reduced late-life survival in two wild ungulate populations. Ecol. Lett. 21, 1001–1009. doi: 10.1111/ELE.12965
Gasaway, W. C., Dubois, S. D., Reed, D. J., and Harbo, S. J. (1986). Estimating Moose Population Parameters From Aerial Surveys. Wisconsin, US: Fairbanks.
Gervasi, V., Nilsen, E. B., Sand, H., Panzacchi, M., Rauset, G. R., Pedersen, H. C., et al. (2012). Predicting the potential demographic impact of predators on their prey: a comparative analysis of two carnivore-ungulate systems in Scandinavia. J. Anim. Ecol. 81, 443–454. doi: 10.1111/j.1365-2656.2011.01928.x
Haagenrud, H. (1978). Layers of secondary dentine in incisors as age criteria in moose. J. Mammol. 59, 857–858. doi: 10.2307/1380153
Hawker, G. A., and King, L. K. (2021). Furthering the links between osteoarthritis and other health conditions. Rheumatology 60, 3964–3965. doi: 10.1093/RHEUMATOLOGY/KEAB376
Heurich, M., Zeis, K., Küchenhoff, H., Müller, J., Belotti, E., Bufka, L., et al. (2016). Selective predation of a stalking predator on ungulate prey. PLoS One 11:e0158449. doi: 10.1371/JOURNAL.PONE.0158449
Hoy, S. R., Forbey, J. S., Melody, D. P., Vucetich, L. M., Peterson, R. O., Koitzsch, K. B., et al. (2022). The nutritional condition of moose co-varies with climate, but not with density, predation risk or diet composition. Oikos 1:e08498. doi: 10.1111/OIK.08498
Hoy, S. R., MacNulty, D. R., Metz, M. C., Smith, D. W., Stahler, D. R., Peterson, R. O., et al. (2021). Negative frequency-dependent prey selection by wolves and its implications for predator-prey dynamics. Anim. Behav. 179, 247–265. doi: 10.1016/j.anbehav.2021.06.025
Hoy, S. R., MacNulty, D. R., Smith, D. W., Stahler, D. R., Lambin, X., Peterson, R. O., et al. (2020). Fluctuations in age structure and their variable influence on population growth. Funct. Ecol. 34, 203–216. doi: 10.1111/1365-2435.13431
Hoy, S. R., Petty, S. J., Millon, A., Whitfield, D. P., Marquiss, M., Davison, M., et al. (2015). Age and sex-selective predation as moderators of the overall impact of predation. J. Anim. Ecol. 84, 692–701. doi: 10.1111/1365-2656.12310
Hudson, P. J., Dobson, A. P., and Newborn, D. (1992). Do parasites make prey vulnerable to predation? Red grouse and parasites. J. Anim. Ecol 61, 681–692. doi: 10.2307/5623
Ingram, D. K. (2000). Age-related decline in physical activity: generalization to to nonhumans. Med. Sci. Sport. Exerc. 32, 1623–1629. doi: 10.1097/00005768-200009000-00016
Jayakody, D. M. P., Friedland, P. L., Martins, R. N., and Sohrabi, H. R. (2018). Impact of aging on the auditory system and related cognitive functions: a narrative review. Front. Neurosci. 12:125. doi: 10.3389/FNINS.2018.00125/BIBTEX
Jiménez, G., Cobo-Molinos, J., Antich, C., and López-Ruiz, E. (2018). Osteoarthritis: trauma vs disease. Adv. Exp. Med. Biol. 1059, 63–83. doi: 10.1007/978-3-319-76735-2_3
Krumm, C. E., Conner, M. M., Hobbs, N. T., Hunter, D. O., and Miller, M. W. (2010). Mountain lions prey selectively on prion-infected mule deer. Biol. Lett. 6:209. doi: 10.1098/RSBL.2009.0742
Lacourt, M., Gao, C., Li, A., Girard, C., Beauchamp, G., Henderson, J. E., et al. (2012). Relationship between cartilage and subchondral bone lesions in repetitive impact trauma-induced equine osteoarthritis. Osteoarthr. Cartil. 20, 572–583. doi: 10.1016/J.JOCA.2012.02.004
Magnhagen, C. (1991). Predation risk as a cost of reproduction. Trends Ecol. Evol. 6, 183–186. doi: 10.1016/0169-5347(91)90210-O
Manly, B. F. J. (1974). A model for certain types of selection experiments. Biometrics 30:281. doi: 10.2307/2529649
McCoy, A. M., Kemper, A. M., Boyce, M. K., Brown, M. P., and Trumble, T. N. (2020). Differential gene expression analysis reveals pathways important in early post-traumatic osteoarthritis in an equine model. BMC Genomics 21:843. doi: 10.1186/S12864-020-07228-Z/TABLES/3
Mech, L., and Delgiudices, G. D. (1985). Limitations of the marrow-fat technique as an indicator of body condition on JSTOR. Wildl. Soc. Bull. 13, 204–206.
Metz, M. C., Smith, D. W., Vucetich, J. A., Stahler, D. R., and Peterson, R. O. (2012). Seasonal patterns of predation for gray wolves in the multi-prey system of Yellowstone National Park. J. Anim. Ecol. 81, 553–563. doi: 10.1111/J.1365-2656.2011.01945.X
Montgomery, R. A., Vucetich, J. A., Peterson, R. O., Roloff, G. J., and Millenbah, K. F. (2013). The influence of winter severity, predation and senescence on moose habitat use. J. Anim. Ecol. 82, 301–309. doi: 10.1111/1365-2656.12000
Montgomery, R. A., Vucetich, J. A., Roloff, G. J., Bump, J. K., and Peterson, R. O. (2014). Where wolves kill moose: the influence of prey life history dynamics on the landscape ecology of predation. PLoS One 9:e91414. doi: 10.1371/journal.pone.0091414
Mysterud, A., Strand, O., and Rolandsen, C. M. (2019). Efficacy of recreational hunters and marksmen for host culling to combat chronic wasting disease in reindeer. Wildl. Soc. Bull. 43, 683–692. doi: 10.1002/WSB.1024
Packer, C., Holt, R. D., Hudson, P. J., Lafferty, K. D., and Dobson, A. P. (2003). Keeping the herds healthy and alert: implications of predator control for infectious disease. Ecol. Lett. 6, 797–802. doi: 10.1046/J.1461-0248.2003.00500.X
Peterson, R. O. (1977). Wolf Ecology And Prey Relationships On Isle Royale. Washington D.C: U.S. National Park Service.
Peterson, R. O., and Page, R. E. (1988). The rise and fall of isle royale wolves, 1975-1986. J. Mammal. 69, 89–99. doi: 10.2307/1381751
Peterson, R. O., Thomas, N. J., Thurber, J. M., Vucetich, J. A., and Waite, T. A. (1998). Population limitation and the wolves of isle royale. J. Mammal. 79, 828–841. doi: 10.2307/1383091
Peterson, R. O., Vucetich, J. A., Bump, J. M., and Smith, D. W. (2014). Trophic cascades in a multicausal world: isle royale and yellowstone. Annu. Rev. Ecol. Evol. Syst. 45, 325–345. doi: 10.1146/annurev-ecolsys-120213-091634
Peterson, R. O., Vucetich, J. A., Fenton, G., Drummer, T. D., and Larsen, C. S. (2010). Ecology of arthritis. Ecol. Lett. 13, 1124–1128. doi: 10.1111/j.1461-0248.2010.01504.x
Pierce, B. M., Bleich, V. C., and Bowyer, T. (2000). Selection of mule deer by mountain lions and coyotes: effects of hunting style, body size, and reproductive status. J. Mammal. 81, 462–472. doi: 10.1093/jmammal/81.2.462
Pivec, R., Johnson, A. J., Harwin, S. F., and Mont, M. A. (2013). Differentiation, diagnosis, and treatment of osteoarthritis, osteonecrosis, and rapidly progressive osteoarthritis. Orthopedics 36, 118–125. doi: 10.3928/01477447-20130122-04
R Core Team (2021). R: A language and environment for statistical computing. Vienna: R Foundation for Statistical Computing. Available online at: https://www.R-project.org/
Rickey, E. J., Cruz, A. M., Trout, D. R., McEwen, B. J., and Hurtig, M. B. (2012). Evaluation of experimental impact injury for inducing post-traumatic osteoarthritis in the metacarpophalangeal joints of horses. Am. J. Vet. Res. 73, 1540–1552. doi: 10.2460/AJVR.73.10.1540
Ripple, W. J., Estes, J. A., Beschta, R. L., Wilmers, C. C., Ritchie, E. G., Hebblewhite, M., et al. (2014). Status and ecological effects of the world’s largest carnivores. Science 343:1241484. doi: 10.1126/SCIENCE.1241484
Rolandsen, C. M., Solberg, E. J., Heim, M., Holmstrøm, F., Solem, M. I., and Sæther, B. E. (2008). Accuracy and repeatability of moose (Alcesalces) age as estimated from dental cement layers. Eur. J. Wildl. Res. 54, 6–14. doi: 10.1007/s10344-007-0100-8
Short, K., Bigelow, M., Kahl, J., Singh, R., Coenen-Schimke, J., Raghavakaimal, S., et al. (2005). Decline in skeletal muscle mitochondrial function with aging in humans. Proc. Natl. Acad. Sci. U.S.A. 102, 5618–5623. doi: 10.1073/PNAS.0501559102
Spear, P., Moore, R. J., Kim, C. B., Xue, J. T., and Tumosa, N. (1994). Effects of aging on the primate visual system: spatial and temporal processing by lateral geniculate neurons in young adult and old rhesus monkeys. J. Neurophysiol. 72, 402–420. doi: 10.1152/JN.1994.72.1.402
Ståhlberg, S., Bassi, E., Viviani, V., and Apollonio, M. (2017). Quantifying prey selection of Northern and Southern European wolves (Canis lupus). Mamm. Biol. 83, 34–43. doi: 10.1016/j.mambio.2016.11.001
Tanaka, H., and Seals, D. (2008). Endurance exercise performance in Masters athletes: age-associated changes and underlying physiological mechanisms. J. Physiol. 586, 55–63. doi: 10.1113/JPHYSIOL.2007.141879
Tanner, E., White, A., Acevedo, P., Balseiro, A., Marcos, J., and Gortázar, C. (2019). Wolves contribute to disease control in a multi-host system. Sci. Reports 2019 91, 1–12. doi: 10.1038/s41598-019-44148-9
Temple, S. A. (1987). Do predators always capture substandard individuals disproportionately from prey populations. Ecology 68, 669–674. doi: 10.2307/1938472
Torretta, E., Serafini, M., Imbert, C., Milanesi, P., and Meriggi, A. (2017). Wolves and wild ungulates in the Ligurian Alps (western Italy): prey selection and spatialoral interactions. Mammalia 81, 537–551.
Valdes, A. M., and Spector, T. D. (2008). The contribution of genes to osteoarthritis. Rheum. Dis. Clin. North Am. 34, 581–603. doi: 10.1016/J.RDC.2008.04.008
Vucetich, J. A., Bruskotter, J. T., and Nelson, M. P. (2015). Evaluating whether nature’s intrinsic value is an axiom of or anathema to conservation. Conserv. Biol. 29, 321–332. doi: 10.1111/COBI.12464
Vucetich, J. A., Bruskotter, J. T., Nelson, M. P., Peterson, R. O., and Bump, J. K. (2017). Evaluating the principles of wildlife conservation: a case study of wolf (Canis lupus) hunting in Michigan. United States. J. Mammal. 98, 53–64. doi: 10.1093/JMAMMAL/GYW151
Vucetich, J. A., Hebblewhite, M., Smith, D. W., and Peterson, R. O. (2011). Predicting prey population dynamics from kill rate, predation rate and predator-prey ratios in three wolf-ungulate systems. J. Anim. Ecol. 80, 1236–1245. doi: 10.1111/j.1365-2656.2011.01855.x
Wang, H., Bai, J., He, B., Hu, X., and Liu, D. (2016). Osteoarthritis and the risk of cardiovascular disease: a meta-analysis of observational studies. Sci. Rep. 6:39672. doi: 10.1038/SREP39672
Wild, M. A., Hobbs, N. T., Graham, M. S., and Miller, M. (2011). The role of predation in disease control: a comparison of selective and nonselective removal on prion disease dynamics in deer. J. Wildl. Dis. 47, 78–93. doi: 10.7589/0090-3558-47.1.78
Keywords: bone disease, senescent related pathology, chronic pathology, selective predation, resource selection, disease dynamics, ungulates, carnivores
Citation: Hoy SR, Vucetich JA and Peterson RO (2022) The Role of Wolves in Regulating a Chronic Non-communicable Disease, Osteoarthritis, in Prey Populations. Front. Ecol. Evol. 10:819137. doi: 10.3389/fevo.2022.819137
Received: 20 November 2021; Accepted: 11 March 2022;
Published: 20 April 2022.
Edited by:
Camilla Wikenros, Swedish University of Agricultural Sciences, SwedenReviewed by:
Astrid VikStronen, University of Ljubljana, SloveniaKrzysztof Schmidt, Mammal Research Institute (PAN), Poland
Copyright © 2022 Hoy, Vucetich and Peterson. This is an open-access article distributed under the terms of the Creative Commons Attribution License (CC BY). The use, distribution or reproduction in other forums is permitted, provided the original author(s) and the copyright owner(s) are credited and that the original publication in this journal is cited, in accordance with accepted academic practice. No use, distribution or reproduction is permitted which does not comply with these terms.
*Correspondence: Sarah R. Hoy, srhoy@mtu.edu