- 1Departamento de Ecología Evolutiva, Museo Nacional de Ciencias Naturales-Consejo Superior de Investigaciones Científicas (CSIC), Madrid, Spain
- 2Área de Biodiversidad y Conservación, Departamento Biología y Geología, Física y Química Inorgánica, Universidad Rey Juan Carlos, Móstoles, Spain
Dispersal decisions are affected by the internal state of the individual and the external environment. Immigrants entering a new population are phenotypically different from residents due to selection that mitigate costs of dispersal and facilitate settlement. Sexual and status signaling traits may influence individual’s ability to settle in a population, either by showing a subordinate phenotype thus reducing aggressive interactions, or by signaling a more competitive phenotype, thus gaining local breeding resources, including mates. By comparing immigrants vs. residents in a common kestrel population across 17 years, we evaluated the influence of dispersal on fitness components (laying date, clutch size and number of fledglings) and investigated if sex, age and phenotypic traits (body size, body condition and plumage coloration) involved in movement and social interactions affected settlement. We found that population characteristics affected sexes and age classes differently, as the admission of females and young males into our population had fewer obstacles than the admission of males. In females, immigrant young were larger, had longer wings and tails, showed better body condition, had less gray tails, started breeding earlier, and laid larger clutches than residents. Adult female immigrants also showed better body condition and less gray tails. In males, immigrants had longer tails and higher number of black spots than residents. Summarizing, immigrants are good-quality individuals and, as deduced from their breeding performance, they benefited by signaling subordination, thus reducing the probability of aggressive encounters and facilitating settlement. Our study highlights the role of phenotypic traits related to signaling to study dispersal.
Introduction
Dispersal is a key life-history trait influencing population dynamics and evolutionary processes (Clobert et al., 2012). Two dispersal movements can be distinguished: natal dispersal, in which young individuals leave their birth site and select another site to breed for their first time, and reproductive dispersal, in which adults change breeding sites between consecutive breeding seasons (Greenwood and Harvey, 1982). Across many taxa, studies aimed to understanding why animals disperse show that far from being a random behavior, a variety of factors influence the costs and benefits of dispersal at the three distinct stages of departure, transience, and settlement (Bowler and Benton, 2005; Cote et al., 2017). Thus, dispersal decisions are found to be both condition-dependent, when individuals rely on a set of external environmental cues such as habitat quality and conspecifics density (e.g., Denno and Roderick, 1992; Doligez et al., 2004; Garant et al., 2005; Matthysen, 2005), and phenotype-dependent, when dispersal depends on phenotypic attributes such as structural size (Camacho et al., 2013, 2015), body mass (Senar et al., 2002; Garant et al., 2005) sex (Trochet et al., 2016), personality (Fraser et al., 2001; Bize et al., 2017), coloration (Saino et al., 2014; Camacho et al., 2018), etc. (reviewed by Clobert et al., 2009).
Dispersers are found to differ from non-dispersers (residents hereafter) in several ecologically important attributes including physiological, morphological, behavioral and life history traits (review in Clobert et al., 2009). This dispersal phenotypes or syndromes (when multiple such traits are shaped in concert) may arise because dispersers and residents face different environmental and social contexts throughout their life, and thus divergent selection shapes their respective phenotypes favoring different traits or combinations of traits (Ronce and Clobert, 2012; Cotto et al., 2014). In general, three main explanations are assumed to underlie phenotypic differences between dispersers and residents. First, some traits are needed to increase dispersal success either by enabling dispersal (e.g., wings) or by facilitating dispersal and reducing its costs (e.g., longer wings, higher energy reserves; Clobert et al., 2009; Ronce and Clobert, 2012). Facing new environments is considered a settling cost within the dispersal process (Bonte et al., 2012), as unfamiliarity with breeding grounds impairs foraging efficiency, the defense of resources and territory acquisition, and survival (Forero et al., 2002; Bonte et al., 2012). Therefore, a second explanation is that some traits not needed for a high dispersal capacity per se benefit dispersers more than residents to optimize performance in new environments e.g., different behavioral responses to optimize performance in new environments such as exploration, locomotor activity or boldness (Fraser et al., 2001; Jokela et al., 2008; Chapman et al., 2011). When selection promotes phenotypic adaptations that mitigate the costs of dispersal, facilitate settlement and/or benefit dispersers more than residents, the fitness of immigrants and residents may be similar (Bélichon et al., 1996). Third, given that dispersal is a costly process, energetic investment in dispersal is traded-off against energetic allocation to other traits (e.g., trade-off between flight capability in insects and fecundity; Roff and Gelinas, 2003).
The idea that dispersal modifies phenotypes because it diverts resources for other functions is particularly interesting in relation to sexual and status signaling traits which are costly to produce and maintain, thus being honest signals of individual quality (Hamilton and Zuk, 1982; Hill, 1991; von Schantz et al., 1999) that are decisively involved in gaining breeding territories and mates (e.g., Greene et al., 2000) and therefore in the settlement phase of the dispersal. On this basis, dispersal might be traded-off against the expression of such traits, yet the role of dispersal in the expression of sexual and social signals or the influence of these on departure and settlement behavior are unknown.
Immigration rates are closely related with individual decisions for settlement, for which knowing environmental and social conditions favoring immigration is essential to delve into the phenomenon of dispersal. It is known that an increase of food abundance (Korpimäki, 1994; Doonan and Slade, 1995; Matthysen, 2012) or breeding sites (Berthier et al., 2012) results in higher immigration rates, probably because immigrants can assess the quality of an area based on these indicators. Yet, the relationship between breeding density and immigration rate is not so clear: whereas a positive relationship has been explained through a conspecific attraction, a large population providing good expectations for mating and/or good environmental conditions favoring settlement (Stamps, 1988; Schaub et al., 2013; Szostek et al., 2014), a negative correlation could occur if the number of territories is limited and immigrants are subordinate to residents (Wilson and Arcese, 2008).
In birds, plumage coloration has long been the focus of research on social selection, including both sexual and status signaling (Andersson, 1994; Hill, 2006; Senar, 2006). In addition, coloration is a phenotypic trait showing a boundless variation in nature, this variability being commonly associated with other phenotypic traits in a genetic, developmental or simply functional way, promoting phenotypic integration (Pigliucci and Preston, 2004). Melanic coloration has been reported to covary with morphological, behavioral, physiological or hormonal traits in different bird species (e.g., Roulin and Ducrest, 2011; Pryke et al., 2012; Kim et al., 2013; Fargallo et al., 2014). Interestingly, recent studies have reported a covariation between melanin coloration and propensity to disperse (van den Brink et al., 2012; Roulin, 2013; Saino et al., 2014; Camacho et al., 2018). In a different perspective, looking for a covariation between colored traits and dispersal predisposition in the origin population may mask other potential functions of colored traits. Quality and status signaling might have an essential role in entering the populations where dispersers settle and might explain dispersal patterns. For this reason, knowing how admittance of foreign individuals in settlement populations works is essential to have a more complete view of dispersal. In this context, the expression of sexual and social status traits may influence individual’s ability of immigrants to enter a population, either by showing a subordinate phenotype thus reducing aggressive interactions, or by signaling a more competitive phenotype, thus gaining local breeding resources, including mates. The former would be particularly relevant for sexually dichromatic species in which young males show delayed plumage maturation, as one of the functions proposed to explain this trait is to signal subordination to more dominant and experienced individuals, which would favor settlement (Lyon and Montgomerie, 1986; Vergara and Fargallo, 2007; Hawkins et al., 2012).
The aim of this study is to investigate whether individual phenotypes and population conditions affected dispersal decisions in the common kestrel Falco tinnunculus, a partially migratory and sexually dichromatic species in which sexually mature young males show delayed plumage maturation and colored plumage traits have been reported to signal quality, playing an important role in social contexts (e.g., Palokangas et al., 1994; Fargallo et al., 2007; Vergara and Fargallo, 2007; Vergara et al., 2009; Vergara and Fargallo, 2008; Zampiga et al., 2008).
By comparing foreign immigrants (dispersed individuals entering the population for the first time) vs. residents in 17 breeding seasons (from 2004 to 2020), we analyzed the influence of dispersal at the settlement phase on fitness components (laying date, clutch size, and number of fledglings) and evaluated if the individual phenotype involved in movement capacity and social interactions (sex, age, body size, feather length, body condition, and plumage coloration) and population characteristics (mean clutch size, density and growth) had an effect on settlement. We predict that good quality immigrants showing a subordinate phenotype may reduce aggressive interactions with conspecifics and thus successfully settle in the population. In addition, male common kestrels are more territorial and philopatric than females (Village, 1990; Vasko et al., 2011; Terraube et al., 2015), making the competition for breeding territories stronger in males than in females, for which we expect more females entering the population than males. Since mean clutch size in our population is considered a proxy of environmental food conditions (Vergara et al., 2009) we predict immigration rate to be positively correlated with mean clutch size. We do not have a clear prediction for the relationship between immigration and density.
Materials and Methods
Study Species and Population
The common kestrel (kestrel hereafter) is a medium-sized raptor with a marked sexual dimorphism in body weight, females being 17% heavier as 1-year old or second calendar year (young hereafter) and 20% as adults. Sexual dimorphism in size is moderate (Supplementary Table 1) with females being less than 5% larger than males in both young and adults. Plumage coloration in kestrels is melanin based (Fargallo et al., 2007) and exhibit a marked sexual dichromatism (Palokangas et al., 1994). Adult females are brown on the head, back and upper side of wings, with black bars and showing a brown-gray color variation in the rump, upper tail-coverts and tail, but always black-barred. Adult males are brownish-red, with black spots on the back and on the upper side of wings. Head, rump, upper tail-coverts and tail are gray and unbarred (Vergara et al., 2009; Vergara and Fargallo, 2011). One-year-old females can also have black-barred gray feathers. Young males are, in general, like adult females, with a proportion of them showing variable amounts of black-barred gray coloration on the rump, upper tail-coverts and tail (Vergara and Fargallo, 2007). There is a great variation in the proportion of molted body feathers among young males, as some birds have changed all of them before they reach a complete year of life, whereas others have replaced very few (Vergara and Fargallo, 2007), showing differential maturation of sexual traits as well (Vergara et al., 2012). Plumage coloration of males and females has been found to correlate with fitness components, such as laying date and fecundity (Palokangas et al., 1994; Vergara et al., 2009; Vergara and Fargallo, 2011), with individual quality in terms of parasite load (Korpimäki et al., 1996), body condition (Fargallo et al., 2007; Vergara and Fargallo, 2011), hunting skills (Palokangas et al., 1994) and competitive capacity (Vergara and Fargallo, 2008) and with female mating preferences (Palokangas et al., 1992; Zampiga et al., 2008). The common kestrel is a monogamous species showing a low rate of polygamy (Costantini and Dell’Omo, 2020). In our kestrel population, an average of 0.9 ± 1.1 males were polygynous each year, representing 3.9 ± 4.0% (n = 398 pairings of 256 males in 18 years). Dispersal in the common kestrel has only been analyzed in one population from Finland (Vasko et al., 2011; Terraube et al., 2015). These studies found that female, yearling and good body condition individuals dispersed longer distances than male, older and poor body condition individuals, body condition particularly affecting females (Vasko et al., 2011; Terraube et al., 2015). Also dispersal distances were longer when food conditions were lower in the spring of settlement (Vasko et al., 2011). In addition, the kestrel is considered almost migrant species in northern, partially migrant in central and sedentary in southern Europe (Village, 1990; Costantini and Dell’Omo, 2020). In our population, and based on ring recoveries and PVC ring resighting, we know that some individuals migrate to Africa to spend the winter while other individuals winter in our study area. Mate retention, i.e., breeding with the same partner the following year, is low in this species (Costantini and Dell’Omo, 2020).
Study Area and Procedures
The study was carried out in the region of Campo Azálvaro, located in central Spain, where about 10–45 breeding common kestrel pairs nest each year in an area of 23 km2. The area is a flat treeless valley at 1,300 m a.s.l., mainly devoted to cattle-raising (Fargallo et al., 2001). The kestrel population has been monitored since 1993 (Fargallo et al., 2001). In 1994 we began to install kestrel nestboxes in the area, adding the last 15 in 2005 to reach 62 boxes in total (Fargallo et al., 2009). Although kestrels have historically used nesting sites other than nestboxes in our study area (Fargallo et al., 2001), this fraction has been gradually decreasing as nestboxes have been installed. In the core 23 km2 region, from 2004 to date, only 13 breeding attempts have been observed in six different nest sites that were not nestboxes, the last being in 2013. In all of them, all breeding females and some males could be captured.
All fledglings born in the study area have been marked since 1994 with metal rings to identify them in subsequent breeding attempts. For this study, we used data collected between 2004 and 2020. During this period there have always been unoccupied nestboxes available for kestrel nesting, ranging from 11 (22%) in 2004 to 51 (84%) in 2018. All nests were checked periodically to record laying date (date of the first laid egg), clutch size and number of fledglings (number of live chicks in the nest 26 days after hatching). To know the identity of breeding adults, reproductive individuals were captured at the age of 10–12 days of the chicks. At capture, metallic ring was recorded, and if there had no ring, we put one. Data on reproductive kestrels included dispersal status (immigrants or residents, see below for details), body mass, body measurements (tarsus, wing and tail length), body condition (weight corrected for size), age (young or adult, see below for details), and plumage coloration (see below for details). In addition, to determine the impact of dispersal on fitness, data on the onset of reproduction (laying date), fecundity (clutch size), and productivity (number of fledglings) have been used.
Dispersal Status and Age
For each year kestrels were classified as “residents” if they were found ringed, or as “immigrants” if they were not. Immigrants were defined as kestrels that were born outside of the study area and dispersed into our population. Immigrants who reproduced again in the population in the subsequent years after their first entrance, were considered as residents the following years. This is so because variation in condition and coloration is greatly influenced by local environmental conditions (Fargallo et al., 2007; Vergara et al., 2009) and because the interest of the study is to compare the phenotypes of the individuals that enter in a given year with those of the individuals already settled in the population in that year. So, resident kestrels include individuals born in the population and ringed as chicks as well as individuals that have dispersed from their origin population but reproduce habitually in our study population. This means that an individual can have a dispersal status for one year and a resident status in subsequent years. The age of the immigrant individuals could be classified as “young” (1-year old) or “adult” (>1-year old) based on plumage characteristics (e.g., Village, 1990).
To examine whether the entry of individuals in the population was associated with annual environmental conditions and population size, we calculated the proportion of dispersed and resident in each age class (young and adults) and sex (males and females) for each breeding season. We used the mean clutch size of the population as a proxy of environmental conditions, mainly food conditions (Vergara et al., 2009). Population density was calculated as the number of breeding pairs in 23 km2.
In our population female modal age is young representing 30% of the individuals, young females + 2-year old females make up the 52% of the population (Supplementary Figure 1). In males, modal age is 2-year old, representing 39% of the population, being young males + 2-year old males the 55% of the population (Supplementary Figure 2).
Plumage Coloration
From 2004 to 2020 field estimations and digital photographs were taken from the back side of the captured individuals to measure color traits as described in Fargallo et al. (2007) and López-Rull et al. (2016). Photographs were taken at an angle of 90° between the objective of the camera and the surface of the body using a Nikon D70 camera (objective: 18–70 mm AF-S Nikkon DX). All photographs were taken under a sunshade in the morning. Images were imported into Photoshop CS5, where color trait variables were measured. A metric scale was set for all pictures prior to measurements by equalizing pixel distance to measurement units (cm). All measurements were performed at real scale.
In the case of females, six color trait variables were measured: rump grayness (RG), tail grayness (TG), width of black terminal tail bands (TTB), width of black tail bars (TB), width of black rump bands (RB) and wing blackness (WB). These traits have been shown to be implicated in the probability of recruitment (López-Rull et al., 2016) and personality (López-Idiáquez et al., 2019) in chicks and to reflect individual quality and environmental conditions in breeding females (Vergara et al., 2009) and chicks (Fargallo et al., 2007). We estimated rump grayness as the percentage of gray with respect to brown covering the whole rump and tail (0% = completely black-barred brown and 100% = completely black-barred gray) by direct observation in the field. Field measurements of rump and tail coloration are highly repeatable and closely correlated with those taken from digital images (Fargallo et al., 2007; Vergara et al., 2009). TTB, TB, RB, and WB were measured from the photographs. To compute band widths the “ruler tool” was used. Measurements of the TTB were performed in the two central tail feathers, whereas TB and RB were measured in four different feathers on the left side of the body. Band widths were calculated as the mean value of these feather measurements. To estimate WB, the proportion of black coloration was calculated in the covert feathers of a known area of the wing. Black spots and bars are easily distinguishable from the brown background of the wing plumage, and thus, they were outlined and their area determined as the number of pixels occupied using the “magic wand tool.” All these color trait measurements have been proved repeatable (López-Rull et al., 2016). In the case of males, three color trait variables were measured: width of black terminal tail bands and the number and size of black spots in the back of males. The width of black terminal tail bands was measured directly in the field with a digital micrometer. The size and number of black spots was estimated from the photographs. Spot size was measured as the mean size value of five randomly selected spots using the “loop” tool as described in López-Idiáquez et al. (2018). These color trait measurements have been proved repeatable (terminal tail band: Vergara et al., 2009; number and size of spots: López-Idiáquez et al., 2018).
In the case of females, the six color variables were first combined in a principal components analysis (PCA) in order to reduce the number of variables and statistical tests. The PCA that resulted in two main axes showing eigenvalues >1 (Supplementary Table 1). The first axis (PC1) explained 32% of the variance and represented a gradient from high to low values of WB, RB and TB, that is, individuals having more blackness in the wing also showed wider bars in rump and tail. The second axis (PC2) explained 31% of the variance and described a gradient of RG, TG and TTB so that females showing grayer rumps and tails also have wider terminal tail bands. In the case of males, combining the three color traits of males in a PCA resulted in two axes, explaining 39 and 38% of the variance, respectively, showing no clear reduction in the number of variables. For this reason, in the case of males, we worked with the three variables separately.
Statistical Procedures
Generalized linear mixed models (GLMMs; binomial distribution and logit link function) where used to analyze the relationship between the proportion of immigrant individuals and population variables (mean clutch size and breeding density). An events/trials double column was used as the dependent variable including the number of immigrants (events) and the number of individuals in the population (trials). Mean clutch size and breeding density were included in the models as independent variables, age and sex as fixed factors and year as a random factor. Interactions between the different terms are also analyzed. We fitted the effect of variables to the observed data following backward stepwise procedure, testing the significance of all the variables and interactions and removing the one with the highest p in each step. The final model is the one that contains the variables that contribute significantly. Non-significant variables remain in the model if they participate in a significant interaction. Phenotypic variables were analyzed using general linear mixed models (LMMs) for females and males separately. In the models, male and female morphology, body mass, coloration and reproduction variables were considered dependent variables, dispersal status (immigrant vs. resident) and age (young vs. adult) were included in the models as fixed factors, tarsus, wing and tail lengths were included as covariates when required and individual identity and year were included as random factors to avoid pseudoreplication. Body condition was determined as body mass corrected in the models for tarsus length as a covariate. Proportions as dependent variables were logit transformed (log (y/[1 - y]). Since proportions equal to 0 and 1 transform to undefined values -∞ and +∞, respectively, for which 0 values were replaced by 0.01 and 1 values by 0.99 (Warton and Hui, 2011). All models were checked for normality of residuals. All analyses were performed in SAS 9.4 statistical software (SAS Institute Inc., Cary, NC, United States).
Results
Proportion of Immigrants
The mean percentage of immigrants in our population was 34.9% (range = 26.1–56.1%). The mean percentage of immigrant females was 21.1 ± 6.4% (range = 11.8–33.9%) of which 42.0% were young (range = 0.0–73.7%). Regarding males, the mean percentage of immigrants was 13.8 ± 5.2% (range = 7.9–25.0%) of which 21.3% were young (range = 0.0–66.7%). The differences observed in the proportion of immigrants between sexes were statistically significant (Table 1 and Figure 1), although they were mainly due to the higher proportion of females with respect to males in the young age class (GLMM, post-hoc comparisons t = -4.13, P < 0.001), which was not significant in adults (GLMM, post-hoc comparisons t = -1.16, P = 0.25; Figure 1). The interaction being significant (Table 1). The proportion of immigrants was positively correlated with the mean clutch size of the population, but not with breeding density (Table 1). The interaction between age and breeding density was significant (Table 1), showing that when breeding density increases there tends to be more young immigrants and fewer adult immigrants. However, neither trend was significant when analyzed separately (GLMM, both P > 0.07).
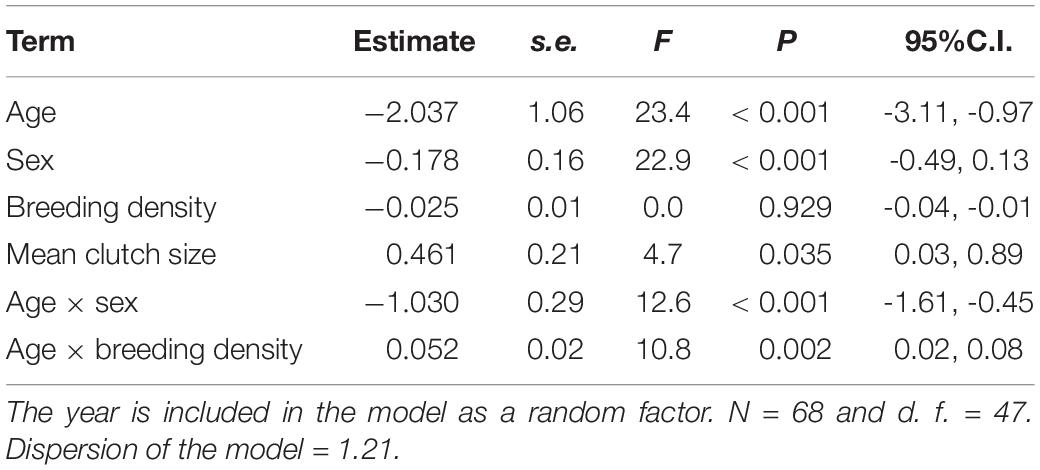
Table 1. Results of GLMMs for the proportion of immigrant common kestrels as a function of age (young vs. adults), breeding density, mean clutch size of the population, the interaction between age and sex and between age and breeding density.
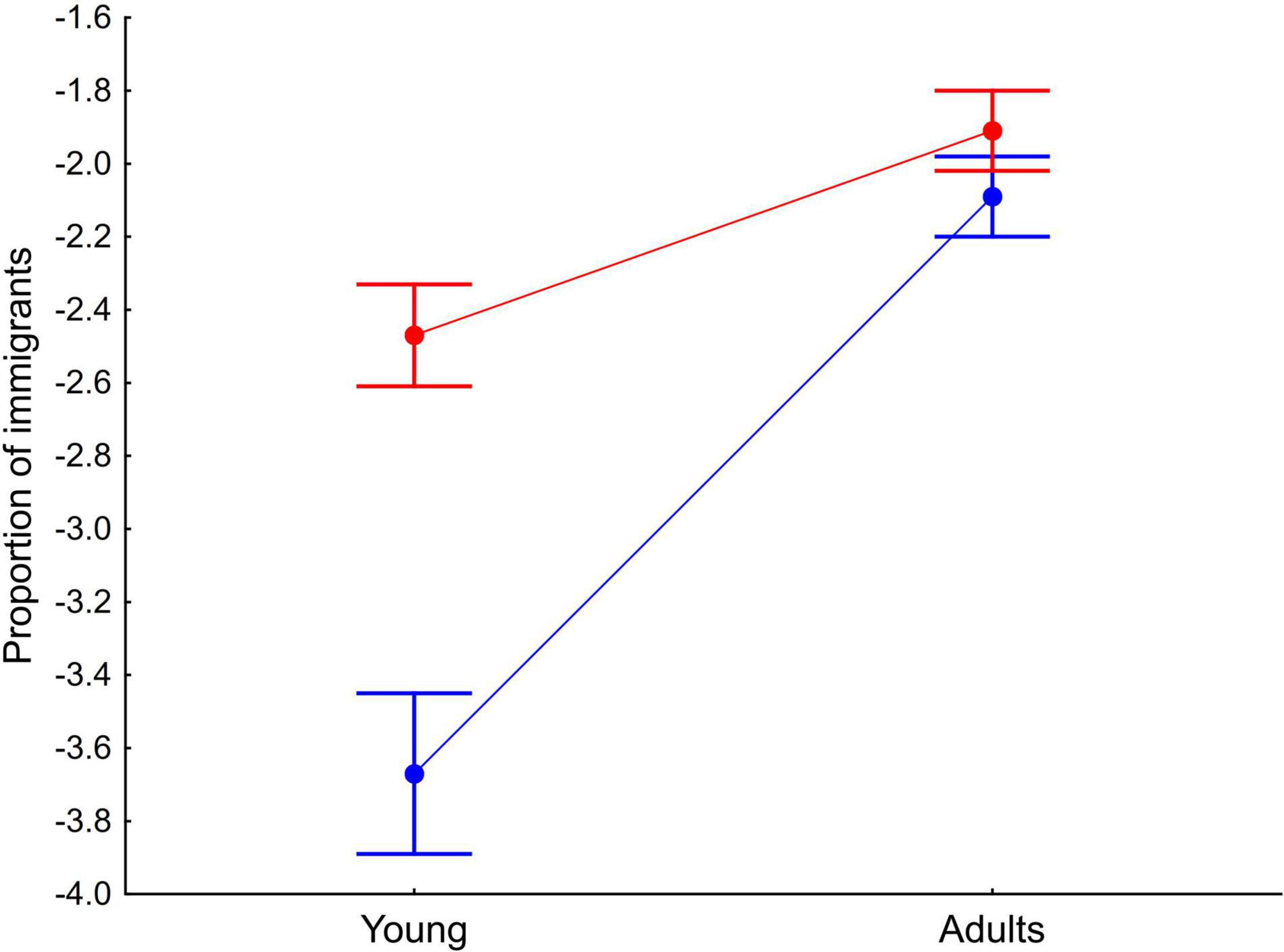
Figure 1. Interaction between sex and age in the proportion of common kestrel immigrants (residuals from the GLMM). Red and blue colors represent females and males, respectively. Dots and whiskers represent mean and s.e. values, respectively.
Female Dispersal Phenotypes
Tarsus length of female immigrants entering the population were similar to that of residents (LMM, P = 0.233). Controlling for tarsus length, differences in wing and tail lengths between immigrant and resident females were not significant (Table 2). Adults showed longer wings and tails than young (Table 2) and in both models the interactions between dispersal status and age were statistically significant (Table 2 and Figure 2). Post-hoc comparisons showed that young immigrants entering the population, but not adult immigrants, had longer wings and tails (Figure 2). Regarding body condition, immigrant females showed higher body condition than resident ones (Table 3 and Figure 3).
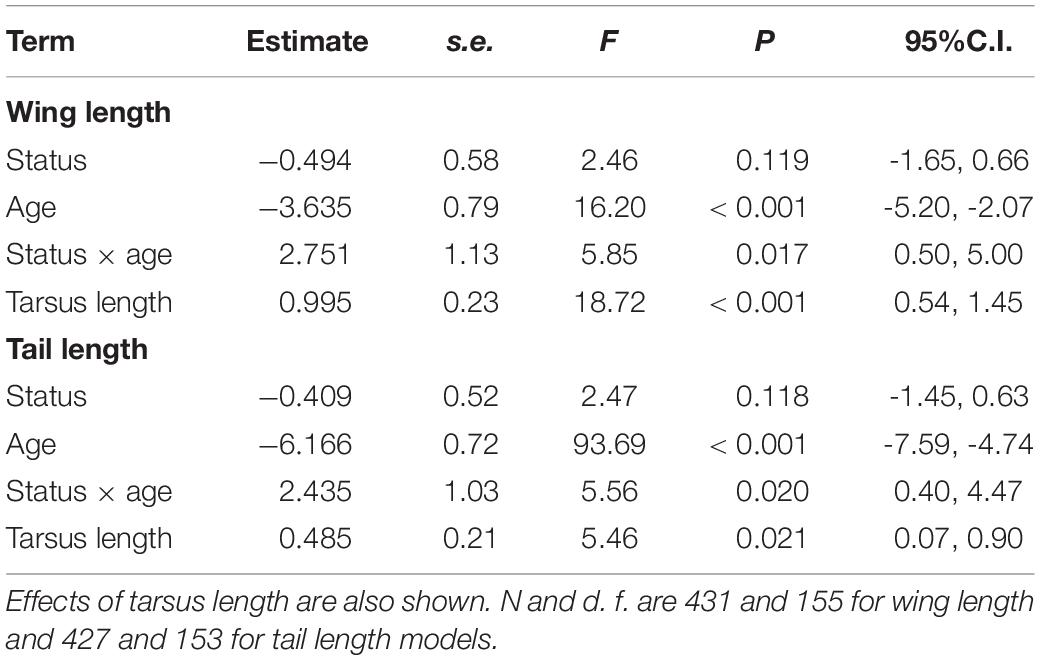
Table 2. Results of LMMs for wing and tail lengths of common kestrel females as a function of dispersal status (immigrant vs. resident), age (young vs. adult), and the interaction between dispersal status and age.
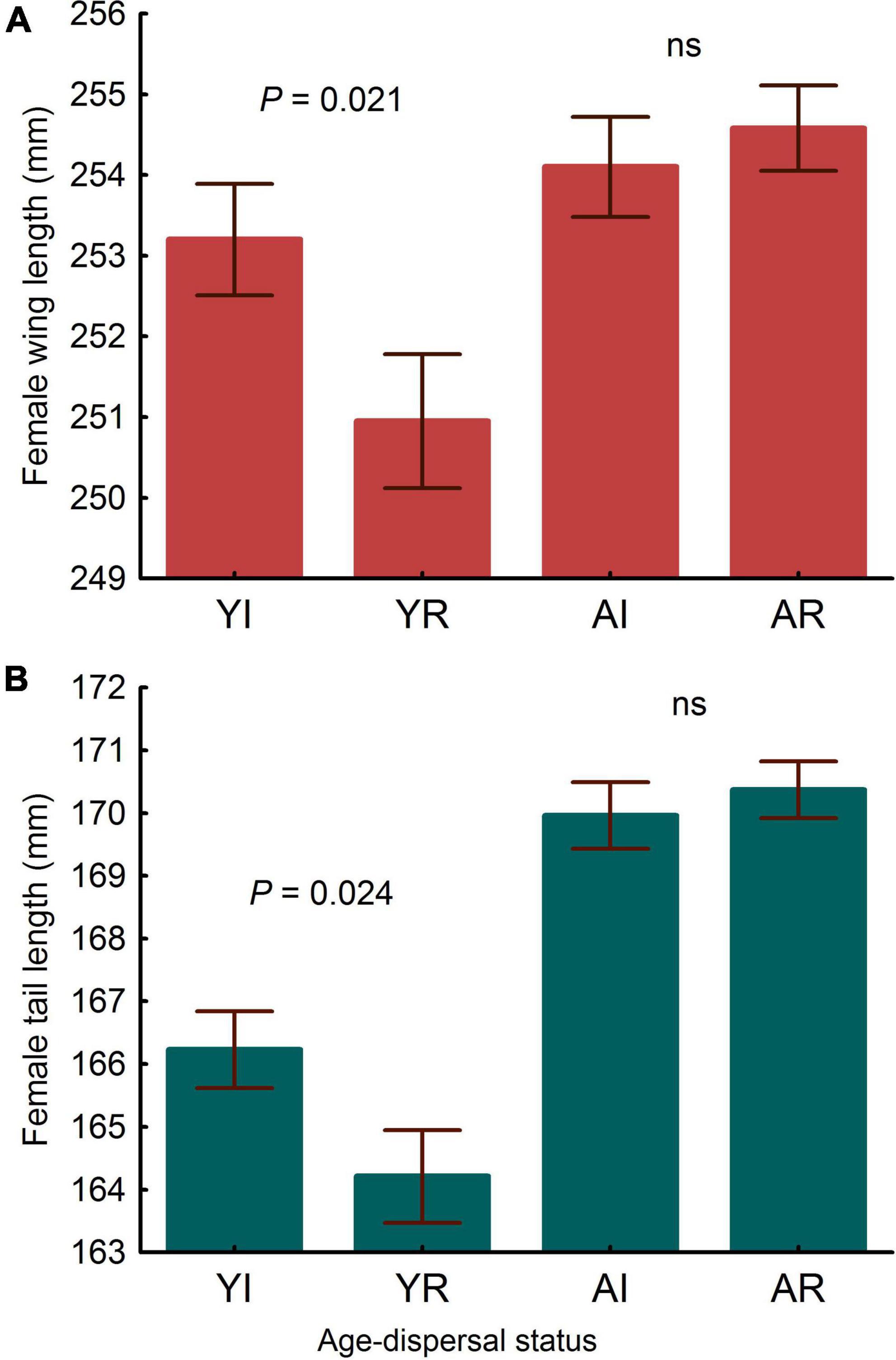
Figure 2. Differences in wing (A) and tail (B) lengths corrected by body size (tarsus length) in breeding females. YI is young immigrant, YR is young resident, AI is adult immigrant and AR is adult resident. P-values correspond to post-hoc comparisons between groups from the interaction dispersal status x age in the LMMs. Values to the left and to the right correspond to differences in young and adults, respectively. “ns” means not significant differences (both P > 0.40). LMMs: wing length in young, estimate ± s.e. = 2.257 ± 0.97, t = 2.33; tail length in young, estimate ± s.e. = 2.026 ± 0.88, t = 2.29. Bars and whiskers represent mean and s.e. values, respectively.
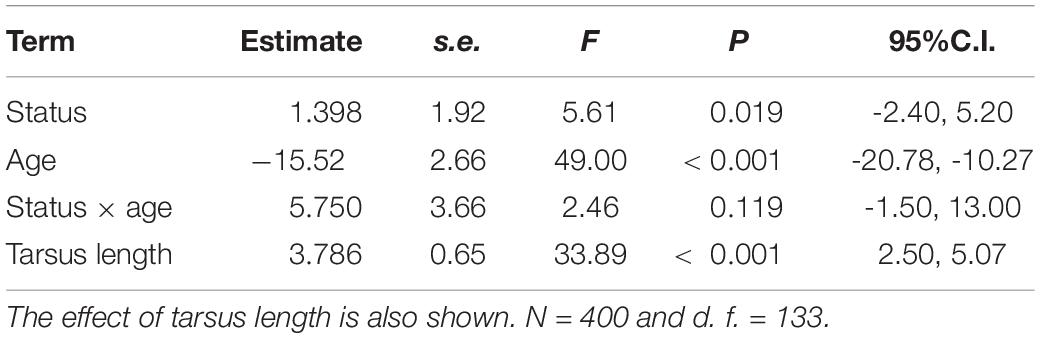
Table 3. Results of LMMs for body condition (body mass corrected by tarsus length) in breeding common kestrel females as a function of dispersal status (immigrant vs. resident), age (young vs. adult) and the interaction between dispersal status and age.
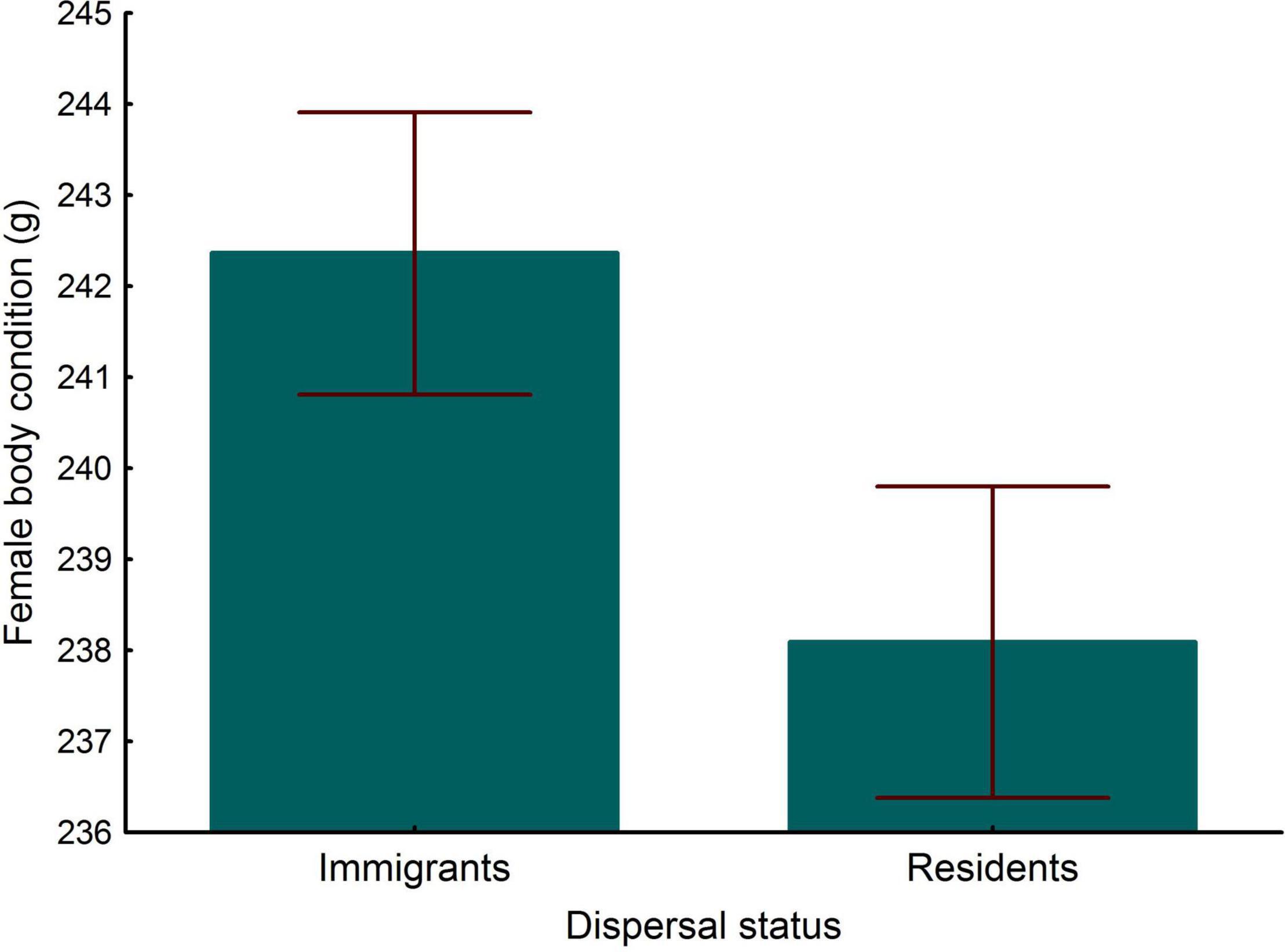
Figure 3. Differences in body condition (body mass corrected by tarsus length) between immigrant and resident breeding females. Bars and whiskers represent mean and s.e. values, respectively.
In relation to plumage coloration, PC1 (combining wing blackness, width of black rump bands, and width of tail bands) did not differ between immigrant and resident females, young and adult females and not significant interaction between both variables was found (LMM, all P > 0.43, n = 392). Removing the interaction term (LMM, P = 0.530) and controlling for age (LMM, P < 0.001), PC2 (combining tail grayness, rump grayness and width of tail terminal bands) showed marginally significant differences between immigrant and resident females [LMM, estimate ± s.e. = -1.120 ± 0.07, F(1, 139) = 3.43, P = 0.066]. To better understand this potential difference, we analyzed the traits separately. Rump grayness and the width of the terminal tail band (corrected for tail length as a covariate in the model) did not show significant differences between immigrant and resident females (LMM, both P > 0.22). However, controlling for age, immigrant females showed less gray tails (browner tails) than resident ones [LMM, estimate ± s.e. = -0.486 ± 0.20, F(1, 139) = 6.12, P = 0.015, n = 392, Figure 4], the interaction between age and dispersal status being not significant (LMM, P = 0.248).
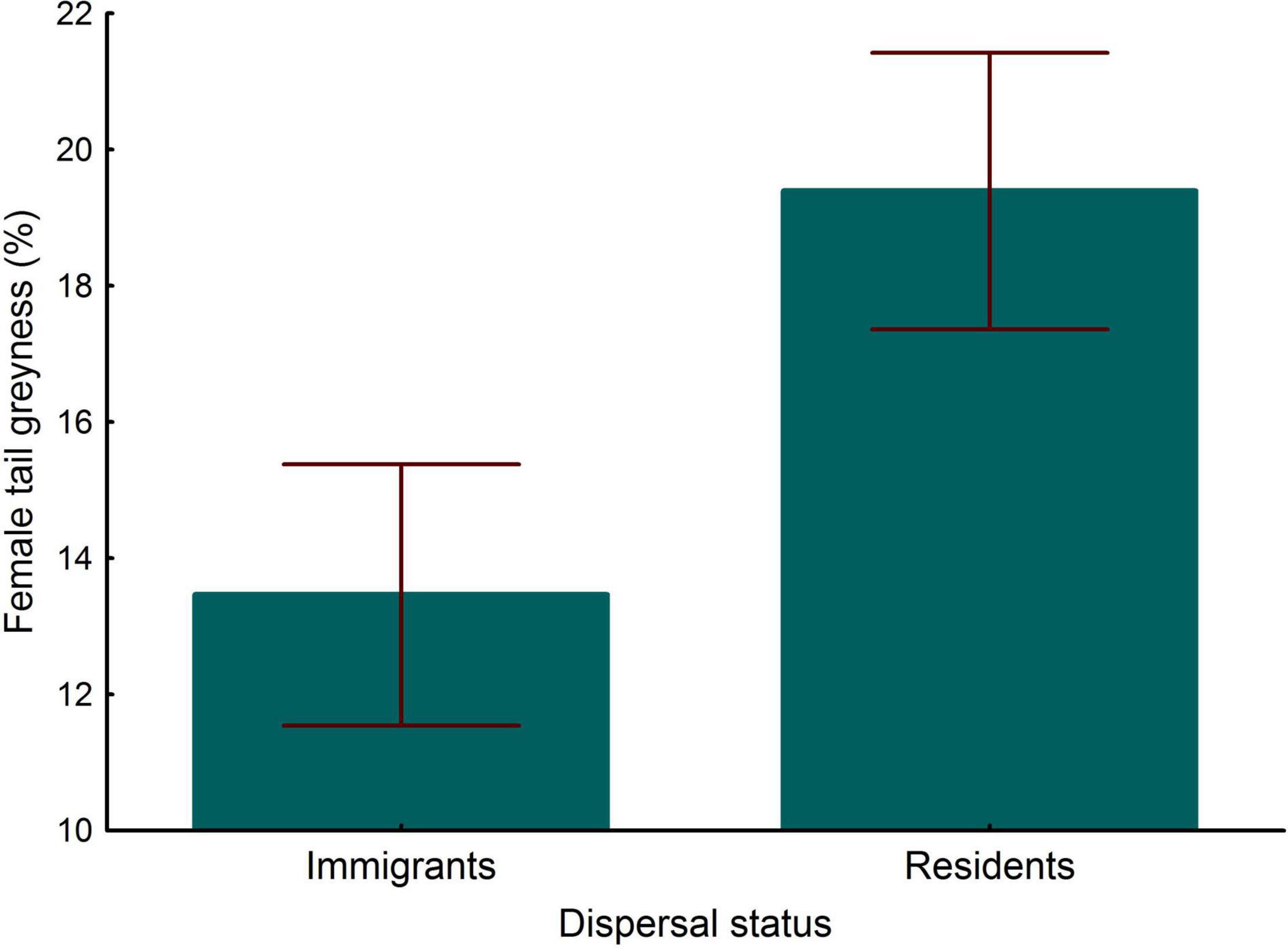
Figure 4. Differences in tail grayness between immigrant and resident breeding females. Bars and whiskers represent mean and s.e. values, respectively.
Female Reproduction
Adult females started laying earlier, laid more eggs, and produced more chicks than young ones (Table 3 and Figure 5). Regarding dispersal status, immigrant females also started laying earlier than resident ones (Table 4). This was mainly due to the difference found in young, but not in adults, as resulted from the significant interaction between dispersal status and age (Table 4 and Figure 5). No differences were found between immigrant and resident females in clutch size or number of fledglings (Table 4), though the interaction between status and age was significant for clutch size and marginally significant for the number of fledglings (Table 4). In the case of clutch size, post-hoc comparisons showed that differences were observed in young females, immigrant individuals having larger clutch sizes than resident ones (Figure 5). With regard to the number of fledglings, marginally significant differences were observed between adults, resident females tending to produce a higher number of fledglings than immigrant ones (Figure 5), being adult residents the most productive female group (Figure 5).
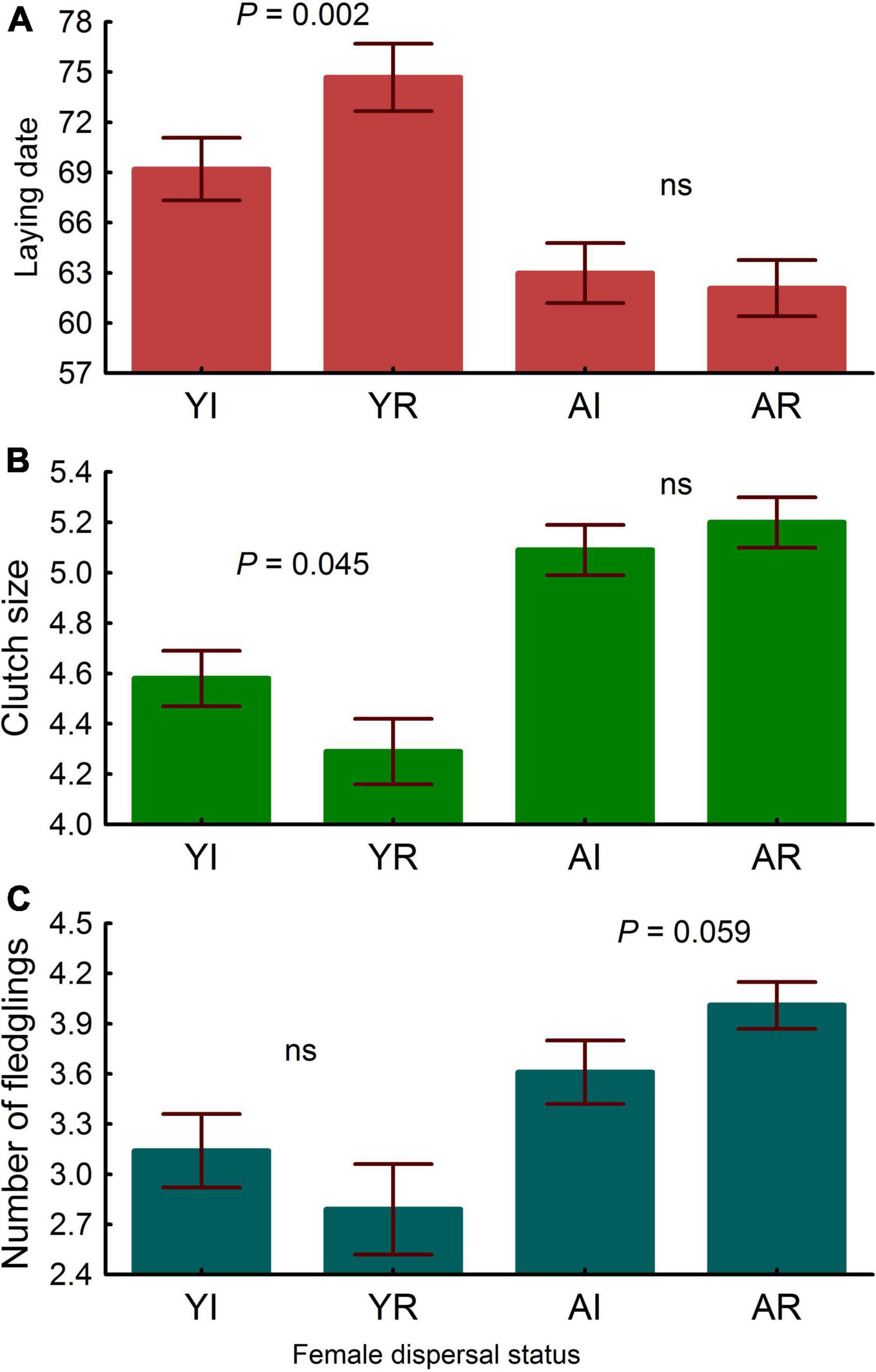
Figure 5. Differences in laying date (A), clutch size (B) and number of fledglings produced (C) in breeding common kestrel females. YI is young immigrant, YR is young resident, AI is adult immigrant and AR is adult resident. P-values correspond to post-hoc comparisons between groups from the interaction dispersal status × age in the LMMs. Values to the left and to the right correspond to differences in young and adults, respectively. “ns” means not significant differences (all P > 0.22). LMMs: laying date young females, estimate ± s.e. = -5.475 ± 1.69, t = 3.24; clutch size young females, estimate ± s.e. = 0.291 ± 0.14, t = 2.02; number of fledglings adult females, estimate ± s.e. = -0.407 ± 0.21, t = 1.90. Bars and whiskers represent mean and s.e. values, respectively.
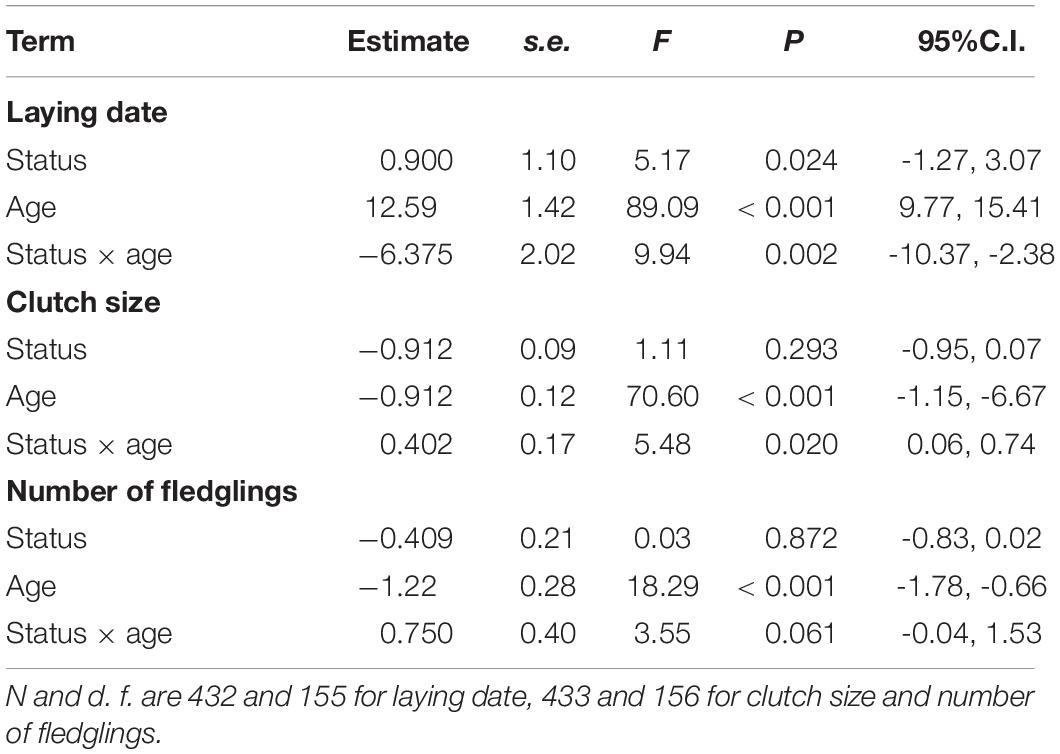
Table 4. Results of LMMs for laying date, clutch size and number of fledglings produced by common kestrel females functions of dispersal status (immigrant vs. resident), age (young vs. adult) and the interaction between dispersal status and age.
Female Tail and Reproduction
Controlling for age and dispersal status, female tail grayness was not correlated with tail length (LMM, P = 0.485). The interaction between dispersal status and age was not significant (LMM, P = 0.229). Since tail length was correlated with tarsus length, we used the residuals of the regression between tail and tarsus lengths to control for body size. Controlling for age and dispersal status, laying date was correlated with female tail length [LMM, estimate ± s.e. = -0.164 ± 0.08, F(1, 152) = 4.73, P = 0.031, n = 428; Figure 6]. The interactions between tail length and dispersal status or age were not significant (LMM, both P > 0.22). Controlling for age and dispersal status, the correlation between clutch size and tail length was positively and marginally significant (LMM, P = 0.062). The interactions between tail length and dispersal status or age were not significant (LMM, both P > 0.28). Including laying date in the model, no trend was found (LMM, P = 0.19). Regarding the number of fledglings and controlling for age and dispersal status, a significant effect of tail length was observed (LMM, P = 0.003) and the interactions between tail length and dispersal status or age were not significant (LMM, both P > 0.30). Including laying date in the model, the positive correlation between the number of fledglings and tail length still remained significant [LMM, estimate ± s.e. = 0.037 ± 0.01, F(1, 153) = 8.04, P = 0.005, n = 426; Figure 6].
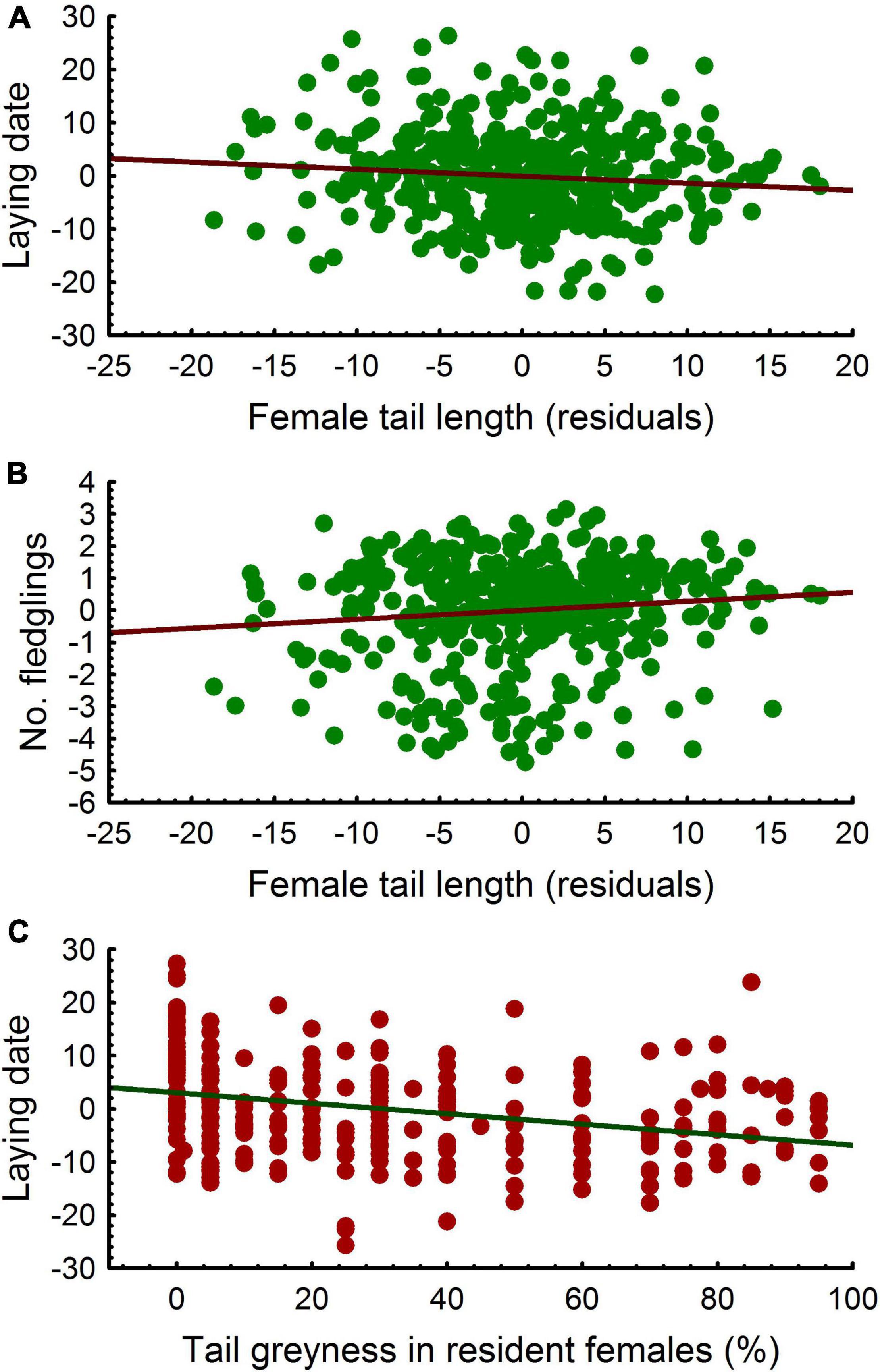
Figure 6. Linear relationship between laying date (residuals) and tail length (A), number of fledglings and tail length (B) and laying date (residuals) and tail grayness (C) in female common kestrels.
Controlling for age and dispersal status, tail grayness of females did not affect significantly laying date (LMM, P = 0.711), but the interaction between tail grayness and dispersal status was significant [LMM, estimate ± s.e. = 1.165 ± 0.25, F(1, 154) = 16.44, P < 0.001, n = 432]. Laying date was not correlated with tail grayness in immigrant females (LMM, P = 0.383, n = 165), whereas a negative correlation was found for resident females [LMM, estimate ± s.e. = -0.559 ± 0.28, F(1, 100) = 3.96, P = 0.049, n = 267; Figure 6]. The interaction between dispersal status and age was not significant (LMM, P = 0.874). Regarding clutch size and controlling for age, a similar significant interaction was found between dispersal status and tail grayness (LMM, P = 0.013), although in this case, no clear significant correlations were observed between clutch size and tail grayness neither in immigrants nor in residents (LMM, both P > 0.07). In the case of the number of fledglings and controlling for age, no significant effects were found for dispersal status, tail grayness and interactions (LMM, all P > 0.12).
Male Dispersal Phenotypes
Controlling for age, immigrant males entering the population were similar in tarsus length to resident ones (LMM, P = 0.59), the interactions between dispersal status and age being also not significant (LMM, P = 0.33). Controlling for body size (tarsus length), no differences were found in wing length and the interaction between dispersal status and age interaction as not significant (LMM, P > 0.90). Controlling for body size, the difference in tail length between immigrant and resident males was significant (Table 5 and Figure 7) and the interaction between dispersal status and age was marginally significant (Table 5). Adults showed higher body condition than young individuals [LMM, estimate ± s.e. = -6.085 ± 2.07, F(1, 102) = 16.20, P < 0.001, n = 348], but no differences were found between immigrant and resident males and the interaction between dispersal status and age was not significant (LMM, both P > 0.57).
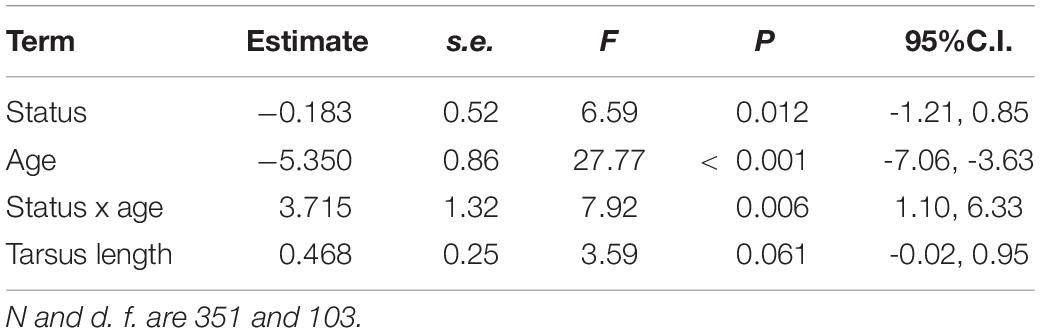
Table 5. Results of LMMs for tail length of common kestrel males as a function of dispersal status (immigrant vs. resident), age (young vs. adult), and the interaction between dispersal status and age.
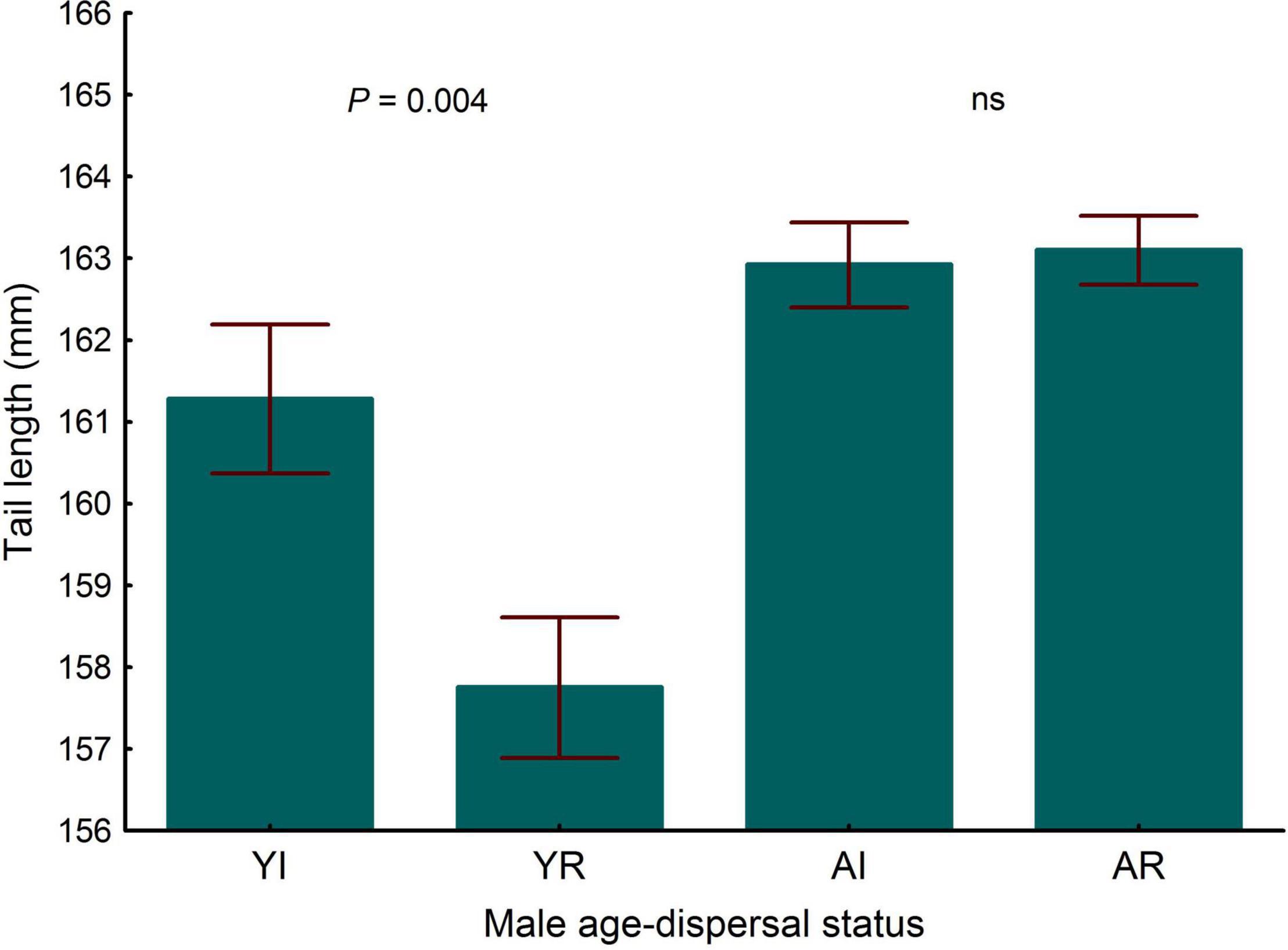
Figure 7. Differences in tail length of breeding common kestrel males. YI is young immigrant, YR is young resident, AI is adult immigrant and AR is adult resident. P-values correspond to post-hoc comparisons between groups from the interaction between dispersal status and age in the LMMs. Values to the left and to the right correspond to differences in young and adults, respectively. “ns” means not significant differences (P > 0.05). LMMs: young, estimate ± s.e. = 3.532 ± 1.21, t = 2.93. Bars and whiskers represent mean and s.e. values, respectively.
In relation to color traits, controlling for age, the size of the spots correlated with wing length [LMM, estimate ± s.e. = 0.098 ± 0.03, F(1, 93) = 8.94, P = 0.005, n = 338] and tarsus length [LMM, estimate ± s.e. = 0.309 ± 0.15, F(1, 93) = 4.02, P = 0.048, n = 338]. Since wing length fitted the linear model better than tarsus length (lower s.e.), we used the former as a covariate in the models to control for male size. Also, controlling for age, the number of spots was not correlated with any measurement of body size (LMM, both P > 0.21). The width of the terminal tail band was positively correlated with tail length [LMM, estimate ± s.e. = 0.092 ± 0.03, F(1, 104) = 11.79, P < 0.001, n = 344], for which this variable was used as covariate in the models to control for body size.
Controlling for wing length, spots were larger in young than in adult males [LMM, estimate ± s.e. = 1.079 ± 0.75, F(1, 91) = 5.07, P = 0.027, n = 338], but not significant effects were found for dispersal status or the interaction between dispersal status and age (LMM, both P > 0.74). In regards to the number of spots, adult males had significantly fewer spots than young individuals (Table 6 and Figure 8) and residents tended, but not significantly so, to have fewer spots than immigrants (Table 6). This trend was due to the lower number of spots in the resident adults than in the immigrant adults, a difference that was not observed in young individuals (Figure 8), as the significant interaction between dispersal status and age shows (Table 6). Controlling for tail length, adults exhibited wider terminal tail bands than young [LMM, estimate ± s.e. = -8.691 ± 0.45, F(1, 102) = 605.28, P < 0.001], but a not significant difference was found between residents and immigrants and a not significant interaction between dispersal status and age was observed (LMM, P > 0.40).
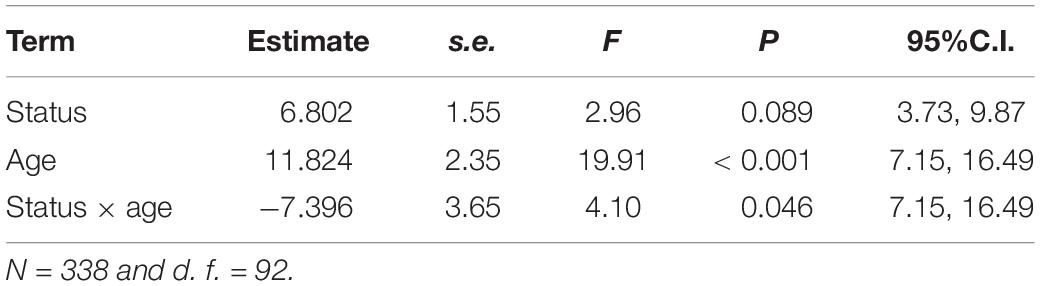
Table 6. Results of LMMs for number of spots of common kestrel males as a function of dispersal status (immigrant vs. resident), age (young vs. adult), and the interaction between dispersal status and age.
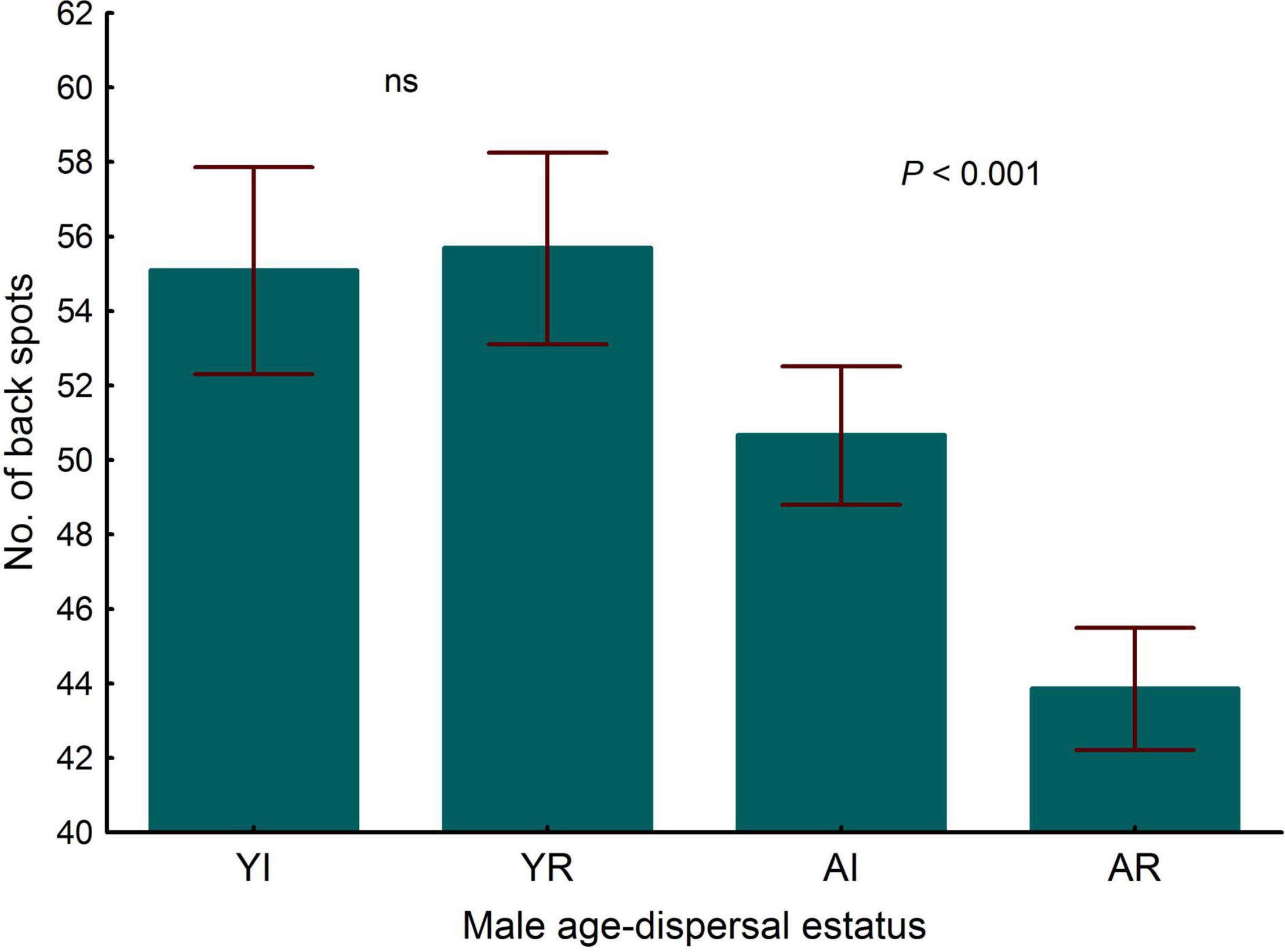
Figure 8. Differences in the number of spots in breeding common kestrel males. YI is young immigrant, YR is young resident, AI is adult immigrant and AR is adult resident. P-values correspond to post-hoc comparisons between groups from the interaction dispersal status x age in the LMMs. Values to the left and to the right correspond to differences in young and adults, respectively. “ns” means not significant differences (P > 0.05). LMMs: adult, estimate ± s.e. = 6.802 ± 1.55, t = 4.40). Bars and whiskers represent mean and s.e. values, respectively.
Male Reproduction
Adult males started breeding earlier than young males [LMM, estimate ± s.e. = 12.371 ± 1.67, F(1, 109) = 57.28, P < 0.001, n = 358]. A marginally significant difference in laying date was observed between immigrant and resident males [LMM, estimate ± s.e. = 0.056 ± 1.12, F(1, 109) = 3.03, P = 0.089] and similarly, a marginally significant interaction was observed between dispersal status and age [LMM, estimate ± s.e. = -4.670 ± 2.65, F(1, 109) = 3.10, P = 0.081]. Post-hoc comparisons showed that no significant difference was observed between dispersed and resident adults (LMM, P = 0.960), but young immigrants tended to breed earlier, although not significantly so, than young residents (LMM, estimate ± s.e. = -4.615 ± 2.38, t = 1.94, P = 0.056). Adult males also paired with females laying more eggs and produced more fledglings than young males (LMM, both P < 0.001), but no differences were found between immigrants and residents and no significant interactions were observed between dispersal status and age neither in clutch size nor in the number of fledglings (LMM, all P > 0.14).
Male Tail Length and Reproduction
Since tail length was correlated with tarsus length, we used the residuals of the regression between tail and tarsus lengths to control for body size. Controlling for age (LMM, P < 0.001), laying date was correlated with tail length in males [LMM, estimate ± s.e. = -0.265 ± 0.08, F(1, 105) = 10.67, P = 0.002, n = 350; Figure 9]. Dispersal status and the interactions dispersal status × age and dispersal status × tail length were not significant (LMM, all P > 0.12). Controlling for age, neither clutch size nor the number of fledglings were significantly correlated with tail length with or without laying date in the models (LMM, all P > 0.16) and no significant effects were observed for dispersal status and remaining interactions (LMM, all P > 0.20).
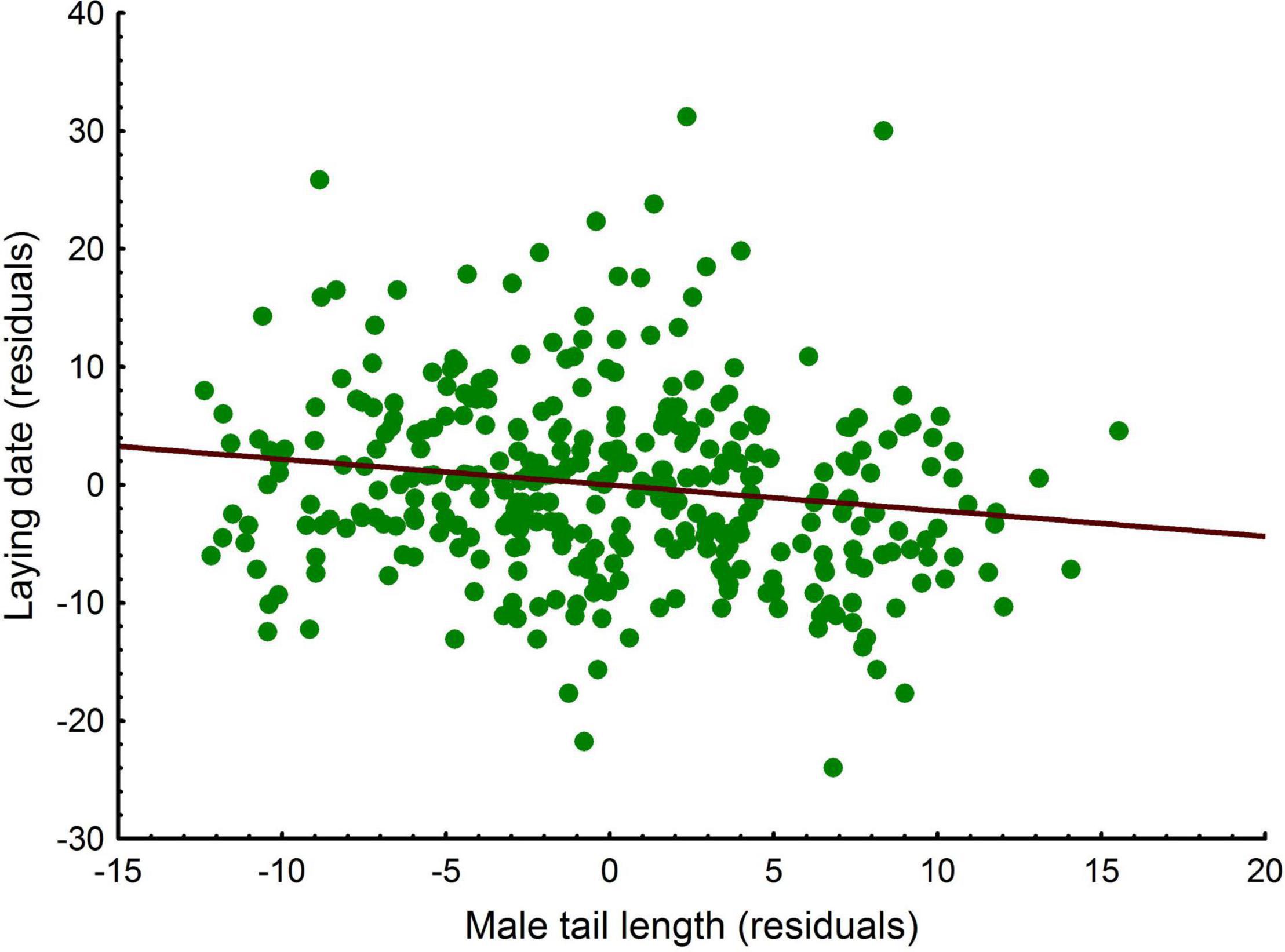
Figure 9. Linear relationship between laying date (residuals) and tail length in male common kestrels.
Discussion
Population Conditions
Immigration rates found in our study (35%) are slightly lower than those found in populations of other bird species that vary between 46 and 54% (Verhulst et al., 1997; Bensch et al., 1998; Frederiksen and Petersen, 2000; Mayer et al., 2009; Schaub et al., 2013. It is also lower than in other related species, such as the Falco naumanni (48% of young and 47% of adults; Serrano et al., 2004), and even considerably lower than in populations of common kestrels (90% in females and 68% in males; Sumasgutner et al., 2014). Good environmental conditions, particularly high food abundance, increases survival (Martin, 1987) and also increases philopatry in the same way that it attracts dispersers (Forero et al., 2002; Matthysen, 2012; Terraube et al., 2015). Furthermore, it is known that sedentary species and populations have lower dispersal rates (Paradis et al., 1998). Our population is one of the densest known for this species (Fargallo et al., 2001) and also shows a high average clutch size (Carrillo and González-Dávila, 2010), indicating that it is a population that offers good food conditions. This, and its sedentary status, might explain the low rate of immigration found. Population results also showed that good environmental food conditions promoting large clutch sizes do not increase the density of kestrel breeding pairs, indicating that environmental and/or demographic variables others than food abundance have a stronger influence in the dynamics of our population reducing density-dependent processes.
Mean clutch size of the population has the potential to explain the entry of foreign immigrants. One reason good feeding conditions attract dispersers is because it reduces settlement costs (Bonte et al., 2012), since it favors access to food resources and relaxes conspecific competition (Forero et al., 2002), thus allowing higher immigration rates in years where the food is abundant (Korpimäki, 1994). This may explain the positive correlation between the proportion of immigrants entering the population and mean clutch size. A greater investment of males in the defense of resources for the attraction of females is one of the ideas proposed to explain the greater tendency of bird females to disperse compared to males (Greenwood, 1980), including raptors (Serrano, 2018). Males would face higher costs if they decide to leave a familiar area. Sex differences in the investment and defense of breeding territories and nest sites might explain the higher immigration rate of females with respect to males observed in our study, although it is only significant in the case of the young. This result suggests that the admission of females into our population had fewer obstacles than the admission of males. We found no clear relationships between breeding density and immigration rate, nor effects of age or sex. Specific studies are needed to understand how birth, death, and immigration rates affect population density.
Reproduction
Although productivity did not differ between immigrant and resident females, young immigrants start laying earlier and lay more eggs than resident females, which does not occur in adults. This implies higher survival prospects for the offspring coming from immigrants as fledglings from earlier breeding attempts have higher body condition (Martínez-Padilla et al., 2017), longer post-fledging dependent periods (Vergara et al., 2010), which is associated with higher recruitment rates (López-Idiáquez et al., 2018). Regarding male reproduction, a tendency was observed for young immigrants to start breeding earlier than young residents, which was not observed in adults. Neither clutch size nor the number of fledglings were affected by dispersal status. Although poorly studied, the immediate consequences of dispersal in raptors are generally improved breeding success (see Serrano, 2018) also in kestrels (Terraube et al., 2015). Our results partially agree with this, since only females, particularly young females, seem to show an improvement in reproductive parameters, suggesting higher dispersal benefits for females than for males which would also explain the higher immigration rates of females with respect to males found in our study.
Body Size and Condition
At the individual level, there were clear differences between young and adult females entering the population. Immigrant young females, had longer wings and tails, and showed better body condition than resident young females. No differences in size or feather length were found in adult females, but young and adult immigrants showed better body condition than residents. In the case of males, no clear differences in size, wing length or body condition between immigrant and resident males were observed. Only in young males we found differences in tail length, immigrant individuals having longer tails than resident ones. The longest wings favor long displacements and it has been found frequently associated with dispersal distances in birds (Paradis et al., 1998; Dawideit et al., 2009). Our results on body condition coincides with that reported by Terraube et al. (2015) who found that in female kestrels, but not in males, dispersal distances were correlated with body condition. Good body condition might provide a higher capacity to buffer the costs linked to long-distance dispersal and pre-breeding prospecting (Barbraud et al., 2003; Delgado et al., 2010; Terraube et al., 2015; see also Clobert et al., 2009). Even without knowing the dispersal distances of the individuals that enter our population, our results suggest that either many of the immigrant females come from distant places and/or that a good body condition favors entry into the population through increased competitive skills or male choosiness. Our study therefore indicates a link between phenotypic traits potentially favoring dispersal movement during the transient phase, such as long wings, and individual quality, such as body condition and tail length (see below) favoring transient and settlement phases of dispersal. This is particularly relevant in young females, suggesting that this association is mainly stablished for natal dispersal within this sex. Overall, these results also point to a sexual difference in dispersal patterns in which selection pressures might be stronger for females, developing phenotypes to favor it.
Plumage Coloration
The common kestrel exhibit sexual dichromatism, so that, with the exception of the terminal tail band, the color traits studied differ between sexes. Our results show that immigrant females show tails with less gray than residents. Recent studies have reported that grayness of females and female-like young males reflects individual quality (Fargallo et al., 2007; Vergara and Fargallo, 2008; Vergara et al., 2009) and is considered a badge of status (Vergara and Fargallo, 2007; López-Idiáquez et al., 2016a). Our results here show that females exhibiting grayer tails start laying earlier, lay more eggs and produce more chicks. Interestingly, it has been reported in experimental studies simulating individual intrusions in kestrel breeding territories that young male intruders elicited different levels of aggressive responses depending on the manipulated color trait (Vergara et al., 2012). Specifically, a gray tail is the trait triggering the highest degree of aggressive behavior in territorial breeding females, concluding that the best phenotype for a young male is the one that creates a balance between signaling quality to get a mate and reducing aggressive behavior of male and female breeders (Vergara et al., 2012). Since phenotypes of young males resemble that of adult females, this conclusion can be applied to the present study. According to this, the relationship between tail grayness and reproductive parameters was observed in resident but not in immigrant females, suggesting that good-quality but inexpert immigrant females who enter new populations should be benefited by signaling subordination (browner tails), thus avoiding confrontations with adult breeders, mainly female breeders, and favoring population settlement.
In the case of males, adults, but not young, entering the population had a higher number of black back spots than resident individuals, and no differences were observed in other measured color traits. Previous studies carried out in our population showed that the number of black spots in males decreases with age and was negatively correlated with clutch size, especially in years of poor food conditions (López-Idiáquez et al., 2016b). The most plausible explanation for the difference we found is that adult immigrants entering the population are younger than the mean age of the adult residents. When all ringed residents are taken into account, the modal male age in the population is 2 years (Supplementary Figure 2A). In the case of immigrants, the most frequent males are 1-year old, these individuals being 70% more common than 2-year old individuals are (Supplementary Figure 2C), which is a drastic difference compared to that found in the residents. This is so because the real age in the immigrant fraction of the population can only be known with individuals who entered at the age of 1 year. If we do this with residents (Supplementary Figure 2B), the distribution of ages are similar to that found in immigrants. All of this suggests two aspects of interest. One is that we are surely underestimating a high proportion of 2-year old individuals entering the population that might explain the higher number of black spots found in adult immigrants with respect to residents, who are older on average. Another aspect is that, the data with which we work in the study does not allow us to differentiate dispersal or settlement patterns between natal and breeding dispersal, since many individuals reproduce for the first time at an age that we consider to be adult, particularly many males start breeding at 2 years of age (López-Rull et al., 2016). Contrary to females, in males we did not observe a clear difference in individual quality signals associated with plumage coloration between different dispersal statuses. In the case of females, and considering the plumage coloration, the individuals that enter the population would be indicating low quality or subordination (Vergara and Fargallo, 2007; Vergara et al., 2012).
Tail Length, Plumage Coloration, and Young Phenotypes
Our study shows that tail length in kestrels is a key trait associated with settlement patterns in both sexes and related to different components of fitness. Longer tails usually means higher costs for flight, making long trips difficult, which explains why migratory birds tend to have shorter tails (Balmford et al., 1993; Thomas and Balmford, 1995). The natal and reproductive dispersal distances found in kestrels are not very long (<200 km; Terraube et al., 2015), but in general kestrels travel long distances after fledging and in premigratory and migratory periods (Adriaensen et al., 1997; Śliwa et al., 2009; Village, 1990; Holte et al., 2016). Kestrels are also migrants in southern Europe, traveling to Africa during the winter, as observed in our population, where at least three individuals born in our population were found dead during the winter in Mauritania and Morocco. Also during reproduction, males can make long-distance trips of more than 10 km (Costantini and Dell’Omo, 2020). Therefore, long tails in this species can lead to significant energy costs. However, long tails may help for hunting. Within the falconids, the kestrel group is characterized by having a smaller wing-tail ratio (Village, 1990), which is related with a higher consumption of terrestrial prey species, mainly small mammals, and an adaptation for flight-hunting or “hovering,” a type of flight used to capture this type of prey (Sale, 2020) a flight for which the common kestrel is well known.
The main function proposed for bird tails is related to stability and maneuverability and not with flight speed, for which it has led to believe that the development of the tail has had a greater margin to evolve as a signal, playing a relevant role in sexual selection (Balmford et al., 1993; Andersson, 1994; Winquist and Lemon, 1994). Palokangas et al. (1992) reported that choosy common kestrel females prefer males with longer tails to mate, however, the length of the tail was not found to be associated with breeding success. In our case, we showed that, irrespective of age and dispersal status, males exhibiting longer tails started breeding earlier, which was also observed in females. Furthermore, in females it was positively correlated with productivity, measured as the number of fledged chicks. In our population, kestrels prey on a variety of prey species, but they have a markedly predilection for voles (Fargallo et al., 2020) and individuals hunting more voles have lower wing-to-tail ratios (Fargallo unpublished data). These results indicate that tail length reflects individual quality in both males and females either by indicating hunting abilities and nutritional state. Thus, both young males and females entering the population are of better quality than young residents, as resulted from the longer tails found in the formers. Since by choosing long-tailed males it is possible to start reproduction earlier, the preference of females for this trait found in previous works (Palokangas et al., 1992) can be explained.
Considering these results together, we propose that longer tails, probably not selected for dispersal movements, but signaling quality, favored the settlement of the young in the new population. In the lazuli bunting Passerina amoena, a sexually dichromatic bird exhibiting delayed plumage maturation, Greene et al. (2000) found that breeding young males show a great variation of plumage coloration from female-like dull brown to bright blue, like adult males. The dullest and the brightest young are more successful in obtaining high-quality territories, pairing with females and siring offspring, than yearlings with intermediate plumage. Females choose males based on the quality of their territories rather than on plumage coloration. Bright blue young have better competitive abilities to gain better territories than dull and intermediate, which facilitates mating. On the other hand, good-quality adult males allow subordinate dull individuals to breed in close proximity to their territories, benefiting from it through the extra-pair paternity (EPP), which is high in this species (49% of nests contained chicks not sired by the resident male; Greene et al., 2000). Female-like plumages in males in kestrels reduce conspecific aggressiveness from adult males (Vergara and Fargallo, 2007) and allow young males breeding closer to adult males than other adult males (Hakkarainen et al., 1993). The frequency of extra-pair paternity (EPP) in the kestrel is low. E. Korpimäki et al. (1996) found EPP in 1.9% of chicks (6 out of 319 chicks) and 3% of nests (2 out of 75 nests) in a 3-year study in a Finnish population. EPP analyzed in our population in years 2005 and 2006 (J. A. Fargallo and J. A. Dávila, unpublished data) show similar values, being 2.5% in chicks-eggs (7 out of 279 chicks-eggs) and 6.3% in nests (4 out of 63 nests). In the Finnish population, one of the two cuckolded males was a young, which is a high frequency (50%) if one takes into account that there were only two breeding young males in the population (2.7%; Korpimäki et al., 1996). In our population, of the four cuckolded males, one was young, the frequency also being higher (25%) than the proportion of young kestrels breeding during those 2 years (14.3%). More precise studies on this matter should be carried out in the kestrel, but it is possible that, as in the lazuli bunting, young kestrels signaling subordination may settle more easily in the population if it represents an opportunity for the adults to increase their fitness via extra-pair paternity.
In conclusion, within a social selection and signaling scheme, our study highlights the importance of phenotypic strategies involved in the final phase of the dispersal, that is, population settlement. In general, individuals entering a population seem to be of higher quality than the mean of the population they enter. This is especially observable in the young class, indicating its importance for natal dispersal. In the case of females, who reach the host population in better body condition, direct benefits were observed in reproduction, as early breeding increases offspring survival prospects. Also, a similar productivity in the young and in the general reproductive performance of the adults is a remarkable achievement in terms of fitness considering the inexperience of immigrants in unfamiliar places and with already established social structures, which are considered to be dispersal costs. Interestingly, some phenotypic traits exhibited in settlement match with those expected for dispersal phenotypes in the transience phase, such as long wings observed in young females. However, other traits, such as long tails in young individuals (males and females) show the contrary pattern to those expected for the transience phase, as long tails that are costly for flight. As a long tail is indicative of good hunting efficiency and promotes early breeding in both males and females and higher productivity in females, this character may be advantageous during settlement and immigrants can enter the population either because they are of good quality and compete better for breeding resources than residents or because they are preferred by mates, at least in the case of males. Our study also suggests that signaling subordination to reduce the aggressive interactions with resident individuals may facilitate the settlement of good quality immigrants. This deduce from the fact that immigrant females (young and adults) showed browner tails irrespective of its length. In addition, as immigrant females enter the population a higher rates, a female-like phenotype could pose fewer obstacles for the settlement of inexpert young males, which is suggested as a selective pressure promoting the existence of delayed plumage maturation. Finally, our study evidences sexual differences in dispersal patterns at the level of size, condition, plumage coloration, and reproduction, suggesting stronger selective dispersal pressures for females and highlighting the importance of the settlement phase to better understand dispersal and the pressures shaping dispersal phenotypes.
Data Availability Statement
The raw data supporting the conclusions of this article will be made available by the authors, without undue reservation.
Ethics Statement
The animal study was reviewed and approved by Comité de Bioética—Museo Nacional de Ciencias Naturales.
Author Contributions
JF did the fieldwork and collected the data. IL-R did part of the fieldwork and collected part of the data. JF and IL-R conceived the study, analyzed the data, and wrote the original draft. Both authors contributed to the article and approved the submitted version.
Funding
Research was funded by the Ministerio de Ciencia e Innovación through projects CGL2007–61395, CGL2010–15726, CGL2013–42451-P, PGC2018–095070-B-I00, and PID2019-111039GA-100.
Conflict of Interest
The authors declare that the research was conducted in the absence of any commercial or financial relationships that could be construed as a potential conflict of interest.
Publisher’s Note
All claims expressed in this article are solely those of the authors and do not necessarily represent those of their affiliated organizations, or those of the publisher, the editors and the reviewers. Any product that may be evaluated in this article, or claim that may be made by its manufacturer, is not guaranteed or endorsed by the publisher.
Acknowledgments
Pablo Vergara, Jesús Martínez-Padilla, David López-Idiáquez, and Juan Navarro-López helped during fieldwork. David López-Idiáquez, Sara García, and Miriam Bravo helped measuring color traits in digital photographs. The study was carried out on the Finat family property with the help of J. San Teodoro. Licenses to study kestrels were granted by the Junta de Castilla y León. This is a contribution of the El Ventorrillo Biological Station.
Supplementary Material
The Supplementary Material for this article can be found online at: https://www.frontiersin.org/articles/10.3389/fevo.2022.810516/full#supplementary-material
References
Adriaensen, F., Verwimp, N., and Dhondt, A. A. (1997). Are belgian kestrels falco tinnunculus migratory: an analysis of ringing recoveries. Ring. Migrat. 18, 91–101. doi: 10.1080/03078698.1997.9674149
Balmford, A., Thomas, A. L., and Jones, I. L. (1993). Aerodynamics and the evolution of long tails in birds. Nature 361, 628–631. doi: 10.1038/361628a0
Barbraud, C., Johnson, A. R., and Bertault, G. (2003). Phenotypic correlates of post-fledging dispersal in a population of greater flamingos: the importance of body condition. J. Anim Ecol. 72, 246–257. doi: 10.1046/j.1365-2656.2003.00695.x
Bélichon, S., Clobert, J., and Massot, M. (1996). Are there differences in fitness components between philopatric and dispersing individuals? Acta Oecol. 17, 503–517.
Bensch, S., Hasselquist, D., Nielsen, B., and Hansson, B. (1998). Higher fitness for philopatric than for immigrant males in a semi-isolated population of great reed warblers. Evolution 52, 877–883. doi: 10.1111/j.1558-5646.1998.tb03712.x
Berthier, K., Leippert, F., Fumagalli, L., and Arlettaz, R. (2012). Massive nest-nox supplementation boosts fecundity, survival and even immigration without altering mating and reproductive behaviour in a rapidly recovered bird population. PLoS One 7:e36028. doi: 10.1371/journal.pone.0036028
Bize, P., Daniel, G., Viblanc, V. A., Martin, J. G., and Doligez, B. (2017). Negative phenotypic and genetic correlation between natal dispersal propensity and nest-defence behaviour in a wild bird. Biol. Lett. 13:20170236. doi: 10.1098/rsbl.2017.0236
Bonte, D., Van Dyck, H., Bullock, J. M., Coulon, A., Delgado, M., Gibbs, M., et al. (2012). Costs of dispersal. Biol. Rev. 87, 290–312.
Bowler, D. E., and Benton, T. G. (2005). Causes and consequences of animal dispersal strategies: relating individual behaviour to spatial dynamics. Biol. Rev. 80, 205–225. doi: 10.1017/s1464793104006645
Camacho, C., Canal, D., and Potti, J. (2013). Nonrandom dispersal drives phenotypic divergence within a bird population. Ecol. Evol. 3, 4841–4848. doi: 10.1002/ece3.563
Camacho, C., Canal, D., and Potti, J. (2015). Testing the matching habitat choice hypothesis in nature: phenotype-environment correlation and fitness in a songbird population. Evol. Ecol. 29, 873–886. doi: 10.1007/s10682-015-9793-4
Camacho, C., Pérez-Rodríguez, L., Abril-Colón, I., Canal, D., and Potti, J. (2018). Plumage colour predicts dispersal propensity in male pied flycatchers. Behav. Ecol. Sociobiol. 72, 1–8.
Carrillo, J., and González-Dávila, E. (2010). Geo-environmental influences on breeding parameters of the eurasian kestrel (Falco tinnunculus) in the western palaearctic. Ornis Fenn 87, 15–25.
Chapman, B. B., Hulthén, K., Blomqvist, D. R., Hansson, L. A., Nilsson, J. Å, Brodersen, J., et al. (2011). To boldly go: individual differences in boldness influence migratory tendency. Ecol. Lett. 14, 871–876. doi: 10.1111/j.1461-0248.2011.01648.x
Clobert, J., Baguette, M., Benton, T. G., and Bullock, J. M. (2012). Dispersal Ecology and Evolution. Oxford: Oxford University Press.
Clobert, J., Le Galliard, J. F., Cote, J., Meylan, S., and Massot, M. (2009). Informed dispersal, heterogeneity in animal dispersal syndromes and the dynamics of spatially structured populations. Ecol. Lett. 12, 197–209. doi: 10.1111/j.1461-0248.2008.01267.x
Costantini, D., and Dell’Omo, G. (2020). The Kestrel: Ecology, Behaviour and Conservation of An Open-Land Predator. Cambridge: Cambridge University Press.
Cote, J., Brodin, T., Fogarty, S., and Sih, A. (2017). Non-random dispersal mediates invader impacts on the invertebrate community. J. Anim Ecol. 86, 1298–1307. doi: 10.1111/1365-2656.12734
Cotto, O., Kubisch, A., and Ronce, O. (2014). Optimal life-history strategy differs between philopatric and dispersing individuals in a metapopulation. Am. Nat. 183, 384–393. doi: 10.1086/675064
Dawideit, B. A., Phillimore, A. B., Laube, I., Leisler, B., and Böhning-Gaese, K. (2009). Ecomorphological predictors of natal dispersal distances in birds. J. Anim Ecol. 78, 388–395. doi: 10.1111/j.1365-2656.2008.01504.x
Delgado, M. D. M., Penteriani, V., Revilla, E., and Nams, V. O. (2010). The effect of phenotypic traits and external cues on natal dispersal movements. J. Anim Ecol. 79, 620–632. doi: 10.1111/j.1365-2656.2009.01655.x
Denno, R. F., and Roderick, G. K. (1992). Density-related dispersal in planthoppers: effects of interspecific crowding. Ecology 73, 1323–1334. doi: 10.2307/1940679
Doligez, B., Pärt, T., Danchin, E., Clobert, J., and Gustafsson, L. (2004). Availability and use of public information and conspecific density for settlement decisions in the collared flycatcher. J. Anim Ecol. 73, 75–87. doi: 10.1111/j.1365-2656.2004.00782.x
Doonan, T. J., and Slade, N. A. (1995). Effects of supplemental food on population dynamics of cotton rats. sigmodon hispidus. Ecology 76, 814–826. doi: 10.1007/s00442-011-2053-6
Fargallo, J. A., Blanco, G., Potti, J., and Viñuela, J. (2001). Nestbox provisioning in a rural population of eurasian kestrels: breeding performance, nest predation and parasitism. Bird Study 48, 236–244. doi: 10.1080/00063650109461223
Fargallo, J. A., Laaksonen, T., Korpimäki, E., and Wakamatsu, K. (2007). A melanin-based trait reflects environmental growth conditions of nestling male eurasian kestrels. Evol. Ecol. 21, 157–171. doi: 10.1007/s10682-006-0020-1
Fargallo, J. A., Martinez-Padilla, J., Vinuela, J., Blanco, G., Torre, I., Vergara, P., et al. (2009). Kestrel-prey dynamic in a mediterranean region: the effect of generalist predation and climatic factors. PLoS One 4:e4311. doi: 10.1371/journal.pone.0004311
Fargallo, J. A., Navarro-López, J., Palma-Granados, P., and Nieto, R. M. (2020). Foraging strategy of a carnivorous-insectivorous raptor species based on prey size, capturability and nutritional components. Sci. Rep. 10, 1–12. doi: 10.1038/s41598-020-64504-4
Fargallo, J. A., Velando, A., López-Rull, I., Gañán, N., Lifshitz, N., Wakamatsu, K., et al. (2014). Sex-specific phenotypic integration: endocrine profiles, coloration, and behavior in fledgling boobies. Behav. Ecol. 25, 76–87. doi: 10.1093/beheco/art088
Forero, M. G., Donázar, J. A., and Hiraldo, F. (2002). Causes and fitness consequences of natal dispersal in a population of black kites. Ecology 83, 858–872. doi: 10.1890/0012-9658(2002)083[0858:cafcon]2.0.co;2
Fraser, D. F., Gilliam, J. F., Daley, M. J., Le, A. N., and Skalski, G. T. (2001). Explaining leptokurtic movement distributions: intrapopulation variation in boldness and exploration. Am. Nat. 158, 124–135. doi: 10.1086/321307
Frederiksen, M., and Petersen, A. E. V. A. R. (2000). The importance of natal dispersal in a colonial seabird, the black guillemot Cepphus grylle. IBIS 142, 48–57. doi: 10.1111/j.1474-919x.2000.tb07683.x
Garant, D., Kruuk, L. E., Wilkin, T. A., McCleery, R. H., and Sheldon, B. C. (2005). Evolution driven by differential dispersal within a wild bird population. Nature 433, 60–65. doi: 10.1038/nature03051
Greene, E., Lyon, B. E., Muehter, V. R., Ratcliffe, L., Oliver, S. J., and Boag, P. T. (2000). Disruptive sexual selection for plumage coloration in a passerine bird. Nature 407, 1000–1003. doi: 10.1038/35039500
Greenwood, P. J. (1980). Mating systems, philopatry and dispersal in birds and mammals. Anim Behav. 28, 1140–1162. doi: 10.1016/s0003-3472(80)80103-5
Greenwood, P. J., and Harvey, P. H. (1982). The natal and breeding dispersal of birds. Ann. Rev. Ecol. Evol. Syst. 13, 1–21. doi: 10.1146/annurev.es.13.110182.000245
Hakkarainen, H., Korpimäki, E., Huhta, E., and Palokangas, P. (1993). Delayed maturation in plumage colour: evidence for the female-mimicry hypothesis in the kestrel. Behav. Ecol. Soc. 33, 247–251. doi: 10.1007/bf02027121
Hamilton, W. D., and Zuk, M. (1982). Heritable true fitness and bright birds: a role for parasites? Science 218, 384–387. doi: 10.1126/science.7123238
Hawkins, G. L., Hill, G. E., and Mercadante, A. (2012). Delayed plumage maturation and delayed reproductive investment in birds. Biol. Rev. 87, 257–274. doi: 10.1111/j.1469-185X.2011.00193.x
Hill, G. E. (1991). Plumage coloration is a sexually selected indicator of male quality. Nature 350, 337–339. doi: 10.1038/350337a0
Hill, G. E. (2006). “Female mate choice for ornamental coloration,” in Bird Coloration II. Function and Evolution, eds G. E. Hill and K. J. McGraw (Harvard University Press).
Holte, D., Köppen, U., and Schmitz-Ornés, A. (2016). Partial migration in a central european raptor species: an analysis of ring re-encounter data of common kestrels falco tinnunculus. Acta Ornithol. 51, 39–54. doi: 10.3161/00016454ao2016.51.1.004
Jokela, M., Elovainio, M., Kivimäki, M., and Keltikangas-Järvinen, L. (2008). Temperament and migration patterns in finland. Psychol. Sci. 19, 831–837. doi: 10.1111/j.1467-9280.2008.02164.x
Kim, S. Y., Fargallo, J. A., Vergara, P., and Martínez-Padilla, J. (2013). Multivariate heredity of melanin-based coloration, body mass and immunity. Heredity 111, 139–146. doi: 10.1038/hdy.2013.29
Korpimäki, E. (1994). Rapid or delayed tracking of multi-annual vole cycles by avian predators? J. Anim Ecol. 63, 619–628. doi: 10.2307/5228
Korpimäki, E., Lahti, K., May, C. A., Parkin, D. T., Powell, G. B., Tolonen, P., et al. (1996). Copulatory behaviour and paternity determined by DNA fingerprinting in kestrels: effects of cyclic food abundance. Anim Behav. 51, 945–955. doi: 10.1006/anbe.1996.0098
López-Idiáquez, D., Fargallo, J. A., López-Rull, I., and Martínez-Padilla, J. (2019). Plumage coloration and personality in early life: sexual differences in signalling. IBIS 161, 216–221. doi: 10.1111/ibi.12665
López-Idiáquez, D., Vergara, P., Fargallo, J. A., and Martinez-Padilla, J. (2016a). Old males reduce melanin-pigmented traits and increase reproductive outcome under worse environmental conditions in common kestrels. Ecology 6, 1224–1235. doi: 10.1002/ece3.1910
López-Idiáquez, D., Vergara, P., Fargallo, J. A., and Martínez-Padilla, J. (2018). Providing longer post-fledging periods increases offspring survival at the expense of future fecundity. PLoS One 13:e0203152. doi: 10.1371/journal.pone.0203152
López-Idiáquez, D., Vergara, P., Fargallo, J. A., and Martínez-Padilla, J. (2016b). Female plumage coloration signals status to conspecifics. Anim Behav. 121, 101–106. doi: 10.1016/j.anbehav.2016.08.020
López-Rull, I., Vergara, P., Martínez-Padilla, J., and Fargallo, J. A. (2016). Early constraints in sexual dimorphism: survival benefits of feminized phenotypes. J. Evol. Biol. 29, 231–240. doi: 10.1111/jeb.12779
Lyon, B. E., and Montgomerie, R. D. (1986). Delayed plumage maturation in passerine birds: reliable signaling by subordinate males? Evolution 40, 605–615. doi: 10.1111/j.1558-5646.1986.tb00511.x
Martin, T. E. (1987). Food as a limit on breeding birds: a life-history perspective. Ann. Rev. Ecol. Syst. 18, 453–487. doi: 10.1146/annurev.es.18.110187.002321
Martínez-Padilla, J., Vergara, P., and Fargallo, J. A. (2017). Increased lifetime reproductive success of first-hatched siblings in common kestrels Falco tinnunculus. Ibis 159, 803–811. doi: 10.1111/ibi.12494
Matthysen, E. (2005). Density-dependent dispersal in birds and mammals. Ecography 28, 403–416. doi: 10.1111/j.0906-7590.2005.04073.x
Matthysen, E. (2012). “Muticausality of dispersal: a review,” in Dispersal Ecology and Evolution, eds J. Clobert, M. Baguette, T. G. Benton, and J. M. Bullock (Oxford University Press).
Mayer, C., Schiegg, K., and Pasinelli, G. (2009). Patchy population structure in a short-distance migrant: evidence from genetic and demographic data. Mol. Ecol. 18, 2353–2364. doi: 10.1111/j.1365-294X.2009.04200.x
Palokangas, P., Alatalo, R. V., and Korpimäki, E. (1992). Female choice in the kestrel under different availability of mating options. Anim Behav. 43, 659–665. doi: 10.1016/s0003-3472(05)81024-3
Palokangas, P., Korpimäki, E., Hakkarainen, H., Huhta, E., Tolonen, P., and Alatalo, R. V. (1994). Female kestrels gain reproductive success by choosing brightly ornamented males. Anim Behav. 47, 443–448. doi: 10.1006/anbe.1994.1058
Paradis, E., Baillie, S. R., Sutherland, W. J., and Gregory, R. D. (1998). Patterns of natal and breeding dispersal in birds. J. Anim Ecol. 67, 518–536. doi: 10.1046/j.1365-2656.1998.00215.x
Pigliucci, M., and Preston, K. (2004). Phenotypic Integration: Studying the Ecology and Evolution of Complex Phenotypes. Oxford: Oxford University Press.
Pryke, S. R., Astheimer, L. B., Griffith, S. C., and Buttemer, W. A. (2012). Covariation in life-history traits: differential effects of diet on condition, hormones, behavior, and reproduction in genetic finch morphs. Am. Nat. 179, 375–390. doi: 10.1086/664078
Roff, D. A., and Gelinas, M. B. (2003). Phenotypic plasticity and the evolution of trade-offs: the quantitative genetics of resource allocation in the wing dimorphic cricket, Gryllus firmus. J. Evol. Biol. 16, 55–63. doi: 10.1046/j.1420-9101.2003.00480.x
Ronce, O., and Clobert, J. (2012). “Dispersal syndromes,” in Dispersal Ecology and Evolution, eds J. Clobert, M. Baguette, T. G. Benton, and J. M. Bullock (Oxford University Press).
Roulin, A. (2013). Ring recoveries of dead birds confirm that darker pheomelanic barn owls disperse longer distances. J. Ornithol. 154, 871–874. doi: 10.1007/s10336-013-0949-0
Roulin, A., and Ducrest, A. L. (2011). Association between melanism, physiology and behaviour: a role for the melanocortin system. Eur. J. Pharmacol. 660, 226–233. doi: 10.1016/j.ejphar.2011.01.036
Saino, N., Romano, M., Scandolara, C., Rubolini, D., Ambrosini, R., Caprioli, M., et al. (2014). Brownish, small and lousy barn swallows have greater natal dispersal propensity. Anim Behav. 87, 137–146. doi: 10.1016/j.anbehav.2013.10.022
Schaub, M., Jakober, H., and Stauber, W. (2013). Strong contribution of immigration to local population regulation: evidence from a migratory passerine. Ecology 94, 1828–1838. doi: 10.1890/12-1395.1
Senar, J. C. (2006). “Color displays as intrasexual signals of aggression and dominance,” in Bird Coloration II. Function and Evolution, eds G. E. Hill and K. J. McGraw (Harvard University Press).
Senar, J. C., Conroy, M. J., and Borras, A. (2002). Asymmetric exchange between populations differing in habitat quality: a metapopulation study on the citril finch. J. Appl. Stat. 29, 425–441. doi: 10.1080/02664760120108791
Serrano, D. (2018). “Dispersal in raptors,” in Birds of Prey, eds J. H. Sarasola, J. M. Grande, and J. J. Negro (Cham: Springer).
Serrano, D., Forero, M. G., Donázar, J. A., and Tella, J. L. (2004). Dispersal and social attraction affect colony selection and dynamics of lesser kestrels. Ecology 85, 3438–3447. doi: 10.1890/04-0463
Śliwa, P., Mokwa, K., and Rejt, L. (2009). Migrations and wintering of the kestrel (Falco tinnunculus) in poland. Ring 31, 59–69. doi: 10.2478/v10050-008-0053-7
Stamps, J. A. (1988). Conspecific attraction and aggregation in territorial species. Am. Nat. 131, 329–347. doi: 10.1086/284793
Sumasgutner, P., Vasko, V., Varjonen, R., and Korpimäki, E. (2014). Public information revealed by pellets in nest sites is more important than ecto-parasite avoidance in the settlement decisions of eurasian kestrels. Behav. Ecol. Soc. 68, 2023–2034. doi: 10.1007/s00265-014-1808-6
Szostek, K. L., Schaub, M., and Becker, P. H. (2014). Immigrants are attracted by local pre-breeders and recruits in a seabird colony. J. Anim Ecol. 83, 1015–1024. doi: 10.1111/1365-2656.12206
Terraube, J., Vasko, V., and Korpimäki, E. (2015). Mechanisms and reproductive consequences of breeding dispersal in a specialist predator under temporally varying food conditions. Oikos 124, 762–771. doi: 10.1111/oik.01974
Thomas, A. L., and Balmford, A. (1995). How natural selection shapes birds’ tails. Am. Nat. 146, 848–868. doi: 10.1086/285828
Trochet, A., Courtois, E. A., Stevens, V. M., Baguette, M., Chaine, A., Schmeller, D. S., et al. (2016). Evolution of sex-biased dispersal. Q. Rev. Biol. 91, 297–320.
van den Brink, V., Dreiss, A. N., and Roulin, A. (2012). Melanin-based coloration predicts natal dispersal in the barn owl. tyto alba. Anim Behav. 84, 805–812. doi: 10.1016/j.anbehav.2012.07.001
Vasko, V., Laaksonen, T., Valkama, J., and Korpimäki, E. (2011). Breeding dispersal of eurasian kestrels Falco tinnunculus under temporally fluctuating food abundance. J. Avian Biol. 42, 552–563. doi: 10.1111/j.1600-048x.2011.05351.x
Vergara, P., and Fargallo, J. A. (2007). Delayed plumage maturation in eurasian kestrels: female mimicry, subordination signalling or both? Anim Behav. 74, 1505–1513. doi: 10.1016/j.anbehav.2007.03.013
Vergara, P., and Fargallo, J. A. (2008). Sex, melanic coloration, and sibling competition during the postfledging dependence period. Behav. Ecol. 19, 847–853. doi: 10.1093/beheco/arn035
Vergara, P., and Fargallo, J. A. (2011). Multiple coloured ornaments in male common kestrels: different mechanisms to convey quality. Nat. Wiss 98, 289–298. doi: 10.1007/s00114-011-0767-2
Vergara, P., Fargallo, J. A., and Martínez-Padilla, J. (2010). Reaching independence: food supply, parent quality, and offspring phenotypic characters in kestrels. Behav. Ecol. 21, 507–512. doi: 10.1093/beheco/arq011
Vergara, P., Fargallo, J. A., Martinez-Padilla, J., and Lemus, J. A. (2009). Inter-annual variation and information content of melanin-based coloration in female eurasian kestrels. Biol. J. Linn. Soc. 97, 781–790. doi: 10.1111/j.1095-8312.2009.01263.x
Vergara, P., Martínez-Padilla, J., and Fargallo, J. A. (2012). Differential maturation of sexual traits: revealing sex while reducing male and female aggressiveness. Behav. Ecol. 24, 237–244. doi: 10.1093/beheco/ars159
Verhulst, S., Perrins, C. M., and Riddington, R. (1997). Natal dispersal of great tits in a patchy environment. Ecology 78, 864–872. doi: 10.1890/0012-9658(1997)078[0864:ndogti]2.0.co;2
von Schantz, T. V., Bensch, S., Grahn, M., Hasselquist, D., and Wittzell, H. (1999). Good genes, oxidative stress and condition–dependent sexual signals. Proc. R. Soc. 266, 1–12. doi: 10.1098/rspb.1999.0597
Warton, D. I., and Hui, F. K. (2011). The arcsine is asinine: the analysis of proportions in ecology. Ecology 92, 3–10. doi: 10.1890/10-0340.1
Wilson, A. G., and Arcese, P. (2008). Influential factors for natal dispersal in an avian island metapopulation. J. Avian Biol. 39, 341–347. doi: 10.1111/j.0908-8857.2008.04239.x
Winquist, T., and Lemon, R. E. (1994). Sexual selection and exaggerated male tail length in birds. Am. Nat. 143, 95–116. doi: 10.1086/285597
Keywords: dispersal, delayed plumage maturation, melanin traits, status signaling, tail length, ornaments, philopatry, coloration
Citation: Fargallo JA and López-Rull I (2022) Settlement Phenotypes: Social Selection and Immigration in a Common Kestrel Population. Front. Ecol. Evol. 10:810516. doi: 10.3389/fevo.2022.810516
Received: 07 November 2021; Accepted: 20 April 2022;
Published: 10 May 2022.
Edited by:
Jesus Martinez-Padilla, Pyrenean Institute of Ecology (IPE—CSIC)—ARAID Senior Researcher, SpainReviewed by:
David Costantini, Muséum National d’Histoire Naturelle, FranceRoi Dor, Open University of Israel, Israel
Copyright © 2022 Fargallo and López-Rull. This is an open-access article distributed under the terms of the Creative Commons Attribution License (CC BY). The use, distribution or reproduction in other forums is permitted, provided the original author(s) and the copyright owner(s) are credited and that the original publication in this journal is cited, in accordance with accepted academic practice. No use, distribution or reproduction is permitted which does not comply with these terms.
*Correspondence: Juan Antonio Fargallo, ZmFyZ2FsbG9AbW5jbi5jc2ljLmVz
†These authors have contributed equally to this work