- 1Chubu University Academy of Emerging Sciences, Kasugai, Japan
- 2Wildlife Research Center of Kyoto University, Kyoto, Japan
- 3Japan Monkey Centre, Inuyama, Japan
- 4Institute for Tropical Biology and Conservation, Universiti Malaysia Sabah, Kota Kinabalu, Malaysia
- 5Primate Research Institute, Kyoto University, Inuyama, Japan
- 6School of Human Sciences, Sugiyama Jogakuen University, Nisshin, Japan
- 7Department of Zoology, Entomology and Fisheries Sciences, College of Natural Sciences, Makerere University, Kampala, Uganda
- 8Clinic of Zoo Animals, Exotic Pets and Wildlife, Vetsuisse Faculty, University of Zurich, Zurich, Switzerland
- 9Department of Animal Sciences, University of Göttingen, Göttingen, Germany
Free-ranging animals make dietary choices that affect their nutritional status and, ultimately, their health and fitness. We investigated food selection by a leaf-eating foregut-fermenting primate, the guereza (Colobus guereza), using multiple criteria, including chemical and mechanical properties, in vitro digestibility and leaf abundance, on the basis of 30 consecutive months of behavioral observations (4308 h in total) of a family group in the Kalinzu Forest, Uganda, as well as vegetation surveys. We noted that leaf toughness may be a proximate cue for the chemical properties of plant foods, especially for protein, which is an important selection factor used by primates. We also found that the in vitro digestibility of plant foods was greatly influenced by the concentrations of fiber and secondary compounds. At a broad level, none of the studied factors, including leaf chemical and mechanical properties, digestibility and abundance, affected whether guerezas consumed specific leaf items. At a more detailed level, however, protein content, digestibility and toughness were related to the percentage of foraging effort that guerezas devoted to specific items in our study site.
Introduction
Tropical forests offer herbivores a variety of potential food sources. Therefore, to meet their nutritional needs and maintain their health and fitness, herbivores must select foods on the basis of criteria such as their chemical and mechanical properties, weighed against the availability of food resources. Since the variety of plant species eaten by herbivores may vary substantially in terms of nutritional content, toughness, secondary compounds, abundance, and digestibility (Janzen, 1973; Crawley, 1983; Onoda et al., 2011), many studies have reported some degree of herbivore food selectivity (Hughes, 1990; Cassini, 1994; Iason and Villalba, 2006). Thus, over the past half century, a considerable amount of knowledge has accumulated with respect to the dietary choices of herbivores, though the same species have often been found to use different dietary choice criteria under different environmental conditions (Cassini, 1994; Ganzhorn et al., 2017); this suggests that further empirical research that evaluates this flexibility is worthwhile.
Foregut-fermenting primates, the colobines, are a group of Afroeurasian monkeys that include over 70 species that are widely distributed throughout Asia and Africa. Their foregut fermentation system features a multi-chambered stomach where a commensal microbiome digests plant cell walls and can detoxify defensive plant chemicals (Chivers, 1994; Matsuda et al., 2022). This fermentation system enables these primates to exploit a diet of leaves in great quantities (Fashing, 2011; Kirkpatrick, 2011). As in many herbivorous mammals (Gordon and Prins, 2019), nutritional studies of colobines have revealed that they generally prefer foods containing more protein and less fiber (Waterman and Kool, 1994; Rothman et al., 2022). However, some colobines do not exhibit a strong preference for protein; in fact, a clear preference for protein is generally only seen in environments with low average protein content (Oftedal, 1991; Ganzhorn et al., 2017; Evans et al., 2021). Additionally, since leaves typically contain many plant secondary metabolites, it is hypothesized that colobines have strategies to detoxify, tolerate or avoid these compounds. Several studies have reported food choices that avoid tannins and/or alkaloids (Oates et al., 1977; Oates, 1988; Fashing et al., 2007). Conversely, there are also reports of a lack of active avoidance of secondary compounds (Davies et al., 1988; Mowry et al., 1996; Huang et al., 2010; Liu et al., 2013; Matsuda et al., 2013). Thus, the combined effects of food chemical properties and other possible factors such as digestibility, toughness and food abundance must be considered to understand the dietary choices of colobines. However, to our knowledge, little is known about the dietary choices of colobines with respect to a larger variety of factors, particularly when they are presented simultaneously.
In addition to chemical properties, variation in the mechanical properties of food plants is believed to influence primate feeding behavior in relation to the morphology of their dentofacial complex (e.g., Lucas and Teaford, 1994; Koyabu and Endo, 2009; Wright and Willis, 2022) and hence their food selection (e.g., Dominy et al., 2001; Huang et al., 2010; Matsuda et al., 2017); in other words, they typically select tender foods. Additionally, in leaf-eating colobines, the selection of leaves with a low degree of toughness may be an adaptive strategy as ingestion rates are negatively correlated, and masticatory investment is positively correlated, with leaf toughness (Dunham and Lambert, 2016). Furthermore, toughness, as assessed by oral sensation, may serve as a proximate cue of the food’s chemical properties, as it depends on the concentration of fiber, such as cellulose, hemicellulose and lignin (Lucas et al., 1997; Dominy et al., 2001). Protein content also has a negative relationship with the toughness of food sources consumed by Asian colobines (Matsuda et al., 2017), supporting the fact that leaf toughness is used as an important cue to evaluate nutritional quality.
Assessing digestibility can be an optimal way to comprehensively quantify food quality as represented by multivariate chemical and physical factors and has been done using in vitro assays. Although some previous studies have used assays combining acid and enzymatic treatments (e.g., Oates et al., 1980; Choo et al., 1981), live gut microbes contained in fresh feces are often used as inoculum, with a specific food as a substrate (e.g., Campbell et al., 2002; Schmidt et al., 2005; Hanya et al., 2020). Recent molecular studies have shown that bacterial communities diverge between the foregut and hindgut in colobines (Clayton et al., 2019), with the higher expression of microbial gene functions for fiber digestion in the foregut than in the hindgut (Liu et al., 2022); thus, feces that contain hindgut-derived microorganisms may not be a representative inoculum source for measuring digestibility in foregut-fermenting colobines. Although limited information is available on the foregut microbial community in colobines (Zhou et al., 2014; Amato et al., 2016; Hayakawa et al., 2018), it should not deviate substantially from that of other foregut-fermenting animals such as artiodactyls (Matsuda and Clauss, 2022), indicating that the most common in vitro method for measuring digestibility in herbivores, using a standardized inoculum and domestic ruminant rumen fluid [e.g., the modified Hohenheim gas test; (Menke et al., 1979)], should also be applicable for foregut-fermenting colobines (Waterman et al., 1980; Chapman and Chapman, 2002; Chapman et al., 2004; Matsuda et al., 2017).
Finally, optimal dietary choices should also depend on energy values, as well as handling and search times (Davies et al., 2012). Hence, it is important to consider the availability of foods and their heterogeneous distribution within forests (Boinski and Garber, 2000). Even in colobines, whose primary food source is leaves, which appear to be ubiquitous and abundant, leaf availability may explain diet selection (e.g., Chapman and Chapman, 2002; Hanya and Bernard, 2012; Matsuda et al., 2017), although some species do not base their diet simply on abundance (Zhou et al., 2006, 2013).
Black-and-white colobuses, or guerezas (Colobus guereza), are widely distributed throughout Africa (Fashing, 2022) and generally form small groups, typically averaging 7–11 individuals including one or two adult males, several adult females and immatures (Fashing, 2022). They have been reported to have considerable intraspecific variability in their diets, both over time and space, ranging from highly folivorous to including large quantities of fruit (Fashing, 2022). As for their dietary choices, protein content is one of the primary indicators in their decision to eat a particular leaf (Chapman et al., 2004; Fashing et al., 2007). Conversely, protein content and/or protein-to-fiber ratios are also positively correlated with the leaf foraging efforts of guerezas in Kibale, Uganda (Chapman et al., 2004), whereas in Kakamega, Kenya, they consume leaves on the basis of fiber, but not protein content (Fashing et al., 2007). Only one study (Chapman et al., 2004) has reported leaf digestibility as a considerable factor in dietary choice: depending on the forest environment (i.e., unlogged or fragmented forests), there is a marked difference between leaf selectivity and digestibility. Conversely, no studies have completed a comprehensive assessment of guereza leaf selectivity, including leaf toughness.
Here using a comprehensive dataset, we examined the relationship between a number of factors (i.e., leaf chemical and mechanical properties, in vitro digestibility, toughness and abundance) and food choice in a guereza population in Kalinzu Forest, Uganda, to establish the factors that are the most relevant proxies for guereza diet selection. First, we examined the relationship between leaf toughness and the chemical properties of the leaves in order to understand the functional traits of the leaves collected in the study site, including those consumed by the guerezas. As in earlier studies reviewed above (Lucas et al., 1997; Dominy et al., 2001; Matsuda et al., 2017), we predicted that leaf toughness would be positively correlated with fiber content, but negatively correlated with protein content. Second, to understand the general pattern of in vitro digestibility in relation to chemical properties of leaves, we also examined the respective relationships. We expected that fiber and secondary compounds would have negative effects on in vitro digestibility (Choo et al., 1981). Third, we compared the multivariate chemical, physical factors and digestibility of leaves eaten and not eaten by the guerezas. We predicted that guerezas would preferentially feed on leaves with lower toughness and higher digestibility than common plant species, rather than just those with higher protein and lower fiber levels (or higher protein: fiber ratio) often demonstrated in dietary choice models of folivorous primates (e.g., Milton, 1979). Fourth, we examined the selectivity within the plant species on which guerezas consumed. Referring to a study in another colobine species which considered multiple factors (Matsuda et al., 2017), we predicted that, apart from nutritionally, mechanically and digestibility advantageous diets, selectivity within the plant species consumed by guerezas would most likely indicate that they choose leaf species that were abundant at the study site. Therefore, in screening whether to eat or not to eat, we expected that food selection by guerezas would take into account nutritional, ingestion and digestive efficiency, but that selectivity within the plant species eaten would prioritize the optimal foraging strategy, i.e., saving travel costs when searching for foods. In our analysis of this comprehensive dataset, we sought to establish the measures that might be the most relevant proxies of dietary choice by guerezas at the study site.
Materials and Methods
Study Site and Collection of Behavioral Data
We conducted our study in a moist, medium-altitude evergreen forest in the Kalinzu Forest, western Uganda, covering an area of 137 km2 [30°07’ E, 0°17’ S; altitude 1000–1500 m above sea level; (Hashimoto et al., 1999)]. Mean minimum and maximum temperatures were approximately 14 and 27°C, respectively, and the total annual precipitation at the site was 1370 mm (Matsuda et al., 2020).
From November 2011 to October 2012, preliminary observations were conducted on several guereza groups before the most habituated group was chosen for the study. During these preliminary observations, members of a focal group were identified by describing their individual physical characteristics. Thus, we successfully followed a well-habituated, identifiable group that included 11 individual guerezas at the end of the preliminary observation period: one alpha male, three adult females, two subadult females, two juveniles and three infants (Matsuda et al., 2020).
From November 2013 to April 2016, behavioral observations were conducted for 10–22 days/month from approximately 7:30 to 16:00 (8.00 ± SD 1.01 observation hours per day and 4,308 h in total) using scan sampling at 10 min intervals. We recorded the activity (feeding, moving, and resting) of all visible adults and subadults ranging from one to seven individuals with a mean number of 4.8 ± SD 1.2 individuals per scan. We recorded the food category and collected samples for later identification when they were feeding (Matsuda et al., 2020). Continuous observations permitted the calculation of time budgets for the adult and subadult monkeys, such as the proportion of the day spent feeding and the time spent feeding on individual food items. Overall, the studied guerezas devoted 87.0% of their feeding time toward young leaves, 9.8% to fruits, 1.1% to flowers, 0.9% to bark, 0.8% to soil and 0.4% to unspecified foods and mature leaves. The numbers of plant species that provided these young leaves, fruits and flowers were 31, 12, and 6, respectively (Matsuda et al., 2020).
Vegetation Survey
On the basis of the focal group’s range data collected during preliminary observations, we selected 12 trails that were 180–900 m long (total 4700 m) within the study site. We labeled trees of ≥ 10 cm in diameter at breast height and vines of ≥5 cm in diameter located ≤1.5 m from the trail; hence, the labeled width was 3 m. The survey area covered 1.41 ha, including 969 trees and 27 vines from 68 species, 57 genera and 35 families (Matsuda et al., 2020). Food abundance was determined as the number of potential food plants for the guerezas.
Leaf Sampling
To compare the chemical properties, toughness and digestibility of young leaves that were both consumed and not consumed by the guerezas, leaf samples were collected in July 2014. For each plant species, young leaf samples were collected from at least four individual trees/vines in the vegetation survey area. We had planned to collect young leaves of all species that accounted for >0.1% of the feeding time (99.4% of the total feeding time) and the 20 most abundant species (defined according to the total number of plant species in the study area as assessed during the vegetation survey); however, because of the logistical difficulties of sampling leaves from treetops, samples from only 16 of the 20 most consumed species could be collected (96.9% of the total feeding time). Seven plant species also overlapped across the two categories, i.e., young leaves of all species that accounted for >0.1% of the feeding time and the 20 most abundant species in the vegetation survey. Thus, 29 plant species were evaluated in this study (i.e., 19 consumed and 10 non-consumed species).
Chemical Analysis
The plant samples collected were dried at ≤60°C immediately after collection in the field station and stored with desiccant in plastic bags for approximately 1 month. The samples were then re-dried at 60°C and milled for chemical analysis at the laboratory. Ground samples were sent to Tokachi Federation of Agricultural Cooperatives (Hokkaido, Japan) for nutrient analysis. The following nutritional components were analyzed using standard procedures (AOAC., 2012): crude protein (CP: AOAC no. 977.02), crude lipid (CL: AOAC no. 963.15), total ash (TA: AOAC no. 942.05), NDF corrected for residual ash (neutral detergent fiber expressed without residual ash: AOAC no. 2002.04). For each tree and vine species, young leaf samples were collected from at least four individual plants in or around the vegetation survey area.
Leaf Toughness
Young leaves from the collected plant species were immediately measured for toughness via a punch test (originally measured as kilogram-force; 1 kgf = 1 kg × 1, G = 1 kg × 9.8, 0665 m/s2 = 9.80665 N, to convert SI units to kPa). To determine the mass needed to penetrate a leaf, a penetrometer (Kurokawa and Nakashizuka, 2008) with a 3 mm diameter column (digital force gauges: IMADA Co., Ltd., Aichi, Japan) was used. The measurement was performed on 30 leaves collected from at least four individual plants per plant species, and the results were averaged for each species.
In vitro Fermentation
All experimental methods were conducted in accordance with relevant guidelines and regulations. A modified Hohenheim gas test (Menke et al., 1979) was used in an in vitro fermentation system to quantify the degradability of the young leaf samples (which had previously been submitted to chemical and toughness analysis). The inoculum was obtained from the rumen fluid of cattle fed a standardized, forage-dominated diet since the inoculum typical for guerezas was not available. However, the use of a standardized inoculum source made it possible to compare the current results with the in vitro results from other studies. The relative abundance of microbes and their taxonomic assignments in the forestomach of colobines should be similar to those in cattle (Zhou et al., 2014; Hayakawa et al., 2018). A total of 200 mg of milled plant tissue was weighed in airtight glass syringes together with the inoculum, as described previously (Hummel et al., 2006), and incubated at 39°C for 24 h. Gas production (Gp) was recorded after 4, 8, 12, and 24 h. Gas produced during fermentation reflects the extent of food degradation; it consists of nearly equal parts of the waste gases of fermentation and the CO2 from the buffer (bicarbonate) reaction with the volatile fatty acids produced during fermentation (Blümmel et al., 1999). Leaves were analyzed with and without 200 mg of polyethylene glycol (PEG), which was added to reduce the negative effects of tannins on digestion (Makkar et al., 1995). Samples were used for two tests on two different days (with two replicates each time). Gp at 24 h was used as the digestibility value of young leaves for guerezas. Additionally, we used the difference in digestibility with and without PEG (i.e., GP [24 h] with PEG and Gp [24 h] without PEG) as an indicator of secondary compounds such as tannin.
Data Analysis
Factors Affecting Leaf Toughness and Digestibility
To examine the relationship between leaf toughness and the chemical properties of the leaves, a linear model was used to establish whether leaf toughness in the tested plants (29 plant species) was affected by chemical factors such as NDF, CP, CL, and TA. The measured toughness value was the normally distributed response variable, and the other factors were treated as explanatory variables. To examine the relationship between in vitro digestibility and leaf traits, a linear model was also used to assess whether the leaf digestibility (Gp at 24 h) of all collected plants (i.e., 29 plant species) was affected by chemical properties, toughness and indicators of secondary compounds. The digestibility (with and without PEG) of young leaves was normally distributed, and the other factors were treated as explanatory variables.
Dietary Choice Between Eaten and Not Eaten Leaves
To determine the factors that may explain guerezas’ leaf preferences, we employed a generalized linear model using the leaf chemical properties, toughness, abundance (number of plants found in the vegetation transects), digestibility (with and without PEG) and indicators of secondary compounds and applied binomial regression family calculations to obtain the AICc. Leaf preference (i.e., the consumed or non-consumed food types) was treated as the categorical response variable, and the other variables were treated as explanatory variables.
Dietary Choice Within Eaten Leaves
We also investigated the effects of leaf traits, abundance, digestibility and indicators of secondary compound for the consumed plant species in terms of the percentage of feeding time [as determined by Matsuda et al. (2020)] using a linear model. The proportion of time spent feeding was logit transformed [log (p/1 − p)] and treated as a normally distributed response variable; the other factors were treated as explanatory variables.
For all models, we verified that the variance inflation factors were smaller than the cut-off value, i.e., less than 10 (Quinn and Keough, 2002); therefore, collinearity among independent factors (explanatory variables) did not affect the results. For model selection, we examined a set of models with all possible combinations of the explanatory variables and ranked them using AIC corrected for small sample sizes, i.e., AICc (Burnham and Anderson, 2002). Following the guidelines published for wildlife research, we selected the best-supported models as those with a ΔAIC(c) score ≤2, where ΔAIC(c) = AIC(c) − minimum AIC(c) within the candidate model set (Burnham and Anderson, 2002). All analyses were conducted using R ver. 4.1.0 (R-Core-Development-Team., 2021), employing the dredge function in the MuMIn package (Barton, 2012). As our data set is based on only 11 individuals belonging to one group of guerezas, extrapolating our results to a population level remains speculative.
Results
Factors Affecting Leaf Toughness and Digestibility
The best-fit model to explain leaf toughness, as evaluated using the AICc, included CP and NDF (Table 1 and Figures 1a,b), although the ΔAICc value of the following models including CP, NDF, TA and indicators of secondary compounds (Figures 1a–d) was also <2.0; leaf toughness increased with increasing TA and decreasing CP content, NDF content and secondary compounds. Out of both model groups for digestibility with/without PEG, with ΔAICc < 2, the best-fit model included NDF and indicators of secondary compounds (Figures 1e–h), which had negative effects (Table 2). The second-best model was the null model in both cases.

Table 1. Summary of model selection for linear models used to examine the effect of leaf chemical properties on leaf toughness (only models with ≤ΔAIC 2 are shown).
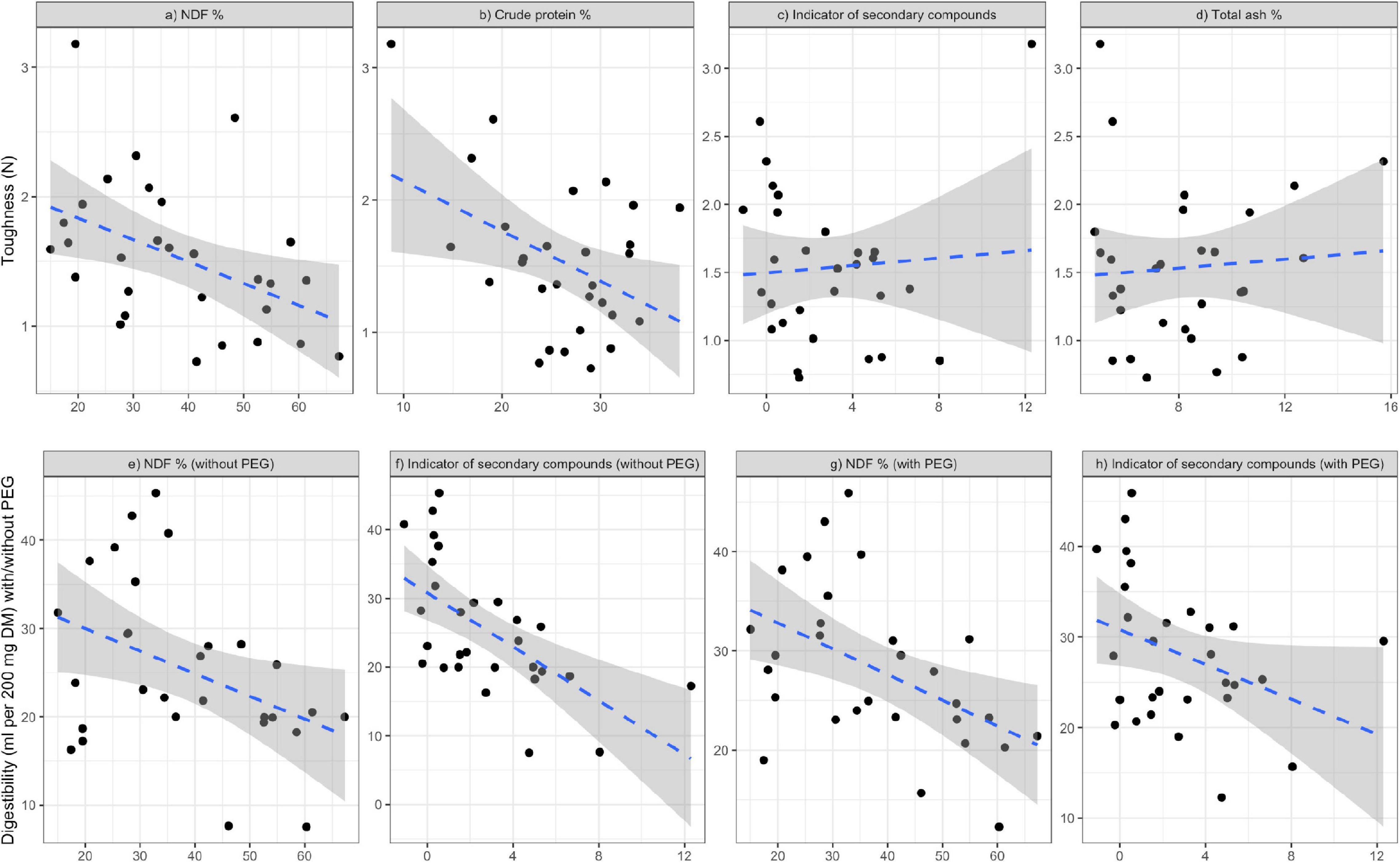
Figure 1. Relationships of leaf toughness (a–d) and in vitro digestibility (e–h) with chemical properties, respectively, based on the model selection Tables 1, 2. Blue dotted lines and shaded areas represent the linear regressions estimated by the “lm” method in R for the observed samples and their 95% confidence interval ranges, respectively.

Table 2. Summary of model selection for linear models used to examine the effects of several leaf traits (e.g., chemical properties, toughness and indicators of secondary compounds) on leaf digestibility (Gp at 24 h) without PEG (A) and with PEG (B) (only models with ≤ΔAIC 2 are shown).
Dietary Choice
Table 3 shows the properties of the consumed and non-consumed leaves. Leaf digestibility (gas production) was generally similar across the consumed and non-consumed leaves, although the consumed leaves produced slightly more gas than the non-consumed leaves, with consistent differences between the in vitro assays with and without PEG. Model selection for the investigation of whether guerezas choose young leaf species on the basis of their chemical properties, abundance, digestibility, indicators of secondary compounds and/or toughness, revealed that the null model was the top model. The second-best models, both with/without PEG, included only leaf abundance, though their ΔAICc values (2.51) were higher than the cut-off of 2.0. This result indicates that these traits were not strongly associated with leaf selection in guerezas.
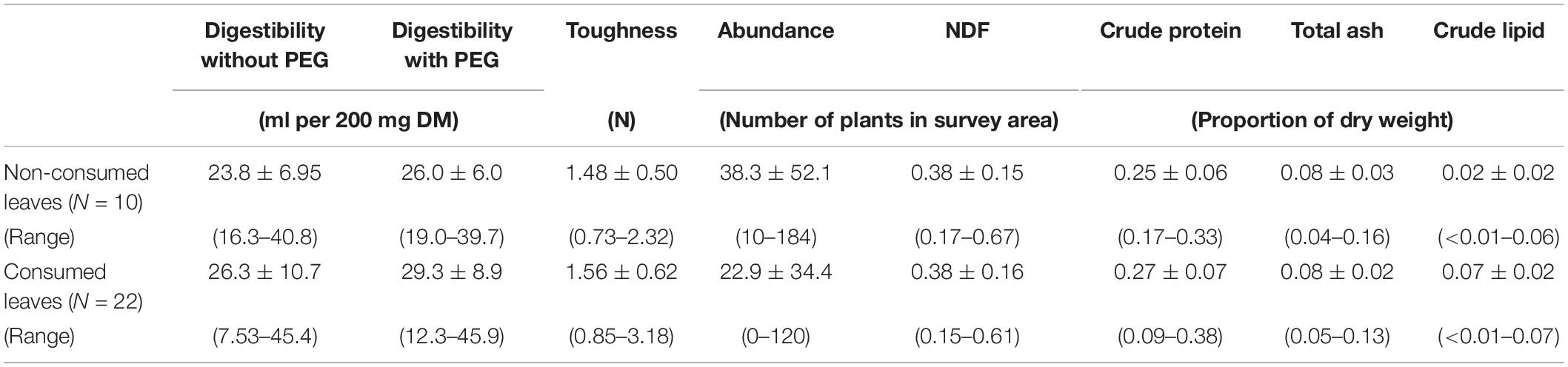
Table 3. Leaf traits and digestibility [mean ± standard deviation] of the young leaves from abundant trees in the study site, with respect to guereza preferences.
By contrast, linear models for the percentage of time spent eating young leaves indicated a positive effect of digestibility (both with and without PEG; Table 4 and Figures 2a,b). Additionally, other models with ΔAICc values of <2.0 included CP, TA, CL, toughness, and indicators of secondary compounds (Figures 2c–g). The animals spent more time eating less tough leaves with more CP, but the trend for the other nutrients was unexpected as the animals spent more time eating leaves containing less CL and TA and more secondary compounds.
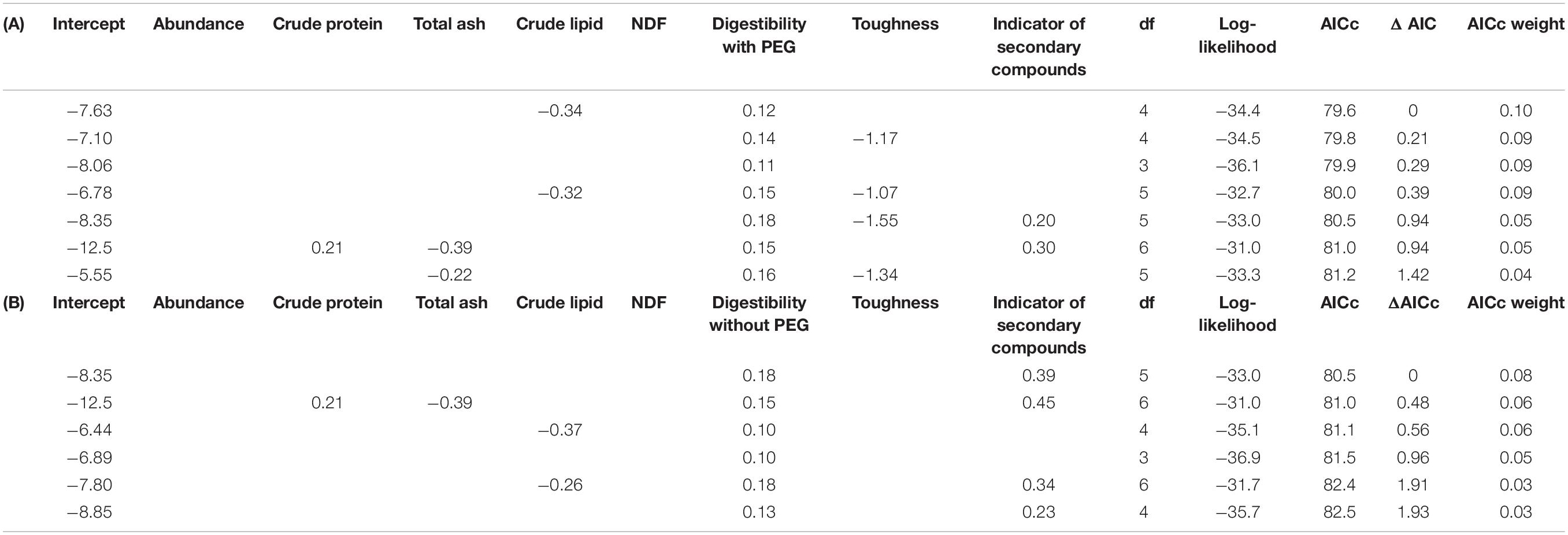
Table 4. Summary of model selection for linear models used to examine the effect of consumed young leaf chemical properties, abundance, digestibility with PEG (A) and without PEG (B) and toughness on the percentage of feeding time (only models with ≤ΔAIC 2 are shown).
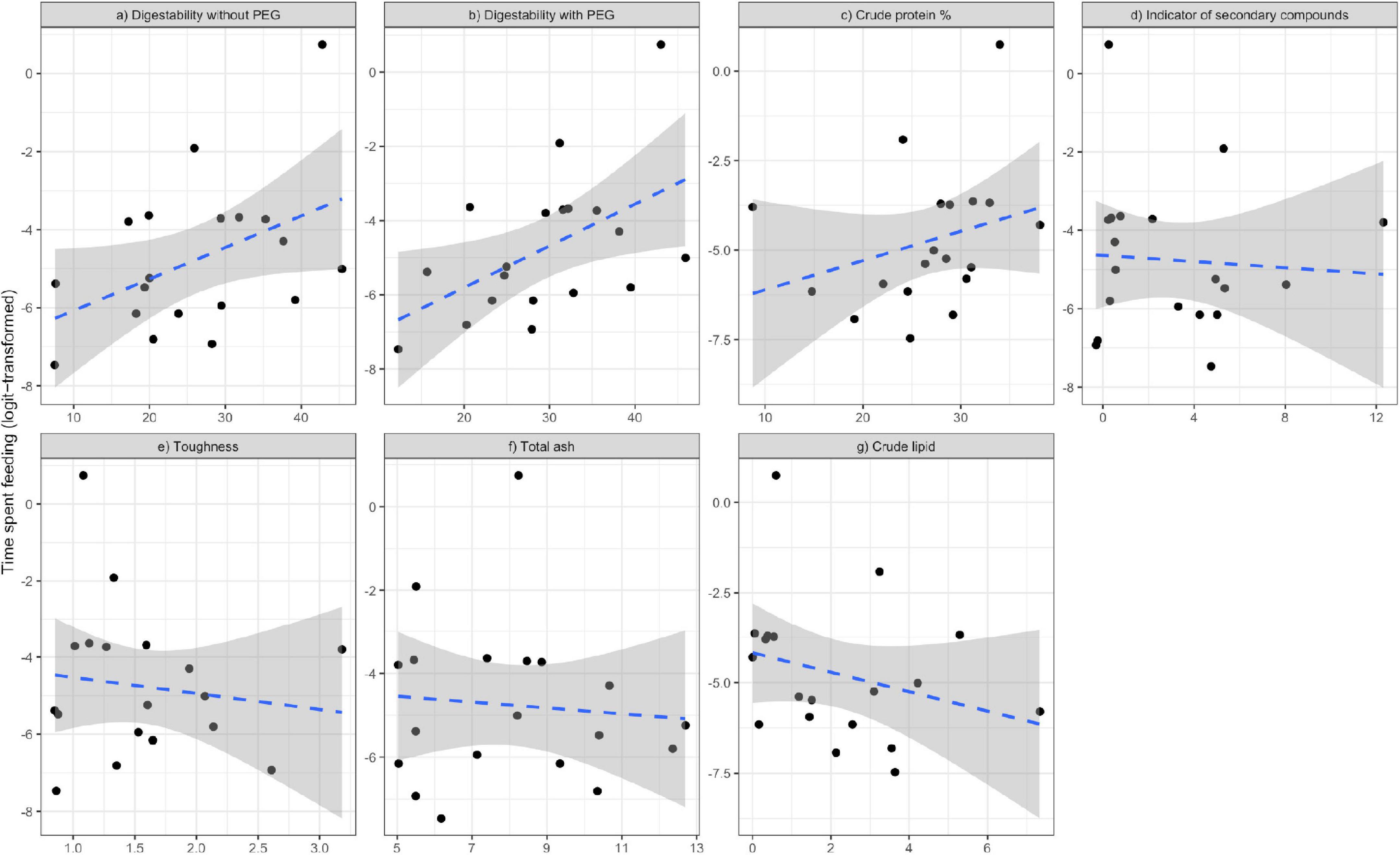
Figure 2. Relationships of the proportion of time spent feeding (logit transformed) with in vitro digestibility (a,b), chemical properties (c,d,f,g) and toughness (e) of consumed leaves by study guerezas, based on the model selection Table 4. Blue dotted lines and shaded areas represent the linear regressions estimated by the “lm” method in R for the observed samples and their 95% confidence interval ranges, respectively.
Discussion
Leaf Toughness and Digestibility
The toughness of young leaves was negatively correlated with CP and fiber (NDF), which are important nutritional factors that influence leaf selection in colobine monkeys (e.g., Fashing, 2001; Chapman and Chapman, 2002; Evans et al., 2021). Thus, the present study indicates that in leaf-eating primates, leaf toughness, as assessed by the oral sensation derived from mechanical properties (Dominy et al., 2001; Huang et al., 2010; Lucas et al., 2011), may be a proximate cue for these key nutrients, especially CP. However, the unexpected negative relationship with NDF indicates that NDF content alone may not be used as an indicator of toughness, as leaf toughness increases with increasing total bulk density and cellulose fraction but decreases with increasing hemicellulose and lignin content (Kitajima et al., 2016). Similarly, a negative correlation between NDF content and toughness has been detected in leaves from the island of Borneo (Matsuda et al., 2017). Thus, not only NDF but also ADF and lignin content should be simultaneously evaluated in relation to leaf toughness to further understand the effects of fiber.
In addition to CP and NDF contents, we also found that leaf toughness was negatively correlated with indicators of secondary compounds, although both toughness and chemical toxicity are expected to increase with leaf age (McKey, 1974; Rhoades and Cates, 1976; Lowman and Box, 1983). Nonetheless, a meta-analysis of 50 studies examined plant growth, plant defense and herbivory in relation to resource availability across latitude and ontogeny, showing that secondary compounds such as phenols and tannins do not exhibit generalizable associations with several leaf traits, including toughness (Endara and Coley, 2011); this may be because the properties and functions of these secondary compounds vary greatly across individual plant species (Kitajima et al., 2012).
The positive relationship between leaf toughness and TA content has, to our knowledge, not been previously reported; whether this is a general trend or specific to our study area would need to be clarified in future studies. Finally, as indicated in some primates (Lucas et al., 1998; Dominy and Lucas, 2001), visual selection of chemical and/or mechanical properties may be an important factor for leaf selectivity in colobines; the color of leaves often changes from red/yellow-green to dark green depending on their age. Future research should consider the associations between the above factors in addition to leaf color and their toughness.
As predicted based on previous studies using both enzymatic and rumen fluid in vitro digestibility assays (Choo et al., 1981), there was a negative relationship of digestibility with some chemical properties in leaves, particularly fiber and condensed tannins. As dietary choice in folivorous primates is generally thought to be influenced by nutritional requirements, dietary constraints on fiber intake and the avoidance of undesirable secondary compounds (Freeland and Janzen, 1974), our findings support the adaptive significance of such dietary choice.
Selection Between Eaten and Not Eaten Leaves
At a broad level, we found that none of the evaluated factors, including the chemical and mechanical properties, digestibility, toughness and abundance of leaves, affected whether guerezas consumed leaves or not. As discussed for Kibale (Evans et al., 2021), which is geographically close to our study site, the overall high level of protein and low level of fiber in the young leaves at our site (Table 3) may have contributed to this result. Indeed, the non-consumed young leaves in our study site had higher CP and lower NDF contents than the young leaves eaten by other foregut-fermenting colobines (e.g., Presbytis rubicunda and Nasalis larvatus) living on the island of Borneo (Matsuda et al., 2013). Thus, the present results support the hypothesis that leaves containing proteins are actively selected only when protein is limited in the environment (Oftedal, 1991; Ganzhorn et al., 2017; Evans et al., 2021). It is important to note, however, that the study group relied on young C. durandii leaves, upon which they fed heavily throughout the study period (58%) (Matsuda et al., 2020). In line with observations of the quality of these leaves in Kibale, Uganda (Chapman et al., 2003; Harris and Chapman, 2007), we found that the leaves were nutrient dense (the second-highest CP content) with a high digestibility, possibly reflecting low contents of difficult-to-digest fiber and secondary compounds, and were relatively abundant (i.e., easy to find and access; Matsuda et al., 2020). Altogether, this indicates that these factors played a role in the high occurrence of these leaves in the diet of the guerezas observed in the present study. Thus, these factors would potentially have an effect on dietary selection.
Selection Within the Eaten Leaves
We found that CP content, digestibility and toughness played an important role in influencing the dietary choices of guerezas, based on a fine grade analysis that considered dietary choice in relation to the percentage of foraging effort devoted to specific items amongst those selected. As mentioned above, the amount of protein in the leaves from this study site was generally high; thus, in terms of selectivity among the eaten leaves, we expected that the guerezas would select leaves on the basis of fiber rather than protein content (e.g., McKey et al., 1981; Chapman et al., 2004; Fashing et al., 2007). As such, the selection of leaves with more protein is difficult to explain. Instead of CP, the available protein (i.e., CP minus the fiber-bound protein), which is suggested to be a more relevant measure (Evans et al., 2021), could theoretically explain our results; however, since available protein and CP are generally positively correlated (Rothman et al., 2008), the use of CP appears nevertheless justified. It is possible that factors other than protein level itself drove dietary selection.
Conversely, our results outlining the selection of more digestible and less tough leaves is in agreement, albeit indirectly, with those of previous studies, which revealed a preference for leaves with lower fiber contents. Leaf NDF content was found to be negatively related with digestibility (Table 2); therefore, digestibility, which can quantify leaf quality as represented not only by fiber but also secondary compounds, may be the main factor that explains the guerezas’ dietary choices in this study. The unexpected negative relationship between NDF and toughness may have led to the selection of tender, but NDF-rich, leaves in our study. Leaf toughness has a decisive influence on colobine feeding behavior in terms of nutrients such as fiber as well as foraging/ingestion efficiency. In fact, ingestion rate (g/min) in Angolan black-and-white colobus monkeys (Colobus angolensis palliatus) is negatively correlated with leaf toughness, whereas masticatory investment (chews/g) is positively correlated with leaf toughness (Dunham and Lambert, 2016). Thus, choosing tender leaves is a reasonable strategy to increase the consumption of better quality foods that are high in protein (but NDF-rich) and can be ingested efficiently.
Outlook
Broadly speaking, we showed that leaf CP content and digestibility in relation to NDF content, secondary compounds and toughness were associated with the dietary choices of guerezas in our study site. Generally, this result is consistent with the food selectivity trends of guerezas documented at other study sites where multiple groups have been surveyed (Chapman et al., 2004; Fashing et al., 2007). However, because we only assessed a single family group, we cannot claim that the whole guereza population at Kalinzu shows the same behavior. Additionally, the potential bias of our study results may be created by the mismatch in time between behavioral data and leaf analyses as leaf traits may vary depending on the seasons (Chapman et al., 2003). Nonetheless, since temporal fluctuations of nutrients in tropical plant material, are smaller than inter-individual variations within the plant species in Kibale National Park, Uganda, it is recommended to sample from several trees at a point in time, as we performed in this study, rather than sampling over time (Chapman et al., 2003). However, the most accurate assessment of nutritional intake can be obtained by analyzing plant materials collected from specific trees selected for consumption, and this should be a future challenge in exploring the more detailed dietary choices of the study animals.
The results of this study reveal some similarity to the food selection practices of N. larvatus, an Asian colobine that is fairly comparable (Matsuda et al., 2017); however, leaf selectivity within the preferred plant species differed, since N. larvatus are more dependent on the abundance of the plant species rather than on its chemical and mechanical properties as well as digestibility. As in this study and that conducted by Matsuda et al. (2017), examining the dietary choice mechanisms of colobines from a variety of indices contributes to establishing relevant proxies and also allows for an understanding of the various functional properties of plants that are specific to each region. Further investigation of these plant properties in different geographical regions may aid in understanding the diverse interspecific and intraspecific foraging strategies of colobines, which may have arisen as an adaptation to the various functional properties of plants.
Data Availability Statement
The raw data supporting the conclusions of this article will be made available by the authors, without undue reservation.
Ethics Statement
Ethical review and approval was not required for the animal study because the study was based on the observation, but all research was conducted in compliance with animal care regulations and applicable Uganda laws.
Author Contributions
IM, MC, and JH conceptualized the initial idea and drafted the manuscript. IM performed the behavioral observation and sample collections with measurement of toughness in the field, arranged the chemical analysis of plant samples, and performed and interpreted the statistical analyses. JH conducted the digestibility analysis. CH, HI, TY, and DB arranged the sampling in the field. All authors contributed to the final version of the manuscript.
Funding
This study was financed by JSPS KAKENHI (#19H03308 to IM, #25304019 to CH, #16H02753 to TY, and #21255006 and #25257409 to IH) and Strategic Young Researcher Overseas Visits Program for Accelerating Brain Circulation from JSPS (to H. Hirai).
Conflict of Interest
The authors declare that the research was conducted in the absence of any commercial or financial relationships that could be construed as a potential conflict of interest.
Publisher’s Note
All claims expressed in this article are solely those of the authors and do not necessarily represent those of their affiliated organizations, or those of the publisher, the editors and the reviewers. Any product that may be evaluated in this article, or claim that may be made by its manufacturer, is not guaranteed or endorsed by the publisher.
Acknowledgments
We are grateful to the Uganda National Council for Science and Technology, the Uganda Forestry Department and Uganda Wildlife Authority for permission to work in the Kalinzu Forest. Our appreciation goes to the research assistants and managers from this project, particularly, Mina Isaji, Hodaka Matsuo, Natsumi Aruga, Reiko Okano, Yasuko Tashiro, and Charles Lakwo because, without their lot of assistance for accommodating and facilitating data collection for this project, it would not have been possible to conduct this study. We also thank Olivia Wanyana Maganyi of the Herbarium of Makerere University for her help in the identification of plants and Rolf Jeromin for his help in measuring the in vitro digestibility. Finally, we are grateful to the reviewers for their fruitful comments that improved this manuscript.
References
Amato, K. R., Metcalf, J. L., Song, S. J., Hale, V. L., Clayton, J., Ackermann, G., et al. (2016). Using the gut microbiota as a novel tool for examining colobine primate GI health. Glob. Ecol. Cons. 7, 225–237. doi: 10.1016/j.gecco.2016.06.004
AOAC. (2012). Official Methods of Analysis of AOAC International. Gaithersburg, MD: Association of Official Analytical Chemists International.
Blümmel, M., Aiple, K. P., Steinga, H., and Becker, K. (1999). A note on the stoichiometrical relationship of short chain fatty acid production and gas formation in vitro in feedstuffs of widely differing quality. J. Anim. Physiol. Anim. Nut. 81, 157–167. doi: 10.1046/j.1439-0396.1999.813205.x
Boinski, S., and Garber, P. A. (2000). On the Move: How and Why Animals Travel in Groups. Chicago: The University of Chicago Press.
Burnham, K. P., and Anderson, D. R. (2002). Model selection and multimodel inference: a practical information-theoretic approach. Verlag: Springer.
Campbell, J. L., Williams, C. V., and Eisemann, J. H. (2002). Fecal inoculum can be used to determine the rate and extent of in vitro fermentation of dietary fiber sources across three lemur species that differ in dietary profile: Varecia variegata, Eulemur fulvus and Hapalemur griseus. J. Nutrit. 132, 3073–3080. doi: 10.1093/jn/131.10.3073
Cassini, M. H. (1994). Behavioral mechanisms of selection of diet components and their ecological implications in herbivorous mammals. J. Mammal. 75, 733–740. doi: 10.2307/1382523
Chapman, C. A., and Chapman, L. J. (2002). Foraging challenges of red colobus monkeys: influence of nutrients and secondary compounds. Comp. Biochem. Physiol. Mol. Integr. Physiol. 133, 861–875. doi: 10.1016/s1095-6433(02)00209-x
Chapman, C. A., Chapman, L. J., Naughton-Treves, L., Lawes, M. J., and McDowell, L. R. (2004). Predicting folivorous primate abundance: validation of a nutritional model. Am. J. Primatol. 62, 55–69. doi: 10.1002/ajp.20006
Chapman, C. A., Chapman, L. J., Rode, K. D., Hauck, E. M., and McDowell, L. R. (2003). Variation in the nutritional value of primate foods: among trees, time periods, and areas. Int. J. Primatol. 24, 317–333.
Chivers, D. (1994). “Functional anatomy of the gastrointestinal tract,” in Colobine monkeys: their ecology, behaviour and evolution, eds A. Davies and J. Oates (Cambridge: Cambridge University Press), 205–227.
Choo, G. M., Waterman, P. G., McKey, D. B., and Gartlan, J. S. (1981). A simple enzyme assay for dry matter digestibility and its value in studying food selection by generalist herbivores. Oecologia 49, 170–178. doi: 10.1007/BF00349185
Clayton, J. B., Shields-Cutler, R. R., Hoops, S. L., Al-Ghalith, G. A., Sha, J. C. M., Johnson, T. J., et al. (2019). Bacterial community structure and function distinguish gut sites in captive red-shanked doucs (Pygathrix nemaeus). Am. J. Primatol. 2019:e22977. doi: 10.1002/ajp.22977
Crawley, M. J. (1983). Herbirory: the dynamics of animal-plant interactions. Studies in Ecology. Oxford: Blackwell Scientific Publications.
Davies, A. G., Bennett, E. L., and Waterman, P. G. (1988). Food selection by two South-east Asian colobine monkeys (Presbytis rubicunda and Presbytis melalophos) in relation to plant chemistry. Biol. J. Linn. Soc. 34, 33–56. doi: 10.1111/j.1095-8312.1988.tb01947.x
Davies, N. B., Krebs, J. R., and West, S. A. (2012). An Introduction to Behavioural Ecology. West Sussex: A John Wiley & Sons, Ltd., Publication.
Dominy, N. J., and Lucas, P. W. (2001). Ecological importance of trichromatic vision to primates. Nature 410, 363–366. doi: 10.1038/35066567
Dominy, N. J., Lucas, P. W., Osorio, D., and Yamashita, N. (2001). The sensory ecology of primate food perception. Evol. Anthropol. Issues News Rev. 10, 171–186. doi: 10.1002/evan.1031
Dunham, N. T., and Lambert, A. L. (2016). The role of leaf toughness on foraging efficiency in Angola black and white colobus monkeys (Colobus angolensis palliatus). Am. J. Phys. Anthropol. 161, 343–54. doi: 10.1002/ajpa.23036
Endara, M.-J., and Coley, P. D. (2011). The resource availability hypothesis revisited: a meta-analysis. Funct. Ecol. 25, 389–398. doi: 10.1111/j.1365-2435.2010.01803.x
Evans, K. D., Foley, W. J., Chapman, C. A., and Rothman, J. M. (2021). Deconstructing protein in the diet and biomass of colobine primates. Int. J. Primatol. 42:9.
Fashing, P. J. (2001). Feeding ecology of guerezas in the Kakamega forest, Kenya: the importance of Moraceae fruit in their diet. Int. J. Primatol. 22, 579–609.
Fashing, P. J. (2011). “African colobine monkeys: their behavior, ecology, and conservation,” in Primates in Perspective, eds C. J. Campbell, A. Fuentes, K. C. MacKinnon, S. K. Bearder, and R. M. Stumpf (Oxford: Oxford University Press), 203–229.
Fashing, P. J. (2022). “Natural history of black-and-white colobus monkeys,” in The Colobines: Natural History, Behaviour and Ecological Diversity (Cambridge Studies in Biological and Evolutionary Anthropology), eds I. Matsuda, C. C. Grueter, and J. A. Teichroeb (Cambridge: Cambridge University Press), 128–145. doi: 10.1017/9781108347150.011
Fashing, P. J., Dierenfeld, E. S., and Mowry, C. B. (2007). Influence of plant and soil chemistry on food selection, ranging patterns, and biomass of Colobus guereza in Kakamega Forest, Kenya. Int. J. Primatol. 28, 673–703. doi: 10.1007/s10764-006-9096-2
Freeland, W. J., and Janzen, D. H. (1974). Strategies in herbivory by mammals: the role of plant secondary compounds. Am. Natural. 108, 269–289. doi: 10.1086/282907
Ganzhorn, J. U., Arrigo-Nelson, S. J., Carrai, V., Chalise, M. K., Donati, G., Droescher, I., et al. (2017). The importance of protein in leaf selection of folivorous primates. Am. J. Primatol. 79, 1–13. doi: 10.1002/ajp.22550
Hanya, G., and Bernard, H. (2012). Fallback foods of red leaf monkeys (Presbytis rubicunda) in Danum Valley. Borneo. Int. J. Primatol. 33, 322–337. doi: 10.1007/s10764-012-9580-9
Hanya, G., Tackmann, J., Sawada, A., Lee, W., Pokharel, S. S., de Castro Maciel, V. G., et al. (2020). Fermentation ability of gut microbiota of wild japanese macaques in the highland and lowland yakushima: in vitro fermentation assay and genetic analyses. Microb. Ecol. 80:1515. doi: 10.1007/s00248-020-01515-8
Harris, T. R., and Chapman, C. A. (2007). Variation in diet and ranging of black and white colobus monkeys in Kibale National Park, Uganda. Primates 48, 208–221. doi: 10.1007/s10329-006-0036-8
Hashimoto, C., Furuichi, T., Tashiro, Y., and Kimura, D. (1999). Vegetation of the Kalinzu Forest, Uganda: ordination of forest types using principal component analysis. Afr. Stud. Monograp. 20, 229–239.
Hayakawa, T., Nathan, S., Stark, D. J., Saldivar, D. A. R., Sipangkui, R., Goossens, B., et al. (2018). First report of foregut microbial community in proboscis monkeys: are diverse forests a reservoir for diverse microbiomes? Environ. Microbiol. Rep. 10, 655–662. doi: 10.1111/1758-2229.12677
Huang, Z., Huo, S., Yang, S., Cui, L., and Xiao, W. (2010). Leaf choice in black-and-white snub-nosed monkeys Rhinopithecus bieti is related to the physical and chemical properties of leaves. Curr. Zool. 56, 643–649. doi: 10.1093/czoolo/56.6.643
Hummel, J., Südekum, K.-H., Streich, W. J., and Clauss, M. (2006). Forage fermentation patterns and their implications for herbivore ingesta retention times. Funct. Ecol. 20, 989–1002. doi: 10.1111/j.1365-2435.2006.01206.x
Iason, G. R., and Villalba, J. J. (2006). Behavioral strategies of mammal herbivores against plant secondary metabolites: the avoidance-tolerance continuum. J. Chem. Ecol. 32, 1115–1132. doi: 10.1007/s10886-006-9075-2
Janzen, D. H. (1973). “Complications in interpreting the chemical defenses of trees against tropical arboreal plant-eating vertebrates,” in The Ecology of Arboreal Folivores, ed. G. G. Montgomery (Washington, D.C: Smithsonian Institution Press), 73–84.
Kirkpatrick, R. C. (2011). “The Asian colobines: diversity among leaf-eating monkeys,” in Primates in Perspective, eds C. J. Campbell, A. Fuentes, K. C. MacKinnon, S. K. Bearder, and R. M. Stumpf (Oxford: Oxford University Press), 189–202. doi: 10.1016/j.ympev.2006.01.017
Kitajima, K., Llorens, A. M., Stefanescu, C., Timchenko, M. V., Lucas, P. W., and Wright, S. J. (2012). How cellulose-based leaf toughness and lamina density contribute to long leaf lifespans of shade-tolerant species. New Phytol. 195, 640–652. doi: 10.1111/j.1469-8137.2012.04203.x
Kitajima, K., Wright, S. J., and Westbrook, J. W. (2016). Leaf cellulose density as the key determinant of inter- and intra-specific variation in leaf fracture toughness in a species-rich tropical forest. Interf. Focus 6:100. doi: 10.1098/rsfs.2015.0100
Koyabu, D. B., and Endo, H. (2009). Craniofacial variation and dietary adaptations of African colobines. J. Hum. Evol. 56, 525–536. doi: 10.1016/j.jhevol.2008.12.009
Kurokawa, H., and Nakashizuka, T. (2008). Leaf herbivory and decomposability in a malaysian tropical rain forest. Ecology 89, 2645–2656. doi: 10.1890/07-1352.1
Liu, R., Amato, K., Hou, R., Gomez, A., Dunn, D. W., Zhang, J., et al. (2022). Specialised digestive adaptations within the hindgut of a colobine monkey. Innovation 3:207.
Liu, X., Stanford, C. B., Yang, J., Yao, H., and Li, Y. (2013). Foods eaten by the Sichuan snub-nosed monkey (Rhinopithecus roxellana) in Shennongjia National Nature Reserve, China, in relation to nutritional chemistry. Am. J. Primatol. 75, 860–871. doi: 10.1002/ajp.22149
Lowman, M. D., and Box, J. D. (1983). Variation in leaf toughness and phenolic content among five species of Australian rain forest trees. Aust. Ecol. 8, 17–25. doi: 10.1111/j.1442-9993.1983.tb01515.x
Lucas, P. W., Copes, L., Constantino, P. J., Vogel, E. R., Chalk, J., Talebi, M., et al. (2011). Measuring the toughness of primate foods and its ecological value. Int. J. Primatol. 33, 598–610. doi: 10.1007/s10764-011-9540-9
Lucas, P. W., Darvell, B. W., Lee, P. K. D., Yuen, T. D. B., and Choong, M. F. (1998). Colour cues for leaf food selection by long-tailed macaques (macaca fascicularis) with a new suggestion for the evolution of trichromatic colour vision. Folia Primatol. 69, 139–154. doi: 10.1159/000021576
Lucas, P. W., Tan, H. T. W., and Cheng, P. Y. (1997). The toughness of secondary cell wall and woody tissue. philosophical transactions of the royal society of london. Ser. B: Biol. Sci. 352, 341–352. doi: 10.1098/rstb.1997.0025
Lucas, P. W., and Teaford, M. F. (1994). “Functional morphology of colobine teeth,” in Colobine Monkets: Their Ecology, Behaviour and Evolution, eds A. G. Davies and F. J. Oates (Cambridge: Cambridge University Press), 173–203.
Makkar, H. P., Blummel, M., and Becker, K. (1995). Formation of complexes between polyvinyl pyrrolidones or polyethylene glycols and tannins, and their implication in gas production and true digestibility in in vitro techniques. Br. J. Nutr. 73, 897–913. doi: 10.1079/bjn19950095
Matsuda, I., and Clauss, M. (2022). “Morphology and physiology of colobine digestive tracts,” in The Colobines: Natural History, Behaviour and Ecological Diversity (Cambridge Studies in Biological and Evolutionary Anthropology), eds I. Matsuda, C. C. Grueter, and J. A. Teichroeb (Cambridge: Cambridge University Press), 64–77. doi: 10.1017/9781108347150.007
Matsuda, I., Clauss, M., Tuuga, A., Sugau, J., Hanya, G., Yumoto, T., et al. (2017). Factors affecting leaf selection by foregut-fermenting proboscis monkeys: new insight from in vitro digestibility and toughness of leaves. Sci. Rep. 7:42774. doi: 10.1038/srep42774
Matsuda, I., Ihobe, H., Tashiro, Y., Yumoto, T., Baranga, D., and Hashimoto, C. (2020). The diet and feeding behavior of the black-and-white colobus (Colobus guereza) in the Kalinzu Forest, Uganda. Primates 61, 473–484. doi: 10.1007/s10329-020-00794-6
Matsuda, I., Takano, T., Shintaku, Y., and Clauss, M. (2022). Gastrointestinal morphology and ontogeny of foregut-fermenting primates. Am. J. Biol. Anthropol. doi: 10.1002/ajpa.24476
Matsuda, I., Tuuga, A., Bernard, H., Sugau, J., and Hanya, G. (2013). Leaf selection by two Bornean colobine monkeys in relation to plant chemistry and abundance. Sci. Rep. 3:1873. doi: 10.1038/srep01873
McKey, D. (1974). Adaptive patterns in alkaloid physiology. Am. Natural. 108, 305–320. doi: 10.1086/282909
McKey, D. B., Gartlan, J. S., Waterman, P. G., and Choo, G. M. (1981). Food selection by black colobus monkeys (Coiobus satanas) in relation to plant chemistry. Biol. J. Linnean Soc. 16, 115–146. doi: 10.1111/j.1095-8312.1981.tb01646.x
Menke, K. H., Raab, L., Salewski, A., Steingass, H., Fritz, D., and Schneider, W. (1979). The estimation of the digestibility and metabolizable energy content of ruminant feedingstuffs from the gas production when they are incubated with rumen liquor in vitro. J. Agricult. Sci. 93, 217–222. doi: 10.1017/s0021859600086305
Milton, K. (1979). Factors influencing leaf choice by howler monkeys: a test of some hypotheses of food selection by generalist herbivores. Am. Natural. 114:362.
Mowry, C. B., Decker, B. S., and Shure, D. J. (1996). The role of phytochemistry in dietary choices of Tana River red colobus monkeys(Procolobus badius rufomitratus). Int. J. Primatol. 17, 63–84. doi: 10.1007/bf02696159
Oates, J. F. (1988). The diet of the olive colobus monkey,Procolobus verus, in Sierra Leone. Int. J. Primatol. 9, 457–478. doi: 10.1007/bf02736220
Oates, J. F., Swain, T., and Zantovska, J. (1977). Secondary compounds and food selection by colobus monkeys. Biochem. Syst. Ecol. 5, 317–321.
Oates, J. F., Waterman, P. G., and Choo, G. M. (1980). Food selection by the South Indian leaf-monkey, Presbytis johnii, in relation to leaf chemistry. Oecologia 45, 45–56.
Oftedal, O. T. (1991). The nutritional consequences of foraging in primates: the relationship of nutrient intakes to nutrient requirements. Philos. Trans. R Soc. Lond. B Biol. Sci. 334, 169–170. doi: 10.1098/rstb.1991.0105
Onoda, Y., Westoby, M., Adler, P. B., Choong, A. M., Clissold, F. J., Cornelissen, J. H., et al. (2011). Global patterns of leaf mechanical properties. Ecol Lett. 14, 301–312. doi: 10.1111/j.1461-0248.2010.01582.x
Quinn, G. P., and Keough, M. J. (2002). Experimental design and data analysis for biologists. Cambridge: Cambridge University Press.
R-Core-Development-Team. (2021). R: a language and environment for statistical computing. Vienna: Foundation for Statistical Computing.
Rhoades, D. F., and Cates, R. G. (1976). “Toward a general theory of plant antiherbivore chemistry,” in Biochemical Interaction Between Plants and Insects. Recent Advances in Phytochemistry, eds J. W. Wallace and R. L. Mansell (Boston: Springer), 168–213.
Rothman, J. M., Chapman, C. A., and Pell, A. N. (2008). Fiber-bound nitrogen in gorilla diets: implications for estimating dietary protein intake of primates. Am. J. Primatol. 70, 690–694. doi: 10.1002/ajp.20540
Rothman, J. M., DePasquale, A., Evans, K. D., and Raboin, D. L. (2022). “Colobine nutritional ecology,” in The Colobines: Natural History, Behaviour and Ecological Diversity (Cambridge Studies in Biological and Evolutionary Anthropology), eds I. Matsuda, C. C. Grueter, and J. A. Teichroeb (Cambridge: Cambridge University Press), 94–107. doi: 10.1017/9781108347150.009
Schmidt, D. A., Kerley, M. S., Dempsey, J. L., Porton, I. J., Porter, J. H., Griffin, M. E., et al. (2005). Fiber digestibility by the orangutan (Pongo abelii): in vitro and in vivo. J. Zoo. Wildl. Med. 36, 571–580. doi: 10.1638/04-103.1
Waterman, P. G., and Kool, K. M. (1994). “Colobine food selection and plant chemistry,” in Colobine Monkeys: Their Ecology, Behaviour and Evolution, eds A. G. Davies and F. J. Oates (Cambridge: Cambridge University Press), 251–310.
Waterman, P. G., Mbi, C. N., McKey, D. B., and Gartlan, J. S. (1980). African rainforest vegetation and rumen microbes: Phenolic compounds and nutrients as correlates of digestibility. Oecologia 47, 22–33. doi: 10.1007/BF00541771
Wright, B. W., and Willis, M. S. (2022). “Relationships between the diet and dentition of Asian leaf monkeys,” in The Colobines: Natural History, Behaviour and Ecological Diversity (Cambridge Studies in Biological and Evolutionary Anthropology), eds I. Matsuda, C. C. Grueter, and J. A. Teichroeb (Cambridge: Cambridge University Press), 44–63. doi: 10.1017/9781108347150.006
Zhou, Q., Tang, Z., Li, Y., and Huang, C. (2013). Food diversity and choice of white-headed langur in fragmented limestone hill habitat in Guangxi, China. Acta Ecol. Sinica 33, 109–113.
Zhou, Q., Wei, F., Li, M., Huang, C., and Luo, B. (2006). Diet and food choice of Trachypithecus francoisi in the Nonggang Nature Reserve, China. Int. J. Primatol. 27, 1441–1460.
Keywords: feeding ecology, folivore, food mechanical properties, nutritional ecology, secondary compounds
Citation: Matsuda I, Hashimoto C, Ihobe H, Yumoto T, Baranga D, Clauss M and Hummel J (2022) Dietary Choices of a Foregut-Fermenting Primate, Colobus guereza: A Comprehensive Approach Including Leaf Chemical and Mechanical Properties, Digestibility and Abundance. Front. Ecol. Evol. 10:795015. doi: 10.3389/fevo.2022.795015
Received: 14 October 2021; Accepted: 16 February 2022;
Published: 14 March 2022.
Edited by:
Cécile Garcia, UMR 7206 Eco Anthropologie et Ethnobiologie (EAE), FranceReviewed by:
Ilaria Agostini, Instituto de Biología Subtropical (IBS), ArgentinaArrilton Araujo, Federal University of Rio Grande do Norte, Brazil
Copyright © 2022 Matsuda, Hashimoto, Ihobe, Yumoto, Baranga, Clauss and Hummel. This is an open-access article distributed under the terms of the Creative Commons Attribution License (CC BY). The use, distribution or reproduction in other forums is permitted, provided the original author(s) and the copyright owner(s) are credited and that the original publication in this journal is cited, in accordance with accepted academic practice. No use, distribution or reproduction is permitted which does not comply with these terms.
*Correspondence: Ikki Matsuda, aWtraS1tYXRzdWRhQGlzYy5jaHVidS5hYy5qcA==; Jürgen Hummel, amh1bW1lbEBnd2RnLmRl
†ORCID: Ikki Matsuda, orcid.org/0000-0002-0861-7801; Chie Hashimoto, orcid.org/0000-0003-3668-3256; Takakazu Yumoto, orcid.org/0000-0002-8924-3388; Marcus Clauss, orcid.org/0000-0003-3841-6207