- 1Department of Earth and Environmental Science, Botswana International University of Science and Technology, Palapye, Botswana
- 2Department of Biological, Geological and Environmental Sciences (BiGeA), Università di Bologna, Bologna, Italy
- 3Department of Geology, University of Johannesburg, Johannesburg, South Africa
- 4School of Geography, Archaeology and Environmental Studies, University of the Witwatersrand, Johannesburg, South Africa
- 5School of Environment, Earth and Ecosystem Sciences, The Open University, Milton Keynes, United Kingdom
The Makgadikgadi Basin in Botswana hosts a system of salt lakes, which developed from the Upper Pleistocene onward due to the gradual shrinking of the giant Lake Palaeo-Makgadikgadi. Stratigraphic and palaeoclimatic studies of this area are difficult due to the influence of several factors, such as a complex history of regional tectonic activities, as well as climatic changes coupled with dryland diagenetic processes. This lake, in the central Kalahari, is the key to understanding the climatic variability in the southern part of Africa in the Quaternary and has played an important role in the evolution of numerous taxa, including our own. In this study, detailed sedimentological analyses (grain size and major elements distribution) of shallow sediments from the Makgadikgadi Pans were combined with the first comprehensive study of the encountered ostracod fauna to establish trends in the environmental changes in the area from the late Quaternary. Ostracod fossil assemblages from the cores of the Makgadikgadi Pans are dominated by the Limnocythere ssp., an opportunistic taxa commonly colonizing the littoral areas of shallow evaporative, ephemeral lakes, together with the subordinate occurrences of Sarcypridopsis ochracea, Sclerocypris cf. bicornis, Candonopsis spp., and Ilyocypris spp. The sediments from the pans show fluctuations in the Cl/K and Ca/Cl ratios, often in phase with the relative abundance of Limnocythere suggesting a cyclicity induced by changes of salinity and alkalinity in the water. This multi-proxy study of the cores collected from the pans suggests a Late Pleistocene shallow, playa lake environment with strongly alkaline water, interrupted by a prolonged drought with sustained aeolian conditions between ∼16 and 2 ka BP. Increasing diversity of ostracod fauna in the top 20–30 cm of the cores indicates that a temporary shift toward higher humidity occurred around 2–1.5 ka BP and lasted through the Medieval Warm Period. This humid period was followed by an overall desiccation trend that started with the Little Ice Age and continues until the present day.
Introduction
The Makgadikgadi Basin (MB) in central Botswana (Figure 1A) is the relict area of a mega-lake system, known as Lake Palaeo-Makgadikgadi (LPM) (Grey and Cooke, 1977; Cooke and Verstappen, 1984; Thomas and Shaw, 1991; Eckardt et al., 2008; Burrough et al., 2009a,b; Riedel et al., 2012, 2014; McFarlane and Long, 2015; Schmidt et al., 2017). Today the MB hosts the world’s largest salt pans complex (e.g., Podgorski et al., 2013), including the Sua and the Ntwetwe Pans (Figure 2), and it represents a key area to investigate the Late Pleistocene–Holocene environmental changes occurring in southern Africa and their possible connection with climate variability (Burrough and Thomas, 2013). However, the age uncertainty of the LPM sediments and the complex depositional dynamics of (ephemeral) saline lacustrine systems fuelled a decade-long controversy regarding the evolution of the central and northern Kalahari (Schmidt et al., 2017).
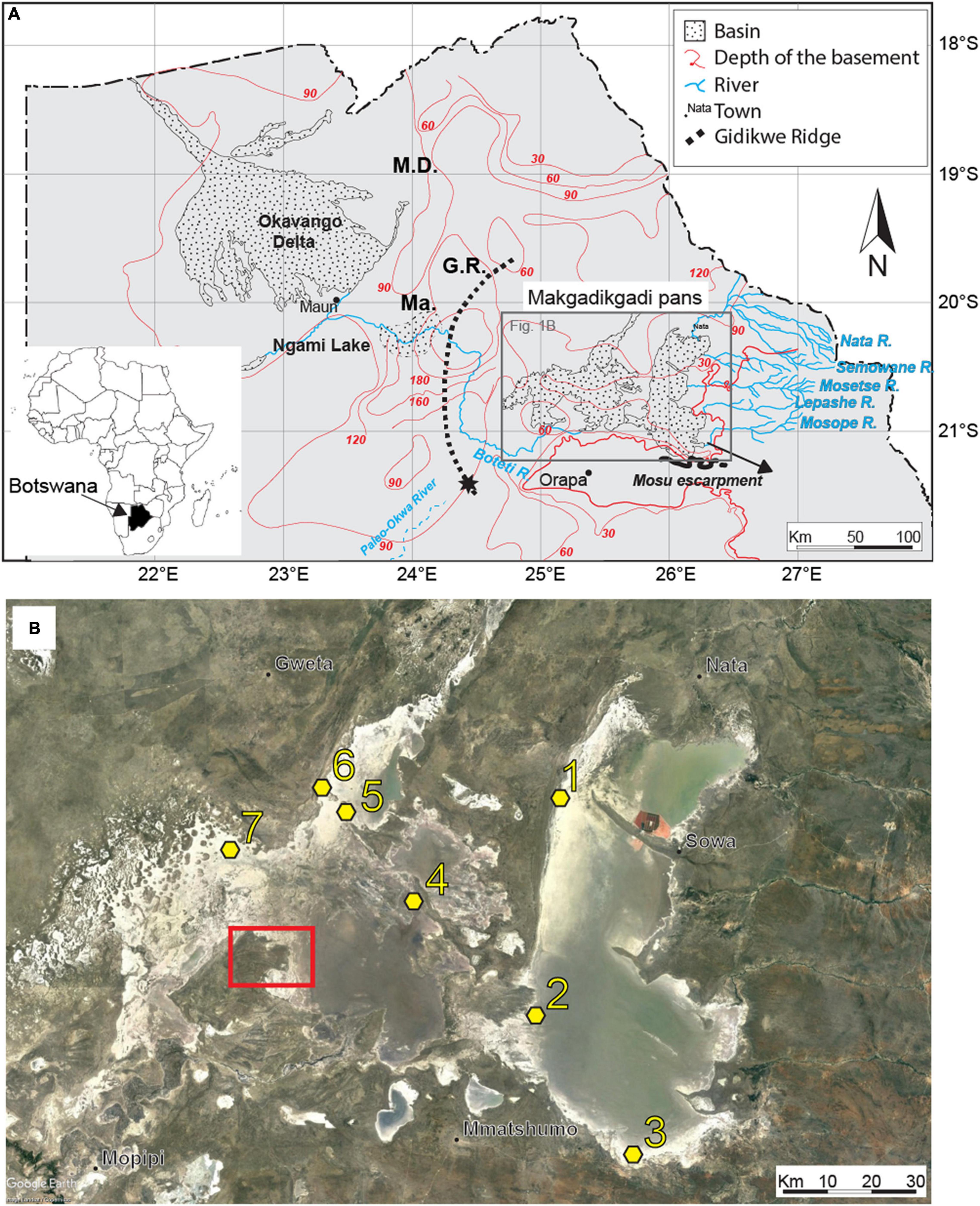
Figure 1. (A) Schematic view of the main basins in northern Botswana, showing the main affluent to the Makgadikgadi Pans and relevant geomorphic features. The star indicates the terminal Okwa Valley (Riedel et al., 2012). Ma, Makalamabedi Basin; G.R., Gidikwe ridge; M.D., Mababe Depression. (B) Satellite image of the Makgadikgadi Pans showing the sampling sites (yellow). 1, Sua Pan Nord core; 2, Kubu Island core, near the core presented in Riedel et al. (2012, 2014); 3, Mosu core; 4, NT mounds cores; 5, LM core; 6, NT shore core; 7, RM mound cores. Source Google Earth, Image Landsat/Copernicus, US Department of State Geographer, 2021. Red square outlines the fan delta described in Franchi et al. (2020), Figure 4 therein.
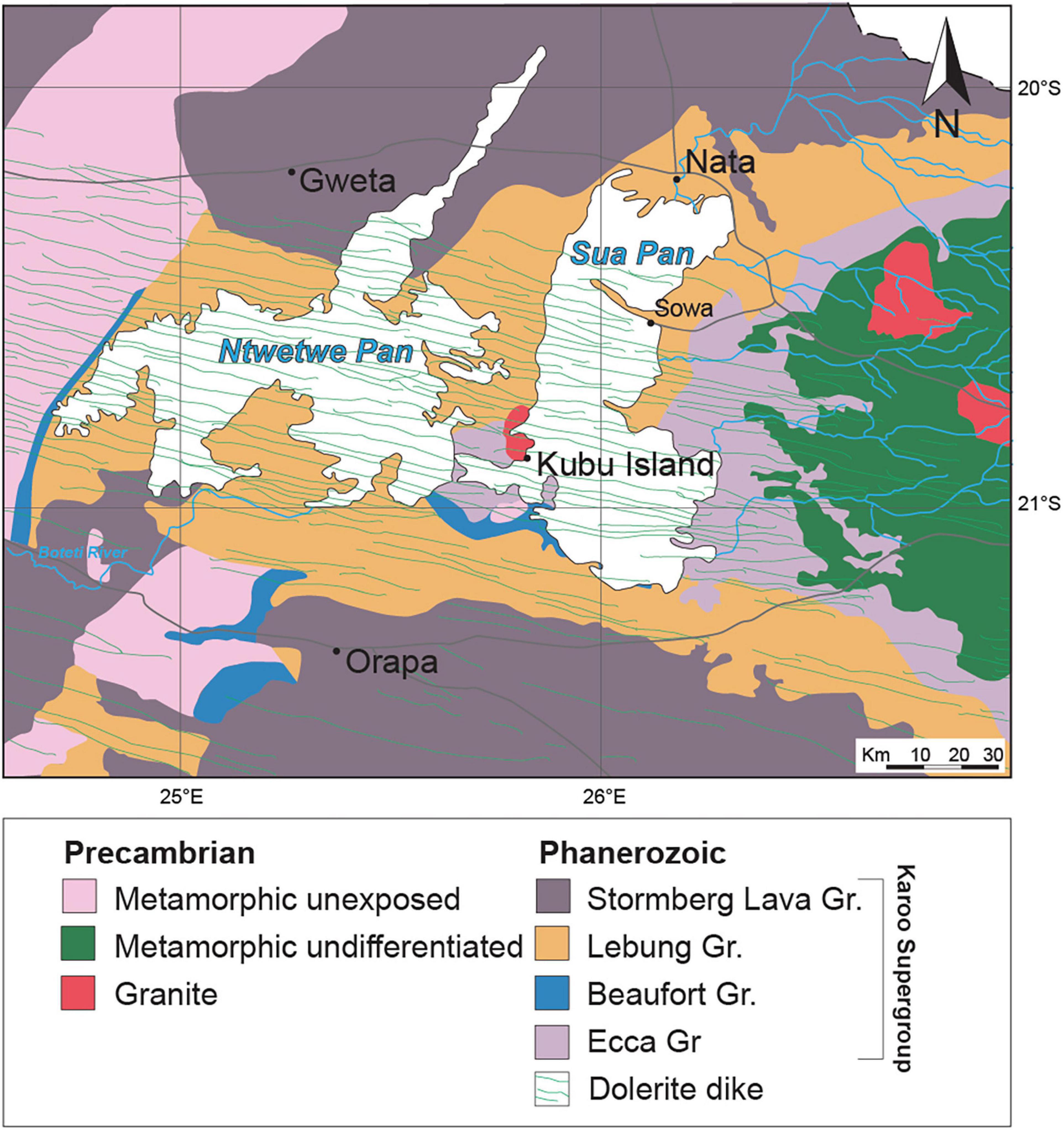
Figure 2. Schematic geological map of the Makgadikgadi Basin. Modified from Key (1997).
Moreover, being hydrologically linked with the Cubango–Okavango River Basin systems as part of the Makgadikgadi–Okavango–Zambezi Basin (MOZB; Ringrose et al., 2005; Huntsman-Mapila et al., 2006), the MB has played a pivotal role in the distribution of surface and underground water reserves in an otherwise arid to semi-arid climate. The MB has been the center of important species radiations (Joyce et al., 2005) and is considered a potential cradle of modern human beings as it provided a suitable environment for settlements as far back as 200 ka (Chan et al., 2019).
Seven highstand phases of Late Pleistocene–Holocene age were identified based on the altitudes of the palaeo-shorelines identified across the LPM. Three highstand phases were dated between 130 and 60 ka by luminescence techniques applied on shoreline sands; the other four took place over the last 40 ka: 38.7 ± 1.8 ka, 26.8 ± 1.2 ka, 17.1 ± 1.6 ka, and 8.5 ± 0.2 ka (Burrough et al., 2009a; Schmidt et al., 2017). On the contrary, a few studies have analyzed the subsurface record of the MB so far (Riedel et al., 2014; Schmidt et al., 2017; Richards et al., 2021) concluding that a spatial correlation of sediments and faunal associations across the basin is still lacking in the literature.
This work is focused on the reconstruction of the evolution trends of the LPM between the penultimate highstand phase (17.1 ± 1.6 ka; Burrough et al., 2009a; Schmidt et al., 2017) and the current condition of the ephemeral lake (playa). Shallow cores, collected from seven sites belonging to both Sua and Ntwetwe pans (Figures 1B, 2), were mainly studied in terms of grain size, major elements distribution, and ostracod fauna. Selected samples from a sampling site in the Sua Pan were dated using the Optical Stimulated Luminescence (OSL) technique and results were compared with existing radiometric ages from literature. Subsurface bio-sedimentary data were then combined for the first time along with cores stratigraphy, with the specific aim to furnish new insights on the palaeoenvironmental dynamics of the Sua and Ntwetwe Pans focusing on the Pleistocene–Holocene transition onwards.
Geological Setting
The bedrock of the MB consists of Carboniferous to Early Jurassic volcano-sedimentary units belonging to the Karoo Supergroup (Figure 2; see details in Modie and Le Hérrissé, 2009; Bordy, 2020; Franchi et al., 2021). The MB is crossed by an ESE–WNW trending doleritic dyke swarm of the Karoo large igneous province (∼187 Ma old; Elburg and Goldberg, 2000; Figure 2). The Karoo Supergroup units are covered by an estimated 50–300 m-thick succession of aeolian sands, lacustrine and fluvial deposits, and duricrusts of pedogenic origin of the Kalahari Group (Thomas and Shaw, 1991; Haddon and McCarthy, 2005).
The LPM system developed within the ∼120,000 km2 MOZB as part of the south-western branch of the East African Rift System (EARS) (Riedel et al., 2014; Schmidt et al., 2017). Its evolution is controlled by NE–SW faults closely related to the EARS propagation toward SW (Modisi et al., 2000; Kinabo et al., 2007). The oldest dated LPM strandlines yielded OSL ages of 288 ± 25 ka (Burrough et al., 2009a); however, the presence of Early Stone Age tools in the basin suggested that a precursor of the LPM was already present ∼500 ka BP (McFarlane and Eckard, 2006).
Some authors attributed the formation of the early lake system in the central Kalahari Basin to the activation (uplift) of the Chobe Fault during the Early Pleistocene (Moore et al., 2012). This early stage of evolution culminated in the formation of the palaeo mega-lake Deception, a precursor of the LPM (Moore et al., 2012). In the Early to Middle Pleistocene, the uplift of the Congo–Zambezi watershed reduced the hydrological input into the basin. Therefore, the LPM outlined by the shoreline at ∼945 m a.s.l. was formed (Thomas and Shaw, 1991; Moore et al., 2012). This phase was followed by a further, progressive contraction of the lake shoreline to ∼936, 920, and 912 m a.s.l (Thomas and Shaw, 1991; Moore et al., 2012). During the Middle to Late Pleistocene, the activation of the Okavango graben in the north-west of the Makgadikgadi Basin led to the formation of the Okavango Delta and the Makgadikgadi Pans (Burrough et al., 2009b; Moore et al., 2012). At ∼46 ky BP the main inflow in the basin was from the palaeo-Boteti and palaeo-Nata rivers and the level of the lake was at ∼912 m a.s.l (Riedel et al., 2014).
During the Last Glacial Maximum, when the lake level rose to 936 m a.s.l (Riedel et al., 2014), the Okwa River (Figure 1A) was one of the main tributaries of the LPM (Shaw et al., 1992; Nash et al., 1994; Nash and McLaren, 2003). Then, during the mid-Holocene the level of the lake dropped to 920 m a.s.l. and then to ∼908 m a.s.l. over the last millennium (Riedel et al., 2014).
Today, the MB receives seasonal surface water from local rainfall and is principally fed by ephemeral rivers flowing from the east and north-east, and seasonally by the Boteti River in the south-west (Figure 1A). The climate of the MB is arid to semi-arid, receiving relatively low mean annual rainfall (∼300 mm yr–1) with precipitation limited to the summer season (Burrough et al., 2009a). During the winter dry season (between April and October), the dominant processes are wind erosion and calcretization under playa conditions (Riedel et al., 2012). Water table fluctuations, between the wet and dry seasons, and the seasonal flooding contribute to the deposition of an ephemeral crust of evaporites and clays on the pan floor (Eckardt et al., 2008; Ringrose et al., 2009), leading to the development of consolidated surfaces (pavements). Moreover, the shallow subsurface of the pan is characterized by sub-cropping duricrusts (e.g., Nash et al., 1994).
Materials and Methods
Field Work, Sedimentological, and Geochemical Analyses
Several field campaigns were conducted between September 2017 and January 2020 across the Makgadikgadi Pans. These activities were focused in the western part of the Sua Pan and along the north-western shores of the Ntwetwe Pan (Figure 2). The western Sua Pan was selected as it is the most accessible site with active precipitation of evaporites and is cyclically inundated (McCulloch et al., 2008). A sampling site near Kubu Island (Point 2 in Figure 1B) was also chosen to allow comparisons with radiocarbon ages from a core described by Riedel et al. (2012, 2014). The north-western shores of the Ntwetwe Pan were selected for being affected by active coastline erosion during the evolution of the pan and for the presence of well-known morphologies such as barchan dunes, layered mounds, and strandlines described in the literature (Cooke, 1980; Burrough and Thomas, 2013; Franchi et al., 2020; Richards et al., 2021). Seven coring sites (Figure 1B and Table 1) were sampled using a manual percussion corer with a 20 cm stainless steel barrel, 5 cm in diameter, and an internal PVC liner, 15 cm in length, to acquire a total of nine cores up to 380 cm long (Table 1). Five cores were collected from mounds in the Ntwetwe Pan (RM3, RM5, NT2, NT3, and LM) and one from the pan strandline (NT shore) (Table 1). Three cores were collected from the Sua Pan floor (∼903 m a.s.l.) along a transect approximately S–N (Table 1). The uppermost 60–80 cm were often composed of fine loose, poorly cohesive sediments that were destructively extracted with an Auger corer every 10 cm. The lowermost 5 cm of cored sediment was retained within the nose of the stainless steel barrel and stored separately. All the cores were described and sub-sampled at 2 cm intervals (when possible) for laboratory analyses.
All sedimentological and geochemical analyses were performed at the Botswana International University of Science and Technology—BIUST (Botswana). The grain size distribution of sediment samples was obtained using a Malvern Mastersizer 3,000 laser diffraction particle size analyzer. Organic matter content was determined using the Loss on Ignition (LOI) method whereby the samples were dried at 60°C for 24 h and subsequently ignited for 2 h in a funnel furnace at 550°C (Table 2).
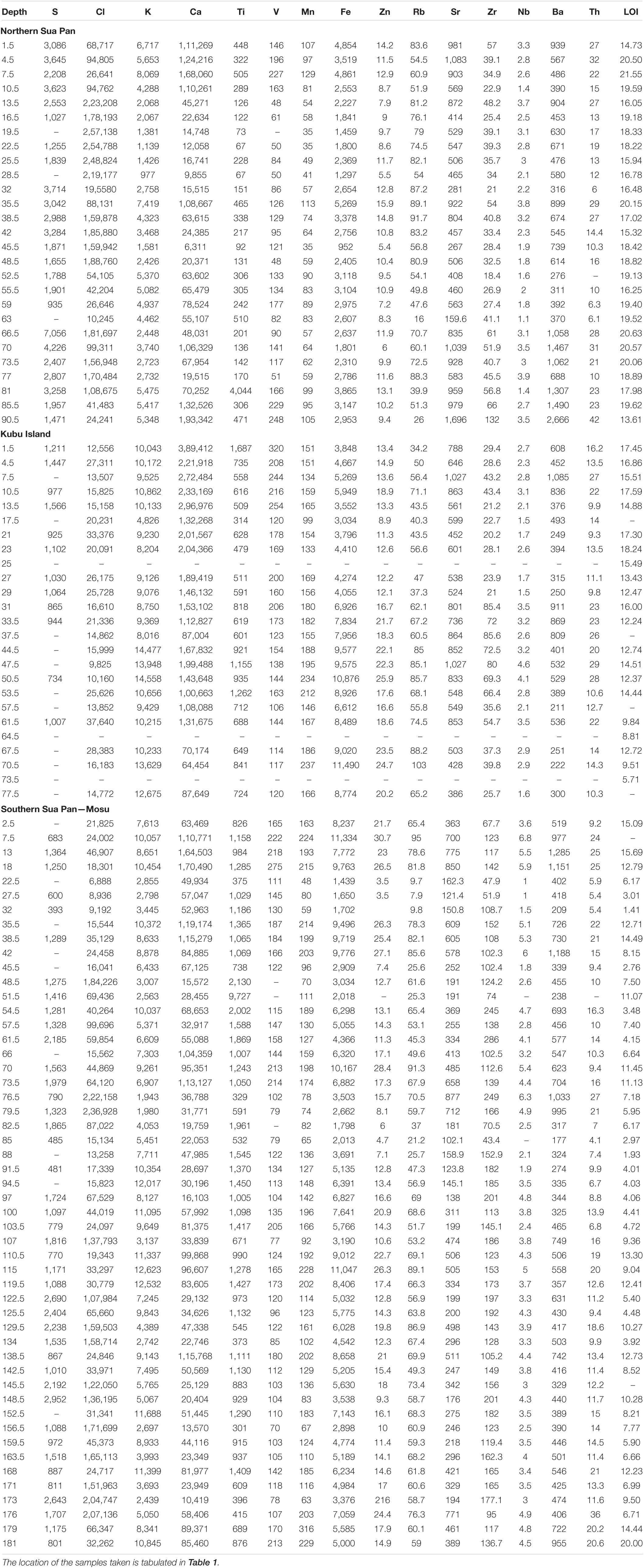
Table 2. Chemical composition (XRF) and organic matter content (LOI) of the sediments from the Sua Pan.
The three cores obtained from the Sua Pan were investigated for their geochemical composition since they represent sites with active evaporite and clay deposition. Major and trace elements distributions were obtained with a Bruker DELTA Premium Handheld X-ray Fluorescence (XRF) spectrometer (Table 2). A detailed description of the vertical variation of selected elements in the cores from the Sua Pan in relationship with sedimentological features and textures is provided in Table 3.
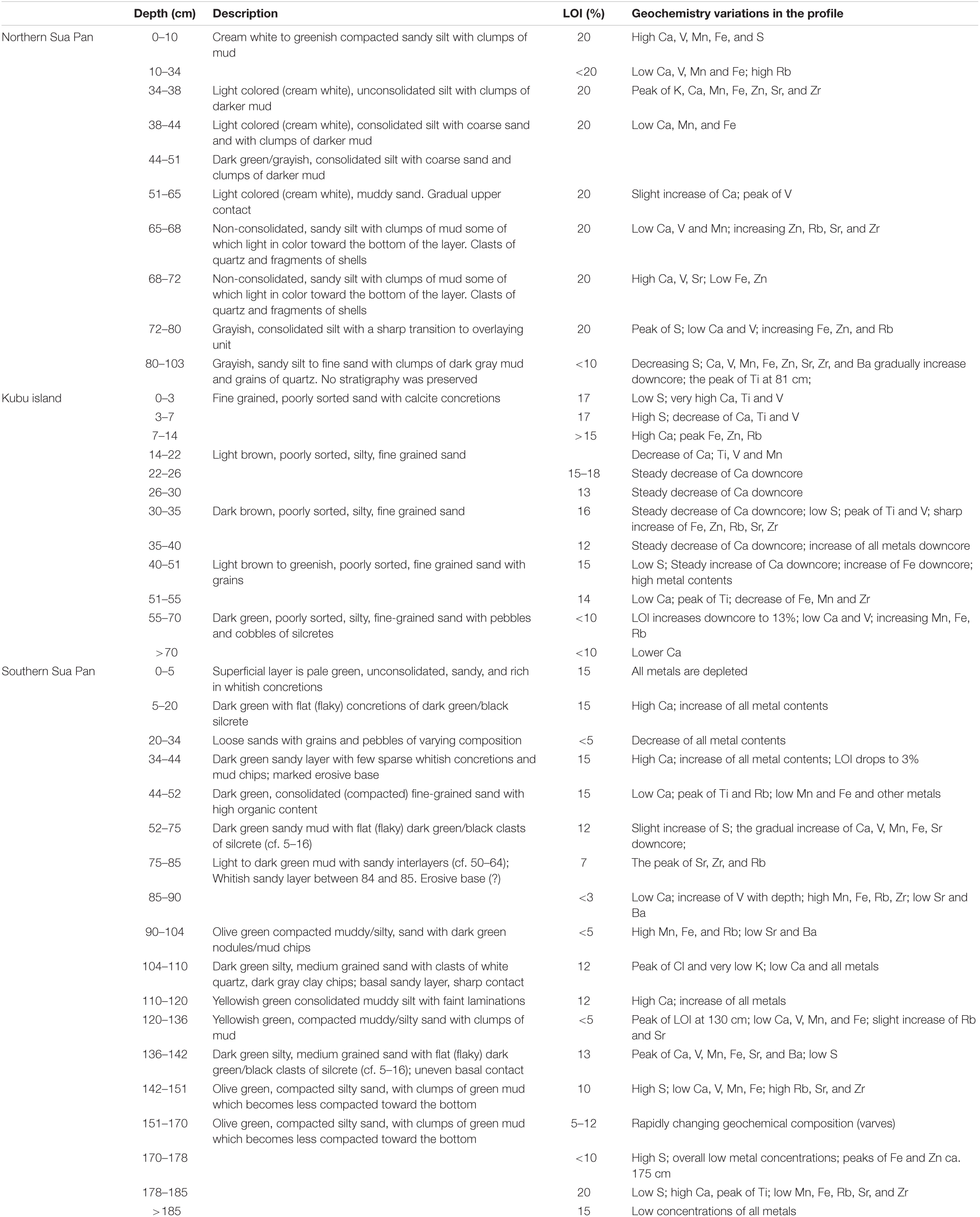
Table 3. Schematic description of the cores from Sua Pan (see Table 1 for location), including a summary of the geochemical composition and organic matter
content (LOI).
Ostracod Analysis
A total of 65 samples were collected along the length of the cores in order to characterize all the encountered lithological units in terms of ostracod content, focusing on the silty-muddy intervals where the presence of other fossil remains (mainly gastropods shells or fragments) was suggestive of an autochthonous fauna. The North Sua Pan core was not analyzed as it shows a stratigraphy almost identical to that recovered at the Kubu Island site (Figure 2).
All samples were prepared at BIUST following a standard procedure (e.g., Amorosi et al., 2014). At least 100 well-preserved valves with morphological features sufficiently developed to allow taxonomic classification was picked and counted (carapaces were considered as two valves), when possible. In the same sediment fraction, heavily recrystallized ostracods were also extracted and analyzed separately. Taxonomic identification of ostracods and autoecological information were based on Meisch (2000) and several reference papers dealing with modern and late Quaternary ostracod fauna from the Makgadikgadi Pans and African inland lakes and wetlands (e.g., Cohen et al., 1983; Martens et al., 1996; Holmes et al., 1998; McCulloch et al., 2008; Park and Cohen, 2011; Bristow et al., 2018; see also Table 4).
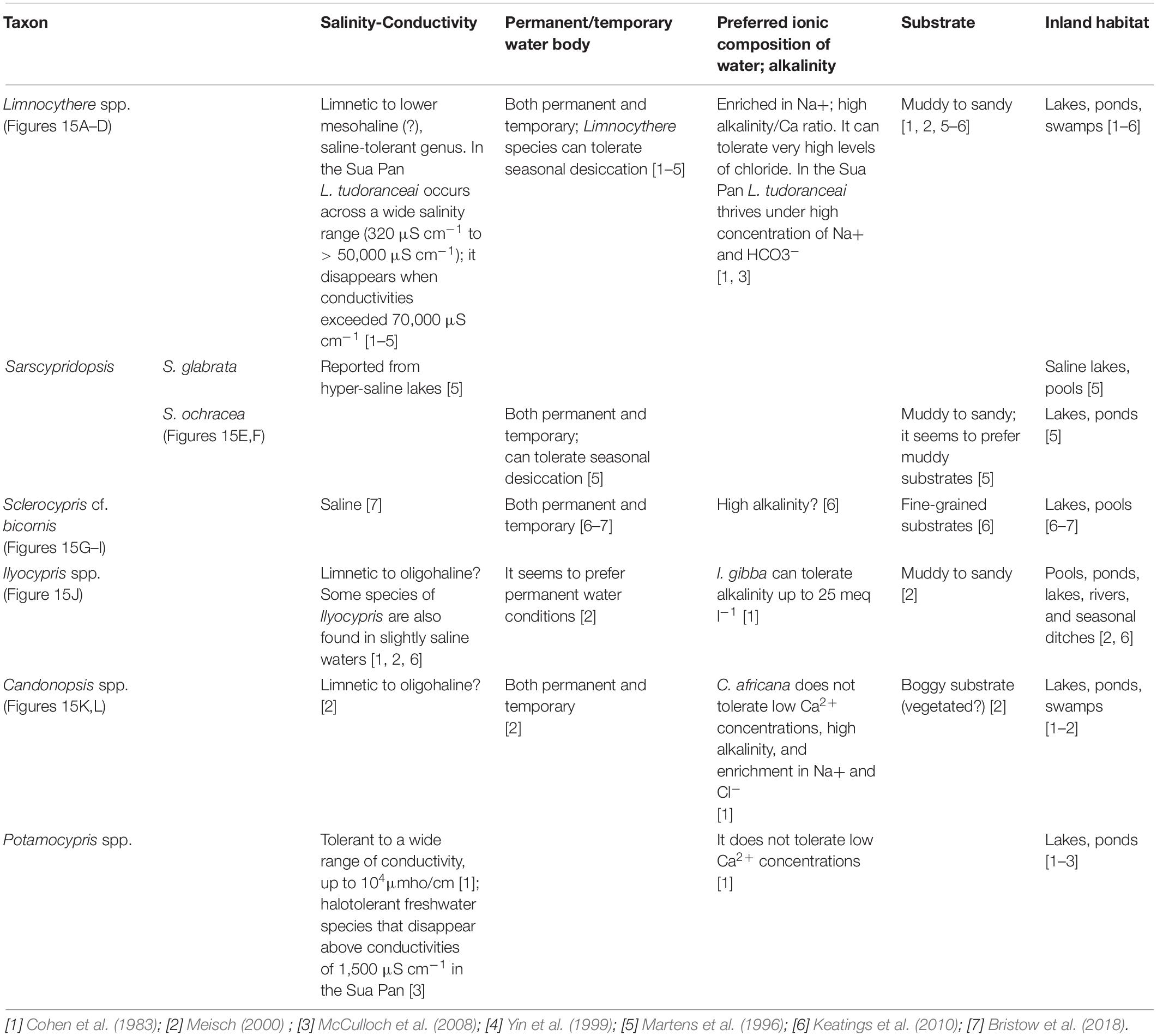
Table 4. Summary of the autecological characteristics of the main ostracod taxa identified in the cored successions.
A selection of ostracod shells was analyzed for morphology and chemical composition using a Jeol JSM-5200 Scanning Electron Microscope (SEM) equipped with an Electron Dispersive Spectrometer (EDS) IXRF Iridium at the BiGeA Department, University of Bologna. EDS analysis was undertaken on different areas of the selected valves in order to evaluate data consistency.
Optical Stimulated Luminescence Dating
Two samples from the Kubu Island core (Table 5) were dated using the OSL dating technique at the Luminescence Dating Laboratory of the University of the Witwatersrand (South Africa). The samples were left intact within their PVC liners and sealed carefully to ensure no exposure to sunlight and were prepared under red, safe-light conditions. Quartz grains with a size range of 180–250 μm were extracted and prepared according to standard procedures (Supplementary Material 1). Equivalent dose (De) values were obtained through calibrating the “natural” optical signal acquired during burial, against “regenerated” optical signals obtained by administering known amounts of laboratory dose following the single-aliquot regenerative-dose (SAR) protocol proposed by Murray and Wintle (2000). Dose rate (DR) was determined by ICP-MS (for the U, Th abundances) and XRF (for K); adjustments were made for water content, and cosmic dose contributions were determined following Prescott and Hutton (1994). Dose rate conversion followed Guérin et al. (2011) and the age was calculated by dividing the De by the DR following procedures in Aitken (1985). All given error terms are computed at one sigma.

Table 5. Results of the OSL analysis on the samples from the Kubu Island core, including ICP-MS value for Th, U, and K.
Results
Ostracod Fauna
Seven main ostracod taxa were identified in the studied cores (Table 4). The most common are: Limnocythere species (Figures 3A–D), Sarscypridopsis ochracea (Sars, 1924; Figures 3E,F), and Sclerocypris cf. S. bicornis (Müller, 1900; Figures 3G–I). Other species of Sarscypridopsis (mainly S. glabrata; Sars, 1924) and valves belonging to genera Ilyocypris (Figure 3J), Candonopsis (Figures 3K,L), and Potamocypris are also locally recorded. Well-preserved valves of Limnocythere species are widely recorded in the dataset, being found at almost all the study sites and throughout the cored successions at different stratigraphic levels. Limnocythere spp. commonly occurs as the predominant taxa (> 50% of the encountered total valves), locally forming monospecific assemblages. The genus is considered tolerant of waters with a wide range of salinity and alkalinity (Table 4). Consistently, Limnocythere-dominated assemblages have been frequently reported from littoral, shallow deposits of modern South Africa lakes and wetlands under high salinity and alkalinity levels as well as over wide ranges of Cl– concentration (Cohen et al., 1983; Martens et al., 1996; Holmes et al., 1998). McCulloch et al. (2008) documented the widespread occurrence of the species Limnocythere tudoranceai (Martens, 1990) across the Sua Pan, highlighting its capability to tolerate the wide fluctuating salinities and hydrochemical conditions of the area also characterized by a high proportion of Na+ and HCO3–.
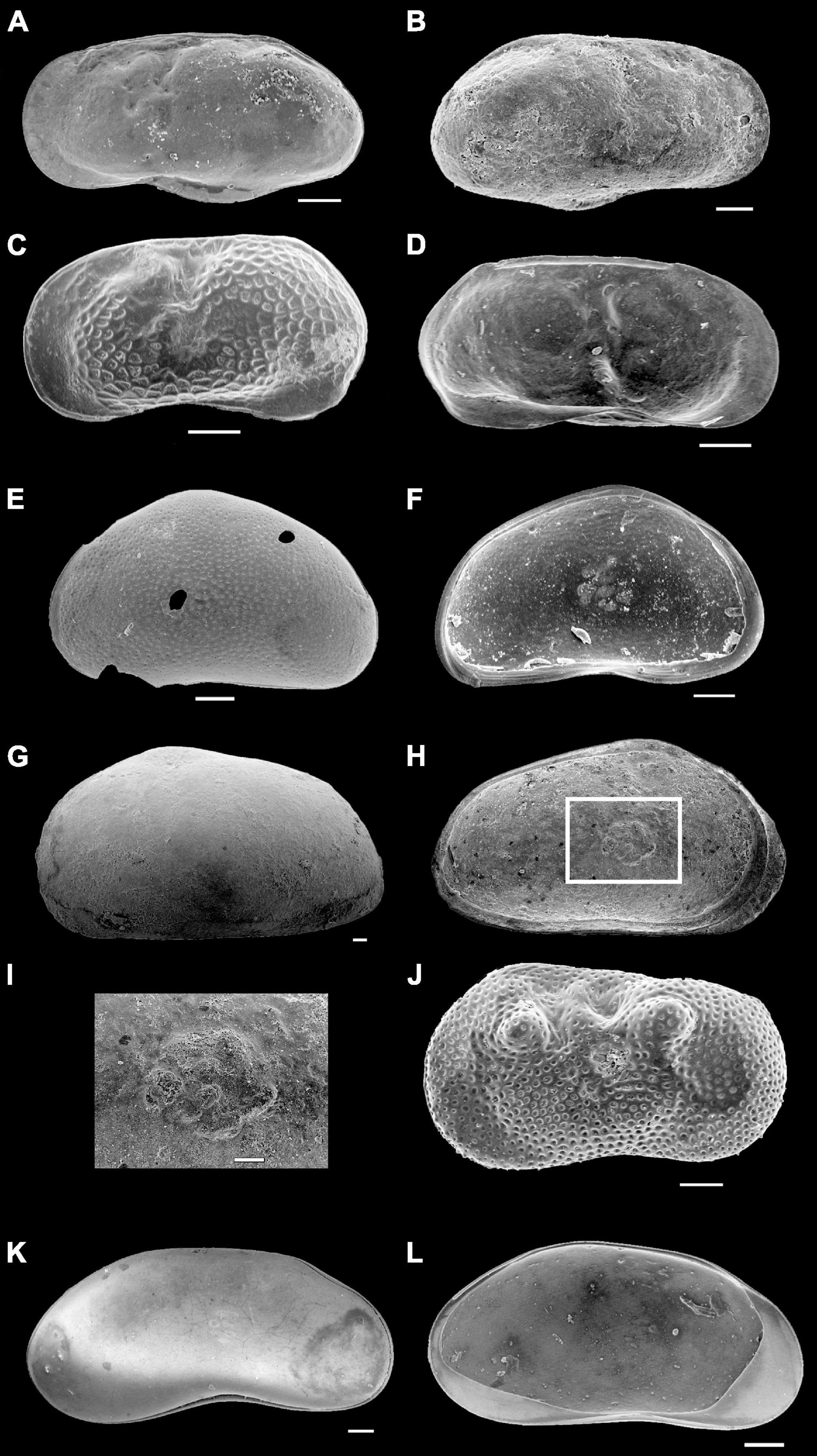
Figure 3. Scanning Electron Microscope (SEM) micrographs of ostracod valves from the Makgadikgadi Pan cores. (A–D) Limnocythere spp. (E,F) Sarscypridopsis ochracea. (G–I) Sclerocypris cf. bicornis, a close-up view of the muscle scars in (H). (J) Ilyocypris spp. (K,L) Candonopsis spp. All scale bars 100 μm.
Sarscypridopsis ochracea is the most common secondary species of the dataset, with a number of valves up to 1/5 of the total assemblage (unique exception one sample where it is the dominant taxa). The genus Sarscypridopsis is mostly an Afrotropical genus (Szwarc et al., 2021) and S. ochracea is one of the most frequently recorded species in southern Africa within both permanent and temporary water bodies (Martens et al., 1996).
Sclerocypris cf. S. bicornis is exclusively recorded from the Ntwetwe Pan with few valves/sample (1–4 valves/sample). The ecology of this species is still poorly known (Table 4). Martens (1988) reported a similar species from the Makgadikgadi Pans, Sclerocypris exserta makarikarensis, considered as endemic hence perfectly adapted to the seasonal desiccation cycles and the high alkalinity of the water. This seems to be confirmed by Bristow et al. (2018) who found specimens with strong similarity to both S. bicornis and S. exserta in the sediments of the Lake Megachad (Sars, 1924). These specimens are more quadrate and show a less prominent posterior point compared to S. exserta. This “sub-quadrate” shape also typifies the Sclerocypris valves found within the cores reported herein (Figures 3G–I). S. bicornis has also been documented from Lake Turkana and Lake Qarun in Egypt (Keatings et al., 2010), under shallow, saline, permanent water conditions (Keatings et al., 2010). The other encountered ostracods, mainly belonging to genera Ilyocypris, Candonopsis, and Potamocypris, are commonly represented by few valves (a total of less than five valves/sample); these genera seem to be sensitive to high salinity conditions and high levels of alkalinity (Table 4).
Morphological observation and SEM-EDS analyses revealed the presence of recrystallized valves and heavily recrystallized carapaces within the majority of the analyzed samples. These ostracod remains retain their overall external shape but are almost entirely silicified, as documented by the EDS intensity spectra that invariably show a marked peak in Si accompanied by a subtle peak in Mg, while the Ca peak appears negligible (Figure 4).
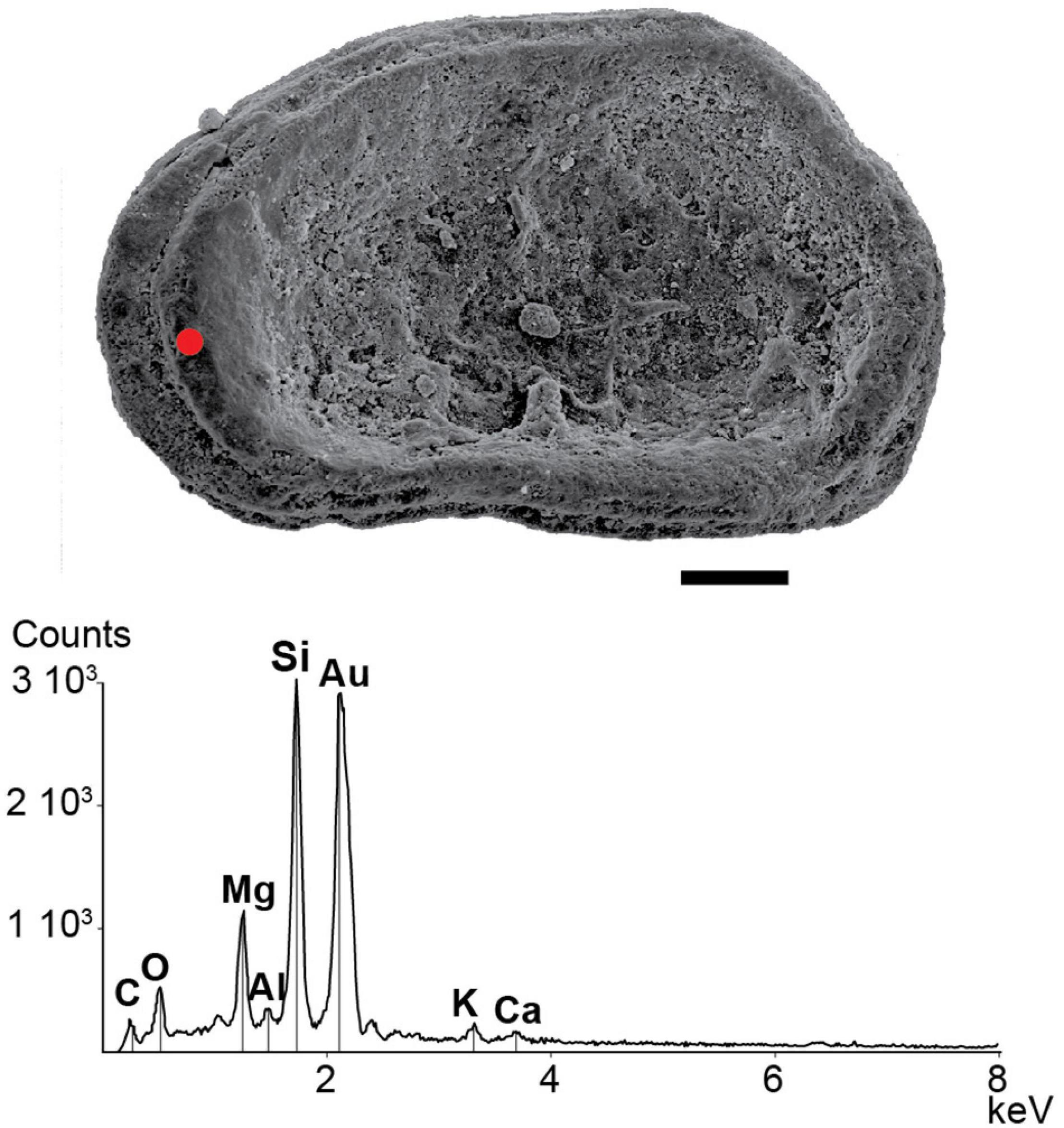
Figure 4. Scanning electron microscope micrograph of a silicified valve of Limnocythere. The EDS spectrum shows the Si-bearing composition of the ostracod shell (red spot) found in the core RM3 at 262–265 cm depth (see Figure 7). The scale bar is 100 μm.
Sua Pan Cores
Northern Sua Pan
The ∼103 cm long core drilled in the northern portion of the Sua Pan (Table 1; point 1 in Figure 1B) is detailed in Table 3 and Figure 5A. This core, collected from the pan floor at an elevation of ∼903 m a.s.l., is typified by opposite changes in K and Cl contents, which show a negative correlation (R2 = 0.75; Table 2 and Figure 6A).
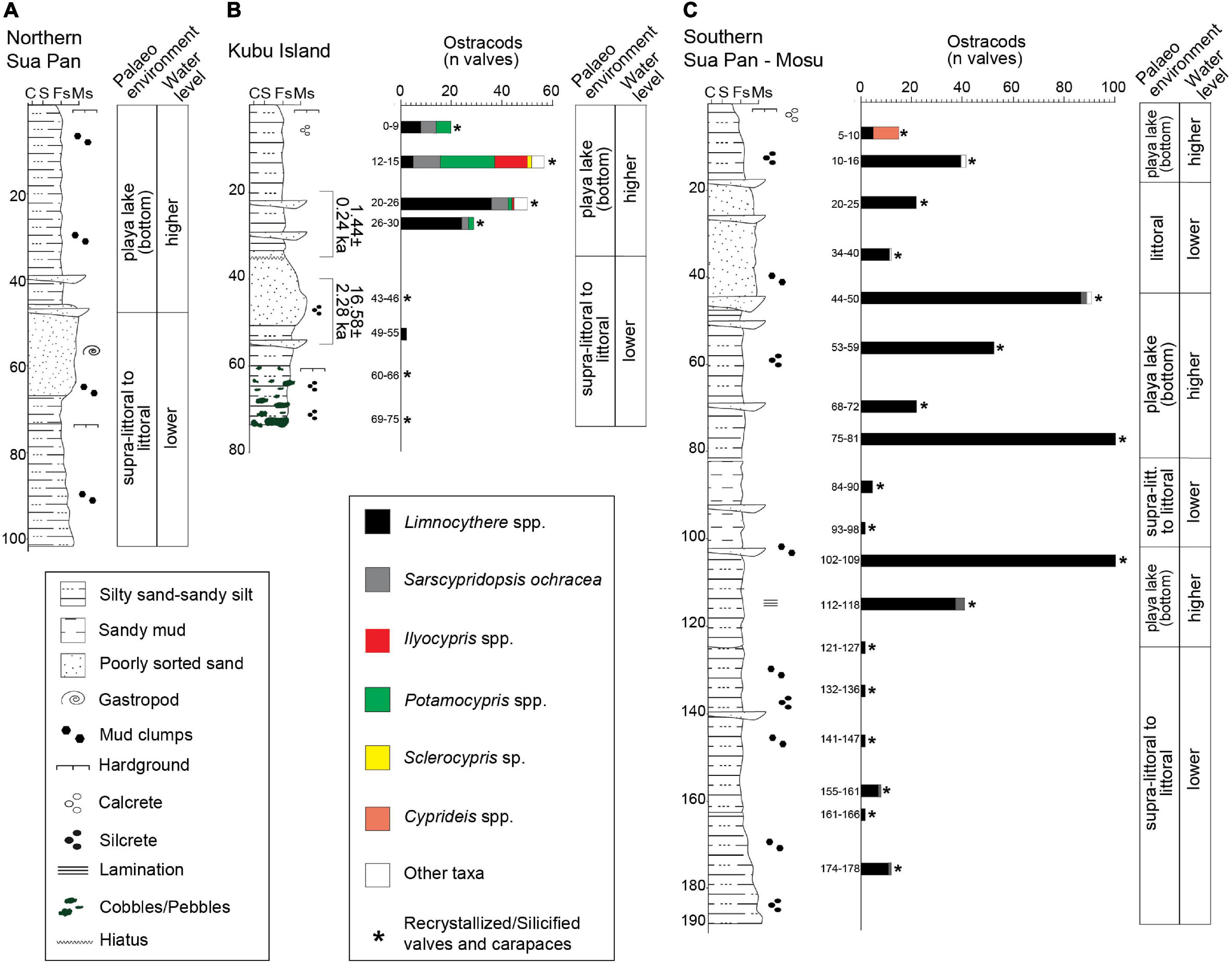
Figure 5. Stratigraphic log, vertical distribution of the main ostracod taxa (reported as number of valves) and palaeoenvironmental reconstruction of the Sua Pan cores. (A) Northern Sua Pan. (B) Kubu Island. (C) Southern Sua Pan-Mosu (see Table 1 and Figure 1B for location).
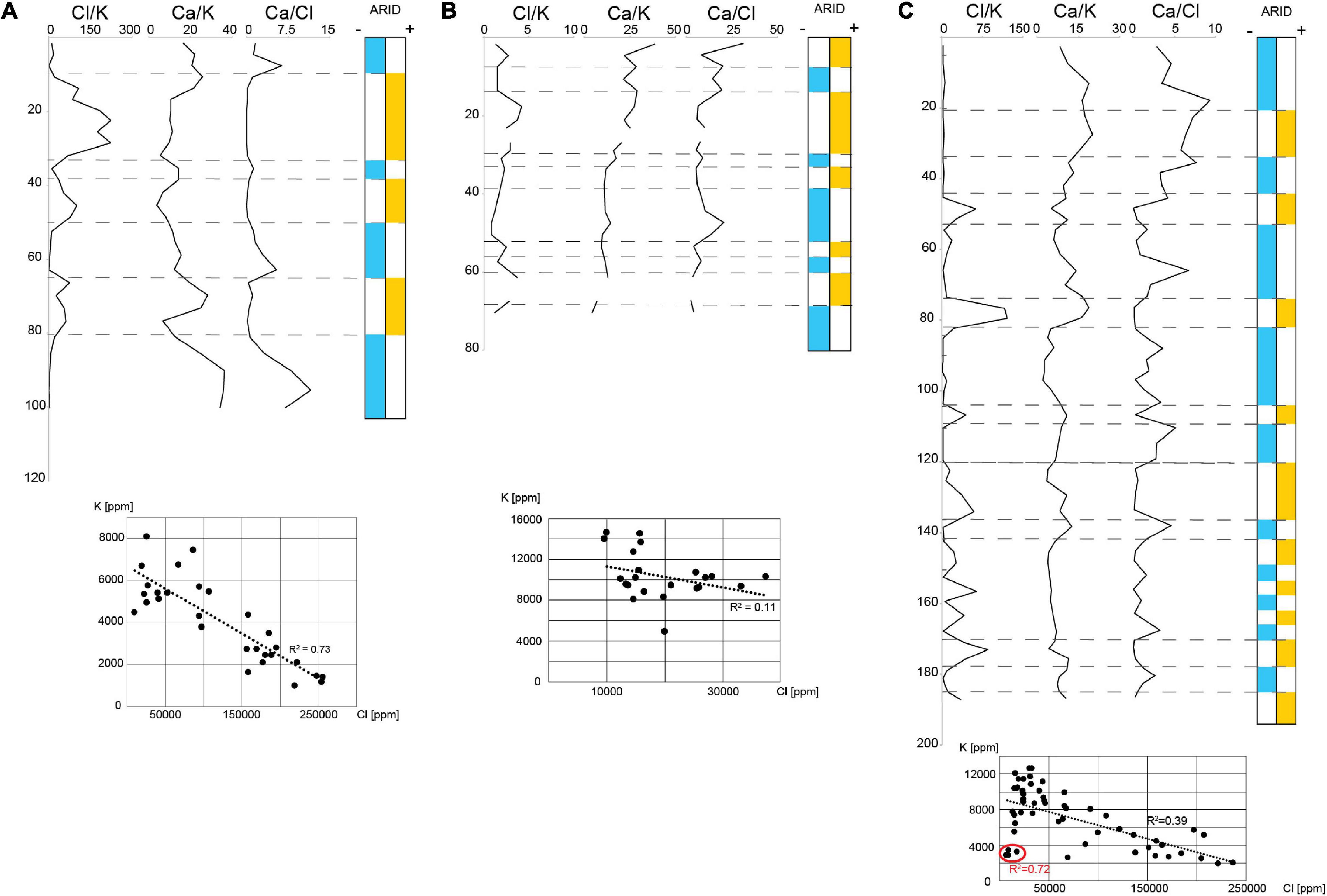
Figure 6. Vertical changes of the Cl, K, and Ca ratios and their interpretation in terms of evaporation rates-dryness in the Northern Sua Pan (A), Kubu Island (B), and Southern Sua Pan-Mosu (C). The cross plots show the degree of correlation between K and Cl.
Sediments at depths between 103 and 65 cm consist of sandy silt to very fine, poorly sorted sand, with a greenish, indurated pavement at ∼75 cm depth. These sediments are characterized by high K, Ca, Sr, and Ba contents that decrease upward. The Cl content shows a marked positive peak corresponding to the pavement. A grain size increase is observed between 65 and 38 cm depth, along with an upward decrease of Ca. An opposite trend is observed for K and Cl contents. All major and trace elements, except Cl, show a positive peak at approximately 38 cm depth. Between 38 cm depth and the top of the core, silt to poorly sorted, silty fine sand occurs. K, Ca, and Fe contents decrease upward, while Cl shows an opposite trend.
Kubu Island
A ∼75 cm long core, collected in the vicinity of Kubu Island (Table 1; point 2 in Figure 1B), is detailed in Table 3 and Figure 5B. This core was collected from the pan floor at an elevation of ∼903 m a.s.l. No evident correlation between the abundances of Cl and K is recorded (Figure 6B).
The sediments present at the depth ranging between ∼75 and 55 cm are characterized by dark green silty, fine sand with abundant pebbles, and cobbles of dark green/blackish calcretes and silcretes. The Cl contents exceed those of K; the Ca values are low but increase upcore while Fe shows an opposite trend. Abundant silicified ostracods occur, while well-preserved valves are not encountered.
Between ∼55 and 35 cm depth a normal graded bed made of poorly sorted, fine sand with grains of coarse sand grading into silty, fine sand occurs. This bed is characterized by an overall increase of Fe and other trace elements such as Sr and Ba. Abundant silicified ostracods and rare valves of Limnocythere species occur close to the lower boundary of the bed. Upcore, at depth of less than 35 cm, silty fine sands become dominant and an abrupt increase in both the richness and diversity of ostracods occurs. The ostracod fauna includes Limnocythere spp. accompanied by S. ochracea and species belonging to Sclerocypris, Ilyocypris, Candonopsis, and Potamocypris genera.
Southern Sua Pan-Mosu Village
A ∼190 cm long core, collected in the vicinity of Mosu village (Table 1; point 3 in Figure 1B), is detailed in Table 3 and Figure 5C. This core was collected from the pan floor at an elevation of ∼903 m a.s.l. The sediments at a depth ranging between ∼190 and 125 cm are characterized by olive green, silty sand with mud clumps, and small silcretes. These sediments are typified by opposite changes in K and Cl contents, although no evident correlation is highlighted (Figure 6C). The other elements (Ca, Fe, Ba, and Sr) vary every 5 cm or less suggesting laminations, although no visual identification was possible. Ba and Sr elements are slightly more abundant in the bottom portion of the core, in association with coarser sediments. Well-preserved ostracod valves are scarce and mostly represented by Limnocythere spp., while silicified ostracods are abundant. Between ∼125 and 100 cm, the dominant lithology is yellowish to light green, very fine, silty sand with interbeds of medium sand. Oscillations in K and Cl contents are broader and the number of ostracod valves, almost exclusively represented by Limnocythere, is relatively high.
Between ∼100 and 80 cm depth, light to dark green sandy mud with sand interlayers occur. In this interval, a sharp increase of Cl, Ba, and Sr contents is encountered along with several valves of Limnocythere spp. A layer of dark green, fine to very fine, silty sand, observed between ∼80 and 50 cm depth, shows an increase of K, while the Cl content drops together with Ba and Sr, as well as the number of Limnocythere valves. Between ∼50 and 20 cm dark green, poorly sorted, fine sand occurs, grading into ∼15 cm of yellowish, medium sand. The Cl content drops while K content is still fluctuating (Figure 6C).
The topmost 20 cm of the core comprises greenish, fine, silty sand organized into a fining upward trend. These sediments are characterized by a very low Cl content, and high values of K, Ca, Ba, and Sr. At the top of the core, the ostracod fauna becomes dominated by Cyprideis spp. with the second occurrence of Limnocythere species.
Ntwetwe Pan Cores
LM Mound
A ∼195 cm long core was drilled along the north-eastern shore of the Ntwetwe Pan at an elevation of ∼919 m a.s.l (point 5 in Figure 1B). The sediments found at a depth between ∼195 and 155 cm consist of a fining upward succession of yellowish, poorly sorted fine sand that grades into grayish, fine sand, and silt (Figure 7A). Only carapaces of silicified ostracods are found. The sediments become much finer between ∼155 and 70 cm depth with dominant sandy silt and horizons of very dark, consolidated muddy silt. This unit is characterized by several valves of Sarscypridopsis species including S. ochracea. At a depth between ∼100 and 70 cm, Limnocythere spp. becomes dominant with the remarkable occurrence of Sarcypridopsis, Candonopsis, and Ilyocypris species. The topmost 70 cm of the core is made up of light gray, poorly sorted, fine, and silty sand barren of ostracods.
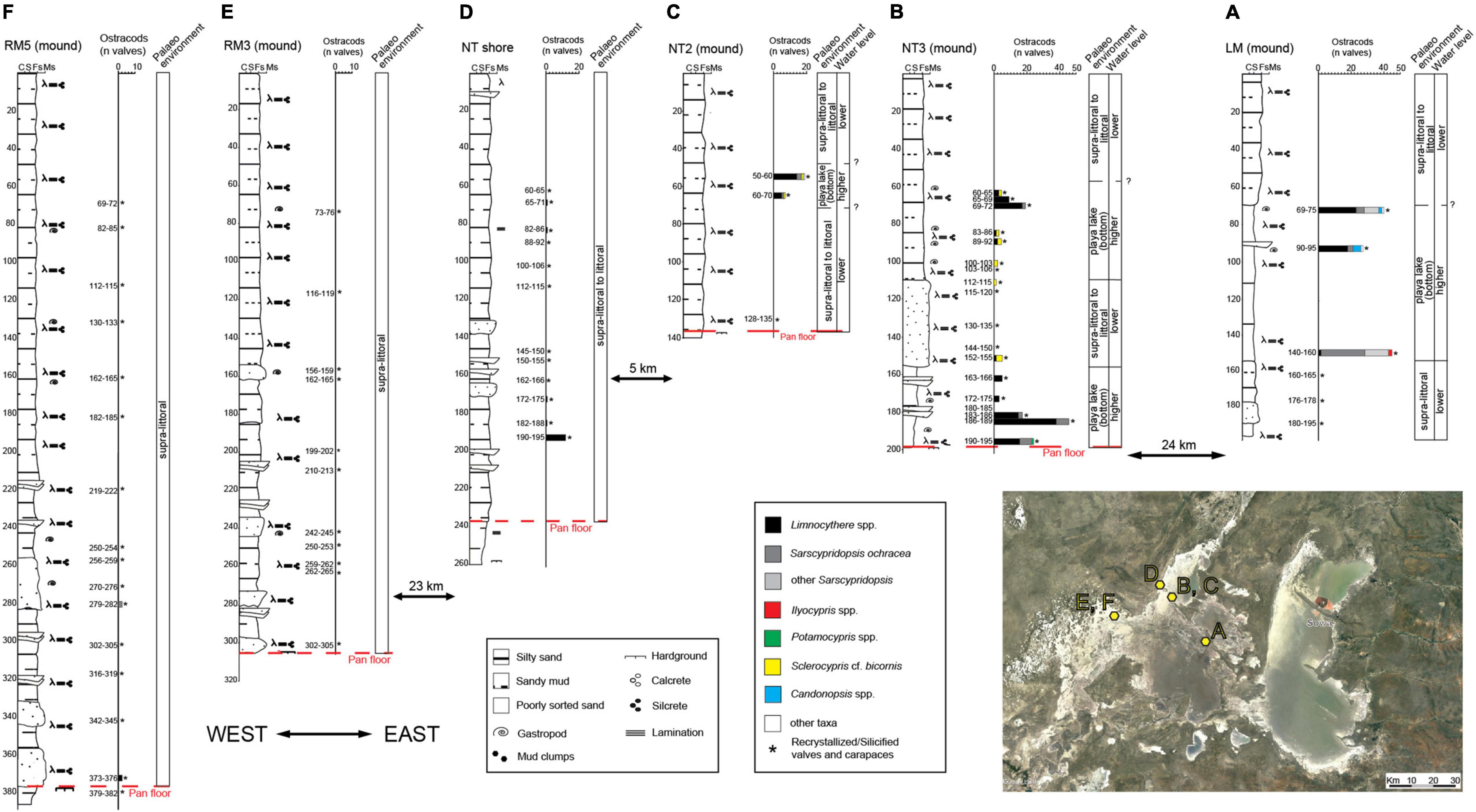
Figure 7. Stratigraphic log, vertical distribution of the main ostracod taxa (reported as number of valves) and palaeoenvironmental reconstruction of the Ntwetwe Pan cores. (A) LM mound. (B) NT3 mound. (C) NT2 mound. (D) NT shore. (E) RM3 mound. (F) RM5 mound (see Table 1 and Figure 1B for location). See legend in Figure 5 for lithological information.
NT Mounds
Two cores were recovered from the top of NT2 and NT3 mounds located in the central northern part of the Ntwetwe Pan at an elevation of ∼916 m a.s.l (Table 1; point 4 in Figure 1B).
The NT3 core, ∼195 cm in length, is characterized by sandy mud with interbeds of fine sand around ∼180 and 165 cm depth (Figure 7B). A faint lamination is observed along with the occurrence of unidentified gastropods and abundant calcretes. Between ∼195 and 180 cm depth, abundant valves of Limnocythere with subordinate S. ochracea are identified. The number of valves decreases between ∼180 and 155 cm depth. Between ∼155 and 110 cm depth well-sorted, fine, silty sand with faint laminations and abundant calcretes occurs. Except for a small number of valves of Sclerocypris cf. bicornis, and to a lesser extent Limnocythere spp., this interval is barren of well-preserved ostracods but several silicified carapaces are found. The uppermost ∼110 cm of sediments are dominantly poorly sorted, fine silty sand. Fragments of gastropod shells and rare valves of Limnocythere spp. and Sclerocypris cf. bicornis are recorded up to 60 cm; around 70–65 cm an increase in ostracod valves is observed.
The core from mound NT2, ∼135 cm in length, shows a quasi-homogeneous lithology dominated by fine to very-fine, silty sand with abundant calcretes (Figure 7C). A layer of olive green, consolidated, muddy sand was identified at ∼135 cm depth where several silicified carapaces of ostracods occur. Between ∼70 and 50 cm, a small number of valves of Limnocythere spp., S. ochracea, and Sclerocypris cf. bicornis are found along with numerous silicified carapaces.
Ntwetwe Shore
One core, ∼260 cm long, was recovered from the north-western shoreline of the Ntwetwe Pan (Table 1; point 6 in Figure 1B). The bottom corresponds to a pavement of consolidated, silty sand (Figure 7D). The sediments between ∼260 and 240 cm are made up of laminated, poorly sorted, silty sand. Between ∼240 and 175 cm depth silty sand with interbeds of medium sand suggest bedding in this portion of the core. Within the uppermost portion of this interval, few Limnocythere valves occur accompanied by abundant silicified carapaces. Between ∼175 and 130 cm depth, the sediments are less sorted and the bedding more evident with alternation of poorly sorted, silty sand and sand, exclusively containing carapaces of silicified ostracods. The topmost part of the core is characterized by 130 cm of silty, fine sand containing very few valves of Limnocythere and abundant silicified carapaces.
RM Mounds
Two cores, RM3 and RM5 (310 and 390 cm long, respectively), were recovered from the western part of the Ntwetwe Pan (Table 1, point 7 in Figure 1B).
The RM3 core shows an overall fining upward succession (Figure 7E). Between the bottom of the core and ∼120 cm depth, a series of normally graded beds can be distinguished with sparse carapaces of silicified ostracods. These beds grade from fine, silty sand into sandy silt; the basal sands of each graded bed are laminated and feature abundant calcretes and plant remains. The topmost 120 cm of the core is characterized by very fine, silty sand including carapaces of silicified ostracods.
At the bottom, the RM5 core shows a pavement of consolidated silty mud, above which an overall fining upward succession occurs (Figure 7F). Between ∼385 and 200 cm depth, a series of normally graded beds have been identified. These beds grade from fine, silty sand to sandy silt; the basal sands of each graded bed are laminated with abundant calcretes and plant remains. Rare valves of Limnocythere spp. and S. ochracea were found in sandy beds at ∼375 and 280 cm depth, otherwise, only carapaces of silicified ostracods have been identified. The topmost 200 cm of the core are characterized by very fine, silty sand with several carapaces of silicified ostracods.
Optical Stimulated Luminescence Ages
The luminescence ages of the two samples from Kubu Island are reported in Table 5 and Supplementary Material 1. The equivalent dose measurement for all samples recorded a large degree of scattering in the data (Supplementary Material 1). The MAM ages obtained from samples Kubu 20–40 cm and Kubu 40–60 cm were 1.44 ± 0.24 ka and 16.58 ± 2.28 ka, respectively.
Discussion
Ostracods were first reported from the sediments of the MB in Grey and Cooke (1977), where a lacustrine environmental context was inferred on the basis of the fauna found along the Gidikwe ridge (Figure 1A). Unidentified ostracod valves were also reported from the 20.5–16 ka old deposits of the Mababe Depression (Figure 1A), located NW of the MB pans (Shaw, 1985). A saline, lacustrine ostracod fauna including Limnocythere species (L. thomasi-group and L. aff. inopinata), Sarscypridopsis glabrata, Potamocypris aff. variegata, and Ilyocypris sp. was described from a Holocene terrace on Kubu Island by Riedel et al. (2012, 2014). The novel ostracod dataset, presented in this study, is coupled for the first time with sedimentological, geochemical, and chronological data along the stratigraphic successions recovered from both the Sua and Ntwetwe pans. This bio-sedimentary record provides new insights into the Late Pleistocene–Holocene palaeoenvironmental dynamics of the central Kalahari and the potential relationships with climate variability, as discussed below.
Sua Pan
Bio-Sedimentary Data and Palaeoenvironments
At the southern reaches of the Sua Pan (site 3 in Figure 1B), the pervasive fossil record of Limnocythere-dominated assemblages across a 190 cm-thick subsurface succession (Figure 5C) documents the long-term persistence of shallow, saline lacustrine conditions (i.e., playa lake sensu Briere, 2000). The modern distribution patterns of Limnocythere species in African wetlands and lakes point to the occurrence of a highly alkaline, chloride-enriched water body, periodically subject to desiccation phases and accompanied by evaporitic conditions (Table 4 and references herein). Northward, a similar ostracod fauna typifies the 80 cm-thick subsurface succession at Kubu Island (site 2 in Figure 1B). Such stratigraphic records extend back into the past the widespread colonization of Limnocythere species across the edges of the Sua Pan (McCulloch et al., 2008).
However, the paucity of well-preserved ostracods within the lowermost portion of the Kubu core (∼75–35 cm core depth) and three distinct intervals of the Mosu core ∼190–125 cm, 100–80 cm, and 45–20 cm core depth) reflects the (repeated) establishment of littoral to supra-littoral conditions under the influence of persistently low water levels and intense evaporitic and diagenetic/alteration processes. This interpretation is consistent with an increase of the sandy fraction and the occurrence of silica-rich duricrusts and/or pebbles-cobbles of silcretes, indicative of a playa lake marginal/littoral setting (Figure 5). Accordingly, the abundance of silicified ostracods (Figure 5), which are invariably predominant with respect to well-preserved valves, suggests the occurrence of strong diagenetic processes in the vadose zone. The recrystallization of ostracod shells can be linked to diagenetic processes driven by acidic, moderately saline conditions in the capillary zone (Ringrose et al., 2005) during periods of subaerial exposure, to which the littoral zone is more exposed. The silicified carapaces are then winnowed by wind erosion, which becomes the dominant geomorphological agent during prolonged low water level phases, and redistributed as aeolian sediments. This would explain why silicified ostracods are almost ubiquitous in the sediments under investigation.
The sandy interval recorded between ∼50 and 35 cm depth at the Kubu Island (Figure 5B) is completely barren in well-preserved ostracods and possibly corresponds to a supra-littoral environment mainly affected by wind deflation.
The uppermost part of the Sua Pan cores (∼20 cm in Mosu and ∼35 cm in Kubu) shows a rather abundant ostracod fauna (Figure 5) mainly composed of Limnocythere spp. and to a secondary extent of other taxa. Overall, the ostracod richness increases near the Kubu Island site (Figure 1B) where valves belonging to S. ochracea and genera Ilyocypris, Potamocypris, Candonopsis, and Sclerocypris also occur (Figure 5). Despite difficulties with ostracod taxonomy and the low degree of knowledge about the autoecology of several species (Table 4), this assemblage is interpreted to reflect a lacustrine stage with sustained water availability and low desiccation rates (i.e., high water level), likely accompanied by a slight decrease in salinity and a moderate alkalinity/Ca ratio (Figure 5B and Table 4).
At the Mosu site, only the uppermost sample (5–10 cm depth) includes an assemblage not dominated by Limnocythere that is largely replaced by Cyprideis (Figure 5C), confirming a recent switch to wetter conditions and an overall drop in alkalinity/Ca ratio (Cohen et al., 1983; Keatings et al., 2010; Wouters, 2017).
Changes in Water Chemistry
Geochemical analyses of sediments from the Sua Pan revealed a clear fluctuation in the relative abundances of Cl, Ca, and K (Figure 6). Variability of the Cl/K, as well as Ca/Cl, ratios might suggest changes in the aridity and an increase in the dryness of the basin. Stratigraphic intervals enriched in Ca and depleted in Cl likely reflect wetter conditions characterized by a lower evaporation rate, as chlorides tend to stay in solution for longer due to their higher solubility with respect to Ca-bearing minerals (e.g., calcite). Wetter periods might also record a higher influx of detrital silicates, including clays that lead to an increase in K contents (and further decrease of Cl/K). By contrast, during dryer periods strong evaporitic conditions promote the formation of chlorides over calcite, resulting in an increase of Cl/K paralleled by a decrease in Ca/Cl (Figure 6).
Interestingly, the highest peaks of the abundance of Limnocythere species in the cores from Kubu (∼20–30 cm depth) and Mosu (∼45, 75, and 100 cm depth) correspond to strong positive peaks in Cl concentration (Figures 5, 6) suggesting a positive relationship between evaporitic conditions and Limnocythere-dominated population, consistently with the autoecological characteristics of this opportunistic genus (Table 4).
Age of the Sediments at the Kubu Site
The sandy interval found at ∼35–50 cm depth within the Kubu Island core is dominated by silicified ostracods indicating littoral to supra-littoral conditions, chronologically constrained by an OSL-age to 16.58 ± 2.28 ka (Figure 5B and Table 5). However, the uppermost 35 cm contains the most abundant and rich ostracod fauna of the entire cored succession, pointing to submerged playa lake conditions that, although characterized by high salinity and alkalinity, were slightly more favorable to life than the present-day conditions (Figure 5B). This interval, which might represent the last wet phase in the pan, is found to be dating back to 1.44 ± 0.24 ka using the OSL method (Table 5). The OSL ages confirm the onset of prolonged dry (lowstand) conditions started around 16 ka ago and continued until ∼2 ka. During the former period, the western edges of the Sua Pan were under constant subaerial conditions with wind erosion and winnowing prevailing over deposition, justifying a potential ∼15 ka hiatus (Figure 5B). Afterward, relative environmental stability under wetter conditions took place (Burrough and Thomas, 2013).
Sediments found 3 m deep from a core ∼1.5 km to the north-east of Kubu Island were radiocarbon dated at ∼37 ka cal BP (Riedel et al., 2012, 2014). The overlying sediments at ∼2–1.6 m were dated at ∼4.6–4.4 ka cal BP, whereas sediments between 1.4 and 0.2 m depth yielded ages spanning between 1.8 and 2.2 ka cal BP (Riedel et al., 2012, 2014). The bottom of the core in the Kubu Island (Figure 5B) consists of dark sediments (Table 3; Supplementary Material 2B) that can be correlated with an organic matter-rich layer described between 60 and 80 cm depth by Riedel et al. (2012) (see also Riedel et al., 2014). The authors reported a radiocarbon age of ∼1.8 ka BP for these dark sediments revealing a marked discrepancy between radiocarbon dating and OSL ages (Table 5). This might be the result of difficulties in stratigraphic correlations between the two cores and/or lateral variation of depositional environments. However, this discrepancy can also depend on intrinsic problems with radiocarbon dating of organic-rich sediments (Lai et al., 2014; Al-Saqarat et al., 2021) in a playa or playa lake environment, where carbonates are constantly forming and dissolving following complex biogeochemical patterns and pH changes.
Ntwetwe Pan
Bio-Sedimentary Data and Palaeoenvironments
At the northern edge of the Ntwetwe Pan, the bio-sedimentary record of the mound sites (point 4 in Figure 1B) documents the succession of palaeoenvironmental changes linked to water level oscillations. At the NT3 mound, the lowermost muddy interval containing a well-preserved ostracod fauna, mostly Limnocythere spp. and S. ochracea, reflects the development of a shallow, playa lake environment (Figures 7, 8); specifically, the predominance of Limnocythere valves points to an alkaline, chloride-enriched water body (Table 4). Upcore, a marked drop in the number of ostracods is paralleled by the appearance of sandy layers that anticipate the deposition of a ∼40 cm-thick laminated sandy interval, containing numerous silicified valves/carapaces and a few scattered valves of Limnocythere spp. and Sclerocypris cf. bicornis (Figure 7B). This sandy interval is interpreted to reflect a shrinking phase of the lake that induced the subaerial exposure of the lacustrine bottom and the development of littoral to supra-littoral zone subject to wind deflation and recrystallization of ostracods. The re-establishment of a submerged environment is testified by the concomitant change in lithology (decrease in the sandy fraction) and ostracod content (increase in well-preserved valves). The co-occurrence of Limnocythere spp., Sclerocypris cf. bicornis, and, locally, S. ochracea points to saline, playa lake conditions developed under rising water levels (Figure 7). Accordingly, a similar environmental trend is typified by ostracod fauna at the nearby NT2 mound site. On the contrary, persistent littoral to supra littoral conditions occurred at the NT shore site, located ∼6 km to the NW (point 6 in Figure 1B), and along the western side of the Ntwetwe Pan in correspondence of the RM mounds (point 7 in Figure 1B), as testified by the scarcity of well-preserved ostracods and the abundance of recrystallized valves/carapaces (Figures 7D–F).
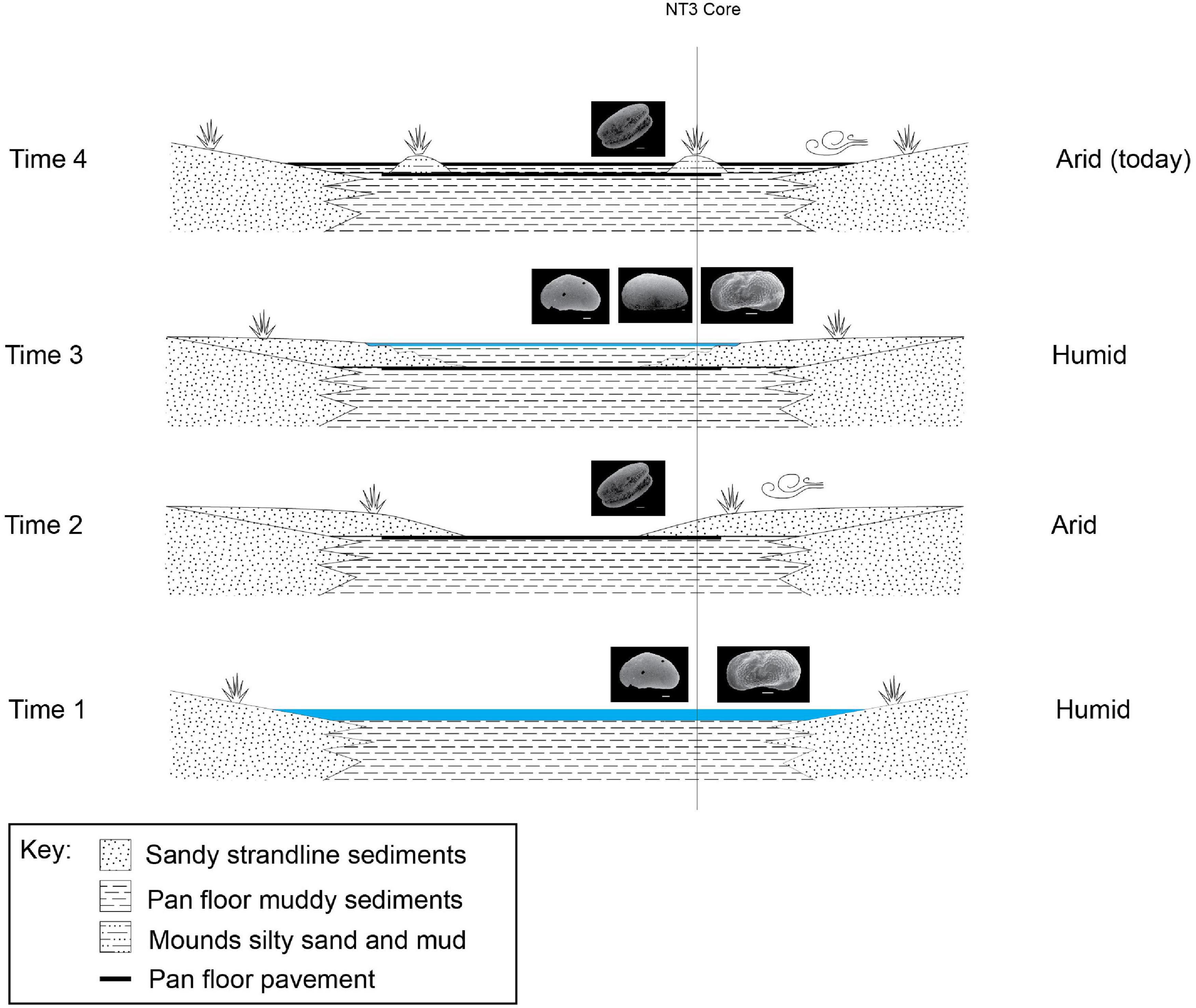
Figure 8. Schematic evolution stages of the LPM since the penultimate highstand phase (17.1 ± 1.6 ka by Burrough et al., 2009a; Schmidt et al., 2017) and the current condition of the ephemeral lake, derived from the cores stratigraphy presented in this study from core NT3 (see Figure 1B and Table 1 for location). The ostracod fauna varies upcore showing a transition from a humid period dominated by L. inopinata and S. ochracea (Time 1) to a more arid period with abundant carapaces of silicified ostracods and no preserved valve (Time 2). The environment turned toward more humid conditions (Time 3) as shown by the occurrence of L. inopinata, S. ochracea, and S. bicornis at around 80–60 cm depth. Eventually, the environment turned to more arid, playa-like conditions in the recent past (Time 4). Time 1 = ∼17 ka BP; Time 2 = ∼17 ka—2 ka BP; Time 3 = ∼2 ka BP—Medieval Warm Period; Time 4 = Little Ice Age to present day.
Unstable environmental conditions affected the eastern side of the Ntwetwe Pan, as testified by the bio-sedimentary data of the LM mound core (point 5 in Figure 1B). The superposition of a ∼1 m-thick muddy interval with a rather abundant and diversified ostracod fauna onto silt-sand deposits exclusively containing recrystallized valves/carapaces reflects an increase in the water level, which led to the submersion of the littoral zone (Figure 7A). The relatively high degree of species diversity and the widespread occurrence of Sarscypridopsis and Candonopsis species likely suggest moderately alkaline waters. The growth of Limnocythere spp. likely tracks a shallowing trend culminating with the deposition of littoral silty sands (Figure 7A).
The Late Pleistocene–Holocene Palaeoenvironmental Evolution of the Makgadikgadi Basin
The period between 135 and 70 ka was reported as a period of the extreme variability of African climate with megadroughts recorded in large tropical lakes (Malawi and Tanganyika) contraposed to extreme wet conditions in the Kenyan rift valley suggesting high interregional variability (Cohen et al., 2007).
If we consider the radiometric ages provided in literature for the shallow sediments off the shorelines of Kubu Island as reliable, we have to assume that the top 3 m of sediments in the MP are representative of the last 37 ka or less (as the cores presented here are much shorter), although this interpretation can be complicated by the discontinuous stratigraphic record and rapid lateral facies variations. During this time span, four highstand periods were identified at ∼38.7, 26.8, 17.1, and 8.5 ka (Burrough et al., 2009a). At ∼37 ka BP, the level of the water in the MB decreased to 908 m a.s.l. (Riedel et al., 2014). The 3 m-deep, sandy unit dated at 37 ka BP as described by Riedel et al. (2014) deposed into a shallow-water, near-shore environment and is followed by a sedimentary hiatus protracted until ∼4.6–4.4 ka BP. The core presented here (Figure 5B) is more proximal to Kubu Island shores (as indicated by the large cobbles and pebbles found at the bottom of the core), hence it records a longer sedimentary hiatus (up to ∼1.4 ka BP) as the area was at a slightly higher elevation and exposed to aeolian erosion under supra-littoral conditions for a longer period during the low stand phase.
The west–east transect in the Ntwetwe Pan shows a clear transition from sandy, littoral environments dominated by L. inopinata in the west (Figures 7D–F) to circa-littoral and shallow lacustrine environment in the east characterized by higher faunal diversity, especially around 2 m depth and at ∼60 cm from the top of the mounds (Figures 7A–C). In summary, the deepest sediments in the Ntwetwe Pan (e.g., core NT3) show high abundances and variability of ostracod fauna corresponding to a phase when the water level was higher than today and conditions allowed different species to survive in the pans. This can represent the last, certain highstand phase (Time 1 in Figure 8) at ∼17 ka BP, or point to more recent times when barchan dunes were stabilized into mounds by the onset of wetter conditions (see section “Discussion” in Burrough and Thomas, 2013). The mega-lake phase recorded by Burrough et al. (2009b) at around 17 ka BP was probably induced by the shift of the Inter-Tropical Convergence Zone (ITCZ) and by significantly colder climate with the consequent reduction in evapotranspiration (Riedel et al., 2014). During this phase, the level in the LPM raised as the system was fed by the inflow from the Okwa River. The mouth of the river has been identified south-west of Lake Xau (Figure 1A) at an elevation of ∼936–938 m a.s.l. by Riedel et al. (2014). The timeline proposed by Riedel et al. (2014) suggests that the Okwa River ceased flowing at around 17 ka BP. The abrupt end of the Okwa River inflow was allegedly due to the southward movement of the winter rainfall zone (linked to the ITCZ) (Riedel et al., 2014).
After the 17 ka BP high-stand phase, the sediments in the Sua Pan cores record a drop in the water level and a prolonged sediments hiatus likely caused by subaerial exposure under playa conditions (Figure 5). The ostracod fauna from the Sua Pan reveals a prolonged desiccation period between ∼17 and 2 ka, probably corresponding to a regional mega-drought (17–8.5 ka reported by Riedel et al., 2014) that dried up the Okwa river and induced a shift to playa conditions similar to those of the present day (see discussion in Richards et al., 2021). Accordingly, the sediments in the Ntwetwe Pan record a protracted arid period during which the size of the inundated part of the pan reduced drastically, and the pan floor was covered by littoral, aeolian deposits (Time 2 in Figure 8). This phase is barren of preserved valves and it is instead rich in carapaces of silicified ostracods. According to Burrough et al. (2009a), this prolonged dry period did not lead to a drying out of the LPM, although this seems to be in discordance with what was presented here.
The last highstand phase identified in the literature, i.e., 8.5 ka, corresponds to the height of the African Humid Period in the early to middle Holocene (e.g., Burrough and Thomas, 2013; Bristow et al., 2018). This highstand was not identified in the present study. This might be related to local variations in the physiography of the basin; the samples described by Burrough et al. (2009a) are located almost 100 km away from the sampling sites presented here and close to river mouths. On the other hand, probably the basin during the Holocene was more stable than thought before and similar to the present-day playa lake. This aligns with the recent findings presented in the study by Richards et al. (2021) that describes the place of formation of the fossil dunes (here called mounds) of the Ntwetwe Pan in an environment very much similar to the present-day one, neither drier nor wetter.
The ostracod data presented here show that the top 40 cm of sediments from the Sua Pan show an incredibly abundant and diverse ostracod fauna followed, in the top 10 cm, by sediments barren of ostracods (Figure 5). This reflects a rapid shift in the last millennia or two from prolonged dry (Time 2 outlined above) conditions to wetter conditions, then back to a more arid, playa-like environment probably protracted until the present day. During this highstand phase, the Sua Pan was connected to the Ntwetwe Pan (Riedel et al., 2012). This would explain why the Kubu Island ostracod fauna was found at an elevation within the range of the mounds found in the Ntwetwe Pan (Table 1). The sediments from the Ntwetwe Pan in fact record a clear return to wetter conditions, after the prolonged dry period, as indicated once again by the higher diversity in the ostracod fauna, with S. bicornis and S. ochracea occurring together with L. inopinata (Time 3 in Figure 8). Such a wetter period can be related to the Medieval Warm Period, which is generally linked to overall humid climatic conditions in Southern Africa (Tyson and Lindesay, 1992; Holmgren et al., 1999; Riedel et al., 2012). The Medieval Warm Period might have seen an inflow of water from the south-west, from the Boteti river, feeding the last lacustrine phase of the MB. Evidence for such abundance of water in the Boteti river have been recently discovered in the palaeo-drainage system of the Ntwetwe Pan. Franchi et al. (2020) have described a relict fan delta that is at an elevation comprised between 912 and 908 m a.s.l. (Figure 1B) considered the mouth of the palaeo-Boteti River. This coincides with the elevation of the oldest living baobabs on the Kubu Island (∼1 ka) (Riedel et al., 2012, 2014).
The Medieval Warm Period is followed, as already seen in the Sua Pan, by a prolonged dry period that continues to the present day and led to the final desiccation of the playa lake (Time 4 in Figure 8). This desiccation phase, probably starting with the onset of the drier Little Ice Age (Tyson and Lindesay, 1992; Holmgren et al., 1999; Riedel et al., 2012), is characterized by a decline in ostracod fauna and dominated by aeolian conditions (Time 4 in Figure 8). Nevertheless, this latest stage of evolution is hard to define since the shallowest sediments analyzed from the Sua Pan yielded discordant radiocarbon ages ranging between 1.8 and 2.2 ka cal. BP (Riedel et al., 2014) and OSL age of 1.4 ka (Table 5). Other authors suggest that the water level in the Makgadikgadi Basin has dropped below 905 m a.s.l. in the last 100–150 years, responding to a greater aridity spell beginning in the early 19th Century (Giannini et al., 2008; Riedel et al., 2012).
Conclusion
Ostracod fauna from the Sua and Ntwetwe pans shows vertical variations across the cores with clear transitions from sediments barren of preserved ostracods and dominated by silicified carapaces, to sediments with a monospecific fauna of Limnocythere species, to units with abundant specific diversity where Limnocythere ssp., Sarcypridopsis spp. (S. ochracea and S. glabrata), Candonopsis spp., and Ilyocypris spp., are found.
Limnocythere is tolerant to a wide range of environmental conditions and seems to prefer shallow waters and very high levels of chlorine in the water. Therefore, a fauna dominated by Limnocythere species can be indicative of a playa lake environment, characterized by very shallow water conditions whereby high evaporation and partial desiccation induce strong environmental stress limiting other taxa.
Sediments of the Makgadikgadi Pans record an overall desiccation trend starting with the highstand at ∼17 ka BP. The lowermost sediments present a fauna dominated by Limnocythere ssp. and S. ochracea indicative of an environment much wetter than the present-day pans. Between 17 and 2 ka BP, the ostracod fauna records prolonged periods of extreme stressful, littoral conditions dominated by Limnocythere and periods of contraction of the pan surface characterized by subaerial exposure, with consequent recrystallization of the ostracod carapaces. This prolonged dry spell is followed, at around 1.4 ka BP, by a relative increase in the lake water level under conditions wetter than the present-day and characterized by an abrupt increase of species variability in the pan sediments. This wet phase was observed to continue well into the Medieval Warm Period, after which a prolonged dry period began during the Little Ice Age and continues to the present day, which has led to the final desiccation of the lake and the onset of playa conditions.
Available data reveal a general trend of greater aridity in the Holocene of the central Kalahari and identify a prolonged dry period between 17 and 2 ka BP. This approach and the study of the cores of shallow sediments have high potential to finally unravel the geological history of the Makgadikgadi Basin. Deeper cores from across the pan will enable this reconstruction to be extended further back in time to provide a comprehensive model of the evolution of the LPM.
Data Availability Statement
The raw data supporting the conclusions of this article will be made available by the authors, without undue reservation.
Author Contributions
FF and VR conceptualized the work. FF, BC, SF, RM, PM, and AP carried out the field campaign and collected the cores. PM, GM, and VR performed the ostracod analysis. RM and PM performed the sedimentological characterization of the cores. ME performed the OSL dating. FF prepared the tables and figures for the final draft of the manuscript. All authors contributed to the final draft of the manuscript and approved the submitted manuscript.
Funding
This research was carried under research permit CMLWS 1/17/4 II (28), granted to FF, by the Ministry of Land Management, Water and Sanitation Services. The field work was partially funded by an IAS Postdoctoral Research Grant (FF) and Europlanet 2024 RI. Europlanet 2024 RI has received funding from the European Union’s Horizon 2020 Research and Innovation Programme under grant agreement number 871149.
Conflict of Interest
The authors declare that the research was conducted in the absence of any commercial or financial relationships that could be construed as a potential conflict of interest.
Publisher’s Note
All claims expressed in this article are solely those of the authors and do not necessarily represent those of their affiliated organizations, or those of the publisher, the editors and the reviewers. Any product that may be evaluated in this article, or claim that may be made by its manufacturer, is not guaranteed or endorsed by the publisher.
Acknowledgments
We would like to thank Omogolo Keobokile and Lefoko Ramakgala (BIUST) for their assistance in the field. We are also grateful to Stefano Claudio Vaiani and Giulia Barbieri for helpful discussion, Giorgio Gasparotto and Maria Roberta Randi for the technical help with the SEM-EDS analysis, and Keyron Hickman-Lewis for proofreading the article.
Supplementary Material
The Supplementary Material for this article can be found online at: https://www.frontiersin.org/articles/10.3389/fevo.2022.818417/full#supplementary-material
Supplementary Material 1 | Optical Stimulated Luminescence protocol and results.
Supplementary Material 2 | Results of the sedimentary analyses of the cores from the Sua Pan showing core pictures, grain size distribution (Dx10 = 10th percentile; D × 50 = median; D × 90 = 90th percentile), and vertical variations of selected elements (see Table 2). (A) Northern Sua Pan core; (B) Kubu Island core; (C) Southern Sua Pan-Mosu core.
Supplementary Material 3 | Results of the sedimentary analyses of the cores from the Ntwetwe Pan showing core pictures and grain size distribution (D × 10 = 10th percentile; D × 50 = median; D × 90 = 90th percentile). (A) LM mound. (B) NT3 mound. (C) NT2 mound. (D) NT shore. (E) RM3 mound. (F) RM5 mound.
References
Al-Saqarat, B. S., Abbas, M., Lai, Z., Gong, S., Alkuisi, M. M., Hamad, A. M., et al. (2021). A wetland oasis at wadi gharandal spanning 125–70 ka on the human migration trail in southern jordan. Quat. Res. 100, 154–169. doi: 10.1017/qua.2020.82
Amorosi, A., Rossi, V., Scarponi, D., Vaiani, S. C., and Ghosh, A. (2014). Postglacial coastal dynamics, N tuscan coast, italy. Boreas 43, 939–954. doi: 10.1111/bor.12077
Bordy, E. M. (2020). Depositional style changes during the permo-carboniferous-early jurassic evolution of the central kalahari karoo sub-basin, botswana. Geol. J. 55, 5514–5539. doi: 10.1002/gj.3751
Briere, P. R. (2000). Playa, playa lake, sabkha: proposed definitions for old terms. J. Arid Environ. 45, 1–7. doi: 10.1006/jare.2000.0633
Bristow, C. S., Holmes, J. A., Mattey, D., Salzmann, U., and Sloane, H. J. (2018). A late holocene palaeoenvironmental ‘snapshot’ of the angamma delta, lake megachad at the end of the african humid period. Quat. Sci. Rev. 202, 182–196. doi: 10.1016/j.quascirev.2018.04.025
Burrough, S. L., and Thomas, D. S. G. (2013). Central southern africa at the time of the african humid period: a new analysis of holocene palaeoenvironmental and palaeoclimate data. Quat. Sci. Rev. 80, 29–46. doi: 10.1016/j.quascirev.2013.08.001
Burrough, S. L., Thomas, D. S. G., and Bailey, R. M. (2009a). Mega-lake in the kalahari: a late pleistocene record of the paleolake makgadikgadi system. Quat. Sci. Rev. 28, 1392–1411. doi: 10.1016/j.quascirev.2009.02.007
Burrough, S. L., Thomas, D. S. G., and Singarayer, J. S. (2009b). Late quaternary hydrological dynamics in the middle kalahari: forcing and feedbacks. Earth Sci. Rev. 96, 313–326. doi: 10.1016/j.earscirev.2009.07.001
Chan, E. K. F., Timmermann, A., Baldi, B. F., Moore, A. E., Lyons, R. J., Lee, S.-S., et al. (2019). Human origins in a southern african palaeo-wetland and first migrations. Nature 575, 185–189. doi: 10.1038/s41586-019-1714-1
Cohen, A. S., Dussinger, R., and Richardson, J. (1983). Lacustrine paleochemical interpretations based on eastern and southern african ostracods. Palaeogeogr. Palaeoclimatol. Palaeoecol. 43, 129–151. doi: 10.1016/0031-0182(83)90051-2
Cohen, A. S., Stone, J. R., Beuning, K. R. M., Park, L. E., Reinthal, P. N., Dettman, D., et al. (2007). Ecological consequences of early late pleistocene megadroughts in tropical africa. PNAS 104, 16422–16427. doi: 10.1073/pnas.0703873104
Cooke, H. J. (1980). Landform evolution in the context of climatic change and neoto-tectonism in the middle kalahari of north-central botswana. Trans. Inst. Br. Geogr. 5, 80–99. doi: 10.2307/622100
Cooke, H. J., and Verstappen, T. H. (1984). The landforms of the western makgadikgadi basin in northern botswana, with a consideration of the chronology of the evolution of lake-paleo makgadikgadi. Zeitschrift du Geomorphol. 28, 1–19.
Eckardt, F. D., Bryant, R. G., McCulloch, G., Spiro, B., and Woode, W. W. (2008). The hydrochemistry of a semi-arid pan basin case study: sua pan, makgadikgadi, botswana. Appl. Geochem. 23, 1563–1580. doi: 10.1016/j.apgeochem.2007.12.033
Elburg, M., and Goldberg, A. (2000). Age and geochemistry of karoo dolerite dykes from northeast botswana. J. Afr. Earth Sci. 31, 539–554. doi: 10.1016/s0899-5362(00)80006-8
Franchi, F., Kelepile, T., Di Capua, A., De Wit, M. C. J., Kemiso, O., Lasarwe, R., et al. (2021). Lithostratigraphy, sedimentary petrography and geochemistry of the upper karoo supergroup in the central kalahari karoo sub-basin, botswana. J. Afr. Earth Sci. 173:104025. doi: 10.1016/j.jafrearsci.2020.104025
Franchi, F., MacKay, R., Selepeng, A. M., and Barbieri, R. (2020). Layered mound, inverted channels and polygonal fractures from the makgadikgadi pan (botswana): possible analogues for martian aqueous morphologies. Planet. Space Sci. 192:105048. doi: 10.1016/j.pss.2020.105048
Galbraith, R. F. (1994). Some applications of radial plots. J. Am. Statist. Assoc. 89, 1232–1242. doi: 10.1080/01621459.1994.10476864
Giannini, A., Biasutti, M., Held, I. M., and Sobel, A. H. (2008). A global perspective on african climate. Clim. Chan. 90, 359–383. doi: 10.1007/s10584-008-9396-y
Grey, D. R. C., and Cooke, H. J. (1977). Some problems in the quaternary evolution of the landforms of northern botswana. Catena 4, 123–133. doi: 10.1016/0341-8162(77)90014-5
Guérin, G., Mercier, N., and Adamiec, G. (2011). Dose-rate conversion factors: update. Ancient TL 29, 5–8.
Haddon, I., and McCarthy, T. (2005). The mesozoic–cenozoic interior sag basins of central africa: the late-cretaceous– cenozoic kalahari and okavango basins. J. Afr. Earth Sci. 43, 316–333. doi: 10.1016/j.jafrearsci.2005.07.008
Holmes, J. A., Fothergill, P. A., Street-Perrott, F. A., and Perrott, R. A. (1998). A high-resolution holocene ostracod record from the sahel zone of north-eastern nigeria. J. Paleolimnol. 20, 369–380.
Holmgren, K., Karlén, W., Lauritzen, S. E., Lee-Thorp, J. A., Partridge, T. C., Piketh, S., et al. (1999). A 3000-year highresolution stalagmite-based record of palaeoclimate for northeastern south africa. Holocene 9, 295–309. doi: 10.1191/095968399672625464
Huntsman-Mapila, P., Ringrose, S., Mackay, A. W., Downey, W. S., Modisi, M., Coetzeed, S. H., et al. (2006). Use of the geochemical and biological sedimentary record in establishing palaeo-environments and climate change in the lake ngami basin, NW Botswana. Quat. Int. 148, 51–64. doi: 10.1016/j.quaint.2005.11.029
Joyce, D. A., Lunt, D. H., Bills, R., Turner, G. F., Katongo, C., Duftner, N., et al. (2005). An extant cichlid fish radiation emerged in an extinct pleistocene lake. Nature 435, 90–95. doi: 10.1038/nature03489
Keatings, K., Holmes, J., Flower, R., Horne, D., Whittaker, J. E., and Abu-Zied, R. H. (2010). Ostracods and the holocene palaeolimnology of lake qarun, with special reference to past human–environment interactions in the faiyum (Egypt). Hydrobiologia 654, 155–176. doi: 10.1007/s10750-010-0379-4
Key, R. M. (1997). “The pre-kalahari geological of the republic of botswana. 1:1,000,000. geophysical information,” in Map Published by Department Geological Survey, eds D. I. Koosimile and H. Koketso (Lobatse, Botswana).
Kinabo, B. D., Atekwana, E. A., Hogan, J. P., Modisi, M. P., Wheaton, D. D., and Kampunzu, A. B. (2007). Fault system at the southeastern boundary of the okavango rift zone, botswana. J. Afr. Earth Sci. 48, 125–136. doi: 10.1016/j.jafrearsci.2007.02.005
Lai, Z., Mischke, S., and Madsen, D. (2014). Paleoenvironmental implications of new OSL dates on the formation of the “shell bar” in the qaidam basin, northeastern qinghai-tibetan plateau. J. Paleolimnol. 51, 197–210. doi: 10.1007/s10933-013-9710-1
Martens, K. (1988). Seven new species and two new subspecies of sclerocypris SARS, 1924 from africa, with new records of some other megalocypridinids (crustacea, ostracoda). Hydrobiologia 162, 243–273. doi: 10.1007/bf00016672
Martens, K. (1990). Revision of african limnocythere s.s. brady, 1867 (crustacea, ostracoda), with special reference to the rift valley lakes: morphology, taxonomy, evolution and (palaeo) ecology. Arch. für Hydrobiol. Suppl. 83, 453–524.
Martens, K., Davies, B. R., Baxter, A. J., and Meadows, M. E. (1996). A contribution to the taxonomy and ecology of the ostracoda (crustacea) from verlorenvlei (western cape, south africa). South Afr. J. Zool. 31, 23–36. doi: 10.1080/02541858.1996.11448392
McCulloch, G. M., Irvine, K., Eckardt, F. D., and Bryant, R. (2008). Hydrochemical fluctuations and crustacean community composition in an ephemeral saline lake (sua pan, makgadikgadi botswana). Hydrobiologia 596, 31–46. doi: 10.1007/s10750-007-9055-8
McFarlane, M. J., and Eckard, F. D. (2006). Lake deception: a new makgadikgadi palaeolake. Botswana Notes Records 38, 195–201.
McFarlane, M. J., and Long, C. W. (2015). Pan flor ‘barchan’ mounds, ntwetwe pan, makgadikgadi, botswana: their origin and paleoclimatic implications. Quatern. Int. 372, 108–119. doi: 10.1016/j.quaint.2014.10.008
Meisch, C. (2000). Freshwater Ostracoda of Western and Central Europe. Süßwasserfauna Von Mitteleuropa 8/3. Stuttgart: Gustav Fischer, 522.
Modie, B. N., and Le Hérrissé, A. (2009). Late paleozoic palynomorph assemblages from the karoo supergroup and their potential for biostratigraphic correlation, karoo kalahari basin, botswana. Br. Geosci. 84, 337–358. doi: 10.3140/bull.geosci.1122
Modisi, M. P., Atekwana, E. A., Kapunzu, A. B., and Mgwisanyi, T. H. (2000). Rift kinematics during the incipient stages of continental fragmentation: evidence from the nascent okavango rift, northwest botswana. Geology 28, 939–942. doi: 10.1130/0091-7613(2000)28<939:rkdtis>2.0.co;2
Moore, A. E., Cotterill, F. P. D., and Eckardt, F. D. (2012). The evolution and ages of makgadikgadi paleolakes: consilient evidence from kalahari drainage evolution. South Afr. J. Geol. 115, 385–413. doi: 10.2113/gssajg.115.3.385
Müller, G. W. (1900). Afrikanische ostracoden, gesammelt von o. neumann im jahre 1893. Zool. Jahrbücher 13, 259–268. doi: 10.5962/bhl.part.27374
Murray, A. S., and Wintle, A. G. (2000). Luminescence dating of quartz using an improved single-aliquot regenerative-dose protocol. Radiat. Measure. 32, 57–73. doi: 10.1016/s1350-4487(99)00253-x
Nash, D. J., and McLaren, S. J. (2003). Kalahari valley calcretes: their nature, origins, and environmental significance. Quat. Int. 111, 3–22. doi: 10.1016/s1040-6182(03)00011-9
Nash, D. J., Shaw, P. A., and Thomas, D. S. G. (1994). Duricrust development and valley evolution: process-landform links in the kalahari. Earth Surface Proc. Land 19, 299–317. doi: 10.1002/esp.3290190403
Park, L. E., and Cohen, A. S. (2011). Paleoecological response of ostracods to early late pleistocene lake-level changes in lake malawi, east africa. Palaeogeogr. Palaeoclimatol. Palaeoecol. 303, 71–80. doi: 10.1016/j.palaeo.2010.02.038
Podgorski, J. E., Green, A. G., Kgotlhang, L., Kinzelbach, W. K. H., Kalscheuer, T., Auken, E., et al. (2013). Paleomegalake and paleo-megafan in southern africa. Geol. 41, 1155–1158. doi: 10.1130/g34735.1
Prescott, J. R., and Hutton, J. T. (1994). Cosmic ray contributions to dose-rates for luminescence and ESR dating: large depths and long terms time variations. Radiat. Meas. 23, 497–500. doi: 10.1016/1350-4487(94)90086-8
Richards, J., Burrough, S., Wiggs, G., Hills, T., Thomas, D., and Moseki, M. (2021). Uneven surface moisture as a driver of dune formation on ephemeral lake beds under conditions similar to the present day: a model-based assessment from the makgadikgadi basin, northern botswana. Earth Surface Proc. Land. 46, 1–18. doi: 10.1002/esp.5215
Riedel, F., Erhardt, S., Chauke, C., Kossler, A., Shemang, E., and Tarasov, R. (2012). Evidence for a permanent lake in sua pan (kalahari, botswana) during the early centuries of the last millennium indicated by distribution of baobab trees (adansonia digitata) on “kubu island”. Quat. Int. 253, 67–73. doi: 10.1016/j.quaint.2011.02.040
Riedel, F., Henderson, A. C. G., Heußner, K. U., Kaufmann, G., Kossler, A., Leipe, C., et al. (2014). Dynamics of a kalahari long-lived mega-lake system—hydromorphological and limnological changes in the makgadikgadi basin (botswana) during the terminal 50 ka. Hydrobiologia 739, 25–53. doi: 10.1007/s10750-013-1647-x
Ringrose, S., Harris, C., Huntsman-Mapila, P., Vink, B. W., Diskins, S., Vanderpost, C., et al. (2009). Origins of strandline duricrusts around the makgadikgadi pans (botswana kalahari) as deduced from their chemical and isotope composition. Sedim. Geol. 219, 262–279. doi: 10.1016/j.sedgeo.2009.05.021
Ringrose, S., Huntsman-Mapila, P., Kampunzu, H., Downey, W. D., Coetzee, S., Vink, B., et al. (2005). Sedimentological and geochemical evidence for palaeo-environmental change in the makgadikgadi subbasin, in relation to the MOZ rift depression, botswana. Palaeogeogr. Palaeoclimatol. Palaeoecol. 217, 265–287. doi: 10.1016/j.palaeo.2004.11.024
Sars, G. O. (1924). The freshwater entomostraca of the cape province (union of south africa). Ostracoda. Ann. South Afr. Museum 20, 105–193.
Schmidt, M., Fuchs, M., Henderson, A. C. G., Kossler, A., Leng, M. J., Mackay, A. W., et al. (2017). Paleolimnological features of a mega-lake phase in the makgadikgadi basin (kalahari, botswana) during marine isotope stage 5 inferred from diatoms. J. Paleolimnol. 58, 373–390. doi: 10.1007/s10933-017-9984-9
Shaw, P. A. (1985). Late quaternary landforms and environmental change in northwest botswana: the evidence of lake ngami and the mababe depression. Trans. Instit. Br. Geogr. 10, 333–346. doi: 10.2307/622182
Shaw, P. A., Thomas, D. S. G., and Nash, D. J. (1992). Late quaternary fluvial activity in the dry valleys (mekgacha) of the middle and southern kalahari, southern africa. J. Quat. Sci. 7, 273–281. doi: 10.1002/jqs.3390070402
Szwarc, A., Martens, K., and Namiotko, T. (2021). Two new cypridopsinae kaufmann, 1900 (crustacea, ostracoda) from southern africa. ZooKeys 1076, 83–107. doi: 10.3897/zookeys.1076.76123
Thomas, D. S. G., and Shaw, P. A. (1991). The Kalahari Environment. Cambridge: Cambridge University Press, 284.
Tyson, P. D., and Lindesay, J. A. (1992). The climate of the last 2000 years in southern africa. Holocene 2, 271–278. doi: 10.1177/095968369200200310
Wouters, K. (2017). On the modern distribution of the euryhaline species Cyprideis torosa (Jones, 1850) (Crustacea, Ostracoda). J. Micropalaeontol. 36, 21–30. doi: 10.1144/jmpaleo2015-021
Keywords: Kalahari, playa lake, evaporites, ostracods, late Quaternary
Citation: Franchi F, Cavalazzi B, Evans M, Filippidou S, Mackay R, Malaspina P, Mosekiemang G, Price A and Rossi V (2022) Late Pleistocene–Holocene Palaeoenvironmental Evolution of the Makgadikgadi Basin, Central Kalahari, Botswana: New Evidence From Shallow Sediments and Ostracod Fauna. Front. Ecol. Evol. 10:818417. doi: 10.3389/fevo.2022.818417
Received: 19 November 2021; Accepted: 10 March 2022;
Published: 13 April 2022.
Edited by:
Laura Parducci, Sapienza University of Rome, ItalyReviewed by:
Zhongping Lai, Shantou University, ChinaIlaria Mazzini, National Research Council (CNR), Italy
Copyright © 2022 Franchi, Cavalazzi, Evans, Filippidou, Mackay, Malaspina, Mosekiemang, Price and Rossi. This is an open-access article distributed under the terms of the Creative Commons Attribution License (CC BY). The use, distribution or reproduction in other forums is permitted, provided the original author(s) and the copyright owner(s) are credited and that the original publication in this journal is cited, in accordance with accepted academic practice. No use, distribution or reproduction is permitted which does not comply with these terms.
*Correspondence: Fulvio Franchi, ZnJhbmNoaWZAYml1c3QuYWMuYnc=