- 1State Key Laboratory of Freshwater Ecology and Biotechnology, Institute of Hydrobiology, Chinese Academy of Sciences, Wuhan, China
- 2University of Chinese Academy of Sciences, Beijing, China
- 3Beijing Osees Technology Co., Limited, Beijing, China
Body size is sensitive to environmental changes and one of the fundamental traits linking ecological functions. Size structure has been suggested as a useful indicator for environmental monitoring and assessment in aquatic ecosystems. However, the organisms’ size structure and the relationship with environmental factors remain seldom addressed in reservoir ecosystems. In this study, we investigated the size spectrum, size diversity of the zooplankton and their relationships with environmental conditions across nitrogen and phosphorus gradients in the Xiangxi Bay of Three Gorges Reservoir, China. We further tested the hypotheses that how nutrient and water temperature affect zooplankton size structure: nutrients indirectly affect zooplankton size spectrum and size diversity via phytoplankton (H1); increasing water temperature will reduce size diversity and result in a steeper size spectrum (H2); size diversity is a more robust metric indicating environment changes than the size spectrum in high dynamic ecosystems (H3). We found that both the size spectrum and size diversity showed high spatiotemporal dynamics. The size spectrum ranged from −3.373 to −0.984. The size diversity ranged from 0.631 to 3.291. Spatially, the lowest values of the size spectrum and size diversity were observed in the upstream areas of Xiangxi Bay, where nitrogen and phosphorus concentrations are high and low, respectively. And in temporal dynamics, lower values of the size spectrum and size diversity were generally observed in March and April. Further analyses based on the structural equation model (SEM) found a clear pathway revealing that nutrient variables affect the zooplankton abundance and size structure, supporting hypothesis H1. That is, dissolved inorganic nitrogen had an indirect effect on the zooplankton abundance, size spectrum, and size diversity by influencing the concentration of phytoplankton chlorophyll a. In addition, results of SEM suggested that increased water temperature had a significant negative effect on the size diversity but had non-significant effects on zooplankton abundance and size spectrum. This finding suggests that size diversity is a reliable and useful index in measuring the zooplankton size structure in reservoir ecosystems with high dynamics, which may have a wide application in environmental monitoring and assessment, especially for complex and dynamic aquatic ecosystems.
Introduction
Zooplankton is an essential component in aquatic ecosystems and plays a fundamental role in energy flow and material cycle in the ecosystem. Zooplankton is the crucial linking of the primary producer to high trophic organisms, which is recognized as essential information in understanding pelagic food webs dynamics (Fenchel, 1988; Eddy et al., 2021; Peng et al., 2021). Because of their small size and short lifecycle, zooplankton is very sensitive to environmental changes and has a wide distribution in aquatic ecosystems (Whitman et al., 2004; García-Comas et al., 2014; Hessen et al., 2019; Rizo et al., 2020). These characteristics make zooplankton become a good indicator for water quality monitoring and environmental change assessment in aquatic ecosystems (García-Comas et al., 2014; Lee et al., 2021; Venello et al., 2021).
Body size has long been considered as a fundamental functional trait in determining community structure and functions and indicating environmental changes (Daufresne et al., 2009; Stouffer et al., 2011; Ye et al., 2013; Verberk et al., 2021). Because of the importance of body size, there has been a growing interest in investigating the size structure of zooplankton community and its response to eutrophication and global warming (Daufresne et al., 2009; Fuchs and Franks, 2010; García-Comas et al., 2014; Gao et al., 2019; Toruan et al., 2021). Global warming is expected to benefit the small size organisms in aquatic ecosystems because of the elevated metabolic rate and energy cost (Daufresne et al., 2009; Yvon-Durocher et al., 2011). And the reduction of body size has even been suggested as a third universal response to global warming (Daufresne et al., 2009). Meanwhile, eutrophication is expected to favor larger organisms in size-based food chains (Davis et al., 2010; Fuchs and Franks, 2010; Toruan et al., 2021). For example, long-term nitrogen and phosphorus enrichment experiments showed that nutrient enrichment will increase the dominance of large body size prey (Davis et al., 2010). Therefore, we hypothesized that the size structure of zooplankton community is closely related to eutrophication and water temperature.
The size spectrum and size diversity are the two widely used metrics representing community size structure (Yvon-Durocher et al., 2011; Ye et al., 2013; Sprules and Barth, 2016; Edwards et al., 2017). Specifically, the slope of the size spectrum represents the relative distribution of individual sizes; a steeper slope indicates a higher contribution of small organisms to the total biomass of a community (García-Comas et al., 2014). Over the past decades, several methods have been developed to fit size spectra and the maximum likelihood estimation method has been suggested as the most reliable method in fitting size spectra (Edwards et al., 2017). Size diversity is a recent emerging metric adapted from the Shannon diversity expression in describing the shape of size distributions (Quintana et al., 2008; Ye et al., 2013; García-Comas et al., 2014). Comparing to the size spectrum, size diversity doesn’t require statistical fitting, which makes this index is more flexible in estimating the size structure (García-Comas et al., 2014). Several studies have reported that zooplankton size spectrum and size diversity are sensitive to environmental changes in marine and lake ecosystems (García-Comas et al., 2014; Toruan et al., 2021). However, how zooplankton size spectrum and size diversity respond to environmental factors remains seldom addressed in reservoir ecosystems.
Different from many other natural water bodies, reservoir ecosystems are unique ecosystems between river ecosystems and lake ecosystems and generally have a high spatial heterogeneity of physicochemical and biological conditions (Ye and Cai, 2011; Shen et al., 2014; Xu et al., 2021). According to the hydrodynamic characteristics, the flooded area of reservoirs can be divided into a riverine zone, transition zone, and lacustrine zone from the backwater area to the dam (Straškraba and Tundisi, 1999), which makes reservoir ecosystems a high spatial heterogeneity (Ye and Cai, 2011; Shen et al., 2014). Ye and Cai (2011) report that the concentration of nitrogen and phosphorus had clear inverse patterns in the Xiangxi Bay of Three Gorges Reservoir (TGR). Specifically, the concentration of nitrate decreased from the downstream to the upstream of the bay. The concentration of soluble reactive phosphorus had a reverse pattern, with the concentration increased from the downstream to the upstream of the bay. Obviously, this kind of nutrient gradients provides a natural experiment site to investigate how zooplankton size structure responds to nutrient enrichment in reservoir ecosystems.
For the above reasons, we investigated the size structure of zooplankton communities in the Xiangxi Bay of Three Gorges Reservoir (Figure 1), which has a clear nutrient gradient to test the theory on how nutrient enrichment influences variations of zooplankton size structure. We are mainly interested in the zooplankton size structure in the Xiangxi Bay and testing the effects of nutrient enrichment and water temperature on the size structure (size spectrum and size diversity). Specifically, the corresponding hypotheses are (H1) nutrient indirectly affects zooplankton size structure via phytoplankton according to the fundamental law of the planktonic food chain; (H2) water temperature increase will reduce size diversity and make a steeper size spectrum because warming will benefit small body size organisms in aquatic ecosystems; (H3) size diversity is a more robust metric indicating environment changes than the size spectrum in high dynamic reservoir ecosystems because the estimation of the size spectrum is affected by the statistical fitting.
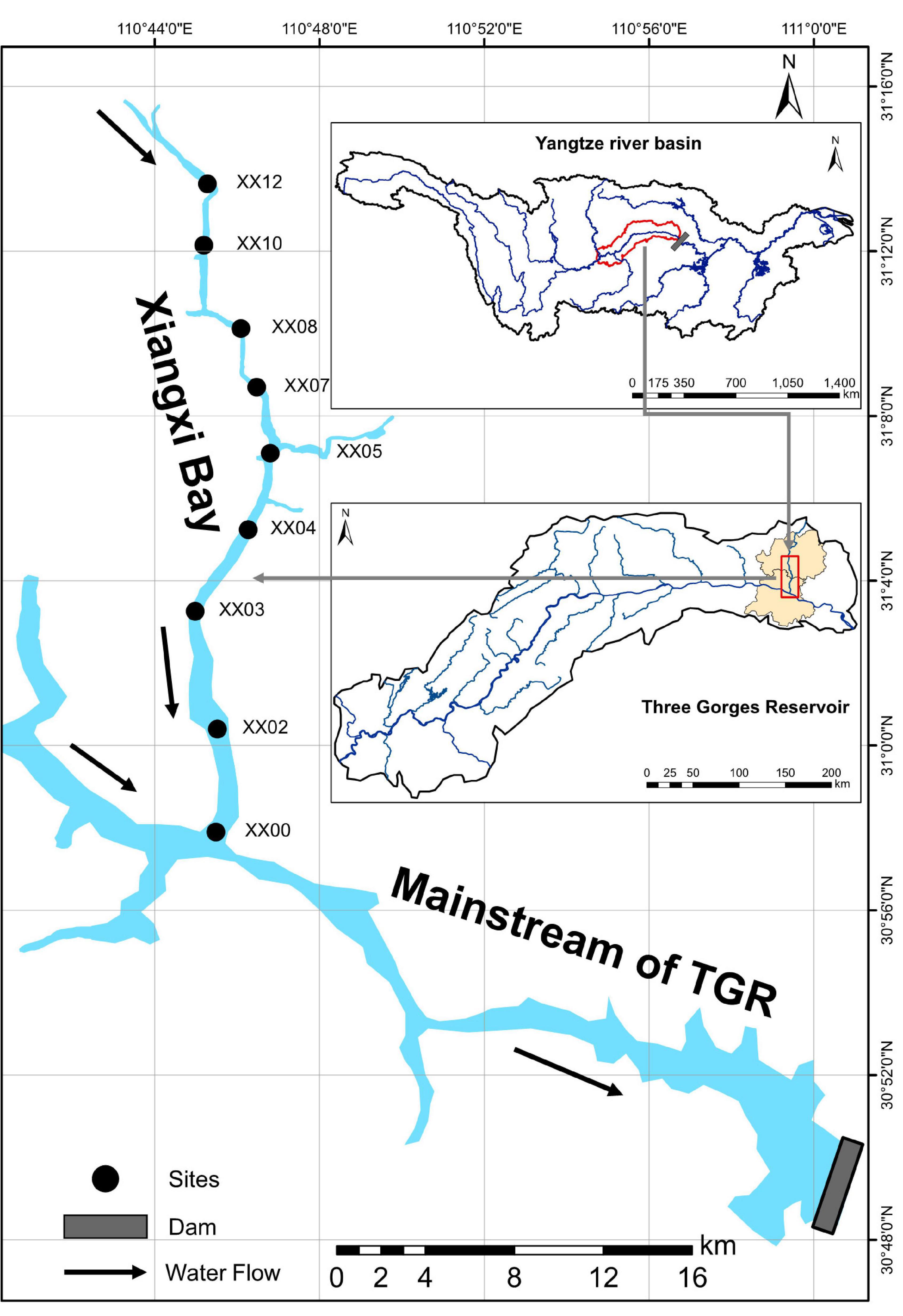
Figure 1. Spatial distribution of the sampling sites in the Xiangxi Bay of Three Gorges Reservoir. Arrows in the figure represent the direction of water flow. This map was created by QGIS (Ver. 3.20.3, https://qgis.org/en/site/).
Materials and Methods
Study Area and Field Sampling
The field study was carried out in the Xiangxi Bay of Three Gorges Reservoir (TGR) (Figure 1), which is the largest strategic freshwater resource reservoir in China, with a storage capacity of 39.3 × 109 m3 (Ye et al., 2016). The Xiangxi Bay, located about 32 km upstream of Three Gorges Dam, is the former Xiangxi River before the impoundment of Three Gorges Reservoir. After the TGR had been filled into the altitude of 175 m above sea level in October 2010, about 28 km downstream of the Xiangxi River was flooded and formed the bay zone. Previous research showed that this bay is facing severe eutrophication problems (Ye et al., 2014) with clear nitrogen and phosphorus gradients (Ye and Cai, 2011). This distinct environmental condition provided a good research area to test our hypothesis that zooplankton size structure will change along the nutrient gradients.
The field sampling was carried out monthly in the spring and summer (March to August) in the year 2014 because the abundance of zooplankton in these months is high enough to calculate a reliable size spectrum (Chen et al., 2020). A total of nine sites reflecting different trophic gradients for dissolved inorganic nitrogen, soluble reactive phosphorus, and dissolved silicate were distributed from the mouth (downstream) of Xiangxi Bay to its upstream (Figure 1). Due to the water level dropping in the summer (June to August), sites XX10 and XX12 became running water and were neglected in the field sampling in the summertime. The detailed information for each sampling site could be found in the checkerboard plot in the supplementary material (Supplementary Figure 1). Zooplankton samples were collected by filtering 20-L water sample with a plankton net (mesh size 60 μm) at each sampling site. Water samples were collected from the surface layer (0.5 m) of each site using a 5-L Van Dorn sampler. All filtered zooplankton samples were fixed and preserved in the formalin solution with a final concentration of 4% immediately.
The FlowCAM integrated system was used to obtain the community and size information of each zooplankton individual in all samples (Wong et al., 2017). In simple terms, the FlowCAM will automatically photograph the particles (zooplankton and non-zooplankton in the samples) that flow through the system and the size attributes (e.g., equivalent spherical diameter, ESD) can be extracted automatically. Based on the captured images, all particles in the sample were calculated and classified into different groups of rotifer, protozoan, cladoceran, copepod, zooplankton egg, and non-zooplankton particle by the taxonomic expert manually for the research. Finally, a total of 74,773 zooplankton individuals (images) were captured by the FlowCAM system in our study. Moreover, the abundance and biovolume for each zooplankton individual were calculated by a standard method provided by the FlowCAM. Details information could be found in the step-by-step procedure for FlowCAM based zooplankton samples processing in the supplementary information (Supplementary Figure 2).
At the same time, the water temperature, dissolved inorganic nitrogen, soluble reactive phosphorus, dissolved silicate, and chlorophyll a for each sample site were measured to analyze the potential environmental driving the zooplankton size structure in the Xiangxi Bay. Specifically, the water temperature (WT) was measured in situ by the multi-parameter water quality sonde (YSI 6600, United States). About 300 mL water sample in the surface layer (0.5 m in depth) of each site was collected for water chemistry analyses, including dissolved inorganic nitrogen (DIN, the sum of the ammonia nitrogen, nitrite nitrogen, and nitrate nitrogen), soluble reactive phosphorus (SRP), and dissolved silicate (DSi). The analyses for the above chemical variables were strictly referencing to the user manual of Skalar by the segmented flow analyzer (Skalar SAN++, Netherlands).
Meanwhile, the concentration of chlorophyll a (Chl-a) was used to estimate the phytoplankton biomass. To measure the concentration of Chl-a, 615 mL water sample collected in each site was filtered through a micro-filter (∼1.2 μm, GF/C Whatman) and stored in the dark at −20°C until the analysis. Before the analysis, filters were conducted in the laboratory with acetone solution with a final concentration of 90% for 24 h. The concentration of Chl-a was measured with a spectrophotometer (Shimadzu UV-1800, Japan) with the trichromatic method of APHA (1998).
Zooplankton Size Structure
The size spectrum and size diversity were used to quantify the zooplankton size structure. With the size data for zooplankton individuals (Supplementary Figure 2), the size spectrum of zooplankton community was estimated by the maximum likelihood estimation, which was reported as the most reliable method in fitting size spectrum (Edwards et al., 2017). Detail plots of the size spectrum estimations for all sites could be found in the supplementary material (Supplementary Figure 3). Size diversity was calculated by following García-Comas et al. (2014). Theoretically, size diversity (μ) is adapted from the Shannon–Wiener index that is commonly used for species diversity (Quintana et al., 2008; Ye et al., 2013):
Where, μ is the size diversity, and pi is the probability that zooplankter belongs to a specific size class. Following García-Comas et al. (2014), the width of each size class in calculating size diversity was doubled with respect to the previous one (geometric 2n series). Specifically, the biovolumes of zooplankton in our study ranged from 0.00011 to 0.62287 mm3, which was divided into 13 size classes for size diversity calculation (Supplementary Table 1).
Statistical Analysis
Structural equation model (SEM) was used to investigate the effects of environmental variables on zooplankton size structure and the affecting paths. SEM is a statistical technique used for analyzing structural relationships (DiLalla, 2000), and has a wide application in ecological and environmental research (Li et al., 2021). Based on the knowledge of the relationship between zooplankton and environmental variables, we assumed that water temperature and nutrient variables (DIN, SRP, and DSi) would affect the zooplankton community (abundance, NBSS slope, and size diversity) directly and indirectly by affecting the phytoplankton. To fit the requirement of the SEM, the zooplankton abundance and Chl-a were log2 transformed in the SEM analyses (Kang et al., 2013). The satisfactory model fit in SEMs was selected by the root of mean square error of approximation (RMSEA,≤0.05), the comparative fit index (CFI, ≥0.95), and the standardized root-mean-squared residual (SRMR,≤0.08), in which the contents in parentheses represent an acceptable range (Hu and Bentler, 1998, 1999). SEM analyses were performed in R (4.0.4) software (R Core Team, 2021) with the “lavaan” package (Rosseel, 2012). Meanwhile, the Mann-Whitney U-test (McKnight and Najab, 2010) was used to test the differences of zooplankton communities among different months.
Results
Environmental Factors
The Chl-a concentration ranged from 1.13 to 152.42 μg/L, with a mean value of 39.39 μg/L (Table 1). Water temperature ranged from 12.99 to 25.75°C (Table 1), with a gradual rise process from the early spring to later summer (Figure 2). The concentrations of DIN, SRP, and DSi during the research period ranged from 0.19 to 2.07 mg/L, 0.02 to 0.48 mg/L, and 0.07 to 3.28 mg/L (Table 1), respectively.

Table 1. Descriptive statistics of environmental factors measured in the Xiangxi Bay of Three Gorges Reservoir.
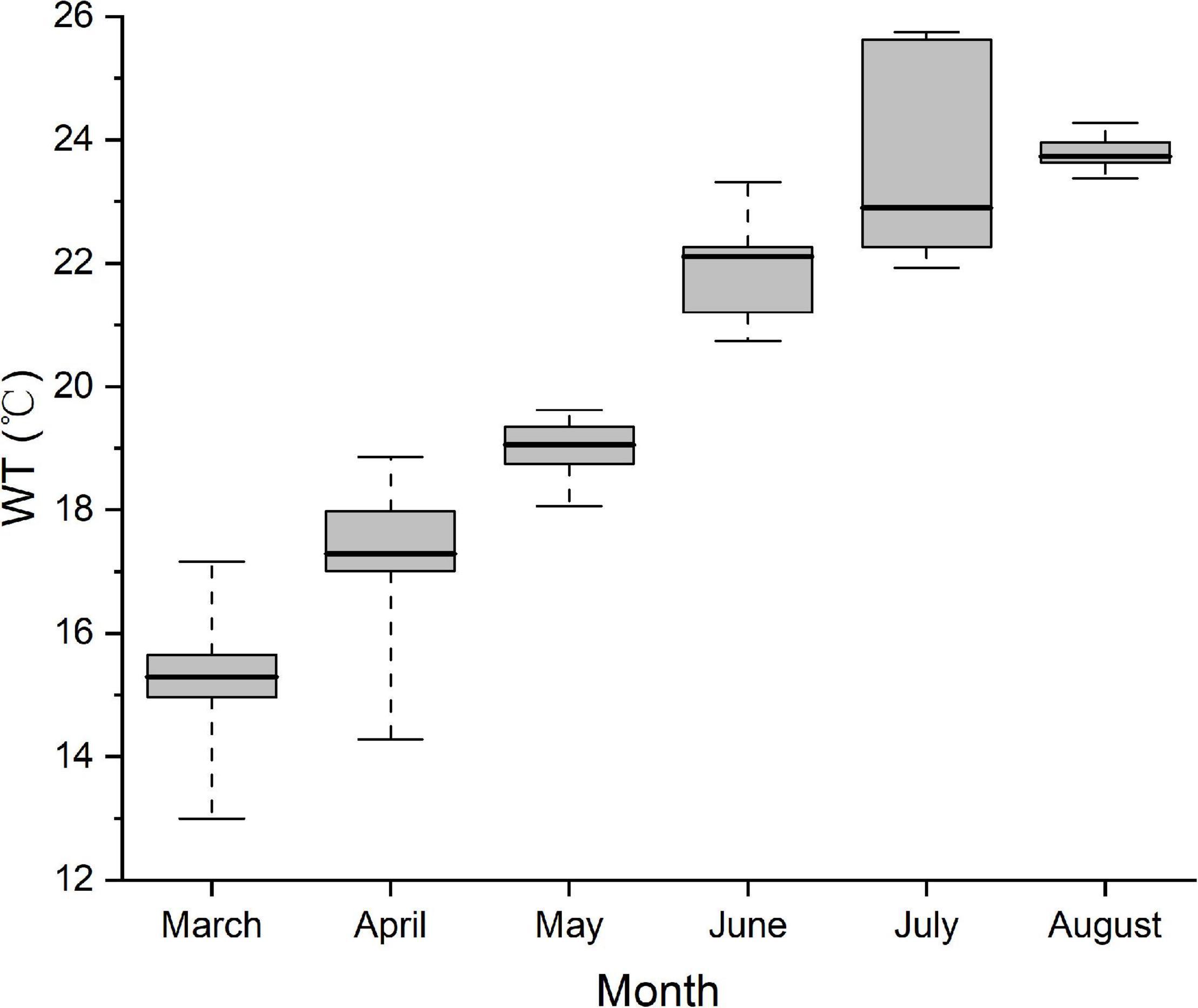
Figure 2. Boxplots showing the spatiotemporal variation of water temperature in the Xiangxi Bay of Three Gorges Reservoir.
Boxplot showed that a clear spatial pattern of environmental variables in the Xiangxi Bay (Figure 3). Specifically, a high concentration of DIN was observed in the mouth of the bay, and the concentration of DIN decreased from the mouth to the upstream of the bay (Figure 3A). The average concentration of DIN in the mouth (XX00) and upstream (XX12) were 1.65 and 0.76 mg/L. On the contrary, the SRP had the reverse spatial pattern comparing to the DIN. That is, a low concentration of SRP was observed in the mouth of the bay, and the concentration of SRP increased from the mouth to the upstream of the bay (Figure 3B). The average concentration of SRP in the mouth (XX00) and upstream (XX12) were 0.07 and 0.28 mg/L. The DSi had a similar spatial pattern with DIN in the Xiangxi Bay (Figure 3C).
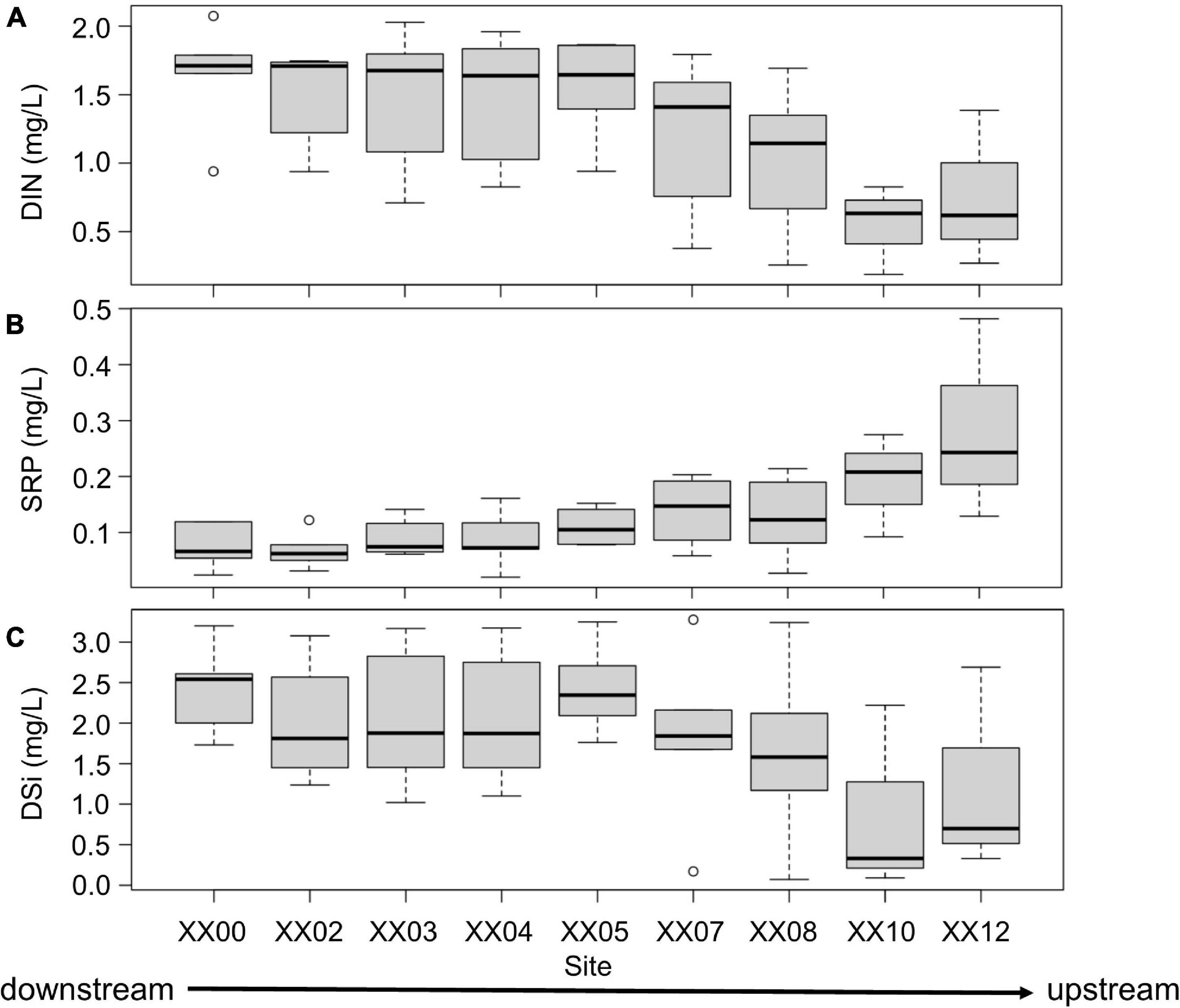
Figure 3. Boxplots showing the spatiotemporal variation of DIN (A), SRP (B), and DSi (C) in the Xiangxi Bay of Three Gorges Reservoir. The sites were arranged by the distance to the mouth of the bay (XX00).
Zooplankton Composition and Size Structure
We found that rotifer is the predominant zooplankton group in the Xiangxi bay in the whole research period (Figure 4). The mean abundance of rotifer among all sites is 327.5 ind./L, which is significantly higher than protozoan (mean abundance is 36.2 ind./L), cladoceran (7.3 ind./L), and copepod (22.0 ind./L) by the Mann–Whitney U-tests (all the tests have met the result of P < 0.001). The high abundance of rotifer was observed in March and April, with the highest value of 2,862.8 ind./L observed in XX05 in April. The high abundance of protozoan was generally observed in the region from the mouth of Xiangxi Bay (XX00) to the middle reach (XX05) in March and May (Figure 4). Copepod had a high relative abundance in the summer (June, July, and August), and cladoceran had a low abundance in the whole research period.
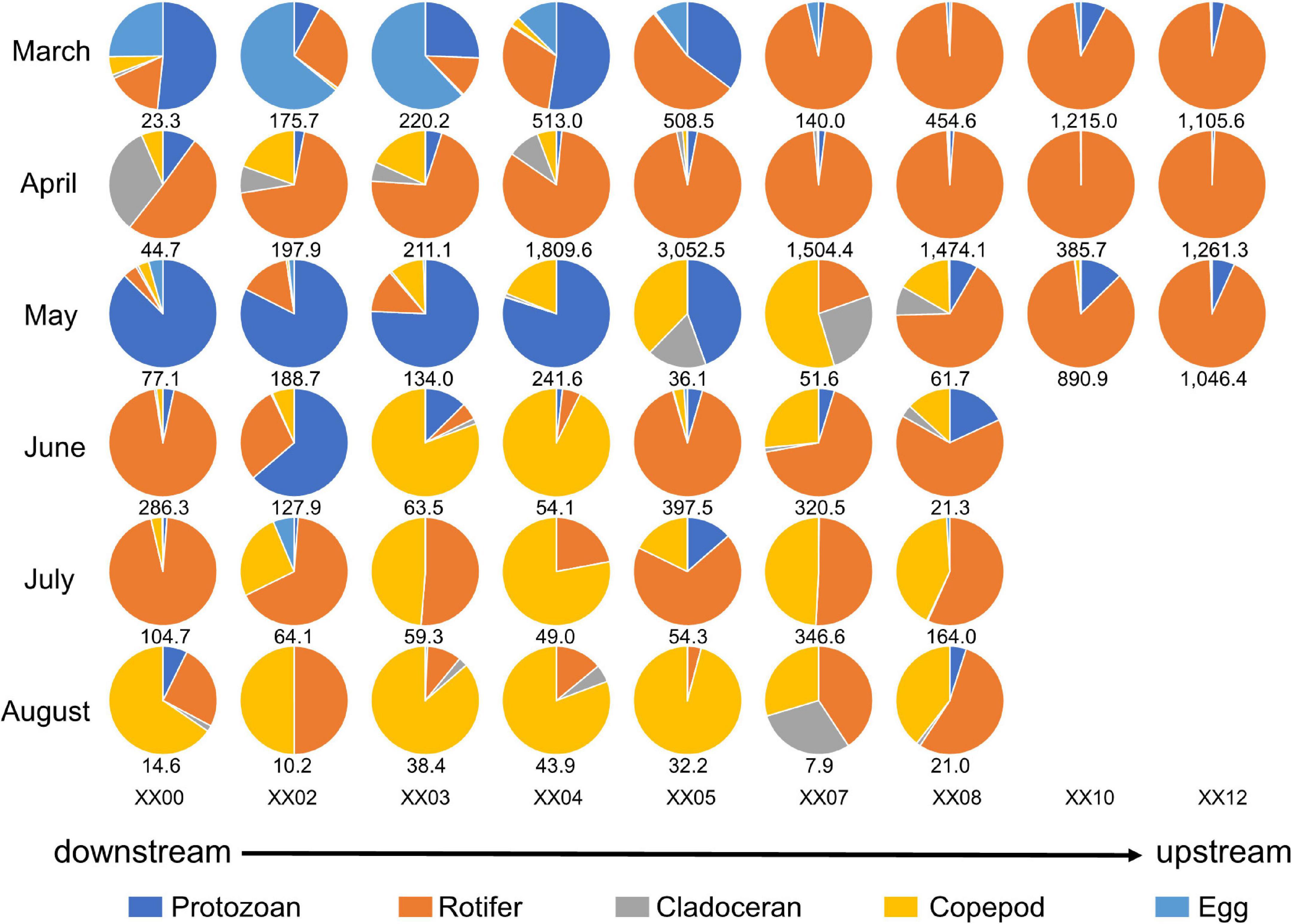
Figure 4. Relative abundance of zooplankton groups in the Xiangxi Bay of Three Gorges Reservoir. Numbers under each pie represent the total abundance (ind./L) for the site.
The estimated size spectra by the maximum likelihood estimation method ranged from −3.373 to −0.984 during the research period (Figure 5A). In spatial variations, the lower size spectra were generally observed in the upstream sites XX10 and XX12, with an average value of −2.462 and −2.761. The average values of size spectra for the downstream sites XX00 and XX02 were −1.474. The size diversity ranged from 0.631 to 3.291 (Figure 5B). The highest value of size diversity was observed in XX07 in May (3.291), and the lowest value was observed in XX12 in April (0.631). The spatial pattern of size diversity was similar to the size spectra (Figure 5B). That is, lower values of size diversity were generally observed in upstream sites XX10 and XX12, with an average value of 1.373 and 1.134. And the average values for the downstream site XX00 and XX02 were 2.414 and 2.292. In temporal dynamics, lower values of size spectra were generally observed in March and April, with mean values of −1.805 and −1.870 (Figure 5A); nevertheless, low values of size diversity were generally observed in March and April, with mean values of 1.963 and 2.015 (Figure 5B).
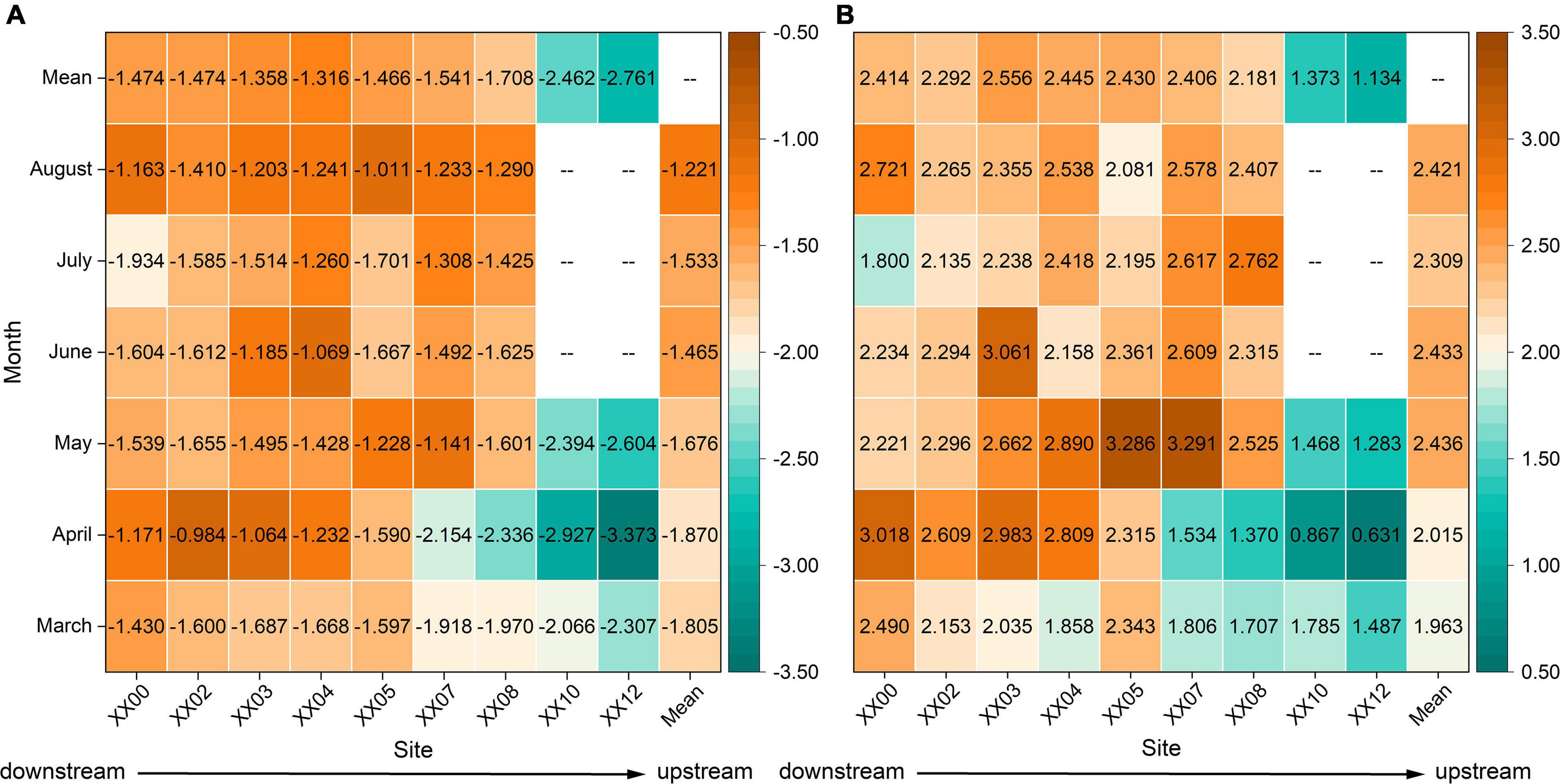
Figure 5. Spatiotemporal variations of zooplankton size spectrum (A), and zooplankton size diversity (B) in the Xiangxi Bay of Three Gorges Reservoir.
Factors Driving Zooplankton Abundance and Size Structure
Our study showed that the SEM achieved an acceptable fit for the spatiotemporal variations of zooplankton size structure (Figure 6). The explanation power of SEM for the zooplankton abundance, size spectrum, size diversity were 60, 66, and 57%. SEMs found that DIN had an indirect effect on the zooplankton abundance, size spectrum, and size diversity by affecting the concentration of Chl-a. The coefficient between DIN and Chl-a was −0.90 (P < 0.001). The coefficient of Chl-a with zooplankton abundance, size spectrum, and size diversity was 0.22 (P = 0.083), −0.38 (P = 0.001), and −0.45 (P = 0.001). We also found that water temperature had a significant negative effect on the size diversity but no significant effects on the zooplankton abundance and size spectrum (Figure 6).
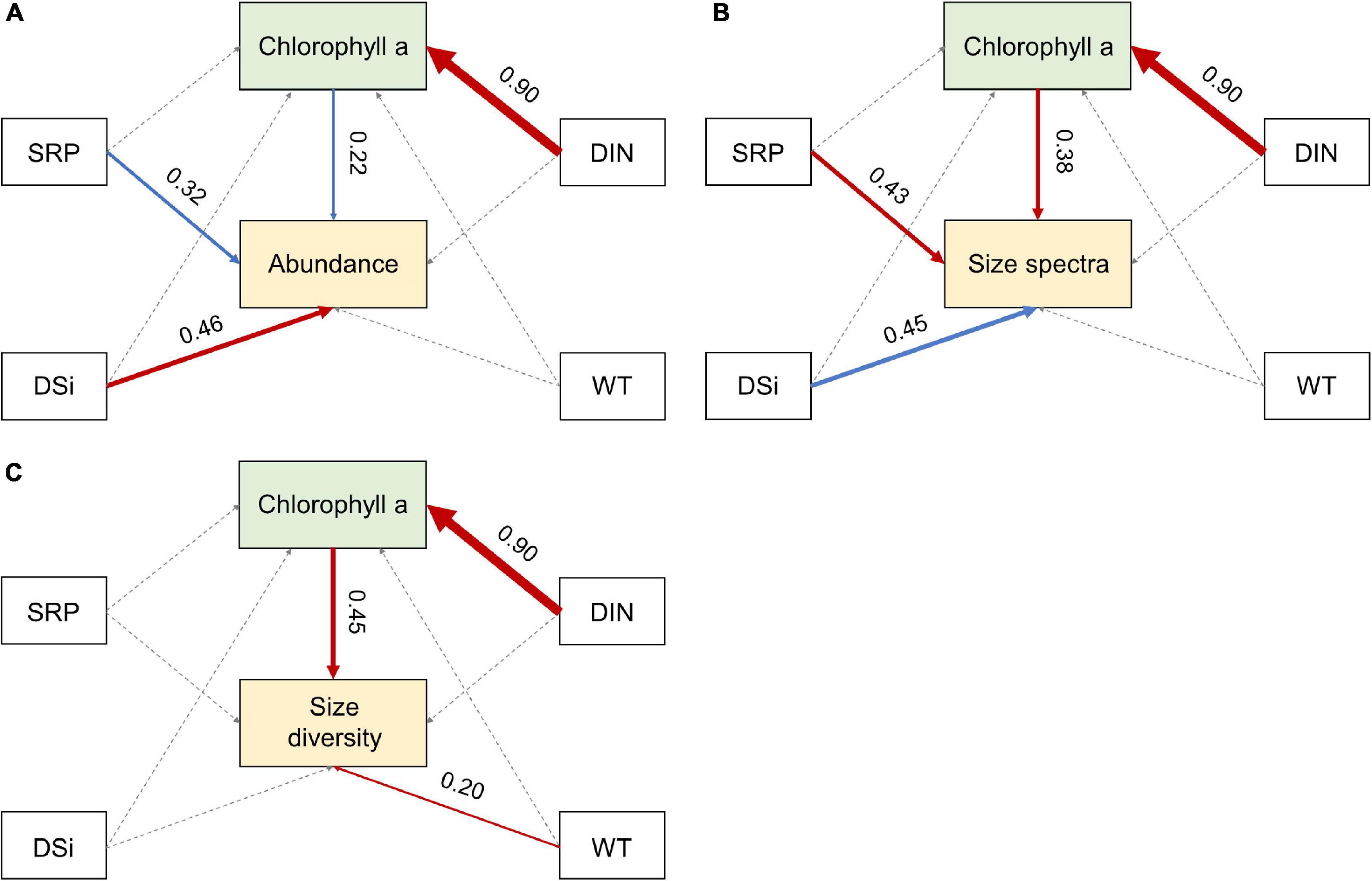
Figure 6. Result of SEM for the direct and indirect effects of the selected environmental variables on zooplankton community (A) Abundance, (B) Size spectra, (C) Size diversity. The red solid arrows indicate the negative effects, blue solid arrows indicate the positive effects, and the dashed gray arrows represent non-significant effects. The width of arrows is weighted according to standardized path coefficients shown above the arrow.
Discussion
Zooplankton Groups and Size Structure
Our study shows that the small body size zooplankton rotifer was the dominant group in the Xiangxi Bay of TGR (Figure 4). This finding is widely observed in reservoirs because of the high reproduction rate of rotifers and their adaptability to the environment (Segers, 2008; Gu et al., 2021). However, because the swimming ability of planktonic rotifers was significantly weaker than that of copepods and cladocerans, the growth of rotifers will be inhibited in an environment with fast water exchange rate or high flow velocity (Holst et al., 1998). This may be the reason explaining why the relative abundance of rotifer decreased in the summertime (June, July, and August) because of the strong hydrological disturbances. For flood control, the water level of TGR needs to be reduced to 145 m above sea level in June (Zhao et al., 2017). As a comprehensive effect of a large amount of inflow water and water level control in the flood season, the water exchange rate in the summertime is very high (Zhao et al., 2017). Therefore, the relative abundance of rotifer decreased in the summertime because of the strong hydrological disturbances.
For the size structure, the average size spectra in our study is −1.619 and the size spectra of most sites in our study are more explanate than the theoretical slope (−2.0) of the maximum likelihood estimation value (Edwards et al., 2017). In terms of the spatial distribution, the upstream sites have a steeper size spectrum (Figure 5A), which is similarly to the spatial pattern of zooplankton communities in the Pearl River estuary in the dry season (Ke et al., 2018). The phenomenon is mainly because the upstream areas are vulnerable to hydrological disturbances and anthropogenic activities, which generally lead to a steeper size spectrum (Dickerson et al., 2010; Ma et al., 2014). Comparing to Brucet et al. (2010), we found that the zooplankton size diversity in the Xiangxi Bay is slightly higher than in some European lakes. This suggests that the zooplankton community in the Xiangxi Bay has relatively high efficiency in grazing phytoplankton because higher zooplankton size diversity is supposed to have a stronger predation effect on phytoplankton (Ye et al., 2013).
How Nutrients Affecting Zooplankton Community
The SEMs showed a clear pathway that how nutrient variables affect the zooplankton size structure with acceptable explanation powers. For the SEMs in our study, the explanation powers for the abundance, size spectrum, and size diversity are similar to the explanation power of the SEMs in explaining the chlorophyll a concentration in reservoir bays (Li et al., 2021). One interesting result of our study is that SEMs found that DIN can affect the zooplankton abundance and size structure by influencing the concentration of Chl-a (Figure 6). This finding provided a mechanistic understanding of how environmental factors affect the zooplankton community in Xiangxi Bay of TGR. We found that DIN had a significant negative correlation with the Chl-a concentration, suggesting that the growth of phytoplankton will uptake the nitrogen and therefore decreased the DIN concentration. Actually, the consumption of nitrogen by phytoplankton was usually observed in the Xiangxi Bay (Ye et al., 2007) and other water bodies (Trommer et al., 2019). As the primary food source for zooplankton, phytoplankton can support the standing stock of zooplankton (Yuan and Pollard, 2018). Therefore, we observed a significant positive relationship between Chl-a concentration and zooplankton abundance in the Xiangxi Bay.
In contrast, we found that the sites with higher concentrations of Chl-a tended to have lower size diversity and steeper size spectra (negative relationships in Figure 6). To explain this opposite finding, we investigated the effects of increasing of Chl-a on the abundance of different groups of zooplankton (Table 2). Interestingly, we found that the increased Chl-a only promotes the abundance of parts of small body-size zooplankters. This suggests that high phytoplankton abundance will promote zooplankton reproduction, further improve the abundance of small body size zooplankters and result in a steep size spectrum and low size diversity. A previous study carried out in the East China Sea also found a similar pattern that increasing food availability increased zooplankton abundance, and more importantly, this increase was due to small body size zooplankton (García-Comas et al., 2014).

Table 2. The mean equivalent spherical diameter (ESD) of each zooplankton group and the results of linear regression analyses between different zooplankton groups and phytoplankton biomass (chlorophyll a) in the Xiangxi Bay of Three Gorges Reservoir.
Meanwhile, SEMs showed that SRP and DSi had direct correlations with zooplankton abundance and size spectrum (Figure 6). Here, we suspected that the direct correlations of the nutrient variables with zooplankton abundance and size spectrum in our study were probably spurious correlations because many research indicated that the effects of nutrients on zooplankton are mainly through the indirect effect of phytoplankton (Li et al., 2019; Lorenz et al., 2019; Trommer et al., 2019).
Size Diversity Is a Good Indicator for Environmental Change
It is worth mentioning that our study found that size diversity is more sensitive to temperature changes than the size spectrum (Figure 6). Our study suggests that zooplankton size diversity is a good indicator for environmental change related research in high dynamic reservoir ecosystems. Despite the widespread use of the size spectrum as the primary indicator of size structure, we found that the size spectrum had a non-significant relationship with the water temperature in the Xiangxi bay. This non-significant relationship could be attributed to the size distribution in some sites were nonlinear (García-Comas et al., 2014), and the statistical fitting of the size spectrum may bring some uncertainties. Thus, the application of the size spectrum in quantifying the size structure of dynamic ecosystems should be aware of the nonlinearity. On the contrary, size diversity measures the continuous analog of size structure (Ye et al., 2013). For this reason, whether the zooplankton community is stable or not will not affect the accuracy in estimating size diversity. Therefore, we suggested that size diversity is a robust index in measuring the size structure.
Conclusion
In this study, we reported the zooplankton size structure and its relationship with the environmental factors in the Xiangxi Bay of TGR. We found that both the size spectra and size diversity had a high spatiotemporal dynamic in the Xiangxi Bay. We further investigated how environmental factors drive the zooplankton size structure by using SEM. Specifically, SEMs revealed a clear pathway that DIN had an indirect effect on the zooplankton abundance, size spectrum, and size diversity through affecting the Chl-a, supporting our hypothesis that nutrient indirectly affects zooplankton size structure via phytoplankton. Besides, SEM found that water temperature has a significant relationship only with the size diversity, but not zooplankton abundance or size spectrum. This finding suggests that size diversity is a robust size structure metric in dynamic aquatic ecosystems. Our study provided some insights into zooplankton size structure and its relationship with environmental factors in dynamic reservoir ecosystems, which can guide the ecological monitoring and research in reservoirs as well as other similar water bodies.
Data Availability Statement
The datasets used and/or analyzed during the current study are available from the corresponding author on reasonable request.
Author Contributions
HL was responsible for the analyses and interpretation of the data, and wrote the original draft. YG conducted the field investigation and sample analysis. QC was responsible for the conception. XD provided the methodology and conducted the sample analysis. LY was responsible for administration and supervision of the project, writing – review and editing, and contributed to the conception. All authors have read and approved the final manuscript.
Funding
This study was supported by the National Key R&D Program of China (2018YFD0900902), the National Natural Science Foundation of China (31670534, 31300392), and the State Key Laboratory FEBL Research Grant (2016FBZ10).
Conflict of Interest
XD was employed by the company Beijing Osees Technology Co., Limited.
The remaining authors declare that the research was conducted in the absence of any commercial or financial relationships that could be construed as a potential conflict of interest.
Publisher’s Note
All claims expressed in this article are solely those of the authors and do not necessarily represent those of their affiliated organizations, or those of the publisher, the editors and the reviewers. Any product that may be evaluated in this article, or claim that may be made by its manufacturer, is not guaranteed or endorsed by the publisher.
Acknowledgments
We thank the students and staff in the Xiangxi River Ecosystem Station for their help in the field sampling. And we also thank Lu Tan for performing the chemical analysis of the water samples used in this study.
Supplementary Material
The Supplementary Material for this article can be found online at: https://www.frontiersin.org/articles/10.3389/fevo.2022.800025/full#supplementary-material
References
APHA (1998). Standard Methods for the Examination of Water and Wastewater, 20th Edn. Washington: American Water Works Association and Water Environment Federation.
Brucet, S., Boix, D., Quintana, X. D., Jensen, E., Nathansen, L. W., Trochine, C., et al. (2010). Factors influencing zooplankton size structure at contrasting temperatures in coastal shallow lakes: implications for effects of climate change. Limnol. Oceanogr. 55, 1697–1711. doi: 10.4319/lo.2010.55.4.1697
Chen, X., Ye, L., Tan, L., and Cai, Q. H. (2020). Crustacean zooplankton community in Xiangxi Bay during the later impoundment period of the Three Gorges Reservoir. Acta Hydrobiol. Sin. 44, 1070–1079. doi: 10.7541/2020.124
Daufresne, M., Lengfellner, K., and Sommer, U. (2009). Global warming benefits the small in aquatic ecosystems. Proc. Natl. Acad. Sci. U. S. A. 106, 12788–12793. doi: 10.1073/pnas.0902080106
Davis, J. M., Rosemond, A. D., Eggert, S. L., Cross, W. F., and Wallace, J. B. (2010). Long-term nutrient enrichment decouples predator and prey production. Proc. Natl. Acad. Sci. U. S. A. 107, 121–126. doi: 10.1073/pnas.0908497107
Dickerson, K. D., Medley, K. A., and Havel, J. E. (2010). Spatial variation in zooplankton community structure Is related to hydrologic flow units in the Missouri River, USA. River Res. Appl. 26, 605–618. doi: 10.1002/rra.1268
DiLalla, L. F. (2000). “Structural equation modeling: uses and issues,” in Handbook of Applied Multivariate Statistics And Mathematical Modeling, eds E. A. T. Howard and D. B. Steven (Salt Lake City: Academic Press), 439–464.
Eddy, T. D., Bernhardt, J. R., Blanchard, J. L., Cheung, W. W. L., Colleter, M., du Pontavice, H., et al. (2021). Energy flow through marine ecosystems: confronting transfer efficiency. Trends Ecol. Evol. 36, 76–86. doi: 10.1016/j.tree.2020.09.006
Edwards, A. M., Robinson, J. P. W., Plank, M. J., Baum, J. K., and Blanchard, J. L. (2017). Testing and recommending methods for fitting size spectra to data. Methods Ecol. Evol. 8, 57–67. doi: 10.1111/2041-210x.12641
Fenchel, T. (1988). Marine plankton food chains. Ann. Rev. Ecol. Syst. 19, 19–38. doi: 10.1146/annurev.es.19.110188.000315
Fuchs, H. L., and Franks, P. J. S. (2010). Plankton community properties determined by nutrients and size-selective feeding. Mar. Ecol. Prog. Ser. 413, 1–15. doi: 10.3354/meps08716
Gao, X. F., Chen, H. H., Govaert, L., Wang, W. P., and Yang, J. (2019). Responses of zooplankton body size and community trophic structure to temperature change in a subtropical reservoir. Ecol. Evol. 9, 12544–12555. doi: 10.1002/ece3.5718
García-Comas, C., Chang, C. Y., Ye, L., Sastri, A. R., Lee, Y. C., Gong, G. C., et al. (2014). Mesozooplankton size structure in response to environmental conditions in the East China Sea: how much does size spectra theory fit empirical data of a dynamic coastal area? Prog. Oceanogr. 121, 141–157. doi: 10.1016/j.pocean.2013.10.010
Gu, Y., Cai, Q. H., Tan, L., Li, B., Ju, S. S., and Ye, L. (2021). Taxonomic and functional diversity of planktonic rotifers along a phosphorus gradient in the Three Gorges Reservoir, China. Fresenius Environ. Bull. 30, 1687–1695.
Hessen, D. O., Jensen, T. C., and Walseng, B. (2019). Zooplankton diversity and dispersal by birds; insights from different geographical scales. Front. Ecol. Evol. 7:74. doi: 10.3389/fevo.2019.00074
Holst, H., Zimmermann, H., Kausch, H., and Koste, W. (1998). Temporal and spatial dynamics of planktonic rotifers in the Elbe Estuary during spring. Estuar. Coast. Shelf Sci. 47, 261–273. doi: 10.1006/ecss.1998.0364
Hu, L. T., and Bentler, P. M. (1998). Fit indices in covariance structure modeling: sensitivity to underparameterized model misspecification. Psychol. Methods 3, 424–453. doi: 10.1037/1082-989X.3.4.424
Hu, L. T., and Bentler, P. M. (1999). Cutoff criteria for fit indexes in covariance structure analysis: conventional criteria versus New Alternatives. Struct. Equ. Modeling 6, 1–55. doi: 10.1080/10705519909540118
Kang, M. Y., Dai, C., Ji, W. Y., Jiang, Y., Yuan, Z. Y., and Chen, H. Y. H. (2013). Biomass and Its allocation in relation to temperature, precipitation, and soil nutrients in inner Mongolia Grasslands, China. PLoS One 8:e69561. doi: 10.1371/journal.pone.0069561
Ke, Z. X., Tan, Y. H., Huang, L. M., Liu, J. X., and Liu, H. X. (2018). Community structure and biovolume size spectra of mesozooplankton in the Pearl River estuary. Aquat. Ecosyst. Health Manage. 21, 30–40. doi: 10.1080/14634988.2018.1432948
Lee, P. W., Hsiao, S. H., Chou, C., Tseng, L. C., and Hwang, J. S. (2021). Zooplankton Fluctuations in the Surface Waters of the Estuary of a Large Subtropical Urban River. Front. Ecol. Evol. 9:598274. doi: 10.3389/fevo.2021.598274
Li, N. X., Wang, J., Yin, W., Jia, H. Y., Xu, J. F., Hao, R., et al. (2021). Linking water environmental factors and the local watershed landscape to the chlorophyll a concentration in reservoir bays. Sci. Total Environ. 758:143617. doi: 10.1016/j.scitotenv.2020.143617
Li, Y., Liu, L., Cui, S. Z., and Chen, F. Z. (2019). Long-term effects of nutrient changes on rotifer communities in a subtropical lake. Limnology 20, 191–201. doi: 10.1007/s10201-018-0567-x
Lorenz, P., Trommer, G., and Stibor, H. (2019). Impacts of increasing nitrogen:phosphorus ratios on zooplankton community composition and whitefish (Coregonus macrophthalmus) growth in a pre-alpine lake. Freshw. Biol. 64, 1210–1225. doi: 10.1111/fwb.13296
Ma, Y. E., Ke, Z. X., Huang, L. M., and Tan, Y. H. (2014). Identification of human-induced perturbations in Daya Bay, China: evidence from plankton size structure. Cont. Shelf Res. 72, 10–20. doi: 10.1016/j.csr.2013.10.012
McKnight, P. E., and Najab, J. (2010). “Mann-Whitney U Test,” in The Corsini Encyclopedia of Psychology, eds I. B. Weiner and W. E. Craighead (New Jersey: John Wiley & Sons Inc.).
Peng, L., Xue, X. G., Liao, J., Zhao, J., Tang, Q. H., Lin, Q. Q., et al. (2021). Potential impact of population increases of non-native tilapia on fish catch and plankton structure: a case study of Tangxi Reservoir in southern China. Aquat. Invasions 16, 329–348. doi: 10.3391/ai.2021.16.2.08
Quintana, X. D., Brucet, S., Boix, D., López-Flores, R., Gascón, S., Badosa, A., et al. (2008). A nonparametric method for the measurement of size diversity with emphasis on data standardization. Limnol. Oceanogr. Methods 6, 75–86. doi: 10.4319/lom.2008.6.75
Rizo, E. Z. C., Liu, P., Niu, H. Y., Yang, Y., Lin, Q. Q., Papa, R. D. S., et al. (2020). Zooplankton in a continuous waterscape: environmental and spatial factors shaping spring zooplankton community structure in a large canyon reservoir at the tropic of cancer. Hydrobiologia 847, 3621–3635. doi: 10.1007/s10750-020-04380-1
Rosseel, Y. (2012). lavaan: an R package for structural equation modeling. J. Stat. Softw. 48, 1–36. doi: 10.18637/jss.v048.i02
Segers, H. (2008). Global diversity of rotifers (Rotifera) in freshwater. Hydrobiologia 595, 49–59. doi: 10.1007/s10750-007-9003-7
Shen, H. L., Li, B., Cai, Q. H., Han, Q. X., Gu, Y., and Qu, Y. M. (2014). Phytoplankton functional groups in a high spatial heterogeneity subtropical reservoir in China. J. Great Lakes Res. 40, 859–869. doi: 10.1016/j.jglr.2014.09.007
Sprules, W. G., and Barth, L. E. (2016). Surfing the biomass size spectrum: some remarks on history, theory, and application. Can. J. Fish. Aquat. Sci. 73, 477–495. doi: 10.1139/cjfas-2015-0115
Stouffer, D. B., Rezende, E. L., and Amaral, L. A. N. (2011). The role of body mass in diet contiguity and food-web structure. J. Anim. Ecol. 80, 632–639. doi: 10.1111/j.1365-2656.2011.01812.x
Straškraba, M., and Tundisi, J. G. (1999). Guidelines of Lake Management (Volume 9): Reservoir Water Quality Management. Kusatsu: International Lake Environment Committee Foundation.
Toruan, R. L., Coggins, L. X., and Ghadouani, A. (2021). Response of zooplankton size structure to multiple stressors in urban lakes. Water 13:2305. doi: 10.3390/w13162305
Trommer, G., Lorenz, P., Lentz, A., Fink, P., and Stibor, H. (2019). Nitrogen enrichment leads to changing fatty acid composition of phytoplankton and negatively affects zooplankton in a natural lake community. Sci. Rep. 9:16805. doi: 10.1038/s41598-019-53250-x
Venello, T. A., Sastri, A. R., Galbraith, M. D., and Dower, J. F. (2021). Zooplankton functional group responses to environmental drivers off the west coast of Vancouver Island, Canada. Prog. Oceanogr. 190:102482. doi: 10.1016/j.pocean.2020.102482
Verberk, W. C. E. P., Atkinson, D., Hoefnagel, K. N., Hirst, A. G., Horne, C. R., and Siepel, H. (2021). Shrinking body sizes in response to warming: explanations for the temperature-size rule with special emphasis on the role of oxygen. Biol. Rev. 96, 247–268. doi: 10.1111/brv.12653
Whitman, R. L., Nevers, M. B., Goodrich, M. L., Murphy, P. C., and Davis, B. M. (2004). Characterization of Lake Michigan coastal lakes using zooplankton assemblages. Ecol. Indic. 4, 277–286. doi: 10.1016/j.ecolind.2004.08.001
Wong, E., Sastri, A. R., Lin, F. S., and Hsieh, C. H. (2017). Modified FlowCAM procedure for quantifying size distribution of zooplankton with sample recycling capacity. PLoS One 12:e0175235. doi: 10.1371/journal.pone.0175235
Xu, H., Yan, M., Long, L. H., Ma, J., Ji, D. B., Liu, D. F., et al. (2021). Modeling the effects of hydrodynamics on thermal stratification and algal blooms in the xiangxi bay of three gorges reservoir. Front. Ecol. Evol. 8:610622. doi: 10.3389/fevo.2020.610622
Ye, L., and Cai, Q. H. (2011). Spring phytoplankton blooms in xiangxi bay of three-gorges reservoir: spatiotemporal dynamics across sharp nutrient gradients. J. Freshw. Ecol. 26, 11–18. doi: 10.1080/02705060.2011.553815
Ye, L., Cai, Q. H., Zhang, M., and Tan, L. (2014). Real-time observation, early warning and forecasting phytoplankton blooms by integrating in situ automated online sondes and hybrid evolutionary algorithms. Ecol. Inform. 22, 44–51. doi: 10.1016/j.ecoinf.2014.04.001
Ye, L., Cai, Q. H., Zhang, M., Tan, L., and Shen, H. L. (2016). Ecosystem metabolism and the driving factors in Xiangxi Bay of Three Gorges Reservoir, China. Freshw. Sci. 35, 826–833. doi: 10.1086/687205
Ye, L., Chang, C. Y., Garcia-Comas, C., Gong, G. C., and Hsieh, C. H. (2013). Increasing zooplankton size diversity enhances the strength of top-down control on phytoplankton through diet niche partitioning. J. Anim. Ecol. 82, 1052–1060. doi: 10.1111/1365-2656.12067
Ye, L., Han, X. Q., Xu, Y. Y., and Cai, Q. H. (2007). Spatial analysis for spring bloom and nutrient limitation in Xiangxi Bay of Three Gorges Reservoir. Environ. Monit. Assess. 127, 135–145. doi: 10.1007/s10661-006-9267-9
Yuan, L. L., and Pollard, A. I. (2018). Changes in the relationship between zooplankton and phytoplankton biomasses across a eutrophication gradient. Limnol. Oceanogr. 63, 2493–2507. doi: 10.1002/lno.10955
Yvon-Durocher, G., Montoya, J. M., Trimmer, M., and Woodward, G. (2011). Warming alters the size spectrum and shifts the distribution of biomass in freshwater ecosystems. Glob. Change Biol. 17, 1681–1694. doi: 10.1111/j.1365-2486.2010.02321.x
Keywords: body size, size spectrum, size diversity, environmental gradient, three gorges reservoir
Citation: Li H, Gu Y, Cai Q, Dong X and Ye L (2022) Zooplankton Size Structure in Relation to Environmental Factors in the Xiangxi Bay of Three Gorges Reservoir, China. Front. Ecol. Evol. 10:800025. doi: 10.3389/fevo.2022.800025
Received: 22 October 2021; Accepted: 05 January 2022;
Published: 10 February 2022.
Edited by:
Arnaud Sentis, Institut National de recherche pour l’agriculture, l’alimentation et l’environnement (INRAE), FranceReviewed by:
Jessica Garzke, University of British Columbia, CanadaJianjun Wang, Nanjing Institute of Geography and Limnology, Chinese Academy of Sciences (CAS), China
Yaoyang Xu, Institute of Urban Environment, Chinese Academy of Sciences (CAS), China
Copyright © 2022 Li, Gu, Cai, Dong and Ye. This is an open-access article distributed under the terms of the Creative Commons Attribution License (CC BY). The use, distribution or reproduction in other forums is permitted, provided the original author(s) and the copyright owner(s) are credited and that the original publication in this journal is cited, in accordance with accepted academic practice. No use, distribution or reproduction is permitted which does not comply with these terms.
*Correspondence: Lin Ye, yelin@ihb.ac.cn